- 1Konrad Lorenz Institute of Ethology, Department of Interdisciplinary Life Sciences, University of Veterinary Medicine, Vienna, Austria
- 2Department of Biology, Université Laval, Québec, QC, Canada
- 3Research Institute of Wildlife Ecology, Department of Interdisciplinary Life Sciences, University of Veterinary Medicine, Vienna, Austria
Air-borne chemicals are highly abundant sensory cues and their use in navigation might be one of the major evolutionary mechanisms explaining the development of olfaction in animals. Despite solid evidence for the importance of olfaction in avian life (e.g., foraging or mating), the importance of chemical cues in avian orientation remains controversial. In particular, songbirds are sorely neglected models, despite their remarkable orientation skills. Here we show that great tits (Parus major) require olfactory cues to orientate toward winter-feeding sites within their home range after displacement. Birds that received an olfaction-depriving treatment were impaired in homing. However, the return rates between olfaction-deprived and control individuals did not differ. Birds with decreased perception of olfactory cues required more time to return to the winter feeding sites. This effect became apparent when the distance between the releasing and capture sites was greater. Our results indicate that even in a familiar environment with possible visual landmarks, scent cues might serve as an important source of information for orientation.
Introduction
The Atlantic salmon (Salmo salar) follows olfactory cues to travel over thousands of kilometers to its spawning sites (Stabell, 1984; Dukes et al., 2004) and sea turtles memorize the scent of their natal beaches to which they return after many years spent in the ocean (Southwood and Avens, 2010). Such spectacular olfactory skills are often found in the animal kingdom and a link between navigation and olfaction is present in many organisms, including arthropods, fish, reptiles, birds and mammals (Stabell, 1984; McGregor and Teska, 1989; Reinhard et al., 2004; Catania, 2006; Southwood and Avens, 2010; Jacobs, 2012; Gagliardo, 2013). Moreover, navigation might be one of the major evolutionary mechanisms explaining the development of olfaction (Jacobs, 2012; Jacobs et al., 2015). Even in humans, recent evidence highlights, that the sense of smell, cognitive abilities and orientation are intrinsically linked and olfactory orientation co-varies with navigation ability (Jacobs et al., 2015; Dahmani et al., 2018). Our knowledge and understanding, about the use of smell for orientating in complex environments, however, is still limited (Jacobs, 2012; Gagliardo, 2013).
There is a growing body of empirical studies showing that species across many taxa memorize air- or waterborne chemicals of their home range or a place of interest (e.g., foraging territories) and after relocation, use olfactory cues to orientate toward a specific destination (e.g., following a gradient of volatile organic compounds) (Waldvogel, 1989; Papi, 1990; Wallraff, 2004; Gagliardo, 2013). Despite the belief that visual and auditory signals are the predominant senses in birds, they are one of the best-known models for olfactory navigation. Birds were considered anosmic for a long time; therefore, to-date there is only little knowledge on the olfactory information birds use to relocate toward a given destination of interest (e.g., type of odor). However, growing evidence confirms that olfaction is ubiquitous in avian species and an important mechanism facilitating orientation and navigation in their environment (e.g., foraging site, nesting site). Homing pigeons remain the most prominent model (Papi et al., 1972; Wallraff, 2004, 2005), but also procellariiformes seabirds and gull species, use olfactory cues over large spatial scales to navigate toward breeding sites and locate their nesting burrows, supporting the idea that birds use their sense of smell for orientation and navigation (Bonadonna et al., 2003, 2005; Nevitt, 2006, 2008; Pollonara et al., 2015; Reynolds et al., 2015).
For songbirds, there is comparatively little evidence that they use olfactory cues in navigation or orientation and where there is, it pertains mainly to migratory birds (Fiaschi et al., 1974; Wallraff et al., 1995; Steiger et al., 2008; Holland et al., 2009; Caspers and Krause, 2011; Rastogi et al., 2011; Gagliardo, 2013; Kishkinev et al., 2019). Catbirds (Dumetella carolinensis) migrating to their wintering sites were found to exhibit impaired orientation behavior after treatment with zinc sulfate. In starlings (Sturnus vulgaris) and swifts (Apus apus) olfactory nerve section significantly affected homing to their breeding sites (Fiaschi et al., 1974; Wallraff et al., 1995; Wallraff, 2003; Holland et al., 2009). These behavioral experiments are corroborated by physiological data, which indicate higher neuronal activity of the olfactory system in migratory passerines during migration (Rastogi et al., 2011, 2016). However, the importance of olfaction in the orientation and navigation of migrating passerines is still controversial (Kishkinev et al., 2019).
Given the phylogenetic predisposition, the question arises whether olfaction is also of importance for orientation within the home range in non-migratory songbirds (Steiger et al., 2008), but to the best of our knowledge, the use of scent in orientation on a small spatial scale has scarcely been investigated (Gagliardo, 2013). Vision and auditory signals are regarded the predominant senses in avian species and in consideration of this, one might assume that within the home range or the territory of a bird species, these two senses might be sufficient to facilitate orientation. However, distinct olfactory cues might also contribute in orientation and odorant sources can serve as additional landmarks to gain information about a given location to optimize orientation within familiar environments. Some species of the family Paridae, for example, blue tits (Cyanistes caeruleus) and great tits, seem to use olfaction to locate and access food in the immediate vicinity (Amo et al., 2013; Mäntylä et al., 2017; Hiltpold and Shriver, 2018), which confirms that passerine species use smell to locate a distinct stimulus (e.g., a specific food resource). Considering that many songbird species spend their entire life in the same area, sometimes even within a permanent territory or home range, memorizing olfactory landmarks, for example scents emanating from foraging sites, within territories and using information resembling an “olfactory landscape” might be even more beneficial than to migrants (Gagliardo, 2013). Whether they require their sense of smell for orientation within their home range to relocate toward their feeding sites and optimize foraging, though, has still to be determined.
To understand whether there is a link between relocating toward known feeding sites and olfaction in non-migratory songbirds, we designed an experiment on a population of resident great tits. We manipulated the olfactory abilities of birds with either zinc sulphate (anosmic) or distilled water (control) and released the individuals in either close vicinity or further away (i.e., on the outskirts of their home range) from their winter feeding sites (Krams et al., 2006). These birds often remain in their breeding grounds during winter and, particularly in urban areas, exploit ephemeral appearing artificial feeding sites provided by humans (van Balen and Hage, 1989; John, 2010). Memorizing the position of olfactory cues may also help to relocate such artificial feeding places quicker and hence could be a beneficial overwintering strategy that allows individuals to reduce foraging time, which could be critical for survival during harsh winter conditions.
Great tits display strong site fidelity and were shown to return to their winter feeding grounds even after displacement of up to 7.8 km (Krištín and Kaňuch, 2017). Therefore, although given the predominant visual competences of birds and their familiarity with the local environment (Wallraff et al., 1994), we expect resident great tits to relocate to the feeders, independently of their olfactory capacities. This is also based on previous findings in starlings, demonstrating no differences in the return rates between control and olfaction-deprived individuals released in vicinity to their nesting grounds (Wallraff et al., 1995). In the study by Wallraff et al. (1995) the birds were released within a minimum distance of 60 km, whereas our experiment involved only short releasing distances (max. 1,500 m). Thus, we do not expect to detect differences in the total return rate between the experimental groups. We further predict that birds with limited abilities of olfactory orientation will require more time to orientate and screen the area to relocate to the feeding sites and the variable “return time” will serve as suitable proxy for this activity. Therefore, we also recorded the return time and considered it as a more sensitive measure to detect a possible function of olfaction in orientation. If olfaction serves in this functional context, we expect a distance and treatment effect e.g., sham-treated individuals to return faster than anosmic birds and this should additionally increase with distance.
Materials And Methods
Study species
We chose to focus our study on the great tit, a European passerine species with a wide distribution range, because they are highly abundant breeding birds in the study area. More importantly, great tits have been suggested to have a well-developed sense of smell and there is indication that they might use chemical cues in predator detection and foraging (Amo et al., 2011, 2013; Mäntylä et al., 2020).
Great tits are partial migrants, but they often remain in their breeding grounds during winter and are within the dominant species observed at artificial winter-feeding sites. They often remain alone or in pairs, but frequently join mixed species flocks. The size of breeding territories is suggested to underlie strong variation between habitats and years but was estimated to range from 0.06 to 14 ha in some populations (Lemel, 1989; Wilkin et al., 2006). Krams et al. (2006) studied individuals and flocks of wintering great tits and estimated home ranges of approximately 5 ha. However, overall there is surprisingly little data available but there is indication that in some populations, birds remaining in their breeding grounds over winter will display high site fidelity (De Laet, 1984; Nakamura and Shindo, 2001) and return after displacement to their local feeding sites (Krištín and Kaňuch, 2017). Considering the high territoriality and the previously shown high return rate to winter-feeding sites after displacement, this species might significantly rely on artificial feeders as over-wintering strategy. However, we have also observed straying individuals that most likely originated from large flocks that pass through the study area during spring migration (own observations indicated by a ring recovery in Russia).
General methods
The study was conducted between the 6th of February and the 11th of March 2014 in the surroundings of the Sacré Coeur School, Pressbaum, Austria (48°18′ N, 16°08′ E; about 320m a.s.l.) (Mahr et al., 2012, 2015, 2016). In total, n = 112 great tits were caught from three feeding sites, which were established in the previous years. Each feeding site was provided with one hopper feeder with a roof and transparent side walls, installed on a branch in a height of approximately 1.7 m. The feeders contained sunflower seeds, but additional feeding opportunities (commercially available fat balls) have been added 2 months before the beginning of the experiment to increase the attractiveness of the feeding site. Until the start of the experiment, the feeding sites were controlled at least twice per week, however, during the experimental period feeders were controlled and refilled daily and the additional food was replaced when necessary.
Mistnetting at the feeding sites was conducted from 0730–1300 between the 6th and 28th of February. Immediately after the capture, birds were transferred to a nearby indoor banding facility and each individual received an aluminum ring and a unique combination of three darvic color rings for further identification. Standard morphological measurements of body mass (with a digital scale, to the nearest 0.1 g) and tarsus length (with a digital caliper, to the nearest 0.1 mm) were taken. Subsequently, individuals were randomly assigned to an experimental (n = 56) and a control group (n = 56). To minimize a possible impact of body condition on the motivation to return and further to reduce adverse effects of the handling procedure (e.g., delay in foraging) on the health of the animals, only individuals with a minimum fat score of 1 (official guidelines used by the “Vogelwarte Radolfzell”) were included in the experiment.
All experimental procedures were in accordance with current laws on animal experimentation in Austria and the European Union. Experimental procedures were approved by the institutional ethics committee and the national authority according to §26 of the Law for Animal Experiments, “Tierversuchsgesetz 2012-TVG 2012.” The studies concern short time experiments without any surgery and no long-term effects on the birds were expected, confirmed by adequate monitoring after release (GZ 68.205/0034-WF/II/3b/2014).
Experimental design
We manipulated the olfactory capacity by rinsing the choanae of individuals with 300 μl of a 4% solution of zinc sulphate (anosmic) (Benvenuti et al., 1993; Benvenuti and Gagliardo, 1996; Holland et al., 2009; Kishkinev et al., 2019). This frequently used method serves in short-term manipulation of scent perception (i.e., induction of anosmia) in avian species, but currently only little information on the efficacy and duration of the treatment is available. In mice, flushing the nasal cavities with zinc sulfate leads to a brief and strong effect, inducing an initial complete anosmia with full recovery (McBride et al., 2003). Overall, the recovery period from zinc sulfate treatment underlies strong individual variation, depending on the concentration of the applied solution, the species and the behavioral tasks (e.g., scent discrimination). However, first signs of recovery were observed after 2–7 days in different species when individuals were treated with similar concentrations of zinc sulfate solution (Slotnick and Gutman, 1977; McBride et al., 2003; Abreu et al., 2017). The effect occurs as the solution leads to the degeneration of the olfactory cells, but has no impact on the basal cell layer, the respiration system and the bird’s health (Slotnick and Gutman, 1977; Cancalon, 1982; Schlund, 1992; Benvenuti et al., 1993; Burd, 1993). Individuals assigned to the control group received a sham manipulation with 300 μl of distilled water (control).
After the handling procedure, we transferred the birds immediately to either of the two releasing sites. These were located in a distance of approximately 400 m (48°11′N 16°05′E) or 1,500 m (48°11′N 16°04′E) from the winter feeding sites. The release distance was equally distributed over the sample size. We chose two open meadow areas with adjacent patches of mixed deciduous forest of similar vegetation height and density of the understory [mainly consisting of young European beech (Fagus sylvatica)] as releasing sites. These landscapes characterize the study area and its surroundings. No urban structures with additional feeding sites were located between either of the two releasing sites and the catching site. However, there are settlements in close proximity of the study area and winter feeding might take place in private gardens.
For each individual, we recorded the exact time of capture and release and the overall handling protocol did not exceed 40 min. However, considering the lack of information on zinc sulfate administration on avian behaviors, we conducted behavioral observations of all individuals immediately after the release. We recorded variables that might indicate adverse effects of the treatment on the individual behavior (Supplementary Table 1). Our analyzes revealed that there were no effects of zinc sulfate treatment on the time of departure from the releasing site (> 40 m from the releasing site) and perching behavior (i.e., how much an individual moved before leaving the area) that might serve as a proxy for orientation (Supplementary Table 1).
By subsequent monitoring at the feeding sites, the return rate and time were recorded. We started the monitoring after the first individuals were released and observed the feeders daily until 11 days after the last bird was caught (6th of February–11th of March 2014). Unless mistnetting was conducted, the monitoring started at 0730 and ended at 1700. Thereby one monitoring circuit consisted of three 15 min observation units at each feeding site and a 5 min break between these units. The observations were continuous and every feeding site was observed an equal amount of time per day. In addition, the sequence of the observations (i.e., which feeding site was observed) was altered daily. To avoid disturbance, we observed the feeders from a distance of 10 m, using binoculars. The ring combination and the exact time of observation was recorded for each individual. All observers were blind to the experimental group (i.e., were unaware of the ring combinations used for each bird).
Statistical analyzes
To reach a sufficient sample size and increase the likelihood of observing target individuals, we pooled all observations and captures from three feeding sites with a maximum distance of approximately 130 m.
All analyzes were performed using the statistical software R 3.2.4 (2016-03-16) (R Core Team, 2016) with the packages MuMIn 1.15.6 (Barton, 2019), MASS 7.3-45 (Venables and Ripley, 2002), lme4 1.1-12 (Bates et al., 2015), and mgcv 1.8-12 (Wood, 2017). The data were tested for normal distribution and statistical analyzes were conducted as appropriate.
To control for an individuals need (motivation) to forage and hence return to the feeders, we incorporated body condition into our models. We defined body condition as body mass corrected for body size (residual body mass). This variable was achieved by fitting a linear regression using body mass as a dependent variable and tarsus length (a proxy for body size and development) as an independent variable (β = 0.6245, SE = 0.2293, p = 0.00753). The individual deviation from the regression line was used for subsequent analysis.
The present study is an experiment from a biological perspective, but from the statistical point of view it presents a correlative study, we therefore followed an information theoretic approach (Burnham and Anderson, 2002).
Firstly, we try to test for possible treatment effects on homing behavior, therefore we used two dependent variables, namely the return rate (categorical variable) and return time (continuous variable). Generalized linear models (GLM) with a binomial link were fitted onto the dataset to investigate the importance of olfactory treatment (anosmic/control) and distance on homing behavior toward the food source. We fitted a negative binomial model that corrected for over dispersion in the data. The variables “anosmic” (c/a) and “distance” (400 m/1,500 m) were included as factors with two levels. Furthermore, the variable “condition” was added as a numeric variable. To test if treatment had an effect on the return rate, the response variable “return rate” was included as a factor with two levels (yes/no). To examine the effect on return time, the response variable “return time” was instead included as a continuous variable.
Secondly, we used linear regression models. For all potential models, the Akaike weights were calculated. Estimates and standard errors were averaged based on Akaike weights (Burnham and Anderson, 2002).
Based on the sum of the Akaike weights of all models containing a variable, the relative variable importance (RVI) was calculated. The RVI is the probability that a given variable will be in the model, which explains the data best. Variables with RVI values > 0.9 are very likely to affect the response variable, variables with values of about 0.7 are likely, the effect of variables with values of 0.5 and below is uncertain and values under 0.2 indicate that the variable is very unlikely to affect the response (Burnham and Anderson, 2002).
Results
In total, 47 of 112 marked individuals returned to the feeders: 24 from the anosmic group and 23 control birds. In relation to the treated (anosmic) birds, 16 returned from the short and eight from the long distance (Figure 1A). In the control group, 14 birds returned from the short and nine from the long distance (Figure 1A). According to the results of the GLM, neither the releasing distance, olfactory treatment, body condition nor the interaction between them were important (Table 1). Similarly, using the information-theoretic approach, the results regarding the return rate revealed low RVI values, including olfactory treatment (0.30), distance (0.51), body condition (0.41) and the interaction (0.04) between the body condition and olfactory treatment. This suggests that it is unlikely that any of the variables are included in the best model and thus there was no effect of the investigated variables on the return rate of great tits to the feeders. The highest value (RVI value = 0.51), for releasing distance, is still low and it is uncertain that this variable would be in the best model.
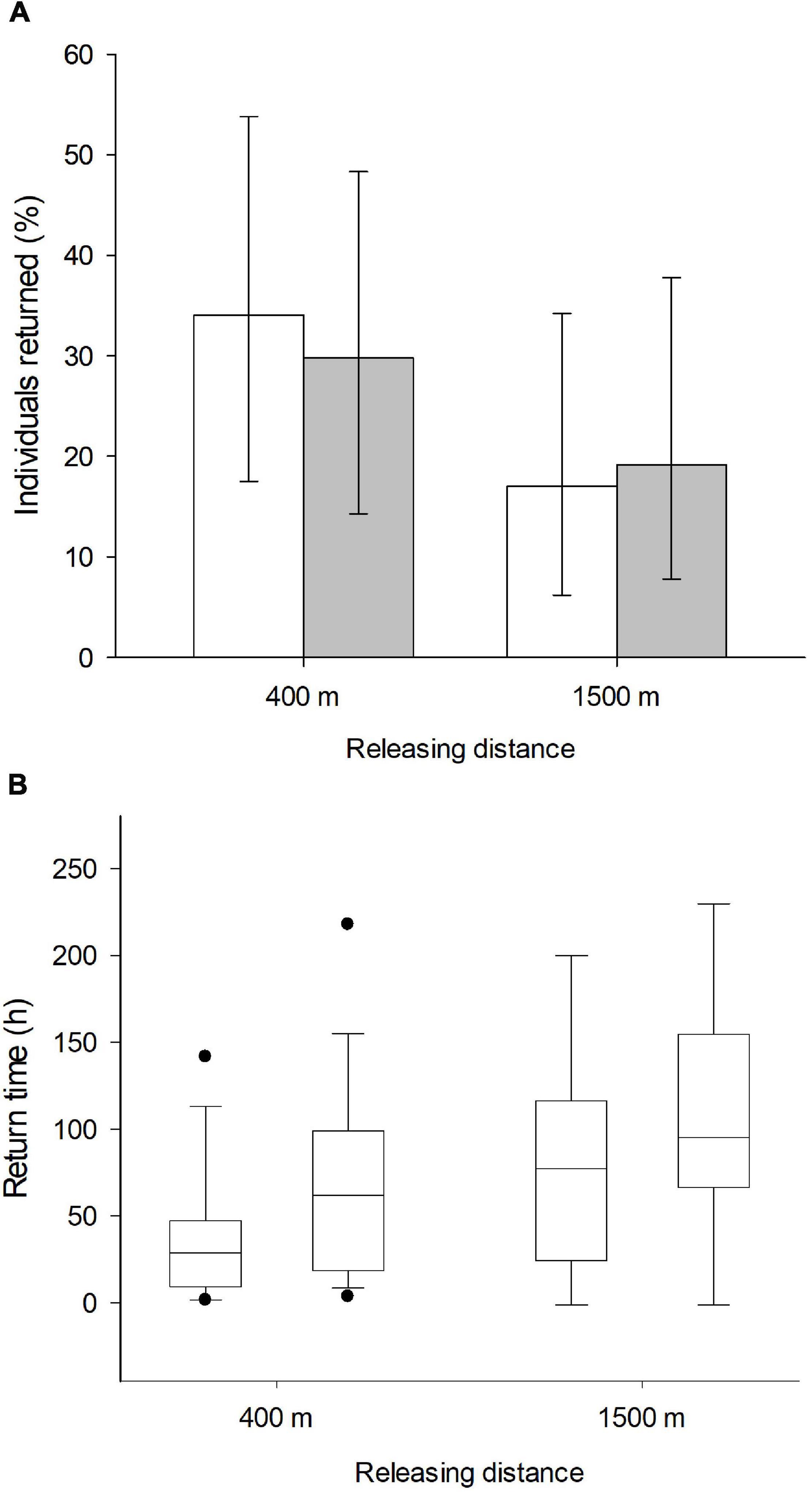
Figure 1. Presented are (A) the average proportion (%) ± 95 confidence limits of birds returned to the feeder. Empty bars present the control group and gray bars the anosmic birds. The box plot (B) shows the time (in h) (medians, quartiles, 5th and 95th percentiles, and extreme values), great tits needed to return to the feeding site in relation to releasing distance (400 m/1,500 m) and their olfactory treatment (left control and right anosmic group, respectively).
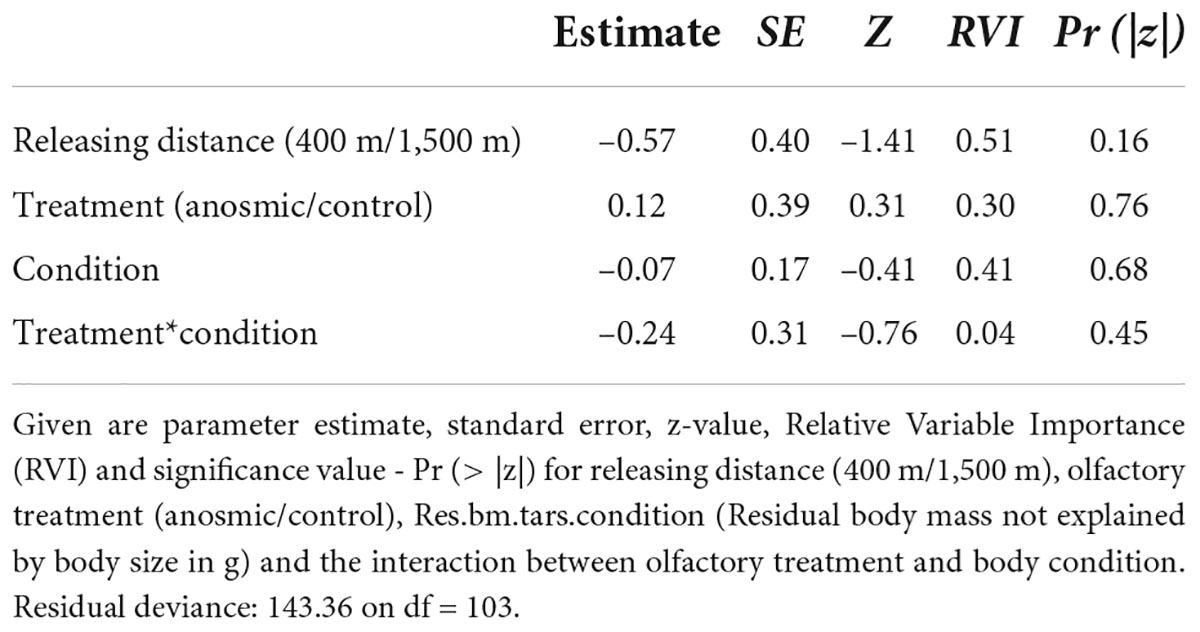
Table 1. Shown are the results of a generalized linear models (GLM) for return rate as the dependent variable based on the model: glm(formula = returned ∼ releasing distance + olfactory treatment * Res.bm.tars.condition, family = binomial).
Regarding the “return time,” however, our results derived from the GLM revealed that the releasing distance and treatment had significant effects on the time the birds needed to return to the feeders (Figure 1B). The birds required more time to return from the long (1,500 m) in comparison to the short distance (400 m) (Figure 1B, Table 2), but also olfactory treatment had a significant effect (Figure 1B, Table 2). Anosmic individuals needed longer to return to the feeders than control individuals. These results were confirmed using the information-theoretic approach. Using the return time as a dependent variable revealed very high RVIs for distance (0.98) and olfactory treatment (0.84). Relative variable importance values for body condition (0.32) and the interaction (0.09) between body condition and olfactory treatment revealed very low values and accordingly, there seems to be no interaction between the dependent variable and the body condition of the birds. The return time of great tits to the feeders seems to have been influenced by the releasing distance and olfactory treatment.
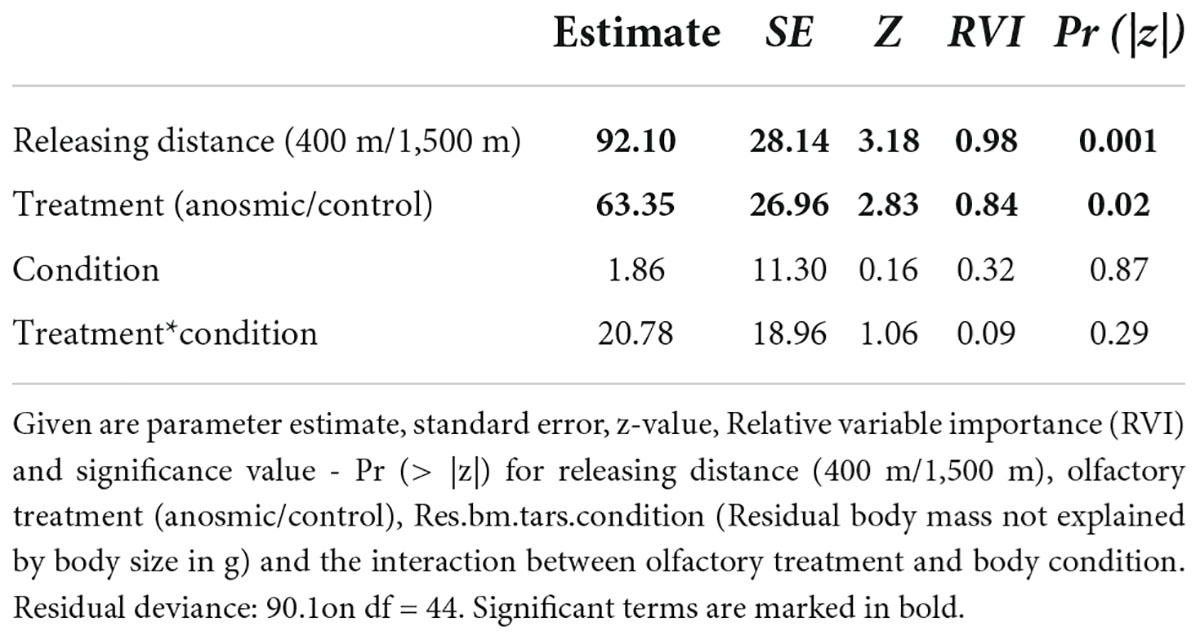
Table 2. Shown are the results of a generalized linear models (GLM) for return time as the dependent variable based on the model: glm(formula = return.time.h ∼ releasing distance + olfactory treatment *Residuals.bm.tars.condition, data = dat).
Discussion
In this study, we show that resident great tits memorize and follow olfactory cues, which might facilitate faster return rates to familiar feeding sites within their wintering territories. As predicted, our relocation experiment demonstrated that experimentally olfaction-deprived great tits required more time to relocate to winter-feeding sites than individuals with unaltered olfactory capacities. In addition, releasing distance (400 m/1,500 m) had an effect on the return time, with individuals released at shorter distance returning faster. Both, birds with full olfactory capacities and anosmic individuals, required more time to return when they were released at greater distance. In addition to the greater distance to be overcome, other factors might affect the prolonged return time, these include age (e.g., yearling or adult), experience and/or familiarity (e.g., breeding or non-breeding birds) with the area. However, there is no indication for a change in the importance of the olfactory capacity with distance, as the interaction between distance and treatment was not significant. Moreover, the difference in the return time between treatments is similar for 400 m and 1,500 m releasing distance (Figure 1), which indicates that overall, olfaction-deprived birds take longer to find the feeding site. The home range size of a species might also be a crucial factor influencing the use - and determining the importance of olfactory cues in homing. Investigating the relationship between releasing distance, return time and olfaction in more detail (e.g., by adding more releasing sites) might bring additional insights.
In line with our predictions regarding the total return rates (i.e., recoveries at the feeding sites), we did not find differences between the treatment groups, or with respect to the different releasing distances. Other passerines, like starlings (Sturnus vulgaris) were impaired in homing depending on the displacement distance (Wallraff and Hund, 1982; Wallraff et al., 1995). The return rates of starlings, for example, were not affected by an olfaction- depriving treatment when they were displaced by 30–60 km (Wallraff et al., 1995). We didn’t expect to find such strong treatment effects on the return rate when displacing great tits by 400 m or 1,500 m, because the birds were not removed from their home range, but remained at the outer boarder of their wintering territories. Our results suggest that measuring return rates depending on the olfactory capacity seems to be of importance over long, but not short distances. On the contrary, return time seems to be a very sensitive measure to detect and evaluate the role of olfaction in orientation even at very short distances. Even more so as in our experiments, the birds were not fitted with tracking devices and information about their exact movements is not available (Holland et al., 2009; Kishkinev et al., 2019). In respect to the short duration of our experiment and the small spatial scale, tracking devices might have provided little information, in particular considering their potential impact on individual behavior (McMahon et al., 2011; Arlt et al., 2013). However, the variable “return time” appears as a good proxy for an increase in movements arising through orientation-related behavior (Pollonara et al., 2015).
It has previously been suggested that within a small spatial scale the relevance and efficiency of smell for navigation decreases, because local environmental odors are too homogenous, and vision persists as predominant sense. Hence, the question arises whether great tits use scent cues for orientation and relocation to winter-feeding sites, or whether individuals simply follow an odorant track arising through the presence of food in the surroundings (Jacobs, 2012). We cannot out-rule this effect, but this explanation seems unlikely for several reasons. Species, which typically use olfactory cues to detect food over long distances, such as vultures, have large olfactory bulbs and complex nasal sinuses (Zelenitsky et al., 2011; Grigg et al., 2017). The small olfactory bulbs (Bang and Cobb, 1968) of passerines seem to have the capacity to detect subtle scents like plant volatiles and predator scents on the nest site (Amo et al., 2008, 2011, 2013; Mäntylä et al., 2017). There is also evidence for a role of olfactory cues in foraging over short distances (Amo et al., 2013; Zungu et al., 2014; Mäntylä et al., 2017; Hiltpold and Shriver, 2018), but it is not suggested to serve as a primary source to locate food (Krause et al., 2016; Mäntylä et al., 2018; Rubene et al., 2019). Furthermore, it is uncertain whether the olfactory cues provided by the feeding sites, consisting of dry sunflower seeds and fat balls, were sufficiently strong stimuli to allow distinct orientation toward feeders from distances of 400 m or 1,500 m, respectively. Sunflower seeds and fat balls are the most common food provided by people at the local winter-feeding sites (personal observation). Private winter-feeding also takes place in our study area. Even though no feeders were located between capture and release site, some feeders might have been provided in the backyards of houses in the proximity of the releasing sites. If food would be the major olfactory stimulus for individuals undergoing the experimental procedure, birds would follow an odorant track arising through the presence of these food sources and we would rarely expect them to return to the original feeder. Considering also that all birds were released at the same sites, the existence of additional feeders is unlikely to explain the differences we found between treatment groups. Thus, one possible explanation for our results is that, in addition to visual landmarks, great tits use olfactory cues for orientation within their home range, which allow them to increase the information they gain about their position. Contrary to pigeons and seabird species, great tits might use these chemical cues to gain additional information about the spatial conditions, which allows a faster position fixing even within a familiar environment. By removing this additional information, anosmic birds might require more time to orientate and relocate to the inner home range and consequently their familiar feeding sites.
In this experiment, only one third of the relocated individuals returned to the original site. We exclude possible effects of individual condition (body mass not explained by body size) on the return time and rate. This measure reflects the nutritional status of individuals and might affect the motivation to return to the feeding sites, but no relationship between body condition and return rate or return time became apparent. In addition, to control for potential direct adverse effects of the treatment, we conducted behavioral observations right after the release. We recorded perching height, number of perches or time until departing from the releasing site, but our results did not reveal differences between the treatment groups (Supplementary Table 1). One possible explanation for the seemingly low return rates could be related to the timing of the experiment. During late February, not only resident birds visit the feeders, but also straying individuals and birds migrating back to their northern breeding habitats (Cramp et al., 1993). These birds might be less likely to return to the feeding site but continue migration to their breeding habitats. However, wintering great tits show remarkably high site fidelity and display strong motivation to return to their winter territories and feeding sites after replacement, as was shown in a previous experiment by Krištín and Kaňuch (2017). They demonstrated that even individuals that were displaced 7.8 km away from their home ranges returned to feeding areas within 4 weeks after relocation. Their results (32.8% recapture) resemble our observed return rates and might represent the natural composition of sedentary and migratory individuals.
We cannot exclude that the treatment affected the birds’ motivation to approach the feeders, because they did not perceive the scent of the provided bird food. Interestingly, a recent study on Scopoli’s shearwaters (Calonectris diomedea) indicated effects of the zinc sulfate treatment (4% solution) on homing behavior but not foraging success (Padget et al., 2017). Whereas this species differs significantly from a small passerine these findings suggest that the treatment resulting in anosmia might affect orientation differently than actual foraging. However, we have not measured feeding behavior or foraging success in the target individuals. Considering though that con- and heterospecific individuals were present at the feeders (own observations) and that birds learn quickly to use artificial feeding sites by observing other individuals, we suggest that this effect might outrule a treatment effect on feeding behavior in this species. Our results also suggest that the majority of birds undergoing the zinc sulfate treatment returned after 4–10 days. There is indication that in other vertebrate species, the effect of the zinc-sulfate treatment diminishes after 2–7 days and birds arriving at the feeding sites might have restored their full olfactory capacity. However, being aware of a possible effect of the treatment on foraging behavior, we also recommend that future experiments should take into account for these effects by e.g., manipulating the odor and appearance of feeding sites.
Conclusion
Our results suggest that the use of chemical cues in addition to visual landmarks might help birds to maximize the information they receive about surrounding environments and optimize their movement in space. Specific olfactory cues in familiar environments might serve as reliable source of information to memorize landmarks and relocate to areas for specific needs (e.g., roosting sites, areas with high food abundance). In particular for species like great tits, which often remain in the breeding territories during winter, olfactory orientation and navigation might serve as an adaptive behavior, allowing individuals to optimize their foraging efficiency during periods of resource limitation (e.g., in winter). To the best of our knowledge, we are within the first to show that olfaction in songbirds might play a role in orientation at short-distance and within a familiar site in a natural setting. The results of our study strongly emphasize that olfaction might be of greater importance in the orientation of avian species than previously thought and contributes to the understanding of the functional contexts of smell in avian life.
Data Availability Statement
The original contributions presented in the study are included in the article/Supplementary Material, further inquiries can be directed to the corresponding author.
Ethics Statement
The animal study was reviewed and approved by the institutional ethics committee and the national authority according to §26 of the Law for Animal Experiments, “Tierversuchsgesetz 2012-TVG 2012.” The studies concern short time experiments without any surgery and no long-term effects on the birds were expected, confirmed by adequate monitoring after release (GZ 68.205/0034-WF/II/3b/2014).
Author Contributions
HH and KM designed the study. LN and KM conducted the experiment and the data collection. KM and HH wrote the manuscript with significant contributions from LN. FK performed statistical analyzes with contributions from HH. All authors have read and approved the manuscript.
Conflict of Interest
The authors declare that the research was conducted in the absence of any commercial or financial relationships that could be construed as a potential conflict of interest.
Publisher’s Note
All claims expressed in this article are solely those of the authors and do not necessarily represent those of their affiliated organizations, or those of the publisher, the editors and the reviewers. Any product that may be evaluated in this article, or claim that may be made by its manufacturer, is not guaranteed or endorsed by the publisher.
Acknowledgments
We thank B. Paces and N. Steurer for their help during data collection, special thanks also go to the teachers and students of the Sacré Coeur school in Pressbaum for hosting the experiment and maintaining the feeders. Furthermore, we are deeply grateful for the contributions of M. Mahr, who supported the research team by assisting in the field work, recruiting students and organizing the facilities. We are also very grateful to R. Hengsberger for copy-editing and assisting during the whole submission process.
Supplementary Material
The Supplementary Material for this article can be found online at: https://www.frontiersin.org/articles/10.3389/fevo.2022.858981/full#supplementary-material
References
Abreu, M. S., Giacomini, A. C. V. V., Rodriguez, R., Kalueff, A. V., and Barcellos, L. J. G. (2017). Effects of ZnSO4-induced peripheral anosmia on zebrafish behavior and physiology. Behav. Brain Res. 320, 275–281. doi: 10.1016/j.bbr.2016.12.014
Amo, L., Galvan, I., Tomas, G., and Sanz, J. J. (2008). Predator odour recognition and avoidance in a songbird. Funct. Ecol. 22, 289–293. doi: 10.1111/j.1365-2435.2007.01361.x
Amo, L., Visser, M. E., and Oers, K. V. (2011). Smelling out predators is innate in birds. Ardea 99, 177–184. doi: 10.5253/078.099.0207
Amo, L., Jansen, J. J., van Dam, N. M., Dicke, M., and Visser, M. E. (2013). Birds exploit herbivore-induced plant volatiles to locate herbivorous prey. Ecol. Lett. 16, 1348–1355. doi: 10.1111/ele.12177
Arlt, D., Low, M., and Part, T. (2013). Effect of Geolocators on Migration and Subsequent Breeding Performance of a Long-Distance Passerine Migrant. PLoS One 8:e82316. doi: 10.1371/journal.pone.0082316
Bang, B. G., and Cobb, S. (1968). The size of the olfactory bulb in 108 species of birds. Auk 85, 55–61. doi: 10.2307/4083624
Bates, D., Mächler, M., Bolker, B., and Walker, S. (2015). Fitting Linear Mixed-Effects Models Using lme4. J. Stat. Softw. 67, 1–48. doi: 10.18637/jss.v067.i01
Benvenuti, S., and Gagliardo, A. (1996). Homing behaviour of pigeons subjected to unilateral zinc sulphate treatment of their olfactory mucosa. J. Exp. Biol. 199, 2531–2535. doi: 10.1242/jeb.199.11.2531
Benvenuti, S., Ioalè, P., and Massa, B. (1993). Olfactory experiments on Cory’s shearwater (Calonectris diomedea): the effect of intranasal zinc sulphate treatment on short-range homing behaviour. Boll. Zool. 60, 207–210. doi: 10.1080/11250009309355811
Bonadonna, F., Bajzak, C., Benhamou, S., Igloi, K., Jouventin, P., Lipp, H. P., et al. (2005). Orientation in the wandering albatross: interfering with magnetic perception does not affect orientation performance. Proc. R. Soc. B 272, 489–495. doi: 10.1098/rspb.2004.2984
Bonadonna, F., Benhamou, S., and Jouventin, P. (2003). ““Orientation in “Featureless” Environments: the Extreme Case of Pelagic Birds,” in Avian Migration, eds P. Berthold, E. Gwinner, and E. Sonnenschein (Berlin: Springer-Verlag), 367–377.
Burd, G. D. (1993). Morphological study of the effects of intranasal zinc sulfate irrigation on the mouse olfactory epithelium and olfactory bulb. Microsc. Res. Techniq. 24, 195–213. doi: 10.1002/jemt.1070240302
Burnham, K. P., and Anderson, D. R. (2002). Model Selection and Multimodel Inference. A Practical Information-Theoretic Approach, 2nd Edn. New York, NY: Springer.
Cancalon, P. (1982). Degeneration and regeneration of olfactory cells induced by ZnSO4 and other chemicals. Tissue Cell 14, 717–733. doi: 10.1016/0040-8166(82)90061-1
Caspers, B. A., and Krause, E. T. (2011). Odour-based natal nest recognition in the zebra finch (Taeniopygia guttata), a colony-breeding songbird. Biol. Lett. 7, 184–186. doi: 10.1098/rsbl.2010.0775
Catania, K. C. (2006). Underwater ‘sniffing’ by semi-aquatic mammals. Nature 444, 1024–1025. doi: 10.1038/4441024a
Cramp, S., Perrins, C. M., and Brooks, D. J. (eds) (1993). Handbook of the Birds of Europe, the Middle East and North Africa: The Birds of the Western Palearctic. Oxford: Oxford University Press.
Dahmani, L., Patel, R. M., Yang, Y., Chakravarty, M. M., Fellows, L. K., and Bohbot, V. D. (2018). An intrinsic association between olfactory identification and spatial memory in humans. Nat. Commun. 9:4162. doi: 10.1038/s41467-018-06569-4
De Laet, J. (1984). Site-related dominance in the Great Tit Parus major major. Ornis Scand. 15, 73–78. doi: 10.2307/3675940
Dukes, J. P., Deaville, R., Bruford, M. W., Youngson, A. F., and Jordan, W. C. (2004). Odorant receptor gene expression changes during the parr-smolt transformation in Atlantic salmon. Mol. Ecol. 13, 2851–2857. doi: 10.1111/j.1365-294X.2004.02252.x
Fiaschi, V., Farina, A., and Ioalé, P. (1974). Homing experiments on swifts Apus apus (L.) deprived of olfactory perception. Monit Zool. Ital. 8, 235–244. doi: 10.1080/00269786.1974.10736238
Gagliardo, A. (2013). Forty years of olfactory navigation in birds. J. Exp. Biol. 216, 2165–2171. doi: 10.1242/jeb.070250
Grigg, N. P., Krilow, J. M., Gutierrez-Ibanez, C., Wylie, D. R., Graves, G. R., and Iwaniuk, A. N. (2017). Anatomical evidence for scent guided foraging in the turkey vulture. Sci. Rep. 7:17408. doi: 10.1038/s41598-017-17794-0
Hiltpold, I., and Shriver, W. G. (2018). Birds Bug on Indirect Plant Defenses to Locate Insect Prey. J. Chem. Ecol. 44, 576–579. doi: 10.1007/s10886-018-0962-0
Holland, R. A., Thorup, K., Gagliardo, A., Bisson, I. A., Knecht, E., Mizrahi, D., et al. (2009). Testing the role of sensory systems in the migratory heading of a songbird. J. Exp. Biol. 212, 4065–4071. doi: 10.1242/jeb.034504
Jacobs, L. F. (2012). From chemotaxis to the cognitive map: the function of olfaction. Proc. Natl. Acad. Sci. USA 109, 10693–10700. doi: 10.1073/pnas.1201880109
Jacobs, L. F., Arter, J., Cook, A., and Sulloway, F. J. (2015). Olfactory Orientation and Navigation in Humans. PLoS One 10:e0129387. doi: 10.1371/journal.pone.0129387
John, R. (2010). [Book review] Birds of Europe: Second Edition by Lars Svensson. 2010. Can. Field-Nat. 124, 61–62. doi: 10.22621/cfn.v124i1.1035
Kishkinev, D., Anashina, A., Ishchenko, I., and Holland, R. A. (2019). Anosmic migrating songbirds demonstrate a compensatory response following long-distance translocation: a radio-tracking study. J. Ornithol. 2019:1698. doi: 10.1007/s10336-019-01698-z
Krams, I., Krama, T., and Igaune, K. (2006). Alarm calls of wintering great tits Parus major: warning, of mate, reciprocal altruism or a message to the predator? J. Avian. Biol. 37, 131–136. doi: 10.1111/j.0908-8857.2006.03632.x
Krause, E. T., Kabbert, J., and Caspers, B. A. (2016). “Exploring the Use of Olfactory Cues in a Nonsocial Context in Zebra Finches (Taeniopygia guttata),” in Chemical Signals in Vertebrates 13, eds B. A. Schulte, T. E. Goodwin, and M. H. Ferkin (Cham: Springer International Publishing), 177–187.
Krištín, A., and Kaňuch, P. (2017). Stay or go? Strong winter feeding site fidelity in small woodland passerines revealed by a homing experiment. J. Ornithol. 158, 53–61. doi: 10.1007/s10336-016-1362-2
Lemel, J. (1989). Habitat Distribution in the Great Tit Parus major in Relation to Reproductive Success, Dominance, and Biometry. Ornis Scand. 20, 226–233. doi: 10.2307/3676917
Mahr, K., Griggio, M., Granatiero, M., and Hoi, H. (2012). Female attractiveness affects paternal investment: experimental evidence for male differential allocation in blue tits. Front. Zool. 9:14. doi: 10.1186/1742-9994-9-14
Mahr, K., Riegler, G., and Hoi, H. (2015). Parental risk management in relation to offspring defence: bad news for kids. Proc. R. Soc. Lond. B 282:20141670. doi: 10.1098/rspb.2014.1670
Mahr, K., Seifert, C. L., and Hoi, H. (2016). Female and male Blue Tits (Cyanistes caeruleus) sing in response to experimental predator exposition. J. Ornithol. 157, 907–911. doi: 10.1007/s10336-016-1345-3
Mäntylä, E., Kipper, S., and Hilker, M. (2020). Insectivorous birds can see and smell systemically herbivore-induced pines. Ecol. Evol. 10, 9358–9370. doi: 10.1002/ece3.6622
Mäntylä, E., Kleier, S., Kipper, S., and Hilker, M. (2017). The attraction of insectivorous tit species to herbivore-damaged Scots pines. J. Ornithol. 158, 479–491. doi: 10.1007/s10336-016-1412-9
Mäntylä, E., Kleier, S., Lindstedt, C., Kipper, S., and Hilker, M. (2018). Insectivorous Birds Are Attracted by Plant Traits Induced by Insect Egg Deposition. J. Chem. Ecol. 44, 1127–1138. doi: 10.1007/s10886-018-1034-1
McBride, K., Slotnick, B., and Margolis, F. L. (2003). Does Intranasal Application of Zinc Sulfate Produce Anosmia in the Mouse? An Olfactometric and Anatomical Study. Chem. Sens. 28, 659–670. doi: 10.1093/chemse/bjg053
McGregor, J. H., and Teska, W. R. (1989). Olfaction as an orientation mechanism in migrating Ambystoma maculatum. Copeia 1989, 779–781. doi: 10.2307/1445516
McMahon, C. R., Collier, N., Northfield, J. K., and Glen, F. (2011). Taking the time to assess the effects of remote sensing and tracking devices on animals. Anim. Welfare 20, 515–521. doi: 10.1371/journal.pone.0255586
Nakamura, M., and Shindo, N. (2001). Effects of snow cover on the social and foraging behavior of the great tit Parus major. Ecol. Res. 16, 301–308. doi: 10.1046/j.1440-1703.2001.00397.x
Nevitt, G. (2006). Olfactory foraging strategies of procellariiform seabirds. Acta Zool. Sin. 52, 510–513.
Nevitt, G. (2008). Sensory ecology on the high seas: the odor world of the procellariiform seabirds. J. Exp. Biol. 211, 1706–1713. doi: 10.1242/jeb.015412
Padget, O., Dell’Ariccia, G., Gagliardo, A., González-Solís, J., and Guilford, T. (2017). Anosmia impairs homing orientation but not foraging behaviour in free-ranging shearwaters. Sci. Rep. 7:9668. doi: 10.1038/s41598-017-09738-5
Papi, F., Fiore, L., Fiaschi, V., and Benvenuti, S. (1972). Olfaction and homing in pigeons. Monit. Zool. Ital. 6, 85–95. doi: 10.1080/00269786.1972.10736187
Pollonara, E., Luschi, P., Guilford, T., Wikelski, M., Bonadonna, F., and Gagliardo, A. (2015). Olfaction and topography, but not magnetic cues, control navigation in a pelagic seabird: displacements with shearwaters in the Mediterranean Sea. Sci. Rep. 5:16486. doi: 10.1038/srep16486
R Core Team (2016). R: A language and environment for statistical computing. R Foundation for Statistical Computing. Vienna: R Foundation for Statistical Computing.
Rastogi, A., Kumari, Y., Rani, S., and Kumar, V. (2011). Phase inversion of neural activity in the olfactory and visual systems of a night-migratory bird during migration. Eur. J. Neurosci. 34, 99–109. doi: 10.1111/j.1460-9568.2011.07737.x
Rastogi, A., Surbhi Malik, S., Rani, S., and Kumar, V. (2016). Annual life-history dependent seasonal differences in neural activity of the olfactory system between non-migratory and migratory songbirds. Behav. Brain Res. 296, 233–239.
Reinhard, J., Srinivasan, M. V., and Zhang, S. (2004). Scent-triggered navigation in honeybees. Nature 427, 411–411. doi: 10.1038/427411a
Reynolds, A. M., Cecere, J. G., Paiva, V. H., Ramos, J. A., and Focardi, S. (2015). Pelagic seabird flight patterns are consistent with a reliance on olfactory maps for oceanic navigation. Proc. R. Soc. B 282, 20150468. doi: 10.1098/rspb.2015.0468
Rubene, D., Leidefors, M., Ninkovic, V., Eggers, S., and Low, M. (2019). Disentangling olfactory and visual information used by field foraging birds. Ecol. Evol. 9, 545–552. doi: 10.1002/ece3.4773
Schlund, W. (1992). Intra-nasal zinc sulphate irrigation in pigeons: effects on olfactory capabilities and homing. J. Exp. Biol. 164, 171–187.
Slotnick, B. M., and Gutman, L. A. (1977). Evaluation of Intranasal Zinc Sulfate Treatment on Olfactory Discrimination in Rats. J. Comp. Physiol. Psychol. 91, 942–950. doi: 10.1037/h0077370
Southwood, A., and Avens, L. (2010). Physiological, behavioral, and ecological aspects of migration in reptiles. J. Comp. Physiol. B 180, 1–23. doi: 10.1007/s00360-009-0415-8
Stabell, O. B. (1984). Homing and olfaction in salmonids: a critical review with special reference to the atlantic salmon. Biol. Rev. 59, 333–388. doi: 10.1111/j.1469-185X.1984.tb00709.x
Steiger, S. S., Fidler, A. E., Valcu, M., and Kempenaers, B. (2008). Avian olfactory receptor gene repertoires: evidence for a well-developed sense of smell in birds? Proc. R. Soc. Lond. B 275, 2309–2317. doi: 10.1098/rspb.2008.0607
van Balen, J. H., and Hage, F. (1989). The Effect of Environmental Factors on Tit Movements. Ornis Scand. 20, 99–104. doi: 10.2307/3676875
Venables, W. N., and Ripley, B. D. (2002). Modern Applied Statistics with S, 4th Edn. New York, NY: Springer.
Waldvogel, J. A. (1989). “Olfactory orientation by birds,” in Current Ornithology, ed. M. Power Dennis (New-York: Plenum Press), 269–321.
Wallraff, H. G. (2003). Zur olfaktorischen Navigation der Vögel. J. Ornithol. 144, 1–32. doi: 10.1007/BF02465514
Wallraff, H. G. (2004). Avian olfactory navigation: its empirical foundation and conceptual state. Anim. Behav. 67, 189–204. doi: 10.1016/j.anbehav.2003.06.007
Wallraff, H. G., and Hund, K. (1982). “Homing Experiments with Starlings (Sturnus vulgaris) Subjected to Olfactory Nerve Section,” in Avian Navigation, eds F. Papi and H. G. Wallraff (Berlin: Springer), 313–318.
Wallraff, H. G., Kiepenheuer, J., Neumann, M. F., and Streng, A. (1995). Homing experiments with starlings deprived of the sense of smell. Condor 97, 20–26. doi: 10.2307/1368979
Wallraff, H. G., Kiepenheuer, J., and Streng, A. (1994). The Role of Visual Familiarity with the Landscape in Pigeon Homing. Ethology 97, 1–25. doi: 10.1111/j.1439-0310.1994.tb01025.x
Wilkin, T. A., Garant, D., Gosler, A. G., and Sheldon, B. C. (2006). Density effects on life-history traits in a wild population of the great tit Parus major: analyses of long-term data with GIS techniques. J. Anim. Ecol. 75, 604–615.
Wood, S. N. (2017). Generalized Additive Models: An Introduction with R. 2nd ed. Boca Raton: CRC Press, Chapman & Hall.
Zelenitsky, D. K., Therrien, F., Ridgely, R. C., McGee, A. R., and Witmer, L. M. (2011). Evolution of olfaction in non-avian theropod dinosaurs and birds. Proc. R. Soc. B 278, 3625–3634. doi: 10.1098/rspb.2011.0238
Zungu, M. M., Hart, L. A., and Downs, C. T. (2014). “The Use of Taste and Smell in Making Foraging Decisions in Frugivorous Birds: An Experimental Test Using Red-Winged Starlings Onychognathus morio,” in Aspects of Fruit Digestion and Selection in Selected Southern African Avian Frugivores, ed. M. M. Zungu (KwaZulu-Natal: University of KwaZulu-Nata), 131–159.
Keywords: olfaction, navigation, homing, passerines, zinc sulfate
Citation: Mahr K, Nowack L, Knauer F and Hoi H (2022) Songbirds use scent cues to relocate to feeding sites after displacement: An experiment in great tits (Parus major). Front. Ecol. Evol. 10:858981. doi: 10.3389/fevo.2022.858981
Received: 20 January 2022; Accepted: 28 June 2022;
Published: 18 July 2022.
Edited by:
Magdalena Ruiz Rodriguez, University of Granada, SpainReviewed by:
Sang-im Lee, Daegu Gyeongbuk Institute of Science and Technology, South KoreaGustavo Tomás, Experimental Station of Arid Zones (CSIC), Spain
Paulo Milet Pinheiro, Federal Rural University of Pernambuco, Brazil
Darren Proppe, St. Edward’s University, United States
Copyright © 2022 Mahr, Nowack, Knauer and Hoi. This is an open-access article distributed under the terms of the Creative Commons Attribution License (CC BY). The use, distribution or reproduction in other forums is permitted, provided the original author(s) and the copyright owner(s) are credited and that the original publication in this journal is cited, in accordance with accepted academic practice. No use, distribution or reproduction is permitted which does not comply with these terms.
*Correspondence: Katharina Mahr, S2F0aGFyaW5hLm1haHJAdmV0bWVkdW5pLmFjLmF0; Herbert Hoi, SGVyYmVydC5ob2lAdmV0bWVkdW5pLmFjLmF0
†These authors have contributed equally to this work