- 1Hunan Provincial Key Laboratory of Traditional Chinese Medicine Diagnostics, Hunan University of Chinese Medicine, Changsha, Hunan, China
- 2School of Traditional Chinese Medicine, Hunan University of Chinese Medicine, Changsha, Hunan, China
- 3Hunan Provincial Key Laboratory for Prevention and Treatment of Ophthalmology and Otolaryngology Diseases with Chinese Medicine, Hunan University of Chinese Medicine, Changsha, Hunan, China
- 4Department of Ophthalmology, The First Affiliated Hospital of Hunan University of Traditional Chinese Medicine, Changsha, Hunan, China
- 5School of Medicine, Hunan University of Chinese Medicine, Changsha, Hunan, China
Retinal cell apoptosis is the primary pathological process in many retinal diseases, including retinitis pigmentosa and age-related macular degeneration, which can cause severe visual impairment and blindness. Lycium barbarum L., a traditional Chinese medicinal botanical drug, has a long history and extensive application in ophthalmic disease prevention and treatment. This study systematically reviewed the key active metabolites in L. barbarum L., including L. barbarum polysaccharides, carotenoids, and flavonoids, that exert retinal protective effects. A comprehensive analysis of the pharmacological effects and underlying molecular mechanisms of L. barbarum L. and its active metabolites in the prevention and treatment of retinal cell apoptosis, including essential aspects such as antioxidant activity, anti-inflammatory properties, autophagy regulation, and mitochondrial function preservation, is essential to establish a comprehensive and solid theoretical basis for further investigation of the medicinal value of L. barbarum L. in ophthalmology and provide a reference for future research directions.
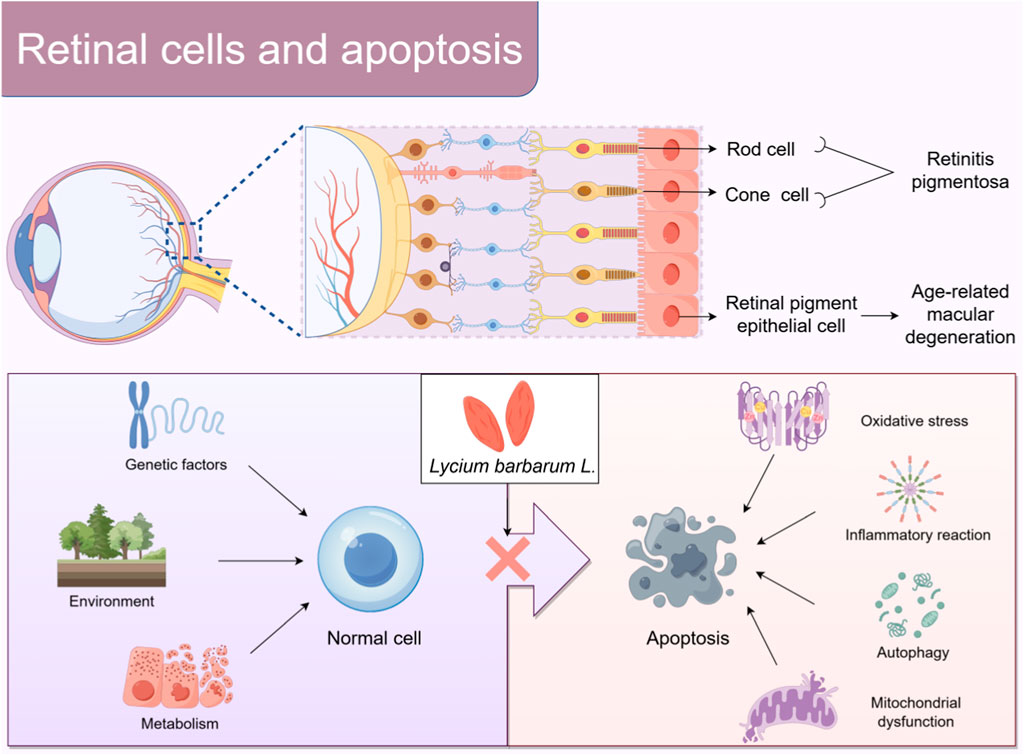
GRAPHICAL ABSTRACT | Under the influence of genetic, environmental, and metabolic factors, normal cells may undergo apoptosis, which may be related to oxidative stress, inflammatory response, autophagy dysfunction, and mitochondrial dysfunction. The proposed mechanism suggests that Lycium barbarum L. is likely to prevent retinal cell apoptosis By Figdraw.
1 Introduction
The photoreceptor cells in the retina, particularly the rod cells and cone cells, are essential components in visual information transmission (Chai et al., 2024). They are responsible for capturing light and converting it into neural signals, which establishes visual perception (Peshenko et al., 2024). Retinal pigment epithelial (RPE) cells function as the “logistical support system” of photoreceptor cells. The RPE absorbs essential nutrients, including glucose, amino acids, and vitamins, from the choroidal capillaries and subsequently transports them to photoreceptor cells to meet their metabolic demands and sustain their normal functions and activities (Li B. et al., 2021). Photoreceptor cells produce substantial metabolic waste, including shed outer disc membrane fragments during the metabolic process. RPE cells phagocytize and degrade these metabolic wastes, maintaining the cleanliness of the retinal microenvironment and preventing metabolic waste accumulation from interfering with retinal function (Zhu et al., 2020). Under physiological conditions, apoptosis influences retinal cell renewal and the clearance of abnormal cells, thereby regulating the maintenance of homeostasis (Yi et al., 2020). Genetic, environmental, metabolic, and other factors can disrupt retinal equilibrium, leading to excessive apoptosis of retinal cells and impairing visual function (Ou et al., 2022). The pathological mechanisms involved in this process are complex, including oxidative stress (Ren et al., 2022), the release of inflammatory mediators (Bishayee et al., 2024), abnormal cellular autophagy (Wang X. et al., 2024), and mitochondrial dysfunction (Wang C. et al., 2024). These mechanisms interact to collectively facilitate retinal cell apoptosis.
Retinal cell apoptosis is the core pathological process in the progression of various retinal degenerative diseases and the main cause of visual impairment and blindness, severely threatening patients’ visual function and quality of life (Chang et al., 2021). Retinitis pigmentosa (RP) (Qiu et al., 2025) and age-related macular degeneration (AMD) (Hu et al., 2024) are representative retinal diseases characterized by retinal cell apoptosis. Their high incidence rate and increasing number of patients have brought a major burden to global health (Fitton et al., 2025; Mosallaei et al., 2025). The strategy for treating retinal cell apoptosis aims to mitigate or prevent this process to protect and restore visual function. While treatment modalities, including antioxidant therapy (Liu Y.chen et al., 2024), gene therapy (Staurenghi et al., 2022), and stem cell transplantation (Maeda et al., 2022), exist, their efficacy and safety require additional validation and optimization (Singh et al., 2020). Therefore, developing new, efficient, and safe prevention and control strategies is imperative.
Goji berries from traditional Chinese medicine (TCM) have attracted much attention for their potential retinal protective effects. The goji berry, Lycium barbarum L., is a perennial shrub in the Solanaceae family. Approximately 80 species of L. barbarum L exist globally, with a predominant concentration on the American continent (Yao et al., 2021). In China, L. barbarum L. has a recorded history exceeding 3,700 years and a cultivation history of >600 years (Yu et al., 2023). Lycium Chinense Mill is a representative variety among them, known for its excellent quality (Yang et al., 2022). Lycium barbarum L., a nutrient-rich edible medicinal plant, is essential in TCM and has a history of >2000 years as a TCM and food supplement (Yun et al., 2022). It possesses properties that nourish the liver and kidneys, replenish essence, and improve eyesight (Ou et al., 2025). Modern scientific research has clarified the potential of L. barbarum L. in protecting the retina and impeding cell apoptosis (Liu et al., 2022). The active metabolites of L. barbarum L. can effectively mitigate retinal damage and improve visual function through various mechanisms, including antioxidant, anti-inflammatory, anti-apoptotic properties, and the promotion of nerve regeneration (Jiang et al., 2024). Lycium barbarum L. is rich in various bioactive metabolites, including L. barbarum polysaccharides (LBP), carotenoids, flavonoids, vitamins (including vitamins A, C, and E), and mineral elements, collectively contributing to its unique nutritional and medicinal value system (Lee and Choi, 2023). Among these metabolites, LBP, carotenoids, and flavonoids are not only abundant, but also play a significant role in the medicinal value of L. barbarum L.
Although the potential of L. barbarum L. in protecting retinal cells has been confirmed, investigations into its specific mechanism of action are still relatively scarce. Therefore, this study aims to systematically clarify the pharmacological mechanisms of L. barbarum L. and its active metabolites, including LBP, carotenoids, and flavonoids, in preventing and treating retinal cell apoptosis, thereby providing a comprehensive theoretical basis for enhancing their medicinal value in ophthalmology.
2 Methodology
In order to explore the potential efficacy and mechanism of L. barbarum L. as a botanical drug for preventing and treating retinal cell apoptosis, we mainly searched relevant articles in databases such as PubMed, Web of Science, and Google Scholar. The search strategy included nine keywords: “L. barbarum L.”, “Lycium Chinense Mill”, “goji berry”, “Gouqizi”, “L. barbarum polysaccharides”, “carotenoids”, “flavonoids”, “traditional Chinese medicine”, and “mechanism”. Two independent reviewers evaluated each article based on predefined inclusion and exclusion criteria. The literature search period was from January 2010 to January 2025. The inclusion criteria were: (1) Articles written in English; (2) Articles published in peer-reviewed journals; (3) Articles that studied the relevant mechanisms and clinical applications of L. barbarum L. and its active metabolites in preventing and treating retinal cell apoptosis. The exclusion criteria were: (1) Non-English articles; (2) Non-peer-reviewed articles, such as conference abstracts, editorials, and non-academic reports; (3) Articles that had nothing to do with L. barbarum L. and its active metabolites; (4) Duplicate articles.
3 The main active metabolites of Lycium barbarum L.
3.1 LBP
LBP, as a distinctive bioactive metabolite in L. barbarum L., has a complex chemical structure comprising various monosaccharides, including mannose, xylose, galacturonic acid, glucose, galactose, and arabinose (Yin et al., 2024). These sugar chain structures endow LBP with unique biological activities, including immune regulation, antioxidant properties, hypoglycemic effects, and lipid-lowering capabilities, which are essential to the pharmacological effects of L. barbarum L. (Liu et al., 2024b; Wu D. et al., 2024).
Regarding retinal protection, LBP exhibits significant inhibitory effects on retinal cell apoptosis through various mechanisms (Figure 1). A previous study has demonstrated that LBP can effectively prevent ROS generation in the retina of mice exposed to light, potentially through the upregulation of antioxidant genes NRF2 and TrxR1, attenuating mitochondrial responses to oxidative stress and enhancing antioxidant capacity (Tang et al., 2018). This regulatory effect weakens the mitochondrial response to oxidative stress, thereby enhancing antioxidant capacity and offering effective protection for photoreceptor cells against light-induced retinal damage. Furthermore, LBP may protect ARPE-19 cells from oxidative stress damage caused by hydrogen peroxide by activating the NRF2/heme oxygenase 1 (HO-1) signaling pathway, demonstrating significant efficacy in reducing oxidative damage and inhibiting cell apoptosis (Liang et al., 2021). The strong antioxidant capacity of LBP can eliminate excessive ROS and free radicals generated in retinal cells, thereby mitigating oxidative stress damage and protecting retinal cells from apoptosis.
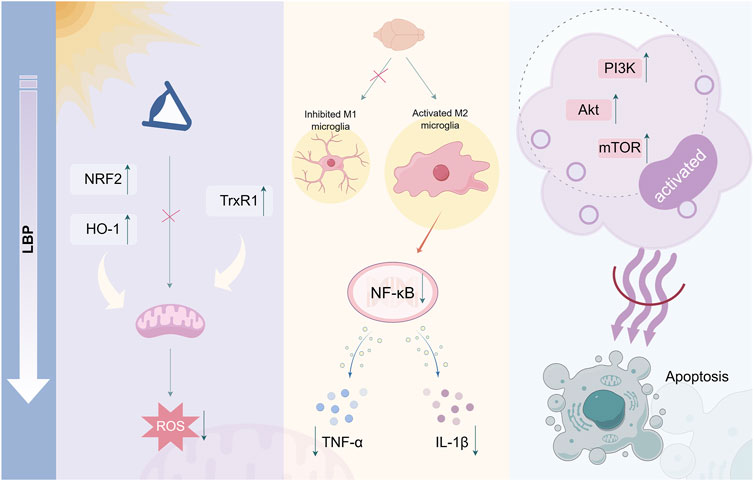
Figure 1. Mechanism of LBP in inhibiting retinal cell apoptosis. LBP inhibits ROS generation and reduces cellular oxidative damage by upregulating antioxidant genes. Simultaneously, it can inhibit the expression of pro-inflammatory cytokines TNF-α and IL-1β through NF-κB signaling. Additionally, LBP can activate the anti-apoptotic signaling pathway PI3K/Akt/mTOR, exerting anti-apoptotic effects By Figdraw.
LBP directly mitigates oxidative stress and indirectly protects the retina by regulating immune cell function. Microglia in the retina are immune cells of the central nervous system that typically oversee and maintain tissue homeostasis (Li L. et al., 2021). Once overactivated, they release a significant amount of inflammatory mediators, resulting in retinal inflammation and damage (Zhang et al., 2022). LBP can regulate microglial activities, inhibit their polarization toward the pro-inflammatory M1 phenotype, facilitate their transformation toward the anti-inflammatory M2 phenotype, and consequently enhance the survival rate of retinal ganglion cells (Li et al., 2019). LBP diminishes neuroinflammation and mitigates chronic retinal inflammation to protect visual function by inhibiting microglial phagocytosis and migration, decreasing the release of inflammatory mediators, including TNF-α and IL-1β, and inhibiting NF-κB signaling pathway activation (Ni et al., 2024). Furthermore, LBP can activate anti-apoptotic signaling pathways in retinal cells, including phosphatidylinositol 3-kinase (PI3K)/protein kinase (Akt)/mammalian target of rapamycin (mTOR), enhance cell survival factor expression, and inhibit the activation of apoptosis-related proteins, directly combating the process of cell apoptosis (Qi et al., 2022). LBP has potential therapeutic value in retinal degenerative diseases, including RP and AMD, which are characterized by retinal cell apoptosis.
3.2 Carotenoids
Lycium barbarum L. is abundant in carotenoids, including β-carotene, lutein, and zeaxanthin. (Neelam et al., 2021), which are complex chemical structures composed of various carbon-hydrogen chains and functional groups, including hydroxyl and ketone groups (Li L. H. et al., 2020).
β-Carotene is crucial for the conversion to retinol in the body, which is essential for retinal photoreceptor cells (Zhong et al., 2023). Retinol, the active form of vitamin A, binds with opsin to form rhodopsin, an essential compound for retinal rod cells to detect low light (Fujiki et al., 2022). Upon exposure to light, rhodopsin decomposes into opsin and retinol, initiating the generation of neural signals and facilitating vision (Radhakrishnan et al., 2024). In the darkness, vitamin A can re-synthesize rhodopsin, maintaining the sensitivity of the retina to dim light. Therefore, the intake of β-carotene is essential for the synthesis of rhodopsin, ensuring the normal function of rod cells, homeostasis of retinal retinas, and promoting visual health. Recent studies have demonstrated that β-carotene can significantly mitigate the pathological structural damage of retinal tissue during RP disease, inhibit the secretion of inflammatory factors including NF-κB, TNF-α, interleukin-6 (IL-6), and IL-1β (Shi et al., 2024), reduce oxidative metabolite accumulation (Li et al., 2017), and effectively restore cone cell function (Moon et al., 2023).
The retina is exposed to light for a long time, particularly blue light, which has high energy and can directly penetrate the eyeball to reach the retina. Excessive blue light can induce photooxidative stress and damage retinal cells (Ziółkowska and Lewczuk, 2022). Lutein and zeaxanthin are densely concentrated in the macular region of the retina and can absorb light within a specific wavelength range (Masri et al., 2024). This optical property enables the filtration of harmful light, including blue light, and reduces photooxidative damage to the retina (Jia et al., 2017). From a biological perspective, the mechanism through which lutein and zeaxanthin protect retinal cells from apoptosis is associated with the regulation of antioxidant capacity and signaling pathways. They engage in the intracellular antioxidant defense system, capturing and neutralizing free radicals through their structure, protecting the lipids, proteins, and nucleic acids of retinal cells from oxidative stress damage (Rozanowska et al., 2023). Additionally, they can regulate cell signaling and maintain the normal order of metabolism, proliferation, and differentiation of retinal cells, thereby protecting the structural and functional integrity of cells and reducing the risk of apoptosis (Liu et al., 2021).
Because of their essential role in protecting retinal photoreceptor cells from apoptosis (Figure 2), carotenoids have exhibited significant potential in various retinal disease treatments and prevention. In retinal degenerative diseases characterized by photoreceptor cell apoptosis, including RP, β-carotene can effectively preserve patients’ dark adaptation ability, expand the visual field, and correct vision (Rotenstreich et al., 2013). Zeaxanthin dipalmitate can markedly improve the survival rate of photoreceptors, improve retinal photo response, and mitigate morphological and functional degeneration of the retina (Chen et al., 2024). In AMD, supplementation with carotenoids (especially lutein and zeaxanthin) slows down the geographic atrophy progression of AMD toward the fovea (Keenan et al., 2025) while protecting visual function (Li X. et al., 2021).
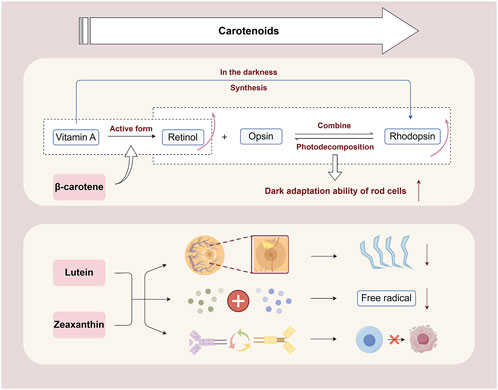
Figure 2. Mechanism of carotenoids in inhibiting retinal cell apoptosis. β-Carotene can help promote the conversion of retinol in the body, maintain the synthesis of rhodopsin, ensure the normal function of rod cells, and promote visual health. Lutein and zeaxanthin can filter blue light and reduce photooxidative damage to the retina. They neutralize free radicals through their own structure and protect retinal cells from oxidative stress damage. Besides, they can regulate cell signaling, maintain normal metabolism, proliferation, and differentiation of retinal cells, and reduce the risk of apoptosis By Figdraw.
3.3 Flavonoids
Flavonoids are a class of essential secondary metabolites widely distributed and abundant in foodborne plants (Liu et al., 2023). Previous studies demonstrated that the flavonoids in L. barbarum L. are primarily composed of rutin, quercetin, kaempferol, isorhamnetin, and luteolin, which exhibit various pharmacological effects, including antioxidant, anti-inflammatory, and vasodilatory properties (Mocan et al., 2019; Li T. et al., 2020).
Rutin has excellent antioxidant properties because the phenolic hydroxyl groups in its molecular structure can provide hydrogen atoms to bind with free radicals, rendering them inactive, reducing oxidative stress damage to cells, and protecting human cells and tissues (Safdari et al., 2022). A previous study has demonstrated that rutin can enhance NRF2 expression by activating the extracellular signal-regulated protein kinases one and 2 (ERK1/2) signaling pathway in RPE cells (Li Y. et al., 2021). It improves tert-butyl hydroperoxide-induced cell death and promotes cell viability by inhibiting the generation of intracellular ROS, demonstrating its potential value in preventing retinal diseases caused by oxidative damage. Some studies have demonstrated that rutin treatment significantly reduces the tortuosity index observed during fundus examination (Gupta et al., 2020), enhances the diameter of retinal arterioles, and reduces the concentrations of pro-inflammatory cytokines, including TNF-α, IL-1β, and IL-6 in tissues (Moldovan et al., 2023). This indicates that rutin may enhance the toughness of retinal capillaries, diminish their permeability, and alleviate inflammatory stimuli on endothelial cells while preserving the integrity of the vascular wall (Palmitessa et al., 2022). Additionally, rutin can inhibit cell apoptosis by modulating intracellular signaling pathways (Ma et al., 2017). It can enhance the expression of cell survival factors, including brain-derived neurotrophic factor (BDNF) and nerve growth factor (NGF), diminish the level of caspase-3 in the retina, and increase the level of B-cell lymphoma-2 (Bcl-2), thereby demonstrating anti-apoptotic activity (Ola et al., 2015). This mechanism of action renders flavonoids significant in delaying the degeneration of retinal photoreceptor cells (Figure 3).
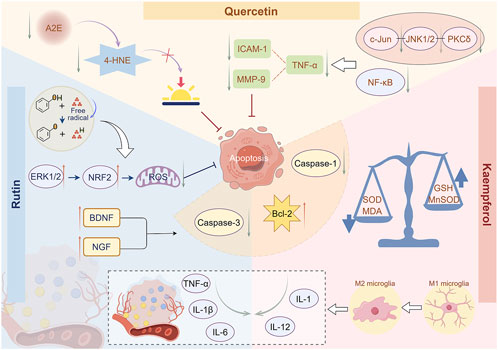
Figure 3. Mechanism of flavonoids in inhibiting retinal cell apoptosis. Rutin can enhance NRF2 expression by activating the ERK1/2 signaling pathway, thereby inhibiting the production of ROS in retinal cells. Rutin can reduce the levels of inflammatory factors, including TNF-α, IL-1β, and IL-6, in tissues, thereby alleviating inflammatory stimulation on retinal cells. Rutin can promote the expression of BDNF and NGF, thereby exhibiting anti-apoptotic activity. Quercetin inhibits A2E formation, reduces 4-HNE release, and thus prevents photooxidation processes in the retina. Quercetin can inhibit inflammatory signaling pathways and reduce the levels of TNF-α, ICAM-1, and MMP-9, thereby suppressing inflammatory responses. Kaempferol can significantly reduce the levels of ROS and MDA and increase the levels of GSH and MnSOD, thereby reducing cellular oxidative stress damage. Kaempferol can reduce the production of inflammatory factors TNF-α and IL-1β and significantly lower the levels of Bcl-2, caspase-1, and caspase-3, thereby inhibiting retinal cell apoptosis By Figdraw.
With advancing age, the dual retinal fluorescent compound A2E, a photoresponsive retinal aldehyde derivative, gradually accumulates in RFE cells (Arunkumar and Bernstein, 2023). Quercetin has been shown to inhibit the formation of photooxidative A2E species from the source, reduce the release of lipid peroxidation product 4-hydroxynonenal (4-HNE), and consequently prevent the photooxidative process in the retina (Wang et al., 2017). Quercetin exhibits anti-inflammatory properties by inhibiting inflammatory signaling pathways, including PKCδ-JNK1/2-c-Jun and NF-κB, decreasing the synthesis of inflammatory mediators, including TNF-α, and suppressing the expression and activity of intercellular adhesion molecule-1 (ICAM-1) and matrix metalloproteinase-9 (MMP-9), thus mitigating the recruitment and dissemination of inflammatory cells (Cheng S. et al., 2019).
The chemical structural similarity between kaempferol and quercetin endows them with similar biological characteristics and functions. It can act on immune cells, regulate cytokine secretion, maintain an appropriate level of the immune response, and prevent excessive immune response leading to inflammation (Tanaka et al., 2022). Diabetic retinopathy (DR) is a common secondary complication of diabetes. The immune system is involved in DR, which affects microglia-mediated retinal immune response (Lim et al., 2024). In the initial phase, it is characterized by the permeability of the blood-retinal barrier, facilitating interaction between the peripheral and retinal immune systems (Kim et al., 2024). A previous study demonstrated that kaempferol treatment promotes phenotypic and functional changes in immune cells, strongly inhibits pro-inflammatory responses during DR progression, diminishes the synthesis of inflammatory factors TNF-α and IL-1β, and significantly reduces the levels of Bcl-2, caspase-1, and caspase-3, consequently inhibiting retinal cell apoptosis (Albalawi et al., 2024). In RPE cells, kaempferol has been shown to significantly reduce the levels of ROS and lipid peroxidation product malondialdehyde (MDA), while increasing the levels of endogenous antioxidants glutathione (GSH) and manganese superoxide dismutase (MnSOD) (Al Sabaani, 2020). Kaempferol can also reduce the production of inflammatory factors including interleukin-1 (IL-1), IL-6, and interleukin-12 (IL-12), enhance RPE cell proliferation activity, and thereby reduce RPE cell apoptosis (Akalın and Selamoglu, 2019; Zhang et al., 2024). However, kaempferol can effectively modulate endothelial cell function, stimulate nitric oxide release, induce vasodilation, improve local blood perfusion, and deliver sufficient nutrients to tissues and organs (Firoz and Talwar, 2022). Experimental results revealed that in a mouse model of oxygen-induced retinopathy, kaempferol inhibited retinal neovascularization and effectively prevented VEGF-induced excessive retinal vascular permeability (Jung et al., 2020). Other flavonoids, including isorhamnetin, have demonstrated similar protective effects that may delay retinal disease progression (Hui et al., 2024).
4 The main mechanisms of retinal cell apoptosis and the targets of Lycium barbarum L. action
4.1 Oxidative stress mechanism and antioxidant intervention of Lycium barbarum L.
During evolution, cells have developed a complete antioxidant defense system. The system includes antioxidant enzymes, such as superoxide dismutase (SOD) and glutathione peroxidase (Selamoglu et al., 2017), which specifically catalyze the dismutation, decomposition, and other reactions of ROS, thereby reducing their concentration (Wang Y. et al., 2021). Concurrently, there are non-enzymatic antioxidant enzymes, including GSH, vitamin C, and vitamin E, which utilize their intrinsic chemical reduction properties to directly neutralize ROS (Monsalves et al., 2020). Under normal physiological conditions, the two work collaboratively to regulate ROS, maintain cellular redox homeostasis, and prevent oxidative damage (Georgescu et al., 2022). However, in a pathological state, the function of the antioxidant defense system is impaired, leading to an imbalance, ROS accumulates in large quantities, and cell damage is exacerbated (Zhou et al., 2021).
The retina is the fundamental region of visual perception, where photoreceptor cells play a crucial role in the initial stage of visual signal transduction (Takeda et al., 2022). Photoreceptor cells exhibit a unique physiological characteristic of a high metabolic rate aimed at fulfilling the energy demands required for visual signal capture and conversion (Oltra et al., 2022). Simultaneously, their microenvironment exhibits a high oxygen partial pressure. This unique metabolic and environmental condition renders photoreceptor cells highly susceptible to oxidative stress (Santhanam et al., 2023). The complex pathological mechanism of retinal lesions involves a disruption of internal homeostasis, leading to a substantial increase in ROS and free radicals (Yang et al., 2020; Al-Sroji et al., 2023), including superoxide anions and hydroxyl radicals (Selamoglu et al., 2020), which possess potent oxidative properties and specifically target photoreceptor cells (Garza et al., 2024). Initially, ROS trigger lipid peroxidation reactions, compromising the lipid bilayer architecture of the cell membrane, resulting in impaired membrane integrity, decreased fluidity, and severe disruption of substance exchange and signal transmission intracellularly and extracellularly (Sui et al., 2024). At the molecular level, ROS can modify amino acid residues, causing conformational changes in proteins and resulting in the loss of their original physiological functions, thereby impeding numerous protein-dependent metabolic pathways within cells. Oxidative damage can adversely affect DNA, leading to base pair mismatches, deletions, and DNA strand breaks, resulting in errors in the storage and transmission of genetic information (Kumar et al., 2022). These oxidative stress damage events are interconnected and progressively activate the meticulously regulated apoptotic signaling pathway within cells, creating latent risk for photoreceptor apoptosis and endangering visual function (Huang et al., 2024).
The various active metabolites in L. barbarum L. exhibit antioxidant properties (Figure 4). NRF2 is the principal transcription factor governing the cellular antioxidant stress response (Jin et al., 2024). Under normal conditions, NRF2 binds to kelch-like ECH-associated protein 1 (KEAP1) and remains in an inactive state within the cytoplasm (Ulasov et al., 2022). Under oxidative stress, photoreceptor cells utilize LBP to specifically bind and modify KEAP1, facilitating the uncoupling of NRF2 and KEAP1, which subsequently activates and translocates NRF2 into the nucleus (Hu et al., 2021; Nguyen et al., 2024). NRF2 translocates to the nucleus, precisely binds to antioxidant response elements (ARE) (Choublier et al., 2022) and activates a cascade of transcription programs for antioxidant enzyme genes, including SOD and glutathione peroxidase 4 (GPX4), leading to their substantial expression (Yu and Xiao, 2021). SOD catalyzes the dismutation of superoxide anions into hydrogen peroxide and oxygen, while GPX4 subsequently reduces hydrogen peroxide to water. The two collaborate to efficiently eliminate excess ROS in cells, reducing oxidative stress at its origin and maintaining the redox homeostasis of the photoreceptor cell environment (García-Pérez et al., 2021). Carotenoids can directly neutralize free radicals, and their polyene chain structure can interact with free radicals to transform them into stable products, thereby reducing the direct assault of free radicals on photoreceptor cells (Welc-Stanowska et al., 2023). Flavonoids can inhibit ROS generation reactions catalyzed by metal ions, including iron and copper ions, by chelating them (Vučkovski et al., 2024). Simultaneously, they can enhance the activity of other antioxidant components, synergistically producing antioxidant effects and inhibiting the oxidative stress-induced apoptosis process of photoreceptor cells through multiple pathways (Shao et al., 2019).
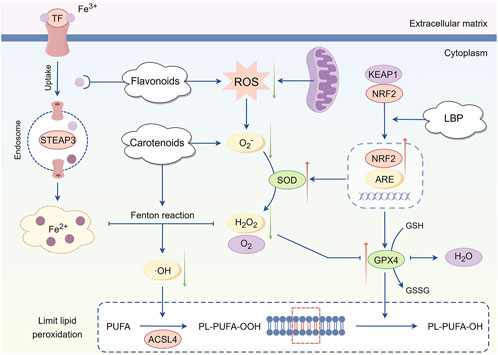
Figure 4. Mechanism of antioxidant intervention in Lycium barbarum L. LBP facilitates the dissociation of NRF2 from KEAP1, leading to the activation of NRF2 and its translocation into the nucleus. This process initiates the transcription and expression of antioxidant enzyme genes, including SOD and GPX4. SOD catalyzes the dismutation of superoxide anions into hydrogen peroxide and oxygen, while GPX4 further reduces hydrogen peroxide to water. Together, these enzymes effectively mitigate excess ROS within cells, thereby reducing oxidative stress at its source and maintaining the redox homeostasis of the photoreceptor cell environment. Additionally, carotenoids directly neutralize free radicals, converting them into stable products, which diminishes the direct assault of free radicals on photoreceptor cells. Flavonoids contribute by chelating metal ions, thereby inhibiting ROS production By Figdraw.
4.2 Inflammatory response mechanism and anti-inflammatory regulation of Lycium barbarum L.
When the retina is damaged or infected, immune cells, including microglia and macrophages, become activated, secreting a substantial amount of inflammatory factors, including TNF-α, IL-1β, and IL-6 (Wang et al., 2025). These inflammatory factors further elicit inflammatory responses in retinal cells by activating downstream signaling pathways, including NF-κB and janus kinase (JAK)/signal transducer and activator of transcription (STAT), resulting in cellular damage and apoptosis (Figure 5).
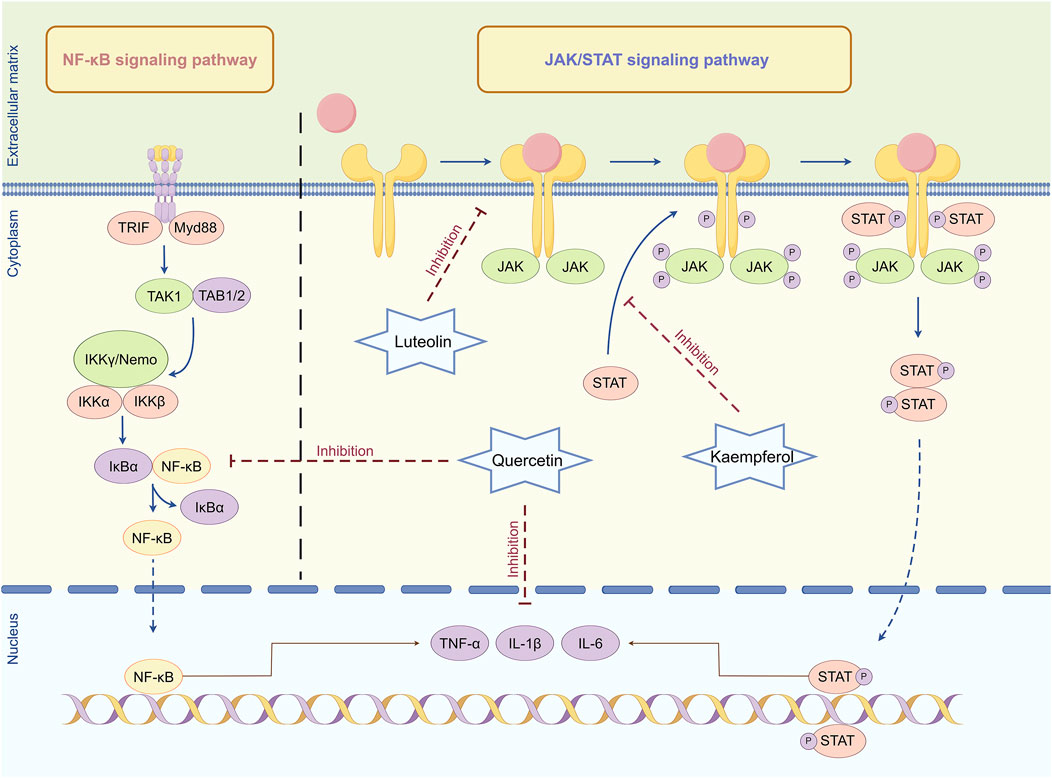
Figure 5. Mechanism of anti-inflammatory regulation in Lycium barbarum L. Quercetin can inhibit the activation of NF-κB and JAK/STAT signaling pathways, reduce the production and release of inflammatory factors, and thus alleviate the damage of inflammatory response to retinal cells. Luteolin can interfere with the binding of cytokines to their corresponding receptors, thereby preventing receptor dimerization and JAK recruitment, thereby inhibiting JAK activation. Kaempferol can inhibit the phosphorylation of receptor tyrosine by activating JAK, blocking the formation of STAT docking sites and inhibiting signal transduction By Figdraw.
NF-κB is a nuclear transcription factor prevalent in eukaryotic cells, crucial for regulating multiple biological processes, including immune response, inflammatory response, cell proliferation, differentiation, and apoptosis (Ravichandran and Selamoglu, 2023; Cornice et al., 2024). NF-κB typically exists in an inactive state within cells and binds to its inhibitory protein inhibitor of NF-κB (IκB) in the cytoplasm (Du et al., 2024). When cells encounter diverse stress stimuli, including ultraviolet radiation, oxidative stress, or cytokines, these stimuli activate signal transduction pathways, resulting in the phosphorylation of IκB and subsequent proteasomal degradation (Mamun et al., 2024). This process facilitates NF-κB release and translocation into the nucleus, where it binds to the promoter regions of specific genes, thereby enhancing their transcription and expression (Shahbazi and Zakerali, 2022). Activation of NF-κB in retinal cells enhances the transcription and expression of various inflammatory genes. These genes encode cytokines, chemokines, adhesion molecules, and acute phase response proteins (Ando et al., 2020). The active metabolites in L. barbarum L., including quercetin, can inhibit the activation of the NF-κB signaling pathway, diminish the synthesis and release of inflammatory factors, and thereby mitigate the detrimental effects of the inflammatory response on retinal cells (Cheng S. C. et al., 2019).
Additionally, the JAK/STAT signaling pathway is essential in the inflammatory response of retinal cells alongside the NF-κB signaling pathway. The JAK/STAT signaling pathway is a series of reactions involving interactions among intracellular proteins, primarily involved in processes including immunity, cell division, apoptosis, and tumorigenesis (Xue et al., 2023). Activation of the JAK/STAT signaling pathway enhances the transcription and expression of inflammatory factors, thereby exacerbating the inflammatory response and resulting in damage and dysfunction of retinal cells (Chen et al., 2022). A previous study has demonstrated that the active metabolites in L. barbarum L. can inhibit the activation of the JAK/STAT signaling pathway. Luteolin can obstruct the binding of cytokines to their respective receptors, thus hindering receptor dimerization and JAK recruitment, thereby inhibiting JAK activation (Tai et al., 2014). Subsequently, kaempferol can inhibit the phosphorylation of receptor tyrosine by activating JAK, thereby obstructing the formation of STAT docking sites and further inhibiting STAT activation and subsequent signal transduction (Li et al., 2023). By inhibiting the activation of the JAK/STAT signaling pathway, quercetin diminishes the transcription and translation of inflammatory factors, thereby mitigating the detrimental effects of the inflammatory response on retinal cells, which aids in preventing and treating inflammation-related retinal diseases (Zou et al., 2024).
4.3 Abnormal autophagy mechanism and regulatory intervention of Lycium barbarum L.
Autophagy is a cellular self-degradation process involving autophagosome formation with a bilayer membrane structure, which encapsulates and degrades proteins, organelles, and other substances to maintain homeostasis (Dragowska et al., 2024). This process is essential in various physiological and pathological mechanisms, including cell survival, development, immunity, and disease manifestation (Zapatería and Arias, 2024). However, the dysregulation of autophagy may lead to impaired retinal cell function, potentially precipitating various retinal diseases (Wang X. et al., 2024).
The PI3K/Akt/mTOR signaling pathway is essential for anti-apoptotic processes. It is a primary regulator of cellular autophagy (Figure 6), which is regulated by sensing the nutritional and energy status inside the cell (Demir et al., 2024). PI3K is the starting point for signal transduction, catalyzing phosphatidylinositol 4,5-bisphosphate (PIP2) to generate phosphatidylinositol 3,4,5-trisphosphate (PIP3), which functions as a second messenger to activate downstream signaling molecules (Li et al., 2022). Akt, a direct downstream target of PI3K, is activated by the recruitment of PIP3 and further activates mTOR through phosphorylation, initiating a cascade reaction (Sun et al., 2022). In a nutrient-rich environment, the high energy and abundant nutrients within cells activate the PI3K/Akt/mTOR signaling pathway (Liu and Sabatini, 2020). As the terminal effector molecule of this pathway, the activated state of mTOR effectively inhibits autophagy by inhibiting the activity of the unc-51-like kinase 1/2 (ULK1/2) complex (autophagy initiating complex) and hindering the formation of autophagosomes (Despotović et al., 2022). This process guarantees that cells can completely exploit the nutritional resources available from their environment to facilitate their growth and proliferation requirements. However, under conditions of nutrient deficiency or injury stress, the activity of the PI3K/Akt signaling pathway weakens, resulting in the dephosphorylation and subsequent inactivation of mTOR (Zi et al., 2022). The mTOR-mediated inhibition of autophagy is subsequently alleviated, facilitating the onset of autophagy (Wang and Zhang, 2019). The ULK1/2 complex is activated, initiating the expression of autophagy-related genes and facilitating the formation and maturation of autophagosomes (Liang et al., 2023). Excessive autophagy can result in the excessive degradation of important nutrients and proteins within cells, resulting in ineffective clearance of autophagosomes, which can compromise cellular structure and function, potentially initiating apoptotic pathways (Wang L. et al., 2021).
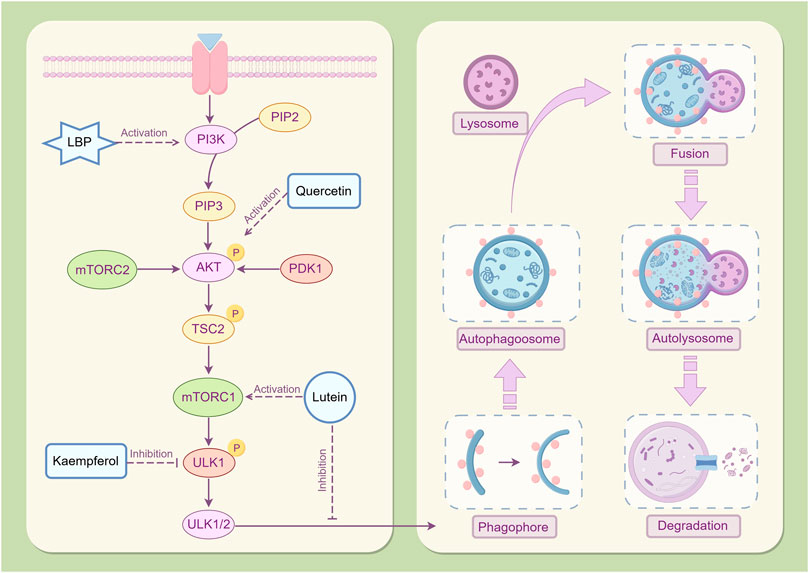
Figure 6. Mechanism of autophagy regulation in Lycium barbarum L. LBP mitigates cellular damage induced by excessive autophagy through the activation of the PI3K/Akt/mTOR signaling pathway, modulation of autophagy-related gene expression, and facilitation of autophagosome formation. Lutein exerts its effects by inhibiting autophagosome formation via mTOR activation, thereby reducing cellular apoptosis. Quercetin has been demonstrated to significantly elevate phosphorylated Akt protein levels in the retina. Moreover, Kaempferol has exhibited efficacy in inhibiting the phosphorylation of mTOR and ULK1, thereby facilitating the removal of senescent and damaged cellular components By Figdraw.
The PI3K/AKT/mTOR signaling pathway is highly activated in RPE cells during proliferative vitreoretinopathy (Cai et al., 2012). LBP can enhance the survival and proliferation of retinal cells by activating the PI3K/Akt/mTOR signaling pathway (Qi et al., 2022) and can inhibit excessive autophagy in RPE cells, thereby safeguarding them from light-induced damage (Gao et al., 2022). This activating effect preserves the nutritional and energy balance within cells, thus preventing cellular damage from excessive autophagy by regulating the expression of autophagy-related genes and the formation of autophagosomes (Yu et al., 2018). Additionally, lutein inhibits the formation of autophagosomes after hypoxia injury by activating the mTOR signaling pathway, thereby enhancing the survival rate of Müller cells and inhibiting cell apoptosis (Fung et al., 2016). After quercetin intervention, the phosphorylated Akt protein level in the diabetic retina increased significantly (Ola et al., 2017). Quercetin may protect neurons in the diabetic retina from damage by inhibiting neuronal apoptosis. Kaempferol was reported to exhibit good effects in mTOR inhibition and ULK1 phosphorylation to eliminate aging and damaged components (Salehi et al., 2018; Kim et al., 2023).
4.4 Mitochondrial dysfunction and the protective effect of Lycium barbarum L.
Mitochondria are the cellular organelles that generate adenosine triphosphate (ATP) and maintain normal physiological functions within cells (Botchway et al., 2022). However, in numerous disease states, the mitochondrial function can be compromised, leading to insufficient energy supply, increased oxidative stress, and apoptosis, a phenomenon known as mitochondrial dysfunction (Huang et al., 2023). For retinal cells, a lack of sufficient energy impedes their ability to maintain normal physiological functions, leading to a significant decrease in the efficiency of capturing and converting light signals and a deceleration or interruption in the transmission of nerve signals (Zhang et al., 2023). This series of chain reactions will ultimately exert a severe impact on the visual system. Mitochondria are energy producers and the main source of intracellular ROS. The impaired mitochondrial function may lead to an abnormal increase in ROS production while the cellular capacity to eliminate ROS diminishes, significantly increasing intracellular oxidative stress levels (Zhao et al., 2022). Oxidative stress is a deleterious process that further damages the DNA, proteins, and membrane structure of mitochondria, forming a vicious cycle where mitochondrial dysfunction increases ROS, thereby exacerbating mitochondrial damage (Sule et al., 2022).
Additionally, mitochondria regulate intracellular calcium ions, which is crucial for maintaining cellular homeostasis (Guo et al., 2023). Impaired mitochondrial function can severely disrupt calcium ion homeostasis, resulting in an abnormal increase in intracellular calcium ion concentration, which may trigger apoptosis or necrosis of retinal cells (Yan et al., 2022). This is an irreversible process of cell demise, whether through programmed apoptosis or necrosis caused by physical or chemical injury, which can lead to a significant decline or complete loss of retinal function.
A previous study demonstrated that LBP significantly ameliorates mitochondrial dysfunction in retinal cells induced by light damage (Wu F. et al., 2024). In the light damage model, LBP can significantly mitigate mitochondrial DNA damage, enhance membrane potential, and restore respiratory chain function, thereby enhancing the energy supply and physiological function of photoreceptor cells. In addition, LBP can inhibit the inflammatory response induced by light damage and mitigate subsequent cellular damage caused by inflammatory factors (Ni et al., 2024). Carotenoids and flavonoids, the main active metabolites in L. barbarum L., may optimize the energy metabolism pathway of mitochondria, promote ATP synthesis, and provide sufficient energy for photoreceptor cells (Pan et al., 2019; Bu et al., 2020). These metabolites can diminish the generation of ROS in mitochondria, safeguard the structural and functional integrity of mitochondria, and consequently inhibit the critical pathway of retinal cell apoptosis at the mitochondrial level (Imran et al., 2019; Ademowo et al., 2024). The specific mechanism is shown in Figure 7.
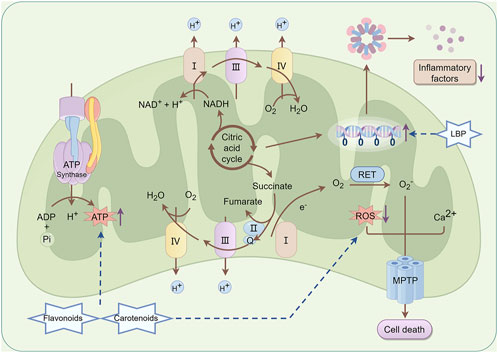
Figure 7. Mechanism of mitochondrial protection in Lycium barbarum L. LBP can effectively mitigate mitochondrial DNA damage, enhance membrane potential, and restore respiratory chain function, thereby improving the energy supply and physiological function of photoreceptor cells. Besides, LBP can inhibit the inflammatory response induced by photodamage and reduce further cellular damage from inflammatory factors. Carotenoids and flavonoids contribute to promoting ATP synthesis and provide adequate energy support for photoreceptor cells. Furthermore, they reduce ROS production within mitochondria, thereby preserving the structural and functional integrity of mitochondria and interrupting the critical pathway of retinal cell apoptosis at the mitochondrial level By Figdraw.
5 Conclusion and perspectives
Compared with TCM treatment methods, modern medicine for retinal diseases often targets a single point. When faced with the complex physiological and pathological processes of the retina, single-target treatment is difficult to address all aspects of the disease comprehensively. In contrast, multiple metabolites in L. barbarum L. can work together to treat retinal diseases. LBP has a unique immune-regulating function, which not only enhances the self-repair ability of retinal cells but also plays a vital role in autophagy regulation. Carotenoids, with their strong antioxidant capacity, can quickly eliminate free radicals within retinal cells, reducing oxidative stress damage and thereby decreasing the risk of cell apoptosis. Flavonoids can suppress the release of inflammatory factors, alleviate the attack of inflammation on retinal cells, and maintain the stability of the cellular microenvironment. These metabolites intervene in retinal cell apoptosis from aspects such as immune regulation, antioxidant activity, anti-inflammation, autophagy regulation, and mitochondrial function protection and can deal with the complex disease mechanisms more comprehensively. In addition, the viewpoint of TCM holds that L. barbarum L. not only acts on eye cells but also regulates overall bodily functions, improves the internal environment, and prevents and treats diseases from the root cause. It effectively complements the local and targeted treatment of modern medicine and opens up new ideas for the treatment of retinal diseases.
Despite the fact that numerous scholars have verified the efficacy of L. barbarum L. in the treatment and prevention of retinal cell apoptosis, there are still several areas in need of improvement. Firstly, neither in vitro nor in vivo models can precisely mimic the physiological and pathological states of the retina. 2D cell cultures lack the complex immune responses and intricate intercellular interactions. Animal models suffer from species-specific differences, which pose significant challenges to accurately reflecting the real-world situation in human retinas. Secondly, there is a conspicuous absence of unified standards in the extraction and identification of L. barbarum L. metabolites. Different laboratories employ diverse extraction methods and experimental procedures, resulting in incomparable research outcomes. This not only hampers the cross-comparison of data but also severely obstructs the in-depth understanding of the underlying mechanisms of action of these metabolites. Thirdly, current clinical research on L. barbarum L. has several limitations. The number of studies is scarce, and the sample sizes are generally small. The experimental designs often lack rigor, and the follow-up periods are short. Although a series of experimental investigations have indicated that L. barbarum L. extracts are safe at normal doses, with no significant toxic reactions observed in animal and cell-based experiments (Mocan et al., 2018), the long-term clinical efficacy and safety of L. barbarum L. remain in a state of uncertainty. With the widespread use of L. barbarum L. in the healthcare and pharmaceutical industries, the potential toxicity at high doses, as well as the long-term cumulative effects, necessitate continuous attention. These aspects could have a profound impact on its clinical applications and safety evaluations. Fourthly, some metabolites of L. barbarum L. have been proven to be highly absorbable. For example, LBP can enter the bloodstream via specific transporters in the intestine, thereby exerting immune-regulating and cell-protecting functions. Nevertheless, further research is urgently required to elucidate the absorption, distribution, metabolism, and excretion of these metabolites under different individual and physiological conditions. This knowledge is crucial for optimizing the clinical utilization and therapeutic efficacy of L. barbarum L.
In the future, the research on L. barbarum L. should focus on several key directions. Developing advanced in vitro models such as 3D organoids or co-cultures, and conducting in vivo research using non-human primates that are more similar to human retinal physiology, can yield more accurate results. These approaches will enhance our understanding of the real-world effects of L. barbarum L. on retinal diseases. The scientific community should also collaborate to establish unified standards, making the results of different laboratories comparable and reproducible. This will promote the integration and transformation of research findings across the field. Additionally, it is of utmost importance to utilize advanced technologies such as single-cell sequencing and multi-omics to deeply explore the molecular mechanisms of L. barbarum L. in preventing retinal apoptosis. In terms of clinical research, large-scale, multi-center, randomized controlled trials should be carried out. By increasing the sample size, extending the follow-up time, and comprehensively evaluating the efficacy, safety, and mechanism of action of L. barbarum L. in preventing and treating diseases related to retinal cell apoptosis, we can gain a more comprehensive understanding of its therapeutic value. Meanwhile, exploring the combined application of L. barbarum L. with other botanical drugs and chemical therapies holds great promise for improving the therapeutic effect, ultimately enhancing the vision and quality of life of patients. Although L. barbarum L. shows great potential in the treatment of retinal diseases, continuous efforts in research methods, mechanism exploration, and clinical transformation are essential to fully realize its therapeutic value.
Author contributions
MX: Conceptualization, Visualization, Writing–original draft, Investigation, Resources, Writing–review and editing. JP: Investigation, Resources, Visualization, Writing–original draft. Shunhua Zhou: Investigation, Resources, Writing–original draft. QG: Investigation, Resources, Writing–original draft. JL: Project administration, Supervision, Writing–review and editing. CO: Project administration, Supervision, Writing–review and editing. HS: Conceptualization, Funding acquisition, Writing–review and editing. QiP: Conceptualization, Funding acquisition, Writing–review and editing.
Funding
The author(s) declare that financial support was received for the research and/or publication of this article. This study was supported by the National Natural Science Foundation of China (no. 82374429), the Guidance Project of Academician Liu Liang’s Expert Workstation (no. 22YS003), the Natural Science Foundation of Hunan Province (no. 2024JJ9428), the Youth Talent Support Project of the Chinese Association of Traditional Chinese Medicine (no. 2024-QNRC2-B25), the Science and Technology Talent Support Project of Hunan Province (no. 2023TJ-N25), the Key Scientific Research Project of Hunan Provincial Department of Education (no. 22A0241), the Scientific Research Project of Hunan Provincial Health Commission (no. 202207024644), and the High-level Talent Project of Hunan Provincial Health Commission (no. 20240304116).
Conflict of interest
The authors declare that the research was conducted in the absence of any commercial or financial relationships that could be construed as a potential conflict of interest.
Generative AI statement
The authors declare that no Generative AI was used in the creation of this manuscript.
Publisher’s note
All claims expressed in this article are solely those of the authors and do not necessarily represent those of their affiliated organizations, or those of the publisher, the editors and the reviewers. Any product that may be evaluated in this article, or claim that may be made by its manufacturer, is not guaranteed or endorsed by the publisher.
References
Ademowo, O. S., Oyebode, O., Edward, R., Conway, M. E., Griffiths, H. R., and Dias, I. H. K. (2024). Effects of carotenoids on mitochondrial dysfunction. Biochem. Soc. Trans. 52, 65–74. doi:10.1042/BST20230193
Akalın, G., and Selamoglu, Z. (2019). Nutrition and foods for skin health. jpc 7, 31–33. doi:10.18502/jpc.v7i1-2.1620
Albalawi, F. E., Alsharif, I., Moawadh, M. S., Alkhoshaiban, A., Falah Alshehri, F., Albalawi, A. E., et al. (2024). Immunomodulatory effects of Kaempferol on microglial and Macrophage cells during the progression of diabetic retinopathy. Int. Immunopharmacol. 133, 112021. doi:10.1016/j.intimp.2024.112021
Al Sabaani, N. (2020). Kaempferol protects against hydrogen peroxide-induced retinal pigment epithelium cell inflammation and apoptosis by activation of SIRT1 and inhibition of PARP1. J. Ocul. Pharmacol. Ther. 36, 563–577. doi:10.1089/jop.2019.0151
Al-Sroji, R. Y., Al-Laham, S., and Almandili, A. (2023). Protective effects of vitamin D3 (cholecalciferol) on vancomycin-induced oxidative nephrotoxic damage in rats. Pharm. Biol. 61, 755–766. doi:10.1080/13880209.2023.2204916
Ando, Y., Sato, Y., Kudo, A., Watanabe, T., Hirakata, A., Okada, A. A., et al. (2020). Anti-inflammatory effects of the NF-κB inhibitor dehydroxymethylepoxyquinomicin on ARPE-19 cells. Mol. Med. Rep. 22, 582–590. doi:10.3892/mmr.2020.11115
Arunkumar, R., and Bernstein, P. S. (2023). Macular pigment carotenoids and bisretinoid A2E. Adv. Exp. Med. Biol. 1415, 15–20. doi:10.1007/978-3-031-27681-1_3
Bishayee, K., Lee, S. H., Heo, Y. J., Cho, M. L., and Park, Y. S. (2024). The unanticipated contribution of Zap70 in retinal degeneration: implications for microglial inflammatory activation. Prog. Neurobiol. 244, 102706. doi:10.1016/j.pneurobio.2024.102706
Botchway, B. O., Okoye, F. C., Chen, Y., Arthur, W. E., and Fang, M. (2022). Alzheimer disease: recent updates on apolipoprotein E and gut microbiome mediation of oxidative stress, and prospective interventional agents. Aging Dis. 13, 87–102. doi:10.14336/AD.2021.0616
Bu, X., Lin, J., Cheng, J., Yang, D., Duan, C., Koffas, M., et al. (2020). Engineering endogenous ABC transporter with improving ATP supply and membrane flexibility enhances the secretion of β-carotene in Saccharomyces cerevisiae. Biotechnol. Biofuels 13, 168. doi:10.1186/s13068-020-01809-6
Cai, N., Dai, S., Liu, N., Liu, L., Zhao, N., and Chen, L. (2012). PI3K/AKT/mTOR signaling pathway inhibitors in proliferation of retinal pigment epithelial cells. Int. J. Ophthalmol. 5, 675–680. doi:10.3980/j.issn.2222-3959.2012.06.05
Chai, Z., Silverman, D., Li, S., Bina, P., and Yau, K. W. (2024). Dark continuous noise from visual pigment as a major mechanism underlying rod-cone difference in light sensitivity. Proc. Natl. Acad. Sci. U. S. A. 121, e2418031121. doi:10.1073/pnas.2418031121
Chang, Y., Lee, Y., Hsu, M., Wang, M., Tsou, S., Chen, C., et al. (2021). Protective effect of quercetin on sodium iodate-induced retinal apoptosis through the reactive oxygen species-mediated mitochondrion-dependent pathway. Int. J. Mol. Sci. 22, 4056. doi:10.3390/ijms22084056
Chen, S., Lathrop, K. L., Kuwajima, T., and Gross, J. M. (2022). Retinal ganglion cell survival after severe optic nerve injury is modulated by crosstalk between Jak/Stat signaling and innate immune responses in the zebrafish retina. Development 149, dev199694. doi:10.1242/dev.199694
Chen, X., Zhang, S., Yang, L., Kong, Q., Zhang, W., Zhang, J., et al. (2024). Zeaxanthin dipalmitate-enriched wolfberry extract improves vision in a mouse model of photoreceptor degeneration. PLoS One 19, e0302742. doi:10.1371/journal.pone.0302742
Cheng, S., Wu, Y., Huang, W., Pang, J. H. S., Huang, T. H., and Cheng, C. (2019b). Anti-inflammatory property of quercetin through downregulation of ICAM-1 and MMP-9 in TNF-α-activated retinal pigment epithelial cells. Cytokine 116, 48–60. doi:10.1016/j.cyto.2019.01.001
Cheng, S. C., Huang, W. C., S Pang, J. H., Wu, Y. H., and Cheng, C. Y. (2019a). Quercetin inhibits the production of IL-1β-induced inflammatory cytokines and chemokines in ARPE-19 cells via the MAPK and NF-κB signaling pathways. Int. J. Mol. Sci. 20, 2957. doi:10.3390/ijms20122957
Choublier, N., Taghi, M., Menet, M.-C., Le Gall, M., Bruce, J., Chafey, P., et al. (2022). Exposure of human cerebral microvascular endothelial cells hCMEC/D3 to laminar shear stress induces vascular protective responses. Fluids Barriers CNS 19, 41. doi:10.1186/s12987-022-00344-w
Cornice, J., Verzella, D., Arboretto, P., Vecchiotti, D., Capece, D., Zazzeroni, F., et al. (2024). NF-κB: governing macrophages in cancer. Genes (Basel) 15, 197. doi:10.3390/genes15020197
Demir, K., Turgut, R., Şentürk, S., Işıklar, H., and Günalan, E. (2024). The therapeutic effects of bioactive compounds on colorectal cancer via PI3K/Akt/mTOR signaling pathway: a critical review. Food Sci. Nutr. 12, 9951–9973. doi:10.1002/fsn3.4534
Despotović, A., Mirčić, A., Misirlić Denčić, S., Harhaji Trajković, L., Trajković, V., Zogović, N., et al. (2022). Combination of ascorbic acid and menadione induces cytotoxic autophagy in human glioblastoma cells. Oxid. Med. Cell Longev. 2022, 2998132. doi:10.1155/2022/2998132
Dragowska, W. H., Singh, J., Wehbe, M., Anantha, M., Edwards, K., Gorski, S. M., et al. (2024). Liposomal formulation of hydroxychloroquine can inhibit autophagy in vivo. Pharmaceutics 17, 42. doi:10.3390/pharmaceutics17010042
Du, Y., Lu, J., Feng, L., Zhao, L., Wu, P., He, Y., et al. (2024). Asperulosidic acid inhibits the PI3K/Akt/NF-κB pathway to suppress endotoxin-induced uveitis. Front. Med. (Lausanne) 11, 1524779. doi:10.3389/fmed.2024.1524779
Firoz, A., and Talwar, P. (2022). COVID-19 and retinal degenerative diseases: promising link “Kaempferol.”. Curr. Opin. Pharmacol. 64, 102231. doi:10.1016/j.coph.2022.102231
Fitton, C. A., Quigley, M. M. R., and Belch, J. J. F. (2025). Risk factors for wet macular degeneration: a systematic review, with novel insights from the Scottish Heart Health Extended Cohort. BMC Ophthalmol. 25, 67. doi:10.1186/s12886-025-03868-5
Fujiki, T., Shinozaki, R., Udono, M., and Katakura, Y. (2022). Identification and functional evaluation of polyphenols that induce regulatory T cells. Nutrients 14, 2862. doi:10.3390/nu14142862
Fung, F. K. C., Law, B. Y. K., and Lo, A. C. Y. (2016). Lutein attenuates both apoptosis and autophagy upon cobalt (II) chloride-induced hypoxia in rat műller cells. PLoS One 11, e0167828. doi:10.1371/journal.pone.0167828
Gao, Y., Li, J., Huang, J., Li, W., and Yu, Y. (2022). Effects of Lycium barbarum polysaccharide on the photoinduced autophagy of retinal pigment epithelium cells. Int. J. Ophthalmol. 15, 23–30. doi:10.18240/ijo.2022.01.04
García-Pérez, E., Ryu, D., Lee, C., and Lee, H. J. (2021). Ochratoxin A induces oxidative stress in HepG2 cells by impairing the gene expression of antioxidant enzymes. Toxins (Basel) 13, 271. doi:10.3390/toxins13040271
Garza, S., James, G., Park, H. G., Baker, P. R. S., and Brenna, J. T. (2024). Analysis of intact in vivo peroxidized phospholipids from bovine retina via LC-MS/MS and GC-MS/MS using autoxidized retina reference standards. Anal. Chem. doi:10.1021/acs.analchem.4c03289
Georgescu, S. R., Mitran, C. I., Mitran, M. I., Matei, C., Popa, G. L., Erel, O., et al. (2022). Thiol-disulfide homeostasis in skin diseases. J. Clin. Med. 11, 1507. doi:10.3390/jcm11061507
Guo, T., Chen, M., Liu, J., Wei, Z., Yuan, J., Wu, W., et al. (2023). Neuropilin-1 promotes mitochondrial structural repair and functional recovery in rats with cerebral ischemia. J. Transl. Med. 21, 297. doi:10.1186/s12967-023-04125-3
Gupta, S. K., Sharma, H. P., Das, U., Velpandian, T., and Saklani, R. (2020). Effect of rutin on retinal VEGF, TNF-α, aldose reductase, and total antioxidant capacity in diabetic rats: molecular mechanism and ocular pharmacokinetics. Int. Ophthalmol. 40, 159–168. doi:10.1007/s10792-019-01165-x
Hu, R., Qi, S., Wang, Y., Li, W., Zou, W., Wang, Z., et al. (2024). Ginsenoside Rg3 improved age-related macular degeneration through inhibiting ROS-mediated mitochondrion-dependent apoptosis in vivo and in vitro. Int. J. Mol. Sci. 25, 11414. doi:10.3390/ijms252111414
Hu, X., Mu, L., Zhu, L., Chang, X., Nie, L., Wang, L., et al. (2021). Lycium barbarum polysaccharides attenuate cardiovascular oxidative stress injury by enhancing the Keap1/Nrf2 signaling pathway in exhaustive exercise rats. Mol. Med. Rep. 24, 643. doi:10.3892/mmr.2021.12282
Huang, H. W., Yang, C. M., and Yang, C. H. (2024). Beneficial effects of fibroblast growth factor-1 on retinal pigment epithelial cells exposed to high glucose-induced damage: alleviation of oxidative stress, endoplasmic reticulum stress, and enhancement of autophagy. Int. J. Mol. Sci. 25, 3192. doi:10.3390/ijms25063192
Huang, L., Gao, W., He, X., Yuan, T., Zhang, H., Zhang, X., et al. (2023). Maternal zinc alleviates tert-butyl hydroperoxide-induced mitochondrial oxidative stress on embryonic development involving the activation of Nrf2/PGC-1α pathway. J. Anim. Sci. Biotechnol. 14, 45. doi:10.1186/s40104-023-00852-1
Hui, Q., Yang, N., Xiong, C., Zhou, S., Zhou, X., Jin, Q., et al. (2024). Isorhamnetin suppresses the epithelial-mesenchymal transition of the retinal pigment epithelium both in vivo and in vitro through Nrf2-dependent AKT/GSK-3β pathway. Exp. Eye Res. 240, 109823. doi:10.1016/j.exer.2024.109823
Imran, M., Rauf, A., Abu-Izneid, T., Nadeem, M., Shariati, M. A., Khan, I. A., et al. (2019). Luteolin, a flavonoid, as an anticancer agent: a review. Biomed. Pharmacother. 112, 108612. doi:10.1016/j.biopha.2019.108612
Jia, Y., Sun, L., Yu, H., Liang, L., Li, W., Ding, H., et al. (2017). The pharmacological effects of lutein and zeaxanthin on visual disorders and cognition diseases. Molecules 22, 610. doi:10.3390/molecules22040610
Jiang, C., Chen, Z., Liao, W., Zhang, R., Chen, G., Ma, L., et al. (2024). The medicinal species of the Lycium genus (goji berries) in East Asia: a review of its effect on cell signal transduction pathways. Plants (Basel) 13, 1531. doi:10.3390/plants13111531
Jin, X., Chen, L., Yang, Y., Tan, R., and Jiang, C. (2024). Adverse effects of Nrf2 in different organs and the related diseases. Antioxid. Redox Signal. doi:10.1089/ars.2024.0586
Jung, E., Jung, W. K., Park, S. B., Kim, H. R., and Kim, J. (2020). Aucuba japonica extract inhibits retinal neovascularization in a mouse model of oxygen-induced retinopathy, with its bioactive components preventing VEGF-induced retinal vascular hyperpermeability. Food Sci. Nutr. 8, 2895–2903. doi:10.1002/fsn3.1590
Keenan, T. D. L., Agrón, E., Keane, P. A., Domalpally, A., and Chew, E. Y.Age-Related Eye Disease Study Research Group (2025). Oral antioxidant and lutein/zeaxanthin supplements slow geographic atrophy progression to the fovea in age-related macular degeneration. Ophthalmology 132, 14–29. doi:10.1016/j.ophtha.2024.07.014
Kim, J. S., Jun, J. H., Lee, J., Park, S., Kim, E., Hwang, S. J., et al. (2024). HDAC6 mediates NLRP3 inflammasome activation in the pathogenesis of diabetic retinopathy. Metabolism 164, 156108. doi:10.1016/j.metabol.2024.156108
Kim, M. J., Song, Y. R., Kim, Y. E., Bae, S. J., Lee, W. Y., Bak, S. B., et al. (2023). Kaempferol stimulation of autophagy regulates the ferroptosis under the oxidative stress as mediated with AMP-activated protein kinase. Free Radic. Biol. Med. 208, 630–642. doi:10.1016/j.freeradbiomed.2023.09.008
Kumar, N., Theil, A. F., Roginskaya, V., Ali, Y., Calderon, M., Watkins, S. C., et al. (2022). Global and transcription-coupled repair of 8-oxoG is initiated by nucleotide excision repair proteins. Nat. Commun. 13, 974. doi:10.1038/s41467-022-28642-9
Lee, H. S., and Choi, C. I. (2023). Black goji berry (Lycium ruthenicum murray): a review of its pharmacological activity. Nutrients 15, 4181. doi:10.3390/nu15194181
Li, B., Jiang, H., Li, H., Zhang, B., Slaughter, M., Yan, Z., et al. (2021a). Direct conversion of adult human retinal pigmented epithelium cells to neurons with photoreceptor properties. Exp. Biol. Med. (Maywood) 246, 240–248. doi:10.1177/1535370220963755
Li, B., Vachali, P. P., Shen, Z., Gorusupudi, A., Nelson, K., Besch, B. M., et al. (2017). Retinal accumulation of zeaxanthin, lutein, and β-carotene in mice deficient in carotenoid cleavage enzymes. Exp. Eye Res. 159, 123–131. doi:10.1016/j.exer.2017.02.016
Li, H., Deng, N., He, X., and Li, Y. (2022). Small biomarkers with massive impacts: PI3K/AKT/mTOR signalling and microRNA crosstalk regulate nasopharyngeal carcinoma. Biomark. Res. 10, 52. doi:10.1186/s40364-022-00397-x
Li, H., Huang, M., Luo, Q., Hong, X., Ramakrishna, S., and So, K. F. (2019). Lycium barbarum (wolfberry) increases retinal ganglion cell survival and affects both microglia/macrophage polarization and autophagy after rat partial optic nerve transection. Cell Transpl. 28, 607–618. doi:10.1177/0963689719835181
Li, L., Liu, J., Xu, A., Heiduschka, P., Eter, N., and Chen, C. (2021b). Expression of purinergic receptors on microglia in the animal model of choroidal neovascularisation. Sci. Rep. 11, 12389. doi:10.1038/s41598-021-91989-4
Li, L. H., Lee, J. C. Y., Leung, H. H., Lam, W. C., Fu, Z., and Lo, A. C. Y. (2020a). Lutein supplementation for eye diseases. Nutrients 12, 1721. doi:10.3390/nu12061721
Li, T., Fan, Y., Qin, H., Dai, G., Li, G., Li, Y., et al. (2020b). Transcriptome and flavonoids metabolomic analysis identifies regulatory networks and hub genes in black and white fruits of Lycium ruthenicum murray. Front. Plant Sci. 11, 1256. doi:10.3389/fpls.2020.01256
Li, X., Holt, R. R., Keen, C. L., Morse, L. S., Yiu, G., and Hackman, R. M. (2021c). Goji berry intake increases macular pigment optical density in healthy adults: a randomized pilot trial. Nutrients 13, 4409. doi:10.3390/nu13124409
Li, Y., Cheng, Z., Wang, K., Zhu, X., Ali, Y., Shu, W., et al. (2021d). Procyanidin B2 and rutin in Ginkgo biloba extracts protect human retinal pigment epithelial (RPE) cells from oxidative stress by modulating Nrf2 and Erk1/2 signalling. Exp. Eye Res. 207, 108586. doi:10.1016/j.exer.2021.108586
Li, Y., Cui, H., Li, S., Li, X., Guo, H., Nandakumar, K. S., et al. (2023). Kaempferol modulates IFN-γ induced JAK-STAT signaling pathway and ameliorates imiquimod-induced psoriasis-like skin lesions. Int. Immunopharmacol. 114, 109585. doi:10.1016/j.intimp.2022.109585
Liang, J., Sun, J., Zhang, W., Wang, X., Xu, Y., Peng, Y., et al. (2023). Novel insights into the roles of N6-methyladenosine (m6A) modification and autophagy in human diseases. Int. J. Biol. Sci. 19, 705–720. doi:10.7150/ijbs.75466
Liang, R., Zhao, Q., Zhu, Q., He, X., Gao, M., and Wang, Y. (2021). Lycium barbarum polysaccharide protects ARPE-19 cells against H2O2-induced oxidative stress via the Nrf2/HO-1 pathway. Mol. Med. Rep. 24, 769. doi:10.3892/mmr.2021.12409
Lim, R. R., Thomas, A., Ramasubramanian, A., and Chaurasia, S. S. (2024). Retinal microglia-derived S100A9 incite NLRP3 inflammasome in a Western diet fed Ossabaw pig retina. bioRxiv, 2024, 10.30.621160. doi:10.1101/2024.10.30.621160
Liu, F., Liu, X., Zhou, Y., Yu, Y., Wang, K., Zhou, Z., et al. (2021). Wolfberry-derived zeaxanthin dipalmitate delays retinal degeneration in a mouse model of retinitis pigmentosa through modulating STAT3, CCL2 and MAPK pathways. J. Neurochem. 158, 1131–1150. doi:10.1111/jnc.15472
Liu, G. Y., and Sabatini, D. M. (2020). mTOR at the nexus of nutrition, growth, ageing and disease. Nat. Rev. Mol. Cell Biol. 21, 183–203. doi:10.1038/s41580-019-0199-y
Liu, H., Sun, J., Zou, J., Li, B., and Jin, H. (2023). MeJA-mediated enhancement of salt-tolerance of Populus wutunensis by 5-aminolevulinic acid. BMC Plant Biol. 23, 185. doi:10.1186/s12870-023-04161-7
Liu, J., Li, Y., Pu, Q., Qiu, H., Di, D., and Cao, Y. (2022). A polysaccharide from Lycium barbarum L.: structure and protective effects against oxidative stress and high-glucose-induced apoptosis in ARPE-19 cells. Int. J. Biol. Macromol. 201, 111–120. doi:10.1016/j.ijbiomac.2021.12.139
Liu, L., Zhao, Y., Huang, Z., Long, Z., Qin, H., Lin, H., et al. (2024b). Effects of Lycium barbarum polysaccharides supplemented to high soybean meal diet on immunity and hepatic health of spotted sea bass Lateolabrax maculatus. Front. Immunol. 15, 1333469. doi:10.3389/fimmu.2024.1333469
Liu, Y., Lin, Y., Lin, Y., Lin, C., Lan, G. Y., Su, Y. C., et al. (2024a). Injectable, antioxidative, and tissue-adhesive nanocomposite hydrogel as a potential treatment for inner retina injuries. Adv. Sci. (Weinh) 11, e2308635. doi:10.1002/advs.202308635
Ma, Y., Yang, L., Ma, J., Lu, L., Wang, X., Ren, J., et al. (2017). Rutin attenuates doxorubicin-induced cardiotoxicity via regulating autophagy and apoptosis. Biochim. Biophys. Acta Mol. Basis Dis. 1863, 1904–1911. doi:10.1016/j.bbadis.2016.12.021
Maeda, T., Mandai, M., Sugita, S., Kime, C., and Takahashi, M. (2022). Strategies of pluripotent stem cell-based therapy for retinal degeneration: update and challenges. Trends Mol. Med. 28, 388–404. doi:10.1016/j.molmed.2022.03.001
Mamun, A. A., Shao, C., Geng, P., Wang, S., and Xiao, J. (2024). Polyphenols targeting NF-κB pathway in neurological disorders: what we know so far? Int. J. Biol. Sci. 20, 1332–1355. doi:10.7150/ijbs.90982
Masri, A., Armanazi, M., Inouye, K., Geierhart, D. L., Davey, P. G., and Vasudevan, B. (2024). Macular pigment optical density as a measurable modifiable clinical biomarker. Nutrients 16, 3273. doi:10.3390/nu16193273
Mocan, A., Cairone, F., Locatelli, M., Cacciagrano, F., Carradori, S., Vodnar, D. C., et al. (2019). Polyphenols from Lycium barbarum (goji) fruit European cultivars at different maturation steps: extraction, HPLC-DAD analyses, and biological evaluation. Antioxidants (Basel) 8, 562. doi:10.3390/antiox8110562
Mocan, A., Moldovan, C., Zengin, G., Bender, O., Locatelli, M., Simirgiotis, M., et al. (2018). UHPLC-QTOF-MS analysis of bioactive constituents from two Romanian Goji (Lycium barbarum L.) berries cultivars and their antioxidant, enzyme inhibitory, and real-time cytotoxicological evaluation. Food Chem. Toxicol. 115, 414–424. doi:10.1016/j.fct.2018.01.054
Moldovan, M., Păpurică, A. M., Muntean, M., Bungărdean, R. M., Gheban, D., Moldovan, B., et al. (2023). Effects of gold nanoparticles phytoreduced with rutin in an early rat model of diabetic retinopathy and cataracts. Metabolites 13, 955. doi:10.3390/metabo13080955
Monsalves, M. T., Ollivet-Besson, G. P., Amenabar, M. J., and Blamey, J. M. (2020). Isolation of a psychrotolerant and UV-C-resistant bacterium from elephant island, Antarctica with a highly thermoactive and thermostable catalase. Microorganisms 8, 95. doi:10.3390/microorganisms8010095
Moon, J., Ramkumar, S., and von Lintig, J. (2023). Genetic tuning of β-carotene oxygenase-1 activity rescues cone photoreceptor function in STRA6-deficient mice. Hum. Mol. Genet. 32, 798–809. doi:10.1093/hmg/ddac242
Mosallaei, P., Purola, P., Tolkkinen, L., Gissler, M., and Uusitalo, H. (2025). The incidence of visual impairment due to retinitis pigmentosa has declined in Finland over the last 40 years. Acta Ophthalmol. 103, 85–92. doi:10.1111/aos.16757
Neelam, K., Dey, S., Sim, R., Lee, J., and Au Eong, K.-G. (2021). Fructus lycii: a natural dietary supplement for amelioration of retinal diseases. Nutrients 13, 246. doi:10.3390/nu13010246
Nguyen, B. Q. H., Le, N. T. H., Nguyen, T. Y. N., Nguyen, H. K. T., Yen, C.-H., and Nguyen, M. H. (2024). Hybridisation of in silico and in vitro bioassays for studying the activation of Nrf2 by natural compounds. Sci. Rep. 14, 31222. doi:10.1038/s41598-024-82559-5
Ni, Y., Hu, Y., Zhu, L., Jiang, X., Zhang, H., Liu, J., et al. (2024). Lycium barbarum polysaccharide-derived nanoparticles protect visual function by inhibiting RGC ferroptosis and microglial activation in retinal ischemia‒reperfusion mice. Adv. Healthc. Mater 13, e2304285. doi:10.1002/adhm.202304285
Ola, M. S., Ahmed, M. M., Ahmad, R., Abuohashish, H. M., Al-Rejaie, S. S., and Alhomida, A. S. (2015). Neuroprotective effects of rutin in streptozotocin-induced diabetic rat retina. J. Mol. Neurosci. 56, 440–448. doi:10.1007/s12031-015-0561-2
Ola, M. S., Ahmed, M. M., Shams, S., and Al-Rejaie, S. S. (2017). Neuroprotective effects of quercetin in diabetic rat retina. Saudi J. Biol. Sci. 24, 1186–1194. doi:10.1016/j.sjbs.2016.11.017
Oltra, M., Martínez-Santos, M., Ybarra, M., Rowland, H., Muriach, M., Romero, J., et al. (2022). Oxidative-induced angiogenesis is modulated by small extracellular vesicle miR-302a-3p cargo in retinal pigment epithelium cells. Antioxidants (Basel) 11, 818. doi:10.3390/antiox11050818
Ou, C., Song, H., Peng, J., Xu, J., Zeng, M., Xie, W., et al. (2025). Lycium barbarum L. and Salvia miltiorrhiza Bunge extract ameliorates retinitis pigmentosa in rd10 mice by affecting endoplasmic reticulum stress. J. Ethnopharmacol. 337, 118971. doi:10.1016/j.jep.2024.118971
Ou, K., Li, Y., Liu, L., Li, H., Cox, K., Wu, J., et al. (2022). Recent developments of neuroprotective agents for degenerative retinal disorders. Neural Regen. Res. 17, 1919–1928. doi:10.4103/1673-5374.335140
Palmitessa, O. D., Durante, M., Somma, A., Mita, G., D’Imperio, M., Serio, F., et al. (2022). Nutraceutical profile of “carosello” (cucumis melo L.) grown in an out-of-season cycle under LEDs. Antioxidants (Basel) 11, 777. doi:10.3390/antiox11040777
Pan, R., Ma, J., Kong, X., Wang, X., Li, S., Qi, X., et al. (2019). Sodium rutin ameliorates Alzheimer’s disease-like pathology by enhancing microglial amyloid-β clearance. Sci. Adv. 5, eaau6328. doi:10.1126/sciadv.aau6328
Peshenko, I. V., Olshevskaya, E. V., and Dizhoor, A. M. (2024). Calcium-sensor proteins but not bicarbonate ion activate retinal photoreceptor membrane guanylyl cyclase in photoreceptors. Front. Mol. Neurosci. 17, 1509366. doi:10.3389/fnmol.2024.1509366
Qi, Y., Duan, G., Fan, G., and Peng, N. (2022). Effect of Lycium barbarum polysaccharides on cell signal transduction pathways. Biomed. Pharmacother. 147, 112620. doi:10.1016/j.biopha.2022.112620
Qiu, R., Yang, M., Jin, X., Liu, J., Wang, W., Zhang, X., et al. (2025). AAV2-PDE6B restores retinal structure and function in the retinal degeneration 10 mouse model of retinitis pigmentosa by promoting phototransduction and inhibiting apoptosis. Neural Regen. Res. 20, 2408–2419. doi:10.4103/NRR.NRR-D-23-01301
Radhakrishnan, R., Lor, A., Li, D., Mudaliar, D., and Lobo, G. P. (2024). Methodology for studying interactions of vitamin A membrane receptors and opsin protein with their ligands in generating the retinylidene protein. J. Vis. Exp. 212. doi:10.3791/67036
Ravichandran, S., and Selamoglu, Z. (2023). Anti-inflammatory influences of royal jelly and melittin and their effectiveness on wound healing. Central Asian J. Med. Pharm. Sci. Innovation 3, 38–47. doi:10.22034/CAJMPSI.2023.02.02
Ren, T., Lin, W., He, S., Yang, X., Xian, M., Zhang, Z., et al. (2022). Integrative analysis of metabolomic and transcriptomic data reveals the antioxidant potential of dietary lutein in chickens. Front. Vet. Sci. 9, 906853. doi:10.3389/fvets.2022.906853
Rotenstreich, Y., Belkin, M., Sadetzki, S., Chetrit, A., Ferman-Attar, G., Sher, I., et al. (2013). Treatment with 9-cis β-carotene-rich powder in patients with retinitis pigmentosa: a randomized crossover trial. JAMA Ophthalmol. 131, 985–992. doi:10.1001/jamaophthalmol.2013.147
Rozanowska, M., Edge, R., Land, E. J., Navaratnam, S., Sarna, T., and Truscott, T. G. (2023). Scavenging of cation radicals of the visual cycle retinoids by lutein, zeaxanthin, taurine, and melanin. Int. J. Mol. Sci. 25, 506. doi:10.3390/ijms25010506
Safdari, F., Gholipour, M. D., Ghadami, A., Saeed, M., and Zandi, M. (2022). Multi-antibacterial agent-based electrospun polycaprolactone for active wound dressing. Prog. Biomater. 11, 27–41. doi:10.1007/s40204-021-00176-1
Salehi, B., Mnayer, D., Özçelik, B., Altin, G., Kasapoğlu, K. N., Daskaya-Dikmen, C., et al. (2018). Plants of the genus lavandula: from farm to pharmacy. Nat. Product. Commun. 13, 1934578X1801301037. doi:10.1177/1934578X1801301037
Santhanam, A., Shihabeddin, E., Wei, H., Wu, J., and O’Brien, J. (2023). Molecular basis of retinal remodeling in a zebrafish model of retinitis pigmentosa. Cell Mol. Life Sci. 80, 362. doi:10.1007/s00018-023-05021-1
Selamoglu, Z., Dusgun, C., Akgul, H., and Gulhan, M. F. (2017). In-vitro antioxidant activities of the ethanolic extracts of some contained-allantoin plants. Iran J. Pharma. Res. 16, 92–98.
Selamoglu, Z., Sevindik, M., Bal, C., Ozaltun, B., Sen, İ., and Pasdaran, A. (2020). Antioxidant, antimicrobial and DNA protection activities of phenolic content of Tricholoma virgatum (Fr.) P.Kumm. Biointerface Res. Appl. Chem. 10, 5500–5506. doi:10.33263/BRIAC103.500506
Shahbazi, S., and Zakerali, T. (2022). The inhibitory role of benzo-dioxole-piperamide on the phosphorylation process as an NF-Kappa B silencer. Biomed. Pharmacother. 145, 112471. doi:10.1016/j.biopha.2021.112471
Shao, Y., Yu, H., Yang, Y., Li, M., Hang, L., and Xu, X. (2019). A solid dispersion of quercetin shows enhanced Nrf2 activation and protective effects against oxidative injury in a mouse model of dry age-related macular degeneration. Oxid. Med. Cell Longev. 2019, 1479571. doi:10.1155/2019/1479571
Shi, E., Wang, X., Jing, H., Xu, Y., Feng, L., He, F., et al. (2024). Synergistic effect of chitosan and β-carotene in inhibiting MNU-induced retinitis pigmentosa. Int. J. Biol. Macromol. 268, 131671. doi:10.1016/j.ijbiomac.2024.131671
Singh, M. S., Park, S. S., Albini, T. A., Canto-Soler, M. V., Klassen, H., MacLaren, R. E., et al. (2020). Retinal stem cell transplantation: balancing safety and potential. Prog. Retin Eye Res. 75, 100779. doi:10.1016/j.preteyeres.2019.100779
Staurenghi, F., McClements, M. E., Salman, A., and MacLaren, R. E. (2022). Minicircle delivery to the neural retina as a gene therapy approach. Int. J. Mol. Sci. 23, 11673. doi:10.3390/ijms231911673
Sui, X., Wang, J., Zhao, Z., Liu, B., Liu, M., Liu, M., et al. (2024). Phenolic compounds induce ferroptosis-like death by promoting hydroxyl radical generation in the Fenton reaction. Commun. Biol. 7, 199. doi:10.1038/s42003-024-05903-5
Sule, R. O., Condon, L., and Gomes, A. V. (2022). A common feature of pesticides: oxidative stress-the role of oxidative stress in pesticide-induced toxicity. Oxid. Med. Cell Longev. 2022, 5563759. doi:10.1155/2022/5563759
Sun, X., Liu, Y., Zhou, S., Wang, L., Wei, J., Hua, R., et al. (2022). Circular dorsal ruffles disturb the growth factor-induced PI3K-AKT pathway in hepatocellular carcinoma Hep3B cells. Cell Commun. Signal 20, 102. doi:10.1186/s12964-022-00911-6
Tai, Z., Lin, Y., He, Y., Huang, J., Guo, J., Yang, L., et al. (2014). Luteolin sensitizes the antiproliferative effect of interferon α/β by activation of Janus kinase/signal transducer and activator of transcription pathway signaling through protein kinase A-mediated inhibition of protein tyrosine phosphatase SHP-2 in cancer cells. Cell Signal 26, 619–628. doi:10.1016/j.cellsig.2013.11.039
Takeda, Y., Sato, K., Hosoki, Y., Tachibanaki, S., Koike, C., and Amano, A. (2022). Mathematical analysis of phototransduction reaction parameters in rods and cones. Sci. Rep. 12, 19529. doi:10.1038/s41598-022-23069-0
Tanaka, M., Yamakage, H., Muranaka, K., Yamada, T., Araki, R., Ogo, A., et al. (2022). Higher serum soluble TREM2 as a potential indicative biomarker for cognitive impairment in inadequately controlled type 2 Diabetes without obesity: the DOR-KyotoJ-1. Front. Endocrinol. (Lausanne) 13, 880148. doi:10.3389/fendo.2022.880148
Tang, L., Bao, S., Du, Y., Jiang, Z., Wuliji, A. O., Ren, X., et al. (2018). Antioxidant effects of Lycium barbarum polysaccharides on photoreceptor degeneration in the light-exposed mouse retina. Biomed. Pharmacother. 103, 829–837. doi:10.1016/j.biopha.2018.04.104
Ulasov, A. V., Rosenkranz, A. A., Georgiev, G. P., and Sobolev, A. S. (2022). Nrf2/Keap1/ARE signaling: towards specific regulation. Life Sci. 291, 120111. doi:10.1016/j.lfs.2021.120111
Vučkovski, M., Filipović, A., Jadranin, M., Korićanac, L., Žakula, J., Bondžić, B. P., et al. (2024). Enhanced bioactivity of quercetin-tetrahydroisoquinoline derivatives: effect on lipophilicity, enzymes inhibition, antioxidant potential, and cytotoxicity. Int. J. Mol. Sci. 25, 13076. doi:10.3390/ijms252313076
Wang, C., Zhang, L., Nie, Z., Liang, M., Liu, H., Yi, Q., et al. (2024a). Mutation of CRYAB encoding a conserved mitochondrial chaperone and anti-apoptotic protein causes hereditary optic atrophy. JCI Insight 10, e182209. doi:10.1172/jci.insight.182209
Wang, K., Liu, Y., Li, S., Zhao, N., Qin, F., Tao, Y., et al. (2025). Unveiling the therapeutic potential and mechanisms of stanniocalcin-1 in retinal degeneration. Surv. Ophthalmol. 70, 106–120. doi:10.1016/j.survophthal.2024.08.001
Wang, L., Sun, J., Yin, Y., Sun, Y., Ma, J., Zhou, R., et al. (2021a). Transcriptional coregualtor NUPR1 maintains tamoxifen resistance in breast cancer cells. Cell Death Dis. 12, 149. doi:10.1038/s41419-021-03442-z
Wang, X., Gao, Y., Yuan, Q., and Zhang, M. (2024b). NLRP3 and autophagy in retinal ganglion cell inflammation in age-related macular degeneration: potential therapeutic implications. Int. J. Ophthalmol. 17, 1531–1544. doi:10.18240/ijo.2024.08.20
Wang, Y., Kim, H. J., and Sparrow, J. R. (2017). Quercetin and cyanidin-3-glucoside protect against photooxidation and photodegradation of A2E in retinal pigment epithelial cells. Exp. Eye Res. 160, 45–55. doi:10.1016/j.exer.2017.04.010
Wang, Y., Pan, Y., Liu, Y., Disasa, D., Akira, M., Xiang, L., et al. (2021b). A new geniposidic acid derivative exerts antiaging effects through antioxidative stress and autophagy induction. Antioxidants (Basel) 10, 987. doi:10.3390/antiox10060987
Wang, Y., and Zhang, H. (2019). Regulation of autophagy by mTOR signaling pathway. Adv. Exp. Med. Biol. 1206, 67–83. doi:10.1007/978-981-15-0602-4_3
Welc-Stanowska, R., Pietras, R., Mielecki, B., Sarewicz, M., Luchowski, R., Widomska, J., et al. (2023). How do xanthophylls protect lipid membranes from oxidative damage? J. Phys. Chem. Lett. 14, 7440–7444. doi:10.1021/acs.jpclett.3c01374
Wu, D., Li, J., Fan, Z., Sun, Z., Zheng, X., Zhang, H., et al. (2024a). Dietary Lycium barbarum polysaccharide modulates growth performance, antioxidant capacity, and lipid metabolism in common carp (Cyprinus carpio) fed with high-fat diet. Antioxidants (Basel) 13, 540. doi:10.3390/antiox13050540
Wu, F., Dang, B., Hu, L., Zhu, S., Liu, Z., Cao, X., et al. (2024b). Lycium barbarum polysaccharide inhibits blue-light-induced skin oxidative damage with the involvement of mitophagy. Photochem Photobiol. 100, 604–621. doi:10.1111/php.13863
Xue, C., Yao, Q., Gu, X., Shi, Q., Yuan, X., Chu, Q., et al. (2023). Evolving cognition of the JAK-STAT signaling pathway: autoimmune disorders and cancer. Signal Transduct. Target Ther. 8, 204. doi:10.1038/s41392-023-01468-7
Yan, Z., Lai, M., Jia, Y., Deng, C., and Zhuo, Y. (2022). CircXPO5 plays a neuroprotective function in the lateral geniculate nucleus of glaucoma by regulating GRIN2A. Brain Sci. 12, 780. doi:10.3390/brainsci12060780
Yang, I. H., Lee, J. J., Wu, P. C., Kuo, H. K., Kuo, Y. H., and Huang, H. M. (2020). Oxidative stress enhanced the transforming growth factor-β2-induced epithelial-mesenchymal transition through chemokine ligand 1 on ARPE-19 cell. Sci. Rep. 10, 4000. doi:10.1038/s41598-020-60785-x
Yang, S., Chen, X., Sun, J., Qu, C., and Chen, X. (2022). Polysaccharides from traditional Asian food source and their antitumor activity. J. Food Biochem. 46, e13927. doi:10.1111/jfbc.13927
Yao, R., Heinrich, M., Zhao, X., Wang, Q., Wei, J., and Xiao, P. (2021). What’s the choice for goji: Lycium barbarum L. or L. chinense Mill. J. Ethnopharmacol. 276, 114185. doi:10.1016/j.jep.2021.114185
Yi, Z., Ke, J., Wang, Y., and Cai, K. (2020). Fluvastatin protects myocardial cells in mice with acute myocardial infarction through inhibiting RhoA/ROCK pathway. Exp. Ther. Med. 19, 2095–2102. doi:10.3892/etm.2020.8413
Yin, J., Zhao, D., Song, J., Gao, R., Wang, X., Rao, H., et al. (2024). Synergistic antioxidant activity of Lycium barbarum polysaccharide and chlorogenic acid and its effect on inflammatory response of NR8383 cells. Foods 13, 3696. doi:10.3390/foods13223696
Yu, C., and Xiao, J.-H. (2021). The keap1-nrf2 system: a mediator between oxidative stress and aging. Oxid. Med. Cell Longev. 2021, 6635460. doi:10.1155/2021/6635460
Yu, Y., Wu, X., Pu, J., Luo, P., Ma, W., Wang, J., et al. (2018). Lycium barbarum polysaccharide protects against oxygen glucose deprivation/reoxygenation-induced apoptosis and autophagic cell death via the PI3K/Akt/mTOR signaling pathway in primary cultured hippocampal neurons. Biochem. Biophys. Res. Commun. 495, 1187–1194. doi:10.1016/j.bbrc.2017.11.165
Yu, Z., Xia, M., Lan, J., Yang, L., Wang, Z., Wang, R., et al. (2023). A comprehensive review on the ethnobotany, phytochemistry, pharmacology and quality control of the genus Lycium in China. Food Funct. 14, 2998–3025. doi:10.1039/d2fo03791b
Yun, D., Yan, Y., and Liu, J. (2022). Isolation, structure and biological activity of polysaccharides from the fruits of Lycium ruthenicum Murr: a review. Carbohydr. Polym. 291, 119618. doi:10.1016/j.carbpol.2022.119618
Zapatería, B., and Arias, E. (2024). Aging, cancer, and autophagy: connections and therapeutic perspectives. Front. Mol. Biosci. 11, 1516789. doi:10.3389/fmolb.2024.1516789
Zhang, H., Liu, C., Gu, C., Jiang, J., and Gao, Y. (2024). Kaempferol alleviates injury in human retinal pigment epithelial cells via STAT1 ubiquitination-mediated degradation of IRF7. Front. Biosci. Landmark Ed. 29, 247. doi:10.31083/j.fbl2907247
Zhang, R., Huang, C., Chen, Y., Li, T., and Pang, L. (2022). Single-cell transcriptomic analysis revealing changes in retinal cell subpopulation levels and the pathways involved in diabetic retinopathy. Ann. Transl. Med. 10, 562. doi:10.21037/atm-22-1546
Zhang, Z., Xie, Z., Chen, S., and Zhang, X. (2023). Mitochondrial dysfunction in glaucomatous degeneration. Int. J. Ophthalmol. 16, 811–823. doi:10.18240/ijo.2023.05.20
Zhao, X., Wang, C., Dai, S., Liu, Y., Zhang, F., Peng, C., et al. (2022). Quercetin protects ethanol-induced hepatocyte pyroptosis via scavenging mitochondrial ROS and promoting PGC-1α-regulated mitochondrial homeostasis in L02 cells. Oxid. Med. Cell Longev. 2022, 4591134. doi:10.1155/2022/4591134
Zhong, Q., Sun, W., Qin, Y., and Xu, H. (2023). Association of dietary α-carotene and β-carotene intake with low cognitive performance in older adults: a cross-sectional study from the national health and nutrition examination survey. Nutrients 15, 239. doi:10.3390/nu15010239
Zhou, Z., Gao, Z., Yan, W., Zhang, Y., Huang, J., and Xiong, K. (2021). Adenosine A3 receptor activated in H2O2 oxidative stress of primary open-angle glaucoma. Ann. Transl. Med. 9, 526. doi:10.21037/atm-20-6154
Zhu, D., Xie, M., Gademann, F., Cao, J., Wang, P., Guo, Y., et al. (2020). Protective effects of human iPS-derived retinal pigmented epithelial cells on retinal degenerative disease. Stem Cell Res. Ther. 11, 98. doi:10.1186/s13287-020-01608-8
Zi, D., Li, Q., Xu, C., Zhou, Z., Song, G.-B., Hu, C., et al. (2022). CXCR4 knockdown enhances sensitivity of paclitaxel via the PI3K/Akt/mTOR pathway in ovarian carcinoma. Aging (Albany NY) 14, 4673–4698. doi:10.18632/aging.203241
Ziółkowska, N., and Lewczuk, B. (2022). Profiles of Rho, Opn4, c-Fos, and Birc5 mRNA expression in Wistar rat retinas exposed to white or monochromatic light. Front. Neuroanat. 16, 956000. doi:10.3389/fnana.2022.956000
Zou, Y., Jiang, J., Li, Y., Ding, X., Fang, F., and Chen, L. (2024). Quercetin regulates microglia M1/M2 polarization and alleviates retinal inflammation via ERK/STAT3 pathway. Inflammation 47, 1616–1633. doi:10.1007/s10753-024-01997-5
Glossary
4-HNE 4-hydroxynonenal
Akt Protein kinase
AMD Age-related macular degeneration
ARE antioxidant response elements
ATP Adenosine triphosphate
Bcl-2 B-cell lymphoma-2
BDNF Brain-derived neurotrophic factor
DR Diabetes retinopathy
ERK1/2 Extracellular signal-regulated protein kinases 1 and 2
GPX4 Glutathione peroxidase 4
GSH Glutathione
HO-1 Heme oxygenase 1
ICAM-1 Intercellular adhesion molecule-1
IL-1 Interleukin-1
IL-12 Interleukin-12
IL-1β Interleukin-1 beta
IL-6 Interleukin-6
IκB Inhibitor of NF-κB
JAK Janus kinase
KEAP1 Kelch-like ECH-associated protein 1
LBP Lycium barbarum polysaccharides
MDA Malondialdehyde
MMP-9 Matrix metalloproteinase-9
MnSOD Manganese superoxide dismutase
mTOR Mammalian target of rapamycin
NF-κB Nuclear factor kappa-B
NGF Nerve growth factor
NRF2 Nuclear factor erythroid 2-related factor 2
PI3K Phosphatidylinositol 3-kinase
PIP2 Phosphatidylinositol 4,5-bisphosphate
PIP3 Phosphatidylinositol 3,4,5-trisphosphate
ROS Reactive oxygen species
RP Retinitis pigmentosa
RPE Retinal pigment epithelial
SOD Superoxide dismutase
STAT Signal transducer and activator of transcription
TCM Traditional Chinese medicine
TNF-α Tumor necrosis factor-alpha
TrxR1 Thioredoxin reductase 1
ULK1/2 Unc-51 like kinase 1/2
Keywords: Lycium barbarum L., retinal cells, apoptosis, retinitis pigmentosa, agerelated macular degeneration
Citation: Xiong M, Peng J, Zhou S, Gao Q, Lu J, Ou C, Song H and Peng Q (2025) Lycium barbarum L.: a potential botanical drug for preventing and treating retinal cell apoptosis. Front. Pharmacol. 16:1571554. doi: 10.3389/fphar.2025.1571554
Received: 05 February 2025; Accepted: 03 March 2025;
Published: 20 March 2025.
Edited by:
Ruyu Yao, Chinese Academy of Sciences (CAS), ChinaReviewed by:
Zeliha Selamoglu, Niğde Ömer Halisdemir University, TürkiyeJianhui Xie, Guangdong Provincial Hospital of Chinese Medicine, China
Yan Chen, Hangzhou Normal University, China
Copyright © 2025 Xiong, Peng, Zhou, Gao, Lu, Ou, Song and Peng. This is an open-access article distributed under the terms of the Creative Commons Attribution License (CC BY). The use, distribution or reproduction in other forums is permitted, provided the original author(s) and the copyright owner(s) are credited and that the original publication in this journal is cited, in accordance with accepted academic practice. No use, distribution or reproduction is permitted which does not comply with these terms.
*Correspondence: Houpan Song, c29uZ2hwQGhudWNtLmVkdS5jbg==; Qinghua Peng, cHFoNDEwMDA3QDEyNi5jb20=
†These authors have contributed equally to this work and share first authorship