- 1Research Team Taxonomy and Biodiversity Conservation TaCoBi. Department of Biodiversity and Environmental Management, University of León, León, Spain
- 2Departamento de Biología (Botánica), Facultad de Ciencias, Universidad Autónoma de Madrid, Madrid, Spain
- 3Centro de Investigación en Biodiversidad y Cambio Global (CIBC-UAM), Facultad de Ciencias, Universidad Autónoma de Madrid, Madrid, Spain
- 4Herbarium LEB Jaime Andrés Rodríguez, CRAI Experimental, University of León, León, Spain
Predicting the distribution of Invasive alien species (IAS) using species distribution models is promising for conservation planning. To achieve accurate predictions, it is essential to explore species niche dynamics. New approaches are necessary for bringing this analysis to real conservation management needs. Using multi-site comparisons can provide great useful insights to better understand invasion processes. Exploring the fine-scale niche overlap between IAS and native species sharing a location can be a key tool for achieving the implementation of local species conservation actions, which can play a fundamental role in the global management of IAS. This can also increase society’s awareness of the threat of IAS. In this context, here, we explored two key research demands. First, we studied the large-scale niche dynamics of the invasive species Paraserianthes lophantha (Willd.) I.C. Nielsen’s considering different invaded areas. The analysis compared niches of the native range (South Western Australia) with the Australian invaded range (eastern Australia); the native range with the European invaded range, and its full Australian range (native plus invaded range) with the European invaded range. Second, we perform a fine-scale niche overlap analysis at landscape scale in Spain. We studied the niche overlap between P. lophantha and a species with remarkable conservation interest (Quercus lusitanica Lam). All the niche analyses were realized following a well-established ordination (principal component analysis) approach where important methodological aspects were compared and analyzed. Our multi-site study of P. lophantha large-scale niche dynamics detected niche shifts between the Australian ranges demonstrating that the species is labile and may potentially adapt to further European climate conditions and spread its invasive range. Comparative analysis between the European and the full Australian ranges supports that calibrate models including the Australian invasive information is promising to accurate predict P. lophantha European potential distribution. The fine-scale study of niche overlap further explained the potential of this IAS and can be used as a model example of how these local studies can be used to promote the implementation of conservation actions in situ as a complement to large-scale management strategies.
1. Introduction
In an era of globalization, invasive alien species (IAS) are increasingly being introduced into new regions worldwide (Meyerson and Mooney, 2007; Van Kleunen et al., 2015; Early et al., 2016; Turbelin et al., 2017). In the places they invade, they cause irreversible damages that scale from species (Bellard et al., 2016) to communities and ecosystems (Charles and Dukes, 2007; Vilà et al., 2010, 2011; Pyšek et al., 2012). This has led IAS to be one of the main threats to biodiversity globally (Vitousek et al., 1997; Mcgeoch et al., 2010; Nic Lughadha et al., 2020). Anthropogenic changes of great impact such as the global climate change or the human transformation of natural ecosystems are accelerating different invasion processes (Vilà et al., 2007; Hellmann et al., 2008). Therefore, there is increasing consensus from the international community that urgent act against IAS is key to protecting biodiversity (Mack et al., 2000; Early et al., 2016; Funk et al., 2020; Van Rees et al., 2022) and reducing socio-economic impacts (Pimentel et al., 2000; Angulo et al., 2021; Diagne et al., 2021; Cuthbert et al., 2022).
Selecting relevant research topics to provide support to the management and to address the concern of the general public will help to better act against IAS (Courchamp et al., 2017; Funk et al., 2020). Avoiding constraining analysis to specific invasion contexts by studying species at multiple sites is needed for increasing in-depth research knowledge to avoid underestimating the expansion potential of an IAS when guiding management initiatives (Kueffer et al., 2013). To integrate into the research of IAS niche dynamics the analysis of the potential impacts on populations of native species at risk can be useful to raise public and administration awareness of the urgency to implement conservation programs (Courchamp et al., 2017; Funk et al., 2020). Performing this kind of study at a fine-scale represents an opportunity to achieve the implementation of local species proactive conservation actions. On the other hand, invasion science has tended to focus on making theoretical integrations or generalizations from insights derived from well-known plant invaders to prioritize management actions (Pyšek et al., 2008; Hulme et al., 2013; Matzek et al., 2015). However, further research on new “model species” can offer novel insights for making robust meaningful recommendations (Kueffer et al., 2013; Gundale et al., 2014). Interesting new breakthroughs can arise from novel model systems such as understudied IAS (Kueffer et al., 2013), species closely related to proven invaders (Kumschick et al., 2015) or recently introduced populations, over which the possibility to successfully control the invasion process increases (Wilson et al., 2011; Cuthbert et al., 2022).
Niche-based modeling of species distributions is one of the most promising tools for conservation planning (Guisan and Thuiller, 2005; Thuiller et al., 2005; Guisan et al., 2013; Fernandes et al., 2019; Van Rees et al., 2022). Information on current IAS potential distributional and range expansion patterns and its projection to future climate-changing conditions is valuable information to elaborate spatial-explicit recommendations for conservation managers (Thomas et al., 2004; Bradley et al., 2010). The potential extent of invasion in the new range is identified by transferring models fitted in the native range under the assumption that species’ ecological niches (niche conservatism hypothesis) are conserved across geographical space (Thuiller et al., 2005). However, niches could not be static and may suffer changes as expansions, contractions or shifts over space and time, what is known as “niche dynamics” (Pearman et al., 2008; Soberón and Nakamura, 2009; Wiens et al., 2010). So, to achieve accurate predictions for introduced IAS and correctly interpret findings, it is needed to first explore its niche dynamics (Pearson and Dawson, 2003; Pearman et al., 2008; Wiens et al., 2010; Parravicini et al., 2015; Sequeira et al., 2018). This kind of research identifies the amount of niche changes but also allows increased understanding of species tolerances to environmental conditions (Chase and Leibold, 2003; Sax et al., 2013), answer key questions for ecology and conservation biology about assumptions of niche conservatism (Wiens et al., 2010) or the potential consequences of global change on future species ranges (Pearman et al., 2008; Petitpierre et al., 2012). Taken together, these results are highly valuable to offer support for the development of management strategies (Sax et al., 2013; Liu et al., 2020a).
Many attempts tried to make generalizations about how common are niche shifts in introduced plants. A widespread debate exists. “Some studies that synthesized findings from studies of many species show that niche shifts are common (Early and Sax, 2014; Dellinger et al., 2016; Atwater et al., 2018) while others found that niche conservatism is more frequent (Petitpierre et al., 2012). What remains clear is that there is still a need for species-specific research (Liu et al., 2020a,b) following the newest methodological recommendations (Liu et al., 2022) and for the use of techniques that can characterize and compare species interactions with the environment to address that question meaningfully (Peterson, 2011; Guisan et al., 2014; Bates and Bertelsmeier, 2021; Liu et al., 2022).
The classical approach to studying IAS niche dynamics consists in comparing niches between the native range and another single invasion range (Pearman et al., 2008; Soberón and Nakamura, 2009; Wiens et al., 2010). However, multi-site comparative studies of climatic niche dynamics between the native and more than one invasion range can offer some important insights to better understand the invasion processes (Broennimann et al., 2014). This kind of study assessing if niche shifts are consistent across several ranges are less frequent (but see Guo et al., 2013; Bulleri et al., 2016; Silva et al., 2016; Hill et al., 2017; Datta et al., 2019; Christina et al., 2020; Dinis et al., 2020; Carlin et al., 2022). Specifically, no studies have explored the niche dynamics of an IAS between (i) the native range and one invaded range and (ii) another different invaded range. Combining data from the native range and one of the invaded ranges may prevent the underestimation of the potential extension of another invaded range.
The need to explore species-environment relationships across different scales and resolutions has been pointed out for our increased understanding of species distributions patterns (Pearson and Dawson, 2003; Guisan and Thuiller, 2005; Vicente et al., 2014). Particularly, examining the species-climate relationships is crucial to assess niche dynamics at coarse-scales (Soberón and Nakamura, 2009), at which climate governs species distribution ranges (Thuiller et al., 2004). This kind of analysis is a prerequisite to address appropriately the potential effects of global change on future distributions (Pearson and Dawson, 2003; Pearman et al., 2008; Bellard et al., 2013; Chevalier et al., 2022). However, complementary finer-scale niche-based studies may be necessary to better examine the realized niches occupied during biological invasions (Guisan et al., 2014). The use of high resolution variables (meters) capturing non-climatic factors can reveal the mechanisms underlying local plant distributions (Wiens, 1989; Guisan and Zimmermann, 2000; Pearson and Dawson, 2003; Guisan and Thuiller, 2005; Mateo et al., 2019a,b). Furthermore, fine-scale niche studies can allow a deeper insight into the potential interactions between IAS and native species sharing a location. Despite various studies have explored IAS-natives niches overlaps (e.g., Alves et al., 2021; Bercê et al., 2021; Castellanos-Mejía et al., 2021), no published study has investigated that question at landscape fine-scale (<1 km res). Results such as the observed overlap from these fine-scale studies can be used as a criterion to determine if the IAS can threaten the conservation of co-occurring vulnerable native species.
Here, we want to answer two research questions. First, to address whether the multi-site study of the niche dynamics between the native range and more than one invasion range can contribute to anticipate the expansion potential of invasive alien species (H1), we performed a large-scale macroclimatic multi-site comparison study of the invasive crested wattle [Paraserianthes lophantha (Willd.) I.C. Nielsen] niches in which we quantified, compared and tested assumptions of niche conservatism. Niches were compared between both its native range (South Western Australia) and its full range (native and invasive) in Australia and two invasion ranges (Europe and eastern Australia). Second, to discuss whether can a micro niche overlap study between an IAS and a native species be used for local conservation purposes (H2), we studied the fine-scale niche overlap between the invasive P. lophantha and the native oak shrub species with special interest for conservation Quercus lusitanica Lam. in their contact area in the Galician massif of Monte Pindo (NW Spain). Several attempts have been made to assess if P. lophantha could threaten Q. lusitanica in this locality (Santamarina et al., 2019, 2022). However, no studies have explicitly assessed that question in terms of niche overlap. To our knowledge, this is the first attempt to integrate into a single niche study a large-scale (macroclimate) analysis of niche dynamics of an IAS between different sites and a finer-scale niche-based analysis of overlap between an IAS and a native species with conservation interest.
2. Materials and methods
2.1. Species
Paraserianthes lophantha (Willd.) I.C. Nielsen is a tree that grows up to 10–15 m tall under favorable conditions but just as a sprawling shrub 1–2 m tall on unfavorable sites (Doran and Turnbull, 1997; Cowan, 1998). It is the closest relative of Australian wattles (Acacia s.s.; Brown et al., 2008) and is natural from the near coastal areas of South-western Australia (Cowan, 1998). P. lophantha has been introduced and become naturalized or invasive in multiple territories around the world such as in the eastern and coastal South-eastern Australia, New Zealand, India, Indonesia, the South Africa, Canary Islands, Madeira, the European continent, United States of America, some South American countries from Colombia and Venezuela to Chile (Randall, 2017). The earliest introduction outside its natural occurrence range corresponds to Australia and could be set around the 1770’s based on occurrence records included in the online plant reference database Global Biodiversity Information Facility (GBIF, 1accessed December 2020). In Europe, the species was first cultivated in 1800 from material collected in its natural range during the “d’Entrecasteaux expedition” that traveled the coasts of Australia (Ventenat et al., 2013). Previous research suggests that events of introduction of P. lophantha were produced from a limited source of native individuals and/or populations both in Australia (Carlyon et al., 2015; Brown et al., 2020) and in Europe (Thompson et al., 2016). Currently, P. lophantha occupies a widespread naturalized range in both Europe and coastal South-eastern Australia.
There was a lapsus of time from the introduction to the year of the first record of the species in the wild in Europe as subspontaneous (1910s), and the “invasive expansion phase” (1990s). Therefore, it can be considered that is in an early invasive in Europe. This phase is occurring in some locations of Portugal and Spain (including the contact area with Quercus lusitanica in Monte Pindo), the species has shown invasive behavior and produced ecological impacts. Specifically, features of invasiveness reported are the prevent of development of native vegetation via shading of dense stands, alteration of habitat conditions due to nitrogen deposition (Martín Osório et al., 2008; Fagúndez, 2019; Santamarina et al., 2022), therefore, an alteration in the habitat structure, community succession and ecosystem dynamics (Grime, 2012).
According to the Worldwide Bioclimatic Classification System, 1996–2018 proposed by Rivas-Martínez et al. (2011), online,2 P. lophantha’s natural distribution occupies territories with Mediterranean pluviseasonal-oceanic bioclimate (Supplementary Figure S2). Therefore, native area is characterized by irregular precipitation (most comes in heavy winter rains and the summer during at least 2 months is dry) and low continentality (not a lot of annual variation in temperature due to high oceanic influence). A portion of its introduced ranges in Australia and in Europe are also under the same bioclimate. However, the invaded distribution in these territories has reach temperate oceanic and hyperoceanic bioclimates (Supplementary Figure S2), so it is expected that the species experience less than 2 aridity months during the summer and low annual oscillation of temperatures. In its Australian native range, it grows in open eucalypt forests, Allocasuarina ssp. and Melaleuca ssp. shrubs, scrublands, and coastal grasslands (Cowan, 1998), at altitudes between sea level and near 200 m. In its Australian invaded range, it grows in open-forest, woodlands, along the banks of rivers and streams or around marshy areas (Doran and Turnbull, 1997) growing up at 700 meters, according to GBIF database records. In the European invasive range, P. lophantha has been closely associated with human-modified environments (García-Duro et al., 2019; Santamarina et al., 2022) and reaches altitudes of near 400 meters.
Quercus lusitanica Lam. is a singular shrubby oak (Amaral Franco, 1990; Llamas et al., 2003) native to several disjoint areas in the west of the Iberian Peninsula and North Africa (Morocco). Q. lusitanica’s main distribution is in the southern half of the Iberian Peninsula and Morocco, where it occupies territories the same bioclimate as P. lophantha in its native Australian range (Mediterranean pluviseasonal-oceanic bioclimate). In this territory, it is found in subsoil of cork oaks or pine forests and scrub, on siliceous soils, especially in sandy soils (Amaral Franco, 1990). Its population of Monte Pindo situated in the North West of Spain (Galician region; Figure 1) represents the northwestern distribution limit of the species (Rivas-Martínez and Sáenz Laín, 1991). Despite being in a territory within the temperate climate (Rivas-Martínez et al., 2014), in Monte Pindo Sub-Mediterranean favorable conditions for the species occur (Amigo and Romero Buján, 2018). Q. luitanica grows in this locality on shrubs placed on steep south-oriented warm sunny stony slopes on underdeveloped soils (Amigo and Romero Buján, 2018). The presence of P. lophantha due to human intervention is one of the potential threats facing the population of Q. lusitanica in this locality (Amigo and Romero Buján, 2018; Santamarina et al., 2019, 2022). The species has a vulnerable status in the Galicia region (Galician Decree 167/2011; DOG 2011). It is also an important component of habitat 4030 ‘European dry heaths’, in the list of Habitats of European Interest of the European Directive 92/43/EEC.
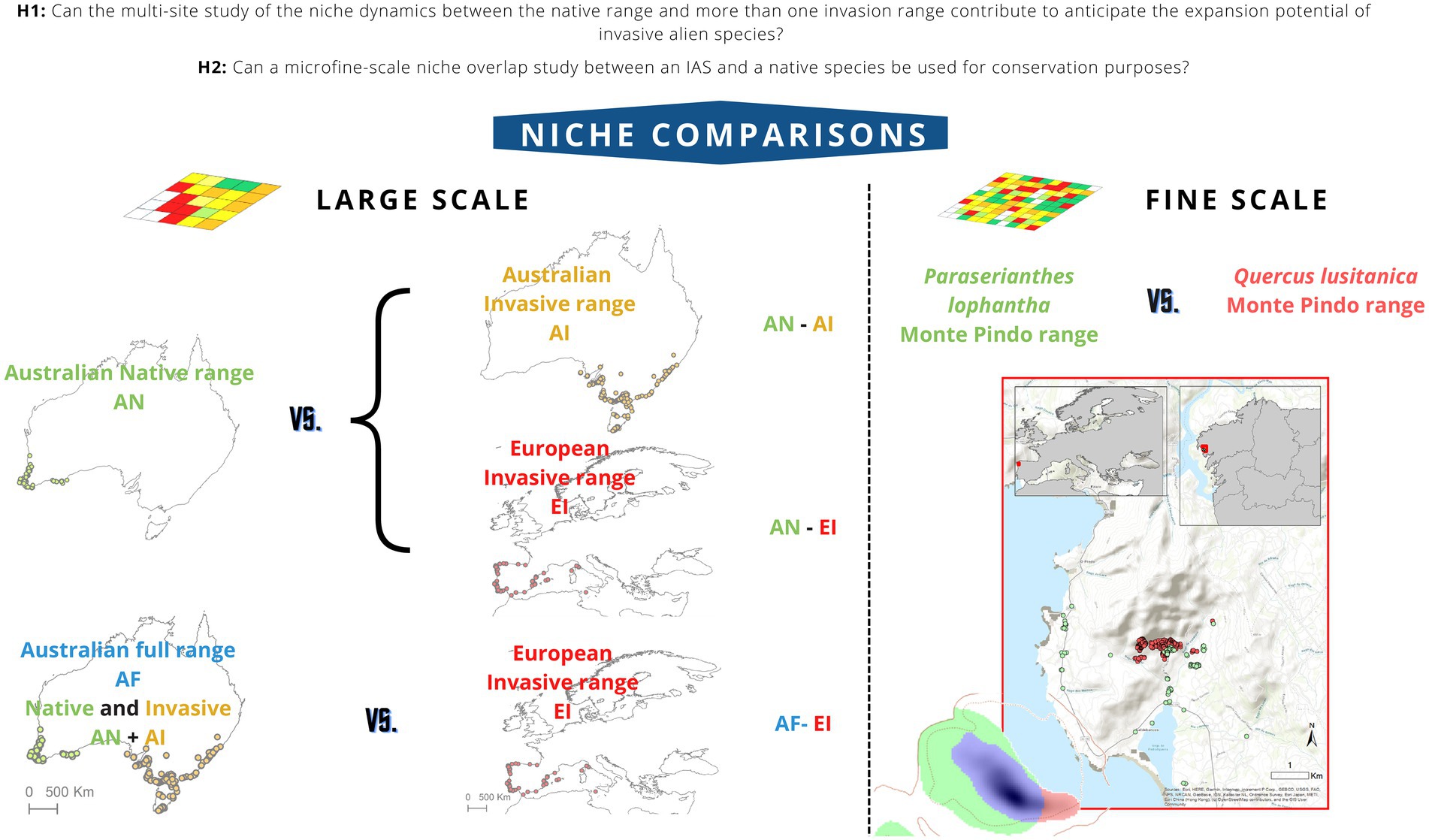
Figure 1. Niche comparisons performed in this work. The large-scale study of Paraserianthes lophantha macroclimatic niche dynamics involves a comparison between niches from its Australian native range and its Australian invasive range (AN-AI comparison) niches from its Australian native range and its European invasive range (AN-EI) niches from its full Australian range (native and invasive) and its European invasive range (AF-EI). The fine-scale study of niche overlap between P. lophantha and Quercus lusitanica involves comparisons between the niches occupied by both species in the local area of Monte Pindo. Fine-scale study of niche overlap between P. lophantha and Quercus lusitanica in Monte Pindo. Solid and dotted contour lines represent 100 and 50% of the available environmental conditions that exist in available environment in Monte Pindo. Green areas represent climates only occupied by P. lophantha, blue areas indicate climates occupied both by the native Q. lusitanica and the introduced P. lophantha, red areas represent climates only occupied by Q. lusitanica. Shading indicates the density of occurrences.
2.2. Niche analysis framework
To answer if the multi-site study of the niche dynamics between the native range and more than one invasion range can avoid to underestimating the expansion potential of an IAS (H1), we analyzed Paraserianthes lophantha macro climatic niche dynamics in two ways: On one hand, niches of P. lophantha were compared between the native range in South Western Australia and each of the two invasive ranges in eastern Australia and Europe (hereafter AN-AI and AN-EI comparisons, respectively). On the other hand, the analysis was performed comparing the full Australian range (including both native and invasive distributions) and the invasive range in Europe (hereafter AF-EI). In order to answer if the results of a micro niche overlap analysis could be used for local conservation purposes (H2), we performed a study of the invasive P. lophantha and the native Quercus lusitanica micro niches taking into account only their distribution ranges in its contact area in the Galician massif of Monte Pindo (Figure 1).
2.3. Background regions
The ordination approach performed (Broennimann et al., 2012; Petitpierre et al., 2012) requires information on environmental conditions from the entire study areas (background regions, BR). The research focused on quantifying niche changes has highlighted the importance of selecting the appropriate extent of BR to improve the accuracy of niche change measures (Guisan et al., 2014; Mateo et al., 2015; Qiao et al., 2017; Chefaoui and Varela-Álvarez, 2018). This is an important issue for the large-scale macroclimatic study of Paraserianthes lophantha niche dynamics since it involves the comparison between niches from different geographic ranges. The use of regions with ecological relevance such as biomes or ecoregions has been recommended to define the study ranges in these cases (Guisan et al., 2014). Furthermore, the use of large biogeographic regions exhibited successful results for invasive species with large and disjunct distributions (Mateo et al., 2015). Therefore, to assess the impact of the definition of the background, we run all the P. lophantha niche dynamics analyses comparing ecoregions, biomes (mapped by Olson et al., 2001), and continents containing P. lophantha occurrence ranges as BR. All the BR pre-selected to capture species-environment relationships and the available climate space to make niche comparisons in both are recompiled in Supplementary Table S1 and represented in Supplementary Figure S1. The use of continents as BR reduced the impact of the non-analogous environments on dynamic indices (see S2 for extensive justification), so we present only that results in this main document and the rest are provided in the Supplementary Figure S3.
Background regions for the fine-scale study of niche overlap between P. lophantha and Quercus lusitanica was defined previously (Monte Pindo, Santamarina et al., 2019). In each of the different BR, the environmental information was extracted from 10.000 randomly generated points (Mateo et al., 2015), The minimum distance between points was 0.0083 and 0.0003 decimal degrees (same as the spatial resolution of variables selected for each study area, to avoid sampling bias; Phillips et al., 2009; Syfert et al., 2013). The R package ‘sp’ (Pebesma and Bivand, 2005) was used to sample random points.
2.4. Species occurrence data
A high-resolution occurrence database (coordinates with errors <1 km for large-scale or < 25 m for fine-scale niche analysis) of Paraserianthes lophantha and Quercus lusitanica contrasted in previous studies (Santamarina et al., 2019) was used in niche analysis. The database compiles records revised by the authors from online plant reference databases GBIF (2018),3 Flora-On (Carapeto et al., 2018), SIVIM (Font, 2013), and Anthos (Aedo, 2012), some of them consulted at their original source and located in samplings or studying the images of Google Earth™. The database was updated with actualized presence data collected from GBIF (GBIF, 4accessed December 2020) and some occurrence data located by new fieldwork. All species distributions newly recorded from GBIF were examined and plotted on maps to detect potentially unreliable citations. We separated the occurrence records at the same distance as the background points to avoid the potential effects of sample selection bias (Phillips et al., 2009; Syfert et al., 2013). The final sice of occurrence data of Paraserianthes lophantha are 71 to Australian native range, 355 to Australian invasive range, 131 to European invasive range, 426 to Australian full range (native and invasive) and 119 to Local Monte Pindo range. The number occurrence records compiled of Q. lusitanica to Local Monte Pindo rage are 256. The spatial distribution of compiled occurrence records of each species is represented in Supplementary Figure S1.
2.5. Environmental variables
To assess large-scale (macroclimate) niche dynamics of Paraserianthes lophantha, candidate variables selected were bioclimatic, which are widely recognized as key drivers of plant species ranges across large scales (Guisan and Thuiller, 2005) and decisive to assess invasion potentials (Thuiller et al., 2005) or global change impacts (Pearson and Dawson, 2003). The 19 bioclimatic variables available globally at 30 arc-seconds spatial resolution (~1x1km) from the CHELSA 2.1 database (Karger et al., 2017)5 were downloaded for the 1981–2010 period. To reduce correlation-related noise, we examined the pair-wise correlations throughout all the study areas together. Among variables with a pairwise Pearson correlation coefficient higher than 0.70 (Elliott and Green, 1980; Dormann et al., 2013), the variable most adequate to be correctly downscaled (Mateo et al., 2019a,b) and the most potential biology meaningfulness (Araújo et al., 2019) was selected. The final selected bioclimatic variables were BIO5 (Max Temperature of Warmest Month), BIO6 (Min Temperature of Coldest Month), BIO7 [Temperature Annual Range (BIO5-BIO6)], BIO13 (Precipitation of Wettest Month), and BIO14 (Precipitation of Driest Month).
To assess fine-scale niche overlaps between P. lophantha and Quercus lusitanica in the local study area (Monte Pindo), we used high-resolution variables (25 × 25m spatial resolution), which are expected to shape plant distributions at the landscape scale (Wiens, 1989; Guisan and Zimmermann, 2000; Pearson and Dawson, 2003; Guisan and Thuiller, 2005). Specifically, we selected the heat load index (HLI), solar radiation aspect index (SRAI) and slope variables to represent microsite topography conditions of Monte Pindo that shown to be good predictors characterizing the local habitat of the species (Santamarina et al., 2019). The soil variable was selected since edaphological conditions have a great importance to explain the ecological preferences of both species in this locality (Amigo and Romero Buján, 2018; García-Duro et al., 2019). We also included a variable related with hidrography (“distance to rivers”) to capture non stressful humidity microsites within the abrupt orography of the massif of Monte Pindo.
The selected bioclimatic variables for the macroclimatic study were downscaled to 25 m resolution following the procedure described in Mateo et al. (2019a). Raster data of temperature variables are downscaled using local linear regressions and those of precipitation variables using resample function of the R package raster (Hijmans and Joe, 2017). The same bioclimatic variables in the two work scales were used so that the analyses are comparable. Variables related to the topography such as heat load index (HLI), solar radiation aspect index (SRAI), streams (intermediate variable to calculate “distance to rivers”) and slope were derived from the digital elevation model, DEM (IGN, 2015). The edaphological variable (soil) was obtained by georeferencing the soil map available for the Monte Pindo study area at a scale of 1:50.000 (Calvo de Anta and Macías Vázquez, 2005), digitizing its categories into a vectorial map and subsequently transforming it into a raster map. Distance to rivers (RiverDistance, the least distance to any stream in m) was calculated from streams variable using geospatial hydrologic modeling extensions of ArcGIS.
All analyses performed using package Ecospat and map matching operations were done using R 1.4.1717 (R Core Team, 2022). Quantum GIS 3.6.3-Noosa (QGIS Geographical Information System6) software was used to species data preparation, generation of background points and final map representation. ArcMap 10.8. from ArcGIS Desktop (ESRI)7 software was used to some mapping.
2.6. Niche comparisons analysis
The method proposed by Petitpierre et al. (2012) and Broennimann et al. (2012) was followed for niche comparisons since has been considered the gold standard approach (Guisan et al., 2014; Di Cola et al., 2017; Atwater et al., 2018). All the analyses were run using the functions included in the R package Ecospat (Di Cola et al., 2017; Broennimann et al., 2021). The analysis workflow was performed for each niche comparison as follows: First, species density of occurrences for each study area were calculated using a kernel smoother density function applied to each cell of a gridded environmental space of 100 × 100 resolution. The gridded environmental space was defined by the first two axes of a principal component analysis (PCA) calculated from the range of environmental conditions present in the study areas (BR). Each cell represents one unique combination of environmental conditions. Then, the widely used Schoener’s D metric of niche overlap (Schoener, 1970) was calculated using the gradients of species occurrence densities in the PCA. This measure provides an overall indication of the match between two niches and ranges from 0 (no overlap) to 1 (complete overlap). We follow the various categories suggested by Rödder and Engler (2011) to easily interpret the results: 0–0.2 = no or very limited overlap, 0.2–0.4 = low overlap, 0.4–0.6 = moderate overlap, 0.6–0.8 = high overlap, 0.8–1.0 = very high overlap.
Equivalency randomization test was run to test the hypothesis of niche conservatism (Warren et al., 2008). Because we wanted to test for niche conservatism, the Ecospat parameter alternative “greater” was selected. The niche equivalency test assesses whether niches of two species in two geographical ranges are statistically equivalent. To test this hypothesis, determine if niche overlap is constant if occurrences are randomly reallocated among groups. If the hypothesis of realized niche equivalency (H0: niches are not more equivalent than expected by chance) is accepted (p > 0.05), then it can be concluded that niches are not significantly conserved between ranges. The test was run with 100 permutations to increase the statistical power to test the conservatism hypothesis (Warren et al., 2008).
Dynamic metrics of niche stability (S), unfilling (U), and expansion (E) were calculated to quantify and decompose the niche changes for the invasive P. lophantha or differences in the densities between P. lophantha and Q. lusitanica (Petitpierre et al., 2012). Niche stability is the proportion of niche A conditions overlapping with niche B. Niche expansion is the proportion of niche B range that does not overlap with niche A. Niche unfilling corresponds to the proportion of the niche A non-present in niche B. The use of non-analogous environments between regions can potentially impact the ability to identify niche shifts accurately in the analysis (Petitpierre et al., 2012). Therefore, P. lophantha large-scale macroclimatic niche shift metrics in AN-EI and AF-EI niche comparisons (involving two different geographical regions) were calculated across the 75th percentile of the marginal climates of the backgrounds being compared, which avoids an overestimation of niche shifts (Guisan et al., 2014). Grids with densities of species occurrence from each study area were plotted in the two-dimensional PCA to visualize and evaluate niche dynamic categories (overlap, unfilling and expansion areas). Niche dynamics along every single variable were also examined to better interpret the niche shifts (Liu et al., 2017).
3. Results
3.1. Large-scale macroclimatic multi-site niche dynamic study of Paraserianthes lophantha’s niches
Results from the niche overlap metric (Schoener’s D) indicated shared environmental conditions between niches compared of Paraserianthes lophantha overall (Table 1). The overlap between the Australian native range and the Australian invasive range (AN-AI) was low (D = 0.2). Niche overlaps between the Australian and the European ranges were most pronounced, achieving moderate values (D = 0.4) between Australian native and European invasive populations and (D = 0.6) between all Australian and European populations (AN-EI and AF-EI, respectively).
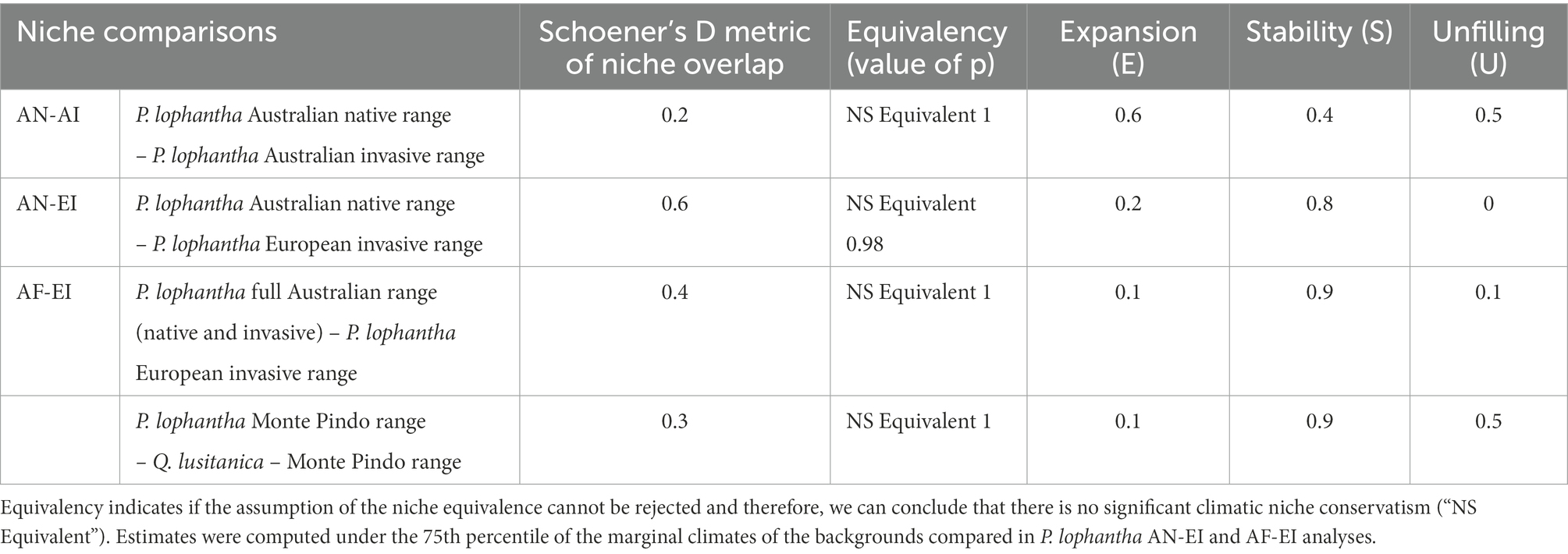
Table 1. Results of niche change indices derived from (1) Paraserianthes lophantha large-scale macroclimatic niche comparisons (2) Fine-scale study of niche overlap between P. lophantha and Quercus lusitanica.
The null hypothesis of the niche equivalency test was accepted in every case (p > 0.05), indicating that all niches of P. lophantha compared are not statistically equivalent (Table 1; AN-AI, AN-EI and AF-EI). Therefore, we can conclude that niches are not significantly conserved between native and invaded ranges (niche equivalency graphs are shown in Supplementary Figure S5).
P. lophantha experienced a large climatic niche expansion (expansion, E) in the Australian non-native range (Table 1; E = 0.6, AN-AI). The expansion was low in the European distribution compared to the Australian native range (E = 0.2, AN-EI) and the full Australian range (E = 0.1, AF-EI). The proportion of the climatic native niche occupied in the native range but not in the invaded range (unfilling, U) was relatively high in the Australian invasive range (U = 0.5, AN-AI). Unfillings detected between Australian and European populations were lowest overall. U ranged from 0 when using only the Australian native range (AN-EI) to 0.1 when niche comparisons were made between the European range and the Australian full range (AF-EI). The proportion of shared climatic space between ranges (stability, S) was lowest between the native and invasive Australian ranges (S = 0.4, AN-AI). Stability ranged from 0.8 when niche comparisons were made between the European range and the Australian native range (AN-EI) to 0.9 when using the Australian full range (AF-EI). Climatic unfilling under non-analogue conditions was detected in the European range in both analyses (AN-EI, AF-EI). Only an area with niche unfilling in analogue climates was detected in the Australian full range-European range comparisons (AF-EI).
A large change both in the climatic niche breadth (expansion) and niche centroid principally along PC1 evidence a niche shift in P. lophantha Australian native-invasive niche comparisons (AN-AI; Figure 2A). In its European invaded range, P. lophantha expanded its niche in several directions of climate space based on the niche envelope breadth along the 2 PCA axes of each PCA elaborated for Australian native - European invasive (AN-EI; Figure 2B) and Australian full range-European invasive comparisons (AF-EI; Figure 2C). In both comparisons (AN-EI, AF-EI), shifts of the niche centroids were detected, but the distance between the centroids was smaller than between native and invasive Australian populations (AN-AI). Therefore, niche positions remained more stable between Australian and European ranges than between Australian ranges.
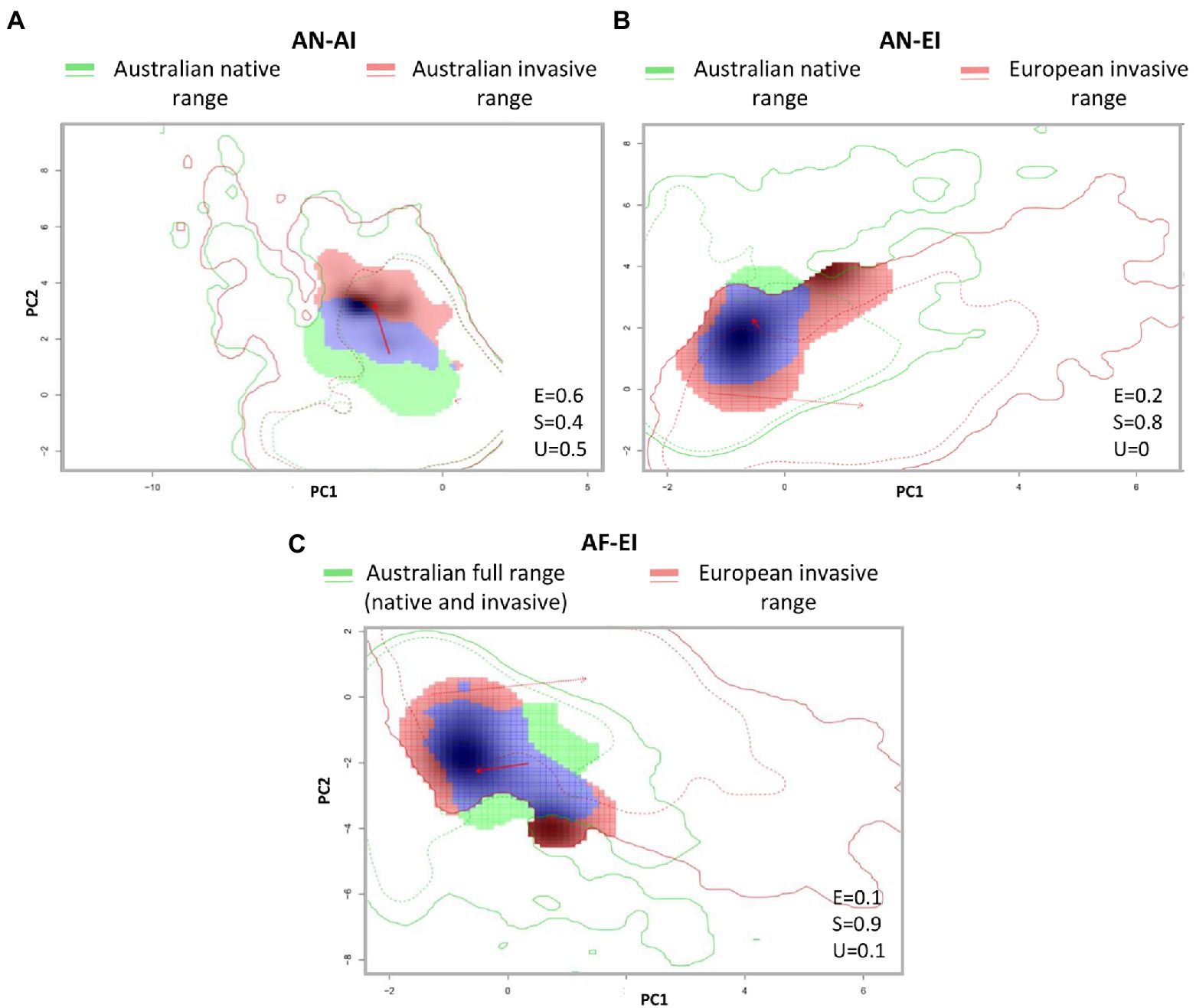
Figure 2. Niche overlaps and dynamics of Paraserianthes lophantha between its native and introduced ranges in climatic space: (A) From Australian native range to Australian invaded range (AN-AI comparison). (B) From Australian native range to European invaded range (AN-EI). (C) from the Australian full range to the European invaded range (AF-EI). Solid and dotted contour lines represent 100 and 50% of the available environmental conditions that exist in the niche A (green lines) and niche B (red lines). Green areas represent climates only occupied in niches A (unfilling areas), blue areas represent climates occupied in niches A and B (stability areas) and red areas represent climates occupied only in niches B (expansion area). Shading indicates the species density in niche B. The red arrows show how the environmental centroid of the species niche (solid) and the background conditions (dotted) changes between regions.
The analysis of niche dynamics along each single niche variable (see Supplementary material S3) shown that climate niche of Paraserianthes lophantha was more conserved among ranges along BIO7 (Temperate annual range) and BIO6 (Min temperature of coldest month). The BIO14 (Precipitation of Driest Month) variable was the less stable in all comparisons (AN-AI, EI-AI and AF-EI). The detail examination of occurrence densities along gridded environmental gradients in univariate niche dynamics indicates that Australian invasive populations are colonizing areas less dry in the driest month (BIO14), with less precipitation in the wettest month (BIO13) and with less extreme high temperatures in the driest month than in its native range (BIO5; AN-AI). European invasive populations are colonizing areas with higher precipitations in the wettest month than in the Australian range (AN-AI and AF-EI). A greater overlap with native dry conditions was detected in Europe than in Australia, as shown in BIO14 (Precipitation of the driest month) univariate niche dynamics. An expansion to less dry conditions was also detected (AN-AI).
3.2. Study of niche overlap between invasive Paraserianthes lophantha and the native species Quercus lusitanica.
The invasive species has the bigger niche breadth. Furthermore, the niche occupied by P. lophantha is discontinuous and has two regions. The overlap between the niches of the two species was found to be low (D = 0.3). Furthermore, the niche equivalency hypothesis could not be rejected (p > 0.05), which evidences the niche differentiation between the species, as the observed niche overlap was lower than expected than chance. However, almost the entire niche of Q. lusitanica overlaps with a part of the niche of P. lophantha, and observed higher density of occurrence of both species occurs in overlapping conditions (Figure 3).
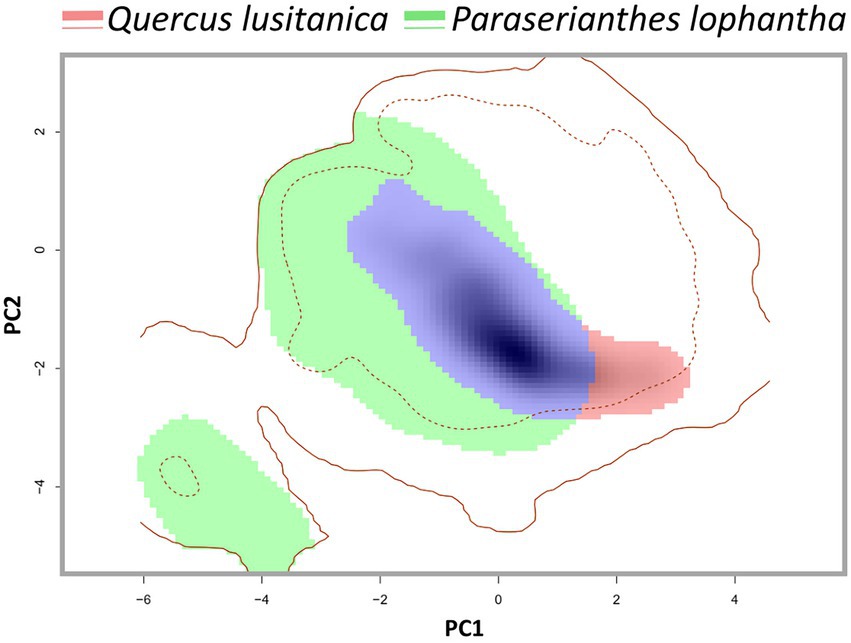
Figure 3. Fine-scale study of niche overlap between Paraserianthes lophantha and Quercus lusitanica in Monte Pindo. Solid and dotted contour lines represent 100 and 50% of the available environmental conditions that exist in available environment in Monte Pindo. Green areas represent climates only occupied by P. lophantha, blue areas indicate climates occupied both by the native Q. lusitanica and the introduced P. lophantha, red areas represent climates only occupied by Q. lusitanica. Shading indicates the density of occurrences.
4. Discussion
4.1. Can the multi-site study of the niche dynamics between the native range and more than one invasion range contribute to anticipating the expansion potential of invasive alien species?
The results of this study indicate that niches of Paraserianthes lophantha in Australia are not significantly conserved in invaded populations, which evidences that climatic niche differentiation in the continent is important. This agrees with previous work that found differences in the climatic relationships of native and invaded populations of P. lophantha in Australia (Brown et al., 2020) and supports previous research that found that niche shifts are frequent in biological invasions (Early and Sax, 2014; Dellinger et al., 2016; Atwater et al., 2018). The capacity of P. lophantha to significantly adapt rapidly to novel climatic conditions could not have been demonstrated if the study had relied only on niche comparisons between the Australian and European ranges. The failure to accept the niche equivalence hypothesis in all comparisons, suggests a lack of niche conservatism in all invaded ranges. However, the study between the Australian native and invaded ranges demonstrated a labile niche, as shown by a high expansion (E = 0.6) and displacement of the native niche centroid. In contrast, the study between the Australian native and European invaded ranges shown very limited expansion (E = 0.2), greater stability (S = 0.8) and very limited displacement in position of introduced niches, supporting a niche conservatism overall (Liu et al., 2020). Therefore, our multi-site study further supports that assessing climatic niche dynamics between the native and more than one invasion range can offer some important insights to better understand the invasion potentials of IAS (Guo et al., 2013; Broennimann et al., 2014; Bulleri et al., 2016; Silva et al., 2016; Hill et al., 2017; Datta et al., 2019; Christina et al., 2020; Carlin et al., 2022) and therefore, our hypothesis H1 can be accepted.
The climatic mismatches among Australian native and invaded ranges of P. lophantha are attributed both to large expansion (E = 0.6) and unfilling (U = 0.5). The high expansion achieved was mostly explained by a niche shift to areas less dry in the driest month, and with less extreme high temperatures in the warmest month than in its native range (Supplementary Figure S4). These new conditions are typical of the Temperate Broadleaf and Mixed Forests biome (Olson et al., 2001; Supplementary Figure S1) of temperate oceanic bioclimate regions (Rivas-Martínez et al., 2011) in which P. lophantha is expanding its invasive distribution like in some areas of eastern Australia and in Europe (Supplementary Figure S2). The drought conditions (Santamarina et al., 2022) have been suggested as can have a great impact on the species. Surrounding the native range, there are hard xeric adverse conditions that may overcome environmental tolerances of P. lophantha to this factor, acting as barriers to its natural dispersion. On the contrary, invasive populations should expand their distribution because are surrounded by a larger accessible area with less-limiting conditions, as the Australian and Europe’s temperate bioclimates (Rivas-Martínez et al., 2011). The accessibility to these suitable environments, together with the high niche unfilling detected suggests that the species has a great territory suitable to be colonized yet in Australia.
In contrast, our study found that the native climatic niche of Paraserianthes lophantha is widely conserved during its early invasion process in Europe (although niches are not statistically equivalent; Figure 2B; Table 1), which corroborates the findings of a great deal of the previous work in plant biological invasions (Petitpierre et al., 2012; Liu et al., 2020a). The Australian native niche has a substantial moderate degree of overlap with the European niche (O = 0.6) and great stability between ranges was detected (S = 0.8). This may be attributed to the presence of the species in suitable Mediterranean pluviseasonal bioclimate conditions (coastal areas of the Mediterranean basin and southern half of the Iberian Peninsula; Supplementary Figure S2). These areas are, as in its native range, characterized by low annual temperature ranges (revealing high oceanic influence) and high minimal temperatures of the coldest month (low exposure to cold and frost stress events), as shown by the he analysis of niche dynamics along each single niche variable. The ability of P. lophantha to colonize fast its climatic favorable environments at the European scale (and also its Australian invasive range) may have been likely boosted by human activity rather than P. lophantha habitat preferences. This hypothesis is supported by two clear pieces of evidence. First, there are a notable number of records of P. lophantha in Europe located at a sufficiently great distance between them to not be attributed its location to the long-dispersal natural capacity of the species (Supplementary Figure S5). Second, P. lophantha presence in invasive ranges is detected mostly close to or within human-modified environments (García-Duro et al., 2019; Santamarina et al., 2019). Taking into account these data, it can be inferred that human activity rather than a natural expansion process may be responsible for the high niche stability achieved between the native and European invasive climatic niches of P. lophantha surprisingly quickly. A note of caution must be done here. Besides the role of human activity discussed above, the dispersion of Paraserianthes lophantha may be related to unlimited dispersal capability and biological processes such as the release of biotic constraints like insect pests such as Melanterius servulus Pascoe (Impson et al., 2011), pathogens (Kleinjan and Hoffmann, 2013) or competitors.
Our results also detected the spread of P. lophantha to new environments in Europe. Despite evidence of niche conservatism and the low expansion dynamic metric obtained (E = 0.2), P. lophantha has a larger niche breadth in Europe than in its Australian native range (Figure 2B). P. lophantha expanded its niche in analogue climates, close to that of its climatic niche in Australia, but not occupied yet by the species. Therefore, we can consider that “true niche shifts” have occurred to some degree (Mandle et al., 2010; Pack et al., 2022). These areas correspond to the European temperate oceanic bioclimate regions (Rivas-Martínez et al., 2011) in which P. lophantha is expanding, showing a proven invasive behavior. One alarming possibility is that P. lophantha continues expanding its niche in Europe if it is not at equilibrium yet (Araújo and Pearson, 2005; Václavík and Meentemeyer, 2012). This is quite likely thanks to the high ability of the species to develop in different climatic conditions, as our study focused on the Australian range demonstrated (AN-AI), the low to moderate overlap achieved and the existence of both analogue and non-analogue unfilling areas in Europe (AN-EI, AF-EI; Figures 2B,C). All together, these results indicate that this species may shift and adapt to further novel environmental conditions or increase the overlap with native niches yet over the course of its spread into new areas. This is worrying, since the species could reach an even more invasive behavior in new invaded areas, as invasives often have successful faster resource use strategies in their non-native ranges (Leishman et al., 2014; Montesinos, 2022).
The existence of unfilling areas in analogue climatic conditions would not have been possible to demonstrate if the study had relied only on niche comparisons between the Australian native and European ranges (AN-EI; Figure 2B). The analysis that compared the full Australian range (including both native and invasive distributions) and the invasive range in Europe (AF-EI, Figure 2C) was the only one that detected a notable unfilling suitable space in analogue conditions and therefore, the only that achieved a score greater than zero in niche unfilling dynamic metric (U = 0.1). This is because it includes information from Australian invasive populations located in Temperate Broadleaf and Mixed Forests biomes (Supplementary Figure S1) of temperate oceanic bioclimate regions Supplementary Figure S2). If analogue unfilling results from colonization time lag, the invasion process may not have been completed and European invasive populations not in equilibrium could spread to the available suitable niche space there in the future. This is worrying given the great extension with these conditions in the European territory. Therefore, the analysis including both native and invasive distributions avoided the underestimation of the invasive potential of the species, which also corroborates our hypothesis H1.
4.2. Can the microfine-scale niche overlap study between an IAS and a native species be particularly interesting for conservation purposes?
Our micro niche study demonstrates that Paraserianthes lophantha has a great potential to continue expanding its geographical range in Monte Pindo if the necessary control measures are not implemented. As shown by its high niche breath, P. lophantha has been able to occupy a considerable amount of the environments in Monte Pindo despite its recent introduction. This demonstrates that it is a successful invader that could threaten the territory, and its local management must be of concern for conservation. The ability of P. lophantha to occupy a broader range of environmental conditions than Q. lusitanica may be explained by its higher ecological flexibility.
Furthermore, we found that P. lophantha shares an important portion of its niche with vulnerable native Q. lusitanica, although niche overlap was found to be low (O = 0.3). Therefore, P. lophantha local management must be of concern for conservation of Quercus lusitanica in Monte Pindo, since its contact could increase if no control is done on the invasive. First, results showed that the invasive species is already established in most of the environments that occupy the population of Q. lusitanica despite having an overall non-overlapping distribution with the native. This question is relevant, in line with those of previous species distribution modeling studies (Santamarina et al., 2019), demonstrates that although there is no complete geographic overlap between the species today, this is likely to occur in the future. Second, the ecological niche overlap between the species occurs in sites where the density of species occurrences of both species is higher. This is worrying since it means that the majority of P. lophantha individuals are adapted to the conditions of the population nucleus of Q. lusitanica. Taken together, our results point that P. lophantha may be a threat to a substantial portion of the population the singular population of Quercus lusitanica in Monte Pindo. Although it has been suggested that P. lophantha could exert an important competitive pressure on the vulnerable native Q. lusitanica through a high propagule pressure (Santamarina et al., 2022) and has potential for invasive advantageous behavior (Martín Osório et al., 2008; Fagúndez, 2019), further work is required to establish the incoming competitive interactions among species.
Since results from the fine-scale study of niche overlap are shown in a graphical way that is easy to understand, can be used to raise public and administration concern of the urgency of the implementation of conservation programs. Therefore, our findings are in agreement with our hypothesis H2, fine-scale niche studies can be used to promote the implementation of proactive conservation actions at a local scale.
4.3. Future directions
The use of species distribution models (Guisan and Zimmermann, 2000; Barbara et al., 2010; Anderson et al., 2011; Mateo et al., 2011; Guisan et al., 2017) may become crucial to predict Paraserianthes lophantha potential geographical distribution and overlap with Quercus lusitanica in a spatially specific way. The niche shifts detected by the study of niche dynamics between the Australian ranges demonstrate that the species is labile and may potentially acclimatize to further European conditions. Therefore, models developed based only on the native range of the species could underestimate the expansion potential of the species (Václavík and Meentemeyer, 2012) and both occurrence data from native and invasive ranges are needed, as suggested previously in other invasion contexts (Broennimann and Guisan, 2008; Ahmad et al., 2019; Pili et al., 2020). The unfilling suitable space in analogue conditions and the high stability observed in the comparative analysis between the native range and the full Australian range (AF-EI) supports that calibrating models including Australian invasive information is promising to accurate predict P. lophantha European potential distribution.
Furthermore, climatic unfillings detected in the European range under non-analogue conditions also reveal climatic areas that can turn to stability under future climate changing conditions. The low unfillings observed in the European range both in the comparative analysis using the native range (U = 0, AN-EI) and the full Australian range (U = 0.1, AF-EI) is due to most unfillings occurring under climate conditions non-analogue to the European invasive range. This may suggest that P. lophantha will never be capable of expanding its current distribution to those conditions in Europe. However, under a scenario of global change, P. lophantha may spread its range to those environments if the conditions in Europe become closer to those of the Australian range currently non-analogue. Detailed predictions of P. lophantha’s future distribution under novel climate will be necessary to answer that question and effectively act against the future spread of the invasive.
5. Conclusion and recommendations
Exploring IAS niche dynamics across different scales and resolutions is highly recommended to provide a better perspective on the management of IAS. From our results, we recommend performing multi-site niche comparisons. Specifically, it is recommended to assess similarities and dissimilarities between the climate space occupied by the IAS among multiple ranges and consider the combination of data from the native and invaded ranges in niche dynamic analysis. This approach may be greatly useful in cases where the IAS has colonized two new territories with similar climates but different to the native. Furthermore, multi-site comparison should be used in the selection of ranges where ecological niche models must be calibrated to ensure robustness in the predictions (Guisan et al., 2014; Mateo et al., 2015; Qiao et al., 2017; Chefaoui and Varela-Álvarez, 2018). With this multi-site comparison approach, we demonstrated the utility of studying also the Paraserianthes lophantha’s Australian invasive niche dynamics to avoid the underestimation of its European invasion potential. Altogether, our result suggest that the IAS may potentially adapt and spread to new Australian and European conditions. Furthermore, global climate change and human activity may facilitate the invasion processes and increase its invasion potential in the future. Further work is required to establish the geographical extension of those territories by using species distribution models. However, its inclusion in catalogues of IAS in the areas where it shows an invasive behavior, should be considered.
Increasing the use of fine-scale niche overlap studies between IAS and local endangered species could represent an important tool for the future implementation of local conservation initiatives as a complement to large-scale management strategies. We recommend the use of fine-scale studies to demonstrate in a graphically and simply way the invasive potential of IAS in local areas and the degree of overlap between co-occurring invasive and native species to raising public awareness of the urgency to implement conservation programs. These kinds of local initiatives can have a very important global impact. Our study of overlap between invasive Paraserianthes lophantha and the iconic native Quercus lusitanica can be used as a model example of how fine-scale niche studies can be used to promote the implementation of proactive conservation actions at a local scale in complement to large-scale management strategies (e.g., at European scale).
Data availability statement
The raw data supporting the conclusions of this article will be made available by the authors, without undue reservation.
Author contributions
SS, RM, EA-S and CA conceived the ideas and designed the study. SS and RM did the formal analyses. SS did data curation and wrote the original draft. All authors contributed to writing, reviewing, and editing the manuscript. All authors contributed to the article and approved the submitted version.
Funding
The Consejería de Educación de la Junta de Castilla y León and the European Social Fund through predoctoral grant held by SSG (ORDEN EDU/51100/2017), as well as, the University of León Research Team Taxonomy and Biodiversity Conservation – TaCoBi, partially supported this study. RGM was funded by the project grant NextDive (PID2021-124187NB-I00) funded by MCIN/AEI/ 10.13039/501100011033 and by “ERDF A way of making Europe”.
Conflict of interest
The authors declare that the research was conducted in the absence of any commercial or financial relationships that could be construed as a potential conflict of interest.
The reviewer VB-R declared a past co-authorship with one of the authors RM to the handling editor.
Publisher’s note
All claims expressed in this article are solely those of the authors and do not necessarily represent those of their affiliated organizations, or those of the publisher, the editors and the reviewers. Any product that may be evaluated in this article, or claim that may be made by its manufacturer, is not guaranteed or endorsed by the publisher.
Supplementary material
The Supplementary material for this article can be found online at: https://www.frontiersin.org/articles/10.3389/fevo.2022.1049142/full#supplementary-material
Footnotes
References
Aedo, C (2012). Anthos. Sistema de Información sobre las plantas de España. http://www.anthos.es/.
Ahmad, R., Khuroo, A. A., Hamid, M., et al. (2019). Predicting invasion potential and niche dynamics of Parthenium hysterophorus (congress grass) in India under projected climate change. Biodivers. Conserv. 28, 2319–2344. doi: 10.1007/s10531-019-01775-y
Alves, D. F. R., de Barros-Alves, S. P., Dolabella, S. S., et al. (2021). Invasive shrimp Cinetorhynchus erythrostictus (Decapoda: Caridea) misidentified in the marine aquarium trade: niche overlap with a native congeneric species. Estuar. Coast. Shelf Sci. 258:107411. doi: 10.1016/j.ecss.2021.107411
Amigo, J., and Romero Buján, M. I. (2018). The dwarf oak plant community, Quercus lusitanica Lam. Fagaceae, Magnoliopsida, in Monte Pindo (A Coruña, Northwest Iberian peninsula). NACC 25, 9–20.
Amaral Franco, J. (1990). “Quercus L,” in Flora Iberica. Plantas vasculares de la Península Ibérica e Islas Baleares. eds. S. Castroviejo, M. Laínz, and G. López González, et al. (Madrid: Real Jardín botánico. C.S.I.C).
Anderson, R. P., Martínez-Meyer, E., Nakamura, M., et al. (2011). Ecological niches and geographic distributions (MPB-49). Princeton, New Jersey: Princeton University Press.
Angulo, E., Ballesteros-Mejia, L., Novoa, A., Duboscq-Carra, V. G., Diagne, C., and Courchamp, F. (2021). Economic costs of invasive alien species in Spain. NeoBiota 67, 267–297. doi: 10.3897/neobiota.67.59181
Araújo, M. B., Anderson, R. P., Barbosa, A. M., et al. (2019). Standards for distribution models in biodiversity assessments. Science (New York, NY: Online) 5, eaat4858–eaat4874. doi: 10.1126/sciadv.aat4858
Araújo, M. B., and Pearson, R. G. (2005). Equilibrium of species’ distributions with climate. Holarct. Ecol. 28, 693–695. doi: 10.1111/J.2005.0906-7590.04253.X
Atwater, D. Z., Ervine, C., and Barney, J. N. (2018). Climatic niche shifts are common in introduced plants. Nat Ecol Evol. 2, 34–43. doi: 10.1038/S41559-017-0396-Z
Barbara, S., Franklin, J., and Miller, Frontmatter J. A. (2010). Mapping species distributions: Spatial inference and prediction-mapping species distributions: Spatial inference and prediction. Cambridge: Cambridge University Press.
Bates, O. K., and Bertelsmeier, C. (2021). Climatic niche shifts in introduced species. Curr. Biol. 31, R1252–R1266. doi: 10.1016/j.cub.2021.08.035
Bellard, C., Cassey, P., and Blackburn, T. M. (2016). Alien species as a driver of recent extinctions. Biol. Lett. 12:20150623. doi: 10.1098/RSBL.2015.0623
Bellard, C., Thuiller, W., Leroy, B., Genovesi, P., Bakkenes, M., and Courchamp, F. (2013). Will climate change promote future invasions? Glob. Chang. Biol. 19, 3740–3748. doi: 10.1111/gcb.12344
Bercê, W., Bello, C., Mendes, C. P., Mendes, C. P., Vancine, M. H., Galetti, M., et al. (2021). Invasive wild boar’s distribution overlap with threatened native ungulate in Patagonia. J. Mammal. 102, 1298–1308. doi: 10.1093/jmammal/gyab099
Bradley, B. A., Blumenthal, D. M., Wilcove, D. S., and Ziska, L. H. (2010). Predicting plant invasions in an era of global change. Trends Ecol. Evol. 25, 310–318. doi: 10.1016/j.tree.2009.12.003
Broennimann, O., Di Cola, V., and Guisan, A. (2021). Ecospat: Spatial ecology miscellaneous methods. R package version 3.2.
Broennimann, O., Fitzpatrick, M. C., Pearman, P. B., Fitzpatrick, M. C., Pearman, P. B., Petitpierre, B., et al. (2012). Measuring ecological niche overlap from occurrence and spatial environmental data. Glob. Ecol. Biogeogr. 21, 481–497. doi: 10.1111/j.1466-8238.2011.00698.x
Broennimann, O., and Guisan, A. (2008). Predicting current and future biological invasions: both native and invaded ranges matter. Biol. Lett. 4, 585–589. doi: 10.1098/rsbl.2008.0254
Broennimann, O., Mráz, P., Petitpierre, B., Guisan, A., and Müller-Schärer, H. (2014). Contrasting spatio-temporal climatic niche dynamics during the eastern and western invasions of spotted knapweed in North America. J. Biogeogr. 41, 1126–1136. doi: 10.1111/jbi.12274
Brown, G. K., James, E. A., Simmons, C. L., and Ahrens, C. W. (2020). Recently naturalized Paraserianthes lophantha subsp. lophantha displays contrasting genetic diversity and climate relationships compared to native populations. Diversity 12, 1–20. doi: 10.3390/d12110422
Brown, G. K., Murphy, D. J., Miller, J. T., and Ladiges, P. Y. (2008). Acacia s.s. and its relationship among tropical legumes, tribe ingeae (Leguminosae: Mimosoideae). Syst. Bot. 33, 739–751. doi: 10.1600/036364408786500136
Bulleri, F., Bruno, J. F., Silliman, B. R., and Stachowicz, J. J. (2016). Facilitation and the niche: implications for coexistence, range shifts and ecosystem functioning. Funct. Ecol. 30, 70–78. doi: 10.1111/1365-2435.12528
Calvo de Anta, R., and Macías Vázquez, F. (2005). Outes. Mapa de Solos de Galicia 1:50.000 [Outes. Soil map of Galicia 1:50.000] ed. X. de Galicia.
Canu, S., Rosati, L., Fiori, M., et al. (2015). Bioclimate map of Sardinia (Italy). J. Maps. 11, 711–718. doi: 10.1080/17445647.2014.988187/SUPPL_FILE/TJOM_A_988187_SM2357.PDF
Carapeto, A., Porto, M., and Vila-Viçosa, C. (2018). Quercus lusitanica Lam. - mapa de distribuição. In: Flora Port. Interactiva, Soc. Port. Botânica
Carlin, T., Bufford, J. L., Hulme, P. E., and Godsoe, W. K. (2022). Global assessment of three Rumex species reveals inconsistent climatic niche shifts across introduced ranges. Res Sq. doi: 10.21203/rs.3.rs-1358439/v1
Carlyon, K., Visoiu, M., Hawkins, C., Richards, K., and Alderman, R. (2015). “Rodondo Island, Bass Strait: Biodiversity & oil Spill Response Survey, January 2015,” in Natural and cultural heritage division, DPIPWE, Hobart, Nature Conservation Report Series 15/04.
Castellanos-Mejía, M. C., Herrera, J., Noguera-Urbano, E. A., et al. (2021). Potential distribution in Colombia of the introduced fish Pangasianodon hypophthalmus (Siluriformes: Pangasiidae) and implications for endangered native fish. Rev. Biol. Trop. 69, 573–587. doi: 10.15517/rbt.v69i2.44223
Charles, H., and Dukes, J. S. (2007). Impacts of invasive species on ecosystem services. Biol. Invasions 193, 217–237. doi: 10.1007/978-3-540-36920-2_13
Chase, J., and Leibold, M. A. (2003). Ecological niches linking classical and contemporary approaches. United States: University of Chicago Press.
Chefaoui, R. M., and Varela-Álvarez, E. (2018). Niche conservatism and spread of seaweed invasive lineages with different residence time in the Mediterranean Sea. Biol. Invasions 20, 423–435. doi: 10.1007/s10530-017-1544-8
Chevalier, M., Zarzo-Arias, A., Guélat, J., Mateo, G., and Guisan, A. R. (2022). Accounting for niche truncation to improve spatial and temporal predictions of species distributions. Front. Ecol. Evol. 10:944116. doi: 10.3389/fevo.2022.944116
Christina, M., Limbada, F., and Atlan, A. (2020). Climatic niche shift of an invasive shrub (Ulex europaeus): a global scale comparison in native and introduced regions. J. Plant Ecol. 13, 42–50. doi: 10.1093/JPE/RTZ041
Courchamp, F., Fournier, A., Bellard, C., Bertelsmeier, C., Bonnaud, E., Jeschke, J. M., et al. (2017). Invasion biology: specific problems and possible solutions. Trends Ecol. Evol. 32, 13–22. doi: 10.1016/j.tree.2016.11.001
Cuthbert, R. N., Diagne, C., Hudgins, E. J., et al. (2022). Biological invasion costs reveal insufficient proactive management worldwide. Sci. Total Environ. 819:153404. doi: 10.1016/j.scitotenv.2022.153404
Datta, A., Schweiger, O., and Kühn, I. (2019). Niche expansion of the invasive plant species Ageratina adenophora despite evolutionary constraints. J. Biogeogr. 46, 1306–1315. doi: 10.1111/JBI.13579
Dellinger, A. S., Essl, F., Hojsgaard, D., et al. (2016). Niche dynamics of alien species do not differ among sexual and apomictic flowering plants. New Phytol. 209, 1313–1323. doi: 10.1111/NPH.13694
Di Cola, V., Broennimann, O., Petitpierre, B., et al. (2017). Ecospat: an R package to support spatial analyses and modeling of species niches and distributions. Holarct. Ecol. 40, 774–787. doi: 10.1111/ecog.02671
Diagne, C., Leroy, B., Vaissière, A. C., Vaissière, A. C., Gozlan, R. E., Roiz, D., et al. (2021). High and rising economic costs of biological invasions worldwide. Nature 592, 571–576. doi: 10.1038/s41586-021-03405-6
Dinis, M., Vicente, J. R., César de Sá, N., et al. (2020). Can niche dynamics and distribution modeling predict the success of invasive species management using biocontrol? Insights from Acacia longifolia in Portugal. Front. Ecol. Evol. 8:358. doi: 10.3389/fevo.2020.576667
DOG (2011). DECRETO 167/2011, de 4 de agosto, por el que se modifica el Decreto 88/2007, de 19 de abril, por el que se regula el Catálogo gallego de especies amenazadas y se actualiza dicho catálogo. Galicia Cons Medio Rural, 23110–23113.
Doran, J. C., and Turnbull, J. W. (1997). Australian trees and shrubs: Species for land rehabilitation and farm planting. ACIAR books online, Camberra, Australia.
Dormann, C. F., Elith, J., Bacher, S., et al. (2013). Collinearity: a review of methods to deal with it and a simulation study evaluating their performance. Holarct. Ecol. 36, 27–46. doi: 10.1111/j.1600-0587.2012.07348.x
Early, R., Bradley, B. A., Dukes, J. S., Bradley, B. A., Dukes, J. S., Lawler, J. J., et al. (2016). Global threats from invasive alien species in the twenty-first century and national response capacities. Nat. Commun. 7, 1–9. doi: 10.1038/ncomms12485
Early, R., and Sax, D. F. (2014). Climatic niche shifts between species’ native and naturalized ranges raise concern for ecological forecasts during invasions and climate change. Glob. Ecol. Biogeogr. 23, 1356–1365. doi: 10.1111/geb.12208
Elliott, J. M., and Green, R. H. (1980). Sampling design and statistical methods for environmental biologists. J. Anim. Ecol. 49:663. doi: 10.2307/4270
Fagúndez, J. (2019). “Plantas exóticas invasoras en Galicia. Doce años de avances en el conocimiento” in Especies Exóticas Invasoras: situación e propostas de mitigación, BADER: Mon. eds. P. Ramil-Rego and C. Vales (Monografías do Ibader, Serie Biodiversidade: Lugo), 62–63.
Fernandes, R. F., Honrado, J. P., Guisan, A., et al. (2019). Species distribution models support the need of international cooperation towards successful management of plant invasions. J. Nat. Conserv. 49, 85–94. doi: 10.1016/J.JNC.2019.04.001
Font, X (2013) SIVIM, Sistema de información de la Vegetación Ibérica y Macaronésica. http://www.sivim.info/sivi/ ().
Funk, J. L., Parker, I. M., Matzek, V., et al. (2020). Keys to enhancing the value of invasion ecology research for management. Biol. Invasions 22, 2431–2445. doi: 10.1007/s10530-020-02267-9
García-Duro, J., Cruz, O., Casal, M., and Reyes, O. (2019). Fire as driver of the expansion of Paraserianthes lophantha (Willd.) I. C. Nielsen in SW Europe. Biol. Invasions 21, 1427–1438. doi: 10.1007/s10530-018-01910-w
Guisan, A., Petitpierre, B., Broennimann, O., Daehler, C., and Kueffer, C. (2014). Unifying niche shift studies: insights from biological invasions. Trends Ecol. Evol. 29, 260–269. doi: 10.1016/j.tree.2014.02.009
Guisan, A., and Thuiller, W. (2005). Predicting species distribution: offering more than simple habitat models. Ecol. Lett. 8, 993–1009. doi: 10.1111/J.1461-0248.2005.00792.X
Guisan, A., Thuiller, W., and Zimmermann, N. E. (2017). Habitat suitability and distribution models: With applications in R. Cambridge: Cambridge University Press.
Guisan, A., Tingley, R., Baumgartner, J. B., Baumgartner, J. B., Naujokaitis-Lewis, I., Sutcliffe, P. R., et al. (2013). Predicting species distributions for conservation decisions. Ecol. Lett. 16, 1424–1435. doi: 10.1111/ele.12189
Guisan, A., and Zimmermann, N. E. (2000). Predictive habitat distribution models in ecology. Ecol. Model. 135, 147–186. doi: 10.1016/S0304-3800(00)00354-9
Gundale, M. J., Pauchard, A., Langdon, B., et al. (2014). Can model species be used to advance the field of invasion ecology? Biol. Invasions 16, 591–607. doi: 10.1007/s10530-013-0610-0
Guo, W. Y., Lambertini, C., Li, X. Z., et al. (2013). Invasion of old world Phragmites australis in the new world: precipitation and temperature patterns combined with human influences redesign the invasive niche. Glob. Chang. Biol. 19, 3406–3422. doi: 10.1111/gcb.12295
Hellmann, J. J., Byers, J. E., Bierwagen, B. G., and Dukes, J. S. (2008). Five potential consequences of climate change for invasive species. Conserv. Biol. 22, 534–543. doi: 10.1111/j.1523-1739.2008.00951.x
Hill, M. P., Gallardo, B., and Terblanche, J. S. (2017). A global assessment of climatic niche shifts and human influence in insect invasions. Glob. Ecol. Biogeogr. 26, 679–689. doi: 10.1111/geb.12578
Hijmans, R. J., and Joe, C. (2017). Geographic Data Analysis and Modeling [R package raster version 2.6-7]. http://CRAN.R-project.org/package=raster
Hulme, P. E., Pyšek, P., Jarošík, V., et al. (2013). Bias and error in understanding plant invasion impacts. Trends Ecol. Evol. 28, 212–218. doi: 10.1016/J.TREE.2012.10.010
IGN, (2015). Modelo digital del terreno con paso de malla de 25m [Digital Elevation Mode 25m cell size]. http://www.ign.es.
Impson, F. A. C., Kleinjan, C. A., Hoffmann, J. H., Post, J. A., and Wood, A. R. (2011). Biological control of Australian acacia species and Paraserianthes lophantha (Willd.) Nielsen (Mimosaceae) in South Africa. African Entomology 19, 186–207. doi: 10.4001/003.019.0210
Karger, D. N., Conrad, O., Böhner, J., et al. (2017). Climatologies at high resolution for the earth’s land surface areas. Sci Data 4:170122. doi: 10.1038/sdata.2017.122
Kleinjan, C. A., and Hoffmann, J. H. (2013). Advances in clarifying the phylogenetic relationships of acacias: relevance for biological control. Acta Oecol. 48, 21–29. doi: 10.1016/J.ACTAO.2013.01.005
Kueffer, C., Pyšek, P., and Richardson, D. M. (2013). Ecological impacts of alien species: quantification, scope, caveats, and recommendations. New Phytol. 200, 615–633. doi: 10.1111/nph.12415
Kumschick, S., Gaertner, M., Vila, M., Essl, F., Jeschke, J. M., Pysek, P., et al. (2015). Ecological impacts of alien species: quantification, scope, caveats, and recommendations. Bioscience 65, 55–63. doi: 10.1093/biosci/biu193
Leishman, M. R., Cooke, J., and Richardson, D. M. (2014). Evidence for shifts to faster growth strategies in the new ranges of invasive alien plants. J. Ecol. 102, 1451–1461. doi: 10.1111/1365-2745.12318
Liu, C., Wolter, C., Xian, W., and Jeschke, J. M. (2020). Most invasive species largely conserve their climatic niche. Proc. Natl. Acad. Sci. U S A 117, 23643–23651. doi: 10.1073/pnas.2004289117
Liu, X., Petitpierre, B., Broennimann, O., Li, X., Guisan, A., and Li, Y. (2017). Realized climatic niches are conserved along maximum temperatures among herpetofaunal invaders. J. Biogeogr. 44, 111–121. doi: 10.1111/JBI.12808
Liu, C., Wolter, C., Courchamp, F., Roura-Pascual, N., and Jeschke, J. M. (2022). Biological invasions reveal how niche change affects the transferability of species distribution models. Ecology 103:e3719. doi: 10.1002/ecy.3719
Liu, C., Wolter, C., Xian, W., and Jeschke, J. M. (2020a). Most invasive species largely conserve their climatic niche. Proc. Natl. Acad. Sci. U. S. A. 117, 23643–23651. doi: 10.1073/pnas.2004289117
Liu, C., Wolter, C., Xian, W., and Jeschke, J. M. (2020b). Species distribution models have limited spatial transferability for invasive species. Ecol. Lett. 23, 1682–1692. doi: 10.1111/ele.13577
Llamas, F., Lence, C., and Acedo, C. (2003). Una nueva nothoespecie de Quercus L. (Fagaceae) en el NO de España. Lagascalia 23, 85–90.
Mack, R. N., Simberloff, D., Lonsdale, W. M., et al. (2000). Biotic Invasions: Causes, Epidemiology, Global Consequences, and Control. Ecol. Appl. 10:689. doi: 10.2307/2641039
Mandle, L., Warren, D. L., Hoffmann, M. H., Warren, D. L., Hoffmann, M. H., Peterson, A. T., et al. (2010). Conclusions about niche expansion in introduced Impatiens walleriana populations depend on method of analysis. PLoS One 5:15297. doi: 10.1371/journal.pone.0015297
Martín, O. V., Wildpret De La Torre, W., Jardim, R., and Silva, L. (2008). “Paraserianthes lophantha (Willd.) I.C. Nielsen” in Flora e Fauna Terrestre Invasora na Macaronésia. TOP 100 nos Açores, Madeira e Canárias. eds. L. Silva, O. Land, and J. L. Elizabeth Rodríguez Luengo (ARENA, Ponta Delgada), 285–287.
Mateo, R. G., Aroca-Fernández, M. J., Gastón, A., et al. (2019a). Looking for an optimal hierarchical approach for ecologically meaningful niche modeling. Ecol. Model. 409:108735. doi: 10.1016/J.ECOLMODEL.2019.108735
Mateo, R. G., Broennimann, O., Petitpierre, B., et al. (2015). What is the potential of spread in invasive bryophytes? Holarct. Ecol. 38, 480–487. doi: 10.1111/ECOG.01014
Mateo, R. G., Felicísimo, Á. M., and Muñoz, J. (2011). Modelos de distribución de especies: Una revisión sintética. Rev. Chil. Hist. Nat. 84, 217–240. doi: 10.4067/S0716-078X2011000200008
Mateo, R. G., Gastón, A., Aroca-Fernández, M. J., et al. (2019b). Hierarchical species distribution models in support of vegetation conservation at the landscape scale. J. Veg. Sci. 30, 386–396. doi: 10.1111/JVS.12726
Matzek, V., Pujalet, M., and Cresci, S. (2015). What managers want from invasive species research versus what they get. Conserv. Lett. 8, 33–40. doi: 10.1111/CONL.12119
Mcgeoch, M. A., Butchart, S. H. M., Spear, D., et al. (2010). Global indicators of biological invasion: species numbers, biodiversity impact and policy responses diversity and distributions. Divers. Distrib. 16, 95–108. doi: 10.1111/j.1472-4642.2009.00633.x
Meyerson, L. A., and Mooney, H. A. (2007). Invasive alien species in an era of globalization. Front. Ecol. Environ. 5, 199–208. doi: 10.1890/1540-9295(2007)5[199:IASIAE]2.0.CO;2
Montesinos, D. (2022). Fast invasives fastly become faster: invasive plants align largely with the fast side of the plant economics spectrum. J. Ecol. 110, 1010–1014. doi: 10.1111/1365-2745.13616
Nic Lughadha, E., Bachman, S. P., Leão, T. C. C., Forest, F., Halley, J. M., Moat, J., et al. (2020). Extinction risk and threats to plants and fungi. Plants, People, Planet 2, 389–408. doi: 10.1002/PPP3.10146
Olson, D. M., Dinerstein, E., Wikramanayake, E. D., et al. (2001). Terrestrial ecoregions of the world: a new map of life on earth. Bioscience 51, 933–938. doi: 10.1641/0006-3568(2001)051[0933:TEOTWA]2.0.CO;2
Pack, K. E., Mieszkowska, N., and Rius, M. (2022). Rapid niche shifts as drivers for the spread of a non-indigenous species under novel environmental conditions. Divers. Distrib. 28, 596–610. doi: 10.1111/ddi.13471
Parravicini, V., Azzurro, E., Kulbicki, M., and Belmaker, J. (2015). Niche shift can impair the ability to predict invasion risk in the marine realm: an illustration using Mediterranean fish invaders. Ecol. Lett. 18, 246–253. doi: 10.1111/ELE.12401
Pearman, P. B., Guisan, A., Broennimann, O., and Randin, C. F. (2008). Niche dynamics in space and time. Trends Ecol. Evol. 23, 149–158. doi: 10.1016/j.tree.2007.11.005
Pearson, R. G., and Dawson, T. P. (2003). Predicting the impacts of climate change on the distribution of species: are bioclimate envelope models useful? Glob. Ecol. Biogeogr. 12, 361–371. doi: 10.1046/J.1466-822X.2003.00042.X
Peterson, A. T. (2011). Ecological niche conservatism: a time-structured review of evidence. J. Biogeogr. 38, 817–827. doi: 10.1111/j.1365-2699.2010.02456.x
Petitpierre, B., Kueffer, C., Broennimann, O., Randin, C., Daehler, C., and Guisan, A. (2012). Climatic niche shifts are rare among terrestrial plant invaders. Science 335, 1344–1348. doi: 10.1126/science.1215933
Phillips, S. J., Dudík, M., Elith, J., et al. (2009). Sample selection bias and presence-only distribution models: implications for background and pseudo-absence data. Ecol. Appl. 19, 181–197. doi: 10.1890/07-2153.1
Pili, A. N., Tingley, R., Sy, E. Y., Diesmos, M. L. L., and Diesmos, A. C. (2020). Niche shifts and environmental non-equilibrium undermine the usefulness of ecological niche models for invasion risk assessments. Sci. Rep. 10, 7972–7918. doi: 10.1038/s41598-020-64568-2
Pimentel, D., Lach, L., Zuniga, R., and Morrison, D. (2000). Environmental and economic costs of nonindigenous species in the United States. Bioscience 50, 53–65. doi: 10.1641/0006-3568(2000)050[0053:EAECON]2.3.CO;2
Pyšek, P., Jarošík, V., Hulme, P. E., Hulme, P. E., Pergl, J., Hejda, M., et al. (2012). A global assessment of invasive plant impacts on resident species, communities and ecosystems: the interaction of impact measures, invading species’ traits and environment. Glob. Chang. Biol. 18, 1725–1737. doi: 10.1111/J.1365-2486.2011.02636.X
Pyšek, P., Richardson, D. M., Pergl, J., et al. (2008). Geographical and taxonomic biases in invasion ecology. Trends Ecol. Evol. 23, 237–244. doi: 10.1016/J.TREE.2008.02.002
Qiao, H., Escobar, L. E., and Peterson, T. A. (2017). Accessible areas in ecological niche comparisons of invasive species: recognized but still overlooked. Sci. Rep. 7, 1213–1219. doi: 10.1038/s41598-017-01313-2
R Core Team. (2022). R: A language and environment for statistical computing. R Foundation for Statistical Computing, Vienna, Austria.
Randall, R. P. (ed) (2017). A global compendium of weeds. 3rd edition, 3rd edn. Perth, Western Australia: National Library of Australia.
Rivas-Martínez, S., Rivas-Sáenz, S., and Penas, A. S. (2011). Worldwide bioclimatic classification system. Glob Geobot 1, 1–634.
Rivas-Martínez, S., and Sáenz Laín, C. (1991). “Enumeración de los Quercus de la Península Ibérica” in Rivasgodaya (Madrid: Departamento de Biología Vegetal II (Botánica), Facultad de Farmacia, Universidad Complutense), 101–110.
Rivas-Martínez, S., Izco, J., Díaz-González, T. E., et al. (2014). The Galician-Potuguese biogeographic sector. An initial advance. Int. J. Geobot. Res. 4, 65–81. doi: 10.5616/ijgr140002
Rödder, D., and Engler, J. O. (2011). Quantitative metrics of overlaps in Grinnellian niches: advances and possible drawbacks. Glob. Ecol. Biogeogr. 20, 915–927. doi: 10.1111/j.1466-8238.2011.00659.x
Santamarina, S., Alfaro-Saiz, E., Llamas, F., and Acedo, C. (2019). Different approaches to assess the local invasion risk on a threatened species: opportunities of using high-resolution species distribution models by selecting the optimal model complexity. Glob Ecol Conserv 20:e00767. doi: 10.1016/j.gecco.2019.e00767
Santamarina, S., Montesinos, D., Alfaro-Saiz, E., and Acedo, C. (2022). Drought affects the performance of native oak seedlings more strongly than competition with invasive crested wattle seedlings. Plant Biol. 24, 1297–1305. doi: 10.1111/PLB.13416
Sax, D. F., Early, R., and Bellemare, J. (2013). Niche syndromes, species extinction risks, and management under climate change. Trends Ecol. Evol. 28, 517–523. doi: 10.1016/j.tree.2013.05.010
Schoener, T. W. (1970). Nonsynchronous spatial overlap of lizards in patchy habitats. Ecology 51, 408–418. doi: 10.2307/1935376
Sequeira, A. M. M., Bouche, T. P. J., Yates, K. L., et al. (2018). Transferring biodiversity models for conservation: opportunities and challenges. Methods Ecol. Evol. 9, 1250–1264. doi: 10.1111/2041-210X.12998
Silva, D. P., Vilela, B., Buzatto, B. A., et al. (2016). Contextualized niche shifts upon independent invasions by the dung beetle Onthophagus taurus. Biol. Invasions 18, 3137–3148. doi: 10.1007/s10530-016-1204-4
Soberón, J., and Nakamura, M. (2009). Niches and distributional areas: concepts, methods, and assumptions. Proc. Natl. Acad. Sci. U. S. A. 106, 19644–19650. doi: 10.1073/PNAS.0901637106
Syfert, M. M., Smith, M. J., and Coomes, D. A. (2013). The effects of sampling bias and model complexity on the predictive performance of MaxEnt species distribution models. PLoS One 8:e55158. doi: 10.1371/JOURNAL.PONE.0055158
Thomas, C. D., Cameron, A., Green, R. E., et al. (2004). Extinction risk from climate change. Nature 427, 145–148. doi: 10.1038/nature02121
Thompson, G. D., Richardson, D. M., Wilson, J. R. U., Bellstedt, D. U., and le Roux, J. J. (2016). Genetic diversity and structure of the globally invasive tree, Paraserianthes lophantha subspecies lophantha, suggest an introduction history characterised by varying propagule pressure. Tree Genet. Genomes 12:27. doi: 10.1007/s11295-016-0984-0
Thuiller, W., Araújo, M. B., and Lavorel, S. (2004). Do we need land-cover data to model species distributions in Europe? J. Biogeogr. 31, 353–361. doi: 10.1046/j.0305-0270.2003.00991.x
Thuiller, W., Richardson, D. M., Pyssek, P., et al. (2005). Niche-based modeling as a tool for predicting the risk of alien plant invasions at a global scale. Glob. Chang. Biol. 11, 2234–2250. doi: 10.1111/j.1365-2486.2005.001018.x
Turbelin, A. J., Malamud, B. D., and Francis, R. A. (2017). Mapping the global state of invasive alien species: patterns of invasion and policy responses. Glob. Ecol. Biogeogr. 26, 78–92. doi: 10.1111/geb.12517
Václavík, T., and Meentemeyer, R. K. (2012). Equilibrium or not? Modeling potential distribution of invasive species in different stages of invasion. Divers. Distrib. 18, 73–83. doi: 10.1111/j.1472-4642.2011.00854.x
Van Kleunen, M., Dawson, W., Essl, F., et al. (2015). Global exchange and accumulation of non-native plants. Nat. Cell Biol. 525, 100–103. doi: 10.1038/nature14910
Van Rees, C. B., Hand, B. K., Carter, S. C., et al. (2022). A framework to integrate innovations in invasion science for proactive management. Biol. Rev. 97, 1712–1735. doi: 10.1111/brv.12859
Ventenat, É. P., Cels, J.-M., and Redouté, H. J. (2013). Description des plantes nouvelles et peu connues: cultivées dans le jardin de J.M. Cels: avec figures
Vicente, J. R., Gonçalves, J., Honrado, J. P., Randin, C. F., Pottier, J., Broennimann, O., et al. (2014). A framework for assessing the scale of influence of environmental factors on ecological patterns. Ecol. Complex. 20, 151–156. doi: 10.1016/J.ECOCOM.2014.10.005
Vilà, M., Corbin, J. D., Dukes, J. S., et al. (2007). “Linking Plant Invasions to Global Environmental Change” in Terrestrial Ecosystems in a Changing World. Global Changue— The IGBP Series. eds. J. G. Canadell, D. E. Pataki, and L. F. Pitelka (Berlin, Heidelberg: Springer), 93–102.
Vilà, M., Basnou, C., Pyšek, P., Josefsson, M., Genovesi, P., Gollasch, S., et al. (2010). How well do we understand the impacts of alien species on ecosystem services? A pan-European, cross-taxa assessment. Front. Ecol. Environ. 8, 135–144. doi: 10.1890/080083
Vilà, M., Espinar, J. L., Hejda, M., Hulme, P. E., Jarošík, V., Maron, J. L., et al. (2011). Ecological impacts of invasive alien plants: a meta-analysis of their effects on species, communities and ecosystems. Ecol. Lett. 14, 702–708. doi: 10.1111/j.1461-0248.2011.01628.x
Vitousek, P. M., Mooney, H. A., Lubchenco, J., and Melillo, J. M. (1997). Human domination of earth’ s ecosystems. Summarized proceedings for the period from and a directory of members as of 277, 494–499. doi: 10.1126/science.277.5325.494
Warren, D. L., Glor, R. E., and Turelli, M. (2008). Environmental niche equivalency versus conservatism: quantitative approaches to niche evolution. Evolution, Lanc 62, 2868–2883. doi: 10.1111/j.1558-5646.2008.00482.x
Wiens, J. J., Ackerly, D. D., Allen, A. P., Anacker, B. L., Buckley, L. B., Cornell, H. V., et al. (2010). Niche conservatism as an emerging principle in ecology and conservation biology. Ecol. Lett. 13, 1310–1324. doi: 10.1111/j.1461-0248.2010.01515.x
Keywords: biological invasions, threatened species, Ecospat, niche conservatism, niche shift
Citation: Santamarina S, Mateo RG, Alfaro-Saiz E and Acedo C (2023) On the importance of invasive species niche dynamics in plant conservation management at large and local scale. Front. Ecol. Evol. 10:1049142. doi: 10.3389/fevo.2022.1049142
Edited by:
Britta Uhl, Goethe University Frankfurt, GermanyReviewed by:
Víctor Bello-Rodríguez, University of La Laguna, SpainIsrael Borokini, University of California, Berkeley, United States
Copyright © 2023 Santamarina, Mateo, Alfaro-Saiz and Acedo. This is an open-access article distributed under the terms of the Creative Commons Attribution License (CC BY). The use, distribution or reproduction in other forums is permitted, provided the original author(s) and the copyright owner(s) are credited and that the original publication in this journal is cited, in accordance with accepted academic practice. No use, distribution or reproduction is permitted which does not comply with these terms.
*Correspondence: Sara Santamarina, ✉ c3NhbmdAdW5pbGVvbi5lcw==