- 1Dauphin Island Sea Lab, Dauphin Island, AL, United States
- 2Department of Biology, Stokes School of Marine and Environmental Sciences, University of South Alabama, Mobile, AL, United States
- 3Department of Biology, California State University Channel Islands, Camarillo, CA, United States
Changing climate conditions are well documented to affect species distribution patterns and migratory phenology, especially for thermally constrained species. Climate induced changes and other natural and anthropogenic factors may affect habitats heterogeneously, altering microhabitats that act as refugia. Here, we used a thermally constrained marine mammal, the West Indian manatee (Trichechus manatus), as a model species to examine how the availability of thermal microrefugia combined with climate driven increases in regional water temperatures may affect the timing and duration of occurrence at the northern margins of the species’ range. We used aerial, thermographic imaging to identify potential thermal anomalies that could act as thermal microrefugia for manatees during unfavorable cold temperatures and assessed manatee occurrence at these sites using citizen-sourced manatee sightings and stranding response data. To further understand how regional and longer-term water temperatures may affect the use of thermal microrefugia and phenology of migration, we compared spatial and temporal distributions of manatee sightings to air and sea surface temperatures on a decadal scale. Thermal anomalies were detected at various sources, and documented manatee sightings at or near these sites support use as thermal microrefugia during cold periods. Cold season manatee sightings at a known western migratory endpoint (Alabama waters) have increased during the last decade, primarily through increased sightings during the late fall and early winter (Nov–Jan) that correspond to increased regional temperatures during the same period. Manatees may use thermal microrefugia to remain at northern latitudes longer, delaying seasonal migrations or overwintering as conditions allow. Climate change is likely to have further effects on the species’ distribution and migration patterns, potentially facilitating modern range expansion that has implications for management and recovery actions for manatees across their range. Our study provides novel insight for manatees but may also be used as a model to understand how other thermally constrained species may expand their ranges into higher latitudes.
1 Introduction
The effects of global climate change on species distributions and habitat selection are broadly documented for terrestrial and aquatic species (e.g., Vergés et al., 2014; Osland et al., 2021). Species distribution models that predict responses to climate change traditionally assume homogenous climate conditions along latitudinal boundaries; however, an increasing body of literature emphasizes the importance of heterogeneity within a species’ range (Thomas et al., 2001; Lembrechts et al., 2019). For example, differences in topography, forest cover, and urbanization in terrestrial habitats and wind, tides, and upwelling in aquatic environments can lead to variability in the microclimatic conditions a species experiences across its distribution (Doniol-Valcroze et al., 2007; Schofield et al., 2009; Lembrechts et al., 2019; Bosso et al., 2022; Müller et al., 2023). This variability has the potential to create microhabitats, which can be selectively used by organisms to improve reproductive success, increase access to prey, avoid predators, or thermoregulate (Doniol-Valcroze et al., 2007; Schofield et al., 2009; Petty et al., 2012; Searcy et al., 2013; Müller et al., 2023). Under changing climate conditions, microhabitats may function as microrefugia, defined as small areas with favorable environmental conditions in which some populations may persist while protected from the broader unfavorable landscape (Rull, 2009; Hannah et al., 2014; Lembrechts et al., 2019). A series of microrefugia may then act as stepping-stones to mediate species’ range shifts, but individual plasticity and local adaptations among populations will influence the extent of distribution shifts under future climate regimes (Hannah et al., 2014; DeMarche et al., 2018).
For migratory species, changing climate conditions not only influence spatial distribution, but also the phenology of migratory activities. For example, dozens of species of migratory birds have been documented changing their total speed of migration to arrive earlier at warmer breeding sites (Schmalijohann and Both, 2017). Changes in total migration speed are primarily linked to earlier departures from winter, non-breeding sites or shorter stop-over periods at refueling sites with abundant food resources (Seebacher and Post, 2015; Schmalijohann and Both, 2017). For these species, changes in migration phenology can have significant consequences on synchrony with favorable habitat conditions and food availability (Mayor et al., 2017). For example, as migratory bird species shift to earlier breeding and nesting dates, they exhibit mismatched timing to peak vegetation and associated insect biomass, reducing prey availability (Visser and Both, 2005; Moore, 2011; Mayor et al., 2017). Conversely, similar species or populations of the same species have not changed the timing of their migration and nesting activities in the face of climate change, illustrating that populations or individuals within the same or similar species may react differently to changing climate conditions (Visser and Both, 2005; Moore, 2011; Neate-Clegg and Tingley, 2022). Other species of birds and insects have ceased migrations in recent years and instead remain year-round at mid-latitude sites that were previously used as stop-over locations (Groepper et al., 2008; Pulito and Berthold, 2010; Moore, 2011; Satterfield et al., 2018). Warming temperatures and mild winters likely increase the duration of annual productivity and resource availability, allowing for year-round survival and migration cessation for some species (Pulito and Berthold, 2010; Moore, 2011). Experimental studies have shown that changes in migratory timing and distance as well as reductions in migratory behavior can occur over short time periods (< 15 years), demonstrating the potential for rapid response of migratory species to changing climate conditions (Pulito and Berthold, 2010).
In the United States (USA), the West Indian manatee (Trichechus manatus; hereafter manatee) is a vulnerable marine mammal species that is thought to comprise a single population of individuals employing various strategies of residency and movement. The species is known to use partial migration, including seasonal short and long-distance movements, making it an ideal study species to examine variation in migratory phenology under changing climate conditions (Deutsch et al., 2008; Cloyed et al., 2021). Manatee distribution within the USA is limited by physiological intolerance to sustained water temperatures < 20°C, traditionally restricting the species’ winter range to peninsular Florida (Irvine, 1983; Bossart et al., 2003). Manatees exposed to cold water temperatures for extended time periods experience cold stress syndrome, with symptoms including skin lesions, malnutrition, and decreased immune function (Bossart et al., 2003; Walsh et al., 2005; Martony et al., 2019). To survive sustained cold periods, manatees use microrefugia with favorable thermal conditions compared to the surrounding environment. For management purposes, such thermal microrefugia are categorized as primary sites, which are well-established to have high manatee use and water temperatures consistently > 20°C, or secondary sites, which have low or unpredictable manatee use and inconsistent water temperatures, especially during severe cold events (Valade et al., 2020). Types of thermal microrefugia typically used by manatees in peninsular Florida include warm water discharges created by industrial facilities, primarily power plants, or natural springs and passive thermal basins that retain heat without a constant inflow of warm water (Laist and Reynolds, 2005a, b; Laist et al., 2013; Valade et al., 2020). During warmer months, manatees disperse from winter refuge sites to access food and other resources throughout their range (Deutsch et al., 2003; Cummings et al., 2014; Hieb et al., 2017; Cloyed et al., 2021).
Between the 1940s and 1970s, newly built power plants in peninsular Florida provided artificial warm water refuge, acting as thermal microrefugia for manatees in areas where the species had not previously occurred. These microrefugia allowed for stepping-stone movements to previously unused sites at more northern latitudes (Laist and Reynolds, 2005a). Correspondingly, sightings of manatees in northern Florida and states west and north of Florida also increased (Fertl et al., 2005; Cummings et al., 2014; Hieb et al., 2017), and manatees are now documented as regular seasonal visitors to these areas (Hieb et al., 2017; Cloyed et al., 2021). Few studies, however, have examined migration and habitat selection in these regions (Deutsch et al., 2003; Cloyed et al., 2021; Cloyed et al., 2022). In particular, no studies have attempted to identify thermal microrefugia outside of Florida that may affect the phenology of seasonal manatee migrations. Understanding range-wide movements under current and future climate conditions will be essential to guide management and recovery efforts for thermally constrained species like manatees.
In this study, manatees serve as a model for thermally constrained migratory species whose distributions are influenced by natural and anthropogenic variation in thermal conditions and for which climate change is likely to further affect the species’ migration patterns and distribution. We examined how thermal microrefugia combined with climate driven increases in regional water temperatures outside of Florida may affect the timing of manatee occurrence and duration of habitat use in the northern Gulf of Mexico (nGOM), a temperate region at the traditional northwestern range margin for manatees. Historically, most manatees were thought to migrate to the nGOM during spring (Apr–May) and depart in the autumn (Sep–Oct), spending the colder months of the year (historically Nov–Mar) in peninsular Florida when nGOM water temperatures drop below 20°C (Hieb et al., 2017; Cloyed et al., 2021). We used aerial thermographic imaging to identify potential warm-water sites that could act as thermal microrefugia for manatees during unfavorable winter conditions and drive changes in the timing of migratory movements. We then defined the timing of potential manatee use of these and nearby sites using citizen-sourced manatee sightings and stranding response data. To relate use of thermal refugia to regional and longer-term water temperatures, we compared temporal distributions of manatee sightings to nGOM air and sea surface temperatures on a decadal scale.
2 Materials and methods
2.1 Study site
Coastal Alabama is a known migratory stopover and endpoint for many manatees on the nGoM coast (Cloyed et al., 2021). Manatee sightings in Alabama waters have increased rapidly in recent years, and manatees are documented to extensively use the waters of the Mobile-Tensaw River Delta, Mobile Bay, and associated nearshore, shallow habitats with ready access to submerged aquatic vegetation (Hieb et al., 2017; Cloyed et al., 2022). Coastal Alabama has a subtropical climate with average minimum temperatures during January (~10°C) and maximum temperatures during July (~28°C). Accordingly, manatee sightings have been documented year-round, with the timing and location of manatee sightings strongly linked to temperature (Hieb et al., 2017; Cloyed et al., 2022). There are a limited number of known but poorly studied natural or man-made warm water sources that have potential to serve as cold season microrefugia for manatees on the Alabama coast. Known warm water sources include Magnolia Springs, a small natural spring at the headwaters of the Magnolia River that drains into southeastern Mobile Bay; the Clifton C. Williams wastewater treatment plant, which is the major treatment facility for the city of Mobile, processing ~30 million gallons of wastewater daily, with an outfall in northeastern Mobile Bay; and the James M. Barry Electric Generating Plant (Barry Steam Plant), which is a coal and natural gas-fired electrical plant that discharges heated cooling water into the Mobile River via a man-made canal located ~35 km north of Mobile Bay.
2.2 Detecting and defining thermal microrefugia
A total of four aerial thermographic surveys were completed during 2016 using a Cessna 172 single engine aircraft flown at an altitude of ~230–320 m and air speed of ~130–185 km hr−1 by an experienced wildlife survey pilot. To capture maximum thermal contrast between ambient waters and potential warm or cool water sources, surveys were flown during cold (Jan, Mar, Dec) and warm (Aug) seasons. Flight paths covered known areas of manatee habitat in Mobile Bay, Perdido Bay, and the Mobile-Tensaw River Delta along major rivers and subembayments of coastal Alabama, USA (Figure S1). Previously identified warm water sources, including Magnolia Springs, the Clifton C. Williams wastewater treatment plant, and the James M. Barry Electric Generating Plant (Barry Steam Plant) were targeted along flight paths as possible microrefugia (Figure S1). Start and end times, refueling events, GPS track lines, air temperature, altitude, and relative humidity were recorded for each survey (Table S1). Surveys during Jan, Mar, and Dec were completed in one day, while the Aug survey was completed during two days. Survey duration was ≤ 6 hrs airtime per day.
Thermal and corresponding standard images were captured by a photographer in the aircraft using a FLIR T620 infrared camera with georeferencing capabilities and a detector resolution of 640 x 480 pixels (FLIR, Wilsonville, Oregon, USA). Object parameters for the thermal camera including emissivity, reflective temperature, exterior optics temperature, and exterior optics transmittance are listed in Table S1. Georeferenced locations for each image along the flight path were manually confirmed in Google Earth and assigned GPS coordinates at the center of water in each image. Images that did not include water, were blurry, or taken at unconfirmed locations were excluded from further analysis. Locations of discrete maximum and minimum temperatures were identified at the pixel level in each image using FLIR Tools Software. To detect anomalous differences between temperatures, linear regression between maximum and minimum temperatures was performed for each survey, and standardized residual values ≤ −2 or ≥ 2 were considered different from expected and defined as thermal anomalies. To determine possible sources of temperature variation detected in these images, follow-up ground-truth surveys were conducted at each location using a FLIR C2 handheld thermal camera to collect thermal and corresponding standard images and a YSI 2030 Pro meter (YSI Inc., Yellow Springs, OH, USA) to measure water temperature (°C) at or near apparent sources.
2.3 Manatee occurrence at thermal microrefugia
To relate thermal anomaly locations to manatee occurrence, manatee sighting and stranding data were obtained from the Dauphin Island Sea Lab’s Manatee Sighting Network, a citizen science program based in coastal Alabama (Pabody et al., 2009; Hieb et al., 2017). We compiled data for Alabama waters from 2007–2019 to include opportunistic, publicly reported sightings and sightings generated during cold-stress related stranding response, which included live distressed animals and carcasses. Live distressed animals were further divided into three outcome categories, including rescued, unknown (i.e., animal moved from sighting location and was not relocated), and died. Stranding response data were collected under U.S. Fish and Wildlife Service permits LOAFC770191-H and MA66525C. Opportunistic sightings were assigned confidence levels of low, medium, or high based on observer description, photographs (when available), or verification by an experienced observer. Low confidence sightings, which included only limited descriptive information and no corroborating photos, and duplicate sightings, which were defined as sightings reported by multiple observers but occurring at the same location, date, and time (within 30 min), were excluded from the dataset.
We then tested cold season sighting and stranding occurrence as potential indicators of use of thermal anomaly locations as thermal microrefugia. To do this, we compared locations of opportunistic and stranding-related manatee sightings to locations of thermal anomalies using ArcMap Version 10.3 (ESRI, 2014). To determine if manatees changed spatial distributions during cold periods relative to locations of thermal microrefugia, we compared locations of opportunistic manatee sightings between warm (Apr–Oct) and cold (Nov–Mar) seasons using the kernel density tool in ArcMap Version 10.3. Warm and cold seasons were defined based on seasonal water temperatures in coastal Alabama, where water temperatures typically fall below 20°C during Nov–Mar and above this temperature during Apr–Oct (Hieb et al., 2017; Cloyed et al., 2022).
2.4 Defining decadal-scale responses
We natural log-transformed the number of cold season sightings and strandings and used general linear models to determine the change in number of cold season manatee sightings and strandings (live distressed animals and carcasses) in Alabama waters through time (Ives, 2015). To avoid duplicate counts of the same stranded animal, live distressed manatees that later died were each counted as a single stranding. To detect changes in timing of manatee sightings in Alabama waters that could indicate changes in migration phenology, we compared the dates of first and last manatee sightings and the duration of manatee occurrence in Julian days among sighting seasons. Sighting seasons and season duration were defined as the period between the first and last sighting dates (Leslie, 2017), and when the last sighting date occurred into the next calendar year, 365 was added to the Julian day. General linear models were used to determine if the timing and duration of sighting seasons changed through time, and the combined monthly sighting frequency distribution during cold seasons was used to determine if potential changes were driven by first or last sighting dates (i.e., manatees arrived in Alabama waters earlier or stayed later each season). All linear model analyses were graphically validated and were performed in R (R Core Team, 2020).
To understand how temperature conditions affected the longer-term timing and duration of manatee sighting seasons, we calculated daily mean air temperatures and daily minimum sea surface temperature during the autumn migration period (Sep–Oct) and the cold season (Nov–Mar) using data from the National Data Buoy Center station DPHA1 on Dauphin Island, AL. We then used these data to calculate the number of days the average air temperature was > 20°C during the autumn migration and cold seasons between 1976 and 2019 and the mean minimum sea surface temperature per year during cold seasons between 1987 and 2019. Different time scales for air and sea surface temperature analyses were based on data availability. We used general linear models to determine if there were increases through time in: a) the number of days with mean temperatures > 20°C during the autumn migration and cold season, b) the mean minimum sea surface temperature during the cold season, and c) the variation (absolute value of the residuals) in minimum temperatures during the cold season. Temperature analyses were performed in R.
3 Results
3.1 Detecting and defining thermal microrefugia
A total of 1,011 thermal and corresponding standard images were taken across all aerial surveys. Of those, 897 images were included in analyses with usable images per survey ranging from 124 (Dec) to 336 (Jan) (Table S1). Air temperatures during surveys were 8–32°C, capturing a broad range of seasonal temperature conditions (Table S1). Water temperatures recorded via thermal imaging ranged from 2°C (Jan) to 53.4°C (Aug). Maximum water temperatures increased with increasing minimum water temperatures for each seasonal survey, allowing detection of minimum and maximum values outside those expected for each survey period (Figure 1; Jan: y = 0.61x + 5.28, F1,334 = 593.5, p < 0.001, R2 = 0.64; Mar: y = 0.41x + 14.44, F1,247 = 41.43, p < 0.001, R2 = 0.14; Aug: y = 1.03x + 1.91, F1,186 = 1004.00, p < 0.001, R2 = 0.84; Dec: y = 0.25x + 13.70, F1,122 = 5.60, p = 0.02, R2 = 0.04). A total of 23 potential thermal anomalies were identified across all surveys (Table S2), representing locations where differences between minimum and maximum temperatures were greater than at other locations. Water temperatures of anomalies ranged from 10.1°C during Jan to 53.4°C during Aug surveys.
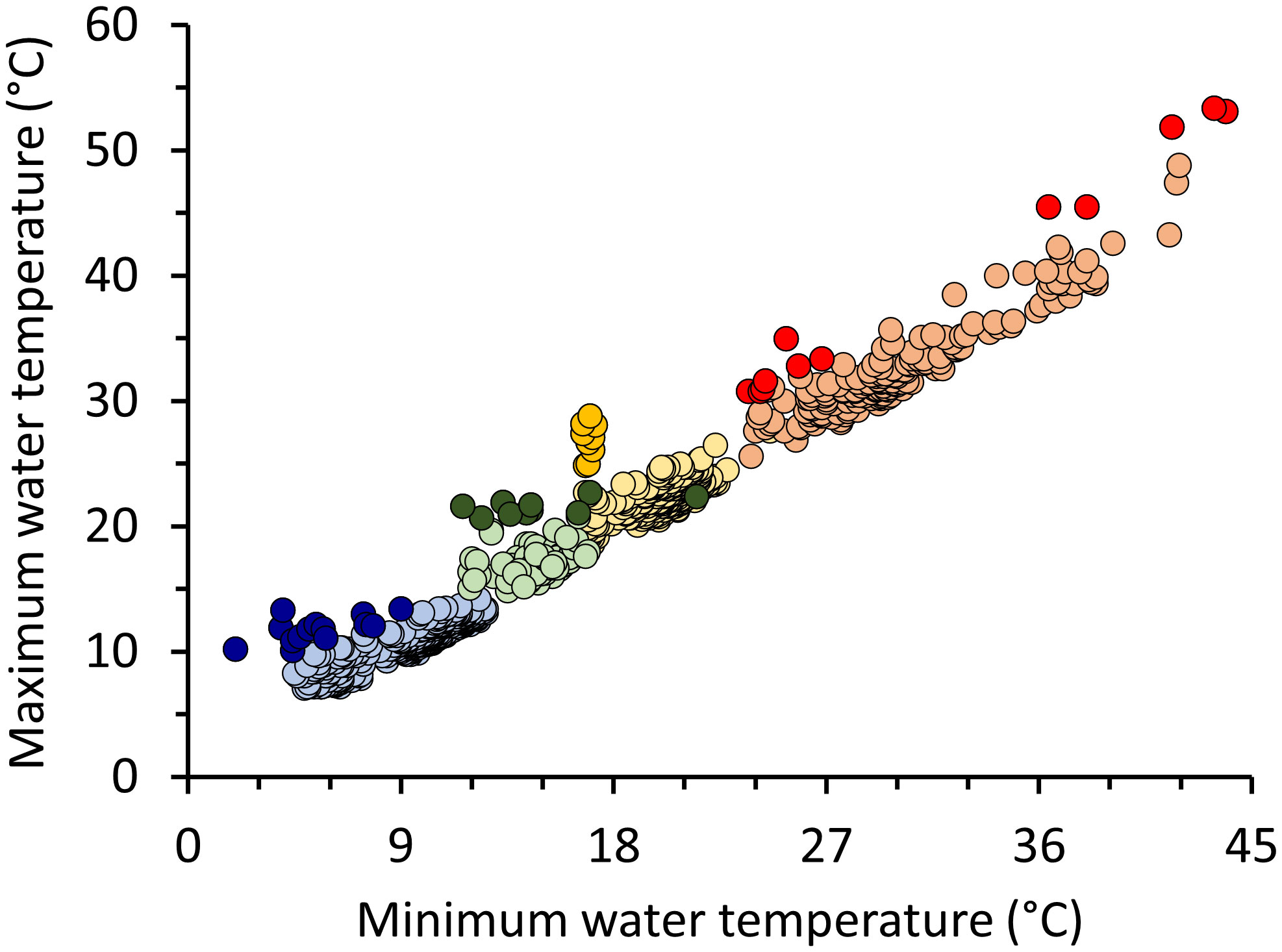
Figure 1 Maximum and minimum water temperatures identified in 897 thermal images collected in Alabama waters and anomalies (darker symbols; ± 2 standardized residuals) to the regression for each seasonal survey. Regression lines not shown for clarity.
Warm water anomalies identified during cold season surveys (Jan, Mar, Dec) included 11 locations in the Mobile-Tensaw Delta that were associated with sediment load or nearshore, marsh habitat (Table S2). The source of one warm water anomaly identified during Jan surveys and located in Rabbit Creek, a tributary of Dog River, was not detected during follow-up ground truth surveys, and therefore was removed from subsequent analyses. Two anomaly locations were identified during Mar and Dec surveys at discharge sites at a coal-fired power station (Barry Steam Plant, Alabama Power; Table S2, Figure 2A). Maximum temperatures recorded at these locations were 28.8°C and 22.7°C in Mar and Dec, respectively. An additional anomaly associated with a wastewater treatment plant outfall (Clifton C. Williams wastewater treatment plant) was identified during Mar surveys and had a maximum water temperature of 21.3°C (Table S2; Figure 2B). During warm season surveys in Aug, the Barry Steam Plant was again identified as a warm water anomaly (maximum temperature = 53.4°C). Cool water anomalies were identified in Fish River (n = 4) and at sites along the eastern shore of Mobile Bay (n = 3) (Table S2; Figures 2C, D). Follow-up ground truth surveys identified sources of anomalies in Fish River as dredged channels and along Mobile Bay as freshwater inflow and run-off sites. Environmental data collected at the dredged channel locations found that temperature and salinity increased with water depth, with an ~4°C increase in temperature and ~12 ppt increase in salinity from surface to bottom. Additional thermal anomalies detected during stranding response for cold-stressed manatees included freshwater inflow and run-off sites at Fly Creek, a man-made canal in the southern Perdido Bay system, and two natural springs at Magnolia Springs on the eastern shore of Mobile Bay and Oak Bayou in the Mobile‐Tensaw River Delta (Figure 3).
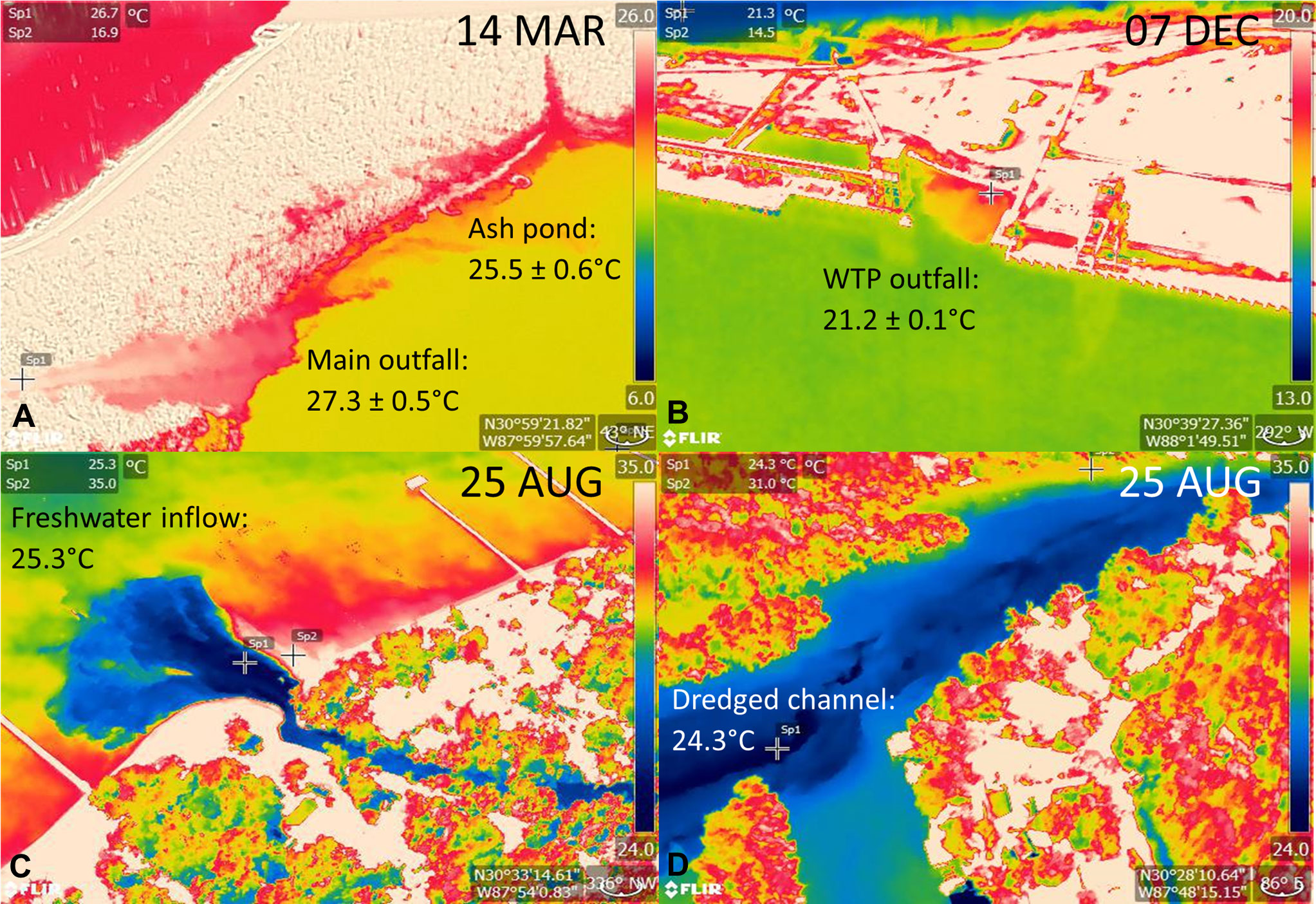
Figure 2 Example thermographic images captured during aerial surveys in coastal Alabama, including warm water anomalies at (A) the Barry Steam Plant main outfall and ash pond discharge sites and (B) Clifton C. Williams wastewater treatment plant (WTP) outfall and cool water anomalies at (C) a freshwater inflow site along the Eastern Shore of Mobile Bay and (D) a dredged channel in Fish River. Note temperature sampling points are indicated by cross bars, and the temperature scale differs among images. Maximum and minimum temperatures are shown for warm and cool water anomalies, respectively with average ± standard error reported in cases when multiple photos were taken of the same anomaly.
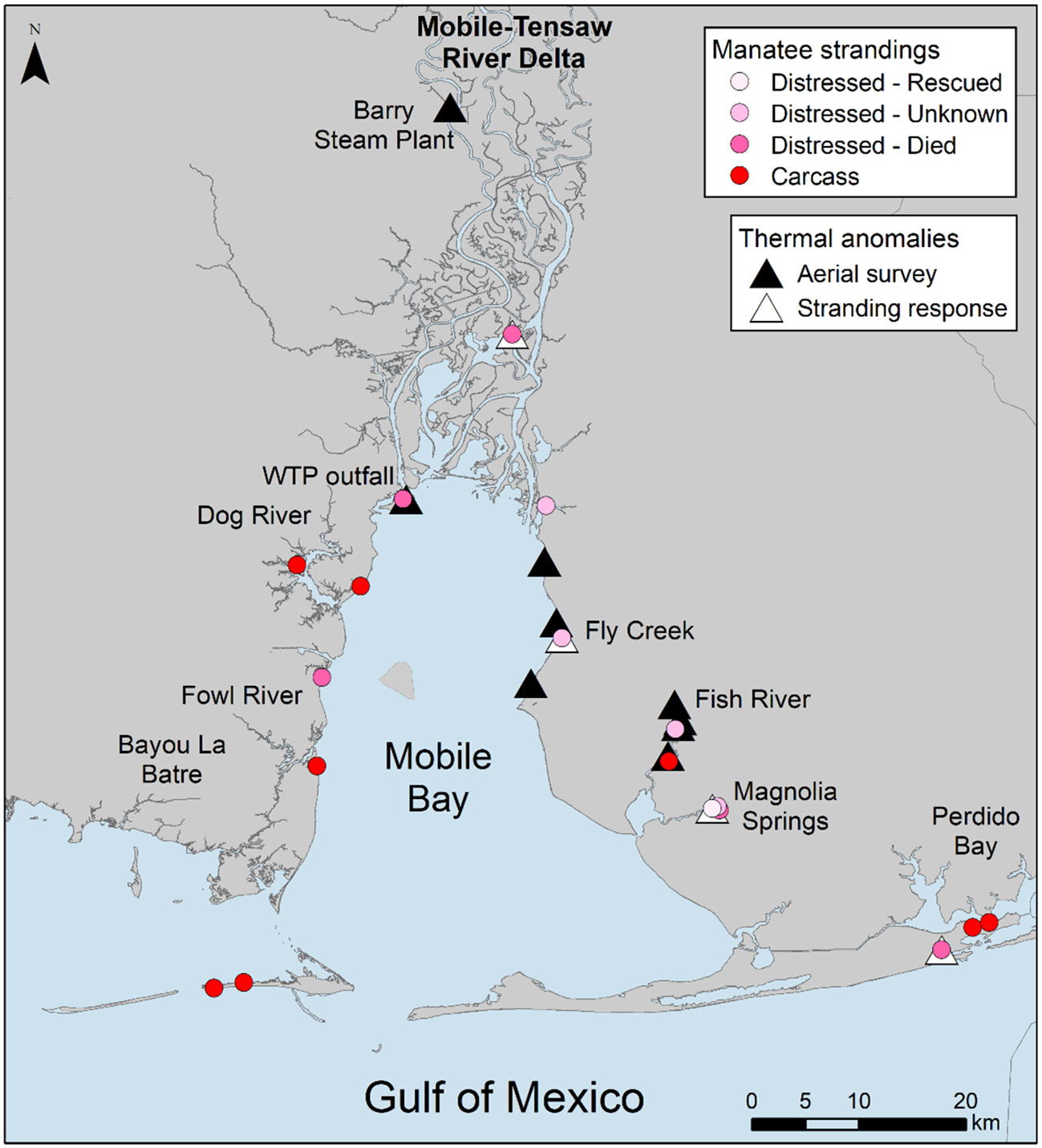
Figure 3 Locations of cold stress related manatee strandings, including live distressed manatees and manatees first found as carcasses, compared to locations of thermal anomalies identified during aerial surveys and stranding response in Alabama waters. WTP outfall is the Clifton C. Williams wastewater treatment plant and Barry Steam Plant is the James M. Barry Electric Generating Plant, both previously known warm water outfalls. Live distressed manatees are separated into categories based on outcomes, including rescued, unknown (i.e., animal moved from sighting location and was not relocated), and died.
3.2 Manatee occurrence at thermal microrefugia
Two thermal anomalies detected during aerial surveys, the Clifton C. Williams wastewater treatment plant outfall and a dredged channel in Fish River, each aligned with the location of 1 live stranded, cold-stressed manatee (Figure 3, Table S3). The outcome for the distressed animals documented at these locations was died and unknown, respectively. A third anomaly detected during surveys, also a dredged channel in Fish River, aligned with a carcass location. A total of 6 live, distressed manatees were documented at thermal anomalies detected during cold stress related stranding response (Figure 3, Table S3). Cold-related manatee strandings that did not align with thermal anomaly locations included 2 live, distressed animals and 7 carcasses. Outcomes for all live, distressed animals included 1 rescued, 4 unknown, and 5 died.
The spatial distribution of opportunistic manatee sightings in Alabama waters during cold seasons (Nov–Mar) followed similar overall patterns to warm seasons (Figure 4). During the warm season, locations with the highest density of manatee sightings included the southwestern Mobile-Tensaw River Delta, Dog River on the western shore of Mobile Bay, embayments in the southern Perdido Bay system, and nearshore Gulf of Mexico waters east of Mobile Bay. During the cold season, manatee sighting density remained high in the embayments of the Perdido Bay system and adjacent Gulf of Mexico waters. Other locations with high cold season sighting densities included Bayou La Batre and Fowl River on the western side of Mobile Bay; the southeastern Mobile-Tensaw River Delta; Fly Creek, Fish River, and Magnolia Springs on the eastern shore of Mobile Bay; and the Intracoastal Waterway between Mobile Bay and Perdido Bay. Of these locations, Fly Creek, Fish River, Magnolia Springs, and the southern Perdido Bay system overlapped with locations of detected thermal anomalies (Figures 3, 4).
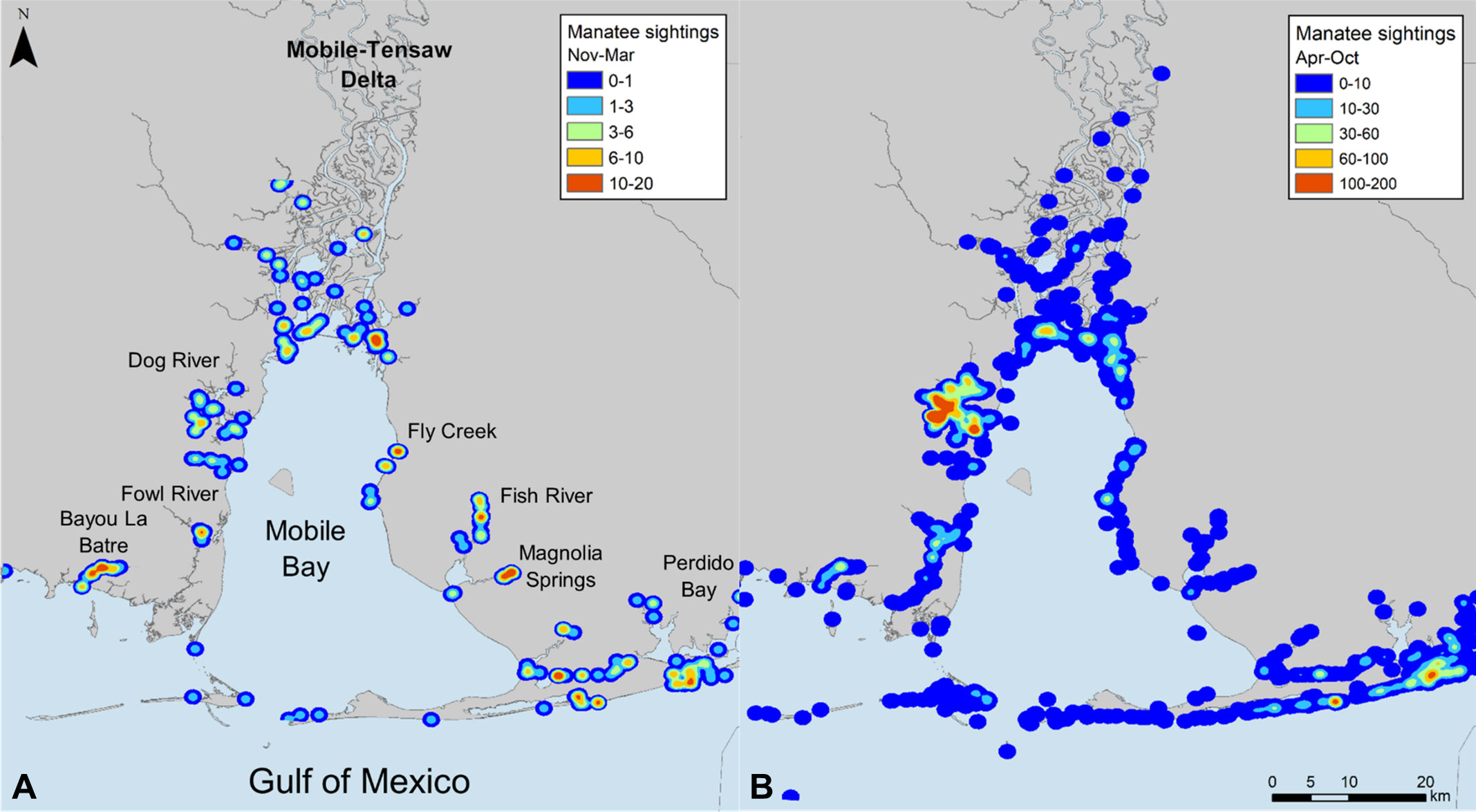
Figure 4 Density of opportunistic manatee sightings in Alabama waters from 2007–2019 during (A) cold (Nov–Mar) and (B) warm (Apr–Oct) seasons, depicted as range of observations expected per 100 km2.
3.3 Decadal-scale effects
A total of 251 opportunistic cold season sightings and 18 cold stress related strandings were recorded in Alabama during the period from 2007–2019 (Figure 5). The number of opportunistic manatee sightings during the cold season increased through time from 2007–2019, ranging from 5 sightings during the 2007–2008 season to 36 sightings during the 2017–2018 season (ey = 0.12x – 229.06, F1, 11 = 14.00, p = 0.003, R2 = 0.52). The number of cold season strandings, however, did not increase during this same period (F1, 11 = 1.38, p = 0.27, R2 = 0.04).
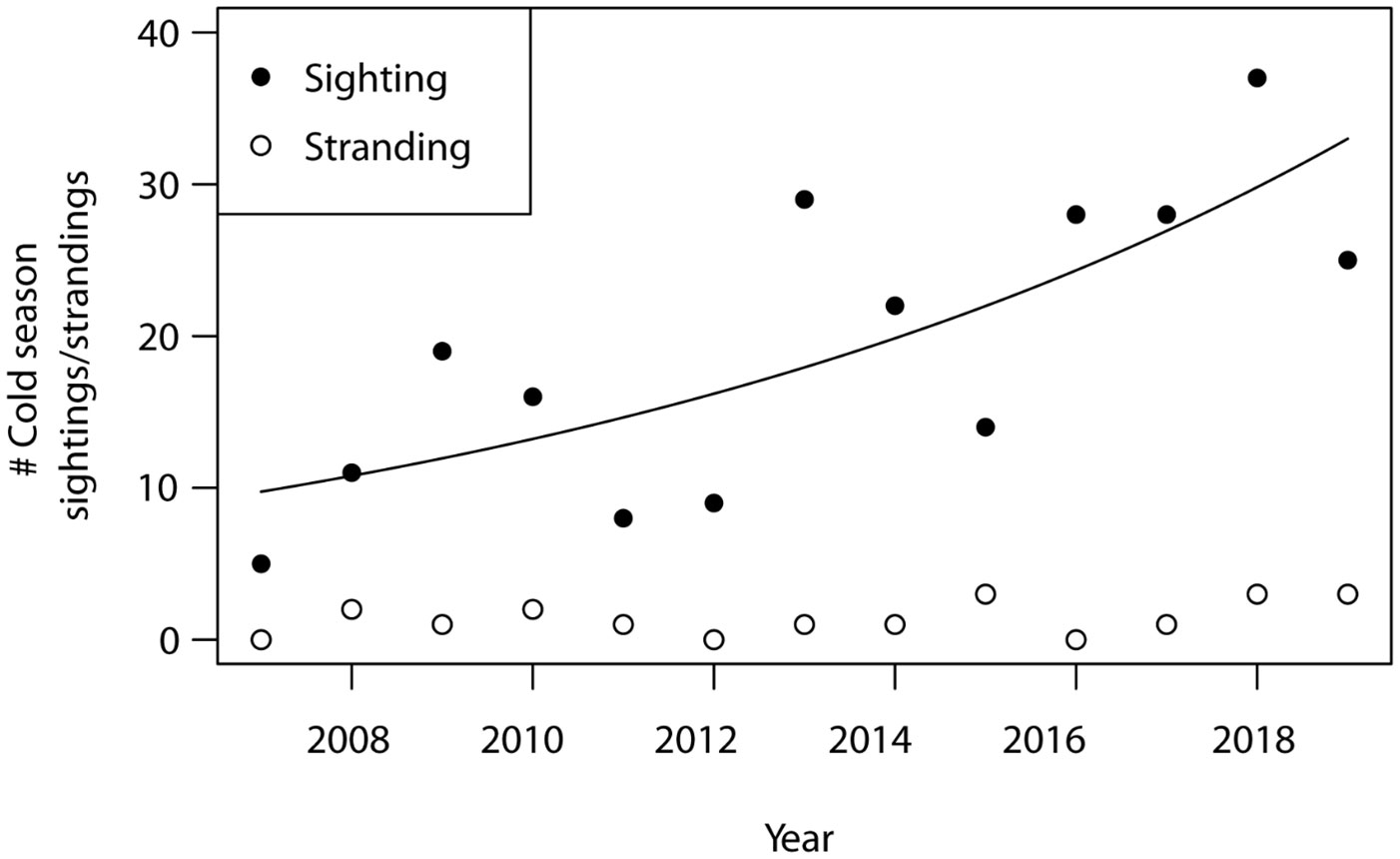
Figure 5 Number of opportunistic manatee sightings and manatee strandings recorded during each annual cold season (Nov–Mar) in Alabama. Cold seasons are represented as single years based on first sighting dates and extend into the following year (e.g., 2007 cold season = 01 Nov 2007–31 Mar 2008).
Dates (Julian day) of the first manatee sightings recorded in Alabama waters each sighting season ranged from 63 (3 Mar) during 2008 to 137 (17 May) during 2007, following initial inception of DISL/MSN, with a median first sighting date of 87 (27 Mar) (Figure 6A). The majority (69%) of first sighting dates fell within a 30-day period from 16 March–14 April. Dates of the last manatee sightings recorded each season ranged from 318 (14 Nov) during 2007 to 418 (22 Feb) during the 2018–2019 season (Figure 6B). The majority (77%) of last sighting dates occurred on 15 Dec or later, with 31% of last sightings recorded during Jan or Feb. Sightings on 15 Dec or later were documented more frequently in recent years (2014–2019). The duration of annual sighting seasons ranged from 181 days in 2007 to 341 days in 2018, with a mean sighting season duration of 272 ± 11 days (Figure 6C). Overall, first and last sighting dates and sighting season duration did not change through time; however, because last sighting dates were found later in the season in recent years, a marginally significant increase through time was detected for last sighting dates (First sighting date: y = −0.62x + 1328.85, R2 = −0.08, F1,11 = 0.15, p = 0.71; Last sighting date: y = 4.06x – 7811.67, R2 = 0.21, F1,11 = 4.26, p = 0.06; Sighting season duration: y = 4.68x – 9140.51, R2 = 0.12, F1,11 = 2.68, p = 0.13). Most cold season sightings were recorded during Nov (n = 150; 60%), followed by Dec (n = 71; 28%) (Figure 7), with a total of 30 sightings recorded during Jan–Mar across all years.
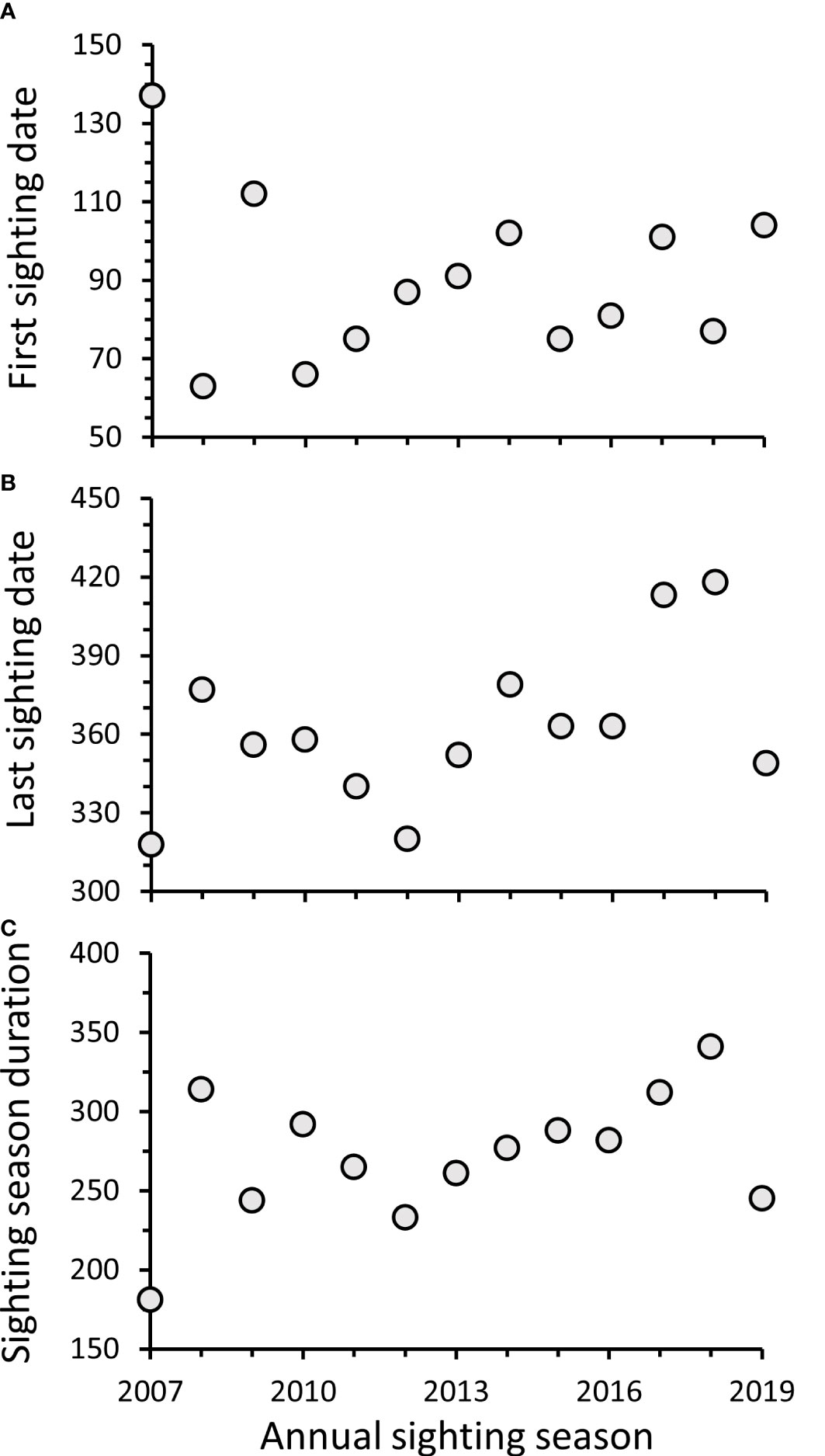
Figure 6 Date in Julian day of (A) first and (B) last manatee sightings reported in Alabama waters during annual sighting seasons and (C) duration of sighting seasons in days. Sighting seasons are represented as single years based on first sighting dates; however, in some seasons, last sightings occurred during Jan or Feb of the following year.
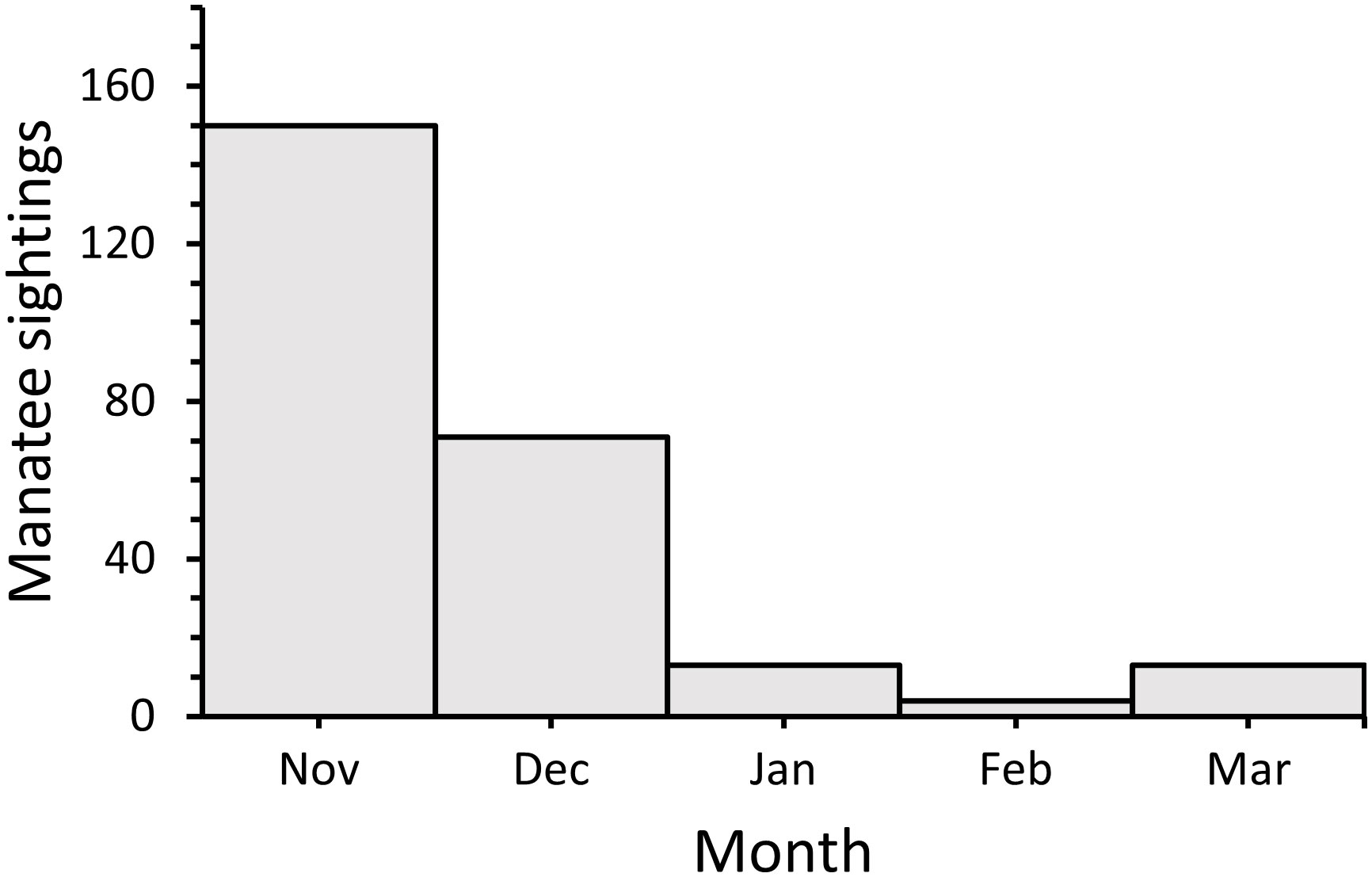
Figure 7 Number of opportunistic manatee sightings in Alabama per month during the cold season (Nov–Mar) from 2007–2019.
Data for nearshore Alabama waters showed an increase in the number of days with mean air temperatures > 20°C for both the manatee migration period and cold season (Figures 8A, B; migration: y = 0.20x – 366.95, F1, 43 = 4.92, p = 0.03, R2 = 0.08; cold season; y = 0.12x – 238.838, F1, 33 = 5.20, p = 0.03, R2 = 0.11). For the period from 1975–2019, cumulative days > 20°C ranged from 7 (1983) to 55 (1984) during the migration period (Sep–Oct) and 0 to 17 (2015) during the cold season (Nov–Mar), with 0 days > 20°C during nine years (1979–1981, 1983, 1987, 1997, 1999, 2009, and 2014). More recent years (2015–2018) had more than 10 days with temperatures > 20°C during the cold season (Figure 8B), which was primarily driven by days during Nov and Mar. Mean minimum sea surface temperatures increased through time during the migration period (y = 0.04x – 54.59, F1, 1298 = 18.22, p < 0.001, R2 = 0.01), but not the cold season (Figure 8C; F1, 3110 = 1.98, p = 0.16, R2 = 0.00), with mean minimum cold season temperatures for all years remaining below the 20°C thermal threshold for manatees. Variation in mean minimum temperatures during the cold season also showed a marginal increase through time (Figure S2; y = 0.03x – 53.55, F1,23 = 2.78, p = 0.11, R2 = 0.07).
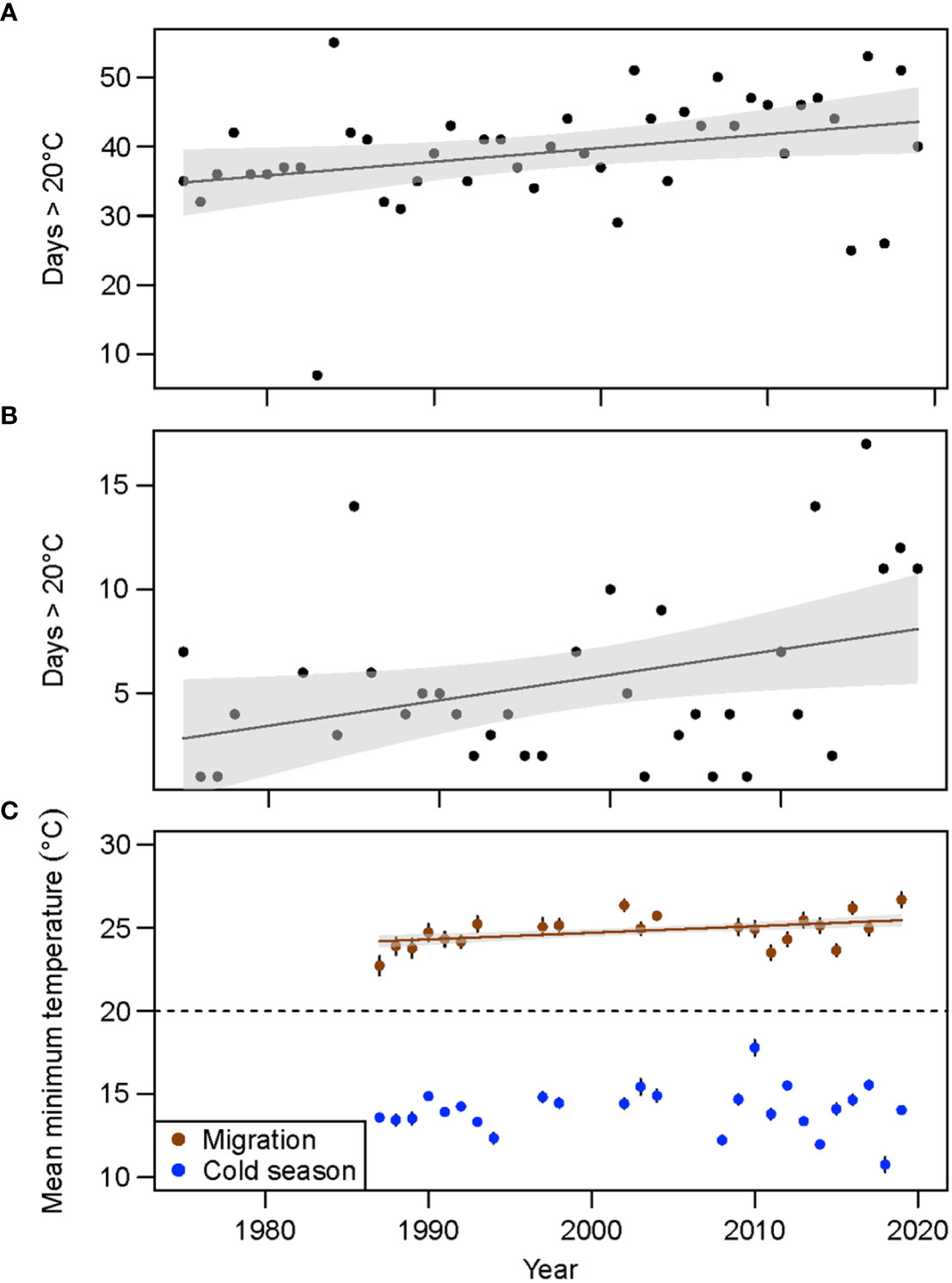
Figure 8 Number of days in the northern Gulf of Mexico with mean air temperature > 20°C during the (A) autumn migration period for manatees (Sep–Oct) and (B) cold season (Nov–Mar) and (C) mean annual minimum sea surface temperature for both periods.
4 Discussion
We found that West Indian manatees are increasingly using waters at a northwestern migratory endpoint in the northern Gulf of Mexico (nGOM) during colder months of the year. Increased sightings during Nov and Dec in recent years suggest that some manatees are changing their migratory phenology and extending the duration of seasonal habitat use in the nGOM, which may be facilitated by use of thermal microrefugia. While we did not find any sites in the study area that were consistently warm enough to support high manatee use (constituting primary thermal refugia), we identified microrefugia that may act as secondary thermal refuge sites. These secondary thermal refugia may be at least suitable to allow manatees to withstand mild cold periods for relatively short durations and delay migrations to primary refuge sites in peninsular Florida. For example, manatees along the Atlantic coast of Florida have delayed migration to primary winter refugia when able to access warm effluents farther north or when ambient temperatures remain warm (Deutsch et al., 2003; Cloyed et al., 2022). Cold season sightings have also been documented with increasing frequency in states at the range margins such as Mississippi, Louisiana, Texas, South Carolina, and North Carolina (Leslie, 2017; Carmichael et al., 2021). Additional study is needed throughout these areas to identify potential warm water sources that may be used by manatees as thermal microrefugia and further alter migratory phenology, dispersal patterns, and habitat use.
The effects of climate change-associated increasing sea surface temperatures on prolonged use of habitats at range margins remains uncertain (Cummings et al., 2014; Cloyed et al., 2022). As water temperatures increase under predicted climate change scenarios, it has been suggested that conditions may become more favorable for manatees to overwinter in areas outside of Florida (Cloyed et al., 2021). Promisingly, we found that despite an increase in cold season manatee sightings in recent years, manatee stranding numbers remained constant, suggesting that most manatees sighted during colder months survived instead of stranding. The contribution of microrefugia to this success is unclear. Our finding that minimum temperatures during the cold season have remained low, despite higher temperatures during key periods of movement, suggests that manatees staying in the nGOM at the end of the season (Jan–Feb) remain at risk of cold stress-related mortality, even if using microrefugia. Recent studies propose that extreme cold events linked to Artic warming are increasing in frequency, especially in mid-latitude regions (Cohen et al., 2021; Michelfelder and Pilotte, 2022) and could pose a risk to animals like manatees that may shift migratory phenology in response to climate change. Our finding of a marginal increase in temperature variation, with some time periods having very low temperatures during the cold season in the nGOM in more recent years, is sufficient to raise concern for increased risk of unexpected, drastic temperature decreases that could lead to increased manatee mortalities. Such mortality was previously seen due to unusually cold temperatures in Florida during 2009–2011 (Hardy et al., 2019). Further study of increasing regional water temperatures and potential for extreme cold events will be important to understand the potential for year-round residency and long-term survival of manatees in the nGOM and other marginal sites.
Thermal microrefugia documented in Alabama waters align with the various types of refugia used by manatees in their winter range in peninsular Florida. Approximately, 65–75% of manatees use warm water discharges from power plants or natural springs as primary refugia (Laist and Reynolds, 2005a; Laist and Reynolds, 2005b; Laist et al., 2013; Valade et al., 2020). In Alabama, a power plant and two natural springs were identified as warm water anomalies, with manatee use documented at the spring sites but not the power plant outfall, during the study period. An additional discharge site identified at a wastewater treatment plant outfall was used by a distressed manatee that was later recovered deceased. Manatees in Florida have also been documented to use wastewater treatment plant outfalls, which are not artificially heated, but are often slightly warmer than surrounding ambient water temperatures (Laist and Reynolds, 2005b). Potential passive thermal basins identified in Alabama included multiple freshwater inflow or run-off sites and dredged channels. Two of these freshwater inflow sites observed in Alabama were documented as cool water microhabitats during summer surveys but may conversely be warmer than surrounding waters during colder months as is the case for some thermal microrefugia in Florida that maintain constant temperature year-round (Laist et al., 2013). In peninsular Florida, passive thermal basins with the highest manatee use are dredged canals where lighter, freshwater flows over heavier, saline water, creating a strong vertical halocline that effectively traps warm water on the bottom (Stith et al., 2011; Stith et al., 2012). A similar mechanism may occur in Alabama at dredged channels identified via thermal imaging. Temperature data collected at one of these sites during response to a distressed manatee in Dec 2017 found a salinity gradient of 0.9–12.6 and temperature gradient of 12.2–15.9°C from surface to bottom over a 4m depth. While temperatures at many of the anomalies identified in this study were < 20°C, the occurrence of animals at these locations suggests they are capable of detecting these thermal differences, and manatees used the various types of available microrefugia in Alabama just as they do in Florida waters.
The ability of manatees to successfully use thermal microrefugia in the nGOM in the future will depend on specific attributes that dictate the availability and suitability of these microhabitats. For example, the Barry Steam Plant was the most consistently documented thermal anomaly detected during seasonal aerial surveys, but warm water discharge at this site is episodic, based on the community’s energy use. Maximum temperatures where the plant discharges into the Mobile River during Dec and Mar were ~22–27°C, above the thermal threshold for manatees, but lower discharge rates at the plant in Jan resulted in greater mixing with ambient water, yielding a maximum water temperature of ~12°C. The location of the power plant at the northern extent of most documented manatee sightings in Alabama may also limit manatee use. Flow rates at natural springs and freshwater inflow sites will change with precipitation, discharge patterns, and human activities that can also affect local hydrology (Wang et al., 2014). Groundwater-fed springs may be more likely than other sources to maintain consistent temperatures, but reductions in groundwater flow from natural or human causes could limit the volume and surface area of warmwater available to manatees at this and other springs, as has been shown in Homosassa Springs, FL, a natural spring heavily used by manatees as a primary thermal refuge (Valade et al., 2020; Chen, 2021). Similarly, dredged channels with temperature inversions linked to haloclines depend on specific hydrology to maintain warm water conditions (Stith et al., 2011; Stith et al., 2012; Decker et al., 2013). Knowledge of the various factors that may affect the volume and consistency of warm water sources, particularly at range margins, will be critical to defining the suitability and availability of thermal microrefugia in the future.
While temperatures are an important determinant of microrefugia use by manatees at their range margins, other factors are likely to be equally influential in determining when and where manatees occur during colder months. Heavily used habitats in Alabama during both warm and cold seasons align with locations of submerged aquatic vegetation (SAV), freshwater access, and shallow water known to be preferred by manatees (Hieb et al., 2017; Cloyed et al., 2022). As regional temperatures cool during autumn and winter, manatees may preferentially remain in these resource abundant areas and delay migration to winter refuge sites where resources are more limited (Deutsch et al., 2003; Haase et al., 2019; Littles et al., 2019; Cloyed et al., 2022). While the availability of thermal microrefugia necessarily enhances manatees’ ability to alter migration phenology, the temperature thresholds at which individual manatees depart warm season foraging habitats and travel to primary winter refugia are also likely dependent on behavioral or physiological variation among manatees (Deutsch et al., 2003). Larger, older individuals may be better able to withstand colder temperatures due to smaller surface area to volume ratios that better sustain homeostasis (Buergelt et al., 1984; Chirachevin, 2017) as well as more experience in managing cold temperatures (Buergelt et al., 1984; Deutsch et al., 2003). The severity of cold fronts in terms of the magnitude of temperature variation and cold duration may also influence whether manatees initiate long distance migrations to primary warm water sites or seek closer, less favorable secondary refugia (Deutsch et al., 2003; Hardy et al., 2019). At least some manatees in the nGOM may follow similar strategies to manatees along the Atlantic coast of Florida, using warm water microhabitats as temporary stepping-stones to withstand cold fronts during autumn and early winter as they travel to primary refuge sites farther south (Reid et al., 1991; Deutsch et al., 2003; Cloyed et al., 2021). This approach may be particularly beneficial if primary refugia in Florida reach carrying capacity and become increasingly resource limited (Littles et al., 2019). To best take advantage of resources in warm season habitats, manatees may take a combined approach of using microhabitats for foraging and thermal microrefugia along migration pathways, with exact strategies dependent on environmental variation and individual plasticity.
While methods used in our study were successful at identifying some thermal anomalies, there are potential limitations to using point-in-time thermal imaging methods alone. For example, during field responses to cold-stressed manatees, we often identified warm water sources not detected during aerial surveys. Thermal cameras were effective at capturing differences in surface water temperature, but anomalies at bottom depths were not always detected if temperature signals dissipated before reaching surface waters. These thermal differences were only observed by directly collecting environmental data from surface to the bottom of the water column. For this reason, use of a vertical profiling system that captures water quality data at various depths is useful to supplement thermal imaging data. In addition, the specific conditions during aerial surveys also likely influenced detection of thermal anomalies, highlighting the importance of higher-resolution, year-round monitoring to help identify potential refuge sites. Thermal microrefugia at some locations also may be ephemeral, existing only under certain conditions that are yet to be defined, and may not be detected using point-in-time sampling methods. In some cases, temperature differences between warm water sources and ambient waters were minimal and not captured as thermal anomalies during our surveys, but higher manatee occurrence at these sites during the cold season indicates manatees are selecting these sites for use, potentially as thermal microrefugia. More work is needed to understand the capability of manatees to detect subtle temperature differences, but Deutsch et al. (2003) found that tagged manatee movements were influenced by temperature changes of only 2.0–3.5°C. In addition to thermal imaging and manatee occurrence data used here, other data such as bathymetry and hydrology of local waterways may be useful in detecting potential warm water sources. Citizen-sourced data and local knowledge can also be informative to supplement traditional scientific data collection in understudied regions (Hieb et al., 2017; Bélisle et al., 2018). While our study focuses on manatees in the nGOM, our approach can be transferred to other species and systems and can complement other types of data collection to better understand changes in species distribution and habitat use.
Thermally dependent species are facing rapid changes in dispersal, migration, and habitat use patterns as climate change and other anthropogenically driven factors increasingly pressure these species to adapt to new conditions. Manatees are an informative case study of changing migratory strategies, potentially linked to microrefugia use and warming temperatures. Predicting manatee use of thermal microrefugia outside of Florida and changes in migratory phenology relative to long-term temperature variation will lead to enhanced understanding of range-wide habitat use during colder months of the year and has the potential to inform management actions to conserve these habitats. As primary refugia within traditional manatee habitats face threats from human impacts and potential for reaching carrying capacity, microhabitats at range margins may be increasingly utilized by manatees. Our study provides novel insight for a thermally constrained migratory marine mammal that may also be used as a model for other thermally constrained species expanding into higher latitudes. To effectively manage and conserve these species and their habitats, future studies should consider local microhabitat use within regional and global scales of temperature change, while also considering individual and population level variation in species responses.
Data availability statement
The raw data supporting the conclusions of this article will be made available by the authors, without undue reservation.
Ethics statement
Ethical review and approval was not required for the animal study because this study uses only citizen-sourced and stranding response data. Citizen-sourced data are non-invasive and do not require approval by an animal ethics committee. Stranding response data were collected under U.S. Fish and Wildlife Service permits LOAFC770191-H and MA66525C.
Author contributions
EH wrote the main draft of the manuscript. EH and CC analyzed the data. KD, AG, and RC contributed to the collection and analysis of data and participated in writing and editing the manuscript. RC conceived of the project. All authors contributed to the article and approved the submitted version.
Funding
This work was funded in part by the Alabama Department of Conservation and Natural Resources, Division of Wildlife and Freshwater Fisheries under traditional Section 6 of the U.S. Fish and Wildlife Service (F16AC00119), the Mobile Bay National Estuary Program, the Northern Gulf Institute, the Gulf of Mexico Energy Security Act (GMSN2017), and the National Science Foundation through the Research Experiences for Undergraduates Program (OCE 1358873).
Acknowledgments
We thank D. Allen for information to optimize use of the FLIR T620, M. Ross (Clearwater Marine Aquarium Research Institute) for guidance on aerial survey and data acquisition methods, R. Kellogg for piloting aerial surveys, and DISL/MSN staff, students, and volunteers for assistance with data collection.
Conflict of interest
The authors declare that the research was conducted in the absence of any commercial or financial relationships that could be construed as a potential conflict of interest.
Publisher’s note
All claims expressed in this article are solely those of the authors and do not necessarily represent those of their affiliated organizations, or those of the publisher, the editors and the reviewers. Any product that may be evaluated in this article, or claim that may be made by its manufacturer, is not guaranteed or endorsed by the publisher.
Supplementary material
The Supplementary Material for this article can be found online at: https://www.frontiersin.org/articles/10.3389/fevo.2023.1211513/full#supplementary-material
References
Bélisle A. C., Asselin H., LeBlanc P., Gauthier S. (2018). Local knowledge in ecological modeling. Ecol. Soc 23 (2), 14. doi: 10.5751/ES-09949-230214
Bossart G. D., Meisner R. A., Rommel S., Ghim S., Jenson A. B. (2003). Pathological features of the Florida manatee cold stress syndrome. Aquat. Mamm. 29, 9–17. doi: 10.1578/016754203101024031
Bosso L., Smeraldo S., Russo D., Chiusano M. L., Bertorelle G., Johannesson K., et al. (2022). The rise and fall of an alien: why the successful colonizer Littorina saxatilis failed to invade the Mediterranean Sea. Biol. Invasions. 24, 3169–3187. doi: 10.1007/s10530-022-02838-y
Buergelt C. D., Bonde R. K., Beck C. A., O'Shea T. J. (1984). Pathologic findings in manatees in Florida. J. Am. Vet. Med. Assoc. 185, 1331–1334.
Carmichael R. H., Hieb E., Aven A., Taylor N., Nelson-Seely C., Delo J., et al. (2021). Dauphin island Sea lab’s manatee sighting network database (1912–2021) (Alabama, USA: Dauphin Island Sea Lab). Available at: https://data.disl.edu/dataset/the-west-indian-manatee-population-in-mobile-bay-al-and-surrounding-waters-1912.
Chen X. (2021). A study of effects of reduction of submarine groundwater discharge on thermal habitats for manatee in a spring-fed estuary using a laterally averaged hydrodynamic model. Ecol. Model. 456, 109653. doi: 10.1016/j.ecolmodel.2021.109653
Chirachevin P. (2017). The relationship between cold stress syndrome mortality and body shape in Florida manatees (Berrien Springs, MI: Andrews University).
Cloyed C. S., Hieb E. E., DaCosta K., Ross M., Carmichael R. H. (2021). West Indian Manatees use partial migration to expand their geographic range into the northern gulf of Mexico. Front. Mar. Sci. 8. doi: 10.3389/fmars.2021.725837
Cloyed C. S., Hieb E. E., DaCosta K. P., Ross M., Carmichael R. H. (2022). Habitat selection and abundance of West Indian manatees Trichechus manatus at the margins of their expanding range. Mar. Ecol. Prog. Ser. 696, 151–167. doi: 10.3354/meps14116
Cohen J., Agel L., Barlow M., Garfinkel C. I., White I. (2021). Linking artic variability and change with extreme winter weather in the united states. Science 373, 1116–1121. doi: 10.1126/science.abi9167
Cummings E. W., Pabst D. A., Blum J. E., Barco S. G., Davis S. J., Thayer V. G., et al. (2014). Spatial and temporal patterns of habitat use and mortality of the Florida manatee (Trichechus manatus latirostris) in the mid-Atlantic states of north Carolina and Virginia from 1991 to 2012. Aquat. Mamm. 40, 126–138. doi: 10.1578/AM.40.2.2014.126
Decker J., Swain E., Stith B., Langtimm C. (2013). Assessing factors affecting the thermal properties of a passive thermal refuge using three-dimensional hydrodynamic flow and transport modeling. J. Waterw. Port. Coast. Ocean Eng. 139, 209–220. doi: 10.1061/(ASCE)WW.1943-5460.0000165
DeMarche M. L., Doak D. F., Morris W. F. (2018). Incorporating local adaptation into forecasts of species’ distribution and abundance under climate change. Glob. Change Biol. 25, 775–783. doi: 10.1111/gcb.14562
Deutsch C. J., Reid J. P., Bonde R. K., Easton D. E., Kochman H. I., O’Shea T. J. (2003). Seasonal movements, migratory, behavior, and site fidelity of West Indian manatees along the Atlantic coast of the united states. Wildlife Monogr. 40, 126–138.
Deutsch C. J., Self-Sullivan C., Mignucci-Giannoni A. (2008). “Trichechus manatus,” in The IUCN red list of threatened species, e.T22103A9356917. doi: 10.2305/IUCN.UK.2008.RLTS.T. Accessed on 16 November 2022).
Doniol-Valcroze T., Berteaux D., Larouche P., Sears R. (2007). Influence of thermal fronts on habitat selection by four rorqual whale species in the gulf of st. Lawrence. Mar. Ecol. Prog. Ser. 335, 207–216. doi: 10.3354/meps335207
Fertl D., Schiro A., Regan G., Beck C., Adimey N., Price-May L., et al. (2005). Manatee occurrence in the northern gulf of Mexico west of Florida. Gulf Caribb. Res. 17, 69–94. doi: 10.18785/gcr.1701.07
Groepper S. R., Gabig P. J., Vrtiska M. P., Gilsdorf J. M., Hygnstrom S. E., Powell L. A. (2008). Population and spatial dynamics of resident Canada geese in southeastern Nebraska. Hum–Wildl Confl. 2, 270–276.
Haase C. G., Fletcher R. J. Jr., Slone D. H., Reid J. P., Butler S. M. (2019). Landscape complementation revealed through bipartite networks: an example with the Florida manatee. Landscape Ecol. 32, 1999–2014. doi: 10.1007/s10980-017-0560-5
Hannah L., Flint L., Syphard A. D., Moritz M. A., Buckley L. B., McCullough I. M. (2014). Fine-grain modeling of species’ response to climate change: holdouts, stepping-stones, and microrefugia. Trends Ecol. Evol. 9, 390–397. doi: 10.1016/j.tree.2014.04.006
Hardy S. K., Deutsch C. J., Cross T. A., de Wit M., Hostetler J. A. (2019). Cold-related Florida manatee mortality in relation to air and water temperatures. PloS One 14, e0225048. doi: 10.1371/journal.pone.0225048
Hieb E. E., Carmichael R. H., Aven A., Nelson-Seely C., Taylor N. (2017). Sighting demographics of the West Indian manatee Trichechus manatus in the north-central gulf of Mexico supported by citizen-sourced data. Endanger. Species Res. 32, 321–332. doi: 10.3354/esr00817
Irvine A. B. (1983). Manatee metabolism and its influence on distribution in Florida. Biol. Conserv. 25, 315–334. doi: 10.1016/0006-3207(83)90068-X
Ives A. R. (2015). For testing the significance of regression coefficients, go ahead and log-transform count data. Methods Ecol. Evol. 6, 828–835. doi: 10.1111/2041-210X.12386
Laist D. W., Reynolds J. E. III. (2005a). Florida Manatees, warm-water refuges, and an uncertain future. Coast. Manage. 33, 279–295. doi: 10.1080/08920750590952018
Laist D. W., Reynolds J. E. III. (2005b). Influence of power plants and other warm-water refuges on Florida manatees. Mar. Mamm. Sci. 21, 739–764. doi: 10.1111/j.1748-7692.2005.tb01263.x
Laist D. W., Taylor C., Reynolds J. E. III. (2013). Winter habitat preferences for Florida manatees and vulnerability to cold. PloS One 8, e58978. doi: 10.1371/journal.pone.0058978
Lembrechts J. J., Nijs I., Lenoir J. (2019). Incorporating microclimate into species distribution models. Ecography 42, 1267–1279. doi: 10.1111/ecog.03947
Leslie D. B. (2017). Seasonal distribution and habitat use of the Florida manatee (Trichechus manatus latirostris) in South Carolina, U.S.A. from 1903-2015 (Charleston, SC: College of Charleston).
Littles C. J., Bonde R. K., Butler S. M., Jacoby C. A., Notestein S. K., Reid J. P., et al. (2019). Coastal habitat change and marine megafauna behavior: Florida manatees encountering reduced food provisions in a prominent winter refuge. Endanger. Spec. Res. 38, 29–43. doi: 10.3354/esr00933
Martony M., Hernandez J. A., de Wit M., St. Leger J., Erlacher-Reid C., Vanderberg J., et al. (2019). Clinicopathological prognostic indicators of survival and pathological findings in cold-stressed Florida manatees Trichechus manatus latirostris. Dis. Aquat. Organ. 132, 85–97. doi: 10.3354/dao03306
Mayor S. J., Guralnick R. P., Tingley M. W., Otegui J., Withey J. C., Emendorf S. C., et al. (2017). Increasing phenological asynchrony between spring green-up and arrival of migratory birds. Sci. Rep. 7, 1902. doi: 10.1038/s441598-017-02045-z
Michelfelder R. A., Pilotte E. A. (2022). Climate change, extreme winter weather and electricity production costs. Electr. J. 35, 107093. doi: 10.1016/j.tej.2022.107093
Müller T. M., Meier C. M., Knaus F., Korner P., Helm B., Amrhein V., et al. (2023). Finding food in a changing world: small-scale foraging habitat preferences of an insectivorous passerine in the Alps. Ecol. Evol. 13, e10084. doi: 10.1002/ece3.10084
Neate-Clegg M. H. C., Tingley M. W. (2022). Adult male birds advance spring migratory phenology faster than females and juveniles across north America. Glob. Change Biol. 29, 341–354. doi: 10.1111/gcb.16492
Osland M. J., Stevens P. W., Lamont M. M., Brusca R. C., Hart K. M., Waddle J. H., et al. (2021). Tropicalization of temperate ecosystems in north America: the northward range expansion of tropical organisms in response to warming winter temperatures. Glob. Change Biol. 27, 3009–3034. doi: 10.1111/gcb.15563
Pabody C. M., Carmichael R. H., Rice L., Ross M. (2009). A new sighting network adds to 20 years of historical data on fringe West Indian manatee (Trichechus manatus) populations in Alabama waters. Gulf Mex. Sci. 27, 6. doi: 10.18785/goms.2701.06
Petty J. T., Hansbarger J. L., Huntsman B. M., Mazik P. M. (2012). Brook trout movement in response to temperature, flow, and thermal refugia within a complex Appalachian riverscape. Trans. Am. Fish. 141, 1060–1073. doi: 10.1080/00028487.2012.681102
Pulito F., Berthold P. (2010). Current selection for lower migratory activity will drive the evolution of residency in a migratory bird population. P. Natl. Acad. Sci. 107, 7341–7346. doi: 10.1073/pnas.0910361107
R Core Team (2020). R: a language and environment for statistical computing (Vienna: R Foundation for Statistical Computing).
Reid J. P., Rathbun G. B., Ross Wilcox J. (1991). Distribution patterns of individually identifiable West Indian manatees (Trichechus manatus) in Florida. Mar. Mamm. Sci. 7, 180–190. doi: 10.1111/j.1748-7692.1991.tb00564.x
Satterfield D. A., Marra P. P., Sillett T. S., Altizer S. (2018). Responses of migratory species and their pathogens to supplemental feeding. Philos. Trans. R. Soc Lond. B Biol. Sci. 373, 20170094. doi: 10.1098/rstb.2017.0094
Schmalijohann H., Both C. (2017). The limits of modifying migration speed to adjust to climate change. Nat. Clim. Change 7, 573–576. doi: 10.1038/nclimate3336
Schofield G., Bishop C. M., Katselidis K. A., Dimopoulos P., Pantis J. D., Hays G. C. (2009). Microhabitat selection by sea turtles in a dynamic thermal marine environment. J. Anim. Ecol. 78, 14–21. doi: 10.1111/j.1365-2656.2008.01454.x
Searcy C. A., Gabbai-Saldate E., Shaffer H. B. (2013). Microhabitat use and migration distance of an endangered grassland amphibian. Biol. Conserv. 158, 80–87. doi: 10.1016/j.biocon.2012.08.033
Seebacher F., Post E. (2015). Climate change impacts on animal migration. Clim. Change Responses. 2, 5. doi: 10.1186/s40665-015-0013-9
Stith B. M., Reid J. P., Langtimm C. A., Swain E. D., Doyle T. J., Slone D. H., et al. (2011). Temperature inverted haloclines provide winter warm-water refugia for manatees in southwest Florida. Estuar. Coast. 34, 106–119. doi: 10.1007/s12237-010-9286-1
Stith B. M., Slone D. H., de Wit M., Edwards H. H., Langtimm C. A., Swain E. D., et al. (2012). Passive thermal refugia provided warm water for Florida manatees during the severe winter of 2009–2010. Mar. Ecol. Prog. Ser. 462, 287–301. doi: 10.3354/meps09732
Thomas C. D., Bodsworth E. J., Wilson R. J., Simmons A. D., Davies Z. G., Musche M., et al. (2001). Ecological and evolutionary processes at expanding range margins. Nature 411, 577–581. doi: 10.1038/35079066
Valade J., Mezich R., Smith K., Merrill M., Calleson T. (2020). Florida Manatee warm-water action plan (Tallahassee, FL: U.S. Fish & Wildlife Service and Florida Fish and Wildlife Conservation Commission).
Vergés A., Steinberg P. D., Hay M. E., Poore A. G. P., Campbell A. H., Ballesteros E. (2014). The tropicalization of temperate marine ecosystems: climate-mediated changes in herbivory and community phase shifts. P. R. Soc B-Biol. Sci. 281, 20140846. doi: 10.1098/rspb.2014.0846
Visser M. E., Both C. (2005). Shifts in phenology due to global climate change: the need for a yardstick. P. R. Soc B-Biol. Sci. 272, 2561–2569. doi: 10.1098/rspb.2005.3356
Walsh C. J., Luer C. A., Noyes D. R. (2005). Effects of environmental stressors on lymphocyte proliferation in Florida manatees, Trichechus manatus latirostris. Vet. Immunol. Immunop. 103, 247–256. doi: 10.1016/j.vetimm.2004.09.026
Keywords: Gulf of Mexico, migration, temperature, thermal refugia, West Indian manatee, microhabitat
Citation: Hieb EE, Cloyed CS, DaCosta KP, Garelick A and Carmichael RH (2023) Thermal microrefugia and changing climate affect migratory phenology of a thermally constrained marine mammal. Front. Ecol. Evol. 11:1211513. doi: 10.3389/fevo.2023.1211513
Received: 24 April 2023; Accepted: 22 June 2023;
Published: 07 July 2023.
Edited by:
Mirko Di Febbraro, University of Molise, ItalyReviewed by:
Sonia Smeraldo, University of Naples Federico II, ItalyLuciano Bosso, Anton Dohrn Zoological Station Naples, Italy
Copyright © 2023 Hieb, Cloyed, DaCosta, Garelick and Carmichael. This is an open-access article distributed under the terms of the Creative Commons Attribution License (CC BY). The use, distribution or reproduction in other forums is permitted, provided the original author(s) and the copyright owner(s) are credited and that the original publication in this journal is cited, in accordance with accepted academic practice. No use, distribution or reproduction is permitted which does not comply with these terms.
*Correspondence: Elizabeth E. Hieb, ZWhpZWJAZGlzbC5vcmc=