- 1Feline Research Center of National Forestry and Grassland Administration, College of Wildlife and Protected Area, Northeast Forestry University, Harbin, China
- 2National School of Forestry, University of Cumbria, Cumbria, United Kingdom
There is evidence that cattle grazing in forests limits big cat abundance. There is concern, too, about competition with wild ungulate prey through bottom-up effects on vegetation. Hence, there have been calls to remove or control forest livestock grazing in aid of restoring endangered large carnivores and their prey. To help inform scientific debate and decision making, we explored cattle-vegetation-prey dynamics in Northeast Tiger and Leopard National Park, northeast China and present a reappraisal of livestock grazing in the context of coexistence and an integrated approach to land use in China. Inside long-term forest grazing enclosures, wild boar (Sus scrofa) density increased with cattle density. Roe deer (Capreolus pygargus) density and occurrence probability of wild boar and roe deer were not influenced by cattle density. Wild ungulate densities were not related with the number of annual shoots, i.e., forage plant abundance. The presence/absence and abundance of annual shoots was not related with cattle density. Wild ungulate density had mixed associations with vegetation structure; arbor tree diameter at breast height (DBH) and habitat “openness” increased with cattle density. Finally, inside and outside enclosures had different vegetation characteristics and wild boar densities, while roe deer densities were equal. We conclude that cattle density and associated changes to vegetation have positive, negative, and neutral effects on two wild ungulate prey species. Each of these factors warrant consideration in evidence-based management decisions in regard to regulating ungulate community composition to support different large predators as preferred prey in core areas and corridors of habitats.
Introduction
Large herbivores have immense capacity to shape landscapes, and have nurtured and maintained biologically rich and productive ecosystems for millennia (Goderie et al., 2013; Ripple et al., 2015). Their size, abundance and behavior stimulate major ecological processes, often defining them as keystone species and ecological engineers (Caro, 2010). As grazing and browsing specialists, they remove vegetation, and trampling creates open spaces within which germination can occur with less competition and the addition of feces as natural fertilizer, providing an important facilitative role in seedling establishment (Hancock et al., 2010; Faust et al., 2011). Seedlings may, however, be damaged by trampling and grazing (Krzic et al., 2006; Wassie et al., 2009; Thakur et al., 2011), yet a sufficiently high percentage may survive to support productive forests under tolerable conditions (Krzic et al., 2006). These interactions are spatially and seasonally complex, shifting vegetation and nutrients through space and time (Kohler et al., 2006; Bernes et al., 2018).
Plants are inherently resilient to herbivory (Bernes et al., 2018), and an abundance of large herbivores promotes a dynamic natural cycle of forested and open grassland landscapes; their absence (or shortage), by contrast, locks in a constrained cycle of closed canopy forest (Goderie et al., 2013). Grazing pressures on saplings create forest gaps, leading to tree regeneration, shrub/herb layer growth, and increases ungulate habitat suitability as they utilize gaps more than closed forests (Kramer et al., 2006; Kuijper et al., 2009; Bernes et al., 2018). This habitat heterogeneity supports the dynamics of predator-prey interactions whereby predators can find habitat to maximize hunting performance and prey species can find habitat that mitigates their predation risk by utilizing areas where predator hunting efficiency is diminished (Miller et al., 2015).
Early domestication of wildlife and animal agriculture have profoundly influenced human societies and landscapes (Food and Agriculture Organization [FAO], 2006). Presently, the livestock sector accounts for more land use than any other human activity, around 30 percent of all terrestrial land (Food and Agriculture Organization [FAO], 2018), and has been a primary driver of habitat loss and reducing wild herbivores and predators in number and distribution (Food and Agriculture Organization [FAO], 2006; Goderie et al., 2013; WWF, 2020). Farmed animals now represent 60% of global mammal biomass, whilst wild mammals represent just 4% (Bar-On et al., 2018). Furthermore, while natural grazing outdoors generally restricts wild mammal presence and abundance through reductions in habitat and resource availability, and habitat complexity, the rapid transition over the recent decades from traditional outdoor farming systems to industrial-scale landless systems is attributed with high externalized costs of land use and environmental pollution (Bai et al., 2018).
By far the greatest increase in meat and milk consumption worldwide during the recent decades has been in China, which saw a 13-fold increase in demand for beef products per capita 1980–2010 (Ministry of Agriculture in China [MOA], 2014). In 2010 the equivalent of 510,000 km2 of arable land (equivalent to 5% of China’s land area) was used for domestic livestock feed production in China, plus an additional 2 million km2 of grasslands (Bai et al., 2018). Modeled scenarios for 2050 suggest that China would need a further 310,000 km2 to produce livestock feed in order to meet projected increases in domestic demand, including for beef and milk which is increasing much faster than other livestock products (Bai et al., 2018). In light of systems-level environmental disruption associated with modern food systems (Gerten et al., 2020), it is perhaps worth considering the need, and urgency, for ecologically balanced alternatives.
The Northeast Tiger and Leopard National Park (NTLNP) is a pilot conservation project launched in 2017 in northeast China. Despite a history of seasonal livestock grazing in the forests of NTLNP, the practice is nationally prohibited in nature reserves except as otherwise provided by laws and administrative regulations [Regulations of the People’s Republic of China on Nature Reserves 1994 (revised 2011 and 2017)]. Within NTLNP, cattle are regarded a major disturbance and threat to vegetation, wild ungulates and predators via bottom-up ecological effects and population constraints, and there are calls for livestock reductions or removals (see for example: Hebblewhite et al., 2012 for Amur tiger (Panthera tigris altaica) conservation landscape planning; Soh et al., 2014; Gu et al., 2018; Dou et al., 2019 for livestock depredation and tiger prey selection; Wang et al., 2016, 2017; Xiao et al., 2018 for cattle effects on tiger, leopard (P. pardus orientalis) and prey abundance; Li Z. L. et al., 2017; Wang et al., 2019 for cattle effects on vegetation; Feng J. W. et al., 2021 for cattle effects on species richness; and Wang et al., 2018; Li et al., 2019; Feng R. N. et al., 2021 for cattle effects on tiger, leopard and prey spatiotemporal patterns). However, the reduction targets are generally unspecified and there remain important gaps in the evidence base, particularly concerning relationships between vegetation factors and wildlife abundance, and their corresponding relationships with long-term cattle grazing. Similar requests have also been made by reserve managers in southern China to limit cattle grazing on the basis of habitat selection and speculated reduced habitat suitability of the conservation-dependent giant panda (Ailuropoda melanoleuca) (Wang et al., 2015, but see Li B. V. et al., 2017 for subsequent analyses).
In regard to food habits and forest vegetation resource use overlap of domestic cattle and wild ungulates, these species exist on a grazer-browser continuum (Goderie et al., 2013). Though cattle are commonly recognized as grazers, their diet is highly variable (Calleja et al., 2019), and a one-year exclosure experiment in the eastern part of NTLNP suggests that cattle reduce twig and leaf biomass during seasonal grazing (Wang et al., 2019), echoing direct foraging observations in another temperate study area which found that around 20% of the cattle diet is woody plants; approximately 80% is grass (Cromsigt et al., 2018). In winter, when cattle are removed from the forest and fed indoors, and wild ungulate abundance is at least partly regulated by food availability, woody plants constitute a major part of the roe deer (Capreolus pygargus) diet, roughly 80–90% (Chen et al., 2014; Li et al., 2014; He et al., 2015; Feng, 2017), and for the wild boar (Sus scrofa), a versatile omnivore and an intermediate feeder (Hofmann, 1989), roughly 5–15% of the diet may be twigs, leaves, bark and fruit, 50–60% herbaceous vegetation, and much of the large remaining part of the diet may comprise roots, buds and shoots (Zhang, 2018).
Despite the narrative of cattle-wildlife conflict and management to reduce or remove cattle from natural systems, in comparable ecosystems to NTLNP, cattle are used as a tool for conservation (Pollock et al., 2005; Kohler et al., 2006; Kramer et al., 2006; Hancock et al., 2010), highlighting the need to review, in a Chinese context, our understanding of how cattle grazing interacts with wild ungulates and vegetation in forests, especially how summer grazing may influence winter food availability as this may be an important factor in regulating population dynamics (Kramer et al., 2006; Svenning et al., 2016). Conservation evidence and management implications of cattle grazing and enclosure fencing in forests is limited, especially in regards to the effects on native wild ungulates (Littlewood et al., 2020). Such data, particularly about cattle density and grazing duration, is of great practical value for management decisions and designs (Pollock et al., 2005). In NTLNP there is an emerging program of widespread removal of livestock and fenced enclosures as part of the national park conservation management plan (Song, 2020); hence, a narrow opportunity to sample longstanding enclosures exists before fences are removed.
In this paper we seek to advance our understanding of seasonal cattle grazing in forests in China. We are aware that livestock grazing within protected areas is a highly contested area, both globally and within China, and within our team there are differences of opinion over this issue. Our aim is to contribute to building an objective evidence base to support data driven decision making. We do this by experimentally investigating the ecological effects of cattle grazing on vegetation and wild ungulates in longstanding enclosures (>10 years old) located within a temperate forest. We hypothesize that: (1) cattle grazing affects the distribution and abundance of wild ungulates via bottom-up effects on vegetation structure; (2) effects of cattle grazing on vegetation structure and wild ungulates vary according to cattle grazing intensity, and (3) fenced cattle grazing enclosures limit wild ungulate population density. We then discuss our results in light of current and future conservation planning with regards to cattle-wildlife coexistence.
Materials and Methods
Study Area
Hunchun National Nature Reserve (HNR, 1,092 km2), Jilin, China is the core area of NTLNP (14,600 km2), bordered by the Russian Far East and Democratic People’s Republic of Korea (Figure 1). The highest elevation is 1,477 m asl, the climate is mid-temperate maritime monsoonal, and average temperature, annual precipitation and frost-free period is 5.7°C, 618 mm, and 140–160 days, respectively. The main forest types are mixed broadleaved and secondary Mongolian oak (Quercus mongolica). Three of the main large mammal species occurring in HNR are roe deer, wild boar and sika deer (Cervus nippon), as well as Amur tiger, and Amur leopard. Hunchun is an important area for cattle farming, more so than other parts of NTLNP, and production is characterized by seasonal fenced and free-roaming forest grazing annually between May and October, with indoor stall feeding over winter.
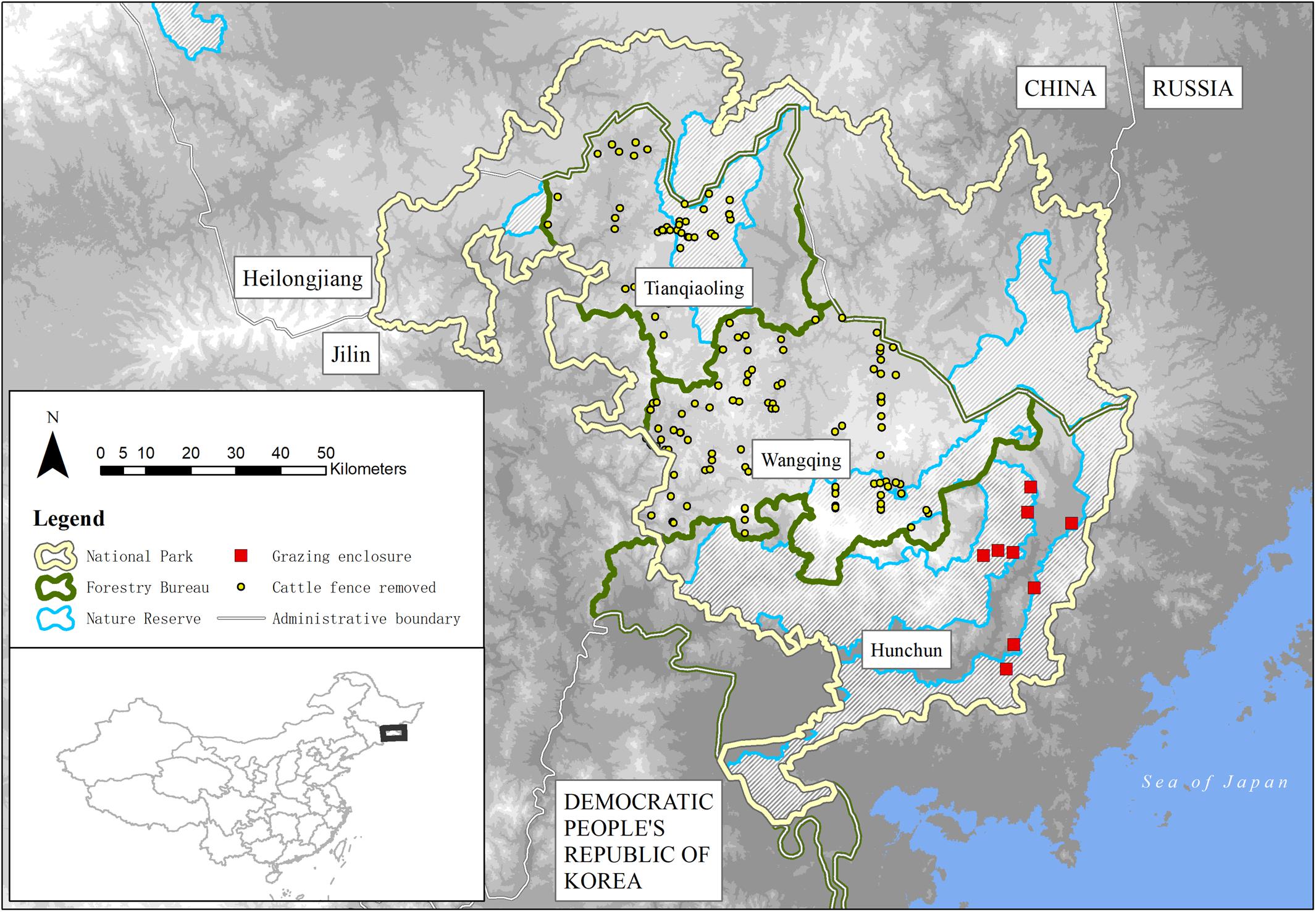
Figure 1. Forestry Bureaus (FBs) and Nature Reserves in Northeast Tiger and Leopard National Park (NTLNP), Jilin/Heilongjiang Province, China sampled by ungulate and vegetation transect surveys. Transect lines intersect grazing enclosures (see section “Materials and Methods”). Base map is a digital elevation model. Cattle fence removals are shown for Tianqiaoling (n = 55; length = 237.0 km) and Wangqing (n = 109; length = 494.8 km) FBs only and do not infer individual fence length, shape, spatial extent or completeness of data (data provided by respective FBs in December 2018 for context only). The names and boundaries shown and the designations used on this map do not imply the expression of opinion whatsoever on the part of the authors concerning the legal status of any country, territory, city or area or of its authorities, or concerning the definitions of its frontiers or boundaries.
Forest Grazing Enclosures
Unlike Wang et al. (2016, 2019), and Li Z. L. et al. (2017) who studied the associations between wildlife, plants and free-roaming forest cattle, our objective was to systematically assess the effects of cattle density on vegetation and wild ungulates by controlled experimentation in longstanding fixed enclosures in the forest. We identified 9 enclosures with access permissions which were spatially independent (Figure 1), represented cattle densities from 10.3 to 54.1 cattle per km2 (average enclosure: 2.7 km2; Supplementary Table 1) and seasonally grazed by cattle from May to October for over 10 years. Based on the survey of Li Z. L. et al. (2017) which confirmed 63 pastures in or around HNR with an average density of 11 cattle per km2, we assumed representative sampling intensity and cattle densities. Cattle were Simmental cattle and enclosures were fenced with barbed wire (1 m height, four strain), though fences were typically in poor condition and as such “semi-permeable”; there was anecdotal evidence from farmers that wildlife could get in and out easily, but cattle were unable to get out.
Vegetation and Ungulate Surveys
Work has already been done on ungulate populations and vegetation structure in summer in regards to free-ranging cattle (Wang et al., 2019), yet these factors remain unclear in the critical winter period of seasonal scarcity after cattle have been removed from the forest and are feeding indoors. In this research, in order to begin to understand how summer cattle grazing may influence winter forage availability, we walked 12 temporary 5 km transect lines which intersected cattle grazing enclosures (i.e., were part inside and part outside enclosures) between 27 and 31 January 2018 (sampling intensity greater than 10 km per 100 km2). Transects were spaced no less than 500 m apart and were entirely inside the forest. We recorded evidence of fresh (<24 h) ungulate footprints and feces in the snow, and recorded GPS coordinates of these events. Every 200 m we defined a vegetation plot of 10 m × 10 m (n = 312), identifying and counting trees larger than 2 m in height, and recording respective diameter at breast height (DBH) (Jiang et al., 2010). We placed 5 smaller quadrats of 2 m × 2 m at the corners and center inside each plot, identifying and counting the number of annual shoots of shrub species less than 1.5 m height. We subsequently calculated total tree abundance, basal area, and food abundance (shoot number) in each plot of each transect line.
Ungulate Density
To be consistent with long-established survey methods in the Sino-Russia area (Stephens et al., 2006a), we estimated ungulate density based on the number of footprints inside each enclosure and for each transect segment outside enclosures (i.e., from fence intersection to end of transect line), considering no more than 1 set of tracks per 100 m intersecting the transect line to reduce pseudo-replication. Values of 2.19 and 3.63 km were used as average daily activity distances for roe deer and wild boar in density estimate calculations, respectively (Stephens et al., 2006b).
Data Analysis
We imported all GPS points of each plot on line transects into ArcGIS and determined distances to human activities from data provided by the local Forestry Bureau. We placed a 200 m × 200 m grid over the data, pooling ungulate observations as binary presence-absence data per grid cell and averaged vegetation data and spatial data where more than one point occurred within a cell, removing non-integers. After averaging/pooling, 242 data points remained. Prior to analyses, we tested response variables for spatial autocorrelation by Moran’s I statistic, spatially rarefying occurrence data at 200 m increments, finalizing at 400 m for roe deer (Moran’s Index = −0.197, z-score = −2.071, p = 0.038) and 600 m for wild boar (Moran’s Index = −0.305, z-score = −1.665, p = 0.096) (Diniz et al., 2003; Boria et al., 2014; Brown, 2014).
We used Mann-Whitney U-test to compare ecological factors inside and outside enclosures. We calculated occurrence probabilities as generalized linear models with data from inside enclosures only, assuming some degree of physical barrier from fencing and that all enclosures were equally permeable. We used Spearman’s Rank (threshold: 0.7; R package “fuzzysim”) to first test for and remove correlated variables, and performed AUC model checking with package “Deducer.” We used a series of linear models, segmented piece regression (package “segmented”) and generalized additive models (package “mgcv”) to test for relationships between variables. We checked model performance by hat values, Cook’s distance, and visual inspection of predicted vs. observed values, and also referred to AIC values in final model selection. We computed a zero-inflated negative binomial (ZINB) model (package “pscl”) for food abundance, checked model dispersion and residuals, and additionally checked the ZINB model performance with package “DHARMa” and rootogram (package “countreg”).
Unless otherwise stated, “cattle density” throughout this manuscript refers to data inside enclosures only, i.e., does not include outside as “0.” We assume some level of free-roaming cattle grazing in our study area outside enclosures; this was not quantified here.
Results
Effects of Vegetation on Wild Ungulates
As annual shoot abundance increased, there was no effect on wild boar [R2Adjusted = 0.04, t(65) = 1.7, p = 0.099; Figure 2A] or roe deer [R2Adjusted = 0.15, t(65) = 1.7, p = 0.092; Figure 2D] density. As Q. mongolica DBH increased (up to 15.9 ± 2.2 cm), roe deer density increased [R2Adjusted = 0.45, t(39) = 4.7, p < 0.001; Figure 2E]. Where average Q. mongolica DBH was greater than this there was no further effect on roe deer density (p = 0.520; Figure 2E). Q. mongolica DBH had no relationship with wild boar density (R = 0.18, t(39) = −0.1, p = 0.934; Figure 2B). Roe deer density also increased with walnut (Juglans spp.) DBH up to 17.0 ± 3.1 cm (R2Adjusted = 0.27, t(21) = 2.6, p = 0.016; Figure 2F); beyond this DBH (or 17.7 ± 7.2 cm for wild boar), there was an observed decreasing density of wild boar (p < 0.001; Figure 2C) but no further effect on roe deer (p = 0.079; Figure 2F). Roe deer occurrence probability also increased with Juglans spp. DBH (z = 2.22, p = 0.027; Supplementary Figure 1). Roe deer density was related with total arbor tree basal area (p < 0.001; Figure 2G). A threshold was found at approximately 4,000 cm2 per 100 m2, approximately equivalent to a density of one tree of 17 cm DBH per 20 m2. Total tree abundance had no effect on wild boar (dropped from final best fitting model; Supplementary Table 2) or roe deer (z = −0.69, p = 0.488; Supplementary Table 3) occurrence probability.
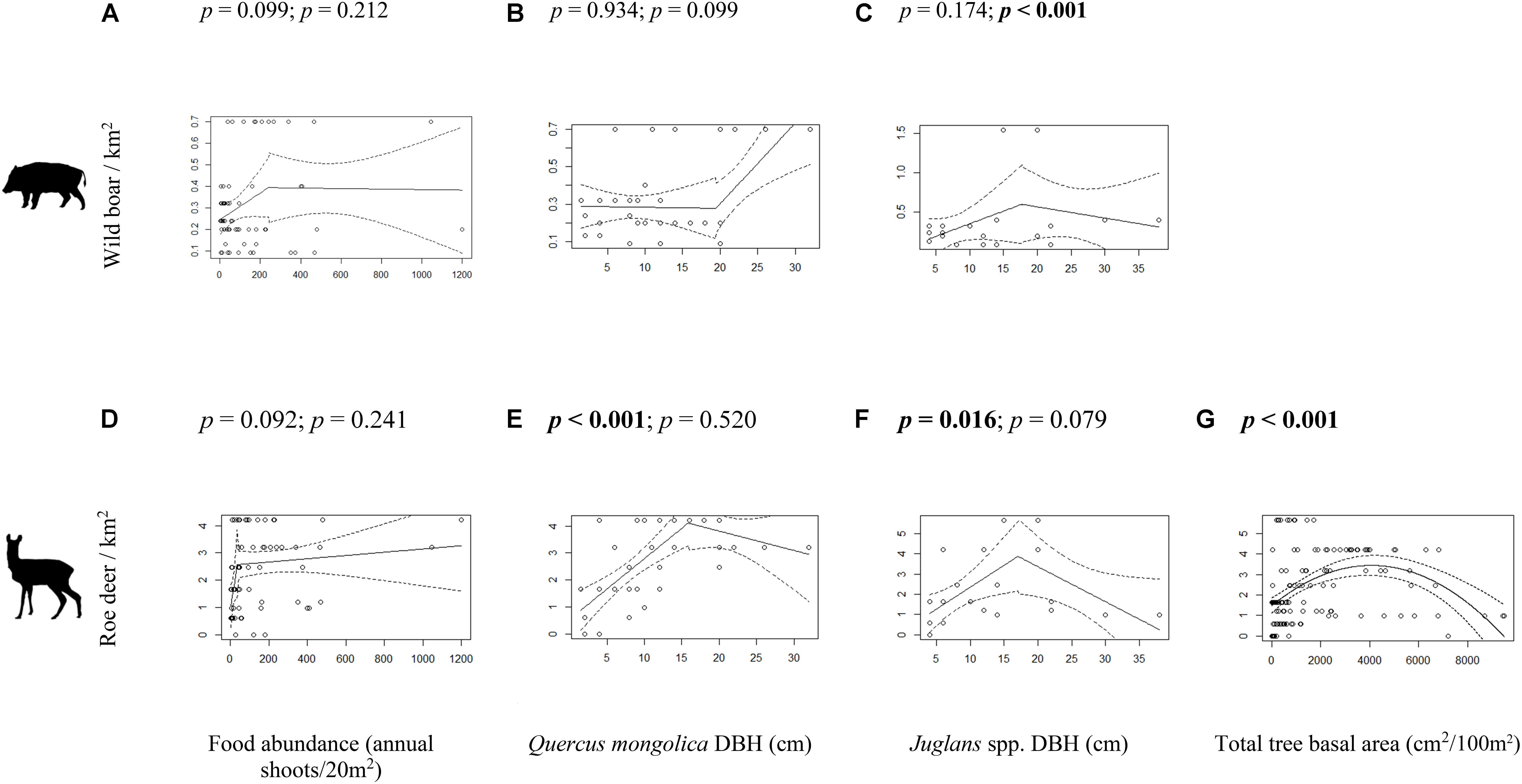
Figure 2. Wild boar (A–C) and roe deer (D–G) density inside forest cattle grazing enclosures in response to food abundance (A,D), Quercus mongolica DBH (B,E), Juglans spp. DBH (C,F) and total arbor tree basal area (G). Wild boar density modeled against total arbor tree basal area is not shown here because all candidate models did not fit the data well. p-values in bold indicate statistical significance (p < 0.05). The segmented models (A–F) show the p-value of the first slope (left) and the difference between the first and second slope (right). Note the scales of y axes.
Effects of Cattle Density and Other Human Activities on Vegetation
Inside enclosures, 49.3% of plots had zero annual shoots (outside: 26.9%). The ZINB model indicated that the chance of zero annual shoots was not related with cattle density (p = 0.056). Where these food plants were present, results indicated that their abundance was not related with cattle density (p = 0.087) but increased with distance to village highway (p = 0.008; Table 1).
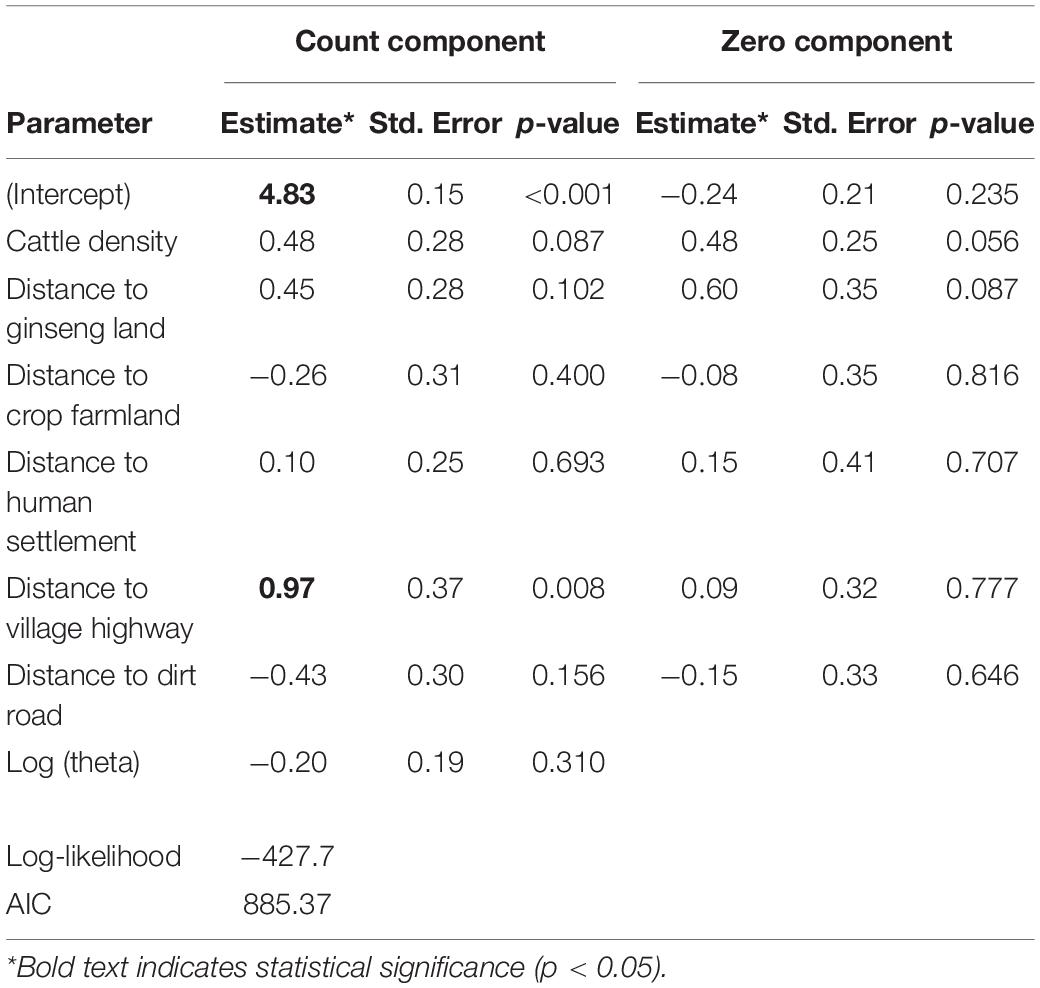
Table 1. Zero-inflated negative binomial model output of food abundance (annual shoot number) inside forest cattle grazing enclosures in response to cattle density and other anthropogenic factors.
Concerning arbor tree density and any relationship with cattle density, candidate models performed poorly, yet data points inside enclosures appeared clustered beneath a “hump-shaped curve” with lowest tree densities found at both the lowest and highest cattle densities. However, increasing cattle density was related with increased Q. mongolica DBH [R2Adjusted = 0.37, F(1, 40) = 25.39, p < 0.001; Figure 3E], Juglans spp. DBH [R2Adjusted = 0.41, F(1, 23) = 17.72, p < 0.001; Figure 3F], and total tree basal area [R2Adjusted = 0.43, t(134) = 5.4, p < 0.001; Figure 3H].
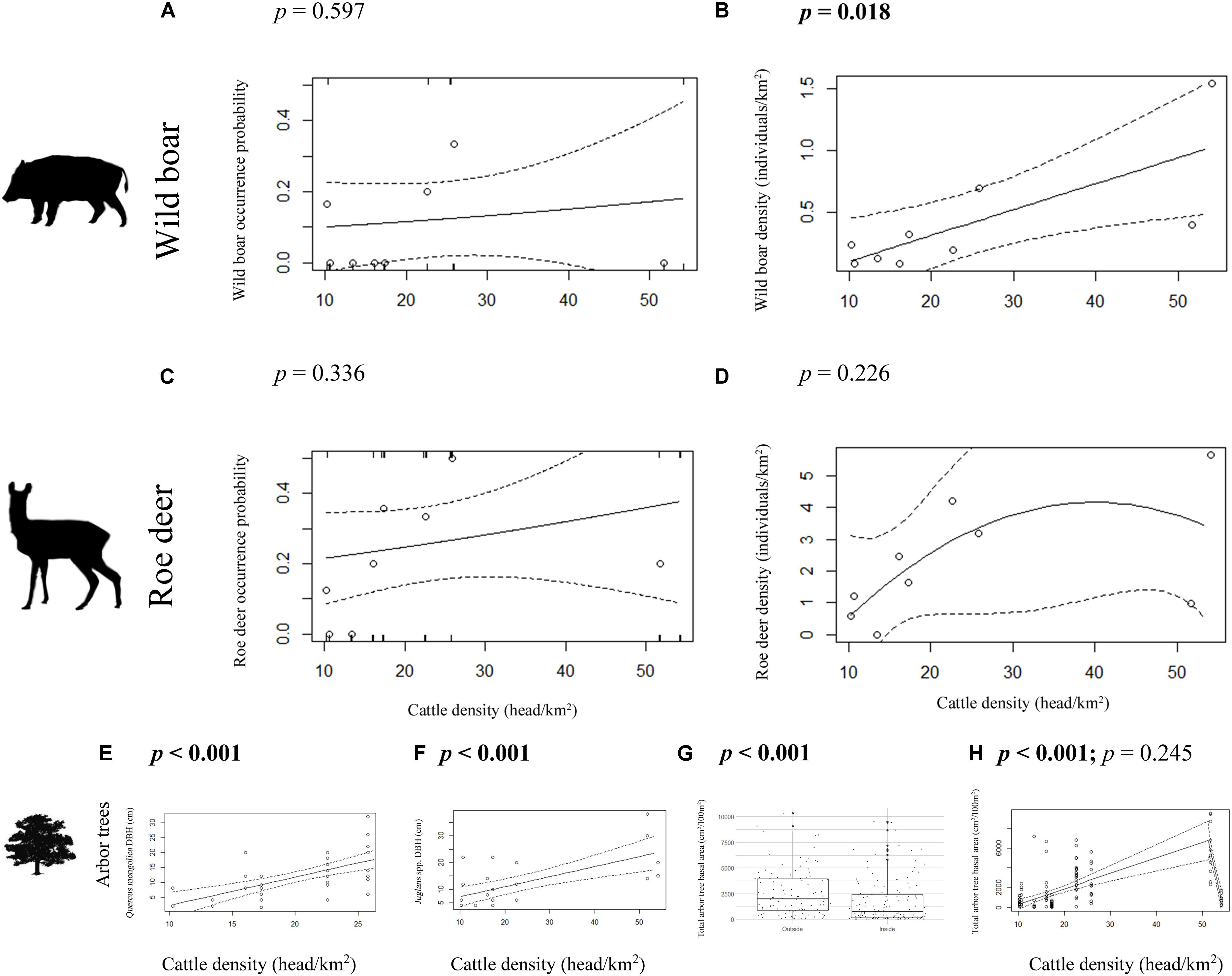
Figure 3. Wild boar (A,B) and roe deer (C,D) occurrence probability (A,C) and density (B,D), and Quercus mongolica DBH (E), Juglans spp. DBH (F), and total arbor tree basal area (H) inside forest cattle grazing enclosures in response to cattle density. Total tree basal area outside (left plot) and inside (right plot) forest cattle grazing enclosures is also shown (G). Note that we assume some level of free-roaming cattle grazing outside enclosures (see section “Materials and Methods”). p-values in bold indicate statistical significance (p < 0.05). The segmented model (H) shows the p-value of the first slope (left) and the difference between the first and second slope (right). Note the scales of x and y axes.
Effects of Cattle Density on Wild Ungulates
Wild boar density increased with cattle density [R2Adjusted = 0.51, F(1, 7) = 9.38, p = 0.0183; Figure 3B]. However, cattle density had no significant effect on wild boar (z = 0.53, p = 0.597; Figure 3A) or roe deer (z = 0.96, p = 0.336; Figure 3C) occurrence probability or roe deer density [R2Adjusted = 0.19, F(2, 6) = 0.22, p = 0.226; Figure 3D].
Effects of Enclosures on Wild Ungulates and Vegetation
Wild boar density was higher outside enclosures than inside (W = 1, p = 0.026, Figure 4A), while roe deer density was no different inside vs. outside (W = 11, p = 0.712, Figure 4B).
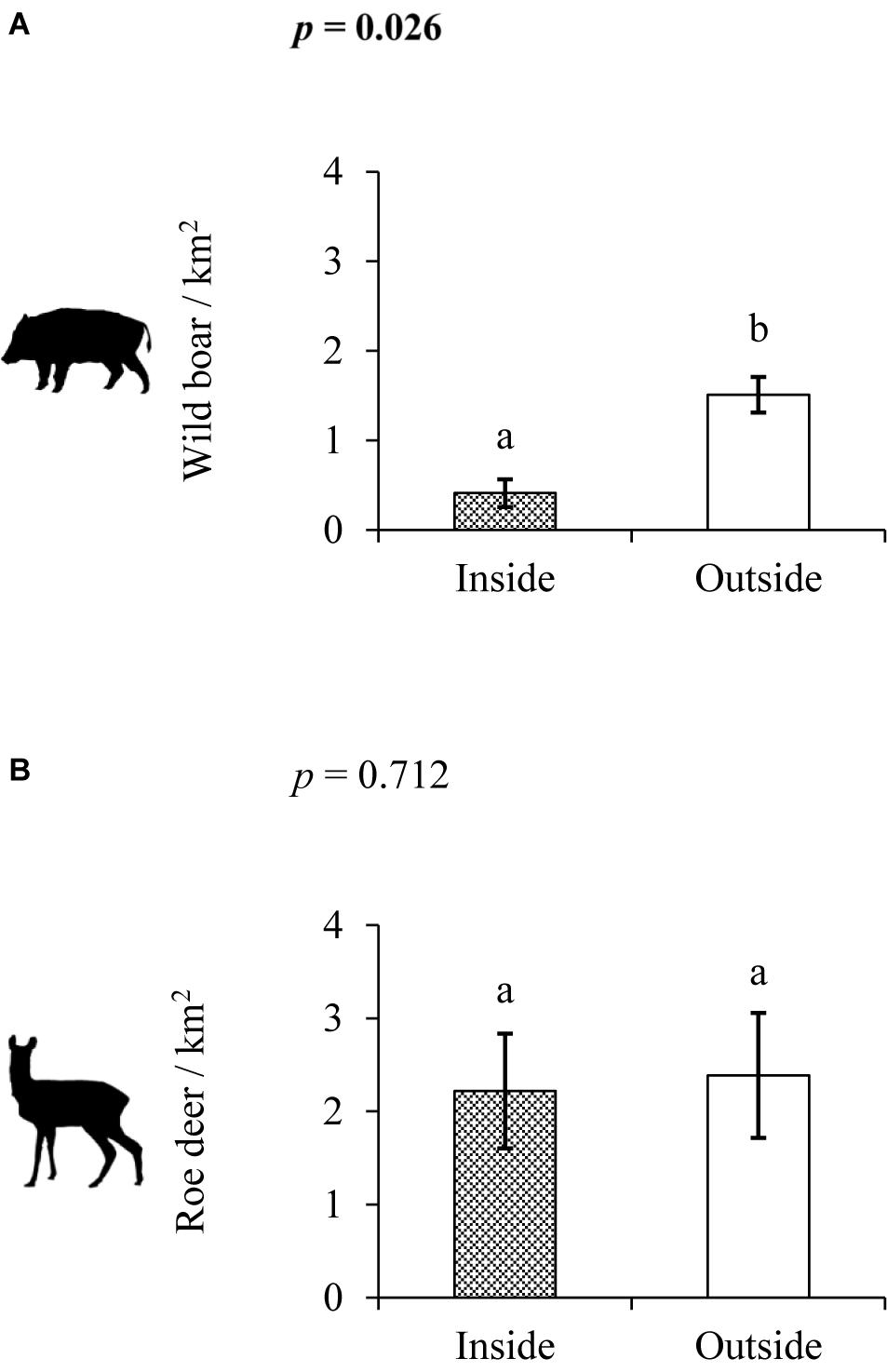
Figure 4. Wild boar (A) and roe deer (B) density inside (shaded) and outside (non-shaded) forest cattle grazing enclosures. Mean and standard errors are shown. p values in bold and different lower case letters above columns indicate statistically significant differences (p < 0.05) within each figure.
Compared with outside enclosures, inside there were fewer annual shoots (W = 4662.5, p < 0.001) and arbor trees (W = 5,831, p = 0.015) and lower basal area (W = 4,939, p < 0.001; Figure 3G); average tree DBH was higher inside (W = 11,616, p < 0.001). Densities of dominant species Q. mongolica (p = 0.320), Juglans spp. (p = 0.460), Siberian elm (Ulmus pumila, p = 0.382), poplar (Populus spp., p = 0.915), and lime (Tilia spp., p = 0.085) were approximately equal inside and outside enclosures. Manchurian ash (Fraxinus mandschurica) density was higher inside enclosures (p = 0.040).
Discussion
Increasing Wild Animal Abundance “Bottom-Up”
Wild ungulate populations in northeast China are considered to be at very low densities (Jiang and Ma, 2015). This is a significant limiting factor in the recovery of two large carnivores, Amur tiger (IUCN Red List endangered) and Amur leopard (IUCN Red List critically endangered; Karanth et al., 2004; Zhang C. Z. et al., 2013). In this paper we have identified factors associated with increasing densities of two (of three) staple ungulate prey species [roe deer, preferred by Amur leopard (Sugimoto et al., 2016), and wild boar, preferred by Amur tiger (Gu et al., 2018; Dou et al., 2019)] and evidenced that these vegetation factors are positively influenced by cattle grazing at certain densities.
First, cattle grazing probably explained lower annual shoot number, (adult) tree density and basal area inside the enclosures. We assume that these long-term ecological effects can be reasonably expected to be detectable as the enclosures have typically been active since 1970 (i.e., the beginning of the new China). Recognizing that cattle seasonally graze on woody vegetation (Cromsigt et al., 2018) and reduce Quercus spp. sapling abundance (Bernes et al., 2018), and that adult trees may have higher growth rates in forest gaps (Kramer et al., 2006), this may at least partly explain why the larger trees were found where cattle densities were higher. Acknowledging missing data on the abundance of immature trees and that bigger trees are less likely to be influenced by cattle grazing, we suggest more comprehensive research is needed to better understand forest composition/structure (including different tree age classes) and the effects of cattle grazing on forest dynamics. We add that while it may be true that cattle abundance is higher closer to human settlements (Feng R. N. et al., 2021), and by extension we might expect corresponding changes to vegetation structure and wild ungulate distribution, we found no such effects of distance to human settlement on the number of annual shoots, and though we found indications that roe deer occurrence probability increased closer to human settlements, wild ungulate abundance and occupancy results in relation to distance to human settlements are mixed, either positive (Feng R. N. et al., 2021) or statistically insignificant (Xiao et al., 2018; Feng J. W. et al., 2021).
Second, there are probably relationships between wild ungulate abundance and average (adult) tree DBH. Correlation analysis revealed that our total arbor tree basal area measurements more closely represented average tree DBH (0.75) than tree abundance (0.38), hence we suspect that a landscape of fewer, larger trees may have higher ungulate densities than a landscape of many smaller trees; the apparent ungulate preference for “open” habitats (Kuijper et al., 2009) supports this, including a wider landscape-scale study in eastern NTLNP finding that roe deer and wild boar occupancy increased with basal area and undergrowth transparency, respectively (Feng J. W. et al., 2021). Reasons for the preference for open habitats may include, for example, higher food availability (e.g., grass and forbs) and reduced predation risk from ambush predators such as tigers (Miller et al., 2015). But, as our data and ecological theory also suggests (Qi et al., 2020), thresholds in habitat suitability and grazing pressure probably exist and a certain level of regeneration (and not just mature trees) is essential for healthy ecosystem function. Understanding how these thresholds function, perhaps by integrating vegetation composition/structure data (described above) and analyzing at fine spatial scales and ecosystem levels, is a potentially important area for further study, including exploring the dynamics of predator-prey relationships in the context of habitat composition and structure.
The ecological relationships observed in this research are interpreted as cause-and-effect (i.e., the effects of cattle density and enclosures on vegetation and wild ungulates) rather than association (i.e., habitat selection by cattle) as the spatial extent of the enclosures (and herd sizes) were selected by people rather than by cattle. Though we assume some level of farmer selection bias, we assume farmer decisions were independent of adult tree DBH/basal area, wild ungulate densities and the respective statistical relationships observed. However, we recognize that this study lacks a control group for direct comparison. Prior to this research, there was understandably limited reason to fence an area to be left intentionally unused. That said, a single-year exclosure experiment in grazed areas in the eastern part of NTLNP in 2016–2017 found higher understory biomass in plots where cattle were excluded (Wang et al., 2019), effectively similar to our results. Moving forward, though exclosure-type experiments may be useful in some contexts (though see Carver and Convery, 2021 regarding fences, and Bernes et al., 2018; Li and Jiang, 2021 for meta-analyses of herbivore impacts), and there is value in determining precise thresholds, we would argue that it is important to recognize that information is always likely to be imperfect, and sometimes it is important to act based on the best available science. Perhaps the key intervention is a long-term adaptive management plan (IUCN, 2020a) that is able to respond to monitoring and emerging best practice.
The wild ungulate densities presented here should be considered as a population index rather than absolute densities. Whilst this level of certainty is sufficient for this study and enables us to achieve our primary goal of determining relationships, more definitive data may be required for further research. We recognize that mis-identification of wild animal tracks sometimes occurs, leading to erroneous abundance estimates and conclusions. To mitigate these risks as much as possible, our field personnel were trained and experienced in distinguishing species based on track morphometry (Pikunov et al., 2004), we analyzed only fresh tracks, and surveying in winter favored track detection and identification in snow as well as greater identification accuracy of pellets (for our occurrence models only) than can be expected in summer (Spitzer et al., 2019); remaining human error is assumed to be even across our dataset. Whether our results are overestimates, underestimates or near true could be a matter for debate, but evidence suggests that Formozov-Malyshev-Pereleshin (FMP) analysis (i.e., our chosen method) may provide higher abundance estimates than those derived from non-invasive genetic capture-mark-recapture analyses (Liu et al., 2015). FMP has however, already been used to estimate ungulate abundance across much of the Amur tiger range (Stephens et al., 2006a; Qi et al., 2021), offering us some reference for comparison. Our roe deer and wild boar densities are lower and higher, respectively, than those in the wider landscape (Qi et al., 2021), perhaps reflecting the spatial variability of density derived from camera trap data (Wang et al., 2016). As an alternative to FMP, camera traps may resolve species identification issues (Roberts, 2011). But, for wild ungulates which lack distinctive unique markings, camera traps could still be limited to providing an index of relative abundance rather than absolute abundance (Gilbert et al., 2020), much as we have reported here. However, new camera trap methods are emerging which may effectively estimate abundance of unmarked animals, including time-to-event and space-to-event models (Moeller et al., 2018; Loonam et al., 2020), the random encounter and staying time model (Nakashima et al., 2018, 2020), and distance sampling with camera trap images (Howe et al., 2017; Harris et al., 2020); these and other new methods could be explored and trialed in future.
For clear interpretation, we remind that all data were collected during a short period in the winter when cattle have been removed from the forest and are feeding indoors and that seasonality was not explicitly included as a variable in this research. However, we can reasonably expect that wild ungulates occur at lower abundances in winter overall (despite localized supplementary feeding; Xiao et al., 2018; Yang et al., 2018a,b; Li et al., 2019), do not exhibit considerable seasonal movement patterns relevant at the scale of this study (Hojnowski et al., 2012; Feng R. N. et al., 2021), and though we have not found any data published in either the English or Chinese language academic literature explicitly collected inside cattle grazing enclosures, summer data in eastern NTLNP suggest that wild boar and roe deer space use is independent of cattle space use (Feng R. N. et al., 2021) and that cattle presence/absence is not a significant determinant of the densities of these wild ungulates (Wang et al., 2019; Feng R. N. et al., 2021); full-year analyses however, found roe deer (but not wild boar) were more abundant where cattle were absent (Wang et al., 2017).
Though we consider our work to be one additional step in understanding cattle-wildlife interactions, our inability to include a control group limits our ability to respond to the question of whether wild ungulate abundance in winter is related with cattle grazing intensity in summer. However, perhaps more to the point and of greater practical significance, is to continue systematically measuring cattle abundance (and not just presence/absence), as it is seemingly evident that relationships with wild ungulate species, as well as community-level mammal species richness, are density dependent (see for example: Wang et al., 2016; Xiao et al., 2018; Feng J. W. et al., 2021; Feng R. N. et al., 2021). Going one step further, we suspect that constrained patterns will emerge between wild animal and cattle abundance that can be used to approximate thresholds, enabling evidence-based target setting and performance evaluation in a spatially explicit adaptive management process. Recognizing also that there will likely be seasonal and inter-annual differences in wild animal abundance and forage plant availability (Groot Bruinderink, 1994; Kramer et al., 2006), such analyses should be at appropriate spatiotemporal scales to capture the dynamic nature of ecosystem structure, animal abundance, and species-habitat interactions, including in response to changes to cattle abundance and cascading effects on vegetation structure (including composition and large-scale habitat shifts between open/closed forests), prey biomass, predator abundance and distribution, and inter-specific competition (Jiang et al., 2015; Qi et al., 2015; Li B. V. et al., 2017; Li Z. L. et al., 2017; Vijayan et al., 2017; Yang et al., 2019).
Unfortunately, our data were too few to offer new insights about sika deer, i.e., the third staple prey ungulate species. There is clearly a positive relationship between sika deer abundance and that of tigers (Wang et al., 2016). Though they may not be the preferred prey species (Kerley et al., 2015; Gu et al., 2018), a diverse prey base with availability of all three ungulate species is important for the coexistence of the two big cat species (Sugimoto et al., 2016). Others report that where cattle are abundant, sika deer are few (Wang et al., 2016; Xiao et al., 2018). To add context for the reader, and offer cautionary advice in the interpretation of literature regarding sika deer abundance in China, we note that sika deer distribution in China is highly concentrated, largely abutting the China-Russia border, clearly limited by the road that runs parallel to the border (see for example Wang et al., 2016Figure 2D). Evidently too, we note that sika deer presence/absence has been found to be more adversely affected by roads than by cattle [e.g., < 10, < 20, and < 80% of occurrence probability variance explained by livestock, primary roads and secondary roads, respectively (Li Z. L. et al., 2017)]. Thus, accepting the commonly cited recommendation to remove or reduce cattle may solve only part of the prey recovery problem.
This discussion reports objectively on the current situation, but there are alternative futures which could be more/less biodiverse depending on interventions.
Historical Reference and Alternative Futures
The ecological responses of plants and wild ungulates to cattle documented in the paper are perhaps not surprising when we consider the environments in which these species and interactions evolved. Archeological evidence reveals widespread occurrence of domestic cattle’s wild ancestor, the aurochs (Bos primigenius) in northeast China and across Eurasia including alongside today’s remnant sympatric ungulates and big cats (Norton and Gao, 2008; Zhang H. C. et al., 2013; Cai et al., 2018). Long evolutionary histories of coexistence and an understanding of differential feeding biology, niche separation and facilitation strongly supports our emerging theory that modern forest ecosystems in NTLNP (and wider landscapes) are potentially missing key ecological functions of the extinct wild cattle, especially if domestic cattle (as potential surrogates) are removed. Further, we note that the large-bodied aurochs was an abundant species in thriving ecosystems (Hofman-Kaminska et al., 2019), likely explaining the relationships observed here at higher cattle densities. However, as Gordon et al. (2021) note, whilst organizations such as Rewilding Europe have vigorously promoted the use of Heck cattle (Bos taurus), which were bred to phenotypically resemble the extinct aurochs, there is no clear evidence that “de-domesticated” breeds have the same ecology as the extinct species and, therefore, the same impact on ecological processes, or indeed the ability to survive in wild landscapes where the risks of predation are much higher. The use of such proxy species for conservation projects is therefore highly contentious. Moreover, domestic livestock are typically kept at densities much above those of wild herbivores [for example, during the summer/grazing periods 2013–2015 in NTLNP, the relative abundance of cattle was 1.17–3.40 times that of the three main wild ungulate species (Xiao et al., 2018; Li et al., 2019; Feng J. W. et al., 2021, but also see Sollmann et al., 2013 concerning relative abundance index detection bias resulting from basic ecological differences)], and hence raises potential concerns of putting in too many cattle and the potential negative impacts that would have.
There is evidence that globally, ecological traits which drive herbivore-environment and interspecific interactions (including body mass, habitat type, diet, and fermentation type) previously lost due to species extinctions, are however, being functionally replaced by approximately 39% by introducing surrogate species with similar traits to the missing species, including use of non-natives (Lundgren et al., 2020). This herbivore or trophic rewilding (“rewilding lite”) is becoming increasingly popular, particularly in Europe (though open to criticism, see Carver and Convery, 2021; Gordon et al., 2021), and so too is the practice of integrating trees, pasture and forage in a single “silvopasture” system. We recognize that rewilding is an emerging area of theory and practice (Carver et al., 2021), and that case studies of herbivore rewilding (Svenning et al., 2016; Tree, 2018; Lundgren et al., 2020) can be helpful in terms of informing conservation planning in China. We also recognize that full trophic rewilding, with the restoration of keystone carnivore species, is also vitally important in maintaining and enhancing biodiversity within landscapes. Yet we should be reminded that rewilding is not about looking back; it should be informed by past ecological conditions, rather than try to recreate them prescriptively (IUCN, 2020b).
This poses four questions: (1) If cattle are absent (or removed), will wildlife abundance and overall ecosystem structure, function and diversity (in time) be greater than if cattle are present (i.e., is there an opportunity cost)? (2) If cattle are present and managed at ecologically sustainable levels, how do we best manage predation risk, loss, and disease, including in a scenario of increased wildlife/cattle/human interaction? (3) Is there a role for large herbivore reintroductions, as in the case of sika deer and red deer (Cervus elaphus) in Wangqing in 2012 and 2013 (Shi, 2016)? and (4) If cattle are to remain within the protected area would it be better to use existing livestock breeds or “back-breed” as part of a de-domestication project?
Designing a Sustainable Forest Grazing Management Plan in a Landscape Matrix
Recognizing that it would be neither possible nor desirable to rewild everywhere (Carver and Convery, 2021), we suggest that forest cattle grazing, when viewed through the lens of conservation, is neither intrinsically “good or bad”; rather it is about making spatially and temporally informed decisions about land use. This necessarily requires an integrated, “joined-up” approach and open honest dialogue between a number of stakeholders, including farmers, conservationists, planners and government officials (amongst others; Wynne-Jones et al., 2021). Livestock grazing probably has a part to play within a landscape matrix comprising of core conservation areas and connected compatible land use where there is also connectivity for wild species alongside human activity (Carver and Convery, 2021). Furthermore, there is increasing recognition of the need to move on from the failed fortress conservation model and develop new conservation approaches that foster collaboration, community engagement and co-existence, whilst also enabling core conservation areas to become nature led and self-willed (IUCN, 2020b).
Acknowledging that in the northeast China landscape Amur tiger and Amur leopard abundance are both limited by the presence/abundance of cattle (Wang et al., 2016), and considering the aim of restoring full trophic webs of native species in core areas, there is a case to be made for removing grazing rights from core conservation areas (such as HNR). However, as the IUCN (2020b) note, monitoring is essential to provide evidence on short and medium-term results with long-term (conservation) goals in mind. To that effect we should make good use of existing long-term monitoring networks (including landscape-scale camera trap arrays throughout NTLNP) to evaluate whether wild animal populations are indeed recovering, and to adapt management plans as necessary. Moreover, recent conservation history is littered with examples of forced removals and community exclusions, and such actions rarely lead to good conservation outcomes (Ancrenaz et al., 2007; Muhumuza and Balkwill, 2013; Soliku and Schraml, 2018). Community participation and meaningful engagement will be vital if cattle grazing is to be reviewed in HNR/NTLNP.
To achieve ecologically sustainable cattle grazing, such as maintaining livestock as part of the ecosystem in areas designated as connected/compatible land use (i.e., “corridors” and/or “matrix”), we recommend assessing the current ecological impacts of domestic and wild herbivores as a key first step in determining suitable forest grazing regimes, aided by freely available tools such as the Woodland Grazing Toolbox (Scottish Forestry, 2020). Where livestock grazing might be permitted and productive, such tools should be investigated collaboratively with farmers and other relevant authorities and stakeholders, and adjusted according to site knowledge, scientific monitoring, and management objectives, to determine density- and/or duration-based management plans. We note that seasonal grazing with winter stall feeding indoors may be preferred for practical reasons, but moderate year-round “natural grazing” outdoors (Vermeulen, 2015) is often preferable as densities are naturally regulated by winter food availability, reducing risk of overgrazing typical of seasonal regimes (Goderie et al., 2013; Tofastrud et al., 2020). In relation to diet selection and density effects, we can expect cattle at moderate densities to have limited effect on woody vegetation (Calleja et al., 2019), and short duration, high density “boom and bust” grazing may reduce woody vegetation but compromise livestock health and production (Teruel-Coll et al., 2019). Ultimately, appropriate grazing is essential to maintain ecosystem function, structure and diversity; if there is evidence of serious degradation caused by years of overgrazing, grazing reduction or exclusion may be a more appropriate option.
In this study, forest cattle grazing enclosures were found to have little direct effect on roe deer but were likely directly limiting wild boar density. The large-scale fence removal across NTLNP since our data collection (Figure 1) is probably, therefore, conducive to wildlife restoration; we strongly encourage evaluation of effectiveness. Furthermore, it is important to understand how fence removal might impact livestock management as there could be implications for farming practice, including identifying stock ownership, managing production yield and loss, and locating the herd. Our research highlights distinct effects of fenced enclosures and cattle density, and we suggest these should be considered as two separate factors in management decisions and evaluations. In practice this may mean the removal of fences and the management of free-roaming cattle at appropriate densities.
Although well managed forest cattle grazing can deliver direct biodiversity benefits (Hancock et al., 2010; England et al., 2020), increase livestock health and production efficiency (Paciullo et al., 2011; England et al., 2020; Tofastrud et al., 2020), and have lower externalized environmental and economic costs compared with crop-fed animal agriculture (Gerten et al., 2020), evidence suggests that livestock depredation risk increases with tree cover (Soh et al., 2014). In and around HNR, livestock losses to predators may be considered numerically few [average 26 cattle per year 2002–2007 (Li et al., 2009) and 53 cattle per year 2008–2014 (Wang et al., 2016); total cattle holdings (in Hunchun): 65,420 in 2008 and 36,320 in 2014 (Wang, 2019)], and though a livestock compensation scheme exists, there are concerns that the financial system unintentionally counters conservation efforts (Li Z. L. et al., 2017). To address these concerns, we propose a shift from these loss-based economics to provision-based economics such as Payments for Ecosystem Services/“eco-compensation” including carbon accounting, recognizing that the carbon sequestration potential of silvopasture is greater than for all other climate change mitigation solutions in the entire agriculture sector, and one of the greatest overall across all solutions assessed (Project Drawdown, 2020).
Finally, we recognize that ungulate density is not only influenced by habitat suitability. Between 2009–2012, patrol teams encountered 233 ungulate snares in and around HNR (Soh et al., 2014). Taking into account imperfect detection of snares and spatial and temporal variability, increasing patrolling and community engagement are recommended in order to reduce human-caused ungulate mortalities and the risk of sink-like habitats emerging, providing employment and education opportunities in core areas and multi-use landscapes (Zhou and Zhang, 2011).
Conclusion and Priorities
Building on the evidence base concerning livestock-wildlife interactions, our research offers new insights about how cattle grazing in forests modifies vegetation structure and how these changes correspond with wild ungulate density, demonstrating mechanisms of coexistence and facilitation and the distinction between effects of cattle density and fences. Recognizing the urgent need for new models in conservation and food production in this crucial decade of ecosystem restoration (Convention on Biological Diversity [CBD], 2020), and global targets to increase coverage of protected areas (Yang et al., 2020), we call upon land use managers and decision makers to respond to these needs in context. While cattle grazing in forests is not intrinsically “right or wrong,” and what works in one location may not necessarily work elsewhere, we argue that there is a strong case for an integrated approach to land use which is objective, spatially and temporally grounded, and based on sound ecological evidence and community engagement, with core areas, connectivity and coexistence (the new 3Cs of conservation, Carver and Convery, 2021).
Moving forward, the key priorities to pursue in order to effectively deliver this long-term holistic restoration plan and vision of ecological civilization in China are: (1) Consider livestock grazing alongside other land use options as part of an integrated approach to land management, comprised of core conservation areas within a matrix of connected compatible land use. (2) In areas where livestock grazing is permitted, validate cattle-vegetation-wildlife ecological relationships and develop grazing plans which foster productivity, coexistence, and provision of ecosystem services. (3) Monitor ecosystem change and collect evidence of interventions systematically and collaboratively, adopting an adaptive management approach which balances human influence and self-regulation of ecosystems.
Data Availability Statement
The raw data supporting the conclusions of this article will be made available by the authors, without undue reservation.
Ethics Statement
Ethical review and approval was not required for the animal study because this research has used non-invasive field methods of wild animals. Written informed consent for participation was not obtained from the owners because field sampling was undertaken in grazing areas when livestock animals were absent and permissions were verbal.
Author Contributions
NR: formal analysis, writing—original draft, and visualization. YZ: conceptualization, methodology, formal analysis, and investigation. IC: writing—original draft. XL: formal analysis and investigation. DS: writing—review and editing. GJ: conceptualization, methodology, writing—original draft, supervision, and funding acquisition. All authors contributed to the article and approved the submitted version.
Funding
This research was funded by the National Natural Science Foundation of China (NSFC 51931872241) and the Biodiversity Survey, Monitoring and Assessment Project of Ministry of Ecology and Environment, China (2019HB2096001006).
Conflict of Interest
The authors declare that the research was conducted in the absence of any commercial or financial relationships that could be construed as a potential conflict of interest.
Publisher’s Note
All claims expressed in this article are solely those of the authors and do not necessarily represent those of their affiliated organizations, or those of the publisher, the editors and the reviewers. Any product that may be evaluated in this article, or claim that may be made by its manufacturer, is not guaranteed or endorsed by the publisher.
Acknowledgments
We thank J. Lai and D. Zhang for assisting data collection and processing, J. Qi and H. Bao for assisting with analysis and interpretation, the reviewers for helping us to improve the manuscript, H. Cao, D. Wen, and S. Liu for graphic visualization, and family and friends for valued discussion. We sincerely thank Hunchun Forestry Bureau for permitting and assisting with fieldwork. NR acknowledges the support from China Scholarship Council.
Supplementary Material
The Supplementary Material for this article can be found online at: https://www.frontiersin.org/articles/10.3389/fevo.2021.680367/full#supplementary-material
References
Ancrenaz, M., Dabek, L., and O’Neil, S. (2007). The costs of exclusion: recognizing a role for local communities in biodiversity conservation. PLoS Biol. 5:e289. doi: 10.1371/journal.pbio.0050289
Bai, Z. H., Ma, W. Q., Ma, L., Velthof, G. L., Wei, Z. B., Havlik, P., et al. (2018). China’s livestock transition: driving forces, impacts, and consequences. Sci. Adv. 4:eaar8534. doi: 10.1126/sciadv.aar8534
Bar-On, Y. M., Phillips, R., and Milo, R. (2018). The biomass distribution on Earth. Proc. Natl. Acad. Sci. U. S. A. 115, 6506–6511. doi: 10.1073/pnas.1711842115
Bernes, C., Macura, B., Jonsson, B. G., Junninen, K., Muller, J., Sandstrom, J., et al. (2018). Manipulating ungulate herbivory in temperate and boreal forests: effects on vegetation and invertebrates: a systematic review. Environ. Evid. 7:13. doi: 10.1186/s13750-018-0125-3
Boria, R. A., Olson, L. E., Goodman, S. M., and Anderson, R. P. (2014). Spatial filtering to reduce sampling bias can improve the performance of ecological niche models. Ecol. Model. 275, 73–77. doi: 10.1016/j.ecolmodel.2013.12.012
Brown, J. L. (2014). SDMtoolbox: a python-based GIS toolkit for landscape genetic, biogeographic and species distribution model analyses. Methods Ecol. Evol. 5, 694–700. doi: 10.1111/2041-210x.12200
Cai, D. W., Zhang, N. F., Zhu, S. Q., Chen, Q. J., Wang, L. X., Zhao, X., et al. (2018). Ancient DNA reveals evidence of abundant aurochs (Bos primigenius) in Neolithic Northeast China. J. Archaeol. Sci. 98, 72–80. doi: 10.1016/j.jas.2018.08.003
Calleja, J. A., Escola, M., Carvalho, J., Forcadell, J. M., Serrano, E., and Bartolome, J. (2019). Cattle grazing fails to control shrub encroachment in mediterranean landscapes. Rangel. Ecol. Manag. 72, 803–811. doi: 10.1016/j.rama.2019.04.005
Caro, T. M. (2010). Conservation by Proxy: Indicator, Umbrella, Keystone, Flagship, and Other Surrogate Species. Washington, DC: Island Press.
Carver, S., and Convery, I. (2021). Rewilding: time to get down off the fence? Br. Wildlife 32, 246–255.
Carver, S., Convery, I., Hawkins, S., Beyers, R., Eagle, A., Kun, Z., et al. (2021). Guiding principles for rewilding. Conserv. Biol. doi: 10.1002/cobi.13730
Chen, G., Li, Y. X., Teng, L. W., Liu, Z. S., Sun, H., and Teng, Y. F. (2014). Winter diet composition of Capreolus pygargus in Liangshui national nature reserve, heilongjiang province, China. J. Econ. Anim. 18, 91–95.
Convention on Biological Diversity [CBD] (2020). Report of the Open-Ended Working Group on the Post-2020 Global Biodiversity Framework on its Second Meeting. Rome: Convention on Biological Diversity.
Cromsigt, J. P. G. M., Kemp, Y. J. M., Rodriguez, E., and Kivit, H. (2018). Rewilding Europe’s large grazer community: how functionally diverse are the diets of European bison, cattle, and horses? Restor. Ecol. 26, 891–899. doi: 10.1111/rec.12661
Diniz, J. A. F., Bini, L. M., and Hawkins, B. A. (2003). Spatial autocorrelation and red herrings in geographical ecology. Glob. Ecol. Biogeogr. 12, 53–64. doi: 10.1046/j.1466-822X.2003.00322.x
Dou, H. L., Yang, H. T., Smith, J. L. D., Feng, L. M., Wang, T. M., and Ge, J. P. (2019). Prey selection of Amur tigers in relation to the spatiotemporal overlap with prey across the Sino-Russian border. Wildlife Biol. 2019:wlb.00508. doi: 10.2981/wlb.00508
England, J. R., O’Grady, A. P., Fleming, A., Marais, Z., and Mendham, D. (2020). Trees on farms to support natural capital: an evidence-based review for grazed dairy systems. Sci. Total Environ. 704:135345. doi: 10.1016/j.scitotenv.2019.135345
Faust, C., Eichberg, C., Storm, C., and Schwabe, A. (2011). Post-dispersal impact on seed fate by livestock trampling – A gap of knowledge. Basic Appl. Ecol. 12, 215–226. doi: 10.1016/j.baae.2011.02.009
Feng, J. W., Sun, Y. F., Li, H. L., Xiao, Y. Q., Zhang, D. D., Smith, J. L. D., et al. (2021). Assessing mammal species richness and occupancy in a Northeast Asian temperate forest shared by cattle. Divers. Distrib. 27, 857–872. doi: 10.1111/ddi.13237
Feng, R. N., Lu, X. Y., Xiao, W. H., Feng, J. W., Sun, Y. F., Guan, Y., et al. (2021). Effects of free-ranging livestock on sympatric herbivores at fine spatiotemporal scales. Landsc. Ecol. 36, 1441–1457. doi: 10.1007/s10980-021-01226-6
Feng, Y. (2017). Winter Nutritional Strategy of the Red Deer (Cervus elaphus xanthopygus) in the Muling Forest Region. Harbin: Northeast Forestry University.
Food and Agriculture Organization [FAO] (2006). Livestock’s Long Shadow: Environmental Issues and Options. Rome: Food and Agriculture Organization of the United Nations.
Food and Agriculture Organization [FAO] (2018). World Livestock: Transforming the Livestock Sector Through the Sustainable Development Goals. Rome: Food and Agriculture Organization of the United Nations.
Gerten, D., Heck, V., Jaegermeyr, J., Bodirsky, B. L., Fetzer, I., Jalava, M., et al. (2020). Feeding ten billion people is possible within four terrestrial planetary boundaries. Nat. Sustain. 3, 200–208. doi: 10.1038/s41893-019-0465-1
Gilbert, N. A., Clare, J. D. J., Stenglein, J. L., and Zuckerberg, B. (2020). Abundance estimation of unmarked animals based on camera-trap data. Conserv. Biol. 35, 88–100. doi: 10.1111/cobi.13517
Goderie, R., Helmer, W., Kerkdijk-Otten, H., and Widstrand, S. (2013). The Aurochs - Born to be Wild: The Comeback of a European Icon. Zutphen: Roodbont Publishers.
Gordon, I. J., Pérez-Barbería, F. J., and Manning, A. D. (2021). Rewilding lite: using traditional domestic livestock to achieve rewilding outcomes. Sustainability 13:3347. doi: 10.3390/su13063347
Groot Bruinderink, G. W. T. A. (1994). Diet and condition of wild boar, Sus scrofa L., without supplementary feeding. J. Zool. 233, 631–648. doi: 10.1111/j.1469-7998.1994.tb05370.x
Gu, J., Yu, L., Hua, Y., Ning, Y., Heng, B., Qi, J., et al. (2018). A comparison of food habits and prey preferences of Amur tiger (Panthera tigris altaica) at the southwest Primorskii Krai in Russia and Hunchun in China. Integr. Zool. 13, 595–603. doi: 10.1111/1749-4877.12322
Hancock, M. H., Summers, R. W., Amphlett, A., Willi, J., Servant, G., and Hamilton, A. (2010). Using cattle for conservation objectives in a Scots pine Pinus sylvestris forest: results of two trials. Eur. J. Forest Res. 129, 299–312. doi: 10.1007/s10342-009-0330-2
Harris, G. M., Butler, M. J., Stewart, D. R., Rominger, E. M., and Ruhl, C. Q. (2020). Accurate population estimation of Caprinae using camera traps and distance sampling. Sci. Rep. 10:17729. doi: 10.1038/s41598-020-73893-5
He, H., Zhang, W. Q., Liu, R., and Zhang, M. H. (2015). Relationship between the composition and tannin content in winter food for roe deer at Zhanhe forest farm of Lesser Xingan Mountains. J. Beijing Forest. Univ. 37, 121–127.
Hebblewhite, M., Zimmermann, F., Li, Z., Miquelle, D. G., Zhang, M., Sun, H., et al. (2012). Is there a future for Amur tigers in a restored tiger conservation landscape in Northeast China? Anim. Conserv. 15, 579–592. doi: 10.1111/j.1469-1795.2012.00552.x
Hofman-Kaminska, E., Bocherens, H., Drucker, D. G., Fyfe, R. M., Guminski, W., Makowiecki, D., et al. (2019). Adapt or die-Response of large herbivores to environmental changes in Europe during the Holocene. Glob. Change Biol. 25, 2915–2930. doi: 10.1111/gcb.14733
Hofmann, R. R. (1989). Evolutionary steps of ecophysiological adaptation and diversification of ruminants: a comparative view of their digestive system. Oecologia 78, 443–457. doi: 10.1007/BF00378733
Hojnowski, C. E., Miquelle, D. G., Myslenkov, A. I., Strindberg, S., Smirnov, E. N., and Goodrich, J. M. (2012). Why do Amur tigers maintain exclusive home ranges? Relating ungulate seasonal movements to tiger spatial organization in the Russian Far East. J. Zool. 287, 276–282. doi: 10.1111/j.1469-7998.2012.00913.x
Howe, E. J., Buckland, S. T., Després-Einspenner, M.-L., and Kühl, H. S. (2017). Distance sampling with camera traps. Methods Ecol. Evol. 8, 1558–1565. doi: 10.1111/2041-210X.12790
IUCN (2020a). “IUCN SSC Position Statement on the Management of Human-Wildlife Conflict”. (IUCN Species Survival Commission (SSC) Human-Wildlife Conflict Task Force. Available online at: www.iucn.org/theme/species/publications/policies-and-position-statements) (accessed February 2, 2021).
IUCN (2020b). “IUCN CEM Principles of Rewilding”. IUCN CEM Rewilding Thematic Group. Available online at: https://www.iucn.org/sites/dev/files/content/documents/principles_of_rewilding_cem_rtg.pdf (accessed July 20, 2021).
Jiang, G. S., and Ma, J. Z. (2015). Population and Habitat of Amur Leopard in China. Beijing: Science Press.
Jiang, G. S., Ma, J. Z., Zhang, M. H., and Stott, P. (2010). Multi-scale foraging habitat use and interactions by sympatric cervids in Northeastern China. J. Wildlife Manag. 74, 678–689. doi: 10.2193/2009-214
Jiang, G. S., Qi, J. Z., Wang, G. M., Shi, Q. H., Darman, Y., Hebblewhite, M., et al. (2015). New hope for the survival of the Amur leopard in China. Sci. Rep. 5:15475. doi: 10.1038/srep15475
Karanth, K. U., Nichols, J. D., Kumar, N. S., Link, W. A., and Hines, J. E. (2004). Tigers and their prey: predicting carnivore densities from prey abundance. Proc. Natl. Acad. Sci. U.S.A. 101, 4854–4858. doi: 10.1073/pnas.0306210101
Kerley, L. L., Mukhacheva, A. S., Matyukhina, D. S., Salmanova, E., Salkina, G. P., and Miquelle, D. G. (2015). A comparison of food habits and prey preference of Amur tiger (Panthera tigris altaica) at three sites in the Russian Far East. Integr. Zool. 10, 354–364. doi: 10.1111/1749-4877.12135
Kohler, F., Gillet, F., Reust, S., Wagner, H. H., Gadallah, F., Gobat, J. M., et al. (2006). Spatial and seasonal patterns of cattle habitat use in a mountain wooded pasture. Landsc. Ecol. 21, 281–295. doi: 10.1007/s10980-005-0144-7
Kramer, K., Bruinderink, G. W. T. A. G., and Prins, H. H. T. (2006). Spatial interactions between ungulate herbivory and forest management. Forest Ecol. Manag. 226, 238–247. doi: 10.1016/j.foreco.2006.01.037
Krzic, M., Newman, R. F., Trethewey, C., Bulmer, C. E., and Chapman, B. K. (2006). Cattle grazing effects on plant species composition and soil compaction on rehabilitated forest landings in central interior British Columbia. J. Soil Water Conserv. 61, 137–144.
Kuijper, D. P. J., Cromsigt, J. P. G. M., Churski, M., Adam, B., Jedrzejewska, B., and Jedrzejewski, W. (2009). Do ungulates preferentially feed in forest gaps in European temperate forest? Forest Ecol. Manag. 258, 1528–1535. doi: 10.1016/j.foreco.2009.07.010
Li, B. V., and Jiang, B. (2021). Responses of forest structure, functions, and biodiversity to livestock disturbances: a global meta-analysis. Glob. Change Biol. 27, 4745–4757. doi: 10.1111/gcb.15781
Li, B. V., Pimm, S. L., Li, S., Zhao, L. J., and Luo, C. P. (2017). Free-ranging livestock threaten the long-term survival of giant pandas. Biol. Conserv. 216, 18–25. doi: 10.1016/j.biocon.2017.09.019
Li, B., Zhang, E., and Liu, Z. (2009). Livestock depredation by Amur tigers in hunchun nature reserve, Jilin, China. Acta Theriol. Sin. 29, 231–238.
Li, L. Y., Teng, L. W., Liu, Z. S., Li, Y. X., and Teng, Y. F. (2014). Winter diet composition of Capreolus pygargus in Sanjiang National Nature Reserve, Heilongjiang Province, China. J. Econ. Anim. 18, 29–32.
Li, Z. L., Kang, A. L., Gu, J. Y., Xue, Y. G., Ren, Y., Zhu, Z. W., et al. (2017). Effects of human disturbance on vegetation, prey and Amur tigers in Hunchun Nature Reserve, China. Ecol. Model. 353, 28–36. doi: 10.1016/j.ecolmodel.2016.08.014
Li, Z. L., Wang, T. M., Smith, J. L. D., Feng, R. N., Feng, L. M., Mou, P., et al. (2019). Coexistence of two sympatric flagship carnivores in the human-dominated forest landscapes of Northeast Asia. Landsc. Ecol. 34, 291–305. doi: 10.1007/s10980-018-0759-0
Littlewood, N. A., Rocha, R., Smith, R. K., Martin, P. A., Lockhart, S. L., Schoonover, R. F., et al. (2020). Terrestrial Mammal Conservation: Global Evidence for the Effects of Interventions for Terrestrial Mammals Excluding Bats and Primates. Synopses of Conservation Evidence Series. Cambridge: University of Cambridge. doi: 10.11647/obp.0234
Liu, H., Jiang, G., and Li, H. (2015). A comparative study on four survey methods used in ungulate population size estimation in winter in North China. Acta Ecol. Sin. 35, 3076–3086. doi: 10.5846/stxb201308172102
Loonam, K., Ausband, D. E., Lukacs, P. M., Mitchell, M. S., and Robinson, H. S. (2020). Estimating abundance of an unmarked, low-density species using Cameras. J. Wildlife Manag. 85, 87–96. doi: 10.1002/jwmg.21950
Lundgren, E. J., Ramp, D., Rowan, J., Middleton, O., Schowanek, S. D., Sanisidro, O., et al. (2020). Introduced herbivores restore Late Pleistocene ecological functions. Proc. Natl. Acad. Sci. U.S.A. 117, 7871–7878. doi: 10.1073/pnas.1915769117
Miller, J. R. B., Jhala, Y. V., Jena, J., and Schmitz, O. J. (2015). Landscape-scale accessibility of livestock to tigers: implications of spatial grain for modeling predation risk to mitigate human-carnivore conflict. Ecol. Evol. 5, 1354–1367. doi: 10.1002/ece3.1440
Ministry of Agriculture in China [MOA] (2014). Ministry of Agriculture. Available online at: www.moa.gov.cn/govpublic/XMYS/201604/t20160420_5101912.htm (accessed March 16, 2021).
Moeller, A. K., Lukacs, P. M., and Horne, J. S. (2018). Three novel methods to estimate abundance of unmarked animals using remote cameras. Ecosphere 9:e02331. doi: 10.1002/ecs2.2331
Muhumuza, M., and Balkwill, K. (2013). Factors affecting the success of conserving biodiversity in National Parks: a review of case studies from Africa. Int. J. Biodiv. 2013:798101. doi: 10.1155/2013/798101
Nakashima, Y., Fukasawa, K., and Samejima, H. (2018). Estimating animal density without individual recognition using information derivable exclusively from camera traps. J. Appl. Ecol. 55, 735–744. doi: 10.1111/1365-2664.13059
Nakashima, Y., Hongo, S., and Akomo-Okoue, E. F. (2020). Landscape-scale estimation of forest ungulate density and biomass using camera traps: applying the REST model. Biol. Conserv. 241:108381. doi: 10.1016/j.biocon.2019.108381
Norton, C. J., and Gao, X. (2008). Hominin-carnivore interactions during the Chinese early Paleolithic: Taphonomic perspectives from Xujiayao. J. Hum. Evol. 55, 164–178. doi: 10.1016/j.jhevol.2008.02.006
Paciullo, D. S. C., de Castro, C. R. T., Gomide, C. A. D., Mauricio, R. M., Pires, M. D. A., Muller, M. D., et al. (2011). Performance of dairy heifers in a silvopastoral system. Livestock Sci. 141, 166–172. doi: 10.1016/j.livsci.2011.05.012
Pikunov, D. G., Miquelle, D. G., Dunishenko, Y. M., Myslenkov, A. I., Nikolaev, I. G., and Seryodkin, I. V. (2004). A Field Guide to Animal Tracks of the Far East. Vladivostok: Dalnauka.
Pollock, M. L., Milner, J. M., Waterhouse, A., Holland, J. P., and Legg, C. J. (2005). Impacts of livestock in regenerating upland birch woodlands in Scotland. Biol. Conserv. 123, 443–452. doi: 10.1016/j.biocon.2005.01.006
Qi, J. Z., Holyoak, M., Ning, Y., and Jiang, G. S. (2020). Ecological thresholds and large carnivores conservation: implications for the Amur tiger and leopard in China. Glob. Ecol. Conserv. 21:e00837. doi: 10.1016/j.gecco.2019.e00837
Qi, J. Z., Shi, Q. H., Wang, G. M., Li, Z. L., Sun, Q., Hua, Y., et al. (2015). Spatial distribution drivers of Amur leopard density in northeast China. Biol. Conserv. 191, 258–265. doi: 10.1016/j.biocon.2015.06.034
Qi, J., Gu, J., Ning, Y., Miquelle, D. G., Holyoak, M., Wen, D., et al. (2021). Integrated assessments call for establishing a sustainable meta-population of Amur tigers in northeast Asia. Biol. Conserv. 261:109250.
Ripple, W. J., Newsome, T. M., Wolf, C., Dirzo, R., Everatt, K. T., Galetti, M., et al. (2015). Collapse of the world’s largest herbivores. Sci. Adv. 1:e1400103. doi: 10.1126/sciadv.1400103
Roberts, N. J. (2011). Investigation into survey techniques of large mammals: surveyor competence and camera-trapping vs. transect-sampling. Biosci. Horizons Int. J. Student Res. 4, 40–49. doi: 10.1093/biohorizons/hzr006
Shi, Q. (2016). Prey Recovery for Amur Tiger in the Changbai Mountains – Adaptability of Sika Deer reintroduction. Harbin: Northeast Forestry University.
Soh, Y. H., Carrasco, L. R., Miquelle, D. G., Jiang, J. S., Yang, J., Stokes, E. J., et al. (2014). Spatial correlates of livestock depredation by Amur tigers in Hunchun, China: relevance of prey density and implications for protected area management. Biol. Conserv. 169, 117–127. doi: 10.1016/j.biocon.2013.10.011
Soliku, O., and Schraml, U. (2018). Making sense of protected area conflicts and management approaches: a review of causes, contexts and conflict management strategies. Biol. Conserv. 222, 136–145.
Sollmann, R., Mohamed, A., Samejima, H., and Wilting, A. (2013). Risky business or simple solution – relative abundance indices from camera-trapping. Biol. Conserv. 159, 405–412. doi: 10.1016/j.biocon.2012.12.025
Song, T. (2020). The exploration of China’s National Park system pilot project: taking Northeast China Tiger and Leopard national park system Pilot area as an example. Int. J. Geoheritage Parks 8, 203–209.
Spitzer, R., Churski, M., Felton, A., Heurich, M., Kuijper, D. P. J., Landman, M., et al. (2019). Doubting dung: eDNA reveals high rates of misidentification in diverse European ungulate communities. Eur. J. Wildlife Res. 65:28.
Stephens, P. A., Zaumyslova, O. Y., Miquelle, D. G., Myslenkov, A. I., and Hayward, G. D. (2006a). Estimating population density from indirect sign: track counts and the Formozov-Malyshev-Pereleshin formula. Anim. Conserv. 9, 339–348. doi: 10.1111/j.1469-1795.2006.00044.x
Stephens, P. A., Zaumyslova, O. Y., Hayward, G. D., and Miquelle, D. G. (2006b). Analysis of the Long-Term Dynamics of Ungulates in Sikhote-Alin Zapovednik, Russian Far East. A Report to the Sikhote-Alin Zapovednik and USDA Forest Service. Bristol: University of Bristol.
Sugimoto, T., Aramilev, V. V., Nagata, J., and McCullough, D. R. (2016). Winter food habits of sympatric carnivores, Amur tigers and Far Eastern leopards, in the Russian Far East. Mamm. Biol. 81, 214–218. doi: 10.1016/j.mambio.2015.12.002
Svenning, J. C., Pedersen, P. B. M., Donlan, C. J., Ejrnaes, R., Faurby, S., Galetti, M., et al. (2016). Science for a wilder Anthropocene: synthesis and future directions for trophic rewilding research. Proc. Natl. Acad. Sci. U.S.A. 113, 898–906. doi: 10.1073/pnas.1502556112
Teruel-Coll, M., Pareja, J., Bartolome, J., Serrano, E., Mentaberre, G., Cuenca, R., et al. (2019). Effects of boom and bust grazing management on vegetation and health of beef cattle used for wildfire prevention in a Mediterranean forest. Sci. Total Environ. 665, 18–22.
Thakur, A. K., Singh, G., Singh, S., and Rawat, G. S. (2011). Impact of Pastoral practices on forest cover and regeneration in the outer fringes of Kedarnath wildlife sanctuary, Western Himalaya. J. Indian Soc. Remote Sens. 39, 127–134. doi: 10.1007/s12524-011-0079-1
Tofastrud, M., Hessle, A., Rekdal, Y., and Zimmermann, B. (2020). Weight gain of free-ranging beef cattle grazing in the boreal forest of south-eastern Norway. Livestock Sci. 233:103955. doi: 10.1016/j.livsci.2020.103955
Vermeulen, R. (2015). Natural Grazing: Practices in the Rewildling of Cattle and Horses. Toernooiveld: Rewilding Europe.
Vijayan, S., Morris, D. W., McLaren, B. E., and Mukherjee, S. (2017). Domestic ungulates in protected areas and the potential for indirect interactions via shared predation. Biodiversity 18, 1–8. doi: 10.1080/14888386.2017.1406405
Wang, F., McShea, W. J., Wang, D. J., and Li, S. (2015). Shared resources between giant panda and sympatric wild and domestic mammals. Biol. Conserv. 186, 319–325. doi: 10.1016/j.biocon.2015.03.032
Wang, L., Feng, J. W., Amarsaikhan, T., Yang, L. M., Huang, C. M., Li, D., et al. (2019). Forest cattle grazing affects understory food resource of ungulates in the eastern part of the Northeast Tiger and Leopard National Park. Acta Theriol. Sin. 39, 386–396.
Wang, T. M., Feng, L. M., Mou, P., Wu, J. G., Smith, J. L. D., Xiao, W. H., et al. (2016). Amur tigers and leopards returning to China: direct evidence and a landscape conservation plan. Landsc. Ecol. 31, 491–503. doi: 10.1007/s10980-015-0278-1
Wang, T. M., Royle, J. A., Smith, J. L. D., Zou, L., Lu, X. Y., Li, T., et al. (2018). Living on the edge: opportunities for Amur tiger recovery in China. Biol. Conserv. 217, 269–279. doi: 10.1016/j.biocon.2017.11.008
Wang, T., Feng, L., Yang, H., Han, B., Zhao, Y., Juan, L., et al. (2017). A science-based approach to guide Amur leopard recovery in China. Biol. Conserv. 210, 47–55. doi: 10.1016/j.biocon.2016.03.014
Wassie, A., Sterck, F. J., Teketay, D., and Bongers, F. (2009). Effects of livestock exclusion on tree regeneration in church forests of Ethiopia. Forest Ecol. Manag. 257, 765–772. doi: 10.1016/j.foreco.2008.07.032
WWF (2020). Living Planet Report 2020 - Bending the Curve of Biodiversity Loss, eds R. E. A. Almond, M. Grooten, and T. Petersen (Gland: WWF).
Wynne-Jones, S., Convery, I., and Carver, S. (2021). Rewilding: Four Tips to Let Nature Thrive. The Conversation May 24th. Available online at: https://theconversation.com/rewilding-four-tips-to-let-nature-thrive-157441 (accessed July 12, 2021).
Xiao, W. H., Hebblewhite, M., Robinson, H., Feng, L. M., Zhou, B., Mou, P., et al. (2018). Relationships between humans and ungulate prey shape Amur tiger occurrence in a core protected area along the Sino-Russian border. Ecol. Evol. 8, 11677–11693. doi: 10.1002/ece3.4620
Yang, H. T., Dou, H. L., Baniya, R. K., Han, S. Y., Guan, Y., Xie, B., et al. (2018a). Seasonal food habits and prey selection of Amur tigers and Amur leopards in Northeast China. Sci. Rep. 8:6930.
Yang, H. T., Zhao, X. D., Han, B. Y., Wang, T. M., Mou, P., Ge, J. P., et al. (2018b). Spatiotemporal patterns of Amur leopards in northeast China: influence of tigers, prey, and humans. Mamm. Biol. 92, 120–128. doi: 10.1016/j.mambio.2018.03.009
Yang, H., Han, S., Xie, B., Mou, P., Kou, X., Wang, T., et al. (2019). Do prey availability, human disturbance and habitat structure drive the daily activity patterns of Amur tigers (Panthera tigris altaica)? J. Zool. 307, 131–140. doi: 10.1111/jzo.12622
Yang, R., Cao, Y., Hou, S. Y., Peng, Q. Y., Wang, X. S., Wang, F. Y., et al. (2020). Cost-effective priorities for the expansion of global terrestrial protected areas: setting post-2020 global and national targets. Sci. Adv. 6:eabc3436. doi: 10.1126/sciadv.abc3436
Zhang, C. Z., Zhang, M. H., and Stott, P. (2013). Does prey density limit Amur tiger Panthera tigris altaica recovery in northeastern China? Wildlife Biol. 19, 452–461. doi: 10.2981/12-090
Zhang, H. C., Paijmans, J. L. A., Chang, F. Q., Wu, X. H., Chen, G. J., Lei, C. Z., et al. (2013). Morphological and genetic evidence for early Holocene cattle management in northeastern China. Nat. Commun. 4:2755. doi: 10.1038/ncomms3755
Zhang, P. (2018). Winter Nutritional Adaptive Strategy of Wild Boar (Sus scrofa) in Northern Forest of Changbai Mountain. Harbin: Northeast Forestry University.
Keywords: livestock grazing, national park, roe deer, sustainable forest, wild boar
Citation: Roberts NJ, Zhang Y, Convery I, Liang X, Smith D and Jiang G (2021) Cattle Grazing Effects on Vegetation and Wild Ungulates in the Forest Ecosystem of a National Park in Northeastern China. Front. Ecol. Evol. 9:680367. doi: 10.3389/fevo.2021.680367
Received: 14 March 2021; Accepted: 29 September 2021;
Published: 18 November 2021.
Edited by:
Jose Marcelo Domingues Torezan, State University of Londrina, BrazilReviewed by:
Adrian M. Shrader, University of Pretoria, South AfricaEmmanuel Serrano Ferron, Universitat Autònoma de Barcelona, Spain
Copyright © 2021 Roberts, Zhang, Convery, Liang, Smith and Jiang. This is an open-access article distributed under the terms of the Creative Commons Attribution License (CC BY). The use, distribution or reproduction in other forums is permitted, provided the original author(s) and the copyright owner(s) are credited and that the original publication in this journal is cited, in accordance with accepted academic practice. No use, distribution or reproduction is permitted which does not comply with these terms.
*Correspondence: Guangshun Jiang, amdzaHVuQDEyNi5jb20=
†These authors have contributed equally to this work