- 1Department of Biological and Environmental Sciences and Technologies (DiSTeBA), University of Salento, Lecce, Italy
- 2RG-ESA (Research Group on the Epistemology of the Sciences of the Artificial), Libera Università di Lingue e Comunicazione (IULM), Milan, Italy
Advancements in the research on so-called “synthetic (artificial) cells” have been mainly characterized by an important acceleration in all sorts of experimental approaches, providing a growing amount of knowledge and techniques that will shape future successful developments. Synthetic cell technology, indeed, shows potential in driving a revolution in science and technology. On the other hand, theoretical and epistemological investigations related to what synthetic cells “are,” how they behave, and what their role is in generating knowledge have not received sufficient attention. Open questions about these less explored subjects range from the analysis of the organizational theories applied to synthetic cells to the study of the “relevance” of synthetic cells as scientific tools to investigate life and cognition; and from the recognition and the cultural reappraisal of cybernetic inheritance in synthetic biology to the need for developing concepts on synthetic cells and to the exploration, in a novel perspective, of information theories, complexity, and artificial intelligence applied in this novel field. In these contributions, we will briefly sketch some crucial aspects related to the aforementioned issues, based on our ongoing studies. An important take-home message will result: together with their impactful experimental results and potential applications, synthetic cells can play a major role in the exploration of theoretical questions as well.
1 Introduction
In recent years, the research on so-called “synthetic (artificial) cells” (SCs) has been characterized by a surprisingly strong momentum. The community of practitioners has grown significantly, also thanks to coordination initiatives like the MaxSynBio consortium in Germany, the BaSyC (Building a Synthetic Cell) project in the Netherlands, the European Synthetic Cell Initiative (SynCellEU), the Build-a-Cell community in the US, the fabriCELL project in the UK, Japanese programs such as CREST-PRESTO, and the research promoted by the Japanese Society for Cell Synthesis Research (Luisi, 2002; Salehi-Reyhani et al., 2017; Schwille et al., 2018; Stano, 2019; Staufer et al., 2021; Guindani et al., 2022).
A very impressive acceleration in experimental studies on the construction of SCs of different types can be identified just by looking at the number and quality of articles published on these subjects, very often in high impact-factor journals. This intense phase of research will probably attract many young and motivated scientists, who will pursue advanced studies on these “bottom-up” synthetic biology (SB) approaches in the upcoming years. Moreover, the combination of several strategies has produced a quite diversified research arena that literally shows the lively developments and the general enthusiasm around this topic—which is probably one of the most exciting among novel technologies. “SC technology,” indeed, does not resemble anything previously existing and shows potential in driving a revolution in future basic and applied sciences.1
Despite this impressive technical progress, important theoretical and epistemological issues have not received significant attention yet. We refer to fundamental questions such as 1) what SCs really are, i.e., what they represent in the context of artificial systems; 2) how their structure, properties, and behavior should be interpreted and with respect to which theoretical framework; and 3) what their role is in advancing scientific knowledge (what sort of knowledge SC research can generate). In this article, we are going to briefly consider some theoretical and epistemological issues related to SCs, selected among a list of subjects we consider particularly relevant to boost theoretical progress in this field (Table 1).
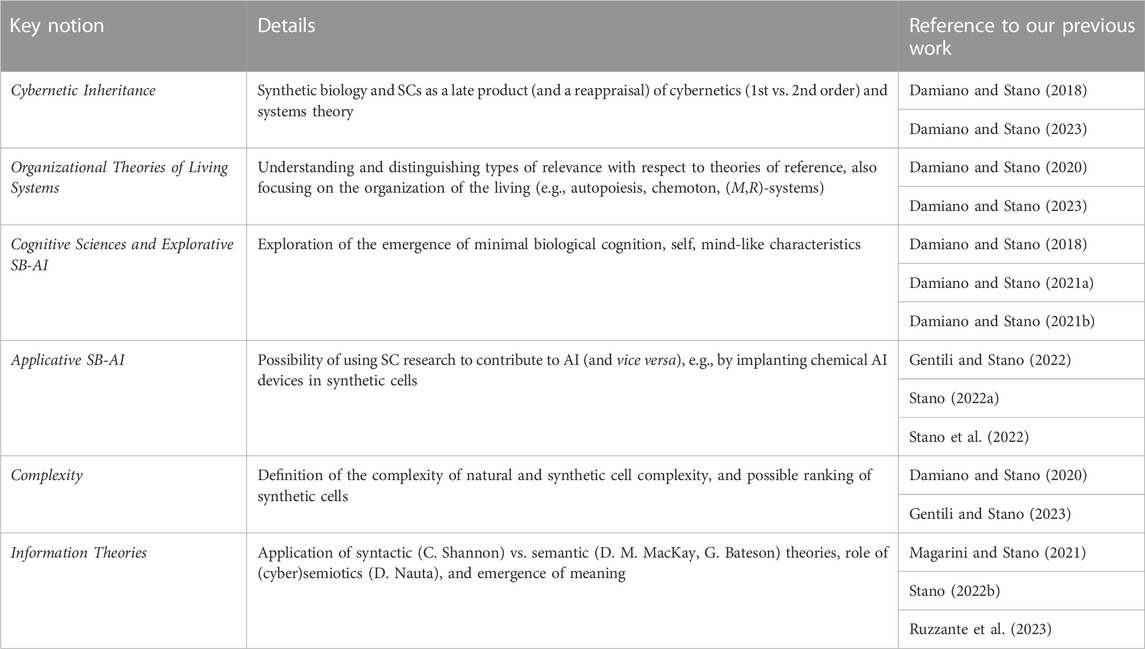
TABLE 1. List of theoretical and epistemological SC-related issues currently investigated by the authors.
In particular, in this Perspective, we will briefly sketch what we believe are crucial aspects of the first three entries in Table 1 and present some preliminary ideas based on our already-published and ongoing studies. This will be an opportunity to present a research path that conjugates chemistry, SB, and philosophy of science questions. An important finding will be observed: together with their impactful experimental results and potential applications, SCs (and, more generally, SB) can play a major role in the exploration of theoretical and epistemological questions.
2 The inheritance from cybernetics
Our path of cross-fertilization between technical and theoretical–epistemological aspects of SC research is grounded in the legacy left by cybernetics for this emerging area and, in particular, in the cybernetic foundations of the synthetic modeling of life and cognition. Conceiving SCs as cellular models is indeed tantamount to considering SCs as scientific tools (perhaps “the” scientific tools par excellence) for investigating the generative mechanisms and the emergence of life at the minimal complexity level, corresponding to simple unicellular organisms. However, as we will clarify in the following paragraphs, cognition is a property closely related, or even coincident, with the property of being alive (e.g., Bich and Damiano, 2012; Damiano and Stano, 2018). It is not surprising, then, that life and cognition have been envisioned as interwoven targets by scientists and philosophers.
As early as 1943, a series of inaugural works from pioneers of cybernetics—specifically, McCulloch and Pitts (1943), Craik (1943), Rosenblueth, Wiener and Bigelow (1943)—proposed epistemological and theoretical frameworks to ground the exploration of biological and cognitive processes via construction and experimental exploration of artificial systems—systems “made by man rather than nature” (Langton, 1989)—functioning as material models of these target biological processes. These authors’ groundbreaking work is not limited to the introduction of the “synthetic method,” i.e., the “understanding-by-building” approach, as a strategy to study experimentally the mechanisms underlying life and cognition not only in their components (analytic method) but also “in their functioning” (synthetic method). As particularly evident in the 1943 McCulloch–Pitts and Rosenblueth–Wiener–Bigelow articles, cybernetics has also associated synthetic modeling with the possibility, for scientific research, of releasing cognition from the “ghostly” status it had assumed in modern (Cartesian) science and reintegrating it among the processes explorable through experimental and quantitative research. In other words: to overcome the mind–body dualism characterizing the Cartesian, or modern, tradition of science and make operational a series of avant-garde theses that today characterize the embodied front of cognitive sciences and artificial intelligence (AI). In a nutshell, they can be summarized in the following two claims: 1) the biological body plays a significant role in natural cognitive processes, and thus, to study cognition based on the synthetic method, effective ways of modeling synthetically body dynamics and interactions are needed. 2) Given the biochemical nature of the body, the synthetic modeling of natural cognitive processes is likely to be more successful when based on biochemical techniques—i.e., wetware models of bodily processes and interactions.
“If an engineer were to design a robot, roughly similar in behavior to an animal organism, he would not attempt at present to make it out of proteins and other colloids. He would probably build it out of metallic parts, some dielectrics and many vacuum tubes. The movement of the robot could readily be much faster and more powerful than those of the original organism. Learning and memory, however, would be quite rudimentary. In future years, as the knowledge of colloids and protein increases, future engineers may attempt the design of robots not only with a behavior, but also with a structure similar to that of a mammal.” (Rosenblueth et al., 1943, p. 23).
This is the cybernetic legacy that, more than 50 years later, is committing SB to engage in the areas of biology and scientific AI. Since the era of cybernetics, SB has been considered by many scholars as the most promising candidate for approaching at the experimental levels the exploration of life and cognition by means of artificial models. Wetware approaches, typical of SB, promise to constitute a third dimension complementing, in a particularly relevant manner, hardware (robotic) and software (AI) approaches, thus forming with them a plural “science of the artificial” (Cordeschi, 2002; Damiano et al., 2011). However, the possibility of concretely offering SB this candidacy, in addition to technical advances, requires addressing a series of open theoretical and epistemological questions.
The following two sections of this short article intend to introduce a few of these questions and the related research lines that we have opened to tackle them.
3 The problem of the relevance of synthetic (SB/SC) models
SB’s transition to the status of an accepted science of life and cognition does not depend only on the soundness of technical solutions in modeling biological and cognitive processes. It also depends primarily, on the possibility, for SB, to address effectively the epistemological open questions concerning the synthetic modeling of life and cognition, which, if left unanswered, threaten its acceptance among the methodological strategies that the scientific community recognizes capable of producing valid insights. Among these issues, a particularly critical issue questions the relevance of synthetic models, understood as the contribution(s) that they can make to the scientific understanding of their target processes.2 The problem is particularly critical since synthetic models often appear to have a merely “imitative” value, whose significance for the advancement of scientific knowledge of life and cognition is uncertain. Furthermore, the current evaluation of synthetic models is often polarized in the sterile alternative between, on one hand, mere behavioral imitation and, on the other hand, full reproduction of target processes, whose relevance is not less problematic. Indeed, although models based on imitation of behaviors of the target systems—i.e., simple functional equivalence—are underdetermined, models that would reproduce in detail all the physicochemical characteristics of the target systems would be overdetermined as their realism would involve the inclusion of physical and functional properties, obscuring, instead of clarifying the mechanism underlying life and cognition.
This epistemological view, diffused in the debate since the era of cybernetics, has been mostly neglected in the context of synthetic modeling, where, since the 1950s, the most popular tool to assess the value of models is the Turing test (Turing, 1950), which focuses on their ability of imitating the target systems’ manifest behavior. Despite many critiques and reformulations, for which we refer to the literature, this test still constitutes a paradigmatic reference for evaluating the relevance of synthetic models, not only in AI but also in the field of explorative SB (Figures 1A,B), where it has been the basis of the first attempts at assessing the life-likeness of SCs (Cronin et al., 2006; Lentini et al., 2017; for a commentary, see Damiano and Stano, 2020). In our view, to effectively address the problem of the relevance of synthetic models, we need new criteria of relevance capable of overcoming both the traditional exclusive attention to imitation and the diffused polarization of the assessment between mere imitation and full reproduction of the target processes.
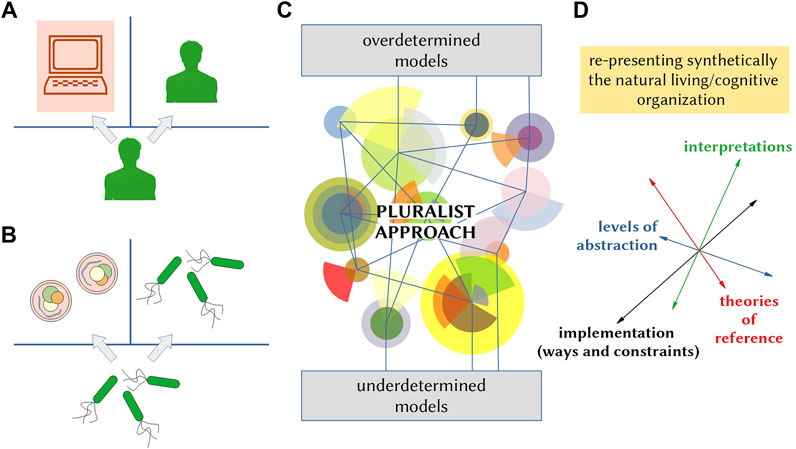
FIGURE 1. Problem of the relevance of synthetic (SB/SC) models. (A) Schematic representation of the classical version of the Turing test (Turing, 1950), also known as the imitation game. The test was devised to bypass the question “can a machine think?” or “what is intelligence?” and substitute it with an operational definition. A human interrogator blindly interacts with a computer or with another human. The machine behavior is defined “intelligent” when the interrogator is not able to distinguish it from a human. The machine “imitates” human intelligence. (B) Schematic representation of the Turing test in a SB scenario (Cronin et al., 2006). Living cells (e.g., bacteria) and SCs have substituted humans and computers. Chemical signaling or other kinds of interactions substitute the written dialogs (questions/answers) on which the original Turing test is based. By analogy, the test can be considered a tool to bypass the question “can a man-made chemical system be alive/cognitive?” or “what is life/cognition?,” providing an operational definition. A synthetic model of living/cognitive systems is alive/cognitive when living cells are not able to distinguish it from other living cells (more generally: when the synthetic model is behaviorally equivalent, for living cells, to what they perceive and dynamically interact with as their own environment). (C) Pictographic representation of the wide research space opened between underdetermined and overdetermined models, when a pluralist approach to the synthetic modeling of life and cognition is adopted. (D) Latter approach intends to overcome mere imitative modeling of life and cognition and proposes the scientific community to attempt at representing the biological/cognitive organizations synthetically by exploring a variety of theories of reference, diverse interpretations of these theories, and multiple options available with regard to the levels of abstraction, as well as the ways (and related constraints) of their implementation.
To fill this gap, we have undertaken an epistemological inquiry into the relevance of synthetic models. This research builds on elements of cybernetic and autopoietic epistemology to determine criteria to assess the different forms of relevance that (hardware, software, and wetware) models can have for the scientific understanding of life and cognition. As mentioned, the aim is the clarification of the contribution that SB can produce to advance scientific knowledge on life and cognition. Let us summarize here the essential elements that emerged from our exploration, a full discussion of which can be found elsewhere (Damiano and Stano, Artificial Life, in press).
Our work generated two relevance criteria for synthetic models which, to overcome the classical imitation paradigm and the related pure imitation/complete reproduction polarization, emphasize the importance of focusing the synthetic exploration of life and cognition on the organization underlying the target processes. This view, in our research, is associated with a pluralist perspective on synthetic modeling, according to which, to recreate synthetically the organizational mechanisms that in nature are responsible for producing a natural process, does not mean to reproduce “the real thing,” since this would require, from an epistemological viewpoint, the availability of a definitive, exhaustive, univocally interpretable, and perfectly implementable theory of the biological and cognitive organization—something beyond the reach of scientific research. In our view, any attempts at reproducing the organization of living and/or cognitive processes have to be based on one or more selections among a multiplicity of options since every target theory of biological and/or cognitive organization can be interpreted in different ways, each of its interpretations can be realized synthetically at a variety of different levels of abstraction, and each of these synthetic realizations, in order to be produced, requires addressing specific implementative constraints, which can be tackled in different ways.
This is the core of the pluralist approach to the synthetic modeling of life and cognition, which opens up a generative research space between underdetermined and overdetermined models (Figure 1C). Indeed, this approach engages the scientific community in implementing a variety of theories of biological/cognitive organization and, with regard to each of them, in exploring a variety of different ways of implementation, based on diverse interpretations of the theory of reference and the multiple options available with regard to the level of abstraction defining the synthetic realization (Figure 1D). In our perspective, scientifically, this approach is more generative than any attempts at overcoming imitation by trying to reproduce “the real thing”, as it is likely to generate a wide multiplicity of valuable insights, in line with the Langtonian ambition of creating a synthetic science of life and cognition as they are and as they “could be” (Langton, 1989).
4 The problem of representing the organizational complexity of life and cognition synthetically
Any attempt to build organizationally relevant synthetic models of biological and/or cognitive processes requires first choosing a theory of reference that offers a scientific description of the organization of life and/or cognition.
Among the organizational theories accessible today, our choice fell on autopoietic cognitive biology (Maturana and Varela, 1973) since this theory, on one hand, thematizes a profound continuity between life and cognition and, on the other hand, proposes a description of the biological and cognitive organization at the level of the minimal living unit. On this basis, this theory offers SB—and, in particular, SC research—a key role in the scientific understanding of cognitive processes. Indeed, autopoiesis generates the theoretical grounds to explore experimentally the controversial thesis that “life, as a process, is a process of cognition” (Maturana, 1969), and in this way, it proposes the experimental option of studying the threshold of minimal life and minimal cognition, as well as their relationship, by physically constructing wetware models of living/cognitive systems characterized by minimal complexity. This thesis, while being controversial, is extremely interesting for the purpose of de-anthropocentering the traditional philosophical and scientific views of cognitive processes. The related wetware experimental approach, proposing a wet version of the “understanding-by-building” methodology, relies on chemical and/or biochemical elements and SC techniques.
However, the design and implementation of autopoietic systems in the laboratory involves, in addition to practical difficulties, a series of conceptual problems. The reason is that, even at the simplest level, autopoietic systems are characterized by an organizational closure. This theoretical notion indicates that the undergoing chemical processes and transformations need to be linked to each other based on a circular/reticular causality. Moreover, the network of transformations designed to constitute an autopoietic system, in order to be considered cognitive, has to be able to perceive environmental perturbations and to cope with some of them, at least, which means to self-regulate successfully, maintaining its own functional coherence and the underlying reticular organization.
Biological autopoietic systems have structures shaped by evolution, whereby the environment has had a participative, co-constructive role. The structure of an autopoietic system is somehow a map of its own history of structural coupling with the environment, which may include other autopoietic systems. In other words, the system embodies, in its peculiar realization and dynamics, “semantic information” about its world, which it co-created through interaction with its niche (Nauta, 1972; Varela, 1979). Such a system indeed learns to react to recurrent perturbations in its environment by associating them with endogenous patterns of self-regulation, i.e., endogenous operational meanings that define in what ways the system compensates for these alterations and maintains itself in the related perturbative external conditions. For example, a certain environmental event linked to a signaling pathway activates a certain gene, a, and not another gene, b, because for the system, only this specific route serves its intrinsic goal of self-maintenance and not another. This route is the operational meaning that the system associates with the related environmental event, based on its (phylogenetic and ontogenetic) history of coupling with its environment.
Therefore, although the technical issue behind the construction of artificial autopoietic systems refers to the practical possibility of designing and constructing such systems (not discussed here), the theoretical question is subtler and refers to the mechanisms of the generation of meaning for these system. In the case of hypothetical autopoietic–and thus cognitive–SCs, where do their meanings come from? Whether or not SCs are built by using biomacromolecules (to closely mimic biological cells), or by using allegedly primitive molecules (to mimic primitive cells), or by using fully artificial molecules (to produce authentic “artificial” cells), or by employing any sort of hybrid approach, the SC structure is ultimately devised by an experimenter. The experimenter decides, a priori, not only the SC structure (intended as the set of reactions, their topology, and dynamics) but also the environment into which SCs are embedded. Technical difficulties translate into simplified—often oversimplified—versions of the target system, while the definition of a “stiff” SC/environment super-system sacrifices the very important moment of meaning generation, which becomes possible only when plastic behavior is allowed. In this respect, it seems that the design of chemical systems more apt to adaptive behavior, plasticity, and easier endogenous reconfiguration is more promising than the design of systems based on the predictable behavior of complex biomacromolecules3. The latter will be performing more in terms of reproducing a series of cell-like behaviors in a programmable manner but probably only partially appropriate to reproduce cognitive features and the emergence of meaning (the question, however, is open to discussion). The sought scenario is somehow resonant with the scientific work realized on the synthetic modeling of cognition by Gordon Pask, another major contributor to (second-order) cybernetics who investigated rudimentary forms of electrochemical systems with the ability to adaptively construct their own sensors, thereby choosing the relationship between their internal states and the world at large (Pask, 1959; Cariani, 1993). Systems chemistry, a recently developed field of chemistry where these sorts of phenomena find a proper collocation, can ally with SB to provide a frontier platform to address these critical theoretical issues (Ruiz-Mirazo et al., 2014; Ashkenasy et al., 2017; Čejková and Cartwright, 2022).
5 Concluding remarks
The enthusiasm born around SB, and in particular around the construction of SCs, has several roots. In addition to the rapid technical development of the field, driven by an original combination of microcompartment technology and microfluidics, cell-free systems, and numerical modeling, which promise innovative contributions to novel biotechnologies, there are philosophical and epistemological scientific interests. They are motivated by the recent actualization of the possibility—prefigured in the cybernetic era—of studying cognition through the construction and experimental exploration of artificial systems capable of reproducing the phenomenological and organizational aspects of biological systems. Among the several open questions on the potential role of SB and SC in the epistemology of the science(s) of the artificial (for example, see Table 1), here, we have briefly discussed questions related to, on one hand, overcoming the imitation paradigm and the polarization ‘mere imitation/full reproduction’ of the target processes, in the context of the synthetic modeling, and, on the other hand, representing synthetically the complexity of the organization underlying natural life and cognition. Furthermore, we promoted a pluralist approach to the synthetic modeling of life and cognition, which aims at making generative the workspace between underdetermined and overdetermined models of biological and cognitive processes by implementing a variety of theories of biological/cognitive organization, and, with regard to each of them, exploring different ways of synthetic realization, based on the Langton-inspired idea of a synthetic science of life and cognition as they are and they could be. However, in these few pages, we could only offer a schematic overview of these issues, and for their appropriate discussion, we must refer to other works (Table 1). The most relevant message that we intended to convey in this short article emphasizes the importance of bringing to the attention of the community not only the technical issues, but also the theoretical and epistemological issues underlying the involvement of SB and, in particular, of SC research in AI, as this is the only way to fully unfold the potential that they can express in this field.
Data availability statement
The original contributions presented in the study are included in the article/Supplementary Material; further inquiries can be directed to the corresponding authors.
Author contributions
PS and LD identified the theoretical aspects that can be relevant to advancing the synthetic cell research field in the context of synthetic biology, artificial life, and cognitive sciences. LD provided the referenced epistemological discussions on cybernetics, autopoiesis, experimental models of life and cognition. Both authors wrote the article.
Funding
This publication was financed through funding allocated to the project “Regenerative Epistemologies” (PIs: M. Ceruti and LD) by the Department of Communication, Arts, and Media “Giampaolo Fabris” of IULM University (Milan, Italy).
Acknowledgments
A preliminary version of the present discussion was presented as a talk at the Second Workshop on Chemistry and Artificial Life Forms (Chemaliforms II, 19 July 2022), organized by Jitka Čejková (University of Chemistry and Technology Prague, Czech Republic), Richard Löffler (University of Trento, Italy), and Tan Phat Huynh (Åbo Akademi, Finland) as a satellite workshop of the 2022 Conference on Artificial Life (18-22 July 2022). An extensive discussion on the topics presented in Section 3 will appear as a full article in the journal Artificial Life, MIT Press.
Conflict of interest
The authors declare that the research was conducted in the absence of any commercial or financial relationships that could be construed as a potential conflict of interest.
Publisher’s note
All claims expressed in this article are solely those of the authors and do not necessarily represent those of their affiliated organizations, or those of the publisher, the editors, and the reviewers. Any product that may be evaluated in this article, or claim that may be made by its manufacturer, is not guaranteed or endorsed by the publisher.
Footnotes
1As suggested by a reviewer, we would like to spend a few words about the definition of “synthetic (or artificial) cell” and its use in the scientific literature. Currently, this expression is widely used to indicate many types of microcompartments, e.g., coacervates, liposomes, and droplets, generated in the laboratory, which host in their volume or on their surface a rather small set of compounds often borrowed from cellular biochemical pathways (such as the set of macromolecules that carry out the transcription–translation reactions, or the enzymes required to catalyze a series of sequential reactions, or small genetic circuits.). They are very simple and non-living cell-like structures, which can be best intended as rudimentary cytomimetic chemical systems. The resulting structures cannot be really compared to living cells, even when “minimal” living cells (actual or hypothetical) are considered. Studies in comparative genomics have shown, indeed, that the minimal genome still counts, in very permissive environmental conditions, 200–250 genes (Mushegian and Koonin, 1996; Luisi et al., 2002; Gil et al., 2004). Experiments with synthetic genomes have shown that a viable minimal living cell—called JCVI-syn3A—is based on 493 genes, of which 452 code for proteins and 38 for RNAs (Hutchison et al., 2016). It should be noted, moreover, that the research on SCs actually originated in connection with origin-of-life problems, attempting to model early “protocells,” which can be considered pre-cellular structures lying at the interface between life and non-life stages (Oberholzer et al., 1995; Szostak et al., 2001; Luisi et al., 2006). Current SC approaches and SC technology, however, are not always directed to address origin-of-life questions (or theoretical biology concepts such as autopoiesis, e.g., Luisi, 2003) but also target applied science, biotechnology, complex bioassays, and nanomedicine.
2It is worth mentioning that the issue of relevance is not limited to wetware models but also affects software and hardware models. For the three forms of synthetic models, indeed, the relationship they currently have with their target processes, and thus their explanatory power, is still unclear (Damiano and Stano, Artificial Life, in press).
3Here, we refer to those designs whereby complex biomacromolecules (e.g., enzymes) are employed mainly because of their efficiency due to the strong constraints they impose on chemical reactions, allowing the very act of “designing” SCs. Biomacromolecule activities are, however, not completely independent from their environment: in turn, they can be allosterically regulated by third parties (activators and inhibitors, which can be elements of the network as well) so that enzyme-based chemical networks also exhibit—in a certain sense—a variation in their structure (e.g., variation of chemical flows, resulting in a change of the relations of productions of components within the network).
References
Ashkenasy, G., Hermans, T. M., Otto, S., and Taylor, A. F. (2017). Systems chemistry. Chem. Soc. Rev. 46, 2543–2554. doi:10.1039/C7CS00117G
Bich, L., and Damiano, L. (2012). Life, autonomy and cognition: An organizational approach to the definition of the universal properties of life. Orig. Life Evol. Biosph. 42, 389–397. doi:10.1007/s11084-012-9300-7
Cariani, P. (1993). To evolve an ear. Epistemological implications of Gordon Pask’s electrochemical devices. Syst. Res. 10, 19–33. doi:10.1002/sres.3850100305
Čejková, J., and Cartwright, J. H. E. (2022). Chemobrionics and systems chemistry. ChemSystemsChem 4, e202200002. doi:10.1002/syst.202200002
Cronin, L., Krasnogor, N., Davis, B. G., Alexander, C., Robertson, N., Steinke, J. H. G., et al. (2006). The imitation game–a computational chemical approach to recognizing life. Nat. Biotechnol. 24, 1203–1206. doi:10.1038/nbt1006-1203
Damiano, L., and Stano, P. (2021b). “Towards autopoietic SB-AI,” in Proceedings of the Artificial Life Conference 2021 (ALIFE 2021). Editors J. Cejkova, S. Holler, L. Soros, and O. Witkowski (Cambridge, MA, United States: MITPress), 179–181.
Damiano, L., Hiolle, A., and Cañamero, L. (2011). “Grounding synthetic knowledge,” in Advances in artificial life, ECAL 2011. Editors T. Lenaerts, M. Giacobini, H. Bersini, P. Bourgine, M. Dorigo, and R. Doursat (Cambridge, MA, USA: MIT Press), 200–207.
Damiano, L., and Stano, P. (2021a). A wetware embodied AI? Towards an autopoietic organizational approach grounded in synthetic biology. Front. Bioeng. Biotechnol. 9, 724023. doi:10.3389/fbioe.2021.724023
Damiano, L., and Stano, P. (2023). Explorative Synthetic Biology in AI. Criteria of relevance and a taxonomy for synthetic models of living and cognitive processes. Artif. Life, in press
Damiano, L., and Stano, P. (2020). On the “life-likeness” of synthetic cells. Front. Bioeng. Biotechnol. 8, 953. doi:10.3389/fbioe.2020.00953
Damiano, L., and Stano, P. (2018). Synthetic Biology and Artificial Intelligence. Grounding a cross-disciplinary approach to the synthetic exploration of (embodied) cognition. Complex Syst. 27, 199–228. doi:10.25088/ComplexSystems.27.3.199
Gentili, P. L., and Stano, P. (2022). Chemical neural networks inside synthetic cells? A proposal for their realization and modeling. Front. Bioeng. Biotechnol. 10, 927110. doi:10.3389/fbioe.2022.927110
Gentili, P. L., and Stano, P. (2023). Monitoring the advancements in the technology of artificial cells by determining their complexity degree: Hints from complex systems descriptors. Front. Bioeng. Biotechnol. 11, 1132546. doi:10.3389/fbioe.2023.1132546
Gil, R., Silva, F. J., Peretó, J., and Moya, A. (2004). Determination of the core of a minimal bacterial gene set. Microbiol. Mol. Biol. Rev. 68, 518–537. doi:10.1128/MMBR.68.3.518-537.2004
Guindani, C., da Silva, L. C., Cao, S., Ivanov, T., and Landfester, K. (2022). Synthetic cells: From simple bio-inspired modules to sophisticated integrated systems. Angew. Chem. Int. Ed. Engl. 61, e202110855. doi:10.1002/anie.202110855
Hutchison, C. A., Chuang, R.-Y., Noskov, V. N., Assad-Garcia, N., Deerinck, T. J., Ellisman, M. H., et al. (2016). Design and synthesis of a minimal bacterial genome. Science 351, aad6253. doi:10.1126/science.aad6253
Langton, C. G. (1989). “Artificial life,” in Artificial life. Editor C. G. Langton (Boston, MX, USA: Addison-Wesley), 1–47.
Lentini, R., Martín, N. Y., Forlin, M., Belmonte, L., Fontana, J., Cornella, M., et al. (2017). Two-way chemical communication between artificial and natural cells. ACS Central Sci. 3, 117–123. doi:10.1021/acscentsci.6b00330
Luisi, P. L. (2003). Autopoiesis: A review and a reappraisal. Naturwissenschaften 90, 49–59. doi:10.1007/s00114-002-0389-9
Luisi, P. L., Ferri, F., and Stano, P. (2006). Approaches to semi-synthetic minimal cells: A review. Naturwissenschaften 93, 1–13. doi:10.1007/s00114-005-0056-z
Luisi, P. L., Oberholzer, T., and Lazcano, A. (2002). The notion of a dna minimal cell: A general discourse and some guidelines for an experimental approach. Helv. Chim. Acta 85, 1759–1777. doi:10.1002/1522-2675(200206)85:6<1759::AID-HLCA1759>3.0
Luisi, P. L. (2002). Toward the engineering of minimal living cells. Anat. Rec. 268, 208–214. doi:10.1002/ar.10155
Magarini, M., and Stano, P. (2021). Synthetic cells engaged in molecular communication: An opportunity for modelling shannon- and semantic-information in the chemical domain. Front. Commun. Netw. 2, 48. doi:10.3389/frcmn.2021.724597
Maturana, H. (1969). “Biology of cognition,” BCL Research Report, 9. Illinois, United States: Biological Computer Laboratory, Department of Electrical Engineering, University of Illinois.
Maturana, H. R., and Varela, F. J. (1973). De Máquinas y Seres Vivos: Una Teoría de la Organizacíon Biológica. Santiago: Editorial Universitaria.
McCulloch, W. S., and Pitts, W. (1943). A logical calculus of the ideas immanent in nervous activity. Bull. Math. Biophysics 5, 115–133. doi:10.1007/BF02478259
Mushegian, A. R., and Koonin, E. V. (1996). A minimal gene set for cellular life derived by comparison of complete bacterial genomes. Proc. Natl. Acad. Sci. U. S. A. 93, 10268–10273. doi:10.1073/pnas.93.19.10268
Oberholzer, T., Wick, R., Luisi, P. L., and Biebricher, C. K. (1995). Enzymatic RNA replication in self-reproducing vesicles: An approach to a minimal cell. Biochem. Biophys. Res. Commun. 207, 250–257. doi:10.1006/bbrc.1995.1180
Pask, G. (1959). “Physical analogues to the growth of a concept,” in Mechanisation of Thought Processes: Proceedings of a Symposium Held at the National Physical Laboratory, Teddington, Middlesex, November 1958. Editor A. Uttley (London, UK), 877–928.
Rosenblueth, A., Wiener, N., and Bigelow, J. (1943). Behavior, purpose and teleology. Philosophy Sci. 10, 18–24. doi:10.1086/286788
Ruiz-Mirazo, K., Briones, C., and de la Escosura, A. (2014). Prebiotic systems chemistry: New perspectives for the origins of life. Chem. Rev. 114, 285–366. doi:10.1021/cr2004844
Ruzzante, B., Del Moro, L., Magarini, M., and Stano, P. (2023). Synthetic cells extract semantic information from their environment. IEEE Trans. Mol. Biol. Multi-Scale Commun. 1, 1. doi:10.1109/TMBMC.2023.3244399
Salehi-Reyhani, A., Ces, O., and Elani, Y. (2017). Artificial cell mimics as simplified models for the study of cell biology. Exp. Biol. Med. (Maywood) 242, 1309–1317. doi:10.1177/1535370217711441
Schwille, P., Spatz, J., Landfester, K., Bodenschatz, E., Herminghaus, S., Sourjik, V., et al. (2018). MaxSynBio: Avenues towards creating cells from the bottom up. Angew. Chem. Int. Ed. Engl. 57, 13382–13392. doi:10.1002/anie.201802288
Stano, P. (2022a). “Chemical neural networks and synthetic cell biotechnology: Preludes to chemical AI,” in Computational intelligence methods for bioinformatics and biostatistics. Lecture notes in computer science. Editors D. Chicco, A. Facchiano, E. Tavazzi, E. Longato, M. Vettoretti, A. Bernasconiet al. (Berlin Germany: Springer International Publishing), 1–12. doi:10.1007/978-3-031-20837-9_1
Stano, P. (2022b). Exploring information and communication theories for synthetic cell research. Front. Bioeng. Biotechnol. 10, 927156. doi:10.3389/fbioe.2022.927156
Stano, P., Rampioni, G., Carrara, P., Damiano, L., Leoni, L., and Luisi, P. L. (2012). Semi-synthetic minimal cells as a tool for biochemical ICT. BioSystems 109, 24–34. doi:10.1016/j.biosystems.2012.01.002
Stano, P., Rampioni, G., Roli, A., Gentili, P. L., and Damiano, L. (2022). “En route for implanting a minimal chemical perceptron into artificial cells,” in Proceedings of the ALIFE 2022: The 2022 Conference on Artificial Life. Editors R. Löffler, and S. Bartlett (Cambridge, MA, United States: MIT Press), 465–467.
Staufer, O., De Lora, J. A., Bailoni, E., Bazrafshan, A., Benk, A. S., Jahnke, K., et al. (2021). Building a community to engineer synthetic cells and organelles from the bottom-up. Elife 10, e73556. doi:10.7554/eLife.73556
Szostak, J. W., Bartel, D. P., and Luisi, P. L. (2001). Synthesizing life. Nature 409, 387–390. doi:10.1038/35053176
Turing, A. M. (1950). I. Computing machinery and intelligence. Mind LIX, 433–460. doi:10.1093/mind/LIX.236.433
Keywords: autopoiesis, artificial or synthetic models, cognition, cybernetics, epistemology of the sciences of the artificial, philosophy of science, synthetic biology, synthetic cells
Citation: Stano P and Damiano L (2023) Synthetic cell research: Is technical progress leaving theoretical and epistemological investigations one step behind?. Front. Robot. AI 10:1143196. doi: 10.3389/frobt.2023.1143196
Received: 12 January 2023; Accepted: 06 March 2023;
Published: 23 March 2023.
Edited by:
Konrad Szaciłowski, AGH University of Science and Technology, PolandReviewed by:
Pier Luigi Luisi, Department of Materials, ETH Zurich, SwitzerlandCopyright © 2023 Stano and Damiano. This is an open-access article distributed under the terms of the Creative Commons Attribution License (CC BY). The use, distribution or reproduction in other forums is permitted, provided the original author(s) and the copyright owner(s) are credited and that the original publication in this journal is cited, in accordance with accepted academic practice. No use, distribution or reproduction is permitted which does not comply with these terms.
*Correspondence: Pasquale Stano, cGFzcXVhbGUuc3Rhbm9AdW5pc2FsZW50by5pdA== Luisa Damiano, bHVpc2EuZGFtaWFub0BpdWxtLml0