- Department of Pharmacology, Institute of Biomedical Sciences, University of São Paulo, São Paulo, SP, Brazil
Substance use has been intertwined with human history for millennia. Throughout the ages, people have consumed various substances for medicinal, spiritual, and recreational reasons, although occasional use differs significantly from substance use disorders (SUDs). Exposure to lifetime stressors constitutes a significant risk factor for both psychiatric disorders and SUD development and relapse. Indeed, hypothalamic–pituitary–adrenal (HPA) axis modulation, alterations in neuroanatomical and neurotransmitter systems, as well as neuroinflammation are common features of stress-related mood disorders and SUDs. In this mini-review, we will explore how stress exposure influences the SUDs' neurobiological basis on different scales—from large neural circuitries to specific molecular mechanisms—and discuss novel targets for potential treatments.
1 Introduction
SUDs are defined as brain diseases characterized by compulsion for drug seeking and intake despite severe negative consequences related to the loss of control and emergence of a negative emotional state (Liu and Li, 2018). According to the 5th Edition of the Diagnostic and Statistical Manual of Mental Disorders (American Psychiatric Association, 2013), SUDs can be classified as mild, moderate or severe. The recently published data from The World Health Organization and the United Nations Office on Drugs and Crime showed that 64 million people worldwide were suffering from SUDs in 2022, which accounts for an increase of 3% over 5 years (Drugs and Crime, 2024), while the global prevalence of mental disorders was 13.0% (Castaldelli-Maia and Bhugra, 2022). Interestingly, the SUD prevalence among individuals with major depressive disorder was 25% (Hunt et al., 2020) and 33% among people with bipolar disorder (Hunt et al., 2016). Also, there is strong evidence of comorbidity of SUD with generalized anxiety disorder (Alegria et al., 2010) and posttraumatic stress disorder (PTSD; McCauley et al., 2012).
Stress is a natural and adaptive response required to sustain life that can be interpreted as any stimulus that changes physiological and/or psychological states (Schneiderman et al., 2005; Le Moal, 2007). Neurons located in the dorsomedial parvocellular subdivision of the paraventricular nucleus of the hypothalamus release corticotropin-releasing factor (CRF) in the hypophyseal portal system in response to stressors, which binds to CRH receptor type 1 (CRHR1) in hypophysis and leads to adrenocorticotropic hormone secretion in the systemic circulation, culminating in glucocorticoids (GCs) release [cortisol in humans and corticosterone (CORT) in rodents]. The GC hormones have genomic (slow) and non-genomic (fast) actions through the mineralocorticoid (MR) or glucocorticoid (GR) receptors. The cytosolic GC-MR/GR complex translocates to the cell nucleus and modulates gene expression by binding to the DNA's glucocorticoid-responsive element (GRE) regions (Beato and Sanchez-Pacheco, 1996) for long-lasting genomic effects. The CORT acts through classical MR and GR inserted in or attached to the plasma membrane for rapid non-genomic action, facilitating or inhibiting ion channels, receptors, and neurotransmitter signaling (Groeneweg et al., 2011). As a crucial stress mediator, GCs play an important role in arousal, cognition, mood, immunity, inflammatory reactions (Oster et al., 2017), and SUD (Mantsch and Gasser, 2015). According to allostasis, depending on the stress nature, intensity, and chronicity, the energy demand may be higher than the organism's resource (allostatic overload), leading to maladaptive responses (McEwen and Wingfield, 2003). Indeed, stress can be a significant risk factor for the development of both psychiatric disorders and SUDs (McGrath and Briand, 2019).
Some limbic regions, such as the ventral tegmental area (VTA), nucleus accumbens (NAc), prefrontal cortex (PFC), amygdala, and bed nucleus of the stria terminalis (BNST), are crucial for governing stress response and different drug use stages. For example, the VTA dopaminergic neurons release dopamine (DA) to other regions responsible for reward processing, such as the NAc and the PFC (Kielbinski et al., 2019). In contrast, the VTA inhibitory interneurons mediate reward-seeking reduction via NAc communication in stressed animals (Lowes et al., 2021). The amygdala is involved in emotional processing, highly responsive to stressors, and strongly related to the withdrawal period, playing a significant role in symptoms such as anxiety, irritability, and unease symptoms present in patients experiencing withdrawal (Stamatakis et al., 2014; Gilpin et al., 2015). More recent data showed that stress disruption of reward responses depends on the amygdala-NAc pathway (Madur et al., 2023). Indeed, the SUD implications in reward and stress (“anti-reward”) systems have long been stated (Volkow et al., 2016).
Given the association between stress and SUD (Nikbakhtzadeh et al., 2023), it is fundamental to clarify what is currently known about the cellular, molecular, and genetic mechanisms governing the relationship between stress and drug use responses to identify new therapeutic targets. We will first address the shared anatomical and neuroendocrine basis of SUD and stress. Then, despite several research models of stress that differ in neurobiological and behavioral effects from each other, we will give a special focus to early life stress (ELS) and cellular stress (i.e., oxidative stress and neuroinflammation) on SUD. Finally, we will explore genetic hallmarks of stress and HPA-axis regulation related to SUD.
1.1 Anatomical and neuroendocrine features of stress and SUD
A three-stage model—including binge/intoxication, withdrawal/negative effects, and preoccupation/anticipation—has been used to explain the transition from drug use to SUD (Koob and Volkow, 2010; Figure 1A). The drug-induced activation of the D1 dopamine receptor in the mesolimbic pathway (from VTA to NAc) and inhibition of D2 receptors in the striatocortical pathway (from the cerebral cortex to striatum) are classically associated with reinforcing, positive drug effects present during binge stage—even though μ-opioid receptors and endocannabinoid systems are also involved (Volkow and Morales, 2015). However, sustained drug intake leads to a dynamic readjustment of physiological parameters, including long-term brain changes that result in increased SUD risk and relapse. This process is referred to as the allostatic theory of addiction (Koob and Le Moal, 1997, 2001), which ultimately leads to withdrawal/negative effects. Indeed, the increase in reward threshold due to dopaminergic system downregulation is an early hallmark of drug-induced neuroadaptations, leading to the deficit in natural reward experience called anhedonia (Volkow et al., 2009). In addition to the dopaminergic system, CRF, dynorphin, and hypocretin are also modulated by chronic drug intake and related to the withdrawal/negative feelings stage. The CRF system is responsible for HPA-axis dysregulation followed by alterations in the extended amygdala, an extra-hypothalamic area composed of the central amygdala (CeA), BNST, and a transition zone in the posterior part of the medial NAc (Koob, 2008). The dynorphin-κ opioid system also modulates the extended amygdala. At the same time, hypocretin (derived exclusively from the lateral hypothalamus) interacts with noradrenergic, cholinergic, serotonergic, histaminergic, and dopaminergic systems, in addition to its role in HPA axis regulation (Koob, 2008). Regarding the preoccupation/anticipation stage, prefrontal cortex (PFC) dysfunction has been associated with the loss of control and compulsive drug-taking characteristic of this stage because of its role in decision-making and self-regulation (Figure 1A). Transcranial direct current stimulation (tDCS) over the dorsolateral prefrontal cortex (DLPFC) reduced craving immediately after the session and 1 month later in individuals with methamphetamine-use disorder (Alizadehgoradel et al., 2020). Moreover, individuals with SUD showed decreased left dorsal anterior cingulate cortex (dACC) and right middle frontal gyrus (MFG) activation compared to healthy controls (Le et al., 2021).
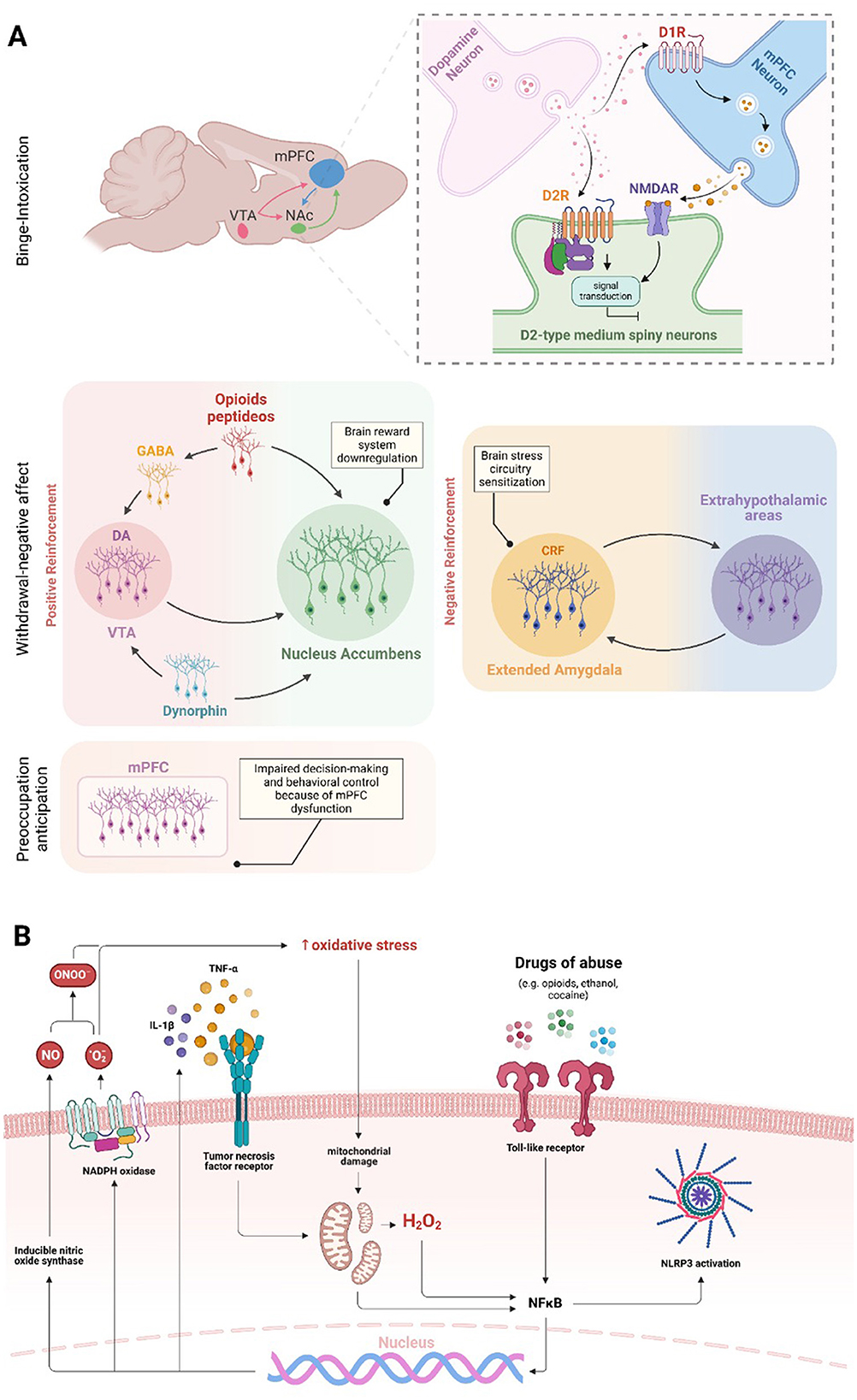
Figure 1. Brain regions and molecular effects involved in substance use and addiction. The three main domains of addiction neurocircuitry correspond to distinct functional areas, including binge/intoxication, associated with reward and incentive salience (activation of D1 dopamine receptor in the VTA-NAc pathway and D2 receptor inhibition in the striatum-cortex pathway—NMDAR are also involved in D2R response); withdrawal/negative affect, linked to negative emotional states and stress (brain reward systems downregulation and stress circuitry sensitization—dopaminergic, CRF, opioid, GABA, and dynorphin systems are involved); and preoccupation/anticipation, related to craving, impulsivity, and executive function; decision making and behavioral control are impaired in consequence of, but not restricted to, mPFC dysfunction (A). Drugs of abuse increase oxidative stress levels in the brain, initiating a continuous cycle that sustains neuroinflammation. The oxidative stress caused by substance use can compromise mitochondrial function, resulting in increased generation of free radicals. Increased oxidative stress contributes to the nuclear translocation and activation of NF-κB in microglial cells and induces the NLRP3 inflammasome activation. In addition, the drug use activates TLR4, which also triggers the activation of microglial NF-κB. Once in the nucleus, NF-κB promotes the increased expression of NOX and iNOS enzymes and pro-inflammatory cytokines, such as TNF-α and IL-1β. The increase in oxidative stress, pro-inflammatory cytokines and NLRP3 ultimately intensifies microglial and astrocytic activation, leading to a cycle of inflammation and oxidative stress in the brain (B).
Notably, the neuroanatomical and neurotransmitter systems governing SUD substantially overlap with stress response. In this regard, ethanol intake was prevented by prior GR (but not MR) antagonism (Koenig and Olive, 2004). Both stress and GCs increase DA synthesis (Baik, 2020) and reduce its clearance (Parnaudeau et al., 2014), which influences the sensitization to psychomotor stimulants, increases substance-induced conditioned place preference and self-administration of cocaine, amphetamine, heroin, and relapse to cocaine seeking (Yap and Miczek, 2008). Given that contextual memory retrieval depends on the hippocampal GR (Roozendaal et al., 2003), it could be part of the mechanism governing the intense craving and anxiety reported by SUD patients in response to stress and drug-cue exposure (Smith et al., 2023). Also, stress and stimulants cause maladaptive decision-making through epigenetic changes in the dorsal striatum (Murphy and Heller, 2022). Therefore, stress influences many substance use aspects, from consumption maintenance through neurotransmitter systems modulation to a contextual association that elicits drug use resumption (Nazeri et al., 2017; Goldfarb and Sinha, 2018; Mukhara et al., 2018).
1.2 The early-life stress implications for SUD
The ELS is among the major risk factors for psychiatric disorders development—for example, substance use, mood, anxiety, and posttraumatic stress disorders are clinical outcomes of severe ELS (Berhe et al., 2022). Neglect, trauma, family dysfunction, or abuse in general has about 3.6 million annual reports, and ~702,000 children are confirmed victims of abuse or neglect (Forster et al., 2018). ELS has been associated with a higher risk of mood disorders (Forster et al., 2018; Andersen, 2019) and SUD (Goodwin et al., 2004; Kirsch and Lippard, 2022). Substance abuse can emerge to alleviate suffering, anxiety, and childhood trauma, resulting in substance dependence to manage their emotional experiences, establishing a vicious cycle (Bushnell et al., 2019).
The proper development of the CNS requires essential cellular processes and must be fine-tuned to ensure its adequate formation (Andersen, 2003). It is known that substance use alters the structure and function of serotonergic and dopaminergic neurons during adolescence, making the developing brain highly susceptible to the neurotoxic effects of drug exposure (Squeglia et al., 2012; Pfefferbaum et al., 2018). For example, neural activation and volume of cortical areas in adolescents predict alcohol consumption and alcohol-related problems (Norman et al., 2011; Cheetham et al., 2014). On the other hand, ELS can affect neurons and glial cells during neurodevelopment (Schafer and Stevens, 2015; Allen and Lyons, 2018; Johnson and Kaffman, 2018; Li and Barres, 2018), including structures and components of the reward system (Lukkes et al., 2009; Hanson et al., 2021; Moustafa et al., 2021). Indeed, there is an important link between ELS and SUD development through adolescent substance use (Kirsch and Lippard, 2022). Rodent models show that different stressors during adolescence or the corresponding pre-adolescence period increase drug consumption during adulthood (Kosten et al., 2000, 2004; Baarendse et al., 2014; Garcia-Pardo et al., 2015). Maternal separation (MS), an ELS closer to the time of birth, has also been shown to increase self-administered alcohol drinking and morphine preference during adulthood (Jaworski et al., 2005; Vazquez et al., 2005; Michaels and Holtzman, 2008; Gondre-Lewis et al., 2016; Lewis et al., 2016). Previous studies reported arginine vasopressin gene expression changes and HPA axis activation after MS (Murgatroyd and Spengler, 2011; de Almeida Magalhaes et al., 2018). The HPA axis's ability to influence substance use seems to be so important that it has been placed as a potential target to assess the probability of relapse in cocaine-dependent individuals (Sinha et al., 2006). The ELS occurring in a range from weaning to early adulthood can affect substance use (McCool and Chappell, 2009; Lopez et al., 2011), suggesting that any period during early life is sensitive to stress effects with crucial implications for SUD development.
1.3 SUD and stress at cellular level
Stress occurs not only at psychological and physiological levels but also at a cellular level, e.g., oxidative stress and neuroinflammation. Recent findings highlight oxidative stress and inflammation as pivotal factors in drug-induced disruption of brain homeostasis (Berrios-Carcamo et al., 2020). For example, research in mice has demonstrated that the administration of indomethacin, a potent anti-inflammatory agent, reduced methamphetamine-induced neuroinflammation (Goncalves et al., 2008) and prolonged use of various addictive substances elevates inflammatory responses in the periphery and central nervous system (CNS; Cahill and Taylor, 2017; Leclercq et al., 2017; Hofford et al., 2019; Kohno et al., 2019). This situation could initiate an inflammatory response through increased microglial and astrocytic reactivity (Kraft and Harry, 2011; Clark et al., 2013; Colombo and Farina, 2016). Moreover, increased microglial and astrocytic reactivity has been observed in response to amphetamines (Zhang et al., 2015), cocaine (Periyasamy et al., 2018), ethanol, nicotine (Alfonso-Loeches et al., 2010; Quintanilla et al., 2018, 2019), opioids (Wang et al., 2012), and cannabinoids (Cutando et al., 2013; Zamberletti et al., 2015). These cells can sense cellular environmental alterations and trigger inflammatory responses through pattern recognition receptors, including Toll-like receptors (TLRs; Kraft and Harry, 2011; Fischer and Maier, 2015). Microglia respond to pro-inflammatory signals by altering their reactivity and gene expression, leading to elevated production of oxidative enzymes like NADPH oxidase (NOX) and inducible nitric oxide synthase (iNOS). This response increases the generation of reactive oxygen species (ROS) and reactive nitrogen species (RNS; Block et al., 2007). Cocaine and opioids induce TNF-α, IL-1β, and IL-6 release through microglial and astrocytic TLR4 activation, and IL-1β, IL-6, IL-18, IL-33, MCP-1, and TNF-α production via NF-κB and NLRP3 inflammasome pathways (Hutchinson et al., 2010; Crews et al., 2013; Northcutt et al., 2015; Pan et al., 2016; Bayazit et al., 2017; Eidson et al., 2017; Berrios-Carcamo et al., 2020; Figure 1B).
Studies in animal models have shown that chronic alcohol use increases pro-inflammatory cytokines, inhibits neurogenesis, and induces long-term behavioral changes (Nixon and Crews, 2002; Pascual et al., 2007). Furthermore, excessive DA released in response to methamphetamine undergoes oxidation, leading to the formation of toxic quinones. This process triggers oxidative stress, causes mitochondrial dysfunction, and damages presynaptic membranes by generating free radicals like superoxide and hydrogen peroxide (Shah et al., 2012). The cause of oxidative stress in the brain may be due to excessive production of free radicals, decreased activity of antioxidant enzymes, or decreased concentration of reducing factors (Lin and Beal, 2006; Kaminski et al., 2024), where ROS and RNS, for example, exert toxic effects on the CNS cellular components, resulting in neuronal death (Berg et al., 2004). Several studies showed that SUDs and oxidative stress are linked since the presence of one correlates with the other's development (Cunha-Oliveira et al., 2010; Zahmatkesh et al., 2017; Kaminski et al., 2024). Cannabis smoke exposure increases oxidative stress, like tobacco's effect (Aguiar et al., 2019), leading to increased ceruloplasmin and lipid hydroperoxides and decreased free thiol (Bayazit et al., 2020). Indeed, tetrahydrocannabinol (THC), a psychoactive substance found in cannabis, increases lipoperoxidation and reduces superoxide dismutase (SOD) enzyme activity in brain tissue (Kopjar et al., 2019). Exposure to amphetamines damages the mitochondrial membrane and oxidates lipids and proteins through increased ROS production (Brown and Yamamoto, 2003; Fitzmaurice et al., 2006; Perfeito et al., 2013; Basmadjian et al., 2021). Cocaine depletes reduced glutathione (GSH) in the heart and liver (Graziani et al., 2016), decreases catalase activity in the striatum and mPFC (Macedo et al., 2005), and glutathione peroxidase and GSH reduction in HPC (Mahoney, 2019). Finally, studies indicate that heroin increases ROS production and oxidative damage to proteins and lipids in the brain and liver (Graziani et al., 2016), decreases SOD, CAT, and GPx activity, and GSH/glutathione disulfide ratio reduction (Cemek et al., 2011; Zahmatkesh et al., 2017; Salarian et al., 2018; Tomek et al., 2019).
1.4 Genetic hallmarks of SUD
Some fundamental questions, such as “Why are some individuals more vulnerable to SUDs than others?” and “Does stress influence individual vulnerability?” remain unanswered. A possible mechanism for individual vulnerability to stress-induced substance use is through epigenetic modulation (Figure 2). There is substantial evidence for ELS-induced epigenetic changes influencing substance use in adulthood (Provencal and Binder, 2015). Also, ELS seems to induce dense DNA methylation of the GR gene (NR3C1), which correlates with major depressive disorder (Holmes et al., 2019). Interestingly, substance use also induces epigenetic changes, such as post-translational modifications, acetylation, methylation, phosphorylation, ubiquitination, SUMOylation, crotonylation, citrullination, and ADP-ribosylation, as well as methylation of the DNA itself (Nestler, 2014; Walker and Nestler, 2018). Several miRNAs are regulated after drug exposure (Doura and Unterwald, 2016), with the expression of some in striatum neurons altering drug-related behaviors (Hollander et al., 2010; Chandrasekar and Dreyer, 2011; Quinn et al., 2015).
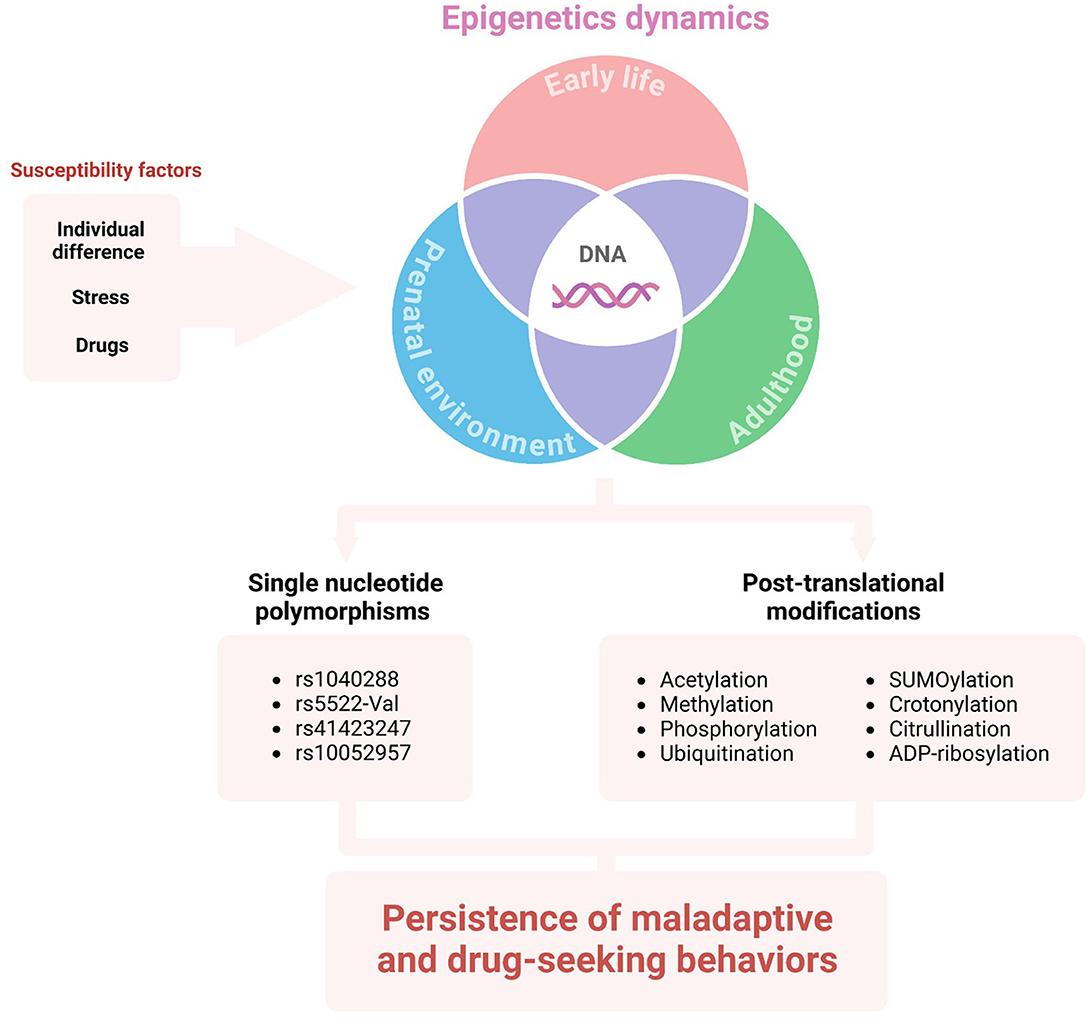
Figure 2. The influence of DNA epigenetics on vulnerability to SUD. Individual differences (e.g., environment, habits, life history, genetic background), as well as stress and/or drug exposure may lead to epigenetic alterations in different life stages. The single nucleotide polymorphisms and post-translational modifications of HPA-axis genes or mediators can sustain maladaptive behavior and substance use disorder.
Additionally, it is well known that minor genetic variations between the population correlate with variations in disorder development risk. Single nucleotide polymorphisms (SNPs) in genes associated with the HPA axis can modify the risk for drug abuse and abstinence symptoms. For example, the NR3C2 gene located in the 4q31.1v chromosome encodes the MR. The rs1040288 SNP results in a displacement of G to C nucleotide in an intronic region of the gene and has been identified as a risk factor for cocaine and heroin abuse in a non-population-specific manner (Levran et al., 2014). Regarding ELS, there is an association between childhood physical neglect and the SNP rs5522-Val allele modulating crack/cocaine abuse (Rovaris et al., 2015). The rs5522 SNP consists of an A/G transition in an exonic region of the gene, which results in a substitution of isoleucine to a valine.
On the other hand, GR is encoded by the NR3C1 gene located at the 5q31-32 chromosome. Atypical GR sensitivity underpins the pathophysiology of drug abuse, continuation, and relapse. The rs41423247 SNP consists of a displacement of G to C nucleotide in an intronic region of the gene. This SNP homozygous mutation has been associated with depression (Peng et al., 2018). Its minor allele C is a risk factor for higher depressive symptoms during early abstinence from crack/cocaine abuse, while the CC genotype appears to correlate with late abstinence (Rovaris et al., 2016). The rs41423247 minor allele C and rs10052957 minor allele G (an SNP that results in displacement of A to G) have been associated with an increased risk for cocaine abuse and a higher burden of depression when combined in a haplotype (Schote et al., 2019).
2 Discussion and conclusions
This mini-review explores how substance use alters brain circuits involved in reward processing and stress response (the “anti-reward” system), linking these changes to the three-stage model of SUD and their anatomical and endocrine features. It integrates cellular stress, which is closely tied to SUD. Depending on its nature, intensity, and duration, stress impacts HPA axis modulation, brain plasticity, and cellular processes (Albernaz-Mariano et al., 2025). Thus, ELS was highlighted due to its strong translational body of evidence and association with blunted stress responses and increased SUD risk (initiation, maintenance, relapse), mediated by ELS-induced reward and stress pathways changes. Finally, individual vulnerability to SUD was examined through (epi)genetics, emphasizing how drug use and life experiences can alter gene expression and increase SUD risk in susceptible individuals.
In conclusion, substance use can disrupt major brain circuits and neuroendocrine systems, resulting in altered behavioral responses to reward and stress. Furthermore, exposure to stress, particularly early-life stress (ELS), may increase susceptibility to substance use disorder (SUD) during adolescence and adulthood. Cellular stress induced by either stress or SUD plays a significant role in this process, offering potential therapeutic targets. Additionally, genetic factors may provide a means to identify at-risk individuals, enabling early intervention and prevention of SUD development.
Author contributions
VJ: Conceptualization, Writing – original draft, Writing – review & editing. KA-M: Conceptualization, Writing – original draft, Writing – review & editing. LC: Writing – original draft, Writing – review & editing. PJ: Writing – original draft, Writing – review & editing. RP: Writing – original draft, Writing – review & editing. AS-d-A: Writing – original draft, Writing – review & editing. LS: Writing – original draft, Writing – review & editing. ED: Writing – original draft, Writing – review & editing. CM: Conceptualization, Funding acquisition, Writing – original draft, Writing – review & editing.
Funding
The author(s) declare that financial support was received for the research and/or publication of this article. This work was supported by the Fundação de Amparo à Pesquisa do Estado de São Paulo (FAPESP 2016/03572-3, 2019/00908-9, and 2021/04077-4) and Conselho Nacional de Desenvolvimento Científico e Tecnológico (CNPq 422523/2016-0) to CM. VJ was supported by FAPESP (2020/06914-8 and 2021/13524-4) and CNPq (PIBIC 2019-1429). KA-M was supported by FAPESP (2021/14690-5). LC was supported by CNPq (166996/2022-0). PJ was supported by FAPESP (2022/08767-8). RP was supported by FAPESP (2023/13386-6) and CAPES. AS-d-A was supported by FAPESP (2019/00400-5 and 2021/12488-4) and CAPES. LS was supported by CNPq (PiBIQ) and CAPES. ED was supported by FAPESP (2022/12679-7 and 2023/09933-1) and CNPq. CM is a research fellow from CNPq. This study was financed in part by the Coordenação de Aperfeiçoamento de Pessoal de Nível Superior -Brasil (CAPES) -Finance Code 001.
Conflict of interest
The authors declare that the research was conducted in the absence of any commercial or financial relationships that could be construed as a potential conflict of interest.
Generative AI statement
The author(s) declare that no Gen AI was used in the creation of this manuscript.
Publisher's note
All claims expressed in this article are solely those of the authors and do not necessarily represent those of their affiliated organizations, or those of the publisher, the editors and the reviewers. Any product that may be evaluated in this article, or claim that may be made by its manufacturer, is not guaranteed or endorsed by the publisher.
References
Aguiar, J. A., Huff, R. D., Tse, W., Stampfli, M. R., McConkey, B. J., Doxey, A. C., et al. (2019). Transcriptomic and barrier responses of human airway epithelial cells exposed to cannabis smoke. Physiol. Rep. 7:e14249. doi: 10.14814/phy2.14249
Albernaz-Mariano, K. A., Juliano, V. A. L., Duque, E. A., Pereira, R. M., Novaes, L. S., Munhoz, C. D., et al. (2025). “Modeling stress-related hormones and delayed anxiety,” in Handbook of the Biology and Pathology of Mental Disorders, eds. C. R. Martin, V. R. Preedy, V. B. Patel, and R. Rajendram (Cham: Springer International Publishing), 1–27. doi: 10.1007/978-3-031-32035-4_11-1
Alegria, A. A., Hasin, D. S., Nunes, E. V., Liu, S. M., Davies, C., Grant, B. F., et al. (2010). Comorbidity of generalized anxiety disorder and substance use disorders: results from the national epidemiologic survey on alcohol and related conditions. J. Clin. Psychiatry 71, 1187–1195; quiz 1252–1183. doi: 10.4088/JCP.09m05328gry
Alfonso-Loeches, S., Pascual-Lucas, M., Blanco, A. M., Sanchez-Vera, I., and Guerri, C. (2010). Pivotal role of TLR4 receptors in alcohol-induced neuroinflammation and brain damage. J. Neurosci. 30, 8285–8295. doi: 10.1523/JNEUROSCI.0976-10.2010
Alizadehgoradel, J., Nejati, V., Sadeghi Movahed, F., Imani, S., Taherifard, M., Mosayebi-Samani, M., et al. (2020). Repeated stimulation of the dorsolateral-prefrontal cortex improves executive dysfunctions and craving in drug addiction: a randomized, double-blind, parallel-group study. Brain Stimul. 13, 582–593. doi: 10.1016/j.brs.2019.12.028
Allen, N. J., and Lyons, D. A. (2018). Glia as architects of central nervous system formation and function. Science 362:181. doi: 10.1126/science.aat0473
American Psychiatric Association (2013). Diagnostic and Statistical Manual of Mental Disorders, 5th Edn. doi: 10.1176/appi.books.9780890425596
Andersen, S. L. (2003). Trajectories of brain development: point of vulnerability or window of opportunity? Neurosci. Biobehav. Rev. 27, 3–18. doi: 10.1016/S0149-7634(03)00005-8
Andersen, S. L. (2019). Stress, sensitive periods, and substance abuse. Neurobiol. Stress 10:100140. doi: 10.1016/j.ynstr.2018.100140
Baarendse, P. J., Limpens, J. H., and Vanderschuren, L. J. (2014). Disrupted social development enhances the motivation for cocaine in rats. Psychopharmacology 231, 1695–1704. doi: 10.1007/s00213-013-3362-8
Baik, J. H. (2020). Stress and the dopaminergic reward system. Exp. Mol. Med. 52, 1879–1890. doi: 10.1038/s12276-020-00532-4
Basmadjian, O. M., Occhieppo, V. B., Marchese, N. A., Silvero, C. M., Becerra, M. C., Baiardi, G., et al. (2021). Amphetamine induces oxidative stress, glial activation and transient angiogenesis in prefrontal cortex via AT(1)-R. Front. Pharmacol. 12:647747. doi: 10.3389/fphar.2021.647747
Bayazit, H., Dulgeroglu, D., and Selek, S. (2020). Brain-derived neurotrophic factor and oxidative stress in cannabis dependence. Neuropsychobiology 79, 186–190. doi: 10.1159/000504626
Bayazit, H., Selek, S., Karababa, I. F., Cicek, E., and Aksoy, N. (2017). Evaluation of oxidant/antioxidant status and cytokine levels in patients with cannabis use disorder. Clin. Psychopharmacol. Neurosci. 15, 237–242. doi: 10.9758/cpn.2017.15.3.237
Beato, M., and Sanchez-Pacheco, A. (1996). Interaction of steroid hormone receptors with the transcription initiation complex. Endocr. Rev. 17, 587–609. doi: 10.1210/edrv-17-6-587
Berg, D., Youdim, M. B., and Riederer, P. (2004). Redox imbalance. Cell Tissue Res. 318, 201–213. doi: 10.1007/s00441-004-0976-5
Berhe, O., Gerhardt, S., and Schmahl, C. (2022). Clinical outcomes of severe forms of early social stress. Curr. Top. Behav. Neurosci. 54, 417–438. doi: 10.1007/7854_2021_261
Berrios-Carcamo, P., Quezada, M., Quintanilla, M. E., Morales, P., Ezquer, M., Herrera-Marschitz, M., et al. (2020). Oxidative stress and neuroinflammation as a pivot in drug abuse. a focus on the therapeutic potential of antioxidant and anti-inflammatory agents and biomolecules. Antioxidants 9:830. doi: 10.3390/antiox9090830
Block, M. L., Zecca, L., and Hong, J. S. (2007). Microglia-mediated neurotoxicity: uncovering the molecular mechanisms. Nat. Rev. Neurosci. 8, 57–69. doi: 10.1038/nrn2038
Brown, J. M., and Yamamoto, B. K. (2003). Effects of amphetamines on mitochondrial function: role of free radicals and oxidative stress. Pharmacol. Ther. 99, 45–53. doi: 10.1016/S0163-7258(03)00052-4
Bushnell, G. A., Gaynes, B. N., Compton, S. N., Dusetzina, S. B., Olfson, M., Sturmer, T., et al. (2019). Incident substance use disorder following anxiety disorder in privately insured youth. J. Adolesc. Health 65, 536–542. doi: 10.1016/j.jadohealth.2019.05.007
Cahill, C. M., and Taylor, A. M. (2017). Neuroinflammation-a co-occurring phenomenon linking chronic pain and opioid dependence. Curr. Opin. Behav. Sci. 13, 171–177. doi: 10.1016/j.cobeha.2016.12.003
Castaldelli-Maia, J. M., and Bhugra, D. (2022). Analysis of global prevalence of mental and substance use disorders within countries: focus on sociodemographic characteristics and income levels. Int. Rev. Psychiatry 34, 6–15. doi: 10.1080/09540261.2022.2040450
Cemek, M., Buyukokuroglu, M. E., Hazman, O., Bulut, S., Konuk, M., Birdane, Y., et al. (2011). Antioxidant enzyme and element status in heroin addiction or heroin withdrawal in rats: effect of melatonin and vitamin E plus Se. Biol. Trace Elem. Res. 139, 41–54. doi: 10.1007/s12011-010-8634-0
Chandrasekar, V., and Dreyer, J. L. (2011). Regulation of MiR-124, Let-7d, and MiR-181a in the accumbens affects the expression, extinction, and reinstatement of cocaine-induced conditioned place preference. Neuropsychopharmacology 36, 1149–1164. doi: 10.1038/npp.2010.250
Cheetham, A., Allen, N. B., Whittle, S., Simmons, J., Yucel, M., Lubman, D. I., et al. (2014). Volumetric differences in the anterior cingulate cortex prospectively predict alcohol-related problems in adolescence. Psychopharmacology 231, 1731–1742. doi: 10.1007/s00213-014-3483-8
Clark, K. H., Wiley, C. A., and Bradberry, C. W. (2013). Psychostimulant abuse and neuroinflammation: emerging evidence of their interconnection. Neurotox. Res. 23, 174–188. doi: 10.1007/s12640-012-9334-7
Colombo, E., and Farina, C. (2016). Astrocytes: key regulators of neuroinflammation. Trends Immunol. 37, 608–620. doi: 10.1016/j.it.2016.06.006
Crews, F. T., Qin, L., Sheedy, D., Vetreno, R. P., and Zou, J. (2013). High mobility group box 1/Toll-like receptor danger signaling increases brain neuroimmune activation in alcohol dependence. Biol. Psychiatry 73, 602–612. doi: 10.1016/j.biopsych.2012.09.030
Cunha-Oliveira, T., Rego, A. C., Garrido, J., Borges, F., Macedo, T., Oliveira, C. R., et al. (2010). Neurotoxicity of heroin-cocaine combinations in rat cortical neurons. Toxicology 276, 11–17. doi: 10.1016/j.tox.2010.06.009
Cutando, L., Busquets-Garcia, A., Puighermanal, E., Gomis-Gonzalez, M., Delgado-Garcia, J. M., Gruart, A., et al. (2013). Microglial activation underlies cerebellar deficits produced by repeated cannabis exposure. J. Clin. Invest. 123, 2816–2831. doi: 10.1172/JCI67569
de Almeida Magalhaes, T., Correia, D., de Carvalho, L. M., Damasceno, S., and Brunialti Godard, A. L. (2018). Maternal separation affects expression of stress response genes and increases vulnerability to ethanol consumption. Brain Behav. 8:e00841. doi: 10.1002/brb3.841
Doura, M. B., and Unterwald, E. M. (2016). MicroRNAs modulate interactions between stress and risk for cocaine addiction. Front. Cell Neurosci. 10:125. doi: 10.3389/fncel.2016.00125
Drugs and Crime (2024). World Drug Report 2024. United Nations Office on Drugs and Crime, Vienna International Centre, Vienna, Austria.
Eidson, L. N., Inoue, K., Young, L. J., Tansey, M. G., and Murphy, A. Z. (2017). Toll-like receptor 4 mediates morphine-induced neuroinflammation and tolerance via soluble tumor necrosis factor signaling. Neuropsychopharmacology 42, 661–670. doi: 10.1038/npp.2016.131
Fischer, R., and Maier, O. (2015). Interrelation of oxidative stress and inflammation in neurodegenerative disease: role of TNF. Oxid. Med. Cell Longev. 2015:610813. doi: 10.1155/2015/610813
Fitzmaurice, P. S., Tong, J., Yazdanpanah, M., Liu, P. P., Kalasinsky, K. S., Kish, S. J., et al. (2006). Levels of 4-hydroxynonenal and malondialdehyde are increased in brain of human chronic users of methamphetamine. J. Pharmacol. Exp. Ther. 319, 703–709. doi: 10.1124/jpet.106.109173
Forster, G. L., Anderson, E. M., Scholl, J. L., Lukkes, J. L., and Watt, M. J. (2018). Negative consequences of early-life adversity on substance use as mediated by corticotropin-releasing factor modulation of serotonin activity. Neurobiol. Stress 9, 29–39. doi: 10.1016/j.ynstr.2018.08.001
Garcia-Pardo, M. P., Blanco-Gandia, M. C., Valiente-Lluch, M., Rodriguez-Arias, M., Minarro, J., Aguilar, M. A., et al. (2015). Long-term effects of repeated social stress on the conditioned place preference induced by MDMA in mice. Prog. Neuropsychopharmacol. Biol. Psychiatry 63, 98–109. doi: 10.1016/j.pnpbp.2015.06.006
Gilpin, N. W., Herman, M. A., and Roberto, M. (2015). The central amygdala as an integrative hub for anxiety and alcohol use disorders. Biol. Psychiatry 77, 859–869. doi: 10.1016/j.biopsych.2014.09.008
Goldfarb, E. V., and Sinha, R. (2018). Drug-induced glucocorticoids and memory for substance use. Trends Neurosci. 41, 853–868. doi: 10.1016/j.tins.2018.08.005
Goncalves, J., Martins, T., Ferreira, R., Milhazes, N., Borges, F., Ribeiro, C. F., et al. (2008). Methamphetamine-induced early increase of IL-6 and TNF-alpha mRNA expression in the mouse brain. Ann. N.Y. Acad. Sci. 1139, 103–111. doi: 10.1196/annals.1432.043
Gondre-Lewis, M. C., Warnock, K. T., Wang, H., June, H. L. Jr., Bell, K. A., Rabe, H., et al. (2016). Early life stress is a risk factor for excessive alcohol drinking and impulsivity in adults and is mediated via a CRF/GABA(A) mechanism. Stress 19, 235–247. doi: 10.3109/10253890.2016.1160280
Goodwin, R. D., Fergusson, D. M., and Horwood, L. J. (2004). Association between anxiety disorders and substance use disorders among young persons: results of a 21-year longitudinal study. J. Psychiatr. Res. 38, 295–304. doi: 10.1016/j.jpsychires.2003.09.002
Graziani, M., Antonilli, L., Togna, A. R., Grassi, M. C., Badiani, A., Saso, L., et al. (2016). Cardiovascular and hepatic toxicity of cocaine: potential beneficial effects of modulators of oxidative stress. Oxid. Med. Cell Longev. 2016:8408479. doi: 10.1155/2016/8408479
Groeneweg, F. L., Karst, H., de Kloet, E. R., and Joels, M. (2011). Rapid non-genomic effects of corticosteroids and their role in the central stress response. J. Endocrinol. 209, 153–167. doi: 10.1530/JOE-10-0472
Hanson, J. L., Williams, A. V., Bangasser, D. A., and Pena, C. J. (2021). Impact of early life stress on reward circuit function and regulation. Front. Psychiatry 12:744690. doi: 10.3389/fpsyt.2021.744690
Hofford, R. S., Russo, S. J., and Kiraly, D. D. (2019). Neuroimmune mechanisms of psychostimulant and opioid use disorders. Eur. J. Neurosci. 50, 2562–2573. doi: 10.1111/ejn.14143
Hollander, J. A., Im, H. I., Amelio, A. L., Kocerha, J., Bali, P., Lu, Q., et al. (2010). Striatal microRNA controls cocaine intake through CREB signalling. Nature 466, 197–202. doi: 10.1038/nature09202
Holmes, L. Jr., Shutman, E., Chinaka, C., Deepika, K., Pelaez, L., and Dabney, K. W. (2019). Aberrant epigenomic modulation of glucocorticoid receptor gene (NR3C1) in early life stress and major depressive disorder correlation: systematic review and quantitative evidence synthesis. Int. J. Environ. Res. Public Health 16:4280. doi: 10.3390/ijerph16214280
Hunt, G. E., Malhi, G. S., Cleary, M., Lai, H. M., and Sitharthan, T. (2016). Comorbidity of bipolar and substance use disorders in national surveys of general populations, 1990-2015: systematic review and meta-analysis. J. Affect. Disord. 206, 321–330. doi: 10.1016/j.jad.2016.06.051
Hunt, G. E., Malhi, G. S., Lai, H. M. X., and Cleary, M. (2020). Prevalence of comorbid substance use in major depressive disorder in community and clinical settings, 1990-2019: systematic review and meta-analysis. J. Affect. Disord. 266, 288–304. doi: 10.1016/j.jad.2020.01.141
Hutchinson, M. R., Zhang, Y., Shridhar, M., Evans, J. H., Buchanan, M. M., Zhao, T. X., et al. (2010). Evidence that opioids may have toll-like receptor 4 and MD-2 effects. Brain Behav. Immun. 24, 83–95. doi: 10.1016/j.bbi.2009.08.004
Jaworski, J. N., Francis, D. D., Brommer, C. L., Morgan, E. T., and Kuhar, M. J. (2005). Effects of early maternal separation on ethanol intake, GABA receptors and metabolizing enzymes in adult rats. Psychopharmacology 181, 8–15. doi: 10.1007/s00213-005-2232-4
Johnson, F. K., and Kaffman, A. (2018). Early life stress perturbs the function of microglia in the developing rodent brain: new insights and future challenges. Brain Behav. Immun. 69, 18–27. doi: 10.1016/j.bbi.2017.06.008
Kaminski, P., Lorek, M., Baszynski, J., Tadrowski, T., Gorzelanczyk, E. J., Feit, J., et al. (2024). Role of antioxidants in the neurobiology of drug addiction: an update. Biomed. Pharmacother. 175:116604. doi: 10.1016/j.biopha.2024.116604
Kielbinski, M., Bernacka, J., and Solecki, W. B. (2019). Differential regulation of phasic dopamine release in the forebrain by the VTA noradrenergic receptor signaling. J. Neurochem. 149, 747–759. doi: 10.1111/jnc.14706
Kirsch, D. E., and Lippard, E. T. C. (2022). Early life stress and substance use disorders: the critical role of adolescent substance use. Pharmacol. Biochem. Behav. 215:173360. doi: 10.1016/j.pbb.2022.173360
Koenig, H. N., and Olive, M. F. (2004). The glucocorticoid receptor antagonist mifepristone reduces ethanol intake in rats under limited access conditions. Psychoneuroendocrinology 29, 999–1003. doi: 10.1016/j.psyneuen.2003.09.004
Kohno, M., Link, J., Dennis, L. E., McCready, H., Huckans, M., Hoffman, W. F., et al. (2019). Neuroinflammation in addiction: a review of neuroimaging studies and potential immunotherapies. Pharmacol. Biochem. Behav. 179, 34–42. doi: 10.1016/j.pbb.2019.01.007
Koob, G. F. (2008). A role for brain stress systems in addiction. Neuron 59, 11–34. doi: 10.1016/j.neuron.2008.06.012
Koob, G. F., and Le Moal, M. (1997). Drug abuse: hedonic homeostatic dysregulation. Science 278, 52–58. doi: 10.1126/science.278.5335.52
Koob, G. F., and Le Moal, M. (2001). Drug addiction, dysregulation of reward, and allostasis. Neuropsychopharmacology 24, 97–129. doi: 10.1016/S0893-133X(00)00195-0
Koob, G. F., and Volkow, N. D. (2010). Neurocircuitry of addiction. Neuropsychopharmacology 35, 217–238. doi: 10.1038/npp.2009.110
Kopjar, N., Fuchs, N., Zunec, S., Mikolic, A., Micek, V., Kozina, G., et al. (2019). DNA damaging effects, oxidative stress responses and cholinesterase activity in blood and brain of wistar rats exposed to delta(9)-tetrahydrocannabinol. Molecules 24:1560. doi: 10.3390/molecules24081560
Kosten, T. A., Miserendino, M. J., and Kehoe, P. (2000). Enhanced acquisition of cocaine self-administration in adult rats with neonatal isolation stress experience. Brain Res. 875, 44–50. doi: 10.1016/S0006-8993(00)02595-6
Kosten, T. A., Sanchez, H., Zhang, X. Y., and Kehoe, P. (2004). Neonatal isolation enhances acquisition of cocaine self-administration and food responding in female rats. Behav. Brain Res. 151, 137–149. doi: 10.1016/j.bbr.2003.08.010
Kraft, A. D., and Harry, G. J. (2011). Features of microglia and neuroinflammation relevant to environmental exposure and neurotoxicity. Int. J. Environ. Res. Public Health 8, 2980–3018. doi: 10.3390/ijerph8072980
Le Moal, M. (2007). Historical approach and evolution of the stress concept: a personal account. Psychoneuroendocrinology 32(Suppl. 1), S3–9. doi: 10.1016/j.psyneuen.2007.03.019
Le, T. M., Potvin, S., Zhornitsky, S., and Li, C. R. (2021). Distinct patterns of prefrontal cortical disengagement during inhibitory control in addiction: a meta-analysis based on population characteristics. Neurosci. Biobehav. Rev. 127, 255–269. doi: 10.1016/j.neubiorev.2021.04.028
Leclercq, S., de Timary, P., Delzenne, N. M., and Starkel, P. (2017). The link between inflammation, bugs, the intestine and the brain in alcohol dependence. Transl. Psychiatry 7:e1048. doi: 10.1038/tp.2017.15
Levran, O., Randesi, M., Li, Y., Rotrosen, J., Ott, J., Adelson, M., et al. (2014). Drug addiction and stress-response genetic variability: association study in African Americans. Ann. Hum. Genet. 78, 290–298. doi: 10.1111/ahg.12064
Lewis, C. R., Bastle, R. M., Manning, T. B., Himes, S. M., Fennig, P., Conrad, P. R., et al. (2016). Interactions between early life stress, nucleus accumbens MeCP2 expression, and methamphetamine self-administration in male rats. Neuropsychopharmacology 41, 2851–2861. doi: 10.1038/npp.2016.96
Li, Q., and Barres, B. A. (2018). Microglia and macrophages in brain homeostasis and disease. Nat. Rev. Immunol. 18, 225–242. doi: 10.1038/nri.2017.125
Lin, M. T., and Beal, M. F. (2006). Mitochondrial dysfunction and oxidative stress in neurodegenerative diseases. Nature 443, 787–795. doi: 10.1038/nature05292
Liu, J. F., and Li, J. X. (2018). Drug addiction: a curable mental disorder? Acta Pharmacol. Sin. 39, 1823–1829. doi: 10.1038/s41401-018-0180-x
Lopez, M. F., Doremus-Fitzwater, T. L., and Becker, H. C. (2011). Chronic social isolation and chronic variable stress during early development induce later elevated ethanol intake in adult C57BL/6J mice. Alcohol 45, 355–364. doi: 10.1016/j.alcohol.2010.08.017
Lowes, D. C., Chamberlin, L. A., Kretsge, L. N., Holt, E. S., Abbas, A. I., Park, A. J., et al. (2021). Ventral tegmental area GABA neurons mediate stress-induced blunted reward-seeking in mice. Nat. Commun. 12:3539. doi: 10.1038/s41467-021-23906-2
Lukkes, J. L., Watt, M. J., Lowry, C. A., and Forster, G. L. (2009). Consequences of post-weaning social isolation on anxiety behavior and related neural circuits in rodents. Front. Behav. Neurosci. 3:18. doi: 10.3389/neuro.08.018.2009
Macedo, D. S., de Vasconcelos, S. M., dos Santos, R. S., Aguiar, L. M., Lima, V. T., Viana, G. S., et al. (2005). Cocaine alters catalase activity in prefrontal cortex and striatum of mice. Neurosci. Lett. 387, 53–56. doi: 10.1016/j.neulet.2005.07.024
Madur, L., Ineichen, C., Bergamini, G., Greter, A., Poggi, G., Cuomo-Haymour, N., et al. (2023). Stress deficits in reward behaviour are associated with and replicated by dysregulated amygdala-nucleus accumbens pathway function in mice. Commun. Biol. 6:422. doi: 10.1038/s42003-023-04811-4
Mahoney, J. J. (2019). Cognitive dysfunction in individuals with cocaine use disorder: potential moderating factors and pharmacological treatments. Exp. Clin. Psychopharmacol. 27, 203–214. doi: 10.1037/pha0000245
Mantsch, J. R., and Gasser, P. J. (2015). “Glucocorticoid involvement in drug abuse and addiction,” in Neuroendocrinology of Stress, eds. J. R. Russell and M. J. Shipston (Hoboken, NJ: Willey), 195–218. doi: 10.1002/9781118921692.ch9
McCauley, J. L., Killeen, T., Gros, D. F., Brady, K. T., and Back, S. E. (2012). Posttraumatic stress disorder and co-occurring substance use disorders: advances in assessment and treatment. Clin. Psychol. 19, 283–304. doi: 10.1111/cpsp.12006
McCool, B. A., and Chappell, A. M. (2009). Early social isolation in male Long-Evans rats alters both appetitive and consummatory behaviors expressed during operant ethanol self-administration. Alcohol. Clin. Exp. Res. 33, 273–282. doi: 10.1111/j.1530-0277.2008.00830.x
McEwen, B. S., and Wingfield, J. C. (2003). The concept of allostasis in biology and biomedicine. Horm. Behav. 43, 2–15. doi: 10.1016/S0018-506X(02)00024-7
McGrath, A. G., and Briand, L. A. (2019). A potential role for microglia in stress- and drug-induced plasticity in the nucleus accumbens: a mechanism for stress-induced vulnerability to substance use disorder. Neurosci. Biobehav. Rev. 107, 360–369. doi: 10.1016/j.neubiorev.2019.09.007
Michaels, C. C., and Holtzman, S. G. (2008). Early postnatal stress alters place conditioning to both mu- and kappa-opioid agonists. J. Pharmacol. Exp. Ther. 325, 313–318. doi: 10.1124/jpet.107.129908
Moustafa, A. A., Parkes, D., Fitzgerald, L., Underhill, D., Garami, J., Levy-Gigi, E., et al. (2021). The relationship between childhood trauma, early-life stress, and alcohol and drug use, abuse, and addiction: an integrative review. Curr. Psychol. 40, 579–584. doi: 10.1007/s12144-018-9973-9
Mukhara, D., Banks, M. L., and Neigh, G. N. (2018). Stress as a risk factor for substance use disorders: a mini-review of molecular mediators. Front. Behav. Neurosci. 12:309. doi: 10.3389/fnbeh.2018.00309
Murgatroyd, C., and Spengler, D. (2011). Epigenetic programming of the HPA axis: early life decides. Stress 14, 581–589. doi: 10.3109/10253890.2011.602146
Murphy, M. D., and Heller, E. A. (2022). Convergent actions of stress and stimulants via epigenetic regulation of neural circuitry. Trends Neurosci. 45, 955–967. doi: 10.1016/j.tins.2022.10.001
Nazeri, M., Ebrahimi, A., Aghaei, I., Ghotbi Ravandi, S., and Shabani, M. (2017). Psychological stress has a higher rate of developing addictive behaviors compared to physical stress in rat offspring. EXCLI J. 16, 903–913. doi: 10.17179/excli2016-685
Nestler, E. J. (2014). Epigenetic mechanisms of drug addiction. Neuropharmacology 76(Pt B), 259–25268. doi: 10.1016/j.neuropharm.2013.04.004
Nikbakhtzadeh, M., Ranjbar, H., Moradbeygi, K., Zahedi, E., Bayat, M., Soti, M., et al. (2023). Cross-talk between the HPA axis and addiction-related regions in stressful situations. Heliyon 9:e15525. doi: 10.1016/j.heliyon.2023.e15525
Nixon, K., and Crews, F. T. (2002). Binge ethanol exposure decreases neurogenesis in adult rat hippocampus. J. Neurochem. 83, 1087–1093. doi: 10.1046/j.1471-4159.2002.01214.x
Norman, A. L., Pulido, C., Squeglia, L. M., Spadoni, A. D., Paulus, M. P., Tapert, S. F., et al. (2011). Neural activation during inhibition predicts initiation of substance use in adolescence. Drug Alcohol Depend. 119, 216–223. doi: 10.1016/j.drugalcdep.2011.06.019
Northcutt, A. L., Hutchinson, M. R., Wang, X., Baratta, M. V., Hiranita, T., Cochran, T. A., et al. (2015). DAT isn't all that: cocaine reward and reinforcement require Toll-like receptor 4 signaling. Mol. Psychiatry 20, 1525–1537. doi: 10.1038/mp.2014.177
Oster, H., Challet, E., Ott, V., Arvat, E., de Kloet, E. R., Dijk, D. J., et al. (2017). The functional and clinical significance of the 24-hour rhythm of circulating glucocorticoids. Endocr. Rev. 38, 3–45. doi: 10.1210/er.2015-1080
Pan, Y., Sun, X., Jiang, L., Hu, L., Kong, H., Han, Y., et al. (2016). Metformin reduces morphine tolerance by inhibiting microglial-mediated neuroinflammation. J. Neuroinflamm. 13:294. doi: 10.1186/s12974-016-0754-9
Parnaudeau, S., Dongelmans, M. L., Turiault, M., Ambroggi, F., Delbes, A. S., Cansell, C., et al. (2014). Glucocorticoid receptor gene inactivation in dopamine-innervated areas selectively decreases behavioral responses to amphetamine. Front. Behav. Neurosci. 8:35. doi: 10.3389/fnbeh.2014.00035
Pascual, M., Blanco, A. M., Cauli, O., Minarro, J., and Guerri, C. (2007). Intermittent ethanol exposure induces inflammatory brain damage and causes long-term behavioural alterations in adolescent rats. Eur. J. Neurosci. 25, 541–550. doi: 10.1111/j.1460-9568.2006.05298.x
Peng, Q., Yan, H., Wen, Y., Lai, C., and Shi, L. (2018). Association between NR3C1 rs41423247 polymorphism and depression: a PRISMA-compliant meta-analysis. Medicine 97:e12541. doi: 10.1097/MD.0000000000012541
Perfeito, R., Cunha-Oliveira, T., and Rego, A. C. (2013). Reprint of: revisiting oxidative stress and mitochondrial dysfunction in the pathogenesis of Parkinson disease-resemblance to the effect of amphetamine drugs of abuse. Free Radic. Biol. Med. 62, 186–201. doi: 10.1016/j.freeradbiomed.2013.05.042
Periyasamy, P., Liao, K., Kook, Y. H., Niu, F., Callen, S. E., Guo, M. L., et al. (2018). Cocaine-mediated downregulation of miR-124 activates microglia by targeting KLF4 and TLR4 signaling. Mol. Neurobiol. 55, 3196–3210. doi: 10.1007/s12035-017-0584-5
Pfefferbaum, A., Kwon, D., Brumback, T., Thompson, W. K., Cummins, K., Tapert, S. F., et al. (2018). Altered brain developmental trajectories in adolescents after initiating drinking. Am. J. Psychiatry 175, 370–380. doi: 10.1176/appi.ajp.2017.17040469
Provencal, N., and Binder, E. B. (2015). The effects of early life stress on the epigenome: from the womb to adulthood and even before. Exp. Neurol. 268, 10–20. doi: 10.1016/j.expneurol.2014.09.001
Quinn, R. K., Brown, A. L., Goldie, B. J., Levi, E. M., Dickson, P. W., Smith, D. W., et al. (2015). Distinct miRNA expression in dorsal striatal subregions is associated with risk for addiction in rats. Transl. Psychiatry 5:e503. doi: 10.1038/tp.2014.144
Quintanilla, M. E., Ezquer, F., Morales, P., Santapau, D., Berrios-Carcamo, P., Ezquer, M., et al. (2019). Intranasal mesenchymal stem cell secretome administration markedly inhibits alcohol and nicotine self-administration and blocks relapse-intake: mechanism and translational options. Stem Cell Res. Ther. 10:205. doi: 10.1186/s13287-019-1304-z
Quintanilla, M. E., Morales, P., Ezquer, F., Ezquer, M., Herrera-Marschitz, M., Israel, Y., et al. (2018). Commonality of ethanol and nicotine reinforcement and relapse in wistar-derived UChB rats: inhibition by N-acetylcysteine. Alcohol. Clin. Exp. Res. 42, 1988–1999. doi: 10.1111/acer.13842
Roozendaal, B., Griffith, Q. K., Buranday, J., De Quervain, D. J., and McGaugh, J. L. (2003). The hippocampus mediates glucocorticoid-induced impairment of spatial memory retrieval: dependence on the basolateral amygdala. Proc. Natl. Acad. Sci. U.S.A. 100, 1328–1333. doi: 10.1073/pnas.0337480100
Rovaris, D. L., Aroche, A. P., da Silva, B. S., Kappel, D. B., Pezzi, J. C., Levandowski, M. L., et al. (2016). Glucocorticoid receptor gene modulates severity of depression in women with crack cocaine addiction. Eur. Neuropsychopharmacol. 26, 1438–1447. doi: 10.1016/j.euroneuro.2016.06.010
Rovaris, D. L., Mota, N. R., Bertuzzi, G. P., Aroche, A. P., Callegari-Jacques, S. M., Guimaraes, L. S., et al. (2015). Corticosteroid receptor genes and childhood neglect influence susceptibility to crack/cocaine addiction and response to detoxification treatment. J. Psychiatr. Res. 68, 83–90. doi: 10.1016/j.jpsychires.2015.06.008
Salarian, A., Kadkhodaee, M., Zahmatkesh, M., Seifi, B., Bakhshi, E., Akhondzadeh, S., et al. (2018). Opioid use disorder induces oxidative stress and inflammation: the attenuating effect of methadone maintenance treatment. Iran. J. Psychiatry 13, 46–54.
Schafer, D. P., and Stevens, B. (2015). Microglia function in central nervous system development and plasticity. Cold Spring Harb. Perspect. Biol. 7:a020545. doi: 10.1101/cshperspect.a020545
Schneiderman, N., Ironson, G., and Siegel, S. D. (2005). Stress and health: psychological, behavioral, and biological determinants. Annu. Rev. Clin. Psychol. 1, 607–628. doi: 10.1146/annurev.clinpsy.1.102803.144141
Schote, A. B., Jager, K., Kroll, S. L., Vonmoos, M., Hulka, L. M., Preller, K. H., et al. (2019). Glucocorticoid receptor gene variants and lower expression of NR3C1 are associated with cocaine use. Addict. Biol. 24, 730–742. doi: 10.1111/adb.12632
Shah, A., Silverstein, P. S., Singh, D. P., and Kumar, A. (2012). Involvement of metabotropic glutamate receptor 5, AKT/PI3K signaling and NF-kappaB pathway in methamphetamine-mediated increase in IL-6 and IL-8 expression in astrocytes. J. Neuroinflamm. 9:52. doi: 10.1186/1742-2094-9-52
Sinha, R., Garcia, M., Paliwal, P., Kreek, M. J., and Rounsaville, B. J. (2006). Stress-induced cocaine craving and hypothalamic-pituitary-adrenal responses are predictive of cocaine relapse outcomes. Arch. Gen. Psychiatry 63, 324–331. doi: 10.1001/archpsyc.63.3.324
Smith, K., Lacadie, C. M., Milivojevic, V., Fogelman, N., and Sinha, R. (2023). Sex differences in neural responses to stress and drug cues predicts future drug use in individuals with substance use disorder. Drug Alcohol Depend. 244:109794. doi: 10.1016/j.drugalcdep.2023.109794
Squeglia, L. M., Pulido, C., Wetherill, R. R., Jacobus, J., Brown, G. G., Tapert, S. F., et al. (2012). Brain response to working memory over three years of adolescence: influence of initiating heavy drinking. J. Stud. Alcohol Drugs 73, 749–760. doi: 10.15288/jsad.2012.73.749
Stamatakis, A. M., Sparta, D. R., Jennings, J. H., McElligott, Z. A., Decot, H., Stuber, G. D., et al. (2014). Amygdala and bed nucleus of the stria terminalis circuitry: implications for addiction-related behaviors. Neuropharmacology 76(Pt B), 320–32328. doi: 10.1016/j.neuropharm.2013.05.046
Tomek, S. E., Stegmann, G. M., and Olive, M. F. (2019). Effects of heroin on rat prosocial behavior. Addict. Biol. 24, 676–684. doi: 10.1111/adb.12633
Vazquez, V., Penit-Soria, J., Durand, C., Besson, M. J., Giros, B., Dauge, V., et al. (2005). Maternal deprivation increases vulnerability to morphine dependence and disturbs the enkephalinergic system in adulthood. J. Neurosci. 25, 4453–4462. doi: 10.1523/JNEUROSCI.4807-04.2005
Volkow, N. D., Fowler, J. S., Wang, G. J., Baler, R., and Telang, F. (2009). Imaging dopamine's role in drug abuse and addiction. Neuropharmacology 56(Suppl. 1), 3–8. doi: 10.1016/j.neuropharm.2008.05.022
Volkow, N. D., Koob, G. F., and McLellan, A. T. (2016). Neurobiologic advances from the brain disease model of addiction. N. Engl. J. Med. 374, 363–371. doi: 10.1056/NEJMra1511480
Volkow, N. D., and Morales, M. (2015). The brain on drugs: from reward to addiction. Cell 162, 712–725. doi: 10.1016/j.cell.2015.07.046
Walker, D. M., and Nestler, E. J. (2018). Neuroepigenetics and addiction. Handb. Clin. Neurol. 148, 747–765. doi: 10.1016/B978-0-444-64076-5.00048-X
Wang, X., Loram, L. C., Ramos, K., de Jesus, A. J., Thomas, J., Cheng, K., et al. (2012). Morphine activates neuroinflammation in a manner parallel to endotoxin. Proc. Natl. Acad. Sci. U.S.A. 109, 6325–6330. doi: 10.1073/pnas.1200130109
Yap, J. J., and Miczek, K. A. (2008). Stress and rodent models of drug addiction: role of VTA-accumbens-PFC-amygdala circuit. Drug Discov. Today Dis. Models 5, 259–270. doi: 10.1016/j.ddmod.2009.03.010
Zahmatkesh, M., Kadkhodaee, M., Salarian, A., Seifi, B., and Adeli, S. (2017). Impact of opioids on oxidative status and related signaling pathways: an integrated view. J. Opioid. Manag. 13, 241–251. doi: 10.5055/jom.2017.0392
Zamberletti, E., Gabaglio, M., Prini, P., Rubino, T., and Parolaro, D. (2015). Cortical neuroinflammation contributes to long-term cognitive dysfunctions following adolescent delta-9-tetrahydrocannabinol treatment in female rats. Eur. Neuropsychopharmacol. 25, 2404–2415. doi: 10.1016/j.euroneuro.2015.09.021
Keywords: HPA axis, neuroinflammation, mood disorders, early life stress, substance use disorder
Citation: Juliano VAL, Albernaz-Mariano KA, Covre LHH, Jucá PM, Pereira RM, Shigeo-de-Almeida A, Sampaio LL, Duque EdeA and Munhoz CD (2025) Neurobiological intersections of stress and substance use disorders. Front. Neurosci. 19:1548372. doi: 10.3389/fnins.2025.1548372
Received: 19 December 2024; Accepted: 07 April 2025;
Published: 01 May 2025.
Edited by:
Valentina Vozella, The Scripps Research Institute, United StatesReviewed by:
Scott Edwards, Louisiana State University, United StatesPaola Palombo, Federal University of São Paulo, Brazil
Natalia Bonetti Bertagna, Universidade Federal de São Paulo, Brazil
Suzanne Nolan, Vanderbilt University, United States
Copyright © 2025 Juliano, Albernaz-Mariano, Covre, Jucá, Pereira, Shigeo-de-Almeida, Sampaio, Duque and Munhoz. This is an open-access article distributed under the terms of the Creative Commons Attribution License (CC BY). The use, distribution or reproduction in other forums is permitted, provided the original author(s) and the copyright owner(s) are credited and that the original publication in this journal is cited, in accordance with accepted academic practice. No use, distribution or reproduction is permitted which does not comply with these terms.
*Correspondence: Carolina Demarchi Munhoz, Y2RtdW5ob3pAdXNwLmJy
†These authors have contributed equally to this work