- 1Study Program of Biology, Faculty of Mathematics and Natural Sciences, Universitas Sumatera Utara, Medan, Indonesia
- 2Department of Pharmaceutical Biology, Faculty of Pharmacy, Universitas Sumatera Utara, Medan, Indonesia
- 3Department of Pharmacology, Faculty of Pharmacy, Universitas Sumatera Utara, Medan, Indonesia
- 4Department of Cell and Molecular Biology, Faculty of Biotechnology and Biomolecular Sciences, Universiti Putra Malaysia, Serdang, Malaysia
Cancer refers to the proliferation and multiplication of aberrant cells inside the human body, characterized by their capacity to proliferate and infiltrate various anatomical regions. Numerous biochemical pathways and signaling molecules have an impact on the cancer auto biogenesis process. The regulation of crucial cellular processes necessary for cell survival and proliferation, which are triggered by phytochemicals, is significantly influenced by signaling pathways. These pathways or components are regulated by phytochemicals. Medicinal plants are a significant reservoir of diverse anticancer medications employed in chemotherapy. The anticancer effects of phytochemicals are mediated by several methods, including induction of apoptosis, cessation of the cell cycle, inhibition of kinases, and prevention of carcinogenic substances. This paper analyzes the phytochemistry of seven prominent plant constituents, namely, alkaloids, tannins, flavonoids, phenols, steroids, terpenoids, and saponins, focusing on the involvement of the MAPK/ERK pathway, TNF signaling, death receptors, p53, p38, and actin dynamics. Hence, this review has examined a range of phytochemicals, encompassing their structural characteristics and potential anticancer mechanisms. It has underscored the significance of plant-derived bioactive compounds in the prevention of cancer, utilizing diverse molecular pathways. In addition, this endeavor also seeks to incentivize scientists to carry out clinical trials on anticancer medications derived from plants.
1 Introduction
Cancer is a pathological condition defined by the excessive and uncontrolled growth of abnormal cells within the human body. These abnormal cells exhibit the ability to proliferate and invade any region of the organism (Brown et al., 2023). Cancer is considered the second leading cause of mortality globally, following stroke and heart disease (Sun and Bhaskar, 2022). Cancer is classified based on the cellular genesis of the tumor. Carcinoma, a cancer that arises from the epithelial cells of the breast, prostate, lung, pancreas, and colon, is responsible for 90% of all cancer-related deaths in humans (Łukasiewicz et al., 2021). Lymphoma, on the other hand, is a cancer that affects immune organs like the spleen, white blood cells, and lymph nodes (Weledji and Orock, 2015). Leukemia is a cancer that affects the blood cells that make up the bone marrow (Bispo et al., 2020). Sarcoma is a cancer that affects the fibrous connective tissue of bones, cartilage, fatty tissue, muscles, and neurons (Vodanovich and M Choong, 2018). Lastly, germ cell tumors originate from pluripotent stem cells found in the testes and ovaries (Hong et al., 2021). Cell metabolism is intricately interconnected within a multifaceted biological network that encompasses various metabolites and ubiquitous mechanisms for sensing these compounds. Signal transduction and epigenetics of these pathways can be regulated by either endogenous or exogenous metabolites. Nevertheless, the occurrence of irregular metabolic reprogramming in cancer might result in atypical suppression and stimulation of metabolite sensing, hence playing a substantial role in the advancement of cancer (Schiliro and Firestein, 2021). Timely identification and efficient therapy enhance the likelihood of survival in cancer patients. Hence, it is imperative to develop a comprehensive strategy aimed at enhancing cancer prevention and treatment. Epidemiological and experimental studies have provided evidence supporting the notion that a substantial consumption of fruits, vegetables, or medicinal plants can effectively mitigate the prevalence of chronic degenerative diseases (Cruz et al., 2024). Moreover, the significance of maintaining a well-balanced diet in the context of cancer prevention has garnered considerable scholarly interest.
Medicinal plants have served as a valuable reservoir of diverse anticancer medications that are being employed in chemotherapy. Bioactive compounds, known as phytochemicals, play a crucial role in anticancer treatment (Yuan et al., 2022). These sources are secure, harmless, economical, and easily accessible, spanning from rural to urban areas and underdeveloped to developed nations (Irianti et al., 2020). Hence, there is growing interest in exploring the possible anticancer properties of herbal substances. Chemoprevention refers to the application of both natural and synthetic chemicals for impeding or decelerating the progression of cancer through the inhibition or disruption of specific molecular signaling pathways (G et al., 2021). The investigation of the impact of phytochemicals on cellular signaling is presently a subject of contemporary scholarly research. These substances have distinct modes of action against tumors. Bioactive compounds (phytochemicals) play a crucial role in the field of anticancer treatment (Situmorang et al., 2022a). The gynogenesis movement is impacted by multiple biochemical pathways and signaling molecules. Signaling pathways and molecular networks play a crucial role in the regulation of vital cellular processes that are required for the survival and proliferation of cells. The etiology of cancer necessitates a correlation with distinct biological pathways (Fawaz et al., 2023). Researchers persist in employing this methodology to further the progress of molecular therapy. Various molecular biology methodologies have been developed to detect and treat cancer, including the targeting of cancer stem cell pathways for treatment, utilization of retroviral therapy, suppression of oncogenes, and the alteration of tumor suppressor genes (Simanullang RH. et al., 2022). The regulation of cell proliferation, differentiation, survival, apoptosis, invasion, migration, angiogenesis, and metastatic spread of cancer cells is connected with various signaling pathways, including mTOR, PI3K, protein kinase B (Akt), MAPK/ERK, Wnt, Notch, and Hedgehog (Kciuk et al., 2022). The anticancer activities are induced by phytochemicals through the regulation of certain pathways or components.
Multiple independently conducted investigations have indicated that phytochemicals induce anticancer effects through various pathways (Choudhari et al., 2019). Nevertheless, there is a lack of detailed reporting regarding the phytochemical composition of seven plant components, namely, alkaloids, tannins, flavonoids, phenols, steroids, terpenoids, and saponins, in relation to their potential as anticancer agents (Simanullang et al., 2022b). There are no reports regarding the regulation of cell proliferation and inhibition of angiogenesis and metastasis through the MAPK/ERK pathway, TNF signaling, death receptors, p53, p38, and actin dynamics. Thus, this review has examined different phytochemicals, including their structures and probable anticancer mechanisms. As a result, it offers comprehensive knowledge on the potential of natural anticancer resources.
2 Role of phytochemicals and antioxidants in inhibiting the resistance of cancer to therapy
Utilizing phytochemicals for cancer chemoprevention is the preferred method for managing cancer. Investigating novel plant-derived chemicals with anticancer characteristics is a crucial objective in pharmacological research as it enables the discovery of new therapeutic targets (Efferth et al., 2017). This review highlights specific chemicals that exhibit potential as effective chemo-preventive agents for cancer. It is worth noting that the consumption of foods containing these bioactive compounds has demonstrated both protective and therapeutic benefits against different forms of cancer (Bakrim et al., 2022). The efficacy of chemotherapy and radiotherapy is enhanced by chemo-preventive medicines through regulation of various signal transduction pathways. Given the significant involvement of oxidative stress in the development of numerous malignancies, the potential of substances with antioxidant properties as a preventive measure against cancer is worth considering (Qi et al., 2022). Plants are rich in antioxidant phytochemicals, which encompass a diverse range of molecules (Simanullang et al., 2022c). These substances have distinct modes of action against tumors. Bioactive compounds (phytochemicals) play a crucial role in the field of anticancer treatment. Medicinal plants or their derivatives currently constitute over 70% of anticancer chemicals, making them the primary focus in the development of anticancer medications (Talib et al., 2020). The anticancer properties of plants are attributed to seven primary bioactive compounds: alkaloids, tannins, flavonoids, phenols, steroids, terpenoids, and saponins. Alkaloids are significant chemical substances that constitute a plentiful resource for the exploration of new drugs. Several alkaloids derived from medicinal plants and herbs have demonstrated antiproliferative and anticancer properties against a diverse range of malignancies, both in laboratory settings (in vitro) and in living organisms (in vivo) (Lu et al., 2012). Vinblastine, vinorelbine, vincristine, and vindesine have been effectively formulated as pharmaceutical agents for the treatment of cancer. Tannins demonstrate a wide range of therapeutic advantages, including their ability to combat cancer, act as an antioxidant, reduce inflammation, and protect the nervous system (Dhyani et al., 2022). Flavonoids are widely recognized for their efficacy as antioxidants and their ability to inhibit angiogenesis. Numerous studies have documented the inhibitory effects of flavonoids on the metabolic activation of carcinogens, hence impeding the proliferation of aberrant cells that have the potential to differentiate into malignant cells (Wang et al., 2022). Phenols in foods have multiple functions, including acting as antioxidants to eliminate cancer-causing free radicals, activating cytoprotective enzymes involved in detoxifying foreign substances, and regulating signal transduction systems (Aghajanpour et al., 2017). The involvement of antioxidants in the activation of the Keap1/Nrf2/ARE pathway leads to heightened levels of phase 2 detoxification enzymes and antioxidant enzymes (Mendonca and Soliman, 2020). The anticancer effects of terpenes may be attributed to their capacity to regulate several signaling pathways associated with cellular proliferation, death, and angiogenesis. The anticancer effects of terpenes may also be attributed to their ability to induce oxidative stress and DNA damage in cancer cells (Wróblewska-Łuczka et al., 2023). Steroids encompass a class of naturally occurring organic compounds that have steroidal anticancer properties. This class of chemicals exhibits a wide range of structural molecular diversity and possesses the capacity to interact with diverse biological targets and pathways. Saponins exhibit several anticancer properties, such as inhibiting cell growth, impeding metastasis, inhibiting angiogenesis, and reversing multidrug resistance (MDR) (Elekofehinti et al., 2021). These effects are caused by the initiation of apoptosis, stimulation of cell differentiation, modulation of the immune system, binding of bile acids, and improvement of cell proliferation caused by carcinogens.
Oxidative stress plays a crucial role in the harmful effects of environmental toxicity in cancer development, and reactive oxygen species (ROS) are produced in response to both internal and external triggers (Chang et al., 2015). Reactive oxygen species (ROS) such as superoxide radicals (O2−.), hydrogen peroxide (H2O2), singlet oxygen (1O2), and hydroxyl radicals (HO.) are harmful to cells and have been linked to the development of different human diseases, including cancer (Afzal et al., 2023). Several carcinogens exert their effects by generating reactive oxygen species (ROS) during their metabolic process (Afzal et al., 2023). Oxidative DNA damage is a significant factor in the development and advancement of carcinogenesis as it can cause mutations (Martemucci et al., 2022). Hence, the crucial role of antioxidants in counteracting elevated levels of reactive oxygen species (ROS) is significant in the context of numerous disorders, including different forms of cancer (Afzal et al., 2023). Epidemiological studies form the primary basis for establishing the correlation between dietary antioxidants and non-communicable diseases, such as cancer. These studies indicate that plant foods and phytochemicals have the capacity to prevent cancer. Certain phytochemicals have the ability to function as both antioxidants and prooxidants (Situmorang and Ilyas, 2018). They can generate reactive oxygen species (ROS) and induce oxidative stress at high levels, particularly when iron and copper are present. Polyphenols, including quercetin, epicatechin, epigallocatechin-3-gallate (EGCG), and gallic acid, have been found to generate reactive oxygen species (ROS) in cell models due to their prooxidant properties (Yang et al., 2020). Phytochemicals are widely recognized for their antioxidant properties, but they can also display prooxidant activity under specific circumstances, such as when administered in excessive amounts or in the presence of metal ions (Yang et al., 2020). The concentration of phytochemicals plays a crucial role in determining whether they exhibit prooxidant or antioxidant activity (Situmorang et al., 2021a). Studies using cell models have highlighted the prooxidant activity of polyphenols, which are known for their antioxidant properties. Specifically, compounds such as quercetin, epicatechin, and epigallocatechin-3-gallate (EGCG) have been found to demonstrate this prooxidant activity. At elevated concentrations, such as 50 μM, quercetin enhances the generation of superoxide radicals (O2−) in isolated mitochondria and cell culture medium (Yang et al., 2020; Safi et al., 2021). Previous research has demonstrated that quercetin can decrease cell viability and thiol content, as well as impair overall antioxidant capacity and the activities of SOD, CAT, and glutathione transferase at higher concentrations (Safi et al., 2021). High amounts of flavonoids can generate reactive oxygen species (ROS) through processes such as autoxidation and redox cycles, as seen in quercetin (Safi et al., 2021).
Dietary phytochemicals can activate many cell signaling pathways, and the specific route triggered by a molecule can vary depending on the type of cell (Hun Lee et al., 2013). Elevated levels of pro-apoptotic p53 and reduced levels of key pro-survival factors, such as epidermal growth factor receptor (EGFR), nuclear factor-kappa B (NF-κB), activator protein 1, signal transducers and activators of transcription (STAT), survivin, metalloproteinases 2 and 9, vascular endothelial growth factor (VEGF), and B-cell leukemia/lymphoma 2 (Bcl-2), are observed under optimal conditions when phytochemicals are administered (Gupta et al., 2012). The effectiveness of phytochemicals in cancer treatment stems from their capacity to influence many signaling pathways concurrently, hence facilitating apoptosis, impeding cellular proliferation and invasion, sensitizing malignant cells, and enhancing immune system functionality (George et al., 2021). The synergistic effect of cytotoxic anticancer drugs and phytochemical inhibitors can synergistically reduce tumor growth (Cheon and Ko, 2022). The phytochemical composition of the seven primary plant constituents, namely, flavonoids, alkaloids, terpenoids, steroids, saponins, phenol, and tannins, as anticancer agents is shown in Supplementary Table S1.
3 Role of phytochemicals in the MAPK/ERK pathway
The MAPK/ERK pathway is a cellular protein chain responsible for transmitting signals from cell surface receptors to DNA within the cell nucleus. The development of certain human disorders, including as Alzheimer’s disease (AD), Parkinson’s disease (PD), amyotrophic lateral sclerosis (ALS), and several types of cancer, has been associated with deviations from strict regulation of the MAPK signaling pathway (Albert-Gascó et al., 2020). This particular route encompasses a multitude of proteins, including mitogen-activated protein kinase (MAPK, formerly known as ERK). These proteins engage in communication by introducing phosphate groups to adjacent proteins, thus functioning as active or inactive switches. MAPKs represent a group of serine/threonine protein kinases that exhibit a high degree of conservation. These kinases play a crucial role in numerous essential cellular processes, including but not limited to proliferation, differentiation, motility, stress response, apoptosis, and survival (Chen et al., 2021). There have been characterizations of at least three MAPK families, namely, extracellular signal-regulated kinase (ERK), Jun kinase (JNK/SAPK), and p38 MAPK. The aforementioned effects are achieved through the modulation of cell-cycle machinery and other proteins associated with cell proliferation (Min and Lee, 2023). Figure 1 investigates the function of this system in conjunction with other signaling pathways to regulate the proliferation of cancer cells. MAPK activation is initiated in a multistep process through the stimulation of receptor tyrosine kinase (RTK) in the MAPK/ERK signaling pathway. The protein kinase activity of RAF kinase is facilitated by the activation of Ras. The phosphorylation and activation of MEK (MEK1 and MEK2) is facilitated by RAF kinase. ERK is activated and phosphorylated by MEK (Santarpia et al., 2012). The role of the MAPK/ERK pathway in the translation of extracellular signals to cellular responses has been demonstrated to be significant. The protein kinase cascade encompasses the presence of MAP kinase (Cargnello and Roux, 2011). The cascade comprises a minimum of three enzymes that are sequentially activated: MAPK kinase kinase (MAPKKK), MAPK kinase (MAPKK), and MAP kinase (MAPK). The MAPK/ERK pathway is involved in multiple processes associated with cancer, such as proliferation, invasion, metastasis, angiogenesis, and apoptosis inhibition. The MAPK/ERK pathway has a significant impact on encouraging cancer cell proliferation and preventing apoptosis due to its diverse actions (Kciuk et al., 2022). For instance, the compound β-carboline has been observed to impede cell growth and trigger apoptosis in SGC-7901 cells. This disruption of the PTEN and ERK balance leads to the inhibition of the MAPK/ERK signaling pathway, ultimately resulting in apoptosis in SGC-7901 cells (Qin et al., 2022). Berberine has the ability to block the EGFR/Raf/MEK/ERK pathway, hence suppressing the aging process in human glioblastoma cells. Sinomenine suppresses the growth of many cancer cells (Liu et al., 2015). The phosphorylation of ERK1/2 and p38 is enhanced by the presence of sinomenine hydrochloride (SH). The phosphorylation of ERK1/2 was considerably increased by the benzo alkaloid chelerythrine chloride, while the phosphorylation of Akt was lowered in a dose-dependent manner (Peng et al., 2023). Table 1 presents the mechanism of action of various phytochemical compounds in the MAPK/ERK pathway in cancer.
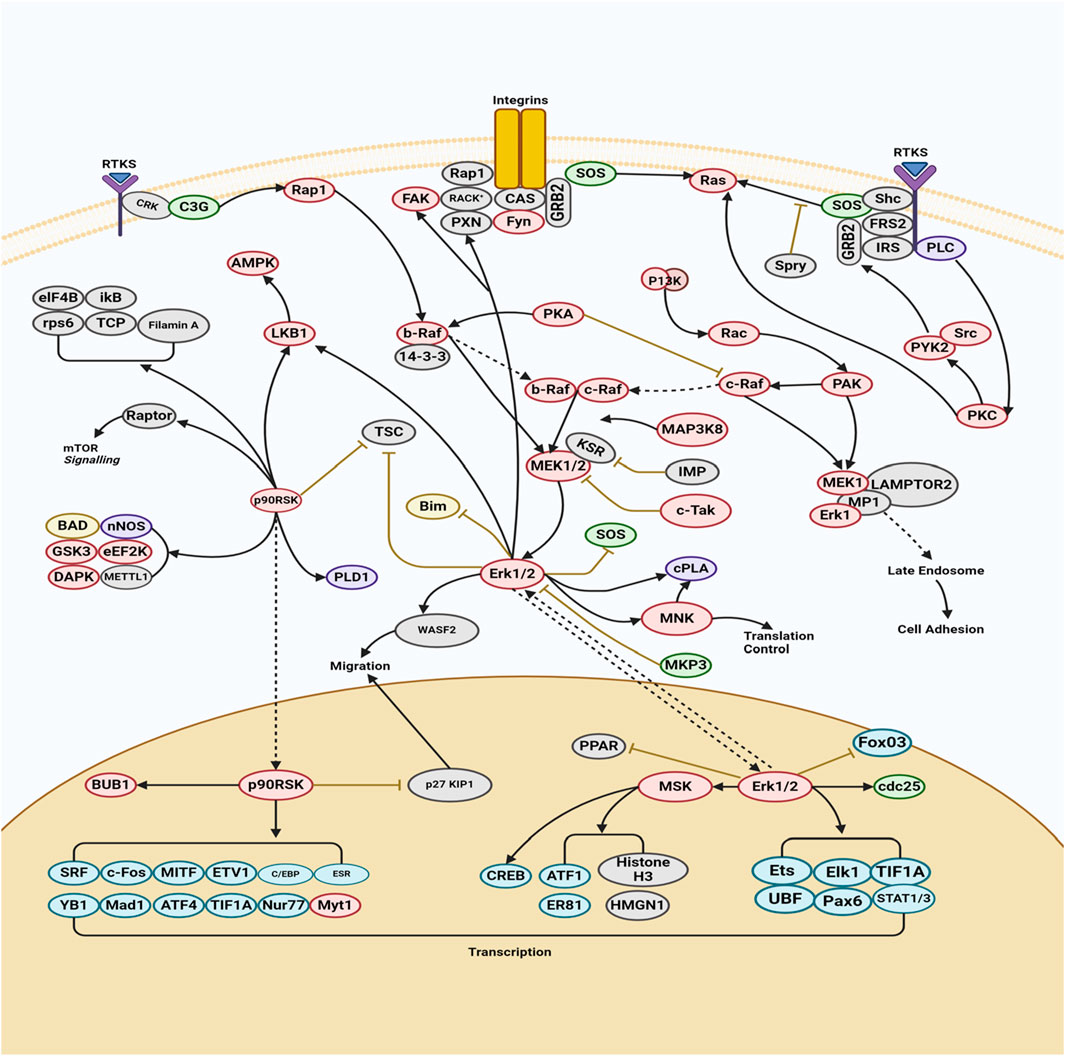
Figure 1. MAPK/ERK pathway in cancer. The activation of MAPK initiates with the stimulation of receptor tyrosine kinase (RTK) in the MAPK/ERK signaling pathway. This leads to the activation of RAF kinase protein kinase activity through Ras activation. Subsequently, MEK (MEK1 and MEK2) is phosphorylated and activated by RAF kinase. Finally, ERK is activated and phosphorylated by MEK (https://www.sinobiological.com/pathways).
4 Role of phytochemicals in the TNF-signaling pathway
The activation of signaling pathways for cell survival, death, and differentiation is facilitated by the tumor necrosis factor (TNF) superfamily of cytokines. TNF signaling has witnessed a growing trend in the therapeutic management of individuals afflicted with inflammatory bowel disease (IBD), including ulcerative colitis (UC) and Crohn’s disease (CD), as well as rheumatologic and dermatological conditions such as rheumatoid arthritis (RA), juvenile idiopathic arthritis, and cancer (Evangelatos et al., 2022). The activation of signal transduction pathways that promote apoptosis can occur in cancer illnesses by the recruitment of death domains (DDs) containing adapters, such as the Fas-associated death domain (FADD) and TNFR-associated DD (TRADD), via TNF signaling (Mak and Yeh, 2002). The activation of transcription factors, such as NF-kappa B and JNK, can be facilitated by the recruitment of TRAF family proteins. This activation promotes cell survival and differentiation, as well as immunological and inflammatory responses. Tumor necrosis factor ligands and receptors were included based on their sequence and structure. The TNF-related ligand is classified as a type II transmembrane protein, characterized by an internal N terminus and an external C terminus, known as the “TNF homology domain” (THD). An essential characteristic of the receptor is the presence of a cysteine-rich domain (CRD), which is composed of three disulfide connections encircling the core motif CXXCXXC, resulting in the formation of an elongated molecule (Suo et al., 2022). Figure 2 illustrates the mechanism by which tumor necrosis factor (TNF) exerts its effects via the tumor necrosis factor receptor (TNFR), hence participating in the extrinsic pathway for induction of apoptosis. The association between the TNFR and procaspases is mediated by adapter proteins such as FADD and TRADD. These adapter proteins have the ability to cleave dormant procaspases, thus initiating the caspase cascade. This cascade ultimately leads to irreversible induction of death in cells (Elmore, 2007). Studies on the apoptotic pathway have identified a malfunction in the breakdown of lysosomal DNA, which triggers macrophages to generate cytokines like IFN2 and TNF, without relying on Toll-like receptors (TLRs) (Brouckaert et al., 2004). The interaction between tumor necrosis factor and tumor cells initiates cytolysis, which is the process of cell death. The inflammatory response can be enhanced by tumor necrosis factor (Li and Beg, 2000). The mechanism of action of various phytochemical compounds in the TNF-signaling pathway in cancer is shown in Table 2.
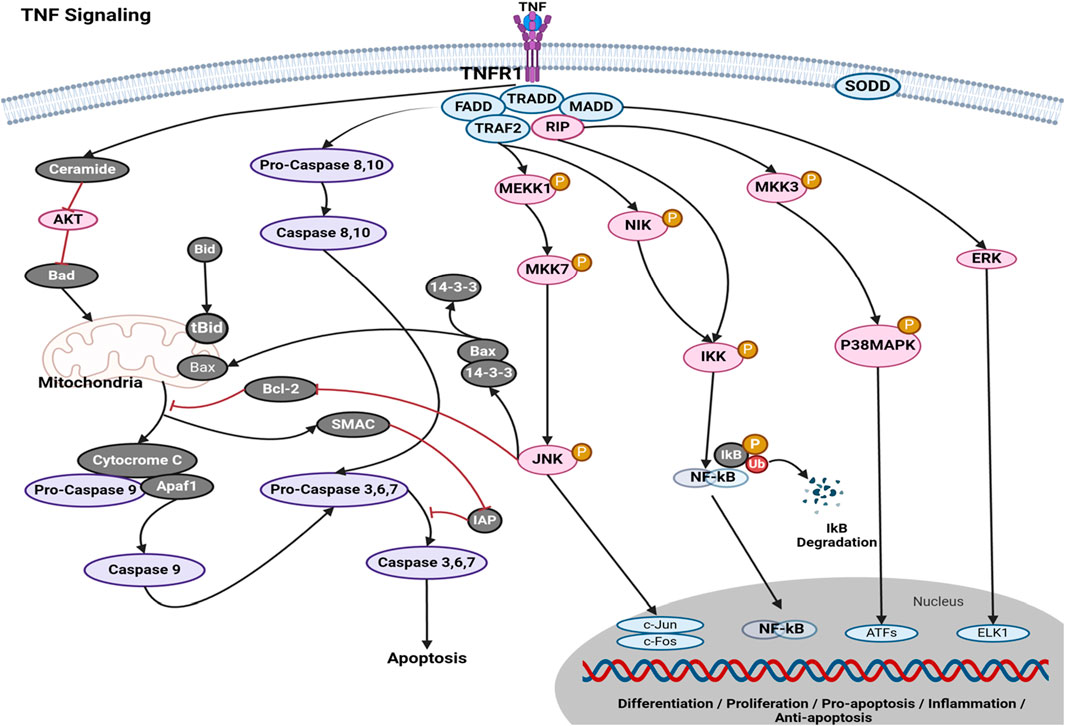
Figure 2. TNF-signaling pathways in cancer. Tumor necrosis factor (TNF) exerts its actions through the tumor necrosis factor receptor (TNFR), hence engaging in the extrinsic pathway to promote apoptosis. The interaction between the TNFR and procaspases is facilitated by adapter proteins, such as FADD and TRADD. These adapter proteins possess the capability to cleave inactive procaspases, hence activating the caspase cascade. This series of events ultimately results in the irreversible initiation of cell death (https://www.sinobiological.com/pathways).
5 Role of phytochemicals in the death receptor pathways
Death receptors are receptors located on the surface of cells that send signals triggering apoptosis. The involvement of death receptors in the process of apoptosis of inflammatory cells, both in vivo and in vitro, is of significant importance in various disorders, including inflammation, hypertension, and cancer (Green, 2022). In certain cases ,T lymphocytes and macrophages exhibit the presence of several TNFR/ligands within plaques (Situmorang et al., 2022b). The TNFR pathway has been linked to the death of T cells and macrophages (Situmorang P. C. et al., 2024). These receptors, like TNFR, are stimulated by specific ligands and play a critical role in instructive apoptosis (Siegmund et al., 2016). Death receptors are classified under the superfamily of tumor necrosis factor receptor (TNFR) genes (Hehlgans and Pfeffer, 2005). So far, eight members of the death receptor family have been identified: TNFR1 (also referred to as DR1, CD120a, p55, and p60), CD95 (also referred to as DR2, APO-1, and Fas), DR3 (also referred to as APO-3, LARD, TRAMP, and WSL1), TRAILR1 (also referred to as DR4 and APO-2), TRAILR2 (also referred to as DR5, KILLER, and TRICK2), DR6, ectodysplasin A receptor (EDAR), and nerve growth factor receptor (NGFR). The death receptors can be identified by the presence of a cytoplasmic region known as the death domain (DD), which has around 80 residues (Hoffmann et al., 2009). In the context of cancer (Figure 3), the activation of these receptors by certain ligands leads to the recruitment of several molecules to the death domain, subsequently initiating a signaling cascade. There are two distinct forms of death receptor signaling complexes. The first group comprises death-inducing signaling complexes (DISCs) that lead to the activation of caspase-8, a pivotal component in the signaling transduction pathways that mediate apoptosis (Gnesutta and Minden, 2003). DISCs are generated on the CD95, TRAILR1, or TRAILR2 receptors. The second category consists of TNFR1, DR3, DR6, and EDAR. The process entails the recruitment of several molecules that assist the translation of signals related to apoptosis and cell survival (Schneider-Brachert et al., 2013). The mechanism of action of various phytochemical compounds in the death receptor pathway in cancer is shown in Table 3.
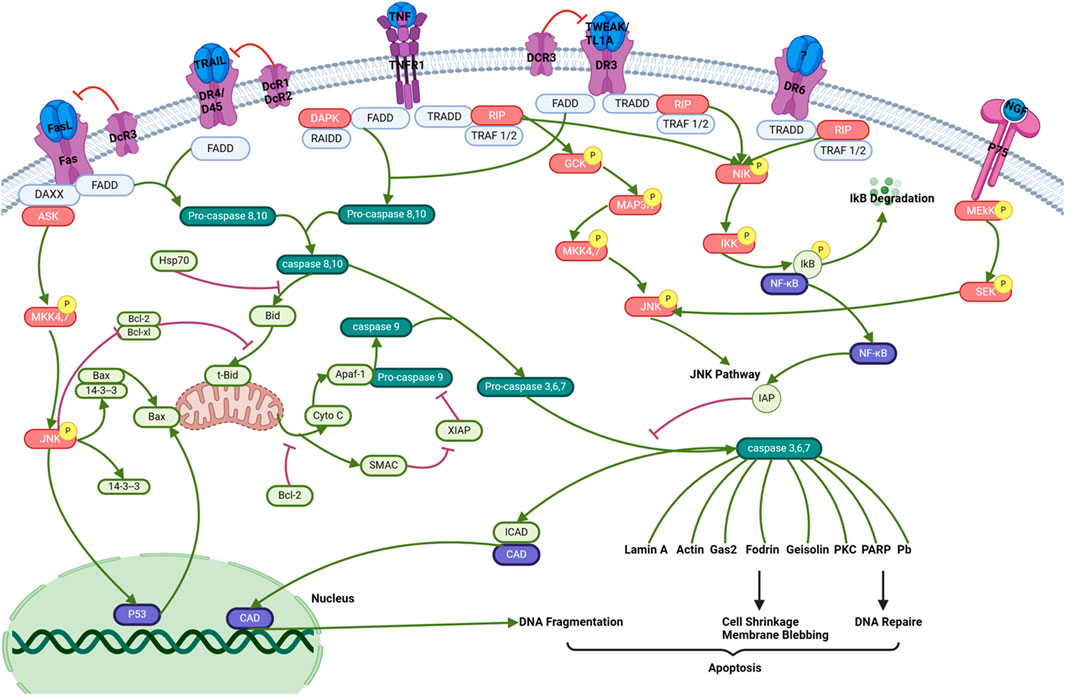
Figure 3. Death receptor pathways in cancer. Activation of these receptors by specific ligands triggers the recruitment of multiple molecules to the death domain, which then initiates a signaling cascade. There are two distinct forms of death receptor signaling complexes. The first group comprises death-inducing signaling complexes (DISCs) that activate caspase-8, a crucial component in apoptotic signaling transduction. DISCs are formed at the CD95, TRAILR1, or TRAILR2 receptors. These processes involve the recruitment of various molecules that facilitate apoptotic and survival signal transduction (https://www.sinobiological.com/pathways).
6 Role of phytochemicals in the p53 pathway
The p53 tumor suppressor is a prominent mechanism involved in apoptosis signaling. Cell loss in various neurological illnesses, such as Alzheimer’s disease, Parkinson’s disease, hypertension, stroke, and cancer, may be attributed to p53-related apoptosis, which is a frequently observed process (Wolfrum et al., 2022). In response to genotoxic or cellular stress, the p53 protein functions as a nuclear transcription factor, exerting regulatory control over the expression of several genes associated with apoptosis, growth arrest, or senescence (Mijit et al., 2020). E3 ubiquitin ligases, such as MDM2, exert a negative regulatory effect on p53 protein levels in cancer (Figure 4). The proteasome-dependent degradation of p53 is facilitated by the E3 ligase, which enhances ubiquitination. In response to various stress stimuli, such as DNA damage, nucleolar stress, metabolic stress, and oncogenic stress, the levels of p53 protein exhibit stability. The cytoplasmic connections between P53 and Bcl-2 family proteins have been observed to facilitate the process of apoptosis. It was demonstrated in the late 1980s and early 1990s that the introduction of the wild-type p53 gene into different human tumor cells resulted in the induction of apoptosis and suppression of cell growth (Dhokia et al., 2022). A curative response was observed in a mouse model system where the function of p53 was precisely reactivated in the tumor. The activation of p53 triggers cell-cycle arrest, facilitating DNA repair and/or apoptosis to inhibit the proliferation of cells with significant DNA damage (Chen, 2016). This is achieved via transactivating target genes that are involved in the initiation of cell cycle arrest and/or apoptosis. The mechanism of action of various phytochemical compounds in the p53 pathway in cancer is shown in Table 4.
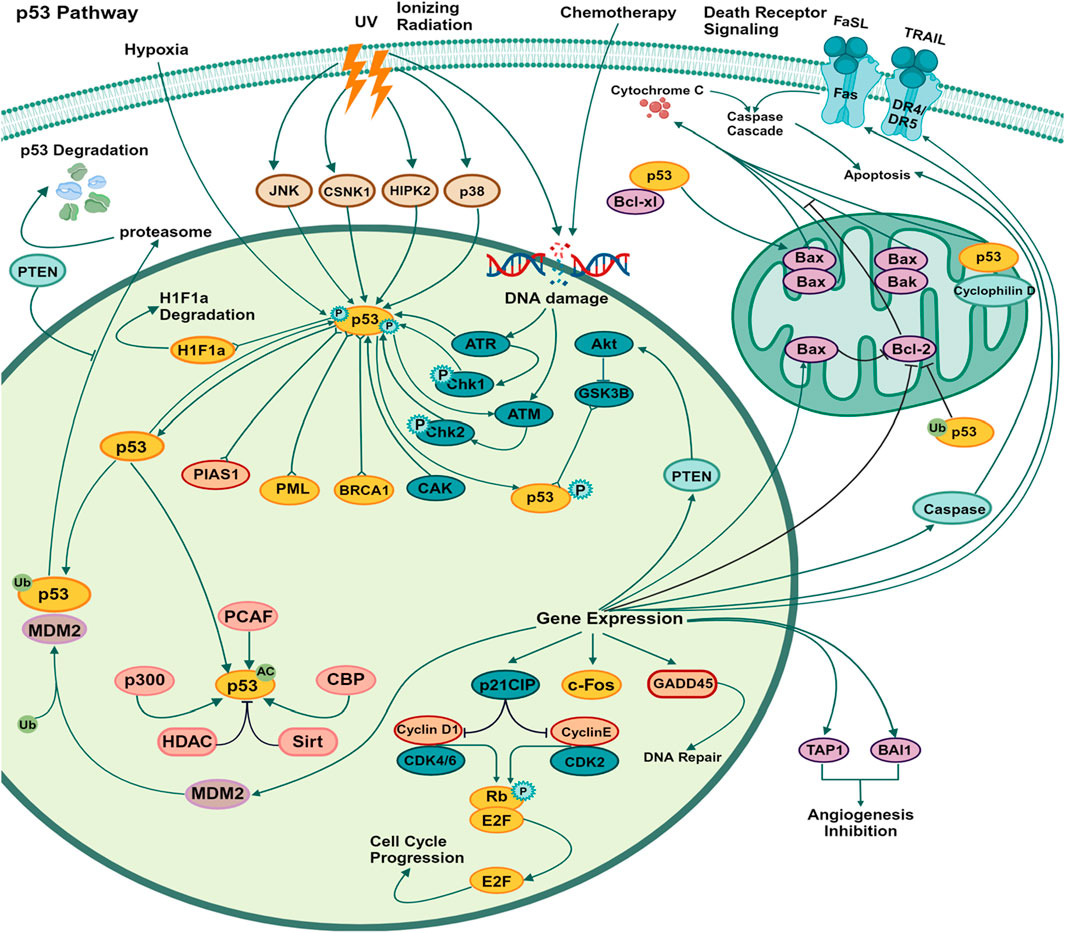
Figure 4. p53 pathways in cancer. The p53 mechanism occurs due to various stress stimuli, such as DNA damage, nucleolar stress, metabolic stress, and oncogenic stress. p53 protein levels show stability. Proteasome-dependent degradation of p53 is facilitated by E3 ligases, which enhance ubiquitination, and cytoplasmic connections between p53 and Bcl-2 family proteins have also been observed to promote apoptosis (https://www.sinobiological.com/pathways).
7 Role of phytochemicals in the p38 pathway
The involvement of p38 MAP kinase (MAPK) in signaling cascades governing cellular reactions to cytokines and stress has been observed (Falcicchia et al., 2020). Four p38 MAP kinases have been found in mammals, namely, p38-α (MAPK14), p38-β (MAPK11), p38-γ (MAPK12/ERK6), and p38-ι (MAPK13/SAPK4). Just like the SAPK/JNK pathway, the activation of p38 MAP kinases occurs in response to several cellular stressors and various illnesses, including osmotic shock, inflammatory cytokines, lipopolysaccharide (LPS), UV light, growth factors, hypertension, and cancer (Asih et al., 2020). Furthermore, the activation of p38 is indirectly induced by oxidative stress and GPCR stimulation (Situmorang et al., 2023). The regulation of downstream targets, such as various kinases, transcription factors, and cytosolic proteins, is governed by p38 MAPK in the context of cancer (Huang et al., 2024). The kinases considered in this study are MAPKAPK2, MAPKAPK3, PRAK, MSK1, and MNK ½ (Figure 5). P38 phosphorylates several crucial transcription factors, such as the tumor suppressor protein p53, CHOP (C/EBP homologous protein), STAT1 (signal transducer and activator of transcription-1), CREB (cAMP response element-binding protein), and Max/Myc complex (Koul et al., 2013). The p38 MAPK pathway plays a crucial role in regulating the production of pro-inflammatory cytokines at both the transcriptional and translational levels. Consequently, several elements within this system hold promise as possible therapeutic targets for autoimmune and inflammatory disorders (Ganguly et al., 2023). The p38 pathway plays a crucial role in controlling cell growth and suppressing tumor growth by influencing many regulators of the cell cycle. The tumor-suppressing role of the p38 pathway suggests that several elements of the p38 pathway hold promise as possible targets for innovative cancer treatments (Martínez-Limón et al., 2020). The mechanism of action of various phytochemical compounds in the p38 pathway in cancer is shown in Table 5.
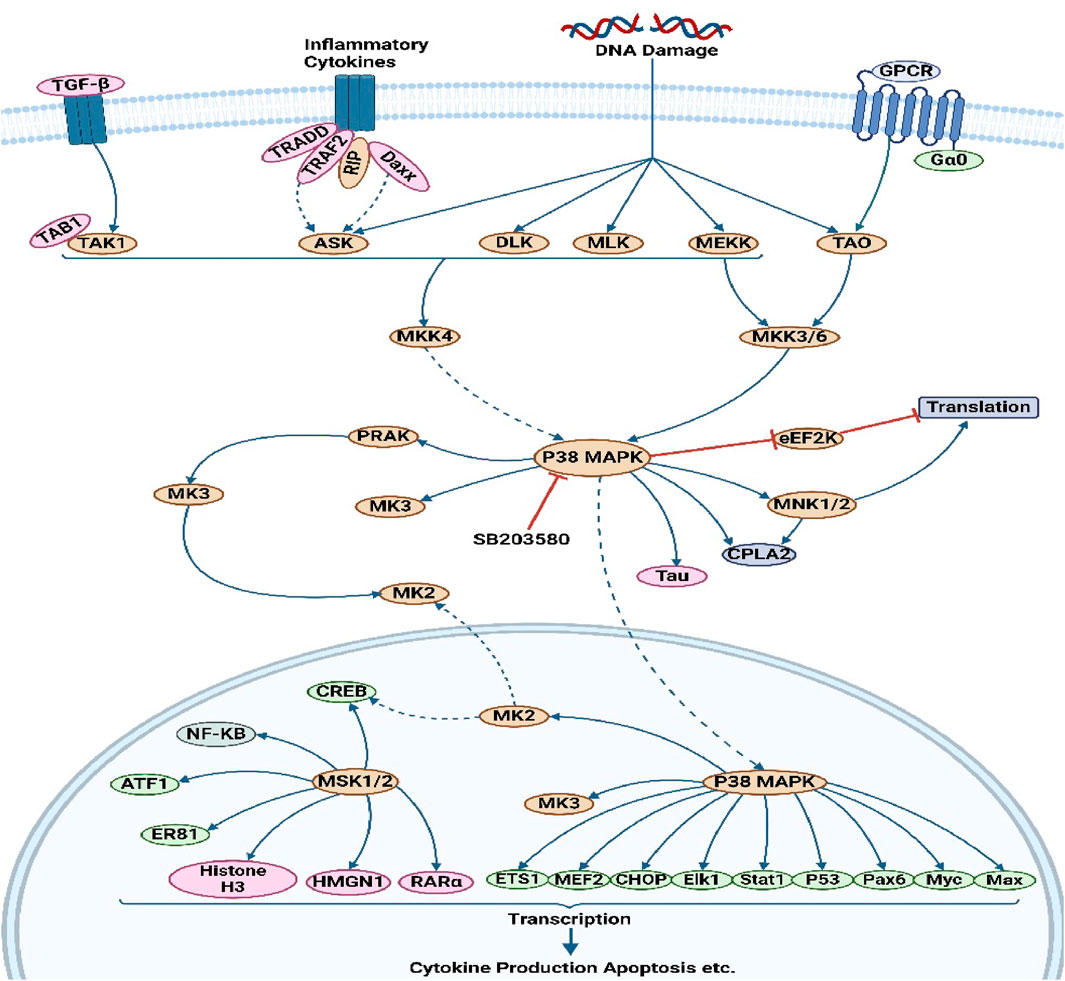
Figure 5. p38 pathways in cancer. p38 MAPK governs the regulation of downstream targets, including different kinases, transcription factors, and cytosolic proteins. The kinases included in this study are MAPKAPK2, MAPKAPK3, PRAK, MSK1, and MNK ½. P38 phosphorylates various important transcription factors, including the tumor suppressor protein p53, CHOP (C/EBP homologous protein), STAT1 (signal transducer and activator of transcription-1), CREB (cAMP response element-binding protein), and Max/Myc complex. The p38 MAPK pathway is essential for controlling the synthesis of pro-inflammatory cytokines at both the transcriptional and translational stages (https://www.sinobiological.com/pathways).
8 Role of phytochemicals in the actin dynamics signaling pathways
G protein-coupled receptors (GPCRs), integrins, and receptor tyrosine kinases (RTKs) are involved in the regulation of actin dynamics by extracellular signals. G protein-coupled receptors (GPCRs) encompass a diverse group of protein receptors that detect extracellular chemicals and initiate intracellular signal transduction pathways, ultimately leading to physiological responses (Cheng et al., 2023). The formation of aberrant thin filaments and the disruption of muscular contraction might result from dysfunctional actin–ATP binding, hence causing muscle weakening and various manifestations of actin accumulation myopathy (Dowling et al., 2021). No ACTA1 gene mutation has been detected in certain individuals with actin accumulation myopathy. In addition to muscular issues, actin dynamics signaling pathways also contribute to the development of cancer. Transmembrane receptors known as integrins serve as intermediaries between cell–cell interactions and the extracellular matrix (ECM) of a cell. In cancer, integrins initiate signaling transduction pathways that affect the cell interior, including the chemical composition and mechanical state of the extracellular matrix (ECM) (Jo et al., 2020). This leads to transcriptional activation, which in turn regulates several cellular processes such as cell cycle regulation, cell shape, the cell’s ability to move, and the addition of additional receptors to the cell membrane (Pang et al., 2023). RTKs, or receptor tyrosine kinases, belong to the extensive group of protein tyrosine kinases. Tyrosine kinase receptors encompass a diverse array of proteins, including EGFR, PDGFR, MCSFR, IGF1R, INSR, NGFR, FGFR, VEGFR, and HGFR (Figure 6). Rho is responsible for modulating intracellular signals that control the cell’s response to external stimulus. Rho is a constituent of the Ras superfamily of tiny GTP-binding proteins that has significant involvement in various biological processes, including the organization of the actin cytoskeleton, dynamics of microtubules, transcription of genes, transformation of cancer cells, development of the cell cycle, adhesion, and epithelial wound repair (Zubor et al., 2020). The activation of GEF (guanine nucleotide exchange factor) is seen (Ilyas et al., 2022). The protein kinase effectors ROCK and PAK are located downstream. The occurrence of immunological diseases, developmental abnormalities, and cancer often involves the disruption of cytoskeletal signaling, leading to the cessation of production of extracellular stimuli and cellular responses (Miller and Zachary, 2017). The mechanism of action of various phytochemical compounds in actin dynamics signaling pathways in cancer is shown in Table 6.
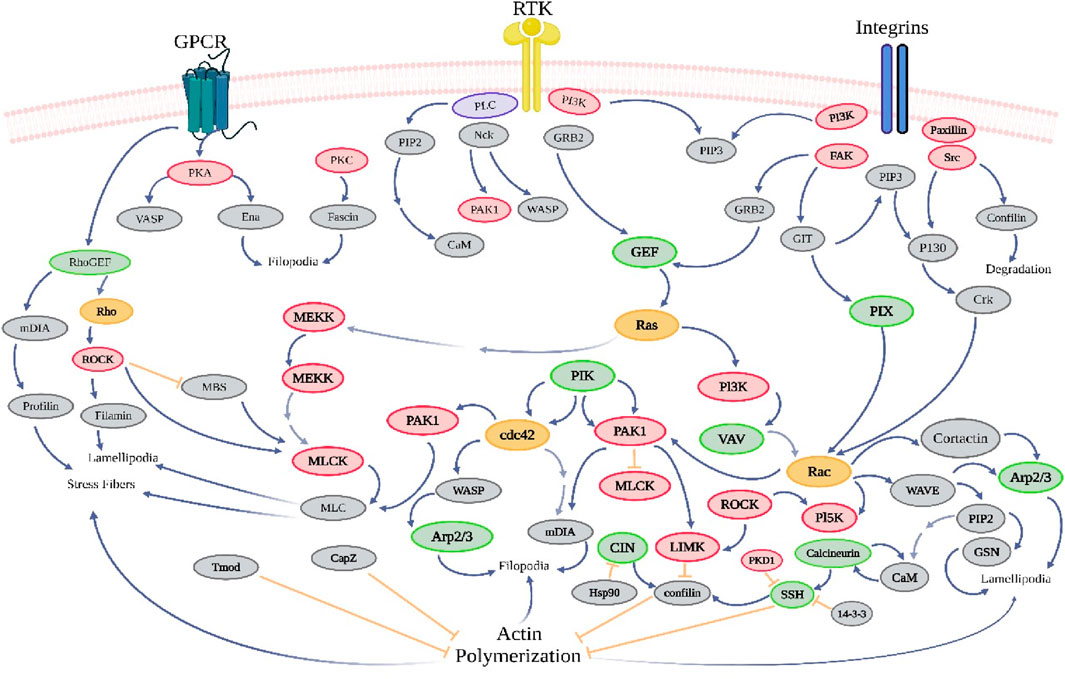
Figure 6. Actin dynamics signaling pathways in cancer. The large protein tyrosine kinase family includes receptor tyrosine kinases (RTKs). Tyrosine kinase receptors, such as EGFR, PDGFR, MCSFR, IGF1R, INSR, NGFR, FGFR, VEGFR, and HGFR, modulate intracellular signals that control the cell’s response to external stimulus and are part of the Ras superfamily of tiny GTP-binding proteins that are involved in many biological processes (https://www.sinobiological.com/pathways).
9 Role of phytochemicals in the autophagy pathways
Autophagy is a cellular recycling mechanism characterized by the dynamic destruction of cytoplasmic contents, aberrant protein aggregates, and excess or damaged organelles through the autophagosome–lysosome process (Gómez-Virgilio et al., 2022). Impaired autophagy function is linked to NAFLD, diabetes, AKD/CKD, heart failure, IBD, and neurodegenerative disorders. These models provide comprehensive data on initial effectiveness, toxicity, pharmacokinetics, and safety, which aids in determining whether a molecule should be further developed for the purpose of conducting clinical studies (Situmorang et al., 2021b; Situmorang et al., 2021c). Nevertheless, in the context of cancer, both the inhibition and augmentation of autophagy significantly contribute to the advancement of the disease via several mechanisms. The activation of mTOR (Akt and MAPK signaling) in cancer leads to the suppression of autophagy, while the negative regulation of mTOR (AMK and p53 signaling) increases autophagy (Verma et al., 2021). ULK functions in a manner comparable to that of yeast Atg1, operating in the downstream region of the mTOR complex. The formation of a substantial complex between ULK, Atg13, and the scaffolding protein FIP200 is observed. The induction of autophagy necessitates the presence of the class III PI3K complex, which comprises hVps34, beclin 1 (the mammalian counterpart of yeast Atg6), p150 (the mammalian counterpart of yeast Vps15), and Atg14-like protein (Atg14L or Barkor) or ultraviolet irradiation resistance-associated gene (UVRAG) (Tran et al., 2021). Rubicon exerts inhibitory effects on the activity of PI3K class III lipid kinase and counteracts the effects of Atg14L, a protein that enhances class III PI3K activity. Autophagosome formation is regulated by the Atg12-Atg5 and LC3-II (Atg8-II) complexes, which are controlled by the Atg gene. Atg12 undergoes conjugation with Atg5 through an ubiquitin-like process, which necessitates the involvement of Atg7 and Atg10 (enzymes with E1-and E2-like properties, respectively). The Atg12–Atg5 conjugate subsequently has a noncovalent interaction with Atg16, resulting in the formation of a substantial complex (Hu and Reggiori, 2022). The protease Atg4 cleaves the C terminus of the second complex, LC3/Atg8, resulting in the formation of cytosolic LC3-I. The process of conjugating LC3-I to phosphatidylethanolamine (PE) involves a ubiquitin-like reaction that necessitates the involvement of Atg7 and Atg3, which are enzymes with E1-and E2-like properties, respectively (Agrotis et al., 2019). LC3-II, a lipidated variant of LC3, adheres to the membrane of autophagosomes (Figure 7). Autophagy and apoptosis have both positive and negative associations, with significant intercommunication observed between these two biological processes. The process of autophagy is a survival strategy that is activated when nutrients are scarce (Xi et al., 2022). However, an excessive amount of autophagy can result in cell death, which is a separate process from apoptosis in terms of its morphology. Autophagy is also induced by other pro-apoptotic signals, including TNF, TRAIL, and FADD. Furthermore, Bcl-2 exerts inhibitory effects on beclin 1-dependent autophagy (Ilyas et al., 2021), thus serving as a dual regulator of both pro-survival and anti-autophagic processes. The mechanism of action of various phytochemical compounds in autophagy pathways in cancer is shown in Table 7.
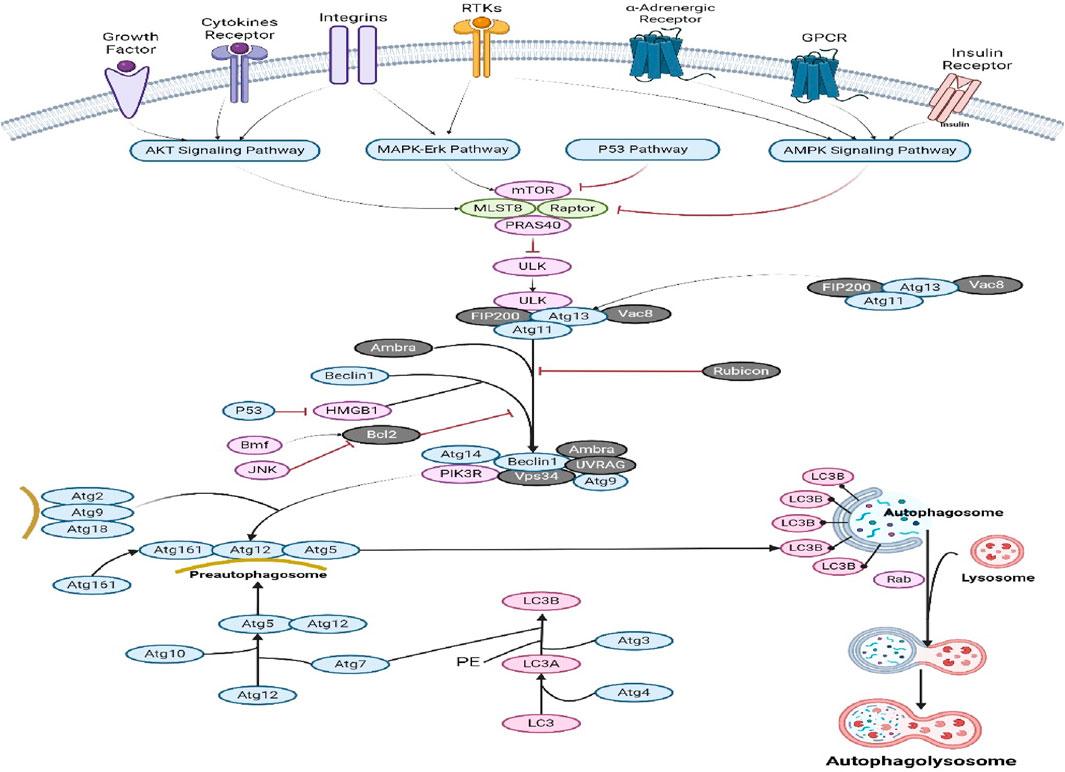
Figure 7. Autophagy pathways in cancer. Activating mTOR (Akt and MAPK signaling) in cancer suppresses autophagy, while negative regulation (AMK and p53 signaling) enhances it. Both autophagy and apoptosis have beneficial and negative effects, and they communicate. Under nutritional constraint, autophagy aids survival. Other pro-apoptotic signals including TNF, TRAIL, and FADD trigger autophagy (https://www.sinobiological.com/pathways).
10 Phytochemicals in clinical trials as anticancer agents
During the practical process of drug development, employing meticulous preclinical screening models can generate promising lead compounds for the development of anticancer drugs. These models provide comprehensive data on initial effectiveness, toxicity, pharmacokinetics, and safety, which aids in determining whether a molecule should be further developed for the purpose of conducting clinical studies (Situmorang et al., 2021b). In the present review, a substantial body of evidence pertaining to the effectiveness of several phytochemicals has been amassed. Clinical research has demonstrated that P. ginseng effectively decreases the occurrence of cancer and exhibits advantageous benefits in individuals with cancer (Chen S. et al., 2014). Previous research has demonstrated that the consumption of fresh ginseng slices, juice, or tea has been associated with a reduced likelihood of developing many types of cancer, such as those affecting the pharynx, larynx, esophagus, stomach, colorectal, pancreatic, liver, lung, and ovarian regions (Lim et al., 2016). The presence of resveratrol in grape powder does not exhibit the ability to inhibit the Wnt pathway in colon cancer. However, it does demonstrate the capacity to inhibit the expression of Wnt target genes in the normal colonic mucosa of individuals diagnosed with colorectal cancer. This implies that resveratrol has advantageous properties in the onset and spread of colon cancer due to its modulation of Wnts and their downstream effectors, which play a crucial role in regulating various processes associated with tumor initiation, tumor growth, cell death, and metastasis (Jin et al., 2016). Furthermore, empirical investigations have demonstrated that flavonoids exhibit antiproliferative and apoptotic properties against diverse tumor cell lines, such as human lymphoma, breast cancer, osteosarcoma, and transformed hepatoma cells. A study including 250 urine samples obtained from Chinese women residing in Shanghai demonstrated a correlation between elevated excretion of total isoflavonoids and total lignans and a decreased likelihood of developing breast cancer (Situmorang PC. et al., 2024). Research has demonstrated that the incorporation of phytosterols into one’s diet can effectively mitigate the likelihood of developing obesity, diabetes, and cancer risk factors. The efficacy of phytosterols as anticancer agents has been demonstrated in several in vivo investigations, employing diverse cancer cell lines and animal models (Dai et al., 2002). Based on the above explanation, it is evident that numerous phytochemical constituents have been subjected to research and have progressed to the clinical trial phase. Herbal phytochemicals promote autophagy, a process by which cells undergo halting of aberrant growth and development. Autophagy is induced by the downregulation of the AKT and mTOR pathways in cancer cells (Ahmed et al., 2022). Hence, phytochemicals modulate antitumor effects in tissues via controlling inflammation, angiogenesis, invasion, and metastasis. The signaling pathways that phytochemicals use to halt carcinogenesis are illustrated in Figure 8.
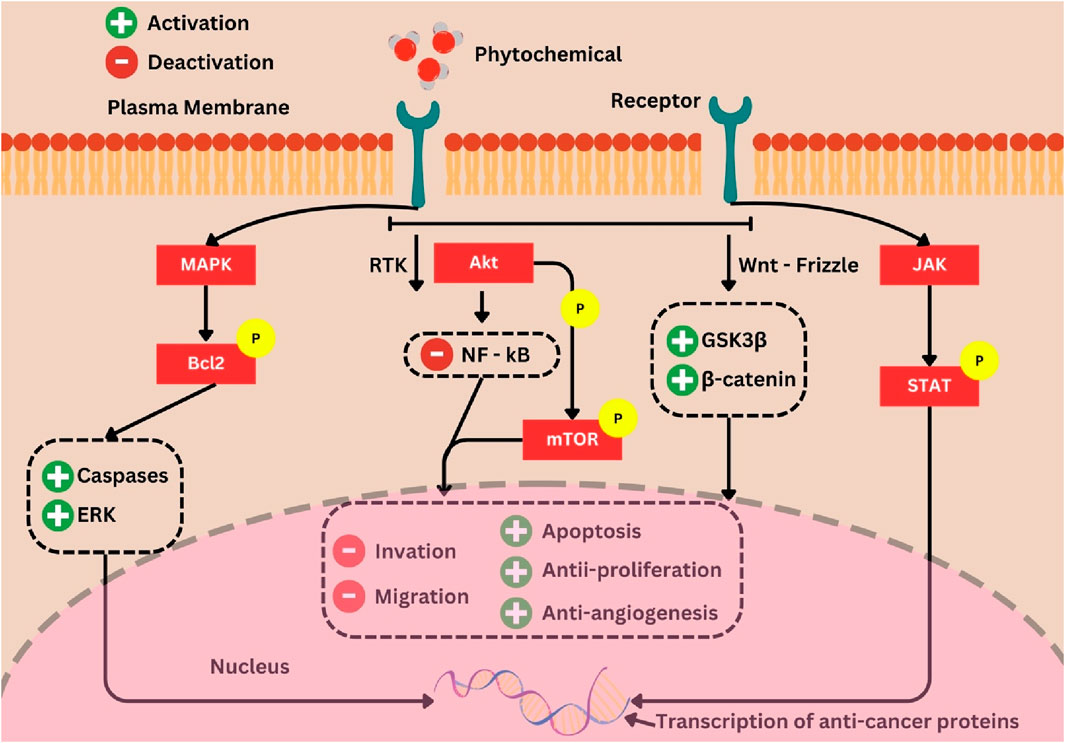
Figure 8. Phytochemicals as chemo-preventives and signaling molecule modulators. In the presence of phytochemicals that increase p53, decrease AKT, and cause cellular apoptosis and cell cycle arrest, tumor suppressor proteins activate the apoptotic cascade. Crosstalk between the p53, MAPK, and JNK pathways also contributes, where the AKT and mTOR pathways are downregulated in cancer cells, causing autophagy, but the presence of herbal phytochemicals can control inflammation, angiogenesis, invasion, and metastasis (Ahmed et al., 2022).
11 Nanoformulation and green synthesis improve cancer-fighting natural chemicals
Various drug delivery technologies, such as synthetic polymers, microcapsules, and liposomes, possess the characteristic of enhancing medication bioavailability and promoting drug accumulation at the intended location. Herbal administration of anticancer medications is crucial since it minimizes or eliminates any adverse effect on healthy tissues. The utilization of nanoformulations is prevalent in the development of sunscreens, antibacterial medications, cholesterol biosensors, and dietary modulators for the purpose of managing diabetes and hyperlipidemia (Khafaga et al., 2023). Furthermore, it is imperative for the drug to possess functional groups that facilitate further alterations aimed at regulating drug release or binding to the target unit. The potential application of silver nanoparticle-based nanosystems as carriers for several therapeutic chemicals, including those possessing anti-inflammatory, antioxidant, antibacterial, and anticancer characteristics, has been the subject of investigation (Burdusel et al., 2018).
The present methodology for synthesizing nanoparticles in an environmentally sustainable manner is distinctive and time-efficient. Extensive research has been carried out on silver nanoparticles (AgNPs) due to their potential anticancer properties, as evidenced by multiple studies on human cancer. AgNPs exhibit significant potential as a highly efficient mechanism for delivering antitumor drugs. AgNPs address these limitations by mitigating adverse effects and enhancing the efficacy of cancer treatment. AgNPs have great potential as pharmaceuticals, as evidenced by the favorable outcomes of prior research and their affordability (Takáč et al., 2023). Several previously documented phytochemicals have demonstrated the potential of phytochemicals as cancer medicines because of their ability to form ionic or covalent connections with gold nanoparticles (AuNPs) and undergoing physical absorption. A hybrid system was formed by covalently linking polyacrylamide/dextran nano-hydrogel to silver nanoparticles, resulting in an in vitro release of 98.5%. Nevertheless, the utilization of AgNPs as therapeutic agents is hindered by certain inherent challenges pertaining to toxicity (Yusuf et al., 2023). In order to surmount these challenges and facilitate their application in preclinical trials involving people or other organisms, it is imperative that AgNPs exhibit biocompatibility, non-toxicity, and the absence of adverse effects. The selection of various mechanistic pathways, such as mitochondrial disruption, DNA fragmentation, cell membrane disruption, cellular signaling pathway disruption, enzyme activity changes, and reactive oxygen species (ROS), by AgNPs holds significant promise for therapeutic applications due to their ability to control the size, shape, and function of the corona surface. Utilizing phytochemicals found in plant extracts, AgNPs were produced by green synthesis for potential cancer treatment. Furthermore, this study also presents recent advancements and accomplishments in the application of silver nanoparticles (AgNPs) produced by green synthesis in the field of cancer therapy (Ratan et al., 2020). It also suggests probable mechanisms through which these nanoparticles may exhibit anticancer and cytotoxic properties. The comprehension of the molecular mechanisms behind the therapeutic effectiveness of AgNPs holds promise for the development of targeted therapies and treatments for cancer, hence presenting a promising avenue for cancer treatment (Wahi et al., 2023).
12 Conclusion
Cancer is characterized by abnormal cell metabolism, which is considered a biological indicator. The preferred approach for cancer treatment is the use of plant compounds for chemoprevention. The observed preventive and therapeutic advantages of these bioactive chemicals against different types of cancer can be attributed to their ability to modulate signal transduction pathways. The consideration of compounds possessing antioxidant properties as a preventive intervention against cancer is warranted due to the substantial role of oxidative stress in the pathogenesis of diverse malignant illnesses. Seven primary bioactive chemicals, namely, alkaloids, tannins, flavonoids, phenols, steroids, terpenoids, and saponins, found in plants have anticancer activities. These compounds play a significant role in modulating cell growth. The process of angiogenesis and metastasis inhibition in cancer is mediated by various mechanisms, including the MAPK/ERK pathway, TNF signaling, death receptors, p53, p38, and actin dynamics. In the context of combating cancer resistance, novel molecular processes necessitate the exploration of novel multitargets. Phytochemical substances, whether transformed into natural small molecules or nanostructured formulations, exhibit considerable potential as multitarget agents due to their reduced side effects and enhanced efficacy. This phenomenon not only exerts an impact on the proliferation of malignant cells but also offers practical insights for the investigation of therapeutic agents that specifically target tumors, hence paving the way for novel avenues in the realm of cancer prevention and therapy.
Author contributions
PS: funding acquisition, methodology, writing–original draft, and writing–review and editing. SI: software, supervision, writing–original draft, and writing–review and editing. SN: formal analysis, investigation, project administration, writing–original draft, and writing–review and editing. RS: formal analysis, writing–original draft, and writing–review and editing. NN: conceptualization, resources, visualization, writing–original draft, and writing–review and editing.
Funding
The author(s) declare that financial support was received for the research, authorship, and/or publication of this article. This study was support by the LPDP in Equity project research PKUU-PT QS WUR RANK 102-300 scheme under contract numbers 7092.1/UN5.1.R/PPM/2023 and 8/UN5.2.3.1/PPM/KPEP/2023.
Conflict of interest
The authors declare that the research was conducted in the absence of any commercial or financial relationships that could be construed as a potential conflict of interest.
Publisher’s note
All claims expressed in this article are solely those of the authors and do not necessarily represent those of their affiliated organizations, or those of the publisher, the editors, and the reviewers. Any product that may be evaluated in this article, or claim that may be made by its manufacturer, is not guaranteed or endorsed by the publisher.
Supplementary material
The Supplementary Material for this article can be found online at: https://www.frontiersin.org/articles/10.3389/fphar.2024.1387866/full#supplementary-material
References
Abbaspour Babaei, M., Zaman Huri, H., Kamalidehghan, B., Yeap, S. K., and Ahmadipour, F. (2017). Apoptotic induction and inhibition of NF-κB signaling pathway in human prostatic cancer PC3 cells by natural compound 2,2’-oxybis (4-allyl-1-methoxybenzene), biseugenol B, from Litsea costalis: an in vitro study. OncoTargets Ther. 10, 277–294. doi:10.2147/OTT.S102894
Afzal, S., Abdul Manap, A. S., Attiq, A., Albokhadaim, I., Kandeel, M., and Alhojaily, S. M. (2023). From imbalance to impairment: the central role of reactive oxygen species in oxidative stress-induced disorders and therapeutic exploration. Front. Pharmacol. 14, 1269581. doi:10.3389/fphar.2023.1269581
Aghajanpour, M., Nazer, M. R., Obeidavi, Z., Akbari, M., Ezati, P., and Kor, N. M. (2017). Functional foods and their role in cancer prevention and health promotion: a comprehensive review. Am. J. cancer Res. 7, 740–769.
Agrotis, A., von Chamier, L., Oliver, H., Kiso, K., Singh, T., and Ketteler, R. (2019). Human ATG4 autophagy proteases counteract attachment of ubiquitin-like LC3/GABARAP proteins to other cellular proteins. J. Biol. Chem. 294, 12610–12621. doi:10.1074/jbc.AC119.009977
Ahmed, M. B., Islam, S. U., Alghamdi, A. A. A., Kamran, M., Ahsan, H., and Lee, Y. S. (2022). Phytochemicals as chemo-preventive agents and signaling molecule modulators: current role in cancer therapeutics and inflammation. Int. J. Mol. Sci. 23 (24), 15765. doi:10.3390/ijms232415765
Akhtar, N., Baig, M. W., Haq, I.-U., Rajeeve, V., and Cutillas, P. R. (2020). Withanolide metabolites inhibit PI3K/AKT and MAPK pro-survival pathways and induce apoptosis in acute myeloid leukemia cells. Biomedicines 8, 333. doi:10.3390/biomedicines8090333
Albert-Gascó, H., Ros-Bernal, F., Castillo-Gómez, E., and Olucha-Bordonau, F. E. (2020). MAP/ERK signaling in developing cognitive and emotional function and its effect on pathological and neurodegenerative processes. Int. J. Mol. Sci. 21, 4471. doi:10.3390/ijms21124471
Almatroodi, S. A., Almatroudi, A., Alsahli, M. A., Khan, A. A., and Rahmani, A. H. (2020). Thymoquinone, an active compound of nigella sativa: role in prevention and treatment of cancer. Curr. Pharm. Biotechnol. 21, 1028–1041. doi:10.2174/1389201021666200416092743
Almatroodi, S. A., Almatroudi, A., Khan, A. A., and Rahmani, A. H. (2023). Potential therapeutic targets of formononetin, a type of methoxylated isoflavone, and its role in cancer therapy through the modulation of signal transduction pathways. Int. J. Mol. Sci. 24, 9719. doi:10.3390/ijms24119719
Asgharian, P., Tazekand, A. P., Hosseini, K., Forouhandeh, H., Ghasemnejad, T., Ranjbar, M., et al. (2022). Potential mechanisms of quercetin in cancer prevention: focus on cellular and molecular targets. Cancer Cell Int. 22, 257. doi:10.1186/s12935-022-02677-w
Ashikawa, K., Majumdar, S., Banerjee, S., Bharti, A. C., Shishodia, S., and Aggarwal, B. B. (2002). Piceatannol inhibits TNF-induced NF-kappaB activation and NF-kappaB-mediated gene expression through suppression of IkappaBalpha kinase and p65 phosphorylation. J. Immunol. 169, 6490–6497. doi:10.4049/jimmunol.169.11.6490
Asih, P. R., Prikas, E., Stefanoska, K., Tan, A. R. P., Ahel, H. I., and Ittner, A. (2020). Functions of p38 MAP kinases in the central nervous system. Front. Mol. Neurosci. 13, 570586. doi:10.3389/fnmol.2020.570586
Azios, N. G., Krishnamoorthy, L., Harris, M., Cubano, L. A., Cammer, M., and Dharmawardhane, S. F. (2007). Estrogen and resveratrol regulate Rac and Cdc42 signaling to the actin cytoskeleton of metastatic breast cancer cells. Neoplasia (New York, N.Y.) 9, 147–158. doi:10.1593/neo.06778
Bai, Y., Chen, J., Hu, W., Wang, L., Wu, Y., and Yu, S. (2022). Silibinin therapy improves cholangiocarcinoma outcomes by regulating ERK/mitochondrial pathway. Front. Pharmacol. 13, 847905. doi:10.3389/fphar.2022.847905
Bakrim, S., El Omari, N., El Hachlafi, N., Bakri, Y., Lee, L.-H., and Bouyahya, A. (2022). Dietary phenolic compounds as anticancer natural drugs: recent update on molecular mechanisms and clinical trials. Foods 11, 3323. doi:10.3390/foods11213323
Balaji, C., Muthukumaran, J., and Nalini, N. (2014). Chemopreventive effect of sinapic acid on 1,2-dimethylhydrazine-induced experimental rat colon carcinogenesis. Hum. Exp. Toxicol. 33, 1253–1268. doi:10.1177/0960327114522501
Balakrishna, P., George, S., Hatoum, H., and Mukherjee, S. (2021). Serotonin pathway in cancer. Int. J. Mol. Sci. 22, 1268. doi:10.3390/ijms22031268
Bhat, S. S., Prasad, S. K., Shivamallu, C., Prasad, K. S., Syed, A., Reddy, P., et al. (2021). Genistein: a potent anti-breast cancer agent. Curr. issues Mol. Biol. 43, 1502–1517. doi:10.3390/cimb43030106
Bispo, J. A. B., Pinheiro, P. S., and Kobetz, E. K. (2020). Epidemiology and etiology of leukemia and lymphoma. Cold Spring Harb. Perspect. Med. 10, a034819. doi:10.1101/cshperspect.a034819
Biswas, P., Dey, D., Biswas, P. K., Rahaman, T. I., Saha, S., Parvez, A., et al. (2022). A comprehensive analysis and anti-cancer activities of quercetin in ROS-mediated cancer and cancer stem cells. Int. J. Mol. Sci. 23, 11746. doi:10.3390/ijms231911746
Borges, G. A., Elias, S. T., Amorim, B., de Lima, C. L., Coletta, R. D., Castilho, R. M., et al. (2020). Curcumin downregulates the PI3K-AKT-mTOR pathway and inhibits growth and progression in head and neck cancer cells. Phytotherapy Res. 34, 3311–3324. doi:10.1002/ptr.6780
Brouckaert, G., Kalai, M., Krysko, D. V., Saelens, X., Vercammen, D., Ndlovu, M. N., et al. (2004). Phagocytosis of necrotic cells by macrophages is phosphatidylserine dependent and does not induce inflammatory cytokine production. Mol. Biol. Cell 15, 1089–1100. doi:10.1091/mbc.e03-09-0668
Brown, J. S., Amend, S. R., Austin, R. H., Gatenby, R. A., Hammarlund, E. U., and Pienta, K. J. (2023). Updating the definition of cancer. Mol. cancer Res. MCR 21, 1142–1147. doi:10.1158/1541-7786.MCR-23-0411
Buhrmann, C., Yazdi, M., Popper, B., Shayan, P., Goel, A., Aggarwal, B. B., et al. (2019). Evidence that TNF-β induces proliferation in colorectal cancer cells and resveratrol can down-modulate it. Exp. Biol. Med. (Maywood, N.J.) 244, 1–12. doi:10.1177/1535370218824538
Burdusel, A. C., Gherasim, O., Grumezescu, A., Mogoantă, L., Ficai, A., and Andronescu, E. (2018). Biomedical applications of silver nanoparticles: an up-to-date overview. Nanomaterials 8, 681. doi:10.3390/nano8090681
Cargnello, M., and Roux, P. P. (2011). Activation and function of the MAPKs and their substrates, the MAPK-activated protein kinases. Microbiol. Mol. Biol. Rev. 75, 50–83. doi:10.1128/MMBR.00031-10
Chan, L.-P., Chou, T.-H., Ding, H.-Y., Chen, P.-R., Chiang, F.-Y., Kuo, P.-L., et al. (2012). Apigenin induces apoptosis via tumor necrosis factor receptor- and Bcl-2-mediated pathway and enhances susceptibility of head and neck squamous cell carcinoma to 5-fluorouracil and cisplatin. Biochimica biophysica acta 1820, 1081–1091. doi:10.1016/j.bbagen.2012.04.013
Chang, C.-I., Chien, W.-C., Huang, K.-X., and Hsu, J.-L. (2017). Anti-inflammatory effects of vitisinol A and four other oligostilbenes from ampelopsis brevipedunculata var. Hancei. Molecules 22, 1195. doi:10.3390/molecules22071195
Chang, Y. J., Hsu, S. L., Liu, Y. T., Lin, Y. H., Lin, M. H., Huang, S. J., et al. (2015). Gallic acid induces necroptosis via TNF-α signaling pathway in activated hepatic stellate cells. PloS one 10, e0120713. doi:10.1371/journal.pone.0120713
Chao, J.-I., Su, W.-C., and Liu, H.-F. (2007). Baicalein induces cancer cell death and proliferation retardation by the inhibition of CDC2 kinase and survivin associated with opposite role of p38 mitogen-activated protein kinase and AKT. Mol. cancer Ther. 6, 3039–3048. doi:10.1158/1535-7163.MCT-07-0281
Chebet, J. J., Ehiri, J. E., McClelland, D. J., Taren, D., and Hakim, I. A. (2021). Effect of d-limonene and its derivatives on breast cancer in human trials: a scoping review and narrative synthesis. BMC cancer 21, 902. doi:10.1186/s12885-021-08639-1
Chen, J. (2016). The cell-cycle arrest and apoptotic functions of p53 in tumor initiation and progression. Cold Spring Harb. Perspect. Med. 6, a026104. doi:10.1101/cshperspect.a026104
Chen, J., Ye, C., Wan, C., Li, G., Peng, L., Peng, Y., et al. (2021). The roles of c-jun N-terminal kinase (JNK) in infectious diseases. Int. J. Mol. Sci. 22, 9640. doi:10.3390/ijms22179640
Chen, J.-C., Wu, C.-H., Peng, Y.-S., Zheng, H.-Y., Lin, Y.-C., Ma, P.-F., et al. (2018). Astaxanthin enhances erlotinib-induced cytotoxicity by p38 MAPK mediated xeroderma pigmentosum complementation group C (XPC) down-regulation in human lung cancer cells. Toxicol. Res. 7, 1247–1256. doi:10.1039/c7tx00292k
Chen, K., Zhang, S., Ji, Y., Li, J., An, P., Ren, H., et al. (2013b). Baicalein inhibits the invasion and metastatic capabilities of hepatocellular carcinoma cells via down-regulation of the ERK pathway. PloS one 8, e72927. doi:10.1371/journal.pone.0072927
Chen, K.-C., Chen, C.-Y., Lin, C.-R., Yang, T.-Y., Chen, T.-H., Wu, L.-C., et al. (2013a). Luteolin attenuates TGF-β1-induced epithelial-mesenchymal transition of lung cancer cells by interfering in the PI3K/Akt-NF-κB-Snail pathway. Life Sci. 93, 924–933. doi:10.1016/j.lfs.2013.10.004
Chen, Q., Zheng, Y., Jiao, D., Chen, F., Hu, H., Wu, Y., et al. (2014a). Curcumin inhibits lung cancer cell migration and invasion through Rac1-dependent signaling pathway. J. Nutr. Biochem. 25, 177–185. doi:10.1016/j.jnutbio.2013.10.004
Chen, S., Wang, Z., Huang, Y., O’Barr, S. A., Wong, R. A., Yeung, S., et al. (2014b). Ginseng and anticancer drug combination to improve cancer chemotherapy: a critical review. Evidence-based complementary Altern. Med. 2014, 168940. doi:10.1155/2014/168940
Cheng, A.-W., Tan, X., Sun, J.-Y., Gu, C.-M., Liu, C., and Guo, X. (2019). Catechin attenuates TNF-α induced inflammatory response via AMPK-SIRT1 pathway in 3T3-L1 adipocytes. PloS one 14, e0217090. doi:10.1371/journal.pone.0217090
Cheng, L., Xia, F., Li, Z., Shen, C., Yang, Z., Hou, H., et al. (2023). Structure, function and drug discovery of GPCR signaling. Mol. Biomed. 4, 46. doi:10.1186/s43556-023-00156-w
Cheon, C., and Ko, S.-G. (2022). Synergistic effects of natural products in combination with anticancer agents in prostate cancer: a scoping review. Front. Pharmacol. 13, 963317. doi:10.3389/fphar.2022.963317
Choudhari, A. S., Mandave, P. C., Deshpande, M., Ranjekar, P., and Prakash, O. (2019). Phytochemicals in cancer treatment: from preclinical studies to clinical practice. Front. Pharmacol. 10, 1614. doi:10.3389/fphar.2019.01614
Chung, K.-S., Choi, J.-H., Back, N.-I., Choi, M.-S., Kang, E.-K., Chung, H.-G., et al. (2010). Eupafolin, a flavonoid isolated from Artemisia princeps, induced apoptosis in human cervical adenocarcinoma HeLa cells. Mol. Nutr. food Res. 54, 1318–1328. doi:10.1002/mnfr.200900305
Collins, H. M., Abdelghany, M. K., Messmer, M., Yue, B., Deeves, S. E., Kindle, K. B., et al. (2013). Differential effects of garcinol and curcumin on histone and p53 modifications in tumour cells. BMC cancer 13, 37. doi:10.1186/1471-2407-13-37
Cruz, M. A. A. S., Coimbra, P. P. S., Araújo-Lima, C. F., Freitas-Silva, O., and Teodoro, A. J. (2024). Hybrid fruits for improving health-A comprehensive review. Foods 13, 219. doi:10.3390/foods13020219
Cui, J., Li, X., Wang, S., Su, Y., Chen, X., Cao, L., et al. (2020). Triptolide prevents bone loss via suppressing osteoclastogenesis through inhibiting PI3K-AKT-NFATc1 pathway. J. Cell. Mol. Med. 24, 6149–6161. doi:10.1111/jcmm.15229
Dai, Q., Franke, A. A., Jin, F., Shu, X.-O., Hebert, J. R., Custer, L. J., et al. (2002). Urinary excretion of phytoestrogens and risk of breast cancer among Chinese women in Shanghai. Cancer Epidemiol. biomarkers Prev. 11, 815–821.
Dembitsky, V. M., Gloriozova, T. A., and Poroikov, V. V. (2021). Antitumor profile of carbon-bridged steroids (CBS) and triterpenoids. Mar. drugs 19, 324. doi:10.3390/md19060324
Dhokia, V., Moss, J. A. Y., Macip, S., and Fox, J. L. (2022). At the crossroads of life and death: the proteins that influence cell fate decisions. Cancers 14, 2745. doi:10.3390/cancers14112745
Dhyani, P., Quispe, C., Sharma, E., Bahukhandi, A., Sati, P., Attri, D. C., et al. (2022). Anticancer potential of alkaloids: a key emphasis to colchicine, vinblastine, vincristine, vindesine, vinorelbine and vincamine. Cancer Cell Int. 22, 206. doi:10.1186/s12935-022-02624-9
Djavan, B., and Nasu, Y. (2001). Prostate cancer gene therapy-what have we learned and where are we going? Rev. urology 3, 179–186.
Dos Santos, M. B., Bertholin Anselmo, D., de Oliveira, J. G., Jardim-Perassi, B. V., Alves Monteiro, D., Silva, G., et al. (2019). Antiproliferative activity and p53 upregulation effects of chalcones on human breast cancer cells. J. enzyme inhibition Med. Chem. 34, 1093–1099. doi:10.1080/14756366.2019.1615485
Dowling, J. J., Weihl, C. C., and Spencer, M. J. (2021). Molecular and cellular basis of genetically inherited skeletal muscle disorders. Nat. Rev. Mol. Cell Biol. 22, 713–732. doi:10.1038/s41580-021-00389-z
Duan, W.-J., Li, Q.-S., Xia, M.-Y., Tashiro, S.-I., Onodera, S., and Ikejima, T. (2011). Silibinin activated ROS-p38-NF-κB positive feedback and induced autophagic death in human fibrosarcoma HT1080 cells. J. Asian Nat. Prod. Res. 13, 27–35. doi:10.1080/10286020.2010.540757
Efferth, T., Saeed, M. E. M., Mirghani, E., Alim, A., Yassin, Z., Saeed, E., et al. (2017). Integration of phytochemicals and phytotherapy into cancer precision medicine. Oncotarget 8, 50284–50304. doi:10.18632/oncotarget.17466
Elekofehinti, O. O., Iwaloye, O., Olawale, F., and Ariyo, E. O. (2021). Saponins in cancer treatment: current progress and future prospects. Pathophysiol. official J. Int. Soc. Pathophysiol. 28, 250–272. doi:10.3390/pathophysiology28020017
Elmore, S. (2007). Apoptosis: a review of programmed cell death. Toxicol. Pathol. 35, 495–516. doi:10.1080/01926230701320337
Evangelatos, G., Bamias, G., Kitas, G. D., Kollias, G., and Sfikakis, P. P. (2022). The second decade of anti-TNF-a therapy in clinical practice: new lessons and future directions in the COVID-19 era. Rheumatol. Int. 42, 1493–1511. doi:10.1007/s00296-022-05136-x
Falcicchia, C., Tozzi, F., Arancio, O., Watterson, D. M., and Origlia, N. (2020). Involvement of p38 MAPK in synaptic function and dysfunction. Int. J. Mol. Sci. 21, 5624. doi:10.3390/ijms21165624
Fawaz, A., Ferraresi, A., and Isidoro, C. (2023). Systems biology in cancer diagnosis integrating omics technologies and artificial intelligence to support physician decision making. J. personalized Med. 13, 1590. doi:10.3390/jpm13111590
Fu, H., Wang, C., Yang, D., Wei, Z., Xu, J., Hu, Z., et al. (2018). Curcumin regulates proliferation, autophagy, and apoptosis in gastric cancer cells by affecting PI3K and P53 signaling. J. Cell. physiology 233, 4634–4642. doi:10.1002/jcp.26190
G, M. S., Swetha, M., Keerthana, C. K., Rayginia, T. P., and Anto, R. J. (2021). Cancer chemoprevention: a strategic approach using phytochemicals. Front. Pharmacol. 12, 809308. doi:10.3389/fphar.2021.809308
Gan, D., He, W., Yin, H., and Gou, X. (2020). β-elemene enhances cisplatin-induced apoptosis in bladder cancer cells through the ROS-AMPK signaling pathway. Oncol. Lett. 19, 291–300. doi:10.3892/ol.2019.11103
Ganguly, P., Macleod, T., Wong, C., Harland, M., and McGonagle, D. (2023). Revisiting p38 mitogen-activated protein kinases (MAPK) in inflammatory arthritis: a narrative of the emergence of MAPK-activated protein kinase inhibitors (MK2i). Pharm. (Basel) 16, 1286. doi:10.3390/ph16091286
Gao, Y., Yin, J., Rankin, G. O., and Chen, Y. C. (2018). Kaempferol induces G2/M cell cycle arrest via checkpoint kinase 2 and promotes apoptosis via death receptors in human ovarian carcinoma A2780/CP70 cells. Molecules 23, 1095. doi:10.3390/molecules23051095
George, B. P., Chandran, R., and Abrahamse, H. (2021). Role of phytochemicals in cancer chemoprevention: insights. Antioxidants (Basel) 10, 1455. doi:10.3390/antiox10091455
Gnesutta, N., and Minden, A. (2003). Death receptor-induced activation of initiator caspase 8 is antagonized by serine/threonine kinase PAK4. Mol. Cell. Biol. 23, 7838–7848. doi:10.1128/MCB.23.21.7838-7848.2003
Gómez-Virgilio, L., Silva-Lucero, M.-D.-C., Flores-Morelos, D.-S., Gallardo-Nieto, J., Lopez-Toledo, G., Abarca-Fernandez, A.-M., et al. (2022). Autophagy: a key regulator of homeostasis and disease: an overview of molecular mechanisms and modulators. Cells 11, 2262. doi:10.3390/cells11152262
Granado-Serrano, A. B., Martín, M. Á., Bravo, L., Goya, L., and Ramos, S. (2012). Quercetin attenuates TNF-induced inflammation in hepatic cells by inhibiting the NF-κB pathway. Nutr. cancer 64, 588–598. doi:10.1080/01635581.2012.661513
Green, D. R. (2022). The death receptor pathway of apoptosis. Cold Spring Harb. Perspect. Biol. 14, a041053. doi:10.1101/cshperspect.a041053
Guillamón, E., Mut-Salud, N., Rodríguez-Sojo, M. J., Ruiz-Malagón, A. J., Cuberos-Escobar, A., Martínez-Férez, A., et al. (2023). In vitro antitumor and anti-inflammatory activities of allium-derived compounds propyl propane thiosulfonate (PTSO) and propyl propane thiosulfinate (PTS). Nutrients 15, 1363. doi:10.3390/nu15061363
Gulati, N., Laudet, B., Zohrabian, V. M., Murali, R., and Jhanwar-Uniyal, M. (2006). The antiproliferative effect of Quercetin in cancer cells is mediated via inhibition of the PI3K-Akt/PKB pathway. Anticancer Res. 26, 1177–1181.
Guo, Y.-B., Bao, X.-J., Xu, S.-B., Zhang, X.-D., and Liu, H.-Y. (2015). Honokiol induces cell cycle arrest and apoptosis via p53 activation in H4 human neuroglioma cells. Int. J. Clin. Exp. Med. 8, 7168–7175.
Gupta, S. C., Patchva, S., Koh, W., and Aggarwal, B. B. (2012). Discovery of curcumin, a component of golden spice, and its miraculous biological activities. Clin. Exp. Pharmacol. physiology 39, 283–299. doi:10.1111/j.1440-1681.2011.05648.x
Gv, V., Ranganathan, P. J., and Palati, S. (2023). Tangeretin’s anti-apoptotic signaling mechanisms in oral cancer cells: in vitro anti-cancer activity. Cureus 15, e47452. doi:10.7759/cureus.47452
Hafeez, B. B., Mustafa, A., Fischer, J. W., Singh, A., Zhong, W., Shekhani, M. O., et al. (2014). α-Mangostin: a dietary antioxidant derived from the pericarp of Garcinia mangostana L. inhibits pancreatic tumor growth in xenograft mouse model. Antioxidants redox Signal. 21, 682–699. doi:10.1089/ars.2013.5212
Hehlgans, T., and Pfeffer, K. (2005). The intriguing biology of the tumour necrosis factor/tumour necrosis factor receptor superfamily: players, rules and the games. Immunology 115, 1–20. doi:10.1111/j.1365-2567.2005.02143.x
Ho, J. F. V., Yaakup, H., Low, G. S. H., Wong, S. L., Tho, L. M., and Tan, S. B. (2020). Morphine use for cancer pain: a strong analgesic used only at the end of life? A qualitative study on attitudes and perceptions of morphine in patients with advanced cancer and their caregivers. Palliat. Med. 34, 619–629. doi:10.1177/0269216320904905
Hoffmann, J. C., Pappa, A., Krammer, P. H., and Lavrik, I. N. (2009). A new C-terminal cleavage product of procaspase-8, p30, defines an alternative pathway of procaspase-8 activation. Mol. Cell. Biol. 29, 4431–4440. doi:10.1128/MCB.02261-07
Hong, T.-K., Song, J.-H., Lee, S.-B., and Do, J.-T. (2021). Germ cell derivation from pluripotent stem cells for understanding in vitro gametogenesis. Cells 10, 1889. doi:10.3390/cells10081889
Hu, C., and He, Y. (2021). Formononetin inhibits non-small cell lung cancer proliferation via regulation of mir-27a-3p through p53 pathway. Oncologie 23, 241–250. doi:10.32604/Oncologie.2021.015828
Hu, Y., and Reggiori, F. (2022). Molecular regulation of autophagosome formation. Biochem. Soc. Trans. 50, 55–69. doi:10.1042/BST20210819
Huang, Y., Wang, G., Zhang, N., and Zeng, X. (2024). MAP3K4 kinase action and dual role in cancer. Discov. Oncol. 15, 99. doi:10.1007/s12672-024-00961-x
Hun Lee, J., Shu, L., Fuentes, F., Su, Z.-Y., and Tony Kong, A.-N. (2013). Cancer chemoprevention by traditional Chinese herbal medicine and dietary phytochemicals: targeting nrf2-mediated oxidative stress/anti-inflammatory responses, epigenetics, and cancer stem cells. J. traditional complementary Med. 3, 69–79. doi:10.4103/2225-4110.107700
Hussain, Y., Cui, J. H., Khan, H., Aschner, M., Batiha, G. E.-S., and Jeandet, P. (2021). Luteolin and cancer metastasis suppression: focus on the role of epithelial to mesenchymal transition. Med. Oncol. N. Lond. Engl. 38, 66. doi:10.1007/s12032-021-01508-8
Ilyas, S., Simanullang, R. H., Hutahaean, S., Rosidah, R., and Situmorang, P. C. (2021). Effect of Zanthoxylum acanthopodium methanol extract on CDK4 expression to cervical cancer. Res. J Pharm. Technol. 14 (11), 5647–5652. doi:10.52711/0974-360X.2021.00982
Ilyas, S., Simanullang, R. H., Hutahaean, S., Rosidah, R., and Situmorang, P. C. (2022). Correlation of myc expression with Wee1 expression by Zanthoxylum acanthopodium in cervical carcinoma histology. Pak J. Biol. Sci. 25 (11), 1014–1020. doi:10.3923/pjbs.2022.1014.1020
Imai-Sumida, M., Chiyomaru, T., Majid, S., Saini, S., Nip, H., Dahiya, R., et al. (2017). Silibinin suppresses bladder cancer through down-regulation of actin cytoskeleton and PI3K/Akt signaling pathways. Oncotarget 8, 92032–92042. doi:10.18632/oncotarget.20734
Irianti, E., Ilyas, S., Hutahaean, S., Rosidah, , and Situmorang, P. C. (2020). Placental histological on preeclamptic rats (Rattus norvegicus) after administration of nanoherbal haramonting (Rhodomyrtus tomentosa). Res. J. Pharm. Tech. 13 (8), 3879–3882. doi:10.5958/0974-360X.2020.00686.1
Jang, J. H., Yang, E. S., Min, K.-J., and Kwon, T. K. (2012). Inhibitory effect of butein on tumor necrosis factor-α-induced expression of cell adhesion molecules in human lung epithelial cells via inhibition of reactive oxygen species generation, NF-κB activation and Akt phosphorylation. Int. J. Mol. Med. 30, 1357–1364. doi:10.3892/ijmm.2012.1158
Jang, J. Y., Im, E., and Kim, N. D. (2022). Mechanism of resveratrol-induced programmed cell death and new drug discovery against cancer: a review. Int. J. Mol. Sci. 23, 13689. doi:10.3390/ijms232213689
Jeong, J.-H., Nakajima, H., Magae, J., Furukawa, C., Taki, K., Otsuka, K., et al. (2009). Ascochlorin activates p53 in a manner distinct from DNA damaging agents. Int. J. cancer 124, 2797–2803. doi:10.1002/ijc.24259
Jia, S., Xu, X., Zhou, S., Chen, Y., Ding, G., and Cao, L. (2019). Fisetin induces autophagy in pancreatic cancer cells via endoplasmic reticulum stress- and mitochondrial stress-dependent pathways. Cell death Dis. 10, 142. doi:10.1038/s41419-019-1366-y
Jin, X., Che, D.-B., Zhang, Z.-H., Yan, H.-M., Jia, Z.-Y., and Jia, X.-B. (2016). Ginseng consumption and risk of cancer: a meta-analysis. J. ginseng Res. 40, 269–277. doi:10.1016/j.jgr.2015.08.007
Jo, J., Abdi Nansa, S., and Kim, D.-H. (2020). Molecular regulators of cellular mechanoadaptation at cell-material interfaces. Front. Bioeng. Biotechnol. 8, 608569. doi:10.3389/fbioe.2020.608569
Johnson, R., Dludla, P. V., Muller, C. J. F., Huisamen, B., Essop, M. F., and Louw, J. (2017). The transcription profile unveils the cardioprotective effect of aspalathin against lipid toxicity in an in vitro H9c2 model. Molecules 22, 219. doi:10.3390/molecules22020219
Jung, J. E., Kim, H. S., Lee, C. S., Park, D.-H., Kim, Y.-N., Lee, M.-J., et al. (2007). Caffeic acid and its synthetic derivative CADPE suppress tumor angiogenesis by blocking STAT3-mediated VEGF expression in human renal carcinoma cells. Carcinogenesis 28, 1780–1787. doi:10.1093/carcin/bgm130
Kang, S. H., Bak, D.-H., Chung, B. Y., Bai, H.-W., and Kang, B. S. (2020). Delphinidin enhances radio-therapeutic effects via autophagy induction and JNK/MAPK pathway activation in non-small cell lung cancer. Korean J. physiology Pharmacol. 24, 413–422. doi:10.4196/kjpp.2020.24.5.413
Kanwal, N., Rasul, A., Hussain, G., Anwar, H., Shah, M. A., Sarfraz, I., et al. (2020). Oleandrin: a bioactive phytochemical and potential cancer killer via multiple cellular signaling pathways. Food Chem. Toxicol. 143, 111570. doi:10.1016/j.fct.2020.111570
Kashafi, E., Moradzadeh, M., Mohamadkhani, A., and Erfanian, S. (2017). Kaempferol increases apoptosis in human cervical cancer HeLa cells via PI3K/AKT and telomerase pathways. Biomed. Pharmacother. 89, 573–577. doi:10.1016/j.biopha.2017.02.061
Kast, R. E. (2003). Ribavirin in cancer immunotherapies: controlling nitric oxide augments cytotoxic lymphocyte function. Neoplasia (New York, N.Y.) 5, 3–8. doi:10.1016/s1476-5586(03)80011-8
Kavitha, K., Kowshik, J., Kishore, T. K. K., Baba, A. B., and Nagini, S. (2013). Astaxanthin inhibits NF-κB and Wnt/β-catenin signaling pathways via inactivation of Erk/MAPK and PI3K/Akt to induce intrinsic apoptosis in a hamster model of oral cancer. Biochimica biophysica acta 1830, 4433–4444. doi:10.1016/j.bbagen.2013.05.032
Kciuk, M., Alam, M., Ali, N., Rashid, S., Głowacka, P., Sundaraj, R., et al. (2023). Epigallocatechin-3-Gallate therapeutic potential in cancer: mechanism of action and clinical implications. Molecules 28, 5246. doi:10.3390/molecules28135246
Kciuk, M., Gielecińska, A., Budzinska, A., Mojzych, M., and Kontek, R. (2022). Metastasis and MAPK pathways. Int. J. Mol. Sci. 23, 3847. doi:10.3390/ijms23073847
Khafaga, D. S. R., El-Khawaga, A. M., Elfattah Mohammed, R. A., and Abdelhakim, H. K. (2023). Green synthesis of nano-based drug delivery systems developed for hepatocellular carcinoma treatment: a review. Mol. Biol. Rep. 50, 10351–10364. doi:10.1007/s11033-023-08823-5
Kikuchi, H., Yuan, B., Yuhara, E., Takagi, N., and Toyoda, H. (2013). Involvement of histone H3 phosphorylation through p38 MAPK pathway activation in casticin-induced cytocidal effects against the human promyelocytic cell line HL-60. Int. J. Oncol. 43, 2046–2056. doi:10.3892/ijo.2013.2106
Kim, H., Lee, M.-J., Kim, J.-E., Park, S.-D., Moon, H.-I., and Park, W.-H. (2010). Genistein suppresses tumor necrosis factor-alpha-induced proliferation via the apoptotic signaling pathway in human aortic smooth muscle cells. J. Agric. food Chem. 58, 2015–2019. doi:10.1021/jf903802v
Kim, H.-J., Hwang, K.-E., Park, D.-S., Oh, S.-H., Jun, H. Y., Yoon, K.-H., et al. (2017). Shikonin-induced necroptosis is enhanced by the inhibition of autophagy in non-small cell lung cancer cells. J. Transl. Med. 15, 123. doi:10.1186/s12967-017-1223-7
Kim, M. E., Na, J. Y., and Lee, J. S. (2018). Anti-inflammatory effects of trans-cinnamaldehyde on lipopolysaccharide-stimulated macrophage activation via MAPKs pathway regulation. Immunopharmacol. Immunotoxicol. 40, 219–224. doi:10.1080/08923973.2018.1424902
Kim, M. S., Kang, H. J., and Moon, A. (2001). Inhibition of invasion and induction of apoptosis by curcumin in H-ras-transformed MCF10A human breast epithelial cells. Archives pharmacal Res. 24, 349–354. doi:10.1007/BF02975105
Kim, N., Chung, G., Son, S.-R., Park, J. H., Lee, Y. H., Park, K.-T., et al. (2023). Magnolin inhibits paclitaxel-induced cold allodynia and ERK1/2 activation in mice. Plants 12, 2283. doi:10.3390/plants12122283
King, S. J., Worth, D. C., Scales, T. M. E., Monypenny, J., Jones, G. E., and Parsons, M. (2011). β1 integrins regulate fibroblast chemotaxis through control of N-WASP stability. EMBO J. 30, 1705–1718. doi:10.1038/emboj.2011.82
Kłósek, M., Mertas, A., Król, W., Jaworska, D., Szymszal, J., and Szliszka, E. (2016). Tumor necrosis factor-related apoptosis-inducing ligand-induced apoptosis in prostate cancer cells after treatment with xanthohumol-A natural compound present in humulus lupulus L. Int. J. Mol. Sci. 17, 837. doi:10.3390/ijms17060837
Ko, A. M.-S., Tu, H.-P., and Ko, Y.-C. (2023). Systematic review of roles of arecoline and arecoline N-oxide in oral cancer and strategies to block carcinogenesis. Cells 12, 1208. doi:10.3390/cells12081208
Kou, Y., Tong, B., Wu, W., Liao, X., and Zhao, M. (2020). Berberine improves chemo-sensitivity to cisplatin by enhancing cell apoptosis and repressing PI3K/AKT/mTOR signaling pathway in gastric cancer. Front. Pharmacol. 11, 616251. doi:10.3389/fphar.2020.616251
Koul, H. K., Pal, M., and Koul, S. (2013). Role of p38 MAP kinase signal transduction in solid tumors. Genes & cancer 4, 342–359. doi:10.1177/1947601913507951
Kretschmer, N., Rinner, B., Stuendl, N., Kaltenegger, H., Wolf, E., Kunert, O., et al. (2012). Effect of costunolide and dehydrocostus lactone on cell cycle, apoptosis, and ABC transporter expression in human soft tissue sarcoma cells. Planta medica. 78, 1749–1756. doi:10.1055/s-0032-1315385
Kurniasih, N., Supriadin, A., Harneti, D., Abdulah, R., Azmi, M., and Supratman, U. (2021). Ergosterol peroxide and stigmasterol from the stembark of aglaia simplicifolia (meliaceae) and their cytotoxic against HeLa cervical cancer cell lines. J. Kim. Val. 1, 46–51. doi:10.15408/jkv.v1i1.20068
Kwon, O. S., Jung, J. H., Shin, E. A., Park, J. E., Park, W. Y., and Kim, S.-H. (2020). Epigallocatechin-3-Gallate induces apoptosis as a TRAIL sensitizer via activation of caspase 8 and death receptor 5 in human colon cancer cells. Biomedicines 8, 84. doi:10.3390/biomedicines8040084
Lecomte, N., Njardarson, J. T., Nagorny, P., Yang, G., Downey, R., Ouerfelli, O., et al. (2011). Emergence of potent inhibitors of metastasis in lung cancer via syntheses based on migrastatin. Proc. Natl. Acad. Sci. U. S. A. 108, 15074–15078. doi:10.1073/pnas.1015247108
Lee, C.-H., Ying, T.-H., Chiou, H.-L., Hsieh, S.-C., Wen, S.-H., Chou, R.-H., et al. (2017). Alpha-mangostin induces apoptosis through activation of reactive oxygen species and ASK1/p38 signaling pathway in cervical cancer cells. Oncotarget 8, 47425–47439. doi:10.18632/oncotarget.17659
Lee, D.-Y., Yun, S.-M., Song, M.-Y., Jung, K., and Kim, E.-H. (2020a). Cyanidin chloride induces apoptosis by inhibiting NF-κB signaling through activation of Nrf2 in colorectal cancer cells. Antioxidants (Basel) 9, 285. doi:10.3390/antiox9040285
Lee, H.-P., Li, T.-M., Tsao, J.-Y., Fong, Y.-C., and Tang, C.-H. (2012). Curcumin induces cell apoptosis in human chondrosarcoma through extrinsic death receptor pathway. Int. Immunopharmacol. 13, 163–169. doi:10.1016/j.intimp.2012.04.002
Lee, M.-G., Lee, K.-S., and Nam, K.-S. (2020b). Anti-metastatic effects of arctigenin are regulated by MAPK/AP-1 signaling in 4T-1 mouse breast cancer cells. Mol. Med. Rep. 21, 1374–1382. doi:10.3892/mmr.2020.10937
Lee, M.-S., Yuet-Wa, J. C., Kong, S.-K., Yu, B., Eng-Choon, V. O., Nai-Ching, H. W., et al. (2005). Effects of polyphyllin D, a steroidal saponin in Paris polyphylla, in growth inhibition of human breast cancer cells and in xenograft. Cancer Biol. Ther. 4, 1248–1254. doi:10.4161/cbt.4.11.2136
Li, C., Yang, X., Chen, C., Cai, S., and Hu, J. (2014). Isorhamnetin suppresses colon cancer cell growth through the PI3K-Akt-mTOR pathway. Mol. Med. Rep. 9, 935–940. doi:10.3892/mmr.2014.1886
Li, D., Wang, G., Jin, G., Yao, K., Zhao, Z., Bie, L., et al. (2019). Resveratrol suppresses colon cancer growth by targeting the AKT/STAT3 signaling pathway. Int. J. Mol. Med. 43, 630–640. doi:10.3892/ijmm.2018.3969
Li, H., Du, G., Yang, L., Pang, L., and Zhan, Y. (2020). The antitumor effects of britanin on hepatocellular carcinoma cells and its real-time evaluation by in vivo bioluminescence imaging. Anti-cancer agents Med. Chem. 20, 1147–1156. doi:10.2174/1871520620666200227092623
Li, M., and Beg, A. A. (2000). Induction of necrotic-like cell death by tumor necrosis factor alpha and caspase inhibitors: novel mechanism for killing virus-infected cells. J. virology 74, 7470–7477. doi:10.1128/jvi.74.16.7470-7477.2000
Li, R., Inbaraj, B. S., and Chen, B.-H. (2023). Quantification of xanthone and anthocyanin in mangosteen peel by UPLC-MS/MS and preparation of nanoemulsions for studying their inhibition effects on liver cancer cells. Int. J. Mol. Sci. 24, 3934. doi:10.3390/ijms24043934
Li, S., Chen, J., Fan, Y., Wang, C., Wang, C., Zheng, X., et al. (2022). Liposomal Honokiol induces ROS-mediated apoptosis via regulation of ERK/p38-MAPK signaling and autophagic inhibition in human medulloblastoma. Signal Transduct. Target. Ther. 7, 49. doi:10.1038/s41392-021-00869-w
Li, X., Zhao, J., Yan, T., Mu, J., Lin, Y., Chen, J., et al. (2021). Cyanidin-3-O-glucoside and cisplatin inhibit proliferation and downregulate the PI3K/AKT/mTOR pathway in cervical cancer cells. J. food Sci. 86, 2700–2712. doi:10.1111/1750-3841.15740
Liang, F., Lv, Y., Qiao, X., Zhang, S., Shen, S., Wang, C., et al. (2023). Cinchonine-induced cell death in pancreatic cancer cells by downregulating RRP15. Cell Biol. Int. 47, 907–919. doi:10.1002/cbin.11987
Liang, X., Wang, P., Yang, C., Huang, F., Wu, H., Shi, H., et al. (2021). Galangin inhibits gastric cancer growth through enhancing STAT3 mediated ROS production. Front. Pharmacol. 12, 646628. doi:10.3389/fphar.2021.646628
Lim, W., Jeong, M., Bazer, F. W., and Song, G. (2016). Curcumin suppresses proliferation and migration and induces apoptosis on human placental choriocarcinoma cells via ERK1/2 and SAPK/JNK MAPK signaling pathways. Biol. reproduction 95, 83. doi:10.1095/biolreprod.116.141630
Lin, F.-L., Hsu, J.-L., Chou, C.-H., Wu, W.-J., Chang, C.-I., and Liu, H.-J. (2011). Activation of p38 MAPK by damnacanthal mediates apoptosis in SKHep 1 cells through the DR5/TRAIL and TNFR1/TNF-α and p53 pathways. Eur. J. Pharmacol. 650, 120–129. doi:10.1016/j.ejphar.2010.10.005
Lin, H.-F., Hsieh, M.-J., Hsi, Y.-T., Lo, Y.-S., Chuang, Y.-C., Chen, M.-K., et al. (2017). Celastrol-induced apoptosis in human nasopharyngeal carcinoma is associated with the activation of the death receptor and the mitochondrial pathway. Oncol. Lett. 14, 1683–1690. doi:10.3892/ol.2017.6346
Liu, M.-J., Wang, Z., Ju, Y., Wong, R. N.-S., and Wu, Q.-Y. (2005). Diosgenin induces cell cycle arrest and apoptosis in human leukemia K562 cells with the disruption of Ca2+ homeostasis. Cancer Chemother. Pharmacol. 55, 79–90. doi:10.1007/s00280-004-0849-3
Liu, Q., Xu, X., Zhao, M., Wei, Z., Li, X., Zhang, X., et al. (2015). Berberine induces senescence of human glioblastoma cells by downregulating the EGFR-MEK-ERK signaling pathway. Mol. cancer Ther. 14, 355–363. doi:10.1158/1535-7163.MCT-14-0634
Liu, T., He, Y., and Liao, Y. (2024). Anacardic acid inhibits the proliferation and inflammation of HaCaT cells induced by TNF- α via the regulation of NF - κB pathway. Trop. J. Pharm. Res. 23, 251–256. doi:10.4314/tjpr.v23i2.3
Lu, J.-J., Bao, J.-L., Chen, X.-P., Huang, M., and Wang, Y.-T. (2012). Alkaloids isolated from natural herbs as the anticancer agents. Evidence-based complementary Altern. Med. 2012, 485042. doi:10.1155/2012/485042
Łukasiewicz, S., Czeczelewski, M., Forma, A., Baj, J., Sitarz, R., and Stanisławek, A. (2021). Breast cancer-epidemiology, risk factors, classification, prognostic markers, and current treatment strategies-an updated review. Cancers 13, 4287. doi:10.3390/cancers13174287
Luo, K. W., Lung, W. Y., Chun-Xie, , Luo, X. L., and Huang, W. R. (2018). EGCG inhibited bladder cancer T24 and 5637 cell proliferation and migration via PI3K/AKT pathway. Oncotarget 9, 12261–12272. doi:10.18632/oncotarget.24301
Madureira, M. B., Concato, V. M., Cruz, E. M. S., Bitencourt de Morais, J. M., Inoue, F. S. R., Concimo Santos, N., et al. (2023). Naringenin and hesperidin as promising alternatives for prevention and Co-adjuvant therapy for breast cancer. Antioxidants (Basel) 12, 586. doi:10.3390/antiox12030586
Mahmoud, M. A., Okda, T. M., Omran, G. A., and Abd-Alhaseeb, M. M. (2021). Rosmarinic acid suppresses inflammation, angiogenesis, and improves paclitaxel induced apoptosis in a breast cancer model via NF3 κB-p53-caspase-3 pathways modulation. J. Appl. Biomed. 19, 202–209. doi:10.32725/jab.2021.024
Mak, T. W., and Yeh, W.-C. (2002). Signaling for survival and apoptosis in the immune system. Arthritis Res. 4 (Suppl. 3), S243–S252. doi:10.1186/ar569
Mari, A., Mani, G., Nagabhishek, S. N., Balaraman, G., Subramanian, N., Mirza, F. B., et al. (2021). Carvacrol promotes cell cycle arrest and apoptosis through PI3K/AKT signaling pathway in MCF-7 breast cancer cells. Chin. J. Integr. Med. 27, 680–687. doi:10.1007/s11655-020-3193-5
Martemucci, G., Costagliola, C., Mariano, M., D’andrea, L., Napolitano, P., and D’Alessandro, A. G. (2022). Free radical properties, source and targets, antioxidant consumption and health. Oxygen 2, 48–78. doi:10.3390/oxygen2020006
Martínez-Limón, A., Joaquin, M., Caballero, M., Posas, F., and de Nadal, E. (2020). The p38 pathway: from biology to cancer therapy. Int. J. Mol. Sci. 21, 1913. doi:10.3390/ijms21061913
Maxwell, T., Lee, K. S., Kim, S., and Nam, K.-S. (2018). Arctigenin inhibits the activation of the mTOR pathway, resulting in autophagic cell death and decreased ER expression in ER-positive human breast cancer cells. Int. J. Oncol. 52, 1339–1349. doi:10.3892/ijo.2018.4271
Mendonca, P., and Soliman, K. F. A. (2020). Flavonoids activation of the transcription factor Nrf2 as a hypothesis approach for the prevention and modulation of SARS-CoV-2 infection severity. Antioxidants (Basel) 9, 659. doi:10.3390/antiox9080659
Meng, X., and Shao, Z. (2021). Ambrosin exerts strong anticancer effects on human breast cancer cells via activation of caspase and inhibition of the Wnt/β-catenin pathway. Trop. J. Pharm. Res. 20, 809–814. doi:10.4314/tjpr.v20i4.22
Mi, X.-J., Choi, H. S., Perumalsamy, H., Shanmugam, R., Thangavelu, L., Balusamy, S. R., et al. (2022). Biosynthesis and cytotoxic effect of silymarin-functionalized selenium nanoparticles induced autophagy mediated cellular apoptosis via downregulation of PI3K/Akt/mTOR pathway in gastric cancer. Phytomedicine 99, 154014. doi:10.1016/j.phymed.2022.154014
Miatmoko, A., Mianing, E. A., Sari, R., and Hendradi, E. (2021). Nanoparticles use for delivering ursolic acid in cancer therapy: a scoping review. Front. Pharmacol. 12, 787226. doi:10.3389/fphar.2021.787226
Mijit, M., Caracciolo, V., Melillo, A., Amicarelli, F., and Giordano, A. (2020). Role of p53 in the regulation of cellular senescence. Biomolecules 10, 420. doi:10.3390/biom10030420
Miller, M. A., and Zachary, J. F. (2017). “Mechanisms and morphology of cellular injury, adaptation, and death,” in Pathologic basis of veterinary disease. Editor J. F. Zachary (Elsevier), 2–43 e19. doi:10.1016/B978-0-323-35775-3.00001-1
Min, H.-Y., and Lee, H.-Y. (2023). Cellular dormancy in cancer: mechanisms and potential targeting strategies. Cancer Res. Treat. 55, 720–736. doi:10.4143/crt.2023.468
Mukhtar, E., Adhami, V. M., Sechi, M., and Mukhtar, H. (2015). Dietary flavonoid fisetin binds to β-tubulin and disrupts microtubule dynamics in prostate cancer cells. Cancer Lett. 367, 173–183. doi:10.1016/j.canlet.2015.07.030
Musial, C., Kuban-Jankowska, A., and Gorska-Ponikowska, M. (2020). Beneficial properties of green tea catechins. Int. J. Mol. Sci. 21, 1744. doi:10.3390/ijms21051744
Ni, J., Wen, X., Yao, J., Chang, H.-C., Yin, Y., Zhang, M., et al. (2005). Tocopherol-associated protein suppresses prostate cancer cell growth by inhibition of the phosphoinositide 3-kinase pathway. Cancer Res. 65, 9807–9816. doi:10.1158/0008-5472.CAN-05-1334
Noori-Daloii, M. R., Momeny, M., Yousefi, M., Shirazi, F. G., Yaseri, M., Motamed, N., et al. (2011). Multifaceted preventive effects of single agent quercetin on a human prostate adenocarcinoma cell line (PC-3): implications for nutritional transcriptomics and multi-target therapy. Med. Oncol. N. Lond. Engl. 28, 1395–1404. doi:10.1007/s12032-010-9603-3
Nozhat, Z., Heydarzadeh, S., Memariani, Z., and Ahmadi, A. (2021). Chemoprotective and chemosensitizing effects of apigenin on cancer therapy. Cancer Cell Int. 21, 574. doi:10.1186/s12935-021-02282-3
Olofinsan, K., Abrahamse, H., and George, B. P. (2023). Therapeutic role of alkaloids and alkaloid derivatives in cancer management. Molecules 28, 5578. doi:10.3390/molecules28145578
Pai, J.-T., Hsu, M.-W., Leu, Y.-L., Chang, K.-T., and Weng, M.-S. (2021). Induction of G2/M cell cycle arrest via p38/p21(Waf1/Cip1)-Dependent signaling pathway activation by bavachinin in non-small-cell lung cancer cells. Molecules 26, 5161. doi:10.3390/molecules26175161
Pandey, N., Tyagi, G., Kaur, P., Pradhan, S., Rajam, M. V., and Srivastava, T. (2020). Allicin overcomes hypoxia mediated cisplatin resistance in lung cancer cells through ROS mediated cell death pathway and by suppressing hypoxia inducible factors. Cell. physiology Biochem. 54, 748–766. doi:10.33594/000000253
Pang, X., He, X., Qiu, Z., Zhang, H., Xie, R., Liu, Z., et al. (2023). Targeting integrin pathways: mechanisms and advances in therapy. Signal Transduct. Target. Ther. 8, 1. doi:10.1038/s41392-022-01259-6
Park, C., Jeong, J.-W., Han, M. H., Lee, H., Kim, G.-Y., Jin, S., et al. (2021). The anti-cancer effect of betulinic acid in u937 human leukemia cells is mediated through ROS-dependent cell cycle arrest and apoptosis. Animal cells Syst. 25, 119–127. doi:10.1080/19768354.2021.1915380
Park, M.-R., Kim, S.-G., Cho, I.-A., Oh, D., Kang, K.-R., Lee, S.-Y., et al. (2015). Licochalcone-A induces intrinsic and extrinsic apoptosis via ERK1/2 and p38 phosphorylation-mediated TRAIL expression in head and neck squamous carcinoma FaDu cells. Food Chem. Toxicol. 77, 34–43. doi:10.1016/j.fct.2014.12.013
Patel, K., and Patel, D. (2021). Biological activity of ascaridole for the treatment of cancers: phytopharmaceutical importance with molecular study. Ann. Hepato-Biliary-Pancreatic Surg. 25, S294. doi:10.14701/ahbps.EP-93
Peng, R., Xu, M., Xie, B., Min, Q., Hui, S., Du, Z., et al. (2023). Insights on antitumor activity and mechanism of natural benzophenanthridine alkaloids. Molecules 28, 6588. doi:10.3390/molecules28186588
Pirpour Tazehkand, A., Salehi, R., Velaei, K., and Samadi, N. (2020). The potential impact of trigonelline loaded micelles on Nrf2 suppression to overcome oxaliplatin resistance in colon cancer cells. Mol. Biol. Rep. 47, 5817–5829. doi:10.1007/s11033-020-05650-w
Pizzi, A. (2021). Tannins medical/pharmacological and related applications: a critical review. Sustain. Chem. Pharm. 22, 100481. doi:10.1016/j.scp.2021.100481
Qi, X., Jha, S. K., Jha, N. K., Dewanjee, S., Dey, A., Deka, R., et al. (2022). Antioxidants in brain tumors: current therapeutic significance and future prospects. Mol. cancer 21, 204. doi:10.1186/s12943-022-01668-9
Qin, R., You, F.-M., Zhao, Q., Xie, X., Peng, C., Zhan, G., et al. (2022). Naturally derived indole alkaloids targeting regulated cell death (RCD) for cancer therapy: from molecular mechanisms to potential therapeutic targets. J. Hematol. Oncol. 15, 133. doi:10.1186/s13045-022-01350-z
Quideau, S., Jourdes, M., Lefeuvre, D., Montaudon, D., Saucier, C., Glories, Y., et al. (2005). The chemistry of wine polyphenolic C-glycosidic ellagitannins targeting human topoisomerase II. Chem. 11, 6503–6513. doi:10.1002/chem.200500428
Rahmani, A. H., Almatroudi, A., Allemailem, K. S., Alwanian, W. M., Alharbi, B. F., Alrumaihi, F., et al. (2023). Myricetin: a significant emphasis on its anticancer potential via the modulation of inflammation and signal transduction pathways. Int. J. Mol. Sci. 24, 9665. doi:10.3390/ijms24119665
Rahmani, A. H., Alsahli, M. A., Almatroudi, A., Almogbel, M. A., Khan, A. A., Anwar, S., et al. (2022). The potential role of apigenin in cancer prevention and treatment. Molecules 27, 6051. doi:10.3390/molecules27186051
Ratan, Z. A., Haidere, M. F., Nurunnabi, M., Shahriar, S. M., Ahammad, A. J. S., Shim, Y. Y., et al. (2020). Green chemistry synthesis of silver nanoparticles and their potential anticancer effects. Cancers 12, 855. doi:10.3390/cancers12040855
Ray, R., Saha, S., and Paul, S. (2022). Two novel compounds, ergosterol and ergosta-5,8-dien-3-ol, from Termitomyces heimii Natarajan demonstrate promising anti-hepatocarcinoma activity. J. Traditional Chin. Med. Sci. 9, 443–453. doi:10.1016/j.jtcms.2022.09.006
Reddy, D., Kumavath, R., Barh, D., Azevedo, V., and Ghosh, P. (2020). Anticancer and antiviral properties of cardiac glycosides: a review to explore the mechanism of actions. Molecules 25, 3596. doi:10.3390/molecules25163596
Rigby, C. M., Roy, S., Deep, G., Guillermo-Lagae, R., Jain, A. K., Dhar, D., et al. (2017). Role of p53 in silibinin-mediated inhibition of ultraviolet B radiation-induced DNA damage, inflammation and skin carcinogenesis. Carcinogenesis 38, 40–50. doi:10.1093/carcin/bgw106
Safi, A., Heidarian, E., and Ahmadi, R. (2021). Quercetin synergistically enhances the anticancer efficacy of docetaxel through induction of apoptosis and modulation of PI3K/AKT, MAPK/ERK, and JAK/STAT3 signaling pathways in MDA-MB-231 breast cancer cell line. Int. J. Mol. Cell. Med. 10, 11–22. doi:10.22088/IJMCM.BUMS.10.1.11
Sali, V. K., Mani, S., Meenaloshani, G., Velmurugan Ilavarasi, A., and Vasanthi, H. R. (2020). Type 5 17-hydroxysteroid dehydrogenase/prostaglandin F synthase (AKR1C3) inhibition and potential anti-proliferative activity of cholest-4-ene-3,6-dione in MCF-7 breast cancer cells. Steroids 159, 108638. doi:10.1016/j.steroids.2020.108638
Sanchez-Martin, V., Plaza-Calonge, M. D. C., Soriano-Lerma, A., Ortiz-Gonzalez, M., Linde-Rodriguez, A., Perez-Carrasco, V., et al. (2022). Gallic acid: a natural phenolic compound exerting antitumoral activities in colorectal cancer via interaction with G-quadruplexes. Cancers 14, 2648. doi:10.3390/cancers14112648
Santarpia, L., Lippman, S. M., and El-Naggar, A. K. (2012). Targeting the MAPK-RAS-RAF signaling pathway in cancer therapy. Expert Opin. Ther. targets 16, 103–119. doi:10.1517/14728222.2011.645805
Schiliro, C., and Firestein, B. L. (2021). Mechanisms of metabolic reprogramming in cancer cells supporting enhanced growth and proliferation. Cells 10, 1056. doi:10.3390/cells10051056
Schneider-Brachert, W., Heigl, U., and Ehrenschwender, M. (2013). Membrane trafficking of death receptors: implications on signalling. Int. J. Mol. Sci. 14, 14475–14503. doi:10.3390/ijms140714475
Senthamizh, N., Vidhyavathi, R., Raj, E., Lobo, V., and Ramu, A. (2020). Reserpine subdued non-small cell lung cancer cells via ROS-mediated apoptosis. J. Conventional Knowl. Holist. Health 4, 1–4. doi:10.53517/JCKHH.2581-3331.422020206
Shen, J., Yang, Z., Wu, X., Yao, G., and Hou, M. (2023). Baicalein facilitates gastric cancer cell apoptosis by triggering endoplasmic reticulum stress via repression of the PI3K/AKT pathway. Appl. Biol. Chem. 66, 10. doi:10.1186/s13765-022-00759-x
Shi, R.-X., Ong, C.-N., and Shen, H.-M. (2004). Luteolin sensitizes tumor necrosis factor-alpha-induced apoptosis in human tumor cells. Oncogene 23, 7712–7721. doi:10.1038/sj.onc.1208046
Shiau, J.-P., Chuang, Y.-T., Tang, J.-Y., Yang, K.-H., Chang, F.-R., Hou, M.-F., et al. (2022). The impact of oxidative stress and AKT pathway on cancer cell functions and its application to natural products. Antioxidants 11, 1845. doi:10.3390/antiox11091845
Si, L., Fu, J., Liu, W., Hayashi, T., Nie, Y., Mizuno, K., et al. (2020). Silibinin inhibits migration and invasion of breast cancer MDA-MB-231 cells through induction of mitochondrial fusion. Mol. Cell. Biochem. 463, 189–201. doi:10.1007/s11010-019-03640-6
Siegmund, D., Kums, J., Ehrenschwender, M., and Wajant, H. (2016). Activation of TNFR2 sensitizes macrophages for TNFR1-mediated necroptosis. Cell death Dis. 7, e2375. doi:10.1038/cddis.2016.285
Silva, L. C., Borgato, G. B., Wagner, V. P., Martins, M. D., Rocha, G. Z., Lopes, M. A., et al. (2022). Cephaeline is an inductor of histone H3 acetylation and inhibitor of mucoepidermoid carcinoma cancer stem cells. J. oral pathology Med. 51, 553–562. doi:10.1111/jop.13252
Simanullang, R. H., Situmorang, P. C., Herlina, M., Noradina, S. B., and Manurung, S. S. (2022a). Histological changes of cervical tumours following Zanthoxylum acanthopodium DC treatment, and its impact on cytokine expression. Saudi J. Biol. Sci. 29 (4), 2706–2718. doi:10.1016/j.sjbs.2021.12.065
Simanullang, R. H., Situmorang, P. C., Herlina, M. N., and Silalahi, B. (2022c). Cytochrome c expression by andaliman (Zanthoxylum acanthopodium) on cervical cancer histology. Pak J. Biol. Sci. 25 (1), 49–55. doi:10.3923/pjbs.2022.49.55
Simanullang, R. H., Situmorang, P. C., Siahaan, J. M., Widjaja, S. S., and Mutiara, M. (2022b). Effects of Zanthoxylum acanthopodium on MMP-9 and GLUT-1 expression and histology changes in rats with cervical carcinoma. Pharmacia 69 (4), 911–920. doi:10.3897/pharmacia.69.e89368
Singh, A. K., and Vinayak, M. (2017). Resveratrol alleviates inflammatory hyperalgesia by modulation of reactive oxygen species (ROS), antioxidant enzymes and ERK activation. Inflamm. Res. 66, 911–921. doi:10.1007/s00011-017-1072-0
Situmorang, P. C., and Ilyas, S. (2018). Review: germinal cell apoptosis by herbal medicine. Asian J Pharm. Clin. Res. 11 (9), 24–31. doi:10.22159/ajpcr.2018.v11i9.26400
Situmorang, P. C., Ilyas, S., Hutahaean, S., and Rosidah, R. (2021a). Components and acute toxicity of nano herbal haramonting (Rhodomyrtus tomentosa). J. Herbmed Pharmacol. 10, 139–148. doi:10.34172/jhp.2021.15
Situmorang, P. C., Ilyas, S., Hutahaean, S., and Rosidah, R. (2021b). Effect of nano herbal andaliman (Zanthoxylum acanthopodium) fruits in NOTCH1 and Hes1 expressions to human placental trophoblasts. Pak J. Biol. Sci. 24 (1), 165–171. doi:10.3923/pjbs.2021.165.171
Situmorang, P. C., Ilyas, S., Hutahaean, S., and Rosidah, R. (2021c). Histological changes in placental rat apoptosis via FasL and cytochrome c by the nano-herbal Zanthoxylum acanthopodium. Saudi J. Bio Sci. 28 (5), 3060–3068. doi:10.1016/j.sjbs.2021.02.047
Situmorang, P. C., Ilyas, S., Siahaan, D. A. S., Restuati, M., Sari, E. R., Chairunisa, C., et al. (2022b). Effect of Rhodomyrtus tomentosa Hassk. on HIF1α and VEGF expressions on hypertension placental. J. Pharm. Pharmacogn. Res. 10 (6), 1076–1086. doi:10.56499/jppres22.1517_10.6.1076
Situmorang, P. C., Ilyas, S., Syahputra, R. A., Nugraha, A. P., Putri, M. S. S., and Rumahorbo, C. G. P. (2024a). Rhodomyrtus tomentosa (Aiton) Hassk. (haramonting) protects against allethrin-exposed pulmo damage in rats:mechanistic interleukins. Front. Pharmacol. 15, 1343936. doi:10.3389/fphar.2024.1343936
Situmorang, P. C., Ilyas, S., Syahputra, R. A., Sari, R. M., Nugraha, A. P., and Ibrahim, A. (2024b). Rhodomyrtus tomentosa as a new anticancer molecular strategy in breast histology via Her2, IL33, EGFR, and MUC1. Front. Pharmacol. 15, 1345645. doi:10.3389/fphar.2024.1345645
Situmorang, P. C., Simanullang, R. H., Syahputra, R. A., Hutahaean, M. M., Sembiring, H., Nisfa, L., et al. (2023). Histological analysis of TGFβ1 and VEGFR expression in cervical carcinoma treated with Rhodomyrtus tomentosa. Pharmacia 70 (1), 217–223. doi:10.3897/pharmacia.70.e96811
Situmorang, P. C., Syahputra R, A., and Simanullang, R. H. (2022a). EGFL7 and HIF-1a expression on human trophoblast placental by Rhodomyrtus tomentosa and Zanthoxylum acanthopodium. Pak J. Biol. Sci. 25 (2), 123–130. doi:10.3923/pjbs.2022.123.130
Škubník, J., Pavlíčková, V. S., Ruml, T., and Rimpelová, S. (2021). Vincristine in combination therapy of cancer: emerging trends in clinics. Biology 10, 849. doi:10.3390/biology10090849
Sohel, M., Sultana, H., Sultana, T., Al Amin, M., Aktar, S., Ali, M. C., et al. (2022). Chemotherapeutic potential of hesperetin for cancer treatment, with mechanistic insights: a comprehensive review. Heliyon 8, e08815. doi:10.1016/j.heliyon.2022.e08815
Son, H.-K., and Kim, D. (2023). Quercetin induces cell cycle arrest and apoptosis in YD10B and YD38 oral squamous cell carcinoma cells. Asian Pac. J. cancer Prev. APJCP 24, 283–289. doi:10.31557/APJCP.2023.24.1.283
Srinivasan, S., Koduru, S., Kumar, R., Venguswamy, G., Kyprianou, N., and Damodaran, C. (2009). Diosgenin targets Akt-mediated prosurvival signaling in human breast cancer cells. Int. J. cancer 125, 961–967. doi:10.1002/ijc.24419
Subramaniam, A., Loo, S. Y., Rajendran, P., Manu, K. A., Perumal, E., Li, F., et al. (2013). An anthraquinone derivative, emodin sensitizes hepatocellular carcinoma cells to TRAIL induced apoptosis through the induction of death receptors and downregulation of cell survival proteins. Apoptosis Int. J. Program. Cell death 18, 1175–1187. doi:10.1007/s10495-013-0851-5
Sun, L., Yuan, W., Wen, G., Yu, B., Xu, F., Gan, X., et al. (2020). Parthenolide inhibits human lung cancer cell growth by modulating the IGF-1R/PI3K/Akt signaling pathway. Oncol. Rep. 44, 1184–1193. doi:10.3892/or.2020.7649
Sun, M.-Y., and Bhaskar, S. M. M. (2022). When two maladies meet: disease burden and pathophysiology of stroke in cancer. Int. J. Mol. Sci. 23, 15769. doi:10.3390/ijms232415769
Suo, F., Zhou, X., Setroikromo, R., and Quax, W. J. (2022). Receptor specificity engineering of TNF superfamily ligands. Pharmaceutics 14, 181. doi:10.3390/pharmaceutics14010181
Takáč, P., Michalková, R., Čižmáriková, M., Bedlovičová, Z., Balážová, Ľ., and Takáčová, G. (2023). The role of silver nanoparticles in the diagnosis and treatment of cancer: are there any perspectives for the future? Life (Basel) 13, 466. doi:10.3390/life13020466
Talib, W. H., Alsalahat, I., Daoud, S., Abutayeh, R. F., and Mahmod, A. I. (2020). Plant-derived natural products in cancer research: extraction, mechanism of action, and drug formulation. Molecules 25, 5319. doi:10.3390/molecules25225319
Tayarani-Najaran, Z., Tayarani-Najaran, N., and Eghbali, S. (2021). A review of auraptene as an anticancer agent. Front. Pharmacol. 12, 698352. doi:10.3389/fphar.2021.698352
Tayeh, M., and Watanapokasin, R. (2020). Antimetastatic potential of rhodomyrtone on human chondrosarcoma SW1353 cells. Evidence-based complementary Altern. Med. eCAM 2020, 8180261. doi:10.1155/2020/8180261
Tian, Z., Sun, C., and Liu, J. (2022). Pelargonidin inhibits vascularization and metastasis of brain gliomas by blocking the PI3K/AKT/mTOR pathway. J. Biosci. 47, 64. doi:10.1007/s12038-022-00281-8
Tran, S., Fairlie, W. D., and Lee, E. F. (2021). BECLIN1: protein structure, function and regulation. Cells 10, 1522. doi:10.3390/cells10061522
Tsai, C.-H., Yang, C.-W., Wang, J.-Y., Tsai, Y.-F., Tseng, L.-M., King, K.-L., et al. (2013). Timosaponin AIII suppresses hepatocyte growth factor-induced invasive activity through sustained ERK activation in breast cancer MDA-MB-231 cells. Evidence-based complementary Altern. Med. eCAM 2013, 421051. doi:10.1155/2013/421051
Tyagi, A., Sharma, S., Wu, K., Wu, S.-Y., Xing, F., Liu, Y., et al. (2021). Nicotine promotes breast cancer metastasis by stimulating N2 neutrophils and generating pre-metastatic niche in lung. Nat. Commun. 12, 474. doi:10.1038/s41467-020-20733-9
Verma, A. K., Bharti, P. S., Rafat, S., Bhatt, D., Goyal, Y., Pandey, K. K., et al. (2021). Autophagy paradox of cancer: role, regulation, and duality. Oxidative Med. Cell. Longev. 2021, 8832541. doi:10.1155/2021/8832541
Villareal, M. O., Chaochaiphat, T., Bejaoui, M., Sato, K., Isoda, H., City, T., et al. (2020). Tara tannin regulates pigmentation by modulating melanogenesis enzymes and melanosome transport proteins expression. Planta Medica Int. Open 7 (01), e34–e44. doi:10.1055/a-1141-0151
Vodanovich, D. A., and M Choong, P. F. (2018). Soft-tissue sarcomas. Indian J. Orthop. 52, 35–44. doi:10.4103/ortho.IJOrtho_220_17
Wahi, A., Bishnoi, M., Raina, N., Singh, M. A., Verma, P., Gupta, P. K., et al. (2023). Recent updates on nano-phyto-formulations based therapeutic intervention for cancer treatment. Oncol. Res. 32, 19–47. doi:10.32604/or.2023.042228
Wang, B.-F., Wang, X.-J., Kang, H.-F., Bai, M.-H., Guan, H.-T., Wang, Z.-W., et al. (2014a). Saikosaponin-D enhances radiosensitivity of hepatoma cells under hypoxic conditions by inhibiting hypoxia-inducible factor-1α. Cell. physiology Biochem. 33, 37–51. doi:10.1159/000356648
Wang, F., Chang, Z., Fan, Q., and Wang, L. (2014b). Epigallocatechin-3-gallate inhibits the proliferation and migration of human ovarian carcinoma cells by modulating p38 kinase and matrix metalloproteinase-2. Mol. Med. Rep. 9, 1085–1089. doi:10.3892/mmr.2014.1909
Wang, J., Li, J., Cao, N., Li, Z., Han, J., and Li, L. (2018). Resveratrol, an activator of SIRT1, induces protective autophagy in non-small-cell lung cancer via inhibiting Akt/mTOR and activating p38-MAPK. OncoTargets Ther. 11, 7777–7786. doi:10.2147/OTT.S159095
Wang, M., Yu, F., Zhang, Y., Chang, W., and Zhou, M. (2022). The effects and mechanisms of flavonoids on cancer prevention and therapy: focus on gut microbiota. Int. J. Biol. Sci. 18, 1451–1475. doi:10.7150/ijbs.68170
Wei, L., Jin, X., Cao, Z., and Li, W. (2016). Evodiamine induces extrinsic and intrinsic apoptosis of ovarian cancer cells via the mitogen-activated protein kinase/phosphatidylinositol-3-kinase/protein kinase B signaling pathways. J. traditional Chin. Med. 36, 353–359. doi:10.1016/s0254-6272(16)30049-8
Wei, M., Li, J., Qiu, J., Yan, Y., Wang, H., Wu, Z., et al. (2020). Costunolide induces apoptosis and inhibits migration and invasion in H1299 lung cancer cells. Oncol. Rep. 43, 1986–1994. doi:10.3892/or.2020.7566
Weledji, E. P., and Orock, G. E. (2015). Surgery for non-hodgkin’s lymphoma. Oncol. Rev. 9, 274. doi:10.4081/oncol.2015.274
Wolfrum, P., Fietz, A., Schnichels, S., and Hurst, J. (2022). The function of p53 and its role in Alzheimer’s and Parkinson’s disease compared to age-related macular degeneration. Front. Neurosci. 16, 1029473. doi:10.3389/fnins.2022.1029473
Woo, S.-M., Choi, Y. K., Kim, A. J., Cho, S.-G., and Ko, S.-G. (2016). p53 causes butein-mediated apoptosis of chronic myeloid leukemia cells. Mol. Med. Rep. 13, 1091–1096. doi:10.3892/mmr.2015.4672
Wróblewska-Łuczka, P., Cabaj, J., Bargieł, J., and Łuszczki, J. J. (2023). Anticancer effect of terpenes: focus on malignant melanoma. Pharmacol. Rep. P. R. 75, 1115–1125. doi:10.1007/s43440-023-00512-1
Wu, D., Liu, Z., Li, J., Zhang, Q., Zhong, P., Teng, T., et al. (2019). Epigallocatechin-3-gallate inhibits the growth and increases the apoptosis of human thyroid carcinoma cells through suppression of EGFR/RAS/RAF/MEK/ERK signaling pathway. Cancer Cell Int. 19, 43. doi:10.1186/s12935-019-0762-9
Wu, H., Du, J., Li, C., Li, H., Guo, H., and Li, Z. (2022a). Kaempferol can reverse the 5-fu resistance of colorectal cancer cells by inhibiting PKM2-mediated glycolysis. Int. J. Mol. Sci. 23, 3544. doi:10.3390/ijms23073544
Wu, M.-F., Huang, Y.-H., Chiu, L.-Y., Cherng, S.-H., Sheu, G.-T., and Yang, T.-Y. (2022b). Curcumin induces apoptosis of chemoresistant lung cancer cells via ROS-regulated p38 MAPK phosphorylation. Int. J. Mol. Sci. 23, 8248. doi:10.3390/ijms23158248
Xi, H., Wang, S., Wang, B., Hong, X., Liu, X., Li, M., et al. (2022). The role of interaction between autophagy and apoptosis in tumorigenesis (Review). Oncol. Rep. 48, 208. doi:10.3892/or.2022.8423
Xiao, J., Gao, M., Fei, B., Huang, G., and Diao, Q. (2020). Nature-derived anticancer steroids outside cardica glycosides. Fitoterapia 147, 104757. doi:10.1016/j.fitote.2020.104757
Xie, X., Zhang, L., Zhang, W., Tayebee, R., Hoseininasr, A., Vatanpour, H., et al. (2020). Fabrication of temperature and pH sensitive decorated magnetic nanoparticles as effective biosensors for targeted delivery of acyclovir anti-cancer drug. J. Mol. Liq. 309, 113024. doi:10.1016/j.molliq.2020.113024
Yamada, M., Iijima, Y., Seo, M., Hino, S., Sano, M., Sakagami, H., et al. (2023). Cancer chemotherapy-associated pigmentation of the oral mucosa. vivo (Athens, Greece) 37, 1880–1885. doi:10.21873/invivo.13280
Yang, J., Yang, Y., Tian, L., Sheng, X.-F., Liu, F., and Cao, J.-G. (2011). Casticin-induced apoptosis involves death receptor 5 upregulation in hepatocellular carcinoma cells. World J. gastroenterology 17, 4298–4307. doi:10.3748/wjg.v17.i38.4298
Yang, L., Zhang, W., Chopra, S., Kaur, D., Wang, H., Li, M., et al. (2020). The epigenetic modification of epigallocatechin gallate (EGCG) on cancer. Curr. drug targets 21, 1099–1104. doi:10.2174/1389450121666200504080112
Yao, H., Chen, Y., Zhang, L., He, X., He, X., Lian, L., et al. (2014). Carnosol inhibits cell adhesion molecules and chemokine expression by tumor necrosis factor-α in human umbilical vein endothelial cells through the nuclear factor-κB and mitogen-activated protein kinase pathways. Mol. Med. Rep. 9, 476–480. doi:10.3892/mmr.2013.1839
You, Y., Wang, R., Shao, N., Zhi, F., and Yang, Y. (2019). Luteolin suppresses tumor proliferation through inducing apoptosis and autophagy via MAPK activation in glioma. OncoTargets Ther. 12, 2383–2396. doi:10.2147/OTT.S191158
Yuan, B., Yang, R., Ma, Y., Zhou, S., Zhang, X., and Liu, Y. (2017). A systematic review of the active saikosaponins and extracts isolated from Radix Bupleuri and their applications. Pharm. Biol. 55, 620–635. doi:10.1080/13880209.2016.1262433
Yuan, M., Zhang, G., Bai, W., Han, X., Li, C., and Bian, S. (2022). The role of bioactive compounds in natural products extracted from plants in cancer treatment and their mechanisms related to anticancer effects. Oxidative Med. Cell. Longev. 2022, 1429869. doi:10.1155/2022/1429869
Yusuf, A., Almotairy, A. R. Z., Henidi, H., Alshehri, O. Y., and Aldughaim, M. S. (2023). Nanoparticles as drug delivery systems: a review of the implication of nanoparticles’ physicochemical properties on responses in biological systems. Polymers 15, 1596. doi:10.3390/polym15071596
Zaafar, D., Khalil, H. M. A., Rasheed, R. A., Eltelbany, R. F. A., and Zaitone, S. A. (2022). Hesperetin mitigates sorafenib-induced cardiotoxicity in mice through inhibition of the TLR4/NLRP3 signaling pathway. PloS one 17, e0271631. doi:10.1371/journal.pone.0271631
Zeng, Q., Che, Y., Zhang, Y., Chen, M., Guo, Q., and Zhang, W. (2020). Thymol isolated from thymus vulgaris L. Inhibits colorectal cancer cell growth and metastasis by suppressing the wnt/β-catenin pathway. Drug Des. Dev. Ther. 14, 2535–2547. doi:10.2147/DDDT.S254218
Zhang, G., Li, Z., Dong, J., Zhou, W., Zhang, Z., Que, Z., et al. (2022). Acacetin inhibits invasion, migration and TGF-β1-induced EMT of gastric cancer cells through the PI3K/Akt/Snail pathway. BMC complementary Med. Ther. 22, 10. doi:10.1186/s12906-021-03494-w
Zhang, L., Yang, C., Huang, Y., Huang, H., Yuan, X., Zhang, P., et al. (2021a). Cardamonin inhibits the growth of human osteosarcoma cells through activating P38 and JNK signaling pathway. Biomed. Pharmacother. = Biomedecine Pharmacother. 134, 111155. doi:10.1016/j.biopha.2020.111155
Zhang, R., Hao, J., Wu, Q., Guo, K., Wang, C., Zhang, W. K., et al. (2020). Dehydrocostus lactone inhibits cell proliferation and induces apoptosis by PI3K/Akt/Bad and ERS signalling pathway in human laryngeal carcinoma. J. Cell. Mol. Med. 24, 6028–6042. doi:10.1111/jcmm.15131
Zhang, Y., Yuan, B., Bian, B., Zhao, H., Kiyomi, A., Hayashi, H., et al. (2021b). Cytotoxic effects of hellebrigenin and arenobufagin against human breast cancer cells. Front. Oncol. 11, 711220. doi:10.3389/fonc.2021.711220
Zhao, X., Liu, Z., Ren, Z., Wang, H., Wang, Z., Zhai, J., et al. (2020). Triptolide inhibits pancreatic cancer cell proliferation and migration via down-regulating PLAU based on network pharmacology of Tripterygium wilfordii Hook F. Eur. J. Pharmacol. 880, 173225. doi:10.1016/j.ejphar.2020.173225
Zhao, X., Tao, X., Xu, L., Yin, L., Qi, Y., Xu, Y., et al. (2016). Dioscin induces apoptosis in human cervical carcinoma HeLa and SiHa cells through ROS-mediated DNA damage and the mitochondrial signaling pathway. Molecules 21, 730. doi:10.3390/molecules21060730
Zhao, Y., Pan, H., Liu, W., Liu, E., Pang, Y., Gao, H., et al. (2023). Menthol: an underestimated anticancer agent. Front. Pharmacol. 14, 1148790. doi:10.3389/fphar.2023.1148790
Zhao, Z., Jiang, Y., Liu, Z., Li, Q., Gao, T., and Zhang, S. (2021). Ampelopsin inhibits cell viability and metastasis in renal cell carcinoma by negatively regulating the PI3K/AKT signaling pathway. Evidence-based complementary Altern. Med. 2021, 4650566. doi:10.1155/2021/4650566
Zhou, B.-G., Wei, C.-S., Zhang, S., Zhang, Z., and Gao, H.-M. (2018). Matrine reversed multidrug resistance of breast cancer MCF-7/ADR cells through PI3K/AKT signaling pathway. J. Cell. Biochem. 119, 3885–3891. doi:10.1002/jcb.26502
Zhou, Q., Wang, S., Zhang, H., Lu, Y., Wang, X., Motoo, Y., et al. (2009). The combination of baicalin and baicalein enhances apoptosis via the ERK/p38 MAPK pathway in human breast cancer cells. Acta Pharmacol. Sin. 30, 1648–1658. doi:10.1038/aps.2009.166
Zhou, W., Cao, W., Wang, M., Yang, K., Zhang, X., Liu, Y., et al. (2023). Validation of quercetin in the treatment of colon cancer with diabetes via network pharmacology, molecular dynamics simulations, and in vitro experiments. Mol. Divers. doi:10.1007/s11030-023-10725-4
Zhu, M.-L., Zhang, P.-M., Jiang, M., Yu, S.-W., and Wang, L. (2020). Myricetin induces apoptosis and autophagy by inhibiting PI3K/Akt/mTOR signalling in human colon cancer cells. BMC complementary Med. Ther. 20, 209. doi:10.1186/s12906-020-02965-w
Zubor, P., Dankova, Z., Kolkova, Z., Holubekova, V., Brany, D., Mersakova, S., et al. (2020). Rho GTPases in gynecologic cancers: in-depth analysis toward the paradigm change from reactive to predictive, preventive, and personalized medical approach benefiting the patient and healthcare. Cancers 12, 1292. doi:10.3390/cancers12051292
Keywords: apoptosis, cancer, herbal, molecular pathway, phytochemicals, plants
Citation: Situmorang PC, Ilyas S, Nugraha SE, Syahputra RA and Nik Abd Rahman NMA (2024) Prospects of compounds of herbal plants as anticancer agents: a comprehensive review from molecular pathways. Front. Pharmacol. 15:1387866. doi: 10.3389/fphar.2024.1387866
Received: 18 February 2024; Accepted: 17 June 2024;
Published: 22 July 2024.
Edited by:
Chunbo He, University of Oklahoma Health Sciences Center, United StatesReviewed by:
Seyed Zachariah Moradi, Kermanshah University of Medical Sciences, IranVenkataswarup Tiriveedhi, Tennessee State University, United States
Copyright © 2024 Situmorang, Ilyas, Nugraha, Syahputra and Nik Abd Rahman. This is an open-access article distributed under the terms of the Creative Commons Attribution License (CC BY). The use, distribution or reproduction in other forums is permitted, provided the original author(s) and the copyright owner(s) are credited and that the original publication in this journal is cited, in accordance with accepted academic practice. No use, distribution or reproduction is permitted which does not comply with these terms.
*Correspondence: Putri Cahaya Situmorang, cHV0cmkuY2FoYXlhQHVzdS5hYy5pZA==