- 1Cereals Division, Council for Scientific and Industrial Research—Crops Research Institute, Kumasi, Ghana
- 2Resource and Crop Management & Socioeconomics Division, Council for Scientific and Industrial Research—Crops Research Institute, Kumasi, Ghana
- 3Department of Agroforestry, Kwame Nkrumah University of Science and Technology (KNUST), Kumasi, Ghana
There is an urgent need to match food production with increasing world population through identification of sustainable land management strategies. However, the struggle to achieve food security should be carried out keeping in mind the soil where the crops are grown and the environment in which the living things survive, especially under rainfed agricultural system. Rainfed agricultural ecosystem is extremely fragile, improving soil fertility and reducing greenhouse gas emission are key factors for developing sustainable agriculture. Moreover, society increasingly expects agriculture to be more sustainable, by decreasing irrigation and mineral fertilizer inputs. Increasing food production sustainably through efficient use of resources will strongly contribute to food security, sustainable agriculture development, and increased climate change resilience. This paper addresses the effects of carbon smart technologies on greenhouse gas emission, soil quality and crop productivity in rainfed agro ecological environment. This paper hypothesized that application of carbon smart technologies could improve soil physical and chemical properties to enhance GHG mitigation and crop production. Carbon smart technologies highlighted in this paper include minimum tillage, crop residue retention, agroforestry, biofuels, integrated nutrient management and land use management systems. This paper review and discusses the work done on carbon smart technologies in different agro-ecological regions so as to understand its impact from the perspectives of the soil, the crop and the environment. The impact of conservation agriculture on greenhouse gas emissions and the underlying mechanism in different agroecological environments have been discussed. A detailed case study and tremendous advancements on the strength of integration of trees and shrub as carbon smart technologies in improving soil and crop productivity is highlighted immensely. The paper concludes with recommendations for encouraging and improving adoption by smallholder farmers to ensure more efficient and sustainable food system. This systematic review will primarily contribute to the achievement of the following Sustainable Development Goals (SDGs), particularly SDG1 (No poverty), SDG2 (Zero hunger), SDG5 (Gender equality) and SDG13 (Climate action).
Introduction
A series of challenges face global food supply in the future, perhaps the most important of which are the combined effects of climate change and population increase. The regions where population increases are predicted to occur are those where there is the greatest food insecurity and where climate change is likely to make the situation worse (Riede et al., 2016; Lenton et al., 2019). In this region, farming systems are barely mechanized and are low input, without artificial fertilizer, plant growth regulators or pesticides, and are rainfed. Sustainable Development Goal (SDG) 2 envisages no hunger by 2030 and is perhaps one of the most difficult challenges that the world has set itself. It is also not enough to produce sufficient food, but the accessible nutritional value of the food produced must enable a healthy and active life and must be safe, as implicitly recognized in the UN definition of Food Security. Moreover, the process of producing sufficient food should not compromised on environmental quality and should be in line with efforts towards addressing SDGs 13 and 15 of climate action and life on land respectively. Yet, in many regions of Africa, including Ghana apart from the Green Revolution failing to have a major impact, crop production practice of moving from field to field on yearly basis in search of fertile soil couple with the effect of climate change would worsen the situation if innovative crop production approaches are ignored.
Climate change is negatively affecting crop production. For example, tomato yields and nutritional quality, is reducing, especially in the case of small holders that produce with minimal inputs. In West Africa, climate scenarios predict a temperature rise of up to 1.2 and 3.2°C by 2035 and 2100 respectively (Qin et al., 2014). Rainfall amounts and patterns will change with shifts in the start of the rainy seasons, most especially in the dryland areas (Adeniyi, 2016; Kim et al., 2017). Using trajectory and time series analysis of temperature anomalies and rainfall distributions for the agro-ecological zones of Ghana, there is strong evidence of local climate change across the country in diverse agro-ecological zones (Adu-Prah et al., 2019). Analysis shows local increases in temperatures for the period ranging from 0.5 to 1.0°C with varying inter-annual rainfall distributions (Adu-Prah et al., 2019) and more temperature extremes projected for the north of Ghana (Ofori-Mensah, 2017). In line with rising annual mean temperatures, the annual number of very hot days (days with daily maximum temperature greater than 35°C) is projected to rise substantially in the different agroecological zones of Ghana (Adjei and Kyerematen, 2018). Thus, projected changes in climate will pose threats to food production and food security, and hence to the livelihood of the people. Typical effects include reduced crop yield, and increased risks of crop failure (Centre for Indigenous Knowledge Organisation Development (CIKOD) Peasant Farmers Association of Ghana (PFAG), 2018). All these factors contribute to making smallholder farmers, particularly those in more ecologically fragile, risk prone agro ecological areas, more vulnerable to food and nutrition insecurity [Centre for Indigenous Knowledge Organisation Development (CIKOD) Peasant Farmers Association of Ghana (PFAG), 2018]. If care is not taken, the effort made in achieving SDG's 1 and 3 of No poverty and Good health and well-being respectively will be compromised.
Ghana's strong economic dependence on agriculture, and the sector's limited adaptive capacity underlines the country's high vulnerability to climate change, especially as <1% of the national crop area is irrigated (FAOSTAT, 2019), and crop yields depend on water availability and are susceptible to drought and heat stress. Staple crops, such as maize and rice, is traditionally rainfed, and yet are of great importance for food security. There is a risk of further intensification of these trends as climate change progresses and the demand for food increases as expected. The major food crops are predominately grown by smallholder farmers under rainfed conditions (www.worldbank.org) with low yield productivity. The low yield is primarily due to water and heat stress, but also due to declining soil fertility, and soil organic matter content; land degradation caused by reduced vegetative cover, soil and water erosion, and forest depletion (Burney et al., 2010). Fertilizer input to increase and stabilize crop yields has been increased by the launch of the Ghana's farm input subsidy programmes (FISP). However, in a recent study by Oppong et al. (2014) crop production data showed that there was no appreciable increase in the yields of the target crops; maize, rice, sorghum and millet. To a large extent, estimates of value-cost ratios suggest that fertilizer use among maize farmers in Ghana is not profitable, in some cases even under the subsidy. This situation poses a great threat to food and nutrition security of the people as well as the health of the environment. Mitigating climate change by changing agricultural practices has big potential (Adiku, 2015; Ahmed, 2016), and the mitigation potential in smallholder farming systems in the developing countries may be realized by implementing elements of climate smart agriculture (http://www.fao.org/climate-smart-agriculture/en/) and conservation agriculture (http://www.fao.org/conservation-agriculture/en/). The potential for carbon sequestration and increased carbon storage in degraded lands are particularly high and can partly be achieved by practices that also improve soil fertility e.g., application of nutrient amendments and organic substrates such as manures, bio-solids, and composts. There is therefore the need for adapting an approach that makes use of carbon smart technologies including conservation agriculture, agroforestry and other improved land used management practices.
The challenge of meeting the demand for food has received great attention worldwide. The current increases (doubled) in food production in the last four decades are due to a seven-fold increase in N fertilization (Shiferaw et al., 2013). However, the indiscriminate use of N fertilizer could result in negative effect on agriculture, socio-economic and environment such as global warming (Giles, 2005). For this reason, present concerns about agricultural and environmental sustainability have stimulated attempts to maximized crop yields while decreasing N input (Shiferaw et al., 2013). According to Snyder et al. (2009) high-yield agriculture has the potential to increase the annual input of crop residue C to soils. In this context, the ability to develop and implement innovative soil management practices plays an important role in maintaining or improving the productive capacity of soils and enhancing the resilience of the agroecosystem which is a key priority to maintain both the quality and quantity of crop production. The adoption of carbon smart technologies such as agroforestry, conservation agriculture, different land use management system and integrated nutrient management principles as part of a change in management system in combination with other sustainable soil management practices (Van den Putte et al., 2010) has been reported to increase crop productivity and carbon inputs (Huang et al., 2008; Yeboah et al., 2016). In an effort to reduce the concentrations of greenhouse gases (GHG) in the atmosphere in order to lessen the potential impacts on global climate, considerable attention has been paid to soil management practices. According to Snyder et al. (2009) cropland has the potential to reduce agricultural greenhouse gas emission by adopting improved soil management practices. Scientific soil management strategies can enhance soil quality and increase crop biomass production (Snyder et al., 2009). These strategies can be achieved by increased input of crop residues while minimizing C loses by erosion, decomposition and carbon emission. Whiles conservation agriculture systems has been noted to improve soil organic C (Andruschkewitsch et al., 2013), conventional plow based farming systems could accelerate carbon mineralization and thus reduce soil C content, which is attributed to soil aggregates disruption and increased oxidization through soil disturbances (Ussiri et al., 2009). According to Yeboah et al. (2016, 2018) conservation tillage combined with residue retention increased field pea yield by 12.5% and spring wheat yield by 14.0% vs. conventional tillage over four years of experiments. Apart from positive effects in both reducing emissions and increasing the sequestration of greenhouse gases, the application of carbon smart technologies will deliver immediate benefits. We postulate that, to counteract the obvious threat of food security and environmental degradation in sub-Saharan Africa, including Ghana, sustainable land use concepts and nature-based solutions that incorporate carbon smart technologies are urgently needed to ensure ecosystems, biodiversity and food security are preserved in the long term. Lastly, we consider future perspectives whether carbon smart technologies offers socio- economic opportunities for smallholder farmers. This paper hypothesized that application of carbon smart technologies could improve soil physical and chemical properties to enhance GHG mitigation and crop production. This proposal aligns perfectly with current national agricultural and climate change policies in SSA and with policies for agricultural developments in Ghana as described by Ministry of Food and Agriculture (http://mofa.gov.gh/site/index.php/about-us/about-the-ministry). The present systematic review presents progress towards the sustainable development goals (SDGs), particularly SDG1 “No poverty,” SDG2 “Zero hunger,” SDG5 “Gender equality” and SDG13 “Climate action” as it addresses the challenges of climate change for smallholder farmers in SSA.
Search Methods
The search methods were not strictly defined or focused in advance, the question for the review evolved and was refined throughout the initial phases of literature searching and data extraction. The research questions became “can carbon smart practices help in addressing problems of poor soil fertility, low crop yield, and high greenhouse gas emission?;” “which of the carbon smart practices is better in terms of improving soil and crop productivity, and maintaining environmental quality?;” “how does carbon smart practices improved soil, crop and environmental health compared to traditional cropping practices?,” “at what extent can carbon smart strategies reduce greenhouse gas emissions whilst improving soil and crop productivity?”. Population, Intervention, Comparison, Outcomes and Study (PICOS) design was used as a framework to formulate eligibility criteria in the systematic reviews (Figure 1).
As the starting point for the synthesis the databases MEDLINE (via EBSCO), CINAHL Complete (via EBSCO), were searched between November 2020 to March 2021. Five key concepts were used for the search; soil and crop productivity; greenhouse gas emission; conservation agriculture; agroforestry for soil and crop production; soil carbon sequestration.
Studies from countries with similar economic and cultural situations and levels of agriculture development were included, using the following criteria: published in English; published between 1985 and 2021 and studies in high income countries: UK, Western Europe, USA, Canada, Australia and New Zealand. The year 1985 was identified as a limit, as this coincides with the period of immense interest in sustainable agriculture.
Quality Appraisal
The criteria proposed by Dixon-Woods et al. (2006), for assessing the quality of all empirical papers for Center for Internet Security (CIS), regardless of study type, were used. These have been adapted from the National Electronic Library for the evaluation of qualitative research.
Minimum Tillage for GHG Mitigation
Soils play an important role in climate change mitigation by storing carbon and decreasing global greenhouse gas emissions in the atmosphere (Lal, 2004). However, poor soil management through unsustainable agricultural practices could release nitrous oxide (N2O), methane (CH4) and carbon dioxide (CO2) into the atmosphere (IPCC, 2013). The adoption of sustainable management of soil resources aims at increasing soil quality favorable for mitigating greenhouse gas emissions (Sharma et al., 2011). The impact of carbon smart strategies on greenhouse gas emission and the mechanism causing emissions has been diverse. It is prudent that a systematic review is conducted to understand the influence of carbon smart strategies on GHG emission and the underlying mechanism in different agro ecological environments.
Rainfed N2O Emissions
According to Saggar et al. (2010) N2O emissions are driven by the applications of fertilizer nitrogen (N), soil tillage and crop type, with their effect dependent on soil and weather conditions. Different results have been reported regarding the influence of tillage and straw practices on N2O flux. Some research has shown that conservation tillage, especially no–tillage, results in increased N2O emissions relative to intensive tillage systems (Hermle et al., 2008). Increased denitrification under reduced tillage has been attributed to decreased water filled pore space and mineral nitrogen concentration (Oorts et al., 2007), reduced gas diffusivity and increased water and reduce soil bulk density content (Hermle et al., 2008). Minimum conservation tillage maintains higher soil moisture levels and surface soil organic matter than where more intensive tillage practices are used. In addition, Bhatia et al. (2010) noted that the emissions of N2O were higher in minimum till vs. conventional till system since soils under minimum tillage were generally more moist and had organic matter concentrated near the soil surface, which favored N2O production. Other studies have observed no difference in N2O emissions between minimum and conventional tillage treatments (Choudhary et al., 2002). However, no–tillage system has been reported to produce less N2O than that of conventional tillage (Duan et al., 2013). This has been attributed to conventional tillage causing more soil organic C decomposition due to higher levels of soil–residue mixing and higher soil temperatures (Duan et al., 2013). In a study conducted by Yeboah et al. (2016) reported that N2O emissions were comparatively higher under conventional tillage treatments soils than those from no–till or minimum tillage treatments with straw retention (Figure 2). The low N2O emission in the minimum tillage plots is therefore, a significant finding in this study as many other agricultural practices that are meant to limit CO2 emission also increase N2O emissions, which does not seem to be the case in this review.
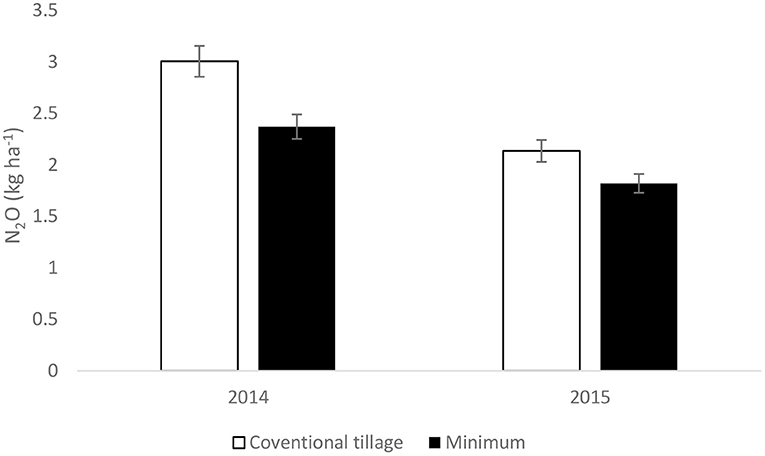
Figure 2. Cumulative N2O emissions in spring wheat as affected by conventional tillage (hollow bars) and minimum tillage (filled bars). Data re-plotted from Yeboah et al. (2016).
These data are in accord with those reported by Chatskikh and Olesen (2007), but contrary to reports by Gregorich et al. (2008) who found that N2O emissions can be higher from no-tillage when compared to conventional tillage plots. The authors attributed the decreased N2O emission by improving soil water drainage and soil structure, as shown by soil bulk density and soil hydraulic conductivity. This result support the assertion that increasing soil carbon stock and reducing soil disturbances could help reduce N2O emission in agricultural systems. In this regards, application of conservation agriculture practices, including minimum tillage and increased residue incorporation has a higher potential to reduce nitrous oxide emission and to promote low emission cropping system.
Rainfed CH4 Emissions
Most previous studies indicate that conservation tillage couple with appropriate straw application acts as a net sinks for methane. However, both increased and decreased CH4 consumption has been reported in no-till soils (Venterea et al., 2005). Changes in land use, especially cultivation of formerly undisturbed soils, strongly decrease the CH4 oxidation and consequently the uptake of atmospheric CH4 by the soil (Hütsch, 1998). Reduced and no-tillage practices have been proposed as alternative system for restoring the CH4 uptake capacity of soils (Hütsch, 2001). This is because the improvement of soil quality through these practices is beneficial to methanotrophs (Hütsch, 1998, 2001). Agricultural soils exhibit both minor emitters of CH4 to sinks for atmospheric CH4 (Mosier et al., 2006). Agricultural soils become sinks of CH4 when methanotrophic bacteria take up CH4 to oxidize it for energy production (Hütsch, 1998). There is evidence that tillage reduces this oxidation in soils leading to less CH4 removal (Hütsch, 1998). In a study by Yeboah et al. (2016), greater uptake of CH4 was observed in the minimum till soils under rainfed conditions (Figure 3).
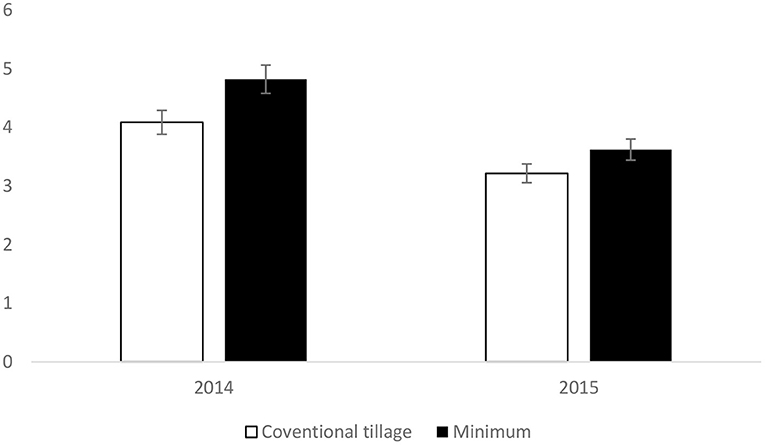
Figure 3. Cumulative CH4 emissions in spring wheat as affected by conventional tillage (hollow bars) and minimum tillage (filled bars). Data re-plotted from Yeboah et al. (2016).
The greater uptake of methane in the no tillage plots, especially with straw application may be due to better aeration and less soil degradation which enhanced methanotroph activity. A well-drained soil provides ideal environment for the methanotrophs which are key bacteria in enhancing CH4 uptake. The mulch layer on top of minimum tillage soils provided soil organic carbon and strengthened the stability of soil aggregates making them resist soil erosion caused by water and wind. When eroded soil particles do not fill pore spaces, porosity is increased and bulk density decreases. McLain and Martens (2006) noted that methanotroph activity was enhanced under adequate diffusion of gases in the niche of microbial activities. Plowing disrupts the ecological niche for methanotrophic bacteria, influence the gaseous diffusivity, and affect the rate of supply of atmospheric CH4 (Hütsch, 1998). Straw returned in no-till system tends to increase soil C and reduce soil density, which may lead to lower risk of CH4 emissions. Mosquera-Losada et al. (2007) reported that soil degradation can reduce the ability of soils to consume or oxidize atmospheric CH4 by as much as 30–90%. However, Omonode et al. (2007), hold a contrary view to the above indicating that anaerobic conditions are prevalent under no-tillage and consequently lead to CH4 emission. The high potential of conservation tillage in reducing methane emission is significant since current increases in atmospheric GHG levels require that innovative strategies are undertaken to mitigate impacts of climate change, particularly management practices capable of improving soil C sequestration.
Rainfed CO2 Emissions
Crop residue retention is related to the increase in organic C concentration and thus reduces CO2 emission (Zhang et al., 2013). Sequestration of C and N in soils could be achieved through adoption of conservation tillage methods and crop residue retention (Zhang et al., 2013). Minimum tillage retains more plant residue on the soil surface and has greater near–surface soil C contents than conventional tillage (Lal, 2009). The decomposition of plant residue is also slower in conservation tillage as a result of reduced soil–residue contact compared with residue that is completely incorporated by conventional tillage (Lal and Pimentel, 2009). According to Reicosky and Archer (2007) the amount of C lost in the form of CO2 due to soil tillage practices is depended on tillage intensity volume of soil disturbed. Lower CO2 emissions were observed in minimum tillage plots with residue (Yeboah et al., 2016, 2018) as shown in Figure 4.
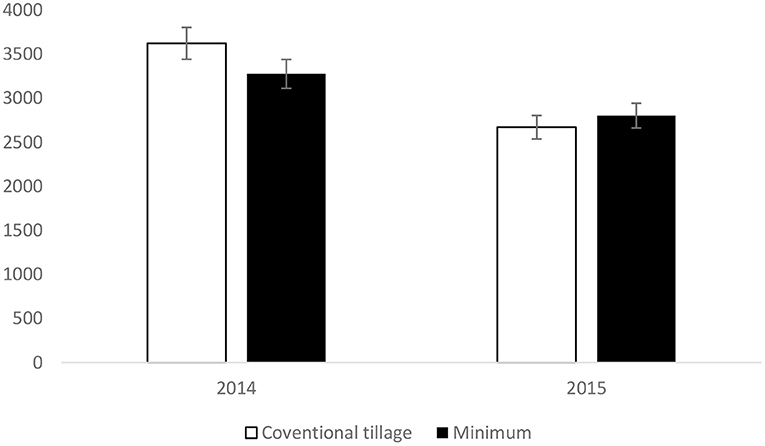
Figure 4. Cumulative CO2 emissions in spring wheat as affected by conventional tillage (hollow bars) and minimum tillage (filled bars). Data re-plotted from Yeboah et al. (2016).
The lower CO2 emissions could be attributed to the significant improvement in the soil organic carbon from the residue retention, which has been reported by other researchers (Zhang et al., 2013; Wang et al., 2020; Tiefenbacher et al., 2021). There is a general consensus that no–till with straw covering on the soil surface decreases soil CO2 emissions, as suggested by Andruschkewitsch et al. (2013), soil disturbances due to tillage increase organic matter contact with microorganism leading to rapid decomposition. Higher CO2 emissions in conventional tillage soils, especially with residue removal was attributed to the increased surface roughness and pores that are created by soil disturbance that accelerate decomposition of SOM (Ussiri et al., 2009). The aggregates 'disruption due to tillage renders the initially protected organic matter (OM) accessible to decomposers. Higher CO2 emissions in conventional treated soils was attributed to the increased surface roughness and voids that are created by soil disturbance that accelerate. Conservation tillage enhance residue cover on the soil surface and have greater upper surface soil C contents than conventional tillage, the decomposition of plant residue is slower in conservation tillage as a result of reduced soil–residue contact compared with residue that is completely incorporated by conventional tillage (Lal, 2009). The review has highlighted the use of minimum tillage to reduce GHG (N2O; CO2; CH4) emissions resulting from agriculture practices. For smallholder famers, there is a unique advantage for encouraging the use of minimum tillage. This because it can easily fit into their farming operations since cultivation and farming operations are on small scale and normally does not involve sophisticated farm implements.
Minimum Tillage and Crop Productivity and C Balance
Previous researches have shown that conservation tillage can improve crop yields (Huang et al., 2008; Zhang et al., 2013). In a study by Yeboah et al. (2016, 2018) minimum tillage couple with straw retention soils improved wheat grain yield by 49.78% on average compared to conventional tillage system with residue removed (Figure 5). The differences in grain yield could be related to the improved soil quality (Huang et al., 2008; Zhang et al., 2013). A possible explanation could be that minimum tillage with residue retention promoted wheat growth by increasing soil water availability and lowering bulk density that enhance root penetration.
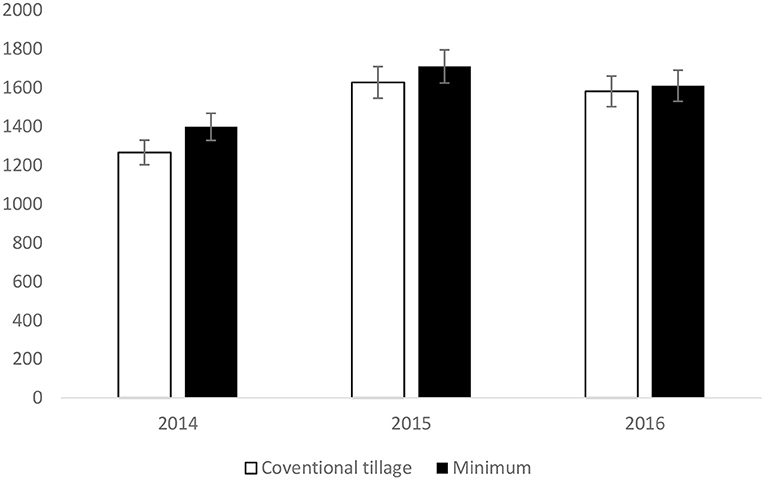
Figure 5. Grain yield in spring wheat as affected by conventional tillage (hollow bars) and minimum tillage (filled bars). Data re-plotted from Yeboah et al. (2016).
In the Mexican highlands improved high-yielding wheat varieties yielded double under conservation agriculture compared to the farmer practice or zero tillage with residue removal, all with the same fertilizer inputs (Govaerts et al., 2005). However, Lampurlanes et al. (2002) found no difference among tillage systems in crop yield. There was a direct and significant relation between the soil quality status of the soil and the crop yield, and no tillage with crop residue retention showed the highest crop yields as well as the highest soil quality status. In contrast, the soil under no tillage and conventional tillage with crop residue removal showed the lowest soil quality and thus produced the lowest yields. This is in line with other studies, for instance Ozpinar and Cay (2006) found that wheat grain yield was greater when tillage practices resulted in improved soil quality. Management strategies in agroecosystems may influence C balance in soil through differences in soil C input and soil C output (Ghoshal and Singh, 2010). In agricultural system when C input to the soil exceeds the C output from the soil, a positive imbalance occurs which subsequently results in C sequestration in soil (Mukherjee and Lal, 2015). Minimum tillage and straw application significantly have been noted to enhanced soil C balance (Figure 6).
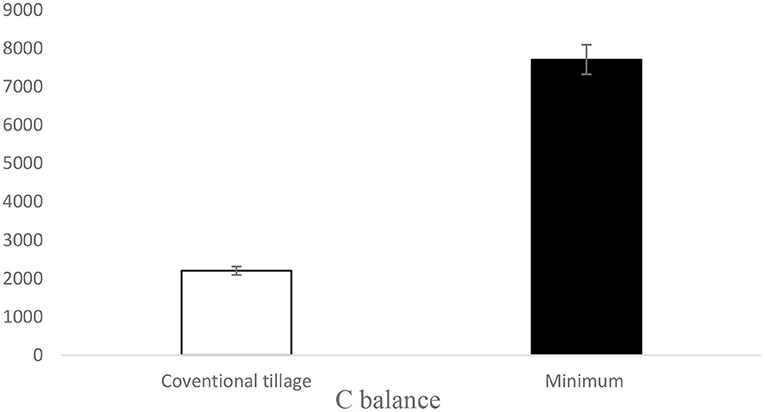
Figure 6. Grain yield in spring wheat as affected by conventional tillage (hollow bars) and minimum tillage (filled bars). Data re-plotted from Yeboah et al. (2016), thesis, unpublished.
Zhang et al. (2012) found the beneficial role of straw returned for C sequestration. When C inputs and outputs are in balance with one another, there is no net change in soil C levels. Also, straw treated plots had higher C sequestration potential in terms of soil C balance particularly that of tillage removal with residue retained plots. On the other hand, soils without carbon inputs with or without tillage treatment had negative C balanced. The increased in annual C inputs could translate into higher C storage in terms of soil C build-up and thus enhanced C sequestration.
Agroforestry
Agroforestry Impact Soil Nutrients and Crop Productivity
Africa just as the whole world faces the change of feeding increasing population against limited land resources in the face of climate change. Technologies that enable improved and sustainable intensification of crop production will be a way forward. The loss of soil organic carbon (SOC) and soil nutrients especially N has been observed to be the most limiting factor of crop production in the tropics and sub-Saharan Africa (Zingore et al., 2015; Liu et al., 2021). Using a crop model Liu et al. (2021) observed that, as a result of N deficiency, the yield of yam a very important food security crop along the West African yam belt including Ghana was limited for 69, 77, 82, and 92% land area at the savannah, forest, forest-savannah transition and coastal savannah respectively of Ghana. Also, APSIM showed that lack of synchronization of sowing date of maize with optimum radiation, rainfall, and nutrients (especially N) is the cause of the huge maize yield gap in Ghana (Owusu Danquah et al., 2020). For rice production, N is the most limiting nutrient followed by Phosphorus (P). The absence of N, P, and Potassium (K) resulted in a yield reduction of 32, 16, and 11%. The study suggested N application of 56, 91, and 122 kg N ha−1 would result in a yield of 3, 5, and 7 t ha−1 respectively (Saito et al., 2019). An on-station and on-farm evaluation on cassava in Uganda and Kenya revealed poor soil fertility as one of the major limiting factors. Cassava productivity was limited as much as 6.7 t ha−1 compared to 5.4 and 5.0 t ha−1 limitations resulting from early water stress and weed management respectively (Fermont et al., 2009). The yield gap of food crops improved with the use of fertilizers and improved seeds. Also, the yield and poverty gap are directly related, and thus yield improvement would reduce poverty and improve farmers' livelihoods (Dzanku et al., 2015). To ensure food security in the face of limited land resources and climate change for the increasing population calls for urgent need to address these yield limitations with improved climate smart technologies. Mueller et al. (2012) observed a high chance of meeting sustainable intensification and food security challenges if changes can be made in soil nutrition and water management. Environmental impact as results of agriculture can be minimized whiles increasing important and major cereals such as maize, wheat, and rice by 30%. This is where agroforestry can play a vital role by providing options that enable efficient and sustainable use of the resources whiles conserving them for future generations. Agroforestry is a collective name for land use practice which incorporate growing trees and shrubs with crops and or livestock so that they interact to facilitate productivity (Nair et al., 2010). This practice has recently received considerable attention as a biological strategy for carbon sequestration for mitigating greenhouse gas emissions recognized by the Kyoto Protocol. This is because interactions between trees and shrubs used in agroforestry systems are managed to facilitate the growth of the associated food crop.
Agroforestry Facilitate Resource Use in Cropping Systems
Agroforestry practice involves the cultivation of more than one plant species on the same piece of land, at least two plant species or more. The selection of the species is very important and relies on the ecological principles of reducing competition and maximizing complementation or facilitation (Erskine et al., 2006; Rao et al., 2007). Competition is where the two or more plant species used in the agroforestry system interact in such a way that at least one exerts a negative influence on the growth and development of the other species. Whiles complementation or facilitation is where the two or more plant species used in the agroforestry system interact in such a way that it exerts a positive influence on at least one or more of the species. When all the species exert positive influence on each other it is termed mutualism (Van Noordwijk et al., 2015). However, in agroforestry systems mutualism is hardly attained. Figure 7 shows how the presence of trees/shrubs in cropping systems facilitates resource use to the benefit of the associated food crop. Yang et al. (2020) observed that rubber tree takes more than 40% of its soil water from the shallow horizon (0–20 cm) of the soil resulting in an interspecific competition between rubber tree and their intercrop. However, the intercrop facilitated soil water to the rubber enabling it to acquire about 9–24% of its soil moisture requirement from the shallow soil horizon (0–20 cm).
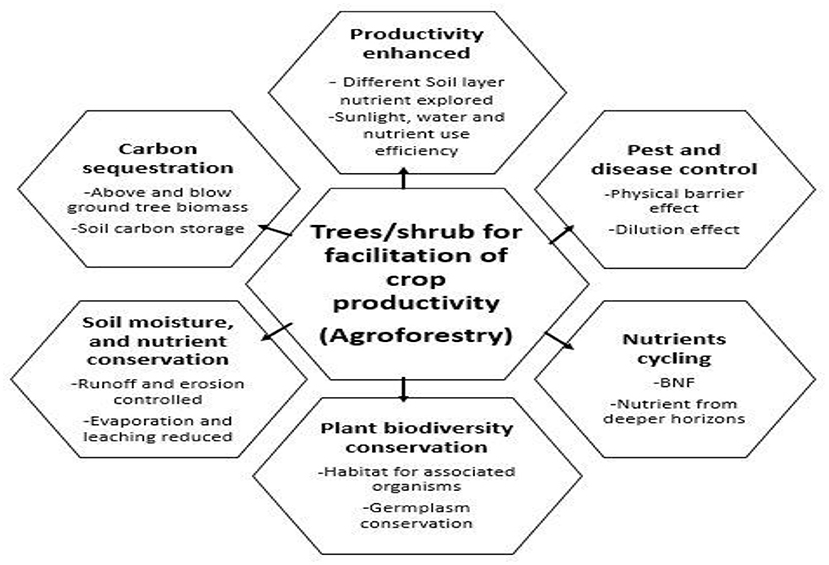
Figure 7. Integration of trees and shrubs into cropping systems for facilitation of resource use and crop productivity. Source: adopted from Malézieux et al. (2009).
Species diversity in an ecosystem is directly related to productivity and income per plant in the plant ecosystem. The income per plant increased for fruits, firewood, and timber but decreased for bananas and cocoa as diversity increases. This suggests stronger complementarity than competition between plants on the higher strata of the canopy (fruits, firewood, and timber trees) and vice versa for the plants occupying the lower canopy (Banana and Cocoa) (Salazar-Díaz and Tixier, 2019). This study has emphasized the need for the selection and arrangement of agroforestry components to achieve optimum benefits. When the management and spatial influence of tree species such as Eucalyptus spp., Sesbania sesban, Grevillea robusta, Calliandra calothyrsus, Markhamia lutea, and Croton macrostachyus were evaluated on water availability and productivity of maize on smallholders' farms in Kenya, it was observed maize productivity increased with leguminous species. Also, the presence of the tree species significantly influenced soil moisture distribution at the field (Nyaga et al., 2019). Moderate shading by trees in a cocoa farm resulted in improved nutrient assimilation and productivity of cocoa (Asare et al., 2017).
Using pigeonpea in cropping systems has provided readily available and rich N biomass which improved N availability and used resulting in improved crop productivity (Kermah et al., 2018; Owusu Danquah, 2020). Figure 8A shows the sunlight reaching yam leaves above, mid, and below the canopy along the stake. Sunlight reaching the yam leaves was influenced by the cropping system. Sunlight intensity on yam leaves at mid-canopy (MC) of PB and sole yam were similar to the sunlight intensity on the yam leaves above canopy (AC) of PA for both locations and years. Sunlight reaching the below canopy (BC) of both PB and Sole yam cropping systems was higher than the sunlight reaching the mid-canopy (MC) of PA fields. This had implications on soil moisture retention, weed control, and N use by the yam. Although the yam leaves of the yam in the PA field were shaded, this facilitated soil moisture retention, control weeds, and improved N use by the yam. Figure 8B shows the mean relative chlorophyll content of the yam leaves. Yam leaves on PA fields had the best leaf chlorophyll content. Farmers' practice (No fertilizer) and half recommended fertilizer rate (23–23–30 N–P2O5-K20 kg/ha) followed the order of PA>PB> sole yam in leaf chlorophyll content whiles the full fertilizer rate (45–45–60 N–P2O5-K2O kg/ha) followed the order of PA=PB>sole yam.
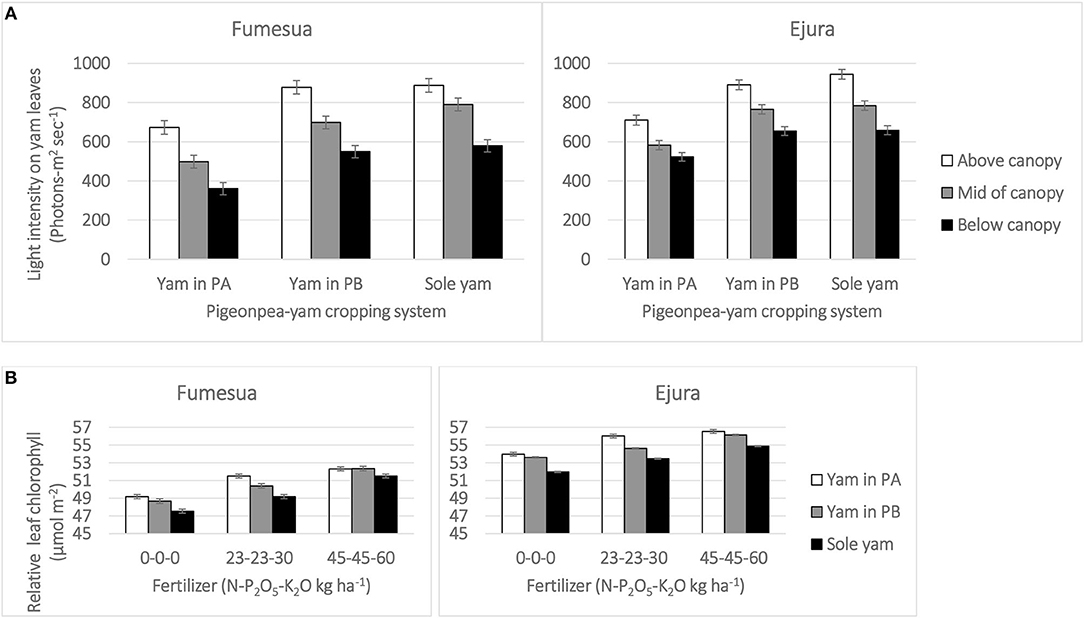
Figure 8. (A) Mean biweekly sunlight photons reaching yam leaves in pigeonpea–yam cropping system. PA, Pigeonpea in alley; PB, Pigeonpea as border. Source (Owusu Danquah 2020). (B) Mean biweekly relative leaf chlorophyll content of yams in pigeonpea–yam cropping system. PA, pigeonpea in alley; PB, pigeonpea as border. Source: Owusu Danquah (2020).
Thus, the presence of the pigeonpea though shaded the yams, shading was positive, it protected the yam ridges from erosion, suppressed weeds, made N available to the yams facilitating yam productivity. As a C3 plant species, it becomes saturated upon receiving 50% of the required light intensity. High environmental temperature conditions around crops especially C3 plants increases oxygenation reaction along the photorespiratory pathway resulting in about 25–30% loss in carbon fixation (Slattery and Ort, 2019). Thus, proving some level of shading is necessary for staple food crops (root and tuber and legume food crops) which are C3 plants so as to operate under full photosynthetic potential for improved productivity (Huang et al., 2020; Owusu Danquah, 2020). In the face of climate change integrating trees into cropping systems on smallholder farmers would play a dual role of shading and conserving moisture and also provide food and income from their produce.
If the trees and shrubs used fixes N through biological nitrogen fixation (BNF) it will improve N availability. This strategy of intentionally using legumes trees and shrubs would facilitate N availability in cropping systems to reduce N inorganic fertilizer application. Since N fixation and availability to the associated crop is a factor of the biomass of the legume grown, the N per unit area fixed by tree legumes are better than major crop legumes used in cropping systems (Mthembu et al., 2018). Also, N fixation can be high when N in the medium or soil is limited (Ennin et al., 2002). Thus, it presents the opportunity to integrate tree legumes into cropping systems in sub-Saharan Africa where the soils are limited in N and other nutrients for sustainable food crop production. Owusu Danquah et al. (2017) observed that when pigeonpea (a legume tree) preceded yam cultivation, half recommended poultry manure (3 t ha−1) and a third recommended fertilizer rate (15–15–20 N–P2O5-K2O kg ha−1) was enough for sustainable yam production on continuously cropped fields. Also, when yam was cultivated in alleys of pigeonpea and the pigeonpea biomass pruned on the soil, half the recommended fertilizer rate (23–23–30 N–P2O5-K2O kg ha−1) was enough for sustainable yam production on continuously cropped fields (Owusu Danquah, 2020).
Improving Productivity and Sustainable Land Use
Integration of trees and shrubs in cropping systems improves the general productivity of the cropping systems compared to their monocrop. This is because the presence of the trees and shrub makes available nutrient-rich biomass which improves nutrient and water use efficiency to the benefit of the associated food crop (Ribeiro-Barros et al., 2018; Kuyah et al., 2019; Akoto et al., 2020). Tsufac et al. (2021), evaluated the role of agroforestry as a sustainable agricultural practice option for soil fertility management in Cameroon. The study revealed a significant improvement in soil with agroforestry practice which was also perceived by most farmers. The productivity of maize significantly improved when integrated with pigeonpea (Musokwa et al., 2019). The productivity and profitability of cowpea, maize, and cassava significantly improved over their monocrop counterpart upon integration with bamboo (Akoto et al., 2020). The integration of Agroforestry trees such as Gliricidia sepium and Leucaena leucocephala into yam cropping system improved yam productivity (Maliki et al., 2017). Table 1 shows the land Equivalent Ratio (LER) and improvement in pigeonpea–yam intercrop compared to their monocrops. More than one (1) LER indicates the significant improvement of yam productivity resulting from the integration of pigeonpea. When yam was planted in alleys of pigeonpea (PA) with pigeonpea as live-stakes, the productivity was better than when yam was planted with the borders of pigeonpea (PB) and pigeonpea stakes cut and used as stakes. Intercropping efficiency of 39–69 and 27–44% were observed for planning yam in alleys of pigeonpea and planning yam with pigeonpea as border respectively. Thus 27–69% land area of the monocrop yam would be needed to achieve a similar yield as the pigeonpea–yam intercrop. This has implications on forest conservation and land use management especially for yam which is associated with deforestation and land degradation (Ennin et al., 2014; Owusu Danquah et al., 2015). According to the report of (Ministry of Food Agriculture (MoFA), 2018), yam production in Ghana increased by 2.39% while the area under yam cultivation within the same period also increased by 2.21 suggesting about 1:1 direct relation between area under cultivation and production. This means yam production in Ghana increases as areas under production increase. This has bad implications for land resource conservation and climate change.
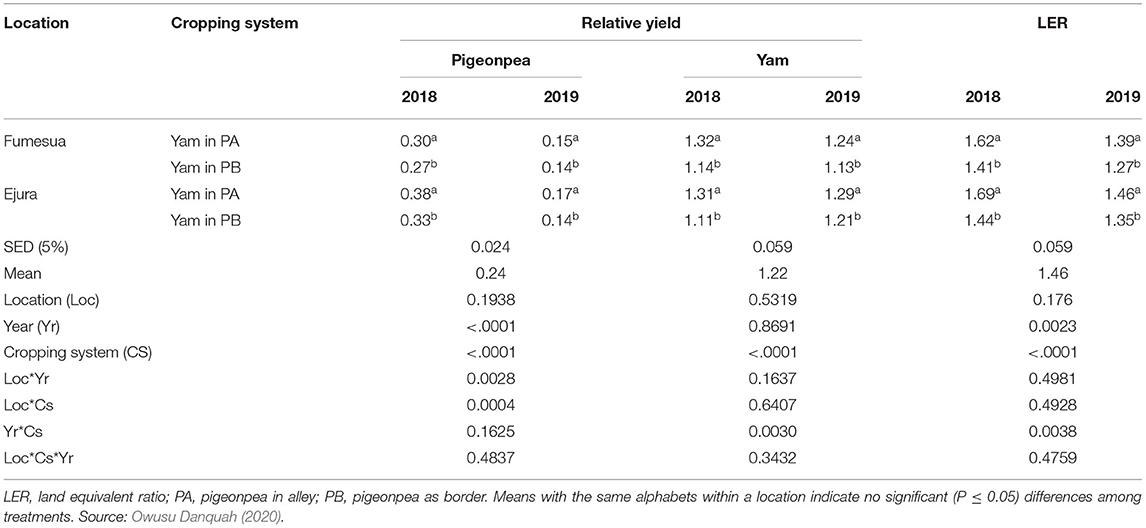
Table 1. The land equivalent ratio (LER) of the pigeonpea–yam cropping system at Fumesua and Ejura for 2018 and 2019 cropping seasons.
The resource use efficiency and productivity increase associated with the integration of trees and shrubs has demonstrated agroforestry's ability to improved and sustained productivity whiles conserving the resources on which production depends (the land). Promotion and adoption of the pigeonpea–yam cropping system as an improved technology option for yam production in Ghana would at least conserved about 27% of the land currently under yam production without affecting production. This is also welcoming since to be able to mitigate climate change, forest and tree conservation would be needed as carbon sinks.
Integration of Trees and Shrubs on Farmlands Facilitates SOC Storage
The increasing greenhouse gases (GHG) (Carbon dioxide, nitrous oxide, methane) in the atmosphere continues to be a challenge and result of global climate change. The warmest earth's surface temperature has been recorded since 2016 resulting in extreme weather conditions and its adverse effect on crop production (Gupta et al., 2017; Jones, 2017). There is, therefore, the need to pursue climate-smart technologies that increase carbon storage especially for smallholder farmers who have been predicted to be worse affected by climate change due to their limited adaptive capacity (Manaye et al., 2021). Agroforestry has been observed to be one of the low costs and sustainable technologies for mitigating climate change. This is because of its ability to provide many ecosystem services which improve agroecosystem biodiversity and productivity (Goncalves et al., 2021). Global agricultural land is about 10% with a carbon stock of about 3–18 t C ha−1 (Zomer et al., 2009). Agroforestry could sequester about 0.29–15.21 and 30–300 Mg C ha−1 year−1 carbon in the biomass above and below ground (soil) respectively (Nair et al., 2009; Nath et al., 2021). Brown et al. (2012) observed that agroforestry systems in East and West Africa alone has the potential to store about 6–22 Mg CO2 ha−1 year−1. Manaye et al. (2021) evaluated tree diversity and carbon stocks in agroforestry systems in Ethiopia and observed a high tree species diversity and potential above and below ground carbon stock of about 77–135 Mg ha−1 on smallholder farmers' fields. The study suggested, agroforestry to be a very important means of storing carbon. Figure 9 shows SOC loss from the Forest, transition, savannah, and coastal soils of Ghana when subjected to pigeonpea residue incorporation in the soil, pigeonpea rotation with yam, and use of sole inorganic fertilizer. Long-term (10 years) stimulation of soil organic matter showed a decline in SOC on all treatments. However, the decline was least when pigeonpea biomass was incorporated (−0.3 Mg ha−1 year−1) followed by when sole fertilizer (−0.43 Mg ha−1 year−1) and pigeonpea rotation with yam (−0.42 Mg ha−1 year−1) with the control of sole inorganic fertilizer recording the worse decline in SOC of −0.51 Mg ha−1 year−1 (Liu et al., 2021).
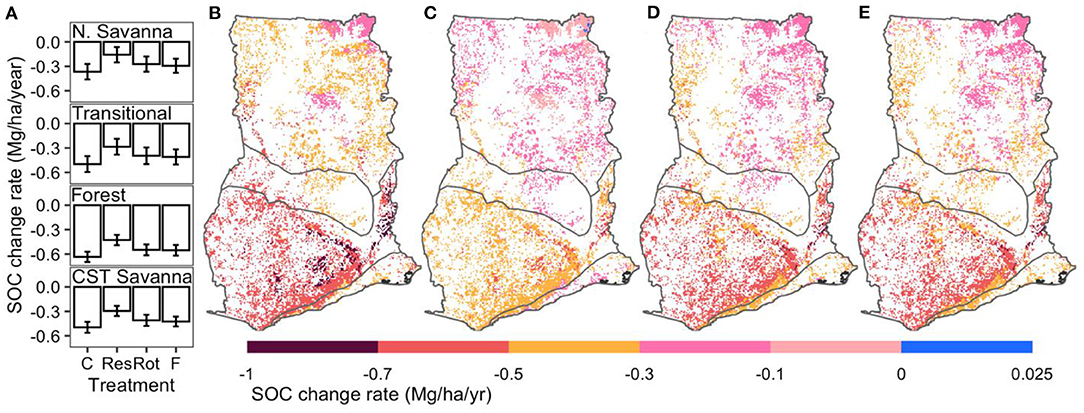
Figure 9. Response of soil organic carbon (SOC) change rate to management treatments across Ghana. (A) Average SOC change rate by agroecological zone under the four treatments, and (B–E) SOC change rate under the four respective treatments: control, pigeonpea residue incorporation, pigeonpea–yam rotation, and yam with fertilizer addition. N. Savanna, northern savanna; CST savanna, coastal savanna; C, control; Res, residue; Rot, rotation, and F, fertilized. Source: Liu et al. (2021).
Under the clean development mechanism (CDM), the approach of afforestation and conservation of forest to serve as carbon sinks is seen as a hindrance to the agenda of increasing food production to feed the increasing population (Apuri et al., 2018; Waldén et al., 2020). Integration of trees and shrubs on farmlands addresses this issue, food production can be sustained whiles maintaining the trees and shrubs to serve as carbon sinks on the same piece of land.
Integrated Soil Fertility Management (ISFM) in the Face of Climate Change
The productivity of crop depends very much on the fertility of the soil. Therefore, improving soil fertility is key to improving smallholder farmers' productivity, income, and livelihood. Soil fertility is on a decline in sub-Saharan African (Nalivata et al., 2017; Stewart et al., 2020). There is a yield gap of about 4.9, 4.5 t ha−1 between potential and actual yields of maize and rice respectively (Rong et al., 2021). According to the [Global Yield Gap and Water Productivity Atlas (GYGA), 2021] (GYGA-www.yieldgap.org), maize a very important food security crop, yield under rainfed conditions is just about 10–30% of the potential yield. Soil nutrition and precipitation have been observed to be the major limiting factors affecting cereals and other crop yields in sub-Saharan Africa (AGRA, 2016; Hadebe et al., 2017; Kihara et al., 2017; Saito et al., 2019; Epule et al., 2021). Fertilizer usage was promoted to address the situation, but the inability of smallholder farmers to afford mineral fertilizers hampers fertilizer usage (Komarek et al., 2017; Patrick et al., 2018). Also, the ability to transport to their fields because of the poor road network and technical know-how in the application and usage of fertilizers are other reasons (Mugwe et al., 2019; Langyintuo, 2020).
The use of fertilizer alone does not promote long-term soil organic carbon buildup, soil fertility, and crop productivity (Raimi et al., 2017; Singh, 2018; Singh et al., 2019). Although, organic fertilizer especially plant biomass has been noted to promote soil health by building soil organic matter, getting sufficient quantities for sustainable soil fertility and food production is a challenge (Place et al., 2003; Biramo, 2018). Therefore, Integrated Soil Fertility Management (ISFM) approach which combines organic and inorganic fertilizers are being promoted as a viable option for sub-Saharan Africa. Integrated Soil Fertility Management (ISFM) is a soil fertility management practice where organic, inorganic (fertilizer), and improved germplasm are combined and adapted to the local conditions resulting in improved nutrient use efficiency and crop productivity (Vanlauwe et al., 2010; Mugwe et al., 2019; Gram et al., 2020). The combined use of organic and Inorganic fertilizers in ISFM resulted in improved productivity of most crops such as maize (Mahmood et al., 2017), Rice (Moe et al., 2017), Tomatoes (Islam et al., 2017), and Yam (Owusu Danquah et al., 2017, 2020). The use of ISFM reduces the inorganic fertilizer requirement whiles improving crop productivity. Figures 10A,B indicates a third and half recommended fertilizer rate of 45–45–60 N–P2O5-K2O kg ha−1 (Ennin et al., 2016) with integration of pigeonpea biomass would be enough for sustainable yam production on continuously cropped fields.
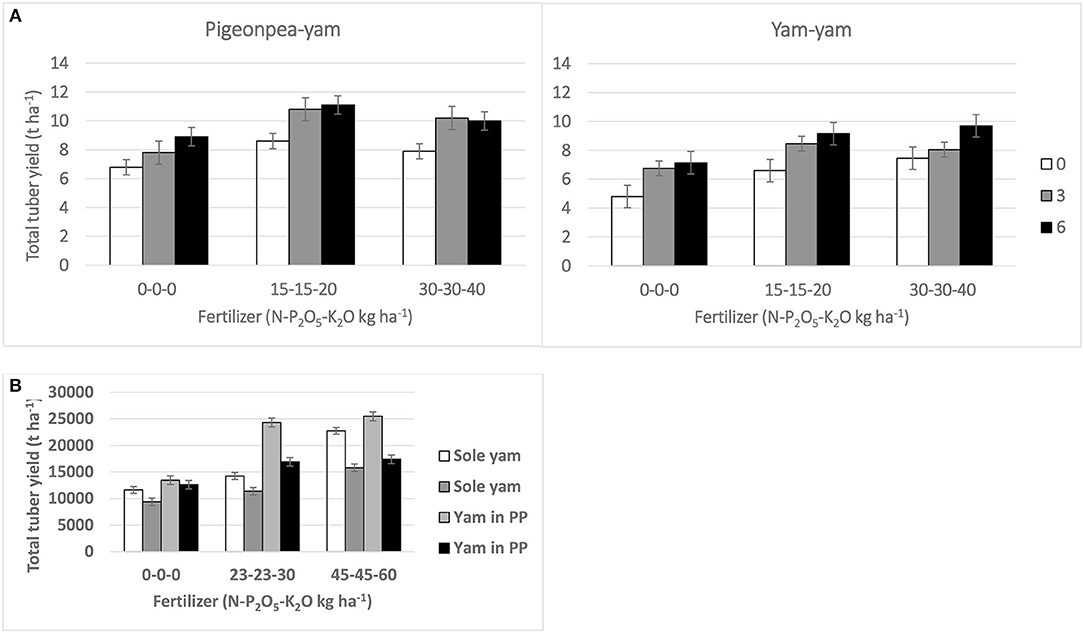
Figure 10. (A) Integrated Soil Fertility Management (ISFM) reduced the inorganic and organic fertilizer (poultry manure) requirement to a third and half respectively for yam production (0–0–0, 15–15–20, 30–30–40 N–P2O5-K2O kg ha−1) Source: Owusu Danquah et al. (2017). (B) Integrated Soil Fertility Management (ISFM) reduced the organic fertilizer requirement to half and improved the tuber yield. PP, pigeonpea biomass. Source: Owusu Danquah (2020).
Preceding yam with pigeonpea resulted in a reduction of the inorganic and organic fertilizers required for sustainable yam production to a third (15–15–15 N–P2O5-K2O kg ha−1) and a half (3 t ha−1) respectively (Figure 10A). Also, the integration of pigeonpea into the yam cropping system resulted in the reduction of the inorganic fertilizer required for sustainable yam production to half (23–23–30 N–P2O5-K2O kg ha−1) (Figure 10B). Thus, the integration of agroforestry trees into cropping systems holds a key in making readily available nutrient reach biomass and reducing the quantities and cost of inorganic fertilizers for sustainable soil fertility management and food security in sub-Saharan Africa.
Conclusion and Way Forward
This paper provides one of the few assessments of the impact of carbon smart technologies on soil, crop and environment and the attendant effect on household livelihood outcomes—food security. Yield gaps are greater in many developing countries, there is considerable need for better assessment of carbon smart technologies to establish viable options for higher productivity. The review showed that conservation agriculture and agroforestry offer the option to increase carbon storage to mitigate the effect of climate change whiles improving and sustaining food production to ensure food security. To be able to improve farmers resilience to climate change and improve food production calls for the adoption of these climate smart technologies by farmers in crop production. Farmer participatory demonstrations of the benefits of these climate smart technologies would make it attractive. Although the adoption of these climate smart technologies would result in improved and sustainable productivity in the long-term, most of these technologies in the short-term require trade-offs in productivity. Well structure incentive schemes should be made available to support farmers to encourage adoption. The use of carbon smart technologies is increasingly gaining recognition under the Kyoto protocol for mitigating climate change. Upon adoption of climate smart farming practices by smallholders, resilience to climate change would improve, resulting in strides towards addressing the SDG's of No poverty (1), No hunger (2), Good health and well-being (3), Climate action (13) and Life on land (15) among others.
Data Availability Statement
The original contributions presented in the study are included in the article/supplementary material, further inquiries can be directed to the corresponding authors.
Author Contributions
SY: conceptualization, methodology, investigation, and writing—original draft. EOD: conceptualization, methodology, and writing—review and editing. PO-D: conceptualization, methodology, and writing—review and editing. KA and ENT: investigation and writing—review and editing. All authors contributed to the article and approved the submitted version.
Conflict of Interest
The authors declare that the research was conducted in the absence of any commercial or financial relationships that could be construed as a potential conflict of interest.
Publisher's Note
All claims expressed in this article are solely those of the authors and do not necessarily represent those of their affiliated organizations, or those of the publisher, the editors and the reviewers. Any product that may be evaluated in this article, or claim that may be made by its manufacturer, is not guaranteed or endorsed by the publisher.
References
Adeniyi, M. O. (2016). The consequences of the IPCC AR5 RCPs 4.5 and 8.5 climate change scenarios on precipitation in West Africa. Clim. Change 139, 245–263. doi: 10.1007/s10584-016-1774-2
Adiku, S. G. K. (2015). “Climate change impacts on west african agriculture: an integrated regional assessment,” in “Handbook of Climate Change and Agroecosystems: The Agricultural Model Intercomparison and Improvement Project (AgMIP) Integrated Crop and Economic Assessments,” eds C. Rosenzweig and D. Hillel (London: Joint Publication with the American Society of Agronomy, Crop Science Society of America, and Soil Science Society of America, Imperial College Press), 25–73
Adjei, V., and Kyerematen, R. (2018). Impacts of changing climate on maize production in the transitional zone of Ghana. Am. J. Clim. Change 7:463. doi: 10.4236/ajcc.2018.73028
Adu-Prah, S., Appiah-Opoku, S., and Aboagye, D. (2019). Spatiotemporal evidence of recent climate variability in Ghana. Afr. Geogr. Rev. 38, 172–190. doi: 10.1080/19376812.2017.1404923
AGRA. (2016). AGRA Annual Progress Report. Available online at: https://agra.org/wp-content/uploads/2021/05/AGRA-2016-Progress-Report.pdf Annual Report (accessed April 3, 2021).
Ahmed, F. (2016). Biochar effects on maize physiology and water capacity of sandy subsoil. Mech. Agric. Conserv. Resourc. 62, 8–13. Available online at: https://stumejournals.com/journals/am/2016/6/8
Akoto, D. S., Partey, S. T., Denich, M., Kwaku, M., Borgemeister, C., and Schmitt, C. B. (2020). Towardz bamboo agroforestry development in Ghana: evaluation of crop performance, soil properties and economic benefit. Agrofor. Syst. 94, 1759–1780. doi: 10.1007/s10457-020-00493-7
Andruschkewitsch, R., Geisseler, D., Koch, H. J., and Ludwig, B. (2013). Effects of tillage on contents of organic carbon, nitrogen, water-stable aggregates and light fraction for four different long-term trials. Geoderma 192, 368–377. doi: 10.1016/j.geoderma.2012.07.005
Apuri, I., Peprah, K., and Achana, G. T. W. (2018). Climate change adaptation through agroforestry: the case of Kassena Nankana West District, Ghana. Environ. Dev. 28, 32–41. doi: 10.1016/j.envdev.2018.09.002
Asare, R., Asare, R. A., Asante, W. A., Markussen, B. O., and Raebild, A. (2017). Influences of shading and fertilization on on-farm yields of cocoa in Ghana. Exp. Agric. 53, 416–431. doi: 10.1017/S0014479716000466
Bhatia, A., Sasmal, S., Jain, N., Pathak, H., Kumar, R., and Singh, A. (2010). Mitigating nitrous oxide emission from soil under conventional and no–tillage in wheat using nitrification inhibitors. Agric. Ecosyst. Environ. 136, 247–253. doi: 10.1016/j.agee.2010.01.004
Biramo, G. (2018). The role of integrated nutrient management system for improving crop yield and enhancing soil fertility under Small holder farmers in Sub-Saharan Africa: a review article. Mod. Concept Dev. Agron. MCDA 547:2. doi: 10.31031/MCDA.2018.02.000547
Brown, S., Grais, A., Ambagis, S., and Pearson, T. (2012). Baseline GHG emissions from the agricultural sector and mitigation potential in countries of East and West Africa. CCAFS Working Paper No. 13. Copenhagen, Denmark: CGIAR Research Program on Climate Change, Agriculture and Food Security (CCAFS). Available online at: www.ccafs.cgiar.org (accessed April 3, 2021).
Burney, J., Woltering, L., Burke, M., Naylor, R. L., and Pasternak, D. (2010). Solar-powered drip irrigation enhances food security in the Sudano-Sahel. Proc. Natl. Acad. Sci. USA. 107, 1848–1853. doi: 10.1073/pnas.0909678107
Centre for Indigenous Knowledge and Organisation Development (CIKOD) and Peasant Farmers Association of Ghana (PFAG). (2018). Assessment of Ghana's Agricultural Development Budget and Farm Input Subsidy Programmes 2008–2017.
Chatskikh, D., and Olesen, J. E. (2007). Soil tillage enhanced CO2 and N2O emissions from loamy sand soil under spring barley. Soil Till. Res. 97, 5–18. doi: 10.1016/j.still.2007.08.004
Choudhary, M. A., Akramkhanov, A., and Saggar, S. (2002). Nitrous oxide emissions from a New Zealand cropped soil: tillage effects, spatial and seasonal variability. Agric. Ecosyst. Environ. 93, 33–43. doi: 10.1016/S0167-8809(02)00005-1
Dixon-Woods, M., Cavers, D., Agarwal, S., Annandale, E., Arthur, A., and Harvey, J. (2006). Conducting a critical interpretive synthesis of the literature on access to healthcare by vulnerable groups. BMC Med. Res. Methodol. 6:35. doi: 10.1186/1471-2288-6-35
Duan, C., Zhang, R., Cai, L., and Zhang, J. (2013). Effects of conservation tillage on daily dynamics of greenhouse gases flux from spring wheat during mature stage in dry land of the Loess Plateau. Chin. Agric. Sci. Bull. 29, 35–40. (in Chinese with English abstract). Available online at: https://en.cnki.com.cn/Article_en/CJFDTotal-ZNTB201321010.htm
Dzanku, F. M., Jirström, M., and Marstorp, H. (2015). Yield gap-based poverty gaps in rural Sub-Saharan Africa. World Dev. 67, 336–362. doi: 10.1016/j.worlddev.2014.10.030
Ennin, S. A., Clegg, M. D., and Francis, C. A. (2002). Resource utilization in soybean/maize intercrops. Afr Crop Sci. J. 10, 251−261. Available online at: https://tspace.library.utoronto.ca/html/1807/20309/cs02025.html
Ennin, S. A., Isaaka, R. N., Acheampong, P. P., Numafo, M., and Owusu Danquah, E. (2014). Mechanization, Fertilization and Staking Options for Environmentally Sound Yam Production. Afr. J. Agric. Res. 9, 2222–2230. doi: 10.5897/AJAR2014.8487
Ennin, S. A., Owusu Danquah, E., Frimpong, F., Akom, M., Osei-Adu, J., Lamptey, J. N. L., et al. (2016). “Yam production and marketing guide,” in CSIR—Crops Research Institute, Ghana, eds H. Adu-Dapaah, J. N. L. Lamptey, I. S. Banning, B. N. Frimpong, J. Osei-Adu and S. A. Ennin.
Epule, T. E., Dhiba, D., Etongo, D., Peng, C., and Lepage, L. (2021). Identifying maize yield and precipitation gaps in Uganda. SN Appl. Sci. 3, 1–12. doi: 10.1007/s42452-021-04532-5
Erskine, P. D., Lamb, D., and Bristow, M. (2006). Tree species diversity and ecosystem function: can tropical multi-species plantations generate greater productivity? For. Ecol. Manag. 233, 205–210. doi: 10.1016/j.foreco.2006.05.013
FAOSTAT (2019). “Ghana Crops and Livestock Products,” 2017. Available online at: http://www.fao.org/faostat/en/#data/TP (accessed April 3, 2021).
Fermont, A. M., Van Asten, P. J., Tittonell, P., Van Wijk, M. T., and Giller, K. E. (2009). Closing the cassava yield gap: an analysis from smallholder farms in East Africa. Field Crops Res. 112, 24–36. doi: 10.1016/j.fcr.2009.01.009
Ghoshal, N., and Singh, K. P. (2010). Impact of addition of various resource quality inputs on soil CO2 flux and C balance in a tropical dryland agroecosystem. 2010, 19 World Congress of Soil Science, Soil Solutions for a Changing World. 1–6 August 2010, Brisbane, Australia
Giles, J. (2005). Nitrogen study fertilizes fears of pollution. Nature 433:791. doi: 10.1038/433791a
Global Yield Gap Water Productivity Atlas (GYGA) (2021). Available online at: GYGA-www.yieldgap.org (accessed May 2021).
Goncalves, N., Andrade, D., Batista, A., Cullen, L., Souza, A., Gomes, H., et al. (2021). Potential economic impact of carbon sequestration in coffee agroforestry systems. Agrofor. Syst. 95, 419–430. doi: 10.1007/s10457-020-00569-4
Govaerts, B., Sayre, K. D., and Deckers, J. (2005). Stable high yields with zero tillage and permanent bed planting? Fields Crop Res. 94, 33–42. doi: 10.1016/j.fcr.2004.11.003
Gram, G., Roobroeck, D., Pypers, P., Six, J., Merckx, R., and Vanlauwe, B. (2020). Combining organic and mineral fertilizers as a climate-smart integrated soil fertility management practice in sub-Saharan Africa: a meta-analysis. PloS ONE 15:e0239552. doi: 10.1371/journal.pone.0239552
Gregorich, E. G., Rochette, P., St–Georges, P., McKim, U. F., and Chan, C. (2008). Tillage effects on N2O emissions from soils under corn and soybeans in eastern Canada. Can. J. Soil Sci. 88, 153–161. doi: 10.4141/CJSS06041
Gupta, R. K., Kumar, V., Sharma, K. R., Buttar, T. S., Singh, G., and Mir, G. (2017). Carbon sequestration potential through agroforestry: a review. Int. J. Curr. Microbiol. Appl. Sci. 6, 211–220. doi: 10.20546/ijcmas.2017.608.029
Hadebe, S. T., Modi, A. T., and Mabhaudhi, T. (2017). Drought tolerance and water use of cereal crops: A focus on sorghum as a food security crop in sub-Saharan Africa. J. Agron. Crop Sci. 203, 177–191. doi: 10.1111/jac.12191
Hermle, S., Anken, T., Leifeld, J., and Weisskopf, P. (2008). The effect of the tillage system on soil organic carbon content under moist, cold-temperate conditions. Soil Till. Res. 98, 94–105. doi: 10.1016/j.still.2007.10.010
Huang, G. B., Zhang, R. Z., Li, G. D., Li, L. L., Chan, K. Y., Heenan, P., et al. (2008). Productivity and sustainability of a spring wheat–field pea rotation in a semi–arid environment under conventional and conservation tillage systems. Field Crop Res. 107, 43–55. doi: 10.1016/j.fcr.2007.12.011
Huang, J., Pan, J., Zhou, L., Zheng, D., Yuan, S., Chen, J., et al. (2020). An improved double-row rubber (Hevea brasiliensis) plantation system increases land use efficiency by allowing intercropping with yam bean, common bean, soybean, peanut, and coffee: A 17-year case study on Hainan Island, China. J. Clean. Prod. 263:121493. doi: 10.1016/j.jclepro.2020.121493
Hütsch, B. W. (1998). Tillage and land use effects on methane oxidation rates and their vertical profiles in soil. Biol. Fertil. Soils 27, 284–292. doi: 10.1007/s003740050435
Hütsch, B. W. (2001). Methane oxidation in non-flooded soils as affected by crop production—invited paper. Eur. J. Agron. 14, 237–260 doi: 10.1016/S1161-0301(01)00110-1
IPCC. (2013). “Climate change 2013: the physical science basis,” in Contribution of Working Group I to the Fifth Assessment Report of the Intergovernmental Panel on Climate Change, eds T. F. Stocker, D. Qin, G.-K. Plattner, M. Tignor, S. K. Allen, J. Boschung, et al. (Cambridge, New York, NY: Cambridge University Press), 1535
Islam, M. A., Islam, S., Ayasha, A., Md Habibur, R., and Dilip, N. (2017). Effect of organic and inorganic fertilizers on soil properties and the growth, yield and quality of tomato in Mymensingh, Bangladesh. Agriculture 7:18. doi: 10.3390/agriculture7030018
Jones, N. (2017). How the world passed a carbon threshold and why it matters. Yale Environ. 360:26. Available online at: http://www.globalwarming-sowhat.com/warm–cool-/how-the-world-passed-a.rtf
Kermah, M., Franke, A. C., Adjei-Nsiah, S., Ahiabor, B. D. K., Abaidoo, R. C., and Giller, K. E. (2018). N2-fixation and N contribution by grain legumes under different soil fertility status and cropping systems in the Guinea savanna of northern Ghana. Agric. Ecosyst. Environ. 261, 201–210. doi: 10.1016/j.agee.2017.08.028
Kihara, J., Sileshi, G. W., Nziguheba, G., Kinyua, M., Zingore, S., and Sommer, R. (2017). Application of secondary nutrients and micronutrients increases crop yields in sub-Saharan Africa. Agron. Sustain. Dev. 37, 1–14. doi: 10.1007/s13593-017-0431-0
Kim, J. H., Kim, Y., and Wang, G. (2017). Impacts of boundary condition changes on regional climate projections over West Africa. J. Geophys. Res. Atmos. 122, 5600–5615. doi: 10.1002/2016JD026167
Komarek, A. M., Drogue, S., Chenoune, R., Hawkins, J., Msangi, S., Belhouchette, H., et al. (2017). Agricultural household effects of fertilizer price changes for smallholder farmers in central Malawi. Agric. Syst. 154, 168–178. doi: 10.1016/j.agsy.2017.03.016
Kuyah, S., Whitney, C. W., Jonsson, M., Sileshi, G. W., Öborn, I., Muthuri, C. W., et al. (2019). Agroforestry delivers a win-win solution for ecosystem services in sub-Saharan Africa. A meta-analysis. Agron. Sustain. Dev. 39:47. doi: 10.1007/s13593-019-0589-8
Lal, R. (2004). Soil carbon sequestration to mitigate climate change. Geoderma 121, 1–22. doi: 10.1016/j.geoderma.2004.01.032
Lal, R. (2009). Challenges and opportunities in soil organic matter research. Eur. J. Soil Sci. 60, 158–169. doi: 10.1111/j.1365-2389.2008.01114.x
Lal, R., and Pimentel, D. (2009). Biofuels: beware crop residues. Science 326, 1345–1346. doi: 10.1126/science.326.5958.1345-c
Lampurlanes, J., Angás, P., and Cantero–Martínez, C. (2002). Tillage effects on water storage during fallow, and on barley root growth and yield in two contrasting soils of the semi–arid Segarra region Spain. Soil Till. Res. 65, 207–220. doi: 10.1016/S0167-1987(01)00285-9
Langyintuo, A. (2020). “Smallholder farmers' access to inputs and finance in Africa,” in The Role of Smallholder Farms in Food and Nutrition Security (Cham: Springer), 133–152. doi: 10.1007/978-3-030-42148-9_7
Lenton, T. M., Rockström, J., Gaffney, O., Rahmstorf, S., Richardson, K., Steffen, W., et al. (2019). Climate tipping points-too risky to bet against. Proc. Natl Acad. Sci. 105, 1786–1793. doi: 10.1038/d41586-019-03595-0
Liu, L., Owusu Danquah, E., Weebadde, C., Bessah, E., and Basso, B. (2021). Modeling soil organic carbon and yam yield under different agronomic management across spatial scales in Ghana. Field Crops Res. 263:108018. doi: 10.1016/j.fcr.2020.108018
Mahmood, F., Khan, I., Ashraf, U., Shahzad, T., Hussain, S., Shahid, M., et al. (2017). Effects of organic and inorganic manures on maize and their residual impact on soil physico-chemical properties. J. Soil Sci. Plant Nutr. 17, 22–22. doi: 10.4067/S0718-95162017005000002
Malézieux, E., Crozat, Y., Dupraz, C., Laurans, M., Makowski, D., Ozier-Lafontaine, H., et al. (2009). “Mixing plant species in cropping systems: concepts, tools and models: a review,” in Sustainable Agriculture, eds E. Lichtfouse, M. Navarrete, P. Debaeke, S. Véronique, and C. Alberola (Dordrecht: Springer). doi: 10.1007/978-90-481-2666-8_22
Maliki, R., Sinsin, B., Parrot, L., Lancon, J., Floquet, A., and Lutaladio, N. (2017). Sustainable agroforestry adoption in a small-scale food production system: the case of yam in rotation with the intercropping gliricidia sepium and herbaceous in the guinea-sudan zone of benin. Int. J. Agric. Innov. Res. 5, 1086–1095.
Manaye, A., Tesfamariam, B., Tesfaye, M., Worku, A., and Gufi, Y. (2021). Tree diversity and carbon stocks in agroforestry systems in northern Ethiopia. Carbon Balance Manag. 16, 1–10. doi: 10.1186/s13021-021-00174-7
McLain, J. E. T., and Martens, D. A. (2006). Moisture controls on trace gas fluxes in semiarid riparian soils. Soil Sci. Soc. Am. J. 70, 367–377. doi: 10.2136/sssaj2005.0105
Ministry of Food and Agriculture (MoFA). (2018). Agriculture in Ghana; facts and figures. Statistics, Research and Information Directorate (SRID), Ministry of Food and Agriculture, Republic of Ghana.
Moe, K., Win, M. K., Win, K. K., and Yamakawa, T. (2017). Combined effect of organic manures and inorganic fertilizers on the growth and yield of hybrid rice (Palethwe-1). Am. J. Plant Sci. 8, 1022–1042. doi: 10.4236/ajps.2017.85068
Mosier, A. R., Halvorson, A. D., Reule, C. A., and Liu, X. J. J. (2006). Net global warming potential and greenhouse gas intensity in irrigated cropping systems in northeastern Colorado. J. Environ. Qual. 35, 1584–1598 doi: 10.2134/jeq2005.0232
Mosquera-Losada, J., Hol, J. M., Rappoldt, C., and Dolfing, J. (2007). Precise soil management as a tool to reduce CH4 and N2O emissions from agricultural soils. Animal Sciences Group Report, 2007, No. 28. Wageningen, The Netherlands
Mthembu, B. E., Everson, C. S., and Everson, T. M. (2018). Tree legumes-temperate grass agroforestry system effects on inorganic soil nitrogen as ecosystem services provision for smallholder farming systems in South Africa. J. Crop Improv. 32, 141–155. doi: 10.1080/15427528.2017.1376239
Mueller, N. D., Gerber, J. S., Johnston, M., Ray, D. K., Ramankutty, N., and Foley, J. A. (2012). Closing yield gaps through nutrient and water management. Nature 490, 254–257. doi: 10.1038/nature11420
Mugwe, J., Ngetich, F., and Otieno, E. O. (2019). Integrated soil fertility management in Sub-Saharan Africa: evolving paradigms toward integration. Zero Hunger. Encyclopedia of the UN Sustainable Development Goals (Cham: Springer). doi: 10.1007/978-3-319-69626-3_71-1
Mukherjee, M., and Lal, R. (2015). Short-term effects of cover cropping on the quality of a typic Argiaquolls in Central Ohio. Catena 131, 125–129. doi: 10.1016/j.catena.2015.02.025
Musokwa, M., Mafongoya, P., and Lorentz, S. (2019). Evaluation of agroforestry systems for maize (Zea mays) productivity in South Africa. South Afric. J. Plant Soil 36, 65–67. doi: 10.1080/02571862.2018.1459898
Nair, P. R., Nair, V. D., Kumar, B. M., and Haile, S. G. (2009). Soil carbon sequestration in tropical agroforestry systems: a feasibility appraisal. Environ. Sci. Policy 12, 1099–1111. doi: 10.1016/j.envsci.2009.01.010
Nair, P. R., Nair, V. D., Kumar, B. M., and Showalter, J. M. (2010). Carbon sequestration in agroforestry systems. Adv. Agron. 108, 237–307. doi: 10.1016/S0065-2113(10)08005-3
Nalivata, P., Kibunja, C., Mutegi, J., Tetteh, F., Tarfa, B., Dicko, M. K., et al. (2017). Integrated soil fertility management in Sub-Saharan Africa. Fertilizer Optim. Sub-Saharan Africa 2017, 113–124. doi: 10.1079/9781786392046.0113
Nath, A. J., Sileshi, G. W., Laskar, S. Y., Pathak, K., Reang, D., Nath, A., et al. (2021). Quantifying carbon stocks and sequestration potential in agroforestry systems under divergent management scenarios relevant to India's Nationally Determined Contribution. J. Cleaner Prod. 281:124831. doi: 10.1016/j.jclepro.2020.124831
Nyaga, J., Muthuri, C. W., Barrios, E., Öborn, I., and Sinclair, F. L. (2019). Enhancing maize productivity in agroforestry systems through managing competition: lessons from smallholders' farms, Rift valley, Kenya. Agrofor. Syst. 93, 715–730. doi: 10.1007/s10457-017-0169-3
Ofori-Mensah, M. (2017). Access to Basic Public Services: Challenges Ghana must Overcome. Accra: The Institute of Economic Affairs.
Omonode, R. A., Vyn, T. J., Smith, D. R., Hegymegi, P., and Gal, A. (2007). Soil carbon dioxide and methane fluxes from long–term tillage systems in continuous corn and corn–soybean rotations. Soil Till. Res. 95, 182–195. doi: 10.1016/j.still.2006.12.004
Oorts, K., Merckx, R., Gréhan, E., Labreuche, J., and Nicolardot, B. (2007). Determinants of annual fluxes of CO2 and N2O in long–term no–tillage and conventional tillage systems in northern France. Soil Till. Res. 95, 133–148. doi: 10.1016/j.still.2006.12.002
Oppong, B. A., Onumah, E. E., Asuming, B., and Samuel, O. (2014). Stochastic frontier modeling of maize production in Brong-Ahafo Region of Ghana. Agris On-line Papers in Econ. Inform. 6, 67–75. doi: 10.22004/ag.econ.182492
Owusu Danquah, E. (2020). Evaluation of pigeonpea—white yam (Canjanus cajan [L.] millsp— Dioscorea rotundata [L] poir) cropping system for improved yam productivity and livelihood of smallholder farmers (Ph. D. Thesis). Department of Plant, Soil and Microbial Sciences, Michigan State University.
Owusu Danquah, E., Beletse, Y., Stirzaker, R., Smith, C., Yeboah, S., Oteng-Darko, P., et al. (2020). Monitoring and modelling analysis of maize (Zea mays L.) yield gap in smallholder farming in Ghana. Agriculture 10:420. doi: 10.3390/agriculture10090420
Owusu Danquah, E., Ennin, S. A., and Acheampong, P. P. (2017). Integrated soil nutrient management option for sustainable yam production. Agron. Afr. 29, 69–81. Available online at: https://search.proquest.com/openview/4922b4424f21ca7290fedd9ce719120b/1?pq-origsite=gscholar&cbl=44156
Owusu Danquah, E., Ennin, S. A., Lamptey, J. N. L., and Acheampong, P. P. (2015). Staking options for sustainable yam production in Ghana. Sustain. Agric. Res. 4, 106–113. doi: 10.5539/sar.v4n1p106
Ozpinar, S., and Cay, A. (2006). Effect of different tillage systems on the quality and crop productivity of a clay-loam soil in semi-arid north-western Turkey. Soil Till. Res. 88, 95–106. doi: 10.1016/j.still.2005.04.009
Patrick, R. A., Mulyungi, P., Mburu, D., Eric, N., and Aiamble, N. (2018). Effect of Transaction Costs on Inorganic Fertilizer Use Intensity in Rwanda. Int. J. Res. Appl. Sci. Eng. Technol. 6, 1433–1439. doi: 10.22214/ijraset.2018.6210
Place, F., Barrett, C. B., Freeman, H. A., Ramisch, J. J., and Vanlauwe, B. (2003). Prospects for integrated soil fertility management using organic and inorganic inputs: evidence from smallholder African agricultural systems. Food Policy 28, 365–378. doi: 10.1016/j.foodpol.2003.08.009
Qin, D., Plattner, G. K., Tignor, M., Allen, S. K., Boschung, J., Nauels, A., et al. (2014). “Climate change 2013: the physical science basis,” in Contribution of Working Group I to the Fifth Assessment Report of the Intergovernmental Panel on Climate Change, eds T. F. Stocker et al., 5–14.
Raimi, A., Adeleke, R., and Roopnarain, A. (2017). Soil fertility challenges and Biofertiliser as a viable alternative for increasing smallholder farmer crop productivity in sub-Saharan Africa. Cogent Food Agric. 3:1400933. doi: 10.1080/23311932.2017.1400933
Rao, K. P. C., Verchot, L. V., and Laarman, J. (2007). Adaptation to climate change through sustainable management and development of agroforestry systems. J. SAT Agric. Res 4, 1–30. Available online at: http://oar.icrisat.org/id/eprint/2561
Reicosky, D. C., and Archer, D. W. (2007). Moldboard plow tillage depth and short-term carbon dioxide release. Soil Till. Res. 94, 109–121 doi: 10.1016/j.still.2006.07.004
Ribeiro-Barros, A. I., Silva, M. J., Moura, I., Ramalho, J. C., Máguas-Hanson, C., and Ribeiro, N. S. (2018). “The potential of tree and shrub legumes in agroforestry systems,” in Nitrogen in Agriculture-Updates, eds K. Amanullah and S. Fahad, 223–239. doi: 10.5772/intechopen.69995
Riede, J. O., Posada, R., Fink, A. H., and Kaspar, F. (2016). “What's on the 5th IPCC Report for West Africa?” in Adaptation to Climate Change and Variability in Rural West Africa (Cham: Springer), 7–23. doi: 10.1007/978-3-319-31499-0_2
Rong, L. B., Gong, K. Y., Duan, F. Y., Li, S. K., Ming, Z. H. A. O., Jianqiang, H. E., et al. (2021). Yield gap and resource utilization efficiency of three major food crops in the world–a review. J. Integr. Agric. 20, 349–362. doi: 10.1016/S2095-3119(20)63555-9
Saggar, S., Harvey, M., Singh, J., Giltrap, D., Pattey, E., Bromley, T., et al. (2010). Chambers, micrometeorological measurements, and the New Zealand Denitrification—decomposition model for nitrous oxide emission estimates from an irrigated dairy-grazed pasture. J. Integr. Environ. Sci. 7, 61–70. doi: 10.1080/19438151003621433
Saito, K., Vandamme, E., Johnson, J. M., Tanaka, A., Senthilkumar, K., Dieng, I., et al. (2019). Yield-limiting macronutrients for rice in sub-Saharan Africa. Geoderma 338, 546–554. doi: 10.1016/j.geoderma.2018.11.036
Salazar-Díaz, R., and Tixier, P. (2019). Effect of plant diversity on income generated by agroforestry systems in Talamanca, Costa Rica. Agrofor. Syst. 93, 571–580. doi: 10.1007/s10457-017-0151-0
Sharma, V. K., Marano, D., Anyanwu, C. N., Okonkwo, G. I., Ibeto, C. N., and Eze, I. S. (2011). Solar cooling: a potential option for energy saving and abatement of greenhouse gas emissions in Africa. Singapore J. Sci. Res. 1, 1–12. doi: 10.3923/sjsres.2011.1.12
Shiferaw, B., Smale, M., Braun, H. J., Duveiller, E., Reynolds, M., and Muricho, G. (2013). Crops that feed the world 10. Past successes and future challenges to the role played by wheat in global food security. Food Security 5, 291–317. doi: 10.1007/s12571-013-0263-y
Singh, B. (2018). Are nitrogen fertilizers deleterious to soil health? Agronomy 8:48. doi: 10.3390/agronomy8040048
Singh, V. K., Dwivedi, B. S., Mishra, R. P., Shukla, A. K., Timsina, J., Upadhyay, P. K., et al. (2019). Yields, soil health and farm profits under a rice-wheat system: long-term effect of fertilizers and organic manures applied alone and in combination. Agronomy 9:1. doi: 10.3390/agronomy9010001
Slattery, R. A., and Ort, D. R. (2019). Carbon assimilation in crops at high temperatures. Plant Cell Environ. 42, 2750–2758. doi: 10.1111/pce.13572
Snyder, C. S., Bruulsema, T. W., Jensen, T. L., and Fixen, P. E. (2009). Review of greenhouse gas emissions from crop production systems and fertilizer management effects. Agric. Ecosyst. Environ. 133, 247–266. doi: 10.1016/j.agee.2009.04.021
Stewart, Z. P., Pierzynski, G. M., Middendorf, B. J., and Prasad, P. V. (2020). Approaches to improve soil fertility in sub-Saharan Africa. J. Exp. Bot. 71, 632–641. doi: 10.1093/jxb/erz446
Tiefenbacher, A., Sandén, T., Haslmayr, H. P., Miloczki, J., Wenzel, W., and Spiegel, H. (2021). Optimizing carbon sequestration in croplands: a synthesis. Agronomy 11:882. doi: 10.3390/agronomy11050882
Tsufac, A. R., Awazi, N. P., and Yerima, B. P. K. (2021). Characterization of agroforestry systems and their effectiveness in soil fertility enhancement in the south-west region of Cameroon. Curr. Res. Environ. Sustain. 3:100024. doi: 10.1016/j.crsust.2020.100024
Ussiri, D., Lal, R., and Jarecki, M. K. (2009). Nitrous oxide and methane emissions from long–term tillage under a continuous corn cropping system in Ohio. Soil Till. Res. 104, 247–253. doi: 10.1016/j.still.2009.03.001
Van den Putte, A., Govers, G., Diels, J., Gillijns, K., and Demuzere, M. (2010). Assessing the effect of soil tillage on crop growth: a meta-regression analysis on European crop yields under conservation agriculture. Eur. J. Agron. 33, 231–241 doi: 10.1016/j.eja.2010.05.008
Van Noordwijk, M., Lawson, G., Hairiah, K., and Wilson, J. (2015). Root distribution of trees and crops: competition and/or complementarity. Tree–Crop Interactions: Agroforestry in a Changing Climate. CABI, Wallingford, 221–257. doi: 10.1079/9781780645117.0221
Vanlauwe, B., Bationo, A., Chianu, J., Giller, K. E., Merckx, R., Mokwunye, U., et al. (2010). Integrated soil fertility management: operational definition and consequences for implementation and dissemination. Outlook Agric. 39, 17–24. doi: 10.5367/000000010791169998
Venterea, R. T., Burger, M., and Spokas, K. A. (2005). Nitrogen oxide and methane emissions under varying tillage and fertilizer management. J. Environ. Qual. 34, 1467–1477. doi: 10.2134/jeq2005.0018
Waldén, P., Ollikainen, M., and Kahiluoto, H. (2020). Carbon revenue in the profitability of agroforestry relative to monocultures. Agrofor. Syst. 94, 15–28. doi: 10.1007/s10457-019-00355-x
Wang, X., He, C., Liu, B., Zhao, X., Liu, Y., Wang, Q., et al. (2020). Effects of residue returning on soil organic carbon storage and sequestration rate in China's croplands: a meta-analysis. Agronomy 10:691. doi: 10.3390/agronomy10050691
Yang, B., Meng, X., Singh, A. K., Wang, P., Song, L., Zakari, S., et al. (2020). Intercrops improve surface water availability in rubber-based agroforestry systems. Agric. Ecosyst. Environ. 298:106937. doi: 10.1016/j.agee.2020.106937
Yeboah, S., Lamptey, S., Cai, L., and Song, M. (2018). Short-term effects of biochar amendment on greenhouse gas emissions from rainfed agricultural soils of the semi-arid loess plateau region. Agronomy 8:74. doi: 10.3390/agronomy8050074
Yeboah, S., Zhang, R., Cai, L., Song, M., Li, L., Xie, L.uo, J. H., et al. (2016). Greenhouse gas emissions in a spring wheat–field pea sequence under different tillage practices in semi-arid Northwest China. Nutr. Cycl. Agroecosyst. 106, 77–91. doi: 10.1007/s10705-016-9790-1
Zhang, A., Bian, R., Pan, G., Cui, L., Hussain, Q., Li, L., et al. (2012). Effects of biochar amendment on soil quality, crop yield and greenhouse gas emission in a Chinese rice paddy: a field study of 2 consecutive rice growing cycles. Field Crops Res. 127, 153–160. doi: 10.1016/j.fcr.2011.11.020
Zhang, R. Z., Huang, G. B., Cai, L. Q., Luo, Z. Z., Li, L. L., and Xie, J. H. (2013). Dry farmland practice involving multi–conservation tillage measures in the Loess Plateau. Chin. J. Eco-Agric. 21, 61–69 (in Chinese with English abstract). Available online at: https://www.cabdirect.org/cabdirect/abstract/20133157436
Zingore, S., Mutegi, J., Agesa, B., Tamene, L., and Kihara, J. (2015). Soil degradation in sub-Saharan Africa and crop production options for soil rehabilitation. Better Crops 99, 24–26. Available online at: https://www.cabdirect.org/cabdirect/abstract/20153138577
Keywords: carbon, climate change, food system, nutrient, resilience
Citation: Yeboah S, Owusu Danquah E, Oteng-Darko P, Agyeman K and Tetteh EN (2021) Carbon Smart Strategies for Enhanced Food System Resilience Under a Changing Climate. Front. Sustain. Food Syst. 5:715814. doi: 10.3389/fsufs.2021.715814
Received: 27 May 2021; Accepted: 31 July 2021;
Published: 26 August 2021.
Edited by:
Abiodun Ogundeji, University of the Free State, South AfricaReviewed by:
Yonas Bahta, University of the Free State, South AfricaTemitope Oluwaseun Ojo, Obafemi Awolowo University, Nigeria
Copyright © 2021 Yeboah, Owusu Danquah, Oteng-Darko, Agyeman and Tetteh. This is an open-access article distributed under the terms of the Creative Commons Attribution License (CC BY). The use, distribution or reproduction in other forums is permitted, provided the original author(s) and the copyright owner(s) are credited and that the original publication in this journal is cited, in accordance with accepted academic practice. No use, distribution or reproduction is permitted which does not comply with these terms.
*Correspondence: Stephen Yeboah, cHJveWVib2FoQHlhaG9vLmNvLnVr