- 1Institute of Biology/Geobotany and Botanical Garden, Martin Luther University Halle-Wittenberg, Halle, Germany
- 2Herbario Nacional de Bolivia, Instituto de Ecología, Carrera de Biología, Universidad Mayor de San Andrés, La Paz, Bolivia
- 3Santiago de Chirca Biological Station, La Paz, Bolivia
- 4Carrera de Biología, Universidad Mayor de San Andrés, La Paz, Bolivia
- 5German Centre for Integrative Biodiversity Research (iDiv) Halle-Jena-Leipzig, Leipzig, Germany
Introduction: Tropical montane forests are diverse ecosystems increasingly threatened by land-use change and frequent uncontrolled fires caused by humans. After these fires, affected areas are often dominated by ferns of the genus Pteridium (bracken), delaying the rate of forest regeneration. This study examined the facilitative and competitive roles of bracken fronds and litter on seedling establishment, recruitment success, and growth after 36 months, and the extent to which these effects depend on seed size in early plant development processes.
Methods: At eight sites, we conducted seed addition and seedling-transplant experiments in plots established in bracken-dominated areas, using the following treatments: (a) fronds and litter intact (F+L+); (b) fronds intact and litter removed (F+L−); (c) fronds removed and litter intact (F−L+); and (d) fronds and litter removed (F−L−).
Results: A total of 46,640 seeds from 24 tree species were sown and 1,070 nursery-raised seedlings transplanted. In treatments with fronds and litter, seedling establishment and recruitment success increased with seed size. In contrast, in the treatment without fronds and litter all the species had low performance. Fronds proved to be highly beneficial for all seed sizes and for all early development processes. Litter, on the other hand, had negative effects on small-seeded species during establishment, but had neutral to positive effects on the recruitment and growth of all species.
Discussion: The disadvantage of small seeds under bracken litter may be related to the high light requirements of seedlings, as litter accumulation reduces light transmission. Species with large seeds and shade tolerance benefit from bracken frond cover, suggesting that restoration strategies in bracken-dominated areas should prioritize these species. Moreover, planting seeds or seedlings shortly after fire, in the presence of bracken fronds and before litter accumulation would be most beneficial for tree species. Additionally, seed dispersers play a crucial role in transporting large seeds into bracken-dominated areas, emphasizing the need for an integrated approach that considers biotic and abiotic factors for effective forest recovery.
1 Introduction
Tropical forests harbor about 50% of the world’s species (Dirzo and Raven, 2003; Pillay et al., 2022). However, their high diversity is threatened by land-use change, which leads to increased deforestation (Achard et al., 2002; Phillips et al., 2017). Agricultural expansion through slash-and-burn techniques often results in uncontrolled fires and extensive fire-deforested areas (Gardner et al., 2009). After fire, some affected regions are dominated by ferns of the genus Pteridium (hereafter referred to as bracken) (Marrs et al., 2000). Historically, fire has been a component of the disturbance dynamics in tropical montane forests (Crausbay et al., 2014). However, the frequency and intensity of fires have increased due to human activity and climate change, leading to environments with fire-dependent vegetation (Asbjornsen and Wickel, 2009). In these environments, superdominant species may become established, with particular concern for bracken, which can sustain continuous fire and hinder forest regeneration (Stewart et al., 2008). Bracken is one of most widespread fern genera in the world, present in temperate an tropical regions (Tryon, 1941; Marrs et al., 2000). However, its occurrence and dominance in tropical montane humid forests are mainly associated with disturbances such as abandoned cultivation, and fires which promote its proliferation (Levy-Tacher et al., 2015; Ssali et al., 2017). In tropical bracken-dominated areas, forest regeneration is slow (Hartig and Beck, 2003; Palomeque et al., 2017), and is often disrupted (Christmann et al., 2023), making ecosystem recovery considerably more difficult. However, the role of bracken in forest succession is unclear, particularly whether it favors or competes with other species during the forest regeneration process.
In plant communities, positive (i.e facilitation) and negative (i.e competition) interactions occur simultaneously (Callaway, 1997; Holmgren et al., 1997). Facilitation occurs when the presence of one plant (the nurse) enhances the fitness of another plant (the beneficiary) by improving microclimatic conditions (Holmgren et al., 1997; Callaway, 2002). In contrast, competition occurs when neighboring plants share the same resources, such as light, soil nutrients, water, or space, often resulting in one plant limiting the growth and survival of the other (Grime, 1977). The balance between facilitation and competition can shift depending on the environmental severity and stress tolerance of the species (Bertness and Callaway, 1994; Wang et al., 2014). During the seedling stage, plant mortality is significantly influenced by biotic and abiotic stress (Alvarez-Clare and Kitajima, 2009). Non-living elements, like rocks or litter can create microsites that modify environmental conditions, sometimes facilitating seedling development or, in other cases, inhibiting it, and as a result influencing competitive dynamics (Loydi et al., 2015; Loayza et al., 2017; Sangsupan et al., 2018). The interaction between the nurse and the beneficiary species can shift between facilitation and competition through early ontogeny (Paterno et al., 2016). Some plants may initially improve seedling establishment (i.e., cotyledon or leaf emergence) (Paterno et al., 2016), and recruitment success (i.e., alive seedlings at the last evaluation) (Barczyk et al., 2024) of certain species but later may compete for resources such as light and soil nutrients (Calcagno-Pissarelli et al., 2023). This dual role highlights the importance of studying the specific effects of bracken fronds and litter to determine whether they act as facilitators or competitors during early recruitment processes.
Bracken can hinder the establishment of other plant species due to shading by its large fronds (Hartig and Beck, 2003; Silva Matos and Belinato, 2010), and also because it produces allelopathic compounds (De Jesus Jatoba et al., 2016). In addition, the deep litter layer of decomposing fronds acts as a barrier and can prevent seeds from reaching the soil, hindering the establishment of seedlings (De Silva and Matos, 2006; Xavier et al., 2016; Carvalho et al., 2022). These patterns suggest that bracken has competitive characteristics that decrease species richness of trees and shrubs as bracken coverage increases (Carvalho et al., 2022; Paz et al., 2022). Although, in tropical regions bracken-dominated areas often show a low diversity and abundance of tree species (Günter et al., 2007; López et al., 2024), it has been found that bracken can improve microclimatic conditions, in comparison to open areas, by reducing photosynthetically active radiation and soil temperature, which particularly benefits shade-tolerant, often non-pioneer and large-seeded species, thereby facilitating their recruitment (Gallegos et al., 2016; Ssali et al., 2019; López et al., 2024). A better understanding of the interactions between bracken and other plant species will help us to identify factors influencing the regeneration of these heavily deforested areas, and to develop effective strategies for promoting forest regeneration.
Functional traits such as seed size play a crucial role in plant regeneration and demography (Violle et al., 2007; Poorter et al., 2008). In particular, larger seeds are frequently associated with higher recruitment success, lower mortality and slower growth (Leishman and Westoby, 1994; Moles and Westoby, 2004; Poorter et al., 2008). Seedlings from large seeds tend to have a better performance under different establishment hazards, such as deep shade, competition, defoliation, drought, nutrient shortage, herbivory, and burial under litter or soil (Moles and Westoby, 2004). These traits make them particularly advantageous in environments with high competition or challenging abiotic conditions. Conversely, seedlings from small-seeded species tend to grow faster and are often favored in open environments such as forest gaps, where rapid growth is advantageous (Grubb et al., 2013). Seed size is also closely linked to dispersal strategies and their demographic consequences. Large seeds, are often dispersed by animals (e.g., birds or mammals), and may benefit from directed dispersal to favorable microsites, enhancing recruitment success. In contrast, small seeds, commonly dispersed by wind, tend to prioritize dispersal distance over establishment probability (Eriksson and Ehrlén, 1992). Understanding the effect of seed size on seedling performance is particularly relevant in disturbed environments such as bracken-dominated areas, where dense litter layers, intense light competition, and microclimatic conditions strongly influence seedling establishment. However, the role of seed size in shaping regeneration patterns in these ecosystems remains underexplored.
This study aims to evaluate the effect of bracken fronds and litter on the performance of tree species during early recruitment processes. Specifically, we aim to: 1) Compare the effects of bracken fronds and litter on different early phases of development of tree species, including seedling establishment, recruitment success and growth, according to seed size. We expect that early seedling performance, including establishment, recruitment and growth will have a positive relationship with seed size in the presence of bracken fronds and litter, because large-seeded species are often better adapted to shaded environments (Gallegos et al., 2015; Ssali et al., 2019; López et al., 2024). 2) Assess the intensity and direction of interactions between bracken fronds and litter on seedling establishment, recruitment and growth as a function of seed size. We hypothesize that the shaded environment created by bracken fronds facilitates the establishment, recruitment and growth of species with large and medium-sized seeds due to their greater shade-tolerance and higher reserves, while bracken fronds compete with small-seeded species for light, thereby affecting their development (Milberg et al., 2000; Baraloto et al., 2005). Regarding bracken litter, we expect that the establishment, recruitment and growth of seedlings of medium- and large-seeds will be facilitated due to the higher moisture content in soils with litter (López et al., 2024). However, as small-seeded species often require high light conditions for germination (Milberg et al., 2000; Pearson et al., 2002), the additional shade created by bracken’s litter could impede their establishment and recruitment. Additionally, we expect that litter accumulation creates a physical barrier that smaller seeded species cannot overcome, hindering their growth (Ghorbani et al., 2006; Ssali et al., 2019). 3) Identify potential bracken management strategies to optimize conditions for seedling establishment, recruitment and growth to promote forest succession. To our knowledge, this is the first study that experimentally evaluates the differential effects of fronds and litter on the early stages of tree seedling performance in bracken-dominated areas.
2 Materials and methods
2.1 Study area
The study was conducted between 2019 and 2023 in the tropical montane humid forests in the Bolivian Andes, in the vicinities of Chulumani and Irupana localities, Sud Yungas, La Paz, Bolivia (16°24’37” S, 67°31’37” W and 16°27’24” S, 67°25’50” W), between 1,850 and 2,450 m asl (Supplementary Figure S1). The mean annual temperature is 20.5°C and the mean annual precipitation is about 1,390 mm (Molina-Carpio et al., 2019). Due to frequent and uncontrolled anthropogenic fires and the expansion of Erythroxylum coca plantations, the montane forest have become highly fragmented (Killeen et al., 2005, 2008; Beck et al., 2024).
The only two large remaining patches of continuous primary montane humid forest in the area, located at the top of the mountains, cover approximately 1,200 and 4,000 ha, respectively (Lippok et al., 2014; Beck et al., 2024). These forests are dominated by tree species such as Alchornea brittonii, Beilschmiedia latifolia, Couepia sp. nov., Coussapoa david-smithii, Ficus crassiuscula, Hedyosmum cuatrecazanum, Ladenbergia oblongifolia, Miconia plumifera, Pectinopitys harmsiana, Piper bolivianum, Tetrorchidium andinum, Weinmannia rhoifolia and different tree ferns (Beck et al., 2024). Surrounding these forests are extensive areas dominated by the bracken fern Pteridium esculentum (Schwartsburd et al., 2018), wind-dispersed trees such as Clethra scabra and Weinmannia sorbifolia, shrubs like Baccharis spp., and some animal-dispersed tree species like Myrsine coriacea, Piper elongatum and Clusia trochiformis, and shrubs like Miconia spp., Gaultheria spp. and Rubus boliviensis (Lippok et al., 2013; Beck et al., 2024). Bracken covers more than 70% of the vegetation, reaching heights of 150–300 cm (Gallegos et al., 2015; Beck et al., 2024), whit its litter covering approximately 70% of the soil, with a depth between 10–50 cm (Lippok et al., 2013; López et al., 2024).
2.2 Experimental design
We selected eight bracken-dominated areas at 100 m from the forest edge (Figure 1A) that had last burned between five and 15 years prior to the experiment and were each at least 1 km apart. The selected sites had similar vegetation structure and slope aspect. At each site, 50 × 50 m plots were established and divided into four 25 × 25 m subplots using a randomized-block design with the following treatments: 1) fronds and litter intact (F+L+); 2) fronds intact and litter removed (F+L−); 3) fronds removed and litter intact (F−L+); and 4) both fronds and litter removed (F−L−) (Figure 1B). To maintain the treatments, fronds were carefully cut at ground level with a machete every four months, and litter was removed manually.
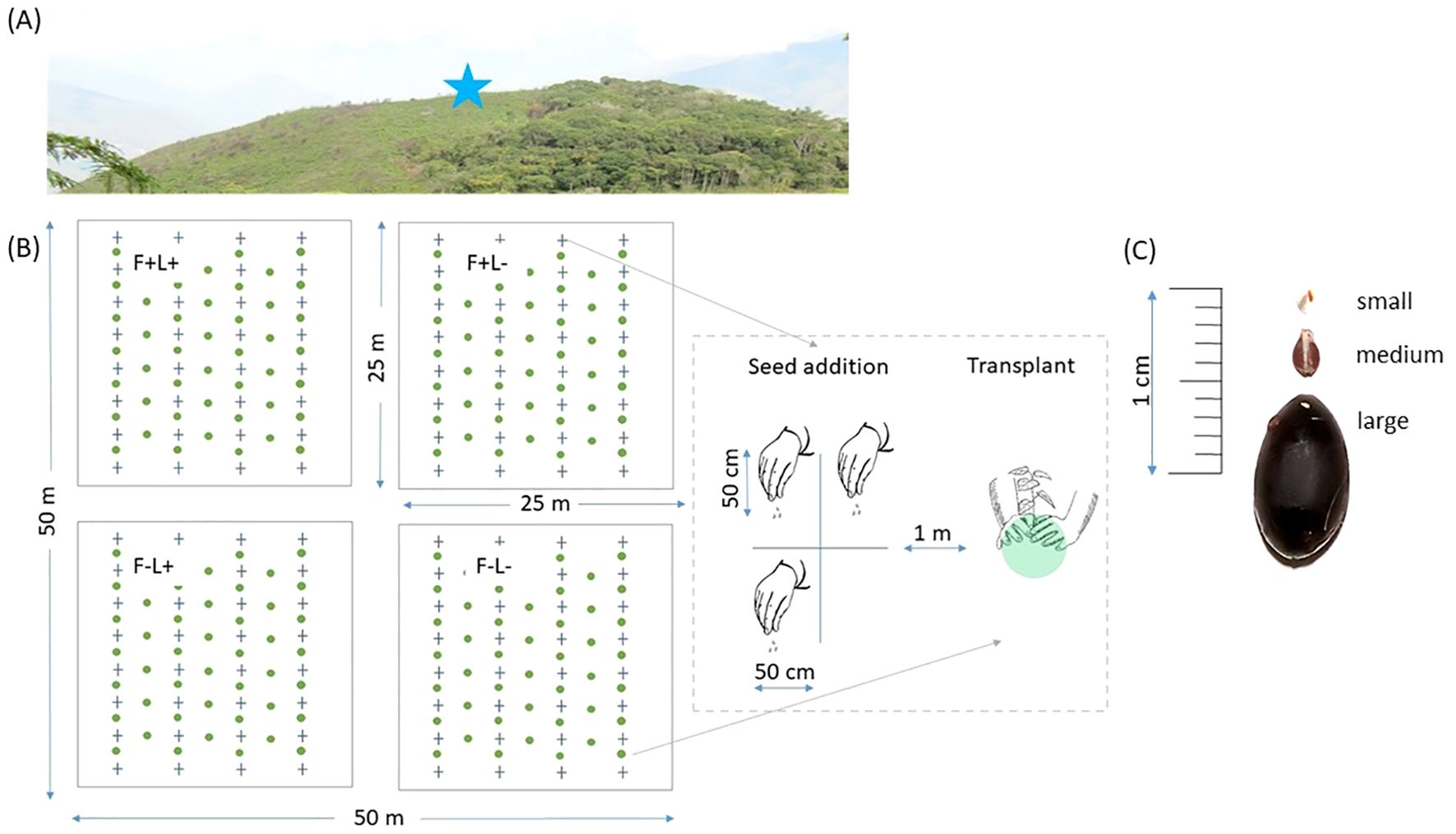
Figure 1. Detailed experimental design for the direct seed addition and transplant experiments with 24 species. (A) Photograph of a bracken-dominated area where the subplots were established, representing different sites (n= 8), (B) Schematic overview of plots and subplots for treatments: (F+) bracken fronds present, (F−) bracken fronds removed, (L+) bracken litter present, and (L−) bracken litter removed, and design of seed addition, and transplant experiments: blue crosses represent quadrats where three species were sown, and green circles represent seedlings from the same cohort transplanted one year after being nursery-raised, interspersed with the sown seeds (C) From top to bottom: examples of small-seeded species (<2 mm), medium-seeded species (2–7 mm), and large-seeded species (>7 mm).
The environmental conditions varied across bracken management treatments (López et al., 2024). For instance, soil temperature in treatments without fronds and litter is approximately 8°C higher than in treatments where fronds and litter are present. Similarly, soil moisture is about 15% lower after bracken removal, and photosynthetically active radiation (PAR) at 20 cm above the ground is nearly 80 μmol m−2 s−1 higher in treatments without fronds compared to those with bracken cover.
2.3 Seed addition and seedling transplant
Based on fruit availability in the study area, 24 common tree species at forest edges and in the forest interior were selected. To enhance response diversity in the experiment, we included species from different families and seed sizes (Palma and Laurance, 2015). (see Supplementary Table S1) for details of species, seed size and number of seeds sown). Seeds were collected from at least ten individuals per species and mixed. All seeds were manually cleaned and counted before sowing. Orthodox seeds were collected once for sowing at all sites, stored in paper bags, and sown within one month. Recalcitrant seeds were collected shortly before sowing at each of the eight sites, and stored in small flasks with damp paper to maintain moisture until sowing within the next two days. In each subplot, seven rows were cautiously delineated to avoid bracken disturbance (Figure 1B). Four rows were designated for the seed addition experiment. In each row, eight 1 × 1 m2 quadrats were established and further divided into four 50 × 50 cm2 sub-quadrats, leaving the lower right sub-quadrant free to facilitate the evaluation of the other three sub-quadrats. These quadrats were separated by 2 m vertically and 3 m horizontally, with a pathway between rows. Because seed germination and survival are positively related to seed size, and small-seeded species tend to produce more seeds (Westoby et al., 1996; Moles and Westoby, 2004), in each sub-quadrant, a different species was sown at different quantities, depending on their seed size and availability, approximately 50 seeds for small-, 15 for medium- and 3 for large-seeded species (Supplementary Table S1). Seeds were dropped to simulate natural dispersion. A total of 46,640 seeds were sown, of which 32,320 (69.3%) were small seeds, 13,376 (28.7%) were medium-sized seeds, and 944 (2%) were large seeds.
Simultaneously, seeds from the same cohort were sown at the greenhouse from the Santiago de Chirca Biological Station (16°23’50.23” S, 67°34’53.53” W, 2080 m asl), with a 50% shade mesh and regular watering, and grown in separated bags. One year later, nursery-raised seedlings were transplanted to the plots in three rows adjacent to the rows with the seeds added (Figure 1B). Both experiments were implemented in the rainy season (November 2019–February 2020) to promote germination and survival (Paterno et al., 2016). Due to the high asynchrony of fruit production in the tropics (Usinowicz et al., 2012) and the lower germination and survival rates of some species (Moles and Westoby, 2004), it was not possible to obtain seedlings from all 24 species to transplant. Therefore, only 14 species were used for the transplant experiment (Supplementary Table S1).
For the seed addition experiment, we assessed seedling establishment and recruitment success. Seedling establishment was calculated as the proportion of sown seeds that developed cotyledons or leaves. Recruitment success was calculated as the proportion of the established seedlings that survived to the final assessment (i.e., after 36 months) (Paterno et al., 2016; Barczyk et al., 2024). Measurements were taken at 6, 12, 24 and 36 months, with a focus on the cumulative outcome at 36 months. For transplanted seedlings, the first evaluation of height took place immediately after planting, with subsequent evaluations of seedling growth after 24 and 36 months. Growth was calculated as the difference between the final and initial height of the transplanted seedlings (Paterno et al., 2016).
2.4 Statistical analysis
To assess the changes in early ontogenetic stages of seedlings across the four bracken management treatments according to seed size, we first conducted separate generalized linear mixed-effects models (GLMMs) for each treatment, treating seed size as a continuous variable. Seedling establishment and seedling recruitment were analyzed using a beta-binomial error distribution to account for over-dispersion (Crawley, 2012), while growth was modeled with a Gaussian distribution. Secondly, we classified species by seed-size categories as follows: small-seeded species (n=8, seed size <2 mm), medium-seeded species (n=13, seed size 2–10 mm), and large-seeded species (n=3, seed size >10 mm). We then performed three separate GLMMs using the same response variables, with bracken treatments, seed-size category, and their interaction as fixed effects, and site as a random effect. Additionally, we conducted separate models for each of the 24 species, using only treatment as a fixed effect and site as a random effect.
To assess the intensity and direction of the interaction between bracken fronds and litter, we used the Relative Interaction Intensity Index (RII), which quantifies the relative effect of the species interactions, ranging from −1 (maximum competition) to +1 (maximum facilitation), while values near to 0 indicate neutral interactions (Armas et al., 2004). The RII was calculated based on the performance of the planted seedlings in the different bracken treatments, using the formula:
where BW represents the performance of each ontogenetic stage and seed size category in the presence of bracken fronds, and B0 represent their performance in the absence of bracken fronds. The same formula was used to assess the effect of litter. We pooled the data from treatments with fronds and litter, respectively. The differences in RII indices for seedling establishment, recruiting success and growth for each seed size category and treatment were tested using generalized linear mixed-effect models (GLMM). In all models we included treatment (fronds and litter), seed size category and their interaction as fixed effects, with sites and species as random effects, and a Gaussian error distribution.
All models were fitted using the ‘glmmTMB’ package (Brooks et al., 2017), and each model was validated analyzing residuals in ‘DHARMa’ package (Hartig, 2022). Post hoc Tukey comparisons among treatments were conducted with the ‘emmeans’ package and all figures were plotted using the ‘ggplot2’ package. Marginal R2 values, representing the proportion of variance explained by the fixed factors were calculated using the r.squaredGLMM function of the ‘MuMIn’ package (Barton, 2018). The significance of the fixed factors in each model was assessed using the ‘mixlm’ package (Liland, 2019). All statistical analyses were carried out using R, version 4.2.1 (R Core Team, 2022).
3 Results
3.1 Addition of seeds and planting of seedlings
We recorded a total of 1,995 established seedlings (4.3%). Seedling establishment and recruitment success significantly and positive increased with seed size in all treatments except in the treatment without fronds and without litter, were both demographic variables remained low (Supplementary Figure S2A, B; Supplementary Table S2). Seedling growth tended to reduce with seed size in all treatments, but the differences were not significant (Supplementary Figure S2A, B; Supplementary Table S2).
Seedling establishment was significantly influenced by treatment (X2 = 158.6, p<0.001), seed size category (X2 = 170.9, p<0.001) and their interaction (X2 = 21.4, p<0.01). Establishment was the lowest in the absence of fronds and litter (F−L−, p<0.001), without an effect of seed size. In contrast, the highest seedling establishment was found in the treatment with fronds and litter for large-seeded species (Figure 2A; Table 1). A similar trend was observed in the treatment with fronds and without litter (F+L−) and in the treatment without fronds with litter (F−L+, Figure 2A).
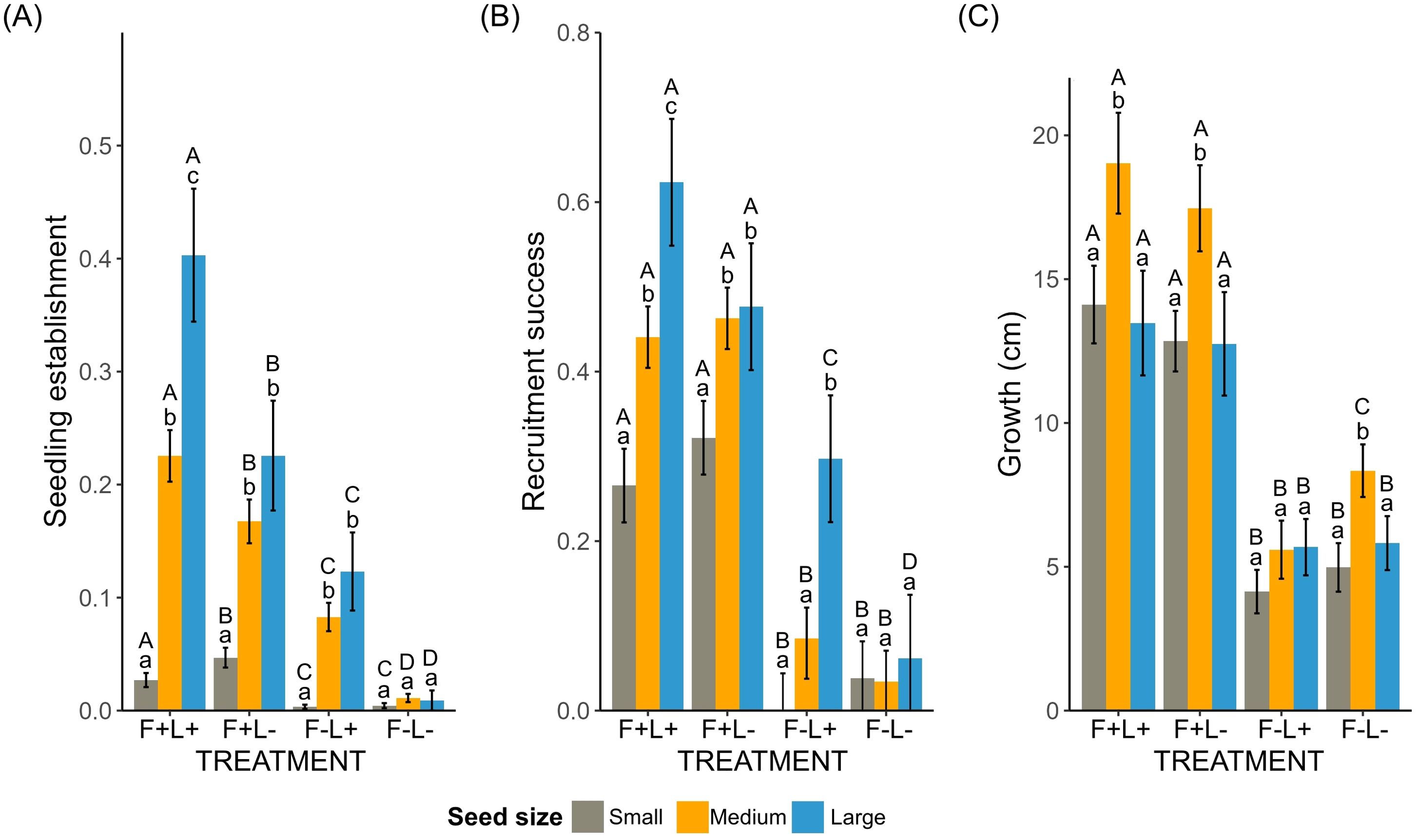
Figure 2. Proportion of (A) seedling establishment, (B) recruitment success, and (C) growth (after 36 months) according to seed size category in experimental bracken treatments: (F+) bracken fronds present, (F−) bracken fronds removed, (L+) bracken litter present, and (L−) bracken litter removed. The bar plots represent predicted values (means ± SE) from the generalized linear mixed-effects models (GLMMs) followed by post-hoc Tukey’s multiple comparisons test, P< 0.05. Different capital letters represent significant differences among treatments for each seed size category, while different lowercase letters denote significant differences among seed size categories in each treatment.
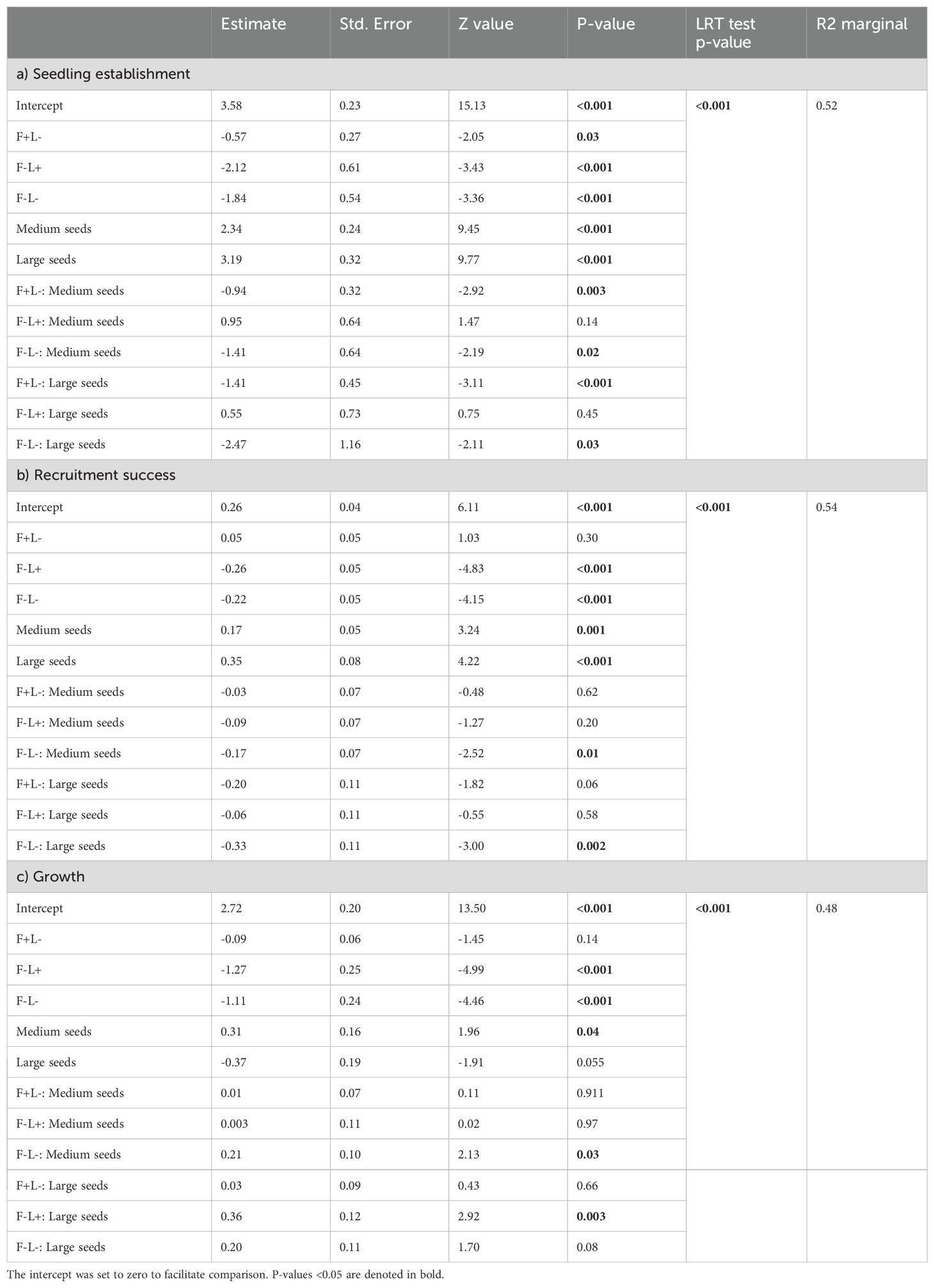
Table 1. Generalized linear mixed effects models (GLMM) for the effect of seed size on a) seedling establishment, b) recruitment success and c) growth, for each treatment analyzed separately.
Recruitment success was significantly influenced by treatment (X2 = 228.4, p<0.001), seed size category (X2 = 19.6, p<0.001) and their interaction (X2 = 15.1, p<0.05). Recruitment success in both treatments without fronds (F−L+ and F−L−) was significantly lower (p<0.001) than in treatments with fronds (F+L+ and F+L−) for all seed size categories (Figure 2B; Table 1).
From the 1,285 transplanted seedlings, 948 (73.7%) survived the first 36 months. Growth was higher in the treatments with fronds (F+L+ and F+L−) than in the treatments without fronds (F−L+ and F−L−, Figure 2C), and this pattern did not change according to seed size (Figure 2C; Table 1; Supplementary Figure S3). Species specific trends can be visualized in Supplementary Figures and Tables (Supplementary Tables S3–S5).
3.2 Intensity and direction of bracken fronds and litter interactions
For all three early recruitment processes and seed-size categories, the relative interaction intensity index (RII) indicated different patterns in both the intensity and direction of interactions. The intensity of facilitative effects was particularly strong for fronds, as the RII values were consistently positive for seedling establishment, recruitment success and growth, for all seed-size categories, indicating beneficial effects (Figure 3).
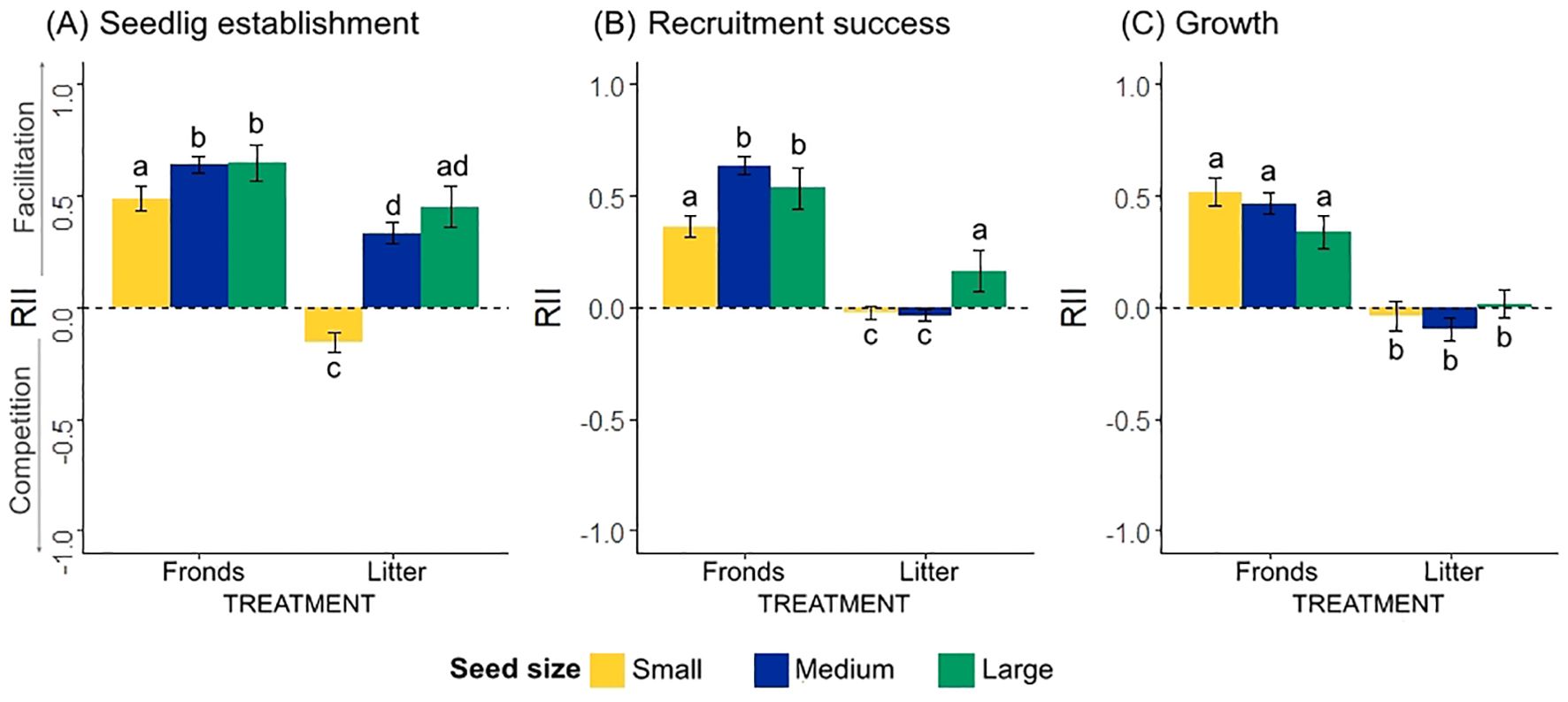
Figure 3. Relative interaction intensity index (RII) between Pteridium fronds and litter and (A) seedling establishment, (B) recruitment success, and (C) growth, according to seed size category. The bar plots represent predicted fit values from the generalized linear mixed-effects models (GLMMs) ± SE. Positive values indicate facilitation and negative values indicate competition. Different letters indicate significant differences at level 0.05 based on post hoc Tukey tests.
On the other hand, the intensity of negative interactions in response to bracken litter was only pronounced for the seedling establishment of small-seeded species, indicating competition, while recruitment and growth showed neutral effects for small-seeded species. In contrast, medium- and large-seeded species showed a strong positive interaction with bracken litter in seedling establishment, indicating facilitative effects. Large-seeded species maintained a positive interaction with litter during recruitment, whereas the interaction was neutral during growth (Figure 3B, C; Table 2).
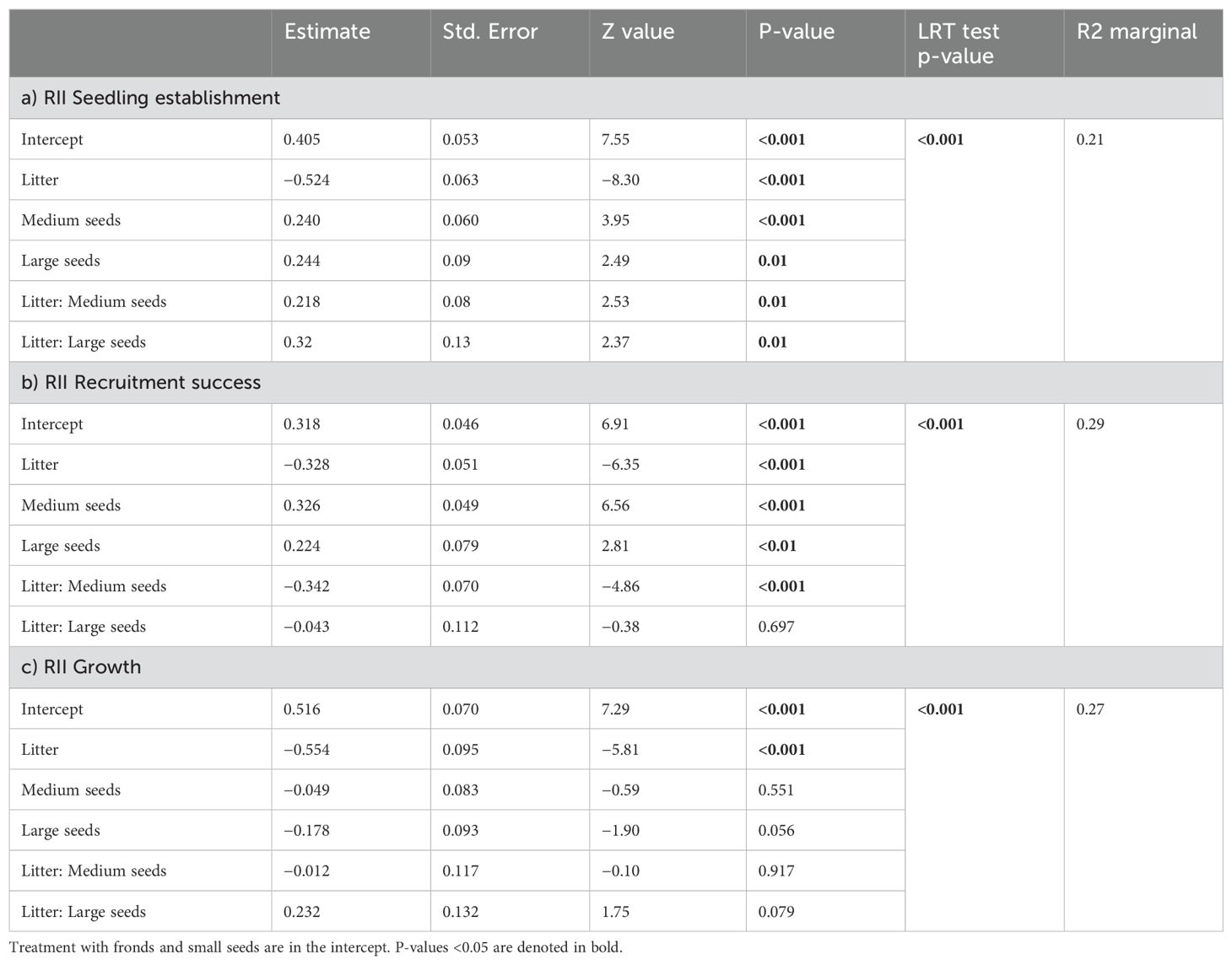
Table 2. Generalized linear mixed effects models (GLMM) for the relative intensity index (RII) of a) seedling establishment, b) recruitment success and c) growth.
4 Discussion
We compared the effects of bracken fronds and litter on the performance of tree species at early development phases as a function of seed size. Seed size positively affected seedling establishment and recruitment success, especially in the treatments with fronds and litter. In contrast, most seedlings had low seedling establishment and recruitment success in the treatment without fronds and without litter regardless their seed size. We found a positive effect of bracken fronds in seedling establishment, recruitment success and growth for all seed size categories. In contrast, litter negatively affected the seedling establishment of small-seeded species but had a neutral effect on their recruitment success and growth. These findings suggest that medium- and large-seeded species benefit from the microclimatic conditions created by bracken, specifically from the fronds. We found no significant differences in growth between small- and large-seeded species.
Our results highlight the dynamics between facilitation and competition in bracken-dominated areas, with seed size being a crucial factor for seedling success during establishment, recruitment and growth. Small-seeded species faced a competitive disadvantage to bracken through litter, hindering their establishment, by limiting light and creating a physical barrier. Our results suggest that bracken facilitates most species during their initial stages, but mainly to medium to large-seeded species. These results highlight the importance of understanding the interactions among different plant life stages when evaluating forest regeneration. Our approach emphasizes the need to prioritize medium- and large-seeded species in restoration strategies to promote forest succession in bracken-dominated areas.
4.1 The effect of bracken fronds and litter on early phases of tree development
As expected, bracken fronds and litter influenced the early development of tree species based on seed size. Seed size was positively related to seedling establishment in all treatments except without fronds and without litter. This pattern is consistent with findings where plants with small seeds have lower probabilities of seedling establishment compared to those with large seeds (Leishman et al., 2000; Wang et al., 2022) in shaded conditions, typical of bracken-dominated areas. This occurs because light requirements for germination and establishment decrease as seed size increases (Milberg et al., 2000). Furthermore, large seeds can produce robust seedlings with thicker stems and larger cotyledons, which not only provide nutrients until the first true leaves develop but also, in some species, contribute to photosynthesis, providing an additional energy source that is advantageous in shaded environments (Kitajima, 2003).
In our seed addition experiment we observed significantly higher recruitment of small-seeded species in treatments with fronds and litter compared to those where fronds and litter had been removed. This pattern might become even more evident with an increase in sample size. For instance, Soliz et al. (in preparation) found similar results using the same species, comparing seedling emergence under natural conditions and in a nursery setting. This result can be explained by the higher temperatures and lower humidity in the absence of fronds and litter (Gallegos et al., 2015; López et al., 2024), conditions that may be too harsh for seedling survival. This is consistent with previous findings showing that fronds ameliorate the harsh conditions of open areas by reducing soil temperature and photosynthetically active radiation, thus improving seedling establishment and recruitment (Gallegos et al., 2015; López et al., 2024).
Bracken-dominated areas are characterized by low species diversity (Günter et al., 2007; Ssali et al., 2017; López et al., 2024). However, in addition to Pteridium, there is a dominance of some pioneer and small-seeded shrub species, particularly from the Asteraceae, Ericaceae and Melastomataceae families (Lippok et al., 2013). Our results suggest that this lower diversity could be due to the fact that only a few small-seeded species can thrive in these ecosystems, and those that could succeed do not arrive, mainly due to the lack of seed dispersers (Saavedra et al., 2015). Among the small-seeded species in our study, Miconia hygrophila (Melastomataceae) exhibited high seedling establishment and recruitment. These results show the variability within small-seeded species, suggesting that other functional traits such as cotyledon type, bark thickness, leaf area, specific leaf area, and wood density, may also play an important role in determining a species ability to thrive in these challenging environments (Green and Juniper, 2004; Moles and Westoby, 2006; Baraloto and Forget, 2007; Poorter et al., 2008), and should be investigated in future studies.
4.2 Intensity and direction of bracken fronds and litter interactions
Our study showed that all seed-size categories benefited from the presence of bracken fronds in the early stages of tree development. All the species, showed improved seedling development under bracken fronds, which highlights the role of bracken as a facilitative species rather than a competitor for resources. These results aligns with recent research has found that ferns promote community assembly by increasing soil stability and other soil properties and reducing competitive pressures (Azevedo-Schmidt et al., 2024).
The competitive effects of bracken through litter on small-seeded species are consistent with previous findings suggesting that small-seeded species are particularly sensitive to litter barriers (Moles and Westoby, 2004; Jessen et al., 2023). This sensitivity may be related to their limited reserves, which make it difficult for them to penetrate or tolerate litter layers (Aud and Ferraz, 2012; Wang et al., 2022). During the establishment phase, bracken litter particularly disadvantages small-seeded species, which often grow rapidly and require a lot of light (Pereira De Souza and Válio, 2001). In addition to the shade provided by the bracken fronds, the litter creates an even shadier environment that is difficult for small-seeded species to overcome (Molofsky et al., 1992; Loydi et al., 2012). Moreover, seeds can become trapped in the litter layer, preventing them from coming into contact with the soil and reducing their probabilities of germination (Donath and Eckstein, 2012).
Although litter showed a facilitative effect for medium- and large-seeded species during seedling establishment, these effects tend to became neutral over time. This ontogenetic shift is consistent with previous research showing that the intensity and direction of interactions could change during ontogeny (Paterno et al., 2016). These findings emphasize the need for long-term studies to better understand the dynamics of facilitation and competition in ecological restoration efforts.
5 Conclusions and future directions
Our findings showed that the presence of bracken fronds and litter creates a complex environment where facilitation and competition coexist, influencing the regeneration dynamics of tropical forests. These results are consistent with previous research, highlighting that larger seeds are more capable of overcoming the physical barrier and low light conditions imposed by bracken (Gallegos et al., 2015; López et al., 2024; Mayta et al., 2024), as these have greater resources to support seedling growth, thereby enhancing their ability to thrive in these low-light conditions (Moles and Westoby, 2004; Baraloto et al., 2005). Given that many studies on plant interactions are limited to short-term observations (Siles et al., 2008), additional monitoring is needed to determine whether Pteridium facilitates long-term recovery and to understand the effect of bracken in subsequent stages of plant ontogeny. As plants mature, their growth rates and resource demands increase, suggesting that the dynamics between neighbors may shift over time (Gómez-Aparicio, 2009). For instance, we found that although litter had facilitative effects for medium- and large-seeded species during seedling establishment, these effects became neutral to negative along time. Since seedlings typically exhibit low growth rates and resource requirements during early stages, demands that intensify as they develop, these requirements could eventually change the intensity and direction of plant interactions (Berkowitz et al., 1995).
Restoration projects and management strategies in bracken-dominated areas should consider the advantages of fronds’ facilitative effects and the negative effects of litter accumulation, especially on establishment of small-seeded species, and probably also for medium-and large-seeded species in the longer term. Identifying tree species with traits that favor their successful establishment, recruitment and growth under bracken is essential to inform restoration strategies to accelerate tropical forest succession. Active restoration practices, such as direct seed addition and seedling transplant, could be implemented in recently burned areas, where bracken fronds enhance microclimatic conditions and the absence of litter help mitigate the initial negative effects of bracken litter accumulation. Transplanting seedlings, which have a survival success exceeding 80%, offers a more reliable approach than seed addition in bracken-dominated areas, especially for small-seeded species. However, to enhance seedling performance of nursery-raised seedlings from small-seeded species, probably seedling transplant should be accompanied by frequent bracken mowing, at least during the first year (Douterlungne et al., 2010), making this option difficult to be set in large areas. Selecting and planting large-seeded shade-tolerant species could be a viable approach to enhance forest recovery in areas dominated by bracken. The incorporation of large-seeded species into active restoration efforts enhanced survival rates and improved long-term recruitment success after land abandonment in areas dominated by grasses (Schubert et al., 2024). Long-term studies conducted on abandoned farmland and cattle-grazed areas, showed that active restoration approaches, such as applied nucleation and plantation, significantly enhance the recruitment of large-seeded late-successional species compared to natural regeneration (Schubert et al., 2024).
Since medium and large-seeded species are particularly favored by bracken, and most of them are mainly dispersed by animals, our results emphasize the importance of attracting seed-dispersing animals, as their activity could be crucial for the transportation of large seeds into bracken-dominated areas (Saavedra et al., 2015). Therefore, incorporating animal attractants such as bird perches or planting animal-dispersed plant species may enhance seed dispersal (Saavedra et al., 2015; López et al., 2024; Mayta et al., 2024). In doing so, including an integrated management approach that considers both biotic and abiotic interactions is crucial for restoration strategies.
Data availability statement
The original contributions presented in the study are included in the article/Supplementary Material, further inquiries can be directed to the corresponding author/s.
Author contributions
CL: Conceptualization, Data curation, Formal analysis, Investigation, Methodology, Software, Writing – original draft, Writing – review & editing, Visualization. CM: Investigation, Methodology, Software, Writing – review & editing. AS: Investigation, Writing – review & editing. IH: Funding acquisition, Resources, Supervision, Validation, Visualization, Writing – review & editing. SG: Investigation, Project administration, Resources, Supervision, Visualization, Writing – review & editing.
Funding
The author(s) declare that financial support was received for the research and/or publication of this article. This work was funded by the German Research Foundation DFG (grant He 3041/23–1) and supported by the Open Access Publication Fund of Martin Luther University Halle-Wittenberg.
Acknowledgments
We are grateful to local communities of Sud Yungas for allowing us to work in their lands, and to S. Tichauer and L. Andrade for permission to work on their properties. We also thank N. Taboada and his family for their help in setting and maintaining the experiment, and to M. Traverso, F. Estrada, J. Domínguez, M. Espejo, S. de la Torre, E. Jimenez, A. Julio, S. Neyrot, D. Ramos, A. Kulkarni, I. Saavedra, V. Villarroel, P. Espinoza and F. Bayá for their invaluable field assistance. We are also grateful to two reviewers for their insightful comments, which improved our work.
Conflict of interest
The authors declare that the research was conducted in the absence of any commercial or financial relationships that could be construed as a potential conflict of interest.
Generative AI statement
The author(s) declare that no Generative AI was used in the creation of this manuscript.
Publisher’s note
All claims expressed in this article are solely those of the authors and do not necessarily represent those of their affiliated organizations, or those of the publisher, the editors and the reviewers. Any product that may be evaluated in this article, or claim that may be made by its manufacturer, is not guaranteed or endorsed by the publisher.
Supplementary material
The Supplementary Material for this article can be found online at: https://www.frontiersin.org/articles/10.3389/fevo.2025.1534920/full#supplementary-material
References
Achard F., Eva H. D., Stibig H. J., Mayaux P., Gallego J., Richards T., et al. (2002). Determination of deforestation rates of the world’s humid tropical forests. Sci. (80-) 297, 999–1002. doi: 10.1126/science.1070656
Alvarez-Clare S., Kitajima K. (2009). Susceptibility of tree seedlings to biotic and abiotic hazards in the understory of a moist tropical forest in Panama. Biotropica 41, 47–56. doi: 10.1111/j.1744-7429.2008.00442.x
Armas C., Ordiales R., Pugnaire F. I. (2004). Measuring plant interactions: A new comparative index. Ecology 85, 2682–2686. doi: 10.1890/03-0650
Asbjornsen H., Wickel B. (2009). “Changing fire regimes in tropical montane cloud forests: a global synthesis,” in Tropical Fire Ecology (Springer, Berlin Heidelberg), 607–626. doi: 10.1007/978-3-540-77381-8_21
Aud F. F., Ferraz I. D. K. (2012). Seed size influence on germination responses to light and temperature of seven pioneer tree species from the Central Amazon. An. Acad. Bras. Cienc. 84, 759–766. doi: 10.1590/S0001-37652012000300018
Azevedo-Schmidt L., Currano E. D., Dunn R. E., Gjieli E., Pittermann J., Sessa E., et al. (2024). Ferns as facilitators of community recovery following biotic upheaval. Bioscience 74, 322–332. doi: 10.1093/biosci/biae022
Baraloto C., Forget P. M. (2007). Seed size, seedling morphology, and response to deep shade and damage in neotropical rain forest trees. Am. J. Bot. 94, 901–911. doi: 10.3732/ajb.94.6.901
Baraloto C., Forget P. M., Goldberg D. E. (2005). Seed mass, seedling size and neotropical tree seedling establishment. J. Ecol. 93, 1156–1166. doi: 10.1111/j.1365-2745.2005.01041.x
Barczyk M. K., Acosta-rojas D. C., Espinosa C. I., Schleuning M., Neuschulz E. L. (2024). Seedling recruitment of small-seeded and large-seeded species in forests and pastures in southern Ecuador. Basic Appl. Ecol. 75, 44–52. doi: 10.1016/j.baae.2024.01.005
Barton K. (2018). MuMIn:Multi-model inference. R package version 1.40.4. Available online at: https://CRAN.R-project.org/package=MuMIn (Accessed June 20, 2024).
Beck S. G., Fuentes A. F., López C. L., Cuba-orozco E., Gallegos S. C. (2024). Los bosques montanos húmedos de la serranía de Apa-Apa (Sud Yungas, La Paz, Bolivia): Un centro de diversidad de plantas y fuente esencial de agua. Ecol. En Boliv. 59, 85–151.
Berkowitz A. R., Canham C. D., Kelly V. R. (1995). Competition vs. Facilitation of tree seedling growth and survival in early successional communities. Ecology 76, 1156–1168. doi: 10.2307/1940923
Bertness M. D., Callaway R. (1994). Positive interactions in communities. Trends Ecol. Evol. 9, 191–193. doi: 10.1016/0169-5347(94)90088-4
Brooks M. E., Kristensen K., van Benthem K. J., Magnusson A., Berg C. W., Nielsen A., et al. (2017).glmmTMB balances speed and flexibility among packages for zero-inflated generalized linear mixed modeling. The R J. 9, 378–400.
Calcagno-Pissarelli M. P., Avila-Nuñez J. L., Alonso-Amelot M. E. (2023). “Mountain Gradients in the Neotropics: A Bracken Fern Perspective,” in Neotropical Gradients and their Analysis, (Cham, Switzerland: Springer International Publishing), 37–115. doi: 10.1007/978-3-031-22848-3_2
Callaway R. M. (1997). Positive interactions in plant communities and the individualistic-continuum concept. Oecologia 112, 143–149. doi: 10.1007/s004420050293
Callaway R. M. (2002). Positive interactions among alpine plants increase with stress. Nature 417, 844–848. doi: 10.1038/nature00805.1
Carvalho T. F., Carvalho A. C., Zanuncio J. C., de Oliveira M. L. R., MaChado E. L. M., José A. C., et al. (2022). Does invasion by Pteridium aquilinum (Dennstaedtiaceae) affect the ecological succession in Atlantic Forest areas after a fire? Environ. Sci. pollut. Res. 29, 14195–14205. doi: 10.1007/s11356-021-16761-7
Christmann T., Palomeque X., Armenteras D., Wilson S. J., Malhi Y., Oliveras Menor I. (2023). Disrupted montane forest recovery hinders biodiversity conservation in the tropical Andes. Glob. Ecol. Biogeogr. 32, 793–808. doi: 10.1111/geb.13666
Crausbay S., Genderjahn S., Hotchkiss S., Sachse D., Kahmen A., Arndt S. K. (2014). Vegetation dynamics at the upper reaches of a tropical montane forest are driven by disturbance over the past 7300 years. Arctic Antarct. Alp. Res. 46, 787–799. doi: 10.1657/1938-4246-46.4.787
De Jesus Jatoba L., Varela R. M., Molinillo J. M. G., Din Z. U., Gualtieri S. C. J., Rodrigues-Filho E., et al. (2016). Allelopathy of bracken fern (pteridium arachnoideum): New evidence from green fronds, litter, and soil. PloS One 11, 1–16. doi: 10.1371/journal.pone.0161670
De Silva Ú.S.R.D., Matos D. M. D. S. (2006). The invasion of Pteridium aquilinum and the impoverishment of the seed bank in fire prone areas of Brazilian Atlantic Forest. Biodivers. Conserv. 15, 3035–3043. doi: 10.1007/s10531-005-4877-z
Dirzo R., Raven P. H. (2003). Global state of biodiversity and loss. Annu. Rev. Environ. Resour. 28, 137–167. doi: 10.1146/annurev.energy.28.050302.105532
Donath T. W., Eckstein R. L. (2012). Litter effects on seedling establishment interact with seed position and earthworm activity. Plant Biol. 14, 163–170. doi: 10.1111/j.1438-8677.2011.00490.x
Douterlungne D., Levy-Tacher S. I., Golicher D. J., Dañobeytia F. R. (2010). Applying indigenous knowledge to the restoration of degraded tropical rain forest clearings dominated by bracken fern. Restor. Ecol. 18, 322–329. doi: 10.1111/j.1526-100X.2008.00459.x
Eriksson O., Ehrlén J. (1992). Seed and microsite limitation of recruitment in plant populations. Oecologia 91, 360–364. doi: 10.1007/BF00317624
Gallegos S. C., Beck S. G., Hensen I., Saavedra F., Lippok D., Schleuning M. (2016). Factors limiting montane forest regeneration in bracken-dominated habitats in the tropics. For. Ecol. Manage. 381, 168–176. doi: 10.1016/j.foreco.2016.09.014
Gallegos S. C., Hensen I., Saavedra F., Schleuning M. (2015). Bracken fern facilitates tree seedling recruitment in tropical fire-degraded habitats. For. Ecol. Manage. 337, 135–143. doi: 10.1016/j.foreco.2014.11.003
Gardner T. A., Barlow J., Chazdon R., Ewers R. M., Harvey C. A., Peres C. A., et al. (2009). Prospects for tropical forest biodiversity in a human-modified world. Ecol. Lett. 12, 561–582. doi: 10.1111/j.1461-0248.2009.01294.x
Ghorbani J., Le Duc M. G., Mcallister H. A., Pakeman R. J., Marrs R. H. (2006). Effects of the litter layer of Pteridium aquilinum on seed banks under experimental restoration. Appl. Veg. Sci. 9, 127–136. doi: 10.1111/j.1654-109X.2006.tb00662.x
Gómez-Aparicio L. (2009). The role of plant interactions in the restoration of degraded ecosystems: A meta-analysis across life-forms and ecosystems. J. Ecol. 97, 1202–1214. doi: 10.1111/j.1365-2745.2009.01573.x
Green P. T., Juniper P. A. (2004). Seed mass, seedling herbivory and the reserve effect in tropical rainforest seedlings. Funct. Ecol. 18, 539–547. doi: 10.1111/j.0269-8463.2004.00881.x
Grime J. P. (1977). Evidence for the existence of three primary strategies in plants and its relevance to ecological and evolutionary theory. Am. Nat. 111, 1169–1194. doi: 10.1086/283244
Grubb P. J., Bellingham P. J., Kohyama T. S., Piper F. I., Valido A. (2013). Disturbance regimes, gap-demanding trees and seed mass related to tree height in warm temperate rain forests worldwide. Biol. Rev. 88, 701–744. doi: 10.1111/brv.12029
Günter S., Weber M., Erreis R., Aguirre N. (2007). Influence of distance to forest edges on natural regeneration of abandoned pastures: A case study in the tropical mountain rain forest of Southern Ecuador. Eur. J. For. Res. 126, 67–75. doi: 10.1007/s10342-006-0156-0
Hartig K., Beck E. (2003). The bracken fern (Pteridium arachnoideum (Kaulf.) Maxon) dilemma in the Andes of Southern Ecuador. Ecotropica 9, 3–13.
Hartig M. (2022). DHARMa: Residual Diagnostics for Hierarchical (Multi-Level / Mixed) Regression Models. R package version 0.4.5. Available online at: https://CRAN.R-project.org/package=DHARMa
Holmgren M., Scheffer M., Huston M. (1997). The interplay of facilitation and competition in plant communities. Ecology 78, 1966–1975. doi: 10.1890/0012-9658(1997)078[1966:TIOFAC]2.0.CO;2
Jessen M., Auge H., Harpole W. S., Eskelinen A. (2023). Litter accumulation, not light limitation, drives early plant recruitment. J. Ecol. 111, 1174–1187. doi: 10.1111/1365-2745.14099
Killeen T. J., Guerra A., Calzada M., Correa L., Calderon V., Soria L., et al. (2008). Total historical land-use change in eastern Bolivia: Who, where, when, and how much? Ecol. Soc 13, 36. doi: 10.5751/ES-02453-130136
Killeen T. J., Siles T. M., Soria L., Correa L. (2005). Estratificación de vegetación y cambio de uso de suelo en los Yungas y Alto Beni de la Paz. Estud. Botánicos La Región Madidi Ecol. En Boliv. 40, 32–69.
Kitajima K. (2003). Impact of cotyledon and leaf removal on seedling survival in three tree species with contrasting cotyledon functions. Biotropica 35, 429–434. doi: 10.1111/j.1744-7429.2003.tb00597.x
Leishman M. R., Westoby M. (1994). The role of seed size in seedling establishment in dry soil conditions – experimental evidence from semi-arid species. J. Ecol. 82, 249. doi: 10.2307/2261293
Leishman M. R., Wright I. J., Moles A. T., Westoby M. (2000). “The evolutionary ecology of seed size,” in Seeds: the ecology of regeneration in plant communities, (Wallingford UK: CABI Publishing), 31–57. doi: 10.1079/9780851994321.0031
Levy-Tacher S. I., Vleut I., Román-Dañobeytia F., Aronson J. (2015). Natural regeneration after long-term bracken fern control with balsa (Ochroma pyramidale) in the Neotropics. Forests 6, 2163–2177. doi: 10.3390/f6062163
Liland K. H. (2019). “Mixlm: mixed model ANOVA and statistics for education,” in R Packag. version 1.2.4. Available at: https://cran.r-project.org/package=mixlm (Accessed June 20, 2024).
Lippok D., Beck S. G., Renison D., Gallegos S. C., Saavedra F. V., Hensen I., et al. (2013). Forest recovery of areas deforested by fire increases with elevation in the tropical Andes. For. Ecol. Manage. 295, 69–76. doi: 10.1016/j.foreco.2013.01.011
Lippok D., Beck S. G., Renison D., Hensen I., Apaza A. E., Schleuning M. (2014). Topography and edge effects are more important than elevation as drivers of vegetation patterns in a neotropical montane forest. J. Veg. Sci. 25, 724–733. doi: 10.1111/jvs.12132
Loayza A. P., Herrera-Madariaga M. A., Carvajal D. E., García-Guzmán P., Squeo F. A. (2017). Conspecific plants are better “nurses” than rocks: Consistent results revealing intraspecific facilitation as a process that promotes establishment in a hyper-arid environment. AoB Plants 9, 1–11. doi: 10.1093/aobpla/plx056
López C. L., Mayta C., Fuentes A. F., Villegas M., Jimenez E., Vasquez V., et al. (2024). Disentangling the roles of bracken fronds and litter on natural seedling recruitment in fire-disturbed tropical montane habitats. For. Ecol. Manage. 566, 122056. doi: 10.1016/j.foreco.2024.122056
Loydi A., Donath T. W., Eckstein R. L., Otte A. (2015). Non-native species litter reduces germination and growth of resident forbs and grasses: allelopathic, osmotic or mechanical effects? Biol. Invasions 17, 581–595. doi: 10.1007/s10530-014-0750-x
Loydi A., Eckstein R. L., Otte A., Donath T. W. (2012). Effects of litter on seedling establishment in natural and semi-natural grasslands: A meta-analysis. J. Ecol. 101, 454–464. doi: 10.1111/1365-2745.12033
Marrs R. H., Le Duc M. G., Mitchell R. J., Goddard D., Paterson S., Pakeman R. J. (2000). The ecology of bracken: Its role succession and implications for control. Ann. Bot. 85, 3–15. doi: 10.1006/anbo.1999.1054
Mayta C., López C. L., Villegas M., Aguirre L. F., Hensen I., Gallegos S. C. (2024). Bird perches and artificial bat roosts increase seed rain and seedling establishment in tropical bracken-dominated deforested areas. Restor. Ecol. 7, e14197. doi: 10.1111/rec.14197
Milberg P., Andersson L., Thompson K. (2000). Large-seeded species are less dependent on light for germination than small-seeded ones. Seed Sci. Res. 10, 99–104. doi: 10.1017/s0960258500000118
Moles A. T., Westoby M. (2004). Seedling survival and seed size: A synthesis of the literature. J. Ecol. 92, 372–383. doi: 10.1111/j.0022-0477.2004.00884.x
Moles A. T., Westoby M. (2006). Seed size and plant strategy across the whole life cycle. Oikos 113, 91–105. doi: 10.1111/j.0030-1299.2006.14194.x
Molina-Carpio J., Espinoza D., Enrique C., Salcedo F., Farfán C., Mamani L., et al. (2019). Clima y variabilidad espacial de la ceja de monte y andino húmedo. Ecol. En Boliv. 54, 40–56.
Molofsky J., Augspurger C. K., Molofsky J. (1992). The effect of leaf litter on early seedling establishment in a tropical forest. Source Ecol. 73, 68–77. doi: 10.2307/1938721
Palma A. C., Laurance S. G. W. (2015). A review of the use of direct seeding and seedling plantings in restoration: What do we know and where should we go? Appl. Veg. Sci. 18, 561–568. doi: 10.1111/avsc.12173
Palomeque X., Günter S., Siddons D., Hildebrandt P., Stimm B., Aguirre N., et al. (2017). Natural or assisted succession as approach of forest recovery on abandoned lands with different land use history in the Andes of Southern Ecuador. New For. 48, 643–662. doi: 10.1007/s11056-017-9590-8
Paterno G. B., Siqueira Filho J. A., Ganade G. (2016). Species-specific facilitation, ontogenetic shifts and consequences for plant community succession. J. Veg. Sci. 27, 606–615. doi: 10.1111/jvs.12382
Paz H., Ortiz-Alcaraz A., del-Val E. (2022). The effects of the aggressive species Pteridium caudatum on the vegetation of Socorro Island: Restoration challenges and opportunities. J. Nat. Conserv. 67, 126160. doi: 10.1016/j.jnc.2022.126160
Pearson T. R. H., Burslem D. F. R. P., Mullins C. E., Dalling J. W. (2002). Germination ecology of neotropical pioneers: Interacting effects of environmental conditions and seed size. Ecology 83, 2798–2807. doi: 10.1890/0012-9658(2002)083[2798:GEONPI]2.0.CO;2
Pereira De Souza R., Válio I. F. M. (2001). Seed size, seed germination, and seedling survival of Brazilian tropical tree species differing in successional status. Biotropica 33, 447–457. doi: 10.1111/j.1744-7429.2001.tb00198.x
Phillips H. R. P., Newbold T., Purvis A. (2017). Land-use effects on local biodiversity in tropical forests vary between continents. Biodivers. Conserv. 26, 2251–2270. doi: 10.1007/s10531-017-1356-2
Pillay R., Venter M., Aragon-Osejo J., González-del-Pliego P., Hansen A. J., Watson J. E. M., et al. (2022). Tropical forests are home to over half of the world’s vertebrate species. Front. Ecol. Environ. 20, 10–15. doi: 10.1002/fee.2420
Poorter L., Wright S. J., Paz H., Ackerly D. D., Condit R., Ibarra-Manríquez G., et al. (2008). Are Functional traits good predictors of demographic rates? Evidence from five netropical forests. Ecology 89, 1908–1920. doi: 10.1890/07-0207.1
Saavedra F., Hensen I., Schleuning M. (2015). Deforested habitats lack seeds of late-successional and large-seeded plant species in tropical montane forests. Appl. Veg. Sci. 18, 603–612. doi: 10.1111/avsc.12184
Sangsupan H. A., Hibbs D. E., Withrow-Robinson B. A., Elliott S. (2018). Seed and microsite limitations of large-seeded, zoochorous trees in tropical forest restoration plantations in northern Thailand. For. Ecol. Manage. 419–420, 91–100. doi: 10.1016/j.foreco.2018.03.021
Schubert S. C., Zahawi R. A., Oviedo-Brenes F., Rosales J. A., Holl K. D. (2024). Active restoration increases tree species richness and recruitment of large-seeded taxa after 16–18 years. Ecol. Appl. 35, e3053. doi: 10.1002/eap.3053
Schwartsburd P. B., Yañez A., Prado J. (2018). Formal recognition of six subordinate taxa within the south american bracken fern, Pteridium esculentum (P. esculentum subsp. arachnoideum s.l.—Dennstaedtiaceae), based on morphology and geography. Phytotaxa 333, 22–40. doi: 10.11646/phytotaxa.333.1.2
Siles G., Rey P. J., Alcántara J. M., Ramírez J. M. (2008). Assessing the long-term contribution of nurse plants to restoration of Mediterranean forests through Markovian models. J. Appl. Ecol. 45, 1790–1798. doi: 10.1111/j.1365-2664.2008.01574.x
Silva Matos D. M., Belinato T. A. (2010). Interference of Pteridium arachnoideum (Kaulf.) Maxon. (Dennstaedtiaceae) on the establishment of rainforest trees. Braz. J. Biol. 70, 311–316. doi: 10.1590/S1519-69842010000200012
Ssali F., Moe S. R., Sheil D. (2017). A first look at the impediments to forest recovery in bracken-dominated clearings in the African Highlands. For. Ecol. Manage. 402, 166–176. doi: 10.1016/j.foreco.2017.07.050
Ssali F., Moe S. R., Sheil D. (2019). The differential effects of bracken (Pteridium aquilinum (L.) Kuhn) on germination and seedling performance of tree species in the African tropics. Plant Ecol. 220, 41–55. doi: 10.1007/s11258-018-0901-8
Stewart G., Cox E., Le Duc M., Pakeman R., Pullin A., Marrs R. (2008). Control of Pteridium aquilinum: Meta-analysis of a multi-site study in the UK. Ann. Bot. 101, 957–970. doi: 10.1093/aob/mcn020
Tryon R. M. (1941). A revision of the genus Pteridium. Contrib. Gray Herb. Harvard Univ. 134, 1–67. doi: 10.5962/p.336249
Usinowicz J., Wright S. J., Ives A. R., Doak D. F. (2012). Coexistence in tropical forests through asynchronous variation in annual seed production. Ecology 93, 2073–2084. doi: 10.1890/11-1935.1
Violle C., Navas M., Vile D., Kazakou E., Fortunel C., Hummel I., et al. (2007). Let the concept of trait be functional! Oikos 116, 882–892. doi: 10.1111/j.2007.0030-1299.15559.x
Wang X., Liang C., Wang W. (2014). Balance between facilitation and competition determines spatial patterns in a plant population. Chin. Sci. Bull. 59, 1405–1415. doi: 10.1007/s11434-014-0142-8
Wang Z., Wang D., Liu Q., Xing X., Liu B., Jin S., et al. (2022). Meta-analysis of effects of forest litter on seedling establishment. Forests 13, 644. doi: 10.3390/f13050644
Westoby M., Leishman M., Lord J. (1996). Comparative ecology of seed size and dispersal. Philos. Trans. R. Soc B Biol. Sci. 351, 1309–1318. doi: 10.1098/rstb.1996.0114
Keywords: Pteridium, restoration strategies, seed addition, tree early development, tropical montane forest
Citation: López CL, Mayta C, Soliz A, Hensen I and Gallegos SC (2025) Facilitative and competitive effects of bracken fronds and litter on tree seedling recruitment. Front. Ecol. Evol. 13:1534920. doi: 10.3389/fevo.2025.1534920
Received: 26 November 2024; Accepted: 10 April 2025;
Published: 07 May 2025.
Edited by:
Carolina Puerta-Pinero, Joint Research Centre (JRC), ItalyReviewed by:
Carmen Guiote, Spanish National Research Council (CSIC), SpainAline Cavalcante De Souza, Rio de Janeiro State University, Brazil
Copyright © 2025 López, Mayta, Soliz, Hensen and Gallegos. This is an open-access article distributed under the terms of the Creative Commons Attribution License (CC BY). The use, distribution or reproduction in other forums is permitted, provided the original author(s) and the copyright owner(s) are credited and that the original publication in this journal is cited, in accordance with accepted academic practice. No use, distribution or reproduction is permitted which does not comply with these terms.
*Correspondence: Cecilia L. López, Y2VjaWxpYS5sb3Blei5hbGlwYXpAZ21haWwuY29t
‡ORCID: Cecilia L. López, orcid.org/0000-0002-1099-2694
Cesar Mayta, orcid.org/0009-0000-3986-2028
Andrea Soliz, orcid.org/0000-0002-4318-0158
Isabell Hensen, orcid.org/0000-0001-6470-9359
Silvia C. Gallegos, orcid.org/0000-0003-4102-7456