- 1Institute of Basic Research in Clinical Medicine, China Academy of Chinese Medical Sciences, Beijing, China
- 2Law Sau Fai Institute for Advancing Translational Medicine in Bone and Joint Diseases, School of Chinese Medicine, Hong Kong Baptist University, Kowloon, Hong Kong SAR, China
- 3Institute of Systems Medicine and Health Sciences, School of Chinese Medicine, Hong Kong Baptist University, Kowloon, Hong Kong SAR, China
- 4Shanghai GuangHua Hospital of Integrated Traditional Chinese and Western Medicine, Institute of Arthritis Research, Shanghai Academy of Chinese Medical Sciences, Shanghai, China
Hepatocellular carcinoma (HCC) has a relatively poor prognosis and a high degree of malignancy. However, the therapeutic drugs are limited. In recent years, abnormal lipid metabolism and its important role in HCC has been reported, and emerging studies found that some formulae and active components of traditional Chinese medicine (TCM) can regulate abnormal lipid metabolism in HCC, showing their good application prospects. Therefore, this article summarizes the changes and the roles of lipid metabolites in HCC progression, and discusses the role of formulae and active components of TCM for the treatment of HCC based on their regulation on abnormal lipid metabolism. A deeper understanding of their relationship may help the precise use of these formulae and active components in HCC.
1 Introduction
Hepatocellular carcinoma (HCC) is a common cancer affecting individuals globally (Vogel et al., 2022). Half of the world’s HCC cases come from China, and HCC ranks as the second most common cause of death in China (Xie et al., 2020). HCC is usually developed through a gradual process: chronic hepatitis, liver fibrosis, liver cirrhosis, and finally HCC (Kanda et al., 2019). Over the past decade, although many breakthroughs in the treatment of HCC have been made, there is still facing difficulties. The high recurrence rate after surgery and interventional therapy, coupled with the difficulty of chemotherapy and targeted medicines to achieve the desired efficacy, makes the overall survival rate of HCC patients not optimistic, and only about 10% of patients can survive the 5-year survival period (Wang and Lu, 2024). Therefore, there is an urgent need to find new strategies for HCC treatments. The metabolic changes in patients with HCC have garnered significant interest in recent years. Abnormal lipid metabolism has shown strong associations with the incidence and development of HCC in previous studies (Sangineto et al., 2020). For example, scientists have found that HCC is typically characterized by upregulation of genes associated with fatty acids (FAs) synthesis (Budhu et al., 2013). In another study, enhanced lipid synthesis as well as the upregulation of some lipid metabolism-related genes was observed in β-catenin-activated HCCs (Berndt et al., 2019a). Consequently, abnormal lipid metabolism has become a new target for treating HCC.
Normal lipid metabolism involves the uptake, synthesis, and decomposition of FAs, phospholipids, and cholesterol. Synthesized and stored lipids function as energy sources for cells, secondary messengers that transmit information, and involved in biofilm synthesis, thereby maintaining normal cell survival. Abnormal lipid metabolism, which manifests as abnormal FA synthesis and lipid accumulation, is observed in patients with HCC (Bort et al., 2020; Xu et al., 2023). It provides cancer cells with a large amount of energy for proliferation, survival, invasion, and metastasis while facilitating FA-induced reprogramming of lipid metabolism (Hu et al., 2020; Peng et al., 2022; Seo et al., 2020). Moreover, it aids in biofilm synthesis and signaling molecules for cancer treatment response and the tumor microenvironment (Bian et al., 2021). Lipids play an important role in signal transduction and molecular recognition, acting as first (extracellular) and second (intracellular) messengers. Specifically, membrane glycerides and sphingolipids are able to hydrolyze to transduce signals to produce biologically active molecules such as ceramides and sphingosine-1-phosphate (S1P). Steroids (including oxysterols, bile acids (BAs), steroid hormones) and FAs are involved in these processes through direct interactions with receptors (Paul et al., 2022). Among them, C24 ceramide is associated with the aggregation of cancer. The proportion of C24 ceramide in tumor-derived exosomes indicates the tumor dry-like phenotype of glioblastoma. Besides, the loss of ceramide and S1P signaling leads to a decrease in the amount of exosome release (Jia et al., 2024). Thus, regulating lipid metabolism is believed to affect the development of HCC. Several studies have observed that drugs targeting key or rate-limiting metabolic enzymes, metabolites, and drivers of metabolic changes are effective in treating HCC. For instance, the likelihood of HCC in individuals with severe liver disease may be diminished through the use of 3-hydroxy-3-methyl-glutaryl-coenzyme A (HMG-CoA) reductase inhibitors, commonly referred to as statins (Hsiang et al., 2015). The inclusion of statins, as has been noted in numerous clinical trials, extends survival for patients suffering from advanced HCC (Kawata et al., 2001; Shao et al., 2015). Fenofibrate, commonly used to treat hypertriglyceridemia and dyslipidemia, is a fibrillic acid derivative. It plays a role in cancer treatment by regulating related lipid metabolism pathways, such as the PPARα/RXR pathway (La Fountaine et al., 2020; Lian et al., 2018). However, efficacy and safety of these drugs in HCC treatment still require further clinical validation.
Formulae and active components of traditional Chinese medicine (TCM) have been used to treat various cancers and metabolic related diseases with good efficacy and safety (Wei et al., 2022). It could regulate metabolic processes by influencing glycolysis, mitochondrial oxidative phosphorylation, glutaminolysis, FA biosynthesis and so on (Wang et al., 2021). Recently, emerging researches revealed that some TCM formulae and active components exert therapeutic effects in patients with HCC by regulating lipid metabolism and the products of lipid metabolism or affecting the pathways of lipid metabolism. However, the unclear action characteristics and therapeutic mechanisms limit the precise application of TCM formulations in clinic. Therefore, we searched the English articles from PubMed in the last 5 years for the keywords: traditional Chinese medicine, hepatocellular carcinoma, and lipid metabolism, hepatocellular carcinoma and linoleic acid, hepatocellular carcinoma and cholesterol, hepatocellular carcinoma and arachidonic acid, hepatocellular carcinoma and triglycerides, hepatocellular carcinoma and lipoprotein, traditional Chinese medicine and linoleic acid, traditional Chinese medicine and cholesterol, traditional Chinese medicine and arachidonic acid, traditional Chinese medicine and triglycerides, traditional Chinese medicine and lipoprotein. Then, we collected and summarized them, so as to describe the changes and the roles of lipid metabolites in HCC progression (Figure 1), and discuss the effect of TCM formulae and active components in the treatment of HCC based on their regulation on abnormal lipid metabolism (Figure 2).
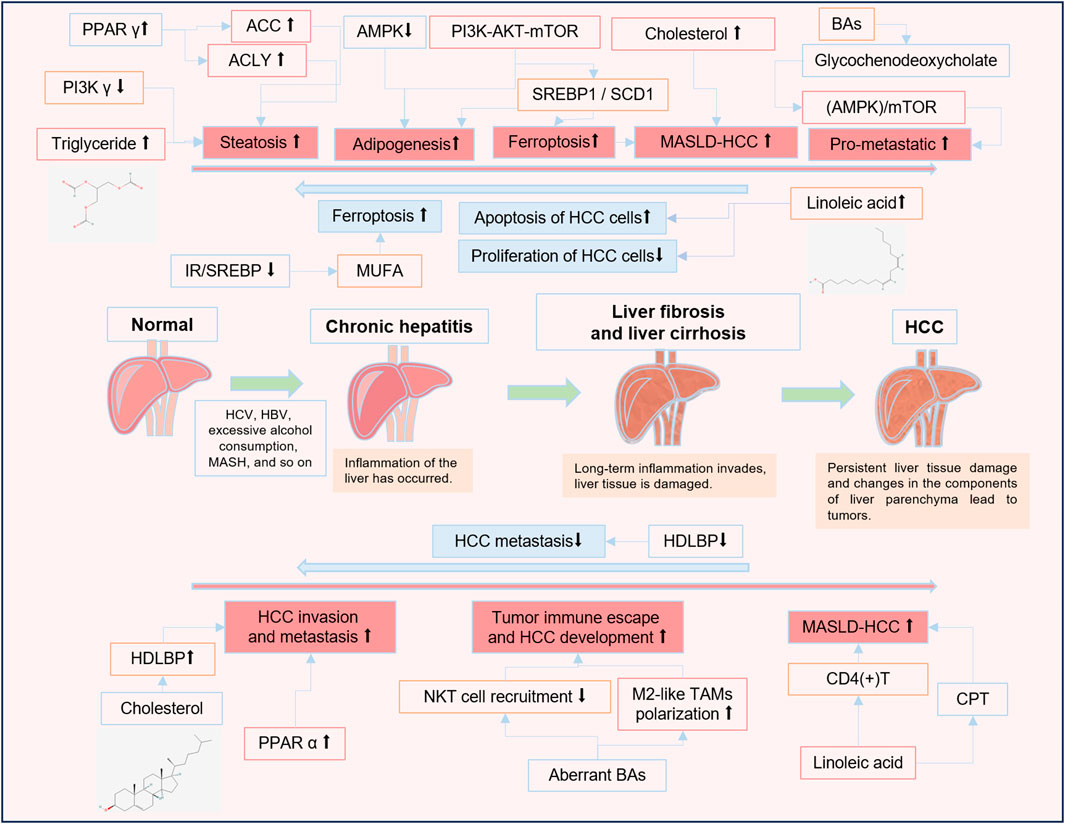
Figure 1. The roles of lipid metabolites and lipid metabolism-related signaling pathways in HCC progression. ↑ An increase in the level or a positive effect. ↓ A decrease in the level or an inhibitory effect.
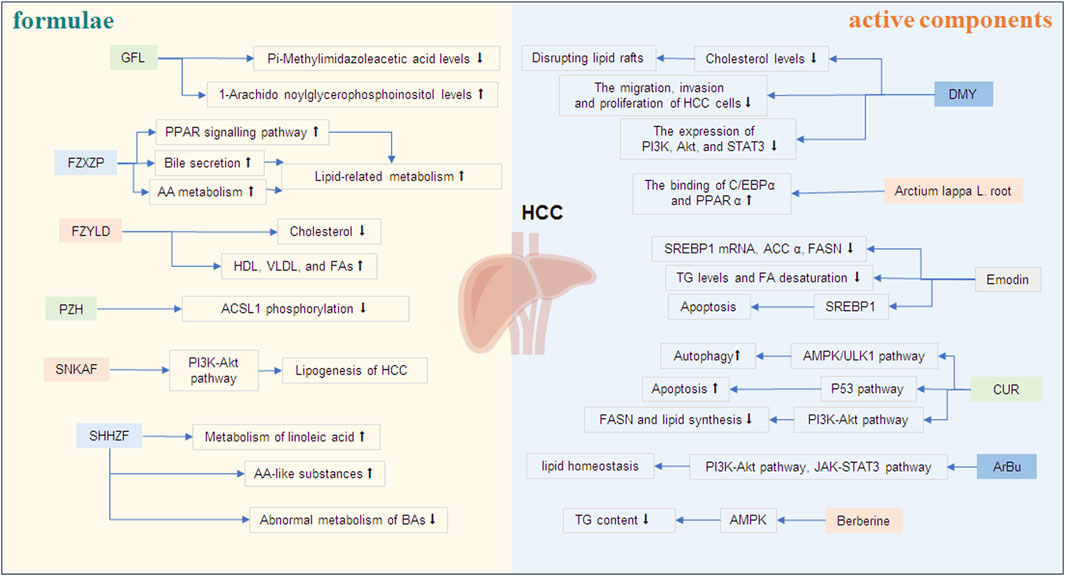
Figure 2. The effects of TCM formulae and active components on lipid metabolism of HCC. ↑ An increase in the level or a positive effect. ↓ A decrease in the level or an inhibitory effect. Note: GFL, Ganfule capsules; FZYLD, Fuzheng Yiliu Decoction; FZXZP, Fuzheng Xiaozheng prescription; PZH, Pien Tze Huang; SNKAF, Sinikangai fang; SHHZF, Shuihonghuazi Formula; DMY, Dihydromyricetin; CUR, Curcumin; ArBu, Arenobufagin.
2 Abnormal lipid metabolism in HCC
2.1 Abnormal FAs levels in HCC
Normally, FAs can be categorized into two primary groups based on their molecular structure: unsaturated fatty acids (UFAs) and saturated fatty acids (SFAs). Within the UFAs category, there are further distinctions made between polyunsaturated fatty acids (PUFAs) and monounsaturated fatty acids (MUFAs). Among PUFAs, specific types include linoleic acid, linolenic acid, and arachidonic acid (AA), et al. It has been observed that abnormal levels of linoleic acid are prevalent in individuals diagnosed with HCC (Cai et al., 2020). Researchers have discovered that the concentration of linoleic acid in the portal vein is reduced in patients with HCC compared to healthy individuals (Liu J. et al., 2022). Vlock EM and colleagues used a mouse model of lean MASH-HCC to determine the changes of linoleic acid levels in HCC. The findings indicated these mice model had a decrease in linoleic acid levels; however, in mice fed choline-deficient, high trans-fat, sucrose, and cholesterol diets, levels increased over time (Vlock et al., 2020). In contrast, AA, another metabolite belonging to PUFA, was elevated in HCC. One experiment used the metabolomics to distinguish HCC patients from those without cancer, researchers discovered that the concentration of AA was notably elevated in HCC cohort relative to the control group (Jee et al., 2018). Additionally, some other FAs were also discovered to be abnormal in HCC. Researchers examined the association between free FAs and advanced liver fibrosis or HCC in hispanics in South Texas who have high rates of HCC. They found that HCC has showed a significant association with low expression of some types of FAs, such as very long chain (VLC) SFAs, odd chain SFAs, and VLC n-3 PUFAs (Jiao et al., 2021).
2.2 Abnormal cholesterol levels in HCC
Cholesterol is an essential lipid for maintaining cellular homeostasis synthesized mainly in the liver (Luo et al., 2020). A study aimed to compare the metabolic signature between MASH and MASH-HCC patients. The results showed that compared with the MASH group, BAs metabolism and cholesterol metabolism were significantly upregulated in MASH-HCC group (Ahmed et al., 2022). Plasma cholesterol in 132 HCC patients and 287 patients without HCC cirrhosis was studied by Venturini et al. and the result suggested that the enhanced cholesterol biosynthesis led to a rise in plasma cholesterol of patients with cancer (Venturini et al., 1999). Besides, researchers assessed the extent of FA synthesis in the liver, as well as levels of triglycerides and cholesterol to investigate the role of unconstrained lipogenesis in human HCC. The findings revealed that the synthesis of FAs, as well as triglyceride and cholesterol levels, was significantly elevated in HCC compared to the surrounding liver tissue (Calvisi et al., 2011). Additionally, it was discovered that HCC patients with shorter survival times exhibited higher serum cholesterol levels (Li et al., 2023a). However, it has also been reported to the contrary that high cholesterol is associated with a low risk of HCC. In one experiment, underwent health screenings in 2009, a total of 8,528,790 patients were analyzed using Cox regression to investigate the hazard ratios for HCC. The findings indicated that as levels of low-density lipoprotein cholesterol and total cholesterol increased, the incidence of HCC progressively decreased. Compared to individuals in the lowest quartile of total cholesterol, those with higher levels of total cholesterol were associated with a reduced risk of developing HCC (Cho et al., 2021).This result suggests that the relationship between HCC progression and cholesterol levels still needs to be further studied.
Bile acids (BAs) play a crucial role in cholesterol metabolism, with the conversion of cholesterol into BAs being the most important metabolic pathway. At the same time, about 40% of the cholesterol in the body of a normal person is converted into BA every day. An enhanced BA pool has been observed in patients with MASH-associated HCC (Conde de la Rosa et al., 2021). The quantification and analysis of 35 types of BAs in the pre- diagnostic sera of 100 patients with HCC and 100 healthy individuals in China revealed the levels of conjugated primary BAs are markedly elevated (Thomas et al., 2021). An increased risk of HCC is associated with higher BAs concentrations, particularly in conjugated primary BAs (Petrick et al., 2020). One study observed an association between the elevated levels of major circulating BAs and the increased risk of HCC through analyzing 233 cases of HCC. With increasing concentrations of BAs, the BAs profile shifted towards a higher proportion of taurine-conjugated BAs, suggesting the progression of HCC and early metabolic changes in BAs metabolism (Stepien et al., 2022). BA synthesis and transport are regulated by farnesoid X receptor (FXR). It has been reported that in mice lacking the FXR, both hepatic and serum levels of BAs are elevated. The accumulation of BAs in these FXR-deficient mice led to a spontaneous development of HCC in nearly 90% of cases (Kim et al., 2007). However, not all studies found that BAs was elevated. In one study, scientists investigated BAs levels and genes associated with BAs homeostasis in 37 patients with HCC in both tumor-adjacent and cancer tissues, finding a 36% reduction in total BAs (Chen W. et al., 2023). In another study involving 348 patients with chronic liver disease and 396 patients with HCC, the findings revealed that the total BAs levels in HCC patients, both prior to and following propensity score matching analysis, were lower compared to those with persistent liver diseases. Additionally, there was a significant reduction in the total BAs levels observed in HCC patients (Dai et al., 2024).
2.3 Abnormal triglycerides levels in HCC
An increase in the levels of saturated triglycerides (TG) had been reported in HCC. Using untargeted metabolomics and lipidomics to study the biomarkers of HCC in patients with cirrhosis, researchers established that TG levels were significantly raised in blood of HCC cases (Rashid et al., 2023). To better comprehend the molecular pathogenesis of lipid metabolism levels, separate tests of blood from patients with HCC, healthy people, and patients with chronic liver disease was used. The results indicated that several predominantly saturated TGs in the blood showed a sustained increase in HCC trajectories (Ismail et al., 2020). Excessive carbohydrates being converted to TG or an increased delivery of TG to the liver causes excessive accumulation of fat in the liver (Lee J. et al., 2017). Experimental results have shown that lipid deposition within the tumor is a characteristic feature of HCCs, with the accumulation of diglycerides and TG being observed in the tumor tissue of mice (Haberl et al., 2021). A study established mouse models of lean and obese MASH-HCC to compare their progression toward MASH-HCC. The results revealed that TG levels in both groups had elevated (Hymel et al., 2022). In another study, the progression of high-fat diet-induced MASLD-MASH-HCC and elevated cholesterol and TG levels were also detected in the mice (Tessitore et al., 2016). Scientists used protein intensity profiles of 11 human HCCs to parameterize tumor-specific kinetic models of cellular lipid metabolism. They found that compared to the HCC tumor-adjacent tissue, TG content in HCC was significantly higher (Berndt et al., 2019b). Logistic regression analysis showed that the TG levels were an separate risk factor for the incidence of HCC (Ali et al., 2022).
2.4 Abnormal lipoprotein and apolipoprotein levels in HCC
Abnormal lipoprotein metabolism is also closely associated with tumorigenesis. There are some typical human lipoproteins: low-density lipoproteins (LDL), very-low-density lipoproteins (VLDL), high-density lipoproteins (HDL) and chylomicrons (CM). Increased secretion of lipoprotein lipase from the tumor cells into the peripheral blood has been observed in patients with HCC. A significant decrease in the serum VLDL levels, and a significant increase in the serum LDL levels have been observed in patients with HCC (Zuo et al., 2021). Apolipoprotein A1 (ApoA-1) is an indispensable protein in the synthesis of HDL. Scientists have found that ApoA-1 levels correlated with the degree of malignancy in HCC. Patients with HCC and tumor recurrence exhibited significantly reduced serum ApoA-1 levels. Additionally, higher serum ApoA-1 levels were observed in patients with reduced levels of circulating tumor cells (Ma X. L. et al., 2016). Analysis of prospective data collected by the Türkiye HCC multi-agency cooperative working group revealed that low HDL and high LDL levels showed significant correlations with an increase in maximum tumor diameter. Elevated LDL levels and reduced HDL levels in plasma are significantly associated with a more aggressive HCC phenotype, especially when these conditions occur together. This combination is also linked to an increased risk of death (Akkiz et al., 2021). Lipoprotein (a) [Lp (a)] is a molecule bound to apolipoprotein (a) (Tasdighi et al., 2024). Scientists assessed the relationship between HCC and Lp(a) levels in serum by Kaplan-Meier curves and log-rank tests. Comparing data from healthy individuals and HCC patients, Lp(a) levels in HCC patients were found to be significantly lower than in healthy individuals. In addition, there is an association between HCC recurrence and Lp(a) levels, HCC patients with low Lp(a) levels have a higher recurrence rate and shorter survival than those with high Lp(a) levels (Gao et al., 2018).
3 Abnormal lipid metabolism affects HCC progression
3.1 Affecting cell proliferation and apoptosis
The recognition of abnormal cholesterol metabolism in HCC has led to an increase in the focus on the effects of it in HCC. In an experiment in which researchers fed mice with high fat/cholesterol, it developed HCC and decreased tryptophan metabolism associated with the gut microbiota, correlation analysis showed that dysbiosis of gut microbiota in MASLD-HCC was associated with cholesterol levels. Germ-free mice gavaged with stools from mice fed high fat/cholesterol manifested hepatic lipid accumulation, inflammation and cell proliferation. Scientists have shown that dietary cholesterol-regulated microbiota promotes MASLD and hepatocyte proliferation by inducing metabolite alterations, thereby promoting cholesterol-induced MASLD-HCC formation (Zhang et al., 2021). Not only cholesterol, but also some FAs can influence the cell proliferation and apoptosis in HCC. Scientists have studied the functional importance of linoleic acid in HCC cell lines. They found that linoleic acid could significantly inhibit the proliferation and induce the apoptosis of HCC cells (Liu J. et al., 2022). Ferroptosis, characterized by lipid peroxidation and iron accumulation that is strongly associated with HCC progression, is an emerging iron-dependent programmed cell death modality (Yang et al., 2024). Accumulating evidence suggests that activation of ferroptosis may prevent HCC cell proliferation (Nie et al., 2018). Lipidomic analysis has shown that promoting the selective uptake of MUFAs could induce an increase in phosphatidylcholine and phosphatidylethanolamine levels in HCC cells, which induces resistance to lipid peroxidation and ferroptosis (Li et al., 2023b). Meanwhile, in another experiment, scientists found that downregulation of IR/SREBP axis-mediated MUFA synthesis could promote ferroptosis in HCC, thus inhibiting HCC (Yuan et al., 2024).
3.2 Affecting tumor invasion and metastasis
The lipid transporter high-density lipoprotein-binding protein (HDLBP) is clinically relevant to tumor metastasis in patients with HCC. Cholesterol-induced HCC metastasis needs HDLBP. Scientists suggested that cholesterol-induced HDLBP promoted HCC metastasis and invasion through BRAF-dependent epithelial-mesenchymal transition (EMT) signaling. Further studies have shown that knockdown or overexpression of HDLBP significantly inhibited or enhanced the metastasis, invasion and EMT of HCC cells, respectively (Yuan et al., 2022). Known as an indispensable adaptive survival mechanism, autophagy was speculated by researchers that may play a role in HCC metastasis by promoting the metastatic colonization of HCC cells (Peng et al., 2013). Scientists found that glycochenodeoxycholate, which is important for the synthesis of BAs, mediated autophagy in HCC cells through the AMP-activated protein kinase (AMPK)/mTOR signaling pathway, exhibiting a pro-metastatic effect both in vitro and in vivo (Gao et al., 2019).
3.3 Affecting the tumor microenvironment
The tumor microenvironment (TME) is an intricate network, predominantly consisting of cancer cells, vascular endothelial cells, penetrating immune cells, carcinoma-linked fibroblasts, and adipose tissues. This milieu is vital for the advancement and multiplication of HCC (Giraldo et al., 2019; Li et al., 2007). Immune cell infiltration including CD4(+) T cells, CD56 NK cells, macrophage and so on (Liu Y. et al., 2022; Bao et al., 2019). In the TME of HCC, the lipid profile of macrophages changes (Yeung et al., 2015). Previous studies have shown that activating M2 macrophage polarization could promote HCC cell invasion and migration (Huang et al., 2023). Aberrant BAs metabolism has been found to modulate the TME by preventing natural killer T (NKT) cell recruitment and increasing M2-like tumor-associated macrophages (TAMs) polarization, thereby promoting tumor immune escape and HCC development (Xia et al., 2022). Immune responses requires CD4(+) T cells to against pathogens and cancer cells (Malyshkina et al., 2023). Previous studies have suggested that the development of HCC may be related to the contribution of CD4(+) T cells loss to the impact. Scientists suggested that linoleic acid can disrupt mitochondrial function and cause more oxidative damage, producing more mitochondria-derived reactive oxygen species (ROS), which could promote the selective loss of intrahepatic CD4(+) T cells, accelerated MASLD-facilitated HCC (Ma C. et al., 2016). Moreover, the long chain of FA is transported from the cytoplasm into the mitochondria by the carnitine palmitoyl transferase (CPT) system and β oxidation occurs. In HCC, induction of the CPT gene increases ROS and leads to apoptosis of CD4(+) T cells. Linoleic acid, on the other hand, can induce CPT gene expression. This suggests that upregulation of the CPT gene by linoleic acid induces CD4(+) T cell apoptosis, thereby promoting HCC development (Brown et al., 2018). This seems to be contrary to the results of the previous experiments, perhaps due to the different models of research, and the role of linoleic acid in serum and cells may be different.
4 Signaling pathways affecting lipid metabolism in HCC
4.1 PPAR related signaling pathways
Peroxisome proliferator-activated receptor (PPAR) is a FA-activated transcription factor. It has three PPAR subtypes: PPAR α, PPAR γ, and PPAR β/δ. PPAR can trigger FA biosynthesis, is the “bridge” between FA imbalance and the maintaining of cancer cell stemness (Feng et al., 2022). PPAR γ is a key transcription factor related to lipid metabolism. The increased expression of PPAR γ, ATP citrate lyase (ACLY) and acetyl-CoA carboxylase (ACC) are associated with steatosis of HCC (Ning et al., 2022). Besides, PPAR γ regulates lipid synthesis by upregulating the transcription of lipid synthesis enzymes (ACLY, ACC, and FASN) (Desvergne et al., 2006). Numerous studies have established the role of PPAR α in lipid and lipoprotein metabolism of HCC, too. After the activation of PPAR α, the expression of its downstream target genes in liver samples and the level of enzymes involved in the β oxidation of FAs increased. And high PPAR α expression in HCC is associated with poor prognosis (Chen et al., 2021). One study showed that activation of PPAR α in rat HCC cell lines led to reduced triglyceride concentrations in liver, plasma, and very low-density lipoprotein (König and Eder, 2006). Besides, increased expression of PPAR α enhances intracellular oxidative stress, thereby promoting HCC invasion and metastasis (Lin et al., 2021). Retinoid X receptor α (RXR α) is a nuclear receptor for retinoid (Sakai et al., 2022). It has been found a dysregulation of both RXR α function and FA metabolism in HCC (Castro-Gil et al., 2022). Scientists believe that the PPAR α/RXR α signaling pathway can be involved in metabolic diseases in humans by regulating oxidative stress, lipid metabolism, and inflammatory pathways (Yang et al., 2022).
4.2 PI3K/AKT related signaling pathways
Phosphatidylininosine-3 kinases (PI3Ks) are promising medicine targets for therapy of HCC, with four PI3Ks: PI3K α, PI3K β, PI3K δ, and PI3K γ. Studies have shown that loss of PI3K γ reduces tumor development of obesity-promoted HCC through multiple cell types and mechanisms, including steatosis (Becattini et al., 2021). Researchers found that the PI3K-AKT-mTOR pathway can activate lipid synthesis and promote NAFLD-HCC progression (Chen et al., 2019). The oncogenic activation of PI3K-AKT-mTOR signaling can activate sterol regulatory element-binding protein 1 (SREBP1), a central transcription factor that regulates lipid metabolism, which then affects adipogenesis in HCC, thereby killing cancer cells. At the same time, stearoyl-coenzyme A desaturase-1 (SCD 1) is the transcriptional target of SREBP1. Hyperactive mutations in PI3K-AKT-mTOR signaling protect cancer cells from oxidative stress and ferroptosis through SREBP1/SCD 1-mediated lipogenesis. Besides, the AKT/mTOR pathway can regulate SREBP1c and thus regulate de novo adipogenesis (Yi et al., 2020; Yecies et al., 2011).
4.3 AMPK related signaling pathways
AMP-activated protein kinases (AMPKs) are key cellular energy sensors (Li et al., 2011). The AMPK signaling pathway is related to lipid synthesis in HCC, and inhibition of the AMPK pathway can induce lipid synthesis in HCC. This is mainly done by regulating its downstream genes, ACC1 and ACLY (Zhang Y. et al., 2022). Previous studies demonstrate that AMPK could inhibit the transactivity of SREBP1. One study found that the interaction between the HCC-associated protein TD26 and the truncated nuclear SREBP1 form (nSREBP1) could block AMPK-mediated SREBP1 inhibition, resulting in increased lipogenesis and enhanced HCC progression (Wang et al., 2018).
4.4 Others
Exosomes are important tools for intracellular communication and have a significant impact on HCC progression and metastasis. A study investigated the changes of lipid classes in HCC derived exosomes. The researcher collected the exosomes from plasma in patients with cirrhosis (31 with HCC and 41 without HCC). The results demonstrated that some lipid species, such as phosphatidylcholines (18:3e/22:4), phosphatidylcholines (16:1e/22:6), TG (18:0/14:0/16:0), TG (25:0/16:0/17:0), monogalactosyldiacylglycerols (16:0/21:6), et al., were detected in the majority of HCC exosomes but in none of the non-HCC exosomes. Further pathway analysis showed that glycerophospholipid metabolism, retrograde endocannabinoid signaling and ferroptosis were associated with the changes in lipid composition in HCC exosomes (Sanchez et al., 2021).
5 TCM formulae and active components alleviates HCC progression by regulating lipid metabolism
Dysregulated lipid metabolism has been identified as a critical driver of HCC progression. Notably, emerging research reveals that specific TCM formulae and active components may exert anti-HCC activity by regulating lipid metabolism through targeting aberrant cholesterol synthesis, fatty acid oxidation, and lipid droplet accumulation (Tables 1, 2; Figure 2). This metabolic regulation not only suppresses HCC growth but also enhances the efficacy of biological agents, offering a dual therapeutic advantage.
5.1 TCM formulae
Ganfule capsules (GFL), approved by the National Medical Products Administration of China, is used as a complementary alternative therapy for the treatment of HCC. It comprised of 21 herbs, including Codonopsis pilosula (Franch.) Nannf, Trionyx sinensis Weigmann, et al. (Ke et al., 2022). Xu F et al. demonstrated that GFL exerted anti-HCC effects by regulating lipid metabolism. Metabolomics identified 426 metabolites and 343 metabolite variants in the positive and negative ion patterns after GFL treatment in a nude murine model of HepG2 cell injection. These genetic variations are potentially associated with lipid metabolic pathways, and the metabolites derived from these pathways have been implicated in mediating the protective actions of GFL against HCC. For example, GFL can increase the 1-Arachido noylglycerophosphoinositol levels while decreasing Pi-Methylimidazoleacetic acid levels (Xu et al., 2022).
Fuzheng Yiliu Decoction (FZYLD) consists of four herbs: Astragalus mongholicus Bunge, Ligustrum lucidum W.T.Aiton, Dioscorea polystachya Turcz., and Rhinacanthus nasutus (L.) Kurz. Scientists have been using FZYLD in clinical practice for the treatment of liver tumors for many years, and it can improve clinical symptoms, slow down physical weakness after chemotherapy or radiotherapy, improve the quality of life, and prolong the survival time of cancer patients (Chen et al., 2014). In recent years, studies have found that FZYLD could also ameliorate energy and lipid metabolism disorders. Zhang Hongcheng established a rat HCC model to observe the therapeutic effect of FZYLD. They found that the levels of endogenous serum metabolites and cholesterol decreased, whereas those of HDL, VLDL, and FAs significantly increased when the rats in the HCC model group received FZYLD. Furthermore, the serum UFA levels in the HCC group exhibited a significant reduction after treatment (Zhang H. et al., 2022).
Fuzheng Xiaozheng prescription (FZXZP), derived from the famous decoction powder recorded in the Book of Wenyilun during the Ming Dynasty, effectively improves liver function and has beneficial effects on patients with HCC (Liu et al., 2022c). In a rat HCC model induced by diethylnitrosamine, scientists found that FZXZP administration significantly inhibited the progression of HCC in rats. Then, they performed microarrays of circRNA, miRNA and mRNA to further explore the mechanism of action. After a series of screening, they established the competing endogenous RNA networks. Go and KEGG analysis showed that FZXZP promoted lipid-related metabolism by activating the PPAR signaling pathway, AA metabolism, and bile secretion (Li et al., 2022). In another study, researchers also found FZXZP improved the pathological characteristics of HCC rats. Moreover, FZXZP influenced lipid metabolic processes by managing substances like AA, linoleic acid and retinol, as well as increased the production of steroid hormones (Liu et al., 2022d).
Pien Tze Huang (PZH), a formula of Chinese patent medicine, has been used for more than 500 years in China and Southeast Asia to treat various inflammation-related diseases such as hepatitis (Liu et al., 2020). It has also been widely employed in clinical settings for treating various human malignancies, including HCC (Yan et al., 2023). PZH has been approved by the National Medical Products Administration of China for the clinical trial of medicines in advanced primary liver cancer (Lin et al., 2023). A study used PZH to treat Hepa1-6 mice, and the results demonstrated that PZH significantly inhibited xenograft tumor growth. Moreover, a four-fold inhibition of ACSL1 phosphorylation, which is associated with FA biosynthesis/degradation pathway, has been observed in PZH-treated mice (Lin et al., 2023).
Sinikangai fang (SNKAF) decoction is a formula that has been widely used for the treatment of HCC in China. Guo et al. used 4-week-old BALB/c nude mice to construct an HCC xenograft mouse model. They revealed that the anti-cancer effect of SNKAF on HCC was related to cell proliferation and apoptosis, and its action pathway involved the PI3K/Akt pathway axis. SNKAF enables PI3K to induce the activation of the core signaling kinase Akt, which controls a number of downstream effector molecules and drives protein and lipid synthesis and cell development (Guo W. et al., 2022).
Shuihonghuazi Formula (SHHZF), a formula of Chinese medicine, is often used in the treatment of HCC. It consists of four herbs: Persicaria orientalis (L.) Spach, Imperata cylindrica (L.) Raeusch., Ophicalcitumb, and Coix lacryma-jobi var. ma-yuen (Rom.Caill.) Stapf. A study used diethylnitrosamine induced rats as HCC animal model, its results showed that SHHZF promoted the absorption and utilization of linoleic acid and oleic acid, increased the content of AA-like substances, translation of phosphatidylethanolamine to phosphatidylcholine, metabolism of linoleic acid, and inhibited the of abnormal metabolism of BAs in rats with HCC (Bao et al., 2017).
Overall, the above studies showed the positive effect of these formulae in the regulation of abnormal lipid metabolism in HCC. However, the specific targets and signaling pathway by which these formulae regulate lipid metabolism remain insufficiently explored in some studies. Moreover, every formula contains multiple active components. Current research has not fully elucidated which specific components drive lipid metabolism regulation. Additionally, whether the different preparation methods of these formulae will affect the effect of lipid metabolism regulation is still obscure. Therefore, the specific mechanism of these formulae on the regulation of abnormal lipid metabolism in HCC is still worth conducting further research.
5.2 TCM active components
Dihydromyricetin (DMY) is the major flavonoid in Ampelopsis grossedentata (Hand.-Mazz.) W.T.Wang and its pharmacological action has attracted increasing attention in recent years (Guo et al., 2019). The researchers gave HCC cells different concentrations of DMY (0, 20, 40, 80, 160, 320 μM) and observed their effects. The result showed that DMY inhibited EGFR and its downstream pathways by lowering the cholesterol levels, thereby disrupting lipid rafts, which resulted in the inhibition of HCC. Pharmacological experiments revealed that DMY inhibited the migration and invasion of HCC cells and reduced cholesterol levels. At the same time, it also inhibited the proliferation of HCC cells. Furthermore, DMY downregulated the expression of lipid raft markers (CAV1 and FLOT1), as well as EGFR, PI3K, Akt, and STAT3 (Zhang et al., 2023).
Arctium lappa L. is an edible medicinal plant. Arctigenin is a dibenzyl butyrolactone lignan which extracted from Arctium lappa L. (Wang et al., 2023). Previous studies investigating the potential anti-tumor effects of arctigenin using HCC cell lines, and luciferase assays have shown that arctigenin targeted the −450 to −400 region of the ankyrin promoter. This region is also a potential binding site for C/EBPα (a putative tumor suppressor that is upregulated in HCC subsets and required for growth and proliferation of cells). HepG2 and Hep-3B cells were treated with 20 μM arctigenin, and the co-immunoprecipitation assays have shown that the presence of arctigenin increases the binding between C/EBPα and PPAR α. Arctigenin can negatively regulate gankyrin by promoting the binding of C/EBPα and PPAR α, thereby inhibiting HCC (Sun et al., 2018). In addition, researchers have found that the ethanolic extract of Arctium lappa L. root also attenuates MASH-related hepatocarcinogenesis. The administration of Arctium lappa L. reduced the total FAs and lipid hydroperoxide levels in a steatohepatitis-induced HCC model (Romualdo et al., 2020).
Emodin is extracted from Chinese herbs, such as Magnolia champaca (L.) Baill. ex Pierre and Reynoutria japonica Houtt., which can affect the synthesis of FAs (Lee K. H. et al., 2017; Guo Y. et al., 2022). Bioinformatics analysis has shown that emodin effectively inhibits the growth and movement of HCC cells (Gao et al., 2024; Zhou et al., 2019). Emodin-treated human HCC cell, Bel-7402, has been used to study intrinsic lipid production. The researchers treated HCC cell line with different concentration of emodin (0, 25, 50, 100, 200, 400, and 600 μmol/L). After examining the cell viability, 100 μmol/L was considered the optimal concentration and experiments were performed. They found that the TG levels and FA desaturation in Bel-7402 cells decreased upon exposure to emodin. In addition, the expression levels of SREBP1 mRNA, and acid metabolism-related proteins (ACC α, FASN, and stearoyl-CoA A desaturase D) were also reduced. These findings indicate that emodin could promote apoptosis of HCC cells by regulating lipid metabolism in an SREBP1-dependent manner (Yang et al., 2019).
Curcumin (CUR), isolated from Curcuma aromatica Salisb., has an inhibitory effect on inflammation, oxidation, and tumors. It exhibits therapeutic benefits in various types of cancers (Bai et al., 2022; Shelash Al-Hawary et al., 2023). In a study based on network pharmacology of CUR-related mechanisms against HCC, scientists treated HepG2 cells with different concentrations of CUR (0, 20, 40, and 60 μmol/L), and the results revealed that CUR treated HCC by regulating the PI3K-Akt pathway, AMPK pathway, apoptosis and autophagy. It promoted HepG2 cell apoptosis through the p53 pathway and HepG2 autophagy through the AMPK/ULK1 pathway (Chen et al., 2022). Another study used CUR combining with sorafenib to treat H22 bearing mice and the results indicated that CUR prevented HCC progression by downregulating FASN and reducing lipid synthesis. The effect of CUR on lipid metabolism disorders is based on the PI3K/Akt pathway (Man et al., 2020).
Arenobufagin (ArBu), a bufadienolide isolated from Ampelopsis delavayana var. glabra (Diels & Gilg) C.L.Li, exhibits broad-spectrum anti-tumor activity (Chen K. et al., 2023). ArBu regulates lipid homeostasis in HCC cells. HepG2 cells and a xenograft model were used to assess the anti-HCC activity of ArBu. Scientists treated HepG2 cells with ArBu at 1.1 ng/mL and 3.3 ng/mL. The results showed that the effect was better at a concentration of 3.3 ng/mL. ArBu decreased the expression of 65 differentially expressed proteins related to autophagy, apoptosis, and lipid metabolism. This may be related to the arbus glycerophospholipid pathway, which is closely related to the PI3K/Akt pathway and JAK-STAT3 signaling pathway and is an important pathway for ArBu to affect lipid homeostasis (Zhao et al., 2020).
Berberine, an isoquinoline alkaloid extracted from Coptis chinensis Franch., promotes autophagic death in cancer cells (La et al., 2017). It has been reported that berberine can effectively inhibit HCC (Shou and Shaw, 2023). Scientists have isolated and synthesized several berberine analogues (A1-A13) and studied their roles in TG production in HepG2 cells. Berberine at a dose of 15 μM and berberine substituted by 9-O-benzoyl decreased intracellular TG content in HepG2 cells by activating AMPK, a major regulator of lipid metabolism (Cao et al., 2018).
6 Conclusion and perspectives
As a refractory malignant tumor that accounts for a large proportion of human cancers, HCC has been attracting much attention. Although the presence of abnormal lipid metabolism in HCC has been confirmed by numerous studies, there are still some contradictions in the results of HCC-related lipid levels, which make it difficult to further elucidate the relationship between HCC and lipid metabolism. The contradictions in the results may be related with the differences in testing sites, test method, evaluation and inclusion criteria, et al. In the future, more rigorous and standardized experimental design as well as testing and analysis techniques in the research need to be used. TCM formulae and active components has shown unique value on the journey against HCC. Some TCM formulae and active components have been proven in clinical and experimental studies to restore abnormal lipid metabolism in HCC, inhibit HCC progression and improve the life quality of the patients. However, research on the regulation of abnormal lipid metabolism in HCC by TCM formulae and active components is still in its nascent stages, with many aspects requiring in-depth exploration. Firstly, the majority of the current studies remain at the fundamental research stage, and the clinical evidence is obviously insufficient. More multicenter clinical trials are necessary to validate the efficacy and advantages of TCM formulae and their active components in restoring abnormal lipid metabolism in HCC. Secondly, current research on the regulation of HCC lipid metabolism by TCM formulae and active components is mostly limited to the formulae and active components themselves. It is also worth exploring whether their combination with existing drugs or biological agents can help better restore abnormal lipid metabolism in HCC. Recently, research in this field began to appear. For example, compound sophora injection is a formula approved by National Medical Products Administration in China, it could alleviate TAM-mediated immunosuppression with TNFR1 and sensitize HCC to sorafenib (Yang et al., 2020). Thirdly, whether the effect of a formula is accidental or not has become a question and needs to be proved by more research evidence. The quality standards, formulations, and usage methods of TCM formulae and active components also need to be further standardized to ensure the accuracy and reproducibility of experimental results. Fourthly, it is still unclear which specific substances in TCM formulae and active components play pivotal roles in regulating abnormal lipid metabolism in HCC, and their specific targets and pathways of action are also unknown. Emerging technologies such as multi-omics integration, high-throughput screening and computational modeling will help overcome this challenge. Besides, recent studies have reported the changes of lipid composition in exosomes from HCC patients, which will become the candidate biomarkers for early detection and treatment of HCC. Nevertheless, whether TCM formulae and active components can regulate the lipid composition in exosomes of HCC is still unknown, which will become a novel research field in the future. Overall, TCM formulae and active components have great promise in the treatment of HCC. In-depth research on the specific mechanism of them in the regulation of abnormal lipid metabolism in HCC will be helpful for the development of more effective therapeutic strategies for HCC.
Author contributions
YL: Writing – original draft. JY: Writing – review and editing. FY: Writing – review and editing. LL: Writing – review and editing. NZ: Writing – review and editing. CL: Writing – review and editing. AL: Writing – review and editing. XH: Supervision, Validation, Writing – review and editing.
Funding
The author(s) declare that financial support was received for the research and/or publication of this article. This work was supported by National Science Foundation of China (No. 81873053) and Scientific and Technological Innovation Project of China Academy of Chinese Medical Sciences (No. CI2023C065YLL).
Conflict of interest
The authors declare that the research was conducted in the absence of any commercial or financial relationships that could be construed as a potential conflict of interest.
The author(s) declared that they were an editorial board member of Frontiers, at the time of submission. This had no impact on the peer review process and the final decision.
Generative AI statement
The author(s) declare that no Generative AI was used in the creation of this manuscript.
Publisher’s note
All claims expressed in this article are solely those of the authors and do not necessarily represent those of their affiliated organizations, or those of the publisher, the editors and the reviewers. Any product that may be evaluated in this article, or claim that may be made by its manufacturer, is not guaranteed or endorsed by the publisher.
References
Ahmed, E. A., El-Derany, M. O., Anwar, A. M., Saied, E. M., and Magdeldin, S. (2022). Metabolomics and lipidomics screening reveal reprogrammed signaling pathways toward cancer development in non-alcoholic steatohepatitis. Int. J. Mol. Sci. 24, 210. doi:10.3390/ijms24010210
Akkiz, H., Carr, B. I., Guerra, V., Donghia, R., Yalçın, K., Karaoğullarından, U., et al. (2021). Plasma lipids, tumor parameters and survival in HCC patients with HBV and HCV. J. Transl. Sci. 7, 421. doi:10.15761/jts.1000421
Ali, F., Hammad, R., Kotb, F. M., Aglan, R. B., and Alrayes, M. H. (2022). Flow cytometry assessment of monocyte subsets alteration in hepatocellular carcinoma post hepatitis C virus infection. Egypt J. Immunol. 29, 33–45. doi:10.55133/eji.290404
Bai, C., Zhao, J., Su, J., Chen, J., Cui, X., Sun, M., et al. (2022). Curcumin induces mitochondrial apoptosis in human hepatoma cells through BCLAF1-mediated modulation of PI3K/AKT/GSK-3β signaling. Life Sci. 306, 120804. doi:10.1016/j.lfs.2022.120804
Bao, D., Zhao, J., Zhou, X., Yang, Q., Chen, Y., Zhu, J., et al. (2019). Mitochondrial fission-induced mtDNA stress promotes tumor-associated macrophage infiltration and HCC progression. Oncogene 38, 5007–5020. doi:10.1038/s41388-019-0772-z
Bao, Y., Wang, S., Yang, X., Li, T., Xia, Y., and Meng, X. (2017). Metabolomic study of the intervention effects of Shuihonghuazi Formula, a Traditional Chinese Medicinal formulae, on hepatocellular carcinoma (HCC) rats using performance HPLC/ESI-TOF-MS. J. Ethnopharmacol. 198, 468–478. doi:10.1016/j.jep.2017.01.029
Becattini, B., Breasson, L., Sardi, C., Zani, F., and Solinas, G. (2021). PI3Kγ promotes obesity-associated hepatocellular carcinoma by regulating metabolism and inflammation. JHEP Rep. 3, 100359. doi:10.1016/j.jhepr.2021.100359
Berndt, N., Eckstein, J., Heucke, N., Gajowski, R., Stockmann, M., Meierhofer, D., et al. (2019a). Characterization of lipid and lipid droplet metabolism in human HCC. Cells 8, 512. doi:10.3390/cells8050512
Berndt, N., Eckstein, J., Heucke, N., Gajowski, R., Stockmann, M., Meierhofer, D., et al. (2019b). Characterization of lipid and lipid droplet metabolism in human HCC. Cells 8, 512. doi:10.3390/cells8050512
Bian, X., Liu, R., Meng, Y., Xing, D., Xu, D., and Lu, Z. (2021). Lipid metabolism and cancer. J. Exp. Med. 218, e20201606. doi:10.1084/jem.20201606
Bort, A., Sánchez, B. G., de Miguel, I., Mateos-Gómez, P. A., and Diaz-Laviada, I. (2020). Dysregulated lipid metabolism in hepatocellular carcinoma cancer stem cells. Mol. Biol. Rep. 47, 2635–2647. doi:10.1007/s11033-020-05352-3
Brown, Z. J., Fu, Q., Ma, C., Kruhlak, M., Zhang, H., Luo, J., et al. (2018). Carnitine palmitoyltransferase gene upregulation by linoleic acid induces CD4(+) T cell apoptosis promoting HCC development. Cell Death Dis. 9, 620. doi:10.1038/s41419-018-0687-6
Budhu, A., Roessler, S., Zhao, X., Yu, Z., Forgues, M., Ji, J., et al. (2013). Integrated metabolite and gene expression profiles identify lipid biomarkers associated with progression of hepatocellular carcinoma and patient outcomes. Gastroenterology 144, 1066–1075.e1. doi:10.1053/j.gastro.2013.01.054
Cai, F. F., Song, Y. N., Lu, Y. Y., Zhang, Y., Hu, Y. Y., and Su, S. B. (2020). Analysis of plasma metabolic profile, characteristics and enzymes in the progression from chronic hepatitis B to hepatocellular carcinoma. Aging (Albany NY) 12, 14949–14965. doi:10.18632/aging.103554
Calvisi, D. F., Wang, C., Ho, C., Ladu, S., Lee, S. A., Mattu, S., et al. (2011). Increased lipogenesis, induced by AKT-mTORC1-RPS6 signaling, promotes development of human hepatocellular carcinoma. Gastroenterology 140, 1071–1083. doi:10.1053/j.gastro.2010.12.006
Cao, S., Yu, S., Cheng, L., Yan, J., Zhu, Y., Deng, Y., et al. (2018). 9-O-benzoyl-substituted berberine exerts a triglyceride-lowering effect through AMPK signaling pathway in human hepatoma HepG2 cells. Environ. Toxicol. Pharmacol. 64, 11–17. doi:10.1016/j.etap.2018.09.007
Castro-Gil, M. P., Torres-Mena, J. E., Salgado, R. M., Muñoz-Montero, S. A., Martínez-Garcés, J. M., López-Torres, C. D., et al. (2022). The transcriptome of early GGT/KRT19-positive hepatocellular carcinoma reveals a downregulated gene expression profile associated with fatty acid metabolism. Genomics 114, 72–83. doi:10.1016/j.ygeno.2021.11.035
Chen, J., Chen, J., Huang, J., Li, Z., Gong, Y., Zou, B., et al. (2019). HIF-2α upregulation mediated by hypoxia promotes NAFLD-HCC progression by activating lipid synthesis via the PI3K-AKT-mTOR pathway. Aging (Albany NY) 11, 10839–10860. doi:10.18632/aging.102488
Chen, K., Li, A., Wang, J., Li, D., Wang, X., Liu, C., et al. (2023b). Arenobufagin causes ferroptosis in human gastric cancer cells by increasing rev-erbα expression. J. Tradit. Complement. Med. 13, 72–80. doi:10.1016/j.jtcme.2022.10.007
Chen, L., Du, J., Dai, Q., Zhang, H., Pang, W., and Hu, J. (2014). Prediction of anti-tumor chemical probes of a traditional Chinese medicine formula by HPLC fingerprinting combined with molecular docking. Eur. J. Med. Chem. 83, 294–306. doi:10.1016/j.ejmech.2014.06.037
Chen, S. Z., Ling, Y., Yu, L. X., Song, Y. T., Chen, X. F., Cao, Q. Q., et al. (2021). 4-phenylbutyric acid promotes hepatocellular carcinoma via initiating cancer stem cells through activation of PPAR-α. Clin. Transl. Med. 11, e379. doi:10.1002/ctm2.379
Chen, W., Ding, M., Ji, L., Yao, J., Guo, Y., Yan, W., et al. (2023a). Bile acids promote the development of HCC by activating inflammasome. Hepatol. Commun. 7, e0217. doi:10.1097/HC9.0000000000000217
Chen, Y., Li, Q., Ren, S., Chen, T., Zhai, B., Cheng, J., et al. (2022). Investigation and experimental validation of curcumin-related mechanisms against hepatocellular carcinoma based on network pharmacology. J. Zhejiang Univ. Sci. B 23, 682–698. doi:10.1631/jzus.B2200038
Cho, Y., Cho, E. J., Yoo, J. J., Chang, Y., Chung, G. E., Jeong, S. M., et al. (2021). Association between lipid profiles and the incidence of hepatocellular carcinoma: a nationwide population-based study. Cancers (Basel) 13, 1599. doi:10.3390/cancers13071599
Conde de la Rosa, L., Garcia-Ruiz, C., Vallejo, C., Baulies, A., Nuñez, S., Monte, M. J., et al. (2021). STARD1 promotes NASH-driven HCC by sustaining the generation of bile acids through the alternative mitochondrial pathway. J. Hepatol. 74, 1429–1441. doi:10.1016/j.jhep.2021.01.028
Dai, S., Zhu, J., Chen, X., Zheng, L., Li, X., and Liu, L. (2024). Alteration of serum bile acid profiles of HBV-related hepatocellular carcinoma identified by LC-MS/MS. J. Cancer Res. Clin. Oncol. 150, 157. doi:10.1007/s00432-024-05686-6
Desvergne, B., Michalik, L., and Wahli, W. (2006). Transcriptional regulation of metabolism. Physiol. Rev. 86, 465–514. doi:10.1152/physrev.00025.2005
Feng, T., Wu, T., Zhang, Y., Zhou, L., Liu, S., Li, L., et al. (2022). Stemness analysis uncovers that the peroxisome proliferator-activated receptor signaling pathway can mediate fatty acid homeostasis in sorafenib-resistant hepatocellular carcinoma cells. Front. Oncol. 12, 912694. doi:10.3389/fonc.2022.912694
Gao, L., Lv, G., Li, R., Liu, W. T., Zong, C., Ye, F., et al. (2019). Glycochenodeoxycholate promotes hepatocellular carcinoma invasion and migration by AMPK/mTOR dependent autophagy activation. Cancer Lett. 454, 215–223. doi:10.1016/j.canlet.2019.04.009
Gao, X. H., Zhang, S. S., Chen, H., Wang, K., Xie, W., and Wang, F. B. (2018). Lipoprotein (a): a promising prognostic biomarker in patients with hepatocellular carcinoma after curative resection. Onco Targets Ther. 11, 5917–5924. doi:10.2147/OTT.S164273
Gao, Y., Li, Y., Zhu, Y., Luo, Q., Lu, Y., Wen, K., et al. (2024). Emodin is a potential drug targeting CD44-positive hepatocellular cancer. Curr. Cancer Drug Targets 24, 510–518. doi:10.2174/0115680096256913231101103719
Giraldo, N. A., Sanchez-Salas, R., Peske, J. D., Vano, Y., Becht, E., Petitprez, F., et al. (2019). The clinical role of the TME in solid cancer. Br. J. Cancer 120, 45–53. doi:10.1038/s41416-018-0327-z
Guo, L., Zhang, H., and Yan, X. (2019). Protective effect of dihydromyricetin revents fatty liver through nuclear factor-κB/p53/B-cell lymphoma 2-associated X protein signaling pathways in a rat model. Mol. Med. Rep. 19, 1638–1644. doi:10.3892/mmr.2018.9783
Guo, W., Yao, X., Lan, S., Zhang, C., Li, H., Chen, Z., et al. (2022a). Metabolomics and integrated network pharmacology analysis reveal SNKAF decoction suppresses cell proliferation and induced cell apoptisis in hepatocellular carcinoma via PI3K/Akt/P53/FoxO signaling axis. Chin. Med. 17, 76. doi:10.1186/s13020-022-00628-1
Guo, Y., Zhang, R., and Li, W. (2022b). Emodin in cardiovascular disease: the role and therapeutic potential. Front. Pharmacol. 13, 1070567. doi:10.3389/fphar.2022.1070567
Haberl, E. M., Pohl, R., Rein-Fischboeck, L., Höring, M., Krautbauer, S., Liebisch, G., et al. (2021). Accumulation of cholesterol, triglycerides and ceramides in hepatocellular carcinomas of diethylnitrosamine injected mice. Lipids Health Dis. 20, 135. doi:10.1186/s12944-021-01567-w
Hsiang, J. C., Wong, G. L., Tse, Y. K., Wong, V. W., Yip, T. C., and Chan, H. L. (2015). Statin and the risk of hepatocellular carcinoma and death in a hospital-based hepatitis B-infected population: a propensity score landmark analysis. J. Hepatol. 63, 1190–1197. doi:10.1016/j.jhep.2015.07.009
Hu, B., Lin, J. Z., Yang, X. B., and Sang, X. T. (2020). Aberrant lipid metabolism in hepatocellular carcinoma cells as well as immune microenvironment: a review. Cell Prolif. 53, e12772. doi:10.1111/cpr.12772
Huang, J., Pan, H., Sun, J., Wu, J., Xuan, Q., Wang, J., et al. (2023). TMEM147 aggravates the progression of HCC by modulating cholesterol homeostasis, suppressing ferroptosis, and promoting the M2 polarization of tumor-associated macrophages. J. Exp. Clin. Cancer Res. 42, 286. doi:10.1186/s13046-023-02865-0
Hymel, E., Vlock, E., Fisher, K. W., and Farazi, P. A. (2022). Differential progression of unhealthy diet-induced hepatocellular carcinoma in obese and non-obese mice. PLoS One 17, e0272623. doi:10.1371/journal.pone.0272623
Ismail, I. T., Elfert, A., Helal, M., Salama, I., El-Said, H., and Fiehn, O. (2020). Remodeling lipids in the transition from chronic liver disease to hepatocellular carcinoma. Cancers (Basel) 13, 88. doi:10.3390/cancers13010088
Jee, S. H., Kim, M., Kim, M., Yoo, H. J., Kim, H., Jung, K. J., et al. (2018). Metabolomics profiles of hepatocellular carcinoma in a Korean prospective cohort: the Korean cancer prevention study-II. Cancer Prev. Res. (Phila) 11, 303–312. doi:10.1158/1940-6207.CAPR-17-0249
Jia, W., Yuan, J., Zhang, J., Li, S., Lin, W., and Cheng, B. (2024). Bioactive sphingolipids as emerging targets for signal transduction in cancer development. Biochim. Biophys. Acta Rev. Cancer 1879, 189176. doi:10.1016/j.bbcan.2024.189176
Jiao, J., Kwan, S. Y., Sabotta, C. M., Tanaka, H., Veillon, L., Warmoes, M. O., et al. (2021). Circulating fatty acids associated with advanced liver fibrosis and hepatocellular carcinoma in South Texas hispanics. Cancer Epidemiol. Biomarkers Prev. 30, 1643–1651. doi:10.1158/1055-9965.EPI-21-0183
Kanda, T., Goto, T., Hirotsu, Y., Moriyama, M., and Omata, M. (2019). Molecular mechanisms driving progression of liver cirrhosis towards hepatocellular carcinoma in chronic hepatitis B and C infections: a review. Int. J. Mol. Sci. 20, 4660. doi:10.3390/ijms20194660
Kawata, S., Yamasaki, E., Nagase, T., Inui, Y., Ito, N., Matsuda, Y., et al. (2001). Effect of pravastatin on survival in patients with advanced hepatocellular carcinoma. A randomized controlled trial. Br. J. Cancer 84, 886–891. doi:10.1054/bjoc.2000.1716
Ke, C., Gao, J., Tu, J., Wang, Y., Xiao, Y., Wu, Y., et al. (2022). Ganfule capsule alleviates bile duct ligation-induced liver fibrosis in mice by inhibiting glutamine metabolism. Front. Pharmacol. 13, 930785. doi:10.3389/fphar.2022.930785
Kim, I., Morimura, K., Shah, Y., Yang, Q., Ward, J. M., and Gonzalez, F. J. (2007). Spontaneous hepatocarcinogenesis in farnesoid X receptor-null mice. Carcinogenesis 28, 940–946. doi:10.1093/carcin/bgl249
König, B., and Eder, K. (2006). Differential action of 13-HPODE on PPARalpha downstream genes in rat Fao and human HepG2 hepatoma cell lines. J. Nutr. Biochem. 17, 410–418. doi:10.1016/j.jnutbio.2005.08.011
La, X., Zhang, L., Li, Z., Yang, P., and Wang, Y. (2017). Berberine-induced autophagic cell death by elevating GRP78 levels in cancer cells. Oncotarget 8, 20909–20924. doi:10.18632/oncotarget.14959
La Fountaine, M. F., Cirnigliaro, C. M., Hobson, J. C., Lombard, A. T., Specht, A. F., Dyson-Hudson, T. A., et al. (2020). Fenofibrate therapy to lower serum triglyceride concentrations in persons with spinal cord injury: a preliminary analysis of its safety profile. J. Spinal Cord. Med. 43, 704–709. doi:10.1080/10790268.2019.1581694
Lee, J., Kim, Y., Friso, S., and Choi, S. W. (2017a). Epigenetics in non-alcoholic fatty liver disease. Mol. Asp. Med. 54, 78–88. doi:10.1016/j.mam.2016.11.008
Lee, K. H., Lee, M. S., Cha, E. Y., Sul, J. Y., Lee, J. S., Kim, J. S., et al. (2017b). Inhibitory effect of emodin on fatty acid synthase, colon cancer proliferation and apoptosis. Mol. Med. Rep. 15, 2163–2173. doi:10.3892/mmr.2017.6254
Li, H., Fan, X., and Houghton, J. (2007). Tumor microenvironment: the role of the tumor stroma in cancer. J. Cell Biochem. 101, 805–815. doi:10.1002/jcb.21159
Li, X., Yu, H., Gong, Y., Wu, P., Feng, Q., and Liu, C. (2022). Fuzheng Xiaozheng prescription relieves rat hepatocellular carcinoma through improving anti-inflammation capacity and regulating lipid related metabolisms. J. Ethnopharmacol. 284, 114801. doi:10.1016/j.jep.2021.114801
Li, Y., Xu, S., Mihaylova, M. M., Zheng, B., Hou, X., Jiang, B., et al. (2011). AMPK phosphorylates and inhibits SREBP activity to attenuate hepatic steatosis and atherosclerosis in diet-induced insulin-resistant mice. Cell Metab. 13, 376–388. doi:10.1016/j.cmet.2011.03.009
Li, Z., Liao, X., Hu, Y., Li, M., Tang, M., Zhang, S., et al. (2023b). SLC27A4-mediated selective uptake of mono-unsaturated fatty acids promotes ferroptosis defense in hepatocellular carcinoma. Free Radic. Biol. Med. 201, 41–54. doi:10.1016/j.freeradbiomed.2023.03.013
Li, Z., Wang, Y., Xing, R., Zeng, H., Yu, X. J., Zhang, Y. J., et al. (2023a). Cholesterol efflux drives the generation of immunosuppressive macrophages to promote the progression of human hepatocellular carcinoma. Cancer Immunol. Res. 11, 1400–1413. doi:10.1158/2326-6066.CIR-22-0907
Lian, X., Wang, G., Zhou, H., Zheng, Z., Fu, Y., and Cai, L. (2018). Anticancer properties of fenofibrate: a repurposing use. J. Cancer 9, 1527–1537. doi:10.7150/jca.24488
Lin, J., Wang, S., Lan, W., Ji, M., and Li, M. (2023). Pien Tze Huang regulates phosphorylation of metabolic enzymes in mice of hepatocellular carcinoma. Sci. Rep. 13, 1897. doi:10.1038/s41598-023-29116-8
Lin, Y. X., Wu, X. B., Zheng, C. W., Zhang, Q. L., Zhang, G. Q., Chen, K., et al. (2021). Mechanistic investigation on the regulation of FABP1 by the IL-6/miR-603 signaling in the pathogenesis of hepatocellular carcinoma. Biomed. Res. Int. 2021, 8579658. doi:10.1155/2021/8579658
Liu, C., Huang, R., Yu, H., Gong, Y., Wu, P., Feng, Q., et al. (2022c). Fuzheng Xiaozheng prescription exerts anti-hepatocellular carcinoma effects by improving lipid and glucose metabolisms via regulating circRNA-miRNA-mRNA networks. Phytomedicine 103, 154226. doi:10.1016/j.phymed.2022.154226
Liu, C., Yu, H., Li, X., Gong, Y., Wu, P., and Feng, Q. S. (2022d). Anti-hepatocellular carcinoma efficacy of Fuzheng Xiaozheng prescription and its interventional mechanism studies. J. Ethnopharmacol. 285, 114913. doi:10.1016/j.jep.2021.114913
Liu, J., Geng, W., Sun, H., Liu, C., Huang, F., Cao, J., et al. (2022a). Integrative metabolomic characterisation identifies altered portal vein serum metabolome contributing to human hepatocellular carcinoma. Gut 71, 1203–1213. doi:10.1136/gutjnl-2021-325189
Liu, S., Wang, R., Lou, Y., and Liu, J. (2020). Uncovering the mechanism of the effects of pien-tze-huang on liver cancer using network pharmacology and molecular docking. Evid. Based Complement. Altern. Med. 2020, 4863015. doi:10.1155/2020/4863015
Liu, Y., Guo, Q., Yang, H., Zhang, X. W., Feng, N., Wang, J. K., et al. (2022b). Allosteric regulation of IGF2BP1 as a novel strategy for the activation of tumor immune microenvironment. ACS Cent. Sci. 8, 1102–1115. doi:10.1021/acscentsci.2c00107
Luo, J., Yang, H., and Song, B. L. (2020). Mechanisms and regulation of cholesterol homeostasis. Nat. Rev. Mol. Cell Biol. 21, 225–245. doi:10.1038/s41580-019-0190-7
Ma, C., Kesarwala, A. H., Eggert, T., Medina-Echeverz, J., Kleiner, D. E., Jin, P., et al. (2016b). NAFLD causes selective CD4(+) T lymphocyte loss and promotes hepatocarcinogenesis. Nature 531, 253–257. doi:10.1038/nature16969
Ma, X. L., Gao, X. H., Gong, Z. J., Wu, J., Tian, L., Zhang, C. Y., et al. (2016a). Apolipoprotein A1: a novel serum biomarker for predicting the prognosis of hepatocellular carcinoma after curative resection. Oncotarget 7, 70654–70668. doi:10.18632/oncotarget.12203
Malyshkina, A., Brüggemann, A., Paschen, A., and Dittmer, U. (2023). Cytotoxic CD4(+) T cells in chronic viral infections and cancer. Front. Immunol. 14, 1271236. doi:10.3389/fimmu.2023.1271236
Man, S., Yao, J., Lv, P., Liu, Y., Yang, L., and Ma, L. (2020). Curcumin-enhanced antitumor effects of sorafenib via regulating the metabolism and tumor microenvironment. Food Funct. 11, 6422–6432. doi:10.1039/c9fo01901d
Nie, J., Lin, B., Zhou, M., Wu, L., and Zheng, T. (2018). Role of ferroptosis in hepatocellular carcinoma. J. Cancer Res. Clin. Oncol. 144, 2329–2337. doi:10.1007/s00432-018-2740-3
Ning, Z., Guo, X., Liu, X., Lu, C., Wang, A., Wang, X., et al. (2022). USP22 regulates lipidome accumulation by stabilizing PPARγ in hepatocellular carcinoma. Nat. Commun. 13, 2187. doi:10.1038/s41467-022-29846-9
Paul, B., Lewinska, M., and Andersen, J. B. (2022). Lipid alterations in chronic liver disease and liver cancer. JHEP Rep. 4, 100479. doi:10.1016/j.jhepr.2022.100479
Peng, J. Y., Cai, D. K., Zeng, R. L., Zhang, C. Y., Li, G. C., Chen, S. F., et al. (2022). Upregulation of superenhancer-driven LncRNA FASRL by USF1 promotes de novo fatty acid biosynthesis to exacerbate hepatocellular carcinoma. Adv. Sci. (Weinh) 10, e2204711. doi:10.1002/advs.202204711
Peng, Y. F., Shi, Y. H., Shen, Y. H., Ding, Z. B., Ke, A. W., Zhou, J., et al. (2013). Promoting colonization in metastatic HCC cells by modulation of autophagy. PLoS One 8, e74407. doi:10.1371/journal.pone.0074407
Petrick, J. L., Florio, A. A., Koshiol, J., Pfeiffer, R. M., Yang, B., Yu, K., et al. (2020). Prediagnostic concentrations of circulating bile acids and hepatocellular carcinoma risk: REVEAL-HBV and HCV studies. Int. J. Cancer 147, 2743–2753. doi:10.1002/ijc.33051
Rashid, M. M., Varghese, R. S., Ding, Y., and Ressom, H. W. (2023). Biomarker discovery for hepatocellular carcinoma in patients with liver cirrhosis using untargeted metabolomics and lipidomics studies. Metabolites 13, 1047. doi:10.3390/metabo13101047
Romualdo, G. R., Silva, E. D. A., Da Silva, T. C., Aloia, T. P. A., Nogueira, M. S., De Castro, I. A., et al. (2020). Burdock (Arctium lappa L.) root attenuates preneoplastic lesion development in a diet and thioacetamide-induced model of steatohepatitis-associated hepatocarcinogenesis. Environ. Toxicol. 35, 518–527. doi:10.1002/tox.22887
Sakai, H., Yamada, Y., Kubota, M., Imai, K., Shirakami, Y., Tomita, H., et al. (2022). The phosphorylated retinoid X receptor-α promotes diethylnitrosamine-induced hepatocarcinogenesis in mice through the activation of β-catenin signaling pathway. Carcinogenesis 43, 254–263. doi:10.1093/carcin/bgab099
Sanchez, J. I., Jiao, J., Kwan, S. Y., Veillon, L., Warmoes, M. O., Tan, L., et al. (2021). Lipidomic profiles of plasma exosomes identify candidate biomarkers for early detection of hepatocellular carcinoma in patients with cirrhosis. Cancer Prev. Res. (Phila) 14, 955–962. doi:10.1158/1940-6207.CAPR-20-0612
Sangineto, M., Villani, R., Cavallone, F., Romano, A., Loizzi, D., and Serviddio, G. (2020). Lipid metabolism in development and progression of hepatocellular carcinoma. Cancers (Basel) 12, 1419. doi:10.3390/cancers12061419
Seo, J., Jeong, D. W., Park, J. W., Lee, K. W., Fukuda, J., and Chun, Y. S. (2020). Fatty-acid-induced FABP5/HIF-1 reprograms lipid metabolism and enhances the proliferation of liver cancer cells. Commun. Biol. 3, 638. doi:10.1038/s42003-020-01367-5
Shao, J. Y., Lee, F. P., Chang, C. L., and Wu, S. Y. (2015). Statin-based palliative therapy for hepatocellular carcinoma. Med. Baltim. 94, e1801. doi:10.1097/MD.0000000000001801
Shelash Al-Hawary, S. I., Abdalkareem Jasim, S., M, M. K., Jaafar Saadoon, S., Ahmad, I., Romero Parra, R. M., et al. (2023). Curcumin in the treatment of liver cancer: from mechanisms of action to nanoformulations. Phytother. Res. 37, 1624–1639. doi:10.1002/ptr.7757
Shou, J. W., and Shaw, P. C. (2023). Berberine activates PPARδ and promotes gut microbiota-derived butyric acid to suppress hepatocellular carcinoma. Phytomedicine 115, 154842. doi:10.1016/j.phymed.2023.154842
Stepien, M., Lopez-Nogueroles, M., Lahoz, A., Kühn, T., Perlemuter, G., Voican, C., et al. (2022). Prediagnostic alterations in circulating bile acid profiles in the development of hepatocellular carcinoma. Int. J. Cancer 150, 1255–1268. doi:10.1002/ijc.33885
Sun, Y., Tan, Y. J., Lu, Z. Z., Li, B. B., Sun, C. H., Li, T., et al. (2018). Arctigenin inhibits liver cancer tumorigenesis by inhibiting gankyrin expression via C/EBPα and PPARα. Front. Pharmacol. 9, 268. doi:10.3389/fphar.2018.00268
Tasdighi, E., Adhikari, R., Almaadawy, O., Leucker, T. M., and Blaha, M. J. (2024). LP(a): structure, genetics, associated cardiovascular risk, and emerging therapeutics. Annu. Rev. Pharmacol. Toxicol. 64, 135–157. doi:10.1146/annurev-pharmtox-031023-100609
Tessitore, A., Cicciarelli, G., Del Vecchio, F., Gaggiano, A., Verzella, D., Fischietti, M., et al. (2016). MicroRNA expression analysis in high fat diet-induced NAFLD-NASH-HCC progression: study on C57BL/6J mice. BMC Cancer 16, 3. doi:10.1186/s12885-015-2007-1
Thomas, C. E., Luu, H. N., Wang, R., Xie, G., Adams-Haduch, J., Jin, A., et al. (2021). Association between pre-diagnostic serum bile acids and hepatocellular carcinoma: the Singapore Chinese health study. Cancers (Basel) 13, 2648. doi:10.3390/cancers13112648
Venturini, I., Amedei, R., Modonesi, G., Cosenza, R., Miglioli, L., Cioni, G., et al. (1999). May plasma cholesterol level be considered a neoplastic marker in liver disease from cirrhosis to hepatocellular carcinoma? Ital. J. Gastroenterol. Hepatol. 31, 61–65.
Vlock, E. M., Karanjit, S., Talmon, G., and Farazi, P. A. (2020). Reduction of polyunsaturated fatty acids with tumor progression in a lean non-alcoholic steatohepatitis-associated hepatocellular carcinoma mouse model. J. Cancer 11, 5536–5546. doi:10.7150/jca.48495
Vogel, A., Meyer, T., Sapisochin, G., Salem, R., and Saborowski, A. (2022). Hepatocellular carcinoma. Lancet 400, 1345–1362. doi:10.1016/S0140-6736(22)01200-4
Wang, C., Tong, Y., Wen, Y., Cai, J., Guo, H., Huang, L., et al. (2018). Hepatocellular carcinoma-associated protein TD26 interacts and enhances sterol regulatory element-binding protein 1 activity to promote tumor cell proliferation and growth. Hepatology 68, 1833–1850. doi:10.1002/hep.30030
Wang, G., Ge, L., Liu, T., Zheng, Z., and Chen, L. (2023). The therapeutic potential of arctigenin against multiple human diseases: a mechanistic review. Phytomedicine 110, 154647. doi:10.1016/j.phymed.2023.154647
Wang, S., Fu, J. L., Hao, H. F., Jiao, Y. N., Li, P. P., and Han, S. Y. (2021). Metabolic reprogramming by traditional Chinese medicine and its role in effective cancer therapy. Pharmacol. Res. 170, 105728. doi:10.1016/j.phrs.2021.105728
Wang, X., and Lu, J. (2024). Immunotherapy for hepatocellular carcinoma. Chin. Med. J. Engl. 137, 1765–1776. doi:10.1097/cm9.0000000000003060
Wei, J., Liu, Z., He, J., Liu, Q., Lu, Y., He, S., et al. (2022). Traditional Chinese medicine reverses cancer multidrug resistance and its mechanism. Clin. Transl. Oncol. 24, 471–482. doi:10.1007/s12094-021-02716-4
Xia, J. K., Tang, N., Wu, X. Y., and Ren, H. Z. (2022). Deregulated bile acids may drive hepatocellular carcinoma metastasis by inducing an immunosuppressive microenvironment. Front. Oncol. 12, 1033145. doi:10.3389/fonc.2022.1033145
Xie, D. Y., Ren, Z. G., Zhou, J., Fan, J., and Gao, Q. (2020). 2019 Chinese clinical guidelines for the management of hepatocellular carcinoma: updates and insights. Hepatobiliary Surg. Nutr. 9, 452–463. doi:10.21037/hbsn-20-480
Xu, F., Li, H., Pan, Y., Zeng, Y., Li, J., and Li, S. (2022). Effects of Ganfule capsule on microbial and metabolic profiles in anti-hepatocellular carcinoma. J. Appl. Microbiol. 132, 2280–2292. doi:10.1111/jam.15307
Xu, K., Xia, P., Chen, X., Ma, W., and Yuan, Y. (2023). ncRNA-mediated fatty acid metabolism reprogramming in HCC. Trends Endocrinol. Metab. 34, 278–291. doi:10.1016/j.tem.2023.02.007
Yan, X., Liu, Y., Li, C., Mao, X., Xu, T., Hu, Z., et al. (2023). Pien-Tze-Huang prevents hepatocellular carcinoma by inducing ferroptosis via inhibiting SLC7A11-GSH-GPX4 axis. Cancer Cell Int. 23, 109. doi:10.1186/s12935-023-02946-2
Yang, B., Lu, L., Zhou, D., Fan, W., Barbier-Torres, L., Steggerda, J., et al. (2022). Regulatory network and interplay of hepatokines, stellakines, myokines and adipokines in nonalcoholic fatty liver diseases and nonalcoholic steatohepatitis. Front. Endocrinol. (Lausanne) 13, 1007944. doi:10.3389/fendo.2022.1007944
Yang, N., Li, C., Li, H., Liu, M., Cai, X., Cao, F., et al. (2019). Emodin induced SREBP1-dependent and SREBP1-independent apoptosis in hepatocellular carcinoma cells. Front. Pharmacol. 10, 709. doi:10.3389/fphar.2019.00709
Yang, R., Gao, W., Wang, Z., Jian, H., Peng, L., Yu, X., et al. (2024). Polyphyllin I induced ferroptosis to suppress the progression of hepatocellular carcinoma through activation of the mitochondrial dysfunction via Nrf2/HO-1/GPX4 axis. Phytomedicine 122, 155135. doi:10.1016/j.phymed.2023.155135
Yang, Y., Sun, M., Yao, W., Wang, F., Li, X., Wang, W., et al. (2020). Compound kushen injection relieves tumor-associated macrophage-mediated immunosuppression through TNFR1 and sensitizes hepatocellular carcinoma to sorafenib. J. Immunother. Cancer 8, e000317. doi:10.1136/jitc-2019-000317
Yecies, J. L., Zhang, H. H., Menon, S., Liu, S., Yecies, D., Lipovsky, A. I., et al. (2011). Akt stimulates hepatic SREBP1c and lipogenesis through parallel mTORC1-dependent and independent pathways. Cell Metab. 14, 21–32. doi:10.1016/j.cmet.2011.06.002
Yeung, O. W., Lo, C. M., Ling, C. C., Qi, X., Geng, W., Li, C. X., et al. (2015). Alternatively activated (M2) macrophages promote tumour growth and invasiveness in hepatocellular carcinoma. J. Hepatol. 62, 607–616. doi:10.1016/j.jhep.2014.10.029
Yi, J., Zhu, J., Wu, J., Thompson, C. B., and Jiang, X. (2020). Oncogenic activation of PI3K-AKT-mTOR signaling suppresses ferroptosis via SREBP-mediated lipogenesis. Proc. Natl. Acad. Sci. U. S. A. 117, 31189–31197. doi:10.1073/pnas.2017152117
Yuan, J., Lv, T., Yang, J., Wu, Z., Yan, L., Yang, J., et al. (2022). The lipid transporter HDLBP promotes hepatocellular carcinoma metastasis through BRAF-dependent epithelial-mesenchymal transition. Cancer Lett. 549, 215921. doi:10.1016/j.canlet.2022.215921
Yuan, Y., Xu, J., Jiang, Q., Yang, C., Wang, N., Liu, X., et al. (2024). Ficolin 3 promotes ferroptosis in HCC by downregulating IR/SREBP axis-mediated MUFA synthesis. J. Exp. Clin. Cancer Res. 43, 133. doi:10.1186/s13046-024-03047-2
Zhang, H., Dai, Q., Zeng, M., Liu, Y., Du, J., Pang, W., et al. (2022b). Investigating the metabolic level of endogenous and exogenous substances on the intervention of traditional Chinese medicine Fuzheng Yiliu decoction in a rat orthotopic liver cancer model. Cancer Manag. Res. 14, 2785–2801. doi:10.2147/cmar.s377621
Zhang, S., Shi, Y. N., Gu, J., He, P., Ai, Q. D., Zhou, X. D., et al. (2023). Mechanisms of dihydromyricetin against hepatocellular carcinoma elucidated by network pharmacology combined with experimental validation. Pharm. Biol. 61, 1108–1119. doi:10.1080/13880209.2023.2234000
Zhang, X., Coker, O. O., Chu, E. S., Fu, K., Lau, H. C. H., Wang, Y. X., et al. (2021). Dietary cholesterol drives fatty liver-associated liver cancer by modulating gut microbiota and metabolites. Gut 70, 761–774. doi:10.1136/gutjnl-2019-319664
Zhang, Y., Xu, J., Qiu, Z., Guan, Y., Zhang, X., Zhang, X., et al. (2022a). STK25 enhances hepatocellular carcinoma progression through the STRN/AMPK/ACC1 pathway. Cancer Cell Int. 22, 4. doi:10.1186/s12935-021-02421-w
Zhao, L. J., Zhao, H. Y., Wei, X. L., Guo, F. F., Wei, J. Y., Wang, H. J., et al. (2020). The lipid homeostasis regulation study of arenobufagin in zebrafish HepG2 xenograft model and HepG2 cells using integrated lipidomics-proteomics approach. J. Ethnopharmacol. 260, 112943. doi:10.1016/j.jep.2020.112943
Zhou, R. S., Wang, X. W., Sun, Q. F., Ye, Z. J., Liu, J. W., Zhou, D. H., et al. (2019). Anticancer effects of emodin on HepG2 cell: evidence from bioinformatic analysis. Biomed. Res. Int. 2019, 3065818. doi:10.1155/2019/3065818
Zuo, D., An, H., Li, J., Xiao, J., and Ren, L. (2021). The application value of lipoprotein particle numbers in the diagnosis of HBV-related hepatocellular carcinoma with BCLC stage 0-A. J. Pers. Med. 11, 1143. doi:10.3390/jpm11111143
Glossary
AA Arachidonic acid
ACC ATP citrate lyase
ACLY Acetyl-CoA carboxylase
AMPK AMP-activated protein kinase
ApoA-1 Apolipoprotein A1
ArBu Arenobufagin
BAs Bile acids
CM Chylomicrons
CPT Carnitine palmitoyltransferase
CUR Curcumin
CYP8B1 Cytochrome P450 family 8 subfamily B member 1
DMY Dihydromyricetin
EMT Epithelial-mesenchymal transition
FAs Fatty acids
FXR Farnesoid X receptor
FZXZP Fuzheng Xiaozheng prescription
FZYLD Fuzheng Yiliu Decoction
GFL Ganfule capsules
HBV Hepatitis B virus
HCC Hepatocellular carcinoma
HCV Hepatitis C virus
HDL High-density lipoproteins
HDLBP High-density lipoprotein-binding protein
HMG-CoA 3-hydroxy-3-methyl-glutaryl-coenzyme A
IDL Intermediate-density lipoprotein
LDL Low-density lipoproteins
LDL-C Low-density lipoprotein cholesterol
Lp (a) Lipoprotein (a)
MASH Metabolic dysfunction-associated steatohepatitis
MASLD Metabolic dysfunction-associated steatotic liver disease
MUFAs Monounsaturated fatty acids
NKT Natural killer T
PPAR Peroxisome proliferator-activated receptor
PUFAs Polyunsaturated fatty acids
PZH Pien Tze Huang
ROS Reactive oxygen species
RXRB Retinoid X receptor β
SCD Stearyl-CoA desaturase
SFAs Saturated fatty acids
SHHZF Shuihonghuazi Formula
SNKAF Sinikangai fang
SREBP1 Sterol regulatory element-binding protein 1
TAMs Tumor-associated macrophages
TCM Traditional Chinese medicine
TG Triglycerides
TME Tumor microenvironment
UFAs Unsaturated fatty acids
VLC Very long chain
VLDL Very-low-density lipoproteins
Keywords: hepatocellular carcinoma, lipid metabolism, traditional Chinese medicine, formulae, active components
Citation: Liu Y, Yang J, Yu F, Li L, Zhao N, Lu C, Lu A and He X (2025) Research advances in traditional Chinese medicine formulae and active components targeting lipid metabolism for hepatocellular carcinoma therapy. Front. Pharmacol. 16:1528671. doi: 10.3389/fphar.2025.1528671
Received: 15 November 2024; Accepted: 11 April 2025;
Published: 25 April 2025.
Edited by:
Rajeev K. Singla, Sichuan University, ChinaReviewed by:
Zhi Yong Du, Capital Medical University, ChinaWentao Jia, Naval Medical Center, China
Greg Potter, Independent Contractor, Halifax, Canada
Copyright © 2025 Liu, Yang, Yu, Li, Zhao, Lu, Lu and He. This is an open-access article distributed under the terms of the Creative Commons Attribution License (CC BY). The use, distribution or reproduction in other forums is permitted, provided the original author(s) and the copyright owner(s) are credited and that the original publication in this journal is cited, in accordance with accepted academic practice. No use, distribution or reproduction is permitted which does not comply with these terms.
*Correspondence: Cheng Lu, bHZfY2hlbmcwODE2QDE2My5jb20=; Aiping Lu, YWlwaW5nbHVAaGtidS5lZHUuaGs=; Xiaojuan He, aHhqMTlAMTI2LmNvbQ==