- Nutritional Insight Ltd, London, United Kingdom
Mycoprotein is a protein-rich fungal-derived sustainable food source that was first discovered in the early 1960's. Since then, a sizeable body of research has investigated the health benefits of mycelium protein. Given this, the present publication aims to systematically review the effects of mycoprotein on human health. A literature search of human studies was conducted using PubMed Central, ClinicalTrials.Gov, Google Scholar and a manual search. Sixteen controlled trials, totaling 432 participants were included – of these 5 studies reported total cholesterol, 5 reported on energy intake, 7 on insulin levels, 8 on glucose levels and 4 studied protein response. Risk of bias showed that 7 studies were good quality although heterogeneity was apparent between studies. Results showed that acute mycoprotein ingestion was associated with reduced total cholesterol levels, particularly amongst those with hyperlipidemia. Evidence was less conclusive for effects on blood glucose and insulin levels. Mycoprotein also appears to be a promising bioavailable source of essential amino acids that could induce muscle protein synthesis. Overall, given growing interest in sustainable proteins and accruing health evidence for mycoprotein, firmer embedment with food-based dietary guidelines is now worthy of consideration.
Introduction
There are growing demands for sustainable food proteins which utilize technologies that are both green (eco innovative) and cost-effective (Fasolin et al., 2019). Plant-proteins have received heightened interest in recent years, particularly their ability to improve markers of health such as blood lipid profile and glycaemic control amongst people with diabetes when substituted for animal protein (Viguiliouk et al., 2015; Li et al., 2017). The COVID-19 pandemic has placed a spotlight on meat supply chains and food security across the world, further propelling demands for plant-based alternatives (FutureBridge, 2020). Subsequently, plant-based food proteins are swiftly being embedded within evolving Food-Based Dietary Guidelines (FBDG). For example, a global review of FBDG found that half of the countries with protein food key messages (33 out of 67) included both plant-based and animal-derived sources of protein (Herforth et al., 2019). Unfortunately, other well-established food proteins, such as fungal-derived proteins appear to have been comparatively overlooked.
Fungal-derived mycoproteins are gaining in popularity due to their healthy nutritional profile, ability to be produced at low cost, environmental benefits and resilience to landscape limitations such as flood or drought (Hashempour-Baltork et al., 2020). The production strain used to grow and harvest mycoprotein (Fusarium Venenatum ATCC 2684) was discovered in the 1960's (Finnigan et al., 2019). Some years later in 1984 after rigorous testing mycoprotein was approved for sale as a food protein source by the Ministry of Agriculture, Fisheries and Food in the United Kingdom (Wiebe, 2002) and may now be sold in all member states of the EU. Further regulatory approvals followed in Switzerland, Norway the USA and Australia, and more recently in Japan, Thailand, Malaysia and Canada. Mycoprotein is mainly consumed within the range of vegan and vegetarian foods under the brand name QuornTM (Finnigan et al., 2019). Today, mycoprotein is produced at scale using fermentation, producing high-quality protein with a relatively benign environmental footprint (Finnigan et al., 2019).
However, despite the growing popularity with consumers, many health professionals do not yet fully understand the potential for fungal proteins to provide a healthy new protein with a low environmental impact (Derbyshire, 2020a). A scientific panel comprised of health professionals – predominantly dietitians, identified that most were unaware of what fungal-derived foods proteins were and the fact that these were a separate kingdom themselves – not plants (Derbyshire, 2020a).
The growing demands for healthy and sustainable new protein sources mean that misconceptions about fungal proteins should be addressed. This review therefore collates evidence in this field, focusing on mycoprotein. We first provide background on the origins of fungal-derived mycelium mycoprotein and then systematically review the health evidence, focusing on cholesterol, energy intake, glucose and insulin levels and protein response.
Fungal Foundations
Fungi are a large and diverse group of eukaryotic organisms that start as microscopic filaments (Alexopoulos et al., 1996). They play fundamental roles in nutrient cycling, acting as predators, pathogens and parasites, and are often found living in symbiotic associations with algae, animals, plants and other organisms (Naranjo-Ortiz and Gabaldon, 2019). Fungi are often considered to be “plant-based” but their cell walls are composed of beta-glucan and chitin rather than cellulose and the absence of chloroplasts makes them distinctly different from plants thus placing them out of this category (Baldauf et al., 2000; Katz et al., 2012).
Mushrooms and truffles are also a type of fungi (Basidiomycetes) but are generally not considered to be suitable meat alternatives due to their lower protein content (Boland et al., 2013; Souza Filho et al., 2019). Mycoprotein is produced from separate member of the fungi family (ascomycetes) and is grown by fermentation (Derbyshire, 2020a). Its overall protein digestibility-corrected amino acid score is 0.996 which has been derived using gold-standard ileostomy methods, demonstrating that it is a high quality protein (Edwards and Cummings, 2010). The filamentous nature of the hyphal creates fibrous bundles that emulate the texture of meat (Figure 1). Recent publications suggest that this structural complexity of the fungal cell wall may provide insights into causal mechanisms for putative benefits to metabolic health (Colosimo et al., 2019, 2020; Colosimoa et al., 2020).
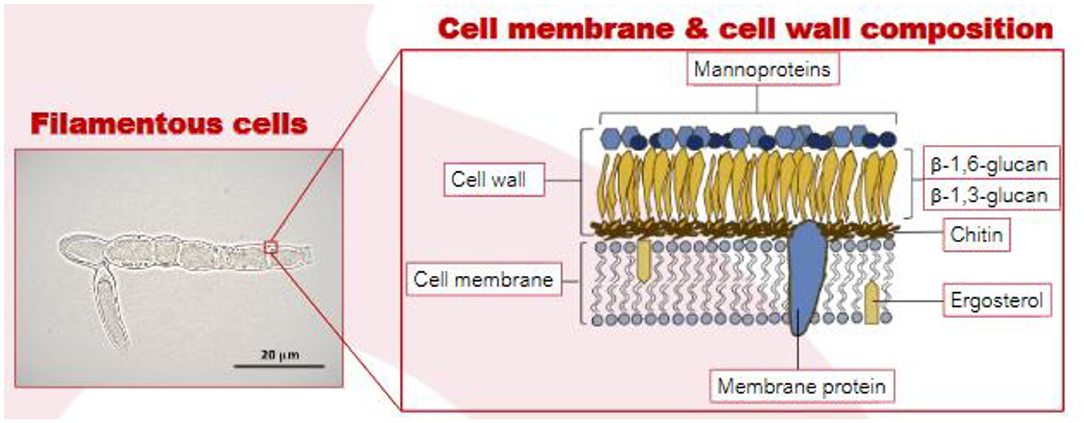
Figure 1. Optimal micrograph of MycoproteinTM filamentous cells (left) and the cell wall/membrane of the filamentous fungi (right). Source: Vega and Kalkum (2012), Colosimo et al. (2019).
Production
A full description of mycoprotein production has been published by Finnigan (2011). The production strain for mycoprotein is first grown using an aerobic fermentation system and carbohydrate and nutrient substrates required for growth (Finnigan, 2011). Next, the mycelium of the fungus is heat-treated to reduce ribonucleic acid content to approved levels. Once the ribonucleic acid levels are lowered, the suspended hyphae are recovered by centrifugation and a supernatant is yielded which is mycoprotein (Gilani and Lee, 2003). During the final phases of production, the processes of steaming, chilling and freezing of mycoprotein result in a meat-like structure, similar to chicken when observed under a microscope. These combined processes along with the final addition of added egg albumen, functional ingredients, flavors, herbs and spices, leads to an end product that mimics the texture of meat (Wiebe, 2002). More recently, plant-based alternatives to egg protein have been discovered that allow vegan products to be produced.
Nutritional Properties
Mycoprotein is a sustainably produced, protein-rich, high-fiber, whole food source (Table 1) (Coelho et al., 2019). Mycoprotein products have a higher weight-percentage protein content than other common plant or fungal sources of protein, though lower than meats. The fiber found in the cell walls of mycoprotein is largely insoluble in the small intestine and is comprised of two-thirds β-glucan and one-third chitin, creating a “fibrous chitin–glucan matrix” (Denny et al., 2003; Finnigan et al., 2019). Mycoprotein according to European Commission standards is “high in fiber” i.e., providing at least 6 g of fiber per 100 g (De Gregori et al., 2006; EC, 2008).
Regarding micronutrients when compared with the other protein food sources mycoprotein does well for vitamin B9 (folate), vitamin B12, calcium, phosphorous, magnesium and zinc. It has also been analyzed for choline which is reported to be ~180 mg per 100 g thus compares well with other foods such as cooked salmon (90 mg/100 g), pork (103 mg/100 g), dried soybeans (116 mg/100 g), bacon (125 mg/100 g), and wheat germ (152 mg/100 g) which have been reported to have some of the highest choline profiles (Zeisel et al., 2003; Wiedeman et al., 2018).
Methods
Search Strategy
A search for relevant human studies was undertaken using the US National Library of Medicine National Institutes of Health Database (PubMed Central). The following search terms were applied: (mycoprotein [All Fields] OR myco-protein [All Fields] OR fungi-derived protein [All Fields] OR Fusarium Venenatum [All Fields] OR quorn [All Fields]) AND health [All Fields] OR cholesterol [All Fields] OR lipids [All Fields] OR insulin* OR glucose levels [All Fields] OR glycaemia [All Fields] OR glycemia [All Fields] OR glycaemic [All Fields] OR energy intake [All Fields] OR protein bioavailability [All Fields] OR protein response OR anabolism [All Fields]).
Manual searches of reference lists were also undertaken to identify additional articles of relevance. Further general searches using the term “mycoprotein” were also undertaken using Google Scholar and ClinicalTrials.Gov to identify further human trials. A manual search of reference lists was also conducted. A cut-off date of November 6th 2020 was applied to the searches.
Approach
The search for human trials used the Preferred Reporting Items for Systematic Reviews and Meta-Analyses (PRISMA) approach as illustrated in Figure 1 (Moher et al., 2009). The PICOS criteria (patients, intervention, comparator, outcome, study design) was applied to determine study eligibility (Cochrane, 2019). The population (P) was defined as: adults, aged 17 years and over. The intervention (I) was the consumption of mycoprotein, fungi-derived protein, Fusarium Venenatum or QuornTM. The comparison (C) was a defined control or placebo group and the outcomes of interest (O) were markers of health which included: Total cholesterol, energy intakes, glucose levels, insulin levels and markers of protein response including muscle protein fractional synthesis rate.
Inclusion/Exclusion Criteria
The present review included: human studies comprised of young people or adults (≥17 years) investigating mycoprotein in any form in relation to markers of health. Animal and mechanistic studies were excluded. Those using multi-interventions which could have skewed results were also not used. Studies that did not clearly specify the intervention were also withdrawn, as were studies that did not specify the level of intake in relation to the intervention being tested. Review papers were not included within the present review.
Data Charting
Data charted from the trials included the following: General details of the study (author, year, and location), participants (age, gender, and health status), methods, intervention (type and amount), the comparator group, health outcome(s) and study outcomes with any reported significant p-values.
Studies were identified by authors and screened based on the specified inclusion and exclusion criteria. Studies were initially checked using their title and were then further verified and screened based on their abstract. The procedure of identification, screening, evaluation of eligibility and inclusion is illustrated in Figure 2. The Jadad scale was used to develop quality scores for each study (Jadad et al., 1996). Quality scores were graded between 1 and 5 with higher scores being indicative of higher quality.
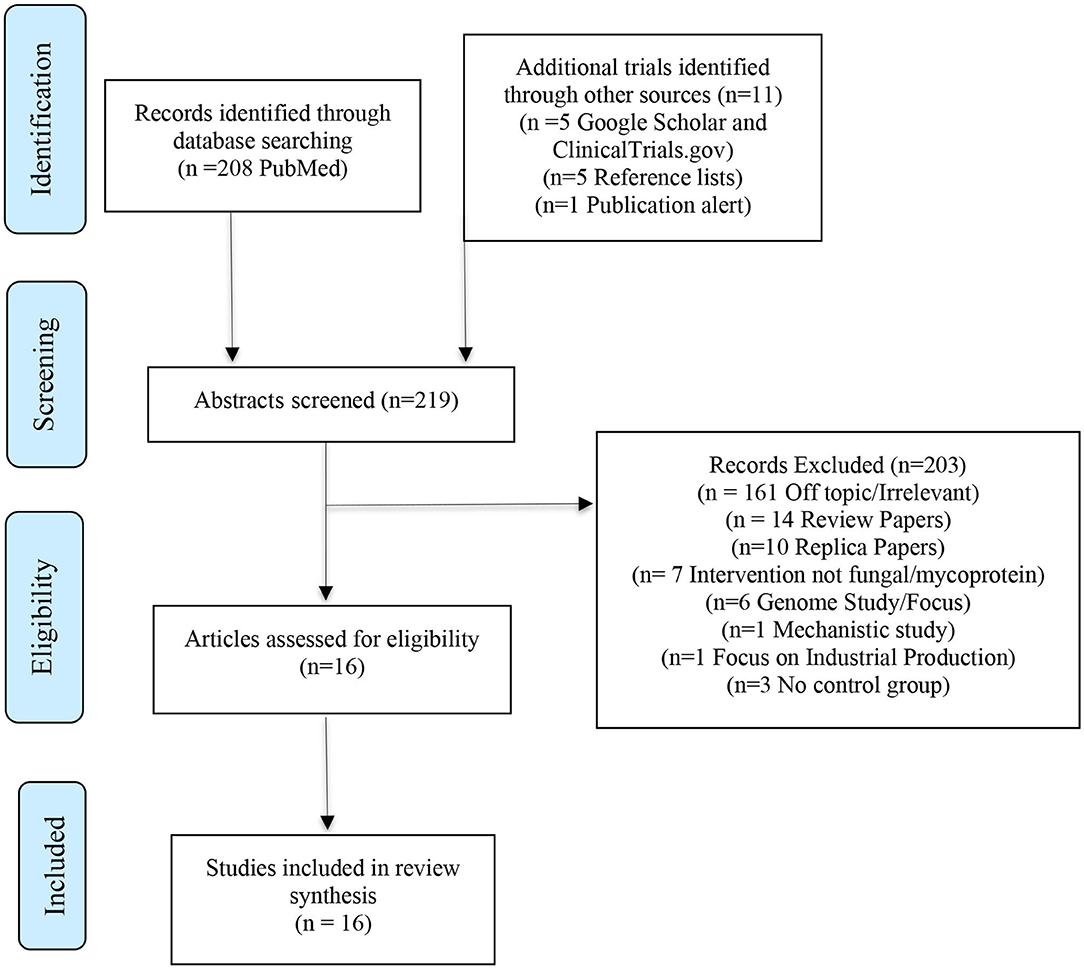
Figure 2. PRISMA approach to identifying trials (Moher et al., 2009).
Results
The PubMed Central search yielded 208 publications for screening. Searches through additional databases and reference lists identified a further 11 publications. A total of 219 publications were subsequently screened. Of these a total of 203 publications were excluded - 161 were off topic/irrelevant, 14 were review papers, 10 replica papers, seven were studies that did not include mycoprotein as an intervention, six papers were genome-focused, three did not have a control group, one was a mechanistic study and one focused on industrial production. After screening for eligibility and reviewing the full text a total of 16 human controlled trials were included in the final review.
Among the included 16 studies the majority were conducted in the United Kingdom (14 studies) and two in the United States. The studied populations were predominantly adults (including young, middle aged, and older adults), with most studies recruiting both males and females. Whilst the majority of studies recruited healthy subjects at baseline some studies recruited overweight adults (Bottin, 2011; Bottin E. et al., 2012; Bottin et al., 2016). Ruxton and McMillan (2010) included adults who reported being in good health but some had high cholesterol levels at baseline. Similarly, Turnbull et al. (1990) included adults with slightly raised cholesterol levels (Turnbull et al., 1990).
As shown in Tables 2, 3 the trials identified focused on the specified outcomes, including total cholesterol (Udall et al., 1984; Turnbull et al., 1990, 1992; Ruxton and McMillan, 2010; Coelho et al., 2020b), energy intake (Burley et al., 1993; Turnbull et al., 1993; Williamson et al., 2006; Bottin E. et al., 2012; Bottin et al., 2016), glucose levels (Turnbull and Ward, 1995; Ruxton and McMillan, 2010; Bottin, 2011; Bottin et al., 2016; Coelho et al., 2020a,b), insulin levels (Turnbull and Ward, 1995; Bottin, 2011; Bottin et al., 2016; Dunlop et al., 2017; Coelho et al., 2020a,b; Monteyne et al., 2020a) and protein response (Udall et al., 1984; Dunlop et al., 2017; Monteyne et al., 2020a,b). As shown in Table 4 seven were good quality, scoring 3 or more after the application of the Jadad criteria (Turnbull et al., 1990; Burley et al., 1993; Bottin et al., 2016; Dunlop et al., 2017; Monteyne et al., 2020a,b). Other studies did not fully report randomization and/or blinding methods and some required translation meaning that such finer details could have been missed.
Health Evidence
Energy Intake
Five studies considered effects on mycoprotein on energy intake. Evidence of energy reduction was determined at subsequent ad libitum meals and post-24 h (Burley et al., 1993; Turnbull et al., 1993; Williamson et al., 2006; Bottin J. H. et al., 2012; Bottin et al., 2016). Burley et al. (1993) and Turnbull et al. (1993) were some of the first investigators to research this (Burley et al., 1993; Turnbull et al., 1993). Burley et al. (1993) recruited 18 lean adults finding that eating a lunch providing mycoprotein reduced evening ad libitum energy intake by 18% when compared with a chicken control, these findings were particularly prominent in males. Turnbull et al. (1993) also found that energy intake was reduced by 24% after healthy weight females consumed 130 g of mycoprotein or chicken as isoenergetic meals and these effects extended into the next day where energy intake was further reduced by 16.5%.
Bottin E. et al. (2012) and Bottin et al. (2016) extended this research by studying effects in overweight adults (Bottin J. H. et al., 2012; Bottin et al., 2016). In both randomized studies reductions in energy intake were observed with up to 132 g of mycoprotein reducing later ad libitum energy intake by 10% at a later meal. The research at the time was not able to provide clear mechanistic explanations although the metabonomic analysis indicated that certain candidate molecules could be worthy of investigation. Williamson et al. (2006) recruited healthy, pre-menopausal females finding that a pasta preload providing 44.3 g mycoprotein significantly reduced energy intakes at the following meal when compared to the chicken control.
Overall, acute mycoprotein ingestion appears effective at reducing energy intake at later ad libitum meals and 24-h post ingestion in lean, overweight, and obese adults. Longer-term trials would be worthwhile and help to decipher whether effects are sustained and potential underpinning mechanisms behind such actions.
Cholesterol Levels
Incorporating modest amounts of fungal mycoprotein into the diet could reduce total cholesterol levels. Presently five human studies have investigated the effects of fungal protein (mycoprotein) in relation to total cholesterol levels (Udall et al., 1984; Turnbull et al., 1990, 1992; Ruxton and McMillan, 2010; Coelho et al., 2020b). Turnbull undertook two studies (1990; 1992). In the first, subjects receiving 191 g mycoprotein over lunch and dinner over 3-weeks led to a 13% reduction in plasma total cholesterol (Turnbull et al., 1990). In a longer 8-week study Turnbull et al. (1992) observed similar reductions in total cholesterol levels although with the intervention (130 g mycoprotein; wet weight) being delivered as cookies (Turnbull et al., 1992).
In a community setting Ruxton and McMillan (2010) used 88 g of wet weight mycoprotein per day and observed significantly lower cholesterol levels amongst those who had higher levels at baseline. These were interesting findings implying that mycoprotein could be a useful food ingredient for the management of blood cholesterol levels although no blinding or randomization was used in this study which could have influenced habitual diet compliance in the control group (Ruxton and McMillan, 2010).
More recently, Coelho et al. (2020b) provided a diet containing 1.2 g of protein per kilogram of body weight which was supplied from QuornTM, meat or fish observing beneficial effects on the plasma lipidome - certain lipid subfractions decreased which included total plasma cholesterol and free-cholesterol in the mycoprotein compared with the control group. It was proposed that these cholesterol-lowering effects could attributed to the amount or type of fiber present (Coelho et al., 2020b). One theory is that short-chain fatty acids (SCFAs) such as acetate, propionate and butyrate which are primary products of fiber fermentation could underpin some of these effects (Cummings et al., 1987; Coelho et al., 2020b). Ongoing research is needed to clarify such potential mechanisms.
Glucose Levels
Eight studies measured glucose levels as a marker of glycaemia (Udall et al., 1984; Turnbull and Ward, 1995; Ruxton and McMillan, 2010; Bottin, 2011; Bottin et al., 2016; Dunlop et al., 2017; Coelho et al., 2020a,b). Overall, results showed a less clear effect of acute mycoprotein ingestion in relation to blood glucose levels. Early research by Turnbull and Ward (1995), for example, observed reductions in glucose values in both the mycoprotein and control group although glycaemia was found to be significantly reduced at 60 min (13% reduced) after the mycoprotein meal (Turnbull and Ward, 1995). Research by Ruxton and McMillan (2010) showed that glucose levels in the mycoprotein condition reduced over time and increased in the control group. Nevertheless, post-hoc test results were not statistically significant (Ruxton and McMillan, 2010).
Bottin (2011) monitored glucose levels, reporting these as Incremental Area Under the Curve (IAUC) values which were lower amongst overweight adults ingesting 30 g mycoprotein (dry weight) compared with the whey protein control, but not to a statistically significant level. Later work by Bottin et al. (2016) which recruited overweight and obese subject did not observe any significant differences in glucose levels after they were fed low, medium or high mycoprotein or energy equivalent chicken meals (Bottin et al., 2016). Dunlop et al. (2017) determined glucose profiles with some evidence of reduction in late postprandial phases after mycoprotein ingestion, although the statistical analyses were not included or discussed in the main body of the paper (Dunlop et al., 2017). Coelho et al. (2020a,b) has undertaken two studies each measuring glucose levels, with no significant differences being reported when compared against controls.
Insulin Levels
Seven trials have studied the effects of acute mycoprotein ingestion in relation to insulin levels (Turnbull and Ward, 1995; Bottin, 2011; Bottin et al., 2016; Dunlop et al., 2017; Coelho et al., 2020a,b; Monteyne et al., 2020a). Turnbull and Ward (1995) first found that post meal insulinemia was significantly reduced in the mycoprotein compared with the control group at 30 and 60 min post ingestion (Turnbull and Ward, 1995). Bottin (2011) measured the IAUC and post-prandial insulin resistance (PPIR) in 10 healthy, overweight adults observing significant reductions in insulin levels 15, 30-, and 45-min post-ingestion. PPIR significantly improved after mycoprotein compared with whey protein ingestion (Bottin, 2011). A larger study by Bottin et al. (2016) showed that mycoprotein meals containing low (44 g), medium (88 g) or high (132 g) mycoprotein significantly reduced insulin concentrations compared with chicken using IAUC calculations, with these reported to be −8% (IAUC low), −12% (IAUC medium), and −21% (IAUC high), respectively (Bottin E. et al., 2012). Such findings are interesting as they occurred under energy-balanced conditions thus are not a result of lower overall energy intake or weight loss.
Dunlop et al. (2017) demonstrated that mycoprotein ingestion led to slower but more sustained hyperinsulinaemia when compared with protein-match milk (Dunlop et al., 2017). Similarly, Monteyne et al. (2020a) found that mycoprotein ingestion (70 g) resulted in a less rapid but more sustained increase in serum insulin levels, peaking at 30 min after consumption when compared with milk protein (Monteyne et al., 2020a). Coelho et al. (2020a,b) did not report any differences in serum insulin responses after mycoprotein ingestion when compared against meat/fish and nucleotide depleted mycoprotein controls (Coelho et al., 2020a,b).
Mycoprotein consumption may be more effective at regulating insulin levels amongst individuals who are overweight or obese at baseline (Bottin, 2011; Bottin et al., 2016). Larger and longer studies are needed to further investigate such effects. Present studies have been heterogeneous in design and laboratory-based which limits the extrapolation and broader application of findings into community settings.
Protein Response
Four studies examined the bioavailability and muscular synthetic effects of mycoprotein (Udall et al., 1984; Dunlop et al., 2017; Monteyne et al., 2020a,b). Udall et al. (1984) was one of the first studies to investigate the digestibility, biological value and net protein utilization of fungal protein (F. graminearium) finding that this was 78, 84, and 65% compared with 95, 85, and 80% for milk, respectively (Udall et al., 1984). Five separate trials conducted in a single-blind, randomized, cross-over design with 12 healthy young males found mycoprotein to be a bioavailable source of dietary protein that could help to stimulate muscle protein synthesis rates; 40 g mycoprotein (18 g total protein) was sufficient in mounting a robust muscle protein synthetic response whilst 60 g mycoprotein (i.e., 27 g total protein) was regarded as ample for optimal stimulation of muscle protein synthesis rates in healthy young men (Dunlop et al., 2017). It was, however, concluded that further intakes beyond the 60 g administered would be unlikely to confer any further health benefits (Dunlop et al., 2017).
Building on this work Monteyne et al. (2020a) undertook a double-blind, randomized, parallel-group study. This trial recruited a slightly larger sample size - 22 healthy males who ingested either 70 g (31.5 g protein; 2.5 g leucine) mycoprotein or 31 g (26.2 g protein; 2.5 g leucine) milk protein. It was observed that mycoprotein stimulated resting and post-resistance exercise muscle protein synthesis rates to a greater extent than a leucine-matched bolus of milk protein (Monteyne et al., 2020a). Other work related to this study concluded that the ingestion of a lower-dose of BCAA-enriched mycoprotein (35 g BCAA-enriched mycoprotein; 18.7 g protein) was effective at stimulating resting and post-exercise muscle protein synthesis but to a lesser extent than the BCAA-matched 70-g mycoprotein bolus (Monteyne et al., 2020b).
Discussion/Perspectives and Conclusion
The present review demonstrates that fungal mycoprotein is a well-established food source with potential benefits for health. Sixteen human trials have studied inter-relationships between fungal mycoprotein consumption and markers of health (Udall et al., 1984; Turnbull et al., 1990, 1992, 1993; Burley et al., 1993; Turnbull and Ward, 1995; Williamson et al., 2006; Ruxton and McMillan, 2010; Bottin, 2011; Bottin E. et al., 2012; Bottin et al., 2016; Dunlop et al., 2017; Coelho et al., 2020a,b; Monteyne et al., 2020a,b).
Overall, acute ingestion of mycoprotein appears to have promising effects on the reduction of total cholesterol levels (Udall et al., 1984; Turnbull et al., 1990, 1992; Ruxton and McMillan, 2010), particularly amongst those with slightly raised cholesterol levels or hyperlipidemia (Turnbull et al., 1990; Ruxton and McMillan, 2010). Further benefits are apparent for energy intake – acute mycoprotein ingestion appears to consistently lower later ad libitum meal and 24-h energy intake. The extended effects on energy intakes in the longer-term in community settings would also be worthy of investigation. Regarding protein response, mycoprotein appears to be a promising food source providing bioavailable essential amino acids that improve muscle protein fractional synthesis rates (Udall et al., 1984; Dunlop et al., 2017; Monteyne et al., 2020a,b)
Findings were less conclusive for glucose and insulin levels. For the former, whilst lower blood glucose levels were reported in some studies, findings were not reported to be statistically significant (Ruxton and McMillan, 2010; Bottin, 2011; Bottin et al., 2016; Coelho et al., 2020a,b). Studying the effects of mycoprotein ingestion amongst those with glucose dysregulation i.e., diabetic patients could help to further determine whether any potential effects exist. Changes in insulin levels were variable between studies although it has been reported elsewhere that there could be benefits for reduced insulinaemia (Cherta-Murillo et al., 2020). Heterogeneity between studies is likely to account for variable differences between trials. Studies need to report study outcomes for both intervention and control groups and apply statistical methods comparing the two so that future comparisons can be made with consistency and clarity. Meta-analytical analysis would also be worthy in the future.
Fungi such as mycoprotein are examples of foods that provide protein, intrinsic fiber, micronutrients and potential bioactive compounds (Coelho et al., 2019; Derbyshire and Ayoob, 2019). Research shows that the structural complexity of the fungal cell wall could contribute to causal mechanisms behind some of its observed physiological benefits (Colosimo et al., 2019; Colosimoa et al., 2020). Work has demonstrated that the presence of fungal cell walls in mycoprotein slows down the kinetics of sugar release during digestion when compared against no cell walls (Colosimoa et al., 2020). Mechanistically, the porosity of mycoprotein fungal cell wall appears to permit the diffusion of α-amylase into the cells which, in turn, results in the entrapment of the enzyme within the hyphal matrix and then subsequent reduced enzymatic activity and starch hydrolysis (Colosimoa et al., 2020). These effects are thought to be potential underpinning mechanisms that could be responsible for mycoproteins ability to potentially modulate postprandial glycaemia/insulinemia (Colosimoa et al., 2020). Other work shows that digestive enzymes are able to diffuse through the cell wall of mycoprotein (due to its porosity), facilitating protein hydrolysis – a process central to digestion and protein release (Colosimo et al., 2019, 2020).
Alongside these health studies the bioactive profiles of specific fungi and their separate health effects are worthy of future investigation. Bioactive compounds are becoming increasingly valuable in the fields of medicine, food and health (Prakash and Namasivayam, 2014). Filamentous fungi are known to produce an array of secondary metabolites which can predominantly be divided into four groups: alkaloids, terpenes, non-ribosomal peptides and polyketides (Liu and Liu, 2018). Fungal lectins also represent an enormous unexplored source of potentially useful and novel lectins (Hassan et al., 2015). These non-immunoglobulin proteins can bind diverse sugar structures with a high degree of selectivity and are gaining interest for their potential antitumor, antiproliferative and immunomodulatory activities (Hassan et al., 2015). So far an analysis of Fusarium Venenatum revealed that this contained diverse compounds with potential pharmacological activities, although further research needs to specify what these are (Prakash and Namasivayam, 2014). The role of fungi in gut health is also gaining interest, with many species of fungi being found in healthy human gastrointestinal systems (Hallen-Adams and Suhr, 2017).
Pigments found in fungi such as ankaflavins, anthraquinone, flavins, melanins, naphthoquinone, and quinones are also growing in interest due to their potential medicinal and food properties (Akilandeswari and Pradeep, 2016; Lagashetti et al., 2019). The production of mycelium with a high ergothioneine content is also attracting growing interest (Liang et al., 2013; Lin et al., 2015). Ergothioneine from a health perspective is defined as a thiol/thione molecule that is synthesized by certain fungi and is a useful diet-derived antioxidant, with reduced blood/plasma levels of ergothioneine being observed in certain diseases e.g., in cases of cognitive impairment and Parkinson disease (Cheah et al., 2016; Hatano et al., 2016; Halliwell et al., 2018). Glutathione, another low molecular-weight thiol has been found to be present in high concentrations in filamentous fungi and yeasts and plays a role in basic cellular functions, cell development, differentiation, mitochondrial structure and membrane integrity (Pócsi et al., 2004; Wu et al., 2004). Glutathione deficiency contributes to oxidative stress which has been attributed to the etiology of certain diseases including Alzheimer's disease, Parkinson's disease, stroke and diabetes, amongst others (Wu et al., 2004).
Future Perspectives
From a broader perspective expanding populations are placing unprecedented pressures on the world's food resources – intensified production of animal-based protein increases GHGEs, land and water use – indeed a “perform storm” accentuating the need for alternative sources (Henchion et al., 2017). Mycoprotein is more efficient in land and water use than animal-derived proteins and has GHGEs lower than beef production (Smetana et al., 2018; The Carbon Trust, 2019). Plant-based diets are less taxing on the environment and thus have rightly been receiving growing interest and gradual incorporation within FBDG, although such messages are not yet universally echoed across countries (Sabaté and Soret, 2014; Herforth et al., 2019). Vegan diets have also been associated with lower serum total cholesterol levels, compared with meat-eaters, fish-eaters and vegetarians, which may be attributed to certain dietary differences (Bradbury et al., 2015). Nevertheless, confusions remain to exist with some health professionals perceiving fungal protein to be “plant-based” which is not the case (Derbyshire, 2020a,b).
Health and mechanistic evidence for fungal mycoprotein has been building. Thus, greater awareness of this alternative whole-food protein is needed from a public and health professional stance. One way to improve this would be to formally embed fungal protein within FBDG, alongside animal and plant-derived proteins (Derbyshire, 2020b). This would help health professionals and consumers to better understand the array of food proteins that are available. Public perceptions appear to be changing about the consumption of fungal protein. For example, changing demographics means that older consumers are now willing to accept alternative, more sustainable protein sources (Grasso et al., 2019). It is also predicted that Asian countries will also become major market sectors with an interest in meat analogs (Ismail et al., 2020).
Lastly, fungal biotechnology has the ability to transform organic materials into nutritious food protein, helping to tackle the urgent global challenges that are becoming increasingly apparent (Finnigan et al., 2019; Meyer et al., 2020). Humans have been tapping into biodiversity for hundreds of thousands of years, yet at no time in history has it been more vital to advance the exploration of how we can utilize these as a means of healthy and sustainable food protein (Antonelli et al., 2019).
Other food production platforms for fungi are also emerging in the accelerating alternative food space which are anticipated to add to this body of evidence in the future. For example, the use of the filamentous fungi belonging to the genus Aspergillus species is advancing within the fields of biotechnology and recombinant protein production (Ntana et al., 2020). Elsewhere, a novel fungi product has been produced from the filamentous fungus Neurospora intermedia, grown on widely available bread waste (Hellwig et al., 2020). A pea-processing by-product has also been found to be an effective medium for filamentous fungi production, enabling production of a vegan-protein concentrate (Souza Filho et al., 2018).
Whilst soy and wheat proteins have had long and established shares of the protein market, additional protein ingredients are advancing with rapidity – especially from plants and fungi, emphasizing the need for protein diversification within modern-day diets (Schweiggert-Weisz et al., 2020). Taking these points into consideration, the time has come to re-assess FBDG and the valuable roles of producing food protein from fungi, which includes mycelium mycoprotein, which has established benefits from a health and environmental stance (Matassa et al., 2016; Fasolin et al., 2019).
Conclusions
In conclusion, the health evidence for the fungal protein mycoprotein has been building over the last half of the century. Sixteen human studies were identified in the present review, with strongest evidence supporting mycoproteins role in reducing total cholesterol levels and short-term energy intake. Its role in the regulation of glucose and insulin levels was less conclusive but mycoprotein shows great promise as a bioavailable protein that can facilitate muscle protein synthesis. Given the advancement of health evidence in this field, coupled with rising concerns about food production and planetary health, now appears to be the ideal time to better consider fungal protein within FBDG.
Data Availability Statement
The original contributions presented in the study are included in the article/supplementary material, further inquiries can be directed to the corresponding author/s.
Author Contributions
All authors listed have made a substantial, direct and intellectual contribution to the work, and approved it for publication.
Conflict of Interest
ED and JD were employed by the company Nutritional Insight Ltd and acted as independent nutrition consultants in the research and writing of this article. The authors declare that this study received funding from Marlow Foods (Quorn Foods) Limited, Stokesley, England. The funder was not involved in the study design, collection, analysis, interpretation of data, the writing of this article or the decision to submit it for publication.
References
Akilandeswari, P., and Pradeep, B. V. (2016). Exploration of industrially important pigments from soil fungi. Appl. Microbiol. Biotechnol. 100, 1631–1643. doi: 10.1007/s00253-015-7231-8
Alexopoulos, C., Mims, C., and Blackwell, M. (1996). Introductory Mycology. New York, NY: John Wiley and Sons.
Antonelli, A., Smith, R. J., and Simmonds, M. S. J. (2019). Unlocking the properties of plants and fungi for sustainable development. Nat. Plants 5, 1100–1102. doi: 10.1038/s41477-019-0554-1
Baldauf, S. L., Roger, A. J., Wenk-Siefert, I., and Doolittle, W. F. (2000). A kingdom-level phylogeny of eukaryotes based on combined protein data. Science 290, 972–977. doi: 10.1126/science.290.5493.972
Boland, M., Rae, A., Vereijken, J., Meuwissen, M., Fischer, A., van Boekel, M., et al. (2013). The future supply of animal-derived protein for human consumption. Trends Food Sci. Technol. 29, 62–73. doi: 10.1016/j.tifs.2012.07.002
Bottin, E., Finnigan, T. J. A., and Frost, G. S. (2012). Mycoprotein reduces energy intake and improves insulin sensitivity compared to chicken. Obesity Facts 5, 55–79.
Bottin, J. (2011). Mycoprotein reduces insulinemia and improves insulin sensitivity. Proc. Nutr. Soc. 70:E372. doi: 10.1017/S0029665111004575
Bottin, J. H., Cropp, E., Finnigan, T. J. A., and Hogban, A. (2012). Mycoprotein reduces energy intake and improves insulin sensitivity compared to chicken. Obes. Facts 5, 55–79.
Bottin, J. H., Swann, J. R., Cropp, E., Chambers, E. S., Ford, H. E., Ghatei, M. A., et al. (2016). Mycoprotein reduces energy intake and postprandial insulin release without altering glucagon-like peptide-1 and peptide tyrosine-tyrosine concentrations in healthy overweight and obese adults: a randomised-controlled trial. Br. J. Nutr. 116, 360–374. doi: 10.1017/S0007114516001872
Bradbury, K. E., Crowe, F. L., Appleby, P. N., Schmidt, J. A., Travis, R. C., and Key, T. J. (2015). Serum concentrations of cholesterol, apolipoprotein A-I and apolipoprotein B in a total of 1694 meat-eaters, fish-eaters, vegetarians and vegans. Eur. J. Clin. Nutr. 69:1180. doi: 10.1038/ejcn.2015.134
Burley, V. J., Paul, A. W., and Blundell, J. E. (1993). Influence of a high-fibre food (myco-protein) on appetite: effects on satiation (within meals) and satiety (following meals). Eur. J. Clin. Nutr. 47, 409–418.
Cheah, I. K., Feng, L., Tang, R. M. Y., Lim, K. H. C., and Halliwell, B. (2016). Ergothioneine levels in an elderly population decrease with age and incidence of cognitive decline; a risk factor for neurodegeneration? Biochem. Biophys. Res. Commun. 478, 162–167. doi: 10.1016/j.bbrc.2016.07.074
Cherta-Murillo, A., Lett, A. M., Frampton, J., Chambers, E. S., Finnigan, T. J. A., and Frost, G. S. (2020). Effects of mycoprotein on glycaemic control and energy intake in humans: a systematic review. Br. J. Nutr. 123, 1321–1332. doi: 10.1017/S0007114520000756
Cochrane (2019). Cochrane Linked Data. PICO ontology. Available online at: https://linkeddata.cochrane.org/pico-ontology
Coelho, M. O. C., Monteyne, A. J., Dirks, M. L., Finnigan, T. J. A., Stephens, F. B., and Wall, B. T. (2020b). Daily mycoprotein consumption for one week does not affect insulin sensitivity or glycaemic control but modulates the plasma lipidome in healthy adults: a randomised controlled trial. Br. J. Nutr. 125, 147–160. doi: 10.1017/S0007114520002524
Coelho, M. O. C., Monteyne, A. J., Dunlop, M. V., Harris, H. C., Morrison, D. J., Stephens, F. B., et al. (2019). Mycoprotein as a possible alternative source of dietary protein to support muscle and metabolic health. Nutr. Res. Rev. 78, 486–497. doi: 10.1093/nutrit/nuz077
Coelho, M. O. C., Monteyne, A. J., Kamalanathan, I. D., Najdanovic-Visak, V., Finnigan, T. J. A., Stephens, F. B., et al. (2020a). Short-communication: ingestion of a nucleotide-rich mixed meal increases serum uric acid concentrations but does not affect postprandial blood glucose or serum insulin responses in young adults. Nutrients 12:1115. doi: 10.3390/nu12041115
Colosimo, R., Warren, F. J., Finnigan, T. J. A., and Wilde, P. J. (2020). Protein bioaccessibility from mycoprotein hyphal structure: in vitro investigation of underlying mechanisms. Food Chem. 330:127252. doi: 10.1016/j.foodchem.2020.127252
Colosimo, R., Warrena, F., Finnigan, T., and Wilde, P. (2019). “The role of fungal cell wall in the control of protein bioaccessibility from Mycoprotein™,” in 6th International Conference on Food Digestion. (Granada).
Colosimoa, R., Warrena, F., Edwardsa, C., Finnigan, T., and Wilde, P. (2020). The interaction of α-amylase with mycoprotein: diffusion through the fungal cell wall, enzyme entrapment, and potential physiological implications. Food Hydrocol. 108:106018. doi: 10.1016/j.foodhyd.2020.106018
Cummings, J. H., Pomare, E. W., Branch, W. J., Naylor, C. P., and Macfarlane, G. T. (1987). Short chain fatty acids in human large intestine, portal, hepatic and venous blood. Gut 28, 1221–1227. doi: 10.1136/gut.28.10.1221
De Gregori, M., Belfer, I., De Giorgio, R., Marchesini, M., Muscoli, C., Rondanelli, M., et al. (2006). Regulation (EC) No 1924/2006 OF THE European parliament and of the council of 20 December 2006 on nutrition and health claims made on foods. Off. J. Euro. Union 18, 244–259.
Denny, A., Aisbitt, B., and Lunn, J. (2003). Mycoprotein and health nutrition. Nutr. Bull. 33, 298–310. doi: 10.1111/j.1467-3010.2008.00730.x
Derbyshire, E. J. (2020b). Is there scope for a novel mycelium category of proteins alongside animals and plants? Foods J. 9, 1–15. doi: 10.3390/foods9091151
Derbyshire, E. J., and Ayoob, K. T. (2019). Mycoprotein nutritional and health properties. Nutr. Today Clin. Nutr. 54, 1–9. doi: 10.1097/NT.0000000000000316
Dunlop, M. V., Kilroe, S. P., Bowtell, J. L., Finnigan, T. J. A., Salmon, D. L., and Wall, B. T. (2017). Mycoprotein represents a bioavailable and insulinotropic non-animal-derived dietary protein source: a dose-response study. Br. J. Nutr. 118, 673–685. doi: 10.1017/S0007114517002409
EC (2008). Commission Directive 2008/100/EC of 28 October 2008 amending council directive 90/496/EEC on nutrition labelling for foodstuffs as regards recommended daily allowances, energy conversion factors and definitions. Off. J. Euro. Union 38, 208–211.
Edwards, G. D., and Cummings, J. (2010). The protein quality of mycoprotein. Proc. Nutri. Soc. 69:OCE4. doi: 10.1017/S0029665110001400
Fasolin, L. H., Pereira, R. N., Pinheiro, A. C., Martins, J. T., Andrade, C. C. P., Ramos, O. L., et al. (2019). Emergent food proteins - towards sustainability, health and innovation. Food Res. Int. 125:108586. doi: 10.1016/j.foodres.2019.108586
Finnigan, T. J. A. (2011). 13 – Mycoprotein: Origins, Production and Properties, Handbook of Food Proteins, Woodhead Publishing Series in Food Science, Technology and Nutrition. doi: 10.1533/9780857093639.335
Finnigan, T. J. A., Wall, B. T., Wilde, P. J., Stephens, F. B., Taylor, S. L., and Freedman, M. R. (2019). Mycoprotein: the future of nutritious nonmeat protein, a symposium review. Curr. Dev. Nutri. 3:nzz021. doi: 10.1093/cdn/nzz021
FutureBridge, F. B. (2020). Impact of COVID-19 on Plant-Based Meat Market. Available online at: https://www.futurebridge.com/industry/perspectives-food-nutrition/impact-of-covid-19-on-plant-based-meat-market/ (accessed January 27, 2021).
Gilani, G. S., and Lee, N. (2003). Protein Sources of Food-Grade Protein. Academic Press. doi: 10.1016/B0-12-227055-X/00834-8
Grasso, A. C., Hung, Y., Olthof, M. R., Verbeke, W., and Brouwer, I. A. (2019). Older consumers' readiness to accept alternative, more sustainable protein sources in the European union. Nutrients 11:1904. doi: 10.3390/nu11081904
Hallen-Adams, H. E., and Suhr, M. J. (2017). Fungi in the healthy human gastrointestinal tract. Virulence 8, 352–358. doi: 10.1080/21505594.2016.1247140
Halliwell, B., Cheah, I. K., and Tang, R. M. Y. (2018). Ergothioneine - a diet-derived antioxidant with therapeutic potential. FEBS Lett. 592, 3357–3366. doi: 10.1002/1873-3468.13123
Hashempour-Baltork, F., Khosravi-Darani, K., Hosseini, H., Farshidi, P., and Reihani, F. (2020). Mycoproteins as safe meat substitutes. J. Clean. Product. 253:119958. doi: 10.1016/j.jclepro.2020.119958
Hassan, M. A., Rouf, R., Tiralongo, E., May, T. W., and Tiralongo, J. (2015). Mushroom lectins: specificity, structure and bioactivity relevant to human disease. Int. J. Mol. Sci. 16, 7802–7838. doi: 10.3390/ijms16047802
Hatano, T., Saiki, S., Okuzumi, A., Mohney, R. P., and Hattori, N. (2016). Identification of novel biomarkers for Parkinson's disease by metabolomic technologies. J. Neurol. Neurosurg. Psychiatry 87, 295–301. doi: 10.1136/jnnp-2014-309676
Hellwig, C., Gmoser, R., Lundin, M., Taherzadeh, M. J., and Rousta, K. (2020). Fungi burger from stale bread? A case study on perceptions of a novel protein-rich food product made from an edible fungus. Foods 9:1112. doi: 10.3390/foods9081112
Henchion, M., Hayes, M., Mullen, A. M., Fenelon, M., and Tiwari, B. (2017). Future protein supply and demand: strategies and factors influencing a sustainable equilibrium. Foods 6:53. doi: 10.3390/foods6070053
Herforth, A., Arimond, M., Alvarez-Sanchez, C., Coates, J., Christianson, K., and Muehlhoff, E. (2019). A global review of food-based dietary guidelines. Adv. Nutr. 10, 590–605. doi: 10.1093/advances/nmy130
Ismail, I., Hwang, Y. H., and Joo, S. T. (2020). Meat analog as future food: a review. J. Anim. Sci. Technol. 62, 111–120. doi: 10.5187/jast.2020.62.2.111
Jadad, A. R., Moore, R. A., Carroll, D., Jenkinson, C., Reynolds, D. J., Gavaghan, D. J., et al. (1996). Assessing the quality of reports of randomized clinical trials: is blinding necessary? Contr. Clin. Trials 17, 1–12. doi: 10.1016/0197-2456(95)00134-4
Katz, L. A., Grant, J. R., Parfrey, L. W., and Burleigh, J. G. (2012). Turning the crown upside down: gene tree parsimony roots the eukaryotic tree of life. Syst. Biol. 61, 653–660. doi: 10.1093/sysbio/sys026
Lagashetti, A. C., Dufosse, L., Singh, S. K., and Singh, P. N. (2019). Fungal pigments and their prospects in different industries. Microorganisms 7:604. doi: 10.3390/microorganisms7120604
Li, S. S., Blanco Mejia, S., Lytvyn, L., Stewart, S. E., Viguiliouk, E., Ha, V., et al. (2017). Effect of plant protein on blood lipids: a systematic review and meta-analysis of randomized controlled trials. J. Am. Heart Assoc. 6:e006659. doi: 10.1161/JAHA.117.006659
Liang, C. H., Ho, K. J., Huang, L. Y., Tsai, C. H., Lin, S. Y., and Mau, J. L. (2013). Antioxidant properties of fruiting bodies, mycelia, and fermented products of the culinary-medicinal king oyster mushroom, Pleurotus eryngii (higher Basidiomycetes), with high ergothioneine content. Int. J. Med. Mushrooms 15, 267–275. doi: 10.1615/IntJMedMushr.v15.i3.40
Lin, S. Y., Chien, S. C., Wang, S. Y., and Mau, J. L. (2015). Submerged cultivation of mycelium with high ergothioneine content from the culinary-medicinal golden oyster mushroom, pleurotus citrinopileatus (higher basidiomycetes). Int. J. Med. Mushrooms 17, 749–761. doi: 10.1615/IntJMedMushrooms.v17.i8.50
Liu, J., and Liu, G. (2018). Analysis of secondary metabolites from plant endophytic fungi. Methods Mol. Biol. 1848, 25–38. doi: 10.1007/978-1-4939-8724-5_3
Matassa, S., Boon, N., Pikaar, I., and Verstraete, W. (2016). Verstraete, microbial protein: future sustainable food supply route with low environmental footprint. Microb. Biotechnol. 9, 568–575. doi: 10.1111/1751-7915.12369
Meyer, V., Basenko, E. Y., Benz, J. P., Braus, G. H., Caddick, M. X., Csukai, M., et al. (2020). Growing a circular economy with fungal biotechnology: a white paper. Fungal Biol. Biotechnol. 7:5. doi: 10.1186/s40694-020-00095-z
Moher, D., Liberati, A., Tetzlaff, J., Altman, D. G., and Group, P. (2009). Preferred reporting items for systematic reviews and meta-analyses: the PRISMA statement. PLoS Med. 6:e1000097. doi: 10.1371/journal.pmed.1000097
Monteyne, A. J., Coelho, M. O. C., Porter, C., Abdelrahman, D. R., Jameson, T. S. O., Finnigan, T. J. A., et al. (2020b). Branched-chain amino acid fortification does not restore muscle protein synthesis rates following ingestion of lower- compared with higher-dose Mycoprotein. J. Nutr. 150, 2931–2941. doi: 10.1093/jn/nxaa251
Monteyne, A. J., Coelho, M. O. C., Porter, C., Abdelrahman, D. R., Jameson, T. S. O., Jackman, S. R., et al. (2020a). Mycoprotein ingestion stimulates protein synthesis rates to a greater extent than milk protein in rested and exercised skeletal muscle of healthy young men: a randomized controlled trial. Am. J. Clin. Nutr. 112, 318–333. doi: 10.1093/ajcn/nqaa092
Naranjo-Ortiz, M. A., and Gabaldon, T. (2019). Fungal evolution: major ecological adaptations and evolutionary transitions. Biol. Rev. Camb. Philos. Soc. 94, 1443–1476. doi: 10.1111/brv.12510
Ntana, F., Mortensen, U. H., Sarazin, C., and Figge, R. (2020). Aspergillus: a powerful protein production platform. Catalysts 10, 1–29. doi: 10.3390/catal10091064
Pócsi, I., Prade, R. A., and Penninckx, M. J. (2004). Glutathione, altruistic metabolite in fungi. Adv. Microb. Physiol. 49, 1–76. doi: 10.1016/S0065-2911(04)49001-8
Prakash, P., and Namasivayam, S. (2014). Screening of bioactive compounds by Gc-Ms from fusarium venenatum. Int. J. PharmTech Res. 6, 1833–1837. Available online at: http://sphinxsai.com/2014/ph_vol6_no6/2/(1833-1837)%20014.pdf
Ruxton, C., and McMillan, B. (2010). The impact of mycoprotein on blood cholesterol levels: a pilot study. Br. Food J. 112:109. doi: 10.1108/00070701011080221
Sabaté, J., and Soret, S. (2014). Sustainability of plant-based diets: back to the future. Am. J. Clin. Nutr. 100 (Suppl 1), 476S–482S. doi: 10.3945/ajcn.113.071522
Schweiggert-Weisz, U., Eisner, P., Bader-Mittermaier, S., and Osen, R. (2020). Food proteins from plants and fungi. Curr. Opin. Food Sci. 32, 156–162. doi: 10.1016/j.cofs.2020.08.003
Smetana, S., Aganovic, K., Irmscher, S., and Heinz, V. (2018). Designing Sustainable Technologies, Products and Policies: From Science to Innovation, (Luxembourg).
Souza Filho, P. F., Andersson, D., Ferreira, J. A., and Taherzadeh, M. J. (2019). Mycoprotein: environmental impact and health aspects. World J. Microbiol. Biotechnol. 35:147. doi: 10.1007/s11274-019-2723-9
Souza Filho, P. F., Nair, R. B., Andersson, D., Lennartsson, P. R., and Taherzadeh, M. J. (2018). Vegan-mycoprotein concentrate from pea-processing industry byproduct using edible filamentous fungi. Fungal Biol. Biotechnol. 5:5. doi: 10.1186/s40694-018-0050-9
The Carbon Trust (2019). Quorn-Product Carbon Footprinting and Labeling. Available online at: https://www.carbontrust.com/our-clients/q/quorn-product-carbon-footprinting-and-labeling/ (accessed January 27, 2021).
Turnbull, W. H., Leeds, A. R., and Edwards, D. G. (1992). Mycoprotein reduces blood lipids in free-living subjects. Am. J. Clin. Nutr. 55, 415–419. doi: 10.1093/ajcn/55.2.415
Turnbull, W. H., Leeds, A. R., and Edwards, G. D. (1990). Effect of mycoprotein on blood lipids. Am. J. Clin. Nutr. 52, 646–650. doi: 10.1093/ajcn/52.4.646
Turnbull, W. H., Walton, J., and Leeds, A. R. (1993). Acute effects of mycoprotein on subsequent energy intake and appetite variables. Am. J. Clin. Nutr. 58, 507–512. doi: 10.1093/ajcn/58.4.507
Turnbull, W. H., and Ward, T. (1995). Mycoprotein reduces glycemia and insulinemia when taken with an oral-glucose-tolerance test. Am. J. Clin. Nutr. 61, 135–140. doi: 10.1093/ajcn/61.1.135
Udall, J. N., Lo, C. W., Young, V. R., and Scrimshaw, N. S. (1984). The tolerance and nutritional value of two microfungal foods in human subjects. Am. J. Clin. Nutr. 40, 285–292. doi: 10.1093/ajcn/40.2.285
Vega, K., and Kalkum, M. (2012). Chitin, chitinase responses, and invasive fungal infections. Int. J. Microbiol. 2012:920459. doi: 10.1155/2012/920459
Viguiliouk, E., Stewart, S. E., Jayalath, V. H., Ng, A. P., Mirrahimi, A., de Souza, R. J., et al. (2015). Effect of replacing animal protein with plant protein on glycemic control in diabetes: a systematic review and meta-analysis of randomized controlled trials. Nutrients 7, 9804–9824. doi: 10.3390/nu7125509
Wiebe, M. G. (2002). Myco-protein from Fusarium venenatum: a well-established product for human consumption. Appl. Microbiol. Biotechnol. 58, 421–427. doi: 10.1007/s00253-002-0931-x
Wiedeman, A. M., Barr, S. I., Green, T. J., Xu, Z., Innis, S. M., and Kitts, D. D. (2018). Dietary choline intake: current state of knowledge across the life cycle. Nutrients 10:1513. doi: 10.3390/nu10101513
Williamson, D. A., Geiselman, P. J., Lovejoy, J., Greenway, F., Volaufova, J., Martin, C. K., et al. (2006). Effects of consuming mycoprotein, tofu or chicken upon subsequent eating behaviour, hunger and safety. Appetite 46, 41–48. doi: 10.1016/j.appet.2005.10.007
Wu, G., Fang, Y. Z., Yang, S., Lupton, J. R., and Turner, N. D. (2004). Glutathione metabolism and its implications for health. J. Nutr. 134, 489–492. doi: 10.1093/jn/134.3.489
Keywords: fungal, mycellium, mycoprotein, evidence-base, understanding, food-based dietary guidelines, health
Citation: Derbyshire EJ and Delange J (2021) Fungal Protein – What Is It and What Is the Health Evidence? A Systematic Review Focusing on Mycoprotein. Front. Sustain. Food Syst. 5:581682. doi: 10.3389/fsufs.2021.581682
Received: 09 July 2020; Accepted: 13 January 2021;
Published: 18 February 2021.
Edited by:
Rakesh Bhardwaj, National Bureau of Plant Genetic Resources (ICAR), IndiaReviewed by:
Jennie Cecile Brand-Miller, The University of Sydney, AustraliaKathleen L. Hefferon, Cornell University, United States
Copyright © 2021 Derbyshire and Delange. This is an open-access article distributed under the terms of the Creative Commons Attribution License (CC BY). The use, distribution or reproduction in other forums is permitted, provided the original author(s) and the copyright owner(s) are credited and that the original publication in this journal is cited, in accordance with accepted academic practice. No use, distribution or reproduction is permitted which does not comply with these terms.
*Correspondence: Emma J. Derbyshire, ZW1tYUBudXRyaXRpb25hbC1pbnNpZ2h0LmNvLnVr