- College of Medicine, Alfaisal University, Riyadh, Saudi Arabia
Lung cancer remains a major global health challenge, characterized by aggressive malignancy and poor prognostic outcomes. This review article focuses on the pivotal role of claudins, a family of tight junction proteins, in the pathophysiology of lung cancer. Claudins are integral to maintaining epithelial barrier function and cellular polarity, yet they are intricately involved in the progression and metastasis of lung cancer. The aberrant expression of claudins has been observed across various histological subtypes of lung cancer, indicating their potential as diagnostic and prognostic biomarkers. Specifically, claudins such as claudin-1, -2, -3, -4, and -7 exhibit diverse expression patterns that correlate with tumor aggressiveness, patient survival rates, and response to therapies. Inflammation and cytokine modulation significantly influence claudin expression, affecting tumor microenvironment dynamics and cancer progression. This review also highlights the therapeutic implications of targeting claudins, particularly in cases resistant to conventional treatments. Recent advances in this area suggest that claudin-modulating agents may enhance the efficacy of existing therapies and offer new avenues for targeted interventions. By integrating the latest research, this article aims to provide a comprehensive understanding of claudin’s roles in lung cancer and encourages further clinical trials to explore claudin-targeting therapies. This could pave the way for more effective management strategies, improving outcomes for lung cancer patients.
1 Introduction
Lung cancer is the leading cause of cancer incidence and deaths globally (1, 2). Recent trends indicate a decreasing incidence of lung cancer in many nations, attributed to a decline in smoking habits (3). The five-year relative survival rates for small cell lung carcinoma (SCLC) and non-SCLC (NSCLC) ranges from 3%–30% and 9%–65%, respectively, depending on the tumor’s stage (4). However, advances into lung cancer molecular biology and immune-based treatments are expected to improve these statistics in the future (5–7).
To understand the complex nature of lung pathologies, it is essential to explore its biological underpinnings, starting at the cellular level where the disease initiates and progresses (8). The lung’s barrier function, crucial for the free diffusion of solutes into airspaces, is facilitated by epithelial cells and tight junctions, comprising key proteins such as occludin, zona-occluden, desmosomes, and claudins (9–11). These structures not only regulate the permeability of the epithelial barrier but also control the passage through the paracellular space (12). Tight junctions are not only vital for barrier integrity, but also facilitate cellular proliferation and differentiation and maintain cellular polarity, an essential factor in cellular communication and signaling (13–17).
Claudins, a family of tetraspan transmembrane proteins, are integral to this barrier function for tight junction (18, 19). All claudins share a similar secondary structure, with significant variation observed in their extracellular domains and cytoplasmic scaffolding interactions, particularly among non-classic claudins (20). The differential expression of claudins across various histological types of lung tumors could be partially attributed to the originating cell type of the tumor (21). Claudins-1, -3, -4, -5, and -7 are predominantly expressed, with each showing distinct patterns of localization and function (22–24). For instance, claudin-18 is primarily found in alveolar epithelium while claudins-4 and -7 are more ubiquitously expressed throughout the respiratory epithelium, highlighting the diversity of claudin expression in different lung regions (23, 25, 26).
In lung cancer, claudins may play an essential role as disease markers, with their expression levels inversely correlating with tumor aggressiveness and patient prognosis (27). Claudins -1, -2, -3, -4, and -7 exhibit diverse expression patterns in lung carcinoma, with variations among different histological types compared to normal lung tissue and even being influenced by smoking habits (28–31). Emerging research also indicates a link between claudin expression and lung cancer metastasis, suggesting their involvement in tumor migration and invasion (32–34). Also, claudin expression has been linked to epithelial-mesenchymal transition in lung squamous cell carcinoma (LSCC) cells through the Tyk2/Stat1 (35) or Wnt/β-catenin signaling pathways (36).
Building upon previous comprehensive reviews which illuminated the role of claudins in head and neck (37), gastrointestinal (38), and genitourinary cancers (39), this paper extends the investigation into the realm of lung cancer. Exploring the intricate mechanisms behind the aberrant expression of claudins in lung cancer is essential, as it may lay the groundwork for identifying novel therapeutic targets in upcoming clinical trials. This paper aims to deliver a comprehensive review of the claudin expression patterns observed in lung cancer and their potential applications in monitoring and managing the disease. We will also delve into the molecular mechanisms leading to irregular claudin expression and discuss strategies for targeting these aberrations in treating lung cancer.
2 Claudins in non-small cell lung cancers
NSCLCs, which include lung adenocarcinomas (LUAD), LSCC, and large cell carcinomas, constitute nearly 85% of all histological lung cancer types (40). NSCLCs are more strongly linked to smoking, which is attributed to three-quarters of all lung cancers globally, than SCLCs (41). Despite advancements in NSCLC management, the unclear mechanisms of disease progression and need for a larger portfolio of targeted therapies leave a lot to be achieved in the future (40). Claudins have been studied extensively in NSCLCs to fill in the gap and guide novel prognostic and therapeutic strategies. In this section, we summarize the evidence describing the expression of claudins in NSCLCs, their disease-modifying effects, and potential prognostic implications (Table 1).

Table 1. Changes in the levels of expression of different claudins in lung cancers compared to normal tissue.
2.1 Claudins in lung adenocarcinomas
LUADs are the most common type of lung cancers globally, and the incidence continues to climb (42). Emerging evidence has demonstrated that aberrant expression of the claudin family mediates LUAD progression (Figure 1). LUADs have reduced claudin-1 messenger ribonucleic acid (mRNA) expression and an absence of the protein (43). When present, claudin-1 can be primarily seen along the tight junctions of LUAD cells, accompanying zona occludins-1 (44). The protein suppresses LUAD cell migration, invasion, and metastasis (45). Claudin-1 also modulates the genetic profile of LUAD cancer cells by promoting the expression of metastasis suppressors and blunting that of metastasis promotors (45). Some studies have revealed that low expression of the protein is independently associated with poor overall survival in these patients (45, 46), while others have been unable to demonstrate an association (47, 48). On the contrary, Sun et al. found that claudin-1 overexpression is associated with poor survival (49). The reasons for the conflicting data are unclear; nevertheless, claudin-1 appears to play important mechanistic and prognostic roles in LUAD patients.
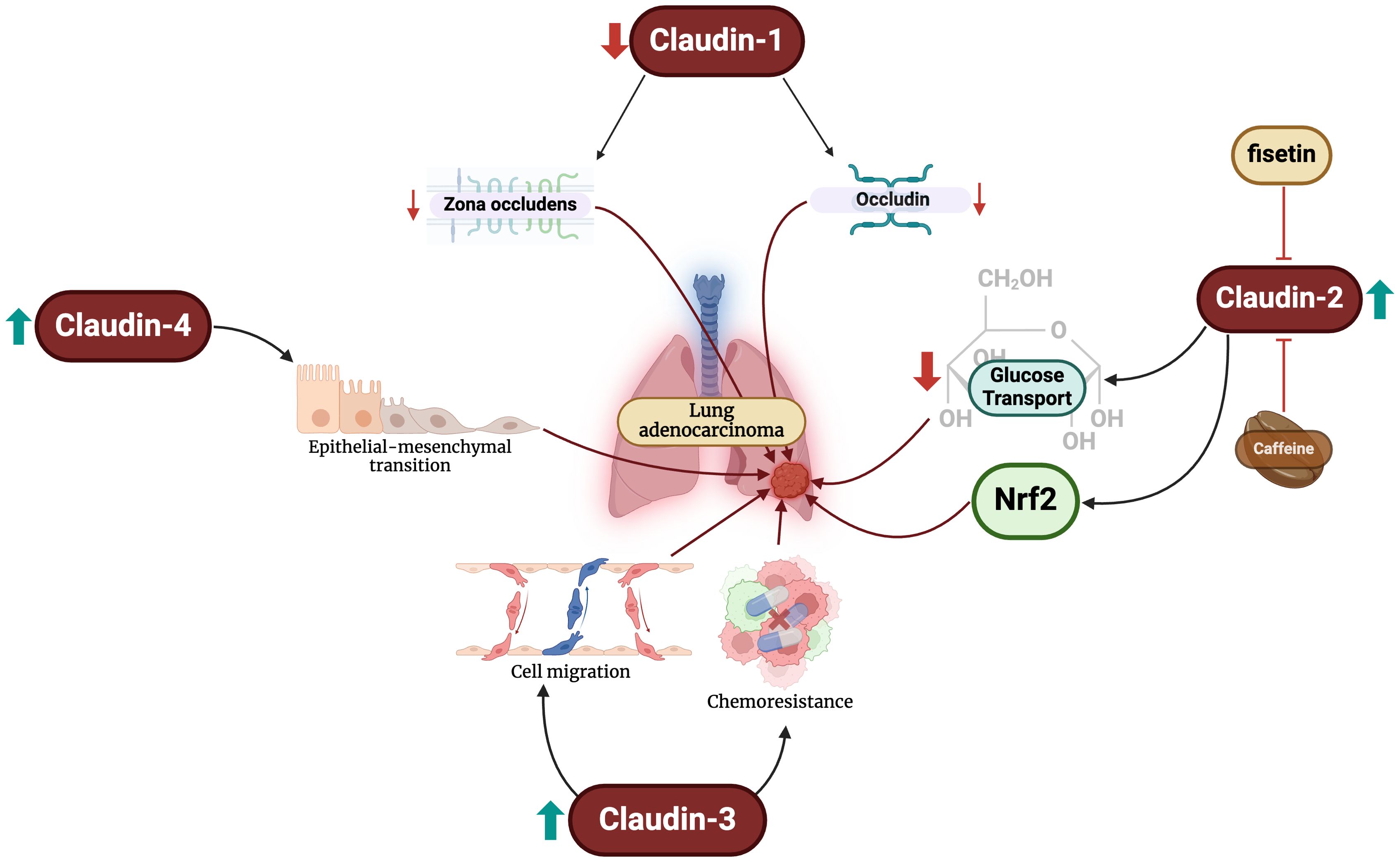
Figure 1. Changes in claudin levels promote the development of LUAD through different mechanisms. Firstly, reductions in claudin-1 levels lead to decreased expression of zona-occludens and occludin. Increased claudin-2 levels blunt glucose transport and activates Nrf2. Furthermore, claudin-3 overexpression promotes malignant cell migration and chemoresistance. Finally, claudin-4 exacerbates epithelial-mesenchymal transition, an important step in oncogenesis.
Claudin-2 has also been extensively study in LUAD. Claudin-2 is highly expressed in LUAD compared to normal lung cells (32, 50, 51). Additionally, claudin-2 protein expression in LUAD is significantly greater than that of SCLCs, elucidating its potential diagnostic value (52). Arai et al. demonstrated that claudin-2 promotes LUAD cell proliferation (50). Claudin-2 promotes chemoresistance to doxorubicin of LUAD cells by inhibiting glucose transport and activating the nuclear factor erythroid 2-related factor 2 (Nrf2) signaling pathways (53). Targeting these pathways, caffeine administration has been shown to enhance lysosomal degradation of claudin-2 and, subsequently, reducing Nrf2 activity and enhancing chemosensitivity (54). Similar effects are also exhibited by fisetin – a flavonoid – and kaempferide through Akt pathway inhibition (55, 56).
Claudin-3 is exhibited in normal bronchial cells in a circular manner at the cellular membrane, but not in pneumocytes (48). The diagnostic utility of claudin-3 appears to be limited in LUADs. Moldvay et al. found increased levels of protein expression in LUADs compared to LSCC (52), while Jung et al. found no difference between the two entities (48). Claudin-3 expression has been linked to poor survival and increased recurrence and/or metastasis in LUAD patients, but not tumor stage or size (57). Forced overexpression of claudin-3 enhances LUAD cell migration, proliferation, and chemoresistance, and its knockout blunts these effects (57).
Claudin-4 is focally expressed in normal pneumocytes and more strongly expressed in the cylindrical cells of the bronchial mucosa (48). Claudin-4 expression is significantly upregulated in LUADs compared to normal and premalignant samples (52, 58, 59) and significantly greater than that of LSCCs (48, 52). Claudin-4 can also be used to differentiate between LUAD and malignant pleural mesotheliomas (60), and specifically epithelioid mesothelioma with a sensitivity and specificity of 97% (61). However, the protein is not associated with any staging or survival parameters in LUAD patients (48). To our knowledge, there have been no studies examining the mechanistic role of claudin-4 in LUAD development. However, changes in claudin-4 expression have been shown to promote epithelial-mesenchymal transition, which is a crucial predisposing factor to the neoplastic process (62). It is crucial for future studies to elucidate the role of claudin-4 in LUADs.
Claudin-7 proteins are strongly expressed in normal bronchial cells (52). In LUADs, mRNA expression does not differ from that in normal tissues (52), but protein levels are significantly reduced (63). Similarly, mRNA levels in LUADs are similar to LSCCs; however, immunohistochemistry has shown elevated levels in LUAD samples (52).
2.2 Claudins in lung squamous cell carcinomas
Following LUAC, LSCCs constitute the second most diagnosed form of NSCLCs (64), resulting in nearly a third of lung cancer in men and a fifth of lung cancer in women (65). Besides their utility in differentiating between LUAC and LSCC, as discussed previously (48, 52), alterations in LSCC compared to normal tissue and prognostic implications have also been reported. Overexpression of claudin-1 mRNA is found in up to 80% of LSCC samples (66, 67). Further solidifying its role, forcibly silencing claudin-1 expression blunts LSCC cell line proliferation and invasiveness (67). However, there has been no association found between its expression and the prognosis of LSCC patients (47).
Reduced claudin-3 levels have been linked to poor overall survival in LSCC patients, due to its promotion of epithelial-mesenchymal transition (68). Che et al. also demonstrated that claudin-7 is downregulated in LSCCs, and its downregulation is linked with poor differentiation and lymphatic metastasis (63). Blunted levels of claudins -5, -7, and -18 promote proliferation of LSCC cells due to phosphorylation and nuclear translocation of Akt and subsequent activation of the G1/S transition in the cell life cycle (69). Finally, claudin-12 has been shown to drive epithelial-mesenchymal transition through activation of the Tyk2/Stat1 signaling (70). Conclusively, claudins are drivers of LSCC pathogenesis; however, further studies are needed to elaborate on their mechanistic roles and prognostic potential.
3 Claudins in small cell lung carcinoma
SCLC is a highly aggressive form of lung cancer, responsible for a significant number of cancer-related deaths worldwide (71, 72). The malignancy of SCLC is characterized by its aggressive biological features, especially its high propensity for metastasis, contributing to its poor prognosis (73, 74).
In the context of claudins, the expression of these proteins in SCLC reveals critical insights into its pathology. Molday et al. observed SCLCs showing strong immunopositivity with claudins-3, -4, and -7, while being less strong for claudin-1 and being entirely negative for claudin-2 (52). However, Sormunen et al. displayed that SCLC exhibit a higher expression of claudin-2 than adenocarcinomas (21), contradicting with Molday et al. (52). Additionally, claudins-3 and -4 mRNA expression in SCLC are found to be 16 times and 3–4 times, respectively, higher than in normal lung tissue (52). Furthermore, in comparison to carcinoid tissue, claudin-3 and -4 mRNA expression in SCLC was found to be 13 times and 3 times higher, respectively (52). Nonetheless, LSCC has 15 times higher claudin-3 mRNA expression compared to SCLC (52). These findings highlight the distinct claudin expression patterns in SCLC, suggesting their potential role in the disease’s aggressive nature.
Expanding upon these observations, further research into claudin expression in SCLC may provide valuable perspectives on key aspects of the disease’s progression and intervention. For instance, Mao et al. identified exosomal miR-375-3p to break the vascular barrier by inhibiting claudin-1, promoting SCLC metastasis (33). In addition, Spi-B–mediated silencing of claudin-2 promotes early dissemination of both SCLC and NSCLC (75). Recently, the identification of differentially expressed genes in SCLC showed claudin-18, among others, significantly correlated to immune infiltration in the tumor microenvironment (76). Moreover, a study identified junctional adhesion molecule 3 as a potential therapeutic target, further reinforcing the importance of exploring these molecules in the development of new treatments for SCLC (77). These insights pave the way for future research, emphasizing the need for a deeper understanding of molecular mechanisms in SCLC to enhance therapeutic strategies and improve patient outcomes.
4 Claudins in pleural mesothelioma
Malignant pleural mesothelioma (MPM) affects 2,000 to 3,000 people annually in the United States and is rising worldwide, with over 5,000 new cases yearly in Western Europe and a peak incidence expected in Japan by 2025 (78–80). MPM, which is more common in men (5:1 male-to-female ratio) and increases with age, is primarily related to occupational asbestos exposure, though familial cases and other factors, such as previous radiation and simian virus-40, have also been identified (78, 81–85). Despite its histologic diversity (epithelioid, biphasic, and sarcomatoid), there are no approved early detection methods, but serum mesothelin-related peptide and osteopontin show promise in diagnosis (86, 87). To date, treatment remains challenging due to the disease’s complexity and limited patient numbers for studies, resulting in an alarmingly low median survival of 9 to 17 months (78). However, recent developments suggest potential advancements in MPM treatment (88, 89).
Studies have shown distinct expression patterns of various claudin subtypes in mesothelioma tissues, with these patterns often being linked to the specific type, severity, and expected outcome of the tumor. In comparison to normal mesothelial tissues, mesothelioma tissues frequently display different claudin expression profiles, hinting at their possible involvement in the development or progression of the cancer. In their study, Soini et al. observed that 40%, 80%, 18%, 23%, 14%, and 43% of mesothelioma cases expressed claudins -1, -2, -3, -4, -5, and -7, respectively (90). Notably, the presence of claudins -1, -3, -4, -5, and -7 was markedly lower in mesothelioma compared to metastatic adenocarcinoma, except for claudin 2, which showed no significant difference. Furthermore, an inverse relationship was found between the presence of claudins -1, -3, -4, -5, and -7 and calretinin positivity. In terms of mesothelioma subtypes, sarcomatoid and biphasic forms showed less positivity for these claudins compared to the purely epithelioid form. However, the study found no link between claudin expression and the survival rates of patients with malignant mesotheliomas (90). Interestingly, Nakashima et al. (91) found that claudin-5 was not expressed in mesothelioma, presenting a stark contrast to the findings of Soini et al. (90), who reported claudin-5 expression in 14% of mesothelioma cases. This contradiction between the studies could potentially be attributed to a bias arising from differences in sample sizes. Such variations in sample sizes can influence the detection and reporting of low-frequency markers like claudin-5 in mesothelioma, leading to differing conclusions in otherwise similar studies. Stefon et al. have reported that claudin-15 may be valuable in subtyping mesotheliomas (92). This finding is particularly significant from a prognostic standpoint, as different subtypes of mesothelioma are known to have varying implications for patient prognosis. The ability to accurately subtype mesotheliomas using markers like claudin-15 could therefore play a crucial role in predicting disease outcomes and tailoring treatment strategies to individual patient needs, enhancing the overall management of this challenging malignancy.
MPM and LUAC often present diagnostic challenges due to their overlapping histological features. Recent studies have highlighted the potential of claudin-4 as a differential marker in this context. The absence of claudin-4 has been found particularly useful in distinguishing between MPM and adenocarcinoma, as almost all carcinoma cases express claudin-4 (93). This contrast in claudin-4 expression between MPM and LUAC has been documented in various research articles (60, 94–98). These findings suggest the inclusion of claudin-4 level assessment could enhance the diagnostic accuracy when distinguishing between MPM and LUACs.
5 Therapeutic implications of claudins in lung cancer
The therapeutic potential of claudins in lung cancer has garnered increasing interest, particularly as the field moves towards more targeted approaches. Recent advancements have incorporated Clostridium perfringens enterotoxin (CPE), particularly due to its ability to bind to specific claudins overexpressed in cancer cells. Claudins such as claudins-3 and -4 serve as receptors for CPE, making it an ideal candidate for targetting claudins. This has led to the development of modified CPE variants, such as CPE-Mut3, which show enhanced binding to a broader range of claudins including CLDN-1 and CLDN-5. These modifications improve the targeting and cytotoxicity against claudin-overexpressing tumors, which is particularly beneficial for NSCLC patients where these claudins are prevalent (99). Furthermore, studies have shown that CPE-mediated therapies can disrupt tight junctions in cancer cells, leading to their destruction and offering a potential pathway for novel cancer treatments (100). Additionally, combining CPE with other therapeutic modalities, such as gold-nanoparticle-mediated laser intervention, has demonstrated significant reduction in tumor cell viability, further enhancing the therapeutic landscape (101). However, the success of these therapies depends on the accessibility of claudins on the cancer cell surface and the specific conditions under which CPE can exert its cytotoxic effects (102).
Beyond CPE, other therapeutic strategies targeting claudins have also shown promise. A groundbreaking study on CLDN6-specific CAR-T cells combined with an amplifying RNA vaccine has demonstrated promising results in patients with relapsed or refractory solid tumors, of which half had lung involvement. This approach leverages the specific expression of CLDN6 in tumors while avoiding on-target/off-tumor toxicity, a major challenge in CAR-T therapies for solid tumors. The ongoing Phase 1 BNT211-01 trial has reported a disease control rate of 67% and an objective response rate of 33%, with manageable safety profiles (103). This innovative therapy could open the door for new therapeutic opportunities; however, this would need to be tested in primary lung neoplasms.
In addition, histone deacetylase inhibitors like tricostatin A and quisinostat have been demonstrated to suppress claudin-2 expression, resulting in reduced tumor cell proliferation and migration, which could represent a potential therapeutic avenue for lung adenocarcinomas (104). Claudin-18, particularly its splice variant 2, has been identified as a viable target for therapeutic antibodies, although clinical trials have yet to show efficacy in its lung-specific form (105). Moreover, claudin-7 has been linked to increased cisplatin sensitivity by promoting pro-apoptotic pathways (106). However, the role of claudin-1 in drug resistance remains complex, with conflicting evidence regarding its impact on chemosensitivity (107, 108).
Conclusively, while claudin-targeting therapies, particularly those involving CPE, hold significant potential, ongoing research and clinical trials are essential to refine these approaches and fully realize their potential in lung cancer treatment.
6 Conclusion
In summary, our review has elucidated the complex role of claudins in the pathophysiology of lung cancer. These tight junction proteins are not only pivotal in maintaining cell polarity and barrier integrity but also significantly influence lung cancer progression and metastasis. The differential expression of claudins among various lung cancer subtypes offers insights into their potential as biomarkers for diagnosis and prognosis. Notably, the overexpression of certain claudins like claudin-2 and claudin-4 has been linked with increased malignancy and poor patient outcomes, while others such as claudin-1 show variable associations depending on the cancer subtype. Furthermore, the interaction of claudins with inflammatory pathways and their modulation by cytokines highlights their role in cancer microenvironment dynamics. The therapeutic potential of targeting specific claudins, supported by emerging studies, suggests a promising avenue for developing personalized treatment strategies, particularly in resistant cases. As research continues to unravel the multifaceted functions of claudins, it is imperative that future clinical trials are designed to explore and validate claudin-targeting therapies in lung cancer management.
Author contributions
TA: Conceptualization, Writing – original draft, Writing – review & editing. WA: Writing – original draft. NO: Writing – original draft. BS: Writing – original draft. NA: Writing – original draft. AO: Conceptualization, Supervision, Writing – review & editing.
Funding
The author(s) declare that no financial support was received for the research, authorship, and/or publication of this article.
Acknowledgments
The figure in this manuscript was made using BioRender.
Conflict of interest
The authors declare that the research was conducted in the absence of any commercial or financial relationships that could be construed as a potential conflict of interest.
Publisher’s note
All claims expressed in this article are solely those of the authors and do not necessarily represent those of their affiliated organizations, or those of the publisher, the editors and the reviewers. Any product that may be evaluated in this article, or claim that may be made by its manufacturer, is not guaranteed or endorsed by the publisher.
References
1. Miller RA, Cagle PT. Lung cancer epidemiology and demographics. In: Cagle PT, Allen TC, Beasley MB, Chirieac LR, Dacic S, Borczuk AC, et al, editors. Precision molecular pathology of lung cancer. Springer International Publishing, Cham (2018). p. 15–7.
2. Thandra KC, Barsouk A, Saginala K, Aluru JS, Barsouk A. Epidemiology of lung cancer. Contemp Oncol (Pozn). (2021) 25:45–52. doi: 10.5114/wo.2021.103829
3. Cornelius ME, Loretan CG, Jamal A, Davis Lynn BC, Mayer M, Alcantara IC, et al. Tobacco product use among adults - United States, 2021. MMWR Morb Mortal Wkly Rep. (2023) 72:475–83. doi: 10.15585/mmwr.mm7218a1
4. Surveillance Research Program NCI. Seer*Explorer: an interactive website for seer cancer statistics (2023). Available online at: https://seer.cancer.gov/statistics-network/explorer/ (Accessed February 15, 2024).
5. Shivani G, Ishani G, Reetika M, Raman K. Perspective chapter: molecular pathology of lung cancer. In: Adem K, Volkan G, Hülya K, editors. Molecular histopathology and cytopathology. IntechOpen, Rijeka (2023). p. Ch. 8.
6. Hayashi R, Inomata M. Small cell lung cancer; recent advances of its biology and therapeutic perspective. Respir Invest. (2022) 60:197–204. doi: 10.1016/j.resinv.2021.10.008
7. Saab S, Zalzale H, Rahal Z, Khalifeh Y, Sinjab A, Kadara H. Insights into lung cancer immune-based biology, prevention, and treatment. Front Immunol. (2020) 11:159. doi: 10.3389/fimmu.2020.00159
8. Arafah MA, Raddaoui E, Kassimi FA, Alhamad EH, Alboukai AA, Alshedoukhy AA, et al. Endobronchial biopsy in the final diagnosis of chronic obstructive pulmonary disease and asthma: A clinicopathological study. Ann Saudi Med. (2018) 38:118–24. doi: 10.5144/0256-4947.2018.118
9. Marchiando AM, Graham WV, Turner JR. Epithelial barriers in homeostasis and disease. Annu Rev Pathology: Mech Dis. (2010) 5:119–44. doi: 10.1146/annurev.pathol.4.110807.092135
10. Koval M. Claudin heterogeneity and control of lung tight junctions. Annu Rev Physiol. (2013) 75:551–67. doi: 10.1146/annurev-physiol-030212-183809
11. Shin K, Fogg VC, Margolis B. Tight junctions and cell polarity. Annu Rev Cell Dev Biol. (2006) 22:207–35. doi: 10.1146/annurev.cellbio.22.010305.104219
12. Diamond JM. Tight and leaky junctions of epithelia: A perspective on kisses in the dark. Fed Proc. (1974) 33:2220–4.
13. Zihni C, Balda MS, Matter K. Signalling at Tight Junctions during Epithelial Differentiation and Microbial Pathogenesis. J Cell Sci. (2014) 127:3401–13. doi: 10.1242/jcs.145029
14. Farkas AE, Capaldo CT, Nusrat A. Regulation of epithelial proliferation by tight junction proteins. Ann N Y Acad Sci. (2012) 1258:115–24. doi: 10.1111/j.1749-6632.2012.06556.x
15. Balda MS, Matter K. Tight junctions in health and disease. Semin Cell Dev Biol. (2014) 36:147–8. doi: 10.1016/j.semcdb.2014.11.001
16. Singh AB, Sharma A, Smith JJ, Krishnan M, Chen X, Eschrich S, et al. Claudin-1 up-regulates the repressor zeb-1 to inhibit E-cadherin expression in colon cancer cells. Gastroenterology. (2011) 141:2140–53. doi: 10.1053/j.gastro.2011.08.038
17. Singh A, Mishra AK, Ylaya K, Hewitt SM, Sharma KC, Saxena S. Wilms tumor-1, claudin-1 and ezrin are useful immunohistochemical markers that help to distinguish schwannoma from fibroblastic meningioma. Pathol Oncol Res. (2012) 18:383–9. doi: 10.1007/s12253-011-9456-x
18. Günzel D, Fromm M. Claudins and other tight junction proteins. Compr Physiol. (2012) 2:1819–52. doi: 10.1002/cphy.c110045
19. Tsukita S, Furuse M. Claudin-based barrier in simple and stratified cellular sheets. Curr Opin Cell Biol. (2002) 14:531–6. doi: 10.1016/s0955-0674(02)00362-9
20. Krause G, Winkler L, Mueller SL, Haseloff RF, Piontek J, Blasig IE. Structure and function of claudins. Biochim Biophys Acta (BBA) - Biomembranes. (2008) 1778:631–45. doi: 10.1016/j.bbamem.2007.10.018
21. Sormunen R, Pääkkö P, Kaarteenaho-Wiik R, Soini Y. Differential expression of adhesion molecules in lung tumours. Histopathology. (2007) 50:282–4. doi: 10.1111/j.1365-2559.2007.02574.x
23. Kaarteenaho R, Merikallio H, Lehtonen S, Harju T, Soini Y. Divergent expression of claudin -1, -3, -4, -5 and -7 in developing human lung. Respir Res. (2010) 11:59. doi: 10.1186/1465-9921-11-59
24. Lappi-Blanco E, Lehtonen ST, Sormunen R, Merikallio HM, Soini Y, Kaarteenaho RL. Divergence of tight and adherens junction factors in alveolar epithelium in pulmonary fibrosis. Hum Pathol. (2013) 44:895–907. doi: 10.1016/j.humpath.2012.08.016
25. Kaarteenaho-Wiik R, Soini Y. Claudin-1, -2, -3, -4, -5, and -7 in usual interstitial pneumonia and sarcoidosis. J Histochem Cytochem. (2009) 57:187–95. doi: 10.1369/jhc.2008.951566
26. Niimi T, Nagashima K, Ward JM, Minoo P, Zimonjic DB, Popescu NC, et al. Claudin-18, a novel downstream target gene for the T/ebp/nkx2.1 homeodomain transcription factor, encodes lung- and stomach-specific isoforms through alternative splicing. Mol Cell Biol. (2001) 21:7380–90. doi: 10.1128/mcb.21.21.7380-7390.2001
27. McDonagh D, Vollmer RT, Shelburne JD. Intercellular junctions and tumor behavior in lung cancer. Mod Pathol. (1991) 4:436–40.
28. Merikallio H, Kaarteenaho R, Pääkkö P, Lehtonen S, Hirvikoski P, Mäkitaro R, et al. Impact of smoking on the expression of claudins in lung carcinoma. Eur J Cancer. (2011) 47:620–30. doi: 10.1016/j.ejca.2010.10.017
29. Wang DW, Zhang WH, Danil G, Yang K, Hu JK. The role and mechanism of claudins in cancer. Front Oncol. (2022) 12:1051497. doi: 10.3389/fonc.2022.1051497
30. Li J. Dysregulated expression of claudins in cancer. Oncol Lett. (2021) 22:641. doi: 10.3892/ol.2021.12902
31. Li J. Targeting claudins in cancer: diagnosis, prognosis and therapy. Am J Cancer Res. (2021) 11:3406–24.
32. Ikari A, Sato T, Takiguchi A, Atomi K, Yamazaki Y, Sugatani J. Claudin-2 knockdown decreases matrix metalloproteinase-9 activity and cell migration via suppression of nuclear sp1 in A549 cells. Life Sci. (2011) 88:628–33. doi: 10.1016/j.lfs.2011.02.002
33. Mao S, Zheng S, Lu Z, Wang X, Wang Y, Zhang G, et al. Exosomal mir-375-3p breaks vascular barrier and promotes small cell lung cancer metastasis by targeting claudin-1. Transl Lung Cancer Res. (2021) 10:3155–72. doi: 10.21037/tlcr-21-356
34. Ma SC, Li Q, Peng JY, Zhouwen JL, Diao JF, Niu JX, et al. Claudin-5 regulates blood-brain barrier permeability by modifying brain microvascular endothelial cell proliferation, migration, and adhesion to prevent lung cancer metastasis. CNS Neurosci Ther. (2017) 23:947–60. doi: 10.1111/cns.12764
35. Sun L, Feng L, Cui J. Increased expression of claudin−12 promotes the metastatic phenotype of human bronchial epithelial cells and is associated with poor prognosis in lung squamous cell carcinoma. Exp Ther Med. (2018) 17:165–74. doi: 10.3892/etm.2018.6964
36. Che J, Yue D, Zhang B, Zhang H, Huo Y, Gao L, et al. Claudin-3 inhibits lung squamous cell carcinoma cell epithelial-mesenchymal transition and invasion via suppression of the wnt/β-catenin signaling pathway. Int J Med Sci. (2018) 15:339–51. doi: 10.7150/ijms.22927
37. Arabi TZ, Algheryafi LA, Alodah NA, Enabi HMK, Alshehry AA, Ouban A. Aberrant expression of claudins in head and neck carcinomas and their prognostic and therapeutic value: A narrative review. Cancers. (2023) 15:4208. doi: 10.3390/cancers15174208
38. Ouban A, Arabi TZ. Expression of claudins in preneoplastic conditions of the gastrointestinal tract: A review. Cancers. (2023) 15:4095. doi: 10.3390/cancers15164095
39. Arabi TZ, Fawzy NA, Sabbah BN, Ouban A. Claudins in genitourinary tract neoplasms: mechanisms, prognosis, and therapeutic prospects. Front Cell Dev Biol. (2023) 11:1308082. doi: 10.3389/fcell.2023.1308082
40. Herbst RS, Morgensztern D, Boshoff C. The biology and management of non-small cell lung cancer. Nature. (2018) 553:446–54. doi: 10.1038/nature25183
41. Sun S, Schiller JH, Gazdar AF. Lung cancer in never smokers–a different disease. Nat Rev Cancer. (2007) 7:778–90. doi: 10.1038/nrc2190
42. Succony L, Rassl DM, Barker AP, McCaughan FM, Rintoul RC. Adenocarcinoma spectrum lesions of the lung: detection, pathology and treatment strategies. Cancer Treat Rev. (2021) 99:102237. doi: 10.1016/j.ctrv.2021.102237
43. Paschoud S, Bongiovanni M, Pache J-C, Citi S. Claudin-1 and claudin-5 expression patterns differentiate lung squamous cell carcinomas from adenocarcinomas. Modern Pathol. (2007) 20:947–54. doi: 10.1038/modpathol.3800835
44. Akizuki R, Maruhashi R, Eguchi H, Kitabatake K, Tsukimoto M, Furuta T, et al. Decrease in paracellular permeability and chemosensitivity to doxorubicin by claudin-1 in spheroid culture models of human lung adenocarcinoma A549 cells. Biochim Biophys Acta Mol Cell Res. (2018) 1865:769–80. doi: 10.1016/j.bbamcr.2018.03.001
45. Chao Y-C, Pan S-H, Yang S-C, Yu S-L, Che T-F, Lin C-W, et al. Claudin-1 is a metastasis suppressor and correlates with clinical outcome in lung adenocarcinoma. Am J Respir Crit Care Med. (2009) 179:123–33. doi: 10.1164/rccm.200803-456OC
46. Zhang Z, Wang A, Sun B, Zhan Z, Chen K, Wang C. Expression of cldn1 and cldn10 in lung adenocarcinoma in situ and invasive lepidic predominant adenocarcinoma. J Cardiothorac Surg. (2013) 8:95. doi: 10.1186/1749-8090-8-95
47. Moldvay J, Fábián K, Jäckel M, Németh Z, Bogos K, Furák J, et al. Claudin-1 protein expression is a good prognostic factor in non-small cell lung cancer, but only in squamous cell carcinoma cases. Pathol Oncol Res. (2017) 23:151–6. doi: 10.1007/s12253-016-0115-0
48. Jung JH, Jung CK, Choi HJ, Jun KH, Yoo J, Kang SJ, et al. Diagnostic utility of expression of claudins in non-small cell lung cancer: different expression profiles in squamous cell carcinomas and adenocarcinomas. Pathol Res Pract. (2009) 205:409–16. doi: 10.1016/j.prp.2008.12.015
49. Sun BS, Yao YQ, Pei BX, Zhang ZF, Wang CL. Claudin-1 correlates with poor prognosis in lung adenocarcinoma. Thorac Cancer. (2016) 7:556–63. doi: 10.1111/1759-7714.12368
50. Arai W, Konno T, Kohno T, Kodera Y, Tsujiwaki M, Shindo Y, et al. Downregulation of angulin-1/lsr induces Malignancy via upregulation of egf-dependent claudin-2 and tgf-β-dependent cell metabolism in human lung adenocarcinoma A549 cells. Oncotarget. (2023) 14:261–75. doi: 10.18632/oncotarget.27728
51. Ikari A, Sato T, Watanabe R, Yamazaki Y, Sugatani J. Increase in claudin-2 expression by an egfr/mek/erk/C-fos pathway in lung adenocarcinoma A549 cells. Biochim Biophys Acta. (2012) 1823:1110–8. doi: 10.1016/j.bbamcr.2012.04.005
52. Moldvay J, Jäckel M, Páska C, Soltész I, Schaff Z, Kiss A. Distinct claudin expression profile in histologic subtypes of lung cancer. Lung Cancer. (2007) 57:159–67. doi: 10.1016/j.lungcan.2007.02.018
53. Ito A, Nasako H, Akizuki R, Takashina Y, Eguchi H, Matsunaga T, et al. Elevation of chemosensitivity of lung adenocarcinoma A549 spheroid cells by claudin-2 knockdown through activation of glucose transport and inhibition of nrf2 signal. Int J Mol Sci. (2021) 22:6582. doi: 10.3390/ijms22126582
54. Eguchi H, Kimura R, Onuma S, Ito A, Yu Y, Yoshino Y, et al. Elevation of anticancer drug toxicity by caffeine in spheroid model of human lung adenocarcinoma A549 cells mediated by reduction in claudin-2 and nrf2 expression. Int J Mol Sci. (2022) 23:15447. doi: 10.3390/ijms232415447
55. Eguchi H, Kimura R, Matsunaga H, Matsunaga T, Yoshino Y, Endo S, et al. Increase in anticancer drug-induced toxicity by fisetin in lung adenocarcinoma A549 spheroid cells mediated by the reduction of claudin-2 expression. Int J Mol Sci. (2022) 23:7536. doi: 10.3390/ijms23147536
56. Eguchi H, Matsunaga T, Endo S, Ichihara K, Ikari A. Kaempferide enhances chemosensitivity of human lung adenocarcinoma A549 cells mediated by the decrease in phosphorylation of akt and claudin-2 expression. Nutrients. (2020) 12:1190. doi: 10.3390/nu12041190
57. Zhang L, Wang Y, Zhang B, Zhang H, Zhou M, Wei M, et al. Claudin-3 expression increases the Malignant potential of lung adenocarcinoma cells: role of epidermal growth factor receptor activation. Oncotarget. (2017) 8:23033–47. doi: 10.18632/oncotarget.14974
58. Yamada G, Murata M, Takasawa A, Nojima M, Mori Y, Sawada N, et al. Increased expressions of claudin 4 and 7 in atypical adenomatous hyperplasia and adenocarcinoma of the lung. Med Mol Morphol. (2016) 49:163–9. doi: 10.1007/s00795-016-0135-6
59. Hanada S, Maeshima A, Matsuno Y, Ohta T, Ohki M, Yoshida T, et al. Expression profile of early lung adenocarcinoma: identification of <I>Mrp3</I> as a molecular marker for early progression. J Pathol. (2008) 216:75–82. doi: 10.1002/path.2383
60. Chaouche-Mazouni S, Scherpereel A, Zaamoum R, Mihalache A, Amir Z-C, Lebaïli N, et al. Claudin 3, 4, and 15 expression in solid tumors of lung adenocarcinoma versus Malignant pleural mesothelioma. Ann Diagn Pathol. (2015) 19:193–7. doi: 10.1016/j.anndiagpath.2015.03.007
61. Kai Y, Amatya VJ, Kushitani K, Kambara T, Suzuki R, Tsutani Y, et al. Mucin 21 is a novel, negative immunohistochemical marker for epithelioid mesothelioma for its differentiation from lung adenocarcinoma. Histopathology. (2019) 74:545–54. doi: 10.1111/his.13775
62. Fujiwara-Tani R, Mori S, Ogata R, Sasaki R, Ikemoto A, Kishi S, et al. Claudin-4: A new molecular target for epithelial cancer therapy. Int J Mol Sci. (2023) 24:5494. doi: 10.3390/ijms24065494
63. Li R, Zhang D, Cai C, Dong J. the clinical significance of claudin-7 and slug expression in lung squamous cell carcinoma and adenocarcinoma. Zhongguo Fei Ai Za Zhi. (2011) 14:492–6. doi: 10.3779/j.issn.1009-3419.2011.06.03
64. Chen JW, Dhahbi J. Lung adenocarcinoma and lung squamous cell carcinoma cancer classification, biomarker identification, and gene expression analysis using overlapping feature selection methods. Sci Rep. (2021) 11:13323. doi: 10.1038/s41598-021-92725-8
65. Polo V, Pasello G, Frega S, Favaretto A, Koussis H, Conte P, et al. Squamous cell carcinomas of the lung and of the head and neck: new insights on molecular characterization. Oncotarget. (2016) 7:25050–63. doi: 10.18632/oncotarget.7732
66. Liu Y, Sun W, Zhang K, Zheng H, Ma Y, Lin D, et al. Identification of genes differentially expressed in human primary lung squamous cell carcinoma. Lung Cancer. (2007) 56:307–17. doi: 10.1016/j.lungcan.2007.01.016
67. Li G, Wu L, Yu J, Zhai S, Deng H, Wang Q. Identification and validation of three-gene signature in lung squamous cell carcinoma by integrated transcriptome and methylation analysis. J Oncol. (2022) 2022:9688040. doi: 10.1155/2022/9688040
68. Che J, Yang Y, Xiao J, Zhao P, Yan B, Dong S, et al. Decreased expression of claudin-3 is associated with a poor prognosis and emt in completely resected squamous cell lung carcinoma. Tumour Biol. (2015) 36:6559–68. doi: 10.1007/s13277-015-3350-1
69. Akizuki R, Shimobaba S, Matsunaga T, Endo S, Ikari A. Claudin-5, -7, and -18 suppress proliferation mediated by inhibition of phosphorylation of akt in human lung squamous cell carcinoma. Biochim Biophys Acta Mol Cell Res. (2017) 1864:293–302. doi: 10.1016/j.bbamcr.2016.11.018
70. Sun L, Feng L, Cui J. Increased expression of claudin-12 promotes the metastatic phenotype of human bronchial epithelial cells and is associated with poor prognosis in lung squamous cell carcinoma. Exp Ther Med. (2019) 17:165–74. doi: 10.3892/etm.2018.6964
71. Torre LA, Siegel RL, Jemal A. Lung cancer statistics. Adv Exp Med Biol. (2016) 893:1–19. doi: 10.1007/978-3-319-24223-1_1
72. Lewis DR, Check DP, Caporaso NE, Travis WD, Devesa SS. Us lung cancer trends by histologic type. Cancer. (2014) 120:2883–92. doi: 10.1002/cncr.28749
73. Sabari JK, Lok BH, Laird JH, Poirier JT, Rudin CM. Unravelling the biology of sclc: implications for therapy. Nat Rev Clin Oncol. (2017) 14:549–61. doi: 10.1038/nrclinonc.2017.71
74. Rudin CM, Poirier JT. Shining light on novel targets and therapies. Nat Rev Clin Oncol. (2017) 14:75–6. doi: 10.1038/nrclinonc.2016.203
75. Du W, Xu X, Niu Q, Zhang X, Wei Y, Wang Z, et al. Spi-B-mediated silencing of claudin-2 promotes early dissemination of lung cancer cells from primary tumors. Cancer Res. (2017) 77:4809–22. doi: 10.1158/0008-5472.Can-17-0020
76. Cao J, Yu C. Identification of immune infiltration and prognostic biomarkers in small cell lung cancer based on bioinformatic methods from 3 studies. Comb Chem High Throughput Screen. (2023) 26:507–16. doi: 10.2174/1386207325666220408092925
77. Yamaguchi M, Hirai S, Idogawa M, Sumi T, Uchida H, Fujitani N, et al. Junctional adhesion molecule 3 is a potential therapeutic target for small cell lung carcinoma. Exp Cell Res. (2023) 426:113570. doi: 10.1016/j.yexcr.2023.113570
78. Tsao AS, Wistuba I, Roth JA, Kindler HL. Malignant pleural mesothelioma. J Clin Oncol. (2009) 27:2081–90. doi: 10.1200/jco.2008.19.8523
79. Robinson BW, Lake RA. Advances in Malignant mesothelioma. N Engl J Med. (2005) 353:1591–603. doi: 10.1056/NEJMra050152
80. Peto J, Decarli A, La Vecchia C, Levi F, Negri E. The european mesothelioma epidemic. Br J Cancer. (1999) 79:666–72. doi: 10.1038/sj.bjc.6690105
81. Larson T, Melnikova N, Davis SI, Jamison P. Incidence and descriptive epidemiology of mesothelioma in the United States, 1999-2002. Int J Occup Environ Health. (2007) 13:398–403. doi: 10.1179/oeh.2007.13.4.398
82. Carbone M, Kratzke RA, Testa JR. The pathogenesis of mesothelioma. Semin Oncol. (2002) 29:2–17. doi: 10.1053/sonc.2002.30227
83. Dogan AU, Baris YI, Dogan M, Emri S, Steele I, Elmishad AG, et al. Genetic predisposition to fiber carcinogenesis causes a mesothelioma epidemic in Turkey. Cancer Res. (2006) 66:5063–8. doi: 10.1158/0008-5472.Can-05-4642
84. Testa JR, Carbone M, Hirvonen A, Khalili K, Krynska B, Linnainmaa K, et al. A multi-institutional study confirms the presence and expression of simian virus 40 in human Malignant mesotheliomas. Cancer Res. (1998) 58:4505–9.
85. Roushdy-Hammady I, Siegel J, Emri S, Testa JR, Carbone M. Genetic-susceptibility factor and Malignant mesothelioma in the cappadocian region of Turkey. Lancet. (2001) 357:444–5. doi: 10.1016/s0140-6736(00)04013-7
86. Robinson BW, Creaney J, Lake R, Nowak A, Musk AW, de Klerk N, et al. Soluble mesothelin-related protein–a blood test for mesothelioma. Lung Cancer. (2005) 49 Suppl 1:S109–11. doi: 10.1016/j.lungcan.2005.03.020
87. Robinson BW, Creaney J, Lake R, Nowak A, Musk AW, de Klerk N, et al. Mesothelin-family proteins and diagnosis of mesothelioma. Lancet. (2003) 362:1612–6. doi: 10.1016/s0140-6736(03)14794-0
88. Vogelzang NJ, Rusthoven JJ, Symanowski J, Denham C, Kaukel E, Ruffie P, et al. Phase iii study of pemetrexed in combination with cisplatin versus cisplatin alone in patients with Malignant pleural mesothelioma. J Clin Oncol. (2003) 21:2636–44. doi: 10.1200/jco.2003.11.136
89. Rice DC, Stevens CW, Correa AM, Vaporciyan AA, Tsao A, Forster KM, et al. Outcomes after extrapleural pneumonectomy and intensity-modulated radiation therapy for Malignant pleural mesothelioma. Ann Thorac Surg. (2007) 84:1685–92; discussion 92-3. doi: 10.1016/j.athoracsur.2007.04.076
90. Soini Y, Kinnula V, Kahlos K, Pääkkö P. Claudins in differential diagnosis between mesothelioma and metastatic adenocarcinoma of the pleura. J Clin Pathol. (2006) 59:250–4. doi: 10.1136/jcp.2005.028589
91. Nakashima Y, Inamura K, Ninomiya H, Okumura S, Mun M, Kirimura S, et al. Frequent expression of conventional endothelial markers in pleural mesothelioma: usefulness of claudin-5 as well as combined traditional markers to distinguish mesothelioma from angiosarcoma. Lung Cancer. (2020) 148:20–7. doi: 10.1016/j.lungcan.2020.07.029
92. Di Stefano I, Alì G, Poma AM, Bruno R, Proietti A, Niccoli C, et al. New immunohistochemical markers for pleural mesothelioma subtyping. Diagnostics. (2023) 13:2945. doi: 10.3390/diagnostics13182945
93. Ordóñez NG. Value of claudin-4 immunostaining in the diagnosis of mesothelioma. Am J Clin Pathol. (2013) 139:611–9. doi: 10.1309/ajcp0b3yjbxwxjii
94. Jo VY, Cibas ES, Pinkus GS. Claudin-4 immunohistochemistry is highly effective in distinguishing adenocarcinoma from Malignant mesothelioma in effusion cytology. Cancer Cytopathology. (2014) 122:299–306. doi: 10.1002/cncy.21392
95. Naso JR, Churg A. Claudin-4 shows superior specificity for mesothelioma vs non–small-cell lung carcinoma compared with moc-31 and ber-ep4. Hum Pathol. (2020) 100:10–4. doi: 10.1016/j.humpath.2020.04.005
96. Ohta Y, Sasaki Y, Saito M, Kushima M, Takimoto M, Shiokawa A, et al. Claudin-4 as a marker for distinguishing Malignant mesothelioma from lung carcinoma and serous adenocarcinoma. Int J Surg Pathol. (2013) 21:493–501. doi: 10.1177/1066896913491320
97. Bernardi L, Bizzarro T, Pironi F, Szymczuk S, Buda R, Fabbri E, et al. The “Brescia panel” (Claudin-4 and brca-associated protein 1) in the differential diagnosis of mesotheliomas with epithelioid features versus metastatic carcinomas. Cancer Cytopathology. (2021) 129:275–82. doi: 10.1002/cncy.22368
98. Facchetti F, Lonardi S, Gentili F, Bercich L, Falchetti M, Tardanico R, et al. Claudin 4 identifies a wide spectrum of epithelial neoplasms and represents a very useful marker for carcinoma versus mesothelioma diagnosis in pleural and peritoneal biopsies and effusions. Virchows Archiv. (2007) 451:669–80. doi: 10.1007/s00428-007-0448-x
99. Piontek A, Eichner M, Zwanziger D, Beier LS, Protze J, Walther W, et al. Targeting claudin-overexpressing thyroid and lung cancer by modified clostridium perfringens enterotoxin. Mol Oncol. (2020) 14:261–76. doi: 10.1002/1878-0261.12615
100. Ogbu CP, Roy S, Vecchio AJ. Disruption of claudin-made tight junction barriers by clostridium perfringens enterotoxin: insights from structural biology. Cells. (2022) 11:903. doi: 10.3390/cells11050903
101. Alnajjar S, Nolte I, Becker A, Schille JT, Trakooljul N, Frank M, et al. Ablation of red stable transfected claudin expressing canine prostate adenocarcinoma and transitional cell carcinoma cell lines by C-cpe gold-nanoparticle-mediated laser intervention. Int J Mol Sci. (2021) 22:12289. doi: 10.3390/ijms222212289
102. Tanaka S, Aoyama T, Ogawa M, Takasawa A, Murata M, Osanai M, et al. Cytotoxicity of clostridium perfringens enterotoxin depends on the conditions of claudin-4 in ovarian carcinoma cells. Exp Cell Res. (2018) 371:278–86. doi: 10.1016/j.yexcr.2018.08.024
103. Mackensen A, Haanen JBAG, Koenecke C, Alsdorf W, Wagner-Drouet E, Borchmann P, et al. Cldn6-specific car-T cells plus amplifying rna vaccine in relapsed or refractory solid tumors: the phase 1 bnt211-01 trial. Nat Med. (2023) 29:2844–53. doi: 10.1038/s41591-023-02612-0
104. Shindo Y, Arai W, Konno T, Kohno T, Kodera Y, Chiba H, et al. Effects of histone deacetylase inhibitors tricostatin a and quisinostat on tight junction proteins of human lung adenocarcinoma A549 cells and normal lung epithelial cells. Histochem Cell Biol. (2021) 155:637–53. doi: 10.1007/s00418-021-01966-1
105. Sahin U, Koslowski M, Dhaene K, Usener D, Brandenburg G, Seitz G, et al. Claudin-18 splice variant 2 is a pan-cancer target suitable for therapeutic antibody development. Clin Cancer Res. (2008) 14:7624–34. doi: 10.1158/1078-0432.Ccr-08-1547
106. Hoggard J, Fan J, Lu Z, Lu Q, Sutton L, Chen YH. Claudin-7 increases chemosensitivity to cisplatin through the upregulation of caspase pathway in human nci-H522 lung cancer cells. Cancer Sci. (2013) 104:611–8. doi: 10.1111/cas.12135
107. Wu JE, Wu YY, Tung CH, Tsai YT, Chen HY, Chen YL, et al. DNA methylation maintains the cldn1-ephb6-slug axis to enhance chemotherapeutic efficacy and inhibit lung cancer progression. Theranostics. (2020) 10:8903–23. doi: 10.7150/thno.45785
Keywords: lung, cancer, claudins, treatment, diagnosis, pathogenesis
Citation: Arabi TZ, Alkattan W, Osman NA, Sabbah BN, Ashraf N and Ouban A (2024) Deciphering the role of claudins in lung cancer. Front. Oncol. 14:1435535. doi: 10.3389/fonc.2024.1435535
Received: 20 May 2024; Accepted: 27 August 2024;
Published: 19 September 2024.
Edited by:
Kang-Seo Park, University of Ulsan, Republic of KoreaReviewed by:
Dario Mizrachi, Brigham Young University, United StatesJian Li, Mianyang Third People’s Hospital, China
Copyright © 2024 Arabi, Alkattan, Osman, Sabbah, Ashraf and Ouban. This is an open-access article distributed under the terms of the Creative Commons Attribution License (CC BY). The use, distribution or reproduction in other forums is permitted, provided the original author(s) and the copyright owner(s) are credited and that the original publication in this journal is cited, in accordance with accepted academic practice. No use, distribution or reproduction is permitted which does not comply with these terms.
*Correspondence: Abderrahman Ouban, YW91YmFuQGFsZmFpc2FsLmVkdQ==
†These authors have contributed equally to this work and share first authorship