- 1Department of Geology and Geophysics, University of Wyoming, Laramie, WY, United States
- 2Environmental Studies, Colby College, Waterville, ME, United States
- 3Department of Earth and Atmospheric Sciences, Cornell University, Ithaca, NY, United States
- 4Paleontological Research Institution, Ithaca, NY, United States
Conservation paleobiology aims to provide a longer-term perspective on environmental problems to inform decisions about natural resource conservation. As such, conservation paleobiology research falls short when geohistorical data and insights do not inform conservation practice, contributing to the well-known idea that a “gap” exists between the production and use of science in the environmental realm. Our study quantified the extent of this research-implementation (or knowing-doing) gap through a systematic literature review and survey questionnaire. We determined whether empirical studies in conservation paleobiology with a link to conservation, management, or restoration documented the use of geohistorical data to implement some form of action or if there was a specific mention of how the geohistorical data could be used in theory. Results indicate that “applied” conservation paleobiology has a poor record of translating research into action. Tangible conservation impacts were evident in only 10.8% of studies. Over half of these studies included coauthors affiliated with a conservation organization. Among the studies coded as having a theoretical application, 25.2% specified how the geohistorical data could be implemented to enhance conservation, management, or restoration actions. All studies documenting action used geohistorical data from the geologically recent past where the species and habitats are familiar to those found today. Drawing insights from the bright spots we identified, we offer some practical suggestions to narrow the gap between knowing and doing in conservation paleobiology.
Introduction
From its beginning, conservation biology has wrestled with defining and assessing its relationship to on-the-ground conservation action and policy implementation. A disconnect between peer-reviewed studies (conservation biologists' research output) and conservation action (conservation biologists' disciplinary goal) contributes to the deeply ingrained perception among researchers that a gap exists between science and practice (Anonymous, 2007; Knight et al., 2008; Arlettaz et al., 2010; Kiessling et al., 2019). Implicit in the gap metaphor is the belief that it is desirable to bring the science and practice of conservation closer together. Efforts to assess this knowing-doing gap in conservation biology have evaluated: (1) evidence of conservation action in published studies (Ehrenfeld, 2000; Knight et al., 2008; Esler et al., 2010; Britt et al., 2018; Mair et al., 2018), (2) evidence of cited research in the bibliography of outputs from conservation practitioners (Stinchcombe et al., 2002; Linklater, 2003), and (3) surveys and/or interviews of researchers and/or practitioners (Knight et al., 2008; Esler et al., 2010; Matzek et al., 2014; Reed et al., 2014; Taft et al., 2020). Quantifying conservation action in conservation research yields humbling results: < 5% of studies published in three issues of Conservation Biology—the flagship journal of the Society for Conservation Biology—discussed conservation achievements (Ehrenfeld, 2000), only 5.7% of conservation assessment studies documented implementation of conservation actions while 29.5% discussed implementation in theory (Knight et al., 2008), 3% of invasion ecology studies referred to “implementation”, with only 2% referring to “policy” (Esler et al., 2010), 38% of conservation genetics studies identified specific conservation or management recommendations (Britt et al., 2018), and only ~30% of conservation science studies across two decades (1997–2017) proposed a conservation response (Williams et al., 2020).
Like conservation biology, conservation paleobiology—the application of long-term perspectives derived from geohistorical records to address modern-day issues in conservation, restoration, and management of biodiversity and natural resources (Dietl and Flessa, 2009, 2011, 2017; Dietl et al., 2015)—must assess and reflect on its own knowing-doing gap. Paleobiology researchers have long recognized the potential for their work to support conservation—for instance, Hunter et al. (1988) drew on paleoecology to develop recommendations for nature reserve design within 3 years of Soulé's (1985) foundational paper defining the field of conservation biology—but the past decade includes both a clear rallying around “conservation paleobiology” by a community of paleontologists aspiring to apply their knowledge and tools to solve conservation problems and a tremendous output of conservation paleobiology research aimed at informing conservation decisions (Dietl and Flessa, 2009; Figure 1 in Tyler, 2018; Dillon et al., 2022).
It is therefore high time to consider the effectiveness of recent efforts to produce actionable conservation paleobiology research results. Are we contributing geohistorical data and insights that are useful to conservation practitioners? Here, we assess the knowing-doing gap in conservation paleobiology through the lens of a literature review and survey that spans the past decade of research effort. In carrying out this necessary but neglected task, we put aside any “unsubstantiated claims and rationalizations” (sensu Ehrenfeld, 2000) that can be used to convince ourselves and others about the relevance of paleontological data and tools in conservation. Only then can we ask the hard questions:
• Do conservation paleobiology studies include evidence of conservation actions?
• Are conservation practitioner co-authors associated with studies documenting conservation actions (Britt et al., 2018)?
• When studies fail to report conservation action, but include specific theory for implementation, does the knowing-doing gap continue beyond publication, or are the actions implemented “off the page”?
• Are particular ecological or taxonomic scales and types of environments or geohistorical data more likely to resonate with conservation practitioners?
• Are near- or deep-time studies more likely to lead to actionable conservation paleobiology results?
• Has the gap between science and practice in conservation paleobiology narrowed over time?
By asking these questions, we aim to quantify the extent and nature of the knowing-doing gap in conservation paleobiology. Guided by “bright spots” (Cvitanovic and Hobday, 2018) we identify in our literature review and survey, where conservation paleobiology research has already contributed to real-world conservation actions, we also offer some practical suggestions for researchers who aspire to “put the dead to work” (Dietl and Flessa, 2011).
Materials and methods
Literature review
We compiled eligible articles for analysis from the Clarivate Web of Science (Core Collection) platform published from January 1, 2010 to July 13, 2020. We used the following boolean terminology to search for articles (English only): (paleo* OR palaeo*) AND (*ecology OR *biology OR ecolog* OR biolog*) AND (conserv* OR restor* OR manag*). Our search cast a wide net to include more than self-identified conservation paleobiology studies. Any study using geohistorical data to inform “conservation”, “restoration”, or “management” was included—even if the authors did not explicitly state the term “conservation paleobiology”. In other words, we targeted “applied” conservation paleobiology (Dillon et al., 2022) studies that were inspired by considerations of practical use. A recent survey of the conservation paleobiology community indicated that it is currently divided on what research approaches could be included under the umbrella of “conservation paleobiology”: “some [respondents] thought that conservation paleobiologists should engage with conservation practitioners and align their research goals with conservation needs, whereas others viewed the field as a separate, more academic undertaking with theoretical implications for conservation” (Dillon et al., 2022, p. 15). Both views have merit—indeed, the practical application of research often depends on unforeseen discoveries from theoretical advances that propel us, sooner or later, in completely unanticipated directions (i.e., basic research driven by the desire for fundamental understanding). But, because authors of “applied” conservation paleobiology articles took an additional step beyond reporting findings and intentionally aligned their research goals with conservation needs and concerns, we chose to design our search query to identify this subset of conservation paleobiology studies with the intent of building the most meaningful and appropriate dataset for assessing the size and nature of the knowing-doing gap. Knight et al. (2008) made a similar decision when they limited their evaluation of the knowing-doing gap in conservation biology to only studies that contained an assessment to identify priority areas for nature conservation activities.
The pre-corpus included n = 1534 articles (e.g., empirical studies, review articles, book chapters, and perspective, essay, opinion, and synthesis articles) on a wide range of topics (e.g., studies in paleoecology, archeology, phylogeography, phylogenetics, biogeography, historical ecology, etc.). Each phase of screening articles was documented in a Preferred Reporting Items for Systematic Reviews and Meta-Analyses (PRISMA) flowchart (Page et al., 2021; Supplementary Figure 1). We initially screened all 1534 articles from the search query for relevancy by reading the title and abstract. We used the terms “conservation”, “management”, or “restoration” to search within an article and determine relevance when it was not apparent in the title or abstract. An “applied” article met the inclusion criteria if it had geohistorical data and a link to conservation, management, or restoration. During screening we excluded non-empirical articles, methods-only articles, modern process /proxy calibration studies without geohistorical data, as well as articles only using geohistorical data for the calibration of phylogenetic analyses (Supplementary Data Sheet 1).
We randomly selected n = 59 articles from the pre-corpus to develop and refine coding criteria and train coders. The final coding criteria were established following four rounds of coding. For each round of coding, the trainees were given the same 14–15 articles to code. We used an inter-rater reliability test (Kappa-Fleiss) to quantify similarity among coders (Landis and Koch, 1977). A threshold score of 0.75 (on a scale of −1 to 1) was used to indicate sufficient agreement among coders for each coded article (Supplementary Table 1). Throughout the coding process, we checked the reliability of our coding method by quantifying the consistency among coders. A non-coder included a reliability check article among every 20 articles coded. Coders had no knowledge of which articles were part of the reliability checks. We used the inter-rater reliability test to quantify similarity for each reliability check article as a check-in for every batch of 20 articles coded (Supplementary Table 1). Coders moved onto the next batch of papers only if there was sufficient agreement (>0.75) and any discrepancies were discussed and agreed upon by all coders.
For data collection, each article was assigned to a coder. First, each coder determined whether an article met the eligibility criteria. At least one other coder provided a second opinion for each article determined to be not relevant. As a group, we discussed individual articles identified as “difficult to code' and coded these by consensus. The final corpus (Supplementary Data Sheet 2) includes each article determined to be relevant.
Basic information collected from each article included publication year and author affiliations (Table 1). Author affiliation was categorized as one or more authors identified as having an affiliation with a conservation or a non-conservation organization. We defined an author affiliation as “conservation” if the organization self-identified as having partnerships with stakeholders in research related to conservation, restoration, or management of biodiversity and/or natural resources. Author affiliations considered “non-conservation” included academic institutions, research institutes, museums, etc. Author affiliation was only coded once for each type of author on an article (e.g., even if several non-conservation co-authors were listed on an article, the article was coded once as non-conservation). An article could also be a combination of conservation and non-conservation co-authors.
For each article, we determined whether the study documented the implementation or application of geohistorical data to conservation, restoration, or management needs (Table 2). We coded articles as an “action” study or “theory” study. An article was coded as an action study if it contained a discussion about the implementation of a conservation, restoration, or management action. An article coded as a theory study was further categorized as either relating the goal or results of the study to conservation, restoration, or management (“theory-general”) or going a step further and specifically mentioning an actual recommendation or management intervention (“theory-specific”).
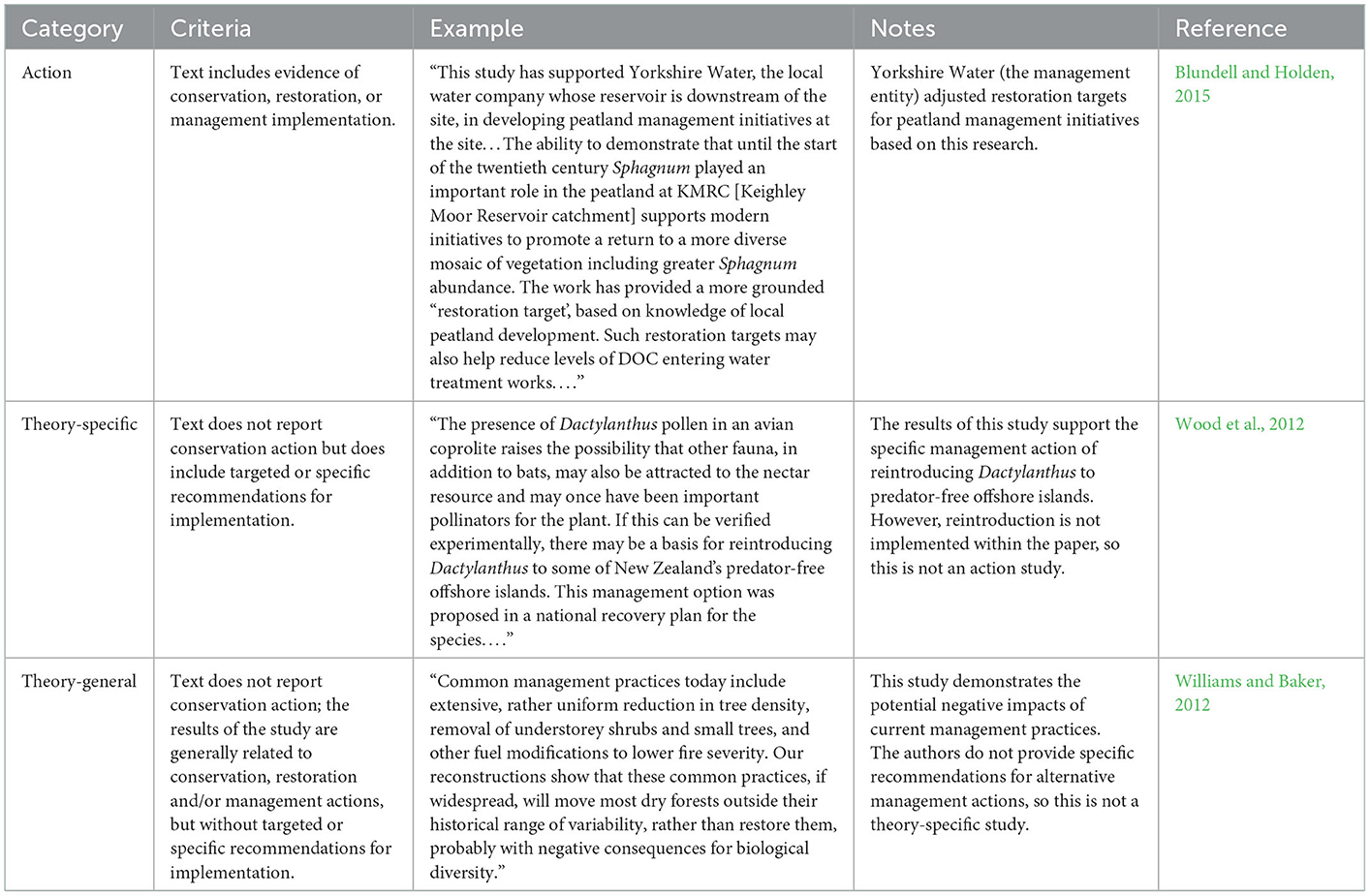
Table 2. Criteria for determining inclusion of an applied conservation paleobiology study into “action”, “theory-general”, and “theory-specific” categories.
Each article was coded to determine if action studies were more likely to focus on a type of environment (freshwater, marine, terrestrial, or any combination thereof; Table 1). Transitional environments were coded based on environmental combinations. For example, wetlands or peatlands were coded both as freshwater and terrestrial and estuaries were coded as both marine and terrestrial. Ecological focus was also coded based on the conservation, restoration, or management goals of the article (Table 1). If the goal of the article focused on a single species or population of the same species, it was coded as “single species/population”. An article that focused on conservation, management, or restoration of several species was coded as “assemblage”. If an article instead had a broader focus on a type of landscape feature (e.g., lake or forest) it was coded as having an “ecosystem” focus. When an organism was the focus of the conservation, restoration, or management efforts, we coded its taxonomic classification as either invertebrate, vertebrate, plant, or other (see Table 1 for finer-scale taxonomic classification of invertebrate and vertebrate categories). Articles with more than one taxonomic classification were coded to allow for multiple combinations of categories for a single article. Taxonomic information was not recorded if solely used as a proxy for environmental conditions (e.g., diatoms used as water quality indicators). Details about the types of proxies (biological, geochemical, lithological, or any combination thereof) used were documented to understand which proxies most commonly have application in conservation, restoration, or management (Table 1; National Research Council, 2005). When an article used more than one type of proxy, we coded for these combinations. The timescale used in each article was coded based on an arbitrary division between “near” and “deep” time using the Pliocene-Pleistocene boundary (2.58 million years ago; Dietl and Flessa, 2017; Table 1). Each article was coded as either, near-time, deep-time, or both near- and deep-time. We further divided near-time at the late Pleistocene—Holocene boundary 11,700 years ago (Holocene-only).
For each category of information we collected, a Pearson's Chi-squared or Fisher's Exact test was used to detect any relationship between article type (action vs. both theory-general and theory-specific articles combined) and each coded category. We asked whether action studies were more likely than theory studies to be co-authored by authors with conservation affiliations (sensu Britt et al., 2018). We also tested whether action studies differed from theory studies in terms of ecological and taxonomic focus, types of environments and proxies, and timescales (Table 1). Chi-squared and Fisher's Exact tests were conducted in R Version 4.2.0 (R Core Team, 2022).
Survey
Research results may not be used by conservation practitioners for several years after they are published in the peer-reviewed literature (Knight et al., 2008). In addition, peer-reviewed publications may not necessarily track the conversation about implementation between researchers and practitioners (Hogg et al., 2018). Therefore, for articles coded as having a theoretically specific recommendation or implementation method (theory-specific), we used a survey to further investigate whether the findings had been used or considered by conservation practitioners outside of the published peer-reviewed article. The survey served to determine if a theory-specific article should be re-classified as action. To aid our interpretation of the knowing-doing gap, we also asked corresponding authors of theory-specific and action studies whether their study was co-designed with practitioners, the time it took for their study's recommendations to be implemented, and to provide additional references for studies demonstrating documented implementation in conservation paleobiology, which we added to our corpus if the study met the eligibility criteria.
Each corresponding author was emailed a short survey (Supplementary Table 2) using Qualtrics XM, approved by the Cornell Institutional Review Board (Protocol Number: IRB0144055). If one of us personally knew a corresponding author, a personal email was sent instead that included the solicitation email and anonymous link to the survey. If an author was the corresponding author for more than one article, they were sent an email that included the solicitation email, survey link, and citations of each article included in our dataset they were corresponding author for and asked to fill out the survey once per article. Reminders were sent via email and through Qualtrics XM to those that had not yet responded to the survey. Follow up emails were sent to select survey respondents to clarify their responses or ask for more information pertaining to their article.
Results
The decisions and methods reported in the PRISMA flowchart led to a final corpus of n = 442 coded peer-reviewed articles out of 1,534 articles (29%) from the initial Web of Science search (Supplementary Figure 1). Two additional articles were added to the corpus that were suggested by survey respondents for a total of n = 444 coded peer-reviewed articles (Supplementary Data Sheet 2).
Documented implementation in the literature review and survey
In the literature review, we found that a small proportion of studies (3.8% or 17 of 444 articles) documented implementation (action) versus only a theoretical link to conservation. From the survey, which had a response rate of 40.5% (58 out of 143 articles), we determined that 311 of the 143 theory-specific studies had been used or considered by a conservation practitioner (Supplementary Table 2; Supplementary Data Sheet 3). We re-coded these 31 articles as “action” for a new total of n = 48 action studies (10.8% or 48 of 444 articles; Figure 1). After re-coding these “theory-specific” studies to “action”, over half of the remaining studies (71.7% or 284 of 396 articles) did not include a specific recommendation (=theory-general; Supplementary Table 3).
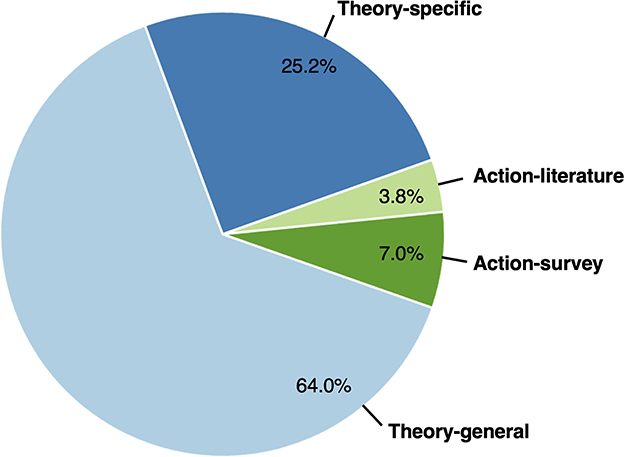
Figure 1. Proportion of documented implementation categories for n = 444 articles coded as action, theory with a specific recommendation, and theory without a specific recommendation.
Action studies—author affiliations
Action studies were more likely than theory studies to be co-authored by authors with conservation affiliations [X2 (1, N = 444) = 18.577, p < 0.001]−45.8% of action studies (22 of 48 articles) were co-authored by authors with both conservation and non-conservation affiliations (Figure 2; Supplementary Tables 3, 5), and three action studies included authors with only a conservation affiliation (or 6.2% of the 48 action studies). Only 6.9% (23 of 331 articles) of studies that did not include a conservation co-author were action studies, however, these studies made up nearly half of all the action studies (47.9% or 23 of 48 articles; Figure 2A).
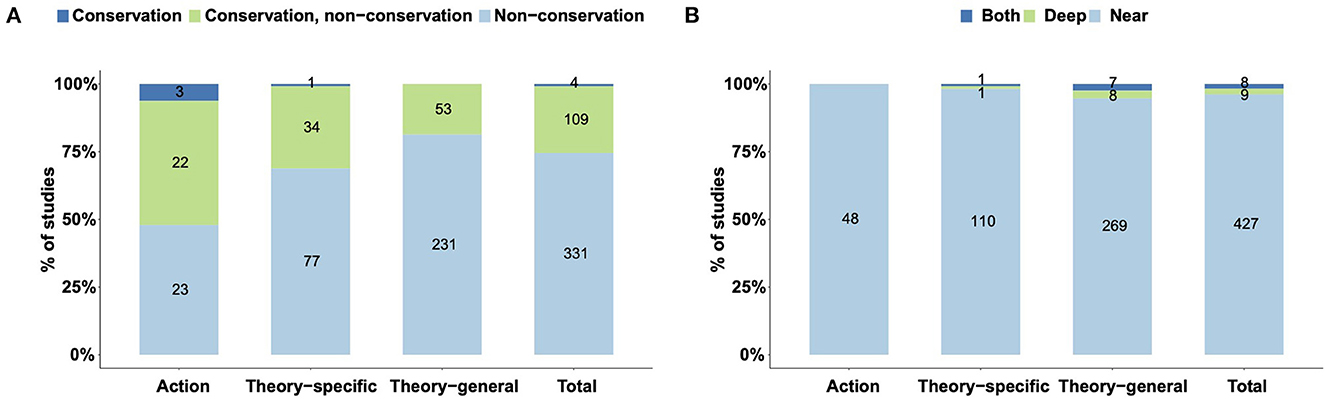
Figure 2. Author affiliations among documented implementation categories. (A) Proportion of studies across the documented implementation categories (action, theory-specific, theory-general) coded as non-conservation, conservation, and a mix of conservation and non-conservation author affiliations. (B) Proportion of studies coded as near-time, deep-time, and a combination of both near- and deep-time. Numbers within bars equal sample size for each category.
Action studies—other attributes
Across the different types of environments, action studies primarily focused on terrestrial environments and rarely on marine environments (55% vs. 8.3%, respectively; Supplementary Figure 2B). The majority of action studies focused on plants (75% or 24 studies; Supplementary Figure 2D), followed by studies with a taxonomic focus on invertebrates (corals and mollusks; 3 and 10% of action studies, respectively) and vertebrates (mammals and bony fish; 9 and 3% of action studies, respectively; Figure 3; Supplementary Tables 3, 4). More than half of the action studies (56.2% or 27 of 48 studies) also focused research questions at the ecosystem scale (Supplementary Figure 2A). All action studies used biological or combinations of biological plus geochemical and lithological proxies (Supplementary Figure 2C; Supplementary Table 3). All action studies focused on near-time scales (Figure 2B). Among these action studies, 87.5% (42 of 48 studies) focused only on the Holocene. No significant patterns between action vs. theory (all) studies were found for environment, taxonomic focus, ecological focus, type of proxy, or timescale (Supplementary Table 5).
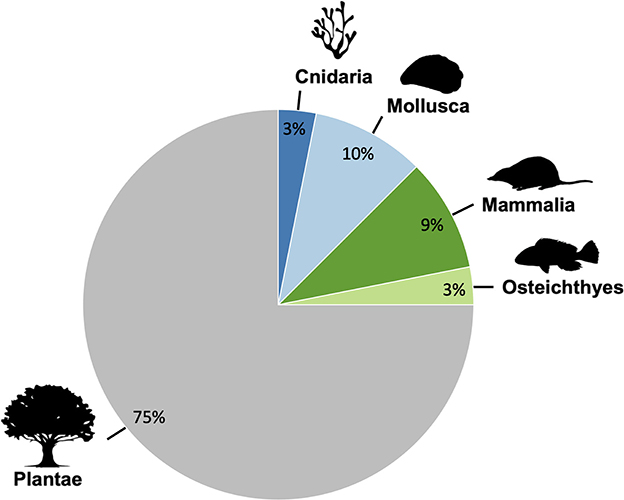
Figure 3. Proportion of action studies (n = 48) that focus on invertebrates (Cnidaria and Mollusca), vertebrates (Mammalia and Osteichthyes), and Plantae. Silhouettes were retrieved and modified from Canva (accessed 18 January 2023).
Discussion
Where is the conservation in conservation paleobiology?
Our literature review estimated that the gap between knowing and doing in conservation paleobiology is wide and even wider when only considering peer-reviewed literature. Sixty-four percent of applied studies did not provide more than a general statement (i.e., theory-general) linking the study's results to conservation, restoration, or management practice or policy. Initially, the literature review indicated that only 3.8% of studies led to a documented conservation implementation. But, when we surveyed authors of theory-specific studies about the conservation outcomes of their research beyond publication, the proportion of studies categorized as action tripled (10.8%). Several (n = 13) theory-specific studies were not co-authored with conservation practitioners but had been co-designed with conservation practitioners (Supplementary Table 2), even though the collaborations were not apparent in the peer-reviewed publications based on author affiliations (i.e., all authors had non-conservation affiliations). Although adding the survey results to the initial literature review narrows the gap between knowing and doing more than the literature alone, these action studies still only account for one tenth of our dataset of applied conservation paleobiology studies. In addition, despite the total number of relevant studies steadily increasing over the past decade, the knowing-doing gap appears to have been relatively stable, in terms of the proportion of studies that documented conservation impacts (Supplementary Figure 3B). Another yardstick of success—the proportion of studies co-authored with conservation partners—also did not appreciably increase over the past decade (Supplementary Figure 3C). Whether we like it or not, and whether we recognize it or not, these inconvenient truths draw attention to a separation between reality and the way conservation paleobiologists who are motivated by a desire to conduct research relevant to conservation would like things to be. Most applied research conducted over the past decade in conservation paleobiology appears to not have informed conservation, restoration, or management actions in a tangible way.
Although it seems reasonable to imagine that the gap is wide in conservation paleobiology because the approach is still relatively new, conservation biology's own longstanding internal struggles with the same problem forewarns that other much more nuanced and complicated factors should also be considered, which will not all easily be addressed from within the prescribed boundaries of the field. Potential reasons to explain the gap in conservation biology include: a disconnect between the type of data created and what is actually needed by practitioners (Arlettaz et al., 2010; Habel et al., 2013; Kiessling et al., 2019), lack of incentives for academic researchers to conduct research with practical applications (Burbidge and Wallace, 1995; Savarese, 2018) or to communicate results to conservation practitioners (Taylor et al., 2017), and research results that are out of sync with policy windows (Cook and Sgrò, 2017). Conservation practitioners also may be unaware of the full range of uses for geohistorical data (Durham and Dietl, 2015; Smith et al., 2018), and often report not using published literature because of technical jargon or abstract framing (Redford and Taber, 2000; Pullin et al., 2004; Bainbridge, 2014; Matzek et al., 2014; Taft et al., 2020). Paywalls or subscriptions imposed by journals further limit conservation practitioners' access to peer-reviewed literature (Fuller et al., 2014). In addition, academic researchers often lack the training to develop skills necessary to collaborate across disciplinary boundaries (Arlettaz et al., 2010; Kelley et al., 2019; Merkle et al., 2019; Kelley and Dietl, 2022). The gap in conservation paleobiology likely stems from some combination of all these factors and others. Which factors are most relevant is a matter for further research, research that should begin without delay.
In good company
Our literature review and follow-up survey of applied conservation paleobiology studies fit a general pattern of limited evidence of conservation implementation in the peer-reviewed conservation sciences literature (Ehrenfeld, 2000; Knight et al., 2008; Esler et al., 2010). We found that 10.8% of the applied conservation paleobiology studies we reviewed either documented conservation implementation or reported that conservation practitioners considered the findings (action studies). Another 25.2% of applied conservation paleobiology studies provided specific recommendations (theory-specific studies) for practitioners, comparable to other conservation biology studies that proposed specific recommendations (Knight et al., 2008; Britt et al., 2018; Williams et al., 2020). It is reasonable to expect that applied conservation paleobiology studies would have been more oriented toward conservation action, given that our corpus comprised studies that self-identified as “conservation”, “restoration”, or “management” relevant based on the search terms of our literature review. If we had reviewed the field of paleobiology more broadly, the low rate of action studies that we found would be more intuitive. Instead, 64.1% of the studies in our corpus included only a general-theory nod to conservation, echoing Ehrenfeld's (2000, p. 107) perceptive, albeit bitter, observation that, “authors would tack on a depressingly predictable final paragraph that would explain how important th[eir] work could eventually be to conservation and why more research was needed.” Such fire-and-forget statements (Gibbons et al., 2011)—about “conservation dividends to be earned in an unspecified future“ (sensu Ehrenfeld, 2000)—risk eroding the credibility of conservation paleobiology and widening the gap between science and practice. Credibility in conservation biology is a relational process, won by building trust between communities (Alagona, 2008). While some researchers may construe their work as improving our understanding of ecological processes relevant to conservation issues (after all, looking for near-term payoffs is a human trait), those outside the field may find statements only vaguely connected to actual conservation contexts unconvincing and overstated. In fact, overstating research implications may have the unintended effect of opening conservation paleobiology up to “damning critiques by the anti-environmentalist lobby…increas[ing] cynicism and complacency about...loss of biodiversity” (Ladle et al., 2005, p. 238). Avoiding this extreme scenario is relatively easy, however, because it only involves recognizing that research does not need to translate directly or immediately to conservation to be valuable (Stinchcombe et al., 2002; Linklater, 2003; Hulme, 2014), and resisting the temptation to tack on vapid claims if our work does not have concrete conservation recommendations.
Collaboration to navigate the knowing-doing gap
Our findings suggest that applied conservation paleobiology research is more likely to lead to conservation action when academic researchers collaborate with conservation practitioners. In our corpus, 113 studies included conservation co-authors: of these, 22 were action studies. This aligns with previous work in conservation: a literature review estimated that conservation genetics studies with non-academic authors were 2.5 times more likely to include specific conservation recommendations than studies with only academic authors (Britt et al., 2018). Wildlife biologists and wildlife managers report that directly involving practitioners in all stages of research increases the likelihood of implementing research results (Merkle et al., 2019). This focus on partnerships is a common theme in the literature on the knowing-doing gap (Bainbridge, 2014; Taft et al., 2020). However, co-authorship is not the only marker of engagement between academic researchers and conservation practitioners (Hogg et al., 2018). In fact, our survey found that author lists are not an accurate proxy for collaboration: over half (56.5% or 13 out of 23) of the survey respondents who had indicated that their study was considered by conservation practitioners in their work also reported co-designing the study with conservation practitioners (Supplementary Table 2), even though it was not apparent in the peer-reviewed publications based on author affiliations (i.e., only non-conservation authors). While collaborations may not appear in author lists, our survey confirms that conservation and non-conservation collaborations occur in conservation paleobiology outside of what is documented in peer-reviewed publications. Indeed, Hulme (2014, p. 1131) affirmed “publishing research in peer-reviewed journals will only ever be a small part of closing the knowing-doing gap.” In contrast, our survey suggests that knowledge exchange (or mutual learning) was incorporated into collaborations between researchers and conservation practitioners, which is largely invisible in the peer-reviewed studies we reviewed (Reed et al., 2014; Cvitanovic et al., 2016).
Action can lag behind publication. Our survey revealed that conservation impacts often emerged 1–3 years after research teams published their findings (Supplementary Figure 4 and Supplementary Table 2). Conservation biologists also note this time lag from research initiation to implementation, suggesting that the lag may explain why few studies bridge the planning stages from status review to implementation to action (Knight et al., 2008; Mair et al., 2018). Hogg et al. (2018) further argued that conservation recommendations are often published in the “gray literature” and not in the peer-reviewed literature (see also Savarese, 2018), or practitioners communicate with researchers they already know before searching peer-reviewed literature. The lag time we found between publication and action reflects active communication and collaboration between academic researchers and conservation practitioners beyond the boundaries of publication.
Conservation paleobiology bright spots
Although action studies represent a small slice of the studies we reviewed, we found bright spots (sensu Cvitanovic and Hobday, 2018) where conservation paleobiology research is leading to real-world conservation actions on the ground around the world (see also Dietl et al., 2015; Boyer et al., 2017; Dietl and Flessa, 2018; Savarese, 2018). Here we highlight a selection of these studies to illustrate where the dead have been put to work.
Researchers and forest managers in the Guadarrama National Park, Spain co-designed a study (Morales-Molino et al., 2017) that used pollen, conifer stomata, charcoal, and dung fungal spores (a proxy for herbivore grazing activity) preserved in 2,500-year-old peat cores to understand the natural range of variability of mountain oak-pine forests in response to disturbances such as grazing. The study provided relevant information for the forest managers who wanted to better understand the natural state of the habitats under their care (Morales-Molino et al., 2017). The forest managers responded to the results of the study, which showed that coppicing and overgrazing are detrimental disturbances to the mountain oak-pine forests in the Guadarrama National Park, by reducing the number of cattle and altering their management strategy (Morales-Molino et al., 2017).
In another study, plant and animal fossils from Makauwahi Cave Reserve (MCR), Kaua'i, Hawaii provided guidance for the successful restoration of land degraded by centuries of agricultural use and non-native introductions to a pre-European state dominated by native plants and Polynesian cultivars. Holocene fossil pollen evidence (Burney et al., 2001) suggested doubling the number of plant species to replant in restoration efforts by including now extinct species, justifying the translocation of closely related plant species from nearby islands to Kaua'i. Invasive plant removal, replanting, maintenance, and monitoring were done by reserve staff, local school children, and volunteers. Animal fossil evidence from MCR guided the design of a large-scale experiment to determine that ecological surrogates like non-native, pet tortoises that could no longer be cared for by their owners could fulfill the same ecological role of weed control—reducing understory plant cover—played out by now extinct herbivores, such as giant flightless ducks and geese (Figure 4A; Burney and Burney, 2016).
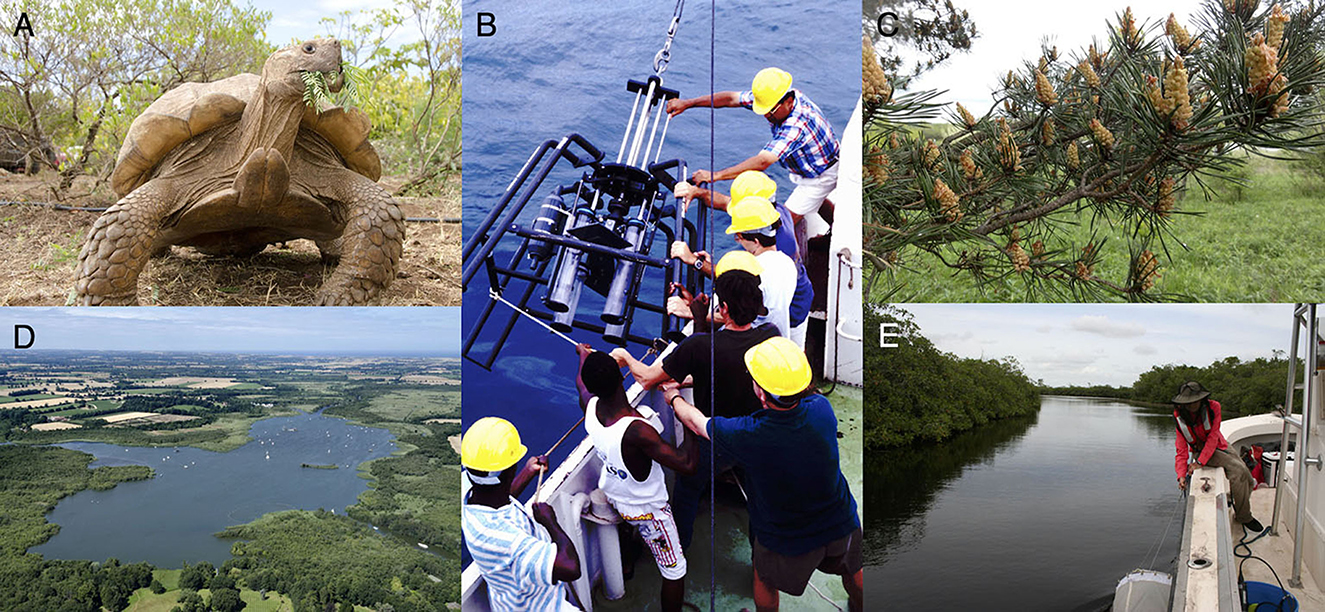
Figure 4. “Bright spots” examples of conservation paleobiology in action. (A) At Makauwahi Cave Reserve on Kaua‘i in the Hawaiian Islands, USA giant tortoises such as this African spurred tortoise (Centrochelys sulcata) act as surrogates for long-lost ecological functions by helping keep invasive grasses and weeds grazed down in securely fenced native plant restorations; photo credit: A. Burney. (B) Researchers deploying a Hedrick-Marrs Multicorer to capture sediments in Lake Tanganyika, Africa's oldest and deepest lake. The sediment cores, containing fish bones and scales, help decipher drivers of fish declines; photo credit: A. Cohen. (C) In Ireland, it was thought that Scots pine (Pinus sylvestris) became extinct, but fossil pollen and pine needle macrofossils demonstrate that a rare stand of Scots pine has persisted locally for centuries and is now used as a seed source for restoration efforts; photo credit: R. A. Nonenmacher; Wikimedia Commons. (D) Barton Broad is a shallow, eutrophic lake in the UK where management has targeted limiting nutrient and sediment influx from surrounding agricultural point sources. Plant macrofossil data from lake sediment cores suggest that only after reducing nutrient levels and providing habitat structure will aquatic vegetation recolonize; photo credit: John Fielding; Wikimedia Commons. (E) United States Geological Survey (USGS) researcher collecting living freshwater snails rafting out into the estuary by doing a plankton tow in Roberts River, Everglades National Park, Florida, USA; photo credit: USGS. The data on living mollusks from the plankton tows are compared to species abundances in sediment cores from the region to estimate past salinity conditions.
In Lake Tanganyika, Africa, fossils from multiple species of fish preserved in lake sediment cores determined that lake warming coincided with declines in economically important fish and their diatom food sources over the past 500 years. The new perspective provided by the past demonstrated to fishery managers and other stakeholders that declines in abundance occurred prior to intensification of contemporary commercial fishing practices in the lake, bringing clarity to the debate over the cause of the population declines (Figure 4B; Cohen et al., 2016). From our survey, we learned that this co-designed project involved working “…directly with UN Global Environmental Facility and Tanzania Fisheries Research Institute personnel in the development …[and]… execution of the research” (A.S. Cohen, personal communication). Also in freshwater environments, Madgwick et al. (2011) used an approach combining historical and macrophyte data with pollen and plant macrofossil data from ~200-year-old lake sediment cores to develop a restoration recommendation for a shallow, high-nutrient lake (Barton Broad, UK; Figure 4D), which is a popular tourist destination supporting the local economy. After three decades of unsuccessful lake restoration using techniques like sediment removal and nutrient reduction, the authors recommended prioritizing efforts to lower the nutrient status and build habitat structure appropriate for aquatic plant communities rather than an approach focused on using a list of aquatic plant species—compiled from anecdotes and historic documents—to restore the lake conditions (Madgwick et al., 2011). A government report for the Broads Authority, Norwich and Natural England on management activities at Broads National Park considered the paleolimnological evidence from Madgwick et al. (2011) by using the study to establish “indicators of restoration success” of aquatic plant species to restore more natural lake conditions (Phillips et al., 2015).
In the U.K., national conservation agencies (e.g., Countryside Council for Wales and Natural England) commissioned paleoecological studies to understand the history of mire degradation and inform conservation management, and decidedly “bridge the gap” (Chambers et al., 2013, 2017). In response to the national call for paleoecologists to collaborate with conservation managers, Chambers et al. (2017, p. 73) generated plant macrofossil, fossil pollen, and magnetic susceptibility data spanning the past 3,000 years in northern England. The geohistorical data impacted the design of action plans for the restoration of peat-forming plants via the translocation of plant species to replace species that are no longer present at the site. This collaboration between managers and researchers ensured that the data informed regional, national, and international agencies (e.g., Yorkshire Peat Partnership (YPP), Natural England, and the International Union for the Conservation of Nature). At West Arkengarthdale, another degraded peatland in the UK, a study funded by the YPP identified plant species that would encourage peatland development based on analyses of humification (a proxy for wet and dry environmental conditions), fossil pollen, and plant macrofossils from a 6,700-year-old peat record (McCarroll et al., 2016). The collaborative approach between researchers and practitioners described in McCarroll et al. (2016) ensured the practitioners' management decisions were informed by the local site history.
One action study, with a mix of conservation and non-conservation author affiliations, focused on an endemic terrestrial mammal, the Hispaniolan solenodon (Solenodon paradoxus; Gibson et al., 2019). By using a combination of fossil and contemporary data sets suggesting anthropogenic factors (rather than Pleistocene and Holocene climatic changes) reduced the geographic range of the Hispaniolan solenodon, Gibson et al. (2019) reported that communicating the results with their conservation partners allowed them to more precisely identify locations for targeted management approaches to rewild. Another action study that was focused on a specific species successfully determined the timing of arrival and slow spread of a disputed invasive plant species, the tree daisy (Olearia lyallii), in the sub-Antarctic Auckland Islands using fossil pollen from peat cores from the past millennium (Wilmshurst et al., 2015). The New Zealand Department of Conservation reacted to the study by continued monitoring of the plant “…rather than trying to eliminate it saving potentially thousands of dollars” (J. Wilmshurst, personal communication). In Ireland, documented implementation of recommendations generated from 6,000-year-old fossil pollen in a lake sediment core included propagating seeds from a rare stand of Scots pine (Pinus sylvestris; Figure 4C) at Rockforest Lough, which was once thought to be extinct in Ireland. The discovery of the Scots pine stand at Rockforest resulted in it becoming listed as a genetic conservation unit (McGeever and Mitchell, 2016), and identified as a seed source for planting Scots pine at two other sites by managers of the Irish National Parks and Wildlife Service (Roche et al., 2018; Roche, 2019).
In a synthesis analysis, monitoring of a network of 15 lakes in the National Park System (NPS) of the Upper Midwest Region, USA, indicated these lakes exceeded the nutrient reference criteria established by the U.S. Environmental Protection Agency. By coupling modern nutrient measurements with pre-1900s conditions based on diatom inferred total phosphorus, pH, and specific conductance from lake sediment cores, the study revealed that the nutrient status of many lakes had not changed historically (pre-1900s), except for shallow, small lakes in the region (VanderMeulen et al., 2016). The study, which was led and co-authored by NPS aquatic ecologists, provided information to refine reference criteria for smaller spatial scales, such as the NPS Great Lakes Inventory and Monitoring Network, one of the first NPS inventory and monitoring frames to combine modern and diatom-inferred water quality data.
In the Greater Everglades Ecosystem in Florida, USA (Figure 4E), the Comprehensive Everglades Restoration Plan (CERP), headed by the Army Corps of Engineers, brought together scientists and practitioners across state and federal levels to develop project teams that were required to produce performance measures and restoration targets. Marshall et al. (2014) developed a model that applied a modern analog dataset to mollusk assemblages from sediment cores to estimate past salinity conditions in Florida Bay to fill a pre-1900s information gap in historical rainfall and salinity records. Results from the study are being used by CERP's Restoration Coordination and Evaluation Southern Coastal Systems team to set targets for how much freshwater flow from the Everglades is needed to lower salinity conditions to a more natural state in the Florida Bay estuary, which has experienced hypersaline conditions since the early 1900s due to a waterflow deficit.
These bright spots underscore a common theme that emerged from our literature review and survey. A reliable route to producing actionable conservation paleobiology research is collaboration, whereby academic researchers and conservation practitioners work together to ensure research results meet the specific needs of conservation practitioners. Aspiring conservation paleobiologists could consult the growing body of literature on this important topic for additional guidance and recommendations on what to do and how to start (e.g., Knight et al., 2008; Cook et al., 2013; Hulme, 2014; Cvitanovic et al., 2016; Beier et al., 2017; Boyer et al., 2017; Savarese, 2018; Gerber et al., 2020).
Moving from potential to application in deep time
From our literature review, research in applied conservation paleobiology focuses almost completely on near-time scales (96%; Figure 2B), with most action studies being restricted to the Holocene (Supplementary Table 3). The scant number of deep-time applied studies in the corpus confirms that they are less systematically pursued by conservation paleobiologists than near-time studies (Dietl and Flessa, 2017; Dietl, 2019). Indeed, a recent survey of the conservation paleobiology community suggests that deep-time records are perceived by many conservation paleobiologists to be too far removed from application to be of immediate value to conservation practice (Dillon et al., 2022): 93% of survey respondents thought near-time scales were useful to conservation practice, in contrast to only 63% for the deep-time fossil record (see also Dietl et al., 2019; Kiessling et al., 2019). Looking to the distant past for guidance in solving today's conservation problems may seem like an unnatural act (Dietl, 2019), but the deep time record of the Earth's history contains abundant opportunity to evaluate pressing questions about biotic responses to a range of environmental stressors outside of our experience, especially unchecked climate change—one of the greatest threats to our ways of life and place in the natural world. Moving from potential to application in deep-time research, however, will require more than motivating participation and publishing additional studies, which, by itself, is not a guarantee of future application. Our findings suggest that a different strategy is more likely to work. Conservation paleobiologists could build collaborations with conservation practitioners and other stakeholders to identify specific decisions that can be informed by deep-time studies, and then jointly define the research questions, to better tailor their research to the immediate needs of the intended end-users.
Limitations
Our approach for assessing the knowing-doing gap in the applied conservation paleobiology literature is not without limitations. First, we note that our corpus was limited to English language peer-reviewed studies. Excluding non-English references could have introduced a geographic selection bias against other regions of the world (Amano et al., 2021). However, Dillon et al. (2022) did not find any non-English empirical research studies to include in their literature review of self-identified conservation paleobiology studies published over the last 20 years even though they were included in their search. They found conservation paleobiology research primarily originated in English-speaking countries (Dillon et al., 2022), which suggests that non-English publications may be rare in the field of conservation paleobiology and may have more to do with where conservation paleobiology studies are being conducted. Second, we recognize limitations to our assessment of the corpus. An uneven distribution of researchers and practitioners working in different ecosystems (terrestrial vs. freshwater vs. marine) or taxa (plants vs. others) may have introduced bias into our analysis of the proportion of action studies associated with each habitat type, ecological focus, proxy, and/or taxonomic focus. This limitation, however, does not affect how we measured the extent of the knowing-doing gap. Third, we did not account for a “clumping effect” in our analysis: there may be specific lab groups, researchers, or networks of researchers that are publishing prolifically in conservation paleobiology. If this clumping effect is real, and the trend in action-oriented work is limited to a small network of researchers, then our estimate of the gap for the applied conservation paleobiology community is, in fact, conservative.
Finally, other errors may have arisen due to a bias in the study design, which could lead to overestimation of the gap. For instance, bias may have been introduced if our search strategy, which targeted applied studies that made a direct connection to conservation, restoration, and management audiences, was inefficient and missed relevant studies from the broader conservation paleobiology community (sensu Dillon et al., 2022). But it is hard to imagine that this limitation of our sampling strategy would change our conclusion (in fact, including research aimed at discovery would likely only widen the gap), given that conservation practitioners often report that a large amount of basic conservation research focuses on issues that are not relevant to conservation practice (Fazey et al., 2005; Cook et al., 2013). We also did not search the gray literature, which may represent a significant proportion of the available documented support for conservation decisions (Linklater, 2003; Haddaway and Bayliss, 2015; Hogg et al., 2018), especially in the tropics where gray literature is often the only source of information available for particular areas or species (Corlett, 2011). Again, however, it is hard to imagine that this limitation would change our conclusions, given we are still in the early stages of the diffusion of the conservation paleobiology innovation. Most conservation practitioners are not aware of the approach (Durham and Dietl, 2015) or are still in the information gathering stage of how the approach might benefit their work. As the conservation paleobiology innovation continues to scale and is adopted by conservation practitioners (i.e., the approach is routinely mentioned in practitioner-generated research outputs—reports, government papers etc.—published in the gray literature), searching for and including action studies from this untapped pool of information will form a vital part of the evidence base for any future assessment of the knowing-doing gap in conservation paleobiology. Until that time comes, although peer-reviewed studies cannot fully capture the relationship between academic researchers and practitioners or research and action (e.g. Savarese, 2018), peer reviewed journals are the forum by which academic researchers communicate with each other and document their work, and thus are the best evidence available to assess the size and nature of the knowing-doing gap in conservation paleobiology.
After considering these limitations and potential biases, we remain confident that we have captured a robust, wide knowing-doing gap in conservation paleobiology, which is remarkably similar to conservation biology and other allied fields in the environmental sciences. The first step in closing the knowing-doing gap is understanding the magnitude of the problem.
Conclusion
Our goal to measure the knowing-doing gap in conservation paleobiology validates what most workers in the field already intuitively suspected—the existence of a sizeable gap between theory and practice. We also found encouraging bright spots where geohistorical data and insights are being used in myriad ways relevant to conservation practitioners, with actionable outcomes. Our findings about collaboration in particular highlight that successfully navigating the knowing-doing gap in conservation paleobiology occurs in spaces where conservation practitioners and researchers engage in active knowledge exchange, where paleontological research informs management actions and management needs inform paleontological research. We recommend deliberate engagement with conservation practitioners through collaborative research opportunities and communication beyond publication between academic researchers and conservation practitioners to build trust and increase the chances of producing actionable conservation paleobiology research. Moving forward, conservation paleobiologists could rally around community-driven initiatives like the Conservation Paleobiology Network2 and redouble efforts to mobilize training opportunities and support for this practice. The knowing-doing gap in conservation paleobiology is real, but not insurmountable.
Data availability statement
All datasets used in this study can be found in the Supplementary material.
Author contributions
DG, CM, and GD conceived the idea. DG, CM, JP, AS, and GD designed the study. DG, JP, and AS compiled the dataset. DG and JP conducted the analyses. DG led the writing of the manuscript, with contributions from GD, JP, and CM. All authors contributed to the content and editing of the manuscript and approved the final version.
Funding
Funding for this study was provided by the Roy J. Shlemon Center for Quaternary Studies, University of Wyoming.
Acknowledgments
We are grateful for research assistance from Matthew Goldsmith, Tiana Issa, and Paige Bober. We thank Jacquelyn Gill for initial conversations sparking our research question, Bryan Shuman and John Handley for helpful data analysis discussions, and the reviewers whose comments helped improve the manuscript. We also thank Andrew Cohen, David Burney, Janet Wilmshurst, and Lynn Wingard for generously providing photos relevant to action studies and/or granting permission to cite their survey responses as personal communications.
Conflict of interest
The authors declare that the research was conducted in the absence of any commercial or financial relationships that could be construed as a potential conflict of interest.
Publisher's note
All claims expressed in this article are solely those of the authors and do not necessarily represent those of their affiliated organizations, or those of the publisher, the editors and the reviewers. Any product that may be evaluated in this article, or claim that may be made by its manufacturer, is not guaranteed or endorsed by the publisher.
Supplementary material
The Supplementary Material for this article can be found online at: https://www.frontiersin.org/articles/10.3389/fevo.2023.1058992/full#supplementary-material
Supplementary Data Sheet 1. Dataset of Web of Science search results including article categorizations made during pre-screening (n = 1534).
Supplementary Data Sheet 2. Corpus of articles (n = 444) that were coded.
Supplementary Data Sheet 3. Response data for three survey questions.
Supplementary Figure 1. PRISMA flow diagram for identification of studies included in the corpus.
Supplementary Figure 2. Proportion of studies categorized by (A) ecological focus, (B) type of environment, (C) types of proxies, and (D) taxonomic focus across three documented implementation categories.
Supplementary Figure 3. Impact measures plotted by publication year from 2010 to 2020 (July 13, 2020). (A) Total number of studies included in the corpus. (B) Proportion of action studies relative to the total number of studies in the corpus. (C) Proportion of action and theory-specific studies with mixed conservation and non-conservation author affiliations relative to total number of studies in the corpus.
Supplementary Figure 4. Time lag from time of publication to impact reported by survey respondents.
Supplementary Table 1. Kappa-Fleiss results for articles included in training sets.
Supplementary Table 2. Results for three survey questions.
Supplementary Table 3. Number of studies in each documented implementation category coded for all coded categories.
Supplementary Table 4. Number of studies in invertebrate and vertebrate groupings for each documented implementation category.
Supplementary Table 5. Results from Chi-squared and Fisher's Exact tests for documented implementation versus author affiliation, taxonomic focus, environment, type of proxy, ecological focus, and timescale.
Footnotes
1. ^Authors of action studies that responded to our survey served as an independent check of the suitability of the criteria (Table 2) we used to identify action studies in the literature review. All authors confirmed that the findings from their study had been used or considered by conservation practitioners.
References
Alagona, P. S. (2008). Credibility. Conserv. Biol. 22, 1365–1367. doi: 10.1111/j.1523-1739.2008.01109.x
Amano, T., Berdejo-Espinola, V., Christie, A. P., Willott, K., Akasaka, M., Báldi, A., et al. (2021). Tapping into non-English-language science for the conservation of global biodiversity. PLoS Biol. 19, e3001296. doi: 10.1371/journal.pbio.3001296
Arlettaz, R., Schaub, M., Fournier, J., Reichlin, T. S., Sierro, A., Watson, J. E. M., et al. (2010). From publications to public actions: when conservation biologists bridge the gap between research and implementation. BioScience 60, 835–842. doi: 10.1525/bio.2010.60.10.10
Bainbridge, I. (2014). Practitioner's perspective: how can ecologists make conservation policy more evidence based? Ideas and examples from a devolved perspective. J. Appl. Ecol. 51, 1153–1158. doi: 10.1111/1365-2664.12294
Beier, P., Hansen, L. J., Helbrecht, L., and Behar, D. (2017). A how-to guide for coproduction of actionable science. Conserv. Lett. 10, 288–296. doi: 10.1111/conl.12300
Blundell, A., and Holden, J. (2015). Using palaeoecology to support blanket peatland management. Ecol. Indic. 49, 110–120. doi: 10.1016/j.ecolind.2014.10.006
Boyer, A. G., Brenner, M., Burney, D. A., Pandolfi, J. M., Savarese, M., Dietl, G. P., et al. (2017). “Conservation paleobiology roundtable: from promise to application” in Conservation paleobiology: Science and Practice, eds. G. P. Dietl and K. W. Flessa (Chicago: University of Chicago Press), 291–302.
Britt, M., Haworth, S. E., Johnson, J. B., Martchenko, D., and Shafer, A. B. A. (2018). The importance of non-academic coauthors in bridging the conservation genetics gap. Biol. Conserv. 218, 118–123. doi: 10.1016/j.biocon.2017.12.019
Burbidge, A. A., and Wallace, K. J. (1995). “Practical methods for conserving biodiversity” in Conserving biodiversity: threats and solutions, eds. R. A. Bradstock, T. D. Auld, D. A. Keith, R. T. Kingsford, D. Lunney, and D. P. Sivertsen (Sidney: Surrey Beatty and Sons), 11–26.
Burney, D. A., and Burney, L. P. (2016). Monitoring results from a decade of native plant translocations at Makauwahi Cave Reserve, Kaua‘i. Plant Ecol. 217, 139–153. doi: 10.1007/s11258-015-0535-z
Burney, D. A., James, H. F., Burney, L. P., Olson, S. L., Kikuchi, W., Wagner, W. L., et al. (2001). Fossil evidence for a diverse biota from Kaua‘i and its transformation since human arrival. Ecol. Monogr. 71, 615–641. doi: 10.1890/0012-9615(2001)071[0615:FEFADB]2.0.CO;2
Chambers, F., Crowle, A., Daniell, J., Mauquoy, D., McCarroll, J., Sanderson, N., et al. (2017). Ascertaining the nature and timing of mire degradation: using palaeoecology to assist future conservation management in Northern England. AIMS Environ. Sci. 4, 54–82. doi: 10.3934/environsci.2017.1.54
Chambers, F. M., Cloutman, E. W., Daniell, J. R. G., Mauquoy, D., and Jones, P. S. (2013). Long-term ecological study (palaeoecology) to chronicle habitat degradation and inform conservation ecology: an exemplar from the Brecon Beacons, South Wales. Biodivers. Conserv. 22, 719–736. doi: 10.1007/s10531-013-0441-4
Cohen, A. S., Gergurich, E. L., Kraemer, B. M., McGlue, M. M., McIntyre, P. B., Russell, J. M., et al. (2016). Climate warming reduces fish production and benthic habitat in Lake Tanganyika, one of the most biodiverse freshwater ecosystems. Proc. Natl. Acad. Sci. U. S. A. 113, 9563–9568. doi: 10.1073/pnas.1603237113
Cook, C. N., Mascia, M. B., Schwartz, M. W., Possingham, H. P., and Fuller, R. A. (2013). Achieving conservation science that bridges the knowledge–action boundary. Conserv. Biol. 27, 669–678. doi: 10.1111/cobi.12050
Cook, C. N., and Sgrò, C. M. (2017). Aligning science and policy to achieve evolutionarily enlightened conservation. Conserv. Biol. 31, 501–512. doi: 10.1111/cobi.12863
Corlett, R. T. (2011). Trouble with the gray literature. Biotropica 43, 3–5. doi: 10.1111/j.1744-7429.2010.00714.x
Cvitanovic, C., and Hobday, A. J. (2018). Building optimism at the environmental science-policy-practice interface through the study of bright spots. Nat. Commun. 9, 3466. doi: 10.1038/s41467-018-05977-w
Cvitanovic, C., McDonald, J., and Hobday, A. J. (2016). From science to action: principles for undertaking environmental research that enables knowledge exchange and evidence-based decision-making. J. Environ. Manage. 183, 864–874. doi: 10.1016/j.jenvman.2016.09.038
Dietl, G. P. (2019). Conservation palaeobiology and the shape of things to come. Philos. Trans. Royal Soc. B. 374, 0190294. doi: 10.1098/rstb.2019.0294
Dietl, G. P., and Flessa, K. W. (2009). Conservation paleobiology: using the past to manage for the future. Paleontol. Soc. Pap. 15, 285.
Dietl, G. P., and Flessa, K. W. (2011). Conservation paleobiology: putting the dead to work. Trends Ecol. E26, 30–37. doi: 10.1016/j.tree.2010.09.010
Dietl, G. P., and Flessa, K. W. (2017). Conservation Paleobiology: Science and Practice. Chicago: University of Chicago Press.
Dietl, G. P., and Flessa, K. W. (2018). “Should conservation paleobiologists save the world on their own time?” in Marine Conservation Paleobiology, eds. C. L. Tyler and C. L. Schneider (Cham: Springer International Publishing), 11–22.
Dietl, G. P., Kidwell, S. M., Brenner, M., Burney, D. A., Flessa, K. W., Jackson, S. T., et al. (2015). Conservation paleobiology: leveraging knowledge of the past to inform conservation and restoration. Annu. Rev. Earth Pl. Sc. 43, 79–103. doi: 10.1146/annurev-earth-040610-133349
Dietl, G. P., Smith, J. A., and Durham, S. R. (2019). Discounting the past: The undervaluing of paleontological data in conservation science. Front. Ecol. Evolut. 7, 108 doi: 10.3389/fevo.2019.00108
Dillon, E. M., Pier, J. Q., Smith, J. A., Raja, N. B., Dimitrijevi,ć, D., Austin, E. L., et al. (2022). What is conservation paleobiology? Tracking 20 years of research and development. Front. Ecol. Evol. 10, 1117. doi: 10.3389/fevo.2022.1031483
Durham, S. R., and Dietl, G. P. (2015). Perspectives on geohistorical data among oyster restoration professionals in the United States. J. Shellfish Res. 34, 227–239. doi: 10.2983/035.034.0204
Ehrenfeld, D. (2000). War and peace and conservation biology. Conserv. Biol. 14, 105–112. doi: 10.1046/j.1523-1739.2000.99325.x
Esler, K. J., Prozesky, H., Sharma, G. P., and McGeoch, M. (2010). How wide is the “knowing-doing” gap in invasion biology? Biol. Invasions 12, 4065–4075. doi: 10.1007/s10530-010-9812-x
Fazey, I., Fischer, J., and Lindenmayer, D. B. (2005). What do conservation biologists publish? Biol. Conserv. 124, 63–73. doi: 10.1016/j.biocon.2005.01.013
Fuller, R. A., Lee, J. R., and Watson, J. E. M. (2014). Achieving open access to conservation science. Conserv. Biol. 28, 1550–1557. doi: 10.1111/cobi.12346
Gerber, L. R., Barton, C. J., Cheng, S. H., and Anderson, D. (2020). Producing actionable science in conservation: best practices for organizations and individuals. Conserv. Sci. Pract. 2, e295. doi: 10.1111/csp2.295
Gibbons, D. W., Wilson, J. D., and Green, R. E. (2011). Using conservation science to solve conservation problems. J. Appl. Ecol. 48, 505–508. doi: 10.1111/j.1365-2664.2011.01997.x
Gibson, L. M., Mychajliw, A. M., Leon, Y., Rupp, E., and Hadly, E. A. (2019). Using the past to contextualize anthropogenic impacts on the present and future distribution of an endemic Caribbean mammal. Conserv. Biol. 33, 500–510. doi: 10.1111/cobi.13290
Habel, J. C., Gossner, M. M., Meyer, S. T., Eggermont, H., Lens, L., Dengler, J., et al. (2013). Mind the gaps when using science to address conservation concerns. Biodivers. Conserv. 22, 2413–2427. doi: 10.1007/s10531-013-0536-y
Haddaway, N. R., and Bayliss, H. R. (2015). Shades of grey: two forms of grey literature important for reviews in conservation. Biol. Conserv. 191, 827–829. doi: 10.1016/j.biocon.2015.08.018
Hogg, C. J., Taylor, H. R., Fox, S., and Grueber, C. E. (2018). Response to Britt et al. 2018 “The importance of non-academic co-authors in bridging the conservation genetics gap” Biological Conservation 218, 118–123. Biol. Conserv. 222, 287–288. doi: 10.1016/j.biocon.2018.04.035
Hulme, P. E. (2014). Bridging the knowing–doing gap: know-who, know-what, know-why, know-how and know-when. J. Appl. Ecol. 51, 1131–1136. doi: 10.1111/1365-2664.12321
Hunter, M. L., Jacobson, G. L., and Webb, III, T. (1988). Paleoecology and the coarse-filter approach to maintaining biological diversity. Conserv. Biol. 2, 375–385. doi: 10.1111/j.1523-1739.1988.tb00202.x
Kelley, P. H., and Dietl, G. P. (2022). Core competencies for training conservation paleobiology students in a wicked world. Front. Ecol. E10, 851014. doi: 10.3389/fevo.2022.851014
Kelley, P. H., Dietl, G. P., and Visaggi, C. C. (2019). Model for improved undergraduate training in translational conservation science. Conserv. Sci. Pract. 1, e5. doi: 10.1002/csp2.5
Kiessling, W., Raja, N. B., Roden, V. J., Turvey, S. T., and Saupe, E. E. (2019). Addressing priority questions of conservation science with palaeontological data. Philos. T. Roy. Soc. B. 374, 20190222. doi: 10.1098/rstb.2019.0222
Knight, A. T., Cowling, R. M., Rouget, M., Balmford, A., Lombard, A. T., Campbell, B. M., et al. (2008). Knowing but not doing: selecting priority conservation areas and the research–implementation gap. Conserv. Biol. 22, 610–617. doi: 10.1111/j.1523-1739.2008.00914.x
Ladle, R. J., Jepson, P., and Whittaker, R. J. (2005). Scientists and the media: the struggle for legitimacy in climate change and conservation science. Interdiscipl. Sci. Rev. 30, 231–240. doi: 10.1179/030801805X42036
Landis, J. R., and Koch, G. G. (1977). The measurement of observer agreement for categorical data. Biometrics 33, 159–174. doi: 10.2307/2529310
Linklater, W. L. (2003). Science and management in a conservation crisis: a case study with rhinoceros. Conserv. Biol. 17, 968–975. doi: 10.1046/j.1523-1739.2003.01449.x
Madgwick, G., Emson, D., Sayer, C. D., Willby, Nigel,., Rose, N. L., et al. (2011). Centennial-scale changes to the aquatic vegetation structure of a shallow eutrophic lake and implications for restoration. Freshwater Biol. 56, 2620–2636. doi: 10.1111/j.1365-2427.2011.02652.x
Mair, L., Mill, A. C., Robertson, P. A., Rushton, S. P., Shirley, M. D. F., Rodriguez, J. P., et al. (2018). The contribution of scientific research to conservation planning. Biol. Conserv. 223, 82–96. doi: 10.1016/j.biocon.2018.04.037
Marshall, F. E., Wingard, G. L., and Pitts, P. A. (2014). Estimates of natural salinity and hydrology in a subtropical estuarine ecosystem: implications for Greater Everglades restoration. Estuaries Coast 37, 1449–1466. doi: 10.1007/s12237-014-9783-8
Matzek, V., Covino, J., Funk, J. L., and Saunders, M. (2014). Closing the knowing–doing gap in invasive plant management: accessibility and interdisciplinarity of scientific research. Conserv. Lett. 7, 208–215. doi: 10.1111/conl.12042
McCarroll, J., Chambers, F. M., Webb, J. C., and Thom, T. (2016). Using palaeoecology to advise peatland conservation: An example from West Arkengarthdale, Yorkshire, UK. J. Nat. Conserv. 30, 90–102. doi: 10.1016/j.jnc.2016.02.002
McGeever, A. H., and Mitchell, F. J. G. (2016). Re-defining the natural range of Scots Pine (Pinus sylvestris L.): a newly discovered microrefugium in western Ireland. J. Biogeogr. 43, 2199–2208. doi: 10.1111/jbi.12761
Merkle, J. A., Anderson, N. J., Baxley, D. L., Chopp, M., Gigliotti, L. C., Gude, J. A., et al. (2019). A collaborative approach to bridging the gap between wildlife managers and researchers. J. Wildlife Manage. 83, 1644–1651. doi: 10.1002/jwmg.21759
Morales-Molino, C., Colombaroli, D., Valbuena-Carabaña, M., Tinner, W., Salomón, R. L., Carrión, J. S., et al. (2017). Land-use history as a major driver for long-term forest dynamics in the Sierra de Guadarrama National Park (central Spain) during the last millennia: implications for forest conservation and management. Global Planet. Change 152, 64–75. doi: 10.1016/j.gloplacha.2017.02.012
National Research Council (2005). The Geological Record of Ecological Dynamics: Understanding the Biotic Effects of Future Environmental Change. Washington, D. C.: The National Academies Press.
Page, M. J., McKenzie, J. E., Bossuyt, P. M., Boutron, I., Hoffmann, T. C., Mulrow, C. D., et al. (2021). The PRISMA 2020 statement: an updated guideline for reporting systematic reviews. Int. J. Surg. 88, 105906. doi: 10.1016/j.ijsu.2021.105906
Phillips, G., Bennion, H., Perrow, M. R., Sayer, C. D., Spears, B. M., Willby, N., et al. (2015). A review of lake restoration practices and their performance in the Broads National Park 1980–2013. Report for Broads Authority, Norwich and Natural England.
Pullin, A. S., Knight, T. M., Stone, D. A., and Charman, K. (2004). Do conservation managers use scientific evidence to support their decision-making? Biol. Conserv. 119, 245–252. doi: 10.1016/j.biocon.2003.11.007
R Core Team (2022). R: A Language and Environment for Statistical Computing. Available at: http://www.rproject.org/ (accessed September 07, 2022).
Redford, K., and Taber, A. (2000). Writing the wrongs: developing a safe-fail culture in conservation. Conserv. Biol. 14, 1567–1568. doi: 10.1046/j.1523-1739.2000.01461.x
Reed, M. S., Stringer, L. C., Fazey, I., Evely, A. C., and Kruijsen, J. H. J. (2014). Five principles for the practice of knowledge exchange in environmental management. J. Environ. Manage. 146, 337–345. doi: 10.1016/j.jenvman.2014.07.021
Roche, J. R. (2019). Recent findings on the native status and vegetation ecology of Scots pine in Ireland and their implications for forestry policy and management. Irish For. 76, 29–54. Available online at: https://journal.societyofirishforesters.ie/index.php/forestry/article/view/10950 (accessed January 18, 2023).
Roche, J. R., Mitchell, F. J. G., Waldren, S., and Stefanini, B. S. (2018). Palaeoecological evidence for survival of Scots Pine through the late Holocene in western Ireland: implications for ecological management. Forests 9, 350. doi: 10.3390/f9060350
Savarese, M. (2018). “Effectively connecting conservation paleobiological research to environmental management: examples from Greater Everglades' restoration of southwest Florida” in Marine Conservation Paleobiology, eds. C. L. Tyler and C. L. Schneider (Cham: Springer), 55–73.
Smith, J. A., Durham, S.R., and Dietl, G. P. (2018). “Conceptions of long-term data among marine conservation biologists and what conservation paleobiologists need to know,” in Marine Conservation Paleobiology, eds C. L. Tyler and C. L. Schneider (Cham: Springer Nature), 23–54. doi: 10.1007/978-3-319-73795-9_3
Stinchcombe, J., Moyle, L., Hudgens, B., Bloch, P., Chinnadurai, S., Morris, W., et al. (2002). The influence of the academic conservation biology literature on endangered species recovery planning. Conserv. Ecol. 6, 2. doi: 10.5751/ES-00444-060215
Taft, H. R., McCoskey, D. N., Miller, J. M., Pearson, S. K., Coleman, M. A., Fletcher, N. K., et al. (2020). Research–management partnerships: An opportunity to integrate genetics in conservation actions. Conserv. Sci. Pract. 2, e218. doi: 10.1111/csp2.218
Taylor, H. R., Dussex, N., and van Heezik, Y. (2017). Bridging the conservation genetics gap by identifying barriers to implementation for conservation practitioners. Glob. Ecol. Conserv. 10, 231–242. doi: 10.1016/j.gecco.2017.04.001
Tyler, C. L. (2018). “A conceptual map of conservation paleobiology: visualizing a discipline” in Marine Conservation Paleobiology Topics in Geobiology, eds. C. L. Tyler and C. L. Schneider (Cham: Springer), 227–254.
VanderMeulen, D. D., Moraska Lafrancois, B., Edlund, M. B., Ramstack Hobbs, J. M., and Damstra, R. (2016). Pairing modern and paleolimnological approaches to evaluate the nutrient status of lakes in Upper Midwest National Parks. J. Am. Water Resour. Assoc. 52, 1401–1419. doi: 10.1111/1752-1688.12458
Williams, D. R., Balmford, A., and Wilcove, D. S. (2020). The past and future role of conservation science in saving biodiversity. Conserv. Lett. 13, e12720. doi: 10.1111/conl.12720
Williams, M. A., and Baker, W. L. (2012). Spatially extensive reconstructions show variable-severity fire and heterogeneous structure in historical western United States dry forests. Glob. Ecol. Biogeogr. 21, 1042–1052. doi: 10.1111/j.1466-8238.2011.00750.x
Wilmshurst, J. M., McGlone, M. S., and Turney, C. S. M. (2015). Long-term ecology resolves the timing, region of origin and process of establishment for a disputed alien tree. AoB Plants 7, plv104. doi: 10.1093/aobpla/plv104
Keywords: boundary science, conservation bright spots, paleoecology, research-implementation gap, resource management, restoration, systematic assessment
Citation: Groff DV, McDonough MacKenzie C, Pier JQ, Shaffer AB and Dietl GP (2023) Knowing but not doing: Quantifying the research-implementation gap in conservation paleobiology. Front. Ecol. Evol. 11:1058992. doi: 10.3389/fevo.2023.1058992
Received: 30 September 2022; Accepted: 10 January 2023;
Published: 09 February 2023.
Edited by:
Pasquale Raia, University of Naples Federico II, ItalyReviewed by:
Cheng-Hsiu Tsai, National Taiwan University, TaiwanCarrie Tyler, University of Nevada, Las Vegas, United States
Copyright © 2023 Groff, McDonough MacKenzie, Pier, Shaffer and Dietl. This is an open-access article distributed under the terms of the Creative Commons Attribution License (CC BY). The use, distribution or reproduction in other forums is permitted, provided the original author(s) and the copyright owner(s) are credited and that the original publication in this journal is cited, in accordance with accepted academic practice. No use, distribution or reproduction is permitted which does not comply with these terms.
*Correspondence: Dulcinea V. Groff, ZHVsY2luZWF2Z3JvZmZAZ21haWwuY29t; Gregory P. Dietl,
Z3BkM0Bjb3JuZWxsLmVkdQ==