- 1Chemistry Department, Tshwane University of Technology, Pretoria, South Africa
- 2Department of Crop Sciences, Tshwane University of Technology, Pretoria, South Africa
Cowpea is the most important seed legume in Africa. Its leaves and seed are consumed to meet the dietary requirements of protein and micronutrient in rural African communities. In this study, leaf protein of 32 cowpea genotypes was 23–40% at Taung (South Africa), 28–40% at Wa and 24–35% at Manga (Ghana). Seed protein level was also up to 40% in landrace Bengpla and more than 30% in nine other genotypes planted at Taung. Trace elements in cowpea leaves showed markedly high concentrations of Fe (2,011 μg.g−1), Zn (150 μg.g−1), Mn (325 μg.g−1), and B (43 μg.g−1) in genotype Apagbaala, in contrast to the very low levels of Fe (273 μg.g−1), Zn (40 μg.g−1), Mn (219 μg.g−1), and B (32 μg.g−1) in genotype Encore. Leaf Fe concentration was highest in genotype Apagbaala (2,011 μg.g−1), followed by Fahari (2,004 μg.g−1), Iron Gray (1,302 μg.g−1), Line 2020 (944 μg.g−1), Bensogla (927 μg.g−1), Omondaw (605 μg.g−1), IT96D-1951 (591 μg.g−1), IT93K-452-1 (574 μg.g−1), Ngonji (569 μg.g−1), and Mchanganyika (566 μg.g−1), and lowest in Bechuana white (268 μg.g−1). Cowpea seed also showed greater concentrations of Fe in genotype Soronko (67 μg.g−1), IT93K-452-1 (67 μg.g−1), Brown Eye (65 μg.g−1), Bensogla (61 μg.g−1), and TVU11424 (62 μg.g−1). Trace elements in cowpea seed differed among genotypes, and ranged from 45.1 to 67.0 μg.g−1 for Fe, 33.9 to 69.2 μg.g−1 for Zn, 10.1 to 17.4 μg.g−1 for Mn, 14.7 to 21.4 μg.g−1 for B, and 5.2 to 8.1 μg.g−1 for Cu. Genotypes Apagbaala, Fahari, Iron Gray, and Line 2020, respectively, exhibited 34.2-, 34.0-, 22.5-, and 18.3-fold higher Fe concentration in leaves than seed, and 3.5-, 2.0-, 2.0-, and 3.5-fold greater Zn in leaves than seed (in that order). The genotypes that accumulated significantly high levels of protein and trace elements in cowpea leaves and seed, were generally high N2-fixers, thus suggesting a link between N2 fixation and cowpea's ability to synthesize protein and accumulate nutrient elements in leaves and seed. Therefore, identifying cowpea genotypes that can enhance protein accumulation and micronutrient density in edible leaves and seed through breeding has the potential to overcome protein-calorie malnutrition and trace element deficiency in rural Africa.
Introduction
Food and nutritional insecurity remain a major problem facing Africa, as about 239 million people are suffering from protein-calorie malnutrition (Fanzo, 2012), and another 232 million from micronutrient deficiency (Andrea and Rose, 2015). In Africa, hunger is the result of food insecurity due to low crop yields stemming from soil moisture deficit from low rainfall, farmer use of nutrient-poor soils for agriculture and unimproved crop varieties, as well as the effects of biotic stress such as insect pests and diseases (Dakora and Keya, 1997). Although N fertilizers can be used to overcome soil infertility and increase crop yields, they are expensive and inaccessible in Africa. On average, only about 8.8 kg NPK fertilizer is applied per hectare by smallholder farmers in Africa (Henao and Baanante, 2006).
Due to low nutritious food production, protein-calorie malnutrition is highly prevalent in African children, and is the outcome of low protein and calorie intake. Although the consumption of meat, dairy products and seafood can overcome protein-calorie malnutrition, these foods are expensive to resource-poor households in rural Africa. So the use of protein-rich plant foods has been the main option for many poor African communities. Leafy vegetables, for example, are a good source of dietary protein (Aletor et al., 2002), however, nodulated legumes are even better due to their ability to fix N2 when in symbiosis with soil bacteria termed “rhizobia.” Here, N2-fixing bacteroids in root nodules are able to reduce atmospheric N2 to NH3, which is incorporated into amino acids and protein, and stored in leaves and seeds. This explains why the edible leaves and seed of legumes (or pulses) are a very high source of dietary protein. Of the cultivated legumes used as food, seed protein is as high as 40% in soybean (Zarkadas et al., 2007), 33% in cowpea (Ddamulira and Santos, 2015), 20–25% in common bean (Broμghton et al., 2003), 20.6% in Bambara groundnut (Mazahib et al., 2013), 21.3% in Kersting's bean (Ayenan and Ezin, 2016), 27–29% in pigeonpea (Saxena et al., 1987), 21–31% in mungbean (Yi-shen et al., 2018), 21.8–25.8% in chickpea (Xu et al., 2016) and 20–30% in groundnut (Toomer, 2018). Additionally, cowpea also contain 34.9% of protein in edible leaves (Enyiukwu et al., 2018).
In addition to protein, the edible leaves and seeds of legumes also contain high levels of dietarily-important mineral nutrients, which are needed for human nutrition and health, especially for overcoming trace element deficiency and promoting brain development. For example, mineral concentrations are also reported to be 142–626 and 60–99 mg.kg−1 for Fe, 49–104 and 44–65 mg.kg−1 Zn, 196–394 and 5–32 mg.kg−1 Mn, 8.6–19.7 and 8.3–14.7 mg.kg−1 Cu and 42–55 and 10–22 mg.kg−1 B in cowpea leaves and seeds, respectively (Belane and Dakora, 2011a). Other studies have reported 22.6 mg.kg−1 Fe, 33.1 mg.kg−1 Zn, 6.7 mg.kg−1 Mn, and 7.5 mg.kg−1 Cu for groundnut seed (Toomer, 2018), as well as 500.0 mg.kg−1 Fe, 405.0 mg.kg−1 Zn, 480.0 mg.kg−1 Mn, and 85.0 mg.kg−1 Cu for chickpea seed (Xu et al., 2016).
Given the inherently low infertility of African soils, as well as the high cost of chemical fertilizers and their polluting effect on the environment, there is a need to develop sustainably green and affordable technologies for increasing the nutritional quality of food legumes for use by resource-poor, smallholder farmers in Africa. The aim of this study was to assess protein level and trace element density in edible leaves and seed of 30–32 cowpea genotypes grown in the field at Wa and Manga in Ghana, and at Taung in South Africa.
Materials and Methods
Site Description
Field trials were conducted in Ghana and South Africa in 2005 and 2006. In Ghana, these field experiments were carried out at Dokpong and Bamahu near Wa in the Upper West Region, and at Manga in the Upper East Region, in 2005 and 2006, while in South Africa, these trials were conducted at Taung. Details of the experimental environments in the countries (altitude, longitude, mean annual rainfall, soil characteristics, cropping history, etc.) have been described elsewhere (Belane and Dakora, 2009, 2010, 2011b; Belane et al., 2011).
Origin of Cowpea Genotypes
The cowpea genotypes used for this study were collected from Ghana, Tanzania, South Africa, and the International Institute of Tropical Agriculture (IITA) in Nigeria, as indicated by Belane and Dakora (2010). The 30 genotypes exhibited different useful biological traits ranging from number of days to 50% flowering and number of days to physiological harvest, to levels of N2 fixation, pest resistance, and seed yield (Belane and Dakora, 2010).
Field Design, Planting, and Pest Management
The experimental design used in this study has been described elsewhere (Belane and Dakora, 2009, 2010, 2011b; Belane et al., 2011), and involved the use of a randomized complete block design with four replicate plots per cowpea genotype in all the experiments. Each plot measured 3 m × 5 m (i.e., 15 m2). The experiments were planted in mid-July each year, with a row-to-row spacing of 60 and 20 cm within-row. Weeds were controlled with a hoe. Two low-dose sprays of lambda cyhalothrin (Karate 2.5 EC) insecticide were applied at flowering and at pod formation to control pests.
Plant Harvest and Processing
Healthy young trifoliate leaves were harvested from 12 plants per plot at 46 and 72 DAP in 2005 and 2006 to assess for any changes in mineral density close to physiological maturity. The leaf samples were oven-dried (60°C), weighed, and ground to fine powder (0.85 mm) for mineral analysis. At physiological maturity, cowpea seeds were harvested and similarly processed for analysis of nutrient elements.
Protein Analysis in Cowpea Leaves and Seed
The percent N in cowpea leaves and seeds was determined using mass spectrometry, as described by Belane and Dakora (2010). The protein in leaves and seeds was estimated as %N of organ ×6.25 (Jones, 1941; Mariotti et al., 2008).
Determination of Micronutrients in Cowpea Leaves and Seeds
Trace elements such as Fe, Zn, Cu, Mn, and B in cowpea leaves and seeds were measured, as described by Belane and Dakora (2011a). Briefly, 1 g of ground cowpea leaf or seed sample was ashed in a porcelain crucible at 500°C overnight, the ash was dissolved in 5 ml of 6 M HCl (analytical grade) and placed in an oven at 50°C for 30 min, after which 35 ml of de-ionized water was added. The mixture was filtered through Whatman No. 1 filter paper, and mineral concentrations determined in leaf and seed extracts from four replicate samples using inductively coupled plasma mass spectrometry (IRIS/AP HR DUO Thermo Electron Corporation, Franklin, Massachusetts, USA) (Ataro et al., 2008).
Correlation Analysis
Correlation analyses were performed for the levels of micronutrients in leaves and seeds of cowpea genotypes to ascertain any relationships that may exist in the translocation of trace elements between the two organs.
Statistical Analysis
The data on protein and micronutrient levels in cowpea leaves and seed were subjected to analysis of variance (ANOVA) using a STATISTICA analytical software program version 7.1. A one-way ANOVA was used to compare protein and micronutrient levels among genotypes. Where significant differences were found, the Duncan's multiple range test was used to separate treatment means at p ≤ 0.05 or p ≤ 0.001.
Results
Leaf Protein Levels of Cowpea Genotypes
The leaf protein of cowpea genotypes used in this study varied markedly between and among genotypes irrespective of location. The leaf protein of 30 cowpea genotypes grown at Wa in the Guinea savanna of the Upper West Region in Ghana also differed significantly, and ranged from about 28% for genotype ITH98-46 to 40% for Soronko (Figure 2). Of the 30 cowpea genotypes tested at Wa, 29 recorded more than 30% protein in their leaves (Figure 2).
Leaf protein was also assessed for 30 cowpea genotypes planted at Manga in the Sudano-Sahelian savanna in the Upper East Region of Ghana. Leaf protein levels also differed among the cowpea genotypes at Manga, and were found to vary from 24 to 35% (Figure 3). Some 12 out of the 30 genotypes studied recorded more than 30% protein in their leaves at Manga, Ghana.
Seed Protein of Cowpea Genotypes
The concentration of protein in cowpea seed was determined for only the 32 genotypes planted at the Taung site in South Africa. The data revealed marked differences in seed protein, which ranged from about 20% in Soronko to 40% in Bengpla (Figure 4). Ten cowpea genotypes, including Bengpla, recorded more than 30% protein in their seed when planted at Taung in South Africa (Figure 4).
Micronutrient Density in Cowpea Leaves
The levels of micronutrients in edible leaves of cowpea genotypes were assessed using ICP-MS analysis for only the 32 cowpea genotypes planted at Taung (Table 1), but not Wa or Manga in Ghana. The concentration of micronutrients in the leaves varied hugely between and among genotypes. As show in Table 1, the level of Fe in cowpea leaves ranged from 268 μg.g−1 in Bechuana white to 2,011 μg.g−1 in Apagbaala landrace. Other genotypes with markedly high leaf Fe levels included Fahari (2,005 μg.g−1), Iron Gray (1,302 μg.g−1), Line 2020 (945 μg.g−1), and Bensogla (927 μg.g−1). In contrast, the genotypes which showed the lowest leaf Fe concentrations were Bechuana white (268 μg.g−1), Encore (273 μg.g−1), IT94D-437-1 (314 μg.g−1), and TVU11424 (313 μg.g−1).
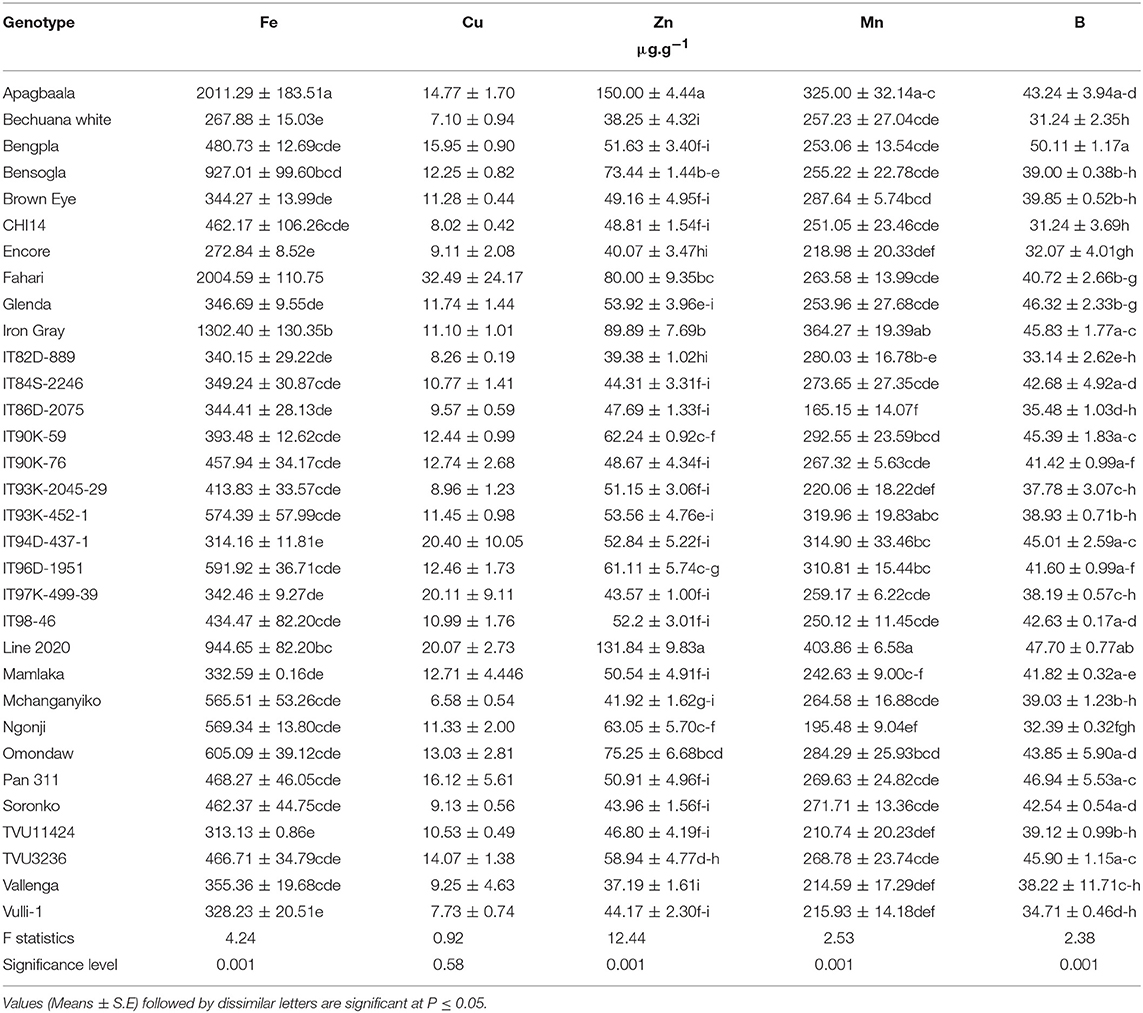
Table 1. Micronutrients in edible leaves of field-grown cowpea varieties harvested at 76 DAP at Taung, South Africa in 2005.
The distribution of Zn in cowpea leaves also differed markedly, and ranged from 37 μg.g−1) in Vallenga to 150 μg.g−1 for Apagbaala (which also recorded the highest Fe concentration; Table 1). Other cowpea genotypes with high levels of Zn in leaves included Line 2020 (132 μg.g−1), Iron Gray (90 μg.g−1), Fahari (80 μg.g−1), and Bensogla (73 μg.g−1), which incidentally also recorded high Fe concentrations in cowpea leaves. However, the genotypes with the least Zn concentration in leaves included Vallenga (37 μg.g−1), Bechuana white (38 μg.g−1), IT82D-889 (39 μg.g−1), and Encore (40 μg.g−1).
The density of Mn in edible cowpea leaves similarly differed among the genotypes, and ranged from 165 μg.g−1 in IT86D-2075 to 404 μg.g−1 in Line 2020 (Table 2). Other genotypes with increased Mn in leaves included Iron Gray (364 μg.g−1) and Apagbaala (325 μg.g−1). Leaf concentration of B also differed with cowpea genotype, with levels ranging from 31 μg.g−1 in Bechuana white to 50 μg.g−1 in Benpla (Table 2). The concentration of Cu in cowpea leaves was similar for all 32 genotypes (Table 2).
Micronutrient Density in Cowpea Seed
The concentrations of trace elements (Fe, Zn, CU, Mn, and B) in cowpea seed were generally lower relative to leaves. As shown in Table 2, Fe levels in seed differed among the genotypes tested, and ranged from 45 μg.g−1 for Bengpla to 67 μg.g−1 in Soronko and IT95K-452-1. Other genotypes with high levels of Fe in seed included Brown Eye (65 μg.g−1), IT98-46 (64 μg.g−1), TVU11424 (62 μg.g−1), IT86D-2075 (62 μg.g−1), and Bensogla (61 μg.g−1). In contrast, the genotypes with low levels of Fe in seed were Bengpla (45 μg.g−1), followed by Mamlaka (50 μg.g−1).
A shown in Table 2, the Cu levels in cowpea seed varied from 5.20 μg.g−1 in genotype IT82D-889 to 8.11 μg.g−1 for genotype IT96D-1951 and 8.06 μg.g−1 for Vallenga (Table 2). Other genotypes with increased levels of Cu in cowpea seed included Bensogla (7.90 μg.g−1), Iron Gray (7.86 μg.g−1), Brown Eye (7.76 μg.g−1), and Pan 311 (7.49). Similarly, Zn concentration in cowpea seed was different for the 32 genotypes tested (Table 3). Genotype TVU11424 recorded the highest levels of Zn (69.15 μg.g−1), followed by Soronko (53.88 μg.g−1), and IT90K-59 (49.78 μg.g−1). In contrast, the lowest Zn concentration was found in Bengpla (33.89 μg.g−1), followed by Mamlaka (34.64 μg.g−1), and Line 2020 (37.86 μg.g−1).
The Mn distribution in cowpea seed ranged from 10.05 μg.g−1 in Bechuana white to 17.43 μg.g−1 in CH14 (Table 2). The highest Mn concentrations in cowpea seed were recorded by genotypes CH14 (17.43 μg.g−1), Iron Gray (17.06 μg.g−1), Bechuana white (16.85 μg.g−1), Fahari (16.46 μg.g−1), and IT86D-2075 (16.21 μg.g−1). By contrast, the lowest Mn levels were produced by Bechuana white (10.05 μg.g−1) and IT82D-889 (10.09 μg.g−1). The B levels in cowpea seed also differed among genotypes, and varied from 14.71 μg.g−1 for IT82D-889 to Brown Eye (21.44 μg.g−1). The highest concentration of B was found in Brown Eye (21.44 μg.g−1), followed by IT94D-437-1 (21.30 μg.g−1), Encore (19.81 μg.g−1), IT90K-59 (19.20 μg.g−1), Bechuana white (19.11 μg.g−1), and IT93K-2045-29 (19.00 μg.g−1). However, the lowest B levels were recorded by IT82D-889 (14.71 μg.g−1), followed by Bensogla (15.57 μg.g−1) and Bengpla (15.71 μg.g−1).
Correlation Analysis of Micronutrients in Cowpea Leaves and Seeds
Leaf Fe was positively correlated with seed Fe, leaf Zn, leaf Mn, and seed Mn (Table 4). Seed Fe was also correlated positively with seed Zn and leaf B, but negatively with seed Cu and seed B. Seed Zn correlated with positively leaf Cu, seed Cu and seed B, but negatively with leaf Mn. Similarly, leaf Mn correlated significantly with seed Mn but negatively with seed Cu, leaf B and seed B, and seed Cu correlated with seed B (Table 4).
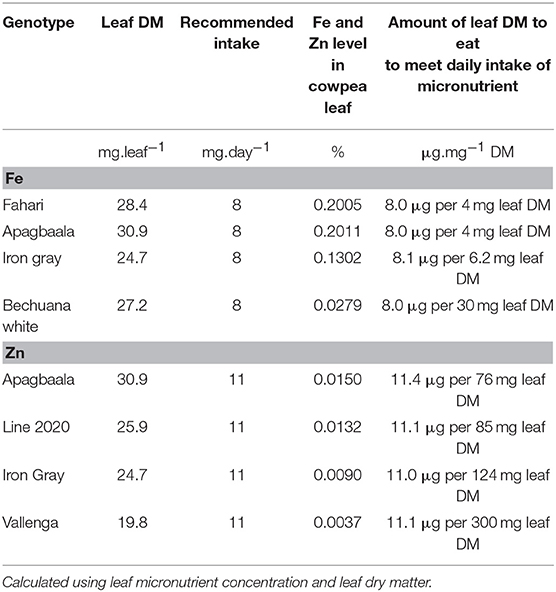
Table 4. Amount of edible cowpea leaf to consume to meet the recommended daily dietary intake of Fe and Zn.
Discussion
Leaf and Seed Protein of Cowpea Genotypes
Food and nutritional insecurity remain a major problem facing Sub-Saharan Africa, as about 239 million people are currently suffering from protein-calorie malnutrition (Fanzo, 2012; Andrea and Rose, 2015). In rural Africa, food/nutritional security and micronutrient deficiency are met through the consumption of leafy vegetables and seed legumes (Belane and Dakora, 2011a), as animal protein is too expensive for resource-poor households. In Sub-Saharan Africa, cowpea is the major food grain legume, cultivated and consumed by the majority of smallholder farming communities and is very important as a food crop in meeting dietary protein requirements, and overcoming micronutrient deficiency.
In this study, we evaluated 32 field-grown cowpea genotypes at Taung in South Africa, and 30 each at Wa and Manga in Ghana for leaf and seed protein, as well as for micronutrient density in the two organs at Taung. The results revealed marked differences in the levels of protein in cowpea leaves independent of location (Figures 1–4), as well as of seed protein and micronutrient density in plant parts at Taung (Tables 1, 2). The leaf protein of 32 cowpea genotypes grown at Taung (South Africa) ranged from 23% for genotype IT96D-1951 to 40% for Bengpla, with nine genotypes recording more than 30% leaf protein (Figure 1). At Wa in the Guinea savanna of Ghana, cowpea leaf protein ranged from 28 to 40% for ITH98-46 and Soronko, respectively, with 29 genotypes accumulating more than 30% protein in their leaves (Figure 2). Similarly, at Manga in the Sudano-Sahelian savanna of Ghana, leaf protein levels varied from 24 to 35%, with 12 out of 30 genotypes recording more than 30% protein in their leaves (Figure 3). The leaves of N2-fixing legumes such as cowpea are very rich in N due to the species ability to reduce N2 into NH3 and subsequently into nitrogenous solutes for plant use (Belane et al., 2014). In plants, N is required for the synthesis of macromolecules such as chlorophyll needed for harvesting light photon energy during photosynthesis and formation of the enzyme ribulose-1,5-bisphosphate carboxylase-oxygenase (Rubisco), which reduces CO2 during photosynthesis. Because Rubisco accounts for over 90% of leaf N (Belane and Dakora, 2015), most of the protein found in green leaves of monocots and dicots consists of Rubisco. Thus, a culturable form of this protein could be a biotech spin-off for enhanced nutritional security.
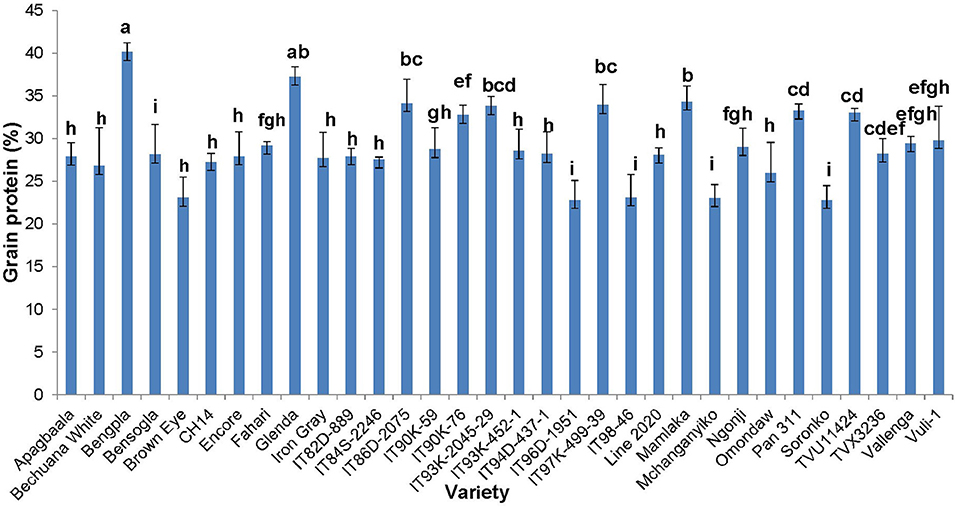
Figure 4. Protein levels in edible seed of 32 cowpea genotypes grown in the field at Taung, South African (dotted line in red denotes 30% or more protein).
Estimates of N2 fixation by cowpea plants sampled from the same field experiments as the materials used in this study showed 43 to 93% N derived from atmospheric N2 fixation at Taung (Belane et al., 2011), 8 to 60% at Manga (Belane and Dakora, 2009) and 64 to 87% at Wa (Belane and Dakora, 2010). Cowpea from farmers' fields could also derive about 30 to 99% of their N nutrition from symbiotic fixation at Wa in the Upper West Region of Ghana (Naab et al., 2009). Similar results from Botswana have shown that field-grown cowpea plants obtained between 19 and 92% of their N nutrition from symbiosis (Pule-Meulenberg et al., 2010). Clearly, the levels of N2 fixation reported in those studies (Belane and Dakora, 2009, 2010, 2011b; Naab et al., 2009) can help to explain the strong variation in protein concentration found in the edible leaves and seed of the cowpea material used in this study.
At Taung in South Africa, cowpea genotypes Fahari, Glenda, IT93K-2045-29, Mamlaka, Pan311, and TVu11424 were among those with the highest amounts of N-fixed (Belane et al., 2011). Coincidentally, however, the same genotypes also revealed more protein in cowpea seed in this study (Figure 4), clearly indicating a direct link between protein concentration in cowpea seed and cowpea symbiotic efficiency, as well as the levels of N-fixed. In the same manner, N2 fixation and photosynthesis are metabolically interlinked at the level of the plant's N and C economy, especially where N2 reduced to NH3 by the enzyme nitrogenase is incorporated with de novo photosynthate into amino acids, needed for protein biosynthesis. In this study, the relationship was however not so clear between percent N derived from fixation and protein levels in cowpea leaves, as found for the seeds. For example, cowpea genotypes Ngonji (56%), Mamlaka (51%) IT90K-59 (48%), IT93K-452-1 (46%), IT90K-59 (48%), and Mchanganyiko (44%), which derived relatively higher N from fixation at Manga in Ghana (Belane and Dakora, 2009), also produced significantly much greater leaf protein in this study (Figure 3). In contrast, Bechuana white, which obtained the highest N from symbiosis (60%) at Manga, was the fourth lowest in leaf protein production, while Fahari, which derived only 25% of its N from fixation, produced the highest leaf protein (Figure 3). The observed anomaly in the relationship between percent N derived from fixation and leaf protein concentration in cowpea genotypes appears to depend on the traffic and pathways of symbiotic N transported to leaves from N2-fixing bacteroids in root nodules, and the subsequent incorporation of fixed-N into protein. The variation in seed protein found among the cowpea genotypes tested in this study is consistent with a recent report by Gerrano et al. (2019).
Trace Element Density in Cowpea Leaves and Seeds
In Africa, about 232 people are suffering from trace element deficiency (Andrea and Rose, 2015), a problem that can be addressed through studies of nodulated legumes that have the ability to accumulate micronutrients in organs. In this study, there were marked differences in the uptake and accumulation of the micronutrients Fe, Zn, Cu, Mn B in leaves of the 32 cowpea genotypes grown in the field at Taung in South Africa (Table 1). Of the five trace elements (Fe, Zn, Cu, Mn, and B) that were measured in cowpea leaves, Fe concentration was highest in genotype Apagbaala (2,011 μg.g−1), followed by Fahari (2,004 μg.g−1), Iron Gray (1,302 μg.g−1), Line 2020 (944 μg.g−1), Bensogla (927 μg.g−1), Omondaw (605 μg.g−1), IT96D-1951(591 μg.g−1), IT93K-452-1 (574 μg.g−1), Ngonji (569 μg.g−1), and Mchanganyika (566 μg.g−1), and lowest in Bechuana white (268 μg.g−1). In that order, the 10 cowpea genotypes were 7.51-, 7.48-, 4.86-, 3.52-, 3.46-, 2.26-, 2.21-, 2.14-, 2.12-, and 2.11-fold greater in leaf Fe concentration than Bechuana white, the lowest Fe accumulation (Table 2).
However, the leaf concentrations of Zn, Mn and B in cowpea were much lower than that of Fe, with Zn distribution ranging from 37.2 μg.g−1 for Vallenga to 150 μg.g−1 for Apagbaala (Table 1). Nine out of the 32 cowpea genotypes studied showed a very high concentration of Zn in edible leaves. These included Apagbaala (150.0 μg.g−1), Line 2020 (131.8 μg.g−1), Iron Gray (89.9 μg.g−1), Fahari (80.0 μg.g−1), Omondaw (75.3 μg.g−1), Bensogla (73.4 μg.g−1), Ngonji (63.1 μg.g−1), IT90K-59 (62.2 μg.g−1), and TVU3236 (58.9 μg.g−1), which (in that order) were 4.0-, 3.5-, 2.4-, 2.2-, 2.0-, 2.0-, 1.7-, 1.7-, and 1.6-fold higher in leaf Zn concentration than Vallenga, the genotype with the lowest Zn accumulation. Similarly, leaf Mn concentration was markedly greater in Line 2020 (403 μg.g−1), followed by Iron Gray (365 μg.g−1), Apagbaala (325 μg.g−1), IT93K-421-1(320 μg.g−1), IT94D-437-1 (315 μg.g−1), and IT96D-1951 (311 μg.g−1); and these were, respectively, 2.5-, 2.2-, 2.0-, 1.9-, 1.9-, and 1.9-fold higher than genotype IT86D-2075, which recorded the least Mn in leaves (165 μg.g−1). Boron concentration in leaves was also much greater in Bengpla (50.1 μg.g−1), followed by Line 2020 (47.7 μg.g−1), Pan 311 (46.9), Glenda (46.3 μg.g−1), TVU3236 (45.9 μg.g−1), Iron Gray (45.8 μg.g−1), IT90K-59 (45.4 μg.g−1), IT94D-437-1 (45.0 μg.g−1), Omondaw (43.9 μg.g−1), and Apagbaala (43.2 μg.g−1), and lowest in Bechuana white (31.2 μg.g−1). As a result, these genotypes were, respectively, 1.60-, 1.53-, 1.50-, 1.48-, 1.47-, 1.47-, 1.45-, 1.44-, 1.40-, and 1.38-fold higher in B than Bechuana white.
Taken together, the results of this study have shown that the concentrations of Fe and Zn (the two most important trace elements) were highest in the leaves of genotypes Apagbaala, Fahari, Iron gray, Line 2020, Bensogla and Omondaw. Furthermore, Fe, but not Zn, was also higher in the leaves of genotypes IT96D-1951 and IT93K-452-1, while conversely Zn, but not Fe, showed increased distribution in Ngonji, IT90K-59 and TVU3236. Interestingly, the leaves of cowpea genotypes Apagbaala, Line 2020, Iron Gray, IT94D-437-1, and Omondaw were similarly very rich in Mn and B, as found for Fe and Zn. These results agree with the findings of Belane and Dakora (2012), and suggest that, with little effort, breeders can easily identify cowpea genotypes with the ability to accumulate high levels of the micronutrients Fe, Zn, Mn, and B in edible leaves for use by farmers to overcome trace element deficiency in Africa.
In this study, the distribution of micronutrients in cowpea seed also differed markedly among the genotypes studied, with a range of 45.1 to 67.0 μg.g−1 for Fe, 33.9 to 69.2 μg.g−1 for Zn, 10.1 to 17.4 μg.g−1 for Mn, 14.7 to 21.4 μg.g−1 for B, and 5.2 to 8.1 μg.g−1 for Cu (Table 2). The strong variation in cowpea micronutrient distribution found in this study is consistent with a report by Gerrano et al. (2019). Furthermore, we found that the cowpea genotypes with higher micronutrient accumulation, recorded much greater concentrations in leaves than seed. In fact, genotypes Apagbaala, Fahari, Iron Gray, and Line 2020, which showed an ability to increase micronutrient density, respectively, exhibited 34.2-, 34.0-, 22.5-, and 18.3-fold higher Fe concentration in leaves than seed, just as the same genotypes (in that order) revealed 3.5-, 2.0-, 2.0-, and 3.5-fold greater Zn distribution in leaves than seed. These results are consistent with the findings of an earlier study which showed that trace element concentration was much greater in cowpea leaves than seed (Belane and Dakora, 2011a). Our data also suggest that the assimilation and translocation of mineral nutrients from leaves to developing ovules to form seed differed among the cowpea genotypes probably as a result of traffic barriers to solute transport. Whatever the case, the observed differences in leaf micronutrient density seems to suggest that, depending on the cowpea genotype, a greater or lesser amount of leaf material must be consumed in order to meet the recommended daily intake of trace elements such as Fe and Zn (Table 3). Thus, a higher concentration of the micronutrients in cowpea leaves generally led to a lower amount (on dry matter basis) of the leaf material needed for consumption in order to meet the daily dietary intake of each trace element, and vice versa. Another factor that seems to define the level of mineral accumulation in nodulated legumes is the symbiotic efficiency of N2-fixing bacteria in root nodules. It has been shown that high N2-fixing legumes generally tend to accumulate more nutrient elements in shoots than their low-fixing counterparts (Belane et al., 2014). In this study, genotypes such as Apagbaala, Fahari, Iron Gray, Line 2020, Bengsogla and Omondaw, which accumulated significantly high concentrations of trace elements in leaves and seed, were earlier reported to be high N2-fixers, and to accumulate large amounts of symbiotic N in their biomass (Belane and Dakora, 2009, 2010).
Correlation analysis revealed some physiological relationships between micronutrients in leaves and seeds (Table 3). During organ development, ovules are generally regarded as sinks for nutrients stored in leaves as sources. The significant correlation (Table 3) between leaf Fe and seed Fe (r = 0.41***), or leaf Mn and seed Mn (r = 0.37***) attest to this source/sink relationship between leaves and seeds (developed ovules) when it comes to nutrient uploading in the phloem and its translocation to ovules that are developing into seeds. It however seems there was co-transport of Zn and Mn from xylem to leaves, just as there was synergy in the translocation of Fe and Mn to seeds. This was evidenced by the positive correlation between leaf Fe and leaf Zn (r = 0.55***), leaf Fe and leaf Mn (r = 0.25*), and/or leaf Mn and seed Mn (r = 0.37***). Conversely, seed Fe was negatively correlated with seed Cu and seed B, and leaf Mn with seed Cu, seed B, and leaf B. This inverse relationship implies that when seed Fe was increasing, seed Cu and B were decreasing; just as when leaf Mn was accumulating, seed Cu, seed B, and leaf B were decreasing (Table 3). However, whether these synergies and/or antagonisms can be usefully exploited during cowpea breeding, remains to be seen.
The recommended daily dietary intake of the micronutrients Fe and Zn is 8 and 11 mg.day−1, respectively (Ross et al., 2011). Assuming cowpea leaves to be the sole source of dietary Fe and Zn (on dry weight basis), the estimated amount to consume in order to meet the daily intake of 8 and 11 mg.day−1 for Fe and Zn was found to vary with cowpea genotype (Table 4). Smaller leaf material of the genotypes with higher leaf concentration of Fe and Zn was needed to meet the daily intake relative to their counterparts with low levels of Fe and Zn. Coincidentally, however, the genotypes with increased Fe and Zn in leaves were reported to be high N2-fixers in different studies (Belane and Dakora, 2009, 2010; Belane et al., 2011). Clearly, the cowpea/rhizobia symbiosis seems to be a major determinant of leaf and seed protein biosynthesis in cowpea, as well as the accumulation of dietary mineral nutrients in edible parts of this species. Therefore, enhancing these traits in cowpea genotypes through breeding has the potential to overcome protein-calorie malnutrition and trace element deficiency in rural Africa.
Data Availability
All datasets generated for this study are included in the manuscript/supplementary files.
Author Contributions
FD designed the experiment and wrote the manuscript. AB undertook the field experiments, collected, analyzed the data, and was a doctoral student of FD.
Funding
This study was supported with funds from the South African Research Chair in Agrochemurgy and Plant Symbioses, the National Research Foundation, and the Tshwane University of Technology to FD, as well as a competitive grant from the McKnight Foundation.
Conflict of Interest Statement
The authors declare that the research was conducted in the absence of any commercial or financial relationships that could be construed as a potential conflict of interest.
References
Aletor, O., Oshodi, A., and Ipinmoroti, K. (2002). Chemical composition of common leafy vegetables and functional properties of their leaf protein concentrates. Food Chem. 78, 63–68. doi: 10.1016/S0308-8146(01)00376-4
Andrea, F., and Rose, M. (2015). Food insecurity and hunger: a review of FAO's annual report on state of food insecurity in the world. Int. J. Multidiscip. Allied Stud. 2, 1–5. Available online at: https://thescholedge.org/index.php/sijmas/issue/view/30
Ataro, A., McCrindle, R. I., Botha, B. M., McCrindle, C. M. E., and Ndibewu, P. P. (2008). Quantification of trace elements in raw cow's milk by inductively coupled plasma mass spectrometry (ICP-MS). Food Chem. 111, 243–248. doi: 10.1016/j.foodchem.2008.03.056
Ayenan, M. A. T., and Ezin, V. A. (2016). Potential of Kersting's groundnut [Macrotyloma geocarpum (Harms) Maréchal & Baudet] and prospects for its promotion. Agric. Food Secur. 5:10. doi: 10.1186/s40066-016-0058-4
Belane, A. K., Asiwe, J., and Dakora, F. D. (2011). Assessment of N2 fixation in 32 cowpea (Vigna unguiculata L. Walp.) genotypes grown in the field at Taung in South Africa, using 15N natural abundance. Afr. J. Biotech. 10, 11450–11458. doi: 10.5897/AJB11.674
Belane, A. K., and Dakora, F. D. (2009). Measurement of N2 fixation in 30 cowpea (Vigna unguiculata L. Walp.) genotypes under field conditions in Ghana, using the 15N natural abundance technique. Symbiosis 48, 47–56. doi: 10.1007/BF03179984
Belane, A. K., and Dakora, F. D. (2010). Symbiotic N2 fixation in 30 field-grown cowpea (Vigna unguiculata L. Walp.) genotypes in the upper west region of ghana measured using 15N natural abundance. Biol. Fertil. Soils 46, 191–198. doi: 10.1007/s00374-009-0415-6
Belane, A. K., and Dakora, F. D. (2011a). Levels of nutritionally-important trace elements and macronutrients in edible leaves and seed of 27 nodulated cowpea (Vigna unguiculata L. Walp.) genotypes grown in the Upper West Region of Ghana. Food Chem. 125, 99–105. doi: 10.1016/j.foodchem.2010.08.044
Belane, A. K., and Dakora, F. D. (2011b). Photosynthesis, symbiotic N and C accumulation in leaves of 30 nodulated cowpea genotypes grown in the field at Wa in the Guinea savanna of Ghana. Field Crops Res. 124, 279–287. doi: 10.1016/j.fcr.2011.07.014
Belane, A. K., and Dakora, F. D. (2012). Elevated concentrations of dietarily-important trace elements and macronutrients in edible leaves and grain of 27 cowpea (Vigna unguiculata L. Walp.) genotypes. Implications for human nutrition and health. Food Nutr. Sci. 3, 377–386. doi: 10.4236/fns.2012.33054
Belane, A. K., and Dakora, F. D. (2015). Assessing the relationship between photosynthetic C accumulation and symbiotic N nutrition in leaves of field-grown nodulated cowpea (Vigna unguiculata L. Walp.) genotypes. Photosynthetica 53, 562–571. doi: 10.1007/s11099-015-0144-z
Belane, A. K., Pule-Meulenberg, F., Makhubedu, T. I., and Dakora, F. D. (2014). Nitrogen fixation and symbiosis-induced accumulation of mineral nutrients by cowpea (Vigna unguiculata L. Walp.). Crop Pasture Sci. 65, 250–258. doi: 10.1071/CP13283
Broμghton, W. J., Hern, G., Blair, M., Beebe, S., Gepts, P., and Vanderleyden, J. (2003). Beans (Phaseolus spp.) – model food legumes. Plant Soil 252, 55–128. doi: 10.1023/A:1024146710611
Dakora, F. D., and Keya, S. O. (1997). Contribution of legume nitrogen fixation to sustainable agriculture in Sub-Saharan Africa. Soil Biol. Biochem. 29, 809–817. doi: 10.1016/S0038-0717(96)00225-8
Ddamulira, G., and Santos, C. (2015). Seed yield and protein content of Brazilian cowpea genotypes under diverse Mgandan environments. Am. J. Plant Sci. 6:2074. doi: 10.4236/ajps.2015.613208
Enyiukwu, D., Amadioha, A., and Ononuju, C. (2018). Nutritional significance of cowpea leaves for human consumption. Greener Trends Food Sci. Nutr. 1, 1–10. doi: 10.15580/GTFSN.2018.1.061818085
Fanzo, J. (2012). The Nutrition Challenge in Sub-Saharan Africa. United Nations Development Programme, Regional Bureau for Africa. Available online at: http://www.africa.undp.org/content/rba/en/home/library/working-papers/nutrition-challenge.html (accessed on August 25, 2019).
Gerrano, A. S., Jansen van Rensburg, W. S., Venter, S. L., Shargie, N. G., Amelework, B. A., Shimelis, H. A., et al. (2019). Selection of cowpea genotypes based on grain mineral and total protein content. Acta Agric. Scand. Sect. B Soil Plant Sci. 69, 155–166. doi: 10.1080/09064710.2018.1520290
Henao, J., and Baanante, C. (2006). Agricultural Production and Soil Nutrient Mining in Africa: Implications for Resource Conservation and Policy Development. Muscle Shoals, AL: An International Center for Soil Fertility and Agricultural Development.
Jones, D. (1941). Factors for Converting Percentages of Nitrogen in Foods and Feeds Into Percentages of Protein. United States Department of Agriculture, Washington, DC, 183.
Mariotti, F., Tomé, D., and Mirand, P. P. (2008). Converting nitrogen into protein — beyond 6.25 and Jones' Factors converting nitrogen into protein. Crit. Rev. Food Sci. Nutr. 48, 177–184. doi: 10.1080/10408390701279749
Mazahib, A. M., Nuha, M. O., Salawa, I. S., and Babiker, E. E. (2013). Some nutritional attributes of bambara groundnut as influenced by domestic processing. Int. Food Res. J. 20, 1165–1171. Available online at: http://www.ifrj.upm.edu.my (accessed on August 23, 2019).
Naab, J. B., Chimphango, S. M. B., and Dakora, F. D. (2009). N2 fixation in cowpea plants grown in farmers' fields in the upper west region of Ghana, measured using 15N natural abundance. Symbiosis 48, 37–46. doi: 10.1007/BF03179983
Pule-Meulenberg, F., Belane, A. K., Krasova-Wade, T., and Dakora, F. D. (2010). Symbiotic functioning and bradyrhizobial biodiversity of cowpea (Vigna unguiculata L. Walp.) in Africa. BMC Microbiol. 10:89. doi: 10.1186/1471-2180-10-89
Ross, C., Taylor, C., Yaktine, A., and Del Valle, H. (2011). Dietary Reference Intakes for Calcium and Vitamin D. Available online at: https://www.ncbi.nih.gov/books/NBK56070 (accessed August 25, 2019).
Saxena, K., Faris, D., Singh, U., and Kumar, R. (1987). Relationship between seed size and protein content in newly developed high protein lines of pigeonpea. Plant Foods Hum. Nutr. 36, 335–340. doi: 10.1007/BF01892354
Toomer, O. T. (2018). Nutritional chemistry of the peanut (Arachis hypogaea). Crit. Rev. Food Sci. Nutr. 58, 3042–3053. doi: 10.1080/10408398.2017.1339015
Xu, Y., Cartier, A., Obielodan, M., Jordan, K., Hairston, T., Shannon, A., et al. (2016). Nutritional and anti-nutritional composition, and in vitro protein digestibility of Kabuli chickpea (Cicer arietinum L.) as affected by differential processing methods. J. Food Meas. Charact. 10, 625–633. doi: 10.1007/s11694-016-9346-8
Yi-shen, Z., Shuai, S., and FitzGerald, R. (2018). Mung bean proteins and peptides: nutritional, functional and bioactive properties. Food Nutr. Res. 62, 1–11. doi: 10.29219/fnr.v62.1290
Zarkadas, C. G., Gagnon, C., Gleddie, S., Khanizadeh, S., Cober, E. R., and Guillemette, R. J. D. (2007). Assessment of the protein quality of fourteen soybean [Glycine max (L.) Merr.] cultivars using amino acid analysis and two-dimensional electrophoresis. Food Res. Int. 40, 129–146. doi: 10.1016/j.foodres.2006.08.006
Keywords: cowpea, food, sustainability, breeding, micronutrients
Citation: Dakora FD and Belane AK (2019) Evaluation of Protein and Micronutrient Levels in Edible Cowpea (Vigna Unguiculata L. Walp.) Leaves and Seeds. Front. Sustain. Food Syst. 3:70. doi: 10.3389/fsufs.2019.00070
Received: 23 May 2019; Accepted: 16 August 2019;
Published: 04 September 2019.
Edited by:
Jose C. Jimenez-Lopez, Consejo Superior de Investigaciones Científicas (CSIC) Granada, SpainReviewed by:
Abe Shegro Gerrano, Agricultural Research Council of South Africa (ARC-SA), South AfricaAinong Shi, University of Arkansas, United States
Copyright © 2019 Dakora and Belane. This is an open-access article distributed under the terms of the Creative Commons Attribution License (CC BY). The use, distribution or reproduction in other forums is permitted, provided the original author(s) and the copyright owner(s) are credited and that the original publication in this journal is cited, in accordance with accepted academic practice. No use, distribution or reproduction is permitted which does not comply with these terms.
*Correspondence: Felix D. Dakora, RGFrb3JhRkRAdHV0LmFjLnph