- 1Department of Endocrinology, The Second Hospital of Jilin University, Changchun, China
- 2Department of Otolaryngology - Head and Neck Surgery, The Second Hospital of Jilin University, Changchun, China
Type 2 diabetes mellitus (T2DM), a chronic condition commonly observed in adults, particularly among the elderly, is characterized by a dysfunctional insulin response that impairs blood glucose regulation, resulting in persistent hyperglycemia. Ginseng, a medicinal plant with significant economic value and a longstanding history of therapeutic use in Asia, has shown efficacy against various diseases. Extensive clinical and experimental studies highlight ginsenosides, its primary bioactive compounds, for their multiple therapeutic effects across a range of conditions, including endocrine, cardiovascular, and central nervous system disorders. Various ginsenoside types have demonstrated potential in lowering blood glucose levels, reducing insulin resistance, and alleviating complications through the modulation of key protein targets and signaling pathways. This review consolidates the pharmacological actions and mechanisms of distinct ginsenosides in managing diabetes and its complications, offering a theoretical foundation for further pharmacological research and novel drug development for T2DM treatment, while also providing robust theoretical support for future clinical applications.
1 Introduction
Diabetes mellitus (DM) is a metabolic disorder characterized by hyperglycemia arising from inadequate insulin secretion and/or compromised insulin efficacy (Wang and Cao, 2025). The International Diabetes Federation (IDF) estimates that DM currently impacts approximately 536.6 million adults globally, with projections indicating an increase to 783.2 million by 2045 (Yang et al., 2024). The rising prevalence of DM, particularly type 2 diabetes mellitus (T2DM), stems from a complex interplay of genetic predispositions—such as obesity, impaired postprandial insulin release, and damage to certain pancreatic β cells—and environmental factors, including obesity, poor dietary habits, physical inactivity, and aging (Młynarska et al., 2025). This results in sustained hyperglycemia and reduced insulin sensitivity, which cumulatively contribute to various metabolic disorders.
T2DM significantly disrupts numerous organ systems by inducing profound alterations across nearly all cellular metabolic pathways. Chronic complications of DM often arise from either insulin deficiency or resistance, accompanied by persistent hyperglycemia, dyslipidemia, and other metabolic irregularities (Bilal and Pratley, 2025). These complications encompass macrovascular diseases, notably cardiovascular and cerebrovascular conditions primarily manifesting as atherosclerosis, and microvascular diseases, which involve thickening of the basement membrane and deposition of transparent material in tissues such as the retina, kidneys, and nerves. Key examples include diabetic retinopathy (DR), diabetic nephropathy (DN), and neuropathy (Alexander et al., 2024). Emerging evidence suggests that this binary classification of DM complications may require refinement, as many complications resist categorization into solely microvascular or macrovascular types. Instead, chronic DM complications can be grouped into pathological categories involving vascular, parenchymal, and mixed tissue, as illustrated in Figure 1. These complications constitute the primary causes of morbidity and mortality associated with DM, imposing a growing strain on healthcare systems worldwide, affecting both developed and developing nations (Singh et al., 2025).
Traditional diabetes therapies, including thiazolidinediones (SU), biguanides (BG), and α-glucosidase inhibitors, frequently carry significant side effects such as hypoglycemia, drug resistance, edema, and weight gain (Zhang et al., 2025). With advancements in diabetes research, therapeutic approaches have shifted from merely enhancing insulin’s hypoglycemic effects to broader strategies that regulate glucose metabolism, increase insulin receptor sensitivity, inhibit insulin resistance, control non-enzymatic glycosylation of proteins, and decrease fatty acid metabolism (Shahcheraghi et al., 2021). Current diabetes management primarily emphasizes insulin or peptide derivatives, oral antidiabetic drugs, and dietary modifications. However, daily intravenous insulin or peptide injections are inconvenient and burdensome for patients (Scairati et al., 2025), while long-term oral administration of chemical drugs poses risks of toxicity. Despite numerous strategies and medications developed for diabetes prevention and treatment, the outcomes remain largely suboptimal. Consequently, there is an urgent demand for new, effective, and safer natural hypoglycemic agents as alternative therapies for diabetes and its complications. Recent years have witnessed a growing interest in traditional Chinese medicine for diabetes treatment, particularly ginseng.
Ginseng, a perennial herb reaching up to 60 cm in height, is primarily cultivated in northeastern China, Korea, North Korea, and Japan (Iqbal et al., 2025). The ginseng shares a basic structure with steroid hormones, containing saponins, polysaccharides, polyacetylene, phenols, and alkaloids, each with a rigid tetracyclic steroid backbone of 17 carbon atoms (Li et al., 2024). Ginsenosides, a principal class of natural triterpene saponins within ginseng, are recognized as key contributors to its antidiabetic properties. To date, nearly 200 ginsenosides have been identified in ginseng plants and heat-processed ginseng products (Qi et al., 2022). Ginsenosides are generally categorized into two subtypes: protopanaxadiol (PPD) and protopanaxatriol (PPT). PPD-type ginsenosides include Rb1, Rb2, Rb3, Rc, Rd, Rh2, Rg3, and F2, while PPT-type ginsenosides encompass Re, Rf, Rg1, Rg2, and Rh1 (Liu et al., 2024). These compounds hold therapeutic potential for treating a wide range of conditions, including diabetes, cancer, digestive diseases, cardiovascular diseases and nervous system disorders (Niu et al., 2025).
Increasing evidence from cell, animal, and clinical studies demonstrates that various ginsenosides exert antidiabetic effects through multiple mechanisms. However, systematic evaluations detailing the specific antidiabetic mechanisms and the preventive effects of different ginsenoside types on diabetes complications remain lacking. This review compiles and analyzes recent studies (from 2020 onward) on ginsenosides in the treatment of diabetes and its complications across different systems or organs, providing a comprehensive theoretical foundation for their application in diabetes management.
2 Specific varieties of ginsenoside ingredients
The active ingredients associated with the ginsenosides used in the treatment of type 2 diabetes and its complications are shown in Table 1.

Table 1. Detailed information on the kinds of beneficial ginsenoside properties in the treatment of type 2 diabetes and its complications with different systems.
2.1 Cardiovascular system
DM is an important risk factor for cardiovascular diseases. Long-term hyperglycemia leads to vascular endothelial damage through oxidative stress, inflammatory response and metabolic disorders, and accelerates the process of atherosclerosis. Coronary atherosclerotic heart disease is often complicated in diabetic patients, which is manifested as angina pectoris and myocardial infarction. At the same time, myocardial microangiopathy can lead to diabetic cardiomyopathy, leading to ventricular remodeling and heart failure.
2.1.1 Endothelial cell dysfunction
Under high glucose conditions, the polyol pathway is activated, and advanced glycation end products accumulate, ultimately triggering an oxidative stress response that damages vascular endothelial cells—an initiating and central factor in diabetes-associated vascular disease (Yang and Liu, 2022).
In response, numerous scholars have systematically investigated this critical mechanism. Findings indicate that Rb1 enhances arterial flexibility, aortic compliance, and endothelium-dependent vasodilation by inhibiting the transforming growth factor-β1 (TGFβ1)/Smad2/3 pathway (Zhang J. H. et al., 2021), reducing oxidative stress (Park et al., 2023), and preventing endothelial-mesenchymal transition (EndMT), apoptosis, and mitochondrial damage (Wang D. S. et al., 2022). Additionally, Rk1 activates the peroxisome proliferator-activated receptor (PPAR)/endothelial nitric oxide synthase (eNOS) pathway, which alleviates endothelial dysfunction and suppresses oxidative stress in diabetic vascular tissue (Miao et al., 2024). Rg1 protects against vascular endothelial dysfunction (VED) by inhibiting the calpain-1/reactive oxygen species (ROS)/protein kinase C-β (PKC-β) axis, thereby mitigating mitochondrial dysfunction and oxidative stress (Lu et al., 2023). At concentrations of 10–40 μM, NR1 downregulates the MyD88/tumor necrosis factor receptor-associated factor 6 (TRAF6)/nuclear factor kappa-B (NF-κB) pathway by upregulating miR-147a, which suppresses oxidative stress, inflammation, and apoptosis while enhancing tube formation (Li and Huang, 2021). Furthermore, a study on Korean Red Ginseng (KRG) demonstrated improved cardiac function in diabetic rats by normalizing ejection fraction, fractional shortening, and vascular reactivity, although the study was limited to animal models and did not extend to cell-level mechanistic analysis (Hossain et al., 2020).
2.1.2 Diabetic cardiomyopathy (DCM)
Diabetic cardiomyopathy (DCM) is a distinct form of cardiomyopathy in patients with DM that is not attributable to hypertensive heart disease, coronary atherosclerosis, or other cardiac conditions. It results in pathological abnormalities, including cardiomyocyte apoptosis, left ventricular dysfunction, cardiac remodeling, inflammation, oxidative reactions, and myocardial metabolic disorders (Zhao et al., 2022). Feng et al. (2024) demonstrated that Rb1 improves myocardial injury in diabetic rats by reducing cardiomyocyte apoptosis and mitigating oxidative damage, although the specific mechanisms were not explored. Recent studies have expanded on this, indicating that Rb1 can not only lower lipid levels through adipocytokine-mediated pathways (Zhang et al., 2022) but also modulate the adenosine 5′-monophosphate-activated protein kinase (AMPK)/nuclear factor erythroid 2-related factor 2 (Nrf2)/heme oxygenase 1 (HO-1) signaling pathway (Bingbing et al., 2023). Furthermore, Rb1 has been shown to alleviate hyperglycemia/hyperlipidemia-induced ventricular diastolic dysfunction, metabolic disorders, oxidative stress, cardiomyocyte apoptosis, and fibrosis by inhibiting mitochondrial damage (Ji et al., 2024). Zhang et al. (2023) highlighted the therapeutic potential of Rg3 in DCM, noting that Rg3 counteracts lipid accumulation-induced dysfunction in cardiac tissue by enhancing adiponectin secretion and signaling. In addition, Lu et al. (Zhen et al., 2024) found that RG1 promotes mesenchymal stem cells (MSCs) to secrete exosomes, which reduce myocardial fibrosis and inflammation by activating the NOTCH signaling pathway to induce macrophage M2 polarization.
These findings underscore that ginsenosides not only enhance cardiovascular function by improving endothelial cell function but also restore myocardial function by addressing pathological alterations in cardiomyocytes, as illustrated in Figure 2. Future directions for research include developing a wider range of ginsenosides, investigating their molecular mechanisms, and exploring combined applications with polymer materials.
2.2 Digestive system
Diabetes digestive diseases are mainly caused by autonomic neuropathy and microcirculation disorders caused by long-term hyperglycemia, which can involve the gastrointestinal tract, liver and pancreas. We mainly describe different kinds of ginsenosides in the treatment of diabetes and its complications from these three aspects.
2.2.1 Diabetic liver disease
Diabetic patients experience impaired glucose metabolism, disrupting the regulatory balance between the liver and pancreas, which in turn diminishes the liver’s capacity to manage blood glucose levels. Hyperglycemia triggers hepatocyte inflammation through mechanisms such as mitochondrial oxidative stress, endoplasmic reticulum (ER) stress, and reduced lysosomal autophagy, causing substantial hepatocyte damage and subsequent liver function decline (Lange et al., 2022). Rh1 not only inhibits elevations in triglyceride (TG), total cholesterol (TC), and low-density lipoprotein cholesterol (LDL-C) levels but also enhances the secretion of G6Pase and phosphoenolpyruvate carboxykinase (PEPCK) in the gluconeogenesis pathway. Histological analyses further indicate that Rh1 mitigates liver tissue apoptosis and suppresses inflammatory mediators, including NF-κB and NOD-like receptor protein 3 (NLRP3), providing initial evidence of Rh1’s protective role against liver damage in T2DM (Su H. et al., 2022). Additionally, Zhu et al. (2021) reported that Rg5 improves liver injury and hepatocyte apoptosis via the insulin receptor substrate-1 (IRS-1)/phosphatidylinositide 3-kinase (PI3K)/protein kinase B (AKT) pathway, alleviates hepatic oxidative stress and inflammation, and promotes mitochondrial biosynthesis in T2DM. Thus, Rg1 and Rg5 show promise as natural interventions for T2DM. Further studies suggest that Rb1 has a strong affinity for 15-PGDH and may enhance hepatic glycogen synthesis through a 15-PGDH-dependent mechanism, offering new insights into Rb1’s positive effects on T2DM (Liang et al., 2023).
Inadequate glycemic control in diabetes increases insulin levels, stimulates fat synthesis, inhibits lipolysis, and impairs the transformation of lipids into lipoproteins, which are essential for lipid transport from the liver. Consequently, these lipids accumulate in hepatocytes, leading to hepatic steatosis. Enhancing lipid metabolism in the liver is, therefore, an essential strategy for addressing diabetic liver disease (Guo et al., 2024). Huang et al. (2022) demonstrated that Red Ginseng significantly reduces fasting blood glucose and TG and TC levels in T2DM rats. Both in vitro and in vivo studies confirm its efficacy in correcting lipid metabolism disorders and alleviating hepatic steatosis, supporting the potential of red ginseng as a functional food for diabetes management. Panax ginseng (PG-MGR), another natural ginseng product, also shows promising effects by lowering fasting blood glucose (FBG), TG, TC, and LDL-C levels, improving insulin resistance and glucose tolerance, and reducing liver damage by decreasing aspartate aminotransferase (AST) and alanine aminotransferase (ALT) expression through the activation of IRS-1/PI3K/AKT and AMPK signaling pathways (Wang D. S. et al., 2022).
To date, research on diabetic liver complications remains limited, focusing mainly on liver function impairment and lipid metabolism. Future studies should aim to develop a wider variety of ginsenosides, investigate their molecular mechanisms in greater detail, and assess potential hepatotoxic effects.
2.2.2 Pancreatic dysfunction
Pancreatic diabetes, a type of diabetes mellitus caused by pancreatic exocrine diseases, is most frequently associated with chronic pancreatitis. Key pathogenic factors include islet dysfunction, insulin insufficiency, insulin resistance, reduced incretin hormone levels, and disruptions in intestinal flora (Vonderau and Desai, 2022). Immune-mediated islet dysfunction plays a critical role in the development of pancreatic diabetes, where macrophage recruitment and activation lead to the release of numerous inflammatory cells, contributing to pancreatic β-cell dysfunction. Studies indicate that the early recruitment and activation of macrophages exacerbate pancreatic cell damage (Wagner et al., 2022). CK has been shown to dose-dependently reduce M1-type inflammatory cytokine expression in macrophages via the PPAR γ/NF-κB signaling pathway, effectively improving insulin resistance and glucose tolerance (Xu et al., 2022). Similarly, Rg1 reduces inflammation and insulin resistance, while also activating AMPK and inhibiting mammalian target of Rapamycin (mTOR)-mediated autophagy and apoptosis (Chen et al., 2023; Zong et al., 2023). Additionally, Rh4 has demonstrated a significant effect in alleviating diabetes symptoms, normalizing glucose metabolism, and enhancing insulin secretion, primarily through increased Nrf2 expression. Elevated pancreatic inflammation levels decrease insulin secretion, but Rh4’s effects include promoting Nrf2 nuclear translocation and boosting insulin production by activating pancreatic and duodenal homeobox-1 (PDX-1) and glucose transporter-2 (GLUT2) signaling pathways. Investigating the Nrf2 pathway offers promising potential as a therapeutic strategy to address pancreatic β-cell dysfunction in diabetes (Liu et al., 2021). Experimental results further indicate that preparations with spherical structures exhibit smaller particle sizes, enhanced penetrative ability, and an encapsulation rate as high as 99.8%, significantly improving fasting insulin (FINS) levels and insulin sensitivity index (ISI) (Han et al., 2024).
Glucagon-like peptide-1 (GLP-1) is a hormone released during the intestinal digestion and absorption of nutrients, which stimulates insulin secretion. However, in cases of pancreatic insufficiency, nutrient absorption is compromised, leading to reduced incretin hormone release and subsequent increases in blood glucose levels. Rk1 has been found to activate the anti-apoptotic effects of the PI3K/AKT/B-cell lymphoma-2 (Bcl-2) signaling pathway by directly targeting and activating the insulin-like growth factor 1 receptor (IGF-1R). Additionally, Rk1 reduces pancreatic weight and increases pancreatic insulin levels, thereby protecting the pancreas from high-fat diet (HFD)-induced diabetes (Vong et al., 2024).
In summary, substantial research has clarified the mechanisms underlying pancreatic diabetes. Future efforts may focus on combining various ginsenosides for diabetes treatment, aiming for complementary effects and exploring multiple drug delivery routes to optimize therapeutic efficacy.
2.2.3 Abnormal intestinal metabolism
L cells, a type of intestinal endocrine cell dispersed throughout the gastrointestinal tract, secrete several critical peptide hormones, including GLP-1, GLP-2, polypeptide YY (PYY), and gastric oxyntic regulator. These hormones play vital roles in promoting insulin secretion, regulating appetite, and managing blood glucose levels and body weight. Recent studies have identified L cells as pivotal targets for diabetes treatment, with CK emerging as the most extensively researched agent. CK has been shown to alleviate ileal epithelial injury and intestinal fibrosis by increasing levels of lithocholic acid (LCA) and deoxycholic acid (DCA). Additionally, CK promotes L-cell transformation and enhances GLP-1 release by upregulating genes associated with L-cell differentiation (Tian et al., 2022a). The underlying mechanisms involve pathways such as the gut microbiota-bile acid (BA)-TGR5 pathway and the RhoA/ROCK/YAP signaling pathway (Tian et al., 2021; Tian et al., 2022a).
The gut microbiota plays a pivotal role in the development of type 2 diabetes, exerting regulatory effects on the body’s metabolic and inflammatory responses. Disturbances in gut microbiota are linked to dysregulation of immune cells and elevated levels of inflammatory cytokines, making them significant contributors to various inflammation-mediated diseases. Rb1 has been shown to reverse intestinal microbiota disorders in diabetic mice by increasing the abundance of Umbellifera mites while decreasing the levels of Aristipes, Preethylene Silkworms, Stinky bacterium, and Anaerobic Proplasma. Furthermore, Rb1 altered the composition of free fatty acids (FFAs) in fecal metabolites, reducing α-linolenic acid, oleic acid, arachidonic acid, palmitic acid, and stearic acid (Zhou et al., 2023). Rd enhanced the abundance of beneficial bacteria through the activation of the AKT pathway, while simultaneously decreasing the abundance of conditionally pathogenic bacteria (Wang et al., 2023). Rg1 also contributed by increasing the proportion of Leptospira and Clostridium leptoilea and decreasing Lactic acid bacteria (Peng et al., 2022). These findings indicate that ginsenosides may function as potential prebiotics, regulating specific gut microbes and related metabolites that are crucial in diabetes-related metabolic disorders and insulin resistance.
These findings not only elucidate the mechanisms through which CK affects intestinal L cells but also establish a molecular foundation for further exploring CK as a potential therapeutic agent for the treatment of T2DM, as illustrated in Figure 3.
2.3 Diabetic nephropathy
DR is one of the most severe complications of diabetes, while DN represents the primary microvascular complication, primarily characterized by diabetic glomerulosclerosis, a glomerular lesion driven by vascular damage.
In its early stages, DN is often asymptomatic, with blood pressure remaining normal or elevated. The incidence of this condition increases with the duration of diabetes. Initially, kidney volume and glomerular filtration rate (GFR) rise, leading to a state of hyperfiltration, followed by the gradual onset of interstitial proteinuria or microalbuminuria (Jung et al., 2021). As the disease progresses, persistent proteinuria, edema, hypertension, and a decrease in GFR can lead to renal insufficiency and uremia, which are significant contributors to diabetes-related mortality.
Research indicates that Fc, CK, and Rg3 can improve urine microalbumin levels in diabetic mice through various mechanisms (Zhou et al., 2020; Chen et al., 2022; Shen et al., 2024). CK mitigates oxidative stress accumulation, decreases levels of pyroptosis-associated proteins, reduces mitochondrial membrane potential collapse, and modulates the expression of mitochondrial fission proteins while increasing mitofusin 2 (Mfn2) expression. CK also remodels the gut microbiota by reducing fungal content and p-prostetones, while increasing lactobacilli levels and decreasing serum concentrations of the histidine-derived microbial metabolite imidazole propionate (IMP). Rg3 treatment activates Toll-like receptor 4 (TLR4), resulting in improved renal histology, significantly reduced apoptosis of renal tubular epithelial cells, and lower fasting blood glucose, creatinine, total cholesterol, and triglyceride levels, as well as reduced expression of inflammatory factors compared to the diabetic group.
Glomerulosclerosis and the hyperperfusion of residual nephron glomeruli in chronic kidney disease are critical factors contributing to further nephron loss. LDL can induce increased apoptosis of mesangial glomerular cells, exacerbating kidney tissue damage. Therefore, alleviating apoptosis is a crucial strategy for treating DN. Chen et al. (2024) reported that Rg3 targets miR-216a-5p, activates the MAPK pathway, inhibits apoptosis, and alleviates kidney damage in diabetic mice. Similarly, Su et al. (2021) found that Rh1 yields comparable effects, with molecular mechanism studies demonstrating that its benefits are linked to apoptosis inhibition via the AMPK/PI3K/AKT signaling pathway. Additionally, He et al. (2022) identified that Rb1 significantly reduces diabetes-induced glomerular injury, podocyte apoptosis, and mitochondrial damage—such as glomerular hypertrophy and mesangial stromal dilation—while decreasing the expression of apoptotic proteins.
Additionally, research demonstrates that ginsenosides exert significant effects primarily by reducing oxidative stress, inflammation, and pathological changes in renal histology, with Rg series ginsenosides being particularly notable. For instance, cell experiments have shown that Rg1 effectively lowers urine protein, serum creatinine, urea nitrogen, blood lipid levels, and renal lipid volume in T2DM mice. Pathological analyses indicate that Rg1 treatment alleviates renal damage and glomerular fibrosis (Han et al., 2023; Ji et al., 2023). This therapeutic effect is mediated through the transient receptor potential cation channel 6 (TRPC6)/nuclear factor of activated T Cells (NFAT2) and NADPH oxidase 4 (NOX4)-MAPK signaling pathways.
Rg3 also contributes positively by upregulating PPARγ activity, thereby reducing inflammatory and fibrosis biomarkers (Sui et al., 2023). Concurrently, it enhances insulin (INS) levels, improves blood lipid profiles, mitigates oxidative stress, and restores renal function via MAPK and NF-κB signaling pathways (Li et al., 2021), leading to improved renal histopathological outcomes. Rg5 further protects against kidney injury in diabetic mice by inhibiting oxidative stress and the activation of the NLRP3 inflammasome, suggesting its potential as a compound for preventing or managing diabetic kidney injury (Zhu et al., 2020), as illustrated in Figure 4.
DN is a chronic, progressive condition where clinical symptoms often manifest late, and once persistent proteinuria occurs, renal function deteriorates irreversibly and progressively. Currently, most studies focus on a limited number of common ginsenosides. Future research should explore the therapeutic effects of ginsenosides from other varieties, aiming to enhance their impact on kidney lesions and experimenting with various drug delivery methods to improve therapeutic efficacy.
2.4 Diabetic neuropathy
Diabetic neuropathy is one of the most prevalent chronic complications of diabetes, impacting both central and peripheral nerves, with distal sensory neuropathy being the most common, accounting for over 50% of all diabetic neuropathies (Mittal et al., 2025).
Currently, there is a lack of published studies on the therapeutic effects of ginsenosides specifically for diabetic peripheral neuropathy. This study aims to explore the effects of three ginsenosides on diabetic central neuropathy through assessments of memory impairment, depression-like behaviors, and cognitive abilities in mice. Dong et al. (2023) demonstrated that Rg1 reduces levels of ROS, inositol triphosphate (IP3), and diacylglycerol (DAG), effectively reversing Ca2+ overload. This is achieved by downregulating the expression of p-PLC, TRPC6, and NFAT1 nuclear translocation, which alleviates amyloid-beta (Aβ) deposition and enhances postsynaptic density-95 (PSD-95) expression in T2DM mice. Additionally, Li et al. (2020) reported that CK treatment significantly improved behavioral impairments in mice, as CK not only lowered fasting blood glucose levels but also enhanced lipid metabolism, glucose tolerance, insulin sensitivity, and dyslipidemia. It further reduced oxidative stress and inhibited inflammatory responses in the hippocampus, alleviating ER stress and suppressing the NLRP3 inflammasome pathway. In addressing cognitive decline associated with diabetes, a 2020 study found that Rb1 improved memory and cognitive function in mice with streptozotocin (STZ)-induced damage. Rb1 also mitigated STZ-induced glucose intolerance by enhancing insulin sensitivity, with these beneficial effects attributed to the inhibition of Cdk5/p35 activity and the upregulation of N-methyl-D-aspartate receptor-1 (NMDAR1) expression in the hippocampus. This research is crucial for understanding the mechanisms by which ginsenoside Rb1 enhances cognitive performance and its implications for the relationship between diabetes and Alzheimer’s disease (AD) (Yang et al., 2020). Furthermore, some researchers have combined Rb1 with small alkali compounds to treat diabetic neuropathy, finding that this combination improved glucose metabolism and insulin resistance and alleviated depression-like behaviors associated with chronic unpredictable stress. Hematoxylin-eosin (HE) and Nissl staining in animal experiments indicated that neurons were protected from pathological and morphological changes. Thus, the combination of small alkali and Rb1 may hold clinical value for treating patients with diabetes and co-occurring depression (Zhang J. H. et al., 2021). Currently, scholarly attention has primarily focused on diabetic central neuropathy, while the therapeutic mechanisms of ginsenosides are extensive. Future research should delve deeper into the role of ginsenosides in the treatment of diabetic peripheral neuropathy, providing a robust theoretical foundation for their clinical application, as illustrated in Figure 5.
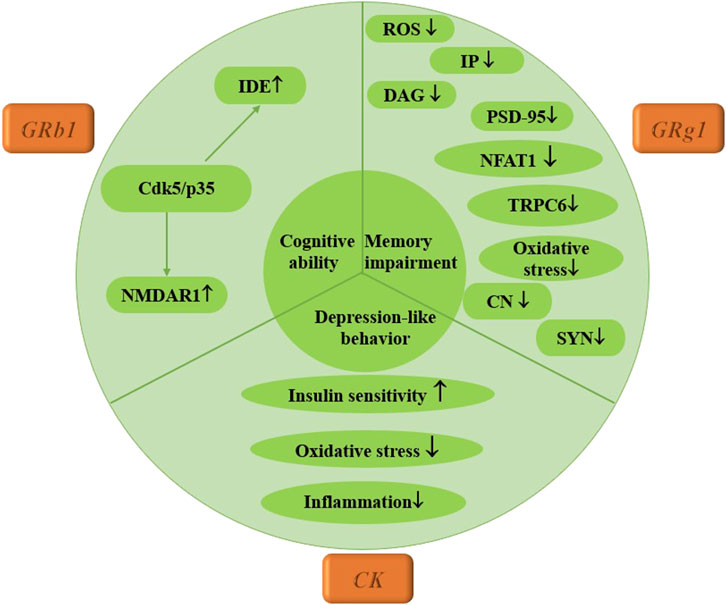
Figure 5. Mechanism of multiple ginsenosides in the treatment of diabetic nervous system complications.
2.5 Diabetic retinopathy
DR is one of the most prevalent microvascular complications of diabetes, resulting from chronic diabetes mellitus and leading to various fundus lesions, including microangiopathy, hard exudates, cotton wool spots, neovascularization, vitreous hyperplasia, macular edema, and even retinal detachment (Bryl et al., 2022).
Wang et al. (2024a) found that Panax notoginseng saponins (PNS) significantly increased retinal core layer thickness and mitigated the rise in retinal cell-free capillaries while markedly reducing microglial activation. Furthermore, PNS inhibits the activation of the NF-κB signaling pathway in M1 cells and suppresses cellular inflammatory responses, thereby alleviating DR and reducing retinal inflammation. Xue et al. (2023) also identified considerable efficacy of Rg1, demonstrating its ability to effectively lower levels of intracellular inflammatory cytokines and growth factors. This suggests a potential therapeutic strategy for DR through the upregulation of miR-216a-5p and the inhibition of the TLR4/NF-κB signaling pathway. Additionally, the PI3K/AKT pathway has been explored, with evidence that Re can counteract high glucose-induced (HG) decreases in RF/6A cell viability, reduce the apoptosis rate, and inhibit oxidation-related enzymes. This action leads to decreased ROS production and mitigates HG-triggered damage to RF/6A cells, providing cytoprotective effects associated with the activation of the PI3K/Akt pathway (Xie et al., 2020). Notably, the use of LY294002, a PI3K inhibitor, partially reversed the effects of Re on apoptosis-related proteins, indicating that Re may improve HG-induced retinal angiogenesis. Diabetes mellitus typically elevates retinal oxidative stress levels, generating large amounts of ROS via pathways such as advanced glycation end products (AGE), the polyol pathway, the hexosamine pathway, and the PKC pathway. This oxidative stress damages the retina, leading to further oxidative damage and apoptosis, creating a vicious cycle by activating additional cytokines that upregulate oxidative stress levels. In this context, Tang et al. (Tang et al., 2022) demonstrated through various in vitro and in vivo experiments that Rd enhances the interaction between AMPK and silent information regulator family protein 1 (SIRT1) by increasing nicotinamide adenine dinucleotide (NAD)/NADH levels and facilitating liver kinase B1 (LKB1) deacetylation in endothelial cells. This mechanism effectively reverses hyperglucose-induced activation of NADPH oxidase 2 (NOX2), oxidative stress, mitochondrial dysfunction, and endothelial cell apoptosis. These findings support the clinical development of Rd as a pharmacological intervention, presenting it as a novel potential vasoprotective agent for early DR. In summary, ginsenosides have shown significant promise in the treatment of DR, highlighting their potential for clinical application in the management of this complication, as illustrated in Figure 6.
2.6 Other complications
In addition to the previously mentioned systemic and organ complications, diabetes can lead to various other complications, which require systematical discussion due to a lack of comprehensive literature.
Tendinopathy, characterized by muscle cell apoptosis and damage to the extracellular matrix, can be influenced by diabetic conditions. CK has demonstrated efficacy in counteracting high glucose-induced apoptosis, inflammation, and oxidative stress in cultured cells. Specifically, CK normalizes the expression of matrix metalloproteinases-9 (MMP-9), MMP-13, and tissue inhibitor of metalloproteinase-1 (TIMP-1), while enhancing the expression of PPARγ and antioxidant enzymes (Cho et al., 2024). This results in improved mitochondrial membrane potential, increased glucose uptake and glycogen synthesis, and enhanced mitochondrial mass (Li et al., 2023), highlighting CK’s therapeutic potential in hyperglycemic tendinopathy. Additionally, in an HFD and HG diabetic mouse model, Rg3 treatment significantly reduced triglyceride and glucose levels in C2C12 myoblasts. It promoted myoblast differentiation, inhibited mitochondrial dysfunction, increased climbing distances, and mitigated muscle atrophy. These beneficial effects are associated with the phosphorylation of AMPK and Forkhead Box O3 (FoxO3) and the inhibition of Smad3 phosphorylation (Wang M. et al., 2024).
Currently, only one study published in 2022 has investigated the role of ginsenosides in treating diabetic lung tissue injury, utilizing Rb1 (Su H. et al., 2022). The findings revealed that Rb1 treatment not only significantly reduced the apoptosis rate of lung tissue cells—2.23 times in the diabetic group compared to 1.73 times in the treatment group—but also decreased oxidative damage and inflammatory infiltration in the lungs. This was achieved by lowering the expression of various inflammatory factors, including interleukin-6 (IL-6), interleukin-1α (IL-1α), and tumor necrosis factor-α (TNF-α).
Poor wound healing is a prevalent chronic complication of diabetes, influenced by factors such as abnormal inflammation, reduced granulation tissue content, impaired angiogenesis at the wound site, and peripheral neuropathy. Rg1 has been shown to enhance the proliferation, migration, and angiogenesis of human umbilical vein endothelial cells (HUVECs) while reducing apoptosis (Huang et al., 2021). Additionally, Rg5 inhibits the expression and activity of SLC7A11 through physical binding, alleviating the negative regulation of anaerobic glycolysis and promoting erythropoiesis of dendritic cells (Xia et al., 2023). Rg1’s effects are mediated by the downregulation of the key nucleic acid miR-489-3p and the activation of the PI3K/AKT/eNOS signaling pathway. Consequently, ginsenosides have potential as adjuvant therapeutic agents to support wound healing in patients, particularly those with diabetic foot ulcers.
Moreover, two clinical studies have explored the effects of ginsenosides. One study found that the addition of ginseng to drug treatment in diabetic patients improved central systolic blood pressure and pulse wave formation, without directly affecting endothelial function (Jovanovski et al., 2020). In another study involving Rg3-KR intervention, a reduction in HbA1c levels was observed (−0.35% ± 0.1% [-3.8 ± 1.1 mmol/mol], p = 0.02) at 12 weeks, with no adverse safety outcomes reported. These findings suggest that ginsenosides may offer clinical benefits when incorporated into polypharmacy and lifestyle interventions for diabetes management (Jovanovski et al., 2021).
3 Prospects of ginsenosides in the treatment of type 2 diabetes and its complications
To date, numerous studies have demonstrated the significant potential of ginsenosides in the treatment of type 2 diabetes, elucidating various related mechanisms to some extent. However, the application of ginsenosides as a therapeutic strategy remains limited. Key challenges include: 1) Most research has focused on a single variety of ginsenosides in cell or animal experiments, with limited exploration of polypharmacy; 2) The predominant route of administration in most studies has been oral, necessitating further investigation of multiple delivery routes to enhance drug utilization; 3) There is a need for in-depth studies on the specific concentrations of ginsenosides in bone and their potential toxic side effects in other organs; and 4) With the emergence of numerous new biochemical materials in recent years, there is an opportunity to explore the synergistic effects of ginsenosides in combination therapies.
4 Conclusion
As research into ginsenosides for diabetes treatment expands, this review outlines the possible mechanisms by which different types of ginsenosides exert therapeutic effects, summarizing their specific signaling pathways and key factor mechanisms. Nevertheless, this therapeutic approach is still in the early stages of clinical translation, facing numerous obstacles to clinical application, such as the need for combination drugs, optimization of routes of administration, bioavailability, and integration with biochemical materials. With the growing recognition of ginsenosides’ roles in diabetes management, they hold considerable promise as a new therapeutic agent for diabetes and its associated complications, positioning them as strong candidates for future drug development.
Author contributions
YL: Writing – original draft. YJ: Writing – review and editing, Investigation. YW: Investigation, Writing – review and editing. XC: Writing – review and editing, Investigation. YS: Writing – review and editing, Investigation. PH: Investigation, Writing – review and editing. YC: Writing – review and editing.
Funding
The author(s) declare that financial support was received for the research and/or publication of this article. This research was supported by Natural Science Foundation of Jilin Province (YDZJ202301ZYTS074).
Acknowledgments
We thank Bullet Edits Limited for the linguistic editing and proofreading of the manuscript.
Conflict of interest
The authors declare that the research was conducted in the absence of any commercial or financial relationships that could be construed as a potential conflict of interest.
Generative AI statement
The author(s) declare that no Generative AI was used in the creation of this manuscript.
Publisher’s note
All claims expressed in this article are solely those of the authors and do not necessarily represent those of their affiliated organizations, or those of the publisher, the editors and the reviewers. Any product that may be evaluated in this article, or claim that may be made by its manufacturer, is not guaranteed or endorsed by the publisher.
References
Alexander, M., Cho, E., Gliozheni, E., Salem, Y., Cheung, J., and Ichii, H. (2024). Pathology of diabetes-induced immune dysfunction. Int. J. Mol. Sci. 25, 7105. doi:10.3390/ijms25137105
Bilal, A., and Pratley, R. (2025). Diabetes and cardiovascular disease in older adults. Ann. N. Y. Acad. Sci. 1543, 42–67. doi:10.1111/nyas.15259
Bingbing, L., Jieru, L. I., Jianchao, S. I., Qi, C., Shengchang, Y., and Ensheng, J. I. (2023). Ginsenoside Rb1 alleviates chronic intermittent hypoxia-induced diabetic cardiomyopathy in db/db mice by regulating the adenosine monophosphate-activated protein kinase/Nrf2/heme oxygenase-1 signaling pathway. J. Tradit. Chin. Med. 43, 906–914. doi:10.19852/j.cnki.jtcm.20221206.004
Bryl, A., Mrugacz, M., Falkowski, M., and Zorena, K. (2022). The effect of diet and lifestyle on the course of diabetic retinopathy-A review of the literature. Nutrients 14, 1252. doi:10.3390/nu14061252
Bu, Q. T., Zhang, W. Y., Chen, Q. C., Zhang, C. Z., Gong, X. J., Liu, W. C., et al. (2012). Anti-diabetic effect of ginsenoside Rb(3) in alloxan-induced diabetic mice. Med. Chem. 8, 934–941. doi:10.2174/157340612802084199
Cai, H. A., Huang, L., Zheng, L. J., Fu, K., Wang, J., Hu, F. D., et al. (2019). Ginsenoside (Rg-1) promoted the wound closure of diabetic foot ulcer through iNOS elevation via miR-23a/IRF-1 axis. Life Sci. 233, 116525. doi:10.1016/j.lfs.2019.05.081
Chen, F., Chen, Y., Kang, X., Zhou, Z., Zhang, Z., and Liu, D. (2012). Anti-apoptotic function and mechanism of ginseng saponins in Rattus pancreatic β-cells. Biol. Pharm. Bull. 35, 1568–1573. doi:10.1248/bpb.b12-00461
Chen, J., Zhu, G., Xiao, W., Huang, X., Wang, K., and Zong, Y. (2023). Ginsenoside Rg1 ameliorates pancreatic injuries via the AMPK/mTOR pathway in vivo and in vitro. Diabetes Metab. Syndr. Obes. 16, 779–794. doi:10.2147/DMSO.S401642
Chen, Q., Ren, D., Liu, L., Xu, J., Wu, Y., Yu, H., et al. (2022). Ginsenoside compound K ameliorates development of diabetic kidney disease through inhibiting TLR4 activation induced by microbially produced imidazole propionate. Int. J. Mol. Sci. 23, 12863. doi:10.3390/ijms232112863
Chen, X., Wei, R., Jin, T., and Du, H. (2019). Notoginsenoside R1 alleviates TNF-α-induced pancreatic β-cell Min6 apoptosis and dysfunction through up-regulation of miR-29a. Artif. Cells Nanomed Biotechnol. 47, 2379–2388. doi:10.1080/21691401.2019.1624368
Chen, Y., Peng, Y., Li, P., Jiang, Y., and Song, D. (2024). Ginsenoside Rg3 induces mesangial cells proliferation and attenuates apoptosis by miR-216a-5p/MAPK pathway in diabetic kidney disease. Aging 16, 9933–9943. doi:10.18632/aging.205907
Cho, W., Oh, H., Abd El-Aty, A. M., Hacimuftuoglu, A., Jeong, J. H., and Jung, T. W. (2024). Therapeutic potential of ginsenoside compound K in managing tenocyte apoptosis and extracellular matrix damage in diabetic tendinopathy. Tissue Cell. 86, 102275. doi:10.1016/j.tice.2023.102275
Cho, W. C., Chung, W. S., Lee, S. K., Leung, A. W., Cheng, C. H., and Yue, K. K. (2006). Ginsenoside re of Panax ginseng possesses significant antioxidant and antihyperlipidemic efficacies in streptozotocin-induced diabetic rats. Eur. J. Pharmacol. 550, 173–179. doi:10.1016/j.ejphar.2006.08.056
Dai, S., Hong, Y., Xu, J., Lin, Y., Si, Q., and Gu, X. (2018). Ginsenoside Rb2 promotes glucose metabolism and attenuates fat accumulation via AKT-dependent mechanisms. Biomed. Pharmacother. 100, 93–100. doi:10.1016/j.biopha.2018.01.111
Dong, C., Liu, P., Wang, H., Dong, M., Li, G., and Li, Y. (2019). Ginsenoside Rb1 attenuates diabetic retinopathy in streptozotocin-induced diabetic rats1. Acta Cir. Bras. 34, e201900201. doi:10.1590/s0102-8650201900201
Dong, X., Kong, L., Huang, L., Su, Y., Li, X., Yang, L., et al. (2023). Ginsenoside Rg1 treatment protects against cognitive dysfunction via inhibiting PLC-CN-NFAT1 signaling in T2DM mice. J. Ginseng Res. 47, 458–468. doi:10.1016/j.jgr.2022.12.006
Du, Y. G., Wang, L. P., Qian, J. W., Zhang, K. N., and Chai, K. F. (2016). Panax notoginseng saponins protect kidney from diabetes by up-regulating silent information regulator 1 and activating antioxidant proteins in rats. Chin. J. Integr. Med. 22, 910–917. doi:10.1007/s11655-015-2446-1
Fan, C., Ma, Q., Xu, M., Qiao, Y., Zhang, Y., Li, P., et al. (2019a). Ginsenoside Rb1 attenuates high glucose-induced oxidative injury via the NAD-PARP-SIRT Axis in rat retinal capillary endothelial cells. Int. J. Mol. Sci. 20, 4936. doi:10.3390/ijms20194936
Fan, C., Qiao, Y., and Tang, M. (2017). Notoginsenoside R1 attenuates high glucose-induced endothelial damage in rat retinal capillary endothelial cells by modulating the intracellular redox state. Drug Des. Dev. Ther. 11, 3343–3354. doi:10.2147/DDDT.S149700
Fan, X., Tao, J., Zhou, Y., Hou, Y., Wang, Y., Gu, D., et al. (2019b). Investigations on the effects of ginsenoside-Rg1 on glucose uptake and metabolism in insulin resistant HepG2 cells. Eur. J. Pharmacol. 843, 277–284. doi:10.1016/j.ejphar.2018.11.024
Fan, X., Zhang, C., Niu, S., Fan, B., Gu, D., Jiang, K., et al. (2019c). Ginsenoside Rg1 attenuates hepatic insulin resistance induced by high-fat and high-sugar by inhibiting inflammation. Eur. J. Pharmacol. 854, 247–255. doi:10.1016/j.ejphar.2019.04.027
Feng, C., Song, J., Deng, L., Zhang, J., Lian, X., Zhen, Z., et al. (2024). Ginsenoside Rb1 reduces oxidative/carbonyl stress damage and dysfunction of RyR2 in the heart of streptozotocin-induced diabetic rats. BMC Cardiovasc Disord. 24, 333. doi:10.1186/s12872-024-04005-8
Gao, Y., Li, J., Chu, S., Zhang, Z., Chen, N., Li, L., et al. (2020). Ginsenoside Rg1 protects mice against streptozotocin-induced type 1 diabetic by modulating the NLRP3 and Keap1/Nrf2/HO-1 pathways. Eur. J. Pharmacol. 866, 172801. doi:10.1016/j.ejphar.2019.172801
Gu, J., Li, W., Xiao, D., Wei, S., Cui, W., Chen, W., et al. (2013). Compound K, a final intestinal metabolite of ginsenosides, enhances insulin secretion in MIN6 pancreatic β-cells by upregulation of GLUT2. Fitoterapia 87, 84–88. doi:10.1016/j.fitote.2013.03.020
Guan, F. Y., Gu, J., Li, W., Zhang, M., Ji, Y., Li, J., et al. (2014). Compound K protects pancreatic islet cells against apoptosis through inhibition of the AMPK/JNK pathway in type 2 diabetic mice and in MIN6 β-cells. Life Sci. 107, 42–49. doi:10.1016/j.lfs.2014.04.034
Guo, M., Guo, G., Xiao, J., Sheng, X., Zhang, X., Tie, Y., et al. (2018a). Ginsenoside Rg3 stereoisomers differentially inhibit vascular smooth muscle cell proliferation and migration in diabetic atherosclerosis. J. Cell. Mol. Med. 22, 3202–3214. doi:10.1111/jcmm.13601
Guo, M., Xiao, J., Sheng, X., Zhang, X., Tie, Y., Wang, L., et al. (2018b). Ginsenoside Rg3 mitigates atherosclerosis progression in diabetic apoE-/- mice by skewing macrophages to the M2 phenotype. Front. Pharmacol. 9, 464. doi:10.3389/fphar.2018.00464
Guo, Y., Zhao, T., Yao, X., Ji, H., Luo, Y., Okeke, E. S., et al. (2024). Acrylamide-aggravated liver injury by activating endoplasmic reticulum stress in female mice with diabetes. Chem. Res. Toxicol. 37, 731–743. doi:10.1021/acs.chemrestox.4c00016
Han, S., Zhang, X., Li, Z., Cui, G., Xue, B., Yu, Y., et al. (2024). A ginsenoside G-Rg3 PEGylated long-circulating liposome for hyperglycemia and insulin resistance therapy in streptozotocin-induced type 2 diabetes mice. Eur. J. Pharm. Biopharm. 201, 114350. doi:10.1016/j.ejpb.2024.114350
Han, Y., Su, Y., Han, M., Liu, Y., Shi, Q., Li, X., et al. (2023). Ginsenoside Rg1 attenuates glomerular fibrosis by inhibiting CD36/TRPC6/NFAT2 signaling in type 2 diabetes mellitus mice. J. Ethnopharmacol. 302, 115923. doi:10.1016/j.jep.2022.115923
He, J. Y., Hong, Q., Chen, B. X., Cui, S. Y., Liu, R., Cai, G. Y., et al. (2022). Ginsenoside Rb1 alleviates diabetic kidney podocyte injury by inhibiting aldose reductase activity. Acta Pharmacol. Sin. 43, 342–353. doi:10.1038/s41401-021-00788-0
Hossain, M. A., Lee, D., Kim, B., Kang, C. W., Kim, N. S., and Kim, J. H. (2020). Korean Red Ginseng attenuates type 2 diabetic cardiovascular dysfunction in Otsuka Long-Evans Tokushima Fatty rats. J. Ginseng Res. 44, 308–311. doi:10.1016/j.jgr.2018.12.003
Huang, G., Lv, J., Li, T., Huai, G., Li, X., Xiang, S., et al. (2016). Notoginsenoside R1 ameliorates podocyte injury in rats with diabetic nephropathy by activating the PI3K/Akt signaling pathway. Int. J. Mol. Med. 38, 1179–1189. doi:10.3892/ijmm.2016.2713
Huang, L., Cai, H. A., Zhang, M. S., Liao, R. Y., Huang, X., and Hu, F. D. (2021). Ginsenoside Rg1 promoted the wound healing in diabetic foot ulcers via miR-489-3p/Sirt1 axis. J. Pharmacol. Sci. 147, 271–283. doi:10.1016/j.jphs.2021.07.008
Huang, R., Zhang, M., Tong, Y., Teng, Y., Li, H., and Wu, W. (2022). Studies on bioactive components of red ginseng by UHPLC-MS and its effect on lipid metabolism of type 2 diabetes mellitus. Front. Nutr. 9, 865070. doi:10.3389/fnut.2022.865070
Hwang, Y. C., Oh, D. H., Choi, M. C., Lee, S. Y., Ahn, K. J., Chung, H. Y., et al. (2018). Compound K attenuates glucose intolerance and hepatic steatosis through AMPK-dependent pathways in type 2 diabetic OLETF rats. Korean J. Intern. Med. 33, 347–355. doi:10.3904/kjim.2015.208
Iqbal, H., Kim, Y., Jin, M., and Rhee, D. K. (2025). Ginseng as a therapeutic target to alleviate gut and brain diseases via microbiome regulation. J. Ginseng Res. 49, 12–21. doi:10.1016/j.jgr.2024.04.005
Ji, L., Han, H., Shan, X., Zhao, P., Chen, H., Zhang, C., et al. (2024). Ginsenoside Rb1 ameliorates lipotoxicity-induced myocardial injury in diabetes mellitus by regulating Mfn2. Eur. J. Pharmacol. 974, 176609. doi:10.1016/j.ejphar.2024.176609
Ji, P., Shi, Q., Liu, Y., Han, M., Su, Y., Sun, R., et al. (2023). Ginsenoside Rg1 treatment alleviates renal fibrosis by inhibiting the NOX4-MAPK pathway in T2DM mice. Ren. Fail 45, 2197075. doi:10.1080/0886022X.2023.2197075
Jiang, S., Ren, D., Li, J., Yuan, G., Li, H., Xu, G., et al. (2014). Effects of compound K on hyperglycemia and insulin resistance in rats with type 2 diabetes mellitus. Fitoterapia 95, 58–64. doi:10.1016/j.fitote.2014.02.017
Jovanovski, E., Lea-Duvnjak, S., Komishon, A., Au-Yeung, F., Zurbau, A., Jenkins, A. L., et al. (2020). Vascular effects of combined enriched Korean Red ginseng (Panax Ginseng) and American ginseng (Panax Quinquefolius) administration in individuals with hypertension and type 2 diabetes: a randomized controlled trial. Complement. Ther. Med. 49, 102338. doi:10.1016/j.ctim.2020.102338
Jovanovski, E., Smircic-Duvnjak, L., Komishon, A., Au-Yeung, F. R., Sievenpiper, J. L., Zurbau, A., et al. (2021). Effect of coadministration of enriched Korean Red Ginseng (Panax ginseng) and American ginseng (Panax quinquefolius L) on cardiometabolic outcomes in type-2 diabetes: a randomized controlled trial. J. Ginseng Res. 45, 546–554. doi:10.1016/j.jgr.2019.11.005
Jung, E., Pyo, M. K., and Kim, J. (2021). Pectin-lyase-modified ginseng extract and ginsenoside Rd inhibits high glucose-induced ROS production in mesangial cells and prevents renal dysfunction in db/db mice. Molecules 26, 367. doi:10.3390/molecules26020367
Kang, K. S., Yamabe, N., Kim, H. Y., Park, J. H., and Yokozawa, T. (2010). Effects of heat-processed ginseng and its active component ginsenoside 20(S)-Rg3 on the progression of renal damage and dysfunction in type 2 diabetic Otsuka Long-Evans Tokushima Fatty rats. Biol. Pharm. Bull. 33, 1077–1081. doi:10.1248/bpb.33.1077
Kaviani, M., Keshtkar, S., Azarpira, N., Hossein Aghdaei, M., Geramizadeh, B., Karimi, M. H., et al. (2019). Cytoprotective effects of ginsenoside Rd on apoptosis-associated cell death in the isolated human pancreatic islets. EXCLI J. 18, 666–676. doi:10.17179/excli2019-1698
Kim, J. M., Park, C. H., Park, S. K., Seung, T. W., Kang, J. Y., Ha, J. S., et al. (2017). Ginsenoside re ameliorates brain insulin resistance and cognitive dysfunction in high fat diet-induced C57bl/6 mice. J. Agric. Food Chem. 65, 2719–2729. doi:10.1021/acs.jafc.7b00297
Kim, K., Kim, D. H., and Kim, H. Y. (2009a). Compound K protects MIN6N8 pancreatic beta-cells against palmitate-induced apoptosis through modulating SAPK/JNK activation. Cell. Biol. Int. 34, 75–80. doi:10.1042/CBI20090020
Kim, K. S., Jung Yang, H., Lee, I. S., Kim, K. H., Park, J., Jeong, H. S., et al. (2015). The aglycone of ginsenoside Rg3 enables glucagon-like peptide-1 secretion in enteroendocrine cells and alleviates hyperglycemia in type 2 diabetic mice. Sci. Rep. 5, 18325. doi:10.1038/srep18325
Kim, M., Ahn, B. Y., Lee, J. S., Chung, S. S., Lim, S., Park, S. G., et al. (2009b). The ginsenoside Rg3 has a stimulatory effect on insulin signaling in L6 myotubes. Biochem. Biophys. Res. Commun. 389, 70–73. doi:10.1016/j.bbrc.2009.08.088
Kim, S. J., Yuan, H. D., and Chung, S. H. (2010). Ginsenoside Rg1 suppresses hepatic glucose production via AMP-activated protein kinase in HepG2 cells. Biol. Pharm. Bull. 33, 325–328. doi:10.1248/bpb.33.325
Kim, S. S., Jang, H. J., Oh, M. Y., Eom, D. W., Kang, K. S., Kim, Y. J., et al. (2014). Ginsenoside Rg3 enhances islet cell function and attenuates apoptosis in mouse islets. Transpl. Proc. 46, 1150–1155. doi:10.1016/j.transproceed.2013.12.028
Kim, Y. J., Park, S. M., Jung, H. S., Lee, E. J., Kim, T. K., Kim, T. N., et al. (2016). Ginsenoside Rg3 prevents INS-1 cell death from intermittent high glucose stress. Islets 8, 57–64. doi:10.1080/19382014.2016.1161874
Lange, N. F., Graf, V., Caussy, C., and Dufour, J. F. (2022). PPAR-targeted therapies in the treatment of non-alcoholic fatty liver disease in diabetic patients. Int. J. Mol. Sci. 23, 4305. doi:10.3390/ijms23084305
Lee, H. M., Lee, O. H., Kim, K. J., and Lee, B. Y. (2012). Ginsenoside Rg1 promotes glucose uptake through activated AMPK pathway in insulin-resistant muscle cells. Phytother. Res. 26, 1017–1022. doi:10.1002/ptr.3686
Lee, K. T., Jung, T. W., Lee, H. J., Kim, S. G., Shin, Y. S., and Whang, W. K. (2011a). The antidiabetic effect of ginsenoside Rb2 via activation of AMPK. Arch. Pharm. Res. 34, 1201–1208. doi:10.1007/s12272-011-0719-6
Lee, M. S., Hwang, J. T., Kim, S. H., Yoon, S., Kim, M. S., Yang, H. J., et al. (2010). Ginsenoside Rc, an active component of Panax ginseng, stimulates glucose uptake in C2C12 myotubes through an AMPK-dependent mechanism. J. Ethnopharmacol. 127, 771–776. doi:10.1016/j.jep.2009.11.022
Lee, O. H., Lee, H. H., Kim, J. H., and Lee, B. Y. (2011b). Effect of ginsenosides Rg3 and Re on glucose transport in mature 3T3-L1 adipocytes. Phytother. Res. 25, 768–773. doi:10.1002/ptr.3322
Li, C. W., Deng, M. Z., Gao, Z. J., Dang, Y. Y., Zheng, G. D., Yang, X. J., et al. (2020). Effects of compound K, a metabolite of ginsenosides, on memory and cognitive dysfunction in db/db mice involve the inhibition of ER stress and the NLRP3 inflammasome pathway. Food Funct. 11, 4416–4427. doi:10.1039/c9fo02602a
Li, J., Zhao, J., Wang, X., Lin, Z., Lin, H., and Lin, Z. (2024). Ginsenoside - a promising natural active ingredient with steroidal hormone activity. Food Funct. 15, 1825–1839. doi:10.1039/d3fo05484e
Li, J. B., Zhang, R., Han, X., and Piao, C. L. (2018). Ginsenoside Rg1 inhibits dietary-induced obesity and improves obesity-related glucose metabolic disorders. Braz. J. Med. Biol. Res. 51, e7139. doi:10.1590/1414-431X20177139
Li, W., Li, H., Zheng, L., Xia, J., Yang, X., Men, S., et al. (2023). Ginsenoside CK improves skeletal muscle insulin resistance by activating DRP1/PINK1-mediated mitophagy. Food Funct. 14, 1024–1036. doi:10.1039/d2fo02026b
Li, W., Zhang, M., Gu, J., Meng, Z. J., Zhao, L. C., Zheng, Y. N., et al. (2012). Hypoglycemic effect of protopanaxadiol-type ginsenosides and compound K on Type 2 diabetes mice induced by high-fat diet combining with streptozotocin via suppression of hepatic gluconeogenesis. Fitoterapia 83, 192–198. doi:10.1016/j.fitote.2011.10.011
Li, X., Zhang, Z., Wang, R., Xia, X., Liu, Y., and Sun, L. (2017). Ginsenoside Rb1 prevents high glucose-induced Schwann cell injury through the mitochondrial apoptosis pathway. J. Tradit. Chin. Med. 37, 746–755. doi:10.1016/s0254-6272(18)30037-2
Li, X. Q., and Huang, T. Y. (2021). Notoginsenoside R1 alleviates high glucose-induced inflammation and oxidative stress in HUVECs via upregulating miR-147a. Kaohsiung J. Med. Sci. 37, 1101–1112. doi:10.1002/kjm2.12433
Li, Y., Hou, J. G., Liu, Z., Gong, X. J., Hu, J. N., Wang, Y. P., et al. (2021). Alleviative effects of 20(R)-Rg3 on HFD/STZ-induced diabetic nephropathy via MAPK/NF-κB signaling pathways in C57BL/6 mice. J. Ethnopharmacol. 267, 113500. doi:10.1016/j.jep.2020.113500
Liang, M., Zhan, W., Wang, L., Bei, W., and Wang, W. (2023). Ginsenoside Rb1 promotes hepatic glycogen synthesis to ameliorate T2DM through 15-PGDH/PGE(2)/EP4 signaling pathway. Diabetes Metab. Syndr. Obes. 16, 3223–3234. doi:10.2147/DMSO.S431423
Liu, D., Zhang, H., Gu, W., Liu, Y., and Zhang, M. (2014). Ginsenoside Rb1 protects hippocampal neurons from high glucose-induced neurotoxicity by inhibiting GSK3β-mediated CHOP induction. Mol. Med. Rep. 9, 1434–1438. doi:10.3892/mmr.2014.1958
Liu, J., Jiang, C., Ma, X., Feng, L., and Wang, J. (2019a). Notoginsenoside Fc accelerates reendothelialization following vascular injury in diabetic rats by promoting endothelial cell autophagy. J. Diabetes Res. 2019, 9696521. doi:10.1155/2019/9696521
Liu, J., Jiang, C., Ma, X., and Wang, J. (2018). Notoginsenoside Fc attenuates high glucose-induced vascular endothelial cell injury via upregulation of PPAR-γ in diabetic Sprague-Dawley rats. Vasc. Pharmacol. 109, 27–35. doi:10.1016/j.vph.2018.05.009
Liu, Q., Zhang, F. G., Zhang, W. S., Pan, A., Yang, Y. L., Liu, J. F., et al. (2017). Ginsenoside Rg1 inhibits glucagon-induced hepatic gluconeogenesis through akt-FoxO1 interaction. Theranostics 7, 4001–4012. doi:10.7150/thno.18788
Liu, R., Xu, L. X., Tong, L. J., Wu, H. Y., Guo, Q., Sun, Z. M., et al. (2024). Therapeutic effects of ginsenosides on osteoporosis for novel drug applications. Eur. J. Pharmacol. 974, 176604. doi:10.1016/j.ejphar.2024.176604
Liu, T., Peng, Y. F., Jia, C., Yang, B. H., Tao, X., Li, J., et al. (2015). Ginsenoside Rg3 improves erectile function in streptozotocin-induced diabetic rats. J. Sex. Med. 12, 611–620. doi:10.1111/jsm.12779
Liu, Y., Deng, J., and Fan, D. (2019b). Ginsenoside Rk3 ameliorates high-fat-diet/streptozocin induced type 2 diabetes mellitus in mice via the AMPK/Akt signaling pathway. Food Funct. 10, 2538–2551. doi:10.1039/c9fo00095j
Liu, Y., Deng, J., and Fan, D. (2021). G-Rh4 improves pancreatic β-cells dysfunction in vivo and in vitro by increased expression of Nrf2 and its target genes. Food Chem. Toxicol. 148, 111925. doi:10.1016/j.fct.2020.111925
Liu, Y. W., Zhu, X., Li, W., Lu, Q., Wang, J. Y., Wei, Y. Q., et al. (2012). Ginsenoside re attenuates diabetes-associated cognitive deficits in rats. Pharmacol. Biochem. Behav. 101, 93–98. doi:10.1016/j.pbb.2011.12.003
Liu, Z., Wang, H., Hou, G., Cao, H., Zhao, Y., and Yang, B. (2019c). Notoginsenoside R1 protects oxygen and glucose deprivation-induced injury by upregulation of miR-21 in cardiomyocytes. J. Cell. Biochem. 120, 9181–9192. doi:10.1002/jcb.28194
Lo, S. H., Hsu, C. T., Niu, H. S., Niu, C. S., Cheng, J. T., and Chen, Z. C. (2017). Ginsenoside Rh2 improves cardiac fibrosis via pparδ-STAT3 signaling in type 1-like diabetic rats. Int. J. Mol. Sci. 18, 1364. doi:10.3390/ijms18071364
Lu, M., Zhao, F., Ran, C., Xu, Y., Zhang, J., and Wang, H. (2023). Ginsenoside Rg1 attenuates diabetic vascular endothelial dysfunction by inhibiting the calpain-1/ROS/PKC-β axis. Life Sci. 329, 121972. doi:10.1016/j.lfs.2023.121972
Miao, L., Zhou, Y., Tan, D., Zhou, C., Ruan, C. C., Wang, S., et al. (2024). Ginsenoside Rk1 improves endothelial function in diabetes through activating peroxisome proliferator-activated receptors. Food Funct. 15, 5485–5495. doi:10.1039/d3fo05222b
Mittal, R., McKenna, K., Keith, G., McKenna, E., Lemos, J., Mittal, J., et al. (2025). Diabetic peripheral neuropathy and neuromodulation techniques: a systematic review of progress and prospects. Neural Regen. Res. 20, 2218–2230. doi:10.4103/NRR.NRR-D-24-00270
Młynarska, E., Czarnik, W., Dzieża, N., Jędraszak, W., Majchrowicz, G., Prusinowski, F., et al. (2025). Type 2 diabetes mellitus: new pathogenetic mechanisms, treatment and the most important complications. Int. J. Mol. Sci. 26, 1094. doi:10.3390/ijms26031094
Namgoong, S., Lee, H., Han, S. K., Lee, H. W., Jeong, S. H., and Dhong, E. S. (2019). Effect of Panax ginseng extract on the activity of diabetic fibroblasts in vitro. Int. Wound J. 16, 737–745. doi:10.1111/iwj.13091
Niu, J., Zhu, G., and Zhang, J. (2025). Ginseng in delaying brain aging: progress and Perspectives. Phytomedicine 140, 156587. doi:10.1016/j.phymed.2025.156587
Park, J., Shin, Y. K., Kim, U., and Seol, G. H. (2023). Ginsenoside Rb(1) reduces hyper-vasoconstriction induced by high glucose and endothelial dysfunction in rat aorta. Pharm. (Basel) 16, 1238. doi:10.3390/ph16091238
Park, M. W., Ha, J., and Chung, S. H. (2008a). 20(S)-ginsenoside Rg3 enhances glucose-stimulated insulin secretion and activates AMPK. Biol. Pharm. Bull. 31, 748–751. doi:10.1248/bpb.31.748
Park, S., Ahn, I. S., Kwon, D. Y., Ko, B. S., and Jun, W. K. (2008b). Ginsenosides Rb1 and Rg1 suppress triglyceride accumulation in 3T3-L1 adipocytes and enhance beta-cell insulin secretion and viability in Min6 cells via PKA-dependent pathways. Biosci. Biotechnol. Biochem. 72, 2815–2823. doi:10.1271/bbb.80205
Peng, M., Wang, L., Su, H., Zhang, L., Yang, Y., Sun, L., et al. (2022). Ginsenoside Rg1 improved diabetes through regulating the intestinal microbiota in high-fat diet and streptozotocin-induced type 2 diabetes rats. J. Food Biochem. 46, e14321. doi:10.1111/jfbc.14321
Qi, L., Zhang, J., Wang, J., An, J., Xue, W., Liu, Q., et al. (2022). Mechanisms of ginsenosides exert neuroprotective effects on spinal cord injury: a promising traditional Chinese medicine. Front. Neurosci. 16, 969056. doi:10.3389/fnins.2022.969056
Qin, L., Wang, J., Zhao, R., Zhang, X., and Mei, Y. (2019a). Ginsenoside-Rb1 improved diabetic cardiomyopathy through regulating calcium signaling by alleviating protein O-GlcNAcylation. J. Agric. Food Chem. 67, 14074–14085. doi:10.1021/acs.jafc.9b05706
Qin, Q., Lin, N., Huang, H., Zhang, X., Cao, X., Wang, Y., et al. (2019b). Ginsenoside Rg1 ameliorates cardiac oxidative stress and inflammation in streptozotocin-induced diabetic rats. Diabetes Metab. Syndr. Obes. 12, 1091–1103. doi:10.2147/DMSO.S208989
Quan, H. Y., Yuan, H. D., Jung, M. S., Ko, S. K., Park, Y. G., and Chung, S. H. (2012). Ginsenoside re lowers blood glucose and lipid levels via activation of AMP-activated protein kinase in HepG2 cells and high-fat diet fed mice. Int. J. Mol. Med. 29, 73–80. doi:10.3892/ijmm.2011.805
Scairati, R., Auriemma, R. S., Del Vecchio, G., Di Meglio, S., Pirchio, R., Graziadio, C., et al. (2025). Diabetes mellitus, vaginal microbiome and sexual function: outcomes in postmenopausal women. Maturitas 194, 108210. doi:10.1016/j.maturitas.2025.108210
Shahcheraghi, S. H., Aljabali, A., Al Zoubi, M. S., Mishra, V., Charbe, N. B., Haggag, Y. A., et al. (2021). Overview of key molecular and pharmacological targets for diabetes and associated diseases. Life Sci. 278, 119632. doi:10.1016/j.lfs.2021.119632
Shang, W., Yang, Y., Zhou, L., Jiang, B., Jin, H., and Chen, M. (2008). Ginsenoside Rb1 stimulates glucose uptake through insulin-like signaling pathway in 3T3-L1 adipocytes. J. Endocrinol. 198, 561–569. doi:10.1677/JOE-08-0104
Shao, X., Li, N., Zhan, J., Sun, H., An, L., and Du, P. (2015). Protective effect of compound K on diabetic rats. Nat. Prod. Commun. 10, 243–245. doi:10.1177/1934578x1501000206
Shen, J., Zhao, Z., Shang, W., Liu, C., Zhang, B., Zhao, L., et al. (2017). Ginsenoside Rg1 nanoparticle penetrating the blood-brain barrier to improve the cerebral function of diabetic rats complicated with cerebral infarction. Int. J. Nanomedicine 12, 6477–6486. doi:10.2147/IJN.S139602
Shen, Y., Chen, W., Lin, K., Zhang, H., Guo, X., An, X., et al. (2024). Notoginsenoside Fc, a novel renoprotective agent, ameliorates glomerular endothelial cells pyroptosis and mitochondrial dysfunction in diabetic nephropathy through regulating HMGCS2 pathway. Phytomedicine 126, 155445. doi:10.1016/j.phymed.2024.155445
Shi, Y., Wan, X., Shao, N., Ye, R., Zhang, N., and Zhang, Y. (2016). Protective and anti-angiopathy effects of ginsenoside re against diabetes mellitus via the activation of p38 MAPK, ERK1/2 and JNK signaling. Mol. Med. Rep. 14, 4849–4856. doi:10.3892/mmr.2016.5821
Singh, A., Shadangi, S., Gupta, P. K., and Rana, S. (2025). Type 2 diabetes mellitus: a comprehensive review of pathophysiology, comorbidities, and emerging therapies. Compr. Physiol. 15, e70003. doi:10.1002/cph4.70003
Song, B., Ding, L., Zhang, H., Chu, Y., Chang, Z., Yu, Y., et al. (2017). Ginsenoside Rb1 increases insulin sensitivity through suppressing 11β-hydroxysteroid dehydrogenase type I. Am. J. Transl. Res. 9, 1049–1057.
Song, W., Wei, L., Du, Y., Wang, Y., and Jiang, S. (2018). Protective effect of ginsenoside metabolite compound K against diabetic nephropathy by inhibiting NLRP3 inflammasome activation and NF-κB/p38 signaling pathway in high-fat diet/streptozotocin-induced diabetic mice. Int. Immunopharmacol. 63, 227–238. doi:10.1016/j.intimp.2018.07.027
Su, H., Tian, C. J., Wang, Y., Shi, J., Chen, X., Zhen, Z., et al. (2022a). Ginsenoside Rb1 reduces oxidative/carbonyl stress damage and ameliorates inflammation in the lung of streptozotocin-induced diabetic rats. Pharm. Biol. 60, 2229–2236. doi:10.1080/13880209.2022.2140168
Su, W. Y., Fan, M. L., Li, Y., Hu, J. N., Cai, E. B., Zhu, H. Y., et al. (2022b). 20(S)-ginsenoside Rh1 alleviates T2DM induced liver injury via the Akt/FOXO1 pathway. Chin. J. Nat. Med. 20, 669–678. doi:10.1016/S1875-5364(22)60201-2
Su, W. Y., Li, Y., Chen, X., Li, X., Wei, H., Liu, Z., et al. (2021). Ginsenoside Rh1 improves type 2 diabetic nephropathy through AMPK/PI3K/Akt-Mediated inflammation and apoptosis signaling pathway. Am. J. Chin. Med. 49, 1215–1233. doi:10.1142/S0192415X21500580
Sui, Z., Sui, D., Li, M., Yu, Q., Li, H., and Jiang, Y. (2023). Ginsenoside Rg3 has effects comparable to those of ginsenoside re on diabetic kidney disease prevention in db/db mice by regulating inflammation, fibrosis and PPARγ. Mol. Med. Rep. 27, 84. doi:10.3892/mmr.2023.12971
Sun, H. Q., and Zhou, Z. Y. (2010). Effect of ginsenoside-Rg3 on the expression of VEGF and TNF-α in retina with diabetic rats. Int. J. Ophthalmol. 3, 220–223. doi:10.3980/j.issn.2222-3959.2010.03.09
Tang, K., Qin, W., Wei, R., Jiang, Y., Fan, L., Wang, Z., et al. (2022). Ginsenoside Rd ameliorates high glucose-induced retinal endothelial injury through AMPK-STRT1 interdependence. Pharmacol. Res. 179, 106123. doi:10.1016/j.phrs.2022.106123
Tian, F., Huang, S., Xu, W., Chen, L., Su, J., Ni, H., et al. (2022a). Compound K attenuates hyperglycemia by enhancing glucagon-like peptide-1 secretion through activating TGR5 via the remodeling of gut microbiota and bile acid metabolism. J. Ginseng Res. 46, 780–789. doi:10.1016/j.jgr.2022.03.006
Tian, F., Wang, X., Ni, H., Feng, X., Yuan, X., and Huang, Q. (2021). The ginsenoside metabolite compound K stimulates glucagon-like peptide-1 secretion in NCI-H716 cells by regulating the RhoA/ROCKs/YAP signaling pathway and cytoskeleton formation. J. Pharmacol. Sci. 145, 88–96. doi:10.1016/j.jphs.2020.11.005
Tian, F., Xu, W., Chen, L., Chen, T., Feng, X., Chen, J., et al. (2022b). Ginsenoside compound K increases glucagon-like peptide-1 release and L-cell abundance in db/db mice through TGR5/YAP signaling. Int. Immunopharmacol. 113, 109405. doi:10.1016/j.intimp.2022.109405
Tian, Z., Ren, N., Wang, J., Zhang, D., and Zhou, Y. (2018). Ginsenoside ameliorates cognitive dysfunction in type 2 diabetic goto-kakizaki rats. Med. Sci. Monit. 24, 3922–3928. doi:10.12659/MSM.907417
Vonderau, J. S., and Desai, C. S. (2022). Type 3c: understanding pancreatogenic diabetes. JAAPA 35, 20–24. doi:10.1097/01.JAA.0000885140.47709.6f
Vong, C. T., Tan, D., Liao, F., Chen, Z., Chen, Z., Tseng, H., et al. (2024). Ginsenoside Rk1 ameliorates ER stress-induced apoptosis through directly activating IGF-1R in mouse pancreatic [formula: see text]-cells and diabetic pancreas. Am. J. Chin. Med. 52, 1195–1211. doi:10.1142/S0192415X24500484
Wagner, R., Eckstein, S. S., Yamazaki, H., Gerst, F., Machann, J., Jaghutriz, B. A., et al. (2022). Metabolic implications of pancreatic fat accumulation. Nat. Rev. Endocrinol. 18, 43–54. doi:10.1038/s41574-021-00573-3
Wang, D. S., Wang, J. M., Zhang, F. R., Lei, F. J., Wen, X., Song, J., et al. (2022a). Ameliorative effects of malonyl ginsenoside from Panax ginseng on glucose-lipid metabolism and insulin resistance via IRS1/PI3K/akt and AMPK signaling pathways in type 2 diabetic mice. Am. J. Chin. Med. 50, 863–882. doi:10.1142/S0192415X22500367
Wang, H., Wu, W., Wang, G., Xu, W., Zhang, F., Wu, B., et al. (2019a). Protective effect of ginsenoside Rg3 on lung injury in diabetic rats. J. Cell. Biochem. 120, 3323–3330. doi:10.1002/jcb.27601
Wang, M., Cai, C., Jin, W., Zhao, Y., Mu, Y., Ren, L., et al. (2024a). 20(S)-ginsenoside Rg3 protects against diabetic muscle atrophy by promoting myoblastic differentiation and protecting mitochondrial function. Phytomedicine 134, 155964. doi:10.1016/j.phymed.2024.155964
Wang, W., Guan, F., Sagratini, G., Yan, J., Xie, J., Jin, Z., et al. (2023). Ginsenoside Rd attenuated hyperglycemia via Akt pathway and modulated gut microbiota in streptozotocin-induced diabetic rats. Curr. Res. Food Sci. 6, 100491. doi:10.1016/j.crfs.2023.100491
Wang, W., Hao, Y., and Li, F. (2019b). Notoginsenoside R1 alleviates high glucose-evoked damage in RSC96 cells through down-regulation of miR-503. Artif. Cells Nanomed Biotechnol. 47, 3947–3954. doi:10.1080/21691401.2019.1671434
Wang, X., and Cao, Y. (2025). A narrative review: relationship between glycemic variability and emerging complications of diabetes mellitus. Biomolecules 15, 188. doi:10.3390/biom15020188
Wang, Y., Sun, X., Xie, Y., Du, A., Chen, M., Lai, S., et al. (2024b). Panax notoginseng saponins alleviate diabetic retinopathy by inhibiting retinal inflammation: association with the NF-κB signaling pathway. J. Ethnopharmacol. 319, 117135. doi:10.1016/j.jep.2023.117135
Wang, Y., Wang, H., Liu, Y., Li, C., Qi, P., and Bao, J. (2012). Antihyperglycemic effect of ginsenoside Rh2 by inducing islet β-cell regeneration in mice. Horm. Metab. Res. 44, 33–40. doi:10.1055/s-0031-1295416
Wang, Z. C., Niu, K. M., Wu, Y. J., Du, K. R., Qi, L. W., Zhou, Y. B., et al. (2022b). A dual Keap1 and p47(phox) inhibitor Ginsenoside Rb1 ameliorates high glucose/ox-LDL-induced endothelial cell injury and atherosclerosis. Cell. Death Dis. 13, 824. doi:10.1038/s41419-022-05274-x
Wei, S., Li, W., Yu, Y., Yao, F., A, L., Lan, X., et al. (2015). Ginsenoside Compound K suppresses the hepatic gluconeogenesis via activating adenosine-5'monophosphate kinase: a study in vitro and in vivo. Life Sci. 139, 8–15. doi:10.1016/j.lfs.2015.07.032
Wei, Y., Yang, H., Zhu, C., Deng, J., and Fan, D. (2020). Hypoglycemic effect of ginsenoside Rg5 mediated partly by modulating gut microbiota dysbiosis in diabetic db/db mice. J. Agric. Food Chem. 68, 5107–5117. doi:10.1021/acs.jafc.0c00605
Wu, Y., Xia, Z. Y., Dou, J., Zhang, L., Xu, J. J., Zhao, B., et al. (2011). Protective effect of ginsenoside Rb1 against myocardial ischemia/reperfusion injury in streptozotocin-induced diabetic rats. Mol. Biol. Rep. 38, 4327–4335. doi:10.1007/s11033-010-0558-4
Xia, W., Zhu, Z., Xiang, S., and Yang, Y. (2023). Ginsenoside Rg5 promotes wound healing in diabetes by reducing the negative regulation of SLC7A11 on the efferocytosis of dendritic cells. J. Ginseng Res. 47, 784–794. doi:10.1016/j.jgr.2023.06.006
Xiao, N., Lou, M. D., Lu, Y. T., Yang, L. L., Liu, Q., Liu, B., et al. (2017). Ginsenoside Rg5 attenuates hepatic glucagon response via suppression of succinate-associated HIF-1α induction in HFD-fed mice. Diabetologia 60, 1084–1093. doi:10.1007/s00125-017-4238-y
Xie, W., Zhou, P., Qu, M., Dai, Z., Zhang, X., Zhang, C., et al. (2020). Ginsenoside re attenuates high glucose-induced RF/6A injury via regulating PI3K/AKT inhibited HIF-1α/VEGF signaling pathway. Front. Pharmacol. 11, 695. doi:10.3389/fphar.2020.00695
Xiong, Y., Shen, L., Liu, K. J., Tso, P., Xiong, Y., Wang, G., et al. (2010). Antiobesity and antihyperglycemic effects of ginsenoside Rb1 in rats. Diabetes 59, 2505–2512. doi:10.2337/db10-0315
Xu, J., Dong, J., Ding, H., Wang, B., Wang, Y., Qiu, Z., et al. (2022). Ginsenoside compound K inhibits obesity-induced insulin resistance by regulation of macrophage recruitment and polarization via activating PPARγ. Food Funct. 13, 3561–3571. doi:10.1039/d1fo04273d
Xu, J., Li, T., Xia, X., Fu, C., Wang, X., and Zhao, Y. (2020). Dietary ginsenoside T19 supplementation regulates glucose and lipid metabolism via AMPK and PI3K pathways and its effect on intestinal microbiota. J. Agric. Food Chem. 68, 14452–14462. doi:10.1021/acs.jafc.0c04429
Xue, B., Sun, L., Li, X., Wang, X., Zhang, Y., Mu, Y., et al. (2012). Ginsenoside Rb1 relieves glucose fluctuation-induced oxidative stress and apoptosis in Schwann cells. Neural Regen. Res. 7, 2340–2346. doi:10.3969/j.issn.1673-5374.2012.30.003
Xue, L., Hu, M., Zhu, Q., Li, Y., Zhou, G., Zhang, X., et al. (2023). GRg1 inhibits the TLR4/NF-kB signaling pathway by upregulating miR-216a-5p to reduce growth factors and inflammatory cytokines in DR. Mol. Biol. Rep. 50, 9379–9394. doi:10.1007/s11033-023-08895-3
Xue, L. P., Fu, X. L., Hu, M., Zhang, L. W., Li, Y. D., Peng, Y. L., et al. (2018). Rg1 inhibits high glucose-induced mesenchymal activation and fibrosis via regulating miR-2113/RP11-982M15.8/Zeb1 pathway. Biochem. Biophys. Res. Commun. 501, 827–832. doi:10.1016/j.bbrc.2018.04.055
Yang, J., and Liu, Z. (2022). Mechanistic pathogenesis of endothelial dysfunction in diabetic nephropathy and retinopathy. Front. Endocrinol. (Lausanne) 13, 816400. doi:10.3389/fendo.2022.816400
Yang, N., Chen, P., Tao, Z., Zhou, N., Gong, X., Xu, Z., et al. (2012). Beneficial effects of ginsenoside-Rg1 on ischemia-induced angiogenesis in diabetic mice. Acta Biochim. Biophys. Sin. (Shanghai) 44, 999–1005. doi:10.1093/abbs/gms092
Yang, R., Jiang, X., He, X., Liang, D., Sun, S., and Zhou, G. (2020). Ginsenoside Rb1 improves cognitive impairment induced by insulin resistance through cdk5/p35-NMDAR-IDE pathway. Biomed. Res. Int. 2020, 3905719. doi:10.1155/2020/3905719
Yang, T., Qi, F., Guo, F., Shao, M., Song, Y., Ren, G., et al. (2024). An update on chronic complications of diabetes mellitus: from molecular mechanisms to therapeutic strategies with a focus on metabolic memory. Mol. Med. 30, 71. doi:10.1186/s10020-024-00824-9
Ying, Y., Zhang, Y. L., Ma, C. J., Li, M. Q., Tang, C. Y., Yang, Y. F., et al. (2019). Neuroprotective effects of ginsenoside Rg1 against hyperphosphorylated tau-induced diabetic retinal neurodegeneration via activation of IRS-1/akt/gsk3β signaling. J. Agric. Food Chem. 67, 8348–8360. doi:10.1021/acs.jafc.9b02954
Yu, H., Zhen, J., Yang, Y., Gu, J., Wu, S., and Liu, Q. (2016). Ginsenoside Rg1 ameliorates diabetic cardiomyopathy by inhibiting endoplasmic reticulum stress-induced apoptosis in a streptozotocin-induced diabetes rat model. J. Cell. Mol. Med. 20, 623–631. doi:10.1111/jcmm.12739
Yu, H. T., Zhen, J., Pang, B., Gu, J. N., and Wu, S. S. (2015). Ginsenoside Rg1 ameliorates oxidative stress and myocardial apoptosis in streptozotocin-induced diabetic rats. J. Zhejiang Univ. Sci. B 16, 344–354. doi:10.1631/jzus.B1400204
Yuan, C., Wang, H., and Yuan, Z. (2019). Ginsenoside Rg1 inhibits myocardial ischaemia and reperfusion injury via HIF-1 α-ERK signalling pathways in a diabetic rat model. Pharmazie 74, 157–162. doi:10.1691/ph.2019.8858
Yuan, H. D., Kim, D. Y., Quan, H. Y., Kim, S. J., Jung, M. S., and Chung, S. H. (2012). Ginsenoside Rg2 induces orphan nuclear receptor SHP gene expression and inactivates GSK3β via AMP-activated protein kinase to inhibit hepatic glucose production in HepG2 cells. Chem. Biol. Interact. 195, 35–42. doi:10.1016/j.cbi.2011.10.006
Zhai, Y., Meng, X., Luo, Y., Wu, Y., Ye, T., Zhou, P., et al. (2018). Notoginsenoside R1 ameliorates diabetic encephalopathy by activating the Nrf2 pathway and inhibiting NLRP3 inflammasome activation. Oncotarget 9, 9344–9363. doi:10.18632/oncotarget.24295
Zhang, B., Zhang, X., Zhang, C., Shen, Q., Sun, G., and Sun, X. (2019). Notoginsenoside R1 protects db/db mice against diabetic nephropathy via upregulation of nrf2-mediated HO-1 expression. Molecules 24, 247. doi:10.3390/molecules24020247
Zhang, C., Han, M., Zhang, X., Tong, H., Sun, X., and Sun, G. (2022). Ginsenoside Rb1 protects against diabetic cardiomyopathy by regulating the adipocytokine pathway. J. Inflamm. Res. 15, 71–83. doi:10.2147/JIR.S348866
Zhang, C., Yu, H., Ye, J., Tong, H., Wang, M., and Sun, G. (2023). Ginsenoside Rg3 protects against diabetic cardiomyopathy and promotes adiponectin signaling via activation of PPAR-γ. Int. J. Mol. Sci. 24, 16736. doi:10.3390/ijms242316736
Zhang, E. Y., Gao, B., Shi, H. L., Huang, L. F., Yang, L., Wu, X. J., et al. (2017). 20(S)-Protopanaxadiol enhances angiogenesis via HIF-1α-mediated VEGF secretion by activating p70S6 kinase and benefits wound healing in genetically diabetic mice. Exp. Mol. Med. 49, e387. doi:10.1038/emm.2017.151
Zhang, J. H., Yang, H. Z., Su, H., Song, J., Bai, Y., Deng, L., et al. (2021a). Berberine and ginsenoside Rb1 ameliorate depression-like behavior in diabetic rats. Am. J. Chin. Med. 49, 1195–1213. doi:10.1142/S0192415X21500579
Zhang, Q. Y., Zhang, H. Y., Feng, S. G., Yao, M. D., Ding, J. J., Li, X. M., et al. (2025). Macrophage metabolic reprogramming ameliorates diabetes-induced microvascular dysfunction. Redox Biol. 79, 103449. doi:10.1016/j.redox.2024.103449
Zhang, X., Wang, L., Guo, R., Xiao, J., Liu, X., Dong, M., et al. (2021b). Ginsenoside Rb1 ameliorates diabetic arterial stiffening via AMPK pathway. Front. Pharmacol. 12, 753881. doi:10.3389/fphar.2021.753881
Zhao, X., Liu, S., Wang, X., Chen, Y., Pang, P., Yang, Q., et al. (2022). Diabetic cardiomyopathy: clinical phenotype and practice. Front. Endocrinol. (Lausanne) 13, 1032268. doi:10.3389/fendo.2022.1032268
Zhao, Y., Liu, Y., Deng, J., Zhu, C., Ma, X., Jiang, M., et al. (2023). Ginsenoside F4 alleviates skeletal muscle insulin resistance by regulating PTP1B in type II diabetes mellitus. J. Agric. Food Chem. 71, 14263–14275. doi:10.1021/acs.jafc.3c01262
Zhen, J., Bai, J., Liu, J., Men, H., and Yu, H. (2024). Ginsenoside RG1-induced mesenchymal stem cells alleviate diabetic cardiomyopathy through secreting exosomal circNOTCH1 to promote macrophage M2 polarization. Phytother. Res. 38, 1745–1760. doi:10.1002/ptr.8018
Zhou, P., Xie, W., Meng, X., Zhai, Y., Dong, X., Zhang, X., et al. (2019). Notoginsenoside R1 ameliorates diabetic retinopathy through PINK1-dependent activation of mitophagy. Cells 8, 213. doi:10.3390/cells8030213
Zhou, R., He, D., Zhang, H., Xie, J., Zhang, S., Tian, X., et al. (2023). Ginsenoside Rb1 protects against diabetes-associated metabolic disorders in Kkay mice by reshaping gut microbiota and fecal metabolic profiles. J. Ethnopharmacol. 303, 115997. doi:10.1016/j.jep.2022.115997
Zhou, T., Sun, L., Yang, S., Lv, Y., Cao, Y., Gang, X., et al. (2020). 20(S)-Ginsenoside Rg3 protects kidney from diabetic kidney disease via renal inflammation depression in diabetic rats. J. Diabetes Res. 2020, 7152176. doi:10.1155/2020/7152176
Zhu, Y., Yang, H., Deng, J., and Fan, D. (2021). Ginsenoside Rg5 improves insulin resistance and mitochondrial biogenesis of liver via regulation of the sirt1/PGC-1α signaling pathway in db/db mice. J. Agric. Food Chem. 69, 8428–8439. doi:10.1021/acs.jafc.1c02476
Zhu, Y., Zhu, C., Yang, H., Deng, J., and Fan, D. (2020). Protective effect of ginsenoside Rg5 against kidney injury via inhibition of NLRP3 inflammasome activation and the MAPK signaling pathway in high-fat diet/streptozotocin-induced diabetic mice. Pharmacol. Res. 155, 104746. doi:10.1016/j.phrs.2020.104746
Keywords: type 2 diabetes mellitus, ginsenoside, traditional Chinese medicine, therapeutic affect, complications
Citation: Liu Y, Ju Y, Wang Y, Cui X, Sun Y, Hu P and Chen Y (2025) Ginsenoside in the treatment of type 2 diabetes and its complications: a promising traditional chinese medicine. Front. Pharmacol. 16:1593780. doi: 10.3389/fphar.2025.1593780
Received: 18 March 2025; Accepted: 25 April 2025;
Published: 13 May 2025.
Edited by:
Rong-Rong He, Jinan University, ChinaReviewed by:
Xiaofei Yang, Beijing University of Chinese Medicine, ChinaXu Liu, Chengdu University of Traditional Chinese Medicine, China
Copyright © 2025 Liu, Ju, Wang, Cui, Sun, Hu and Chen. This is an open-access article distributed under the terms of the Creative Commons Attribution License (CC BY). The use, distribution or reproduction in other forums is permitted, provided the original author(s) and the copyright owner(s) are credited and that the original publication in this journal is cited, in accordance with accepted academic practice. No use, distribution or reproduction is permitted which does not comply with these terms.
*Correspondence: Yan Chen, amRleWx5eUBqbHUuZWR1LmNu