- 1Institute of Chinese Medicine, Heilongjiang University of Traditional Chinese Medicine, Harbin, Heilongjiang, China
- 2The Four Hospital of Heilongjiang University of Traditional Chinese Medicine, Harbin, Heilongjiang, China
Background: Essential oils, often referred to as “liquid gold,” are renowned for their broad biological activity. Ancient Egyptians used essential oils’ antibacterial and antiseptic effects to preserve mummies, ancient Greeks used olive oil for sun protection, and ancient Chinese used essential oils to treat wounds. When essential oils are applied to the facial skin, their potent anti-inflammatory, antioxidant, and antibacterial pharmacological characteristics provide various benefits, including sunscreen, skin-whitening, and anti-aging effects.
Purpose: This paper aims to summarize the application of plant essential oil in skin whitening, anti-inflammatory, antioxidant and antibacterial in recent years, and deeply analyzes the internal relationship between essential oil and modern drug delivery system, expounds how to overcome the limitations of essential oil through specific drug delivery system, to enhance its biological activity and stability, realize sustained release and reduce its potential toxicity, and also discusses the positive effects of essential oil on brain function through olfactory pathway, emphasizes the possible safety risks in the use of essential oil, and puts forward corresponding suggestions for use.
Methods: Using keywords such as “essential oils,” “antioxidant,” “anti-tyrosinase,” Antibacterial Effects and anti-inflammatory,” “anti-anxiety,” and “drug carrier delivery systems,” a comprehensive search was conducted in the PubMed, CNKI, Baidu, and Wanfang databases to summarize articles from the past 5 years. Further screening was performed to select studies demonstrating the efficacy of essential oils through topical or external application.
Results: Various essential oils showed their efficacy as strong oxidants, antibacterial agents, anti-inflammatory agents, and skin-whitening agents. Combined with a new drug delivery system, it not only enhances the biological activity of essential oil but also reduces the inherent defects of essential oil, such as volatility, irritation, and toxicity, and has a targeted delivery effect. At the same time, the integration of essential oil into skin care products can make use of the dual functions of smell and the epidermal system to nourish and repair the skin and maximize the pharmacological effects of essential oil.
Conclusion: This review delves into the application of essential oils and delivery systems, advocating for a broader integration of natural plant resources with modern technology. By strategically utilizing essential oils, we can promote the sustainable development of the global economy. However, extensive clinical trials are still required to evaluate the effectiveness and safety of essential oil delivery systems.
1 Introduction and historical uses of essential oils in skincare
Essential oils (EOs) are concentrated plant extracts obtained through processes such as steam distillation (Kapadia et al., 2022). They are typically extracted from the leaves, flowers, skins, roots, seeds, pericarps, and other parts of aromatic plants and consist of a variety of volatile chemical metabolites, such as terpenes, esters, alcohols, and aldehydes (Bolouri et al., 2022). Owing to their diverse biological activities, environmental friendliness, and ease of acquisition, EOs have long been used in various traditional medicine approaches (Khalil et al., 2021).
EO applications date back thousands of years to ancient civilizations such as Egypt, Arabia, Greece, and China (Long et al., 2022). In ancient Egypt, EOs with antibacterial and preservative properties, such as Boswellia sacra Flück., Commiphora myrrha (T.Nees) Engl., and Cinnamon (Cinnamomum verum J. Presl), were used to embalm bodies during mummification (Vora et al., 2024). Similarly, Lavender (Lavandula angustifolia Mill.) was used in traditional medicine across Asia, Europe, ancient Greece, and Rome, and is mentioned in both the Bible and ancient Jewish texts for its anti-inflammatory and antibacterial effects (Hancianu et al., 2013). The ancient Romans applied oils, including EOs from Clove (Syzygium aromaticum (L.) Merr. and L.M.Perry) and Lavender, on their skin for fragrance, whereas the Greeks used olive oil as sunscreen (Liu and Zhang, 2017). Monoï oil, widely used among the Tahitian tribes in Polynesia for skincare, is primarily applied to hydrate the skin and safeguard it against sun exposure (McMullen and Dell’Acqua, 2023). In 1919, French chemist René-Maurice Gattefossé accidentally burned his hand during a laboratory explosion. Subsequently, he used Lavender EO to treat the infected wound and discovered its remarkable healing effects. This discovery has prompted researchers to conduct in-depth research on the medicinal value of EOs and promote the wide application of EOs in medical and skin care fields.
This paper summarizes the latest research findings and academic perspectives on the applications of plant EOs in skin whitening, anti-inflammatory, antioxidant, and antibacterial treatments, along with a brief introduction to the structure and function of the skin. It provides an in-depth analysis of the intrinsic relationship between EOs and drug delivery systems, elucidating how specific drug delivery systems can overcome the limitations of EOs. Additionally, the paper discusses the positive effects of EOs on brain function through the olfactory pathway and highlights potential safety risks associated with their use, offering corresponding recommendations. These insights provide valuable references for further research.
2 Constitution of the skin
The skin serves as the body’s first line of protection against harmful external stimuli and performs several crucial functions. First, it prevents excessive water loss, thereby preserving the body’s hydration balance (Afshari et al., 2024). Secondly, the skin acts as an effective barrier against toxins and infections. As shown in Figure 1, facial skin primarily comprises the epidermis, dermis, and subcutaneous tissue (Nascimento et al., 2024). Keratinocytes within the epidermis play a key role in forming the skin’s protective barrier and help distribute melanin produced by melanocytes. Melanocytes in the basal layer of the epidermis are responsible for melanin synthesis and influence skin color. EOs can interact with stratum corneum proteins, causing conformational changes that disrupt the lipid phase, thereby weakening the skin barrier. This process promotes the transdermal delivery of both hydrophobic and hydrophilic metabolites, enhances the deep penetration of active metabolites, and improves the anti-aging and whitening properties of products (McMullen and Dell’Acqua, 2023; Yang et al., 2024a). Below the epidermis lies the dermis, which is rich in collagen and elastic fibers that provide skin elasticity and firmness, maintaining a youthful appearance. The subcutaneous layer, comprising fatty tissue, lymphatic vessels, nerves, and blood vessels, provides nourishment and support. Moreover, the skin plays a pivotal role in metabolic processes, material absorption, and protection against biological, physical, and chemical threats (Michalak et al., 2021). In summary, the skin is not only a protective barrier but also an organ essential for maintaining overall physical health and beauty.
3 Pharmacological activities of essential oils
3.1 Whitening effect of essential oils
Melanin, the primary pigment in human hair, eyes, and skin, is synthesized in melanosomes within melanocytes (Yu et al., 2024). Tyrosinase (TYR) is a vital copper-containing metalloenzyme crucial for melanin synthesis in mammals (Ma and Lu, 2020). In melanocytes, tyrosine undergoes a series of oxidation processes mediated by TYR and other enzymes. Specifically, tyrosinase-related protein (TRP) converts dopachrome into 5,6-dihydroxy indole-2-carboxylic acid (DHICA), while TRP-1 facilitates the conversion of DHICA to indole-5,6-quinone-2-carboxylic acid, ultimately resulting in melanin production. The microphthalmia-associated transcription factor (MITF) plays a pivotal role in regulating the expression of TYR, TRP-1, and TRP-2, thereby significantly impacting melanin synthesis. Furthermore, MITF is activated through Mitogen-Activated Protein Kinase (MAPK) signaling cascades, which stimulate melanogenesis in response to diverse external stimuli (Kim et al., 2021; Yang et al., 2023a). This process is summarized in Figure 2. The synthesized melanin is subsequently released from melanocytes into the intercellular space as pigment particles and gradually transferred to the cytoplasm of surrounding keratinocytes, ultimately reaching the stratum corneum. While an appropriate amount of melanin protects the skin from detrimental environmental factors (He et al., 2023), an excess can lead to pigmentation issues, a dull skin tone, and spot development (Nurani et al., 2023).
Kojic acid, arbutin, ascorbic acid, and hydroquinone (Draelos et al., 2020; Mahalapbutr et al., 2023; Zahoor et al., 2023) are well-known chemical inhibitors of TYR. However, their use can pose potential health risks. Since 2001, hydroquinone has been excluded from skincare products due to cancer risks associated with continuous exposure (Zolghadri et al., 2023). Kojic acid, while effective for skin whitening, is limited by its carcinogenic potential and instability during storage (Liu et al., 2024a). When arbutin is applied to the skin, microbes or ultraviolet radiation (UVR) can convert it into hydroquinone (Boo, 2021). Conversely, plants are increasingly valued in skin-whitening cosmetics, with up to 3/5 of products in medical skincare deriving from plant-specific metabolic metabolites (Capetti et al., 2021). Plant extracts and metabolites have demonstrated efficacy in preventing excessive melanin formation in the epidermis (Kanlayavattanakul and Lourith, 2018). For instance, in vitro experiments utilizing EO from Echinophora chrysantha Freyn and Sint. Have demonstrated an inhibitory effect on TYR activity, subsequently affecting melanin synthesis and reducing its content (Kanbolat et al., 2024). In vivo experiments on zebrafish have demonstrated that Boswellia papyrifera (Caill. Ex Delile) Hochst. EO can effectively inhibit melanin production, with no significant toxic effects observed within the tested concentration range (Yan et al., 2024). Table 1 summarizes plant EOs that exert skin-whitening effects by inhibiting the expression of melanin, TYR, or TRP through in vivo or in vitro experiments.
3.2 Anti-aging effect of essential oils
The aging of facial skin is a progressive process, resulting from the interplay between internal aging factors and external influences (Carvalho et al., 2023; Liu et al., 2024c). Signs such as increased wrinkles, reduced firmness and elasticity, dry keratinization, and excessive pigment deposition indicate a loss of skin suppleness due to aging (Banov et al., 2023). Prevailing theories on skin aging highlight the roles of free radicals (Harman, 1956) and photoaging (Fisher et al., 1996). The antioxidant activity of EOs efficiently counteracts free radicals, thereby slowing the aging process (Maache et al., 2023). EOs also absorb UV rays, reducing skin damage and promoting the repair and regeneration of skin cells.
3.2.1 Free radical theory
Harman introduced the free radical aging hypothesis in 1954, suggesting that both internal and external oxidants and free radicals damage cells, altering their structure and function (Harman, 1956). External factors, such as light, radiation, and heavy metals (Chen et al., 2023a; Xie et al., 2020), as well as normal cell metabolism, produce free radicals. Increased free radical levels accelerate aging and contribute to aging-related diseases (Tamagno et al., 2022). Normally, the body’s antioxidant system mitigates free radicals to prevent their accumulation (Di Meo and Venditti, 2020). However, with age, the body’s defenses weaken, leading to an abnormal accumulation of free radicals, particularly reactive oxygen species (ROS). Excess ROS reacts with unsaturated fats, creating lipid peroxides (LPO) through lipid peroxidation (Zhou et al., 2024), thereby damaging cellular membranes and organelles. This process contributes to cell damage, necrosis, aging, and an increased risk of disease. High free radical levels can also cause protein breakdown, DNA mutations, and potentially cancer (Bakheit et al., 2024) (Figure 3).
Synthetic antioxidants, such as butylated hydroxyanisole and 2,6-di-tert-butyl-p-cresol, are widely utilized in the food and skincare sectors (Xu et al., 2021). However, research suggests that they may increase the risk of cancer (Riaz et al., 2023). Consequently, EOs have garnered remarkable interest due to their superior antioxidant properties and safety profile. They contain various antioxidant metabolites that effectively eliminate excess free radicals, donate electrons or hydrogen, and inhibit lipid peroxidation, thereby preventing skin damage and aging. Notably, the anti-aging effects of EOs are achieved by inhibiting free radical-driven signal transduction (Guo et al., 2021). Table 2 summarizes EOs with antioxidant properties and briefly presents the relevant experimental results.
3.2.2 Photoaging theory
The skin, which covers approximately 1.5–2 m2 of the human body, serves as its primary physiological barrier (Xu et al., 2023) and encounters external stimuli such as UVR, smoke, and harmful pollutants (Ge et al., 2024). UVR, particularly UVA (wavelength range 320–400 nm) and UVB (wavelength range 290–320 nm) (Gęgotek et al., 2017), is a major exogenous factor contributing to skin aging (Yang et al., 2023b). Prolonged UVR exposure can lead to hyperpigmentation, skin laxity, severe wrinkling, and an increased risk of skin cancer (Kim et al., 2023; Liu et al., 2024b). UVR also triggers skin inflammation, increasing ROS levels that activate signaling in keratinocytes and fibroblasts, thereby upregulating matrix metalloproteinases (MMPs) (Sim et al., 2023). These proteolytic enzymes degrade collagen, elastin, and other connective tissue proteins, disrupt the extracellular matrix, and inhibit new collagen synthesis (Apte and Parks, 2015). Specifically, UVR exposure enhances the activity of MMPs—such as collagenase, 92-kd gelatinase, and stromelysin—in the skin (Birkedal-Hansen, 1987). As a result, these enzymes accelerate the breakdown of endogenous type I collagen fibers by up to 58%, thereby accelerating the skin aging process. Moreover, adverse skin reactions from the overuse of chemicals in sunscreens are becoming more common (Messias et al., 2023). Therefore, identifying natural and effective sunscreen metabolites is critical. EOs have shown significant potential in sun protection due to their ability to absorb UV radiation while scavenging free radicals, ultimately reducing photodamage and delaying skin aging. The EOs listed in Table 3 are renowned for their sun protection efficacy and demonstrate significant potential for natural skincare applications.
3.3 Antibacterial effects of essential oils
Skin is not only a physical barrier isolating the body from the outside world but also a habitat for various microorganisms (Dessinioti and Katsambas, 2024). An imbalance in the skin microbial community can lead to diseases such as acne, atopic dermatitis, and eczema. Many chemical preservatives (phenoxyethanol, methyl p-hydroxybenzoate [p-HBA], butyl p-hydroxybenzoate [BuP], chlorobenzyl ether, and sodium benzoate [SB]) are added to skincare products to prevent microbial contamination during production, storage, and use, and to prolong shelf life (Alshehrei, 2024; Głaz et al., 2023). However, they cannot protect the skin from bacterial pollution and may be harmful to users. For instance, p-HBA can impact nervous system development in zebrafish (Merola et al., 2024) and reduce male fertility (Shen et al., 2023). BuP is hepatotoxic in aquatic animals (Yin et al., 2023), negatively affects heart development (Zhu et al., 2023), and inhibits neural crest cell proliferation in zebrafish larvae, causing craniofacial deformities (Li et al., 2023b). Additionally, high concentrations of SB may be toxic to insects (Asejeje et al., 2024). The accumulation of these chemical preservatives on the skin may promote aging and pigmentation. Excessive or improper use of chemical preservatives poses risks to human health and the environment (Nowak-Lange et al., 2022). With increasing awareness of health and environmental protection, the reliance on chemical preservatives in skincare is a growing concern (Jafarizadeh-Malmiri et al., 2022). Plant EOs, with their natural composition, favorable safety profile, and potent antibacterial properties, are frequently utilized as alternatives to chemical preservatives (Cunha et al., 2022). As lipophilic substances, EOs can disrupt lipids and proteins, increase membrane permeability, and cause leakage of cellular contents (Álvarez-Martínez et al., 2021) (Figure 4). For instance, in vitro studies show that Lemon verbena EOs can damage the cell walls of cultivated yellow croakers, increasing membrane permeability and causing leakage of cellular contents, ultimately leading to cell death (Gao et al., 2023). The antibacterial properties of plant EOs hold great potential for use as natural preservatives (Table 4 summarizes the related EOs).
3.4 Anti-inflammatory effect of essential oils
Inflammation is a complex defense mechanism that counteracts harmful external stimuli or abnormal internal signals. The immune system plays a central role in this process by eliminating the cause of disease and preserving the structural integrity of cells and tissues to maintain homeostasis. When the skin experiences irritation, bacterial infection, or damage, an inflammatory response is triggered. While moderate inflammation aids in repairing skin damage, excessive inflammation can lead to chronic skin diseases such as dermatitis, rosacea, and acne vulgaris. During this process, immune cells, including T cells, B cells, and dendritic cells, secrete inflammatory factors, most notably cytokines. Following skin damage, keratinocytes also become activated. The recognition and binding of specific pathogens or damaging factors to the pattern recognition receptor TLR (toll-like receptor)4 on the cell membrane triggers the release of inflammatory substances and subsequent immune cell activation (de Sousa et al., 2023; Jiang et al., 2020; Li et al., 2024a; Piipponen et al., 2020; Zhang et al., 2024a) (Figure 5). Current primary treatments for inflammation include corticosteroids, NSAIDs, and biologicals. However, drug resistance compromises their efficacy, necessitating novel therapeutic approaches (Russo et al., 2024). EOs exhibit potent anti-inflammatory properties by reducing capillary permeability and inhibiting both inflammatory cell activation and cytokine release. Thus, EOs are increasingly used as alternatives to traditional drugs for treating inflammation (Shen et al., 2017). For example, adapalene (ADA) is a commonly used gel for treating acne. When a tea tree EO nanoemulsion was combined with ADA, it produced a stronger therapeutic effect than ADA alone in clinical experiments, without increasing adverse effects (Najafi-Taher et al., 2022). Another clinical study revealed that adding 3% kānuka EOs to cream significantly improved eczema to a greater extent than the vitamin C control (Shortt et al., 2022). Table 5 summarizes EOs and their anti-inflammatory properties.
4 Challenges in essential oil application
In summary, EOs have unique biological activity and can be used in medicine, food, and skin care products. However, their high volatility and lipophilicity compromise stability during storage and use, particularly upon exposure to light, heat, O2, and UVR. Such exposure can diminish the active metabolites of EOs, thereby decreasing their efficacy. More critically, EOs may produce oxidation and isomerization products that are highly toxic and allergenic (Yi et al., 2022). For instance, the main component of anise, Clove, Cinnamomum verum J. Presl, and thyme EOs, trans-anethole, isomerizes into cis-anethole under ultraviolet light or high temperatures. Additionally, trans-anethole can be completely oxidized into benzaldehyde or isomerized into cis-anethole after being stored in light for 2 months at room temperature. Studies have shown that cis-anethole may cause stronger skin irritation, such as erythema, itching, and contact dermatitis, and increase the risk of allergic reactions (Castro et al., 2010). Additionally, a patch test conducted by Geier et al. on 12 EOs in 10,930 dermatitis patients revealed that 908 patients (8.3%) reacted to at least one EO. Among these, only six EOs (ylang-ylang, lemongrass, jasmine, sandalwood, Clove, and neroli) elicited positive patch test reactions in more than 1% of the patients (Geier et al., 2022). Although sensitization reactions to EOs do occur, they are generally uncommon. Nonetheless, safety concerns in practical applications should not be overlooked.
5 Essential oil’s drug delivery system
In summary, the volatility, instability, poor solubility, and skin irritation of EOs lead to uneven curative effects and low bioavailability, making it impractical to use them alone. However, advances in natural plant research and the rapid development of drug delivery systems offer new solutions to these issues. A drug delivery system can effectively prevent the degradation of EOs by encapsulating them in or adsorbing them onto carriers, thereby improving their stability and bioavailability. These systems also allow controlled and targeted release, enabling EOs to act on the target area more accurately and effectively, reduce the toxicity, and further expand their application scenarios (Figure 6) (Chhetri et al., 2024; Lim et al., 2023; Ye et al., 2024).
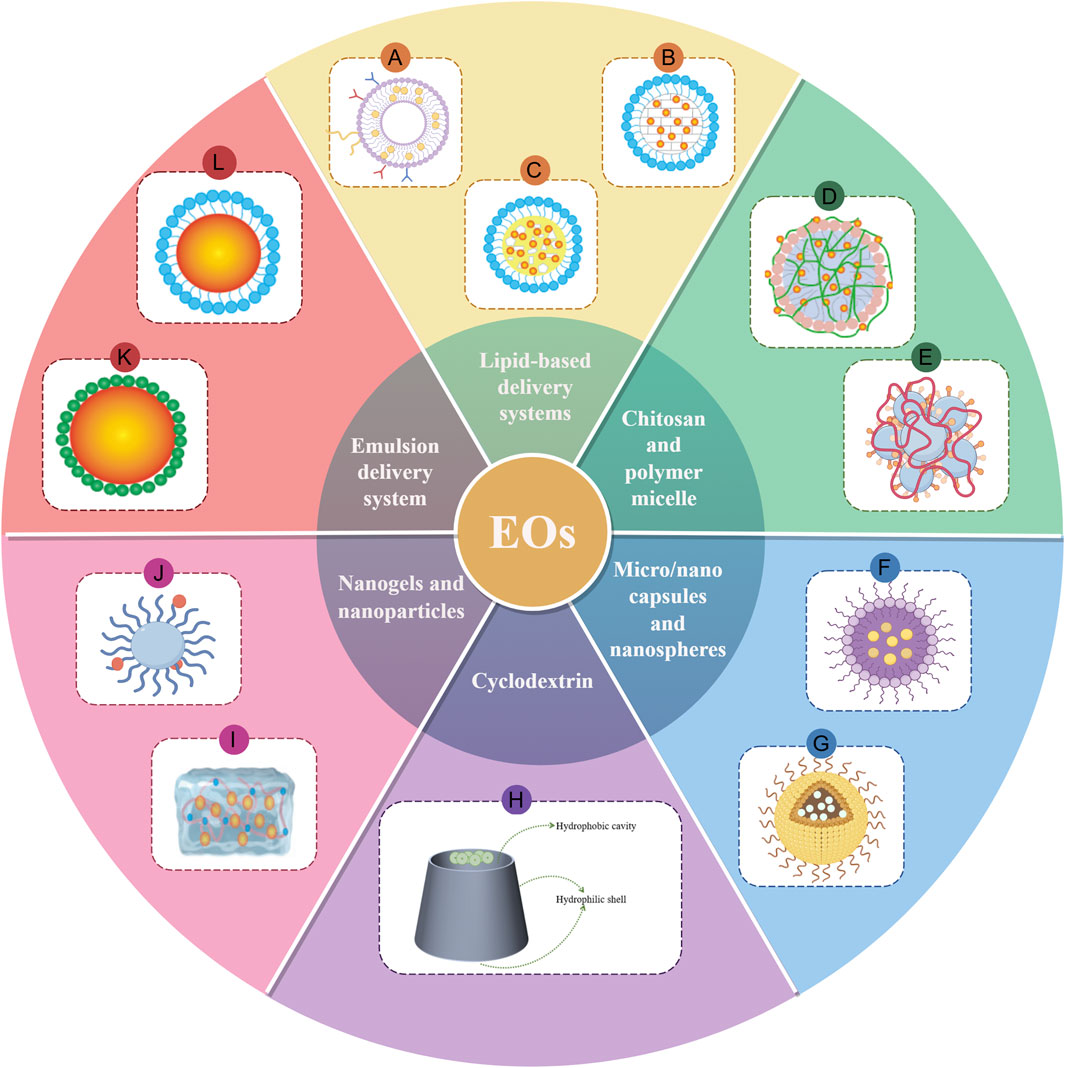
Figure 6. Summary of Different drug delivery system for EOs. Note: A, liposome; B, solid lipid nanoparticles; C, nanostructured lipid carrier; D, chitosan; E, polymer micelle; F, micro/nano capsules; G, nanospheres; H, cyclodextrin; I, nanogel; J, nanoparticles; K, Pickering emulsion; L, nano/micron emulsion. (By Figdraw).
5.1 Lipid-based delivery systems
5.1.1 Liposomes (LS)
LS are vesicular structures that spontaneously assemble from one or more phospholipid bilayers surrounding an aqueous core, capable of encapsulating hydrophilic molecules within their internal aqueous phase and hydrophobic molecules within the phospholipid bilayers (Bedoya-Agudelo et al., 2024). LS, as the most mature nano-drug carrier, effectively addresses the instability and low solubility of EOs caused by external factors (such as reduced bioavailability) through the integration of EOs with LS technology (Hedayati et al., 2024). For instance, embedding Origanum Vulgar L. EO into LS can significantly enhance antioxidant activity and increase cytotoxicity against MCF-7 cancer cells (Kryeziu et al., 2022). Nanoliposomes (NLS) are LS with diameters typically under 200 nm, suited for high-precision targeting and controlled-release applications, such as local dermatological treatments and cancer therapy. Ellboudy et al. (2023) doped Cinnamon EO into an NLS formula to enhance its stability, thereby extending the release time and improving its antibacterial activity.
However, LS has limitations, such as poor repeatability, uneven particle size, and remarkable lipid oxidation. Consequently, the development of new carriers based on LS is on the rise. For instance, Baldim incorporated CD in LS to deliver Lippia sidoides Cham. and Syzygium aromaticum (L.) EO, finding that the EOs-in-CD-in-LS systems retained volatile metabolites and increased their physicochemical stability (Baldim et al., 2022). Similarly, Gan et al. (2024) enhanced NLS using the layer-by-layer electrostatic deposition method with chitosan and three anionic polymers (pectin, gum arabic, and carrageenan) as the first and second coating polymers, respectively, to encapsulate lemongrass EO, resulting in improved EO stability.
5.1.2 Solid lipid nanoparticles (SLNs)
SLNs represent a novel colloidal drug delivery system that offers better stability compared to traditional LS. Comprised of three main metabolites—active metabolites (or drugs), solid lipids, and surfactants (Subroto et al., 2023)—SLNs utilize commonly biocompatible substances such as mono-, di-, and triglycerides, fatty acids, fatty alcohols, and waxes for the lipid core (Llaguno-Munive et al., 2024). The non-toxic and non-irritating nature of these lipids makes SLNs particularly suitable for injured or inflamed skin (Safta et al., 2024). Using a nanostructure design, EOs are encapsulated in SLNs as submicron capsules or nanoparticles, considerably enhancing the stability and bioavailability of the oils (AbouAitah and Lojkowski, 2022). For instance, Fuentes et al. (2024) developed an SLN delivery system based on Mentha × piperita L. EO and evaluated its thermal stability. The SLNs markedly reduced the evaporation loss of active metabolites in the EO, exhibited excellent thermal stability at 50°C and boosted the EO’s antibacterial properties. Additionally, SLNs can enhance the therapeutic efficacy of EOs. Due to their lipophilic nature and ease of penetration through the bacterial cell wall, SLNs can facilitate the delivery of Cinnamon EO across the cellular membrane, thereby enhancing its antibacterial activity against E. coli (Nemattalab et al., 2022). Similarly, Kotb et al. (2023) prepared Boswellia sacra Flück. EO into SLNs (FO-SLNs) via high-shear homogenization and assessed their anti-photoaging activity through in vivo experiments, demonstrating that FO-SLNs provided enhanced protection against UVB-induced epidermal thickening, dermal collagen degradation, and a decrease in inflammatory cell numbers compared to pure EO alone. While SLNs effectively protect EOs and improve their stability, they also present challenges such as complex production, high costs, and stability issues.
5.1.3 Nanostructured Lipid Carriers (NLCs):
NLCs are second-generation lipid nanoparticles based on SLNs, formed from a mixture of solid lipids (cocoa, murumuru butter, and beeswax) and liquid lipids (olive oil or sesame oil). The presence of liquid lipids creates larger cavities within the solid lipids, ensuring a high drug-loading capacity and enhanced solubility of active metabolites. Importantly, the active metabolites rarely leak during storage (Cimino et al., 2021; Uchida et al., 2021). For instance, using NLCs to encapsulate Lippia sidoides Cham. and Caryophyllus aromaticus L. EOs results in a high encapsulation efficiency of the marker compound, ranging from 84.6% to 100%, significantly enhancing the therapeutic efficacy of EOs (Almeida et al., 2024). Pires et al. prepared seven types of NLCs; one formulation composed of Geranium EO and beeswax allowed storage for 210 days at 25°C. Meanwhile, NLCs prepared with encapsulated lemongrass EOs using murumuru butter, Cinnamon EOs with cocoa butter, Clove EOs, and Origanum vulgare L. EOs with beeswax demonstrated that encapsulation can improve the bioavailability, stability, and biocompatibility of EOs while reducing their photodegradation and toxicity (Pires et al., 2024).
In the EO-NLC system, liquid lipids may consist solely of EOs or be combined with additional liquid lipids to improve EO stability and enhance therapeutic efficacy. For instance, Santos Pimentel et al. (2024) prepared NLCs using Cinnamon, sage, and thyme as liquid matrices, combined with other solid matrices and surfactants. Experimental results demonstrated improved stability of all developed EO-based NLC formulations, while bioactivity remained unchanged. Satrialdi et al. (2024) prepared a Clove EO-loaded NLC (COE-NLC) via an emulsification-ultrasonication process, achieving an encapsulation efficiency of 97% and good stability. Furthermore, compared to free COE, COE-NLC exhibited enhanced free radical scavenging activity and effectively protected fibroblast cells from oxidative stress. The capacity of NLCs for EO loading is greater than that of LS and SLNs, although the use of surfactants in the preparation process is inevitable.
5.2 Emulsion delivery system
5.2.1 Microemulsion (ME)
ME is a clear, uniform liquid mixture composed of a co-surfactant, water phase, oil phase, and surfactant. MEs can be categorized as O/W, W/O, or bicontinuous types. The nanoscale droplets created by surfactants in ME weaken the skin’s barrier function, making it suitable for transdermal drug delivery. The oil phase can improve drug absorption efficiency at the site of action, and using plant EOs, which dissolve the stratum corneum, as the oil phase further enhances ME permeability (Chen et al., 2024; Thakur et al., 2021). Wang et al. synthesized a ME of Matricaria recutita L. EO (MRME) using the phase inversion emulsification method and examined its anti-inflammatory and eczema-treating properties. Their findings indicated that MRME improved EO stability, lowered irritancy, and maintained anti-inflammatory and eczema-treating effectiveness (Wang et al., 2024a). Meanwhile, an ME loaded with Torreya grandis Fortune ex Lindl. EO (TaEO) showed higher stability and enhanced biological activity than pure TaEO (Wang et al., 2023a). Encapsulating Lavender, Basil, and Clove EOs using an ME, antibacterial experiments demonstrated more effective activity against S. aureus and E. coli at lower concentrations than those of pure EOs (Manzoor et al., 2023). Although ME significantly improves the efficacy of EOs, it has certain limitations, such as low encapsulation efficiency, poor stability, the use of surfactants during preparation, and residual organic solvents.
5.2.2 Nanoemulsion (NE)
NEs are emulsion or colloidal dispersion systems composed of two immiscible liquids formed by surfactants, oil, and water, resulting in oil-in-water (O/W) or water-in-oil (W/O) types. Due to their high stability and greater resistance to environmental factors, NEs have found increased application in the food, cosmetics, and pharmaceutical industries. They enhance the stability, solubility, and bioavailability of active metabolites while producing a sustained-release effect (Huang et al., 2024a; Pandey et al., 2023; Shahabi et al., 2024; Sharma et al., 2024b). Sun et al. prepared an NE of Litsea cubeba (Lour.) Pers. EO (LEON) using ultrasonic emulsification; experimental data indicated that LEON significantly enhances the antibacterial efficacy and antioxidant capacity of the EO (Sun et al., 2024). Moreover, NE can also improve the antibacterial effect of EOs. For example, the NE of Callistemon citrinus (Curtis) Skeels EO exhibits 15 times the antibacterial activity against E. coli compared to pure EO and remains stable for up to 6 months (Haghbayan et al., 2024). Ling et al. prepared traditional emulsions (BDT) and NE (Bneo) of Illicium verum Hook. f. EO using ultrasonic methods; antibacterial results showed that the antifungal activity of Bneo against Fusarium proliferatum was 5.8 times higher than that of BDT, with good stability (Ling et al., 2024). However, the process inevitably requires surfactants and faces challenges related to thermodynamic instability and high production costs.
5.2.3 Pickering Emulsion (PE)
Compared to emulsions traditionally stabilized with surfactants, PEs utilize solid particles to stabilize oil–water mixtures, including both O/W and W/O types. The stabilizing principle involves creating a solid barrier around the oil droplets, effectively isolating the oil phase from the water phase. The particles used as stabilizers are diverse, encompassing materials such as chitosan, starch, and cellulose. Owing to their low emulsifier dosage, ease of operation, strong biocompatibility, and high safety, they are increasingly favored. PE serves as an excellent carrier for EOs by reducing their sensitivity to evaporation and oxidation, thereby improving stability (Apostolidis et al., 2023; Cahyana et al., 2022; Pandita et al., 2024; Zhang et al., 2022b). For instance, a PE stabilized by modified cinnabar can enhance the thermal stability and in vitro dissolution rate of Acorus tatarinowii Schott EOs (Ru et al., 2024). PE can also carry lysozyme and tea tree EO, maintaining lysozyme activity while alleviating the volatilization of tea tree EO. Furthermore, the synergistic effect of the two metabolites exhibited a strong bactericidal effect against drug-resistant bacteria in vitro (Yao et al., 2024). Additionally, PE can improve the bioavailability of EOs, enhancing therapeutic efficacy and enabling sustained release. The PE of Cinnamon EOs demonstrates stronger antibacterial and antioxidant properties than pure EOs (Ly et al., 2024).
5.2.4 Self-emulsifying drug delivery systems (SEDDS)
SEDDS are homogeneous mixtures of oil, surfactant, and co-surfactant. When in contact with an aqueous medium and subjected to gentle stirring, they spontaneously form an emulsion (AboulFotouh et al., 2017). Moreover, SEDDS avoid steps such as heating or solvent evaporation that may damage EO active metabolites or cause volatile losses, thereby overcoming issues like poor solubility, limited absorption, and inadequate stability (Liu et al., 2022a). For example, Chaisri prepared an SEDDS using EOs from Cymbopogon citratus (DC.) Stapf and lemongrass, finding that its stability was superior to that of the crude oils during both long-term storage and accelerated conditions (Chaisri et al., 2024). However, droplets formed by SEDDS for lipophilic, poorly water-soluble drugs may not release the active metabolites at all (Malkawi et al., 2020). An improved version, the Self-Nanoemulsified Delivery System (SNEDDS), features smaller emulsion droplets and generally better emulsifying properties, enabling the rapid formation of stable, consistent emulsions. When combined with other medications, Mentha × piperita L. and Lavender EOs can be encapsulated and released at the absorption site without interference from the surrounding environment (Ali et al., 2022; Alissa et al., 2023). Nonetheless, research in this direction remains limited.
5.3 Microcapsules and nanocapsules
5.3.1 Microcapsules (MCs)
Introduced in the 1950s, MC technology involves encasing microscopic particles or droplets in polymer materials to create a core–shell structure. The outer layer protects the core (EOs) from harsh external conditions by efficiently blocking light, oxygen, and water. By precisely controlling the core material’s release, MCs mask its original odor and color while optimizing properties such as dispersion and solubility (Ma et al., 2024; Xiao et al., 2022). For example, Zhu et al. prepared MCs containing tea tree EO, which retained up to 87.1% of their content after high-temperature treatment for 70 min. Additionally, MCs enhance the inhibitory effect of EOs against S. aureus and E. coli (Zhu et al., 2024). While providing sustained release, MCs encapsulating Clove EO also boost the oil’s antioxidant properties (Li et al., 2024c). MCs containing lime peel EOs demonstrate good thermal stability, withstanding temperatures up to 122°C and maintaining an oil content of 38% over 4 weeks at room temperature (Indriyani et al., 2024). Composite MCs containing tea tree EO were fabricated using gelatin, Arabic gum, and n-butyl cyanoacrylate as wall materials via in-situ polymerization combined with composite solidification. Test results indicate that these capsules exhibit exceptional stability in osmotic environments and offer effective slow release and antioxidant capabilities under typical skin conditions (Yang et al., 2024b). However, MC preparation requires the use of surfactants.
5.3.2 Nanocapsules (NCs)
NCs, which have a smaller diameter than that of MCs, consist of a core material designed to encapsulate lipophilic chemicals, enclosed within a polymer shell. This design considerably enhances the functionality of EOs by providing exceptional stability and controlled-release characteristics (Zhang et al., 2024b). Due to their nanoscale size, NCs can maintain prolonged contact with the skin, offering enhanced residence time. For example, NCs loaded with EO can completely inhibit Pseudomonas acnes and scavenge ROS, thereby protecting human skin cells and demonstrating strong skin permeability (Ivanova et al., 2023). Compared to free EOs, NCs containing EOs from Rosmarinus officinalis L. and Lavandula dentata L. improve deposition in the stratum corneum (Silva-Flores et al., 2023). Additionally, studies encapsulating basil EOs in NCs have shown that the NCs effectively mask the EO odor while preserving their antibacterial and antioxidant properties (Gorzin et al., 2024).
However, NCs require stringent storage conditions and are prone to leakage. Conversely, lipid NCs (LNCs) represent a monodisperse system capable of efficiently encapsulating hydrophobic active metabolites and achieving sustained drug release. For instance, combining Lavender oil with apocynin in LNCs enhanced targeting efficiency and prolonged residence time (Youssef et al., 2024). These LNCs also display superior permeability properties. The resulting LO-LNCs have improved the penetration of ASP through rat skin by encapsulating Lavender oil within LNCs. In vivo pharmacokinetic studies have shown that transdermal administration of LO-LNCs can quadruple the maximum plasma concentration of ASP, increase its bioavailability by up to 52%, and offer sustained release for up to 3 days compared to oral suspensions (El-Tokhy et al., 2023).
5.4 Polymer and gel delivery systems
5.4.1 Polymeric Micelles (PMs)
PMs are self-assembled from amphiphilic copolymers, featuring a hydrophobic core for drug loading and a hydrophilic shell for drug absorption. As a delivery system for active molecules, PMs are easy to prepare, have a high drug-loading capacity, and their surfaces can be functionalized to achieve targeted delivery or extended circulation (Lodovichi et al., 2022). However, in recent years, there have been few studies on the encapsulation of EOs using PMs alone, with most efforts combining them with other carriers. For instance, Origanum vulgare L. Eos were first encapsulated into PMs and then incorporated into a binary hydrogel based on a Pluronic F127/L31 block-copolymer mixture. The pH compatibility of the EO-loaded poloxamer binary hydrogel with skin was investigated, and it exhibited sufficient spreadability and consistency. Furthermore, the formulation lowered the generation of inflammatory cytokines and limited the migration and proliferation of HaCaT cells (Bora et al., 2022). Most PM delivery systems focus on a single component rather than a complex EO, and future research should concentrate on loading EO mixtures.
5.4.2 Nanogels (NGs)
NGs are nanoscale, three-dimensional network structures with a particle size range of 20–200 nm. They encapsulate active metabolites through self-assembly or crosslinking. NGs have broad application prospects in local administration due to their ease of use, high loading capacity, and excellent physical and chemical stability. Compared to LSs and NEs, NGs exhibit greater physical stability (Maurya et al., 2024; Zarenezhad et al., 2021). In a comparison between an NG and an NE-containing Cuminum cyminum L. EO, the IC50 value of the NG against A-375 human melanoma cells was lower than that of the NE. Furthermore, the inhibition rates of 5,000 μg/mL NG and NE against Pseudomonas aeruginosa and S. aureus were 100% and 80%, respectively (Ranjbar et al., 2023). Chitosan is often used as the cross-linking polymer in NGs. The EO of Zataria multiflora Boiss. And nisin were co-encapsulated in chitosan NGs to extend the shelf life of cheese. Experimental results demonstrated that within 60 days of storage, the chitosan NG slowed pH changes in the cheese, and no coliforms were detected, indicating sustained EO release (Hosseini, et al., 2024). Moreover, NGs can reduce the EO-induced irritancy caused by EOs. For instance, NGs containing Lippia sidoides Cham. EOsEO did not exhibit acute skin irritation in a rat excisional wound healing model and significantly reduced the final wound area (Pires Rodrigues de Almeida Ribeiro et al., 2024). In addressing multidrug-resistant strains, where effective treatments remain limited, Aldawsari et al. successfully encapsulated lemongrass EO using nanoparticles formulated with PVA and PLGA. This formulation maintained EO stability and bioactivity while enabling precise delivery to the target site, thereby enhancing LGO effectiveness against antibiotic-resistant P. aeruginosa (Aldawsari et al., 2023).
5.4.3 Hydrogels
Hydrogels are hydrophilic, three-dimensional network gels capable of retaining large amounts of water and exhibiting excellent biocompatibility with bodily fluids. They can load active metabolites and release them in a controlled manner, making them suitable for the prolonged delivery of non-toxic, environmentally friendly bioactive substances. Additionally, they exhibit good air permeability and are used in wound healing (Alven et al., 2022; Elshahawy et al., 2024). Patchouli EO (PEO) shows limited absorption and low stability; therefore, a PEO-NE was developed and incorporated into a hydrogel composed of ROS/thermo dual-sensitive Bletilla striata polysaccharides. When administered rectally, PEONE-RTH adhered to the inflammatory site for an extended period and enhanced the antioxidant and repair activities of the EO (Huang et al., 2024b). As an external wound adhesive, hydrogels can load and promote the sustained release of EOs at injury sites, thereby improving bioavailability. For example, R. officinalis L., Curcuma longa L., and Thuja occidentalis L. EOs were loaded into a CCFG-CA hydrogel film; in vitro studies confirmed the film’s biocompatibility, antioxidant, and antimicrobial properties. Furthermore, in vivo wound healing studies showed that 14% of wounds healed and re-epithelialized within 99 days, with the hydrogel degradation time extended to 15 days (Tanwar et al., 2024).
The combined use of carriers can further reduce EO volatility and improve bioavailability. For example, Eucalyptus EOs in an NE prepared by physical crosslinking, when incorporated into a hydrogel, reduced the bacterial load of wounds and significantly downregulated inflammatory factor expression in vivo (Cai et al., 2023). In another example, a hydrogel containing an ME of Alpinia officinarum rhizome EO was developed for local application; the hydrogel maintained good physical stability after heating and cooling cycles at 4°C and during long-term storage (3 months) (Chittasupho et al., 2022).
5.5 Other delivery systems
5.5.1 Chitosan (CS)
CS, a polysaccharide derived from the deacetylation of chitin, is a versatile and environmentally friendly biopolymer. Besides exhibiting antibacterial, antioxidant, and antitumor properties, CS is widely used due to its biodegradability, biocompatibility, and non-toxic characteristics. In combination with EOs, the bioactivity of CS can be further enhanced (Abenaim and Conti, 2023; Ding et al., 2022; Liu et al., 2024f). For instance, the CS-thyme EO combination retained an antiseptic effect after being stored for 12 days at low temperatures (Liu et al., 2024d). Rosmarinus officinalis L. EO exhibited high physical and chemical stability in CS and showed the highest inhibitory activity against B. subtilis, E. coli, and free radicals as measured by ABTS and DPPH assays (Akhter et al., 2024). CS particles loaded with geranium and lemongrass EO showed superior activity, better than that of pure CS or EO, by reducing the mixed biofilm of C. albicans and Streptococcus mutans on a glass slide and lowering the toxicity of EOs to RAW 264.7 cells (Garcia et al., 2023).
CS nanoparticles (CSNPs) are nano-sized particles derived from CS. By encapsulating Piper betle L. EO in CSNPs, the permeability of the EO can be improved, showing better solubility and efficacy than free EO (Muthusamy et al., 2024). In another study, SLNs and CSNPs were used as carriers to load tea tree EO. The MICs of EO-CS, EO-SLN, and pure EO against S. aureus and P. aeruginosa were 35 and 45, 130 and 170 μg/mL, and 380 and 410 μg/mL, respectively, with EO-CS exhibiting a considerably high antibacterial effect (Vase et al., 2023). While various EOs have been encapsulated by CS, limitations such as mechanical strength and water solubility mean that using CS as a sole carrier may not fulfill the requirements of some applications. Consequently, it is often necessary to compound or blend CS with other carriers, such as starch, cellulose, or protein, which can increase production costs.
5.5.2 Cyclodextrin (CD)
CD is a water-soluble, biodegradable cyclic oligosaccharide that produces a mixture of α-CD, β-CD, and γ-CD composed of 6, 7, and 8 glucopyranose units, respectively. The hydrophobic cavity of CD can form complexes with various water-insoluble substances (such as EOs), thereby enhancing its stability and solubility and delaying volatilization (Ma et al., 2023; Paiva-Santos et al., 2022; Rodrigues Arruda et al., 2022). Among these, β-CD, containing seven sugar units, is widely used owing to its moderate size, which allows it to encapsulate most EOs with low sensitivity and irritation to the skin (Wu et al., 2024). β-CD active bacterial nanocellulose (BNC) nanopapers containing Salvia officinalis L. EO (SEO) were prepared. Experimental results demonstrated that adding SEO-βCD complexes improved the thermal properties of the BNC nanopapers, enhanced the antibacterial effect against L. monocytogenes, and increased antioxidant capacity (Mohammadzadeh et al., 2024). Ben et al. prepared β-CD/Eucalyptus globulus Labill. EO (EGEO) inclusion complexes, and the results showed that compared to the free EO (8.38 ± 1.95 mg/g), the β-CD/EGEO inclusion complexes exhibited an LC50 of 5.03 ± 1.16 mg/g against Ephestia kuehniella larvae, indicating enhanced EO activity (Ben Amara et al., 2024). Preparing an inclusion compound of fennel EO and hydroxypropyl-β-CD regulated EO release extended its release duration and improved its stability under varying temperature and relative humidity conditions (Song et al., 2024). Through β-CD encapsulation, the release of Cinnamon EO was delayed to about 90 h, achieving a high growth inhibition rate of almost 100% for E. coli and S. aureus (Li et al., 2023a).
5.5.3 Mesoporous Silica Nanoparticles (MSNPs)
MSNPs possess a high surface area and well-ordered protective pore structure, exhibiting excellent chemical stability, biocompatibility, and biodegradability. Embedding EO into MSNP can improve its stability and water solubility while achieving more sustained therapeutic efficacy through controlled release. After 2 months, the retention rate of the encapsulated compound reaches 50%. The synthesis method of MSNPs is simple and economical, making it suitable for large-scale industrial production (Gulin-Sarfraz et al., 2022; Lai et al., 2023; Weisany et al., 2024). The encapsulation of Lippia graveolens Kunth EO in MSNPs preserved its antioxidant and antibacterial properties, exhibiting a release rate of up to 42 days and inhibiting the growth of pathogenic and spoilage microorganisms (Matadamas-Ortiz et al., 2022). The surface modification of mesoporous silica particles (MSPs) reduces the hydrophobicity of EO, improves its solubility in the water phase, and enables it to penetrate into fungal cells, destroying the cell membrane and eventually causing cell death. Chakroun et al. encapsulated Ammoides pusilla (Brot.) Breistr. EO (AP-EO) into MSPs using an impregnation method and coated the surface with CS. In agar medium contact tests, the antifungal activity of AP-EO in MSPs without CS increased threefold. However, the CS coating slowed EO release. Therefore, using multiple carriers can further improve the stability of EO, achieve slow release, and enhance therapeutic effects (Chakroun et al., 2023).
To sum up, combining EO with modern drug delivery systems can minimize their defects and fully exploit their therapeutic potential. This section briefly summarizes modern drug delivery systems for encapsulating EOs. However, it is worth noting that EO delivery systems face some limitations. Therefore, researchers are urged to develop new strategies to overcome these shortcomings and optimize the effective delivery of EOs. For instance, Carneiro et al. utilized HPH technology to prepare NEs and NLCs containing Piper aduncum L. Eos, which were then thickened with hydrogel, enhancing both the viscosity and skin adherence of the nanoformulations (Carneiro et al., 2022). Chittasupho et al. successfully applied Kaempferia galanga L. EOs in ME to hydrogel, showing good physical stability and potential as a local sunscreen preparation (Chittasupho et al., 2022). For another example, garlic EO is limited in its application because of its irritation, poor water solubility, and low bioavailability. Therefore, Zhang et al. combined garlic EO with β-CD inclusion liposome to prepare a double-layer delivery system, which not only masked the smell of EO but also significantly improved its embedding efficiency and reduced the release rate (Zhang et al., 2024c). Elsewedy et al. integrated NE-containing fusidic acid and Cinnamomum cassia (L.) J. Presl EO into a hydrogel matrix, which prolonged the in vitro release time and enhanced the stability and antibacterial effects (Elsewedy et al., 2024). New carriers or materials have also been developed and utilized. For instance, a novel delivery system of nanoemulgel, combining NE with a gel matrix, merges the small particle size and high stability of NE with the controlled release and good skin adhesion of the gel. This includes the Zanthoxylum armatum DC. and R. officinalis L. EO nanoemulgel (Noor et al., 2023) and the nanoemulgel of Pituranthos tortuosus EO (Bahloul et al., 2024). Moreover, Fan et al. developed PE co-loaded with tannic acid and Cinnamomum cassia (L.) J. Presl EO based on zein and tannic acid complexes through interfacial engineering. This improved the stability and controlled-release performance of EOs compared to using PE alone (Fan et al., 2024). ME is widely used in the field of EO encapsulation; however, a common limitation is the use of potentially toxic surfactants and co-surfactants. Shen et al. used rhamnolipids instead of surfactants with rose and eucalyptus EOs to formulate MEs. Compared to regular emulsions, the EO-ME exhibited better slow-release behavior. The EO-ME significantly lowered the MIC against S. aureus (39 mg/L, compared to 1,250 mg/L with EO alone), demonstrating stable performance during storage (Shen et al., 2024a). Furthermore, Cheng and colleagues developed carrier-free nanodelivery systems characterized by self-assembly from active metabolites without surfactants or carriers (Cheng et al., 2024).
6 The drug delivery system enhances the physical and chemical properties of essential oil
One of the primary challenges in broadening the application of EOs is enhancing their bioavailability. Over the past decade, the use of drug delivery systems for encapsulating EOs has gradually increased. Numerous studies show that these systems can improve the stability and targeting of EOs, reduce their toxicity, and facilitate slow release, thus effectively improving EO bioavailability.
6.1 Sustained release of essential oil
Maintaining drugs within the therapeutic concentration range is crucial for chronic diseases requiring long-term treatment. However, frequent drug administration not only inconveniences patients but can also lead to drug resistance. Research has shown that drug delivery systems can maintain the slow release of EOs, achieving continuous treatment effects. For instance, Long et al. conducted an in vitro release study on Ligusticum striatum DC. EO (CXEO)-LS, which indicated a gradual increase in cumulative release within 48–72 h and demonstrated good storage stability at 4°C for at least 25 days (Long et al., 2023). Additionally, researchers have developed a photoresponsive LS for the controlled release of Alpinia galanga EO by utilizing the photoreactivity of Pheophorbide-a. This system has shown strong sustained-release effects and can be stored for up to 28 days at 4°C (Ge et al., 2023). Shen et al. developed PEs using potato protein-CS composite nanoparticles (PCCNs) for encapsulating Zanthoxylum bungeanum Maxim. EO (ZBEO). Confocal laser scanning microscopy revealed that PCCNs adsorbed onto the EO surface to form a dense interfacial layer, significantly enhancing the stability of ZBEO-PEs and enabling sustained EO release (Shen et al., 2024b). Alam et al. encapsulated fennel EO (FEO) into poly (lactic-co-glycolic acid) nanoparticles (FEO–PLGANPs) and incorporated them into NGs. In an in vitro drug release study, over 60% of the pure EO was released within 120 min, whereas the release amounts for FEO–PLGANPs NGs and FEO–PLGANPs were 31.43% and 26.76%, respectively, indicating that encapsulation within nanoparticles and NGs significantly delayed the drug release rate (Alam et al., 2022). Thus, EO drug delivery systems provide a viable solution for maintaining long-term therapeutic concentrations and improving patient convenience.
6.2 Enhancing the stability of essential oil
The stability of EOs is often disturbed by external environmental factors. Encapsulating EOs within drug delivery systems can effectively isolate them from these external conditions, thereby improving their physical and chemical stability. Wei et al. developed thyme EO-ME (TEO-M) and incorporated it into pullulan-sodium alginate (PS) films, finding that TEO-M endowed the PS films with antioxidant and UV-blocking properties, which remained stable for 10 days at 4°C (Wei et al., 2023). The NE of Calotropis gigantea EO remained stable after being stored at various temperatures for 50 days (Sharma, et al. 2024a). Cinnamon EO-MC prepared using the coagulation method extended its shelf life beyond 10 days when applied to kraft paper wrapped around citrus (Liu, et al. 2024e). Additionally, combining two carrier systems can further stabilize EOs. Xing et al. prepared NEs encapsulating Cinnamon EOs using hydroxypropyl-β-CD/lauroyl arginate inclusion complexes and found that under 100 MPa pressure and after seven cycles, the NEs exhibited excellent storage stability and thermal stability (Xing et al., 2024).
6.3 Enhancing the targeting function of essential oil
Drug delivery systems for EOs can be engineered to incorporate specific ligands on the carrier surface, thereby enabling active targeting of EOs to lesions through receptor-ligand interactions and enhancing therapeutic efficacy. For instance, Mahmoud et al. encapsulated thyme EO within O-quaternized, ultrasound-mediated deacetylated chitosan NCs, which not only exhibited excellent stability and release properties but also demonstrated selective targeting toward the SARS-CoV-2 virus (Mahmoud et al., 2024). Researchers have developed a novel lipid nanocapsule that encapsulates apocynin, is coated with lactoferrin, and incorporates Lavender EO as a bioactive additive. This system effectively targets the brain, where Lavender EO and apocynin work synergistically to alleviate seizures induced by pentylenetetrazol (Youssef et al., 2024). Additionally, Chen et al. prepared LS of Clove EO by embedding casein using the freeze-thaw method and then coupled Campylobacter jejuni antibodies to the LS surface via the post-insertion method, producing protease-activated, antibacterial LS with bacterial targeting capabilities (Chen et al., 2023b).
6.4 Reducing the toxic effects of essential oil
EOs are rarely applied directly to the skin without dilution because they may cause severe irritation. Therefore, the EU Cosmetics Regulation (EC) No 1223/2009 restricts the use of certain EO metabolites (such as coumarin and camphor) and mandates that known allergens (such as linalool and limonene) be labeled when their concentrations exceed specified limits. Additionally, products containing EOs must undergo safety assessments before being marketed. In 2001, the Scientific Committee on Cosmetic Products stipulated that the total concentration of furocoumarins in cosmetics must not exceed 1 ppm (Kejlová et al., 2010). In practical applications, EOs are typically diluted with carrier oils (such as vegetable oils, fixed oils, or fats), cream bases, or gels, with concentrations generally ranging from 2% to 5%. For more irritating EOs, the maximum dilution concentration should be reduced to 0.5% to ensure safe use (Dontje et al., 2024). Recent studies have shown that combining EOs with drug delivery systems can effectively reduce their toxicity and skin irritation. For instance, when using NLC to load Red Sacaca EO (NLC-RSO), the cytotoxicity of NLC-RSO was significantly lower than that of free RSO, indicating a cytoprotective effect of NLC on EO delivery (Chura et al., 2023). When applied topically, Cinnamon EO may cause skin irritation and phototoxicity. However, Essid et al. reduced its cytotoxicity by encapsulating Cinnamon EO in CSNPs, achieving a fourfold decrease compared to pure Cinnamon EO (Essid et al., 2023). Emtiazi et al. encapsulated Achillea millefolium EO using NLS/nano-niosomes, noting that the encapsulated EO exhibited lower toxicity than that exhibited by its non-encapsulated form (Emtiazi et al., 2022). Alam et al. encapsulated FEO into FEO–PLGANPs and further integrated them into NGs. In vitro cell viability assays using the normal cell line L929 demonstrated that both FEO–PLGANPs and FEO–PLGANPs NGs exhibited low toxicity and excellent biocompatibility, making them highly suitable for topical applications without irritation (Alam et al., 2022).
7 Effects of plant essential oil-based skin care on the nervous system
When individuals experience mental stress and fatigue, neuromodulators are released from skin nerve fibers, which can impair skin function and reduce its resilience to environmental stressors. Due to their rich active metabolites, EO-based skincare products not only nourish and repair the skin but also positively impact human psychology. For instance, a study showed that inhalation of Vetiver EOs dramatically altered gene expression in the lateral region of the central amygdala, displaying anti-anxiety characteristics (Saiyudthong et al., 2015). Díaz-Cantón et al. demonstrated that inhaling the EO of Litsea glaucescens Kunth increases brain-derived neurotrophic factor levels in the brains of mice, thereby exhibiting significant anxiolytic effects (Díaz-Cantón et al., 2024). Li et al., using the Forced Swim Test, Sucrose Preference Test, and Open Field Test, showed that inhaling Lavender EO significantly alleviated depressive-like behaviors in rats undergoing alcohol withdrawal (Li et al., 2024b). Sgoifo et al. explored the stress resistance properties of EO-rich skincare through self-administration experiments; their findings indicate that this approach reduces stress and promotes psychological recovery by lowering performance anxiety, state anxiety, and nonverbal behavioral patterns (Sgoifo et al., 2021). Therefore, incorporating plant EOs into skincare leverages the dual effects of the olfactory and epidermal systems to nourish and repair the skin, enhance the user’s emotional state, and regulate physiological functions simultaneously. Figure 7 summarizes EOs used to improve human negative emotions, and some EOs can produce dual pharmacological effects, both through inhalation and topical application, which can provide far-reaching effects when added to skin care products.
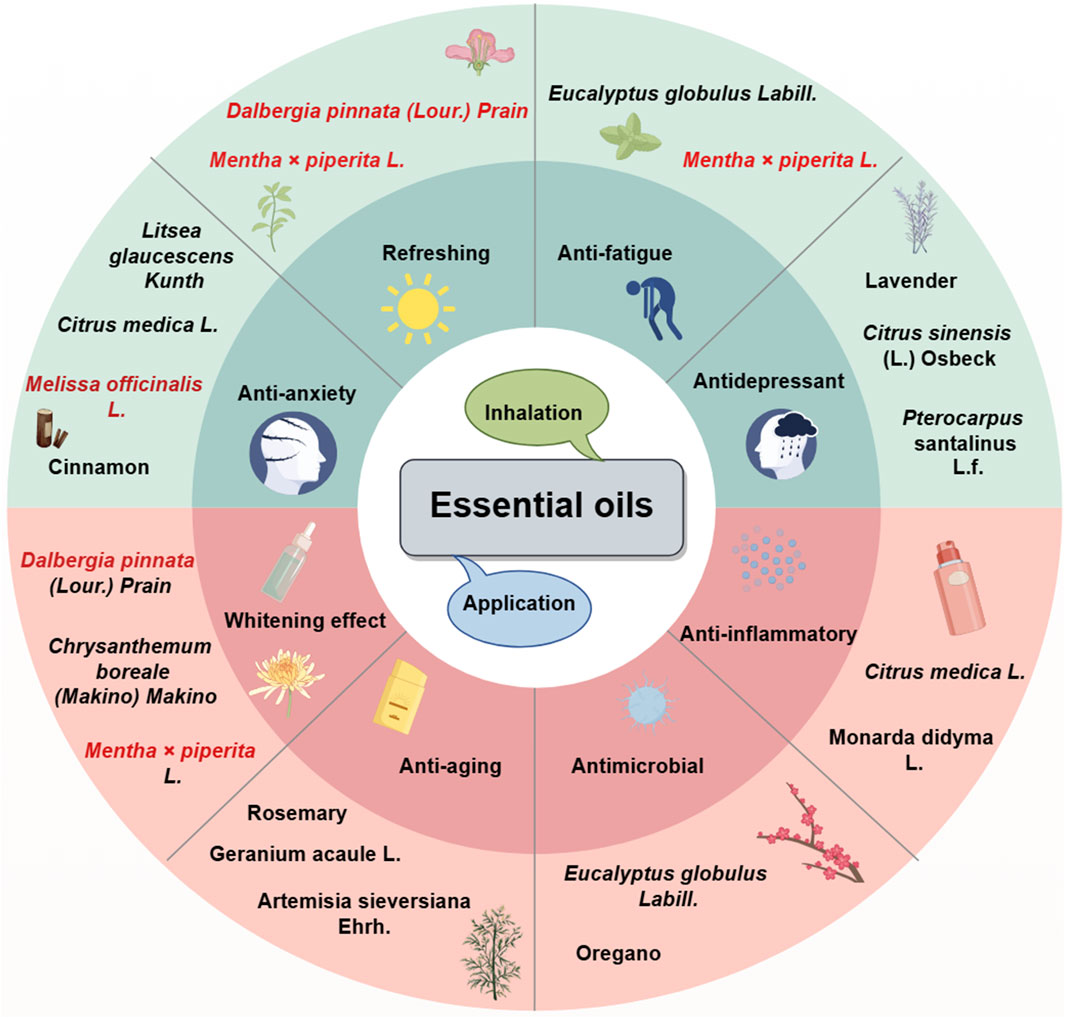
Figure 7. EOs with positive effects caused by inhalation. Note: Layer 1: EOs can be administered in two primary ways: inhalation and daubing. Layer 2: Inhalation, represented by green, is associated with benefits such as anti-anxiety, anti-depression, anti-fatigue, and a refreshing effect, while topical application, denoted by pink, delivers skin care benefits, including whitening, anti-aging, anti-bacterial, and anti-inflammatory properties. Layer 3: List the EOs related to the second layer, respectively. Some EOs (marked in red font) can be inhaled and smeared at the same time, indicating that EOs can nourish and repair the skin and also have a positive impact on the mental health of users. (By Figdraw).
8 Future perspectives and conclusion
As interest in natural and healthy skincare methods grows, EOs are increasingly utilized in skincare for their unique efficacy and natural purity. Projections for 2026 estimate that the global EO market will reach $16 billion (Tsitlakidou et al., 2023). The skin-whitening, UV resistance, antioxidative, antibacterial, and anti-inflammatory properties of EOs are well recognized. However, several challenges remain. First, the complexity of EO metabolites makes them susceptible to factors such as the plant’s growth stage, the specific parts used for extraction (Al-Snafi et al., 2024), and the extraction techniques adopted (Park et al., 2024). All these factors can significantly affect EO quality and the concentration of active metabolites. Additionally, isolating these metabolites from complex mixtures is challenging, and their release mechanisms are not yet fully elucidated. Second, direct application of EOs on the skin may cause adverse reactions, including phototoxicity, eye irritation, and skin allergies. Therefore, encapsulation represents a crucial strategy for broadening the safe application of EOs. Third, as the EO market continues to expand, counterfeit products and consumer misinformation are becoming more prevalent, leading to suboptimal usage outcomes.
Given the high sensitivity of EOs to environmental conditions, their storage requires special considerations. Employing various carriers has proven effective in mitigating the adverse effects of changes in O2, temperature, humidity, and pH, thereby ensuring the quality and stability of EOs. This paper briefly discusses several important carrier delivery systems and related preparation technologies. Combining EOs with these systems enhances their dispersion, stability, and release kinetics, improving overall efficacy compared to free EOs (Dupuis et al., 2022). However, several challenges persist. First, many existing EO delivery systems are complex to prepare, costly, and lack long-term stability. For instance, nano-lipid carriers may transform into a thermodynamically stable crystalline state during storage, reducing their drug-carrying capacity and potentially causing abrupt drug release (Pereira et al., 2018). Second, safety concerns arise because nanocarriers, while enhancing therapeutic effects by improving permeability, also increase the risk of cytotoxicity and potential harm to the nervous system. Moreover, the use of organic solvents or surfactants in nanopreparation processes may leave residues that adversely affect human health (Najahi-Missaoui et al., 2020). Third, current studies typically focus on encapsulating a single EO with one carrier; exploring the possibility of encapsulating multiple EOs within a single carrier presents significant research opportunities. Fourth, each delivery system has its limitations. Although combining two or more carriers can further enhance EO stability, cost considerations must also be taken into account.
In summary, to expand the application of EOs in the skincare field, it is essential to advance experimental research on transdermal drug delivery beyond in vitro studies and increase focus on both acute and chronic toxicity, including potential impacts on the liver, nervous system, and other organs following skin absorption. Further investigation is required to determine safe concentration ranges and ensure that experimental findings consistently translate into clinical therapeutic outcomes. In addition, standardizing extraction methods for various EOs, strengthening market regulation, and establishing clear laws and guidelines are necessary steps to safeguard consumers. Enhanced consumer education regarding the safe use of EOs and the identification of counterfeit products will also promote a healthier industry.
Concurrently, improving existing EO delivery systems by enhancing encapsulation efficiency and optimizing sustained-release performance to reduce toxicity and irritation while minimizing the use of harmful solvents is a key research priority. Ultimately, establishing a comprehensive safety evaluation system that spans from cell experiments and animal models to human trials is vital for fully assessing the potential risks of EOs. This comprehensive approach will drive their application in skincare, offering a safer, more effective, and environmentally friendly therapeutic solution.
Author contributions
WY: Conceptualization, Methodology, Visualization, Writing – original draft. DJ: Supervision, Validation, Writing – review and editing. WY: Investigation, Project administration, Writing – review and editing. MG: Formal Analysis, Writing – review and editing. ZX: Funding acquisition, Resources, Supervision, Validation, Writing – review and editing.
Funding
The author(s) declare that financial support was received for the research and/or publication of this article. This work was supported and funded by the Heilongjiang Special financial aid to post-doctor research fellows (number LBH-TZ1620) and the Science and Key Project of “Revealing the List and Taking the Lead” in Heilongjiang Province (number 023ZXJ02C02) and the Technology Plan of the Health and Wellness Committee of Heilongjiang Province (number 0222121021039) and the Science and Technology Innovation Project of Heilongjiang University of Traditional Chinese Medicine (number KY 2023-04).
Acknowledgments
We would like to thank all the researchers involved in this study. Additionally, our gratitude extends to Editage (www.editage.cn) for their assistance with English language editing.
Conflict of interest
The authors declare that the research was conducted in the absence of any commercial or financial relationships that could be construed as a potential conflict of interest.
Generative AI statement
The author(s) declare that no Generative AI was used in the creation of this manuscript.
Publisher’s note
All claims expressed in this article are solely those of the authors and do not necessarily represent those of their affiliated organizations, or those of the publisher, the editors and the reviewers. Any product that may be evaluated in this article, or claim that may be made by its manufacturer, is not guaranteed or endorsed by the publisher.
References
Abenaim, L., and Conti, B. (2023). Chitosan as a control tool for insect pest management: a review. Insects 14, 949. doi:10.3390/insects14120949
AbouAitah, K., and Lojkowski, W. (2022). Nanomedicine as an emerging technology to foster application of essential oils to fight cancer. Pharm. (Basel) 15, 793. doi:10.3390/ph15070793
AboulFotouh, K., Allam, A. A., El-Badry, M., and El-Sayed, A. M. (2017). Development and in vitro/in vivo performance of self-nanoemulsifying drug delivery systems loaded with candesartan cilexetil. Eur. J. Pharm. Sci. 109, 503–513. doi:10.1016/j.ejps.2017.09.001
Adewinogo, S. O., Sharma, R., Africa, C. W. J., Marnewick, J. L., and Hussein, A. A. (2021). Chemical composition and cosmeceutical potential of the essential oil of oncosiphon suffruticosum (L.) källersjö. Plants Basel, Switz. 10, 1315. doi:10.3390/plants10071315
Afshari, M., Kolackova, M., Rosecka, M., Čelakovská, J., and Krejsek, J. (2024). Unraveling the skin; a comprehensive review of atopic dermatitis, current understanding, and approaches. Front. Immunol. 15, 1361005. doi:10.3389/fimmu.2024.1361005
Ailli, A., Handaq, N., Touijer, H., Gourich, A. A., Drioiche, A., Zibouh, K., et al. (2023). Phytochemistry and biological activities of essential oils from six aromatic medicinal plants with cosmetic properties. Antibiot. Basel, Switz. 12, 721. doi:10.3390/antibiotics12040721
Akhter, R., Masoodi, F. A., and Wani, T. A. (2024). Chitosan, gelatin and pectin based bionanocomposite films with rosemary essential oil as an active ingredient for future foods. Int. J. Biol. Macromol. 272, 132813. doi:10.1016/j.ijbiomac.2024.132813
Alam, A., Foudah, A. I., Salkini, M. A., Raish, M., and Sawale, J. (2022). Herbal fennel essential oil nanogel: formulation, characterization and antibacterial activity against Staphylococcus aureus. Gels 8, 736. doi:10.3390/gels8110736
Aldawsari, M. F., Foudah, A. I., Rawat, P., Alam, A., and Salkini, M. A. (2023). Nanogel-based delivery system for lemongrass essential oil: a promising approach to overcome antibiotic resistance in Pseudomonas aeruginosa infections. Gels 9, 741. doi:10.3390/gels9090741
Ali, S. A., Alhakamy, N. A., Hosny, K. M., Alfayez, E., Bukhary, D. M., Safhi, A. Y., et al. (2022). Rapid oral transmucosal delivery of zaleplon-lavender oil utilizing self-nanoemulsifying lyophilized tablets technology: development, optimization and pharmacokinetic evaluation. Drug Deliv. 29, 2773–2783. doi:10.1080/10717544.2022.2115165
Alissa, M., Hjazi, A., Abusalim, G. S., Aloraini, G. S., Alghamdi, S. A., Rizg, W. Y., et al. (2023). Development and optimization of a novel lozenge containing a metronidazole-peppermint oil-tranexamic acid self-nanoemulsified delivery system to Be used after dental extraction: in vitro evaluation and in vivo appraisal. Pharmaceutics 15, 2342. doi:10.3390/pharmaceutics15092342
Almeida, L. F., Gil, G. A., Moraes, L. N., Furtado, F. B., Kakuda, L., Grotto, R. M. T., et al. (2024). Nanostructured lipid carriers loaded with essential oils: a strategy against SARS-CoV-2. J. Microencapsul. 41, 284–295. doi:10.1080/02652048.2024.2348463
Al-Mijalli, S. H., Mrabti, N. N., Ouassou, H., Sheikh, R. A., Assaggaf, H., Bakrim, S., et al. (2022). Chemical composition and antioxidant, antimicrobial, and anti-inflammatory properties of Origanum compactum benth essential oils from two regions: in vitro and in vivo evidence and in silico molecular investigations. Mol. Basel, Switz. 27, 7329. doi:10.3390/molecules27217329
Alomar, H. A., Elkady, W. M., Abdel-Aziz, M. M., Ibrahim, T. A., and Fathallah, N. (2023). Anti-heliobacter pylori and anti-inflammatory potential of salvia officinalis metabolites: in vitro and in silico studies. Metabolites 13, 136. doi:10.3390/metabo13010136
Al-Qudah, M. A., Al-Jaber, H. I., Abu Orabi, F. M., Hasan, H. S., Aldahoun, A. K., Alhamzani, A. G., et al. (2023). Unveiling the impact of drying methods on phytochemical composition and antioxidant activity of anthemis palestina. Plants Basel, Switz. 12, 3914. doi:10.3390/plants12223914
Alshehrei, F. M. (2024). Microbiological quality assessment of skin and body care cosmetics by using challenge test. Saudi J. Biol. Sci. 31, 103965. doi:10.1016/j.sjbs.2024.103965
Al-Snafi, A. E., Teibo, J. O., Shaheen, H. M., Akinfe, O. A., Teibo, T. K. A., Emieseimokumo, N., et al. (2024). The therapeutic value of Myrtus communis L.: an updated review. Naunyn-Schmiedeberg's archives Pharmacol. 397, 4579–4600. doi:10.1007/s00210-024-02958-3
Álvarez-Martínez, F. J., Barrajón-Catalán, E., Herranz-López, M., and Micol, V. (2021). Antibacterial plant compounds, extracts and essential oils: an updated review on their effects and putative mechanisms of action. Phytomedicine 90, 153626. doi:10.1016/j.phymed.2021.153626
Alven, S., Peter, S., and Aderibigbe, B. A. (2022). Polymer-based hydrogels enriched with essential oils: a promising approach for the treatment of infected wounds. Polym. (Basel) 14, 3772. doi:10.3390/polym14183772
Alves-Silva, J. M., Moreira, P., Cavaleiro, C., Pereira, C., Cruz, M. T., and Salgueiro, L. (2023). Effect of ferulago lutea (poir.) grande essential oil on molecular hallmarks of skin aging. Plants Basel, Switz. 12, 3741. doi:10.3390/plants12213741
Apostolidis, E., Stoforos, G. N., and Mandala, I. (2023). Starch physical treatment, emulsion formation, stability, and their applications. Carbohydr. Polym. 305, 120554. doi:10.1016/j.carbpol.2023.120554
Apte, S. S., and Parks, W. C. (2015). Metalloproteinases: a parade of functions in matrix biology and an outlook for the future. Matrix Biol. J. Int. Soc. Matrix Biol. 44-46, 1–6. doi:10.1016/j.matbio.2015.04.005
Asbabou, A., Hanane, T., Gourich, A. A., Siddique, F., Drioiche, A., Remok, F., et al. (2024). Phytochemical profile, physicochemical, antioxidant and antimicrobial properties of Juniperus phoenicea and Tetraclinis articulate: in vitro and in silico approaches. Front. Chem. 12, 1397961. doi:10.3389/fchem.2024.1397961
Asejeje, F. O., Alade, T. F., Oyibo, A., and Abolaji, A. O. (2024). Toxicological assessment of sodium benzoate in Drosophila melanogaster. J. Biochem. Mol. Toxicol. 38, e23586. doi:10.1002/jbt.23586
Ashmawy, N. S., Gad, H. A., and El-Nashar, H. A. S. (2023). Comparative study of essential oils from different organs of Syzygium cumini (pamposia) based on GC/MS chemical profiling and in vitro antiaging activity. Mol. Basel, Switz. 28, 7861. doi:10.3390/molecules28237861
Badalamenti, N., Salbitani, G., Cianciullo, P., Bossa, R., De Ruberto, F., Greco, V., et al. (2023). Chemical composition of salvia fruticosa Mill. Essential oil and its protective effects on both photosynthetic damage and oxidative stress in conocephalum conicum L. Induced by environmental heavy metal concentrations. Antioxidants Basel, Switz. 12, 1990. doi:10.3390/antiox12111990
Bader, A., Abdalla, A. N., Obaid, N. A., Youssef, L., Naffadi, H. M., Elzubier, M. E., et al. (2023). In vitro anticancer and antibacterial activities of the essential oil of forsskal's basil growing in extreme environmental conditions. Life Basel, Switz. 13, 651. doi:10.3390/life13030651
Bahloul, B., Ben Bnina, E., Hamdi, A., Castillo Henríquez, L., Baccar, D., Kalboussi, N., et al. (2024). Investigating the wound-healing potential of a nanoemulsion-gel formulation of Pituranthos tortuosus essential oil. Gels 10, 155. doi:10.3390/gels10030155
Bakheit, A. H., Wani, T. A., Al-Majed, A. A., Alkahtani, H. M., Alanazi, M. M., Alqahtani, F. R., et al. (2024). Theoretical study of the antioxidant mechanism and structure-activity relationships of 1,3,4-oxadiazol-2-ylthieno[2,3-d]pyrimidin-4-amine derivatives: a computational approach. Front. Chem. 12, 1443718. doi:10.3389/fchem.2024.1443718
Baldim, I., Oliveira, A. M., Souto, E. B., and Oliveira, W. P. (2022). Cyclodextrins-in-Liposomes: a promising delivery system for Lippia sidoides and Syzygium aromaticum essential oils. Life (Basel) 12, 95. doi:10.3390/life12010095
Banov, D., Carvalho, M., Schwartz, S., and Frumento, R. (2023). A randomized, double-blind, controlled study evaluating the effects of two facial serums on skin aging. Skin Res. Technol. official J. Int. Soc. Bioeng. Skin (ISBS) Int. Soc. Digital Imaging Skin (ISDIS) Int. Soc. Skin Imaging (ISSI) 29, e13522. doi:10.1111/srt.13522
Bedoya-Agudelo, J. P., López-Carvajal, J. E., Quiguanás-Guarín, E. S., Cardona, N., Padilla-Sanabria, L., and Castaño-Osorio, J. C. (2024). Assessment of antimicrobial and cytotoxic activities of liposomes loaded with curcumin and Lippia origanoides essential oil. Biomolecules 14, 851. doi:10.3390/biom14070851
Belcadi, H., Aknouch, A., El Amrani, S., Chraka, A., Lachkar, M., Mouhib, M., et al. (2023). Gamma-irradiation effect on the chemical composition and antibacterial activity of the moroccan tanacetum annuum L. essential oil. Heliyon 9, e16625. doi:10.1016/j.heliyon.2023.e16625
Ben Amara, F., Akermi, S., Driss, F., Marques, H. C., Costa, N., Smaoui, S., et al. (2024). Exploring the bio-insecticidal activity of Eucalyptus globulus essential oil/β-cyclodextrin inclusion complexes: in vitro and in silico assessment against Ephestia kuehniella larvae. Pestic. Biochem. Physiol. 202, 105917. doi:10.1016/j.pestbp.2024.105917
Berechet, M. D., Gaidau, C., Nešić, A., Constantinescu, R. R., Simion, D., Niculescu, O., et al. (2023). Antioxidant and antimicrobial properties of hydrolysed collagen nanofibers loaded with ginger essential oil. Mater. Basel, Switz. 16, 1438. doi:10.3390/ma16041438
Birkedal-Hansen, H. (1987). Catabolism and turnover of collagens: collagenases. Methods Enzym. 144, 140–171. doi:10.1016/0076-6879(87)44177-3
Boga, M., Ersoy, E., Eroglu, O. E., Cinar, E., Mataraci, K. E., Yesil, C. Y., et al. (2021). Volatile and phenolic profiling of a traditional medicinal plant, Hypericum empetrifolium with in vitro biological activities. J. Ethnopharmacol. 272, 113933. doi:10.1016/j.jep.2021.113933
Bolouri, P., Salami, R., Kouhi, S., Kordi, M., Asgari, L. B., Hadian, J., et al. (2022). Applications of essential oils and plant extracts in different industries. Molecules 27, 8999. doi:10.3390/molecules27248999
Boo, Y. C. (2021). Arbutin as a skin depigmenting agent with antimelanogenic and antioxidant properties. Antioxidants Basel, Switz. 10, 1129. doi:10.3390/antiox10071129
Bora, L., Burkard, T., Juan, M. H. S., Radeke, H. H., Muț, A. M., Vlaia, L. L., et al. (2022). Phytochemical characterization and biological evaluation of Origanum vulgare L. Essential oil formulated as polymeric micelles drug delivery systems. Pharmaceutics 14, 2413. doi:10.3390/pharmaceutics14112413
Cahyana, Y., Putri, Y. S. E., Solihah, D. S., Lutfi, F. S., Alqurashi, R. M., and Marta, H. (2022). Pickering emulsions as vehicles for bioactive compounds from essential oils. Molecules 27, 7872. doi:10.3390/molecules27227872
Cai, K., Liu, Y., Yue, Y., Liu, Y., and Guo, F. (2023). Essential oil nanoemulsion hydrogel with anti-biofilm activity for the treatment of infected wounds. Polym. (Basel) 15, 1376. doi:10.3390/polym15061376
Capetti, F., Tacchini, M., Marengo, A., Cagliero, C., Bicchi, C., Rubiolo, P., et al. (2021). Citral-containing essential oils as potential tyrosinase inhibitors: a bio-guided fractionation approach. Plants Basel, Switz. 10, 969. doi:10.3390/plants10050969
Caprari, C., Fantasma, F., Monaco, P., Divino, F., Iorizzi, M., Ranalli, G., et al. (2023). Chemical profiles, in vitro antioxidant and antifungal activity of four different Lavandula angustifolia L. EOs. Mol. Basel, Switz. 28, 392. doi:10.3390/molecules28010392
Carneiro, S. B., Kreutz, T., Limberger, R. P., Teixeira, H. F., da Veiga Júnior, V. F., and Koester, L. S. (2022). Piper aduncum essential oil rich in dillapiole: development of hydrogel-thickened nanoemulsion and nanostructured lipid carrier intended for skin delivery. Pharmaceutics 14, 2525. doi:10.3390/pharmaceutics14112525
Carvalho, M. J., Pedrosa, S. S., Mendes, A., Azevedo-Silva, J., Fernandes, J., Pintado, M., et al. (2023). Anti-aging potential of a novel ingredient derived from sugarcane straw extract (SSE). Int. J. Mol. Sci. 25, 21. doi:10.3390/ijms25010021
Castro, H. T., Martínez, J. R., and Stashenko, E. (2010). Anethole isomerization and dimerization induced by acid sites or UV irradiation. Molecules 15, 5012–5030. doi:10.3390/molecules15075012
Chaisri, W., Aneknan, T., Khonkarn, R., Tiwananthagorn, S., Suriyasathaporn, W., Pangprasit, N., et al. (2024). Self-emulsifying drug delivery systems (SEDDS) containing Cymbopogon citratus essential oil: enhancing the stability and acaricide efficacy against Rhipicephalus (Boophilus) microplus. Vet. Parasitol. 328, 110171. doi:10.1016/j.vetpar.2024.110171
Chakroun, Y., Snoussi, Y., Chehimi, M. M., Abderrabba, M., Savoie, J. M., and Oueslati, S. (2023). Encapsulation of Ammoides pusila essential oil into mesoporous silica particles for the enhancement of their activity against Fusarium avenaceum and its enniatins production. Molecules 28, 3194. doi:10.3390/molecules28073194
Chao, W. W., Su, C. C., Peng, H. Y., and Chou, S. T. (2017). Melaleuca quinquenervia essential oil inhibits α-melanocyte-stimulating hormone-induced melanin production and oxidative stress in B16 melanoma cells. Phytomedicine Int. J. phytotherapy Phytopharm. 34, 191–201. doi:10.1016/j.phymed.2017.08.024
Chen, G., Wang, X., He, Z., Li, X., Yang, Z., Zhang, Y., et al. (2023a). Light-elicited and oxygen-saved iridium nanocapsule for oxidative damage intensified oncotherapy. Molecules 28, 4397. doi:10.3390/molecules28114397
Chen, H., Xiao, Y., Wang, Y., Jiang, Y., Xu, Y., Wang, J., et al. (2024). Two-dimensional correlation infrared spectroscopy elucidated the volatilization process of the microemulsion composed of peppermint essential oil and composite herbal extract. Spectrochim. Acta A Mol. Biomol. Spectrosc. 311, 124009. doi:10.1016/j.saa.2024.124009
Chen, X., Yang, H., Li, C., Hu, W., Cui, H., and Lin, L. (2023b). Enhancing the targeting performance and prolonging the antibacterial effects of clove essential oil liposomes to Campylobacter jejuni by antibody modification. Food Res. Int. 167, 112736. doi:10.1016/j.foodres.2023.112736
Cheng, F., Chen, M., Duan, Z., Zou, Y., He, Y., Zeng, F., et al. (2024). Fabrication, characterization, and bioactivity of self-assembled carrier-free colloidal dispersions from Citrus × Limon 'Rosso' essential oil and tea polyphenols. Food Chem. 457, 140058. doi:10.1016/j.foodchem.2024.140058
Chhetri, S., Arora, S., Parcha, V., Kumar, D., and Rawat, D. S. (2024). Nano encapsulation of an essential oil transpire the therapeutic approach. Curr. Pharm. Biotechnol. 25. doi:10.2174/0113892010291682240423095306
Chittasupho, C., Ditsri, S., Singh, S., Kanlayavattanakul, M., Duangnin, N., Ruksiriwanich, W., et al. (2022). Ultraviolet radiation protective and anti-inflammatory effects of Kaempferia galanga L. Rhizome oil and microemulsion: formulation, characterization, and hydrogel preparation. Gels 8, 639. doi:10.3390/gels8100639
Chura, S. S. D., Memória, K. A. S., Lopes, A. T., Pelissari, F. M., Da Silveira, J. V. W., Bezerra, J. A., et al. (2023). Red sacaca essential oil-loaded nanostructured lipid carriers optimized by factorial design: cytotoxicity and cellular reactive oxygen species levels. Front. Pharmacol. 14, 1176629. doi:10.3389/fphar.2023.1176629
Cimino, C., Maurel, O. M., Musumeci, T., Bonaccorso, A., Drago, F., Souto, E. M. B., et al. (2021). Essential oils: pharmaceutical applications and encapsulation strategies into lipid-based delivery systems. Pharmaceutics 13, 327. doi:10.3390/pharmaceutics13030327
Cunha, C., Ribeiro, H. M., Rodrigues, M., and Araujo, A. (2022). Essential oils used in dermocosmetics: review about its biological activities. J. Cosmet. dermatology 21, 513–529. doi:10.1111/jocd.14652
de Sousa, D. P., Damasceno, R. O. S., Amorati, R., Elshabrawy, H. A., de Castro, R. D., Bezerra, D. P., et al. (2023). Essential oils: chemistry and pharmacological activities. Biomolecules 13, 1144. doi:10.3390/biom13071144
Dessinioti, C., and Katsambas, A. (2024). The microbiome and acne: perspectives for treatment. Dermatology Ther. 14, 31–44. doi:10.1007/s13555-023-01079-8
Díaz-Cantón, J. K., Torres-Ramos, M. A., Limón-Morales, O., León-Santiago, M., Rivero-Segura, N. A., Tapia-Mendoza, E., et al. (2024). Inhaled Litsea glaucescens K. (Lauraceae) leaves' essential oil has anxiolytic and antidepressant-like activity in mice by BDNF pathway activation. J. Ethnopharmacol. 321, 117489. doi:10.1016/j.jep.2023.117489
Di Meo, S., and Venditti, P. (2020). Evolution of the knowledge of free radicals and other oxidants. Oxidative Med. Cell. Longev. 2020, 9829176. doi:10.1155/2020/9829176
Ding, X., Zhao, L., Khan, I. M., Yue, L., Zhang, Y., and Wang, Z. (2022). Emerging chitosan grafted essential oil components: a review on synthesis, characterization, and potential application. Carbohydr. Polym. 297, 120011. doi:10.1016/j.carbpol.2022.120011
Do, T. T. H., Nguyen, T. U., Nguyen, T. T. H., Ho, T. Y., Pham, T. L. H., Le, T. S., et al. (2022). Essential oils from the leaves, stem, and roots of blumea lanceolaria (roxb.) druce in vietnam: determination of chemical composition, and in vitro, in vivo, and in silico studies on anti-inflammatory activity. Mol. Basel, Switz. 27, 7839. doi:10.3390/molecules27227839
Dong, Y., Wei, Z., Yang, R., Zhang, Y., Sun, M., Bai, H., et al. (2023). Chemical compositions of essential oil extracted from eight thyme species and potential biological functions. Plants Basel, Switz. 12, 4164. doi:10.3390/plants12244164
Dontje, A., Schuiling-Veninga, C. C. M., van Hunsel, F., Ekhart, C., Demirci, F., and Woerdenbag, H. J. (2024). The therapeutic potential of essential oils in managing inflammatory skin conditions: a scoping review. Pharm. (Basel) 17, 571. doi:10.3390/ph17050571
Draelos, Z. D., Deliencourt-Godefroy, G., and Lopes, L. (2020). An effective hydroquinone alternative for topical skin lightening. J. Cosmet. Dermatol 19, 3258–3261. doi:10.1111/jocd.13771
Dupuis, V., Cerbu, C., Witkowski, L., Potarniche, A. V., Timar, M. C., Żychska, M., et al. (2022). Nanodelivery of essential oils as efficient tools against antimicrobial resistance: a review of the type and physical-chemical properties of the delivery systems and applications. Drug Deliv. 29, 1007–1024. doi:10.1080/10717544.2022.2056663
Dylenova, E. P., Zhigzhitzhapova, S. V., Emelyanova, E. A., Tykheev, Z. A., Chimitov, D. G., Goncharova, D. B., et al. (2023). Chemical diversity of artemisia rutifolia essential oil, antimicrobial and antiradical activity. Plants Basel, Switz. 12, 1289. doi:10.3390/plants12061289
El Hachlafi, N., Fikri-Benbrahim, K., Al-Mijalli, S. H., Elbouzidi, A., Jeddi, M., Abdallah, E. M., et al. (2024). Tetraclinis articulata (Vahl) Mast. essential oil as a promising source of bioactive compounds with antimicrobial, antioxidant, anti-inflammatory and dermatoprotective properties: in vitro and in silico evidence. Heliyon 10, e23084. doi:10.1016/j.heliyon.2023.e23084
El Hachlafi, N., Mrabti, H. N., Al-Mijalli, S. H., Jeddi, M., Abdallah, E. M., Benkhaira, N., et al. (2023). Antioxidant, volatile compounds; antimicrobial, anti-inflammatory, and dermatoprotective properties of cedrus atlantica (endl.) manetti ex carriere essential oil: in vitro and in silico investigations. Mol. Basel, Switz. 28, 5913. doi:10.3390/molecules28155913
Ellboudy, N. M., Elwakil, B. H., Shaaban, M. M., and Olama, Z. A. (2023). Cinnamon oil-loaded Nanoliposomes with potent antibacterial and antibiofilm activities. Molecules 28, 4492. doi:10.3390/molecules28114492
El Omari, N., Mrabti, H. N., Benali, T., Ullah, R., Alotaibi, A., Abdullah, A. D. I., et al. (2023). Expediting multiple biological properties of limonene and α-pinene: main bioactive compounds of pistacia lentiscus L., essential oils. Front. Biosci. Landmark Ed. 28, 229. doi:10.31083/j.fbl2809229
Elsewedy, H. S., Shehata, T. M., Genedy, S. M., Siddiq, K. M., Asiri, B. Y., Alshammari, R. A., et al. (2024). Enhancing the topical antibacterial activity of fusidic acid via embedding into cinnamon oil nano-lipid carrier. Gels 10, 268. doi:10.3390/gels10040268
Elshahawy, M. F., Mohamed, R. D., Ali, A. E., Raafat, A. I., and Ahmed, N. A. (2024). Electron beam irradiation developed cinnamon oil- (polyvinyl alcohol/gum tragacanth)/graphene oxide dressing hydrogels: antimicrobial and healing assessments. Int. J. Biol. Macromol. 277, 134384. doi:10.1016/j.ijbiomac.2024.134384
El-Tokhy, F. S., Abdel-Mottaleb, M. M. A., Abdel, M. S. S., Mahmoud, A. M. A., El-Ghany, E. A., and Geneidi, A. S. (2023). Boosting the in vivo transdermal bioavailability of asenapine maleate using novel lavender oil-based lipid nanocapsules for management of schizophrenia. Pharmaceutics 15, 490. doi:10.3390/pharmaceutics15020490
Emtiazi, H., Salari Sharif, A., Hemati, M., Fatemeh Haghiralsadat, B., and Pardakhti, A. (2022). Comparative study of nano-liposome and nano-niosome for delivery of Achillea millefolium essential oils: development, optimization, characterization and their cytotoxicity effects on cancer cell lines and antibacterial activity. Chem. Biodivers. 19, e202200397. doi:10.1002/cbdv.202200397
Essid, R., Ayed, A., Djebali, K., Saad, H., Srasra, M., Othmani, Y., et al. (2023). Anti-Candida and anti-leishmanial activities of encapsulated Cinnamomum verum essential oil in chitosan nanoparticles. Molecules 28, 5681. doi:10.3390/molecules28155681
Fan, S., Yang, Q., Wang, D., Zhu, C., Wen, X., Li, X., et al. (2024). Zein and tannic acid hybrid particles improving physical stability, controlled release properties, and antimicrobial activity of cinnamon essential oil loaded Pickering emulsions. Food Chem. 446, 138512. doi:10.1016/j.foodchem.2024.138512
Fayez, S., Gamal El-Din, M. I., Moghannem, S. A., Azam, F., El-Shazly, M., Korinek, M., et al. (2023). Eucalyptus-derived essential oils alleviate microbes and modulate inflammation by suppressing superoxide and elastase release. Front. Pharmacol. 14, 1218315. doi:10.3389/fphar.2023.1218315
Feng, S., Xu, G., Fu, Y., Ding, Q., and Shi, Y. (2023). Exploring the mechanism of bergamot essential oil against asthma based on network pharmacology and experimental verification. ACS omega 8, 10202–10213. doi:10.1021/acsomega.2c07366
Fisher, G. J., Datta, S. C., Talwar, H. S., Wang, Z. Q., Varani, J., Kang, S., et al. (1996). Molecular basis of sun-induced premature skin ageing and retinoid antagonism. Nature 379, 335–339. doi:10.1038/379335a0
Fraternale, D., Dufat, H., Albertini, M. C., Bouzidi, C., D'Adderio, R., Coppari, S., et al. (2022). Chemical composition, antioxidant and anti-inflammatory properties of Monarda didyma L. essential oil. PeerJ 10, e14433. doi:10.7717/peerj.14433
Fuentes, J. M., Jofré, I., Tortella, G., Benavides-Mendoza, A., Diez, M. C., Rubilar, O., et al. (2024). The mechanistic insights of essential oil of Mentha piperita to control Botrytis cinerea and the prospection of lipid nanoparticles to its application. Microbiol. Res. 286, 127792. doi:10.1016/j.micres.2024.127792
Gan, N., Li, Q., Li, Y., Li, M., Li, Y., Chen, L., et al. (2024). Encapsulation of lemongrass essential oil by bilayer liposomes based on pectin, gum Arabic, and carrageenan: characterization and application in chicken meat preservation. Int. J. Biol. Macromol. 281, 135706. doi:10.1016/j.ijbiomac.2024.135706
Gao, X., Liu, J., Li, B., and Xie, J. (2023). Antibacterial activity and antibacterial mechanism of Lemon verbena essential oil. Mol. Basel, Switz. 28, 3102. doi:10.3390/molecules28073102
Garcia, L. G. S., Rocha, M. G. D., Freire, R. S., Nunes, P. I. G., Nunes, J. V. S., Fernandes, M. R., et al. (2023). Chitosan microparticles loaded with essential oils inhibit duo-biofilms of Candida albicans and Streptococcus mutans. J. Appl. Oral Sci. 31, e20230146. doi:10.1590/1678-7757-2023-0146
Gaspar, A. L., Gaspar, A. B., Contini, L. R. F., Silva, M. F., Chagas, E. G. L., Bahú, J. O., et al. (2022). Lemongrass (Cymbopogon citratus)-incorporated chitosan bioactive films for potential skincare applications. Int. J. Pharm. 628, 122301. doi:10.1016/j.ijpharm.2022.122301
Ge, X., Hu, Y., Shen, H., Liang, W., Sun, Z., Zhang, X., et al. (2023). Pheophorbide-a as a light-triggered liposomal switch: for the controlled release of Alpinia galanga (A. Galanga) essential oil and its stability, antioxidant, and antibacterial activity assessment. J. Agric. Food Chem. 71, 1667–1678. doi:10.1021/acs.jafc.2c07082
Ge, Y., Li, M., Bai, S., Chen, C., Zhang, S., Cheng, J., et al. (2024). Doxercalciferol alleviates UVB-induced HaCaT cell senescence and skin photoaging. Int. Immunopharmacol. 127, 111357. doi:10.1016/j.intimp.2023.111357
Gęgotek, A., Rybałtowska-Kawałko, P., and Skrzydlewska, E. (2017). Rutin as a mediator of lipid metabolism and cellular signaling pathways interactions in fibroblasts altered by UVA and UVB radiation. Oxidative Med. Cell. Longev. 2017, 4721352. doi:10.1155/2017/4721352
Geier, J., Schubert, S., Reich, K., Skudlik, C., Ballmer-Weber, B., Brehler, R., et al. (2022). Contact sensitization to essential oils: IVDK data of the years 2010-2019. Contact Dermat. 87, 71–80. doi:10.1111/cod.14126
Głaz, P., Rosińska, A., Woźniak, S., Boguszewska-Czubara, A., Biernasiuk, A., and Matosiuk, D. (2023). Effect of commonly used cosmetic preservatives on healthy human skin cells. Cells 12, 1076. doi:10.3390/cells12071076
Gorzin, M., Saeidi, M., Javidi, S., Seow, E. K., and Abedinia, A. (2024). Nanoencapsulation of Oliveria decumbens Vent./basil essential oils into gum Arabic/maltodextrin: improved in vitro bioaccessibility and minced beef meat safety. Int. J. Biol. Macromol. 270, 132288. doi:10.1016/j.ijbiomac.2024.132288
Guedes, J. B., do Nascimento, A. L., Costa, W. K., de Veras, B. O., de Aguiar, J., Navarro, D., et al. (2023). Eugenia gracillima essential oil has pharmaceutical applications in pain and inflammation without toxic effects in mice. J. Ethnopharmacol. 303, 115941. doi:10.1016/j.jep.2022.115941
Gulin-Sarfraz, T., Kalantzopoulos, G. N., Haugen, J. E., Axelsson, L., Raanaas, K. H., and Sarfraz, J. (2022). Controlled release of volatile antimicrobial compounds from mesoporous silica nanocarriers for active food packaging applications. Int. J. Mol. Sci. 23, 7032. doi:10.3390/ijms23137032
Guo, Y., Pizzol, R., Gabbanini, S., Baschieri, A., Amorati, R., and Valgimigli, L. (2021). Absolute antioxidant activity of five phenol-rich essential oils. Mol. Basel, Switz. 26, 5237. doi:10.3390/molecules26175237
Ha, S. Y., Jung, J. Y., and Yang, J. K. (2021). Camellia japonica essential oil inhibits α-MSH-induced melanin production and tyrosinase activity in B16F10 melanoma cells. Evidence-based complementary Altern. Med. eCAM 2021, 6328767. doi:10.1155/2021/6328767
Haghbayan, H., Moghimi, R., Sarrafi, Y., Taleghani, A., and Hosseinzadeh, R. (2024). Enhancing bioactivity of Callistemon citrinus (Curtis) essential oil through novel nanoemulsion formulation. J. Biomater. Sci. Polym. 35, 2660–2681. doi:10.1080/09205063.2024.2386787
Hancianu, M., Cioanca, O., Mihasan, M., and Hritcu, L. (2013). Neuroprotective effects of inhaled lavender oil on scopolamine-induced dementia via anti-oxidative activities in rats. Phytomedicine Int. J. phytotherapy Phytopharm. 20, 446–452. doi:10.1016/j.phymed.2012.12.005
Harman, D. (1956). Aging: a theory based on free radical and radiation chemistry. J. gerontology 11, 298–300. doi:10.1093/geronj/11.3.298
Hashemi, S. M. B., Gholamhosseinpour, A., and Barba, F. J. (2023). Rosmarinus officinalis L. Essential oils impact on the microbiological and oxidative stability of sarshir (kaymak). Mol. Basel, Switz. 28, 4206. doi:10.3390/molecules28104206
He, J., Chen, W., Chen, X., Xie, Y., Zhao, Y., Tian, T., et al. (2023). Tetrahedral framework nucleic acid loaded with glabridin: a transdermal delivery system applicated to anti-hyperpigmentation. Cell Prolif. 56, e13495. doi:10.1111/cpr.13495
Hedayati, S., Tarahi, M., Iraji, A., and Hashempur, M. H. (2024). Recent developments in the encapsulation of lavender essential oil. Adv. Colloid Interface Sci. 331, 103229. doi:10.1016/j.cis.2024.103229
Henríquez, J. C., Duarte, L. V., Sierra, L. J., Fernández-Alonso, J. L., Martínez, J. R., and Stashenko, E. E. (2023). Chemical composition and in vitro antioxidant activity of salvia aratocensis (lamiaceae) essential oils and extracts. Mol. Basel, Switz. 28, 4062. doi:10.3390/molecules28104062
Ho, Y. T., Liu, I. H., Chang, S. T., Wang, S. Y., and Chang, H. T. (2023). In vitro and in vivo antimelanogenesis effects of leaf essential oil from Agathis dammara. Pharmaceutics 15, 2269. doi:10.3390/pharmaceutics15092269
Hosseini, S. M., Tavakolipour, H., Mokhtarian, M., and Armin, M. (2024). Co-encapsulation of Shirazi thyme (Zataria multiflora) essential oil and nisin using caffeic acid grafted chitosan nanogel and the effect of this nanogel as a bio-preservative in Iranian white cheese. Food Sci. Nutr. 12, 4385–4398. doi:10.1002/fsn3.4105
Hsiao, W. W., Kumar, K. J. S., Lee, H. J., Tsao, N. W., and Wang, S. Y. (2021). Anti-Melanogenic activity of calocedrus formosana wood essential oil and its chemical composition analysis. Plants Basel, Switz. 11, 62. doi:10.3390/plants11010062
Huang, Y., Huang, H., Wu, J., Feng, Q., Li, Y., Li, Q., et al. (2024a). Preparation and properties of PCL coaxial electrospinning films with shell loaded with CEO and core coated LEO nanoemulsions. Food Res. Int. 194, 114817. doi:10.1016/j.foodres.2024.114817
Huang, Y., Wang, P., Zhao, P., Cheng, W., Fu, H., Zheng, X., et al. (2024b). ROS/Thermo dual-sensitive hydrogel loaded with a nanoemulsion of patchouli essential oil for ulcerative colitis. Int. J. Biol. Macromol. 281, 136542. doi:10.1016/j.ijbiomac.2024.136542
Huang, Y., Xu, H., Ding, M., Li, J., Wang, D., Li, H., et al. (2023). Screening of rosemary essential oils with different phytochemicals for antioxidant capacity, keratinocyte cytotoxicity, and anti-proliferative activity. Mol. Basel, Switz. 28, 586. doi:10.3390/molecules28020586
Indriyani, N. N., Al-Anshori, J., Wahyudi, T., Nurzaman, M., Nurjanah, S., Permadi, N., et al. (2024). An optimized chitosan/alginate-based microencapsulation of lime peel essential oil and its application as an antibacterial textile. J. Biomater. Sci. Polym. 35, 989–1007. doi:10.1080/09205063.2024.2313829
Ivanova, K., Ramon, E., Ivanova, A., Sanchez-Gomez, S., and Tzanov, T. (2023). Bio-based nano-enabled cosmetic formulations for the treatment of cutibacterium acnes-associated skin infections. Antioxidants (Basel) 12, 432. doi:10.3390/antiox12020432
Jafarizadeh-Malmiri, H., Anarjan, N., and Berenjian, A. (2022). Developing three-component ginger-cinnamon-cardamom composite essential oil nanoemulsion as natural food preservatives. Environ. Res. 204, 112133. doi:10.1016/j.envres.2021.112133
Jaradat, N., Hawash, M., Abualhasan, M. N., Qadi, M., Ghanim, M., Massarwy, E., et al. (2021). Spectral characterization, antioxidant, antimicrobial, cytotoxic, and cyclooxygenase inhibitory activities of Aloysia citriodora essential oils collected from two Palestinian regions. BMC complementary Med. Ther. 21, 143. doi:10.1186/s12906-021-03314-1
Jiang, Y., Tsoi, L. C., Billi, A. C., Ward, N. L., Harms, P. W., Zeng, C., et al. (2020). Cytokinocytes: the diverse contribution of keratinocytes to immune responses in skin. JCI Insight 5, e142067. doi:10.1172/jci.insight.142067
Kanbolat, Ş., Şahin, O., Ulaş Çolak, N., Badem, M., Subaş, T., Şener, S., et al. (2024). Investigation of antioxidant and enzyme inhibitory activities of Echinophora chrysantha: microwave-assisted hydrodistillation and SPME analysis of its essential oils. Chem. Biodivers. 22, e202401366. doi:10.1002/cbdv.202401366
Kanlayavattanakul, M., and Lourith, N. (2018). Plants and natural products for the treatment of skin hyperpigmentation - a review. Planta medica. 84, 988–1006. doi:10.1055/a-0583-0410
Kapadia, P., Newell, A. S., Cunningham, J., Roberts, M. R., and Hardy, J. G. (2022). Extraction of high-value chemicals from plants for technical and medical applications. Int. J. Mol. Sci. 23, 10334. doi:10.3390/ijms231810334
Kejlová, K., Jírová, D., Bendová, H., Gajdoš, P., and Kolářová, H. (2010). Phototoxicity of essential oils intended for cosmetic use. Toxicol Vitro 24, 2084–2089. doi:10.1016/j.tiv.2010.07.025
Khalil, H. E., Ibrahim, H. M., Darrag, H. M., and Matsunami, K. (2021). Insight into analysis of essential oil from anisosciadium lanatum boiss.-chemical composition, molecular docking, and mitigation of Hepg2 cancer cells through apoptotic markers. Plants Basel, Switz. 11, 66. doi:10.3390/plants11010066
Kim, D. Y., Won, K. J., Kim, Y. Y., Yoo, D. Y., and Lee, H. M. (2024). Potential wound healing and anti-melanogenic activities in skin cells of aralia elata (miq.) seem. Flower essential oil and its chemical composition. Pharmaceutics 16, 1008. doi:10.3390/pharmaceutics16081008
Kim, H. S., Kim, H. J., Hong, Y. D., Son, E. D., and Cho, S. Y. (2023). β-endorphin suppresses ultraviolet B irradiation-induced epidermal barrier damage by regulating inflammation-dependent mTORC1 signaling. Sci. Rep. 13, 22357. doi:10.1038/s41598-023-49886-5
Kim, K., Huh, Y., and Lim, K. M. (2021). Anti-pigmentary natural compounds and their mode of action. Int. J. Mol. Sci. 22, 6206. doi:10.3390/ijms22126206
Kotb, E. A., El-Shiekh, R. A., Abd-Elsalam, W. H., El, S. N., El, T. N., and El Senousy, A. S. (2023). Protective potential of frankincense essential oil and its loaded solid lipid nanoparticles against UVB-induced photodamage in rats via MAPK and PI3K/AKT signaling pathways; A promising anti-aging therapy. PLoS One 18, e0294067. doi:10.1371/journal.pone.0294067
Kryeziu, T. L., Haloci, E., Loshaj-Shala, A., Bagci, U., Oral, A., Stefkov, G. J., et al. (2022). Nanoencapsulation of Origanum vulgare essential oil into liposomes with anticancer potential. Pharmazie 77, 172–178. doi:10.1691/ph.2022.1230
Kumar, K. J. S., Vani, M. G., Wu, P. C., Lee, H. J., Tseng, Y. H., and Wang, S. Y. (2020). Essential oils of Alpinia nantoensis retard forskolin-induced melanogenesis via ERK1/2-mediated proteasomal degradation of MITF. Plants Basel, Switz. 9, 1672. doi:10.3390/plants9121672
Kuttithodi, A. M., Narayanankutty, A., Visakh, N. U., Job, J. T., Pathrose, B., Olatunji, O. J., et al. (2023). Chemical composition of the Cinnamomum malabatrum leaf essential oil and analysis of its antioxidant, enzyme inhibitory and antibacterial activities. Antibiot. Basel, Switz. 12, 940. doi:10.3390/antibiotics12050940
Lai, H., Chen, S., Su, X., Huang, X., Zheng, Q., Yang, M., et al. (2023). Sponge-liked silica nanoporous particles for sustaining release and long-term antibacterial activity of natural essential oil. Molecules 28, 594. doi:10.3390/molecules28020594
Lee, D. K., Won, K. J., Kim, D. Y., Kim, Y. Y., and Lee, H. M. (2023). Chemical composition and skin-whitening activities of siegesbeckia glabrescens makino flower absolute in melanocytes. Plants Basel, Switz. 12, 3930. doi:10.3390/plants12233930
Li, D., Liu, Z., Zhang, L., Bian, X., Wu, J., Li, L., et al. (2024a). The lncRNA SNHG26 drives the inflammatory-to-proliferative state transition of keratinocyte progenitor cells during wound healing. Nat. Commun. 15, 8637. doi:10.1038/s41467-024-52783-8
Li, J., Bao, Y., Li, Z., Cui, H., Jiang, Q., Hou, C., et al. (2023a). Dual-function β-cyclodextrin/starch-based intelligent film with reversible responsiveness and sustained bacteriostat-releasing for food preservation and monitoring. Int. J. Biol. Macromol. 253, 127168. doi:10.1016/j.ijbiomac.2023.127168
Li, X., Xiao, D., Li, C., Wu, T., Li, L., Li, T., et al. (2024b). Lavender essential oil alleviates depressive-like behavior in alcohol-withdrawn rats: insights from gut metabolites and hippocampal transcriptome analysis. Biomed. Pharmacother. 176, 116835. doi:10.1016/j.biopha.2024.116835
Li, Y., Bian, B., Tang, R., and Zhang, K. (2024c). Characterization of a clove essential oil slow-release microencapsulated composite film and its preservation effects on blueberry. ACS Omega 9, 12643–12656. doi:10.1021/acsomega.3c07169
Li, Z., Jia, K., Chen, X., Guo, J., Zheng, Z., Chen, W., et al. (2023b). Exposure to butylparaben induces craniofacial bone developmental toxicity in zebrafish (Danio rerio) embryos. Ecotoxicol. Environ. Saf. 265, 115523. doi:10.1016/j.ecoenv.2023.115523
Lim, X. Y., Li, J., Yin, H. M., He, M., Li, L., and Zhang, T. (2023). Stabilization of essential oil: polysaccharide-based drug delivery system with plant-like structure based on biomimetic concept. Polym. (Basel) 15, 3338. doi:10.3390/polym15163338
Ling, C. Q., Liao, H. X., Wen, J. R., Nie, H. Y., Zhang, L. Y., Xu, F. R., et al. (2024). Investigation of the inhibitory effects of Illicium verum essential oil nanoemulsion on Fusarium proliferatum via combined transcriptomics and metabolomics analysis. Curr. Microbiol. 81, 182. doi:10.1007/s00284-024-03724-7
Ling, Q., Zhang, B., Wang, Y., Xiao, Z., Hou, J., Xiao, C., et al. (2022). Chemical composition and antioxidant activity of the essential oils of citral-rich chemotype Cinnamomum camphora and Cinnamomum bodinieri. Mol. Basel, Switz. 27, 7356. doi:10.3390/molecules27217356
Liu, D., Liu, R., Zhuang, Z., Yao, B., Qin, C., Ma, F., et al. (2023). Preparation of self-microemulsion solids of Kaempferia galanga (L.) volatile oil and its effect on rats with gastric ulcer. AAPS PharmSciTech 24, 243. doi:10.1208/s12249-023-02693-7
Liu, F., Xu, T., He, J., Jiang, Y., Qu, L., Wang, L., et al. (2024a). Exploring the potential of white birch sap: a natural alternative to traditional skin whitening agents with reduced side effects. Heliyon 10, e26715. doi:10.1016/j.heliyon.2024.e26715
Liu, H., Dong, J., Du, R., Gao, Y., and Zhao, P. (2024b). Collagen study advances for photoaging skin, Photodermatol. Photoimmunol. Photomed., Collagen study advances for photoaging skin. Photodermatol. Photoimmunol. and Photomed. 40: e12931. doi:10.1111/phpp.12931
Liu, L., Cao, W., Xia, M., Tian, C., Wu, W., Cai, Y., et al. (2022a). Self-emulsifying drug delivery system enhances tissue distribution of cinnamaldehyde by altering the properties of the mucus layer. AAPS PharmSciTech 23, 261. doi:10.1208/s12249-022-02416-4
Liu, P., and Zhang, A. (2017). Historical evolution of western fitness culture. J. Phys. Educ. 24, 20–26. doi:10.16237/j.cnki.cn44-1404/g8.20170723.005
Liu, X., Li, X., and Ma, J. (2024c). Beverage consumption and facial skin aging: evidence from Mendelian randomization analysis. J. Cosmet. dermatology 23, 1800–1807. doi:10.1111/jocd.16153
Liu, X. J., Li, Y., Su, S. L., Wei, D. D., Yan, H., Guo, S., et al. (2022b). Comparative analysis of chemical composition andAntibacterial and anti-inflammatory activities of theEssential oils from Chrysanthemum morifolium ofDifferent flowering stages and different parts. Evidence-based complementary Altern. Med. eCAM 2022, 5954963. doi:10.1155/2022/5954963
Liu, Y., Geng, C., Zeng, H., Kai, Y., and Lu, Y. (2024d). Chitosan coatings containing thyme essential oil enhance the quality of snakehead (Channa striata) during chilled storage verified by metabolomics approaches. J. Food Sci. 89, 7397–7409. doi:10.1111/1750-3841.17442
Liu, Y., Pan, L., Li, T., Tang, T., Xu, R., Duan, X., et al. (2024e). Improving the performance of kraft paper by cinnamon essential oil/soybean protein isolate microcapsules and konjac glucomannan for citrus preservation. Int. J. Biol. Macromol. 277, 134308. doi:10.1016/j.ijbiomac.2024.134308
Liu, Z., Wang, S., Liang, H., Zhou, J., Zong, M., Cao, Y., et al. (2024f). A review of advancements in chitosan-essential oil composite films: better and sustainable food preservation with biodegradable packaging. Int. J. Biol. Macromol. 274, 133242. doi:10.1016/j.ijbiomac.2024.133242
Llaguno-Munive, M., Vazquez-Lopez, M. I., and Garcia-Lopez, P. (2024). Solid lipid nanoparticles, an alternative for the treatment of triple-negative breast cancer. Int. J. Mol. Sci. 25, 10712. doi:10.3390/ijms251910712
Lodovichi, J., Landucci, E., Pitto, L., Gisone, I., D'Ambrosio, M., Luceri, C., et al. (2022). Evaluation of the increase of the thymoquinone permeability formulated in polymeric micelles: in vitro test and in vivo toxicity assessment in Zebrafish embryos. Eur. J. Pharm. Sci. 169, 106090. doi:10.1016/j.ejps.2021.106090
Lohani, A., Mishra, A. K., and Verma, A. (2019). Cosmeceutical potential of geranium and calendula essential oil: determination of antioxidant activity and in vitro sun protection factor. J. Cosmet. dermatology 18, 550–557. doi:10.1111/jocd.12789
Long, Y., Li, D., Yu, S., Zhang, Y. L., Liu, S. Y., Wan, J. Y., et al. (2022). Natural essential oils: a promising strategy for treating cardio-cerebrovascular diseases. J. Ethnopharmacol. 297, 115421. doi:10.1016/j.jep.2022.115421
Long, Y., Yu, S., Li, D., Shi, A., Ma, Y., Deng, J., et al. (2023). Preparation, characterization and safety evaluation of Ligusticum chuanxiong essential oils liposomes for treatment of cerebral ischemia-reperfusion injury. Food Chem. Toxicol. 175, 113723. doi:10.1016/j.fct.2023.113723
Ly, T. B., Bui, B. T. A., Nguyen, Y. T. H., Le, K. A., Tran, V. T., and Le, P. K. (2024). Innovative ultrasonic emulsification of cinnamon essential oil pickering emulsion stabilized by rice straw-derived cellulose nanocrystals. Int. J. Biol. Macromol. 276, 134084. doi:10.1016/j.ijbiomac.2024.134084
Ma, D., Yang, B., Zhao, J., Yuan, D., and Li, Q. (2024). Advances in protein-based microcapsules and their applications: a review. Int. J. Biol. Macromol. 263, 129742. doi:10.1016/j.ijbiomac.2024.129742
Ma, J., Fan, J., Xia, Y., Kou, X., Ke, Q., and Zhao, Y. (2023). Preparation of aromatic β-cyclodextrin nano/microcapsules and corresponding aromatic textiles: a review. Carbohydr. Polym. 308, 120661. doi:10.1016/j.carbpol.2023.120661
Ma, Z. Y., and Lu, Y. (2020). Melanin synthesis and regulation in vivo and commonly used melanin inhibitors from natural products and traditional Chinese medicine. Zhongguo Zhong yao za zhi = Zhongguo zhongyao zazhi = China J. Chin. materia medica 45, 5898–5916. doi:10.19540/j.cnki.cjcmm.20200818.601
Maache, S., Zbadi, L., Ghouizi, A. E., Soulo, N., Saghrouchni, H., Siddique, F., et al. (2023). Antioxidant and antimicrobial effects of essential oils from two salvia species with in vitro and in silico analysis targeting 1AJ6 and 1R4U proteins. Sci. Rep. 13, 14038. doi:10.1038/s41598-023-41178-2
Mahalapbutr, P., Sabuakham, S., Nasoontorn, S., Rungrotmongkol, T., Silsirivanit, A., and Suriya, U. (2023). Discovery of amphotericin B, an antifungal drug as tyrosinase inhibitor with potent anti-melanogenic activity. Int. J. Biol. Macromol. 246, 125587. doi:10.1016/j.ijbiomac.2023.125587
Mahendran, G., and Vimolmangkang, S. (2023). Chemical compositions, antioxidant, antimicrobial, and mosquito larvicidal activity of Ocimum americanum L. and Ocimum basilicum L. leaf essential oils. BMC complementary Med. Ther. 23, 390. doi:10.1186/s12906-023-04214-2
Mahmoud, N. M., Abdel Moneim, A. M. Y., Darweesh, O., El Zahaby, E. I., Elshaarawy, R. F. M., Hassan, Y. A., et al. (2024). Fabrication and characteristics of new quaternized chitosan nanocapsules loaded with thymol or thyme essential oil as effective SARS-CoV-2 inhibitors. RSC Adv. 14, 29330–29343. doi:10.1039/d4ra03298e
Malkawi, A., Jalil, A., Nazir, I., Matuszczak, B., Kennedy, R., and Bernkop-Schnürch, A. (2020). Self-emulsifying drug delivery systems: hydrophobic drug polymer complexes provide a sustained release in vitro. Mol. Pharm. 17, 3709–3719. doi:10.1021/acs.molpharmaceut.0c00389
Mansour, R. B., Wasli, H., Bourgou, S., Khamessi, S., Ksouri, R., Megdiche-Ksouri, W., et al. (2023). Insights on juniperus phoenicea essential oil as potential anti-proliferative, anti-tyrosinase, and antioxidant candidate. Mol. Basel, Switz. 28, 7547. doi:10.3390/molecules28227547
Manzoor, A., Asif, M., Khalid, S. H., Ullah, K. I., and Asghar, S. (2023). Nanosizing of lavender, basil, and clove essential oils into microemulsions for enhanced antioxidant potential and antibacterial and antibiofilm activities. ACS Omega 8, 40600–40612. doi:10.1021/acsomega.3c05394
Matadamas-Ortiz, A., Hernández-Hernández, E., Castaño-Tostado, E., Amaro-Reyes, A., García-Almendárez, B. E., Velazquez, G., et al. (2022). Long-term refrigerated storage of beef using an active edible film reinforced with mesoporous silica nanoparticles containing oregano essential oil (Lippia graveolens Kunth). Int. J. Mol. Sci. 24, 92. doi:10.3390/ijms24010092
Maurya, R., Misro, L., Boini, T., Radhakrishnan, T., Nair, P. G., Gaidhani, S. N., et al. (2024). Transforming medicinal oil into advanced gel: an update on advancements. Gels 10, 342. doi:10.3390/gels10050342
McMullen, R. L., and Dell’Acqua, G. (2023). History of natural ingredients in cosmetics. Cosmetics 10, 71. doi:10.3390/cosmetics10030071
Merola, C., Caioni, G., Bertolucci, C., Lucon-Xiccato, T., Savaşçı, B. B., Tait, S., et al. (2024). Embryonic and larval exposure to propylparaben induces developmental and long-term neurotoxicity in zebrafish model. Sci. total Environ. 912, 168925. doi:10.1016/j.scitotenv.2023.168925
Messias, M. A., Ferreira, S. M., Tavares, L., and Santos, L. (2023). A comparative study between onion peel extracts, free and complexed with β-cyclodextrin, as a natural UV filter to cosmetic formulations. Int. J. Mol. Sci. 24, 15854. doi:10.3390/ijms242115854
Michalak, M., Pierzak, M., Kręcisz, B., and Suliga, E. (2021). Bioactive compounds for skin health: a review. Nutrients 13, 203. doi:10.3390/nu13010203
Moghrovyan, A., Sahakyan, N., Babayan, A., Chichoyan, N., Petrosyan, M., and Trchounian, A. (2019). Essential oil and ethanol extract of oregano (Origanum vulgare L.) from Armenian flora as a natural source of terpenes, flavonoids and other phytochemicals with antiradical, antioxidant, metal chelating, tyrosinase inhibitory and antibacterial activity. Curr. Pharm. Des. 25, 1809–1816. doi:10.2174/1381612825666190702095612
Mohammadzadeh, P., Marand, S. A., Almasi, H., Zeynali, F., and Moradi, M. (2024). Bacterial nanocellulose-based nanopaper activated by β-cyclodextrin/Salvia officinalis essential oil complexes for shelf life extension of shrimp. Int. J. Biol. Macromol. 275, 133354. doi:10.1016/j.ijbiomac.2024.133354
Mohanta, O., Ray, A., Jena, S., Sahoo, A., Panda, S. S., Das, P. K., et al. (2023). Mesosphaerum suaveolens essential oil attenuates inflammatory response and oxidative stress in LPS-stimulated RAW 264.7 macrophages by regulating NF-κB signaling pathway. Mol. Basel, Switz. 28, 5817. doi:10.3390/molecules28155817
Mohanty, S., Ray, A., Naik, P. K., Sahoo, A., Jena, S., Das, P. K., et al. (2023). Variation in yield, chemical composition and biological activities of essential oil of three Curcuma species: a comparative evaluation of hydrodistillation and solvent-free microwave extraction methods. Molecules 28, 4434. doi:10.3390/molecules28114434
Muthusamy, V., Govindhan, T., Amirthalingam, M., Pottanthara Ashokan, A., Thangavel, H., Palanisamy, S., et al. (2024). Chitosan nanoparticles encapsulated Piper betle essential oil alleviates Alzheimer's disease associated pathology in Caenorhabditis elegans. Int. J. Biol. Macromol. 279, 135323. doi:10.1016/j.ijbiomac.2024.135323
Najafi-Taher, R., Jafarzadeh, K. A., Eslami Farsani, V., Mehdizade, R. N., Moghimi, H. R., Ehsani, A., et al. (2022). A topical gel of tea tree oil nanoemulsion containing adapalene versus adapalene marketed gel in patients with acne vulgaris: a randomized clinical trial. Arch. Dermatol Res. 314, 673–679. doi:10.1007/s00403-021-02267-2
Najahi-Missaoui, W., Arnold, R. D., and Cummings, B. S. (2020). Safe nanoparticles: are we there yet? Int. J. Mol. Sci. 22, 385. doi:10.3390/ijms22010385
Narimani, R., Moghaddam, M., Mahmoodi, S. M., and Samiei, L. (2022). A comparative study on essential oil profile and antioxidant activity in different parts of three ferulago species. Chem. and Biodivers. 19, e202100513. doi:10.1002/cbdv.202100513
Nascimento, N. S., Torres-Obreque, K. M., Oliveira, C. A., Rabelo, J., Baby, A. R., Long, P. F., et al. (2024). Enzymes for dermatological use. Exp. Dermatol. 33, e15008. doi:10.1111/exd.15008
Nasir Shah, S., Khan, I., Tul, M. S., Hayat, A., Ur, R. M., Ali, S. T., et al. (2023). Bioactive, antioxidant and antimicrobial properties of chemically fingerprinted essential oils extracted from Eucalyptus globulus: in-vitro and in-silico investigations. Front. Chem. 11, 1287317. doi:10.3389/fchem.2023.1287317
Navabhatra, A., Maniratanachote, R., and Yingngam, B. (2022). Antiphotoaging properties of Zingiber montanum essential oil isolated by solvent-free microwave extraction against ultraviolet B-irradiated human dermal fibroblasts. Toxicol. Res. 38, 235–248. doi:10.1007/s43188-021-00107-z
Nemattalab, M., Rohani, M., Evazalipour, M., and Hesari, Z. (2022). Formulation of Cinnamon (Cinnamomum verum) oil loaded solid lipid nanoparticles and evaluation of its antibacterial activity against Multi-drug Resistant Escherichia coli. BMC Complement. Med. Ther. 22, 289. doi:10.1186/s12906-022-03775-y
Nikolic, D., Bosco, L., Moschetti, M., Tinnirello, V., Pucci, M., Corleone, V., et al. (2023). Anti-inflammatory properties of an aldehydes-enriched fraction of grapefruit essential oil. J. food Sci. 88, 1172–1187. doi:10.1111/1750-3841.16461
Noor, A., Jamil, S., Sadeq, T. W., Mohammed Ameen, M. S., and Kohli, K. (2023). Development and evaluation of nanoformulations containing timur oil and rosemary oil for treatment of topical fungal infections. Gels 9, 516. doi:10.3390/gels9070516
Nowak, A., Duchnik, W., Makuch, E., Kucharski, Ł., Ossowicz-Rupniewska, P., Cybulska, K., et al. (2021). Epilobium angustifolium L. Essential oil-biological activity and enhancement of the skin penetration of drugs-in vitro study. Mol. Basel, Switz. 26, 7188. doi:10.3390/molecules26237188
Nowak-Lange, M., Niedziałkowska, K., and Lisowska, K. (2022). Cosmetic preservatives: hazardous micropollutants in need of greater attention? Int. J. Mol. Sci. 23, 14495. doi:10.3390/ijms232214495
Nurani, A. M., Kikuchi, K., Iino, M., Shirasugi, Y., Sonoki, A., Fujimura, T., et al. (2023). “Development of a method for evaluating skin dullness: a mathematical model explaining dullness by the color, optical properties, and microtopography of the skin,” Skin. Res. Technol., 29. e13407. doi:10.1111/srt.13407
Obiștioiu, D., Hulea, A., Cocan, I., Alexa, E., Negrea, M., Popescu, I., et al. (2023). Boswellia essential oil: natural antioxidant as an effective antimicrobial and anti-inflammatory agent. Antioxidants Basel, Switz. 12, 1807. doi:10.3390/antiox12101807
Paiva-Santos, A. C., Ferreira, L., Peixoto, D., Silva, F., Soares, M. J., Zeinali, M., et al. (2022). Cyclodextrins as an encapsulation molecular strategy for volatile organic compounds- Pharmaceutical applications. Colloids Surf. B Biointerfaces 218, 112758. doi:10.1016/j.colsurfb.2022.112758
Pandey, V. K., Srivastava, S., Ashish, , Dash, K. K., Singh, R., Dar, A. H., et al. (2023). Bioactive properties of clove (Syzygium aromaticum) essential oil nanoemulsion: a comprehensive review. Heliyon 10, e22437. doi:10.1016/j.heliyon.2023.e22437
Pandita, G., de Souza, C. K., Gonçalves, M. J., Jasińska, J. M., Jamróz, E., and Roy, S. (2024). Recent progress on Pickering emulsion stabilized essential oil added biopolymer-based film for food packaging applications: a review. Int. J. Biol. Macromol. 269, 132067. doi:10.1016/j.ijbiomac.2024.132067
Pandur, E., Balatinácz, A., Micalizzi, G., Mondello, L., Horváth, A., Sipos, K., et al. (2021). Anti-inflammatory effect of lavender (Lavandula angustifolia Mill.) essential oil prepared during different plant phenophases on THP-1 macrophages. BMC complementary Med. Ther. 21, 287. doi:10.1186/s12906-021-03461-5
Panyod, S., Wu, W. K., Hsieh, Y. C., Tseng, Y. J., Peng, S. Y., Chen, R. A., et al. (2024). Ginger essential oil prevents NASH progression by blocking the NLRP3 inflammasome and remodeling the gut microbiota-LPS-TLR4 pathway in mice. Nutr. Diabetes 14, 65. doi:10.1038/s41387-024-00306-1
Park, M. K., Cha, J. Y., Kang, M. C., Jang, H. W., and Choi, Y. S. (2024). The effects of different extraction methods on essential oils from orange and tangor: from the peel to the essential oil. Food Sci. Nutr. 12, 804–814. doi:10.1002/fsn3.3785
Pastare, L., Berga, M., Kienkas, L., Boroduskis, M., Ramata-Stunda, A., Reihmane, D., et al. (2023). Exploring the potential of supercritical fluid extraction of Matricaria chamomilla white ray florets as a source of bioactive (cosmetic) ingredients. Antioxidants Basel, Switz. 12, 1092. doi:10.3390/antiox12051092
Pavlić, B., Teslić, N., Zengin, G., Đurović, S., Rakić, D., Cvetanović, A., et al. (2021). Antioxidant and enzyme-inhibitory activity of peppermint extracts and essential oils obtained by conventional and emerging extraction techniques. Food Chem. 338, 127724. doi:10.1016/j.foodchem.2020.127724
Pedreiro, S., Figueirinha, A., Cavaleiro, C., Cardoso, O., Donato, M. M., Salgueiro, L., et al. (2023). Exploiting the crithmum maritimum L. Aqueous extracts and essential oil as potential preservatives in food, feed, pharmaceutical and cosmetic industries. Antioxidants Basel, Switz. 12, 252. doi:10.3390/antiox12020252
Pereira, I., Zielińska, A., Ferreira, N. R., Silva, A. M., and Souto, E. B. (2018). Optimization of linalool-loaded solid lipid nanoparticles using experimental factorial design and long-term stability studies with a new centrifugal sedimentation method. Int. J. Pharm. 549, 261–270. doi:10.1016/j.ijpharm.2018.07.068
Piipponen, M., Li, D., and Landén, N. X. (2020). The immune functions of keratinocytes in skin wound healing. Int. J. Mol. Sci. 21, 8790. doi:10.3390/ijms21228790
Pires, H. M., Bastos, L. M., da Silva, E. F., Fonseca, B. B., Sommerfeld, S., de Oliveira Junior, R. J., et al. (2024). Antimicrobial activity of essential-oil-based nanostructured lipid carriers against Campylobacter spp. Isolated from chicken carcasses. Pharmaceutics 16, 922. doi:10.3390/pharmaceutics16070922
Pires Rodrigues de Almeida Ribeiro, F., Fernandes, M. L., Brito, Q. D., Botelho, M. A., de Souza Siqueira, B. D. R., Santana de Lima, R., et al. (2024). Wound healing effect of Lippia sidoides and myracrodruon urundeuva nanogel. Chem. Biodivers. 21, e202302043. doi:10.1002/cbdv.202302043
Qiu, Y., Wang, Y., and Li, Y. (2022). Solvent-free microwave extraction of essential oils from Litsea cubeba (lour.) Pers. At different harvesting times and their skin-whitening cosmetic potential. Antioxidants Basel, Switz. 11, 2389. doi:10.3390/antiox11122389
Raka, R. N., Zhiqian, D., Yue, Y., Luchang, Q., Suyeon, P., Junsong, X., et al. (2022). Pingyin rose essential oil alleviates LPS-Induced inflammation in RAW 264.7 cells via the NF-κB pathway: an integrated in vitro and network pharmacology analysis. BMC complementary Med. Ther. 22, 272. doi:10.1186/s12906-022-03748-1
Ranjbar, R., Zarenezhad, E., Abdollahi, A., Nasrizadeh, M., Firooziyan, S., Namdar, N., et al. (2023). Nanoemulsion and nanogel containing Cuminum cyminum L essential oil: antioxidant, anticancer, antibacterial, and antilarval properties. J. Trop. Med. 2023, 5075581. doi:10.1155/2023/5075581
Riaz, M., Khalid, R., Afzal, M., Anjum, F., Fatima, H., Zia, S., et al. (2023). Phytobioactive compounds as therapeutic agents for human diseases: a review. Food Sci. Nutr. 11, 2500–2529. doi:10.1002/fsn3.3308
Rodrigues Arruda, T., Campos Bernardes, P., Robledo Fialho, E. M. A., and de, F. F. S. N. (2022). Natural bioactives in perspective: the future of active packaging based on essential oils and plant extracts themselves and those complexed by cyclodextrins. Food Res. Int. 156, 111160. doi:10.1016/j.foodres.2022.111160
Ru, H., Luan, F., Shi, Y., Zhang, X., Guo, D., Zhai, B., et al. (2024). Modified Cinnabaris-stabilized Pickering emulsions loaded with the essential oil of Acorus tatarinowii Schott: preparation, characterization and in vitro evaluation. RSC Adv. 14, 31367–31384. doi:10.1039/d4ra05168h
Russo, C., Lombardo, G. E., Bruschetta, G., Rapisarda, A., Maugeri, A., and Navarra, M. (2024). Bergamot byproducts: a sustainable source to counteract inflammation. Nutrients 16, 259. doi:10.3390/nu16020259
Safta, D. A., Bogdan, C., and Moldovan, M. L. (2024). SLNs and NLCs for skin applications: enhancing the bioavailability of natural bioactives. Pharmaceutics 16, 1270. doi:10.3390/pharmaceutics16101270
Saiyudthong, S., Pongmayteegul, S., Marsden, C. A., and Phansuwan-Pujito, P. (2015). Anxiety-like behaviour and c-fos expression in rats that inhaled vetiver essential oil. Nat. Prod. Res. 29, 2141–2144. doi:10.1080/14786419.2014.992342
Salem, M. A., Manaa, E. G., Osama, N., Aborehab, N. M., Ragab, M. F., Haggag, Y. A., et al. (2022). Coriander (Coriandrum sativum L.) essential oil and oil-loaded nano-formulations as an anti-aging potentiality via TGFβ/SMAD pathway. Sci. Rep. 12, 6578. doi:10.1038/s41598-022-10494-4
Sangthong, S., Promputtha, I., Pintathong, P., and Chaiwut, P. (2022). Chemical constituents, antioxidant, anti-tyrosinase, cytotoxicity, and anti-melanogenesis activities of etlingera elatior (jack) leaf essential oils. Mol. Basel, Switz. 27, 3469. doi:10.3390/molecules27113469
Santos Pimentel, L., Sommerfeld, S., Fernanda de Sousa Braga, P., Flores, C. A., Beatriz Fonseca, B., Machado, B. L., et al. (2024). Antitumor activity of essential oils-based nanostructured lipid carriers on prostate cancer cells. Int. J. Pharm. 657, 124149. doi:10.1016/j.ijpharm.2024.124149
Satrialdi, H. N. N., Rihad, R. Q., and Utami, R. A. (2024). The incorporation of clove essential oil into nanostructured lipid carrier for improvement of the delivery and antioxidant effects on the fibroblast cells. Pharm. Nanotechnol. doi:10.2174/0122117385304491240320040711
Sgoifo, A., Carnevali, L., Pattini, E., Carandina, A., Tanzi, G., Del Canale, C., et al. (2021). Psychobiological evidence of the stress resilience fostering properties of a cosmetic routine. Stress Amsterdam, Neth. 24, 53–63. doi:10.1080/10253890.2020.1750590
Shahabi, N., Fallah, A. A., Sami, M., and Habibian, D. S. (2024). Effect of tragacanth gum-chitin nanofiber film containing free or nano-encapsulated cumin essential oil on the quality of chilled Turkey burgers packed with oxygen absorber. Food Sci. Nutr. 12, 5605–5618. doi:10.1002/fsn3.4202
Sharma, A. D., Chhabra, R., Rani, J., Chauhan, A., Kaur, I., and Kapoor, G. (2024a). Oil/water (O/W) nanoemulsions developed from essential oil extracted from wildly growing Calotropis gigantea (Linn.) Aiton F.: synthesis, characterization, stability and evaluation of anti-cancerous, anti-oxidant, anti-inflammatory and anti-diabetic activities. J. Biomater. Sci. Polym. Ed. 35, 2506–2527. doi:10.1080/09205063.2024.2384801
Sharma, R., Nath, P. C., Das, P., Rustagi, S., Sharma, M., Sridhar, N., et al. (2024b). Essential oil-nanoemulsion based edible coating: innovative sustainable preservation method for fresh/fresh-cut fruits and vegetables. Food Chem. 460, 140545. doi:10.1016/j.foodchem.2024.140545
Shaverdi, M., Rafiee, Z., Razmjoue, D., Oryan, A., Ghaedi, M., and Abidi, H. (2024). Antibacterial, antioxidant, and anti-giardia properties of the essential oil, hydroalcoholic extract, and green synthesis of the silver nanoparticles of Salvia mirzayanii plant. Sci. Rep. 14, 22866. doi:10.1038/s41598-024-74039-7
Shen, C., Li, J., Meng, Q., Xu, L., and Zhang, G. (2024a). Rhamnolipids stabilized essential oils microemulsion for antimicrobial and fruit preservation. Food Chem. 457, 140167. doi:10.1016/j.foodchem.2024.140167
Shen, C. Y., Jiang, J. G., Zhu, W., and Ou-Yang, Q. (2017). Anti-inflammatory effect of essential oil from citrus aurantium L. Var. amara Engl. J. Agric. food Chem. 65, 8586–8594. doi:10.1021/acs.jafc.7b02586
Shen, G., Qiu, X., Hou, X., Li, M., Zhou, M., Liu, X., et al. (2024b). Development of Zanthoxylum bungeanum essential oil Pickering emulsions using potato protein-chitosan nanoparticles and its application in Mandarin preservation. Int. J. Biol. Macromol. 277, 134100. doi:10.1016/j.ijbiomac.2024.134100
Shen, X., Zhan, M., Wang, Y., Tang, W., Zhang, Q., and Zhang, J. (2023). Exposure to parabens and semen quality in reproductive-aged men. Ecotoxicol. Environ. Saf. 264, 115453. doi:10.1016/j.ecoenv.2023.115453
Shortt, N., Martin, A., Kerse, K., Shortt, G., Vakalalabure, I., Barker, L., et al. (2022). Efficacy of a 3% Kānuka oil cream for the treatment of moderate-to-severe eczema: a single blind randomised vehicle-controlled trial. EClinicalMedicine 51, 101561. doi:10.1016/j.eclinm.2022.101561
Silva-Flores, P. G., Galindo-Rodríguez, S. A., Pérez-López, L. A., and Álvarez-Román, R. (2023). Development of essential oil-loaded polymeric nanocapsules as skin delivery systems: biophysical parameters and dermatokinetics ex vivo evaluation. Molecules 28, 7142. doi:10.3390/molecules28207142
Sim, W. J., Kim, J., Baek, K. S., Lim, W., and Lim, T. G. (2023). Porcine placenta peptide inhibits UVB-induced skin wrinkle formation and dehydration: insights into MAPK signaling pathways from in vitro and in vivo studies. Int. J. Mol. Sci. 25, 83. doi:10.3390/ijms25010083
Sobhy, M., Ali, S. S., Cui, H., Lin, L., and El-Sapagh, S. (2023). Exploring the potential of 1,8-cineole from cardamom oil against food-borne pathogens: antibacterial mechanisms and its application in meat preservation. Microb. Pathog. 184, 106375. doi:10.1016/j.micpath.2023.106375
Song, Q., Lu, Q., Zhang, S., Zhang, Z., Huang, J., Li, X., et al. (2024). Preparation and characterization of fennel (Foeniculum vulgare miller) essential oil/hydroxypropyl-β-cyclodextrin inclusion complex and its application for chilled pork preservation. Food Chem. 456, 139887. doi:10.1016/j.foodchem.2024.139887
Sosa, L., Espinoza, L. C., Valarezo, E., Bozal, N., Calpena, A., Fábrega, M. J., et al. (2023). Therapeutic applications of essential oils from native and cultivated Ecuadorian plants: cutaneous candidiasis and dermal anti-inflammatory activity. Mol. Basel, Switz. 28, 5903. doi:10.3390/molecules28155903
Subroto, E., Andoyo, R., and Indiarto, R. (2023). Solid lipid nanoparticles: review of the current research on encapsulation and delivery systems for active and antioxidant compounds. Antioxidants (Basel) 12, 633. doi:10.3390/antiox12030633
Sun, R., Li, Y., Su, R., Cai, X., Kong, Y., Jiang, T., et al. (2024). Antibacterial effect of ultrasound combined with Litsea cubeba essential oil nanoemulsion on Salmonella Typhimurium in kiwifruit juice. Int. J. Food Microbiol. 426, 110898. doi:10.1016/j.ijfoodmicro.2024.110898
Tamagno, W. A., Santini, W., Alves, C., Vanin, A. P., Pompermaier, A., Bilibio, D., et al. (2022). Neuroprotective and antioxidant effects of pitaya fruit on Cu-induced stress in adult zebrafish. J. Food Biochem. 46, e14147. doi:10.1111/jfbc.14147
Tansaoui, H., Bouazizi, N., Behary, N., Campagne, C., El-Achari, A., and Vieillard, J. (2023). Assessing alternative pre-treatment methods to promote essential oil fixation into cotton and polyethylene terephthalate fiber: a comparative study. Polymers 15, 1362. doi:10.3390/polym15061362
Tanwar, M., Rani, A., Gautam, N., Talegaonkar, S., and Gupta, R. K. (2024). Essential oils loaded carboxymethylated Cassia fistula gum-based novel hydrogel films for wound healing. Int. J. Biol. Macromol. 278, 134682. doi:10.1016/j.ijbiomac.2024.134682
Thakur, D., Kaur, G., Puri, A., and Nanda, R. (2021). Therapeutic potential of essential oil-based microemulsions: reviewing state-of-the-art. Curr. Drug Deliv. 18, 1218–1233. doi:10.2174/1567201818666210217161240
Tian, M., Xie, D., Yang, Y., Tian, Y., Jia, X., Wang, Q., et al. (2023). Hedychium flavum flower essential oil: chemical composition, anti-inflammatory activities and related mechanisms in vitro and in vivo. J. Ethnopharmacol. 301, 115846. doi:10.1016/j.jep.2022.115846
Tian, Y., Jia, X., Wang, Q., Lu, T., Deng, G., Tian, M., et al. (2022). Antioxidant, antibacterial, enzyme inhibitory, and anticancer activities and chemical composition of Alpinia galanga flower essential oil. Pharm. Basel, Switz. 15, 1069. doi:10.3390/ph15091069
Tsitlakidou, P., Tasopoulos, N., Chatzopoulou, P., and Mourtzinos, I. (2023). Current status, technology, regulation and future perspectives of essential oils usage in the food and drink industry. J. Sci. food Agric. 103, 6727–6751. doi:10.1002/jsfa.12695
Uchida, D. T., Siqueira, G. F., Dos Reis, E. M., Hegeto, F. L., Medina Neto, A., Reis, A. V., et al. (2021). Design of nanostructured lipid carriers containing cymbopogon martinii (palmarosa) essential oil against Aspergillus nomius. Molecules 26, 4825. doi:10.3390/molecules26164825
Vase, H., Nemattalab, M., Rohani, M., and Hesari, Z. (2023). Comparison of chitosan and SLN nano-delivery systems for antibacterial effect of tea tree (Melaleuca alternifolia) oil against P. aeruginosa and S. aureus. Lett. Appl. Microbiol. 76, ovad130. doi:10.1093/lambio/ovad130
Vella, F. M., Calandrelli, R., Cautela, D., Fiume, I., Pocsfalvi, G., and Laratta, B. (2020). Chemometric screening of fourteen essential oils for their composition and biological properties. Mol. Basel, Switz. 25, 5126. doi:10.3390/molecules25215126
Visakh, N. U., Pathrose, B., Chellappan, M., Ranjith, M. T., Sindhu, P. V., and Mathew, D. (2023). Extraction and chemical characterisation of agro-waste from turmeric leaves as a source of bioactive essential oils with insecticidal and antioxidant activities. Waste Manag. 169, 1–10. doi:10.1016/j.wasman.2023.06.030
Vora, L. K., Gholap, A. D., Hatvate, N. T., Naren, P., Khan, S., Chavda, V. P., et al. (2024). Essential oils for clinical aromatherapy: a comprehensive review. J. Ethnopharmacol. 330, 118180. doi:10.1016/j.jep.2024.118180
Wang, D., Wang, W., Zhang, Q., Liu, C., Li, X., Zuo, K., et al. (2024a). Studies on the preparation of a microemulsion formulation of Matricaria recutita essential oil and the treatment of 2,4-dinitro-chlorobenzene- induced eczema in mice by inhibiting inflammation. Curr. Drug Deliv. 22. doi:10.2174/0115672018315617240826133041
Wang, H., Zheng, Y., Tang, X., and Zhang, T. (2023a). Formulation of a stable oil-in-water microemulsion of Torreya grandis cv. Merrillii aril essential oil and its application in loquat fruit preservation. Foods 12, 4005. doi:10.3390/foods12214005
Wang, S. Y., Cai, L., Yang, N., Xu, F. F., Wu, Y. S., and Liu, B. (2023b). Chemical composition of the Kaempferia galanga L. essential oil and its in vitro and in vivo antioxidant activities. Front. Nutr. 10, 1080487. doi:10.3389/fnut.2023.1080487
Wang, Y. L., Lang, D. Q., Wu, C., Chen, Q. C., Lin, S. X., Li, X. Y., et al. (2024b). Chemical composition and antibacterial and antiulcerative colitis activities of essential oil from pruni semen. J. Agric. food Chem. 72, 1096–1113. doi:10.1021/acs.jafc.3c06442
Węglarz, Z., Kosakowska, O., Pióro-Jabrucka, E., Przybył, J. L., Gniewosz, M., Kraśniewska, K., et al. (2022). Antioxidant and antibacterial activity of Helichrysum italicum (roth) G. Don. From central Europe. Pharm. Basel, Switz. 15, 735. doi:10.3390/ph15060735
Wei, Z., Huang, L., Feng, X., Cui, F., Wu, R., Kong, Q., et al. (2023). Development of functional, sustainable pullulan-sodium alginate-based films by incorporating essential oil microemulsion for chilled pork preservation. Int. J. Biol. Macromol. 253, 127257. doi:10.1016/j.ijbiomac.2023.127257
Weisany, W., Yousefi, S., Soufiani, S. P., Pashang, D., McClements, D. J., and Ghasemlou, M. (2024). Mesoporous silica nanoparticles: a versatile platform for encapsulation and delivery of essential oils for food applications. Adv. Colloid Interface Sci. 325, 103116. doi:10.1016/j.cis.2024.103116
Won, Y. R., Won, K. J., Kim, D. Y., Kim, M. J., Hong, B. S., and Lee, H. M. (2022). Chemical composition of impatiens textori miq. Flower absolute and its potential wound repair and anti-melanogenesis-promoting activities in skin cells. Pharm. Basel, Switz. 15, 1397. doi:10.3390/ph15111397
Wu, H., Jiang, X., Dong, Z., Fan, Q., Huang, J., Liu, H., et al. (2024). New insights into the influence of encapsulation materials on the feasibility of ultrasonic-assisted encapsulation of Mosla chinensis essential oil. Ultrason. Sonochem 103, 106787. doi:10.1016/j.ultsonch.2024.106787
Xiao, Z., Xia, J., Zhao, Q., Niu, Y., and Zhao, D. (2022). Maltodextrin as wall material for microcapsules: a review. Carbohydr. Polym. 298, 120113. doi:10.1016/j.carbpol.2022.120113
Xie, J., Wang, C., Wang, N., Zhu, S., Mei, L., Zhang, X., et al. (2020). Graphdiyne nanoradioprotector with efficient free radical scavenging ability for mitigating radiation-induced gastrointestinal tract damage. Biomaterials 244, 119940. doi:10.1016/j.biomaterials.2020.119940
Xing, Z., Xu, Y., Feng, X., Gao, C., Wu, D., Cheng, W., et al. (2024). Fabrication of cinnamon essential oil nanoemulsions with high antibacterial activities via microfluidization. Food Chem. 456, 139969. doi:10.1016/j.foodchem.2024.139969
Xu, X., Liu, A., Hu, S., Ares, I., Martínez-Larrañaga, M. R., Wang, X., et al. (2021). Synthetic phenolic antioxidants: metabolism, hazards and mechanism of action. Food Chem. 353, 129488. doi:10.1016/j.foodchem.2021.129488
Xu, Y., Zhao, M., Cao, J., Fang, T., Zhang, J., Zhen, Y., et al. (2023). Applications and recent advances in transdermal drug delivery systems for the treatment of rheumatoid arthritis. Acta Pharm. Sin. B 13, 4417–4441. doi:10.1016/j.apsb.2023.05.025
Yan, X., Ma, X., Dai, D., Yan, X., Han, X., Bao, X., et al. (2024). Potent pigmentation inhibitory activity of incensole-enriched frankincense volatile oil-identification, efficacy and mechanism. J. Cosmet. Dermatol 23, 244–255. doi:10.1111/jocd.15887
Yang, J., Lee, S. Y., Jang, S. K., Kim, K. J., and Park, M. J. (2023a). Inhibition of melanogenesis by essential oils from the citrus cultivars peels. Int. J. Mol. Sci. 24, 4207. doi:10.3390/ijms24044207
Yang, N., Ai, X., Cheng, K., Wu, Y., Lu, Z., Liu, Z., et al. (2024a). A compound essential oil alters stratum corneum structure, potentially promoting the transdermal permeation of hydrophobic and hydrophilic ingredients. Curr. Drug Deliv. 21, 744–752. doi:10.2174/1567201820666230120122206
Yang, W., Gong, Y., Wang, Y., Wu, C., Zhang, X., Li, J., et al. (2024b). Design of gum Arabic/gelatin composite microcapsules and their cosmetic applications in encapsulating tea tree essential oil. RSC Adv. 14, 4880–4889. doi:10.1039/d3ra08526k
Yang, Y. L., Zhou, C., Chen, Q., Shen, S. Z., Li, J. D., Wang, X. L., et al. (2023b). YAP1/Piezo1 involve in the dynamic changes of lymphatic vessels in UVR-induced photoaging progress to squamous cell carcinoma. J. Transl. Med. 21, 820. doi:10.1186/s12967-023-04458-z
Yao, Y., Feng, J., Ao, N., Zhang, Y., Zhang, J., Wang, Y., et al. (2024). Natural agents derived Pickering emulsion enabled by silica nanoparticles with enhanced antibacterial activity against drug-resistant bacteria. J. Colloid Interface Sci. 678, 1158–1168. doi:10.1016/j.jcis.2024.09.066
Ye, Z. W., Yang, Q. Y., Lin, Q. H., Liu, X. X., Li, F. Q., Xuan, H. D., et al. (2024). Progress of nanopreparation technology applied to volatile oil drug delivery systems. Heliyon 10, e24302. doi:10.1016/j.heliyon.2024.e24302
Yi, W., Na, W., Yang, L., Jiabao, L., Yutian, Z., Zhenfeng, W., et al. (2022). Stability improvement of Chinese medicinal essential oils based on delivery systems and their application in medical field. China J. Chin. Materia Medica 47, 603–610. doi:10.19540/j.cnki.cjcmm.20210915.602
Yin, Y., Xie, Z., Sun, X., Wu, X., Zhang, J., Shi, H., et al. (2023). Effect of butyl paraben on oxidative stress in the liver of mauremys sinensis. Toxics 11, 915. doi:10.3390/toxics11110915
Youssef, J. R., Boraie, N. A., Ismail, F. A., Bakr, B. A., Allam, E. A., and El-Moslemany, R. M. (2024). Brain targeted lactoferrin coated lipid nanocapsules for the combined effects of apocynin and lavender essential oil in PTZ induced seizures. Drug Deliv. Transl. Res. 15, 534–555. doi:10.1007/s13346-024-01610-0
Yu, Z., Lv, H., Zhou, M., Fu, P., and Zhao, W. (2024). Identification and molecular docking of tyrosinase inhibitory peptides from allophycocyanin in Spirulina platensis. J. Sci. food Agric. 104, 3648–3653. doi:10.1002/jsfa.13249
Zahoor, A. F., Hafeez, F., Mansha, A., Kamal, S., Anjum, M. N., Raza, Z., et al. (2023). Bacterial tyrosinase inhibition, hemolytic and thrombolytic screening, and in silico modeling of rationally designed tosyl piperazine-engrafted dithiocarbamate derivatives. Biomedicines 11, 2739. doi:10.3390/biomedicines11102739
Zarenezhad, E., Agholi, M., Ghanbariasad, A., Ranjbar, A., and Osanloo, M. (2021). A nanoemulsion-based nanogel of Citrus limon essential oil with leishmanicidal activity against Leishmania tropica and Leishmania major. J. Parasit. Dis. 45, 441–448. doi:10.1007/s12639-020-01318-1
Zejli, H., Fitat, A., Lefrioui, Y., Siddique, F., Bourhia, M., Bousseraf, F. Z., et al. (2023). Phytochemical analysis and biological activities of essential oils extracted from Origanum grossii and Thymus pallidus: in vitro and in silico analysis. Sci. Rep. 13, 20021. doi:10.1038/s41598-023-47215-4
Zekri, N., Elazzouzi, H., Ailli, A., Gouruch, A. A., Radi, F. Z., El Belghiti, M. A., et al. (2023). Physicochemical characterization and antioxidant properties of essential oils of M. pulegium (L.), M. suaveolens (ehrh.) and M. spicata (L.) from Moroccan middle-atlas. Foods Basel, Switz. 12, 760. doi:10.3390/foods12040760
Zhang, B., Tang, M., Zhang, W., Zhangb, C., Ai, Y., Liang, X., et al. (2021). Chemical composition of Blumea balsamifera and Magnolia sieboldii essential oils and prevention of UV-B radiation-induced skin photoaging. Nat. Prod. Res. 35, 5977–5980. doi:10.1080/14786419.2020.1809401
Zhang, C., Jin, H., Kang, Y., Wu, Y., Zheng, R., Zhang, Z., et al. (2024a). IL-17A-neutralizing antibody ameliorates inflammation and fibrosis in rosacea by antagonizing the CXCL5/CXCR2 axis. FASEB J. 38, e70096. doi:10.1096/fj.202400006R
Zhang, M., Li, M., Zhang, D., Yu, Y., Zhu, K., Zang, X., et al. (2024b). Preparation and investigation of sustained-release nanocapsules containing cumin essential oil for their bacteriostatic properties. Foods 13, 947. doi:10.3390/foods13060947
Zhang, S., Zhang, L., Yu, M., Luo, D., Chen, S., Liu, W., et al. (2022a). Essential oils of Zingiber officinale: chemical composition, in vivo alleviation effects on TPA induced ear swelling in mice and in vitro bioactivities. Front. Nutr. 9, 1043175. doi:10.3389/fnut.2022.1043175
Zhang, W., Jiang, H., Rhim, J. W., Cao, J., and Jiang, W. (2022b). Effective strategies of sustained release and retention enhancement of essential oils in active food packaging films/coatings. Food Chem. 367, 130671. doi:10.1016/j.foodchem.2021.130671
Zhang, X., Guo, X., Sun, J., Chen, Y., Zhang, M., Tang, X., et al. (2024c). Evaluating the hypolipidemic effect of garlic essential oil encapsulated in a novel double-layer delivery system. Colloids Surf. B Biointerfaces 237, 113835. doi:10.1016/j.colsurfb.2024.113835
Zhang, X., Xu, H., Hua, J., Zhu, Z., and Wang, M. (2022c). Protective effects of grapefruit essential oil against Staphylococcus aureus-induced inflammation and cell damage in human epidermal keratinocytes. Chem. and Biodivers. 19, e202200205. doi:10.1002/cbdv.202200205
Zhang, Y., Li, W., Li, J., Zhu, T., and Guo, J. (2024d). Essential oil of Cinnamomum camphora mitigates depression-like behavior in mice by regulating Nrf2/HO-1 pathway and inhibiting inflammatory cytokines. China J. Chin. Materia Medica 49, 779–788. doi:10.19540/j.cnki.cjcmm.20231013.705
Zhao, A., Zhang, Y., Li, F., Chen, L., and Huang, X. (2023). Analysis of the antibacterial properties of compound essential oil and the main antibacterial components of unilateral essential oils. Mol. Basel, Switz. 28, 6304. doi:10.3390/molecules28176304
Zhou, L., Li, J., Kong, Q., Luo, S., Wang, J., Feng, S., et al. (2021). Chemical composition, antioxidant, antimicrobial, and phytotoxic potential of Eucalyptus grandis × E. Urophylla leaves essential oils. Mol. Basel, Switz. 26, 1450. doi:10.3390/molecules26051450
Zhou, W., He, Y., Lei, X., Liao, L., Fu, T., Yuan, Y., et al. (2020). Chemical composition and evaluation of antioxidant activities, antimicrobial, and anti-melanogenesis effect of the essential oils extracted from Dalbergia pinnata (Lour.) Prain. J. Ethnopharmacol. 254, 112731. doi:10.1016/j.jep.2020.112731
Zhou, Y., He, L., Zhang, N., Ma, L., and Yao, L. (2022). Photoprotective effect of artemisia sieversiana ehrhart essential oil against UVB-induced photoaging in mice. Photochem. Photobiol. 98, 958–968. doi:10.1111/php.13561
Zhou, Y., Liao, Q., Li, D., Chen, L., Zhang, H., and Yi, B. (2024). Vitamin D receptor alleviates lipid peroxidation in diabetic nephropathy by regulating ACLY/Nrf2/Keap1 pathway. FASEB J. 38, e70060. doi:10.1096/fj.202401543R
Zhu, H., Liao, D., Mehmood, M. A., Huang, Y., Yuan, W., Zheng, J., et al. (2023). Systolic heart failure induced by butylparaben in zebrafish is caused through oxidative stress and immunosuppression. Ecotoxicol. Environ. Saf. 268, 115692. doi:10.1016/j.ecoenv.2023.115692
Zhu, L., Liu, Y., Liu, J., Qiu, X., and Lin, L. (2024). Preparation and characterization of tea tree essential oil microcapsule-coated packaging paper with bacteriostatic effect. Food Chem. X 23, 101510. doi:10.1016/j.fochx.2024.101510
Zolghadri, S., Beygi, M., Mohammad, T. F., Alijanianzadeh, M., Pillaiyar, T., Garcia-Molina, P., et al. (2023). Targeting tyrosinase in hyperpigmentation: current status, limitations and future promises. Biochem. Pharmacol. 212, 115574. doi:10.1016/j.bcp.2023.115574
Glossary
EOs Essential oils
TYR tyrosinase
DHI 5,6-dihydroxyindol; DOPAdihydroxyphenylalanine
DHICA 5,6-dihydroxy indole-2-carboxylic acid
DCT Dopachrome tautomerase
TRP tyrosinase-related protein
MITF microphthalmia-associated transcription factor
MAPK Mitogen-Activated Protein Kinase
IC50 half-maximal inhibitory concentration
TD50 half-maximal toxicity dose
μM micromolar
ROS reactive oxygen species
B16-F10 malignant melanoma cell line of C57BL/6 J mice
A375 human melanoma cell line
B16BL6 melanoma-associated cell line
α-MSH Alpha-Melanocyte-Stimulating Hormone
B16 melanoma-associated cell line
UVR ultraviolet radiation
LPO lipid peroxide
USFA unsaturated fatty acids
ROS reactive oxygen species
CAT antioxidant enzyme catalase
GST glutathione-S-transferase
BHT 2, 6-di-tert-butyl-p-cresol
FRAP ferric ion reducing antioxidant power
DPPH 2, 2-diphenyl-1-picrylhydrazyl
ABTS 2,2′-azino-bis(3-ethylbenzothiazoline-6-sulfonic acid
EC50 median effective concentration
IL interleukin
IL-6 Interleukin-6
IL-1β Interleukin-1β
COX-2 Cyclooxygenase-2
PGE2 Prostaglandin E2
MMP-1 and MMP-3 Matrix Metalloproteinases-1,-3
TNF-α Tumor Necrosis Factor-α
HaCaT human keratinocyte cell line
HDFn human dermal fibroblast cells
SPF sun protection factor
SOD superoxide dismutase
GSH-Px glutathione peroxidase
MDA malondialdehyde
MIC minimum inhibitory concentration
MBC minimum bactericidal concentration
DIZ diameter of inhibition zone
TNF tumor necrosis factor
LPS lipopolysaccharide
DSS dextran sulfate sodium
PGE prostaglandin E
COX cyclooxygenase
TLR toll-like receptor
p-HBA methyl p-hydroxybenzoate
BuP butyl p-hydroxybenzoate chlorobenzyl etherSBsodium benzoate
ATP adenosine triphosphate
ADP adenosine diphosphate
G+ Gram-positive bacteria
G- Gram-negative bacteria
ZOI zone of inhibition
MIC minimum inhibitory concentration
MBC minimum bactericidal concentration
E. coli Escherichia coli
B. subtilis Bacillus subtilis
S. aureus Staphylococcus aureus
C. albicans Candida albicans
P. aeruginosa Pseudomonas aeruginosa
B. bronchiseptica Bordetella bronchiseptica
S epidermidis Staphylococcus epidermidis
L. monocytogenes Listeria monocytogenes
S. typhimurium Salmonella typhimurium
S. typhi Salmonella typhi
DIZ diameter of inhibition zone
5-LOX Lipoxygenase
NO nitric oxide
iNOS nitric oxide synthase
AA Arachidonic Acid
LS Liposomes
NLS Nanoliposomes
SLNs Solid Lipid Nanoparticles
NLCs Nanostructured Lipid Carriers
ME Microemulsion
NE Nanoemulsion
PE Pickering Emulsion
SEDDS Self-emulsifying drug delivery systems
SNEDDS Self-Nanoemulsified Delivery System
MCs Microcapsules
NCs Nanocapsules
PMs Polymeric Micelles
NGs Nanogels
CS Chitosan
CD Cyclodextrin
MSNPs Mesoporous Silica Nanoparticles.
Keywords: phytotherapy, essential oil, skin care, delivery system, intrinsic efficacy
Citation: Yihan W, Jinjin D, Yingqi W, Guanai M and Xiwu Z (2025) Advances in plant essential oils and drug delivery systems for skincare. Front. Pharmacol. 16:1578280. doi: 10.3389/fphar.2025.1578280
Received: 17 February 2025; Accepted: 03 April 2025;
Published: 17 April 2025.
Edited by:
Yuanyuan Wu, Nanjing University of Chinese Medicine, ChinaReviewed by:
Ravendra Kumar, G. B. Pant University of Agriculture and Technology, IndiaRahul Kumar Maurya, Washington University in St. Louis, United States
Copyright © 2025 Yihan, Jinjin, Yingqi, Guanai and Xiwu. This is an open-access article distributed under the terms of the Creative Commons Attribution License (CC BY). The use, distribution or reproduction in other forums is permitted, provided the original author(s) and the copyright owner(s) are credited and that the original publication in this journal is cited, in accordance with accepted academic practice. No use, distribution or reproduction is permitted which does not comply with these terms.
*Correspondence: Zhang Xiwu, MTQ5NzcyMTA1QHFxLmNvbQ==
†These authors have contributed equally to this work and share first authorship