- School of Life Sciences, Zhaoqing University, Zhaoqing, Guangdong, China
Sanchezia oblonga is a very important urban garden tree species. In order to investigate the ecological adaptability of S. oblonga against various stresses, this study examined physiological response of S. oblonga to biotic (feeding by the snail Achatina fulica) and abiotic (simulated pruning) independent interference. The changes in malondialdehyde content, catalase activity, and peroxidase activity were assessed over time. Our findings demonstrated that A. fulica significantly damaged S. oblonga, consuming an average of 0.22 ± 0.01 grams per hour. Furthermore, both simulated pruning and snail feeding significantly impacted the levels of malondialdehyde content and the activities of catalase and peroxidase in the plant’s leaves. Compared to the control group, both stresses induced increased malondialdehyde synthesis and heightened catalase and peroxidase activity. Interestingly, both snail feeding and leaf cutting stresses can induce more persistent defense responses in S. oblonga. Additionally, the activity of defense enzymes declined over time following stress exposure, with a more sustained effect observed in the simulated pruning group compared to the snail feeding group. Overall, the study highlights ecological adaptability of plant to biotic and abiotic stresses, emphasizing the crucial roles of malondialdehyde, catalase, and peroxidase in response to adversity and stress in S. oblonga. These findings hold significant implications for breeding programs aimed at improving stress resistance and for developing cultivation practices that optimize the health of garden plants. This study present a new perspective on understanding plant ecological adaptability of plants to biotic and abiotic stress in urban gardens.
1 Introduction
The adaptation of plants to adversity, including biotic and abiotic stresses such as temperature, mechanical stimulation, and animal feeding, is an ecological and evolutionary issue that they undergo during their growth process (Chuang et al., 2010; Atkinson et al., 2013). Sanchezia oblonga, an important urban garden plant, is a shrub belonging to the genus Sanchezia within the Acanthaceae family. It features light green leaves with prominent golden-yellow veins running down the center. These leaves are opposite, nearly sessile, and have a glossy sheen. S. oblonga grows as an upright shrub with long ovate leaves (Deng and Deng, 2002; Liang et al., 2010). Plants in the Acanthaceae family generally thrive in warm climates and are predominantly found in southern China. According to the Flora of China, there are 68 genera and over 300 species documented in China, exhibiting a wide variety of forms. Their natural habitats encompass forests, deserts, and even mangrove ecosystems. Acanthaceae species play a valuable role in landscaping applications such as flower beds, potted plants, hedges, slope stabilization, and vertical gardens. Additionally, they hold promise for developing new ornamental flowers and cultivated plants due to their fast reproduction, strong vitality, and relative resistance to pests and diseases (Tang and Liao, 2013). S. oblonga blooms in summer and autumn, producing small yellow flowers nestled within red bracts. The ideal planting medium is a loose, fertile, moist, and well-draining soil. S. oblonga can tolerate shaded environments with low light year-round. It is valued for its high ornamental appeal and potential medicinal properties (Deng, 2000; Deng, 2001; Lin and Zhang, 2001). Native to tropical regions of the Americas, S. oblonga was introduced to China for cultivation and has become widely used in landscaping projects. It is considered a significant landscaping tree species in southern China (Xie et al., 2022).
Both biotic and abiotic stresses significantly impact plant growth and development. S. oblonga, a prominent garden plant, is susceptible to various stresses encountered during cultivation and management. For instance, gardening often involves pruning branches and leaves, while herbivory by the snail Achatina fulica further disrupts S. oblonga. These stresses can significantly hinder photosynthesis, affecting normal growth and diminishing the plant’s aesthetic value in landscaping applications. Achatina fulica, an omnivorous mollusk belonging to the family Achatinidae and genus Achatina, thrives in humid environments, particularly after rain or at night. Native to East Africa, it has spread globally through human trade activities (Zhou et al., 1989). Notably, A. fulica is one of the first 16 invasive alien species documented in China. Classified as a Class II quarantine pest for import and export within China, it also serves as an intermediate host for various human and animal parasites and pathogens, including those responsible for tuberculosis and eosinophilic meningitis. The first recorded instance of A. fulica in China occurred in Xiamen, Fujian Province (Lv et al., 2009).
Understanding plant physiological responses and ecological adaptability to biotic and abiotic stresses is crucial for improving plant resilience and productivity. Existing research on S. oblonga primarily focuses on rapid propagation, cultivation techniques, ornamental value analysis, and applied applications (Deng, 2001; Lin and Zhang, 2001; Deng and Deng, 2002). However, there is a critical gap in our understanding physiological response mechanisms and ecological adaptability of S. oblonga under stress. During cultivation and garden management, S. oblonga frequently encounters various biotic and abiotic stresses, including herbivory by the snail A. fulica and mechanical pruning. This study aimed to address this knowledge gap by investigating the effects of biotic stress (feeding by A. fulica) and abiotic stress (garden pruning) on S. oblonga. We quantified changes in malondialdehyde (MDA) content, catalase (CAT) activity, and peroxidase (POD) activity, all indicators of plant defense responses. By elucidating the defense mechanisms of S. oblonga under these stress conditions, this study seeks to provide a theoretical foundation for breeding programs focused on stress resistance or pest control in S. oblonga. Additionally, these findings hold significant implications for guiding improved cultivation and management practices for S. oblonga in gardens. At the same time, it has a promoting effect on understanding the ecological and evolutionary adaptability of plants to adversity.
2 Materials and methods
2.1 Plant and animal
Plant materials for this experiment were S. oblonga specimens cultivated in the Zhaoqing University green nursery. The animals used were A. fulica individuals of similar size and vitality.
2.2 Measurement of the height and ground diameter of S. oblonga and the appetite of A. fulica
The height of 69 S. oblonga trees was measured with a ruler, and the ground diameter of 69 S. oblonga trees was measured with Vernier calipers (Wang et al., 2021). This investigation aimed to assess feeding damage caused by snail A. fulica in the campus green nursery and to evaluate the quality of 12 leaves from 12 trees consumed by the snails over a three-hour period.
2.3 Leaf clipping stimulation and snail feeding treatment
Sanchezia oblonga leaves of similar size and in good health were selected for the experiment. The experiment is timed to begin when the snail consumed about one-third of the leaf area (about takes 5 hours) in the treatment group, and at the same time, cuts off the same area (one-third of the leaf area) as the snail eats as the leaf cutting stress group (Zhao et al., 2024). The control group leaves received no treatment. Snails are surrounded by breathable nets on a single leaf, and two leaves from one tree are selected to be placed with one snail each. After 3, 9, and 15 hours, leaves from each group were collected to determine malondialdehyde (MDA) content, catalase (CAT) activity, and peroxidase (POD) activity. Each test group was set with 3 repetitions (Figure 1). Each repetition contained 5 leaves, and only one leaf was selected for a tree.
2.4 Determination of malondialdehyde content after stimulated by leaf cutting and snail bite
The determination of malondialdehyde content was based on the method previous research reported, but with some improvements (Bailly et al., 2010; Zhang et al., 2015). One gram of leaves was weighed, cut into pieces, and placed in a mortar. Two milliliters of 10% Trichloroacetic Acid (TCA) solution and a small amount of quartz sand are added. The mixture was ground into a homogenate. An additional 8 mL of 10% TCA solution was added, and grinding was continued until complete homogenization was achieved. The homogenate was then centrifuged for 10 minutes, and the supernatant was collected as the sample extract. Two milliliters of the extract were combined with 2 milliliters of 0.6% Thiobarbituric acid (TBA) solution, mixed well, and incubated in a boiling water bath for 15 minutes. After rapid cooling, the solution was centrifuged again. The supernatant was used to measure the optical density at 450 nm, 532 nm, and 600 nm. The MDA content in the sample extract was calculated using the formula provided in the experimental principle. This value was then substituted into a separate formula to determine the final MDA content in the plant tissue sample.
Note: C is the concentration of MDA (μmol/L); V is the volume of the extraction solution (mL); m is the fresh weight of leaves (g).
2.5 Determination of catalase activity after stimulation by leaf cutting and snail bite
Other reported papers had been used to determine catalase activity (Zhang et al., 2015; Yin et al., 2022), but it had been optimized in this study. A 0.5 g sample was weighed and homogenized with 5.0 mL of extraction buffer solution under ice bath conditions. The homogenate was centrifuged at 4°C for 30 minutes at 12,000 rpm. The supernatant, containing the enzyme extract, was collected, and its total volume measured. The extract was stored at a low temperature for further use. The enzymatic reaction system was prepared by combining 2.9 mL of 20 mmol/L H2O2 solution with 100 μL of the enzyme extract. Distilled water was used as the reference blank. The initial optical density (OD0) of the reaction system was measured at 240 nm after 15 seconds of reaction. The optical density (OD2) was then measured again at 2 minutes, and this process was repeated three times. The change in optical density (ΔOD) per minute was calculated based on the obtained values.
Note: △OD is the change value of the optical density of the reaction mixture per minute, OD2 is the optical density value of the reaction mixture for 2 minutes, and OD0 is the initial optical density value of the reaction mixture, t2 is the end time of the reaction, t1 is the initial time of the reaction. The following formula was used to quantify CAT activity:
Where, U refers to CAT activity, V is the total volume of sample extract (mL), Vs is the measured volume of sample extract (mL), and m is the sample mass (g).
In addition, a standard curve was generated using different concentrations of H2O2. CAT activity was then expressed by the consumption of substrate H2O2 solution, as determined by the change in optical density value relative to the standard curve.
2.6 Determination of peroxidase activity after stimulation by leaf cutting and snail bite
Based on the experimental methods of others (Sahu et al., 2010; Zhang et al., 2015), the method for measuring peroxidase activity had been improved as follows. One gram of plant material was homogenized with 10 mL of 20 mmol/L KH2PO4solution in a mortar and pestle. After centrifugation at 4000 rpm for 15 minutes, the supernatant (enzyme solution) was collected and stored at a low temperature. Two cuvettes were prepared: a control cuvette containing 3 mL of reaction mixture and 1 mL of KH2PO4solution, and a test cuvette containing 3 mL of reaction mixture and 1 mL of the enzyme solution. Following enzyme addition, the change in optical density (ΔOD) at 470 nm was measured every minute for 6 minutes, starting immediately and repeated three times. POD activity was then expressed in units of U.
2.7 Data analysis
The average of tree height and ground diameter were combined, and their variance and multiple comparisons were analyzed. Similarly, the average of MDA content, POD activity, and CAT activity were analyzed using two-way analysis of variance (ANOVA) followed by Tukey’s Honestly Significant Difference (HSD) test for pairwise comparisons (P = 0.05). Principal component analysis (PCA) selects principal components based on the criteria of cumulative contribution rate exceeding 70% and eigenvalues greater than 1, and then conducts detailed analysis and plotting according eigenvalues, contribution rates, and cumulative contribution rates of the selected principal components. All statistical analyses were performed using SPSS 20.0 (IBM SPSS Statistics, Chicago, IL, USA), and data visualization was done with Origin 2018 (OriginLab Inc., Northampton, UK).
3 Results and analysis
3.1 The tree height and ground diameter of S. oblonga and the phenomenon of A. fulica feeding hazards
In the present study, the average of plant reached a height of 112.77 ± 1.02 cm and a ground diameter of 1.65 ± 0.02 cm. Details regarding this survey data can be found in Supplementary Table S1. Sanchezia oblonga faces challenges during garden maintenance. Trimming machinery frequently stimulates the plant, and concurrently, the giant African snail, A. fulica, significantly damages its leaves through feeding. This extensive leaf loss (Figure 2) disrupted the plant’s normal photosynthesis and reduced its aesthetic value. We found that healthy S. oblonga leaves weighed an average of 4.87 ± 0.03 g, while leaves eaten by snails for 3 hours had an average weight of 4.22 ± 0.04 g. In those 3 hours, the snails gained an average weight of 0.65 ± 0.02 g, translating to an average hourly food intake of 0.22 ± 0.01 g (Supplementary Table S2).
3.2 Stress-induced temporal changes of malondialdehyde content in leaves of S. oblonga
From the results of two-way ANOVA, there is a significant difference in the effect of the time factor on the malondialdehyde (MDA) content after stress, and the main effect exists (P < 0.05). However, there was no significant difference in the effect of stress treatment factors on the MDA content (P > 0.05). The p-value of time*stress treatment is also greater than 0.05, indicating that there is no significant difference in the interaction between the two factors of time and stress mode on the MDA content.
The effect of leaf-cutting stress and snail bite timing treatment on the MDA content in the leaves of S. oblonga was significantly different (P < 0.05). Under the same stress, different time sequence treatments had different effects on the MDA content of the leaves: 3 h (0.00090) > 9 h(0.00066) > 15 h (0.00041 μmol·L-1), and after snail bite treatment: 15 h (0.00074) > 3 h (0.00058) > 9 h (0.00057 μmol·L-1) (Figure 3).
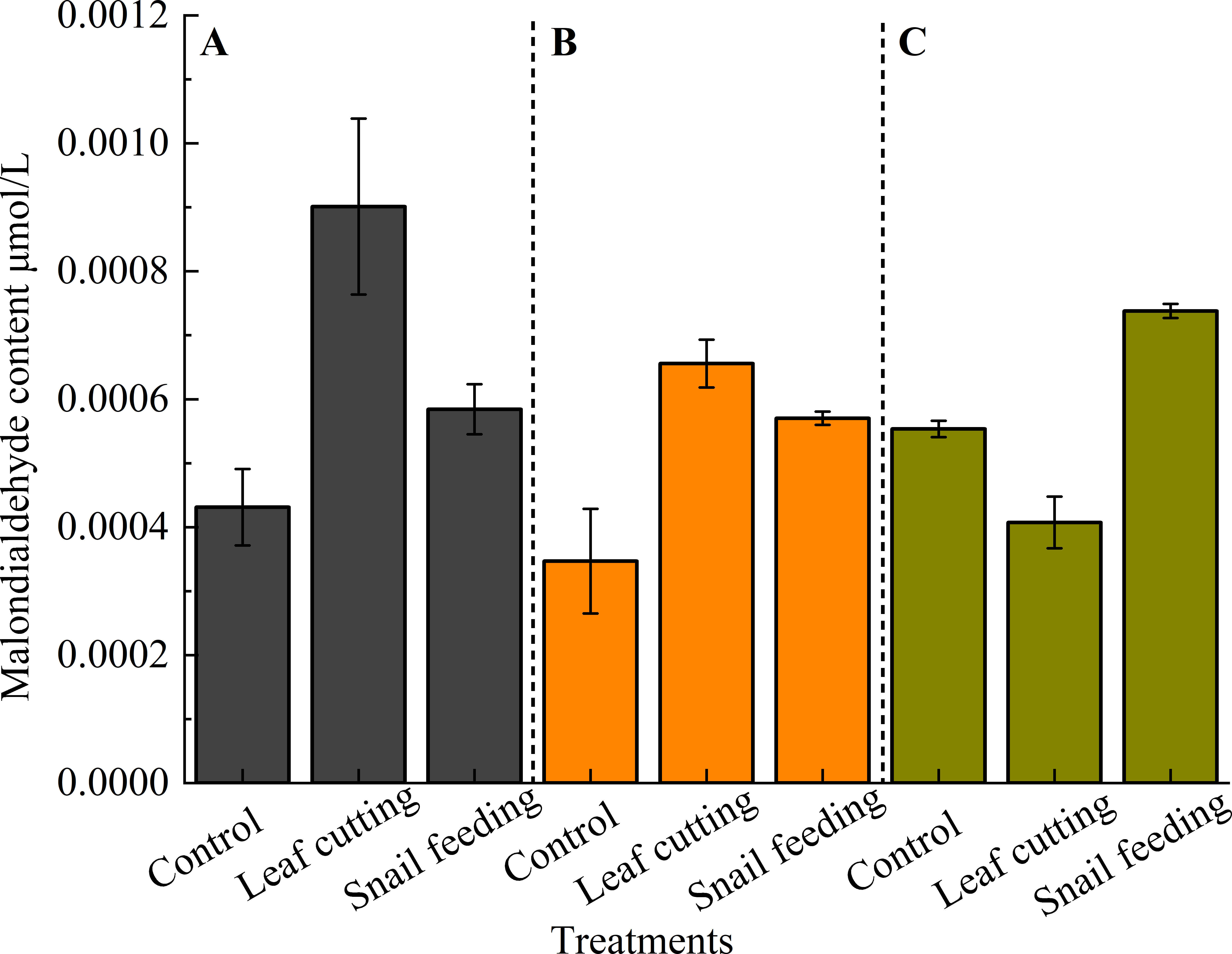
Figure 3. The MDA content of S. oblonga leaves under different stress conditions. (A) refers to the duration of 3 hours after stress, (B) refers to the duration of 9 hours after stress, and (C) refers to the duration of 15 hours after stress. Different letters indicated that the MDA content of leaves of different groups had significant difference at α = 0.05 level for the same time after stress.
Three hours after stress, the level of MDA in the leaves followed this order: leaf cutting (0.00090) > snail bite (0.00058) > control (0.00043 μmol·L-1). A similar pattern emerged after 9 hours of stress, with leaf cutting (0.00066) > snail bite (0.00057) > control (0.00035 μmol·L-1). Interestingly, the trend reversed at 15 hours, where snail bite (0.00074) > control (0.00055) > leaf cutting (0.00041 μmol·L-1) (Figure 3).
3.3 Stress-induced temporal changes of catalase activity in leaves of the S. oblonga
According the results of two-way ANOVA, it can be seen that the time factor after stress has a significant impact on catalase (CAT) activity (P < 0.05). The impact of stress treatment factors on CAT activity also showed significant differences (P < 0.05). The p-value of time*stress treatment is less than 0.05, indicating a significant difference in the interaction between the two factors of time and stress treatment on CAT activity.
Three hours after stress, CAT activity followed the order: snails bite (0.4500 U) > leaf cutting (0.2814 U) > control (0.1829 U). This pattern remained comparable at 9 hours, with snail bite (0.3657 U) showing the highest activity, followed by leaf cutting (0.2900 U) and control (0.2143 U). Interestingly, the trend reversed at the 15-hour mark, where the control group exhibited the highest CAT activity (0.3557 U), surpassing leaf cutting (0.3014 U) and snails bite (0.2329 U) (Figure 4).
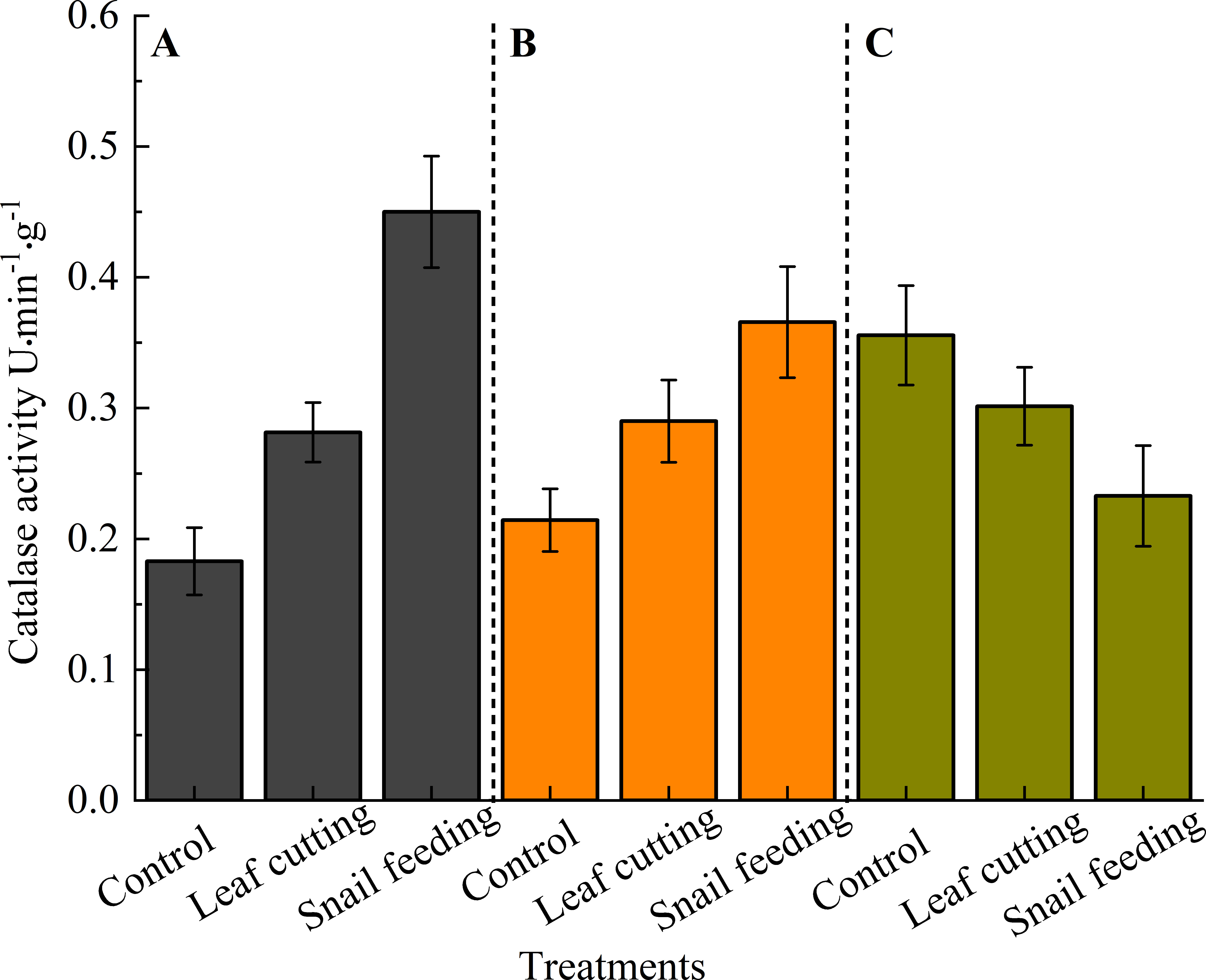
Figure 4. The CAT activity of S. oblonga leaves under different stress conditions. (A) refers to the duration of 3 hours after stress, (B) refers to the duration of 9 hours after stress, and (C) refers to the duration of 15 hours after stress. Different letters indicated that the CAT activity of leaves of different groups had significant difference at α = 0.05 level for the same time after stress.
The pattern of CAT activity after sequential treatment of healthy leaves followed: 15 h (0.3557 U) > 9 h (0.2143 U) > 3 h (0.1829 U). In contrast, leaf-cutting displayed a different trend: 15 h (0.3014 U) > 9 h (0.2900 U) > 3 h (0.2814 U). Interestingly, snail feeding exhibited a contrasting pattern: 3 h (0.4500 U) > 9 h (0.3657 U) > 15 h (0.2329 U).
3.4 Stress-induced temporal changes of peroxidase activity of S. oblonga leaves
From the results of the two-way analysis of ANOVA, it can be seen that there are significant differences in the effects of time factors and stress treatment factors on peroxidase (POD) activity after stress (P < 0.05). The p-value of time*stress treatment is less than 0.05, indicating a significant difference in the interaction between the two factors of time and stress treatment on POD activity.
POD activity was highest following 3 hours of snail bite stress (1.6580 U), followed by leaf cutting (1.4027 U) and the control (1.1505 U). Leaf-cutting stress resulted in the highest POD activity at 9 hours (1.7636 U) compared to the control (1.7095 U) and snail bite stress (0.5963 U). Finally, at 15 hours, the control group had the highest POD activity (1.6166 U), followed by leaf cutting (1.4083 U) and snail bite stress (0.1334 U) (Figure 5). After leaf cutting, POD activity followed the order: 9 h (1.7636 U) > 15 h (1.4083 U) > 3 h (1.4027 U). Similarly, after snail bites, POD activity was highest at 3 h (1.6580 U), followed by 9 h (0.5963 U) and 15 h (0.1334 U).
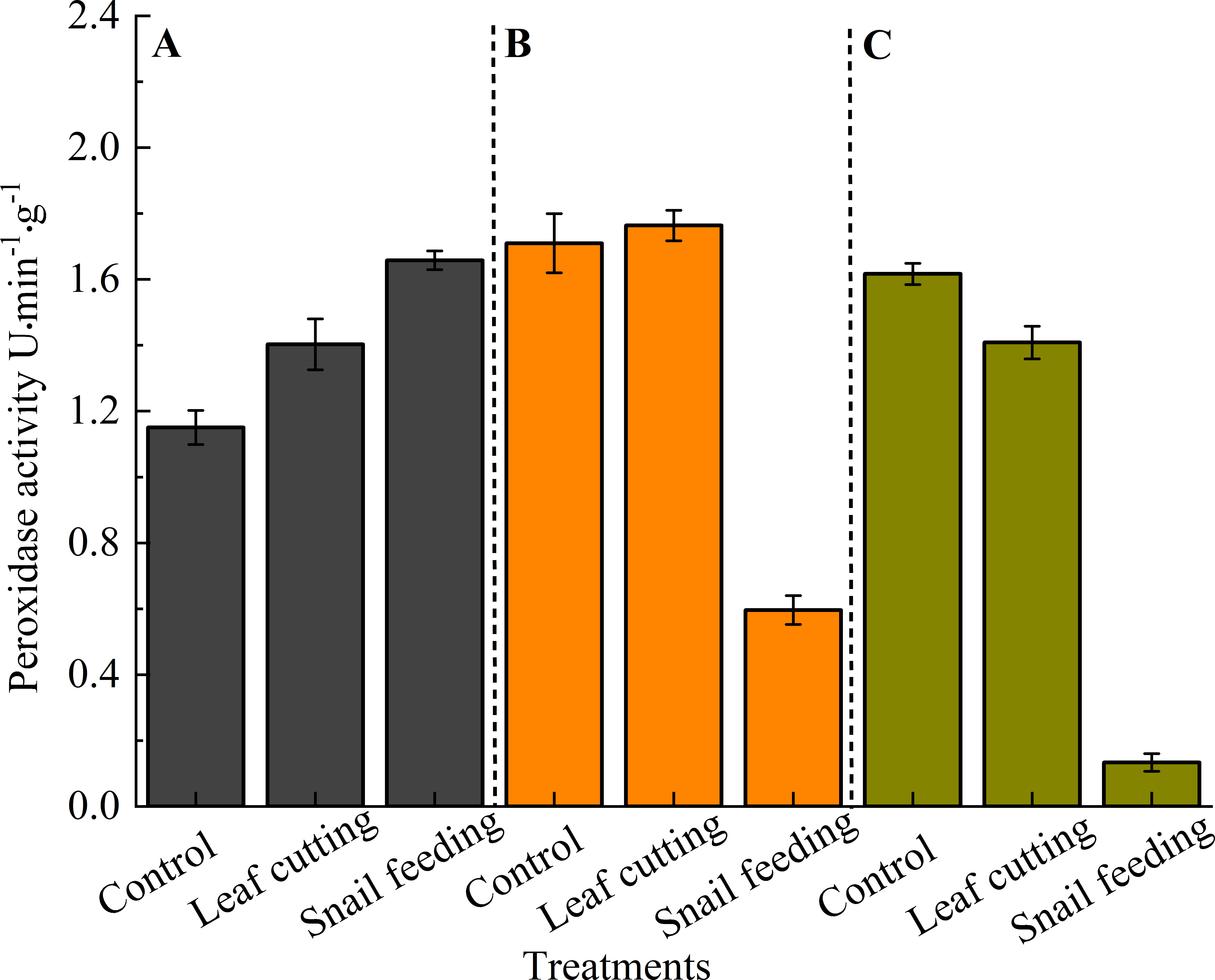
Figure 5. The POD activity of S. oblonga leaves under different stress conditions. (A) refers to the duration of 3 hours after stress, (B) refers to the duration of 9 hours after stress, and (C) refers to the duration of 15 hours after stress. Different letters indicated that the POD activity of leaves of different groups had significant difference at α = 0.05 level for the same time after stress.
3.5 Principal component analysis of the ecological adaptability of Sanchezia oblonga
Figure 6 shows that the malondialdehyde (MDA) content and the catalase (CAT) activity are the main factors affecting the ecological adaptability of S. oblonga to biotic and abiotic stress. After being subjected to biotic and abiotic stress for 3, 9, and 15 hours, the eigenvalue of MDA content were the highest, followed by CAT activity, and the lowest was peroxidase (POD) activity. After being subjected to stress for three different durations, the contribution rate of the first principal component (MDA content) was the highest, and the contribution rate of the second principal component (CAT activity) was also higher. The cumulative contribution rate of the two principal components (MDA content and CAT activity) exceeded 95%, while the contribution rate of POD activity was the lowest.
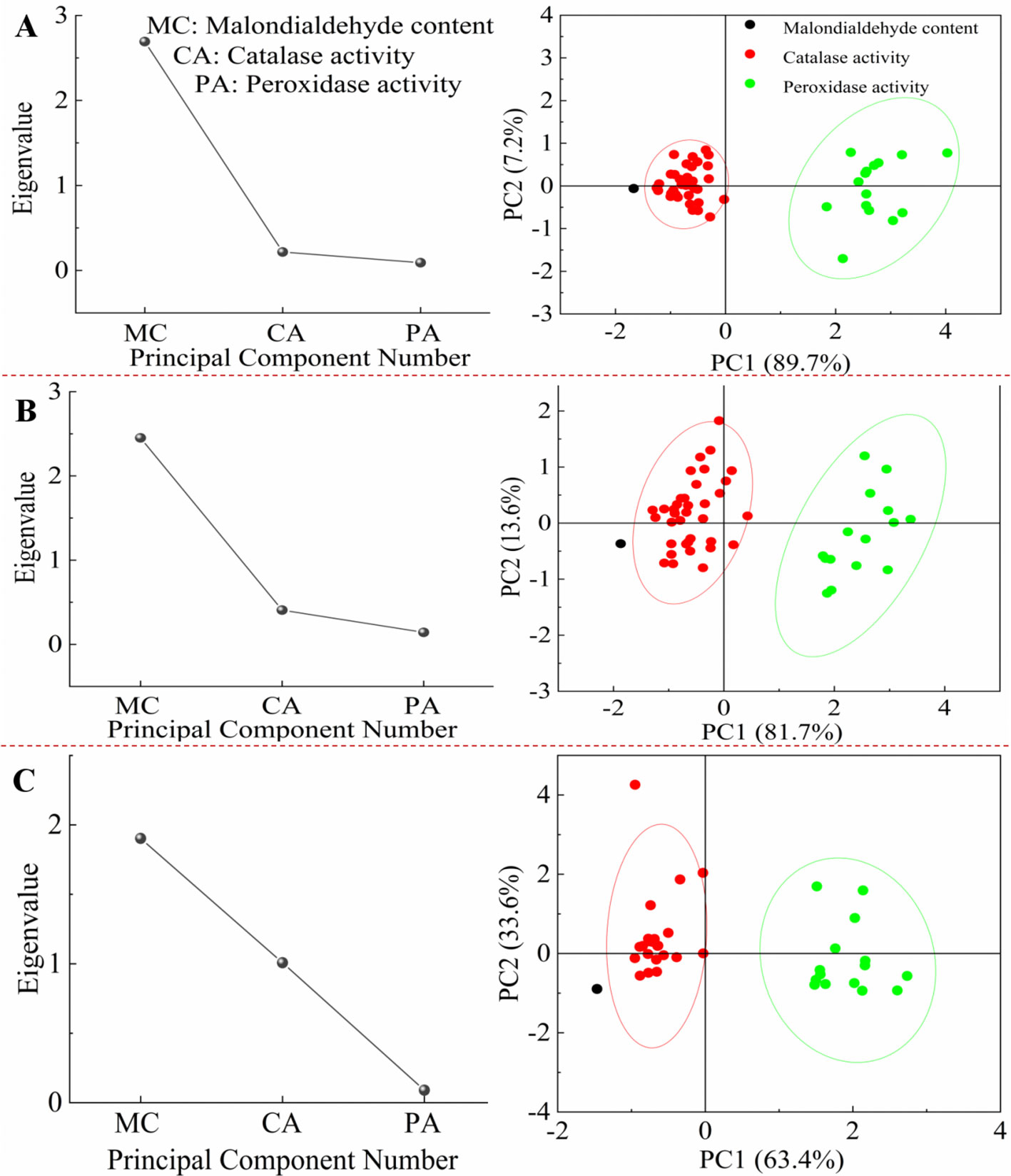
Figure 6. The scree plot and score plot analysis of the ecological adaptability of S. oblonga under different stress conditions. (A) refers to the duration of 3 hours after stress, (B) refers to the duration of 9 hours after stress, and (C) refers to the duration of 15 hours after stress.
After being subjected to biotic and abiotic stress for three different durations, the eigenvalue of the first principal component (MDA content) showed a continuous decreasing trend (2.6920, 2.4512, and 1.9025), while the eigenvalue of the second principal component (CAT activity) showed a continuous increasing trend (4.701, 0.2170, 0.4069, and 1.0077). After being subjected to biotic and abiotic stress for 3 hours, the contribution rate of the first principal component (MDA content) was 89.7324%, and the contribution rate of the second principal component (CAT activity) was 7.2332%. The cumulative contribution of the two principal components was 96.9656%. After 9 hours of stress, the contribution rate of the first principal component (MDA content) was 81.7051%, the contribution rate of the second principal component (CAT activity) was 13.5638%, and the cumulative contribution of the two principal components was 95.2689%. After 15 hours of stress, the contribution rate of the first principal component (MDA content) was 63.4512%, the contribution rate of the second principal component (CAT activity) was 33.5892%, and the cumulative contribution of the two principal components was 97.0044%.
4 Discussion
Sanchezia oblonga, a significant gardening plant in southern China, faced threats from both biotic and abiotic stresses (Deng, 2000; Deng, 2001; Lin and Zhang, 2001; Deng and Deng, 2002; Liang et al., 2010; Xie et al., 2022). This study have investigated the ecological adaptability of S. oblonga to biotic and abiotic stress in urban gardens. Achatina fulica is a major biotic stressor, with a documented fondness for S. oblonga. Its large appetite can lead to extensive leaf damage across affected areas. Furthermore, the size of A. fulica is large, long lifespan, rapid growth, and prolific reproduction contribute to its agricultural and forestry pest status. Its wide feeding range, encompassing over 500 plant species, results in significant economic losses (Guo et al., 2015). In addition to snail feeding, S. oblonga encounters abiotic stress during cultivation and management practices, such as the mechanical stimulation caused by pruning. Therefore, explores the defense response of S. oblonga to both biotic (snail feeding) and abiotic (pruning) stresses have been explored in this study.
The application of various stress treatments (biotic and abiotic) and time sequences following stress exposure significantly impacted the content and activity of growth-related substances in S. oblonga compared to the control group. Our experimental results revealed that different stresses and post-stress time points exerted distinct effects on the in vivo responses of S. oblonga leaves. Notably, malondialdehyde (MDA) content, catalase (CAT) activity, and peroxidase (POD) activity exhibited dynamic changes over time following insect feeding and mechanical injury. Both snail feeding and leaf cutting triggered defense responses in S. oblonga, with some responses exhibiting a time lag. However, the changes in the content and activity of defense-related substances differed between biotic and abiotic stresses over time. Both animal feeding and leaf cutting stress can induce more persistent defense responses in S. oblonga, which may be due to the synthesis of more defense substances and/or enhanced activity of defense enzymes induced by biotic and abiotic stress. The differential effects of biotic and abiotic stresses on plants can be attributed to the distinct mechanisms by which these stresses induce damage. Mechanical leaf cutting inflicts solely physical injury, whereas animal feeding, a form of biotic stress, not only causes physical damage but also introduces chemical damage via saliva and other secretions (Lou and Cheng, 1997). This study confirmed the presence of mucus produced by snails feeding on S. oblonga leaves. The chemical stimuli present in snail secretions are likely responsible for the more rapid activation of defense responses in S. oblonga observed in this study.
Animal feeding stress elicited a more sustained defense response and increased MDA synthesis in S. oblonga compared to the transient response induced by mechanical leaf cutting. MDA, as the end product of membrane lipid peroxidation, reflects the extent of membrane damage and the plant’s overall stress response (Li et al., 2010). Leaf-cutting directly inflicts mechanical injury on plant cells, triggering a defense response. However, insect feeding introduces an additional layer of complexity. Beyond physical damage from feeding wounds, insect saliva contains a cocktail of effectors that target various components of the plant’s Pathogen-Triggered Immunity (PTI) pathway. These effectors manipulate the plant’s immune response by inhibiting or suppressing specific defense mechanisms to facilitate insect feeding (Dong et al., 2021). Examples include NcSP84 from the black-tailed leafhopper (Nephotettix bipunctatus) and NlSEF1 from the brown planthopper (Nilaparvata lugens), which prevent screen tube blockage or inhibit calcium signaling by chelating free calcium ions (Hattori et al., 2012; Ye et al., 2017). During snail feeding, plant cells are subjected to both physical and chemical stimuli. It is highly conceivable that the components in snail saliva likely trigger a more rapid defense response and enhanced synthesis of defense substances in S. oblonga. While both stimuli ultimately induce defense responses, the response timing differs between leaf-cutting and snail feeding. The mechanical damage caused by leaf cutting elicits a faster initial response in terms of MDA synthesis compared to snail feeding. This observation aligns with the findings of other reported, who proposed that the action time of mechanical damage differs between leaf-cutting and insect feeding (Yuan et al., 2009). Insect feeding is a gradual process that inflicts damage over time, while leaf cutting delivers a single, immediate physical injury devoid of the secretory components associated with insect feeding.
Plant defense enzymes (catalase and peroxidase) play critical roles in plant stress tolerance and adaptation, positively impacting plant growth and development. Their activity can be harnessed as a physiological indicator to assess a plant’s stress resistance (Ding et al., 2013). During normal plant cell metabolism, both biotic and abiotic stresses elevate cellular levels of reactive oxygen species (ROS) and peroxides. The interaction between CAT and POD contributes to the ecological adaptation of plants to different stress conditions. After 3 h of S. oblonga under stress, both CAT and POD were higher in snail feeding than in leaf cutting. After 15h, the situation was reversed; the lowest activity of CAT and POD was in snail feeding treatment. But after 9 hours of treatment, CAT activity is higher in snail feeding, while POD activity is higher in leaf cutting. From the above, it can be seen that the two enzymes undergo dynamic changes in plant response to stress. CAT and POD are essential protective enzymes, safeguarding cells from ROS-mediated damage. They function by scavenging O2-, H2O2, and peroxides, preventing or minimizing the formation of highly destructive hydroxyl radicals. Consequently, they maintain the integrity of the cellular membrane system (Guo et al., 2015). Additionally, POD plays a role in lignin biosynthesis, pathogen defense, and cell wall polysaccharide cross-linking, further contributing to plant stress resistance (Shasmitaa et al., 2019).
Both snail feeding and leaf-cutting stimulation significantly enhanced the activities of catalase and peroxidase compared to the control group, indicating that both biotic and abiotic stresses triggered defense responses in S. oblonga. This response involves increased activity of antioxidant enzymes to mitigate the oxidative stress caused by cellular damage. While both stresses induce mechanical damage, animal feeding, a form of biotic stress, introduces an additional layer of complexity: chemical stimulation by insect saliva. Dong Yumei et al. demonstrated that insect secretions, including saliva, oviposition fluids, feces, and honeydew, contain compounds that can be recognized by plant Pattern Recognition Receptors (PRRs) on the cell membrane (Dong et al., 2021). These signals activate defense signaling pathways, leading to a more intricate defense response than purely mechanical damage. As McCloud and Baldwin highlighted, plant recognition of herbivorous insects is primarily driven by specific elicitors in insect saliva. These elicitors trigger specific signaling cascades within the plant, ultimately inducing the expression of defense genes and the accumulation of toxic metabolites and defense proteins (Mccloud and Baldwin, 1997). Notably, in this study, the catalase and peroxidase activity enhancement in response to snail feeding lagged behind that observed with leaf-cutting stimulation. This suggests that snail feeding elicits a delayed but ultimately robust activation of defense enzymes. Leaf-cutting represents a simple, rapid, and short-term physical stimulus. Conversely, animal feeding encompasses a continuous, comprehensive process involving mechanical injury and chemical stimulation via saliva (Yuan et al., 2009). A similar pattern was reported on Dendrolimus tabulaeformis, where the plant’s defense response to insect feeding exhibited an “on-off effect” (Shi et al., 2017). In other words, the plant’s defense system activates upon reaching a certain threshold of external stimuli but may be deactivated if the stimulus becomes excessive (Shi et al., 2017).
Achatina fulica, a terrestrial mollusk listed among the 100 worst invasive species by the International Union for Conservation of Nature (IUCN), poses a significant threat to landscaping plants (Guo et al., 2015). Effective control strategies for A. fulica are crucial for successful cultivation and management of ornamental plants. Current control methods, including quarantine, pesticides, and manual trapping, have limited research and may not be optimal solutions (Tian et al., 2014). However, promising new avenues are emerging. The development of a novel A. fulica baiting agent formulated with extracts from two invasive plants (Ipomoea cairica and Mikania micrantha) combined with snail pheromones, demonstrating promising efficacy was reported (Wang et al., 2022). Based on a new phenomenon in which A. fulica prefer to feed on S. oblonga, future research could explore the potential of plant-derived attractants based on the volatile compounds of S. oblonga for A. fulica control.
5 Conclusion
Understanding the ecological adaptability of landscaping plants to both biotic stress or abiotic stress is critical for optimizing cultivation practices, stress-resistant breeding programs, and integrated pest management strategies. This study revealed that the Achatina fulica significantly damages S. oblonga leaves by consuming large quantities of foliage, compromising the plant’s aesthetic value. Our findings demonstrate that both biotic and abiotic stresses significantly impact plant defense responses, with biotic stresses eliciting a stronger response compared to abiotic stresses. Malondialdehyde (MDA), catalase (CAT), and peroxidase (POD) play crucial roles in plant stress responses and can be important targets for stress-resistance breeding programs in ornamental plants. Both leaf cutting (abiotic stress) and snail feeding (biotic stress) stimulated increased synthesis of MDA, a defense compound, in the leaves and significantly affected catalase and peroxidase activities. These findings provide a new perspective on understanding plant ecological adaptability and an important theoretical foundation for optimizing landscaping plant cultivation, stress resistance breeding, and integrated pest management strategies.
Data availability statement
The original contributions presented in the study are included in the article/Supplementary Material. Further inquiries can be directed to the corresponding author/s.
Author contributions
CC: Conceptualization, Methodology, Project administration, Writing – original draft, Writing – review & editing. YP: Investigation, Methodology, Writing – original draft. LL: Data curation, Investigation, Writing – original draft. XC: Data curation, Investigation, Writing – original draft. ZC: Data curation, Investigation, Writing – original draft. XM: Data curation, Writing – original draft. DY: Data curation, Writing – original draft. YW: Data curation, Writing – original draft.
Funding
The author(s) declare that financial support was received for the research and/or publication of this article. This research was supported by the 2023 Zhaoqing Science and Technology Special Project (2023N010), the 2024 Science and Technology Innovation Guidance Project of Zhaoqing (241223100090425), the 2023 Scientific Research Fund Funding Project of Zhaoqing university (QN202331), and the 2023 Doctoral Initiation Fund Project of Zhaoqing university (611/230009).
Conflict of interest
The authors declare that the research was conducted in the absence of any commercial or financial relationships that could be construed as a potential conflict of interest.
Generative AI statement
The author(s) declare that no Generative AI was used in the creation of this manuscript.
Publisher’s note
All claims expressed in this article are solely those of the authors and do not necessarily represent those of their affiliated organizations, or those of the publisher, the editors and the reviewers. Any product that may be evaluated in this article, or claim that may be made by its manufacturer, is not guaranteed or endorsed by the publisher.
Supplementary material
The Supplementary Material for this article can be found online at: https://www.frontiersin.org/articles/10.3389/fevo.2025.1514533/full#supplementary-material.
References
Atkinson N. J., Lilley C. J., Urwin P. E. (2013). Identification of genes involved in the response of Arabidopsis to simultaneous biotic and abiotic stresses. Plant Physiol. 162, 2028–2041. doi: 10.1104/pp.113.222372
Bailly C., Benamar A., Corbineau F., Come D. (2010). Changes in malondialdehyde content and in superoxide dismutase, catalase and glutathione reductase activities in sunflower seeds as related to deterioration during accelerated aging. Physiol Plant 97, 104–110. doi: 10.1111/j.1399-3054.1996.tb00485.x
Chuang H. W., Harnrak A., Chen Y. C., Hsu C. M. (2010). A harpin-induced ethylene-responsive factor regulates plant growth and responses to biotic and abiotic stresses. Biochem. Biophys. Res. Commun. 402, 414–420. doi: 10.1016/j.bbrc.2010.10.047
Deng Q. C., Deng Q. L. (2002). Key cultivation points of sanchezia oblonga. Southwest Hortic 30, 40.
Ding Y. M., Ma L. H., Zhou X. G., Yao C. X., Dong L. F., Sun M. L., et al. (2013). Change of proline and malondialdehyde content in potato leaves under drought stress and correlation analysis with drought tolerance. J. Southwest Agric. 26, 106–110.
Dong Y. M., Zhang M. Q., Shen H., Huang X. G., Yang Y. X., Li J. F., et al. (2021). Research progress on salivary effectors and elicitors of phytophagous insects. Acta Entomol Sin. 64, 982–997. doi: 10.16380/j.kcxb.2021.08.010
Guo J., Zhang J. E., Wu R. S., Zhao B. L., Yang H. R. (2015). Research status and prospect of Achatina fulica in China. J. South. Agric. 46, 626–630. doi: 10.3969/j:issn.2095-1191.2015.4.626
Hattori M., Nakamura M., Komatsu S., Tsuchihara K., Tamura Y., Hasegawa T., et al. (2012). Molecular cloning of a novel calcium-binding protein in the secreted saliva of the green rice leathopper Nephotettix cincticeps. Insect Biochem. Mol. Biol. 42, 1–9. doi: 10.1016/j.ibmb.2011.10.001
Li Z. H., Dong X. H., Tong F. P. (2010). Four season changes and stress resistance of protective enzymes and malondialdehyde in leaves of Broussonetia papyrifera in mining area. J. Cent. South Univ. For Technol. 30, 106–109 + 138. doi: 10.14067/j.cnki.1673-923x.2010.05.031
Liang X., Zhang J. M., Ruan J. C. (2010). Cultivation and management of acanthaceae. Chin. Hortic. Abstr 26, 105 + 78.
Lin Y. J., Zhang X. R. (2001). Sanchezia oblonga is a good leaf viewing. Bonsai Flower Trees 11), 28–29.
Lou Y. G., Cheng J. A. (1997). Induced insect resistance of plants. Acta Entomol Sin. 40, 320–331. doi: 10.16380/j.kcxb.1997.03.018
Lv S., Zhang Y., Liu H. X., Hu L., Yang K., Steinmann P., et al. (2009). Invasive snails and an emerging infectious disease: Results from the first national survey on Angiostrongylus cantonensis in China. PloS Neglected Trop. Dis. 3, e368. doi: 10.1371/journal.pntd.0000368
Mccloud E. S., Baldwin I. T. (1997). Herbivory and caterpillar regurgitants amplify the wound-induced increases in jasmonic acid but not nicotine in nicotiana sylvestris. Planta 203, 430–435. doi: 10.1007/s004250050210
Sahu S., Das P., Ray M., Sabat S. C. (2010). Osmolyte modulated enhanced rice leaf catalase activity under salt-stress. Adv. Biosci Biotechnol. 1, 39–46. doi: 10.4236/abb.2010.11006
Shasmitaa B., Debasish M., Pradipta K. M., Mohapatra D., Mohapatra P. K., Naik S. K., et al. (2019). Priming with salicylicacid induces defense against bacterial blight disease by modulating riceplant photosystem I and antioxidant enzymes activity. Physiol. Mol. Plant Pathol. 108, 101427–101437. doi: 10.1016/j.pmpp.2019.101427
Shi Y. Y., Feng J. Z., Yu L. H., Gao B. J. (2017). Induction effect of insect feeding and leaf cutting stimulation on some defense substances in Pinus tabulaeformis needles. J. Hebei Agric. Univ. 40, 81–86. doi: 10.13320/j.cnki.jauh.2017.0015
Tang H. M., Liao L. J. (2013). The species of Acanthaceae and their application in gardens. Modern Agric. Sci. Technol. 9), 194–195.
Tian Z. L., Huang X. D., Li W. Y. (2014). Preliminary study on occurrence characteristics and control methods of Achatina fulica. Friends Farmers Getting Rich 4), 58.
Wang X. F., Li Z. Y., Liu B., Qiao X., Wan F. H., Qian W. Q., et al. (2022). Research on a new bait and kill agent for Achatina fulica based on invasive plants and snail pheromones. J. BSL 31, 289–294. doi: 10.3969/j.issn.2095-1787.2022.03.014
Wang X., Sun H., Tan C., Wang X. W., Xia M. (2021). Effects of film mulching on plant growth and nutrients in artificial soil: a case study on high altitude slopes. Sustainability 13, 1–15. doi: 10.3390/su131911026
Xie Q. L., Huang X. T., Wang J. (2022). Analysis on the application of Acanthaceae ornamental plants in Zhanjiang gardens. Modern GDN 45, 157–160.
Ye W. F., Yu H. X., Jian Y. K., Zeng J. M., Ji R., Chen H. D., et al. (2017). A salivary EF-hand calcium-binding protein of the brown planthopper Nilaparvata lug ens functions as an effector for defense responses in rice. Sci. Rep. 7, 40498. doi: 10.1038/srep40498
Yin Y. Q., Tian X., Yang J., Yang Z. F., Tao J., Fang W. M. (2022). Melatonin mediates isoflavone accumulation in germinated soybeans (Glycine max L.) under ultraviolet-B stress. Plant Physiol. Biochem. 175, 23–32. doi: 10.1016/j.plaphy.2022.02.001
Yuan H. E., Yan S. C., Tong L. L., Gao L. L., Wang Y. J. (2009). Differences in the induction of condensed tannins in Larix gmelinii needles by leaf cutting injury and insect feeding. J. Ecol. 29, 1415–1420.
Zhang X. H., Yu X. Z., Yue D. M. (2015). Phytotoxicity of dimethyl sulfoxide (DMSO) to rice seedlings. Int. J. Environ. Sci. Technol. 13, 607–614. doi: 10.1007/s13762-015-0899-6
Zhao Y. N., Sun T. H., Liu J., Zhang R. B., Yu Y. J., Zhou G. N., et al. (2024). The key role of plant hormone signaling transduction and flavonoid biosynthesis pathways in the response of Chinese pine (Pinus tabuliformis) to feeding stimulation by pine caterpillar (Dendrolimus tabulaeformis). Int. J. Mol. Sci. 25, 6354. doi: 10.3390/ijms25126354
Keywords: leaf-cutting stimulation, feeding stress, Achatina fulica, malondialdehyde, catalase
Citation: Chen C, Peng Y, Liu L, Cai X, Cao Z, Mu X, Yan D and Wen Y (2025) The ecological adaptability of Sanchezia oblonga to biotic and abiotic stress in urban gardens. Front. Ecol. Evol. 13:1514533. doi: 10.3389/fevo.2025.1514533
Received: 21 October 2024; Accepted: 31 March 2025;
Published: 24 April 2025.
Edited by:
Ziming Ma, Max Planck Institute of Molecular Plant Physiology, GermanyReviewed by:
Aliya Baidourela, Xinjiang Agricultural University, ChinaSanja Manitašević Jovanović, University of Belgrade, Serbia
Copyright © 2025 Chen, Peng, Liu, Cai, Cao, Mu, Yan and Wen. This is an open-access article distributed under the terms of the Creative Commons Attribution License (CC BY). The use, distribution or reproduction in other forums is permitted, provided the original author(s) and the copyright owner(s) are credited and that the original publication in this journal is cited, in accordance with accepted academic practice. No use, distribution or reproduction is permitted which does not comply with these terms.
*Correspondence: Cong Chen, Y29uZ2NoZW4yMDA3QDEyNi5jb20=