- 1Key Laboratory of Mountain Surface Processes and Ecological Regulation, Institute of Mountain Hazards and Environment, Chinese Academy of Sciences, Chengdu, China
- 2College of Resources and Environment, University of Chinese Academy of Sciences, Beijing, China
The response mechanism of ecosystem respiration (Re) and soil respiration (Rs) to different water conditions is of great significance for understanding the carbon cycle under future changes in the precipitation patterns. We used seven precipitation treatments to investigate the effects of precipitation on Re and Rs on a typical alpine steppe in Northern Tibet. Precipitation was captured and relocated to simulate the precipitation rates of −25, −50, −75, 0 (CK), +25, +50, and +75%. The soil moisture was influenced by all the precipitation treatments. There was a positive linear relationship between the soil moisture and Re, Rs in the study area during the experiment (July–October). Soil volumetric water content (VWC), absolute water content (AWC), soil temperature (ST), aboveground biomass (AGB), bulk density, soil total nitrogen (TN), and alkaline hydrolysis nitrogen (AHN) were the predictors of Re and Rs. The multiple linear regression analysis showed that ST and AWC could explain 90.6% of Rs, and ST, AWC, and AHN could explain 89.4% of Re. Ecosystem respiration was more sensitive to the increased precipitation (+29.5%) whereas Rs was more sensitive to the decreased precipitation (−23.8%). An appropriate increase in water (+25 and +50%) could improve the Re and Rs, but a greater increase (+75%) would not have a significant effect; it could have an effect even lower than those of the first two. Our study highlights the importance of increased precipitation and the disadvantage of decreased precipitation on Re and Rs in an arid region. The precipitation changes will lead to significant changes in the soil properties and AGB, and affect Re and Rs, to change the climate of the alpine steppe in Northern Tibet in the future. These findings contribute to our understanding of the regional patterns of environmental C exchange and soil C flux under the climate change scenarios and highlight the importance of water availability to the regulating ecosystem processes in semi-arid steppe ecosystems. In view of these findings, we urge future researchers to focus on manipulating the precipitation over longer time scales, seasonality, and incorporating more environmental factors to improve our ability to predict and model Re and Rs and feedback from climate change.
Introduction
Ecosystem respiration (Re), one of the major fluxes in the terrestrial ecosystems and the atmospheric carbon cycle, is the main way that carbon is removed from an ecosystem (Johnston et al., 2021). Therefore, Re is an important factor affecting the carbon matter and energy balance of ecosystems and has received widespread attention (Valentini et al., 2000; Janssens et al., 2001; Saleska et al., 2003; Fuchslueger et al., 2014; Sun et al., 2021). Many scholars found that Re is more representative of the difference in carbon sink flux observation points than Gross Ecosystem Production (Valentini et al., 2000; Pilegaard et al., 2001; Noormets et al., 2007; Walsh et al., 2017). Therefore, the dynamic patterns of Re and its response to the environmental factors have been the subject of considerable research in recent years. Many studies have shown that air temperature, relative air humidity, soil temperature (ST), soil moisture content, plant aboveground biomass (AGB), aboveground respiration, and groundwater level affect the monthly total change of Re and seasonal dynamics; however, different regions exhibited different responses (Huang et al., 2009; Fang et al., 2012).
Soil respiration (Rs) is the main component of Re, and its carbon cycle process has been a focus of ecological research (Nie et al., 2019). Soil carbon flux is the main mechanism of soil carbon storage and the global carbon cycle (Gougoulias et al., 2014). Soil carbon flux can not only affect the carbon storage capacity of an ecosystem but also significantly affect the concentration of greenhouse gases in the atmosphere, which in turn affect the global climate (Dixon et al., 1994; Burton and Pregitzer, 2003; Dhaliwal et al., 2019). Soil contains approximately two times as much carbon as that stored in the atmosphere; therefore, even small soil carbon flux changes can have a significant impact on the global ecosystem carbon cycle (Lee et al., 2017). In addition, many predictions suggest that Rs will increase as temperatures rise due to global warming (Schuur et al., 2015). At the same time, the loss of carbon dioxide in the soil will induce the positive feedback that further exacerbates global warming (Woodwell et al., 1998; Rustad et al., 2000; Jeong et al., 2018). Therefore, quantifying Rs has become the initial task for predicting the future changes in the atmospheric CO2 concentration (Hirano et al., 2003; Wang et al., 2015). Precipitation is usually the driving factor of soil moisture dynamics (Chen et al., 2016). Any changes in the root biomass, soil organic matter, or root and microbial activities due to the precipitation changes may influence Rs (Li et al., 2010). Considerable research has been conducted on the influence of precipitation on Rs (Liu et al., 2016; Du et al., 2020). Several studies have suggested that increasing precipitation can stimulate belowground productivity, resulting in a greater supply of C to Rs, particularly in dryland areas (Yan et al., 2010; Zhang et al., 2017). In hot and humid tropical forests, drought can stimulate Rs by reducing overall soil hypoxia (Cleveland et al., 2010). Soil respiration in a semi-arid steppe increased non-linearly with increased precipitation, and the effect of precipitation change on the soil heterotrophic respiration was greater than that of autotrophic respiration (Zhang et al., 2019). In summary, the precipitation changes affect Rs mainly through the changes in the ecosystem processes, such as plant growth (Yan et al., 2011; Zhou et al., 2016), soil microbial activity (Zhao et al., 2016a; Ren et al., 2018), and temperature sensitivity (Liu et al., 2016). Many previous studies on the response of Rs to precipitation in the alpine steppe have concluded that Rs increases with an increase in precipitation (Zhang et al., 2019). However, there is no consensus on the range of increase in Rs, the linear or non-linear trend, and whether the responses to water reduction and water increase are consistent (Chen et al., 2008; Wang et al., 2019). The effects of different precipitation rates in Rs and its relationship with soil moisture have not been well-investigated, particularly in the subtropics steppe. Therefore, it is imperative to improve our mechanistic understanding of Rs responses to the precipitation and soil moisture changes.
Northern Tibet is located at the hinterland of the Qinghai-Tibet Plateau. Under global warming, the precipitation has shown a significant increasing trend in the past 50 years in this region (Li, 2018). The Qinghai-Tibet Plateau has exhibited a trend of “warm and humid” (Zhuo et al., 2018); however, extreme precipitation events have also become more frequent in the period of 1961–2017 (Ma et al., 2020). It is not clear how Re and Rs will respond under future changes, and how they may differ. We propose two hypotheses, as follows, to investigate this problem. Hypothesis 1: the increase in precipitation will increase Re and Rs, and as precipitation increases in the alpine grassland area, respiration would become stronger. Hypothesis 2: Re and Rs may be different in sensitivity or response to water increase or decrease due to dry climate. Water is the critical factor of environmental change in the region of the semi-arid climate. All aspects of the alpine grassland ecosystem would be implicated in precipitation change. For specific performance, variation in precipitation could contribute to the alterations in the process of Re and Rs, and lead to changes in the CO2 concentration. Ultimately, the carbon cycle of terrestrial ecosystem and the process of carbon balance would be changed (Phillips et al., 2017). If the extent and direction of responses to increased or decreased precipitation for Re and Rs are discrepant, then it is helpful for us to predict the trend of variation of Re and Rs by means of precipitation change and evaluate accurately the alpine grassland carbon pool in the future. Here, we selected the northern Tibet alpine grassland at Xainza Experimental Station, as the study area. We simulated different precipitation conditions through the field experiments and observed changes in Re and Rs rates. This study provides insight into the effects of Re and Rs on the ST and moisture, and the responses of Re and Rs to the changes in external factors, such as AGB and soil physicochemical properties. It is expected that this study can provide theoretical support for future research on the Re and Rs changes in grassland ecosystem.
Materials and Methods
Study Site
The experiment was conducted in Xainza Alpine grassland and the Wetland Ecosystem Observation and Experimental Station (30°57′N, 88°42′E, 4,675 m elevation) in Xainza County, Nagqu City, Tibet Autonomous Region (Figure 1). The region belongs to the semi-arid climate of the plateau subfrigid zone, with thin air and a cold and dry climate. The mean annual temperature is 0.4°C and mean annual precipitation is 298.6 mm which is concentrated from May to September. The area has an annual average wind speed of 3.8 m/s. The annual mean wind speed is more than eight reaches 104.3 days, the frost period lasts 279.1 days, and there are 2915.5 h of sunshine annually. The soil type in this area is mainly alpine grassland soil with a thin soil layer and is easily eroded. The alpine grasslands are the main vegetation types and the floral community is relatively simple, mainly Stipa purpurea and Carex Moocroftii. Accompanying species are Leontopodium, Oxytropis, Artemisia capillaris, Bulegrass, and Stellera Chamaejasme.
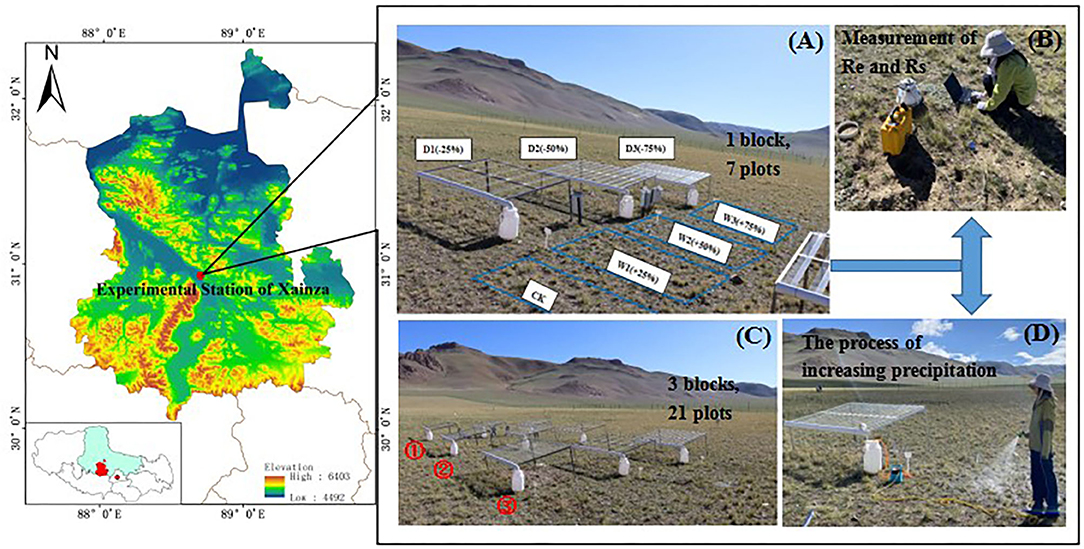
Figure 1. Location of the study area and design of the experiment. (A) Diagram of the seven precipitation treatments; (B) ecosystem respiration (Re) and soil respiration (Rs) measurements; (C) a complete experimental site with three blocks and 21 plots; (D) the process of increasing precipitation in the experiment.
Experimental Design
The precipitation treatment experiment commenced in early June 2020. The experiment used seven levels and three blocks with a total of 21 plots, each 3 × 2 m in size (Figure 1C). The seven levels of precipitation gradients were a 25% decrease (D1), 50% decrease (D2), 75% decrease (D3), control (CK), 25% increase (W1), 50% increase (W2), and 75% increase (W3). The spacing between the two adjacent decreased precipitation treatments was 2 m, and the spacing between the increased and decreased precipitation treatments was 1.5 m to avoid a marginal effect between the plants (Figure 1C). Rain shelter were set up on each decreased precipitation treatments. The rain shutters were fixed to the steel pillars by lag spike; the polyethylene plates were fixed in “V” shapes to intercept the precipitation and allow rainfall to flow along the PVC pipe into the water storage bucket on the right to decrease precipitation (Figure 1A). Within 12 h of precipitation, the rainwater in the water storage bucket was sprayed equably to the corresponding quadrat on the right to increase precipitation (Figure 1D). The angle of the polyethylene rain shield was 120° and the width was 15 cm. The water storage bucket could accommodate rainwater trapped by rainfall with radius of 20 cm and height of 60 cm. The area-specific gravity method was used to regulate the water gradient. In terms of the rain shutter setup, five rain shutters were uniformly spread out to cover 25% of the plot in D1 to retain 25% of the precipitation, and 10 and 15 rain shutters were used in D2 and D3 treatments, respectively, to intercept 50 and 75% precipitation. CK was a control treatment for ambient rainfall conditions (Figure 1A).
Index Measurements
Respiration, ST, and Volumetric Water Content Measurements
Ecosystem respiration and Rs were measured using the Li-8100 connected to a soil chamber (LI-8100, LI-COOR Inc., Lincoln, NE, USA). Ecosystem respiration and Rs were measured in two polyvinyl chloride (PVC) soil collars (20 cm diameter, 15 cm height, 0.5 cm thickness) which were permanently inserted in each plot with 5 cm of the collars remaining above the soil surface. The PVC collars were inserted into the places in each plot where the vegetation coverage was uniform to allow measurement of Re. To reduce the influence of soil disturbance on the measurement results, the surrounding areas were compacted with soil and were not removed during the experiment (Wu et al., 2013). The plants inside the collars were clipped to the ground level after the insertion of the collars and whenever they were found in the collars, to eliminate the aboveground plant respiration during the measurement of Rs (Yan et al., 2011). The litter that had fallen into the collars was retained in the collars to account for CO2 released from litter decomposition (Ren et al., 2014; Wei et al., 2014). The measurements commenced on July 16, 2020 and were conducted every 5 days from 10:00 to 12:00 a.m. (Figure 1B). The interval time could be adjusted appropriately according to the weather conditions in the test area. To reduce the error in the Rs rate caused by long observations and large temperature changes, the measurements were completed in the sunny conditions.
Soil temperature and moisture sensors (Oset HOBO U30-NRC-SYS-ADV, America) were buried at a 10-cm depth in each plot near the soil collars (see below) to continuously monitor the ST and volumetric water content (VWC). The data were recorded every hour.
AGB and Soil Physicochemical Properties
Aboveground biomass was obtained by the harvesting method on July 24 and August 26, 2020, and the biomass was measured by randomly selecting a 0.5 × 0.5 m plot in each precipitation treatment plot. The aboveground parts of the harvested plants were taken back to the laboratory and dried at 65°C to constant weight after drying in an oven at 105°C, their weights were then recorded. After cutting the plants at ground level, the soil at a 0–10 cm depth was removed using the soil drill method. Three soil samples were taken from each sample square and were placed into sealed bags, transported to the laboratory, and were naturally air-dried for measuring the soil physicochemical properties. The total nitrogen (TN) in the soil was determined using the Kjeldahl method (Song, 2019) and the alkaline hydrolysis nitrogen (AHN) was measured using the alkali-diffusion method (Na, 2014). Soil bulk density was determined using the valve bag method (Zhang et al., 2012). Soil absolute water content (AWC) was determined by sampling drying method because this method was relatively accurate and the operation process was simple (Wang and Shu, 2017).
In Equations (1) and (2), DSW denotes the dry weight of soil; SV denotes the volume of soil; and WSW denotes the wet weight of soil; and DSD denotes the dry weight of soil.
Statistical Analysis
The statistical analysis was conducted using R version 4.0.3 (R Core Team 2020) with the packages “vegan” and “ggplot2” (Wickham, 2016; Oksanen et al., 2020) as well as SPSS (IBM, Chicago, IL USA). Before the analysis, the Shapiro–Wilk test was used to check the normality of all data (P < 0.05) and Bartlett's test was used to check the homogeneity of variances (P > 0.05). The one-way ANOVA test was used when the values for both normality of data and the homogeneity of the variances passed their tests. The non-parametric tests were used when both normality and homogeneity of variance did not pass their tests. In this study, the Kruskal–Wallis test method was used for Re and Rs and the one-way ANOVA test was used to analyze ST, VWC, AGB, AWC, TN, and AHN under different precipitation treatments. Duncan's new multiple-range test was used for post-hoc multiple comparisons (Harter, 2008). In addition, to compare the differences among the increased precipitation treatment, decreased precipitation treatment, and overall ambient treatment, the Rs and Re under three increased precipitation treatment gradients were integrated into wet treatments (W) while the Rs and Re under three decreased precipitation treatment gradients were integrated into drought treatments (D). The linear model was used to determine the relationship between Re and Rs and VWC, AWC, AGB, TN, AHN, and bulk density. The quadratic function model was used to analyze the relationship among Re, Rs, and ST. After conducting the correlation analyses for the environmental factors and Rs and Re, we also constructed a multiple linear regression model to establish the relationships between each factor and Rs and Re to allow us to determine which factor had the greatest influence on Rs and Re. We used stepwise regression analysis and set the probability of F as 0.05 and 0.1 for entry and removal, respectively. During this analysis, we eliminated independent variables that were not significant in the model test and were finally left with the three independent variables of ST, AWC, and AHN. The of the mode of Rs and Re reached 0.906 and 0.894, indicating a strong goodness of fit (Table 2). Furthermore, all P-values in the variance tests were <0.001, indicating that the variance tests and the overall model passed significance tests. Finally, we were able to express the multiple linear regression from the results of parameter tests as follows (as shown in Tables 2, 3):
A software ArcGIS (Environmental Systems Research Institute, Inc., CA, USA) was used to generate the geographical location map of the study area. Origin (Origin Lab 2021; Microcal, MA, USA) and Sigmaplot (Systat Software, Inc., CA USA) were used for figures.
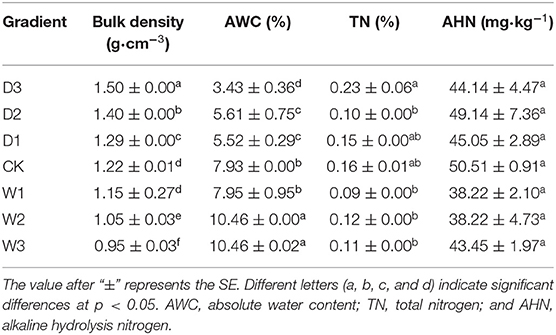
Table 1. The mean values of soil physical properties of the precipitation treatments from July to August.

Table 2. Summary of the results of multiple linear stepwise regression analysis of the effects of environmental factors on soil respiration (Rs).

Table 3. Summary of the results of multiple linear stepwise regression analysis of the effects of environmental factors on ecosystem respiration (Re).
Results
Rs and Re Under Precipitation Change
There were significant differences in the Rs (χ2 = 47.079, P < 0.01) and Re (χ2 = 97.027, P < 0.01) among the seven precipitation treatments during the experiment. For Re, W2 had the highest mean rate (i.e., 2.46) and D3 had the lowest mean rate (i.e., 1.23). However, Re was significantly higher in W1, W2, and W3 than in CK, D1, D2, and D3. Among the three decreased precipitation treatments, the Re and Rs in D3 were the lowest. For Rs, the mean rate was the highest in the W3 treatment (i.e., 1.32), there was no significant difference from that of W2, while that in D3 was the lowest (i.e., 0.82). The Rs was significantly higher in W1 and CK than in D2, and there was no significant difference between D1 and D2 (Figures 2A,B). In general, the mean Rs and Re values under drought treatment were 0.96 and 1.52 μmol·m−2·s−1, respectively, which were significantly lower than those under increased precipitation (1.29 and 2.28 μmol·m−2·s−1, respectively) and ambient treatment (1.26 and 1.76 μmol·m−2·s−1, respectively) (Figure 3). We found that Re and Rs decreased by 13.6 and 23.8%, respectively, under decreased precipitation compared with the ambient treatment. At the same time, Re and Rs increased by 29.5 and 2.4%, respectively, under increased precipitation compared with ambient precipitation. This indicated that Re and Rs were more sensitive to the increases and decreases, respectively, in precipitation.
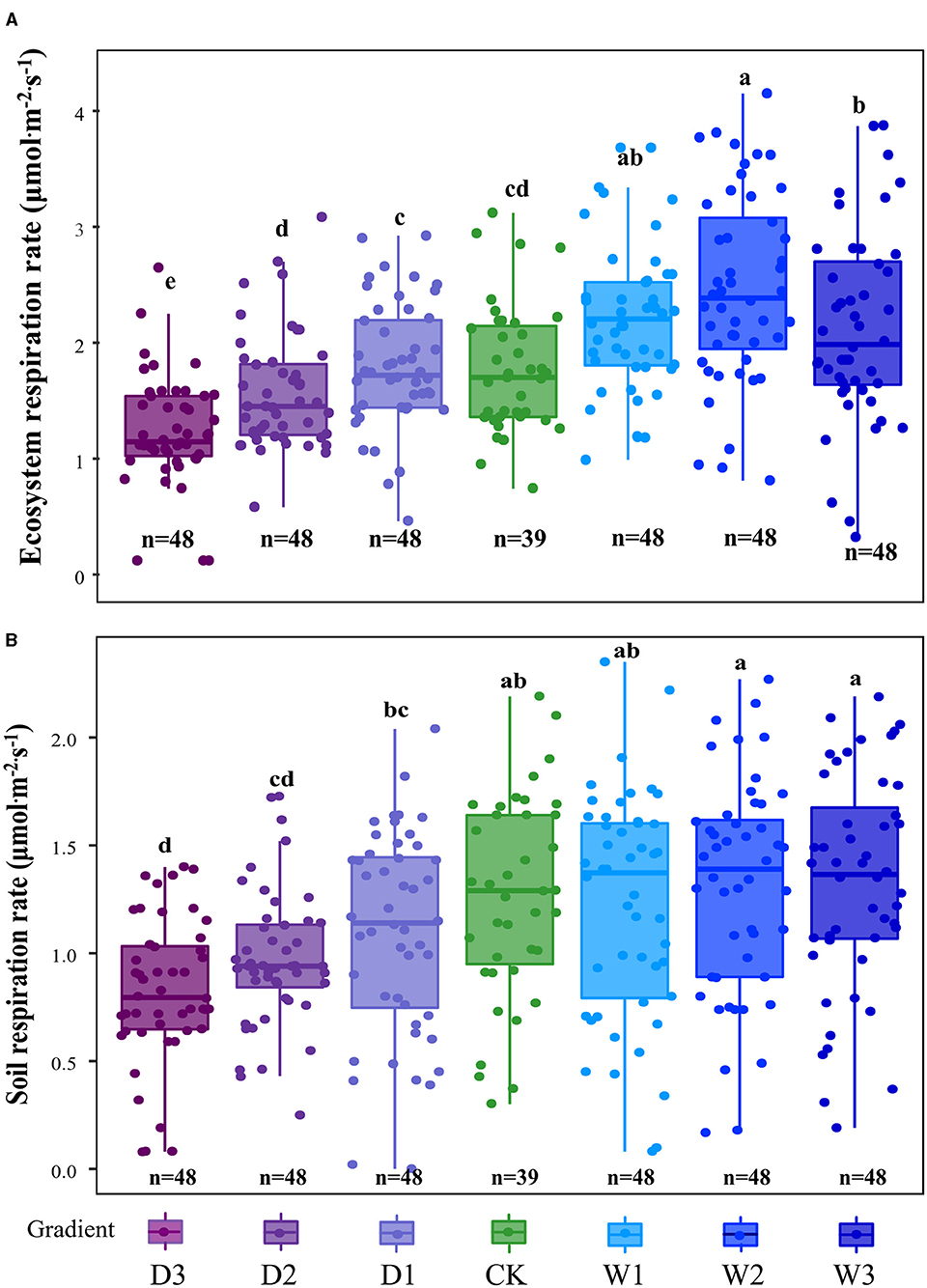
Figure 2. (A) Ecosystem respiration rate and (B) Rs rate under different precipitation treatments; 25% reduction (D1), 50% reduction (D2), 75% reduction (D3), control (CK), 25% increase (W1), 50% increase (W2), and 75% increase (W3). “n” denotes the number of measurements. Different letters (a, b, c, d, and e) represent the significant differences at p < 0.05.
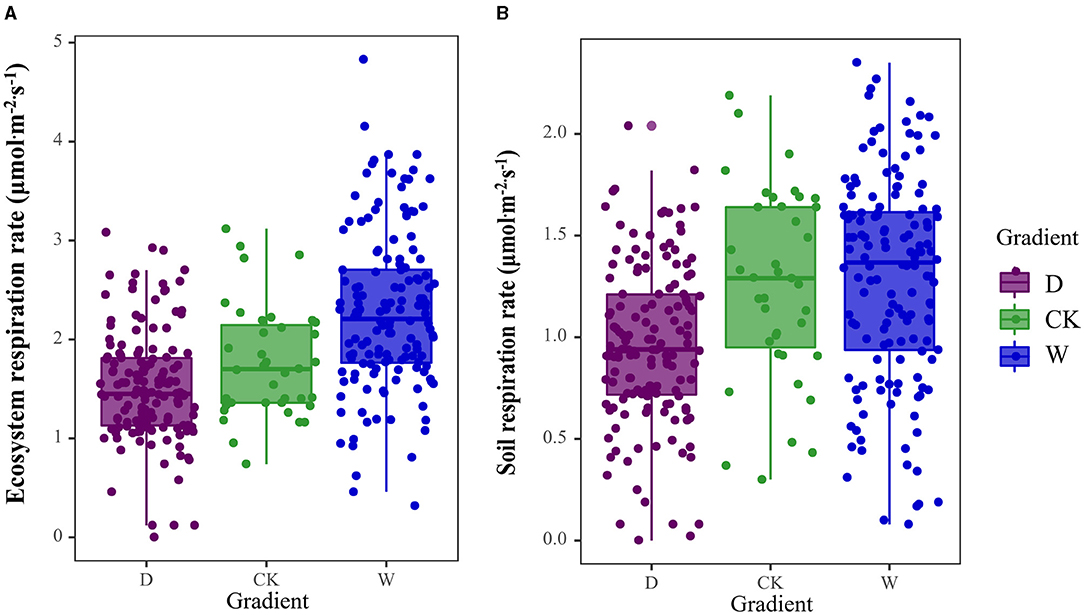
Figure 3. Ecosystem respiration (A) and soil respiration (B) under the three precipitation treatments. D represents drought treatment; W represents wet treatment; CK represents ambient precipitation. Different letters (a, b, c, d, and e) indicate significant differences at p < 0.05.
Environmental Characteristics Under Precipitation Change
Aboveground Biomass
In general, the AGB of W2 was the highest (89.3 g·m−2) and was significantly higher than that of D1, D2, and D3 (F = 5.21, P < 0.01). The AGB of D3 was the lowest (27.16 g·m−2). There was no significant difference in AGB between CK and W3, D2, or D1 (Figure 4). The mean AGB from July to August decreased as follows: W2 > W1 > W3 > D2 > CK > D1 > D3. Aboveground biomass of D1, D2, and D3 decreased by 23.6, 17.3, and 59.3%, respectively, compared with the control. D3 was extremely short of water due to the 75% reduction in water; therefore, the AGB levels were extremely low. However, both a 50 and 25 reduction in water reduced aboveground productivity due to the decrease in precipitation. The AGB in W1 and W2 increased by 30.7 and 33.5%, respectively, while AGB in W3 decreased by 0.07%.
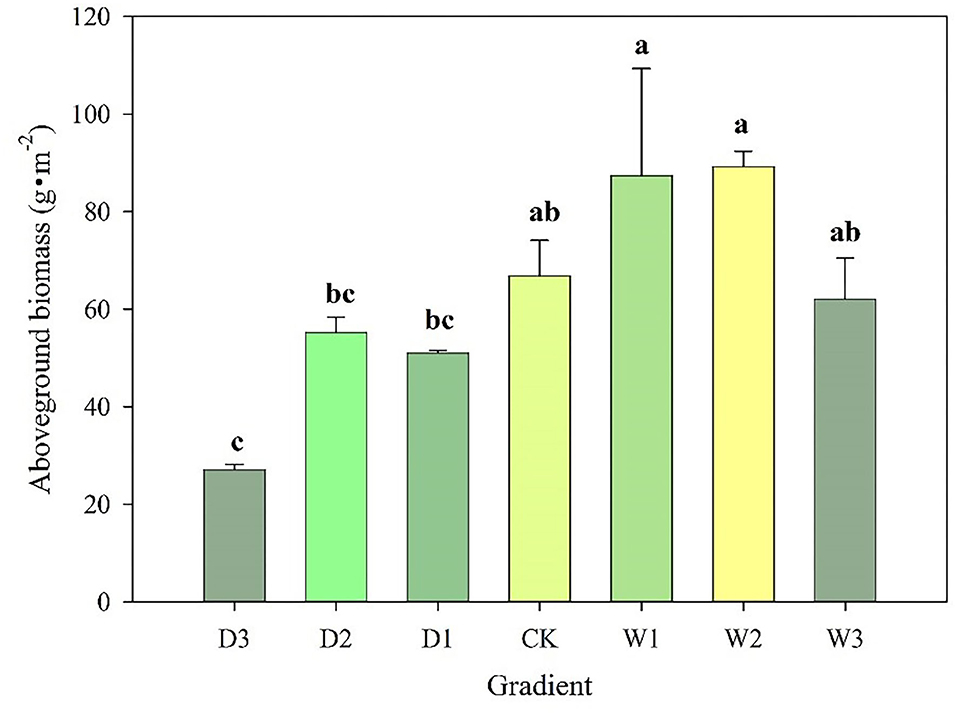
Figure 4. Aboveground biomass (AGB) in the precipitation treatments from July to August; 25% increase (W1), 50% increase (W2), 75% increase (W3), control (CK), 25% reduction (D1), 50% reduction (D2), and 75% reduction (D3). Different letters (a, b, c, and d) indicate significant differences at p < 0.05.
Soil Physicochemical Properties
There were significant differences in soil bulk density among the different moisture gradients, which increased with a decrease in soil moisture. The bulk density of W3 was the lowest (0.95 g·cm−3), and that of D3 was the highest (1.50 g·cm−3) (F = 97.447, P < 0.01). The bulk density decreased from D3 > D2 > D1 > CK, W1 > W2 > W3. The difference in the soil bulk density between CK and W1 was not significant. The soil AWC ranged from 3.43 to 10.46%, and the difference between the precipitation treatments was significant. The AWC in W2 and W3 were significantly higher than those in CK and W1, which were significantly higher than those in D1 and D2; D3 had the lowest AWC (3.43%; F = 26.891, P < 0.01). The highest soil TN content in D3 was 0.23%, followed by D1 and CK, which were 0.15 and 0.16%, respectively. D2, W1, W2, and W3 were significantly lower than D3, which were 0.1, 0.09, 0.12, and 0.11%, respectively. There was no significant difference in soil AHN, which was between 38.22 and 50.05 mg kg−1, under each treatment; it was clear that the average content of AHN in water-reducing treatments were slightly higher than those in water-increasing treatments. Soil AHN in CK was the highest (50.51 mg·kg−1), and the average content of AHN in water reduction treatments were slightly higher than that in water increase treatments, but the difference was not significant.
ST and VWC
The variation trend of ST and VWC under precipitation treatments was consistent during the experiment period (Figure 5). The ST increased gradually from July 16 to August 21, reached the maximum value (D3; 21.15°C) on August 21, and then, showed a decreasing trend and dropped to the minimum value (W1; 9.25°C) on October 17 (Figure 5A). Soil VWC showed two peaks in July–August, i.e., 18 and 17% on July 31 and August 31, respectively. Subsequently, soil VWC gradually decreased and reached its minimum value (D2; 4%) on October 17 (Figure 5B). The mean ST and VWC in CK were 15.86°C and 11.7%, respectively. The soil VWCs in D1, D2, and D3 decreased by approximately 15, 51, and 45% compared with the CK plot, respectively. The mean STs of D2 and D3 increased by approximately 2.4 and 12.5%, respectively. The soil VWC in W1, W2, and W3 plots increased by approximately 6.2, 14.3, and 8.6%, respectively. Compared with CK, the mean STs of W1, W2, and W3 decreased by approximately 6, 5.4, and 4.9%, respectively.
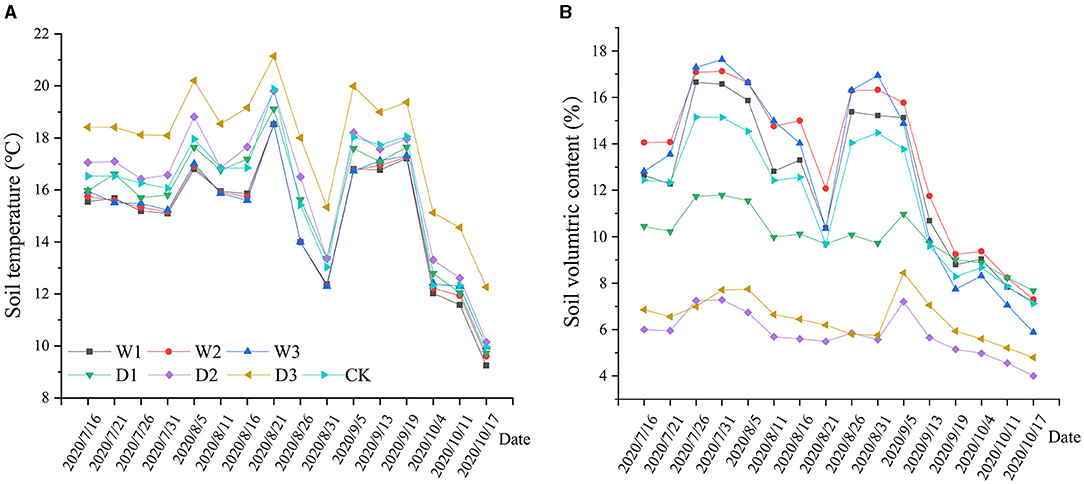
Figure 5. Daily mean (A) soil temperature (ST) and (B) moisture in each precipitation treatment; 25% increase (W1), 50% increase (W2), 75% increase (W3), control (CK), 25% reduction (D1), 50% reduction (D2), and 75% reduction (D3).
Relationship Between Environmental Factors and Re and Rs
Correlations Between Environmental Factors and Re and Rs
Aboveground biomass was positively correlated with Re and Rs (Figure 6) and accounted for 69.8% of the variations in Re; however, the positive correlation between AGB and Rs was not significant. The linear fitting results showed a significant linear relationship between the VWC and Re and Rs, with of 0.4 and 0.152, respectively (Figure 7A). Soil temperature and Re and Rs—the latter two of which first increased with the increase of ST—were fit by a quadratic linear model. When the ST reached about 15°C, Re and Rs began to decrease (Figure 7B). In addition, soil bulk density was significantly negatively correlated with Re and Rs, with reaching 0.694 and 0.728, respectively (Figure 8A). Absolute water content was significantly positively correlated with Re and Rs the reached 0.767 and 0.798, respectively (Figure 8B). The TN had a weak negative correlation with Re and Rs significantly (Figure 8C). Alkaline hydrolysis nitrogen had a weak negative correlation with Re significantly, and AHN had no significant negative correlation with Rs (Figure 8D).
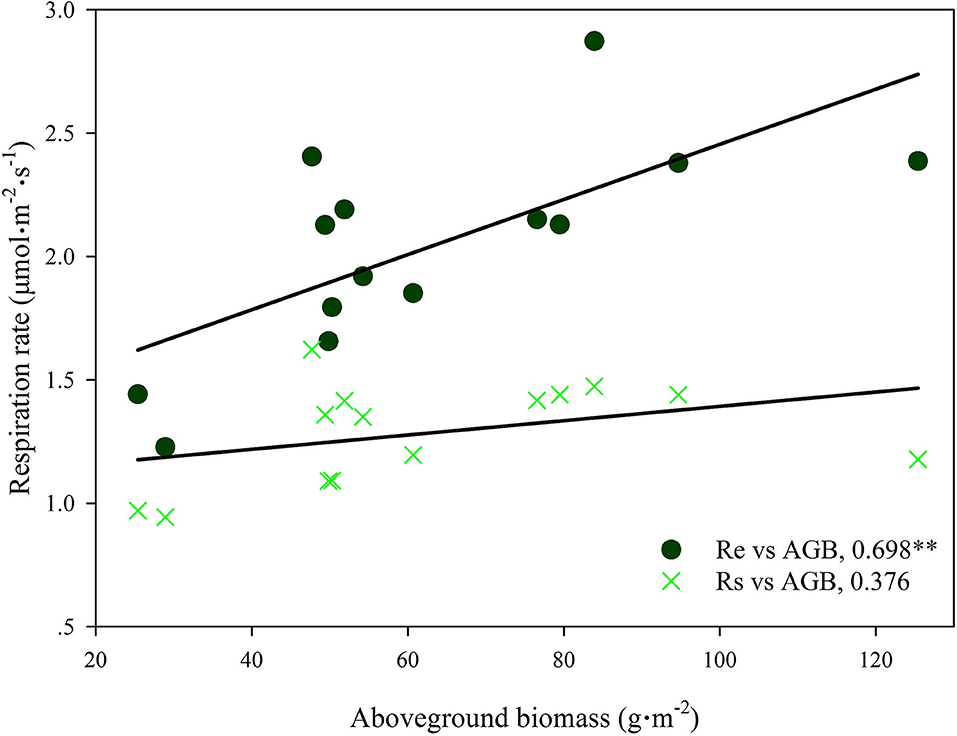
Figure 6. Relationships between AGB and Re and Rs. Each point is the average value through time. *p < 0.05 and **p < 0.01.
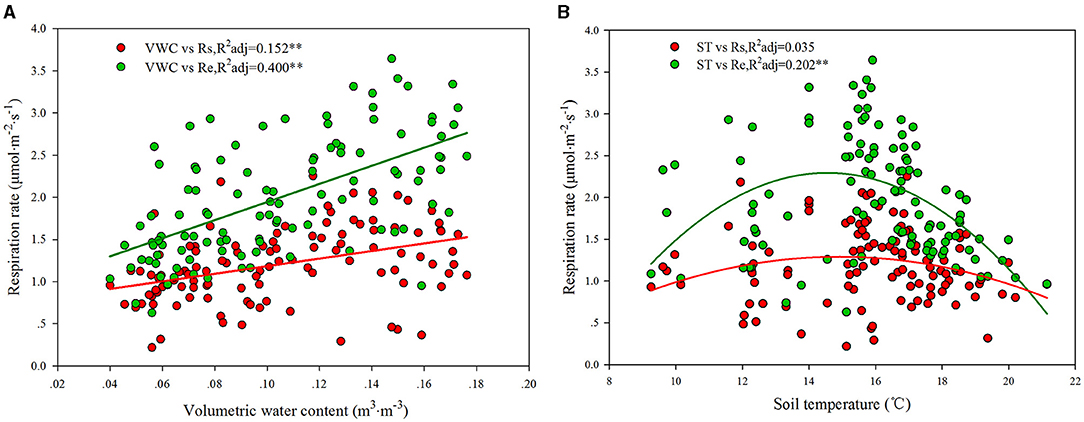
Figure 7. Relationships between volumetric water content (VWC), Rs and Re (A); Relationships between ST and Rs and Re (B). **p < 0.01.
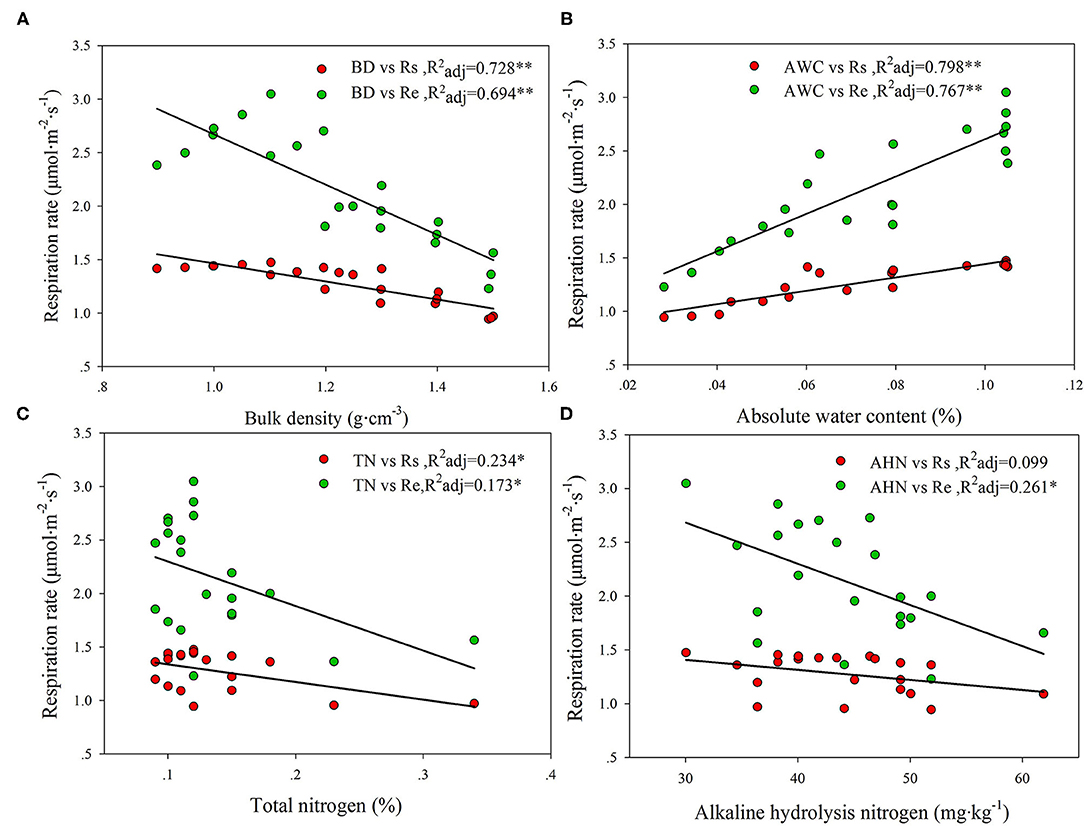
Figure 8. Relationships between the (A) bulk density, (B) absolute water content (AWC), (C) total nitrogen (TN), (D) alkaline hydrolysis nitrogen (AHN), and Rs and Re. *p < 0.05 and **p < 0.01.
Multiple Linear Regression Analysis
Based on the multiple linear regression results, expressions (3) and (4) showed that the main factors affecting Rs were ST and AWC, the regression coefficient showed that ST and Rs had an inverse growth relationship and increases in ST may have inhibited Rs. Absolute water content and Rs grew in the same direction. The main factors affecting Re were ST, AHN, and AWC. Soil temperature and AHN showed negative growth with Re while AWC showed positive growth with Re.
Discussion
Effects of Precipitation Changes on Re and Rs Characteristics in the Growing Season
Moisture is often the most important limiting factor in the alpine regions (Green et al., 2019; Wang Y. et al., 2020). When the precipitation changes, the environment in the study area will change accordingly, thus affecting the release of carbon (Liu et al., 2009; Carbone et al., 2011; MatÍAs et al., 2011; Correia et al., 2012). Many previous studies have indicated highly variable effects of precipitation manipulation experiments on Rs (Beier et al., 2012). It is widely assumed that the precipitation can influence Rs by altering the soil moisture, which directly influences the production and breakdown of the organic matter that forms the substrate for heterotrophic respiration, as well as the physiological processes of roots and microorganisms (Borken et al., 2006). Thus, a decrease in the precipitation is expected to lead to low soil CO2 emissions, and several precipitation exclusion experiments have indeed shown that Rs decreased under decreased precipitation (Miao et al., 2017; Li et al., 2020; Wang J. et al., 2020). In this paper, Re and Rs were between 1.23–2.46 and 0.82–1.32 μmol·m−2·s−1, respectively, in the growing season (Figures 2A,B). In addition, Re and Rs increased significantly under the increased precipitation treatment (Figure 2), which reflected the difference between the semi-arid ecosystems and forest ecosystems. It is reported that Rs in the subtropical forests showed little response to precipitation increase, even when the precipitation was doubled (Deng et al., 2012). But a large increase of 31% in Rs was reported in the arid and semiarid grassland with 30% increase in annual precipitation (Liu et al., 2009). The different responses might be attributed to the differences in climate type (temperature and precipitation), soil condition (e.g., infiltration rates, slopes, textures, depths, and impermeable layers), and vegetation (types and covers) at these study sites (Daniels, 2016; Ma et al., 2018; Shi et al., 2020). In turn, reducing the precipitation reduced the Rs, which was consistent with a study in the Mediterranean that decreased the Rs by 11% through 3-year rainfall exclusion experiment (Misson et al., 2010). The study area in this paper was located in the arid and semi-arid alpine region, which was characterized by high altitude, scarce year-round precipitation, and sparse vegetation (Green et al., 2019). Water is the main limiting factor of environmental change. So, Re and Rs were very sensitive to the changes in precipitation. Increased precipitation increases the soil moisture, enhances substrate supply, and stimulates increased root and plant biomass and microbial activity, which releases more nutrients from soil organic matter and increases Re and Rs (Liu et al., 2009, 2016; Deng et al., 2017; Song et al., 2019). This is consistent with the response of Re and Rs of many grassland ecosystems to precipitation change (Chen et al., 2013; Wang et al., 2019). Differing from a study on a subtropical forest (Deng et al., 2012), the effect of precipitation on Rs was offset by the decrease of ST sensitivity, and the slight increase of soil microbial biomass and AGB had no significant effect on Rs and Re. In addition, combining the precipitation treatment with the increase and decrease in Re and Rs rates, it could be concluded that Re was more sensitive to the increased precipitation, and Rs was more sensitive to the reduced precipitation (Figure 3). This is because there are many strong winds weather in the study area, which make the evaporation strong (Gao, 2016). Therefore, when the precipitation increases slightly, the evaporation of soil surface water is more vigorous, and only a little part of it infiltrates, so the Rs will not be greatly improved. As for Rs in this study area, reduced precipitation increased the scarcity of water in the arid soil, which would seriously inhibit microorganism and root activities and the transformation of soil organic matter, leading to a significant decline in Rs (Wani et al., 2013; Wang and Wang, 2017). When the increments of water reached 50 and 75%, the sufficient soil moisture stimulated the increase of AGB and captured soil water; therefore, Re increased significantly (Flanagan and Johnson, 2005; Wang et al., 2021). Our results suggest that if the precipitation increases in the future, the Re in the study area may significantly increase, and Rs may not notably increase. Whereas, if less precipitation occurs in the future, Rs may significantly decrease.
Relationships Among Re, Rs, and Environmental Factors Under Precipitation Changes
The precipitation changes affect the soil CO2 flux by changing the soil micrometeorological conditions, such as soil moisture and ST (Yang et al., 2017). Generally, with the increase of precipitation, soil moisture will increase, and the availability and mobility of soil soluble organic matter will be enhanced. The increase of organic matter will promote microbial activity and provide sufficient substrate for microbial reproduction, thus increasing Rs (Chen et al., 2014). At the same time, the higher soil water content is conducive to the growth of plant roots and stems, promotes photosynthesis of aboveground plants, and allocates photosynthates to the carbon emission in the rhizosphere. Microbial populations will use this carbon source and the microbial respiration rate will be higher, thus enhancing the Re (Smith et al., 2014). Therefore, soil VWC and AWC were significantly positively correlated with Re and Rs (Figures 7A, 8B). Our study found that increases in ST do not always increase CO2 emissions. When the ST exceeded 15°C, Re and Rs tended to decrease (Figure 7B). The response of Rs to temperature is not invariable. The sensitivity of Rs to temperature increase will reduce when the temperature further increases or prolongs the high temperature (Oechel et al., 2000; Tang et al., 2018), which leads to the decrease of the amount of Rs with the rising of temperature, showing the adaptation of Rs to temperature. Previous scholars also called this phenomenon the temperature saturation of Rs (Qi, 1994). The polyethylene plates used in this study prevented heat loss, resulting in STs in the decreased precipitation being higher than that in the increased precipitation. In addition, the soil moisture of decreased precipitation was lower, so Rs and Re were inhibited. This was particularly evident in D3, in which the ST was high but respiration was weak (Figures 5A, 7B).
The TN content in soil reflects the status of soil nitrogen cycle and is an important index to measure soil fertility and evaluate soil resources (Buondonno et al., 1997). Soil alkali-hydrolyzed nitrogen, also known as soil available nitrogen (Wang, 2020), is the sum of ammonium nitrogen, nitrate nitrogen, amino acids, amides, and easily hydrolyzed protein nitrogen (Zhang, 2020). Compared with soil TN, AHN can better reflect the seasonal or recent nitrogen supply capacity of soil (Liu and Huang, 2005), and is one of the important indexes to measure the soil fertility (Meng et al., 2021). It is reported that the net nitrification rate and nitrate leaching in the surface soil increased significantly under summer rainfall enhancement treatment, while the total organic nitrogen content in the soil decreased significantly (Schaeffer et al., 2013). Increasing rainfall will increase soil moisture, promote inorganic nitrogen leaching, denitrification, plant and microbial nitrogen uptake, accelerate soil nitrogen mineralization rate, and reduce soil inorganic nitrogen content. On the contrary, the decrease of precipitation will lead to the decrease of soil moisture, the inhibition of plant and microbial activities, the decrease of soil nitrogen mineralization rate and N2O emission, and the increase of soil inorganic nitrogen content (Cregger et al., 2014; Ju et al., 2016). This is consistent with the results of this study. We found that the TN content of soil under water increasing treatment was lower, while that under water reducing treatment was higher, especially D3. The mean value of alkali-hydrolyzed nitrogen under water reduction treatment was slightly higher than that under water increase treatment (Table 1). In addition, carbon and nitrogen cycles are easily decoupled under the drought conditions, which affect the ecological environment and productivity. A study showed that the soil inorganic nitrogen content increased by five times compared with the control after summer drought treatment in the Grassland of the United States. However, due to the limitation of soil water, the above-ground and underground biomass and soil CO2 emission were significantly lower than the control, indicating that the decoupling of carbon and nitrogen cycle may occur (Evans and Burke, 2013). Additionally, there are short-term experiments that show that the precipitation pulses lead to carbon and nitrogen decoupling. In arid ecosystems, the precipitation pulses stimulate the microbial activity and AGB synchronously, but stimulate nitrogen loss (Dijkstra et al., 2012). In our study, it was found that the soil nitrogen (TN and AHN) content was lower under increased precipitation and was negatively correlated with Re and Rs (Figures 8C,D). The soil nitrogen content was higher, but the AGB, Re and Rs were smaller under decreased precipitation. This may be due to the decoupling of carbon and nitrogen cycle under drought conditions that reduces Re and Rs, which may also represent a trend of decoupling of carbon and nitrogen.
The AGB could indicate Re and Rs rates to a certain extent, and precipitation played an important role in the change in the AGB (Ji et al., 2016; Zhao et al., 2016b). The higher AGB and canopy coverage of the increased precipitation treatment may have inhibited surface evaporation, so the Re and Rs rates corresponding to the more abundant AGB were also larger (Gang et al., 2018). At the same time, Re and Rs were strong, which reflected the strong activity of plants and microorganisms and the more efficient use of water and nutrients. Abundant AGB and strong plant metabolic capacity increase the rates of Re and Rs (Tiwari et al., 2021). Therefore, the AGB was positively correlated with Re and Rs (Figure 6).
Soil bulk density reflects the smoothness of Rs discharge channels. Soil is porous and CO2 released by roots and soil microorganisms gathers in the pores and is then gradually released into the atmosphere in accordance with the physical diffusion principle (Ryan and Law, 2005; Fang and Wang, 2007). Due to the lack of water in the soil under the decreased precipitation, the water-stable aggregates were reduced, resulting in decreased water and air permeability which hindered the smooth discharge of gas. The soil bulk density was lower with increased precipitation than in the D1, D2, especially D3 (Table 1); however, Re and Rs were higher under increased precipitation treatment (Figure 8A). This is so because the soil under the increased precipitation treatment was more conducive to the entry of O2 required by heterotrophic respiration and the discharge of CO2 due to respiration (Cao et al., 2004; Dong et al., 2005; Xu et al., 2012). Low soil bulk density was associated with higher porosity, resulting in higher availability of soil oxygen to improve the respiration rate, which was consistent with the previous findings (Chen et al., 2010; Wani et al., 2013). Multiple linear regression analysis showed that ST and AWC could explain 90.6% of Rs, while ST, AWC, and AHN could explain 89.4% of Re (Tables 2, 3), which indeed indicated that the soil moisture and temperature were the most important factors in the alpine and cold areas (Tang et al., 2018; Green et al., 2019). Factors, such as AGB and soil bulk density may also be induced by precipitation change. The significant effect of AHN on Re may be related to the internal mechanism of carbon and nitrogen cycle decoupling, which is an interesting and novel discovery, but it needs further research to be more clear (Evans and Burke, 2013).
Limitation of the Study
In this study, we selected an alpine grassland ecosystem in the north of Qinghai–Tibet and tested the effects of increased and decreased precipitation on Re and Rs. One shortcoming of the experimental design was that Re and Rs were only measured during July–October 2020 (i.e., in the peak growing season and at the end of the growing season). If a complete precipitation control experiment for a year or more is conducted, the variation characteristics and differences of Re and Rs in each gradient under the difference between precipitation and snowfall can be analyzed simultaneously (Chen et al., 2017), as well as the effects of freeze-thaw cycle and plant growth stages on Re and Rs (Lu and Liao, 2015; Gao et al., 2021). Soil respiration includes root respiration, soil microbial respiration, and soil animal respiration, but not aboveground plants. However, the respiration of the plants includes aboveground plants and belowground root respiration different from that Rs. Another limitation was that the chosen plot with PVC collars was too small in area to represent the overall field plot, in spite of choosing the plot of relative uniform conditions. Otherwise, due to the frequent rainfall in study site, we had to delay the measurement schedule resulted in irregular interval days for the 16 times. It might influence the evaluation of mean Re and Rs (Zhang et al., 2014). Although we tried to minimize the influence of clipped aboveground plants respiration by removing grass in advance and correcting the influence of changed soil environments on Rs in the PVC collars, there were still some biases of the dynamic chamber method should be noted in partitioning Rs (Subke et al., 2006; Zhang et al., 2016). The microbial activity, litter, and roots also play a crucial role in the changes in Re and Rs. To acquire the changes of microbial activity, litter, and roots respiration, it is necessary to divide detailed components of Re and Rs to compare. In the future research, we will include these indicators in the next step of our research and focus on analyzing their potential influence mechanisms on Re and Rs. Therefore, the inferences regarding the differences between Re and Rs in response to the precipitation changes in alpine steppe ecosystems should be carefully interpreted. The studies in multiple growing seasons are needed to verify the response characteristics of the Re and Rs to provide a theoretical basis for further predicting carbon emissions under the trend of precipitation change.
Conclusion
In summary, our research showed that the increased precipitation treatments increased Re, Rs, and AGB. Furthermore, when precipitation was increased by 50% compared with ambient precipitation, Re and Rs were the highest, whereas, when precipitation was decreased, the Re and Rs in D3 (75% decrease) were the smallest. This proved that hypothesis 1 was not valid. The Rs and Re were stronger with moderate increase in the water levels than with excessive increase, but respiration was the weakest with excessive decrease in the water levels. Ecosystem respiration was more sensitive to the increased precipitation and Rs was more sensitive to the decreased precipitation, which indicated that hypothesis 2 was applicable in our study area. Soil temperature and AWC were the main factors differentiating Re and Rs responses under different precipitation gradients. Alkaline hydrolysis nitrogen was also the dominant factor causing Re differences and may be involved in the decoupling of carbon and nitrogen cycles. The precipitation changes caused significant changes in ST, soil moisture, and soil physicochemical properties (i.e., bulk density, VWC, AWC, TN, and AHN) in the 0–10 cm soil layer had significant effects on the Re and Rs under different precipitation treatments.
Data Availability Statement
The raw data supporting the conclusions of this article will be made available by the authors, without undue reservation.
Author Contributions
XL carried out the measurement of Rs and Re, collected soil samples, assisted in the determination of soil physical properties, acquired, collected, and analyzed the experimental data, and wrote manuscripts. As the applicant of this natural science fund project, YY obtained financial and instrument support, designed the site layout of the precipitation control experiment, assembled the ST and humidity monitor, guided the installation of polyvinyl chloride soil collars, guided and assisted the collection of soil and plant samples, discussed data analysis, and revised the manuscript. LF helped complete the layout of the experimental site. All the authors made important contributions to this study.
Funding
The research was supported by the National Natural Science Foundation of China (Grant No. 41871049).
Conflict of Interest
The authors declare that the research was conducted in the absence of any commercial or financial relationships that could be construed as a potential conflict of interest.
Publisher's Note
All claims expressed in this article are solely those of the author and do not necessarily represent those of their affiliated organizations, or those of the publisher, the editors and the reviewers. Any product that may be evaluated in this article, or claim that may be made by its manufacturer, is not guaranteed or endorsed by the publisher.
Acknowledgments
We are very grateful to the Xainza Alpine grassland and the Wetland Ecosystem Observation and Experimental Station for providing instruments and facilities for measuring respiration rates and collecting samples, and to the Laboratory of Institute of Mountain Hazards and Environment for providing assistance for measuring soil physical properties. Thanks to researcher Xuyang Lu and associate Researcher Jihui Fan for their valuable comments on this study. Thanks to Dr. Yang Hu for her help in drawing. We would like to thank Editage (www.editage.com) for English language editing.
References
Beier, C., Beierkuhnlein, C., Wohlgemuth, T., Penuelas, J., Emmett, B., Körner, C., et al. (2012). Precipitation manipulation experiments – challenges and recommendations for the future. Ecol. Lett. 15, 899–911. doi: 10.1111/j.1461-0248.2012.01793.x
Borken, W., Savage, K., Davidson, E., and Trumbore, S. (2006). Effects of experimental drought on soil respiration and radiocarbon efflux from a temperate forest soil. Glob. Change Biol. 12, 177–193. doi: 10.1111/j.1365-2486.2005.001058.x
Buondonno, A., Coppola, E., Palmieri, G., Benedetti, A., Dell'Orco, S., Németh, K., et al. (1997). Monitoring nitrogen forms in soil/plant systems under different fertilizer managements: a preliminary investigation. Eur. J. Agron. 7, 293–300. doi: 10.1016/S1161-0301(97)00008-7
Burton, A. J., and Pregitzer, K. S. (2003). Field measurements of root respiration indicate little to no seasonal temperature acclimation for sugar maple and red pine. Tree Physiol. 23, 273–280. doi: 10.1093/treephys/23.4.273
Cao, G., Tang, Y., Mo, W., Wang, Y., Li, Y., and Zhao, X. (2004). Grazing intensity alters soil respiration in an alpine meadow on the Tibetan plateau. Soil Biol. Biochem. 36, 237–243. doi: 10.1016/j.soilbio.2003.09.010
Carbone, M. S., Still, C. J., Ambrose, A. R., Dawson, T. E., Williams, A. P., Boot, C. M., et al. (2011). Seasonal and episodic moisture controls on plant and microbial contributions to soil respiration. Oecologia 167, 265–278. doi: 10.1007/s00442-011-1975-3
Chen, M., Zhang, B., Ren, T., Wang, S., and Chen, S. (2016). Responses of soil moisture to precipitation pattern change in semiarid grasslands in Nei Mongol, China. Chin. J. Plant Ecol. 40, 658–668. doi: 10.17521/cjpe.2015.0155
Chen, Q., Wang, Q., Han, X., Wang, s., and Li, L. (2010). Temporal and spatial variability and controls of soil respiration in a temperate steppe in northern China. Glob. Biogeochem. Cycles 24. doi: 10.1029/2009GB003538
Chen, R., Liu, Q., Wang, J., Liu, W., and Sainju, U. M. (2014). Response of soil “Birch Effect” to simulated rainfalls in dry croplands. Acta Ecol. Sin. 36, 306–317. doi: 10.5846/stxb201403120428
Chen, S., Lin, G., Huang, J., and He, M. (2008). Responses of soil respiration to simulated precipitation pulses in semiarid steppe under different grazing regimes. J. Plant Ecol. 1, 237–246. doi: 10.1093/jpe/rtn020
Chen, W., Zheng, X., Chen, Q., Wolf, B., Butterbach-Bahl, K., Brüggemann, N., et al. (2013). Effects of increasing precipitation and nitrogen deposition on CH4 and N2O fluxes and ecosystem respiration in a degraded steppe in Inner Mongolia, China. Geoderma 192, 335–340. doi: 10.1016/j.geoderma.2012.08.018
Chen, Z., Xu, Y., Zhou, X., Tang, J., Kuzyakov, Y., Yu, H., et al. (2017). Extreme rainfall and snowfall alter responses of soil respiration to nitrogen fertilization: a 3-year field experiment. Glob. Change Biol. 23, 3403–3417. doi: 10.1111/gcb.13620
Cleveland, C. C., Wieder, W. R., Reed, S. C., and Townsend, A. R. (2010). Experimental drought in a tropical rain forest increases soil carbon dioxide losses to the atmosphere. Ecology 91, 2313–2323. doi: 10.1890/09-1582.1
Correia, A. C., Minunno, F., Caldeira, M. C., Banza, J., Mateus, J., Carneiro, M., et al. (2012). Soil water availability strongly modulates soil CO2 efflux in different mediterranean ecosystems: model calibration using the Bayesian approach. Agric. Ecosyst. Environ. 161, 88–100. doi: 10.1016/j.agee.2012.07.025
Cregger, M. A., McDowell, N. G., Pangle, R. E., Pockman, W. T., and Classen, A. T. (2014). The impact of precipitation change on nitrogen cycling in a semi-arid ecosystem. Funct Ecol. 28, 1534–1544. doi: 10.1111/1365-2435.12282
Daniels, W. L. (2016). “The Nature and Properties of Soils, 15th Edition Ray R. Weil and Nyle C. Brady. Pearson Press, Upper Saddle River NJ, 2017. 1086 p. Soil Sci. Soc. Am. J. 80, 1428–1428. doi: 10.2136/sssaj2016.0005br
Deng, Q., Aras, S., Yu, C.-L., Dzantor, E. K., Fay, P. A., Luo, Y., et al. (2017). Effects of precipitation changes on aboveground net primary production and soil respiration in a switchgrass field. Agric. Ecosyst. Environ. 248, 29–37. doi: 10.1016/j.agee.2017.07.023
Deng, Q., Hui, D., Zhang, D., Zhou, G., Liu, J., Liu, S., et al. (2012). Effects of precipitation increase on soil respiration: a three-year field experiment in subtropical forests in China. PLoS ONE 7:e41493. doi: 10.1371/journal.pone.0041493
Dhaliwal, S., Naresh, R., and Chandra, M. (2019). Soil organic carbon storage, ecosystem dynamics and climate change: current agro-environmental perspectives and future dimensions: a review. Int. J. Chem. Stud. 7, 192–204.
Dijkstra, F. A., Augustine, D. J., Brewer, P., and von Fischer, J. C. (2012). Nitrogen cycling and water pulses in semiarid grasslands: are microbial and plant processes temporally asynchronous? Oecologia 170, 799–808. doi: 10.1007/s00442-012-2336-6
Dixon, R. K. K., Solomon, A. M., Brown, S., Houghton, R. A., Trexier, M. C., and Wisniewski, J. (1994). Carbon pools and flux of global forest ecosystems. Science 263, 185–190. doi: 10.1126/science.263.5144.185
Dong, Y., Yang, X., Geng, Y., Liu, L., and Li, M. (2005). Effects of grazing on carbon dioxide and methane fluxes in typical temperate grassland in inner Mongolia, China. Resour. Sci. 103–109. doi: 10.1360/biodiv.050058
Du, Y., Wang, Y., Su, F., Jiang, J., Wang, C., Yu, M., et al. (2020). The response of soil respiration to precipitation change is asymmetric and differs between grasslands and forests. Glob. Change Biol. 26, 6015–6024. doi: 10.1111/gcb.15270
Evans, S. E., and Burke, I. C. (2013). Carbon and Nitrogen decoupling under an 11-year drought in the shortgrass steppe. Ecosystems 16, 20–33. doi: 10.1007/s10021-012-9593-4
Fang, J., and Wang, W. (2007). Soil respiration as a key belowground process: issues and perspectives? Chin. J. Plant. Ecol. 31, 345–347. doi: 10.17521/cjpe.2007.0041
Fang, R., Zhang, Z., Cha, T., Tan, J., Wang, X., Chen, J., et al. (2012). Ecosystem and soil respiration of a poplar plantation on a sandy floodplain in Northern China. Acta Ecol. Sin. 32, 2400–2409. doi: 10.5846/stxb201103070273
Flanagan, L. B., and Johnson, B. G. (2005). Interacting effects of temperature, soil moisture and plant biomass production on ecosystem respiration in a northern temperate grassland. Agric. For. Meteorol. 130, 237–253. doi: 10.1016/j.agrformet.2005.04.002
Fuchslueger, L., Bahn, M., Fritz, K., Hasibeder, R., and Richter, A. (2014). Experimental drought reduces the transfer of recently fixed plant carbon to soil microbes and alters the bacterial community composition in a mountain meadow. New Phytol. 201, 916–927. doi: 10.1111/nph.12569
Gang, F., Zhen, S., and Zhang, X. (2018). Increased precipitation has stronger effects on plant production of an alpine meadow than does experimental warming in the Northern Tibetan Plateau. Agric. For. Meteorol. 249, 11–21. doi: 10.1016/j.agrformet.2017.11.017
Gao, D., Liu, Z., and Bai, E. (2021). Effects of in situ freeze-thaw cycles on winter soil respiration in mid-temperate plantation forests. Sci. Total Environ. 793, 148567. doi: 10.1016/j.scitotenv.2021.148567
Gao, Y. (2016). The Study of Evapotranspiration of the Subalpine Meadow in Tianlaochi Catchment in the Upper Reaches of Heibe River. Master, Lanzhou University (in Chinese).
Gougoulias, C., Clark, J. M., and Shaw, L. J. (2014). The role of soil microbes in the global carbon cycle: tracking the below-ground microbial processing of plant-derived carbon for manipulating carbon dynamics in agricultural systems. J. Sci. Food Agric. 94, 2362–2371. doi: 10.1002/jsfa.6577
Green, J. K., Seneviratne, S. I., Berg, A. M., Findell, K. L., Hagemann, S., Lawrence, D. M., et al. (2019). Large influence of soil moisture on long-term terrestrial carbon uptake. Nature 565, 476–479. doi: 10.1038/s41586-018-0848-x
Harter, H. L. (2008). Critical values for Duncan's new multiple range test. Int. Biometr. Soc. 16, 671–685. doi: 10.2307/2527770
Hirano, T., Kim, H., and Tanaka, Y. (2003). Long-term half-hourly measurement of soil CO2 concentration and soil respiration in a temperate deciduous forest. J. Geophys. Res. 108:4631. doi: 10.1029/2003JD003766
Huang, L., Wang, Z., Wei, Y., and Zhang, X. (2009). Ecosystem respiration and its controlling factors in the riparian wetland of Yangtze River. Acta Ecol. Sin. 29, 3621–3628. doi: 10.3321/j.issn:1000-0933.2009.07.021
Janssens, I. A., Lankreijer, H., Matteucci, G., Kowalski, A. S., Buchmann, N., Epron, D., et al. (2001). Productivity overshadows temperature in determining soil and ecosystem respiration across European forests. Glob. Change Biol. 7, 269–278. doi: 10.1046/j.1365-2486.2001.00412.x
Jeong, S., Eom, J., Park, J., Chun, J., and Lee, J. (2018). Effect of precipitation on soil respiration in a temperate broad-leaved forest. J. Ecol. Environ. 42, 10. doi: 10.1186/s41610-018-0071-6
Ji, C., Luo, Y., Xia, J., Shi, Z., Jiang, L., Niu, S., et al. (2016). Differential responses of ecosystem respiration components to experimental warming in a meadow grassland on the Tibetan Plateau. Agric. For. Meteorol. 220, 21–29. doi: 10.1016/j.agrformet.2016.01.010
Johnston, A. S. A., Meade, A., Ardö, J., Arriga, N., Black, A., Blanken, P. D., et al. (2021). Temperature thresholds of ecosystem respiration at a global scale. Nat. Ecol. Evol. 5, 487–494. doi: 10.1038/s41559-021-01398-z
Ju, H., Shen, G., Xu, W., Zhao, C., Su, L., Wang, Y., et al. (2016). The emission of CH4, CO2, and N2O in the typical forest soils of Shennongjia under the precipitation reduction. Acta Ecol. Sin. 36, 6397–6408. doi: 10.5846/stxb201503240560
Lee, J., Eom, J., Jeong, S., Hong, S., Park, E., and Lee, J. (2017). Influence of carbonized crop residue on soil carbon storage in red pepper field. J. Ecol. Environ. 41, 40. doi: 10.1186/s41610-017-0059-7
Li, B. (2018). Dynamic Change of Grassland in Northern Tibet Plateau Based on Remoting Sensing and IBIS Model. Graduate Master, Lanzhou University (in Chinese).
Li, X., Han, S., Guo, Z., Shao, D., and Xin, L. (2010). Changes in soil microbial biomass carbon and enzyme activities under elevated CO2 affect fine root decomposition processes in a Mongolian oak ecosystem. Soil Biol. Biochem. 42, 1101–1107. doi: 10.1016/j.soilbio.2010.03.007
Li, Y., Zhou, Z., Lei, L., Ru, J., Song, J., Zhong, M., et al. (2020). Asymmetric responses of soil respiration in three temperate steppes along a precipitation gradient in northern China revealed by soil-monolith transplanting experiment. Agric. For. Meteorol. 294, 108–126. doi: 10.1016/j.agrformet.2020.108126
Liu, H., and Huang, J. (2005). Dynamic of soil properties under secondary succession forest communities in Mt. Jiyun. J. Appl. Ecol. 16, 37–42. doi: 10.13287/j.1001-9332.2006.0287
Liu, L., Wang, X., Lajeunesse, M. J., Miao, G., Piao, S., Wan, S., et al. (2016). A cross-biome synthesis of soil respiration and its determinants under simulated precipitation changes. Glob. Change Biol. 22, 1394–1405. doi: 10.1111/gcb.13156
Liu, W., Zhang, Z., and Wan, S. (2009). Predominant role of water in regulating soil and microbial respiration and their responses to climate change in a semiarid grassland. Glob. Change Biol. 15, 184–195. doi: 10.1111/j.1365-2486.2008.01728.x
Lu, X., and Liao, Y. (2015). Effects of tillage on soil respiration and root respiration under rain-fed summer corn field. Environ. Sci. 36, 2266–2273. doi: 10.13227/j.hjkx.2015.06.047
Ma, W., Huang, F., Zhou, Q., Chen, Q., Liu, F., and Chen, Y. (2020). Characteristics of extreme precipitation over the Qinghai-Tibet Plateau from 1961 to 2017. Nat. Resour. J. 35, 3039–3050. doi: 10.31497/zrzyxb.20201218
Ma, Z., Zhao, W., Liu, M., and Liu, Q. (2018). Responses of soil respiration and its components to experimental warming in an alpine scrub ecosystem on the eastern Qinghai-Tibet Plateau. Sci. Total Environ. 643, 1427–1435. doi: 10.1016/j.scitotenv.2018.06.243
MatÍAs, L., Castro, J., and Zamora, R. (2011). Soil-nutrient availability under a global-change scenario in a Mediterranean mountain ecosystem. Glob. Change Biol. 17, 1646–1657. doi: 10.1111/j.1365-2486.2010.02338.x
Meng, X., Huang, H., Chen, C., and Wang, R. (2021). Effect of different cultivation modes on soil fertility in Paddy field. Hun. Agric. Sci. 2021, 45–48 (in Chinese). doi: 10.16498/j.cnki.hnnykx.2021.002.012
Miao, Y., Han, H., Du, Y., Zhang, Q., Jiang, L., Hui, D., et al. (2017). Nonlinear responses of soil respiration to precipitation changes in a semiarid temperate steppe. Sci. Rep. 7, 45782. doi: 10.1038/srep45782
Misson, L., Rocheteau, A., Rambal, S., Ourcival, J.-M., Limousin, J.-M., and Rodriguez, R. (2010). Functional changes in the control of carbon fluxes after 3 years of increased drought in a Mediterranean evergreen forest? Glob Chang Biol. 16, 2461–2475. doi: 10.1111/j.1365-2486.2009.02121.x
Na, W. (2014). Comparison between two methods of available nitrogen in soil. Tibet J. Agric. Sci. 36, 30–34 (in Chinese). doi: 10.3969/j.issn.1005-2925.2014.01.007
Nie, C., Li, Y., Niu, L., Liu, Y., Shao, R., Xu, X., et al. (2019). Soil respiration and its Q10 response to various grazing systems of a typical steppe in Inner Mongolia, China. PeerJ. 7, e7112. doi: 10.7717/peerj.7112
Noormets, A., Chen, J., and Crow, T. R. (2007). Age-dependent changes in ecosystem carbon fluxes in managed forests in Northern Wisconsin, USA. Ecosystems 10, 187–203. doi: 10.1007/s10021-007-9018-y
Oechel, W. C., Vourlitis, G. L., Hastings, S. J., Zulueta, R. C., Hinzman, L., and Kane, D. (2000). Acclimation of ecosystem CO2 exchange in the Alaskan Arctic in response to decadal climate warming. Nature 406, 978–981. doi: 10.1038/35023137
Oksanen, J., Blanchet, F. G., Friendly, M., Kindt, R., Legendre, P., McGlinn, D., et al. (2020). vegan: Community Ecology Package.
Phillips, C. L., Bond-Lamberty, B., Desai, A. R., Lavoie, M., Risk, D., Tang, J., et al. (2017). The value of soil respiration measurements for interpreting and modeling terrestrial carbon cycling. Plant Soil 413, 1–25. doi: 10.1007/s11104-016-3084-x
Pilegaard, K., Hummelshøj, P., Jensen, N. O., and Chen, Z. (2001). Two years of continuous CO2 eddy-flux measurements over a Danish beech forest. Agric. For. Meteorol. 107, 29–41. doi: 10.1016/S0168-1923(00)00227-6
Qi, Y. (1994). Human-Induced Biospheric Change and the Global Carbon Cycl: A Spatial Modeling Approach and Its Application to Tropical Asia. State University of New York College of Environmental Science and Forestry (in Chinese).
Ren, C., Chen, J., Lu, X., Doughty, R., Zhao, F., Zhong, Z., et al. (2018). Responses of soil total microbial biomass and community compositions to rainfall reductions. Soil Biol. Biochem. 116, 4–10. doi: 10.1016/j.soilbio.2017.09.028
Ren, Z., Gao, B., Huang, T., and Ju, X. (2014). The contribution of root respiration to soil respiration under different crop rotations and managements. Acta Sci. Circum. 34, 2367–2375. doi: 10.13671/j.hjkxxb.2014.0552
Rustad, L. E., Huntington, T. G., and Boone, R. D. (2000). Controls on soil respiration: implications for climate change. Biogeochemistry 48, 1–6. doi: 10.1023/A:1006255431298
Ryan, M. G., and Law, B. E. (2005). Interpreting, measuring, and modeling soil respiration. Biogeochemistry 73, 3–27. doi: 10.1007/s10533-004-5167-7
Saleska, S. R., Miller, S. D., Matross, D. M., Goulden, M. L., Wofsy, S. C., Rocha, H. R. D., et al. (2003). Carbon in Amazon forests: unexpected seasonal fluxes and disturbance-induced losses. Science 302, 1554–1557. doi: 10.1126/science.1091165
Schaeffer, S. M., Sharp, E., Schimel, J. P., and Welker, J. M. (2013). Soil-plant N processes in a High Arctic ecosystem, NW Greenland are altered by long-term experimental warming and higher rainfall. Glob. Change Biol. 19, 3529–3539. doi: 10.1111/gcb.12318
Schuur, E. A. G., McGuire, A. D., Schädel, C., Grosse, G., Harden, J. W., Hayes, D. J., et al. (2015). Climate change and the permafrost carbon feedback. Nature 520, 171–179. doi: 10.1038/nature14338
Shi, P., Qin, Y., Liu, Q., Zhu, T., Li, Z., Li, P., et al. (2020). Soil respiration and response of carbon source changes to vegetation restoration in the Loess Plateau, China. Sci. Total Environ. 707, 135507. doi: 10.1016/j.scitotenv.2019.135507
Smith, N. G., Rodgers, V. L., Brzostek, E. R., Kulmatiski, A., Avolio, M. L., Hoover, D. L., et al. (2014). Toward a better integration of biological data from precipitation manipulation experiments into Earth system models. Rev. Geophys. 52, 412–434. doi: 10.1002/2014RG000458
Song, J., Wan, S., Piao, S., Knapp, A. K., Classen, A. T., Vicca, S., et al. (2019). A meta-analysis of 1,119 manipulative experiments on terrestrial carbon-cycling responses to global change. Nat. Ecol. Evol. 3, 1309–1320. doi: 10.1038/s41559-019-0958-3
Song, X. (2019). Improvement of the method for determination of total nitrogen in soil by kjeldahl method. Environ. Dev. 31, 120–121 (in Chinese). doi: 10.16647/j.cnki.cn15-1369/X.2019.08.068
Subke, J.-A., Inglima, I., and Francesca Cotrufo, M. (2006). Trends and methodological impacts in soil CO2 efflux partitioning: a metaanalytical review. Glob. Chang Biol. 12, 921–943. doi: 10.1111/j.1365-2486.2006.01117.x
Sun, S., Che, T., Gentine, P., Chen, Q., Wang, L., Yan, Z., et al. (2021). Shallow groundwater inhibits soil respiration and favors carbon uptake in a wet alpine meadow ecosystem. Agric. For. Meteorol. 297, 108254. doi: 10.1016/j.agrformet.2020.108254
Tang, J., Valolahti, H., Kivimäenpää, M., Michelsen, A., and Rinnan, R. (2018). Acclimation of biogenic volatile organic compound emission from subarctic heath under long-term moderate warming. J. Geophys. 123, 95–105. doi: 10.1002/2017JG004139
Tiwari, P., Bhattacharya, P., Rawat, G. S., Rai, I. D., and Talukdar, G. (2021). Experimental warming increases ecosystem respiration by increasing above-ground respiration in alpine meadows of Western Himalaya. Sci. Rep. 11, 2640. doi: 10.1038/s41598-021-82065-y
Valentini, R., Matteucci, G., Dolman, A. J., Schulze, E. D., Rebmann, C., Moors, E. J., et al. (2000). Respiration as the main determinant of carbon balance in European forests. Nature 404, 861–865. doi: 10.1038/35009084
Walsh, B., Ciais, P., Janssens, I. A., Peñuelas, J., Riahi, K., Rydzak, F., et al. (2017). Pathways for balancing CO2 emissions and sinks. Nat. Commun. 8, 14856. doi: 10.1038/ncomms14856
Wang, D., and Shu, Y. (2017). Research progress in determination methods for soil water content. J. Mount. Agric. Biol. 36, 61–65. doi: 10.15958/j.cnki.sdnyswxb.2017.02.013
Wang, J., Liu, Y., Cao, W., Li, W., Wang, X., Zhang, D., et al. (2020). Effects of grazing exclusion on soil respiration components in an alpine meadow on the north-eastern Qinghai-Tibet Plateau. Catena 194, 104750. doi: 10.1016/j.catena.2020.104750
Wang, L., Manzoni, S., Ravi, S., Riveros-Iregui, D., and Caylor, K. (2015). Dynamic interactions of ecohydrological and biogeochemical processes in water-limited systems. Ecosphere 6, art133. doi: 10.1890/ES15-00122.1
Wang, S. (2020). Study on the Performance of Micro Silicon Powder Suspended Liquid Film and Its Effect on Vegetation Growth. Master Master, Qinghai University (in Chinese).
Wang, S., and Wang, H. (2017). Response of soil respiration to a severe drought in Chinese Eucalyptus plantations. J. For. Res. 28, 841–847. doi: 10.1007/s11676-017-0366-y
Wang, Y., Ma, Y., Li, H., and Yuan, L. (2020). Carbon and water fluxes and their coupling in an alpine meadow ecosystem on the northeastern Tibetan Plateau. Theor. Appl. Climatol. 142, 1–18. doi: 10.1007/s00704-020-03303-3
Wang, Y., Xie, Y., Rapson, G., Ma, H., Jing, L., Zhang, Y., et al. (2021). Increased precipitation enhances soil respiration in a semi-arid grassland on the Loess Plateau, China. PeerJ. 9, e10729. doi: 10.7717/peerj.10729
Wang, Z., McKenna, T. P., Schellenberg, M. P., Tang, S., Zhang, Y., Ta, N., et al. (2019). Soil respiration response to alterations in precipitation and nitrogen addition in a desert steppe in northern China. Sci. Total Environ. 688, 231–242. doi: 10.1016/j.scitotenv.2019.05.419
Wani, A., Joshi, P., Singh, O., and Bhat, J. (2013). Estimating soil carbon storage and mitigation under temperate coniferous forests in the southern region of Kashmir Himalayas. Mitig. Adapt. Strateg. Glob. Change 19, 1179–1194. doi: 10.1007/s11027-013-9466-y
Wei, S., Luo, B., Wei, S., Sun, L., Wen, Z., and Hu, H. (2014). Methods of measuring of soil respiration forest escosystems: a review. Ecol. Environ. Sci. 23, 504–514. doi: 10.3969/j.issn.1674-5906.2014.03.021 (in Chinese).
Woodwell, G. M., Mackenzie, F. T., Houghton, R. A., Apps, M., Gorham, E., and Davidson, E. (1998). Biotic feedbacks in the warming of the earth. Clim. Change 40, 495–518. doi: 10.1023/A:1005345429236
Wu, Q., Li, Y., Liu, X., Li, H., and Mao, S. (2013). Ecosystem respiration and carbon sink strength of the Aapline Weeds Meadow in Qinghai-Tibet Plateau under grazing gradient. Chin. J. Agrometeorol. 34, 390–395 (in Chinese). CNKI:SUN:ZGNY.0.2013-04-003
Xu, H., Zhang, H., and Zhang, W. (2012). Influence of soil physical and chemical properties on soil respiration rate of alpine meadow in the Northweatern Plateau of Sichuan Province. J. Sichuan Normal Univ. Nat. Sci. 35, 835–841 (in Chinese). doi: 10.3969/j.issn.1001-8395.2012.06.024
Yan, L., Chen, S., Huang, J., and Lin, G. (2010). Differential responses of auto- and heterotrophic soil respiration to water and nitrogen addition in a semiarid temperate steppe. Glob. Change Biol. 16, 2345–2357. doi: 10.1111/j.1365-2486.2009.02091.x
Yan, L., Chen, S., Huang, J., and Lin, G. (2011). Water regulated effects of photosynthetic substrate supply on soil respiration in a semiarid steppe. Glob. Change Biol. 17, 1990–2001. doi: 10.1111/j.1365-2486.2010.02365.x
Yang, Q., Tian, D., Zeng, H., and Niu, S. (2017). Main factors driving changes in soil respiration under altering precipitation regimes and the controlling processes. Chin. J. Plant Ecol. 41, 1239–1250. doi: 10.17521/cjpe.2017.0208
Zhang, B., Li, S., Chen, S., Ren, T., Yang, Z., Zhao, H., et al. (2016). Arbuscular mycorrhizal fungi regulate soil respiration and its response to precipitation change in a semiarid steppe. Sci. Rep. 6, 19990. doi: 10.1038/srep19990
Zhang, B., Li, W., Chen, S., Tan, X., Wang, S., Chen, M., et al. (2019). Changing precipitation exerts greater influence on soil heterotrophic than autotrophic respiration in a semiarid steppe. Agric. For. Meteorol. 271, 413–421. doi: 10.1016/j.agrformet.2019.03.019
Zhang, B., Yang, Z., Chen, S., Yan, L., and Ren, T. (2014). Evaluating the influences of measurement time and frequency on soil respiration in a semiarid temperate grassland. Chin. Sci. Bull. 59, 2726–2730. doi: 10.1007/s11434-014-0265-y
Zhang, X., Tan, Y., Zhang, B., Li, A., Daryanto, S., Wang, L., et al. (2017). The impacts of precipitation increase and nitrogen addition on soil respiration in a semiarid temperate steppe. Ecosphere 8, e01655. doi: 10.1002/ecs2.1655
Zhang, Y. (2020). Study on the Characteristics of Soil Fertility Quality Under Artificially Planted Vegetation in Photovoltaic Power Plants. Master Master, Inner Mongolia Agricultural University (in Chinese).
Zhang, Z., Gong, H., Huang, Y., Mo, L., He, C., Xu, G., et al. (2012). A Method for Determining Soil Bulk Density. Chinese Patent Application.
Zhao, C., Miao, Y., Yu, C., Zhu, L., Wang, F., Jiang, L., et al. (2016a). Soil microbial community composition and respiration along an experimental precipitation gradient in a semiarid steppe. Sci. Rep. 6, 24317. doi: 10.1038/srep24317
Zhao, J., Luo, T., Li, R., Li, X., and Tian, L. (2016b). Grazing effect on growing season ecosystem respiration and its temperature sensitivity in alpine grasslands along a large altitudinal gradient on the central Tibetan Plateau. Agric. For. Meteorol. 218–219, 114–121. doi: 10.1016/j.agrformet.2015.12.005
Zhou, X., Zhou, L., Nie, Y., Fu, Y., Du, Z., Shao, J., et al. (2016). Similar responses of soil carbon storage to drought and irrigation in terrestrial ecosystems but with contrasting mechanisms: a meta-analysis. Agric. Ecosyst. Environ. 228, 70–81. doi: 10.1016/j.agee.2016.04.030
Keywords: ecosystem respiration, soil respiration, precipitation change, soil moisture, aboveground biomass
Citation: Li X, Yan Y and Fu L (2021) Effects of Rainfall Manipulation on Ecosystem Respiration and Soil Respiration in an Alpine Steppe in Northern Tibet Plateau. Front. Ecol. Evol. 9:708761. doi: 10.3389/fevo.2021.708761
Received: 12 May 2021; Accepted: 07 September 2021;
Published: 15 October 2021.
Edited by:
Giovanna Battipaglia, University of Campania Luigi Vanvitelli, ItalyReviewed by:
Yang Wang, Hebei Normal University, ChinaSu Xukun, Research Center for Eco-Environmental Sciences (CAS), China
Copyright © 2021 Li, Yan and Fu. This is an open-access article distributed under the terms of the Creative Commons Attribution License (CC BY). The use, distribution or reproduction in other forums is permitted, provided the original author(s) and the copyright owner(s) are credited and that the original publication in this journal is cited, in accordance with accepted academic practice. No use, distribution or reproduction is permitted which does not comply with these terms.
*Correspondence: Yan Yan, eXlAaW1kZS5hYy5jbg==