- 1Association Cancer et Métabolisme, Nîmes, France
- 2Institut de NeuroPhysiopathologie (INP), CNRS UMR 7051, Marseille, France
Corticosteroids (CSs), widely used in oncology for their anti-inflammatory and immunosuppressive properties, help manage cancer-related symptoms and side effects. However, their long-term use may negatively affect patient survival and exacerbate tumor progression. Elevated glucose and glutamine metabolism, disruption of vitamin D levels, and alterations in the microbiome are some of the key factors contributing to these adverse outcomes. Approaches such as ketogenic diets, fasting, sartans, and vitamin D supplementation have shown promise in providing similar benefits to CSs while mitigating the risks associated with the mechanisms identified as contributing to tumor progression. This perspective underscores the necessity for a reevaluation of CSs use in cancer care and advocates for further research into safer, more effective therapeutic strategies.
1 Introduction
Corticosteroids (CSs) are synthetic drugs with a broad range of effects on cells and tissues, widely used in oncology due to their ability to regulate inflammation and modulate the immune response (Goodman et al., 2023). These include hydrocortisone, prednisone, prednisolone, methylprednisolone, and dexamethasone (Faggiano et al., 2022). While hydrocortisone has both glucocorticoid (anti-inflammatory and immunosuppressive) and mineralocorticoid (electrolyte balance) activities, the other CSs mentioned, including prednisone, prednisolone, methylprednisolone, and dexamethasone, exhibit little to no mineralocorticoid activity. In oncology, high-dose CSs are commonly used for a variety of purposes: managing cancer-related symptoms such as fatigue, shortness of breath, pain from bone metastases, or cerebral edema caused by brain metastases; reducing side effects of cancer treatments; managing oncological emergencies; enhancing anti-cancer effects, particularly in hematologic cancers; and treating comorbidities like autoimmune diseases (Faggiano et al., 2022). In this review, we will explore the effects of CSs on survival and the long-term side effects associated with their prolonged use. We will then investigate the mechanisms by which CSs may influence tumor progression. Four key mechanisms have been identified that could explain the negative impact of CSs on survival: altered cancer cell metabolism, the renin-angiotensin system (RAS), vitamin D metabolism, and the microbiome. Based on these mechanisms, we propose therapeutic strategies that may complement or potentially replace CSs in oncological care.
2 Corticosteroids use in oncology: impact on survival, risks, and potential adverse effects
CSs have been widely used in oncology for decades, often as part of standardized treatment protocols for various hematologic and solid malignancies. However, as monotherapy, CSs have not been able to induce long-lasting remissions, let alone cures (Pearson and Eliel, 1950). Their primary role has been in symptom management and as adjuncts to other therapies, rather than as standalone curative agents. In this section, we will examine the role of CSs in oncology, assessing their true impact on treatment outcomes, their contribution to observed survival benefits, and the risks associated with prolonged exposure.
2.1 Effect on survival in hematological cancers
In Non-Hodgkin Lymphoma (NHL), individuals with relapsed or refractory large B-cell lymphoma (LBCL) treated with axi-cel showed an association between higher cumulative corticosteroid doses and shorter progression-free survival (PFS) and overall survival (OS) particularly when corticosteroids were used early or for a prolonged duration (Strati et al., 2021). Furthermore, alternative chemotherapy regimens have shown promising results without CSs. For instance, the bendamustine + rituximab (B + R) regimen, which does not contain CSs, achieved a significantly longer PFS (69.5 vs. 31.2 months; p < 0.0001) and demonstrated better tolerability compared to Rituximab + Cyclophosphamide + Doxorubicine + Vincristine + Prednisone (R-CHOP). However, this improvement in PFS did not translate into a significant overall survival benefit. These findings support B + R as a preferred first-line treatment option for patients with previously untreated indolent lymphoma (Rummel et al., 2013). In Diffuse Large B-Cell Lymphoma (DLBCL), recent evidence also challenges the necessity of CSs. Chimeric Antigen Receptor T-cell (CAR-T cell) therapy has shown higher response rates compared to various alternative chemotherapy regimens, including Cyclophosphamide + Doxorubicine + Vincristine + Prednisone (CHOP, with CSs) (Sermer et al., 2020). Additionally, the ZUMA-1 trial demonstrated that the CAR-T therapy axicabtagene ciloleucel (73% without CSs) provided durable responses in patients with relapsed or refractory large B-cell lymphoma, after failure of multiple prior therapies, including R-CHOP with CSs (Neelapu et al., 2017). A similar trend is observed in Hodgkin Lymphoma (HL). Studies comparing Mustargen (mechlorethamine) + Oncovin (vincristine) + Procarbazine + Prednisone (MOPP, which includes prednisone) and Adriamycine + Bléomycine + Vinblastine + Dacarbazine (ABVD, which does not) indicate that ABVD achieves superior efficacy in terms of complete response rate and failure-free survival, with fewer hematologic toxicities. However, overall survival at 5 years did not differ significantly between the treatment groups (Canellos et al., 1992). Alternating MOPP/ABVD regimens remained similar to ABVD monotherapy (Duggan et al., 2003). Another study found no significant difference in survival between MOPP and ABVD (Ng et al., 2004). The role of CSs in multiple myeloma (MM) can also be questioned. An analysis of 18 clinical trials found no significant difference in overall efficacy between melphalan + prednisone (M + P) and combination chemotherapy (CCT) (Gregory et al., 1992) M+P was more effective in patients with good prognosis (P = .02), while CCT tended to be better for those with poor prognosis (P = .07) (Gregory, Richards et al. 1992). More recent studies suggest that adding CSs to lenalidomide maintenance may not provide significant survival benefits (Bringhen et al., 2017; Bonello et al., 2019). Collectively, these findings suggest that while CSs have historically played a key role in lymphoid malignancies, they are not always indispensable. Their use should be carefully reassessed on a case-by-case basis, weighing potential benefits against toxicity risks. The only cancer type where their necessity could remain unquestioned is leukemia, particularly acute lymphoblastic leukemia (ALL) (Pourhassan et al., 2024). Even in leukemia, certain clinical situations could justify exploring alternative therapeutic approaches. Glucocorticoid resistance in ALL is a major challenge that compromises treatment efficacy (Olivas-Aguirre et al., 2021). Additionally, recent research on CAR T-cell therapy reported that 30 patients received CAR-T anti-CD19. Among them, nine received tocilizumab to manage cytokine release syndrome (CRS), and six required additional CSs. Most patients were successfully treated without the need for CSs (Maude et al., 2014). This further suggests that even in ALL, alternative strategies could be viable. This assumption needs further validation, as the widespread standardization of CSs in this setting has limited the availability of comparative data. More studies are required to evaluate their true indispensability.
2.2 Effect on survival in solid tumors
In the case of solid tumors, their use is more uncertain and may be either beneficial or detrimental. The administration of dexamethasone in glioblastoma, at the start of radiotherapy is associated with reduced median survival and poorer clinical outcomes. Patients on steroids had significantly lower OS (12 vs. 17 months) and PFS (5.3 vs. 6.4 months) (Pitter et al., 2016). The negative effects of steroids were especially pronounced in patients who received radiotherapy alone. These effects were less pronounced, or even non-significant, in those treated with combined chemotherapy and radiotherapy (Pitter et al., 2016). This is confirmed in a meta-analysis of 22 studies including 8,752 patients, which showed that the use of CSs in patients with glioblastoma is associated with a significant reduction in OS (HR = 1.54; 95% CI: 1.37-1.75; p < 0.01) and PFS (HR = 1.28; 95% CI: 1.1-1.49; p < 0.01) (Petrelli et al., 2021). A recent meta-analysis, which included 76 studies and a total of 83,614 patients, revealed that CSs use in advanced solid cancers is associated with decreased OS (hazard ratio [HR] = 1.18, 95% confidence interval [CI]: 1.10–1.26; P < .01, based on 69 studies). PFS was also negatively impacted in steroid users compared to non-users (HR = 1.13, 95% CI: 1.01–1.26; P = .03, based on 40 studies). The study analyzed the effects of steroids across various solid tumors, including non-small cell lung cancer (NSCLC), prostate cancer, breast cancer, gastrointestinal cancers, melanoma, pancreatic cancer, ovarian cancer, and Kaposi’s sarcoma. Steroid use was evaluated in different settings, such as palliative care, in combination with immunotherapies, chemotherapies, and hormonal treatments, as well as post-surgical management (Petrelli et al., 2020). Similarly, a recent systematic review and meta-analysis of 4,045 patients found that steroid use in individuals undergoing immune checkpoint inhibitor (ICI) therapy increased the risk of disease progression and death by 34% and 54% respectively, compared to non-steroid users (Petrelli et al., 2020). Additionally, another meta-analysis demonstrated that CSs administration for cancer-related indications was significantly associated with worse PFS (HR = 1.735, 95% CI: 1.381–2.180) and OS (HR = 1.936, 95% CI: 1.587–2.361) in ICI-treated patients (Wang et al., 2021).
2.3 Adverse effects of corticosteroids
Their use is associated with numerous side effects affecting various systems. Metabolically, they can lead to hyperglycemia, hypertension and weight gain (Kulkarni et al., 2022). Endocrinologically, they increase the risk of Cushing’s syndrome (Oray et al., 2016) and may cause adrenal insufficiency (Caplan et al., 2017). Gastrointestinally, CSs promote the development of gastritis, peptic ulcers, and increase the risk of gastrointestinal bleeding, particularly when combined with nonsteroidal anti-inflammatory drugs (NSAIDs) (Caplan et al., 2017). Cardiovascularly, they raise blood pressure and the risk of heart disease (Pimenta et al., 2012). The risk of hypertension is increased by ∼2-fold in patients treated with CSs regardless of treatment duration (Wei et al., 2004). Furthermore, it has been reported that the use of CSs increases the risk of coronary heart disease, ischemic heart disease, heart failure and even sudden death (Wei et al., 2004). The ALL AIEOP/BFM 2000 study demonstrated a significant increase in deaths during induction therapy with dexamethasone (10 mg/m2), mainly due to severe bacterial and fungal infections, particularly in patients aged ≥10 years (4.5% vs. 2.4% with prednisone) (Schrappe et al., 2008).
Although CSs are effective in symptom management, their long-term survival benefits remain questionable. Given their potential long-term adverse effects, they are not recommended as a prolonged treatment for survival improvement. However, their short-term use can be beneficial in urgent oncological situations such as spinal cord compression, and superior vena cava syndrome, where they play a crucial role in reducing inflammation and temporarily alleviating symptoms (Faggiano et al., 2022).
3 Impact of corticosteroids on cancer cells: unraveling tumor progression and systemic risks
CSs influence various metabolic processes, including glucose and glutamine metabolism, the renin-angiotensin system, vitamin D metabolism, and the microbiome. While these drugs are often considered from the perspective of their beneficial effects, their impact on cancer progression and the systemic balance of patients must be better understood to optimize their use and limit their harmful effects. This section explores these mechanisms to highlight the associated risks and the need for alternative approaches.
3.1 Cancer cell metabolism: a therapeutic contradiction
The metabolism of cancer cells relies heavily on glucose and glutamine as their primary energy sources, and an increase in glucose and glutamine levels may enhance malignancy (Seyfried et al., 2020). The “Warburg effect,” described the last century (Warburg, 1956), highlights the critical role of glucose, while the recent recognition of glutamine’s role has reinforced the idea that both substrates are crucial for tumor growth (Seyfried et al., 2020; Lee et al., 2024). However, CSs use leads to a marked increase in blood glucose levels. A study by Limbachia et al. (2024) demonstrated that all CSs increase glucose concentrations, but dexamethasone and methylprednisolone induce more significant spikes compared to prednisolone or hydrocortisone. Consequently, 10%–30% of cancer patients experience acute hyperglycemia episodes (Hwangbo and Lee, 2017), and some develop corticosteroid-induced diabetes (Suh and Park, 2017). Notably, one study found that 20% of non-diabetic patients treated with dexamethasone for gastrointestinal cancer developed steroid-induced diabetes (Jeong et al., 2016). These metabolic effects have direct clinical consequences: a meta-analysis of 2,168 patients revealed that hyperglycemia, whether pre-existing or corticosteroid-induced, is associated with a significant reduction in overall survival in brain cancers (Liu et al., 2016). Similarly, increased glutamine levels under CSs treatment (Thibault et al., 2008), due to the activation of glutamine synthetase (Kazazoglou et al., 2021) and glutaminase (Sarantos et al., 1992), may promote tumor proliferation.
These findings raise a critical question: is the routine use of CSs, particularly in palliative care patients, truly justified when fatigue remains one of the most prevalent symptoms? While CSs are known to modulate inflammation and improve certain aspects of quality of life, their metabolic effects could paradoxically exacerbate fatigue rather than alleviate. Moreover, their impact on glucose and glutamine metabolism presents a therapeutic contradiction. By increasing circulating glucose and glutamine levels—key fuels for tumor growth—CSs might unintentionally accelerate disease progression. This duality underscores the complexity of their use in oncology, highlighting the need for a more nuanced approach that weighs their symptomatic benefits against potential long-term oncologic risks.
3.2 Renin-angiotensin system: an amplifying mechanism
The RAS plays a key role in maintaining systemic adaptation in cancer (Martinez and Sabatier, 2025). The renin-angiotensin system (RAS) is initiated when the liver releases angiotensinogen, a precursor protein that is cleaved by renin to generate angiotensin I, an inactive form. Angiotensin I is subsequently converted into angiotensin II (Ang II) by the angiotensin-converting enzyme (ACE), which is primarily produced in the liver and functions mainly in the lungs. Ang II exerts its physiological effects through two main receptor types: angiotensin type 1 receptor (AT1R) and type 2 receptor (AT2R) (Catarata et al., 2020). AT1R is frequently overexpressed on the cell surface in many types of cancer (Roth et al., 2019), where it promotes proangiogenic and proinflammatory processes. The use of CSs has been linked to an increase in ACE levels (Fishel et al., 1995), which leads to elevated Ang II concentrations and enhanced expression of AT1R (Shelat et al., 1999). A study highlights that the overexpression of the AT1R receptor is associated with poor overall survival in patients with ESCC and that the angiotensin II/AT1R signaling pathway promotes tumor growth, partly through mTOR activation (Li et al., 2016). The impact of CSs on the renin-angiotensin system, by increasing the expression of angiotensin II and its AT1R receptor, could enhance pro-angiogenic and pro-inflammatory processes, thereby promoting tumor progression and metastasis.
3.3 Vitamin D metabolism: an underestimated impact?
CSs also alter vitamin D metabolism, a hormone with well-recognized anticancer properties (Bikle, 2016). Chronic use of CSs significantly reduces vitamin D levels (Skversky et al., 2011), possibly due to the increase in fat mass they induce (Oray et al., 2016) and the subsequent sequestration of vitamin D in adipose tissues following weight gain (Wortsman et al., 2000). A recent meta-analysis indicates that sufficient vitamin D levels are associated with a reduced risk of cancer-related mortality (Keum et al., 2022).
3.4 Microbiome: an aggravating factor?
Many cancers are generally associated with dysbiosis of the microbiome (an imbalance in gut flora biodiversity), including those affecting the airways and lungs, esophagus, gastric, small intestine, large intestine, liver, pancreas, and kidneys (see Table 2 in Martinez and Sabatier, 2025). Dysbiosis can promote tumor progression and metastases (Sevcikova et al., 2023). A recent study showed that chronic CS use significantly alters gut microbial diversity and composition (Couch et al., 2023), potentially reducing their efficacy and increasing the risk of infection (Chan et al., 2024). Beyond these disruptions, CSs have been linked to specific microbiome alterations in patients with inflammatory bowel disease (IBD) and irritable bowel syndrome (IBS) (Vich Vila et al., 2020). In IBD patients using oral CSs, an increased abundance of Methanobrevibacter smithii—a microorganism associated with enhanced caloric harvest and weight gain (Mathur et al., 2013)—was observed, which is a well-known side effect of corticosteroids (Kulkarni et al., 2022). Meanwhile, IBS patients using inhaled CSs showed an increased abundance of Streptococcus mutans and Bifidobacterium dentium (Vich Vila et al., 2020). A recent study showed that prenatal exposure to dexamethasone alters the composition of the gut microbiota by reducing alpha microbial diversity as well as its function (Lu et al., 2024), which may lead to long-term health consequences, such as diabetes (Greene et al., 2013). CSs, administered at the start of ICI treatment, are associated with reduced overall survival in several cancers, such as non-small cell lung cancer and melanoma. This decrease in survival appears to be linked to the impact of corticosteroids on T-cell mediated inflammation and their influence on the microbiome, thereby altering the response to ICIs (Spakowicz et al., 2020).
The microbiome disruptions induced by these treatments (CSs) raise concerns about their long-term impact on immunity and response to anticancer therapies.
The complex interplay of CSs with glucose and glutamine metabolism, the renin-angiotensin system, vitamin D, and the microbiome underscores the need for a balanced approach in oncology. These effects are summarized in Figure 1.
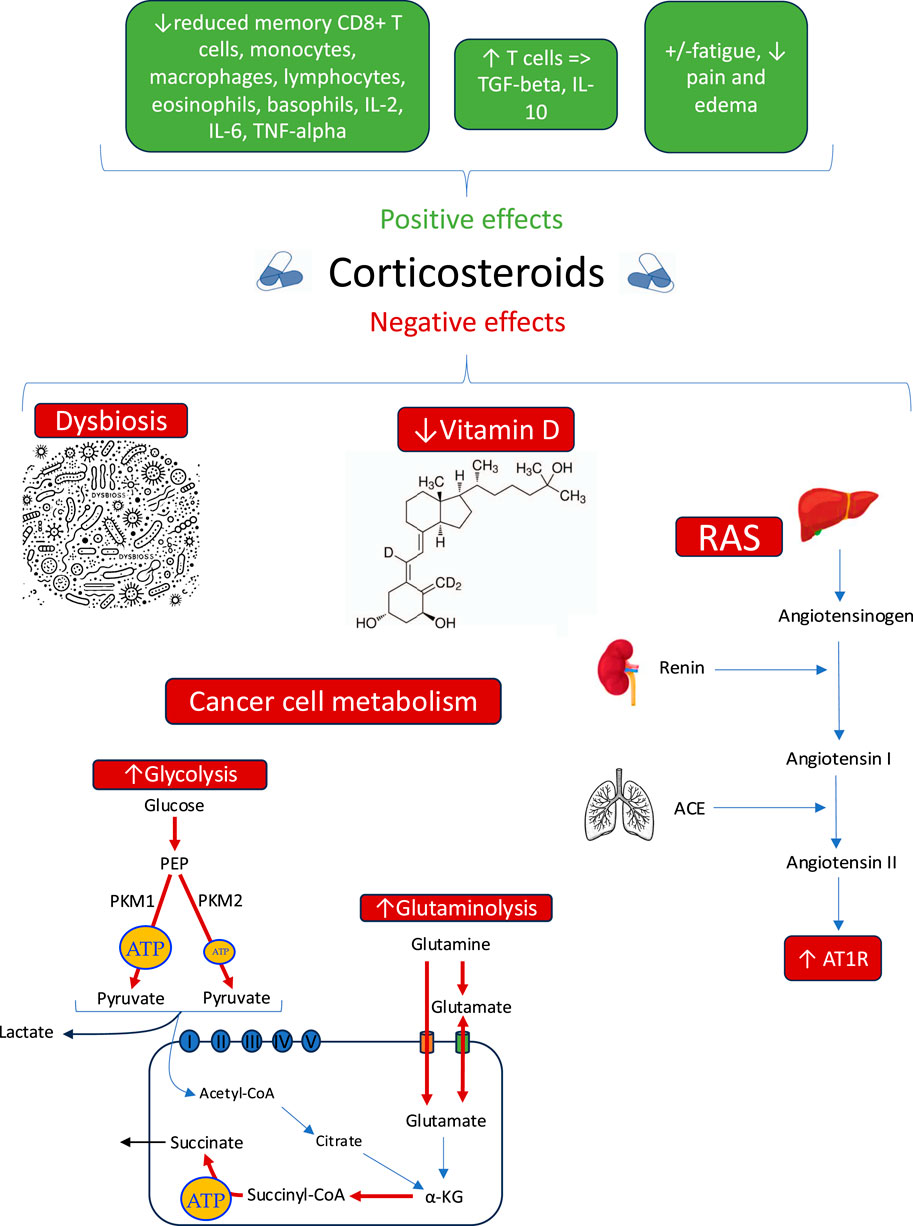
Figure 1. Summary of benefits and risk of corticosteroids use in cancer progression. a-KG: Alpha-ketoglutarate (cycle de Krebs), ACE: Angiotensin-converting enzyme, AT1R: Angiotensin II type 1 receptor, PEP: Phosphoenolpyruvate (glycolyse), PKM1: Pyruvate kinase M1, PKM2: Pyruvate kinase M2, RAS: Renin-angiotensin system.
Given their complex effects on tumor progression, it is becoming essential to explore alternative strategies, such as combining them with metabolic modulators, RAS blockers, or microbiome-targeted interventions, which we will discuss in the next section.
4 Opportunities, challenges, and future directions
While CSs can be beneficial in certain cases, the potential risks highlighted in this article underscore the urgent need to explore alternative therapeutic strategies. For instance, dexamethasone, widely used to manage vasogenic edema associated with tumors or brain metastases, negatively interacts with the metabolic and molecular mechanisms previously described. In murine models, dexamethasone has been shown to reduce the effectiveness of radiotherapy by inducing p21 expression, a cell cycle inhibitor that causes cell accumulation in the G1 phase—a phase resistant to irradiation (Pitter et al., 2016). Despite its effectiveness in managing edema, dexamethasone provides no survival benefit, raising questions about its systematic use in oncology (Pitter et al., 2016). Exploring safer alternatives to CSs is essential to optimizing cancer treatment strategies. Several promising alternatives have been identified, including dietary interventions, renin-angiotensin system (RAS) blockers (sartans), and vitamin D optimization. These approaches not only address inflammation, edema, and pain—key targets of CSs—but also counteract the deleterious metabolic and molecular mechanisms associated with their use. Table 1 below summarizes the potential benefits, additional advantages, and limitations of these alternative strategies.
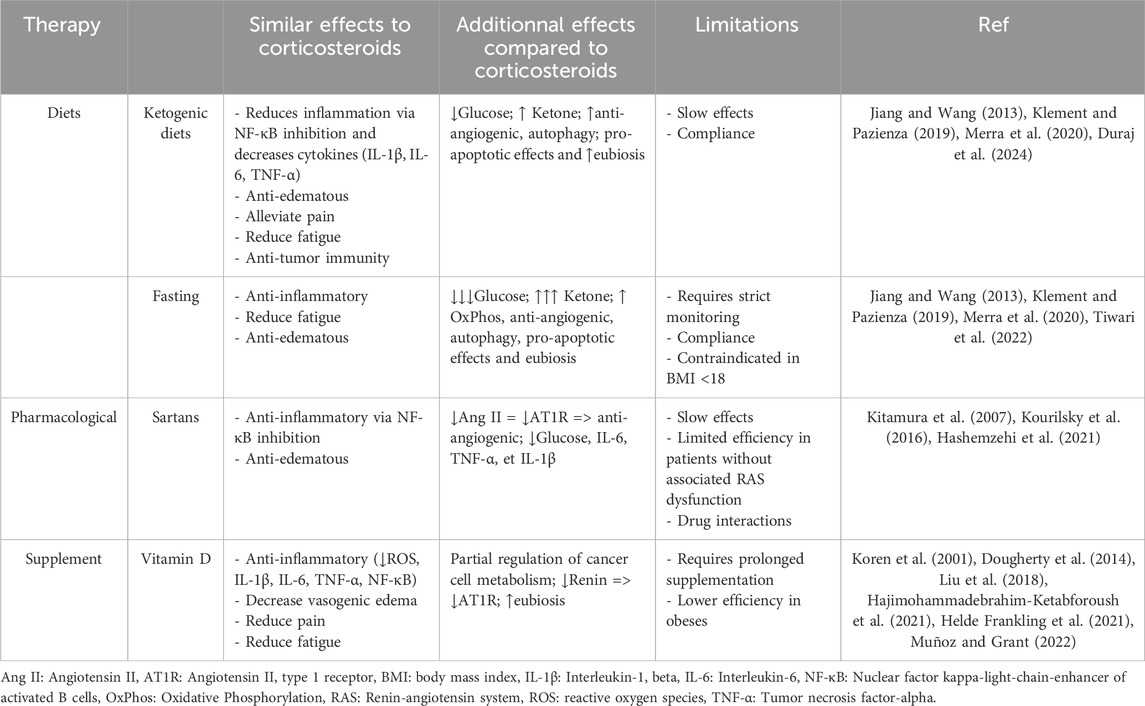
Table 1. Comparison of the positive effects, additional effects, and limitations of therapies compared to corticosteroid use.
4.1 Dietary strategies
Targeting both glucose and glutamine metabolism through dietary interventions and pharmacological agents while maintaining therapeutic ketosis represents a promising avenue. Various dietary strategies, such as the strict ketogenic diet (caloric restriction), the Paleolithic diet (Cruwys et al., 2020; Duraj et al., 2024), prolonged water fasting (3–7 days) (Phillips et al., 2022), and the fast-mimicking diet (Nencioni et al., 2018), have demonstrated benefits in reducing glycolysis pathway activity in cancer. For patients who cannot fast due to a BMI <18, modified fasting approaches provide viable alternatives. A 5-day cyclic fasting-mimicking diet, when combined with standard cancer therapies, has been demonstrated to enhance systemic metabolism and bolster antitumor immunity (Vernieri et al., 2022). Fasting also stimulates autophagy, which may protect normal cells while rendering cancer cells more vulnerable to treatment by modulating stress-related gene expression (p21, p16, and p53) (Erlangga et al., 2023). A meta-analysis in mice suggest that ketogenic diets alone can prolong survival and slow tumor progression compared to carbohydrate-rich diets (Klement et al., 2016). In clinical settings, a small observational study on glioblastoma patients found that adherence to a ketogenic diet for more than 6 months was associated with a significantly higher 3-year survival rate (66.7% vs. 8.3%, p = 0.0114), suggesting a potential impact on patient outcomes (Kiryttopoulos et al., 2025). These metabolic interventions exert anti-angiogenic, anti-edematous, anti-inflammatory, and pro-apoptotic effects (Jiang and Wang, 2013). Additionally, fasting, the Mediterranean diet, the ketogenic diet, and the Paleolithic diet contribute to restoring microbiota eubiosis (a balanced and healthy state of the microbiome), enhancing microbial diversity, and strengthening gut barrier function (Klement and Pazienza, 2019; Merra et al., 2020). Eubiosis contributes to reducing inflammation by promoting gut barrier homeostasis, decreasing angiogenesis, regulating epigenetic processes, and reducing metastasis (Devoy et al., 2022). In colorectal cancer models, the ketogenic diet induces shifts in the gut microbiota, increasing microbial production of stearic acid. This metabolite, along with others, exhibits pro-apoptotic effects, reducing tumor growth by inducing apoptosis in cancer cells. These benefits persist even after microbiome transplantation into germ-free mice, suggesting a causal role of the microbiota in mediating the diet’s anticancer effects (Tsenkova et al., 2025). Weight loss is also associated with the regulation of the microbiome (Koutoukidis et al., 2022). For these dietary approaches to be effective, achieving therapeutic levels of ketosis is critical. This can be monitored using the glucose/ketone index (GKI), with optimal therapeutic levels characterized by glycemia below 5 mM and ketonemia above 1–2 mM, maintaining GKI values below 2.0, ideally near 1.0 (Meidenbauer et al., 2015; Duraj et al., 2024). A combination diet-drug called Ketogenic Metabolic Therapy, may further enhance these effects. Mebendazole, for instance, inhibits glycolysis and glutaminolysis (specifically Glutaminase C for glutaminolysis) (Mukherjee et al., 2023), while 6-diazo-5-oxo-L-norleucine (DON), a pan-glutaminase inhibitor, exhibits potent antitumor activity (Olsen et al., 2015) by simultaneously inhibits glycolysis and glutaminolysis pathways (Leone et al., 2019). While acute lymphoblastic leukemia (ALL) remains one of the few cancers where CSs provide substantial therapeutic benefit, resistance mechanisms involving increased glycolytic activity (Olivas-Aguirre et al., 2021) further support the need for metabolic-targeting strategies in cancer therapy.
4.2 Sartans: RAS inhibitors
Another promising option involves the use of sartans, inhibitors of the RAS. Sartans can inhibit tumor growth and progression, invasion, and metastasis in many cell lines and animal model systems (see Table 2 of (Roth et al., 2019)). A recent study showed that the use of sartans improves 5-year overall survival in Nasopharyngeal carcinoma (Lin et al., 2021). A case report also demonstrated that irbesartan, led to a nearly complete radiological improvement and a significant reduction in Carcinoembryonic Antigen levels in a patient with colorectal cancer (Jones et al., 2016). Indeed, sartans can reduce vasogenic edema (Kourilsky et al., 2016), decrease blood glucose levels (Kitamura et al., 2007), and counteract pro-tumor effects linked to ACE, Ang II, and the overexpression of AT1R (Almutlaq et al., 2021). Sartans reduce inflammation by blocking the AT1R receptor, which decreases the production of pro-inflammatory cytokines such as IL-6, TNF-α, and IL-1β, (Hashemzehi et al., 2021), which are often involved in chronic inflammatory processes and the tumor microenvironment.
4.3 Vitamin D
Vitamin D emerges as another viable alternative. It inhibits renin, thereby lowering ACE levels, Ang II, and AT1R expression (Dougherty et al., 2014). Vitamin D also partially helps to restore normal cellular metabolism (Sheeley et al., 2022; Farahmand et al., 2023) and has been shown to reduce brain edema following brain tumor surgery (Hajimohammadebrahim-Ketabforoush et al., 2021). Vitamin D has powerful effects: it decreases ROS (reactive oxygen species), IL-1β (interleukin-1 beta), IL-6, IL-8, IL-17A, TNF-α (tumor necrosis factor-alpha), and NF-κB (nuclear factor kappa-light-chain-enhancer of activated B cells), while increasing MKPs (mitogen-activated protein kinase phosphatases), IL-4, and IL-10 (interleukin-4 and interleukin-10) (Koren et al., 2001; Liu et al., 2018; Muñoz and Grant, 2022). Vitamin D supplementation appears to primarily reduce cancer mortality, rather than significantly impacting overall cancer incidence (Keum et al., 2022). However, it is important to consider the limitations of vitamin D, especially in obesity-related cancers, as excess fat tissue can sequester it within the body’s fat stores (Wortsman et al., 2000).
Historically, CSs have played a central role in oncology, particularly in managing symptoms and complications associated with cancer treatments. However, an increasing number of studies highlight their potential deleterious effects on survival and tumor progression. Underlying mechanisms such as increased glucose and glutamine metabolism, disruption of the renin-angiotensin system, alterations in gut microbiota, and vitamin D underscore the complex and sometimes detrimental impacts of CS use in oncology. Several studies illustrate the need to limit the use of CSs. The study by Petrelli et al. (2020) recommends avoiding or restricting their use, particularly in patients with advanced solid tumors. In standard care for glioblastoma multiforme, it is recommended to minimize steroid use, as well as their dose and duration (Petrelli et al., 2021). Moreover, prolonged CS use in oncology, especially in cancer survivors, is not recommended in the absence of a clear clinical benefit, particularly for chronic pain relief (Paice et al., 2016). Clinical trials have also shown that prolonged CS use may be unnecessary or even harmful. In a randomized study on patients with brain metastases, Vecht et al. (1994) demonstrated that a reduced dose of dexamethasone (4 mg) was as effective as a higher dose (16 mg) while limiting side effects such as muscle weakness and Cushing’s syndrome. Furthermore, a shorter duration of CSs treatment does not increase the risk of recurrence or mortality (Gupta et al., 2022), while high-dose or prolonged use is associated with a significant reduction in PFS and OS (Terao et al., 2023). Additionally, CSs do not appear to provide a significant clinical benefit in managing cancer-related fatigue, as demonstrated in a systematic review (Sandford et al., 2023). Given these findings, CSs should be used in oncology only when unavoidable (e.g., emergencies or certain lymphoproliferative cancers), at the minimum effective dose, with regular reassessment and gradual tapering whenever possible (Ryken et al., 2010).
In light of these observations, further research is imperative to explore alternatives to CSs and optimize therapeutic strategies. Promising approaches include vitamin D optimization, the use of sartans, and dietary strategies—such as Ketogenic Diets—which could, in some cases, replace CSs in combination with other treatments. The future of cancer treatment relies on a balanced, evidence-based approach to maximize therapeutic benefits while minimizing the risks associated with CS use.
Data availability statement
The original contributions presented in the study are included in the article/supplementary material, further inquiries can be directed to the corresponding authors.
Author contributions
PM: Writing–original draft, Writing–review and editing. J-MS: Writing–original draft, Writing–review and editing.
Funding
The author(s) declare that no financial support was received for the research, authorship, and/or publication of this article.
Conflict of interest
The authors declare that the research was conducted in the absence of any commercial or financial relationships that could be construed as a potential conflict of interest.
The author(s) declared that they were an editorial board member of Frontiers, at the time of submission. This had no impact on the peer review process and the final decision.
Publisher’s note
All claims expressed in this article are solely those of the authors and do not necessarily represent those of their affiliated organizations, or those of the publisher, the editors and the reviewers. Any product that may be evaluated in this article, or claim that may be made by its manufacturer, is not guaranteed or endorsed by the publisher.
References
Almutlaq, M., Alamro, A. A., Alamri, H. S., Alghamdi, A. A., and Barhoumi, T. (2021). The effect of local renin angiotensin system in the common types of cancer. Front. Endocrinol. (Lausanne) 12, 736361. doi:10.3389/fendo.2021.736361
Bikle, D. D. (2016). Extraskeletal actions of vitamin D. Ann. N. Y. Acad. Sci. 1376 (1), 29–52. doi:10.1111/nyas.13219
Bonello, F., Pulini, S., Ballanti, S., Gentile, M., Spada, S., Annibali, O., et al. (2019). Lenalidomide maintenance with or without prednisone in newly diagnosed myeloma patients: a pooled analysis. Cancers (Basel) 11 (11), 1735. doi:10.3390/cancers11111735
Bringhen, S., Offidani, M., Musto, P., Liberati, A. M., Benevolo, G., Cascavilla, N., et al. (2017). Long term outcome of lenalidomide-dexamethasone (rd) vs melphalan-lenalidomide-prednisone (MPR) vs cyclophosphamide-prednisone-lenalidomide (CPR) as induction followed by lenalidomide-prednisone (RP) vs lenalidomide (R) as maintenance in a community-based newly diagnosed myeloma population: updated analysis of EMN01 phase III study. Blood 130, 901. doi:10.1182/blood.v130.suppl_1.901.901
Canellos, G. P., Anderson, J. R., Propert, K. J., Nissen, N., Cooper, M. R., Henderson, E. S., et al. (1992). Chemotherapy of advanced Hodgkin's disease with MOPP, ABVD, or MOPP alternating with ABVD. N. Engl. J. Med. 327 (21), 1478–1484. doi:10.1056/NEJM199211193272102
Caplan, A., Fett, N., Rosenbach, M., Werth, V. P., and Micheletti, R. G. (2017). Prevention and management of glucocorticoid-induced side effects: a comprehensive review: a review of glucocorticoid pharmacology and bone health. J. Am. Acad. Dermatol 76 (1), 1–9. doi:10.1016/j.jaad.2016.01.062
Catarata, M. J., Ribeiro, R., Oliveira, M. J., Robalo Cordeiro, C., and Medeiros, R. (2020). Renin-angiotensin system in lung tumor and microenvironment interactions. Cancers (Basel) 12 (6), 1457. doi:10.3390/cancers12061457
Chan, M., Ghadieh, C., Irfan, I., Khair, E., Padilla, N., Rebeiro, S., et al. (2024). Exploring the influence of the microbiome on the pharmacology of anti-asthmatic drugs. Naunyn Schmiedeb. Arch. Pharmacol. 397 (2), 751–762. doi:10.1007/s00210-023-02681-5
Couch, C. E., Neal, W. T., Herron, C. L., Kent, M. L., Schreck, C. B., and Peterson, J. T. (2023). Gut microbiome composition associates with corticosteroid treatment, morbidity, and senescence in Chinook salmon (Oncorhynchus tshawytscha). Sci. Rep. 13 (1), 2567. doi:10.1038/s41598-023-29663-0
Cruwys, T., Norwood, R., Chachay, V. S., Ntontis, E., and Sheffield, J. (2020). An important part of who I am: the predictors of dietary adherence among weight-loss, vegetarian, vegan, paleo, and gluten-free dietary groups. Nutrients 12 (4), 970. doi:10.3390/nu12040970
Devoy, C., Flores Bueso, Y., and Tangney, M. (2022). Understanding and harnessing triple-negative breast cancer-related microbiota in oncology. Front. Oncol. 12, 1020121. doi:10.3389/fonc.2022.1020121
Dougherty, U., Mustafi, R., Sadiq, F., Almoghrabi, A., Mustafi, D., Kreisheh, M., et al. (2014). The renin-angiotensin system mediates EGF receptor-vitamin d receptor cross-talk in colitis-associated colon cancer. Clin. Cancer Res. 20 (22), 5848–5859. doi:10.1158/1078-0432.CCR-14-0209
Duggan, D. B., Petroni, G. R., Johnson, J. L., Glick, J. H., Fisher, R. I., Connors, J. M., et al. (2003). Randomized comparison of ABVD and MOPP/ABV hybrid for the treatment of advanced Hodgkin's disease: report of an intergroup trial. J. Clin. Oncol. 21 (4), 607–614. doi:10.1200/JCO.2003.12.086
Duraj, T., Kalamian, M., Zuccoli, G., Maroon, J. C., D'Agostino, D. P., Scheck, A. C., et al. (2024). Clinical research framework proposal for ketogenic metabolic therapy in glioblastoma. BMC Med. 22 (1), 578. doi:10.1186/s12916-024-03775-4
Erlangga, Z., Ghashang, S. K., Hamdan, I., Melk, A., Gutenbrunner, C., and Nugraha, B. (2023). The effect of prolonged intermittent fasting on autophagy, inflammasome and senescence genes expressions: an exploratory study in healthy young males. Hum. Nutr. and Metabolism 32, 200189. doi:10.1016/j.hnm.2023.200189
Faggiano, A., Mazzilli, R., Natalicchio, A., Adinolfi, V., Argentiero, A., Danesi, R., et al. (2022). Corticosteroids in oncology: use, overuse, indications, contraindications. An Italian association of medical oncology (AIOM)/Italian association of medical diabetologists (AMD)/Italian society of endocrinology (SIE)/Italian society of pharmacology (SIF) multidisciplinary consensus position paper. Crit. Rev. Oncology/Hematology 180, 103826. doi:10.1016/j.critrevonc.2022.103826
Farahmand, M. A., Daneshzad, E., Fung, T. T., Zahidi, F., Muhammadi, M., Bellissimo, N., et al. (2023). What is the impact of vitamin D supplementation on glycemic control in people with type-2 diabetes: a systematic review and meta-analysis of randomized controlled trails. BMC Endocr. Disord. 23 (1), 15. doi:10.1186/s12902-022-01209-x
Fishel, R. S., Eisenberg, S., Shai, S. Y., Redden, R. A., Bernstein, K. E., and Berk, B. C. (1995). Glucocorticoids induce angiotensin-converting enzyme expression in vascular smooth muscle. Hypertension 25 (3), 343–349. doi:10.1161/01.hyp.25.3.343
Goodman, R. S., Johnson, D. B., and Balko, J. M. (2023). Corticosteroids and cancer immunotherapy. Clin. Cancer Res. 29 (14), 2580–2587. doi:10.1158/1078-0432.CCR-22-3181
Greene, N. H., Pedersen, L. H., Liu, S., and Olsen, J. (2013). Prenatal prescription corticosteroids and offspring diabetes: a national cohort study. Int. J. Epidemiol. 42 (1), 186–193. doi:10.1093/ije/dys228
Gregory, W. M., Richards, M. A., and Malpas, J. S. (1992). Combination chemotherapy versus melphalan and prednisolone in the treatment of multiple myeloma: an overview of published trials. J. Clin. Oncol. 10 (2), 334–342. doi:10.1200/JCO.1992.10.2.334
Gupta, S., Garcia-Carro, C., Prosek, J. M., Glezerman, I., Herrmann, S. M., Garcia, P., et al. (2022). Shorter versus longer corticosteroid duration and recurrent immune checkpoint inhibitor-associated AKI. J. Immunother. Cancer 10 (9), e005646. doi:10.1136/jitc-2022-005646
Hajimohammadebrahim-Ketabforoush, M., Shahmohammadi, M., Vahdat Shariatpanahi, Z., and Zali, A. (2021). Preoperative serum level of vitamin D is a possible protective factor for peritumoral brain edema of meningioma: a cross sectional study. Nutr. Cancer 73 (11-12), 2842–2848. doi:10.1080/01635581.2020.1861311
Hashemzehi, M., Rahmani, F., Khoshakhlagh, M., Avan, A., Asgharzadeh, F., Barneh, F., et al. (2021). Angiotensin receptor blocker Losartan inhibits tumor growth of colorectal cancer. Excli J. 20, 506–521. doi:10.17179/excli2020-3083
Helde Frankling, M., Klasson, C., Sandberg, C., Nordström, M., Warnqvist, A., Bergqvist, J., et al. (2021). Palliative-D'-Vitamin D supplementation to palliative cancer patients: a double blind, randomized placebo-controlled multicenter trial. Cancers (Basel) 13 (15), 3707. doi:10.3390/cancers13153707
Hwangbo, Y., and Lee, E. K. (2017). Acute hyperglycemia associated with anti-cancer medication. Endocrinol. Metab. Seoul. 32 (1), 23–29. doi:10.3803/EnM.2017.32.1.23
Jeong, Y., Han, H. S., Lee, H. D., Yang, J., Jeong, J., Choi, M. K., et al. (2016). A pilot study evaluating steroid-induced diabetes after antiemetic dexamethasone therapy in chemotherapy-treated cancer patients. Cancer Res. Treat. 48 (4), 1429–1437. doi:10.4143/crt.2015.464
Jiang, Y. S., and Wang, F. R. (2013). Caloric restriction reduces edema and prolongs survival in a mouse glioma model. J. Neurooncol 114 (1), 25–32. doi:10.1007/s11060-013-1154-y
Jones, M. R., Schrader, K. A., Shen, Y., Pleasance, E., Ch'ng, C., Dar, N., et al. (2016). Response to angiotensin blockade with irbesartan in a patient with metastatic colorectal cancer. Ann. Oncol. 27 (5), 801–806. doi:10.1093/annonc/mdw060
Kazazoglou, T., Panagiotou, C., Mihailidou, C., Kokkinopoulou, I., Papadopoulou, A., and Moutsatsou, P. (2021). Glutamine synthetase regulation by dexamethasone, RU486, and compound A in astrocytes derived from aged mouse cerebral hemispheres is mediated via glucocorticoid receptor. Mol. Cell Biochem. 476 (12), 4471–4485. doi:10.1007/s11010-021-04236-9
Keum, N., Chen, Q. Y., Lee, D. H., Manson, J. E., and Giovannucci, E. (2022). Vitamin D supplementation and total cancer incidence and mortality by daily vs. infrequent large-bolus dosing strategies: a meta-analysis of randomised controlled trials. Br. J. Cancer 127 (5), 872–878. doi:10.1038/s41416-022-01850-2
Kiryttopoulos, A., Evangeliou, A. E., Katsanika, I., Boukovinas, I., Foroglou, N., Zountsas, B., et al. (2025). Successful application of dietary ketogenic metabolic therapy in patients with glioblastoma: a clinical study. Front. Nutr. 11, 1489812. doi:10.3389/fnut.2024.1489812
Kitamura, N., Takahashi, Y., Yamadate, S., and Asai, S. (2007). Angiotensin II receptor blockers decreased blood glucose levels: a longitudinal survey using data from electronic medical records. Cardiovasc Diabetol. 6, 26. doi:10.1186/1475-2840-6-26
Klement, R. J., Champ, C. E., Otto, C., and Kämmerer, U. (2016). Anti-tumor effects of ketogenic diets in mice: a meta-analysis. PLoS One 11 (5), e0155050. doi:10.1371/journal.pone.0155050
Klement, R. J., and Pazienza, V. (2019). Impact of different types of diet on gut microbiota profiles and cancer prevention and treatment. Med. Kaunas. 55 (4), 84. doi:10.3390/medicina55040084
Koren, R., Hadari-Naor, I., Zuck, E., Rotem, C., Liberman, U. A., and Ravid, A. (2001). Vitamin D is a prooxidant in breast cancer cells. Cancer Res. 61 (4), 1439–1444.
Kourilsky, A., Bertrand, G., Ursu, R., Doridam, J., Barlog, C., Faillot, T., et al. (2016). Impact of Angiotensin-II receptor blockers on vasogenic edema in glioblastoma patients. J. Neurol. 263 (3), 524–530. doi:10.1007/s00415-015-8016-9
Koutoukidis, D. A., Jebb, S. A., Zimmerman, M., Otunla, A., Henry, J. A., Ferrey, A., et al. (2022). The association of weight loss with changes in the gut microbiota diversity, composition, and intestinal permeability: a systematic review and meta-analysis. Gut Microbes 14 (1), 2020068. doi:10.1080/19490976.2021.2020068
Kulkarni, S., Durham, H., Glover, L., Ather, O., Phillips, V., Nemes, S., et al. (2022). Metabolic adverse events associated with systemic corticosteroid therapy-a systematic review and meta-analysis. BMJ Open 12 (12), e061476. doi:10.1136/bmjopen-2022-061476
Lee, D. C., Ta, L., Mukherjee, P., Duraj, T., Domin, M., Greenwood, B., et al. (2024). Amino acid and glucose fermentation maintain ATP content in mouse and human malignant glioma cells. ASN Neuro 16 (1), 2422268. doi:10.1080/17590914.2024.2422268
Leone, R. D., Zhao, L., Englert, J. M., Sun, I. M., Oh, M. H., Sun, I. H., et al. (2019). Glutamine blockade induces divergent metabolic programs to overcome tumor immune evasion. Science 366 (6468), 1013–1021. doi:10.1126/science.aav2588
Li, S. H., Lu, H. I., Chang, A. Y., Huang, W. T., Lin, W. C., Lee, C. C., et al. (2016). Angiotensin II type I receptor (AT1R) is an independent prognosticator of esophageal squamous cell carcinoma and promotes cells proliferation via mTOR activation. Oncotarget 7 (41), 67150–67165. doi:10.18632/oncotarget.11567
Limbachia, V., Nunney, I., Page, D. J., Barton, H. A., Patel, L. K., Thomason, G. N., et al. (2024). The effect of different types of oral or intravenous corticosteroids on capillary blood glucose levels in hospitalized inpatients with and without diabetes. Clin. Ther. 46 (2), e59–e63. doi:10.1016/j.clinthera.2023.11.013
Lin, Y. T., Wang, H. C., Tsai, M. H., Su, Y. Y., Yang, M. Y., and Chien, C. Y. (2021). Angiotensin II receptor blockers valsartan and losartan improve survival rate clinically and suppress tumor growth via apoptosis related to PI3K/AKT signaling in nasopharyngeal carcinoma. Cancer 127 (10), 1606–1619. doi:10.1002/cncr.33391
Liu, H., Liu, Z., Jiang, B., Ding, X., Huo, L., Wan, X., et al. (2016). Prognostic significance of hyperglycemia in patients with brain tumors: a meta-analysis. Mol. Neurobiol. 53 (3), 1654–1660. doi:10.1007/s12035-015-9115-4
Liu, W., Zhang, L., Xu, H. J., Li, Y., Hu, C. M., Yang, J. Y., et al. (2018). The anti-inflammatory effects of vitamin D in tumorigenesis. Int. J. Mol. Sci. 19 (9), 2736. doi:10.3390/ijms19092736
Lu, X., Chen, B., Xu, D., Hu, W., Wang, X., Dai, Y., et al. (2024). Epigenetic programming mediates abnormal gut microbiota and disease susceptibility in offspring with prenatal dexamethasone exposure. Cell Rep. Med. 5 (2), 101398. doi:10.1016/j.xcrm.2024.101398
Martinez, P., and Sabatier, J.-M. (2025). Malignant tumors in vagal-innervated organs: exploring its homeostatic role. Cancer Lett. 617, 217539. doi:10.1016/j.canlet.2025.217539
Mathur, R., Amichai, M., Chua, K. S., Mirocha, J., Barlow, G. M., and Pimentel, M. (2013). Methane and hydrogen positivity on breath test is associated with greater body mass index and body fat. J. Clin. Endocrinol. Metab. 98 (4), E698–E702. doi:10.1210/jc.2012-3144
Maude, S. L., Frey, N., Shaw, P. A., Aplenc, R., Barrett, D. M., Bunin, N. J., et al. (2014). Chimeric antigen receptor T cells for sustained remissions in leukemia. N. Engl. J. Med. 371 (16), 1507–1517. doi:10.1056/NEJMoa1407222
Meidenbauer, J. J., Mukherjee, P., and Seyfried, T. N. (2015). The glucose ketone index calculator: a simple tool to monitor therapeutic efficacy for metabolic management of brain cancer. Nutr. Metab. (Lond) 12, 12. doi:10.1186/s12986-015-0009-2
Merra, G., Noce, A., Marrone, G., Cintoni, M., Tarsitano, M. G., Capacci, A., et al. (2020). Influence of mediterranean diet on human gut microbiota. Nutrients 13 (1), 7. doi:10.3390/nu13010007
Mukherjee, P., Greenwood, B., Aristizabal-Henao, J., and Kiebish, M. (2023). Ketogenic diet as a metabolic vehicle for enhancing the therapeutic efficacy of mebendazole and devimistat in preclinical pediatric glioma.
Muñoz, A., and Grant, W. B. (2022). Vitamin D and cancer: an historical overview of the epidemiology and mechanisms. Nutrients 14 (7), 1448. doi:10.3390/nu14071448
Neelapu, S. S., Locke, F. L., Bartlett, N. L., Lekakis, L. J., Miklos, D. B., Jacobson, C. A., et al. (2017). Axicabtagene ciloleucel CAR T-cell therapy in refractory large B-cell lymphoma. N. Engl. J. Med. 377 (26), 2531–2544. doi:10.1056/NEJMoa1707447
Nencioni, A., Caffa, I., Cortellino, S., and Longo, V. D. (2018). Fasting and cancer: molecular mechanisms and clinical application. Nat. Rev. Cancer 18 (11), 707–719. doi:10.1038/s41568-018-0061-0
Ng, A. K., Li, S., Neuberg, D., Silver, B., Stevenson, M. A., Fisher, D. C., et al. (2004). Comparison of MOPP versus ABVD as salvage therapy in patients who relapse after radiation therapy alone for Hodgkin's disease. Ann. Oncol. 15 (2), 270–275. doi:10.1093/annonc/mdh067
Olivas-Aguirre, M., Torres-López, L., Pottosin, I., and Dobrovinskaya, O. (2021). Overcoming glucocorticoid resistance in acute lymphoblastic leukemia: repurposed drugs can improve the protocol. Front. Oncol. 11, 617937. doi:10.3389/fonc.2021.617937
Olsen, R. R., Mary-Sinclair, M. N., Yin, Z., and Freeman, K. W. (2015). Antagonizing Bcl-2 family members sensitizes neuroblastoma and Ewing's sarcoma to an inhibitor of glutamine metabolism. PLoS One 10 (1), e0116998. doi:10.1371/journal.pone.0116998
Oray, M., Abu Samra, K., Ebrahimiadib, N., Meese, H., and Foster, C. S. (2016). Long-term side effects of glucocorticoids. Expert Opin. Drug Saf. 15 (4), 457–465. doi:10.1517/14740338.2016.1140743
Paice, J. A., Portenoy, R., Lacchetti, C., Campbell, T., Cheville, A., Citron, M., et al. (2016). Management of chronic pain in survivors of adult cancers: American society of clinical oncology clinical practice guideline. J. Clin. Oncol. 34 (27), 3325–3345. doi:10.1200/JCO.2016.68.5206
Pearson, O. H., and Eliel, L. P. (1950). Use of pituitary adrenocorticotropic hormone (ACTH) and cortisone in lymphomas and leukemias. J. Am. Med. Assoc. 144 (16), 1349–1353. doi:10.1001/jama.1950.02920160023005
Petrelli, F., Bukovec, R., Perego, G., Luisa, R., Luciani, A., Zaniboni, A., et al. (2020). Association of steroid use with survival in solid tumours. Eur. J. Cancer 141, 105–114. doi:10.1016/j.ejca.2020.09.020
Petrelli, F., De Stefani, A., Ghidini, A., Bruschieri, L., Riboldi, V., Dottorini, L., et al. (2021). Steroids use and survival in patients with glioblastoma multiforme: a pooled analysis. J. Neurol. 268 (2), 440–447. doi:10.1007/s00415-020-09731-5
Petrelli, F., Signorelli, D., Ghidini, M., Ghidini, A., Pizzutilo, E. G., Ruggieri, L., et al. (2020). Association of steroids use with survival in patients treated with immune checkpoint inhibitors: a systematic review and meta-analysis. Cancers (Basel) 12 (3), 546. doi:10.3390/cancers12030546
Phillips, M. C. L., Leyden, J., McManus, E. J., Lowyim, D. G., Ziad, F., Moon, B. G., et al. (2022). Feasibility and safety of a combined metabolic strategy in glioblastoma multiforme: a prospective case series. J. Oncol. 2022, 4496734. doi:10.1155/2022/4496734
Pimenta, E., Wolley, M., and Stowasser, M. (2012). Adverse cardiovascular outcomes of corticosteroid excess. Endocrinology 153 (11), 5137–5142. doi:10.1210/en.2012-1573
Pitter, K. L., Tamagno, I., Alikhanyan, K., Hosni-Ahmed, A., Pattwell, S. S., Donnola, S., et al. (2016). Corticosteroids compromise survival in glioblastoma. Brain 139 (Pt 5), 1458–1471. doi:10.1093/brain/aww046
Pourhassan, H., Murphy, L., and Aldoss, I. (2024). Glucocorticoid therapy in acute lymphoblastic leukemia: navigating short-term and long-term effects and optimal regimen selection. Curr. Hematol. Malig. Rep. 19 (4), 175–185. doi:10.1007/s11899-024-00735-w
Roth, I. M., Wickremesekera, A. C., Wickremesekera, S. K., Davis, P. F., and Tan, S. T. (2019). Therapeutic targeting of cancer stem cells via modulation of the renin-angiotensin system. Front. Oncol. 9, 745. doi:10.3389/fonc.2019.00745
Rummel, M. J., Niederle, N., Maschmeyer, G., Banat, G. A., von Grünhagen, U., Losem, C., et al. (2013). Bendamustine plus rituximab versus CHOP plus rituximab as first-line treatment for patients with indolent and mantle-cell lymphomas: an open-label, multicentre, randomised, phase 3 non-inferiority trial. Lancet 381 (9873), 1203–1210. doi:10.1016/S0140-6736(12)61763-2
Ryken, T. C., McDermott, M., Robinson, P. D., Ammirati, M., Andrews, D. W., Asher, A. L., et al. (2010). The role of steroids in the management of brain metastases: a systematic review and evidence-based clinical practice guideline. J. Neurooncol 96 (1), 103–114. doi:10.1007/s11060-009-0057-4
Sandford, A., Haywood, A., Rickett, K., Good, P., Khan, S., Foster, K., et al. (2023). Corticosteroids for the management of cancer-related fatigue in adults with advanced cancer. Cochrane Database Syst. Rev. 1 (1), Cd013782. doi:10.1002/14651858.CD013782.pub2
Sarantos, P., Abouhamze, A., and Souba, W. W. (1992). Glucocorticoids regulate intestinal glutaminase expression. Surgery 112 (2), 278–283.
Schrappe, M., Zimmermann, M., Möricke, A., Mann, G., Valsecchi, M. G., Bartram, C. R., et al. (2008). Dexamethasone in induction can eliminate one third of all relapses in childhood acute lymphoblastic leukemia (ALL): results of an international randomized trial in 3655 patients (trial AIEOP-BFM ALL 2000). Blood 112 (11), 7. doi:10.1182/blood.v112.11.7.7
Sermer, D., Batlevi, C., Palomba, M. L., Shah, G., Lin, R. J., Perales, M. A., et al. (2020). Outcomes in patients with DLBCL treated with commercial CAR T cells compared with alternate therapies. Blood Adv. 4 (19), 4669–4678. doi:10.1182/bloodadvances.2020002118
Sevcikova, A., Mladosievicova, B., Mego, M., and Ciernikova, S. (2023). Exploring the role of the gut and intratumoral microbiomes in tumor progression and metastasis. Int. J. Mol. Sci. 24 (24), 17199. doi:10.3390/ijms242417199
Seyfried, T. N., Arismendi-Morillo, G., Mukherjee, P., and Chinopoulos, C. (2020). On the origin of ATP synthesis in cancer. iScience 23 (11), 101761. doi:10.1016/j.isci.2020.101761
Sheeley, M. P., Andolino, C., Kiesel, V. A., and Teegarden, D. (2022). Vitamin D regulation of energy metabolism in cancer. Br. J. Pharmacol. 179 (12), 2890–2905. doi:10.1111/bph.15424
Shelat, S. G., Flanagan-Cato, L. M., and Fluharty, S. J. (1999). Glucocorticoid and mineralocorticoid regulation of angiotensin II type 1 receptor binding and inositol triphosphate formation in WB cells. J. Endocrinol. 162 (3), 381–391. doi:10.1677/joe.0.1620381
Skversky, A. L., Kumar, J., Abramowitz, M. K., Kaskel, F. J., and Melamed, M. L. (2011). Association of glucocorticoid use and low 25-hydroxyvitamin D levels: results from the national health and nutrition examination survey (NHANES): 2001-2006. J. Clin. Endocrinol. Metab. 96 (12), 3838–3845. doi:10.1210/jc.2011-1600
Spakowicz, D., Hoyd, R., Muniak, M., Husain, M., Bassett, J. S., Wang, L., et al. (2020). Inferring the role of the microbiome on survival in patients treated with immune checkpoint inhibitors: causal modeling, timing, and classes of concomitant medications. BMC Cancer 20 (1), 383. doi:10.1186/s12885-020-06882-6
Strati, P., Ahmed, S., Furqan, F., Fayad, L. E., Lee, H. J., Iyer, S. P., et al. (2021). Prognostic impact of corticosteroids on efficacy of chimeric antigen receptor T-cell therapy in large B-cell lymphoma. Blood 137 (23), 3272–3276. doi:10.1182/blood.2020008865
Suh, S., and Park, M. K. (2017). Glucocorticoid-induced diabetes mellitus: an important but overlooked problem. Endocrinol. Metab. Seoul. 32 (2), 180–189. doi:10.3803/EnM.2017.32.2.180
Terao, T., Kitamura, W., Fujii, N., Asada, N., Kamoi, C., Fujiwara, K., et al. (2023). Negative prognostic impact of high-dose or long-term corticosteroid use in patients with relapsed or refractory B-cell lymphoma who received tisagenlecleucel. Transpl. Cell Ther. 29 (9), 573.e1–573.e8. doi:10.1016/j.jtct.2023.06.018
Thibault, R., Welch, S., Mauras, N., Sager, B., Altomare, A., Haymond, M., et al. (2008). Corticosteroids increase glutamine utilization in human splanchnic bed. Am. J. Physiol. Gastrointest. Liver Physiol. 294 (2), G548–G553. doi:10.1152/ajpgi.00461.2007
Tiwari, S., Sapkota, N., and Han, Z. (2022). Effect of fasting on cancer: a narrative review of scientific evidence. Cancer Sci. 113 (10), 3291–3302. doi:10.1111/cas.15492
Tsenkova, M., Brauer, M., Pozdeev, V. I., Kasakin, M., Busi, S. B., Schmoetten, M., et al. (2025). Ketogenic diet suppresses colorectal cancer through the gut microbiome long chain fatty acid stearate. Nat. Commun. 16 (1), 1792. doi:10.1038/s41467-025-56678-0
Vecht, C. J., Hovestadt, A., Verbiest, H. B., van Vliet, J. J., and van Putten, W. L. (1994). Dose-effect relationship of dexamethasone on Karnofsky performance in metastatic brain tumors: a randomized study of doses of 4, 8, and 16 mg per day. Neurology 44 (4), 675–680. doi:10.1212/wnl.44.4.675
Vernieri, C., Fucà, G., Ligorio, F., Huber, V., Vingiani, A., Iannelli, F., et al. (2022). Fasting-mimicking diet is safe and reshapes metabolism and antitumor immunity in patients with cancer. Cancer Discov. 12 (1), 90–107. doi:10.1158/2159-8290.CD-21-0030
Vich Vila, A., Collij, V., Sanna, S., Sinha, T., Imhann, F., Bourgonje, A. R., et al. (2020). Impact of commonly used drugs on the composition and metabolic function of the gut microbiota. Nat. Commun. 11 (1), 362. doi:10.1038/s41467-019-14177-z
Wang, Y., Yang, M., Tao, M., Liu, P., Kong, C., Li, H., et al. (2021). Corticosteroid administration for cancer-related indications is an unfavorable prognostic factor in solid cancer patients receiving immune checkpoint inhibitor treatment. Int. Immunopharmacol. 99, 108031. doi:10.1016/j.intimp.2021.108031
Warburg, O. (1956). On the origin of cancer cells. Science 123 (3191), 309–314. doi:10.1126/science.123.3191.309
Wei, L., MacDonald, T. M., and Walker, B. R. (2004). Taking glucocorticoids by prescription is associated with subsequent cardiovascular disease. Ann. Intern Med. 141 (10), 764–770. doi:10.7326/0003-4819-141-10-200411160-00007
Keywords: corticosteroids, glucocorticoids, glycolysis, glutaminolysis, renin-angiotensin system, vitamin D, microbiome
Citation: Martinez P and Sabatier J-M (2025) Rethinking corticosteroids use in oncology. Front. Pharmacol. 16:1551111. doi: 10.3389/fphar.2025.1551111
Received: 24 December 2024; Accepted: 07 March 2025;
Published: 26 March 2025.
Edited by:
Heike Wulff, University of California, Davis, United StatesReviewed by:
David Diaz-Jimenez, National Institute of Environmental Health Sciences (NIH), United StatesCopyright © 2025 Martinez and Sabatier. This is an open-access article distributed under the terms of the Creative Commons Attribution License (CC BY). The use, distribution or reproduction in other forums is permitted, provided the original author(s) and the copyright owner(s) are credited and that the original publication in this journal is cited, in accordance with accepted academic practice. No use, distribution or reproduction is permitted which does not comply with these terms.
*Correspondence: Jean-Marc Sabatier, c2FiYXRpZXIuam0xQGdtYWlsLmNvbQ==