- 1Department of Plant Pathology, University of Wisconsin, Madison, WI, United States
- 2Michael Fields Agricultural Institute, East Troy, WI, United States
As recognition increases of the benefits of reducing soil disturbance to preserve soil health, there is mounting interest in developing innovative methods of using cover crops as living mulches to control weeds in organic grain systems. Spring-planted winter cereal rye (Secale cereale L.) interseeded with soybeans (Glycine max. [L.] Merr.) is a promising, yet untested, living mulch system because rye exhibits vigorous growth in the early spring during the critical weed free period, but then dies back as the soybean canopy matures. The objectives of this study were to compare a rye living mulch system with a tilled “organic business-as-usual” control, and to understand the risks and benefits associated with delaying soybean planting date to manage the weed seed bank prior to establishment of rye and soybeans. Three treatments including (1) a June-planted rye and soybean living mulch system, (2) a June planted tilled control, and (3) a May planted tilled control, were compared in terms of weed prevalence and soybean grain yield in a randomized complete block experimental design with four replications implemented across 3 site years from 2019 to 2020. Interseeding rye as a living mulch resulted in consistently higher weed pressure as compared to tilled controls. Increased weed pressure in May- over June-planted controls in 2 of 3 site years indicate planting date influences weed dynamics. Rye biomass was positively correlated with soybean yield (R2 = 0.76, r = 0.87, p < 0.05) and negatively correlated with weed biomass (R2 = 0.63, r = −0.79, p < 0.05). Under optimal conditions where rye biomass was maximized, interseeding rye adequately suppressed weeds without reducing soybean yields as compared to tilled controls. However, under drier conditions with lower rye production, increased weed pressure and reduced yields emphasize the risks associated with living mulch systems.
Introduction
Organic agriculture provides an alternative management paradigm that limits environmental externalities through the adoption of practices that promote ecosystem services; most notably soil health (Tuck et al., 2013; Reganold and Wachter, 2016; Muller et al., 2017). However, concerns over organic grain production systems' dependence on tillage to control weeds, terminate cover crops, manage disease, and incorporate crop residues has prompted substantial research and innovation efforts to advance the development of reduced or no-till organic cropping systems (Carr, 2017; Silva and Delate, 2017; Silva and Vereecke, 2019). In recent years, advances in no-till organic soybean production have arisen through the strategic use of fall-planted cover crops, most commonly winter cereal rye, to suppress weed growth in place of tillage (Silva, 2014; Silva and Delate, 2017; Vincent-Caboud et al., 2019). Winter rye is typically seeded in September in the Upper Midwest (Silva, 2014; Silva and Delate, 2017) to reach the recommended minimum threshold of 8,000 kg ha−1 of biomass for consistent weed suppression (Mirsky et al., 2013; Vincent-Caboud et al., 2019) before being terminated with a roller crimper in the spring to create a thick mulch layer to cover the soil. Meeting these early rye planting date requirements to achieve adequate weed suppression can be challenging following corn, which almost always appears before soybeans in rotations and is often not harvested for grain until November in the Upper Midwest. Therefore, organic producers are seeking adaptive management strategies to achieve weed suppression while maintaining reduced tillage systems without relying on fall-planted covers. Interseeding winter rye simultaneously with soybeans as a living mulch has been demonstrated as an alternative weed control approach that minimizes soil disturbance in organic soybean production (Thelen et al., 2004; Uchino et al., 2009; Nelson et al., 2011), would not rely on operational timing in the fall, and could even enable stale seedbed techniques in the spring prior to planting.
Living mulches maintain both weed suppression and cash crop yield when selected living mulch species have morphological and physiological differences from cash crops that limit competition for light, nutrients, and water (Verret et al., 2017; Bhaskar et al., 2021). Winter rye is an ideal living mulch for soybeans because its life cycle is complementary to soybean's. Due to winter rye's vernalization requirement, it will not set seed when spring sown (Bàrberi, 2002; Uchino et al., 2009), precluding issues from rye seedbank or soy seed lot contamination. Winter cereal rye creates a living mulch that is highly competitive with the early season germinating weeds through light interception, soil resource competition, and allelopathic effects (Brainard and Bellinder, 2004; Reberg-Horton et al., 2005; Datta et al., 2017; Vollmer et al., 2020; Bhaskar et al., 2021). However, winter rye begins to senesce as temperatures increase and soybean demand for water and nutrients intensifies, thereby potentially limiting the competitive effects of the living mulch on soybean grain yield (Robinson and Dunham, 1954; Ateh and Doll, 1996; Thelen et al., 2004).
While spring-seeding winter rye as a living mulch in soybean production systems was initially proposed in the 1950's, knowledge on agronomic best management practices remains limited. Explorations into variations on rye seeding rate (Ateh and Doll, 1996; Nelson et al., 2011), soybean seeding rate (Thelen et al., 2004), rye and soybean planting dates (Thelen et al., 2004; Nelson et al., 2011), and soybean row spacings (Nelson et al., 2011) have been examined in the literature. High soybean seeding rates and narrow rows have been recommended as cultural weed control strategies that can result in earlier canopies and greater direct competition with weeds (Holshouser and Whittaker, 2002; Mortensen et al., 2012; Datta et al., 2017). However, planting soybeans on narrow rows increases risk as it precludes the opportunity to perform cultivation if weed control from the rye living mulch becomes inadequate (Uchino et al., 2009; Nelson et al., 2011). Planting rye prior to soybean planting has been seen as impractical as rye growth competes strongly with soybeans for resources (Robinson and Dunham, 1954; Uchino et al., 2009). Seeding rye in the weeks following soybean planting have limited impacts on soybean vigor and grain yield; however, delayed seeding prohibits winter rye's niche of competing with the early season weeds thereby requiring additional soil disturbance to control weeds prior to rye interseeding (Uchino et al., 2009).
A previous experience at the University of Wisconsin has indicated that there is value to delaying rye and soybean planting dates later than typically seeded under standard organic management practices to decrease the risk from rye vernalization occurring as well as to lower the weed seed bank through additional stale seed bedding prior to planting (Rasmussen, 2004; Boyd et al., 2017). However, delaying planting to reduce weed populations represents a potential trade off as delaying planting dates beyond mid-May in the Upper Midwest can result in the loss of yield potential (Pedersen and Lauer, 2004; De Bruin and Pedersen, 2008; Hu and Wiatrak, 2012). Therefore, there remains a need to elucidate the dynamics of winter rye and soybean planting dates to determine agronomic management systems that balance weed suppression with soybean production.
Given the potential utility of spring-seeded rye as a reduced tillage weed control measure in organic agriculture, further research is required to optimize management and address yield losses (Uchino et al., 2009; Nelson et al., 2011). The objectives of this study were to understand potential risks and benefits from (1) interseeding winter rye with soybeans and (2) delaying soybean planting date within the context of a spring-seeded rye system on weed prevalence and soybean production in the Upper Midwest to further guide adaptation of organic weed management strategies.
Materials and methods
Field history and site description
A field experiment to examine spring-seeded winter rye with soybeans was implemented at the Arlington Agricultural Research Station (AARS) (43°30'N, 89°34'W) in Columbia County, WI in 2019–2020. The experimental location contained a Plano series silt loam soil (Fine-silty, mixed, superactive, mesic Typic Argiudolls) (USDA, NRCS, 2021). These trials were implemented following a corn silage crop on certified organic land (Midwest Organic Services Agency. Viroqua, WI). Weather data for the research site was acquired from the National Weather Service and accessed through the Wisconsin State Climatology Office (https://www.aos.wisc.edu/~sco/). Plots were established under rainfed conditions without irrigation in all site years. Cumulative annual precipitation was 1,180 mm and 944 mm in 2019 and 2020, respectively, as compared against the 57-year historical average of 861 mm. In-season precipitation trends from June through August were above the historical average by 43.6 mm in 2019 and 23.5 mm in 2020 (Figure 1).
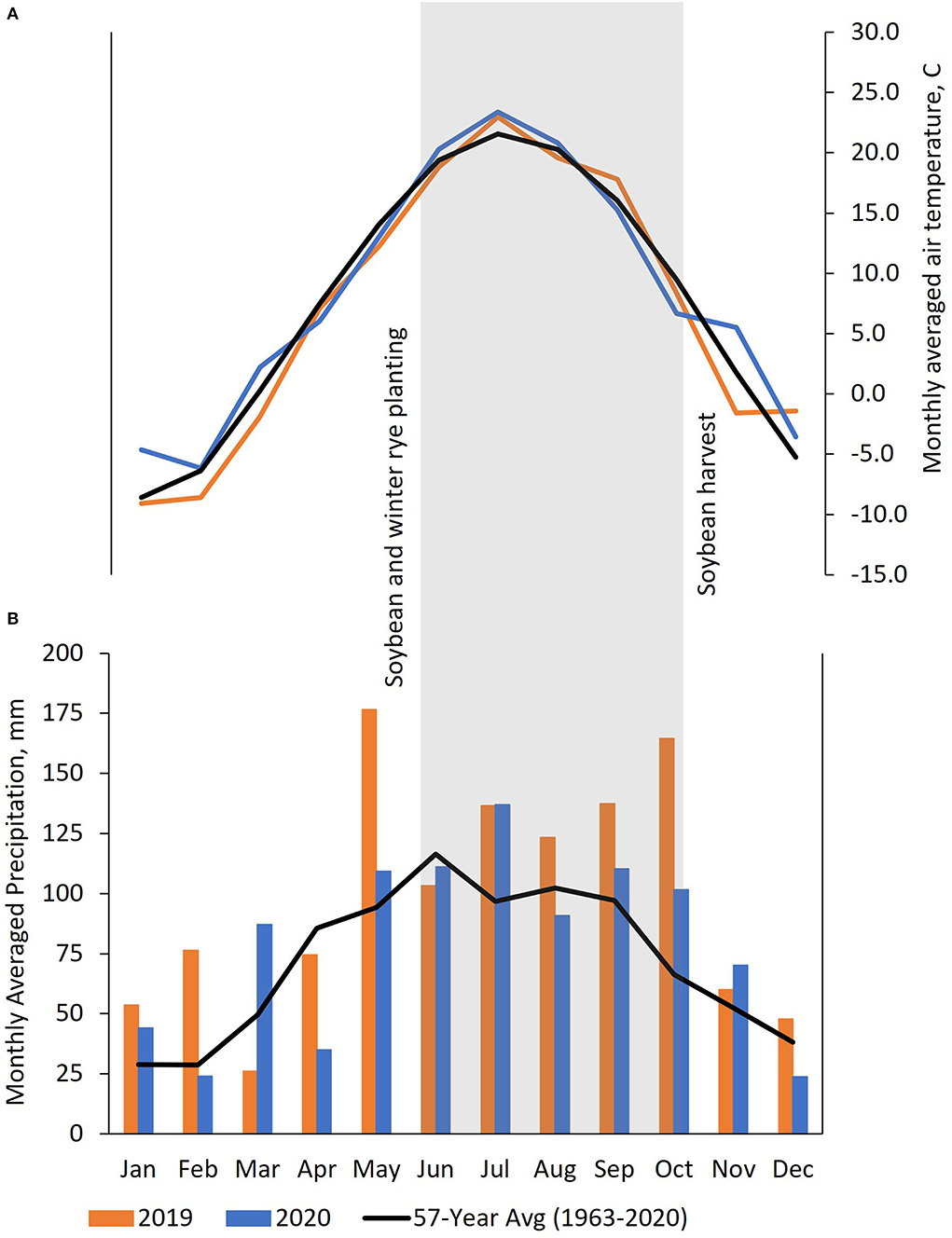
Figure 1. (A) Monthly averaged air temperatures (°C) and (B) precipitation (mm) plotted against 57-year averages (1963–2020) at the Arlington Agricultural Research Station, Arlington, WI, 2019–2020. Area shaded in gray signifies the active growing season of soybean during the 2 study years.
A third site year was conducted at the Michael Fields Agricultural Institute's (MFAI) research farm (42°48'N, 88°26'W) in Walworth County, WI in 2020. The MFAI location contained a St. Charles silt loam soil (Fine-silty, mixed, superactive, mesic Typic Hapludalfs) (USDA, NRCS, 2021). The MFAI experimental site land has been certified organic since 2017, and the previous crop before soybeans in this experiment was an alfalfa + grass mix. Precipitation was only recorded in-season at the MFAI site. Monthly cumulative precipitation of 52.1 mm in June, 78.7 mm in July, and 55.9 mm in August were recorded. These observed values fall 74.5, 156, and 274 mm below the established 7-year site history (2014–2020) averages for June, July, and August, respectively, indicating abnormally dry conditions during the winter rye and soybean growing season.
Treatments and experimental design
A randomized complete block design with three treatments examining the presence or absence of winter rye as a living mulch and soybean planting date were replicated four times in all site years. Treatments contrasted a winter cereal rye living mulch (“June Rye + Soy”) with (1) a May-planted tilled soybean control using standard weed control practices of tine weeding and inter-row cultivation (“May Control”), and to a (2) June-planted tilled soybean control that allows for additional stale seedbed preparation to manage the weed seed bank prior to in-season tine weeding and inter-row cultivation (“June Control”) (Figure 2). A May-planted living mulch with soybean treatment was excluded from the experimental design based on previous experience at the University of Wisconsin indicating the early seeding of rye increases risk of rye vernalization and higher weed pressures due to fewer opportunities to manage the weed seed bank prior to planting. Plot size was 68 × 5 m in 2019 and lengthened to 137 m in 2020.

Figure 2. Cropping systems diagram illustrating conceptual differences and timing of weed control activities, soybean growth, and winter rye cover when managed under three experimental treatments (June Rye + Soy, June Control, and May Control) as implemented at the Arlington Agricultural Research Station, Arlington, WI and the Michael Fields Agricultural Institute, East Troy, WI, 2019–2020.
Crop management
Organic fertility sources were not applied either before or after planting during the duration of the study in all site years. Pre-plant cultivation occurred as a stale seedbed technique to reduce the weed seedbank prior to crop establishment (Travlos et al., 2020) using a field cultivator (Sunflower Manufacturing, Beloit, KS) at AARS and a disc + finisher at the MFAI site, from late April until planting (Table 1). Winter rye and soybeans were seeded on the same day with rye planted immediately following soybean sowing (Table 1). Soybeans (Viking O.1706N) were planted (JD 1750, John Deere, Moline, IL) 3.8-cm deep on 76-cm row spacing according to the treatment structure at 531,265 seeds ha−1. Winter rye was interseeded with a no-till drill 2-cm deep on 19-cm row spacing to maintain four rows of rye between each soybean row. A seeding rate of 4.9 million seeds ha−1 using Spooner (University of Wisconsin, Madison, WI) and Aroostook (Soil Conservation Service Plant Materials Center et. al, Big Flatts, NY) winter rye varieties in 2019 and 2020, respectively, in accordance with Wisconsin interseeded spring rye seeding rates as described by Ateh and Doll (1996). Tine weeding and rotary hoeing were performed 1 to 2 times as necessary to adequately disrupt emerging weed seedlings with minimal soil disturbance (Table 1) following soybean and rye sowing. However, no mechanical methods of weed control were utilized until the rye two-leaf growth stage (Zadoks 12) and soybean cotyledon stage (VC) to minimize damage to emerging crops (Zadoks et al., 1974). Rye growth then was allowed to control weeds without the use of mechanical weed management tools after initial tine weeding and rotary hoe passes were completed in the June Rye + Soy treatment. In contrast, the no-rye control treatments followed typical weed control management practices for organic soybeans by receiving 2–5 post-plant tillage operations using a field cultivator to achieve adequate weed control until canopy closure of soybean rows. All treatments were harvested for soybean grain upon reaching crop maturity (Table 1).
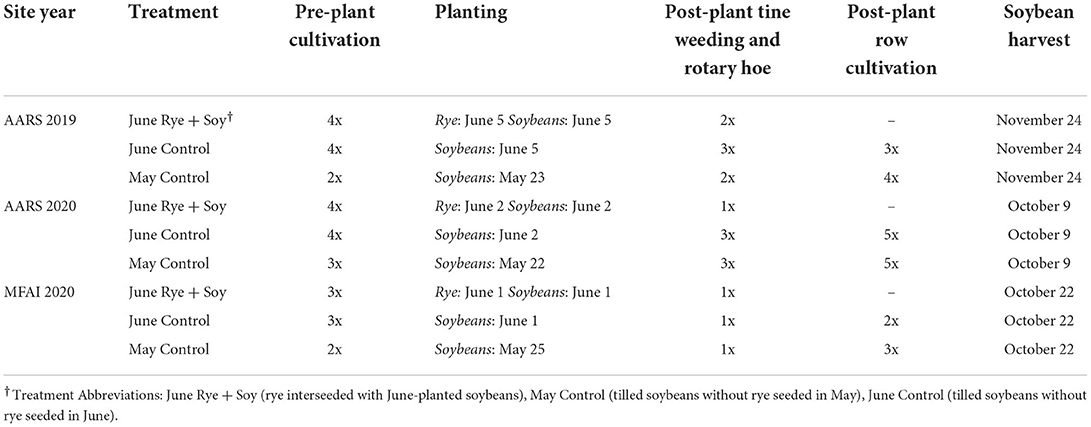
Table 1. Frequency of pre-plant and post-plant tillage operations for spring-seeded rye and tilled control treatments located at the Arlington Agricultural Research Station (AARS) in Arlington, WI and the Michael Fields Agricultural Institute (MFAI), East Troy, WI, 2019–2020.
Data collection
Individual weeds in each plot were counted and separated by grasses and broadleaves as a measure indicating weed abundance. At the AARS location in 2019 and 2020, weed abundance was recorded in the early August by counting all weed plants in three locations per plot using a frame of 0.25 m2. At MFAI 2020, weed abundance was recorded on July 1 at two locations per plot using a frame of 0.25 m2. In early August at all site years, broadleaf and grass weeds and rye biomass were cut at ground level at the time of rye senescence, and fractions were separated upon collection to understand the community structure of present weeds. Biomass samples were dried in a forced air oven at 60°C until completely dry. Soybean plant stand was determined by counting all soybeans on 1/1,000 of an acre of row length in three locations per plot in mid-July. At AARS, the plots were machine harvested with yield determined by weighing harvested soybean grain and correcting to 13% moisture from harvested moisture as determined by a soil moisture meter (Dickey-John GAC 2500). At MFAI, soybean grain yields were determined by cutting all soybean plants within a 1-m2 quadrat per plot, hand threshing grain from pods, and weighing grain. The yields reported were adjusted to 13% moisture.
Data analysis
Data analysis was performed with RStudio statistical software, version 4.1.0 (R Core Team, 2021). The data was separated and analyzed by individual site year due to significant differences in site year when pooled. A two-way ANOVA linear model tested differences between total weed, grass, and broadleaf biomass as well as soybean stand counts and grain yield. Assumptions of normality and homogeneity of variances were confirmed through examination of residual plots using the ggResidpanel package (Goode and Rey, 2019), Levene's test, and the Shapiro–Wilk normality test. All weed biomass measurements were log(1 + x) transformed to validate the models. The total weed, grass, and broadleaf abundance was analyzed using a negative binomial generalized linear model through the MASS package (Venables and Ripley, 2002). The mean separation was performed using Tukey's HSD at p < 0.05 using the agricolae package (de Mendiburu, 2021) while Pearson's correlations were examined using the hmisc package (Harrel and Dupont, 2021). Principle component (PC) analysis was performed using the FactoMineR package (Lê et al., 2008) to calculate the PC scores as well as loadings for each parameter.
Results
Effect on weed abundance and biomass
There was an interaction between location and year for weed abundance. At 2 site years, total weed abundance was greatest in the June Rye + Soy treatment and was similar to the May Control at AARS 2019 (Table 2). The June Control treatment experienced the lowest total weed abundance at AARS 2019 and MFAI 2020, and was similar to the May Control at AARS 2020 (Table 2). In breaking out the counts by species, the weed abundance counts for grass and broadleaf weeds were statistically similar to total weed abundance results among treatments, and both grass and broadleaf weeds were present at similar levels within a treatment (Table 2).
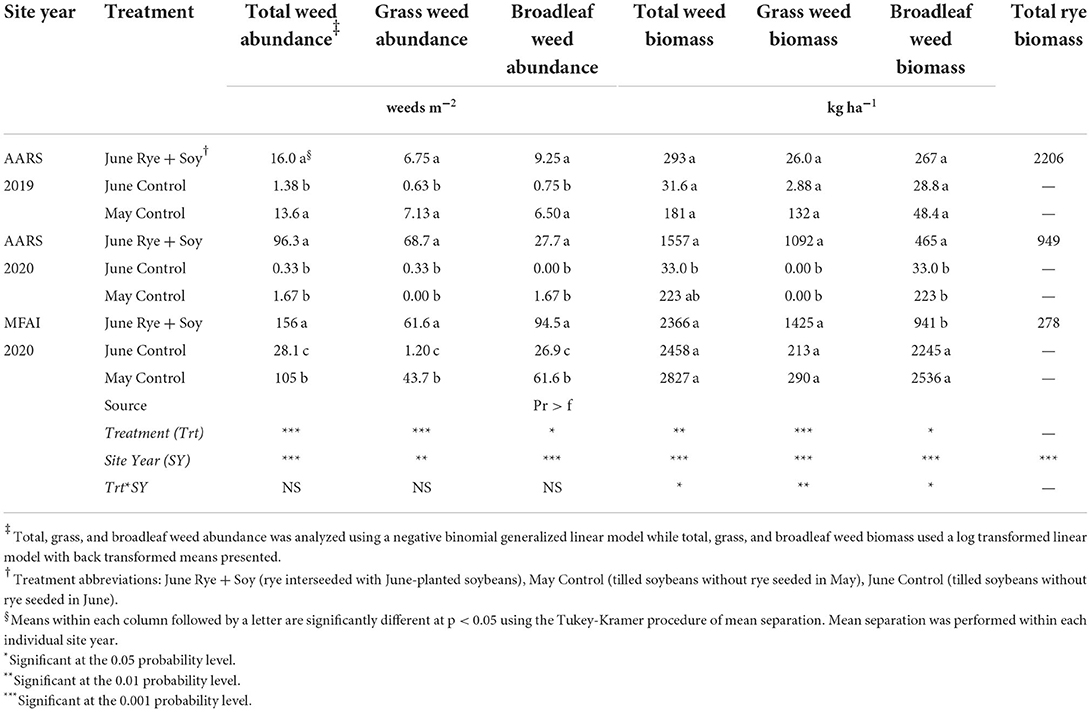
Table 2. Analysis of variance and treatment means of biomass (dry matter basis) and abundance of total, broadleaf, and grass weeds located at the Arlington Agricultural Research Station (AARS) in Arlington, WI and the Michael Fields Agricultural Institute (MFAI), East Troy, WI, 2019–2020.
Fewer differences were observed among treatments in total weed biomass compared to abundance counts, likely due to the high variance often encountered when measuring weed biomass. The only differences in total weed biomass among treatments was detected at AARS 2020, where weed biomass was greater in the June Rye + Soy than the June Control, and the May Control was similar to both treatments (Table 2). In examining grass vs. broadleaf biomass, the only notable difference from the abundance results occurred at MFAI 2020, where broadleaf weed biomass was lower in the June Rye + Soy than the May and June Controls (the opposite trend as that observed from weed abundance; Table 2). Although weed biomass was not statistically different between the May and the June Control treatments, at all 3 site years weed biomass trended higher in the May Control (Table 2), similar to trends observed in previous studies (in this case, unusually high variability precluded detection of statistical differences; Supplementary Figures 1, 2).
A principal component (PC) analysis revealed that study location primarily separated along PC 1, with the AARS locations associated with higher soybean yields and stand counts, and the MFAI 2020 location strongly affected by weed biomass, particularly broadleaves (Figure 3). Treatment effects separated mainly along PC 2, with the tilled control treatments not strongly associating with any particular measured variables (but weakly associated with broadleaf weed biomass), and the June Rye + Soy weakly associated with grass weed biomass (Figure 3).
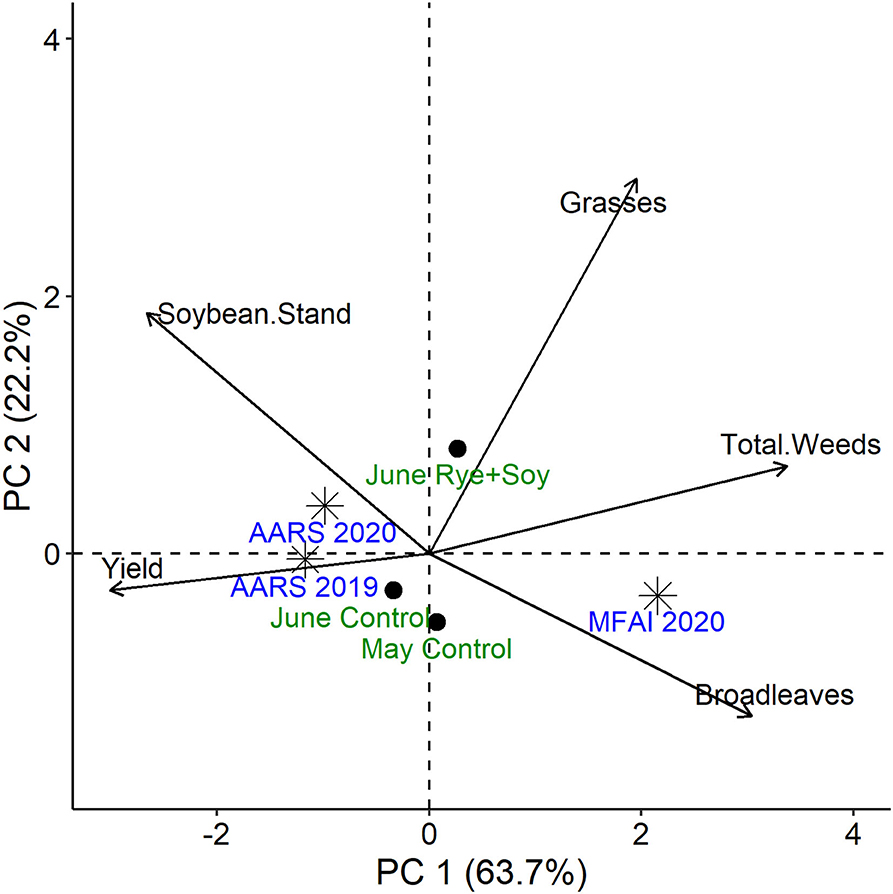
Figure 3. Principal component (PC) plot superimposed with eigenvectors of treatments and site years in relation to measures of weed biomass (kg ha−1), soybean plant stand (plants ha−1), and soybean grain yield (Mg ha−1). Treatment abbreviations: June Rye + Soy (rye interseeded with June-planted soybeans), May Control (tilled soybeans without rye seeded in May), June Control (tilled soybeans without rye seeded in June). Site year abbreviations: AARS, Arlington Agricultural Research Station; MFAI, Michael Fields Agricultural Institute.
Soybean plant stand
Significant differences in soybean plant stand among treatments were noted in 2 of 3 site years (Table 3). At AARS 2019, higher soybean plant stands were recorded in June Rye + Soy as compared to June Control. May Control exhibited lower soybean stand in relation to either of the June-planted treatments at MFAI 2020 (Table 3), due to relatively wet and cold soil conditions persisting into the end of May in that site year. The Pearson correlation coefficients indicated that soybean stand counts were significantly related to broadleaf weed abundance indicating that soybean stands are important for competing with germinating broadleaf weeds (Table 4). In contrast, soybean stand counts were not correlated with grass weed abundance. Similar correlation trends were observed between the soybean stand counts and the weed biomass, as for weed abundance (Table 4).
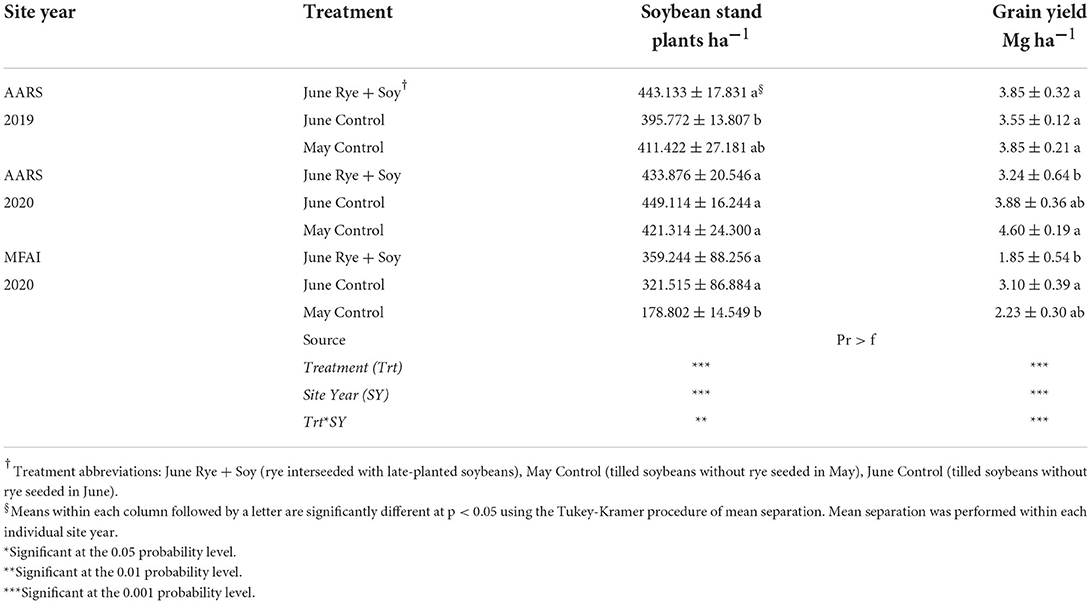
Table 3. Analysis of variance and mean soybean stand counts and soybean grain yield, shown with standard deviation, at the Arlington Agricultural Research Station (AARS), Arlington, WI and Michael Fields Agricultural Institute (MFAI), East Troy, WI, 2019–2020.
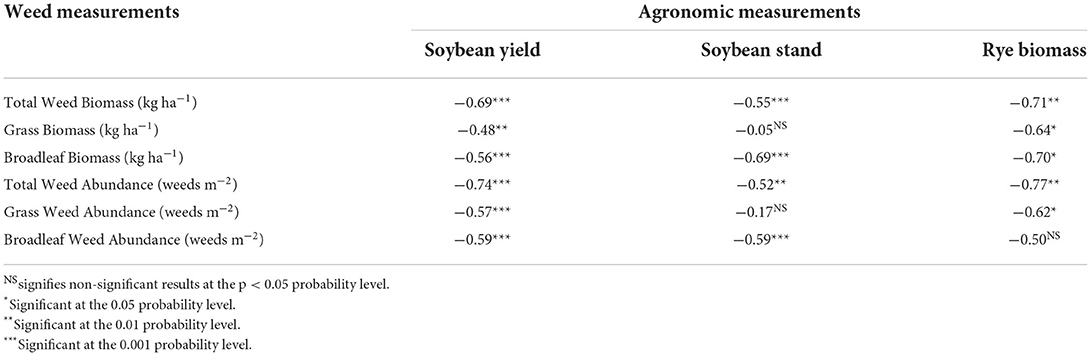
Table 4. Pearson correlation coefficients (r values) between the soybean grain yield (Mg ha−1), the soybean stand (plants ha−1), and the rye biomass (kg ha−1) with measures of weed parameters located at the Arlington Agricultural Research Station in Arlington, WI and the Michael Fields Agricultural Institute, East Troy, WI, 2019–2020.
Rye biomass
Winter rye biomass production was strongly affected by site year (Table 2) with AARS 2020 and MFAI 2020 achieving only 43 and 12.6% of the rye biomass observed at AARS 2019. The relationship between the rye biomass and the weed biomass was best fit with a quadratic polynomial regression indicating that achieving adequate rye growth is essential for effective weed control (Figure 4A). Both biomass and abundance of broadleaf and grass weed components showed negative correlations with rye biomass with the exception of broadleaf weed abundance (p < 0.1; Table 4).
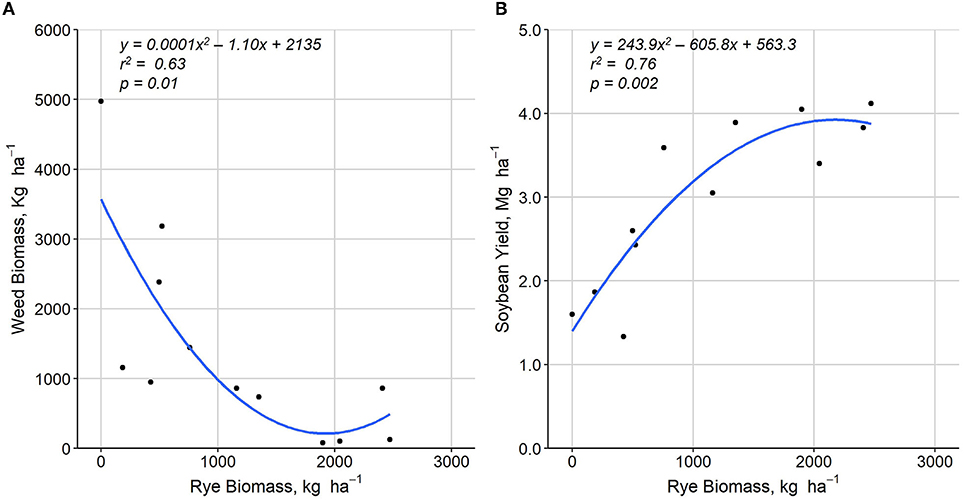
Figure 4. Effects of winter rye biomass on (A) weed biomass and (B) soybean yield measured at the Arlington Agricultural Research Station in Arlington, WI and the Michael Fields Agricultural Institute, East Troy, WI, 2019–2020.
Soybean yield
Soybean yields were significantly different among treatments in 2 of 3 site years with no difference in yield reported at AARS 2019 (Table 3). At AARS 2020, soybean grain yields were greater in the May Control than the June Rye + Soy, whereas yields in the June Control were similar to the other two treatments (Table 3). At MFAI 2020, soybean grain yields were greatest in the June Control and lowest in the June Rye + Soy, whereas grain yields in the May Control were similar to the other two treatments (Table 3). Soybean grain yield exhibited a quadratic relationship with rye biomass (Figure 4B) indicating higher soybean yields as rye biomass increased (R2 = 0.76, p < 0.001) likely achieved through improved weed suppression. Soybean yield was most strongly correlated with total weed abundance but exhibited negative correlations with all measured indicators of weed prevalence (Table 4).
Discussion
While it appears that rye successfully controlled weeds to an extent, total weed abundance remained higher for June Rye + Soy at all 3 site years matching reports of greater weed prevalence for rye living mulch systems seen in the literature (Robinson and Dunham, 1954; Ateh and Doll, 1996). Weed pressure and rye biomass in this study varied substantially by location likely as a combined result of soil moisture conditions and weed seed bank. Lower rye biomass at MFAI 2020 as compared to other site years was likely a result of dry conditions following rye planting, giving the rye a slow start, whereas the high rye biomass observed at AARS 2019 was likely driven by well above average precipitation throughout the growing season. Rye and weed biomass observed at MFAI 2020 match observations from Nelson et al. (2011) who saw rye dry weights below 400 kg ha−1 resulting in heavy weed pressure exceeding 2,400 kg ha−1 and soybean yields below 2.0 Mg ha−1. In contrast Geddes and Gulden (2021) observed rye mulch exceeding 1,000 kg ha−1 that reduced volunteer canola stands without lowering soybean yield in 2 of 3 site years. These results fit with the trends observed in the present study where a strong positive relationship between rye biomass and soybean yield coupled with a negative relationship between rye biomass and weed biomass suggest that achieving adequate rye biomass is essential to controlling weeds in this system. The previous studies have noted that when rye seeding rates are increased to obtain greater ground cover, soybean yield drops due to greater competition for soil moisture (Ateh and Doll, 1996; Nelson et al., 2011). Therefore, under high precipitation conditions as observed at AARS 2019, the moisture competition from rye may be mitigated while providing substantial biomass to suppress weeds. Following these results, when spring and the early summers are predicted, we would recommend growers not use rye as a living mulch for soybeans.
Study results indicate that a winter rye living mulch may provide better suppression against broadleaf weeds as opposed to grass weeds indicating that the type of dominant weed present may impact the weed control ability of rye. In this study, interseeding rye was associated with higher levels of grass weeds as compared to broadleaf weeds. Furthermore, at MFAI 2020 which experienced relatively higher proportions of broadleaf weeds as compared to grasses, June Rye + Soy reduced broadleaf weed biomass even though higher broadleaf abundance was observed. This effect was less pronounced for grasses indicating a greater propensity for rye and soybean companion crops to together compete with broadleaf weed types as opposed to grasses. Interestingly, measures of weed abundance proved to be a more sensitive indicator of differences in weed pressure as opposed to weed biomass in this study. While this is likely partially affected by high variability in weed biomass sampling, it may also reflect an ability of rye to reduce weed growth potential through allelopathic effects. Allelopathy in winter rye has been well-established showing that rye's allelochemicals can reduce both weed germination and growth (Barnes and Putnam, 1983; Schulz et al., 2013; Grint et al., 2022). The previous research has suggested that broadleaf weeds tend to exhibit greater sensitivity to the benzoxazinoid allelochemicals produced by rye forming a major component of their weed suppression ability (Barnes and Putnam, 1986; Gavazzi et al., 2010; Schulz et al., 2013).
Soybean yield was not significantly different between the June Control and the May Control in any of the locations. With the exception of MFAI 2020, June Control yielded numerically lower than May Control at AARS 2019 and AARS 2020 indicating the potential for a loss of yield potential as indicated in the literature (Pedersen and Lauer, 2004; De Bruin and Pedersen, 2008; Hu and Wiatrak, 2012). The numeric yield increase of June Control over May Control at MFAI 2020 may be due to a higher weed abundance and lower plant stands reported with May Control. This indicates that while it is likely that yield potential is being lost by delaying planting date, the potential for higher weed pressure due to fewer stale seedbed passes may offset potential increases in yield potential by pushing planting dates earlier. Therefore, earlier planting dates must be balanced with the ability to reduce the weed seed bank prior to planting through stale seedbed practices.
In this study, establishing winter rye as a living mulch showed both neutral and negative effects on soybean yield throughout the course of this study which match the generally negative (Ateh and Doll, 1996; Thelen et al., 2004; Nelson et al., 2011) and generally neutral effects (Robinson and Dunham, 1954; Geddes and Gulden, 2021) observed on soybean yield by year when interseeding rye as a living mulch as compared to a chemically managed or tilled control. Yield reductions when utilizing rye as a living mulch have been attributed to competition for soil moisture (Thelen et al., 2004; Uchino et al., 2009; Geddes and Gulden, 2021) and insufficient weed control (Nelson et al., 2011) which are in accordance with results obtained from the present study. This system proved most successful when under high precipitation conditions that maximized rye biomass growth without competing with soybeans for available moisture, as observed at AARS 2019.
Conclusion
Rye was unable to provide as consistent or as effective weed control as the tilled controls evidenced by higher weed abundance throughout the study. However, the negative relationship observed between rye biomass and weed biomass coupled with the positive affect of rye biomass on soybean yields suggest that under high precipitation conditions rye biomass can be maximized without inducing competitive effects for soil moisture. June Control trended toward lower soybean grain yields as compared to May Control indicating a yield potential loss by pushing the planting dates into June. However, consistently higher weed abundance in May Control represents a tradeoff of maximizing yield potential with achieving adequate weed control. Therefore, delaying establishment of a winter rye living mulch with soybeans until June can aid in reducing weed populations through stale seedbed techniques. Ultimately, successful implementation of a rye living mulch system, demonstrated specifically at AARS 2019, was achieved by June planting rye and soybeans under high precipitation conditions that allowed substantial rye growth to reduce weed populations without competing with soybeans for soil moisture. This study confirms the potential for spring-seeded rye with reduced tillage as an effective adaptive management approach to increase crop biodiversity without compromising soybean yield in organic crop production systems. However, these results also underscore the risks associated with intensifying agricultural management and minimizing mechanical weed control.
Data availability statement
The raw data supporting the conclusions of this article will be made available by the authors, without undue reservation.
Author contributions
LV and NT implemented field trials and collected data. BB and NT provided data analysis and drafted manuscript with contributions from ES and LV. All authors provided substantial intellectual contributions and have agreed upon the final presented results.
Acknowledgments
We would like to acknowledge the field staff at the Arlington Agricultural Research Station and the Michael Fields Agricultural Institute who provide field operations that make this research possible.
Conflict of interest
The authors declare that the research was conducted in the absence of any commercial or financial relationships that could be construed as a potential conflict of interest.
Publisher's note
All claims expressed in this article are solely those of the authors and do not necessarily represent those of their affiliated organizations, or those of the publisher, the editors and the reviewers. Any product that may be evaluated in this article, or claim that may be made by its manufacturer, is not guaranteed or endorsed by the publisher.
Supplementary material
The Supplementary Material for this article can be found online at: https://www.frontiersin.org/articles/10.3389/fsufs.2022.926606/full#supplementary-material
References
Ateh, C. M., and Doll, J. D. (1996). Spring-planted winter rye (Secale cereale) as a living mulch to control weeds in soybean (Glycine max). Weed Technol. 10, 347–353. doi: 10.1017/S0890037X00040070
Bàrberi, P. (2002). Weed management in organic agriculture: are we addressing the right issues? Weed Res. 42, 177–193. doi: 10.1046/j.1365-3180.2002.00277.x
Barnes, J. P., and Putnam, A. R. (1983). Rye residues contribute weed suppression in no-tillage cropping systems. J. Chem. Ecol. 9, 1045–1057. doi: 10.1007/BF00982210
Barnes, J. P., and Putnam, A. R. (1986). Evidence for allelopathy by residues and aqueous extracts of rye (Secale cereale). Weed Sci. 34, 384–390. doi: 10.1017/S0043174500067035
Bhaskar, V., Westbrook, A. S., Bellinder, R. R., and DiTommaso, A. (2021). Integrated management of living mulches for weed control: a review. Weed Technol. 35, 856–868. doi: 10.1017/wet.2021.52
Boyd, N. S., Brennan, E. B., and Fennimore, S. A. (2017). Stale seedbed techniques for organic vegetable production. Weed Technol. 20, 1052–1057. doi: 10.1614/WT-05-109.1
Brainard, D., and Bellinder, R. (2004). Weed suppression in a broccoli–winter rye intercropping system. Weed Sci. 2, 281–290. doi: 10.1614/WS-03-031R
Carr, P. M. (2017). Guest editorial: conservation tillage for organic farming. Agriculture 7:19. doi: 10.3390/agriculture7030019
Datta, A., Ullah, H., Tursun, N., Pornprom, T., Knezevic, S., and Chauhan, B. (2017). Managing weeds using crop competition in soybean [Glycine max (L.) Merr.]. Crop Prot. 95, 60–68. doi: 10.1016/j.cropro.2016.09.005
De Bruin, J. L., and Pedersen, P. (2008). Soybean seed yield response to planting date and seeding rate in the Upper Midwest. Agron. J. 100, 696–703. doi: 10.2134/agronj2007.0115
de Mendiburu, F. (2021). agricolae: Statistical Procedures for Agricultural Research. Available online at: https://CRAN.R-project.org/package=agricolae
Gavazzi, C., Schulz, M., Marocco, A., and Tabaglio, V. (2010). Sustainable weed control by allelochemicals from rye cover crops: from the greenhouse to field evidence. Allelop. J. 25, 259–274. doi: 10.4081/ija.2013.e5
Geddes, C. M., and Gulden, R. H. (2021). Wheat and cereal rye inter-row living mulches interfere with early season weeds in soybean. Plants 10:2276. doi: 10.3390/plants10112276
Goode, K., and Rey, K. (2019). ggResidpanel: Panels and Interactive Versions of Diagnostic Plots Using 'ggplot2'. R package version 0.3.0. Available online at: https://CRAN.R-project.org/package=ggResidpanel
Grint, K. R., Arneson, N. J., Oliveira, M. C., Smith, D. H., and Werle, R. (2022). Cereal rye cover crop terminated at crop planting reduces early-season weed density and biomass in Wisconsin corn-soybean production. Agrosyst. Geosci. Environ. 5:e20245. doi: 10.1002/agg2.20245
Harrel, F. E. Jr., and Dupont, C. (2021). Hmisc: Harell miscellaneous. R package version 4.6-0. Available online at: https://hbiostat.org/R/Hmisc
Holshouser, D. L., and Whittaker, J. P. (2002). Plant population and row-spacing effects on early soybean production systems in the Mid-Atlantic USA. Agron. J. 94, 603–611. doi: 10.2134/agronj2002.6030
Hu, M., and Wiatrak, P. (2012). Effect of planting date on soybean growth, yield, and grain quality. Agron. J. 104, 785–790. doi: 10.2134/agronj2011.0382
Lê, S., Josse, J., and Husson, F. (2008). FactoMineR: a package for multivariate analysis. J. Stat. Softw. 25, 1–18. doi: 10.18637/jss.v025.i01
Mirsky, S. B., Ryan, M. R., Teasdale, J. R., Curran, W. S., Reberg-Horton, C. S., Spargo, J. T., et al. (2013). Overcoming weed management challenges in cover crop-based organic rotational no-till soybean production in the Eastern United States. Weed Technol. 27, 193–203. doi: 10.1614/WT-D-12-00078.1
Mortensen, D. A., Egan, J. F., Maxwell, B. D., Ryan, M. R., and Smith, R. G. (2012). Navigating a critical juncture for sustainable weed management. BioScience 62, 75–84. doi: 10.1525/bio.2012.62.1.12
Muller, A., Schader, C., Scialabba, N. E., Brüggemann, J., Isensee, A., Erb, K., et al. (2017). Strategies for feeding the world more sustainably with organic agriculture. Nat. Commun. 8:1290. doi: 10.1038/s41467-017-01410-w
Nelson, K. A., Smeda, R. J., and Smoot, R. L. (2011). Spring-interseeded winter rye seeding rates influence weed control and organic soybean yield. Int. J. Agron. 2011, 1–7. doi: 10.1155/2011/571973
Pedersen, P., and Lauer, J. G. (2004). Soybean growth and development response to rotation sequence and tillage system. Agron. J. 96, 1005–1012. doi: 10.2134/AGRONJ2004.1005
R Core Team (2021). R: A Language and Environment for Statistical Computing. R Found. Stat. Comput. Vienna, Austria. https://www.R-project.org/
Rasmussen, I. A. (2004). The effect of sowing date, stale seedbed, row width and mechanical weed control on weeds and yields of organic winter wheat. Weed Res. 44, 12–20. doi: 10.1046/j.1365-3180.2003.00367.x
Reberg-Horton, S. C., Burton, J. D., Danehower, D. A., Ma, G., Monks, D. W., Murphy, J. P., et al. (2005). Changes over time in the allelochemical content of ten cultivars of rye (Secale cereale L.). J. Chem. Ecol. 31, 179–193. doi: 10.1007/s10886-005-0983-3
Reganold, J. P., and Wachter, J. M. (2016). Organic agriculture in the twenty-first century. Nat. Plants 2:15221. doi: 10.1038/nplants.2015.221
Robinson, R., and Dunham, R. (1954). Companion crops for weed control in soybeans 1. Agron. J. 46, 278–281. doi: 10.2134/agronj1954.00021962004600060010x
Schulz, M., Marocco, A., Tabaglio, V., Macias, F. A., and Molinillo, J. M. G. (2013). Benzoxazinoids in rye allelopathy–From discovery to application in sustainable weed control and organic farming. J. Chem. Ecol. 39, 154–174. doi: 10.1007/s10886-013-0235-x
Silva, E. M. (2014). Screening five fall-sown cover crops for use in organic no-till crop production in the Upper Midwest. Agroecol. Sustain. Food Syst. 38, 748–763. doi: 10.1080/21683565.2014.901275
Silva, E. M., and Delate, K. (2017). A decade of progress in organic cover crop-based reduced tillage practices in the Upper Midwestern USA. Agriculture 7:44. doi: 10.3390/agriculture7050044
Silva, E. M., and Vereecke, L. (2019). Optimizing organic cover crop-based rotational tillage systems for early soybean growth. Org. Agr. 9, 471–481. doi: 10.1007/s13165-019-00243-9
Thelen, K. D., Mutch, D. R., and Martin, T. E. (2004). Utility of interseeded winter cereal rye in organic soybean production systems. Agron. J. 96, 281–284. doi: 10.2134/agronj2004.2810
Travlos, I., Gazoulis, I., Kanatas, P., Tsekoura, A., Zannopoulos, S., and Papastylianou, P. (2020). Key factors affecting weed seeds' germination, weed emergence, and their possible role for the efficacy of false seedbed technique as weed management practice. Front. Agron. 2:1. doi: 10.3389/fagro.2020.00001
Tuck, S. L., Winqvist, C., Mota, F., Ahnström, J., Turnbull, L. A., and Bengtsson, J. (2013). Land-use intensity and the effects of organic farming on biodiversity: a hierarchical meta-analysis. J. Appl. Ecol. 51, 746–755. doi: 10.1111/1365-2664.12219
Uchino, H., Iwama, K., Jitsuyama, Y., Yudate, T., and Nakamura, S. (2009). Yield losses of soybean and maize by competition with interseeded cover crops and weeds in organic-based cropping systems. Field Crops Res. 113, 342–351. doi: 10.1016/j.fcr.2009.06.013
USDA, NRCS. (2021). Web Soil Survey. Available online at: http://websoilsurvey.sc.egov.usda.gov/ (accessed October 30, 2021).
Venables, W. N., and Ripley, B. D. (2002). Modern Applied Statistics With S, Fourth edition. New York, NY: Springer.
Verret, V., Gardarin, A., Pelzer, E., Médiène, S., Makowski, D., and Valantin-Morison, M. (2017). Can legume companion plants control weeds without decreasing crop yield? A meta-analysis. Field Crops Res. 204, 158–168. doi: 10.1016/j.fcr.2017.01.010
Vincent-Caboud, L., Casagrande, M., David, C., Ryan, M. R., Silva, E. M., and Peigné, J. (2019). Using mulch from cover crops to facilitate organic no-till soybean and maize production. A review. Agron. Sustain. Dev. 39:45. doi: 10.1007/s13593-019-0590-2
Vollmer, K. M., Besancon, T. E., Carr, B. L., VanGessel, M. J., and Scott, B. A. (2020). Spring-seeded cereal rye suppresses weeds in watermelon. Weed Technol. 34, 42–47. doi: 10.1017/wet.2019.102
Keywords: organic agriculture, living mulch, interseeding, winter cereal rye, soybeans
Citation: Brockmueller B, Tautges NE, Vereecke L and Silva EM (2022) Spring-seeded winter rye living mulches enhance crop biodiversity and promote reduced tillage organic soybeans. Front. Sustain. Food Syst. 6:926606. doi: 10.3389/fsufs.2022.926606
Received: 22 April 2022; Accepted: 15 July 2022;
Published: 12 August 2022.
Edited by:
Ashley Conway, University of Missouri, United StatesReviewed by:
Rajani Srivastava, Banaras Hindu University, IndiaAjit Singh, University of Nottingham Malaysia Campus, Malaysia
Copyright © 2022 Brockmueller, Tautges, Vereecke and Silva. This is an open-access article distributed under the terms of the Creative Commons Attribution License (CC BY). The use, distribution or reproduction in other forums is permitted, provided the original author(s) and the copyright owner(s) are credited and that the original publication in this journal is cited, in accordance with accepted academic practice. No use, distribution or reproduction is permitted which does not comply with these terms.
*Correspondence: Ben Brockmueller, YnJvY2ttdWVsbGVyQHdpc2MuZWR1
†Present Address: Léa Vereecke, Rodale Institute, Madison, WI, United States