- 1Department of Nephrology, Guangdong Provincial Hospital of Chinese Medicine, The Second Affiliated Hospital of Guangzhou University of Chinese Medicine, Guangzhou, China
- 2Department of Pharmacology, School of Pharmaceutical Sciences, Guangzhou University of Chinese Medicine, Guangzhou, China
Kidney injuries may trigger renal fibrosis and lead to chronic kidney disease (CKD), but effective therapeutic strategies are still limited. Quercetin is a natural flavonoid widely distributed in herbal medicines. A large number of studies have demonstrated that quercetin may protect kidneys by alleviating renal toxicity, apoptosis, fibrosis and inflammation in a variety of kidney diseases. Therefore, quercetin could be one of the promising drugs in the treatment of renal disorders. In the present study, we review the latest progress and highlight the beneficial role of quercetin in kidney diseases and its underlying mechanisms. The pharmacokinetics and bioavailability of quercetin and its proportion in herbal medicine will also be discussed.
1 Introduction
Kidney diseases are one of the life-threatening diseases with high mortality rates (Li et al., 2021b). Renal injuries could be triggered by various insults such as nephrotoxins, oxidative stress, or inflammation. These pathogenic factors act as the major driving force to promote renal injuries towards fibrosis (Gu et al., 2020b), which may eventually lead to chronic kidney disease (CKD) or end-stage renal disease (ESRD). To date, the effective drugs and therapeutic strategies for renal injury are still limited.
Natural products have been used in the clinical management of the renal disease. The constituent compounds of herbal medicine receive considerable attention in experimental models of kidney disease both in vivo and in vitro (Chen et al., 2018). Quercetin is one of the most abundant flavonoids present in natural plants. Due to its antioxidative, anti-hypertensive, and anti-diabetic effects, quercetin has been suggested as an effective flavonoid that plays a beneficial role in the treatment of cancer, cardiovascular disease, and metabolic disease (Sok Yen et al., 2021).
Although quercetin has been studied in many studies, we could not locate a recent overview of quercetin’s action in kidney diseases. In the present review, we discuss and explore the biological effects of quercetin on kidney injuries such as nephrotoxicity, renal inflammation, fibrosis, hyperglycemia damage, and oxidative stress. We also identified the pathogenic mechanisms of renal disease and focused on the signaling pathways that are potentially associated with quercetin treatment.
2 Pharmacokinetics and bioavailability of quercetin
Quercetin, also known as 3,5,7,3′,4′-pentahydroxyflavone, is a natural flavonoid compound. In nature, it exists in various forms in different plants and can be found as either quercetin aglycone or derivatives, while the most abundant form in the diet is glycosides (Owumi et al., 2019). Quercetin is highly soluble in lipids and alcohol. Due to its hydrophobicity, quercetin has relatively poor solubility in water (0.17–7 μg/ml), gastric fluids (5.5 μg/ml) and small intestine fluids (28.9 μg/ml), which have reduced its bio-accessibility (Bağdatlıoğlu, 2016). Quercetin aglycone exhibit a poor oral bioavailability of about 2%. However, depending on different radicals bound to the quercetin aglycone backbone, the solubility and biochemical activity of quercetin derivatives vary. The glycoside is much more soluble compared to aglycone, as the glycosyl group increases the water solubility. After the intake of quercetin-rich supplements in human bodies, quercetin quickly disappeared in the body with a 1–2 h removal half-life (Graefe et al., 1999).
Quercetin can be transported through sodium-dependent glucose transporter 1(SGLT1). The process begins with the hydrolysis of quercetin glycosides by lactase phloridzin hydrolase (LPH) and intracellular ß-Glucosidases and releases quercetin aglycone. Quercetin aglycone is then primarily metabolized in the gastrointestinal tract (Graf et al., 2006). In enterocytes, the biotransformation of quercetin includes glucuronidation by UDP-glucuronyltransferases (UGT), methylation by catechol O-methyltransferases (COMT), and sulfation by sulfotransferases (SULT). 3-O-glucuronide and quercetin 3′-O-sulfate are the two main metabolites passing from the enterocyte and diffusing into the hepatic portal vein to undergo a second transformation in the liver before returning to the bloodstream (Figure 1). Afterward, about 80% of aglycone or metabolites are bound to plasma albumin and the remaining 20% free form can enter the tissues, therefore the aglycone amount is relatively low in the blood. The metabolism process takes place in the intestines, liver and kidneys and the accumulation tends to happen in those organs.
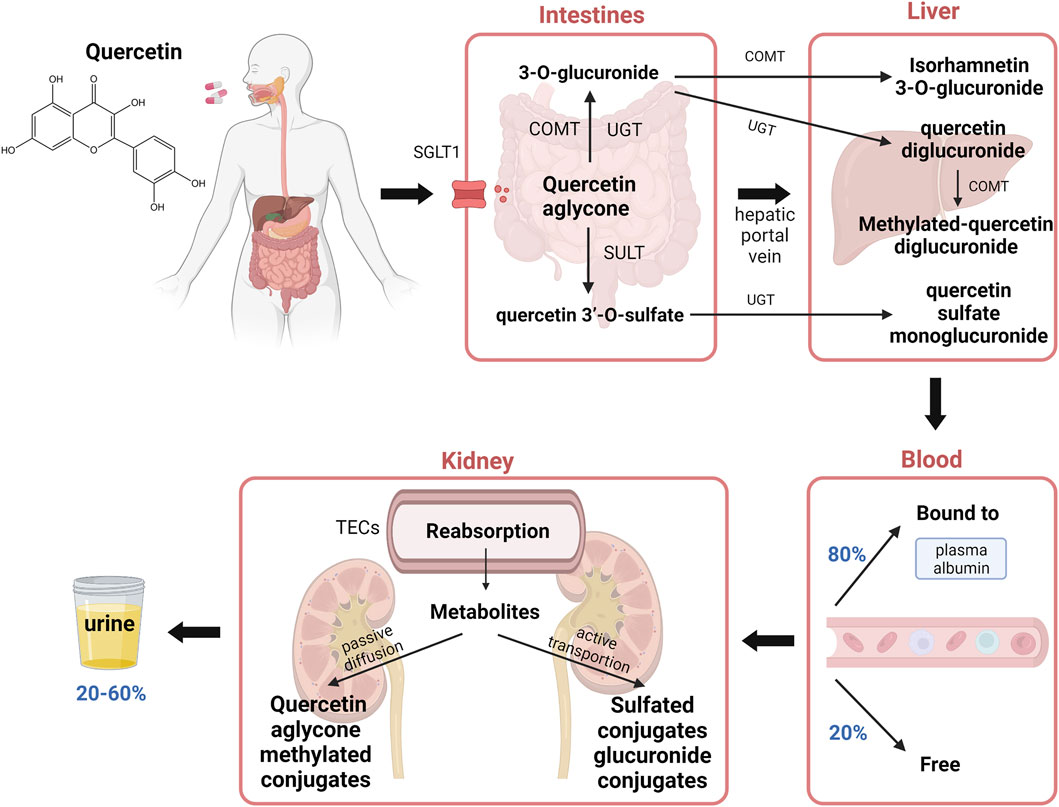
FIGURE 1. Chemical structure of quercetin and its pharmacokinetics in the body. Quercetin is transported by SGLT1, the process begins with hydrolysis in the gastrointestinal tract and releases quercetin aglycone. Biotransformation reactions include glucuronidation, methylation, and sulfation of quercetin aglycone are catalyzed by UGT, COMT, and SULT, respectively. Main metabolites such as 3-O-glucuronide and quercetin 3′-O-sulfate undergo a second transformation in the liver and 80% of the metabolites are bound to plasma albumin and the remaining 20% are free to enter tissues. The metabolites are reabsorbed in the TECs and enter cells by passive diffusion or active transportation. Up to 20%–60% of the quercetin intake may be secreted into the urine. Abbreviations: SGLT1, sodium-dependent glucose transporter 1; UGT, UDP-glucuronyltransferases; COMT, catechol O-methyltransferases; SULT, sulfotransferases; TECs, tubular epithelial cells.
In the kidney, the metabolites from the plasma go through the glomerular filtration process, followed by dispersion into the tubular. The metabolites are partially reabsorbed by tubular epithelial cells (TECs), and the remaining part passes into the urine. The transportation to proximal TECs primarily occurs in the basolateral membrane and apical membrane (Wong et al., 2011). Quercetin aglycone and methylated conjugates across the basolateral membrane by passive diffusion, while sulfated conjugates and glucuronide conjugates use active transport to enter the cells, due to their high affinity for organic anion transporters (OATs). The metabolites are formed in the tubular cells and secreted into the urine. In the human body, the quercetin metabolites excreted through urine take up 20%–60% of total quercetin intake and they are mainly composed of monoglucuronide sulfates, methylated quercetin monoglucuronides, and quercetin diglucuronide (Graf et al., 2006; Mullen et al., 2006).
Previous experiments and studies have revealed the pharmacodynamics of quercetin, it is found that due to its chemical structure, it has low water solubility, oral absorption rate, rapid elimination, and low bioavailability (Diniz et al., 2020). Such characteristics greatly hindered the application of quercetin in pre-employment drug testing and clinical practice (Heeba and Mahmoud, 2016). Casanova et al. (2021) encapsulated quercetin with Pluronic F127 to make micelles and found that it had higher water solubility with good bioavailability, and the protective effect on the kidney had been greatly improved (Gu et al., 2020a). Although more in-depth drug experiments and clinical trials are needed, it is believed that the utilization of quercetin can be improved in the future.
3 Quercetin in traditional herbal medicines
Except for various food and supplements, quercetin is widely abundant in flowers, leaves and fruits of plants. It was determined in nearly 200 kinds of traditional Chinese herbal medicines, such as Sophora japonicum, Radix Bupleuri (Sen-ming, 2013), Gynostemmae Pentaphylli Herba (Conglei Pan, 2019).
High-performance liquid chromatography (HPLC) was mainly used to determine the content of quercetin in herbal medicine. As shown in Table 1, the content of quercetin in different species can vary from less than 1 mg/g to more than 300 mg/g. According to the theory of traditional Chinese medicine (TCM), the efficacy of these herbal medicines containing quercetin can be summarized as follows: 1) heat clearing: the heat described in TCM is somehow related to the inflammatory response in the body and due to quercetin’s significant antioxidant property, it can relieve pain and inflammation. For instance, stranguria is a common urologic disease that is considered to cause by excessive damp heat in the lower energizer. In clinical practice, Houttuyniae cordata (Arky Jane Langstieh et al., 2021), Pyrrosiae lingua (Chen Junhua et al., 2014), and Centella asiatica (Mohammad Azmin and Mat Nor, 2020) are frequently selected, which contained 315.8 mg/g, 234.6 mg/g, and 77.6 mg/g quercetin, respectively. Other representative herbal medicines with heat-clearing effect include Mori follum, Sophora japonica L., and Fallopia multiflora Herba (Vetrova et al., 2017), and Fallopia multiflora Herba (Bao Lidao et al., 2015); 2) urination promotion, swelling, or edema reduction: by dilating the renal arteries, quercetin can increase blood and urine volume, therefore alleviating the edema. The common ingredients are Ephedra Herba (Saida Ibragic, 2015), Hedysarum Multijugum Maxim (Fu Juan and Huang, 2013), and Plantaginis Semen (Cao Xuesong and Huang, 2019); 3) promoting kidney recovery: other herbal medicines also exert a nourishing and strengthening effect on the kidney, such as Lycii Fructus (Kim Le and Ng, 2007), Herba Taxilli (Zhu Kaixin et al., 2011), and Rubi Fructus (Zhang Jing and Yan, 2020). Crataegi Folium (Deng Ting et al., 2021) (12.73 mg/g) was reported to attain a cardiovascular protection effect by lowering blood lipid, while the contents of quercetin in Ginkgo Folium (Qiu et al., 2017) and Inulae Flos (Hongmei, 2008) are relatively low (less than 1 mg/g).
4 Renal protective effects of quercetin in kidney disease
4.1 Nephrotoxicity
When exposed to certain toxic substances or harmful pollution for a long period, one may occur nephrotoxicity. Due to the special biological structure and physiological role, the kidneys are important organs for drug metabolism and are susceptible to toxins including antineoplastics, antibiotics and many kinds of agents. As summarized in Table 2, many experimental studies and mechanism exploration of multifaceted signal transduction and pathways suggest that quercetin has great potential in reducing renal toxicity.
Antineoplastic agents such as cisplatin (Li et al., 2016a), methotrexate (Erboga et al., 2015), doxorubicin (Heeba and Mahmoud, 2016), and cyclophosphamide may cause side effects in clinical treatment due to dose-related nephrotoxicity. The nephroprotective effect of quercetin against cisplatin-induced oxidative stress was demonstrated by Almaghrabi (2015). In cisplatin-treated rats, quercetin can reduce tubular injury, downregulate the pro-inflammatory mediators and maintain renal blood flow. Moreover, quercetin also exhibited antioxidant and anti-apoptotic effects, therefore reducing the apoptosis of non-tumor cells caused by cisplatin treatment (Almaghrabi, 2015; Casanova et al., 2021). It is worth mentioning that quercetin did not interfere with the antitumor activity of cisplatin (Sánchez-González et al., 2017). Furthermore, experimental results have shown that quercetin may enhance the activity of cisplatin against cancer (Li et al., 2016a). Likewise, quercetin may protect against cyclophosphamide-induced hepatic and renal injury by immunosuppressing the IDO/TDO pathway (Ebokaiwe et al., 2021). It is hypothesized that this effect may be due to the combination of quercetin’s ability to scavenge reactive oxygen species (ROS) and inhibition of malondialdehyde (MDA) formation. The production of free radicals and ROS are key triggers for the activation of Nrf2 (nuclear factor erythroid 2-related factor 2) and HO-1 (renal heme oxygenase 1). Regarding nephrotoxicity, Nrf2/HO-1 pathway may play an important role in boosting the GSH, GPx, and SOD antioxidant moieties (Arab et al., 2021). Quercetin supplementation could markedly activate the mRNA expression of Nrf2 and HO-1 in copper sulfate-induced renal injury mice (Peng et al., 2020).
Dosage is of great importance in the understanding of the pharmacological effects of quercetin. Of note, evidence also support that when applied with high dose, such as 100 mg/kg/d, quercetin did not show significant improvement in renal function or protection against doxorubicin-induced renal injury (Heeba and Mahmoud, 2016). Nevertheless, quercetin protects kidneys against antineoplastic drugs through the inhibition of inflammatory response, enhancement of the antioxidant system, and exertion of anti-apoptotic effects.
Oral pretreatment of quercetin in rats with gentamicin-induced renal injury (50 mg/kg) for 10 days revealed an improvement in renal injury. The mechanisms of the protective effect of quercetin could be the rebalancing of the antioxidant system and the modulation of renal biomarkers (Rahdar et al., 2021). A study reported by Dallak et al. (2020) showed that toxic doses of acetaminophen formed severe damage to glomerular ultrastructural compartments after 24 h, and apoptosis was observed in renal tissues. Pretreatment with resveratrol and quercetin exerted a protective effect, namely the reduction of p53 expression in the renal tissue, as well as the decrease of blood urea, creatinine, and oxidative biomarkers.
In addition, quercetin also exerts renoprotective effects on antiretroviral combination therapy involving multiple drugs. Valproic acid (VPA) is widely used to intervene in epilepsy and control multiple seizures. It was deduced from experimental studies that the effectiveness of quercetin in protecting against VPA-induced kidney injury and toxicity relies on its ability to scavenge free radicals and alter antioxidant status (Chaudhary et al., 2015). Quercetin showed the potential to improve kidney damage caused by cArt through inhibiting oxidative stress and inflammatory processes. As a result, quercetin participates in the scavenging of toxins, improves the cellular structure of organs and maintains normal biological chemical activity (Gu et al., 2020a).
Specifically, with the development of industrial technology and the progress of science and technology, the damage of chemical raw materials to the kidney has grown immensely prominent. Data from animal models have shown that the protective effect of quercetin is closely related to the clearance of free radicals and reduction of lipid peroxidation in both industrial chemical raw materials and heavy metal and diesel particulate pollution (Li et al., 2016b; Qi et al., 2017; Uthra et al., 2017; Alshanwani et al., 2020; Morsi et al., 2022). Moreover, Quercetin was also found to reduce organophosphorus pesticide mixture-induced nephrotoxicity by regulating fatty acid, energy and sex hormone metabolism, protecting antioxidant defense systems and reducing DNA damage (Qi et al., 2017). Quercetin may regulate the metabolism of phospholipids, energy, fatty acids and amino acids to protect the kidney against acrylamide-induced nephrotoxicity (Bao et al., 2017; Bo et al., 2018). All these findings have shown that quercetin can produce significant protective effects in alleviating nephrotoxicity and renal insults caused by drug treatments (Figure 2).
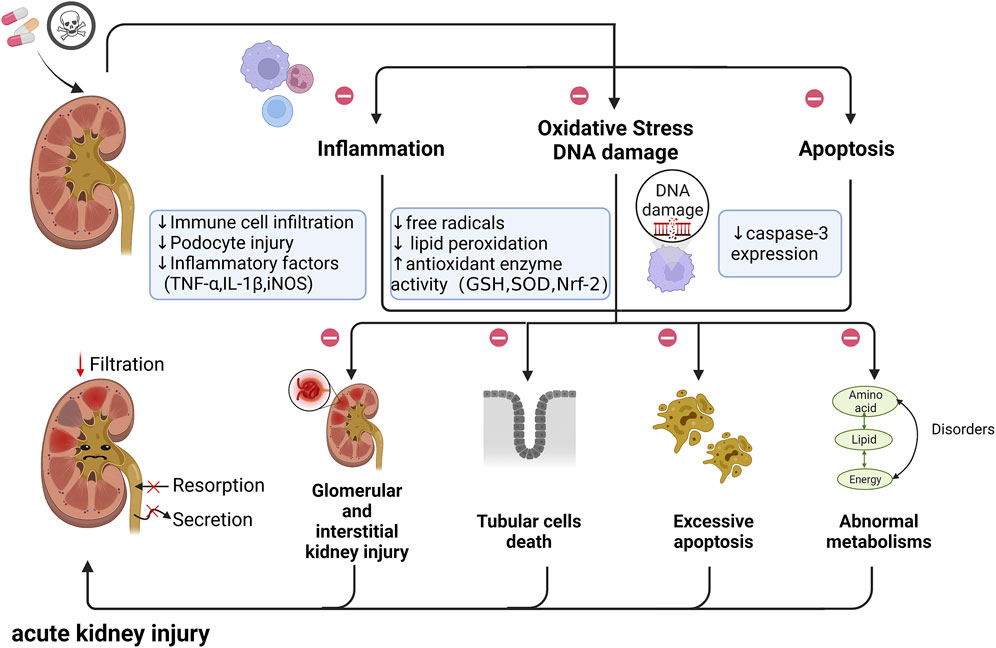
FIGURE 2. The potential role of renal toxins in the pathogenesis of AKI. Nephrotoxins may cause glomerular and interstitial kidney injury, tubular cell necrosis, and excessive apoptosis. In addition, the normal metabolism of the body can also be disrupted. This contributes to the reduction of kidney filtration and the impaired function of reabsorption and secretion, and eventually leads to acute kidney injury. (㊀ indicates therapeutic targets of quercetin; ↑ and ↓ indicates the regulatory role of quercetin on pathogenic changes); Abbreviations: TNF-α, tumor necrosis factor α; IL-1β, interleukin 1β; iNOS, inducible nitric oxide synthase; GSH, glutathione; SOD, superoxide dismutase; Nrf-2,Nuclear factor erythroid 2-related factor 2.
4.2 Acute and chronic renal injury
4.2.1 Acute kidney injury
Injuries from mesangial cells, endothelial cells (ECs), podocytes, TECs, and inflammatory cells could also lead to glomerular and interstitial fibrosis. Unresolved renal inflammation could also trigger cell apoptosis and fibrosis by releasing pro-apoptotic, pro-fibrotic growth factors, cytokines, and chemokines (Gu et al., 2021a).
Cell apoptosis and glomerular injuries are observed during renal ischemia. Quercetin can effectively prevent glomerular loss caused by renal hypochlorous ischemia (Gonçalves et al., 2021). The pathogenesis of renal ischemia/reperfusion injury (IRI) involves oxidative stress responses in the kidneys and distal organs, and the antioxidant effect of quercetin can prevent partial IRI (Gholampour and Sadidi, 2018). Regarding apoptosis, iron apoptosis is the iron-dependent regulatory necrosis that contributes to the progression of acute kidney injury (AKI), quercetin inhibits iron apoptosis in proximal renal TECs, thereby reducing AKI (Wang et al., 2021).
Carvedilol can relieve AKI caused by renal IRI and quercetin restores renal function by reducing inflammation (Rezk et al., 2021). Quercetin may also prevent AKI by regulating Mincle/Syk/NF-κB signaling to inhibit macrophage inflammation (Tan et al., 2020). Quercetin improves kidney damage by regulating macrophage polarization (Lu et al., 2018). Lipopolysaccharide (LPS) induces AKI in mice, and quercetin pretreatment protects mice from LPS-induced renal inflammation by inhibiting the TLR4/NF-κB signaling pathway (Tan et al., 2019). Quercetin may prevent sepsis-associated AKI by inhibiting NF-κB activation and upregulating Sirt1 expression (Lu et al., 2021). Besides, CD38 plays an important role in macrophage activation during sepsis-induced AKI. In the LPS-induced AKI mouse model, quercetin induces the blockade of CD38, thus significantly alleviating renal dysfunction and the infiltration of inflammatory cells (Shu et al., 2018).
Interestingly, as kidneys are one of the targets of SARS-CoV-2, up to 36% of SARS-CoV-2-infected patients develop AKI. COVID-19-induced inflammation is closely associated with AKI. Quercetin restores renal function by inhibiting the inflammatory and apoptosis-related signaling pathways (Gu et al., 2021b). Quercetin may potentially target SARS-CoV-2 3Clpro, which might inhibit the invasion of coronavirus, the life-threatening inflammation and cytokines storm in AKI (Diniz et al., 2020).
4.2.2 Chronic kidney injury and renal fibrosis
One of the notable pathological characteristics of CKD is renal fibrosis, a prolonged wound-healing process that responds to multiple tissue injuries in the kidney. This process is characterized by glomerulosclerosis, tubular atrophy, and interstitial fibrosis. Studies have shown that renal fibrosis could be triggered by chronic inflammation. Renal injuries promote the recruitment of inflammatory cells and the release of related cytokines, chemokines, and ROS. This inflammatory process eventually activates fibroblasts and promotes the synthesis and accumulation of extracellular matrix (ECM) proteins. It is demonstrated that quercetin alleviated inflammation by upregulating the miR-124/NF-κB pathway in LPS-stimulated TECs (Guo et al., 2020). Of note, quercetin can also reduce macrophage accumulation and the expression of inflammatory cytokines in the kidneys of obstructive, therefore inhibiting renal fibrosis (Ren et al., 2016).
Transforming growth factor beta (TGF-β) is a major cytokine that promotes ECM accumulation. It may also induce the apoptosis of podocytes and promote epithelial to mesenchymal transition (EMT) progression (Arauz et al., 2015). One study has demonstrated that quercetin downregulated TGF-β signaling and reduced the expression of EMT-related proteins to halt the progression of glomerulosclerosis (Liu et al., 2019b). Other studies have also suggested that quercetin suppressed TGF-β signaling via Sonic Hedgehog, PTEN/TIMP3 and PI3k/Akt signaling pathways (Cao et al., 2018; Liu et al., 2019a; Tu et al., 2021). All the studies have suggested the anti-fibrotic role of quercetin in chronic kidney injury.
4.3 Diabetic nephropathy
4.3.1 Anti-hyperglycemic effect
As shown in Figure 3, the regulatory roles of the signaling pathways involved in diabetic nephropathy (DN) are complex. Both hyperglycemia and dyslipidemia can induce structural and functional damage in diabetic kidneys (Sun et al., 2019). On one hand, quercetin decreases blood glucose levels by increasing the release of insulin while reducing hepatic glucose production. Mechanistically, quercetin may also enhance glucose uptake by regulating the expression and function of GLUT4 and the insulin receptor beta subunit (Ali et al., 2020).
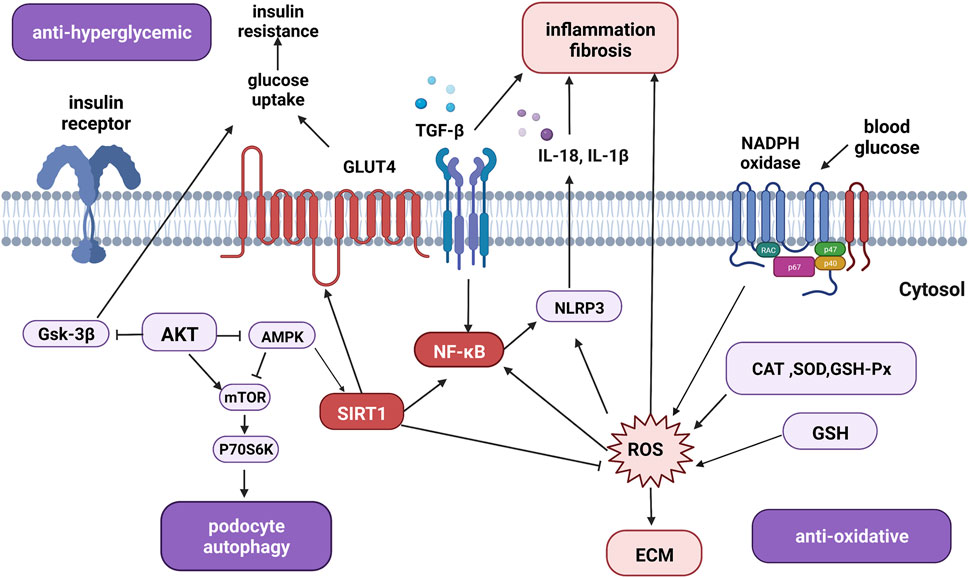
FIGURE 3. The overview of signaling pathways and therapeutic targets of quercetin in the treatment of diabetic nephropathy. Quercetin acts as an anti-hyperglycemic agent by regulating glucose-related signaling pathways. Quercetin also targets fibrotic, inflammatory, and oxidative mediators such as TGF-β, SIRT1, AKT, and NF-κB to inhibit inflammation, fibrosis, oxidative stress, apoptosis, and promote autophagy to exert renal protective effects. Abbreviations: Gsk-3β, glycogen synthase kinase-3; AKT, protein kinase B; AMPK, AMP-activated protein kinase; mTOR, mammalian target of rapamycin; P70S6K, 70-kDa ribosomal protein S6 kinase; GLUT4, glucose transporter protein type-4; TGF-β, transforming growth factor beta; NF-κB, nuclear factor κ-light-chain-enhancer of activated B cells; SIRT1, silent information regulator 1; NLRP3, NLR family pyrin domain containing 3; CAT, catalase; SOD, superoxide dismutase; GSH-Px, glutathione peroxidase; GSH, glutathione; ROS, reactive oxygen species; ECM, extracellular matrix; IL-18, interleukin-18; IL-1β, interleukin-1β; (Figure created with BioRender.com).
On the other, hyperglycemia also induces metabolic alterations, resulting in the disturbance of protein, fat, and carbohydrate metabolism. Disorders of these metabolites also increase the burden on the diabetic kidneys. Quercetin at a dosage of 10 mg/kg/d can reduce blood glucose and triglycerides serum levels (Gomes et al., 2015). Likewise, at the early stage of DN, 50 or 100 mg/kg/d quercetin could improve lipid metabolism by alleviating albuminuria and renal function. In terms of lipid metabolism, quercetin reduces serum cholesterol, and triglycerides, and increases low-density lipoprotein cholesterol through the SCAP-SREBP2-LDLr signaling pathway in the diabetic rat model (Jiang et al., 2019).
4.3.2 Anti-oxidative effect
The excessive expression of intracellular ROS is one of the significant changes in DN. ROS induces the activity of apoptosis-related enzymes, causing damage to the podocytes and promoting the proliferation of fibrotic cells to induce the synthesis of ECM (Ma et al., 2018). These processes result in renal fibrosis and inflammation and turn out to the progression of DN. Quercetin has acted as a free radical scavenger in DN animal models. For instance, one study measured the antioxidant-related enzymes and histopathological changes in kidneys and found that quercetin alleviated the damage by preventing oxidative stress (Elbe et al., 2015). As reported by other studies, dihydro quercetin exerts a renal protective effect on DN rats at the dose of 100 mg/kg/day, with the downregulated expression of ROS-related proteins and NLRP3 inflammasome (Ding et al., 2018). Besides, another study has revealed that both quercetin and quercetin-nanoparticle complex reduced structural damage to the kidney, improved renal function and alleviated oxidative stress by downregulating the expression of ICAM-1 (Tong et al., 2017).
4.3.3 Autophagy promotion
Autophagy plays a crucial role in the intracellular degradation system for cellular homeostasis. As for kidney diseases, autophagy may protect functions in both glomerular and tubular compartments by suppressing excessive inflammation and fibrosis in AKI, CKD, and DN (Kimura et al., 2017; Bhatia and Choi, 2020). For example, hyperglycemia induces the dysregulation of autophagy in major types of resident kidney cells, mainly the impairment of podocytes. Autophagy is primarily regulated by signaling pathways such as the serine/threonine protein kinase mammalian target of rapamycin (mTOR), AMP activated protein kinase (AMPK), and sirtuins. High glucose can inhibit podocyte autophagy through AMPK pathway (Platé et al., 2020) and activate the mTOR signaling to inhibit podocyte autophagy. Evidence has suggested a quercetin-rich fruit, guava, is able to protect against type 2 diabetes mellitus-induced renal and pancreatic dysfunction by preventing cell apoptosis, autophagy, and pyroptosis (Lin et al., 2016). More studies have also demonstrated quercetin regulating blood glucose/lipid levels and improving renal fibrosis, potential mechanisms could be the modulation of the AMPK-dependent autophagy process, inhibition of mTORC1/p70S6K signaling, or the activation of Hippo pathways in vitro and in vivo (Lu et al., 2015; Lei et al., 2019; Lai et al., 2021). Further studies should focus on the glycemic regulating role and underlying mechanisms of quercetin treatment on DN.
4.4 Senolytic therapy for kidney disease
As clinical interest in kidney aging rapidly arises, the progression of cellular senescence relates closely to the stable cell cycle arrest. The accumulation of renal senescent cells (SCs) promotes inflammation and fibrosis, leading to multiple kidney disorders. The senolytics are a class of drugs that may selectively clear SCs. Quercetin, together with dasatinib, acts as the novel pharmacological senolytic agent for a number of kidney diseases (Kirkland and Tchkonia, 2020).
Senescent TECs are the driving force in renal fibrosis progression, which may activate fibroblasts. The combination of quercetin and dasatinib may specifically induce apoptosis of senescent TECs, therefore restoring renal function and ameliorating fibrosis (Li et al., 2021a). Another study has also shown that the combination of quercetin and dasatinib can alleviate renal insufficiency and damage in animal models of renal ischemia. In vivo study has revealed that senolytic therapy of quercetin and dasatinib improved renal artery stenosis by reducing the p21 positive stenotic TECs and attenuating mesenchymal transition (Kim et al., 2021). Notably, obesity could promote cellular senescence and impair renal function. Researchers have found an increased expression of renal markers of senescence, such as p16, p19, and p53, in a high-fat-diet-induced mouse model. Renal function and fibrosis are improved in quercetin-treated mice (Kim et al., 2019). Similarly, an open-label Phase 1 pilot study (NCT02848131) in patients with diabetic kidney disease showed that the combination of quercetin and dasatinib can eliminate senescent cells and significantly reduce senescent cell burden in adipose and skin tissues within 11 days. The possible mechanisms of their protective effects may be associated with the decrease of p16-, p21 expressing cells and the downregulation of senescence-associated secretory phenotype (including the expression of pro-inflammatory cytokines IL-6, IL-1α, and MMP-9) (Hickson et al., 2019). More explorations are needed to investigate the mechanism of analytic therapy and verify its efficacy and safety. The combination of quercetin and dasatinib could serve as new therapeutic agents to hinder renal senescence.
4.5 Other renal disorders
Dietary intake of the flavonoid quercetin has been proven effective in lowering blood pressure and restoring endothelial dysfunction in animal models of hypertension. Quercetin intake improves endothelium-dependent relaxation and inhibits α1-adrenoceptor mediated contractions in aortic rings from hypertensive rats. In addition, quercetin treatment in high dose promotes a significant reduction in blood pressure in spontaneously hypertensive rats compared to the control group (Choi et al., 2016; Elbarbry et al., 2020). These data not only demonstrate the anti-hypertensive effect of quercetin but also provide evidence for its role as a novel cardioprotective compound.
Renal cell carcinoma (RCC) has become a common subtype of kidney cancer, which has the highest propensity to manifest as metastatic disease. We lack knowledge of the correlation between migration and invasion in RCC, thus few therapeutic options are available (Meng et al., 2015). Intriguingly, recent studies have found that quercetin has anti-tumor effects against diverse types of cancers via multiple signaling pathways (Zhu et al., 2018). For example, a study explored the anti-tumoral effect of a potential chemopreventive effect of quercetin, the combination of quercetin and anti-sense oligo gene therapy provides stronger suppressive effects on RCC cells rather than a solo treatment. These studies have provided the possibility of quercetin as a novel treatment for renal cancer (Meng et al., 2015).
Autosomal dominant polycystic kidney disease (ADPKD) is a monogenic disease characterized by the massive enlargement of fluid-filled cysts in the kidney. One study has found that quercetin dramatically inhibited the formation and growth of the cyst, suggesting that quercetin could hinder renal cyst progression and should be represented as a novel candidate strategy for the treatment of ADPKD (Zhu et al., 2018). Nevertheless, speaking of kidney stones, quercetin also reduces the reabsorption of sodium, calcium, and water, thereby preventing the formation of a kidney stone in the urinary tract (Nirumand et al., 2018).
5 Conclusion and future perspectives
Quercetin, an active compound from natural products, has shown a significant protective effect in various models of kidney diseases. However, most of the studies have reported observational results and phenotype changes rather than the mechanisms of action related to the crucial pathogenesis. Besides, although experimental research has focused on the therapeutic effects and mechanisms of quercetin, it could hardly be used in the clinical setting due to its poor solubility and low oral bioavailability. Nevertheless, further research on nanoparticles, liposomes, micelles, or novel materials is in urgent need to improve the drug delivery system of quercetin and bring this natural compound to the forefront of therapeutic agents for the treatment of kidney disease.
Author contributions
Y-QC, H-YC, and Q-QT contributed equally to writing and revising the manuscript. Y-FL wrote and checked the manuscript. F-HL and X-SL supervised and conducted the study. Y-YG conducted, wrote, and revised the manuscript. All authors contributed to the manuscript conception development and discussed the manuscript writing and revising.
Funding
This work was supported by the National Natural Science Foundation of China (No. 81903956), the China Postdoctoral Science Foundation (2021M690042, 2022T150148), the Basic and Applied Basic Research Project of Guangzhou Science and Technology Department (202102020011) and the Research Fund for Bajian Talents of Guangdong Provincial Hospital of Chinese Medicine (No. BJ2022KY03).
Conflict of interest
The authors declare that the research was conducted in the absence of any commercial or financial relationships that could be construed as a potential conflict of interest.
Publisher’s note
All claims expressed in this article are solely those of the authors and do not necessarily represent those of their affiliated organizations, or those of the publisher, the editors and the reviewers. Any product that may be evaluated in this article, or claim that may be made by its manufacturer, is not guaranteed or endorsed by the publisher.
References
Abdel-Wahhab, M. A., Aljawish, A., El-Nekeety, A. A., Abdel-Aziem, S. H., and Hassan, N. S. (2017). Chitosan nanoparticles plus quercetin suppress the oxidative stress, modulate DNA fragmentation and gene expression in the kidney of rats fed ochratoxin A-contaminated diet. Food Chem. Toxicol. 99, 209–221. doi:10.1016/j.fct.2016.12.002
Abdelhalim, M. A. K., Qaid, H. A., Al-Mohy, Y., and Al-Ayed, M. S. (2018). Effects of quercetin and arginine on the nephrotoxicity and lipid peroxidation induced by gold nanoparticles in vivo. Int. J. Nanomedicine 13, 7765–7770. doi:10.2147/ijn.S183281
Al-Asmari, A. K., Khan, H. A., Manthiri, R. A., Al-Khlaiwi, A. A., Al-Asmari, B. A., and Ibrahim, K. E. (2018). Protective effects of a natural herbal compound quercetin against snake venom-induced hepatic and renal toxicities in rats. Food Chem. Toxicol. 118, 105–110. doi:10.1016/j.fct.2018.05.016
Ali, A. M., Gabbar, M. A., Abdel-Twab, S. M., Fahmy, E. M., Ebaid, H., Alhazza, I. M., et al. (2020). Antidiabetic potency, antioxidant effects, and mode of actions of citrus reticulata fruit peel hydroethanolic extract, hesperidin, and quercetin in nicotinamide/streptozotocin-induced wistar diabetic rats. Oxid. Med. Cell. Longev. 2020, 1730492. doi:10.1155/2020/1730492
Alidadi, H., Khorsandi, L., and Shirani, M. (2018). Effects of quercetin on tubular cell apoptosis and kidney damage in rats induced by titanium dioxide nanoparticles. Malays. J. Med. Sci. 25 (2), 72–81. doi:10.21315/mjms2018.25.2.8
Almaghrabi, O. A. (2015). Molecular and biochemical investigations on the effect of quercetin on oxidative stress induced by cisplatin in rat kidney. Saudi J. Biol. Sci. 22 (2), 227–231. doi:10.1016/j.sjbs.2014.12.008
Alshanwani, A. R., Shaheen, S., Faddah, L. M., Alhusaini, A. M., Ali, H. M., Hasan, I., et al. (2020). Manipulation of quercetin and melatonin in the down-regulation of HIF-1α, HSP-70 and VEGF pathways in rat's kidneys induced by hypoxic stress. Dose. Response. 18 (3), 1559325820949797. doi:10.1177/1559325820949797
Andreucci, M., Faga, T., Pisani, A., Serra, R., Russo, D., De Sarro, G., et al. (2018). Quercetin protects against radiocontrast medium toxicity in human renal proximal tubular cells. J. Cell. Physiol. 233 (5), 4116–4125. doi:10.1002/jcp.26213
Arab, H. H., Eid, A. H., Gad, A. M., Yahia, R., Mahmoud, A. M., and Kabel, A. M. (2021). Inhibition of oxidative stress and apoptosis by camel milk mitigates cyclosporine-induced nephrotoxicity: Targeting Nrf2/HO-1 and AKT/eNOS/NO pathways. Food Sci. Nutr. 9 (6), 3177–3190. doi:10.1002/fsn3.2277
Arauz, J., Rivera-Espinoza, Y., Shibayama, M., Favari, L., Flores-Beltrán, R. E., and Muriel, P. (2015). Nicotinic acid prevents experimental liver fibrosis by attenuating the prooxidant process. Int. Immunopharmacol. 28 (1), 244–251. doi:10.1016/j.intimp.2015.05.045
Arky Jane Langstieh, J. B. W., Jane Lyngdoh, C., Jaba, I., Sarkar, C., and Brahma, D. K. (2021). Estimation of quercetin and rutin content in hyouttunia cordata and Centella asiatica plant extracts using UV-spectrophotometer. Int. J. Pharm. Sci. Rev. Res. 71 (2), 130–132. doi:10.47583/ijpsrr.2021.v71i02.021
Bağdatlıoğlu, M. B. K. (2016). Bioavailability of quercetin. Curr. Res. Nutr. Food Sci. 4, 1. doi:10.12944/CRNFSJ.4.Special-Issue-October.20
Bao Lidao, D. H., Zhao, Y., Wu, L., and Song, J. (2015). Simultaneous determination of four active components in polygonum aviculare L by HPLC. Liaoning J. Traditional Chin. Med. 43 (06), 1266–1268.
Bao, W., Cao, C., Li, S., Bo, L., Zhang, M., Zhao, X., et al. (2017). Metabonomic analysis of quercetin against the toxicity of acrylamide in rat urine. Food Funct. 8 (3), 1204–1214. doi:10.1039/c6fo01553k
Bhatia, D., and Choi, M. E. (2020). Autophagy in kidney disease: Advances and therapeutic potential. Prog. Mol. Biol. Transl. Sci. 172, 107–133. doi:10.1016/bs.pmbts.2020.01.008
Bo, L., Liu, Y., Jia, S., Liu, Y., Zhang, M., Li, S., et al. (2018). Metabonomics analysis of quercetin against the nephrotoxicity of acrylamide in rats. Food Funct. 9 (11), 5965–5974. doi:10.1039/c8fo00902c
Cao Xuesong, W. J., and Huang, Z. (2019). Determination of quercetin, kaempferol, luteolin, apigenin in semen Plantaginis by RP-HPLC. China Pharm. 18 (12), 32–34.
Cao, Y., Hu, J., Sui, J., Jiang, L., Cong, Y., and Ren, G. (2018). Quercetin is able to alleviate TGF-β-induced fibrosis in renal tubular epithelial cells by suppressing miR-21. Exp. Ther. Med. 16 (3), 2442–2448. doi:10.3892/etm.2018.6489
Casanova, A. G., Prieto, M., Colino, C. I., Gutiérrez-Millán, C., Ruszkowska-Ciastek, B., de Paz, E., et al. (2021). A micellar formulation of quercetin prevents cisplatin nephrotoxicity. Int. J. Mol. Sci. 22 (2), E729. doi:10.3390/ijms22020729
Chaudhary, S., Ganjoo, P., Raiusddin, S., and Parvez, S. (2015). Nephroprotective activities of quercetin with potential relevance to oxidative stress induced by valproic acid. Protoplasma 252 (1), 209–217. doi:10.1007/s00709-014-0670-8
Chen, D. Q., Hu, H. H., Wang, Y. N., Feng, Y. L., Cao, G., and Zhao, Y. Y. (2018). Natural products for the prevention and treatment of kidney disease. Phytomedicine. 50, 50–60. doi:10.1016/j.phymed.2018.09.182
Chen Junhua, Z. G., Peng, G., Qin, H., Cheng, H., and Shen, J. (2014). Content determination of chlorogenic acid, quercetin and kaempferol in pyrrosia lingua(thumb.) farwell by HPLC. Chin. J. Inf. Traditional Chin. Med. 21 (8), 67–69.
Choi, S., Ryu, K. H., Park, S. H., Jun, J. Y., Shin, B. C., Chung, J. H., et al. (2016). Direct vascular actions of quercetin in aorta from renal hypertensive rats. Kidney Res. Clin. Pract. 35 (1), 15–21. doi:10.1016/j.krcp.2015.12.003
Conglei Pan, H. L., and Lu, H. (2019). Preparative separation of quercetin, ombuin and kaempferide from Gynostemma pentaphyllum by high-speed countercurrent chromatography. J. Chromatogr. Sci. 57 (3), 265–271. doi:10.1093/chromsci/bmy110
Dallak, M., Dawood, A. F., Haidara, M. A., Abdel Kader, D. H., Eid, R. A., Kamar, S. S., et al. (2020). Suppression of glomerular damage and apoptosis and biomarkers of acute kidney injury induced by acetaminophen toxicity using a combination of resveratrol and quercetin. Drug Chem. Toxicol. 45, 1–7. doi:10.1080/01480545.2020.1722156
Deng Ting, Q. T., Xu, Y., and Sun, T. (2021). Simultaneous detection of 6 flavonoids in Hawthorn Leave total flavonoids and evaluation of antioxidant activity in vitro. Pharm. Clin. Chin. Materia Medica 12 (05), 27–32.
Ding, T., Wang, S., Zhang, X., Zai, W., Fan, J., Chen, W., et al. (2018). Kidney protection effects of dihydroquercetin on diabetic nephropathy through suppressing ROS and NLRP3 inflammasome. Phytomedicine. 41, 45–53. doi:10.1016/j.phymed.2018.01.026
Diniz, L. R. L., Souza, M. T. S., Duarte, A. B. S., and Sousa, D. P. (2020). Mechanistic aspects and therapeutic potential of quercetin against COVID-19-associated acute kidney injury. Molecules 25 (23), E5772. doi:10.3390/molecules25235772
Ebokaiwe, A. P., Obasi, D. O., Njoku, R. C. C., Osawe, S., Olusanya, O., and Kalu, W. O. (2021). Cyclophosphamide instigated hepatic-renal oxidative/inflammatory stress aggravates immunosuppressive indoleamine 2, 3-dioxygenase in male rats: Abatement by quercetin. Toxicology 464, 153027. doi:10.1016/j.tox.2021.153027
Elbarbry, F., Abdelkawy, K., Moshirian, N., and Abdel-Megied, A. M. (2020). The antihypertensive effect of quercetin in young spontaneously hypertensive rats; role of arachidonic acid metabolism. Int. J. Mol. Sci. 21 (18), E6554. doi:10.3390/ijms21186554
Elbe, H., Vardi, N., Esrefoglu, M., Ates, B., Yologlu, S., and Taskapan, C. (2015). Amelioration of streptozotocin-induced diabetic nephropathy by melatonin, quercetin, and resveratrol in rats. Hum. Exp. Toxicol. 34 (1), 100–113. doi:10.1177/0960327114531995
Erboga, M., Aktas, C., Erboga, Z. F., Donmez, Y. B., and Gurel, A. (2015). Quercetin ameliorates methotrexate-induced renal damage, apoptosis and oxidative stress in rats. Ren. Fail. 37 (9), 1492–1497. doi:10.3109/0886022x.2015.1074521
Fu Juan, Y. S., and Huang, L. (2013). Simultaneous determination of six flavonoid active components in Radix astragali by UPLC. Chin. Pharm. J. 48 (11), 916–919.
Gholampour, F., and Sadidi, Z. (2018). Hepatorenal protection during renal ischemia by quercetin and remote ischemic perconditioning. J. Surg. Res. 231, 224–233. doi:10.1016/j.jss.2018.05.036
Gholampour, F., and Saki, N. (2019). Hepatic and renal protective effects of quercetin in ferrous sulfateinduced toxicity. Gen. Physiol. Biophys. 38 (1), 27–38. doi:10.4149/gpb_2018038
Gomes, I. B., Porto, M. L., Santos, M. C., Campagnaro, B. P., Gava, A. L., Meyrelles, S. S., et al. (2015). The protective effects of oral low-dose quercetin on diabetic nephropathy in hypercholesterolemic mice. Front. Physiol. 6, 247. doi:10.3389/fphys.2015.00247
Gonçalves, G. F. B., Silva, M. E. M., Sampaio, F. J. B., Pereira-Sampaio, M. A., and de Souza, D. B. (2021). Quercetin as a nephroprotector after warm ischemia: Histomorphometric evaluation in a rodent model. Int. Braz J. Urol. 47 (4), 796–802. doi:10.1590/s1677-5538.Ibju.2020.0358
Graefe, E. U., Derendorf, H., and Veit, M. (1999). Pharmacokinetics and bioavailability of the flavonol quercetin in humans. Int. J. Clin. Pharmacol. Ther. 37 (5), 219–233.
Graf, B. A., Ameho, C., Dolnikowski, G. G., Milbury, P. E., Chen, C. Y., and Blumberg, J. B. (2006). Rat gastrointestinal tissues metabolize quercetin. J. Nutr. 136 (1), 39–44. doi:10.1093/jn/136.1.39
Gu, Y., Huang, X., Wu, Y., Liu, X., and Lan, H. (2020a). Quercetin alleviates cisplatin-induced acute kidney injury by rebalancing TGF-beta/Smad signaling. Nephrology 25 (S1), 478.
Gu, Y. Y., Dou, J. Y., Huang, X. R., Liu, X. S., and Lan, H. Y. (2021a). Transforming growth factor-β and long non-coding RNA in renal inflammation and fibrosis. Front. Physiol. 12, 684236. doi:10.3389/fphys.2021.684236
Gu, Y. Y., Liu, X. S., Huang, X. R., Yu, X. Q., and Lan, H. Y. (2020b). Diverse role of TGF-β in kidney disease. Front. Cell Dev. Biol. 8, 123. doi:10.3389/fcell.2020.00123
Gu, Y. Y., Zhang, M., Cen, H., Wu, Y. F., Lu, Z., Lu, F., et al. (2021b). Quercetin as a potential treatment for COVID-19-induced acute kidney injury: Based on network pharmacology and molecular docking study. PLoS One 16 (1), e0245209. doi:10.1371/journal.pone.0245209
Guan, T., Xin, Y., Zheng, K., Wang, R., Zhang, X., Jia, S., et al. (2021). Metabolomics analysis of the effects of quercetin on renal toxicity induced by cadmium exposure in rats. Biometals. 34 (1), 33–48. doi:10.1007/s10534-020-00260-2
Guo, S., Sun, J., and Zhuang, Y. (2020). Quercetin alleviates lipopolysaccharide-induced inflammatory responses by up-regulation miR-124 in human renal tubular epithelial cell line HK-2. Biofactors 46 (3), 402–410. doi:10.1002/biof.1596
Heeba, G. H., and Mahmoud, M. E. (2016). Dual effects of quercetin in doxorubicin-induced nephrotoxicity in rats and its modulation of the cytotoxic activity of doxorubicin on human carcinoma cells. Environ. Toxicol. 31 (5), 624–636. doi:10.1002/tox.22075
Hickson, L. J., Langhi Prata, L. G. P., Bobart, S. A., Evans, T. K., Giorgadze, N., Hashmi, S. K., et al. (2019). Senolytics decrease senescent cells in humans: Preliminary report from a clinical trial of Dasatinib plus Quercetin in individuals with diabetic kidney disease. EBioMedicine 47, 446–456. doi:10.1016/j.ebiom.2019.08.069
Hongmei, G. (2008). Simultaneous determination of 4 flavonoids in inula britannica by RP-HPLC. China Pharm. 20 (27), 2122–2123.
Jia, S., Guan, T., Zhang, X., Liu, Y., Liu, Y., and Zhao, X. (2020). Serum metabonomics analysis of quercetin against the toxicity induced by cadmium in rats. J. Biochem. Mol. Toxicol. 34 (4), e22448. doi:10.1002/jbt.22448
Jiang, X., Yu, J., Wang, X., Ge, J., and Li, N. (2019). Quercetin improves lipid metabolism via SCAP-SREBP2-LDLr signaling pathway in early stage diabetic nephropathy. Diabetes Metab. Syndr. Obes. 12, 827–839. doi:10.2147/dmso.S195456
Khalil, S. R., Mohammed, A. T., Abd El-Fattah, A. H., and Zaglool, A. W. (2018). Intermediate filament protein expression pattern and inflammatory response changes in kidneys of rats receiving doxorubicin chemotherapy and quercetin. Toxicol. Lett. 288, 89–98. doi:10.1016/j.toxlet.2018.02.024
Kim Le, F. C., and Ng, K. (2007). Identification and quantification of antioxidants in Fructus lycii. Food Chem. 105 (1), 353–363. doi:10.1016/j.foodchem.2006.11.063
Kim, S. R., Jiang, K., Ogrodnik, M., Chen, X., Zhu, X. Y., Lohmeier, H., et al. (2019). Increased renal cellular senescence in murine high-fat diet: Effect of the senolytic drug quercetin. Transl. Res. 213, 112–123. doi:10.1016/j.trsl.2019.07.005
Kim, S. R., Puranik, A. S., Jiang, K., Chen, X., Zhu, X. Y., Taylor, I., et al. (2021). Progressive cellular senescence mediates renal dysfunction in ischemic nephropathy. J. Am. Soc. Nephrol. 32 (8), 1987–2004. doi:10.1681/asn.2020091373
Kimura, T., Isaka, Y., and Yoshimori, T. (2017). Autophagy and kidney inflammation. Autophagy 13 (6), 997–1003. doi:10.1080/15548627.2017.1309485
Kirkland, J. L., and Tchkonia, T. (2020). Senolytic drugs: From discovery to translation. J. Intern. Med. 288 (5), 518–536. doi:10.1111/joim.13141
Lai, L. L., Lu, H. Q., Li, W. N., Huang, H. P., Zhou, H. Y., Leng, E. N., et al. (2021). Protective effects of quercetin and crocin in the kidneys and liver of obese Sprague-Dawley rats with Type 2 diabetes: Effects of quercetin and crocin on T2DM rats. Hum. Exp. Toxicol. 40 (4), 661–672. doi:10.1177/0960327120954521
Lei, D., Chengcheng, L., Xuan, Q., Yibing, C., Lei, W., Hao, Y., et al. (2019). Quercetin inhibited mesangial cell proliferation of early diabetic nephropathy through the Hippo pathway. Pharmacol. Res. 146, 104320. doi:10.1016/j.phrs.2019.104320
Li, C., Shen, Y., Huang, L., Liu, C., and Wang, J. (2021a). Senolytic therapy ameliorates renal fibrosis postacute kidney injury by alleviating renal senescence. Faseb J. 35 (1), e21229. doi:10.1096/fj.202001855RR
Li, Q. C., Liang, Y., Hu, G. R., and Tian, Y. (2016a). Enhanced therapeutic efficacy and amelioration of cisplatin-induced nephrotoxicity by quercetin in 1, 2-dimethyl hydrazine-induced colon cancer in rats. Indian J. Pharmacol. 48 (2), 168–171. doi:10.4103/0253-7613.178834
Li, S., Cao, C., Shi, H., Yang, S., Qi, L., Zhao, X., et al. (2016b). Effect of quercetin against mixture of four organophosphate pesticides induced nephrotoxicity in rats. Xenobiotica. 46 (3), 225–233. doi:10.3109/00498254.2015.1070443
Li, X., Chen, W., Feng, J., and Zhao, B. (2021b). Incidence of death from kidney diseases among cancer patients: A US population-based analysis. Int. Urol. Nephrol. 53 (12), 2627–2633. doi:10.1007/s11255-021-02801-1
Lin, C. F., Kuo, Y. T., Chen, T. Y., and Chien, C. T. (2016). Quercetin-rich guava (psidium guajava) juice in combination with trehalose reduces autophagy, apoptosis and pyroptosis formation in the kidney and pancreas of type II diabetic rats. Molecules 21 (3), 334. doi:10.3390/molecules21030334
Liu, X., Sun, N., Mo, N., Lu, S., Song, E., Ren, C., et al. (2019a). Quercetin inhibits kidney fibrosis and the epithelial to mesenchymal transition of the renal tubular system involving suppression of the Sonic Hedgehog signaling pathway. Food Funct. 10 (6), 3782–3797. doi:10.1039/c9fo00373h
Liu, Y., Dai, E., and Yang, J. (2019b). Quercetin suppresses glomerulosclerosis and TGF-β signaling in a rat model. Mol. Med. Rep. 19 (6), 4589–4596. doi:10.3892/mmr.2019.10118
Liu, Y., Zhang, X., Guan, T., Jia, S., Liu, Y., and Zhao, X. (2020). Effects of quercetin on cadmium-induced toxicity in rat urine using metabonomics techniques. Hum. Exp. Toxicol. 39 (4), 524–536. doi:10.1177/0960327119895811
Lu, H., Wu, L., Liu, L., Ruan, Q., Zhang, X., Hong, W., et al. (2018). Quercetin ameliorates kidney injury and fibrosis by modulating M1/M2 macrophage polarization. Biochem. Pharmacol. 154, 203–212. doi:10.1016/j.bcp.2018.05.007
Lu, Q., Ji, X. J., Zhou, Y. X., Yao, X. Q., Liu, Y. Q., Zhang, F., et al. (2015). Quercetin inhibits the mTORC1/p70S6K signaling-mediated renal tubular epithelial-mesenchymal transition and renal fibrosis in diabetic nephropathy. Pharmacol. Res. 99, 237–247. doi:10.1016/j.phrs.2015.06.006
Lu, S., Zhou, S., Chen, J., Zheng, J., Ren, J., Qi, P., et al. (2021). Quercetin nanoparticle ameliorates lipopolysaccharide-triggered renal inflammatory impairment by regulation of Sirt1/NF-KB pathway. J. Biomed. Nanotechnol. 17 (2), 230–241. doi:10.1166/jbn.2021.3031
Ma, Y., Chen, F., Yang, S., Chen, B., and Shi, J. (2018). Protocatechuic acid ameliorates high glucose-induced extracellular matrix accumulation in diabetic nephropathy. Biomed. Pharmacother. 98, 18–22. doi:10.1016/j.biopha.2017.12.032
Meng, F. D., Li, Y., Tian, X., Ma, P., Sui, C. G., Fu, L. Y., et al. (2015). Synergistic effects of snail and quercetin on renal cell carcinoma Caki-2 by altering AKT/mTOR/ERK1/2 signaling pathways. Int. J. Clin. Exp. Pathol. 8 (6), 6157–6168.
Mohammad Azmin, S. N. H., and Mat Nor, M. S. (2020). Chemical fingerprint of Centella Asiatica’s bioactive compounds in the ethanolic and aqueous extracts. Adv. Biomark. Sci. Technol. 2, 35–44. doi:10.1016/j.abst.2020.10.001
Morsi, A. A., Fouad, H., Alasmari, W. A., and Faruk, E. M. (2022). The biomechanistic aspects of renal cortical injury induced by diesel exhaust particles in rats and the renoprotective contribution of quercetin pretreatment: Histological and biochemical study. Environ. Toxicol. 37 (2), 310–321. doi:10.1002/tox.23399
Mullen, W., Edwards, C. A., and Crozier, A. (2006). Absorption, excretion and metabolite profiling of methyl-glucuronyl-glucosyl- and sulpho-conjugates of quercetin in human plasma and urine after ingestion of onions. Br. J. Nutr. 96 (1), 107–116. doi:10.1079/bjn20061809
Nirumand, M. C., Hajialyani, M., Rahimi, R., Farzaei, M. H., Zingue, S., Nabavi, S. M., et al. (2018). Dietary plants for the prevention and management of kidney stones: Preclinical and clinical evidence and molecular mechanisms. Int. J. Mol. Sci. 19 (3), E765. doi:10.3390/ijms19030765
Owumi, S. E., Danso, O. F., and Effiong, M. E. (2019). Dietary quercetin abrogates hepatorenal oxidative damage associated with dichloromethane exposure in rats. Acta Biochim. Pol. 66 (2), 201–206. doi:10.18388/abp.2018_2771
Peng, X., Dai, C., Zhang, M., and Das Gupta, S. (2020). Molecular mechanisms underlying protective role of quercetin on copper sulfate-induced nephrotoxicity in mice. Front. Vet. Sci. 7, 586033. doi:10.3389/fvets.2020.586033
Platé, M., Guillotin, D., and Chambers, R. C. (2020). The promise of mTOR as a therapeutic target pathway in idiopathic pulmonary fibrosis. Eur. Respir. Rev. 29 (157), 200269. doi:10.1183/16000617.0269-2020
Qi, L., Cao, C., Hu, L., Chen, S., Zhao, X., and Sun, C. (2017). Metabonomic analysis of the protective effect of quercetin on the toxicity induced by mixture of organophosphate pesticides in rat urine. Hum. Exp. Toxicol. 36 (5), 494–507. doi:10.1177/0960327116652460
Qiu, J., Chen, X., Netrusov, A. I., Zhou, Q., Guo, D., Liu, X., et al. (2017). Screening and identifying antioxidative components in Ginkgo biloba pollen by DPPH-HPLC-PAD coupled with HPLC-ESI-MS2. PLoS One 12 (1), e0170141. doi:10.1371/journal.pone.0170141
Rahdar, A., Hasanein, P., Bilal, M., Beyzaei, H., and Kyzas, G. Z. (2021). Quercetin-loaded F127 nanomicelles: Antioxidant activity and protection against renal injury induced by gentamicin in rats. Life Sci. 276, 119420. doi:10.1016/j.lfs.2021.119420
Ren, J., Li, J., Liu, X., Feng, Y., Gui, Y., Yang, J., et al. (2016). Quercetin inhibits fibroblast activation and kidney fibrosis involving the suppression of mammalian target of rapamycin and β-catenin signaling. Sci. Rep. 6, 23968. doi:10.1038/srep23968
Rezk, A. M., Ibrahim, I., Mahmoud, M. F., and Mahmoud, A. A. A. (2021). Quercetin and lithium chloride potentiate the protective effects of carvedilol against renal ischemia-reperfusion injury in high-fructose, high-fat diet-fed Swiss albino mice independent of renal lipid signaling. Chem. Biol. Interact. 333, 109307. doi:10.1016/j.cbi.2020.109307
Saida Ibragic, E. S., and Sofic, E. (2015). Chemical composition of various Ephedra species. Bosn. J. Basic Med. Sci. 15 (3), 21–27. doi:10.17305/bjbms.2015.539
Sánchez-González, P. D., López-Hernández, F. J., Dueñas, M., Prieto, M., Sánchez-López, E., Thomale, J., et al. (2017). Differential effect of quercetin on cisplatin-induced toxicity in kidney and tumor tissues. Food Chem. Toxicol. 107 (1), 226–236. doi:10.1016/j.fct.2017.06.047
Sen-ming, Z. (2013). Improvements of flavonoids and saponins determination in Radix Bupleuri by HPLC method. Mod. Chin. Med. 15 (09), 744–747.
Shu, B., Feng, Y., Gui, Y., Lu, Q., Wei, W., Xue, X., et al. (2018). Blockade of CD38 diminishes lipopolysaccharide-induced macrophage classical activation and acute kidney injury involving NF-κB signaling suppression. Cell. Signal. 42, 249–258. doi:10.1016/j.cellsig.2017.10.014
Sok Yen, F., Shu Qin, C., Tan Shi Xuan, S., Jia Ying, P., Yi Le, H., Darmarajan, T., et al. (2021). Hypoglycemic effects of plant flavonoids: A review. Evid. Based. Complement. Altern. Med. 2021, 2057333. doi:10.1155/2021/2057333
Sun, H. J., Wu, Z. Y., Cao, L., Zhu, M. Y., Liu, T. T., Guo, L., et al. (2019). Hydrogen sulfide: Recent progression and perspectives for the treatment of diabetic nephropathy. Molecules 24 (15), E2857. doi:10.3390/molecules24152857
Tan, J., He, J., Qin, W., and Zhao, L. (2019). Quercetin alleviates lipopolysaccharide-induced acute kidney injury in mice by suppressing TLR4/NF-κB pathway. Nan Fang. Yi Ke Da Xue Xue Bao 39 (5), 598–602. doi:10.12122/j.issn.1673-4254.2019.05.16
Tan, R. Z., Wang, C., Deng, C., Zhong, X., Yan, Y., Luo, Y., et al. (2020). Quercetin protects against cisplatin-induced acute kidney injury by inhibiting Mincle/Syk/NF-κB signaling maintained macrophage inflammation. Phytother. Res. 34 (1), 139–152. doi:10.1002/ptr.6507
Tong, F., Liu, S., Yan, B., Li, X., Ruan, S., and Yang, S. (2017). Quercetin nanoparticle complex attenuated diabetic nephropathy via regulating the expression level of ICAM-1 on endothelium. Int. J. Nanomedicine 12, 7799–7813. doi:10.2147/ijn.S146978
Tu, H., Ma, D., Luo, Y., Tang, S., Li, Y., Chen, G., et al. (2021). Quercetin alleviates chronic renal failure by targeting the PI3k/Akt pathway. Bioengineered 12 (1), 6538–6558. doi:10.1080/21655979.2021.1973877
Uthra, C., Shrivastava, S., Jaswal, A., Sinha, N., Reshi, M. S., and Shukla, S. (2017). Therapeutic potential of quercetin against acrylamide induced toxicity in rats. Biomed. Pharmacother. 86, 705–714. doi:10.1016/j.biopha.2016.12.065
Vetrova, E. V., Maksimenko, E. V., Borisenko, S. N., Lekar, A. V., Borisenko, N. I., and Minkin, V. I. (2017). Extraction of rutin and quercetin antioxidants from the buds of Sophora Japonica (Sophora japonica L.) by subcritical water. Russ. J. Phys. Chem. B 11, 1202–1206. doi:10.1134/s1990793117070193
Vicente-Vicente, L., González-Calle, D., Casanova, A. G., Hernández-Sánchez, M. T., Prieto, M., Rama-Merchán, J. C., et al. (2019). Quercetin, a promising clinical candidate for the prevention of contrast-induced nephropathy. Int. J. Mol. Sci. 20 (19), E4961. doi:10.3390/ijms20194961
Wang, Y., Quan, F., Cao, Q., Lin, Y., Yue, C., Bi, R., et al. (2021). Quercetin alleviates acute kidney injury by inhibiting ferroptosis. J. Adv. Res. 28, 231–243. doi:10.1016/j.jare.2020.07.007
Wong, C. C., Botting, N. P., Orfila, C., Al-Maharik, N., and Williamson, G. (2011). Flavonoid conjugates interact with organic anion transporters (OATs) and attenuate cytotoxicity of adefovir mediated by organic anion transporter 1 (OAT1/SLC22A6). Biochem. Pharmacol. 81 (7), 942–949. doi:10.1016/j.bcp.2011.01.004
Yuksel, Y., Yuksel, R., Yagmurca, M., Haltas, H., Erdamar, H., Toktas, M., et al. (2017). Effects of quercetin on methotrexate-induced nephrotoxicity in rats. Hum. Exp. Toxicol. 36 (1), 51–61. doi:10.1177/0960327116637414
Zhang Jing, T. C., and Yan, F. (2020). Simultaneous determination of seven constituents in rubus chingii by HPLC. China Pharm. 23 (12), 2496–2499. doi:10.1016/S1674-6384(16)60051-5
Zhong Yuekui, C. Q., and Qiu, Z. (2021). Determination of 4 flavonoids in mulberry leaves from different habitats by ultra performance liquid chromatography. J. Food Saf. Qual. 12 (05), 1855–1860.
Zhu Kaixin, Z. X., Zhao, M., Pei, H., Su, B., and Li, Y. (2011). Determination of quarcetin from four kinds of Herba Taxilli parasiticed in mulberry. Lishizhen Med. Materia Medica 22 (10), 2395–2397.
Keywords: quercetin, natural product, herbal medicine, kidney injury, renal disease
Citation: Chen Y-Q, Chen H-Y, Tang Q-Q, Li Y-F, Liu X-S, Lu F-H and Gu Y-Y (2022) Protective effect of quercetin on kidney diseases: From chemistry to herbal medicines. Front. Pharmacol. 13:968226. doi: 10.3389/fphar.2022.968226
Received: 13 June 2022; Accepted: 28 July 2022;
Published: 02 September 2022.
Edited by:
Dan-Qian Chen, Northwest University, ChinaReviewed by:
Milad Ashrafizadeh, Sabancı University, TurkeyYaser Hosny Ali Elewa, Zagazig University, Egypt
Maria Russo, Institute of Food Sciences (CNR), Italy
Copyright © 2022 Chen, Chen, Tang, Li, Liu, Lu and Gu. This is an open-access article distributed under the terms of the Creative Commons Attribution License (CC BY). The use, distribution or reproduction in other forums is permitted, provided the original author(s) and the copyright owner(s) are credited and that the original publication in this journal is cited, in accordance with accepted academic practice. No use, distribution or reproduction is permitted which does not comply with these terms.
*Correspondence: Fu-Hua Lu, bHVmdWh1YUBnenVjbS5lZHUuY24=; Yue-Yu Gu, Z3V5eUBnenVjbS5lZHUuY24=
†These authors have contributed equally to this work