- 1Department of Biology, Texas A&M University, College Station, TX, United States
- 2Institute for Neuroscience, Texas A&M University, College Station, TX, United States
Echolocating bats are able to discriminate between different surface textures based on the spectral properties of returning echoes. This capability is likely to be important for recognizing prey and for finding suitably perching sites along smooth cave walls. Previous studies showed that bats may exploit echo spectral interference patterns in returning echoes to classify surface textures, but a systematic assessment of the limits of their discrimination performance is lacking and may provide important clues about the neural mechanisms by which bats reconstruct target features based on echo acoustic cues. We trained three Mexican free-tailed bats (Tadarida brasiliensis) on a Y-maze to discriminate between the surfaces of 10 different sheets of aluminum-oxide abrasive sandpapers differing in standardized grit sizes ranging from 40 grit (coarse, 425 μm mean particle diameter) to 240 grit (fine, 54 μm mean particle diameter). Bats were rewarded for choosing the coarsest of two choices. All three bats easily discriminated all abrasive surfaces from a smooth plexiglass control and between all sandpaper comparisons except the two with the smallest absolute difference in mean particle sizes, the 150 vs. 180 grit (92 vs. 82 μm) and the 220 vs. 240 grit (68 vs. 54 μm) surfaces. These results indicate that echolocating free-tailed bats can use slight variations in the echo spectral envelope to remotely classify very fine surface textures with an acuity of at least 23 μm, which rivals direct tactile discrimination performance of the human hand.
Introduction
Echolocating bats and cetaceans not only detect and localize objects in their environment but are also able to classify shapes and textures based on the acoustic cues embedded in returning echoes (Accomando et al., 2020; Ming et al., 2021). The biosonar discriminatory capabilities of these animals supersede those of man-made sonar systems in their capacity to recognize shapes and classify different types of insects or fish from different angles or under noisy conditions, prompting questions about both the acoustic nature of information in the echoes and how their auditory system analyzes echoes to achieve such high performance in real time. Previous research revealed that bats that emit downward frequency-modulated (FM-type) pulses can exploit amplitude modulations in the echo spectral envelope to evaluate variations in surface depth (Simmons et al., 1974; Habersetzer and Vogler, 1983; Schmidt, 1988; Simon et al., 2014). These results led to the hypothesis that information about surface shapes and textures is captured by spectral interference patterns emerging in the reflected echoes. Those experiments were designed to delineate the relative contributions of spectral vs. temporal cues used by bats to reconstruct target shapes and textures, but did not systematically explore the bats’ resolution limits. Echoes reflected off of rough or irregularly shaped natural objects are chaotic and may not directly reflect any specific shape or texture (Grunwald et al., 2004), but bats typically inspect targets with many pulses from multiple angles and may thereby collect sufficient information to assemble a statistical representation of a target’s general physical features. Thus, naturally behaving bats may possess higher spatial resolving powers than previously reported owing to their abilities to integrate information from multiple echoes over time and space.
Free-tailed bats are a cave and crevice-dwelling species that is commonly found roosting in a variety of artificial stone and concrete structures, such as in bridges, stadiums and parking garages. To land on the ceiling of a cave or on a concrete wall, these bats must first inspect the surface in search of places where their toenails can reliably take hold. They are able to detect the presence of fine cracks, small protrusions and other surface imperfections that provide sufficient substrate for perching. Adult free-tailed bats, which weigh 10–12 g, cannot land on a very smooth surface such as painted concrete, but they can discriminate between smooth and slightly rougher surfaces, and demonstrate a preference for landing on rougher surfaces for perching. Thus, texture discrimination may be important to the behavioral ecology of this species because it reduces the time spent searching for suitable perches, and current evidence suggests that FM-type bats would do so by evaluating the spectral features of returning echoes. This led us to hypothesize that free-tailed bats may be particularly well-adapted to use biosonar to evaluate surface textures, and that conducting a behavioral assay of surface texture discrimination in this species in parallel with ongoing neurophysiological studies of their auditory system (Macias et al., 2020a,b, 2022) might lead to new insights into the acoustic and neurophysiological basis of biosonar texture discrimination (Ming et al., 2021).
When broadband echolocation pulses reflect off of multiple closely spaced surfaces, the overlapping echoes convolve to produce a single echo comprised of spectral interference notches (Simmons et al., 1974). By using artificially generated echo mimics, it was confirmed that these spectral cues were used by bats to infer surface textures (Schmidt, 1988) and may be used in combination with temporal cues and amplitude modulations to infer many details about an ensonified object’s shape (Simmons et al., 1990). A behavioral assay conducted with Myotis myotis found flying bats could discriminate between plates with holes drilled in them that differed in depth by as little as 600 μm, with anecdotal evidence that their resolution might conceivably extend to as little as 30 μm (Habersetzer and Vogler, 1983). A more recent study with echolocating nectar feeding bats (Glossophaga soricina) reported structural resolution limits of at least 380 μm, and further confirmed that the bats’ discriminatory capabilities were dependent upon the spectral quality of the echoes (Simon et al., 2014). Here, we extend those observations by providing a comprehensive assessment of the texture resolving powers of an FM-type bat that routinely evaluates surface textures when searching for a place to perch. Free-tailed bats (Tadarida brasiliensis) were trained to discriminate between different commercially available aluminum oxide sandpapers spanning a range of very rough to very fine textures. Commercial sandpapers are categorized by grit number, which is directly related to the mean diameter of particles adhered to their surface. The results showed that free-tailed bats were able to consistently discriminate between surface textures differing by as little as 23 μm in mean particle diameter, but failed when the differences in particle size were smaller than this.
Materials and methods
Acoustic measurements
To characterize the acoustic properties of the echoes reflected off of the different sandpaper grits and confirm that they generated echoes with distinctive acoustic features, we recreated the experimental setting in an anechoic chamber and placed the different targets 50 cm in front of an ultrasonic loudspeaker positioned below a Brüel & Kjaer type 4139 1/4” microphone and recorded the echoes generated by artificial frequency-modulated (FM) sweeps. Pulse-echo pairs were digitized at a 250 kHz sampling rate using a National Instrument card (NI USB-6356, National Instruments Co.) and recorded and analyzed with custom-made software in MatLab (R2018a, MathWorks). The artificial FM pulses swept downwards from 60 to 20 kHz over a 5 ms period and were delivered at an intensity of 80 dB re 20 μPa, digitally compensated to produce a flat spectrum throughout the sweep by correcting for the frequency response of the playback system. Note that the electronic loudspeaker system could not replicate the full multi-harmonic bandwidth of the bats natural pulse bandwidths because they extended up to more than 100 kHz. Our rationale for using a single harmonic artificial FM sweep was to confirm that broadband FM echoes reflected off of the different sandpaper grits reliably produced distinctive spectral cues available to the bats while performing the discrimination assay. We slightly extended the upper frequency range of our test pulses to 60 kHz to enhance the resolution of spectral notch patterns such as those likely to be available to the bats. The bats’ own pulses have a fundamental harmonic that drops from roughly 50 to 20 kHz, a prominent second harmonic that drops from 100 to 40 kHz, and sometimes a third harmonic is evident (Figure 1C). Consequently, their natural pulses would produce slightly different echo modulation patterns than those derived with the synthetic pulses. The free-tailed bat auditory system is distinguished by possessing a prominent auditory fovea, or overrepresented frequency range of 20–30 kHz and an overall sensitivity range of about 12–80 kHz. The auditory fovea begins with biomechanical specializations in the cochlea (Vater and Siefer, 1995), continues through the inferior colliculus (Pollak et al., 1978) and extends to the auditory cortex (Macias et al., 2020b), and is believed to provide greater sensitivity for detecting prey at longer ranges and higher speeds. Thus, while their pulses extend above 100 kHz, neurophysiological results indicate that their auditory system is mostly constrained to a bandwidth of 20–80 kHz and especially sensitive to the first harmonic of the emitted pulses.
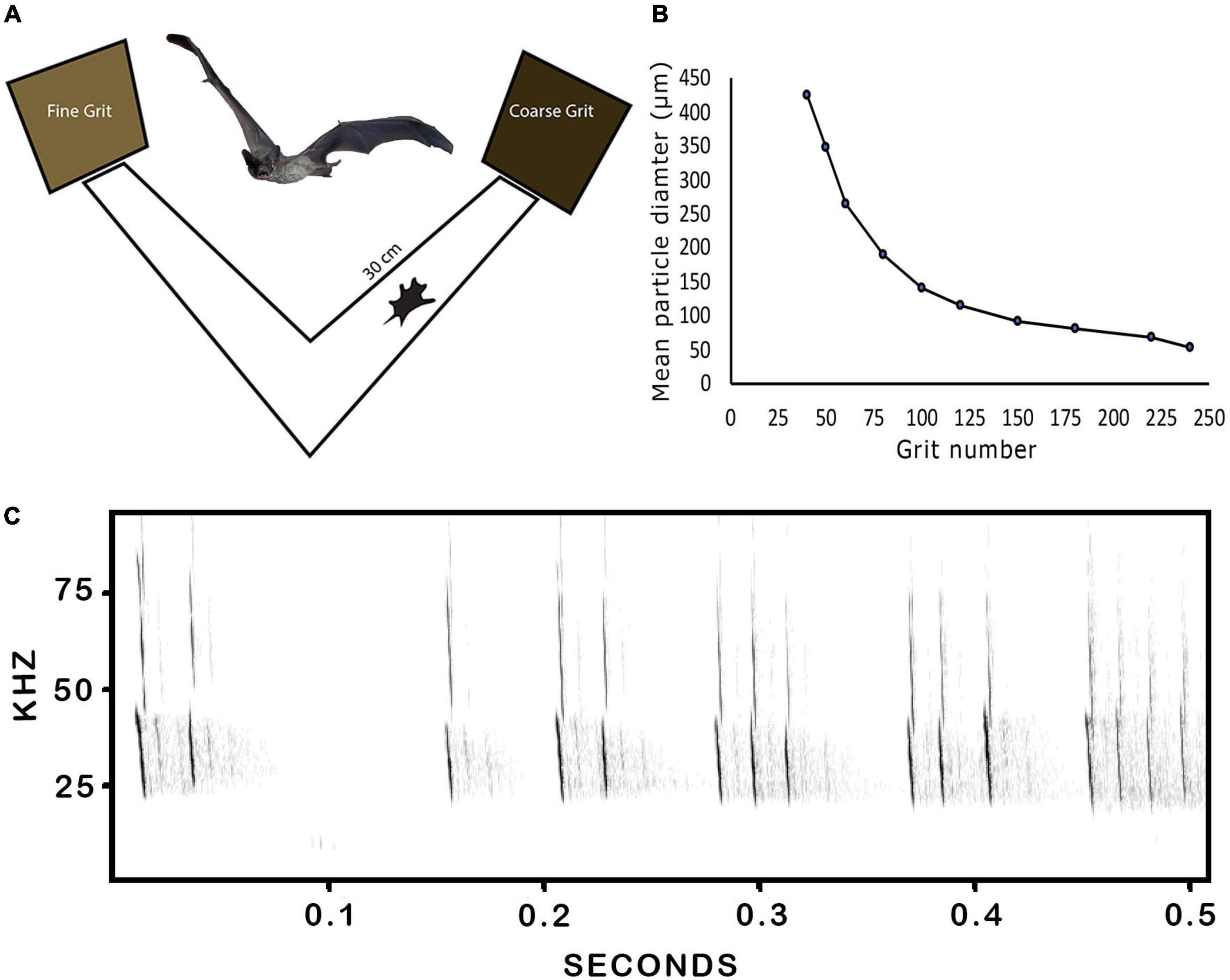
Figure 1. (A) Schematic diagram of the y-maze apparatus with cartoon free-tailed bat drawn to scale. A photograph of a flying free-tailed bat is included for reference purposes. (B) Plot of the relationship between different sandpaper grit numbers and their corresponding mean particle diameters. (C) Spectrogram of a representative echolocation pulse sequence emitted by a bat on the maze as it surveyed the targets. Two prominent harmonics and the tail-end of a higher third harmonic are visible, plus echo wave fronts reflected off the platform toward the microphone positioned 1 m in front of maze and facing toward the bat.
Behavioral experiments
The animals used in these experiments were 3 wild-caught adult Mexican free-tailed bats (Tadarida brasiliensis, 1 male and 2 female) housed socially in an artificial bat vivarium. The bats were allowed opportunities to fly daily and had access to water ad libitum. Husbandry and experimental procedures were approved by the Texas A&M institutional animal care and use committee and complied with all N.I.H. guidelines for the care and use of animals. All training and experiments were conducted in a dimly lit, adjacent flight room at 25°C. It was necessary to carry out the training and experimental trials under low light conditions rather than complete darkness for two reasons: first, during the training phase bats often flew off the perch and had to be retrieved by the handlers, and secondly, because the reward (live mealworm) was delivered by hand with forceps immediately after the bats made a choice, and this was difficult to do in complete darkness. The bats were trained in the two-alternative forced choice procedure on an elevated y-maze in which they received a food reward for evaluating, approaching and touching the coarser of two target surfaces positioned at the ends of the two maze arms. The y-shaped experimental platform was mounted on a tripod 90 cm above the floor. The two arms were padded with a soft synthetic material that was easy for the bats to grasp and move on, and both arms were 50 cm long and oriented away from each other at a 90-degree angle (Figure 1A). The targets were 8″× 10″ (20 cm × 25 cm) sheets of aluminum oxide abrasive sandpaper (3M, St. Paul MN) adhered to 8″× 10″ flat plexiglass boards. The experimenter stood behind the platform and wore a GoPro HERO8 on the forehead and tilted downwards to record trials, and all data were tabulated by a second observer reviewing the videos. In a subset of trials an ultrasonic microphone (UltraSoundGate 116 with CM16 polarized condenser microphone, Avisoft Bioacoustics, Berlin) was positioned 1 meter in front of the y-maze to assess the number and pattern of biosonar pulses emitted by the bats as they evaluated targets and made their selections. We hypothesized that pulse emission rates or total numbers might increase when the bats were challenged with more difficult discrimination tasks, and therefore recorded pulse emissions of all three bats on an easy task (80-grit vs. 180-grit) and a hard task (150-grit vs. 180-grit). From these data sets we calculated the mean ± SD pulse rates for the 2 s preceding the bats decision. Pulses were recorded at 16-bit, 196 kHz sample rate and recordings were analyzed using Avisoft SASLab Pro.
The bats were first trained to demonstrate a preference for rough vs. smooth surfaces by exploiting their innate preference to climb onto vertical surfaces. When naive bats were given a choice between a smooth Plexiglass target and a medium (60 grit) sandpaper target that they could easily mount and climb, they sought out and mounted the rougher surface. We reinforced this choice with a mealworm food reward each time they mounted the rough surface. Remarkably, within 3 days of training, each bat exclusively chose the 60-grit sandpaper over the plexiglass surface, running toward the sandpaper immediately after being placed at the maze center. After eating their reward, the experimenter returned the bat to the maze center to initiate another trial. Position of the coarser target (S +) was alternated with the smoother target (S-) in an irregular (pseudorandom) pattern between trials within sets, and the sequence changed daily. During training, the trials continued until each bat received 15 mealworms per day. For every trial, choice was defined as whichever target the bat made physical contact with first, typically by dragging a thumb nail across the surface before attempting to climb onto it. In some instances, bats approached a target, inspected if from close range (∼10 cm), and then returned to the alternate maze arm and inspected the other target. On more difficult grit comparisons, bats sometimes went back and forth between targets multiple times before choosing. The time to decide was not measured, but was rarely longer than 10 s. On occasions where the bat chose incorrectly (S-), it was removed from the target, held in a gloved palm for 60 s, which routinely evoked protest vocalizations, and then returned to the center of the maze for a new trial. Each experimental set consisted of two or three warm-ups, then 15 consecutive video-recorded trials, and additional unmarked trials as needed to ensure each bat always received 15 worms per day regardless of performance.
Once trained, we proceeded to change the sandpaper targets (S + and S-) to include a range of surface textures varying from 40 to 240 grit (Figure 1B). First, the full range of 10 sandpaper grits were tested three times in random order against plexiglass, which required 30 days and produced a strongly reinforced behavior. Following completion of the plexiglass control sets, the bats were subsequently challenged to discriminate between two targets differing in sandpaper grit size. We transitioned to the second phase by first presenting them with a choice between a very fine grit vs. very coarse grit. After confirming that the bats extended the learned preference to choosing the coarsest of the two abrasive targets, the sequence of tests were pseudo-randomly arranged to complete a 9 × 9 matrix comparing each grit vs. the other eight grits and one flat surface (smooth plexiglass). This resulted in 36 different comparisons, 8 where the bats were tested with sandpapers vs. flat, and 28 in which they were tested with two different sandpaper grits. Each challenge was repeated three times each for a total of 108 sets per bat. Testing was conducted every day for approximately 4 months. The bats were challenged with a single challenge (one pair of surfaces) of 15 trials per day. Each possible combination of target comparisons were tested on three different days to produce a total of 45 trials per comparison per bat. At the midpoint and after the conclusion of the study, we challenged the bats with a series of three control sets wherein the bats were presented with identical targets, and confirmed that in each case their performance dropped to chance (50%). Statistical analyses were performed by summing each bats performance across all 45 trials collected over 3 days for each comparison and calculating the percentage of times the bat chose S +. Following similar previous studies (Habersetzer and Vogler, 1983; Lamb, 1983; Schmidt, 1988; Simmons et al., 1998) we analyzed the results of the two-alternative forced choice test using exact binomial tests with 50% expectations and considered the classical limen threshold of 75% as indicative of performing better than chance.
Controls for the contribution of vision
Different sandpaper grits vary slightly in their shade of color, with coarse grits being of a darker brown shade and finer grits becoming progressively lighter in shade. Consequently, it might have been possible for the bats to use vision to aid in their discrimination. To assess the contributions of vision we conducted two different control experiments: First, we tested all three bats on the 80-grit vs. 180-grit challenge in complete darkness, except that the handler wore a red LED headlamp and was instructed to aim the headlamp downward while the bats scanned the targets. We chose the 80-grit vs. 180-grit challenge because all three bats performed well on this discrimination task and because these two targets represented two very different shades of brown. In the second experiment, we repeated the 80-grit vs. 180-grit trials in dim light, but with both targets behind a clear plexiglass sheet; if the bats choices were biased toward the darker shaded sandpapers, then they would be predicted to show a preference for the 80-grit paper despite there being no difference in echo acoustic features.
Results
Acoustic properties of echoes reflected off of sandpapers
We collected and averaged the power spectra from 25 echoes for each sandpaper grit size. Representative recordings of echoes reflected off of the different sandpaper grits are provided in Figure 2, with oscillograms (left column), spectrograms (middle) and power spectra (right column) of the echoes. The electronically generated pulses are visible in the oscillograms and spectrograms, appearing with distortions at lower amplitudes than the subsequent echoes owing to directional effects of the loudspeaker and microphone. Different sandpaper grit sizes reliable produced different overall spectral envelopes, and several included distinctive spectral notch patterns. These results confirm that the different grits produced different echo acoustic cues that were available to the bats during these experiments. Repeating our echo acoustic measurements with three different samples of the same sandpaper grit produced consistent results. Our echo measurements showed that the range of particle diameters used here were too small to produce a detectable change in echo amplitudes when measured head on (normal incidence). However, changing the angle of incidence by 15 degrees, which corresponded to what the bats could achieve with head scans, attenuated the echo by up to 12 dB and significantly altered the spectral envelope in complex, non-linear ways that differed for each sandpaper grit size. Coarser sandpapers showed slightly less peak attenuation with changing angle but more dramatic manipulations in spectral envelope shape. We documented but did not try to quantify or model the effects of incident angle on echo acoustic properties because this would have required more detailed information about surface microstructures than was available. Bats scanning the targets with multiple pulses from half-way down one arm of the maze would have been able to alter the incident angle of their emissions on the target surface by ± 15 degrees, so it must be assumed that the bats collected an array of different echo patterns from each target sandpaper and therefore had much more information at their disposal than what is shown in Figure 2. Since the bats physically interacted with the different sandpaper surfaces while learning the task, it is possible that they learned to correlate different echo collages with somatosensory experiences gained from touching and climbing on the targets.
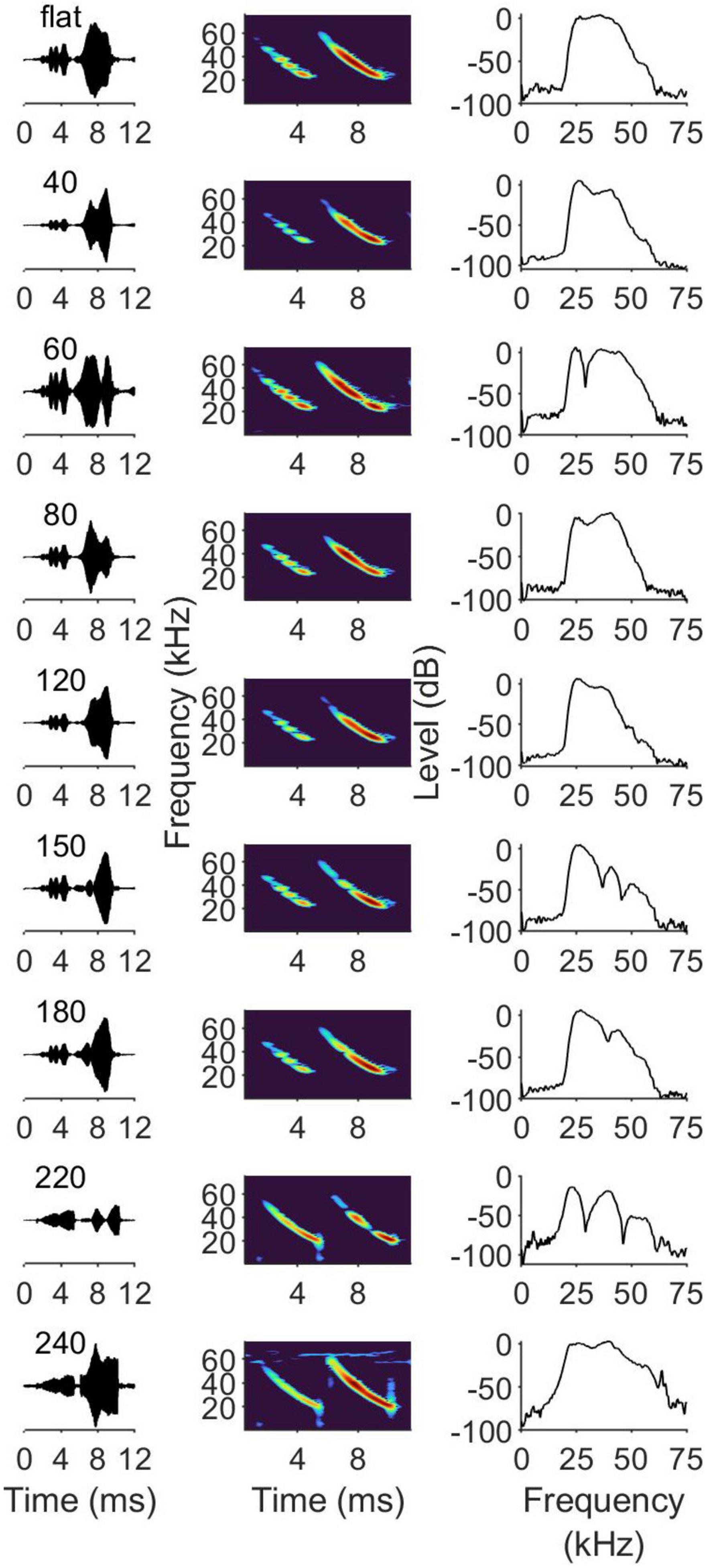
Figure 2. (Left column) Oscillogram of outgoing synthetic pulse followed by returning echo reflected off of different sandpaper grits. Both the microphone and speaker were positioned centrally at normal incidence to the target surface. The microphone was positioned above and slight behind the loudspeaker such that the recorded pulse amplitude is significantly lower than the recorded echo amplitude. (Middle column) Shows the accompanying spectrograms for the oscillograms on the left. Within the middle column, the pulse (Left) spectral envelope is highly distorted when recorded from this aspect. The recorded echo (Right) reveals the spectral notches induced by spectral interference when the pulse reflects off of the irregular sandpaper surface. Discreet spectral notches (also evident in the oscillograms) appeared at different positions in the echo depending on sandpaper grit size. The (Right column) shows the mean power spectrum of 25 echoes, illustrating complex variations in the spectral envelope that occurred addition to appearance of discreet notches. Echoes reflected off of different sandpaper grits contained unique patterns of spectral modulations that the bats could use to discriminate between surface textures.
Discrimination performance
At the beginning of each trial when the trained bats were first placed at the center of the y-maze facing forward, they emitted pulses at a rate of 5–15 pulses per second (9.9 ± 4.5; n = 3 bats, 90 trials per bat) and oriented their body and head alternately toward both targets. A preliminary choice was usually made within 3–5 s, and pulse rates increased as the bats crawled toward one of the targets. During locomotion, the bats displayed frequent vertical and horizontal head scanning movements, and paused to scan the target or crawled back and forth toward the targets before finally choosing which one to mount. Acoustic recordings showed that the bats often, but not always, emitted pulses in irregular bursts (or strobe groups) as they scanned the targets. However, we saw no evidence of a correlation between the appearance of pulse bursts and sandpaper grit number or difficulty of comparison. Individual bats were highly variable in their temporal emission patterns both within sets and across days, and pulse emission rates appeared to vary with the bats motivational state (e.g., hunger levels) and crawling speed more so than with differences in task difficulty. After completing all sets, we conducted a follow experiment in which we measured the pulse emission rates of the bats during the final 2 s before choosing a target, first while performing a simple (80 grit vs. 180 grit) comparison and secondly during a difficult (150 grit vs. 180 grit) comparison, and found no significant difference in emission rates or temporal patterns between the two challenges (9.9 ± 3.5 Hz vs. 10.5 ± 3.9 Hz; t-test, n = 45, p = 0.62).
Figure 3 shows the combined discrimination assay results for all three bats. All three bats easily discriminated all sandpaper grit sizes from a smooth plexiglass surface with success rates above 85%. Each individual bat discriminated all but two sandpaper grit comparisons with better than 78% success rates (mean 86% success). Errors tended to occur toward the end of set when the bats became impatient and ran to a target without scanning. All three bats failed to perform better than chance on the same two comparisons, the 150 grit vs. 180 grit (92 vs. 83 μm particle diameter; 60, 64, and 58% individual success rates) and also when comparing 220 grit vs. 240 grit (68 vs. 54 μm; 60, 62, and 64% individual success rates). After completing the entire series of 36 comparison sets, we revisited the two failed tests to allow each bat an additional week of practice time on these comparisons, but saw no improvements in performance with experience.
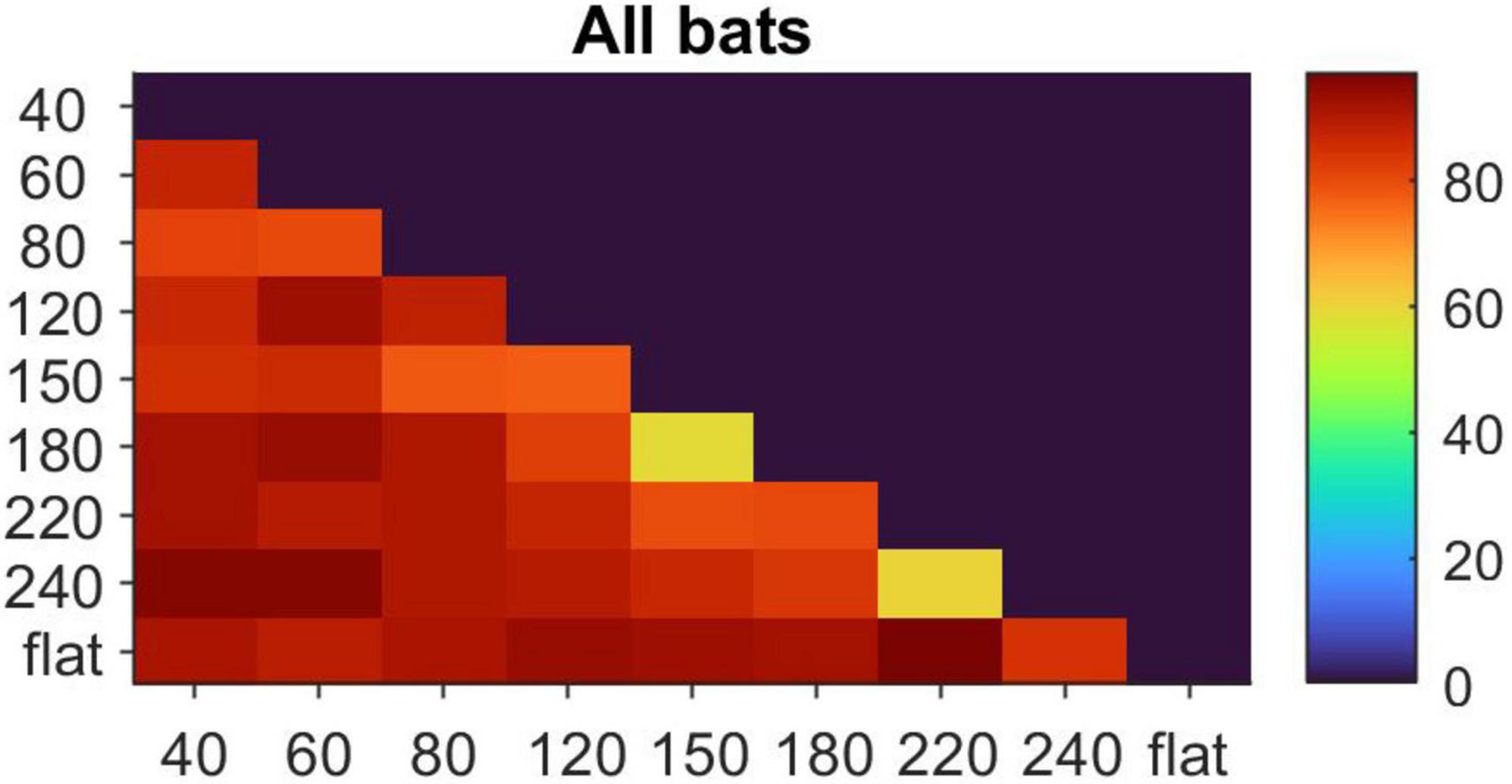
Figure 3. Summary of the pooled results of discrimination assay for all three bats (45 trials per bat, per comparison). Scale bar depicts percentage correct. All three bats performed similarly across all comparisons, falling below chance on only two tests (yellow bins). “Flat” refers to a smooth plexiglass surface, which the bats discriminated against all sandpaper grits.
All three bats easily discriminated each of the sandpaper grits from the flat plexiglass surface. Figure 4 plots the combined performances of all three bats vs. the absolute and relative differences in mean particle diameters corresponding to all 28 different grit comparisons. The 220 grit vs. 240 grit challenge compared the two grits with the smallest mean particle diameters (68 vs. 54 μm) corresponding to an absolute difference of 14 μm, but this wasn’t the smallest absolute difference. The 150 grit vs. 180 grit sandpapers differed by only 10 μm. The next smallest absolute differences after these two were 23 and 24 μm (120 vs. 150 and 150 vs. 220) and all three bats successfully discriminated between those grits better than chance (77 and 79%, respectively). These data indicate that absolute difference in mean particle diameters mattered more than absolute size when performing the discrimination task, and that a mean diameter difference of at least 23 μm was necessary for the bats to successfully discriminate between surface roughness.
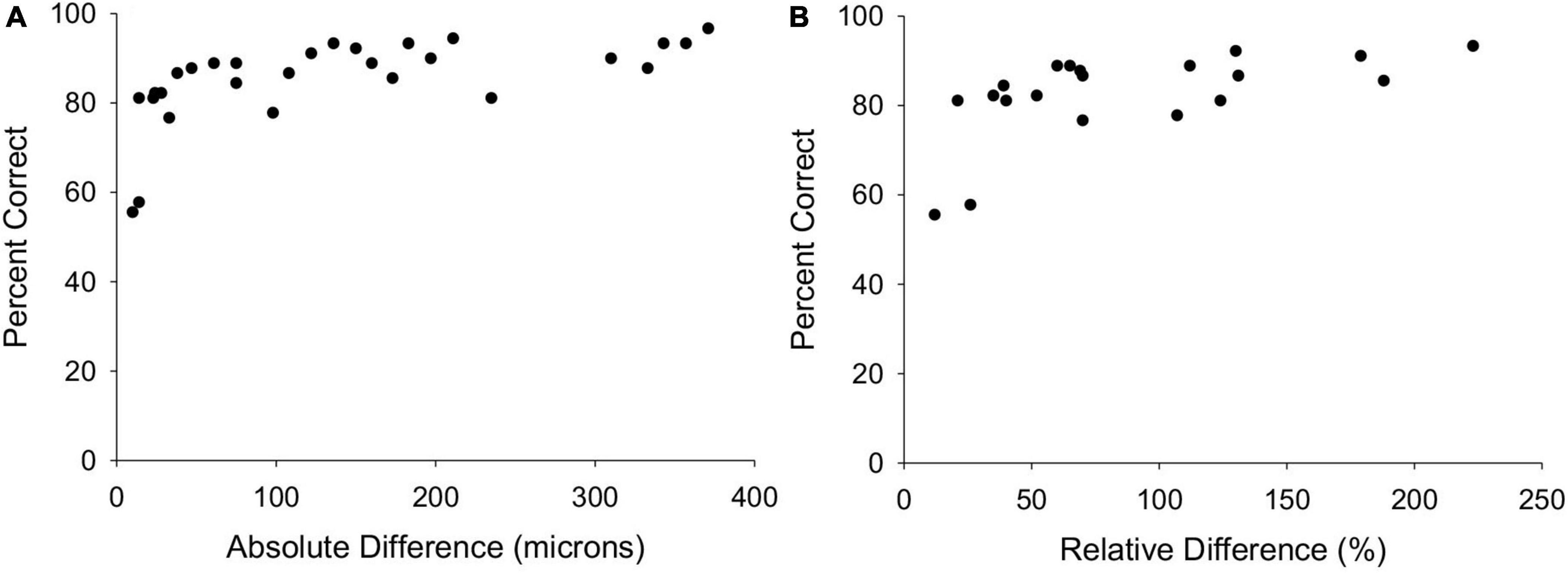
Figure 4. (A) Plot of the percentage correct choices for all three bats vs. the absolute difference in mean particle diameters for all 28 sandpaper comparisons. (B) Plots the same performance data vs. relative difference in mean particle diameters for each grit size comparison (larger size divided by smaller size).
To test for the contributions of vision, we tested all three bats in the 80-grit vs. 180-grit challenge under dark conditions while the handler wore a red LED headlamp. Under dark conditions, all three bats continued to perform well above chance (93, 96, 89; n = 45 trials). In a second test, we repeated the 80-grit vs. 180-grit challenge in dim light, but with both targets pressed behind a clear plexiglass plate. This way the targets would have offered the same visual cues but lacked variance in echo acoustic cues. Under this condition all three bats were equally likely to choose either target (46, 49, and 40%), indicating that their choices were not biased by the shade of the sandpaper samples. This does not completely exclude the possibility that bats integrated visual cues with auditory feedback during the discrimination trials, but indicates that visual cues alone were insufficient to account for the bat’s performance.
Discussion
In this set of experiments, we sought to determine the texture resolving limits of a cave-dwelling, echolocating bat that emits broadband, multi-harmonic downward FM sweeps. The range of surface textures used here spanned an ecologically relevant range, from very rough (40 grit) sandpapers that the bats could easily land and climb upon, to very fine textures too smooth to climb on. Previous research with other bat species has concluded that FM bats rely heavily upon spectral interference patterns in the returning echoes to infer target texture and shape cues (Simmons et al., 1974; Habersetzer and Vogler, 1983; Schmidt, 1988; Mogdans et al., 1993), with some results hinting at the possibility that the bats might be able to discriminate between surface textures varying by as little as a few hundred microns. However, while some of these studies used controlled artificial echoes to isolate spectral from temporal cues, none systematically investigated the bats behavioral texture resolution limits. Electronic artificial echo mimics are ideal for controlling and manipulating the acoustic cues available to bats when performing a discrimination task, but using natural surfaces and allowing bats to scan the surfaces from multiple distances and angles affords the bats the opportunity to integrate information from many echoes, providing a more natural test of their discrimination capabilities. The neural integration of information from across multiple echoes takes place within the bats auditory system to support an important cognitive process that presumably facilitates better texture discrimination performances under natural conditions. The results of current experiments show that naturally behaving free-tailed bats are capable of much finer texture discrimination than what has been documented previously.
We trained bats to show a preference for the coarser of two surfaces and took advantage of the wide range of commercially available standardized sandpaper grits to challenge the bats with 28 different comparisons. We had no a priori expectations for where the resolution limit might lie, or if indeed one would appear from within the available comparisons. The bats were first trained to prefer a course surface over a flat plexiglass surface, and with reinforcement the bats generalized this learned preference to when comparing any two rough surfaces. The bats easily discriminated all sandpapers from a smooth plexiglass surface, up to and including the finest grit size tested, a 240 grit sandpaper, equal to a mean diameter difference of 54 μm. The bats distinguished between all but two grit-grit sandpaper comparisons, with those two representing the two smallest absolute differences in mean particle diameters between sandpapers. The bats could not discriminate between sandpapers grits varying by 10 and 14 μm, but they were able to discriminate between grits varying by 23 and 24 μm. Their performances on the 120 vs. 150 grit (23 μm difference) and the 150 grit vs. 220 grit (24 μm difference) were statistically better than chance but also produced the lowest percentage success rates (77 and 79%, respectively), supporting the conclusion that 23 μm is very close to their behavioral resolving limit.
All grit sizes used in this study refer to the standardized scale and tolerances for commercially available sandpapers set by the American National Standards Institute (ANSI) and the Coated Abrasive Manufacturers Institute (CAMI). Sandpaper grit sizes reference the mean particle diameter adhered to the paper surface, but the complex reflective surface is not perfectly predicted by mean particle diameter. Importantly, the abrasive particles are not perfectly spherical and their diameters alone cannot be used to predict the complex spectral interference patterns that arise in the echoes, but instead reflect the convergence of multipath trajectories indirectly related to particle diameter and shape. A previous study used scanning electron microscopy and a contact-profile meter to directly measure the surface unevenness of commercially available sandpapers and confirmed that although peak-to-peak amplitudes did not always align perfectly with standardized particle sizes, the mean amplitudes displayed a consistent monotonic relationship with the assigned grit number (Miyaoka et al., 1999). Thus, our acoustic measurements and published physical analyses indicated that commercial sandpapers provide reproducible acoustic stimuli across samples, and the range of sandpaper grits used in this study provided for a sufficiently variable range of spectrotemporal cues by which the bats could discriminate different surface textures. Lastly, surface roughness can influence echo loudness, which could by itself potentially account for the bat’s discrimination performance.
Sandpapers are made by adhering particles of a certain size range to a paper surface, and the grit number corresponds to a standardized mean particle diameter. The particles are collected by filtering crushed aluminum oxide through a series of progressively smaller sieves to produce pools of irregularly shaped particles. The production processes are standardized to produce a pool of material with less than 3% of the remaining particles being smaller than the corresponding sieve diameter. When a biosonar pulse reflects off of the sandpaper, some of the sound reflects off of the front-facing surfaces of the particles while some reflects off of the paper backing. If the particles were perfectly flat, this would produce a simple 2-glint echo pair that would overlap in time to create a single echo with a spectral interference pattern that is directly correlated to the time delay between the two echoes, which is determined by particle diameter. For the sandpaper grits used here, simple 2-glint echoes would have produced spectral notches at 240 kHz or higher, which is outside the bat’s hearing range. However, as shown in Figure 2, the sandpapers produced spectral notches and other changes in the spectral envelope of the echoes at lower frequencies corresponding to the first harmonic of outgoing pulse and well within the hearing range of the bats: these modulations can be accounted for by the occurrence of multi-path echoes reverberating between irregularly shaped particles to produce chaotic spectral interference patterns. While the reflections were chaotic, each sandpaper type generated a consistently reproducible echo spectral pattern that was distinctive to each grit size. It remains unknown whether the bats can directly infer surface textures based upon these chaotic spectral interference patterns, or if instead they learn an association between a collage of echo acoustic features and the physical properties of the surface that generated them. In current experiments the bats were allowed to touch and climb upon there sandpapers while learning to perform the discrimination assay, so it is possible that they learned the associations that subsequently guided their choices.
In Figure 2 it is evident that the echoes reflected from 150 grit and 180 grit sandpapers contained different patterns of spectral notches, and yet the bats were unable to discriminate between these two surfaces. Previous work using electronically generated echoes showed that bats use the spectral notch patterns to discriminate between 2-glint echoes (Schmidt, 1988; Simmons et al., 1990), but for some reason that was not the case here. Other sandpapers, such as the 40, 80, and 120 grits, produced echoes that had distinctive spectral envelopes but lacked prominent spectral notches, and yet the bats still easily discriminated these from one another. However, the bats may have had access to additional cues derived from head scanning and moving back and forth toward the targets; our acoustic measurements showed that a distinctive range of notch patterns unique to these grits could emerge from different incident angles, and prior research has shown that bats can exploited these patterns in their decision-making during foraging (Zsebok et al., 2013; Baier et al., 2019; Geipel et al., 2019). However, their inability to discriminate 150 grit from 180 grit suggests that the presence of spectral notches alone is insufficient to account for the free-tailed bat’s behavioral performance. These data suggest that free-tailed bats are evaluating and comparing the complete spectral envelope, and that additional features such as the rate of attenuation at higher frequencies are important for the discrimination of fine surface textures. The free-tailed bat is an FM bat that uses broadband, multi-harmonic pulses, but their auditory system is largely constrained to frequencies below 80 kHz, with more than half of the auditory substrate to committed processing frequencies in the range of 20–30 kHz. These auditory neurophysiological properties are somewhat different from other species used to study biosonar texture discrimination that display slightly better high-frequency hearing, including Eptesicus fuscus (Simmons et al., 1974; Mogdans et al., 1993), Megaderma lyra (Schmidt, 1988), Myotis myotis (Habersetzer and Vogler, 1983), and Glossophaga soricina (Simon et al., 2014). Foraging free-tailed bats and fast flyers that rely heavily upon narrow-bandwidth search phase pulses in the 20–30 kHz bandwidth, and their auditory system shows enhanced sensitivity to this bandwidth. Thus, both the acoustic properties of the free-tailed bats’ biosonar pulses and their auditory system appear mainly adapted to foraging at high speeds in open spaces. Despite this, they were able to discriminate between different surface textures differing by as little as 23 μm. Like other FM-emitting bats, free-tailed bats shift to broadband multi-harmonic pulses for short range targets. At short ranges, the second and third harmonics of the echoes can be relatively loud and therefore contribute to texture resolution despite the a animals lacking very sensitive high-frequency hearing.
Humans are better able to discriminate fine surface textures by touch than by vision, and a similar study measured the tactile discrimination performance of humans moving their fingers over sandpapers of different grit sizes (Miyaoka et al., 1999). That study found reported difference thresholds in the range of 2–3 μm mean particle diameters. Free-tailed bat sonar is not quite as good at human touch for texture discrimination, but they come surprisingly close without having to physically touch the surfaces. This is presumed to facilitate their ability to quickly and safely find a landing spot within a cave. That they can do this which such high resolution provides valuable new insights for understanding the evolution of echolocation pulse structure and the behavioral and neural processes by which bats use their biosonar to resolve details about the shape and texture of objects in their environment.
Data availability statement
The raw data supporting the conclusions of this article will be made available by the authors, without undue reservation.
Ethics statement
This animal study was reviewed and approved by the Texas A&M University Institutional Animal Care and Use Committee.
Author contributions
MS designed, executed, and analyzed the experiments and wrote the manuscript. TC conducted experiments and analyzed data. SM analyzed data, composed figures, and helped write the manuscript. All authors contributed to the article and approved the submitted version.
Funding
This study was supported by the US Office of Naval Research (Grant ONRN00014-17-1-2736).
Acknowledgments
We thank Mikayla Hobbs, Stephen Odunsi, Ana Houde, and Alexandra Goff for help with the animal care, training, and experiments.
Conflict of interest
The authors declare that the research was conducted in the absence of any commercial or financial relationships that could be construed as a potential conflict of interest.
Publisher’s note
All claims expressed in this article are solely those of the authors and do not necessarily represent those of their affiliated organizations, or those of the publisher, the editors and the reviewers. Any product that may be evaluated in this article, or claim that may be made by its manufacturer, is not guaranteed or endorsed by the publisher.
References
Accomando, A. W., Mulsow, J., Houser, D. S., and Finneran, J. J. (2020). Classification of biosonar target echoes based on coarse and fine spectral features in the bottlenose dolphin (Tursiops truncatus). J. Acoust. Soc. Am. 148, 1642–1646. doi: 10.1121/10.0001976
Baier, A. L., Wiegrebe, L., and Goerlitz, H. R. (2019). Echo-imaging exploits an environmental high-pass filter to access spatial information with a non-spatial sensor. iScience 14, 335–344. doi: 10.1016/j.isci.2019.03.029
Geipel, I., Steckel, J., Tschapka, M., Vanderelst, D., Schnitzler, H. U., Kalko, E. K. V., et al. (2019). Bats actively use leaves as specular reflectors to detect acoustically camouflaged prey. Curr. Biol. 29:2731–2736.e3. doi: 10.1016/j.cub.2019.06.076
Grunwald, J. E., Schornich, S., and Wiegrebe, L. (2004). Classification of natural textures in echolocation. Proc. Natl. Acad. Sci. U.S.A. 101, 5670–5674. doi: 10.1073/pnas.0308029101
Habersetzer, J., and Vogler, B. (1983). Discrimination of surface-structured targets by the echolocating bat myotis-myotis during flight. J. Comp. Physiol. 152, 275–282.
Lamb, G. D. (1983). Tactile discrimination of textured surfaces: Psychophysical performance measurements in humans. J. Physiol. 338, 551–565.
Macias, S., Bakshi, K., Garcia-Rosales, F., Hechavarria, J. C., and Smotherman, M. (2020a). Temporal coding of echo spectral shape in the bat auditory cortex. PLoS Biol. 18:e3000831. doi: 10.1371/journal.pbio.3000831
Macias, S., Bakshi, K., and Smotherman, M. (2020b). Functional organization of the primary auditory cortex of the free-tailed bat Tadarida brasiliensis. J. Comp. Physiol. A. Neuroethol. Sens. Neural. Behav. Physiol. 206, 429–440. doi: 10.1007/s00359-020-01406-w
Macias, S., Bakshi, K., and Smotherman, M. (2022). Faster repetition rate sharpens the cortical representation of echo streams in echolocating bats. eNeuro 9:ENEURO.410–ENEURO.421. doi: 10.1523/ENEURO.0410-21.2021
Ming, C., Haro, S., Simmons, A. M., and Simmons, J. A. (2021). A comprehensive computational model of animal biosonar signal processing. PLoS Comput. Biol. 17:e1008677. doi: 10.1371/journal.pcbi.1008677
Miyaoka, T., Mano, T., and Ohka, M. (1999). Mechanisms of fine-surface-texture discrimination in human tactile sensation. J. Acoust. Soc. Am. 105, 2485–2492. doi: 10.1121/1.426852
Mogdans, J., Schnitzler, H. U., and Ostwald, J. (1993). Discrimination of two-wavefront echoes by the big brown bat, Eptesicus fuscus: Behavioral experiments and receiver simulations. J. Comp. Physiol. A 172, 309–323. doi: 10.1007/BF00216613
Pollak, G. K., Marsh, D. S., Bodenhamer, R., and Souther, A. (1978). A single-unit analysis of inferior colliculus in unanesthetized bats: Response patterns and spike-count functions generated by constant-frequency and frequency-modulated sounds. J. Neurophysiol. 41, 677–691. doi: 10.1152/jn.1978.41.3.677
Schmidt, S. (1988). Evidence for a spectral basis of texture perception in bat sonar. Nature 331, 617–619. doi: 10.1038/331617a0
Simmons, J. A., Ferragamo, M. J., and Moss, C. F. (1998). Echo-delay resolution in sonar images of the big brown bat, Eptesicus fuscus. Proc. Natl. Acad. Sci. U.S.A. 95, 12647–12652.
Simmons, J. A., Lavender, W. A., Lavender, B. A., Doroshow, C. A., Kiefer, S. W., Livingston, R., et al. (1974). Target structure and echo spectral discrimination by echolocating bats. Science 186, 1130–1132.
Simmons, J. A., Moss, C. F., and Ferragamo, M. (1990). Convergence of temporal and spectral information into acoustic images of complex sonar targets perceived by the echolocating bat, Eptesicus fuscus. J. Comp. Physiol. A 166, 449–470. doi: 10.1007/BF00192016
Simon, R., Knornschild, M., Tschapka, M., Schneider, A., Passauer, N., Kalko, E. K., et al. (2014). Biosonar resolving power: Echo-acoustic perception of surface structures in the submillimeter range. Front. Physiol. 5:64. doi: 10.3389/fphys.2014.00064
Vater, M., and Siefer, W. (1995). The cochlea of Tadarida brasiliensis: Specialized functional organization in a generalized bat. Hear. Res. 91, 178–195. doi: 10.1016/0378-5955(95)00188-3
Keywords: bat, echolocation, biosonar, texture, discrimination, hearing, auditory
Citation: Smotherman MS, Croft T and Macias S (2022) Biosonar discrimination of fine surface textures by echolocating free-tailed bats. Front. Ecol. Evol. 10:969350. doi: 10.3389/fevo.2022.969350
Received: 16 June 2022; Accepted: 18 October 2022;
Published: 03 November 2022.
Edited by:
M. Jerome Beetz, Julius Maximilian University of Würzburg, GermanyReviewed by:
Manfred Kössl, Goethe University Frankfurt, GermanyYossi Yovel, Tel Aviv University, Israel
Leonie Baier, Smithsonian Tropical Research Institute, Panama
Copyright © 2022 Smotherman, Croft and Macias. This is an open-access article distributed under the terms of the Creative Commons Attribution License (CC BY). The use, distribution or reproduction in other forums is permitted, provided the original author(s) and the copyright owner(s) are credited and that the original publication in this journal is cited, in accordance with accepted academic practice. No use, distribution or reproduction is permitted which does not comply with these terms.
*Correspondence: Michael S. Smotherman, c21vdGhlcm1hbkB0YW11LmVkdQ==