- 1Discipline of Paediatrics and Child Health, School of Clinical Medicine, Faculty of Medicine and Health, UNSW Sydney, Sydney, NSW, Australia
- 2Department of Respiratory Medicine, Sydney Children’s Hospital, Sydney, NSW, Australia
- 3Molecular and Integrative Cystic Fibrosis Research Centre, UNSW Sydney, Sydney, NSW, Australia
- 4School of Biomedical Sciences, Faculty of Medicine and Health, UNSW Sydney, Sydney, NSW, Australia
- 5Department of Respiratory Medicine, Concord Hospital, Sydney, NSW, Australia
- 6Faculty of Medicine, University of Sydney, Sydney, NSW, Australia
Introduction: Primary Ciliary Dyskinesia (PCD) is a rare genetic disorder affecting motile cilia, leading to impaired mucociliary clearance and increased susceptibility to respiratory infections. These infections contribute to long-term complications such as bronchiectasis and lung function decline.
Objectives: This review explores both the acute and long-term impact of respiratory infections in children with PCD, while highlighting the multiple contributors to infection susceptibility. The review also evaluates emerging personalized approaches such as gene and mRNA therapy that hold promise for restoring ciliary function and reducing the burden of acute infections in pediatric PCD.
Key findings and conclusions: Acute respiratory infections have a significant impact on morbidity in pediatric PCD, driving progressive airway remodeling. While current treatment strategies focus on managing infections directly, emerging therapies targeting inflammation and genetic causes hold promise for reducing infection burden and improving long-term outcomes. Future advances in personalized medicine could further enhance therapeutic approaches in this population.
1 Introduction
Primary Ciliary Dyskinesia (PCD) is a rare, incurable, progressive genetic disorder that affects ciliary structure and function, and results in impaired mucociliary clearance (1, 2). The most common symptoms of this mucostasis are daily wet cough, recurrent respiratory tract infections, chronic rhinosinusitis and recurrent otitis media (3).
Acute infections have a substantial impact on children with PCD causing significant morbidity (4). Every exacerbation has the potential to cause a permanent decline in lung function (5) and hasten the progression of bronchiectasis (6). This review will explore the epidemiology, risks, complications, management and prevention of acute infections in children with PCD, including an exploration of the role of the microbiome in acute infections and novel personalized approaches that hold promise for restoring ciliary function.
2 Acute infections in PCD
Children with PCD suffer from recurrent acute and chronic upper and lower respiratory tract infections (7). In the upper airway, the ears and sinuses are the most common sites of acute infection (3). In the lower airway, acute bacterial or viral infections are the major cause of respiratory exacerbations, with symptoms such as increased cough, change in sputum volume and/or colour, shortness of breath or fevers (8). A respiratory exacerbation is not always associated with an acute infection (9). Distinguishing an infective from a non-infective exacerbation can be challenging however due to their similar clinical presentation. Therefore, for the purposes of this review, both acute infections and exacerbations are discussed simultaneously, acknowledging that they often overlap yet remain distinct clinical events. A complex interplay of factors contribute to the high burden of acute infections in children with PCD (Figure 1).
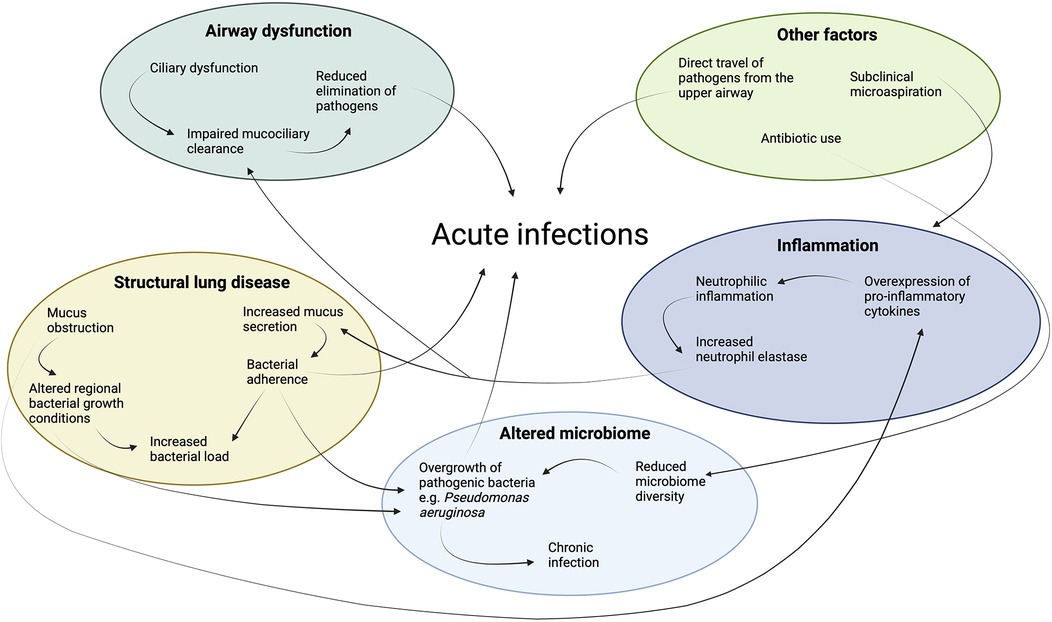
Figure 1. Key factors contributing to the high burden of acute infections in children with Primary Ciliary Dyskinesia (PCD). Airway dysfunction leads to impaired mucociliary clearance, promoting microbial colonization. Structural lung disease, such as bronchiectasis, exacerbates infection risk by creating areas of localized mucus obstruction and bacterial persistence. An altered microbiome in the respiratory tract contributes to the imbalance of pathogens, fostering chronic infection. Persistent inflammation due to infection and the inflammatory response causes further lung remodeling and impairment of host defences such as mucociliary clearance.
3 Epidemiology of acute infections in children with PCD
Acute respiratory tract infections are common in childhood but have a more significant burden in children with PCD. Historically, collection of accurate epidemiological data on exacerbation frequency was challenging in PCD due to differing definitions in clinical practice and research. However, a consensus definition for lower respiratory exacerbations in children and adults with PCD is now available. It defines a pulmonary exacerbation as the presence of 3 or more of the following: increased cough; change in sputum colour and/or volume; decision to start or change antibiotic treatment because of perceived pulmonary symptoms; increased shortness of breath perceived by the patient or parent; new or increased hemoptysis; temperature >38 °C; and malaise, tiredness, fatigue or lethargy (8). A large international prospective cohort study was the first to collect data using this definition, reporting an incidence rate of 3.1 respiratory exacerbations per person per year in patients with PCD (10). The number of exacerbations increases with age, disease severity as measured by lung function and CT chest scores, and chronic infection with Pseudomonas aeruginosa (10, 11). Consensus definitions for sinonasal and otologic exacerbations are also available (12), which will facilitate collection of accurate data on these additional clinically important variables in patients with PCD.
4 Bacterial and viral causes of infections
Respiratory viruses are the most common cause of an acute exacerbation. In children with bronchiectasis respiratory viruses, most commonly rhinovirus, parainfluenza, influenza and coronavirus, are detected in up to 48% of exacerbations (13). The most common causative organisms for bacterial respiratory infections in PCD are Haemophilus influenzae, P.aeruginosa, Streptococcus pneumoniae, Moraxella catarrhalis and Staphylococcus aureus (6, 7, 14, 15). H.influenzae is a particularly important pathogen in children and adolescents with PCD as a cause for both acute and chronic infections, with several studies reporting a prevalence of up to 80% in this group (6, 7). The prevalence declines with age and is 21% in adults (7). Respiratory viruses can cause direct damage to the ciliated epithelial cells (16), and bacterial infections such as P.aeruginosa can slow ciliary beat frequency (17). This further impairs mucociliary clearance predisposing to secondary infections.
5 Risks and complications of acute infections in children with PCD
Acute exacerbations can cause a reduction in lung function, and approximately 25% of children with PCD fail to recover back to baseline lung function within 3 months (5). Although the overall rate of FEV1 decline in PCD is approximately 0.49 FEV1% predicted per year (18), this accelerates with a decline of 1.95 FEV1% predicted per hospitalization, based on evidence in pediatric bronchiectasis (19). Hemoptysis can occur due to acute or chronic infection and inflammation, and can be life-threatening due to rupture of a tortuous blood vessel, requiring embolization (20).
Acute infections also impact psychological wellbeing. Frequent hospitalizations have a profound impact on quality of life, leading to social isolation, missed school days, and psychological distress (4). Caregivers also experience significant mental health challenges, with acute exacerbations causing a significant worsening in quality of life and parental mental health scores (4). Strategies that reduce the frequency and severity of exacerbations have the potential to reduce the impact of having a child with PCD on the wider family.
6 Airway remodeling and bronchiectasis in PCD
Acute infections contribute to structural changes in the airway walls by initiating and propagating the “vicious vortex” cycle of infection, inflammation, mucociliary dysfunction and structural lung disease (Figure 2) (21). Understanding this vortex is vital in bronchiectasis, whatever the underlying cause.
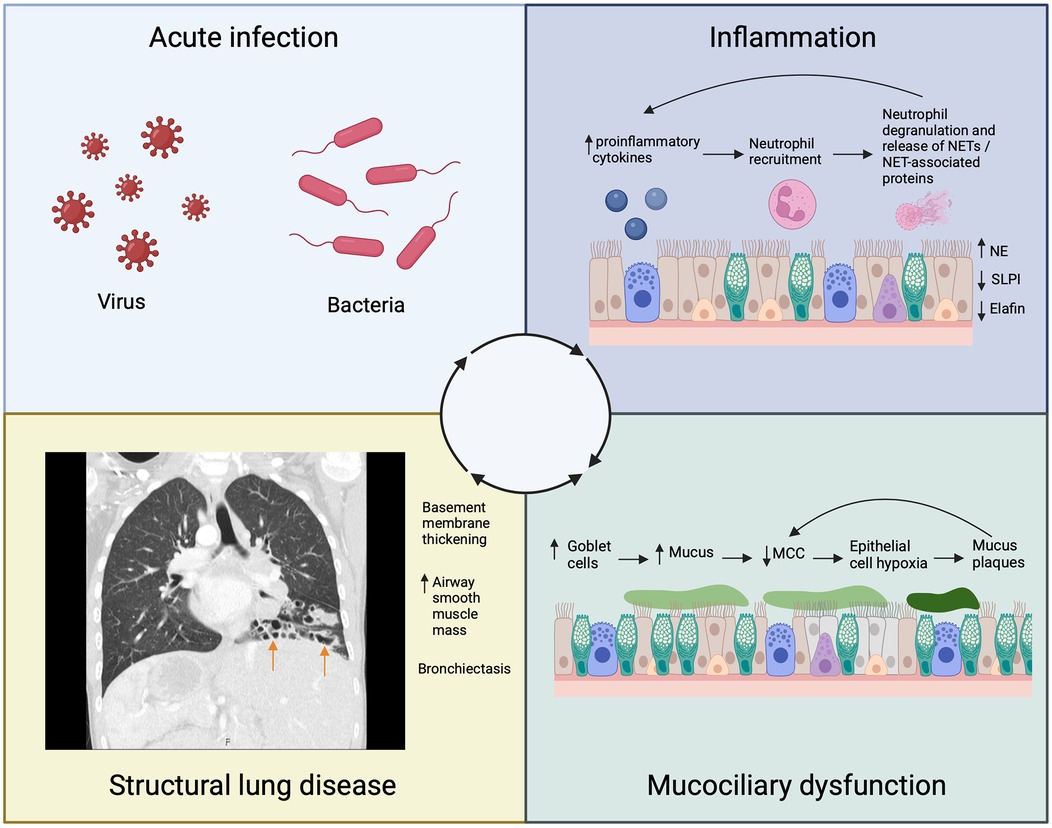
Figure 2. Schematic representing the contribution of acute infection to the inflammatory cycle that leads to airway remodeling and bronchiectasis (arrows) in Primary Ciliary Dyskinesia (PCD). NSPs, neutrophil serine proteases; NETs, neutrophil extracellular traps; SLPI, secretory leukocyte protease inhibitor; NE, neutrophil elastase; MCC, mucociliary clearance.
During an acute exacerbation, there is a significant rise in proinflammatory cytokines that attract neutrophils to the PCD airway (22). Neutrophils phagocytose pathogens and degranulate, releasing neutrophil extracellular traps (NETs) and NET-associated proteins including neutrophil elastase (NE) (23). Neutrophil elastase is a serine protease that induces goblet cell metaplasia, stimulates excess mucus production, and further impairs mucociliary clearance (24–26). The ensuing obstruction of the airway with mucus causes local epithelial hypoxia, perpetuating further pro-inflammatory events promoting neutrophil recruitment (27). Neutrophil elastase additionally impairs antimicrobial and anti-inflammatory responses by cleaving proteins such as elafin and secretory leukocyte protease inhibitor (SLPI) (24). These events further amplify the inflammatory response. In addition to driving inflammation, chronic epithelial hypoxia causes hyperconcentration of mucus due to increased transepithelial sodium and fluid absorption (28, 29). As mucus concentration increases, mucociliary transport rates further decline due to formation of mucus plaques which themselves impair ciliary function particularly in more distal airways, perpetuating further obstruction and chronic hypoxia (27).
Our understanding of the consequences of neutrophilic inflammation on airway remodeling in PCD is evolving. Bronchial epithelial reticular basement membrane thickening is seen in children with PCD on endobronchial biopsies (30, 31), and this thickening correlates with both ventilation heterogeneity measured by LCI2.5 and the concentration of neutrophils in lavage fluid (30). An increase in the number of myocytes and airway smooth muscle volume is also seen in children with PCD (32). Bronchiectasis is a key feature of airway remodeling in PCD, with a prevalence of 61% in children and 98% in adults (6). The degree of bronchiectasis correlates with proinflammatory cytokine and NE levels in the sputum of children with PCD (33). These findings suggest that airway remodeling may be related to the degree of neutrophilic inflammation, and the structural changes have a functional impact. As airway remodeling progresses, it likely contributes to the long-term decline in lung function observed in children with PCD emphasising the need for further research to understand the relationship between airway inflammation, remodeling and disease progression.
7 Role of the lung microbiome in acute infections
The human microbiome plays a crucial role in the development and progression of acute airway infections. A diverse and balanced lung microbiome helps protect the airway from infection. Beneficial microbes such as Prevotella and Veillonella limit the ability of harmful pathogens to colonise the lungs. Disruption of this balance, termed dysbiosis, allows pathogenic organisms like H.influenzae, P.aeruginosa or S.pneumoniae to cause infection (34). The lung microbiome additionally modulates the host's immune response, protecting against infection (35). Pathogen-pathogen interactions within the microbiome may also impact the course of infections. Viral infections such as influenza or rhinovirus can disrupt the upper airway microbiome, creating an environment favourable for bacterial entry into the lower respiratory tract (36). Each acute infection further alters the microbiome, creating a self-amplifying cycle of inflammation, infection, and microbiome disturbance.
Several factors contribute to alterations in the balance and composition of the lung microbiome in PCD. These include direct travel of pathogens along the airway mucosa from the upper airway, impaired mucociliary clearance, structural lung disease impacting regional bacterial growth conditions and frequent or long-term use of antibiotics (37). Many of these factors change over time, highlighting the dynamic nature of the PCD microbiome (38).
Our understanding of the PCD microbiome is evolving. Rogers et al. (39) used culture-independent methods to assess the lower airway microbiota of 24 adults and children with PCD using sputum and/or broncho-alveolar lavage samples. Members of the genera Pseudomonas, Streptococcus and Haemophilus were most abundant (39), which is in contrast to the microbiota of healthy individuals where Streptococcus, Veillonella and Prevotella are most abundant (40). Bacterial load was not found to correlate with lung function, however the abundance of P.aeruginosa was strongly negatively associated with lung function. Several other studies have linked chronic P.aeruginosa colonisation and more severe disease measured by lung function (41) and CT scores (11, 41) in adults and children with PCD. However, other studies have found no such association (11, 15, 42), highlighting the need for more research in this area. Tural et al. (43) went on to assess the composition and structure of the airway microbiota in 7 sibling pairs of children with a diagnosis of PCD and 9 healthy parents. In contrast to Rogers et al. (39), the most common genera were Haemophilus, Actinobacillus and Veillonella in patients with PCD, and Ralstonia, Moraxella and Haemophilus in their parents (43). Significantly less bacterial diversity was seen in patients who had a positive clinical bacterial airway culture or H.influenzae growth in the previous year. The microbial community tended to cluster by several clinical factors including age, lung function indices, pulmonary exacerbation status and the presence of H.influenzae growth. Interestingly, microbial communities did not cluster by families. These studies provide early insight into the complexity of the airway microbiota in PCD and highlight the importance of ongoing work to better understand the relationship between the microbiome and susceptibility to acute infections in PCD.
8 Management and prevention of acute infections
Current management and prevention strategies for acute infections in children with PCD are largely based on expert opinion and extrapolation of evidence and guidelines from other causes of bronchiectasis. Consensus recommendations for management of PCD are available from North America (1) and the European Respiratory Society (9), as well as an international consensus for infection prevention and control (44). Management of acute infections generally includes antibiotics and airway clearance techniques (ACTs). Commonly used preventative strategies include vaccinations, maintenance antibiotics, ACTs, maintenance of nutrition and exercise.
8.1 Antibiotic therapy
Current consensus suggests that treatment of acute exacerbations with a prolonged course of antibiotics for 14–21 days is required (45–48), but the data upon which this guidance is based are limited. Antibiotic choice is guided by the pathogens isolated from the patient's sputum or oropharyngeal cough swabs, clinical severity, local antibiotic susceptibility patterns and patient tolerance (1, 46). In the case of a severe infection or failure to respond to oral therapy, intravenous antibiotics, monitoring and intensive ACTs may be required (9, 46). If P.aeruginosa is isolated on lower airway samples, eradication is usually attempted (44). While there are no established P.aeruginosa eradication guidelines in PCD, international pediatric bronchiectasis guidelines suggest the use of IV antibiotics for 2 weeks followed by inhaled antibiotics for 4–12 weeks, if symptomatic. If asymptomatic, treatment with oral and/or inhaled antibiotics for 2 weeks followed by inhaled antibiotics for 4–12 weeks is suggested (49). The choice of antibiotics depends on local availability and preference, and P.aeruginosa susceptibility profiles (49). However numerous alternative eradication strategies are published and used worldwide in PCD (50–52), highlighting the need for further research to determine the optimal regime.
8.2 Airway clearance techniques
Airway clearance techniques commonly employed for both prevention and management of exacerbations in children with PCD include breathing techniques, manual techniques such as percussions and vibrations, positioning, positive expiratory pressure (PEP) adjuncts and oscillating devices (53). Aerobic exercise can also form an important airway clearance modality (54, 55). Studies comparing techniques have found no significant difference in lung function, acute exacerbation rate or time to first exacerbation with different methods (56–58). Therefore review by a specialist respiratory physiotherapist should be performed to decide on the most appropriate technique for each patient, taking into account factors such as their age, preference and level of experience.
8.3 Nebulized therapies
Hypertonic saline is the most commonly used nebulized agent in children with PCD as an adjunct to ACTs (59). Nebulized hypertonic saline osmotically hydrates airway secretions and stimulates cough (60). In a study comparing nebulized 7% hypertonic saline with 0.9% isotonic saline in 22 patients with PCD, no differences in quality-of-life outcomes was found between the two groups (61). This study had several limitations including the small sample size and lack of disease specific outcome measures. In children with non-CF bronchiectasis, 3% hypertonic saline with ACTs improves lung function parameters and reduces the number of exacerbations, compared with ACTs alone (62).
There is currently insufficient evidence to support the routine use of nebulized mucolytics such as dornase alpha in children with PCD. In adults with bronchiectasis, one large randomized controlled trial found that treatment with nebulized dornase alpha was associated with an increase in frequency of respiratory exacerbations and a greater rate of lung function decline compared with placebo, suggesting potentially harmful effects in this population (63). Despite subsequent recommendations against the use of dornase alpha in PCD (9, 48), there are several published case studies reporting improvement in lung function and symptoms in children with PCD when treated with dornase alpha, suggesting possible benefit (64–66).
8.4 Maintenance antibiotics
Long-term macrolide antibiotic therapy is widely used to reduce exacerbation frequency in PCD due to its anti-inflammatory, bacteriostatic and immunomodulatory properties (67). Azithromycin is the most commonly prescribed macrolide for this purpose in children, but roxithromycin, erythromycin and clarithromycin have also been studied (68). A randomized controlled trial comparing maintenance azithromycin with placebo in adults and children with PCD found that azithromycin halved the rate of respiratory exacerbations (69). In vitro, azithromycin has been shown to inhibit production of cytokines and promote cell proliferation in airway basal cells isolated from patients with PCD (70).
9 Emerging preventative therapies
Ultimately, preventing acute exacerbations from occurring is the most effective long-term strategy to preserve lung function and improve outcomes in pediatric PCD. Emerging therapies such as sodium channel blockers, oral mucolytics, dipeptidyl peptidase (DPP)-1 inhibitors and gene or mRNA therapies are innovative approaches aimed at enhancing airway hydration, reducing inflammation and ultimately restoring mucociliary clearance. As research progresses, these preventative strategies hold the potential to transform PCD care, shifting the focus from reactive treatment to proactive disease modification.
9.1 Sodium channel blockers
A potential explanation for the apparent reduced efficacy of nebulized hypertonic saline in patients with PCD compared to those with CF is the presence of a functional cystic fibrosis transmembrane conductance regulator (CFTR) chloride channel in the PCD airway. In patients with CF, the lack of a functional CFTR channel allows the sodium-chloride (NaCl) deposited into the airway through nebulization to remain present, osmotically attracting water to hydrate mucus (71). In contrast, in PCD, the presence of a functional CFTR channel could facilitate rapid absorption of the NaCl, reducing its osmotic hydrating effect. Blocking epithelial sodium channels could theoretically slow sodium and thereby chloride and fluid absorption, improving airway hydration. This theory was investigated in CLEAN-PCD, a randomized controlled trial assessing the use of the sodium channel blocker idrevloride in patients with PCD (72). Treatment with idrevloride in hypertonic saline for 28 days led to a mean absolute change from baseline FEV1 of 1% predicted, compared with hypertonic saline alone. In the second part of the study, coadministration of the CFTR modulator therapy ivacaftor with idrevloride in hypertonic saline was found to result in additional improvement in FEV1 of 4.7% predicted from baseline. No improvement in FEV1 was seen in those treated with ivacaftor and nebulized placebo. Therefore, it was suggested by the authors that the increase in lung function seen in the ivacaftor with idrevloride in hypertonic saline group was due to the effect of prolonged treatment with idrevloride in hypertonic saline, rather than due to ivacaftor. These results highlight the potential of combining sodium channel blockers with hypertonic saline as a novel approach to enhance airway hydration, improve mucociliary clearance and potentially reduce exacerbation frequency in PCD.
9.2 Oral mucolytics
Oral mucolytics are an additional potential strategy to assist with preventing acute infections and reducing pulmonary exacerbations. The evidence for benefit is currently low in children with PCD. In a small placebo controlled trial of oral N-acetylcysteine (NAC), no difference in outcomes including clinical symptoms, lung function and sputum bacteriology was detected (73). REPEAT is a randomized controlled trial evaluating exacerbation rate following treatment of 104 adults and child with PCD with either azithromycin plus placebo, or azithromycin plus the oral mucolytic erdosteine (74). This study is complete and the results are in press.
9.3 DPP-1 inhibitors
Dipeptidyl peptidase-1 inhibitors are a particularly promising new developing therapy to prevent exacerbations in PCD, targeting neutrophilic inflammation. Neutrophil serine proteases including NE are cleaved and activated by DPP-1 during maturation of neutrophils in the bone marrow (75). DPP-1 inhibitors therefore prevent the activation of NSPs. In the phase 2 WILLOW trial, the DPP-1 inhibitor brensocatib prolonged time to first exacerbation and reduced sputum neutrophil elastase activity in adults with bronchiectasis (76). A phase 3 global study, ASPEN, has since completed enrolment and results are expected in early 2025 (77). A second DPP-1 inhibitor, BI 1291583, is being studied in the phase 2 Airleaf trial which has also finished recruitment with results pending publication (78).
9.4 Personalized gene and mRNA therapies
Ultimately, restoration of ciliary function and mucociliary clearance using personalized treatment approaches such as gene or messenger RNA (mRNA) therapy would be the ideal strategy to reduce the burden of acute infections in children with PCD. Gene therapy can successfully restore ciliary beat frequency (CBF) in PCD cells in vitro, such as those with DNAI1 deficiency, using lenti-virus-mediated delivery of complementary DNA (cDNA) (79, 80). Likewise, gene editing using tools like transcription activator-like effector nucleases (TALENs) has shown promise in correcting PCD gene defects (81). mRNA therapy, which delivers corrected mRNA encoding for a target protein to airway cells to restore protein function, has also shown great potential in PCD animal models (82, 83). Nebulized mRNA therapies targeting DNAI1 (83) and CCDC40 (84) have been developed, with promising results in the preclinical trials.
While gene and mRNA therapies hold great promise for restoring ciliary function in patients with PCD, several barriers and challenges exist. These include potential difficulties with immune related inhibition of gene or mRNA transfer (2, 85), the potential for formation of anti-drug antibodies (86–88), the unknown effect of the limited half-life of ciliated epithelial cells (89), and the genetic heterogeneity of PCD necessitating separate formulations to target each genetic defect (90). Ultimately, the successful application of gene therapy and mRNA therapies could revolutionize the management of PCD in the future.
10 Future directions: patient-derived airway models for studying infection and personalized therapies in PCD
The development of patient-derived airway epithelial cell models offers a transformative tool for advancing both our understanding of the infection burden in PCD and the discovery of personalized therapeutic interventions. These models, derived from nasal or bronchial epithelial cells of PCD patients, are cultured at the air-liquid interface (ALI), closely mimicking the in vivo respiratory environment (91). Importantly, they retain the genetic and functional characteristics of the donor (92, 93), making them invaluable for studying disease-specific pathophysiology and testing personalized drug responses. Ultimately, patient-derived airway models hold great promise for personalized drug discovery in PCD. They allow for the screening of therapeutic interventions, including antibiotics, airway clearance techniques, and novel pharmacological agents, in a patient-specific manner (92). This approach not only accelerates the development of effective treatments but also ensures that therapies are tailored to the genetic and functional characteristics of each patient, paving the way for more individualized and effective prevention and management of acute infections in children with PCD.
11 Conclusion
An intact mucociliary escalator is vital to protect the airway against infection. In children with PCD, acute infection has traditionally been treated as a cause of exacerbation, but it now seems clearer that both acute and chronic infection are potent drivers of chronic inflammation, precipitating and perpetuating the “vicious vortex”. The future of PCD management will require us to understand this interplay more carefully, but will also open the door to exciting opportunities to make meaningful change to the landscape for patients with PCD.
Author contributions
MF: Writing – original draft, Writing – review & editing. BP: Writing – review & editing. LO: Writing – review & editing. SW: Writing – review & editing. LM: Conceptualization, Writing – review & editing.
Funding
The author(s) declare that financial support was received for the research and/or publication of this article. MF is supported by a Rotary Club of Sydney Cove Grant and National Health and Medical Research Council (NHMRC) Postgraduate Scholarship.
Conflict of interest
The authors declare that the research was conducted in the absence of any commercial or financial relationships that could be construed as a potential conflict of interest.
Generative AI statement
The author(s) declare that no Generative AI was used in the creation of this manuscript
Publisher's note
All claims expressed in this article are solely those of the authors and do not necessarily represent those of their affiliated organizations, or those of the publisher, the editors and the reviewers. Any product that may be evaluated in this article, or claim that may be made by its manufacturer, is not guaranteed or endorsed by the publisher.
References
1. Shapiro AJ, Zariwala MA, Ferkol T, Davis SD, Sagel SD, Dell SD, et al. Diagnosis, monitoring, and treatment of primary ciliary dyskinesia: PCD foundation consensus recommendations based on state of the art review. Pediatr Pulmonol. (2016) 51(2):115–32. doi: 10.1002/ppul.23304
2. Paff T, Omran H, Nielsen KG, Haarman EG. Current and future treatments in primary ciliary dyskinesia. Int J Mol Sci. (2021) 22(18):9834. doi: 10.3390/ijms22189834
3. Goutaki M, Meier AB, Halbeisen FS, Lucas JS, Dell SD, Maurer E, et al. Clinical manifestations in primary ciliary dyskinesia: systematic review and meta-analysis. Eur Respir J. (2016) 48(4):1081–95. doi: 10.1183/13993003.00736-2016
4. Kapur N, Masters IB, Newcombe P, Chang AB. The burden of disease in pediatric non-cystic fibrosis bronchiectasis. Chest. (2012) 141(4):1018–24. doi: 10.1378/chest.11-0679
5. Sunther M, Bush A, Hogg C, McCann L, Carr SB. Recovery of baseline lung function after pulmonary exacerbation in children with primary ciliary dyskinesia. Pediatr Pulmonol. (2016) 51(12):1362–6. doi: 10.1002/ppul.23479
6. Noone PG, Leigh MW, Sannuti A, Minnix SL, Carson JL, Hazucha M, et al. Primary ciliary dyskinesia: diagnostic and phenotypic features. Am J Respir Crit Care Med. (2004) 169(4):459–67. doi: 10.1164/rccm.200303-365OC
7. Alanin MC, Nielsen KG, von Buchwald C, Skov M, Aanaes K, Hoiby N, et al. A longitudinal study of lung bacterial pathogens in patients with primary ciliary dyskinesia. Clin Microbiol Infect. (2015) 21(12):1093 e1–7. doi: 10.1016/j.cmi.2015.08.020
8. Lucas JS, Gahleitner F, Amorim A, Boon M, Brown P, Constant C, et al. Pulmonary exacerbations in patients with primary ciliary dyskinesia: an expert consensus definition for use in clinical trials. ERJ Open Res. (2019) 5(1):00147-2018. doi: 10.1183/23120541.00147-2018
9. Barbato A, Frischer T, Kuehni CE, Snijders D, Azevedo I, Baktai G, et al. Primary ciliary dyskinesia: a consensus statement on diagnostic and treatment approaches in children. Eur Respir J. (2009) 34(6):1264–76. doi: 10.1183/09031936.00176608
10. Schreck LD, Goutaki M, Pedersen ES, Copeland F, Fernández TL, group LwPpaet al.Incidence and predictors of pulmonary exacerbations in primary ciliary dyskinesia: an international prospective cohort study. medRxiv. 2025:2025.01.13.25320478).
11. Piatti G, De Santi MM, Farolfi A, Zuccotti GV, D’Auria E, Patria MF, et al. Exacerbations and Pseudomonas aeruginosa colonization are associated with altered lung structure and function in primary ciliary dyskinesia. BMC Pediatr. (2020) 20(1):158. doi: 10.1186/s12887-020-02062-4
12. Goutaki M, Lam YT, Anagiotos A, Armengot M, Burgess A, Campbell R, et al. Definition of sinonasal and otological exacerbation in patients with primary ciliary dyskinesia: an expert consensus. ERJ Open Res. (2024) 10(6):00218-2024. doi: 10.1183/23120541.00218-2024
13. Kapur N, Mackay IM, Sloots TP, Masters IB, Chang AB. Respiratory viruses in exacerbations of non-cystic fibrosis bronchiectasis in children. Arch Dis Child. (2014) 99(8):749–53. doi: 10.1136/archdischild-2013-305147
14. Davis SD, Ferkol TW, Rosenfeld M, Lee HS, Dell SD, Sagel SD, et al. Clinical features of childhood primary ciliary dyskinesia by genotype and ultrastructural phenotype. Am J Respir Crit Care Med. (2015) 191(3):316–24. doi: 10.1164/rccm.201409-1672OC
15. Maglione M, Bush A, Nielsen KG, Hogg C, Montella S, Marthin JK, et al. Multicenter analysis of body mass index, lung function, and sputum microbiology in primary ciliary dyskinesia. Pediatr Pulmonol. (2014) 49(12):1243–50. doi: 10.1002/ppul.22984
16. Robinot R, Hubert M, de Melo GD, Lazarini F, Bruel T, Smith N, et al. SARS-CoV-2 infection induces the dedifferentiation of multiciliated cells and impairs mucociliary clearance. Nat Commun. (2021) 12(1):4354. doi: 10.1038/s41467-021-24521-x
17. Kanthakumar K, Taylor G, Tsang KW, Cundell DR, Rutman A, Smith S, et al. Mechanisms of action of Pseudomonas aeruginosa pyocyanin on human ciliary beat in vitro. Infect Immun. (1993) 61(7):2848–53. doi: 10.1128/iai.61.7.2848-2853.1993
18. Shah A, Shoemark A, MacNeill SJ, Bhaludin B, Rogers A, Bilton D, et al. A longitudinal study characterising a large adult primary ciliary dyskinesia population. Eur Respir J. (2016) 48(2):441–50. doi: 10.1183/13993003.00209-2016
19. Kapur N, Masters IB, Chang AB. Longitudinal growth and lung function in pediatric non-cystic fibrosis bronchiectasis: what influences lung function stability? Chest. (2010) 138(1):158–64. doi: 10.1378/chest.09-2932
20. Lim RK, Tremblay A, Lu S, Somayaji R. Evaluating hemoptysis hospitalizations among patients with bronchiectasis in the United States: a population-based cohort study. BMC Pulm Med. (2021) 21(1):392. doi: 10.1186/s12890-021-01762-6
21. Flume PA, Chalmers JD, Olivier KN. Advances in bronchiectasis: endotyping, genetics, microbiome, and disease heterogeneity. Lancet. (2018) 392(10150):880–90. doi: 10.1016/S0140-6736(18)31767-7
22. Ratjen F, Waters V, Klingel M, McDonald N, Dell S, Leahy TR, et al. Changes in airway inflammation during pulmonary exacerbations in patients with cystic fibrosis and primary ciliary dyskinesia. Eur Respir J. (2016) 47(3):829–36. doi: 10.1183/13993003.01390-2015
23. Lacy P. Mechanisms of degranulation in neutrophils. Allergy Asthma Clin Immunol. (2006) 2(3):98–108. doi: 10.1186/1710-1492-2-3-98
24. Johnson E, Long MB, Chalmers JD. Biomarkers in bronchiectasis. Eur Respir Rev. (2024) 33(173):230234. doi: 10.1183/16000617.0234-2023
25. Fischer B, Voynow J. Neutrophil elastase induces MUC5AC messenger RNA expression by an oxidant-dependent mechanism. Chest. (2000) 117(5 Suppl 1):317S–20. doi: 10.1378/chest.117.5_suppl_1.317S
26. Krotova K, Khodayari N, Oshins R, Aslanidi G, Brantly ML. Neutrophil elastase promotes macrophage cell adhesion and cytokine production through the integrin-Src kinases pathway. Sci Rep. (2020) 10(1):15874. doi: 10.1038/s41598-020-72667-3
27. Hill DB, Button B, Rubinstein M, Boucher RC. Physiology and pathophysiology of human airway mucus. Physiol Rev. (2022) 102(4):1757–836. doi: 10.1152/physrev.00004.2021
28. Mikami Y, Grubb BR, Rogers TD, Dang H, Asakura T, Kota P, et al. Chronic airway epithelial hypoxia exacerbates injury in muco-obstructive lung disease through mucus hyperconcentration. Sci Transl Med. (2023) 15(699):eabo7728. doi: 10.1126/scitranslmed.abo7728
29. Boucher RC. Muco-obstructive lung diseases. N Engl J Med. (2019) 380(20):1941–53. doi: 10.1056/NEJMra1813799
30. Koucký V, Uhlík J, Hoňková L, Koucký M, Doušová T, Pohunek P. Ventilation inhomogeneity and bronchial basement membrane changes in chronic neutrophilic airway inflammation. Chest. (2020) 157(4):779–89. doi: 10.1016/j.chest.2019.10.023
31. Hilliard TN, Regamey N, Shute JK, Nicholson AG, Alton EW, Bush A, et al. Airway remodelling in children with cystic fibrosis. Thorax. (2007) 62(12):1074–80. doi: 10.1136/thx.2006.074641
32. Regamey N, Ochs M, Hilliard TN, Mühlfeld C, Cornish N, Fleming L, et al. Increased airway smooth muscle mass in children with asthma, cystic fibrosis, and non-cystic fibrosis bronchiectasis. Am J Respir Crit Care Med. (2008) 177(8):837–43. doi: 10.1164/rccm.200707-977OC
33. Sagel SD, Kupfer O, Wagner BD, Davis SD, Dell SD, Ferkol TW, et al. Airway inflammation in children with primary ciliary dyskinesia. Ann Am Thorac Soc. (2023) 20(1):67–74. doi: 10.1513/AnnalsATS.202204-314OC
34. Drigot ZG, Clark SE. Insights into the role of the respiratory tract microbiome in defense against bacterial pneumonia. Curr Opin Microbiol. (2024) 77:102428. doi: 10.1016/j.mib.2024.102428
35. Brown RL, Sequeira RP, Clarke TB. The microbiota protects against respiratory infection via GM-CSF signaling. Nat Commun. (2017) 8(1):1512. doi: 10.1038/s41467-017-01803-x
36. Wolter N, Tempia S, Cohen C, Madhi SA, Venter M, Moyes J, et al. High nasopharyngeal pneumococcal density, increased by viral coinfection, is associated with invasive pneumococcal pneumonia. J Infect Dis. (2014) 210(10):1649–57. doi: 10.1093/infdis/jiu326
37. Dickson RP, Huffnagle GB. The lung microbiome: new principles for respiratory bacteriology in health and disease. PLoS Pathog. (2015) 11(7):e1004923. doi: 10.1371/journal.ppat.1004923
38. Dickson RP, Martinez FJ, Huffnagle GB. The role of the microbiome in exacerbations of chronic lung diseases. Lancet. (2014) 384(9944):691–702. doi: 10.1016/S0140-6736(14)61136-3
39. Rogers GB, Carroll MP, Zain NM, Bruce KD, Lock K, Walker W, et al. Complexity, temporal stability, and clinical correlates of airway bacterial community composition in primary ciliary dyskinesia. J Clin Microbiol. (2013) 51(12):4029–35. doi: 10.1128/JCM.02164-13
40. Li R, Li J, Zhou X. Lung microbiome: new insights into the pathogenesis of respiratory diseases. Signal Transduct Target Ther. (2024) 9(1):19. doi: 10.1038/s41392-023-01722-y
41. Cohen-Cymberknoh M, Weigert N, Gileles-Hillel A, Breuer O, Simanovsky N, Boon M, et al. Clinical impact of Pseudomonas aeruginosa colonization in patients with primary ciliary dyskinesia. Respir Med. (2017) 131:241–6. doi: 10.1016/j.rmed.2017.08.028
42. Holgersen MG, Marthin JK, Raidt J, Qvist T, Johansen HK, Omran H, et al. Long-term lung function and Pseudomonas aeruginosa infection in genotyped primary ciliary dyskinesia. Ann Am Thorac Soc. (2025) 22(2):216–25. doi: 10.1513/AnnalsATS.202404-340OC
43. Ademhan Tural D, Kasikci M, Eryilmaz Polat S, Ozsezen B, Hizal M, Sunman B, et al. The airway microbiota in siblings with primary ciliary dyskinesia: related factors and correlation with clinical characteristics. Pediatr Pulmonol. (2024) 59(3):695–706. doi: 10.1002/ppul.26816
44. Marthin JK, Lucas JS, Boon M, Casaulta C, Crowley S, Destouches DMS, et al. International BEAT-PCD consensus statement for infection prevention and control for primary ciliary dyskinesia in collaboration with ERN-LUNG PCD core network and patient representatives. ERJ Open Res. (2021) 7(3):00301–2021. doi: 10.1183/23120541.00301-2021
45. Abbott L, Plummer A, Hoo ZH, Wildman M. Duration of intravenous antibiotic therapy in people with cystic fibrosis. Cochrane Database Syst Rev. (2019) 9(9):CD006682. doi: 10.1002/14651858.CD006682.pub6
46. Chang AB, Bell SC, Byrnes CA, Dawkins P, Holland AE, Kennedy E, et al. Thoracic Society of Australia and New Zealand (TSANZ) position statement on chronic suppurative lung disease and bronchiectasis in children, adolescents and adults in Australia and New Zealand. Respirology. (2023) 28(4):339–49. doi: 10.1111/resp.14479
47. Hill AT, Sullivan AL, Chalmers JD, De Soyza A, Elborn SJ, Floto AR, et al. British Thoracic Society Guideline for bronchiectasis in adults. Thorax. (2019) 74(Suppl 1):1–69. doi: 10.1136/thoraxjnl-2018-212463
48. Polineni D, Davis SD, Dell SD. Treatment recommendations in Primary Ciliary Dyskinesia. Paediatr Respir Rev. (2016) 18:39–45. doi: 10.1016/j.prrv.2015.10.002
49. Chang AB, Fortescue R, Grimwood K, Alexopoulou E, Bell L, Boyd J, et al. European Respiratory Society guidelines for the management of children and adolescents with bronchiectasis. Eur Respir J. (2021) 58(2):2002990. doi: 10.1183/13993003.02990-2020
50. Arndal E, Johansen HK, Haagensen JAJ, Bartell JA, Marvig RL, Alanin M, et al. Primary ciliary dyskinesia patients have the same P. aeruginosa clone in sinuses and lungs. Eur Respir J. (2020) 55(1):1901472. doi: 10.1183/13993003.01472-2019
51. Gatt D, Shaw M, Wee W, Solomon M, Dell SD, Ratjen F. Efficacy of antibiotic eradication therapy of early Pseudomonas aeruginosa infection in children with primary ciliary dyskinesia. Ann Am Thorac Soc. (2023) 20(6):854–60. doi: 10.1513/AnnalsATS.202210-858OC
52. Crowley S, Holgersen MG, Nielsen KG. Variation in treatment strategies for the eradication of Pseudomonas aeruginosa in primary ciliary dyskinesia across European centers. Chron Respir Dis. (2019) 16:1479972318787919. doi: 10.1177/1479972318787919
53. Schofield LM, Duff A, Brennan C. Airway clearance techniques for Primary Ciliary Dyskinesia; is the Cystic Fibrosis literature portable? Paediatr Respir Rev. (2018) 25:73–7. doi: 10.1016/j.prrv.2017.03.011
54. Schofield L, Lloyd N, Kang R, Marsh G, Keenan V, Wilkins H. Physiotherapy English national standards of care for children with primary ciliary dyskinesia. J ACPRC. (2018) 50:71.
55. Phillips GE, Thomas S, Heather S, Bush A. Airway response of children with primary ciliary dyskinesia to exercise and beta2-agonist challenge. Eur Respir J. (1998) 11(6):1389–91. doi: 10.1183/09031936.98.11061389
56. Gokdemir Y, Karadag-Saygi E, Erdem E, Bayindir O, Ersu R, Karadag B, et al. Comparison of conventional pulmonary rehabilitation and high-frequency chest wall oscillation in primary ciliary dyskinesia. Pediatr Pulmonol. (2014) 49(6):611–6. doi: 10.1002/ppul.22861
57. Vandervoort B, De Beuckeleer D, Huenaerts E, Schulte M, Vermeulen F, Proesmans M, et al. The short term influence of chest physiotherapy on lung function parameters in children with cystic fibrosis and primary ciliary dyskinesia. Front Pediatr. (2022) 10:858410. doi: 10.3389/fped.2022.858410
58. Bingol I, Gokdemir Y, Yilmaz-Yegit C, Ergenekon P, Atag E, Bas Ikizoglu N, et al. Comparison of conventional chest physiotherapy and oscillatory positive expiratory pressure therapy in primary ciliary dyskinesia. Pediatr Pulmonol. (2020) 55(12):3414–20. doi: 10.1002/ppul.25099
59. Gardner RA, Ferkol TW, Davis SD, Rosenfeld M, Sagel SD, Dell SD, et al. Therapies used by children with primary ciliary dyskinesia: a natural history study. Pediatr Pulmonol. (2024) 60:e27412. doi: 10.1002/ppul.27412
60. Maiz Carro L, Martinez-Garcia MA. Nebulized hypertonic saline in noncystic fibrosis bronchiectasis: a comprehensive review. Ther Adv Respir Dis. (2019) 13:1753466619866102. doi: 10.1177/1753466619866102
61. Paff T, Daniels JM, Weersink EJ, Lutter R, Vonk Noordegraaf A, Haarman EG. A randomised controlled trial on the effect of inhaled hypertonic saline on quality of life in primary ciliary dyskinesia. Eur Respir J. (2017) 49(2):1601770. doi: 10.1183/13993003.01770-2016
62. Anuradha KWDA, Gunathilaka PKG, Wickramasinghe VP. Effectiveness of hypertonic saline nebulization in airway clearance in children with non-cystic fibrosis bronchiectasis: a randomized control trial. Pediatr Pulmonol. (2021) 56(2):509–15. doi: 10.1002/ppul.25206
63. O'Donnell AE, Barker AF, Ilowite JS, Fick RB. Treatment of idiopathic bronchiectasis with aerosolized recombinant human DNase I. rhDNase study group. Chest. (1998) 113(5):1329–34. doi: 10.1378/chest.113.5.1329
64. Desai M, Weller PH, Spencer DA. Clinical benefit from nebulized human recombinant DNase in Kartagener’s syndrome. Pediatr Pulmonol. (1995) 20(5):307–8. doi: 10.1002/ppul.1950200509
65. El-Abiad NM, Clifton S, Nasr SZ. Long-term use of nebulized human recombinant DNase1 in two siblings with primary ciliary dyskinesia. Respir Med. (2007) 101(10):2224–6. doi: 10.1016/j.rmed.2007.05.014
66. ten Berge M, Brinkhorst G, Kroon AA, de Jongste JC. DNase treatment in primary ciliary dyskinesia–assessment by nocturnal pulse oximetry. Pediatr Pulmonol. (1999) 27(1):59–61. doi: 10.1002/(SICI)1099-0496(199901)27:1%3C59::AID-PPUL11%3E3.0.CO;2-D
67. Knowles MR, Daniels LA, Davis SD, Zariwala MA, Leigh MW. Primary ciliary dyskinesia. Recent advances in diagnostics, genetics, and characterization of clinical disease. Am J Respir Crit Care Med. (2013) 188(8):913–22. doi: 10.1164/rccm.201301-0059CI
68. Kelly C, Chalmers JD, Crossingham I, Relph N, Felix LM, Evans DJ, et al. Macrolide antibiotics for bronchiectasis. Cochrane Database Syst Rev. (2018) 3(3):Cd012406. doi: 10.1002/14651858.CD012406.pub2
69. Kobbernagel HE, Buchvald FF, Haarman EG, Casaulta C, Collins SA, Hogg C, et al. Efficacy and safety of azithromycin maintenance therapy in primary ciliary dyskinesia (BESTCILIA): a multicentre, double-blind, randomised, placebo-controlled phase 3 trial. Lancet Respir Med. (2020) 8(5):493–505. doi: 10.1016/S2213-2600(20)30058-8
70. Varenyiova Z, Rojas-Hernandez LS, Spano J, Capek V, Rosenberg-Hasson Y, Holmes T, et al. Azithromycin promotes proliferation, and inhibits inflammation in nasal epithelial cells in primary ciliary dyskinesia. Sci Rep. (2023) 13(1):14453. doi: 10.1038/s41598-023-41577-5
71. Elkins MR, Robinson M, Rose BR, Harbour C, Moriarty CP, Marks GB, et al. A controlled trial of long-term inhaled hypertonic saline in patients with cystic fibrosis. N Engl J Med. (2006) 354(3):229–40. doi: 10.1056/NEJMoa043900
72. Ringshausen FC, Shapiro AJ, Nielsen KG, Mazurek H, Pifferi M, Donn KH, et al. Safety and efficacy of the epithelial sodium channel blocker idrevloride in people with primary ciliary dyskinesia (CLEAN-PCD): a multinational, phase 2, randomised, double-blind, placebo-controlled crossover trial. Lancet Respir Med. (2024) 12(1):21–33. doi: 10.1016/S2213-2600(23)00226-6
73. Stafanger G, Garne S, Howitz P, Morkassel E, Koch C. The clinical effect and the effect on the ciliary motility of oral N-acetylcysteine in patients with cystic fibrosis and primary ciliary dyskinesia. Eur Respir J. (1988) 1(2):161–7. doi: 10.1183/09031936.93.01020161
74. Chang AB, Morgan LC, Duncan EL, Chatfield MD, Schultz A, Leo PJ, et al. Reducing exacerbations in children and adults with primary ciliary dyskinesia using erdosteine and/or azithromycin therapy (REPEAT trial): study protocol for a multicentre, double-blind, double-dummy, 2 × 2 partial factorial, randomised controlled trial. BMJ Open Respir Res. (2022) 9(1):e001236. doi: 10.1136/bmjresp-2022-001236
75. Hamon Y, Legowska M, Hervé V, Dallet-Choisy S, Marchand-Adam S, Vanderlynden L, et al. Neutrophilic cathepsin C is maturated by a multistep proteolytic process and secreted by activated cells during inflammatory lung diseases. J Biol Chem. (2016) 291(16):8486–99. doi: 10.1074/jbc.M115.707109
76. Chalmers JD, Haworth CS, Metersky ML, Loebinger MR, Blasi F, Sibila O, et al. Phase 2 trial of the DPP-1 inhibitor brensocatib in bronchiectasis. N Engl J Med. (2020) 383(22):2127–37. doi: 10.1056/NEJMoa2021713
77. Chalmers JD, Burgel P-R, Daley CL, De Soyza A, Haworth CS, Mauger D, et al. Brensocatib in non–cystic fibrosis bronchiectasis: ASPEN protocol/baseline characteristics. ERJ Open Res. (2024) 10(4):00151–2024. doi: 10.1183/23120541.00151-2024
78. Chalmers JD, Gupta A, Chotirmall SH, Armstrong A, Eickholz P, Hasegawa N, et al. A Phase 2 randomised study to establish efficacy, safety and dosing of a novel oral cathepsin C inhibitor, BI 1291583, in adults with bronchiectasis: Airleaf. ERJ Open Res. (2023) 9(3):00633–2022. doi: 10.1183/23120541.00633-2022
79. Chhin B, Negre D, Merrot O, Pham J, Tourneur Y, Ressnikoff D, et al. Ciliary beating recovery in deficient human airway epithelial cells after lentivirus ex vivo gene therapy. PLoS Genet. (2009) 5(3):e1000422. doi: 10.1371/journal.pgen.1000422
80. Ostrowski LE, Yin W, Patel M, Sechelski J, Rogers T, Burns K, et al. Restoring ciliary function to differentiated primary ciliary dyskinesia cells with a lentiviral vector. Gene Ther. (2014) 21(3):253–61. doi: 10.1038/gt.2013.79
81. Lai M, Pifferi M, Bush A, Piras M, Michelucci A, Di Cicco M, et al. Gene editing of DNAH11 restores normal cilia motility in primary ciliary dyskinesia. J Med Genet. (2016) 53(4):242–9. doi: 10.1136/jmedgenet-2015-103539
82. Woo CJ, Allawzi A, Clark N, Kaushal N, Efthymiou T, Thamsen M, et al. Inhaled delivery of a lipid nanoparticle encapsulated messenger RNA encoding a ciliary protein for the treatment of primary ciliary dyskinesia. Pulm Pharmacol Ther. (2022) 75:102134. doi: 10.1016/j.pupt.2022.102134
83. Hennig M, Ishimaru D, Liston DR, Eby JK, Corona CC, Casillas JE, et al. Rescue of Ciliary Function in Cell-Based Primary Ciliary Dyskinesia Models Using Nebulized, Lipid Nanoparticle (LNP)-Formulated mRNA. A62 CYSTIC FIBROSIS AND NON-CF BRONCHIECTASIS: MECHANISTIC INSIGHTS. p. A1998-A).
84. Rudolph C, Plank C, Langenickel T, Wawrzinek C. ETHRIS Pipeline (2024). Available at: https://www.ethris.com/pipeline/ (Accessed June 14, 2024).
85. Rohner E, Yang R, Foo KS, Goedel A, Chien KR. Unlocking the promise of mRNA therapeutics. Nat Biotechnol. (2022) 40(11):1586–600. doi: 10.1038/s41587-022-01491-z
86. Zhu X, Yin L, Theisen M, Zhuo J, Siddiqui S, Levy B, et al. Systemic mRNA therapy for the treatment of fabry disease: preclinical studies in wild-type mice, fabry mouse model, and wild-type non-human primates. Am J Hum Genet. (2019) 104(4):625–37. doi: 10.1016/j.ajhg.2019.02.003
87. An D, Schneller JL, Frassetto A, Liang S, Zhu X, Park JS, et al. Systemic messenger RNA therapy as a treatment for methylmalonic acidemia. Cell Rep. (2017) 21(12):3548–58. doi: 10.1016/j.celrep.2017.11.081
88. Jiang L, Park JS, Yin L, Laureano R, Jacquinet E, Yang J, et al. Dual mRNA therapy restores metabolic function in long-term studies in mice with propionic acidemia. Nat Commun. (2020) 11(1):5339. doi: 10.1038/s41467-020-19156-3
89. Rawlins EL, Hogan BL. Ciliated epithelial cell lifespan in the mouse trachea and lung. Am J Physiol Lung Cell Mol Physiol. (2008) 295(1):L231–4. doi: 10.1152/ajplung.90209.2008
90. Keiser NW, Cant E, Sitaraman S, Shoemark A, Limberis MP. Restoring ciliary function: gene therapeutics for primary ciliary dyskinesia. Hum Gene Ther. (2023) 34(17-18):821–35. doi: 10.1089/hum.2023.102
91. Cholon DM, Gentzsch M. Recent progress in translational cystic fibrosis research using precision medicine strategies. J Cyst Fibros. (2018) 17(2S):S52–60. doi: 10.1016/j.jcf.2017.09.005
92. Lee DDH, Cardinale D, Nigro E, Butler CR, Rutman A, Fassad MR, et al. Higher throughput drug screening for rare respiratory diseases: readthrough therapy in primary ciliary dyskinesia. Eur Respir J. (2021) 58(4):2000455. doi: 10.1183/13993003.00455-2020
Keywords: primary ciliary dyskinesia, acute infection, airway infection, exacerbation, bronchiectasis, airway remodeling, mucociliary clearance, microbiome
Citation: Frohlich M, Prentice B, Owens L, Waters S and Morgan L (2025) Beyond the present: current and future perspectives on the role of infections in pediatric PCD. Front. Pediatr. 13:1564156. doi: 10.3389/fped.2025.1564156
Received: 21 January 2025; Accepted: 4 March 2025;
Published: 18 March 2025.
Edited by:
Ron Rubenstein, Washington University in St. Louis, United StatesReviewed by:
Katherine Carr, Washington University in St. Louis, United StatesThomas Saba, University of Michigan, United States
Copyright: © 2025 Frohlich, Prentice, Owens, Waters and Morgan. This is an open-access article distributed under the terms of the Creative Commons Attribution License (CC BY). The use, distribution or reproduction in other forums is permitted, provided the original author(s) and the copyright owner(s) are credited and that the original publication in this journal is cited, in accordance with accepted academic practice. No use, distribution or reproduction is permitted which does not comply with these terms.
*Correspondence: Megan Frohlich, TWVnYW4uRnJvaGxpY2gxQGhlYWx0aC5uc3cuZ292LmF1