- College of Plant Protection, Northwest A&F University, Yangling, China
Clubroot is a devastating disease threatening global cruciferous vegetable production caused by Plasmodiophora brassicae (Pb). We have evaluated the positive effects of the Alcaligenes faecalis Juj3 on cabbage growth promotion and Pb stress alleviation through pot and field experiments. The Juj3 strain was isolated from a healthy cabbage rhizosphere with growth-promoting characteristics and was identified as A. faecalis based on morphological traits and phylogeny. Seed germination assays revealed that Juj3 inoculation enhances cabbage bud shoot and root growth. In pot experiments, inoculation with Juj3 fermentation powder at cabbage sowing dates significantly improved the seedling biomass. Combining seed treatments with root irrigation after transplanting considerably reduced the clubroot disease index and resulted in appreciable biocontrol efficacy (83.7%). Gene expression analyses of cabbage after Juj3 inoculation showed that PR2 and EIN3 expression were significantly up-regulated. Physiologically, Juj3 inoculation enhanced cabbage chlorophyll content and root activity in a normal environment. Irrespective of whether plants were under normal environment or Pb stresses, Juj3 improved photosynthesis. Field trial analyses revealed that Juj3 exhibits satisfactory biocontrol efficacy in cabbage (51.4%) and Chinese cabbage (37.7%). Moreover, Juj3 could also enhance cabbage and Chinese cabbage biomass to improve the yield quality. These findings pave the way for future use of A. faecalis as biocontrol agents for clubroot and reveal the great potential of the rhizobacterium for plant growth-promoting applications in agriculture and horticulture.
Introduction
Brassica crops provide vital sources for vegetable, cooking oil, and condiment uses for human consumption (Karamat et al., 2021). In recent years, soilborne diseases have become increasingly severe as the cultivated areas of cruciferous vegetables have increased. Clubroot, as one of the most economically severe diseases of cruciferous vegetables, caused a substantial proportion of these losses (Strelkov and Hwang, 2014) and is elicited by the obligate biotrophic parasite, Plasmodiophora brassicae (Pb) (Zhang et al., 2019). The pathogen induces abnormal cell division and enlargement of host root cells to produce spindle-like, spherical, knobby, or club-shaped root galls (Kageyama and Asano, 2009). Pb infections result in carbohydrate sinks from the viable gall cells, leading to wilting or plant death (Ludwig-Müller and Schuller, 2008; Ludwig-Müller et al., 2009). Hence, Pb substantially alters the morphology, development, and physiology of diseased plants (Malinowski et al., 2012) resulting in nutrients and severe yield losses in cruciferous vegetables. To ensure sustainable vegetable yields in cruciferous agrosystems during ever-increasing Pb stresses, integrated strategies involving host resistance, crop rotation, fungicides (Peng et al., 2014), and biocontrol agents (BCAs) (Zhu et al., 2019) are necessary for clubroot management. Among the various methods, the use of plant growth-promoting rhizobacteria (PGPR) has attracted the attention of many researchers.
The rhizosphere provides the frontline defense for plant roots against attack by soilborne pathogens (Cook et al., 1995). Their interactions have improved plant growth, yield, and stress tolerance of crops, and can be included in biofertilizers and biopesticide applications, which makes them a very effective organic alternative (Qessaoui et al., 2019; Singh et al., 2020). Several rhizosphere bacteria, including Pseudomonas spp., Bacillus spp., and Alternaria spp., are being used to successfully control soilborne diseases that have emerged in vegetable production (Agrillo et al., 2019; Zhang et al., 2020; Huang et al., 2021). PGPRs support plant growth under biotic stress by at least one of the direct and indirect mechanisms. For example, PGPRs increase the availability of nutrients to growing plants under nutritional imbalance conditions by influencing solubility or uptake of nutrients to improve plant vigor and increase root development (Etesami and Maheshwari, 2018). They can indirectly repress pathogens by stimulating the plant's defense system. Induced systemic resistance (ISR) is typically activated upon colonization of plant roots by beneficial microorganisms, and it is associated with priming for enhanced defense rather than direct activation of defense (Pozo et al., 2008). This priming leads to an enhanced expression of host defense-related genes upon subsequent attack by a pathogen.
Alcaligenes faecalis is a gram-negative bacterium that is widespread in soils. As a PGPR, A. faecalis has been reported to have an active role in promoting the growth and development of plants (Li, 2011). Several investigations have shown that A. faecalis has antifungal activity against Aspergillus niger, A. flavus, Paecilomyces variotii, Candida albicans, Alternaria alternata, Cercospora arachicola, Rhizoctonia solani, and Fusarium oxysporum (Honda et al., 1999; Santoa et al., 2011; Sayyed and Patel, 2011). In recent years, A. faecalis has been found to biodegrade organic pollutants such as anionic surfactants (Fedeila et al., 2018), which is of great significance to research in the field of environmental protection. However, to our knowledge, there is no evidence as to whether A. faecalis can induce tolerance against Pb stress in cruciferous vegetables. In this study, we have isolated microorganisms from a healthy cabbage rhizosphere and have selected a bacterium, A. faecalis Juj3, with growth-promoting characteristics. To characterize the roles of A. faecalis on cabbage responses to Pb stresses, we assessed the effects of inoculating cabbage with Juj3 on (i) plant growth and development, (ii) disease resistance responses, and (iii) the infestation levels of Pb in greenhouse and field trials.
Materials and methods
Isolation of rhizospheric bacteria
Rhizobacteria soil samples of healthy cabbage were collected from a field infected with Pb at Baoji, Shaanxi, China (107°18′35″ E, 34°2′51″ N). Isolation of bacteria from cabbage rhizospheres was performed as previously stated (Cordovez et al., 2018). Briefly, the floating soil of the cabbage root was shaken off, and a sterile brush was used to gently brush the soil within 4 mm near the root as rhizosphere soil. Subsequently, the soil sample was air-dried for 3 days and passed through a 200-mesh sieve. Ten g rhizosphere soil was placed in 100 mL sterile distilled water (SDW) and shaken for 30 min at 180 r/min. The supernatant was serially diluted in SDW (10−1 to 10−7) and plated on Luria-Bertani (LB) medium (10 g NaCl, 10 g tryptone, 5 g yeast extract, and 15 g agar per liter). After incubation at 30°C for 1–2 d, different colonies on the agar plates were observed. The morphologically distinct colonies of isolates were selected and streaked on LB plates. Purified bacterial cultures were preserved in 50% v/v glycerol stock at −80°C for further experiments.
Inoculum production for the isolates
The isolated bacteria were cultivated on LB plates for 24 h at 30°C. A single colony was selected and incubated in conical flasks containing liquid LB (250 mL conical flasks, 100 mL each) at 30°C with shaking at 180 rpm for 48 h. The fermentation broth (FB) was then harvested and prepared for root irrigation test. After centrifuging at 8,000 rpm for 15 min, the supernatant was filtered through a 0.22 μm cellulose nitrate filter as cell-free suspensions used for metabolite detection. The cells were collected by centrifugation and resuspended in SDW as bacterial suspension (BS) used for seed treatment.
For formulations preparation, solid fermentations were carried out following the method described by Guo et al. (2020) with a slight modification. One kilogram powder could be generated with solid fermentation material composed of 600 g soybean meal, 600 g bran, 100 g sucrose, 100 g yeast extract, 1 g MgSO4, 0.2 g MnSO4, and 1,800 mL water. After all, the ingredients were mixed for about 60 min, and the solid fermentation medium was packed in 250 mL conical flasks (80 g per flask) and autoclaved for sterilization. The FB was inoculated in the sterile solid fermentation medium in a volume ratio of 1/10 and incubated at 30°C for 72 h. Finally, the semi-processed fermentation medium was dried in a 40°C oven for 96 h, crushed by a grinder, and the powder was collected from across a 200-mesh sieve used for the greenhouse trial.
Pathogen inoculum preparation
Clubroot galls were collected from diseased cabbages in a heavily infested field, washed, dried, and stored at −20°C until used. The crude resting spores were extracted based on the method of Zhu et al. (2019) with slight modifications. Briefly, about 10 g of stored galls were immersion in 50 mL SDW at 25°C for 2 h to soften the tissue and then were ground in a mortar. The resulting suspension was filtered through 8 layers of gauze. The obtained Pb suspension was estimated for resting spores concentration with a hemacytometer and diluted with SDW to 1 × 107 spores/mL as crude inoculum. For Pb inoculation, a soil-free planting mix was infested with a Pb suspension (1 × 107 resting spores/mL) to result in an infestation level of about 2 × 106 spores per gram of growth medium. The growth medium was then sealed for more than 48 h at 23°C before planting.
Screening of bio-control strains with plant growth-promoting traits
Siderophore production
The detection of siderophore production was performed according to a modified method by Bonaldi et al. (2015). A mixture of 10 mL of Chrome-Azurol S (CAS) reagent and 90 mL of sterilized LB agar medium was used for siderophore detection in CAS agar plates. After incubation, the formation of a clear zone around the colonies was reported as positive. The experiments were conducted in triplicate.
IAA-like compounds production
Indole-3-acetic acid (IAA) production was determined via Salkowski's colorimetric method (Sachdev et al., 2009). Briefly, 1 mL of cell-free suspensions was added to 2 mL of Salkowski reagent. After 30 min of incubation, the appearance of the color pink indicated a positive production of IAA. The experiments were conducted in triplicate.
Growth in nitrogen-free medium
The strains were evaluated for their nitrogen fixation ability on Burk's modified N-free medium (pH 7.00) (Franche et al., 2008). In short, the appearance of bacterial growth on the medium was taken as a positive test for the nitrogen fixation experiment. The experiments were conducted in triplicate.
Phosphate solubilization
The phosphate-solubilizing activities of the strains were evaluated on the organophosphorus medium (Yang and Yang, 2020). Following bacterial culture at 30°C for 5 d, the occurrence of a transparent phosphorus solubilizing circle on the medium was interpreted as the bacteria having the capability of dissolving organic phosphorus. The experiments were conducted in triplicate.
In vitro plant growth promotion assays
For in vitro assays, the Haoyuan (Shanghai Xinglv Vegetable Seedling Research Institute) cabbage variety that is susceptible to clubroot disease was used in this study. Cabbage seeds were surface-sterilized by soaking in 70% ethanol for 2 min, immersed in 10% sodium hypochlorite for 5 min, and rinsed four times with ddH2O to remove residues. At least 27 sterile seeds were soaked in bacterial suspensions at concentrations of 1 × 106 colony-forming units (CFU)/mL or SDW for 2 h to make Juj3 colonize on the cabbage seeds, followed by rinsing three times in ddH2O. Seeds were then germinated on 90 mm diameter Petri dishes containing two water-soaked filter papers in a climate cabinet at 23°C (16 h: 8 h, light: dark). The germination rates of the seeds were tested on the second day, and three replicates were used per treatment.
To observe the effects of the isolates on cabbage growth at the seedling stage, a root elongation test was conducted in a growth pouch. Surface-sterilized cabbage seeds were inoculated with the isolates described above. Twenty-five seeds per treatment were transferred into sterile growth pouches that had been saturated with sterile Hoagland's nutrient solution. Three replicates were used for each treatment, and the seedlings were kept in the dark at 23°C for 1–2 days until they germinated. They were then cultured at 23°C for 5 days under a 16-h photoperiod, and their plant heights and root lengths were measured.
Identification of Juj3
Morphological and molecular characteristics of Juj3 were used for taxonomic identification after streaking on LB plates and incubation at 30°C for 48 h and observations by scanning electron microscope (Hitachi model S-3400N, Japan). To characterize isolates phylogenetically, 16S rRNA genes were amplified by Polymerase Chain Reaction (PCR) with the primers 27F (5′-ATGCCATTCGTGCGGAGGTTG-3′) and 1492R (5′-CGTCTCTGCTGTCATCACTTCGTAT-3′) (Zhang et al., 2021). Genomic DNA extractions from the Juj3 strain were performed by use of the Bacterial Genomic DNA Extraction kit (Tiangen Biotech co. LTD). The 25-μL reaction mixtures contained 2 μL of DNA template, 1 μL (0.4 μM) of each primer, and 12.5 μL Taq Mix (Vazyme). PCR conditions for 16S rRNA gene amplification were as follows: 95°C for 5 min, followed by 35 cycles of 95°C for 30 s, 55°C for 30 s, and 72°C for 1.5 min. A final extension step was carried out at 72°C for 10 min. The amplified 16S rRNA fragments were sequenced (Qingke, Xian, China) and submitted to the EzTaxon-e server (http://www.ezbiocloud.net/) for comparative analyses. Phylogenetic trees were constructed with MEGA 7.0 software using the Maximum Likelihood method with 1,000 bootstrap replications (Kumar et al., 2016b).
Effects of Juj3 on photosynthesis and root viability of cabbage seedlings
Experimental design
Photosynthesis and root viability assays were carried out under normal and stress conditions to determine whether Juj3 could enhance plant health and growth. For the Pb stress test, sterile cabbage seeds were sowed in the plant plug trays (15 × 7-well) filled with a growth medium containing Pb (2 × 106 spores/g). Fermentations with different root irrigation concentrations of Juj3 (1.00 × 106, 1.00 × 105, and 1.00 × 104 CFU/mL) with 25 mL per pot were performed at 7 days and 14 days after seeding. Six hundred times dilutions of cyazofamid (20%) with 25 mL per pot were served as positive controls, and blank controls received 25 mL of water only. The non-stress experiments were conducted in plant plug trays filled with V9 soilless planting mix in which sterile cabbage seeds were inoculated with Juj3, cyazofamid, and water, respectively, as described above. All treated plants were placed in a growth cabinet at 23°C with a 16-h photoperiod. Each treatment consisted of five plants in a trial, and the trial was replicated at three different times. The physiological indexes such as photosynthesis, chlorophyll content, and root viability were determined after 30 days of sowing.
Photosynthesis measurement
Leaf photosynthetic characteristics (i.e., the net photosynthetic rate, stomatal conductance, intercellular CO2 concentration, and transpiration rate) were measured on top leaves with a CIRAS-3 portable photosynthesis system (PP SYSTEMS CIRAS-3) on sunny days. The chlorophyll content was determined as described previously (Zhang et al., 2016; Zhou et al., 2021). In brief, 0.10 g of fresh cabbage leaf tissue was harvested from each treatment, homogenized in 80% acetone, and centrifuged at 8000 r/min for 5 min. Then, the supernatant was obtained, and absorbance was measured through the UV-Vis spectrophotometer at 645 nm and 663 nm.
Analysis of root activity
Root activities were measured by the triphenyl tetrazolium chloride (TTC) method. After weighing the roots, they were immersed in 10 mL of an equally mixed solution of 1% TTC and phosphate buffer and kept in the dark at 37°C for 1 h. Subsequently, 2 mL of 1 M H2SO4 was added to end the reaction with the root. Samples, carefully wiped and cut into segments, were then dried and ground with 3–4 mL ethyl acetate and a small amount of quartz sand to extract triphenyl tetrazolium formazan (TTF). Transfer the red extract into the test tube with a small amount of ethyl acetate, washing the residue two to three times, and then transferring the residue into the test tube. Finally, add ethyl acetate to make the total amount of 10 ml, and the absorbance of the extract at 485 nm was recorded. Root activity was expressed as TTC reduction intensity, and the formulae are described below.
Relative expression of cabbage defense-related genes
Surface-sterilized cabbage seeds were inoculated with the Juj3 as described in Section In vitro plant growth promotion assays, and seeds soaked in SDW were used as controls. After being fully air-dried, the seeds were then sowed in the plant plug trays (15 × 7-well) filled with the growth medium containing Pb (2 × 106 spores/g). All treated plants were placed in a growth cabinet at 23°C with a 16-h photoperiod. Each treatment consisted of five plants in a trial, and the trial was replicated at three different times. Fourteen days after sowing, roots from three cabbages (a replicate) as well as the first true leaves of each plant were collected. All harvested samples were immediately put into liquid nitrogen. Total RNA from cabbages were extracted using Trizol and converted to cDNA using Hipure Plant RNA Mini Kit (Magen) protocols.
To examine the defense gene expression in cabbage, a quantitative real-time PCR (qRT-PCR)-based assay was developed for several putative genes, such as pathogenesis-related protein 1 (PR1), PR2, PR3, transcription factor ethylene insensitive 3 (EIN3), phenylalanine ammonia-lyase (PAL), and NIMI-interacting 2 (NIMI2). The gene Actin was chosen as the internal control gene. Sequences for gene-specific primer pairs were designed based on sequences available in the GenBank database, and primer sequences were designed using Primer Premier 5.0. Details of these genes and their primer sequences are given in Supplementary Table S3. The qRT-PCR was performed using UltraSYBR Mixture (CWBIO, China), and the amplification was performed according to the manufacturer's instructions. The relative fold change of target gene expression in Juj3-treated plants was calculated and normalized against its expression levels in water-treated plants, using the ΔΔCt method as previously described method (Kenneth and Thomas, 2001).
Greenhouse experiments
Effects of Juj3 on cabbage seedling growth
For seedling growth assays, surface-sterilized cabbage seeds were sown in substrates mixed with different doses of Juj3 solid fermentation powder. The numbers of viable bacteria in the Juj3 bacteria power were counted. The Juj3 bacterial powder and sterile substrates were mixed uniformly at a mass ratio of 1:9, 1:19, 1:39, 1:79, and 1:159 to provide final bacteria concentrations in the soil of 1.00 × 107, 5.00 × 106, 2.50 × 106, 1.25 × 106, and 6.25 × 105 CFU/g, respectively. Seeds were germinated in 72-cell plant plug trays filled with the above growth medium and were sown in sterile substrates as blank controls. Two seeds were sown per hole, and each treatment was repeated three times. The fresh weight, dry weight, root weight, shoot weight, stem diameter, and plant heights were measured 35 days after sowing.
Biocontrol assay
To evaluate the biocontrol efficacies of the Juj3 strain against clubroot under greenhouse conditions, seeds were processed by combining Juj3 solid formulations and fermentation broth. Based on the experimental results of the Section Effects of Juj3 on cabbage seedling growth protocols, a Juj3 formulation of 2.50 × 106 CFU/g was mixed evenly with the soil-less mix. Seeds were sown in plant plug trays filled with the mixture and grown under the same conditions as above. Then after 35 days of culture, single cabbage seedlings were transplanted into nutrient bowls filled with the Pb mix. For root irrigations, the initial Juj3 fermentation broth was diluted with SDW to produce 2-, 4-, and 8-fold diluted suspensions. Root irrigations were performed at transplanting time, 7 days, and 14 days after transplanting with 25 mL of solution for each pot. Positive controls consisted of 600-fold dilutions of 20% cyazofamid, and SDW was used as a blank control. Each treatment involved 30 transplanted seedlings, and the experiment was repeated three times. The treated plants were kept in a growth chamber at 23°C (16-h photoperiod, 90% relative humidity for 40 days. A disease severity index (DSI) was then calculated by the Klewer (2009) formula:
where n is the number of plants in a class; N is the total number of plants in an experimental unit; and 0, 1, 2, 3, and 4 are the symptom severity classes. The control efficiency formula was:
where CDI refers to the DSI of the control group, and TDI denotes the DSI of the treatment group.
Field trials
Experimental design
To evaluate the biocontrol effects of Juj3 on clubroot disease under field conditions, we chose the Haoyuan cabbage and Chinese cabbage (Qingza No. 3, Qingdao Xingye Seeds Co., Ltd.) as the target vegetables. The experimental test plot was located in Tangkou Village, Taibai County, Shaanxi province (107°18′35″ E, 34°2′51″ N). Two fields infested with Pb were used for testing from March 2018 to October 2018. The preceding crop was Chinese cabbage. Before winter, subsoiling and basal fertilizers of N, P, and K 100 kg/667 m2 were applied to the experimental plots.
Seeds were sown in 72-cell plant plug trays filled with substrate, with 2 seeds per hole to ensure germination. After sowing, the seedling trays were placed on pre-leveled seedling beds in the experimental field, and the soils were kept moist throughout the seedling stage. Both Cabbage and Chinese cabbage field trials included seven treatments with three replications consisting of a planting density of 35 × 50 cm in a completely randomized block design. There were 5 concentration gradients of fermentation broth in biocontrol treatment, and 2-fold serial dilutions (2-, 4-, 8-, 16-, and 32-fold) were made in SDW. The 600-fold dilutions of 20% cyazofamid were used as a positive control, and SDW acted as a blank control. Root irrigation was carried out at transplanting time, 7 days, and 14 days after transplanting, and the amount of root irrigation was 100 mL/plant per time. The area of each plot was 0.0012 hm2, and there were 42 plots in this trial. An isolation zone of 1 m was set up to prevent water and gas flow. A protection line was set around the test site, and the field was routinely managed.
Measurements
A five-point sampling method was used, and at least 10 plants were obtained to calculate the cabbage and Chinese cabbage disease indexes in each plot. The total number of inspected plants and the number of diseased plants at each grade were recorded. Infected cruciferous vegetables were scored according to the following: 0, no galls; 1, a few small clubs on the lateral roots; 3, most lateral roots swelling and obvious swelling of expanding primary roots; 5, enlarged napiform taproot, no wilting and yellowing symptoms; 7, all taproots are swollen but not rotted, plant wilting or yellowing and growth cessation; 9, swollen and rotten roots, and nearly dead plants. Field disease severity indexes (FDSI) were calculated as follows, and the field control efficacy was counted as described above in Section Biocontrol assay.
To assess the effects of Juj3 on the cabbage's growth and quality, ten plants per plot were used for the experiments. The relevant growth-promotion parameters, including cabbage height, commodity fresh weight, and stem diameter, were evaluated at the yield stage. Concurrently, the height and commodity fresh weight of Chinese cabbage were investigated. A group of treatments with the best control effect of Juj3 was selected to determine cabbage leaf quality. The nitrate, total amino acid, vitamin C and reducing sugar contents were measured using salicylate spectrophotometric, ninhydrin colorimetry, ultraviolet spectrophotometry, and 3, 5-dinitrosalicylic acid (DNS) method (Miller, 1959; Stevens et al., 2006; Wei et al., 2018), respectively.
Data analysis
All data were presented as mean values ± standard deviation (SD), except for data from field experiments, and the values are presented as ± standard error mean (SEM). Data were subjected to analysis of variance (ANOVA) followed by Duncan's multiple range tests using SPSS11.0 (SPSS Inc., Chicago, IL, USA). Different lowercase letters indicate a significant difference at the 0.05 level. Data were analyzed by the two-tailed t-test using the Student's t-test, as appropriate.
Results
PGP attributes of the isolates
Eighteen morphologically different bacteria strains (numbered Juj1 to Juj6, Cot1 to Cot4, Gra1 to Gra5, and Cab1 to Cab3) were isolated from rhizosphere soils collected in Taibai County, Shaanxi Province, China (data not shown). The isolates were analyzed for their plant growth-promoting (PGP) attributes. Isolates Juj3, Juj4, Juj5, Cot1, Gra1, and Gra3 were promising for PGP attributes (Supplementary Table S1), which showed nitrogen fixation abilities (Supplementary Figure S1) and siderophore production (Supplementary Figure S2). The Juj3 strain was positive for phosphorous solubilization ability (Supplementary Figure S3). None of the six isolates affected cabbage seed germination (Figures 1A,B). Among these isolates, Juj3 was rated the best because it could enhance the length of cabbage seedlings and facilitate plant growth. As shown in Figure 1B, although there were no significant differences from the control, the germination rates of cabbage seeds treated with Juj3 were 5.2% higher than the control group. The significant effects of Juj3 on the root length and bud length of the seeds at early time points (5 dpi) increased by 49.0% (Figure 1C) and 13.4% (Figure 1D) respectively compared with the control (Figure 1E).
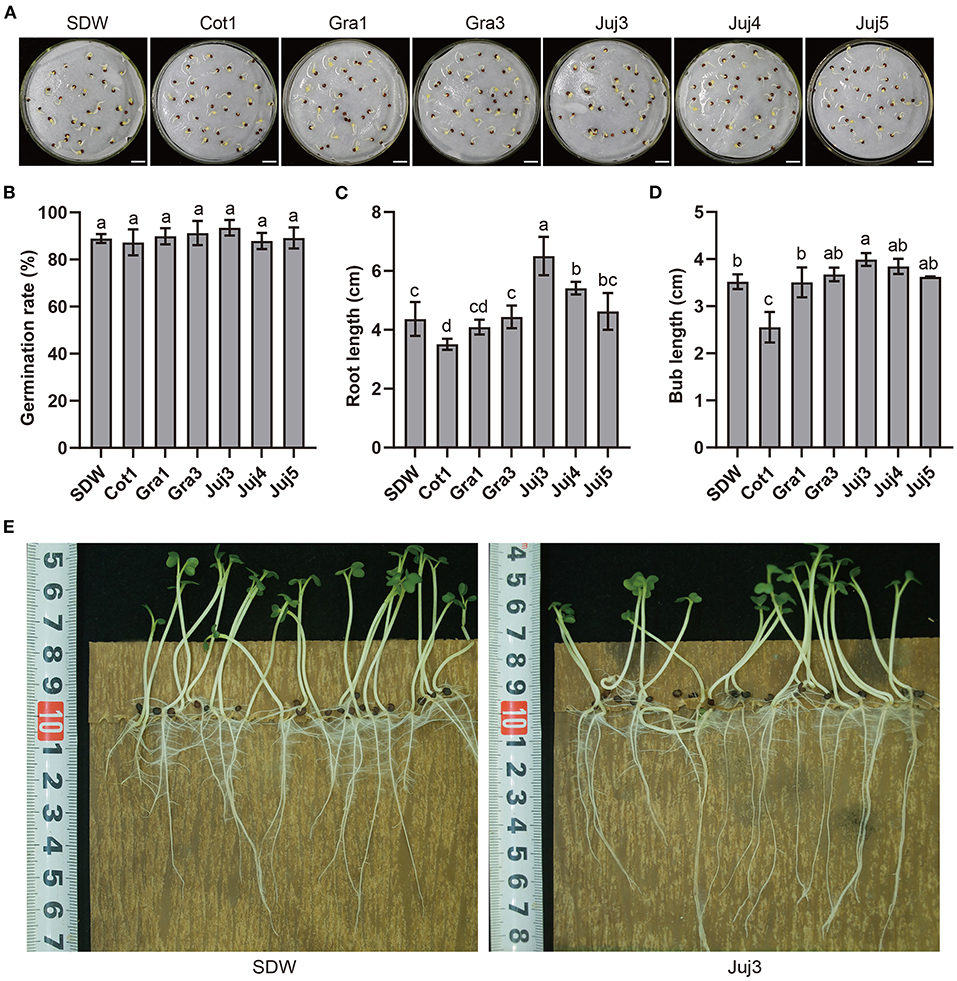
Figure 1. Cabbage seeds growth-promoting effects of the isolates. (A) Effect of six isolates on cabbage seed germination. Bar = 1 cm. (B) Seed germination rates after isolate treatments. Germination rates were determined 2 days after sowing. Values in the chart were mean ± SD. Different lowercase letters indicate significant differences at the 0.05 percent levels by the Duncan's new multiple range test. (C,D) Average root and bud lengths (cm) of cabbage treated with the six isolates. (E) Effects of Juj3 on cabbage growth at the 5-day-old seedling stage.
Identification of Juj3
The rhizosphere bacterium Juj3 was identified as Alcaligenes faecalis based on phenotypic characterization coupled with phylogeny analyses of 16S rRNA gene sequences. Morphologically, the Juj3 colonies after 48 h incubation on LB medium were creamy white in appearance, had a smooth surface, with smooth, regular margins, and were opaque, moist, and did not produce pigment (Figure 2A). Under the scanning electron microscope, the Juj3 cells were rod-shaped with peritrichous flagella and consisted of cell sizes between 0.6 and 1.0 μm that developed into long and elliptical spores (Figure 2B).
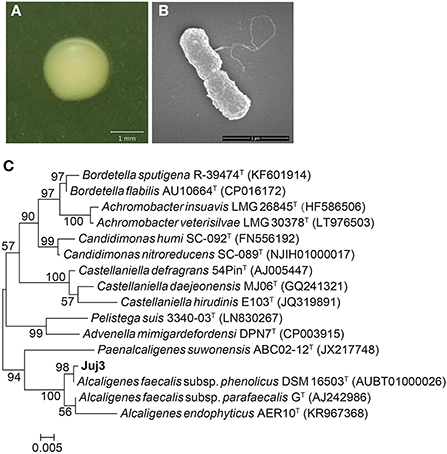
Figure 2. Identification of Juj3. (A) Morphological features of Juj3 on LB agar plates. Bar = 1 cm. (B) Scanning electron microscopy (SEM) photo of Juj3. Bar = 1 μm. (C) Phylogenetic tree based on the partial nucleotide sequences of 16S rRNA genes. Numbers between the segments represent bootstrap support values, and “T” above represents the strain as a model strain.
To confirm the Juj3 phylogenetic relationship with A. faecalis, we used the original 16S rRNA sequences available in the NCBI SRA database with the accession number ON028636 to conduct alignments in the EzTaxon-e server. Analysis of the 16S rRNA sequences demonstrated that Juj3 matches A. faecalis subsp. Phenolicus DSM 16503 at 99.3% in the EzTaxon-e server, and they cluster into a single group (Figure 2C). These results clearly show that strain Juj3 is A. faecalis.
Photosynthetic rate, chlorophyll content, and root activity analysis
To further investigate the physiological impacts of Juj3 on cabbages, we determined the photosynthetic rates, chlorophyll content of leaves, and root activity of Juj3 treatments during normal and Pb-stress conditions.
Photosynthetic rate
Photosynthesis is the basis of plant growth and development, and the rate of photosynthesis directly determines the growth rate of plants. As shown in Figure 3, photosynthesis of Pb-infected cabbage plants was significantly lower than healthy plants. Stomatal conductance was also markedly (P < 0.01) reduced after Pb infection and the CK treatment values under Pb stress were 57.5% lower than that of healthy plants (CK-normal) (Figure 3B). Regardless of whether cabbages were grown under normal conditions, all photosynthesis-related parameters except for intercellular CO2 concentrations were maintained at relatively high levels or were significantly improved after the addition of Juj3 at concentrations of 1.00 × 106 CFU/mL (T1) (Figure 3D). Under Pb stress after the addition of Juj3, the leaf assimilation rates, stomatal conductance, and transportation rates increased by 45.2% (Figure 3A), 0.2% (Figure 3B), and 7.8% (Figure 3C), respectively, each of which were significantly higher than that of the CK group. Similar trends (~1.3-fold greater than CK treatments) were observed under normal conditions when the concentration of Juj3 was 1.00 × 106 CFU/mL (Figure 3A).
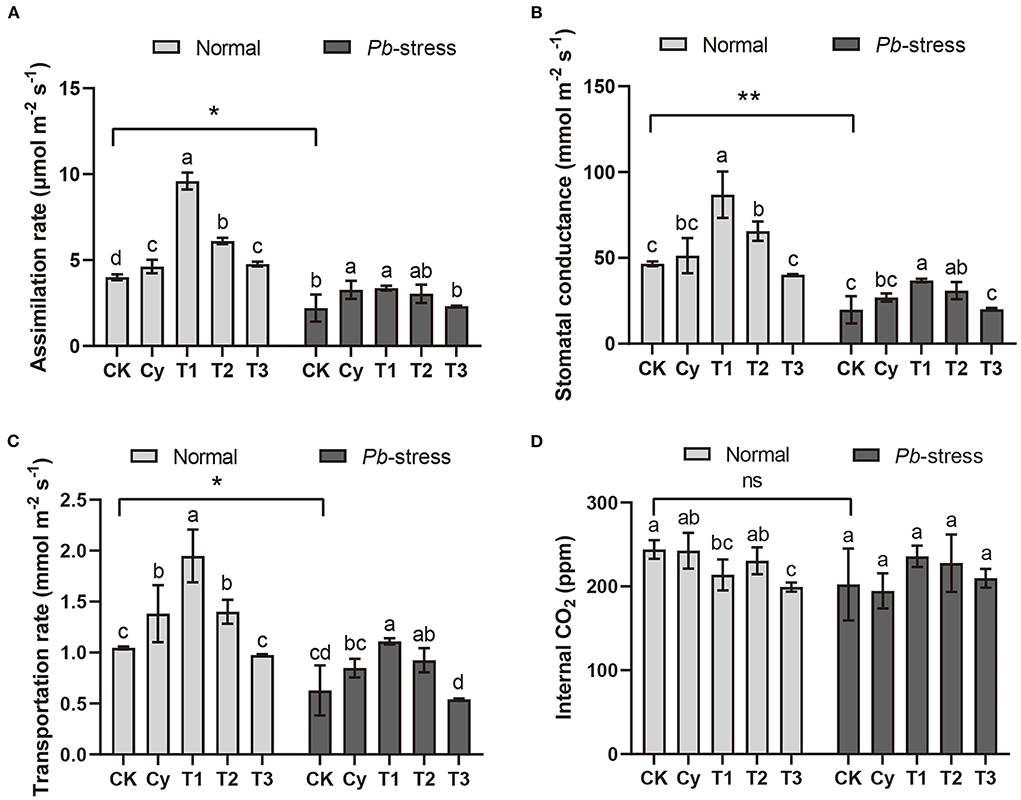
Figure 3. Photosynthetic parameters of cabbage leaves under different treatments. (A) assimilation rate; (B) stomatal conductance; (C) transpiration rate; and (D) intercellular CO2. CK: SDW; Cy (50 mg/L, cyazofamid). T1 (1 × 106 CFU/mL), T2 (1 × 105 CFU/mL), and T3 (1 × 104 CFU/mL) with different concentrations of Juj3 fermentation broth. Values in the chart show the mean ± SD. Different lowercase letters indicate a significant difference at the 0.05 percent levels by Duncan's new multiple range test. An asterisk indicates significant differences based on the unpaired two-tailed Student's t-test with P-values marked (*P < 0.05; **P < 0.01) between the normal-CK and Pb-stress-CK treatments. “ns” indicates no significant difference.
Chlorophyll content
To explore whether changes in photosynthetic pigment content resulted in photosynthesis alterations, we examined three different photosynthetic pigment content traits: chlorophyll a, chlorophyll b, and total chlorophyll (Table 1). In summary, the chlorophyll content of all treatment groups after Pb infection was higher than under the normal environment, and the major changes were for chlorophyll b. In the case of Pb-stress, the chlorophyll content of the T1 treatment (1.00 × 106 CFU/mL) group decreased significantly, compared with the control group, and there was no significant difference with cyazofamid (Cy) treatment. Under normal conditions, each concentration of Juj3 increased the chlorophyll a and total chlorophyll contents to different degrees. When the concentration was 1.00 × 106 (T1) and 1.00 × 105 CFU/mL (T2), the chlorophyll a content was enhanced significantly compared with the control. The T1 treatment increased total chlorophyll contents, and there was no significant difference in comparison to the CK. The T2 treatment increased the total chlorophyll content by 23.3% compared to the control.
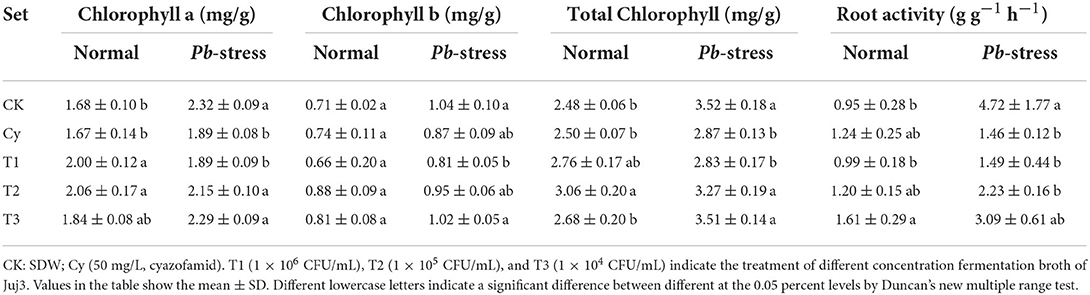
Table 1. Effects of Juj3 and cyazofamid on chlorophyll content and root activity in cabbage seedlings.
Root activity
As a good root metabolic reactive agent, TTC receives electrons directly from the electron transport link, and transport reductions are directly related to the mitochondrial respiration rate (Comas et al., 2000; Ruf and Brunner, 2003). In our experiments, there was a significant increase in the root TTC activity under Pb stress (Table 1). For CK treatments, the TTC reductive intensity in roots with Pb-infected was 4.0-fold higher than in healthy cabbage roots and the root activities of CK treatments were higher than in other Pb stress treatments. The T1 (1.00 × 106 CFU/mL) and T2 (1.00 × 105 CFU/mL) treatments were significantly different CK treatments and were very similar to the Cy treatments. The TTC reductive intensity in all three concentrations of Juj3 was higher than with CK, and the most significant difference was from the T3 treatment.
Beneficial rhizobacteria trigger changes in the expression of defense-related genes
We evaluated if the application of strain Juj3 could activate the expression of defense-related genes in cabbage. When cabbage plants were treated with Juj3 plus Pb, gene EIN3 (transcription factor ethylene insensitive 3) activity generally and its expression increased 3.4- and 1.1-fold in the roots and leaves relative to Pb inoculated (Figure 4). The gene expression related to PR2 protein biosynthesis (β-1,3-glucanase) was also induced by inoculation with Juj3 plus Pb. Even though the gene expression of PR2 was increased 2.8-fold compared with the controls, the expression of this gene did not change significantly in leaves. The genes PR1 and NIMI2, associated with salicylic acid (SA) signaling pathways, showed the same expression tendency. That is, gene expression was not significantly different from control in the root but significantly decreased in leaves. The gene PR3, encoding a class I chitinase, was down-regulated in leaves of treated plants, although the level of gene PR3 expression in roots was not significantly different from the control. Only expression of gene PAL (involved in the phenylpropanoid pathways) decreased substantially in roots and leaves.
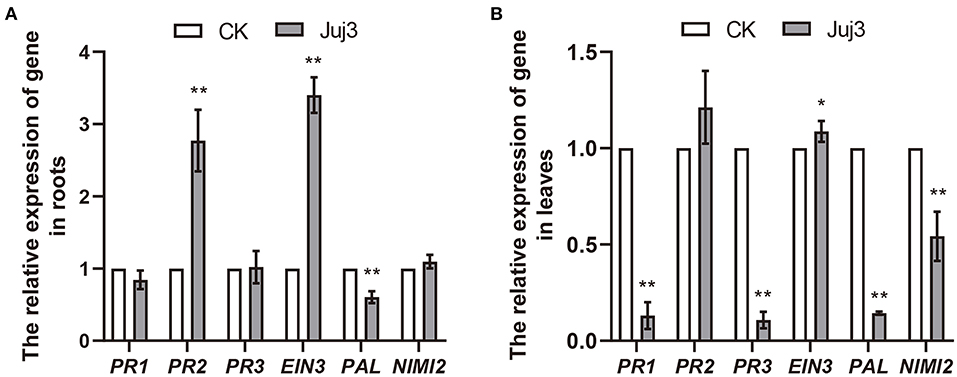
Figure 4. Effects of Juj3 on expression levels of cabbage disease resistance-related genes. (A,B) represent the expression of disease resistance-related genes in cabbage roots and leaves. Values in the chart show the mean ± SD. An asterisk indicates significant differences between the CK and Juj3 treatments based on the unpaired two-tailed Student's t-test with P-values marked (*P < 0.05; **P < 0.01).
Growth-promoting effects of Juj3 on cabbage in planta and plot experiments
Growth-promoting effects of Juj3 on cabbage at the seedling stage were assessed after 35 days of seeding in the pot experiments. As shown in Figure 5A, cabbage treated with Juj3 have better developmental processes. However, seedling emergence of cabbage was inhibited by 1.00 × 107 CFU/g concentrations of Juj3 (data not shown). According to the bar graph (Figures 5B–G), among 6 parameters, the cabbage dry weight, stem diameter, and height significantly increased during Juj3 treatment. Concentrations of 2.50 × 106 and 1.25 × 106 CFU/g significantly increased the numerical data for all the test parameters compared to the SDW control and exhibited the best growth-promoting effects among the treatments.
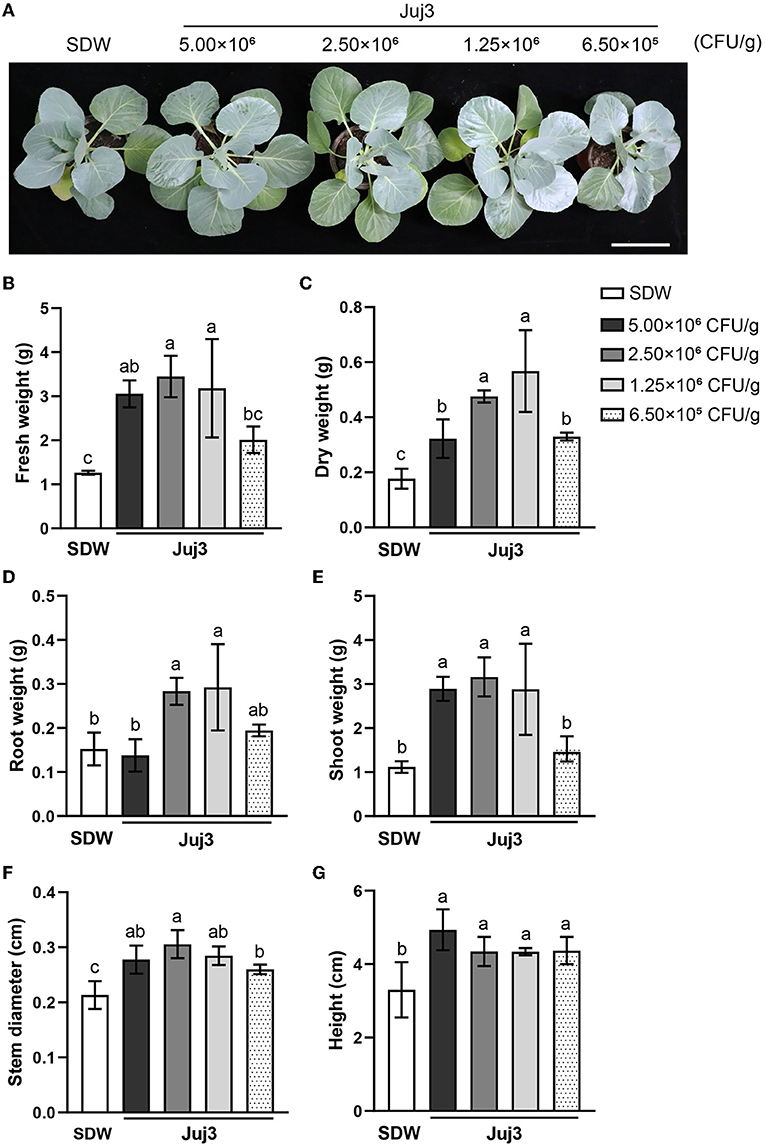
Figure 5. Dosage effects of various concentrations of Juj3 solid fermentation powder (SFP) on cabbage seedling growth. (A) Cabbage growth at 35 days after sowing. Soil treatments were carried out with different Juj3 SFP doses before sowing. SDW: sterile distilled water. Bar = 10 cm. Effect of Juj3 on the (B) fresh weight, (C) dry weight, (D) root weight, (E) shoot weight, (F) stem diameter, and (G) height of cabbage seedlings. Values in the chart are the mean ± SD. Different lowercase letters indicate significant differences at the 0.05 percent levels by Duncan's new multiple range test.
In field trials, the cabbage and Chinese cabbage biomasses were measured at the harvest stage. The fresh weights of plants treated with Juj3 and combined with 8-fold (8n) and 16-fold (16n) dilutions of fermentation broth had significant improvements in fresh weight in both cabbage (Figure 6A) and Chinese cabbage (Figure 6B) when compared to the controls (CK). As shown in Supplementary Table S2, 2-fold (2n), 4-fold (4n), and 8-fold (8n) dilutions of fermentation broth significantly increased the cabbage and Chinese cabbage height and cabbage stem diameter. Additionally, the results of quality parameter measurements demonstrated that Juj3 fermentation broth resulted in a significant improvement in cabbage quality. Juj3 reduced the nitrate content in cabbage leaves to <19.3% of the control-treated cabbages and conspicuously elevated the reducing sugar contents to 26.0 % over the SDW treatment (Table 2). Unexpectedly, the vitamin C content in cabbages treated with Juj3 was 175.0 mg/100 g, ~1.17-fold higher than that of in-control plants.
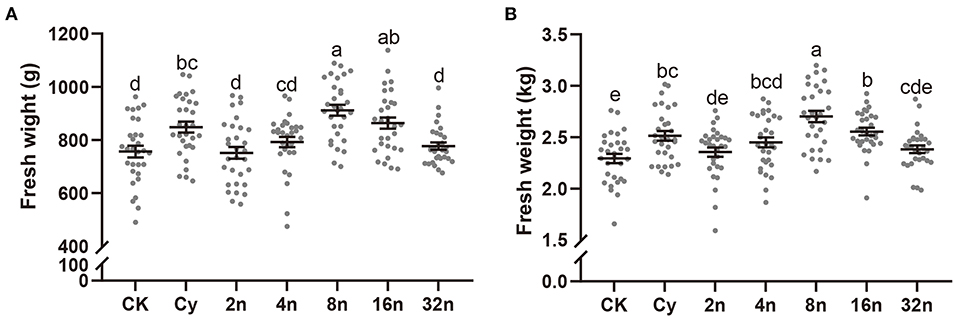
Figure 6. Commodities fresh weight of plants treated with Juj3 in the field. (A) cabbage fresh weight and (B) Chinese cabbage fresh weight. Cy (50 mg/L, cyazofamid). The Juj3 initial fermentation broth concentration was 1.0 × 107 CFU/mL, and “n” refers to the dilution factor. Values in the chart represent the mean ± SEM. Different lowercase letters indicate significant differences between treatments at the 0.05 percent levels by Duncan's new multiple range test.
Control effects of Juj3 on cabbage clubroot in pot and field experiments
In the pot experiments, all treatments inoculated with Juj3 (2.50 × 106 CFU/g) in the sowing period exhibited significantly reduced disease indices when compared with the blank control (CK) (Figures 7A,B). Even roots irrigated with SDW at transplanting had significantly lower disease indexes (51.7) than the control (65.6). Among all treatments, the 2-fold dilution treatment (2n) resulted in the best cabbage clubroot biocontrol effects of 83.7%, which were significantly higher than the 73.7% cyazofamid treatment (Figure 7C).
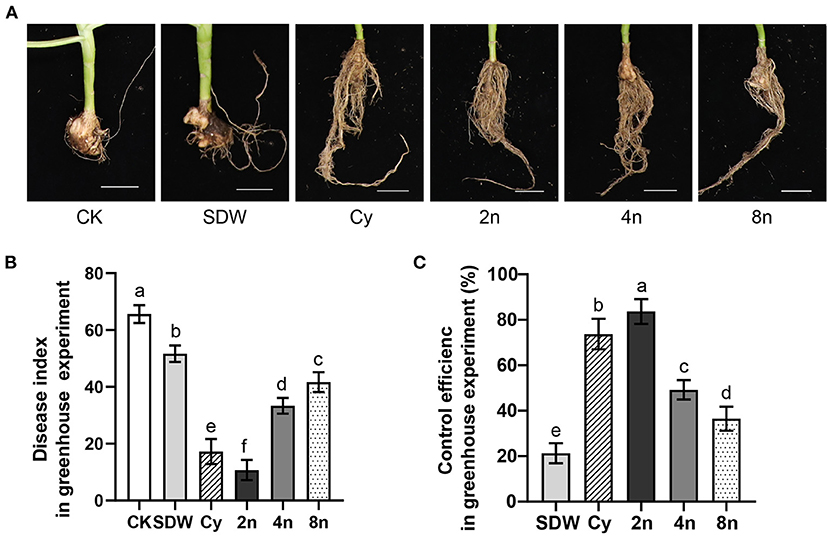
Figure 7. Biocontrol efficacy of Juj3 against cabbage clubroot in a growth chamber. (A) Effects of various treatments on the extent of cabbage clubroot development. The soil was mixed with SFP (final concentration, 2.50 × 106 CFU/g) at sowing and combined with root irrigation with different concentrations of Juj3 fermentation broth during transplanting. CK shows the blank control. Cabbage roots were irrigated with SDW, cyazofamid (Cy) and 2-fold (2n), 4-fold (4n), and 8-fold (8n) dilutions of Juj3 fermentation broth. The clubroot disease severity index was calculated at 40 days after transplanting. The Juj3 initial fermentation broth concentration was 1.0 × 107 CFU/mL, and “n” refers to the dilution factor. Bar = 5 cm. (B) Clubroot disease index for each treatment. Values in the chart show the mean ± SD. Different lowercase letters indicate a significant differences between treatments at the 0.05 percent levels by Duncan's new multiple range test. (C) The clubroot control efficiecy of each treatment.
In the field experiments, the clubroot disease severity was assessed according to the disease index at the harvest stage (Table 3). Viewed as a whole, the disease indices of the cabbage plots were generally lower than those of the Chinese cabbage plots. However, all five tested Juj3 concentrations had significant effects on disease severity compared with the control treatments, with the cabbage DSIs ranging from 18.0 to 30.4 and the Chinese cabbage ranges from 30.4 to 38.2. Among all of the Juj3 concentrations, in contrast, the 8-fold dilution treatment (8n) exhibited high control efficiency for clubroot in both cabbage and Chinese cabbage fields. The 8n treatment provided the highest control efficiency in the cabbage fields (51.4%) and was significantly higher than the cyazofamid group (32.7%). Taken together, these results indicate that Juj3 is a very effective clubroot biological control agent.
Discussion
Plant growth-promoting rhizobacteria (PGPR) provide attractive sustainable ways for agriculture to cope up with biotic and abiotic stresses (Alexander et al., 2021). These agents improve plant growth and development directly and indirectly. Direct effects include more effective nitrogen fixation, phosphate solubilization, siderophore, and phytohormone production and indirect effects are as biocontrol actions or activation of ISR in host plants (Vessey, 2003; Van Loon, 2007). Although Alcaligenes faecalis has been reported to enhance rice growth, it can also inhibit the growth of various pathogenic fungi (Li, 2011; Santoa et al., 2011). However, the roles that A. faecalis might play in the resistance of cabbage to Pb stresses have not been previously investigated. In this study, we isolated the A. faecalis Juj3 strain from healthy cabbage rhizospheres in severely infested disease fields and found that Juj3 can enhance cabbage growth and development and induce defense-related gene expression under Pb stresses, as well as decrease the clubroot disease index.
PGPR enhances the growth of crops either by the production of growth substances produced by them or by improving plant uptake of environmental nutrients (Khan et al., 2019). The results suggest that PGPR helps promote plant growth by an improving combination of physiological attributes such as asymbiotic N2 fixation, production of phytohormones such as IAA, siderophore production, solubilization of inorganic phosphate, zinc, potassium, and various trace elements, as well as by inhibiting deleterious fungal pathogen infections (Whipps, 2001; Kumar et al., 2020). Previous studies have demonstrated that A. faecalis affects PGPR due to the production of IAA, ACC-deaminase, the fungicidal siderophore bavistin, and phosphate solubilization and fixation of atmospheric nitrogen (Sayyed and Chincholkar, 2009; Kumar et al., 2016a). In the current study of Juj3, we tested multiple PGP traits during the initial screening process. Among the 18 rhizobacterial isolates evaluated, A. faecalis Juj3 had the most efficient nitrogen fixation, phosphate solubilization, and siderophore production properties. Moreover, Juj3 was shown to possess positive plant growth promotion activities that enhanced the growth of cabbage seedlings. Therefore, we selected the Juj3 as a PGPR to determine its biological role in the cabbage rhizosphere.
Bacterial inoculation to enhance the productivity of different crops has been practiced since the discovery of beneficial bacterial effects on plants. Applications of seed treatments contribute mainly to the survival efficiency of the bacteria in the soil and on seeds. Previous researchers have reported that seed treatments with PGPR improved germination and seedling vigor (Liu et al., 2019), plant emergence rates, biomass, and significantly increased plant health (Kusstatscher et al., 2020). Our results are compatible with these conclusions. We found that bio-inoculation with Juj3 fermentation powder before sowing improves the growth of cabbage and decreases the clubroot disease index at the seedling stage. In our field trials, the optimal root irrigation concentration for Juj3 fermentation broth was determined; it could promote cabbage and Chinese cabbage growth, improve the quality of cabbage leaves, and provide a positive effect on clubroot control. The results herein establish the foundation for Juj3 application in agricultural production practices.
Improved photosynthesis can increase plant growth and stress resistance (Bendaly et al., 2016). Therefore, studying the effects of PGPR Juj3 on plant photosynthetic parameters under Pb stress is of great significance for improving biotic tolerance. It is well-known that most pathogen invasions lead to a decline in the photosynthesis rate of the host (Walters et al., 2008; Kasuga et al., 2021). The net photosynthesis rate and related parameters of susceptible tomato leaves are affected by inoculation with Fusarium oxysporum f. sp. lycopersici or Verticillium albo-atrum (Lorenzini et al., 1997). The photosynthesis capacity of maize leaves is also inhibited by infection with Colletotrichum musae and Fusarium moniliforme, and these inhibitions are accompanied by sharp decreases in chlorophyll content (Pinto et al., 2000). Similarly, we found that Pb stress can reduce cabbage photosynthesis. It has been reported that PGPR improves the stomatal aperture to result in increased water uptake via roots and enhanced stomatal CO2 conductance compared to non-PGPR inoculated plants (Vejan et al., 2016). Other PGPR, such as Bacillus siamensis and Pseudomonas aeruginosa, have been reported to improve leaf photosynthesis and chlorophyll maintenance under stressful environmental conditions (Sarma and Saikia, 2013; Awan et al., 2020). Our study suggests that the Juj3 strain can effectively alleviate stress conditions by enhancing cabbage assimilation rates, transportation rates, and stomatal conductance.
Root activity reflects the ability of roots to synthesize, assimilate and transform essential substances such as amino acids and hormones. Several early studies found that Pb infection stimulates meristematic activity in the hypocotyl (Malinowski et al., 2012). In growing root galls, the pathogen alters host metabolism, especially plant hormones such as auxins and cytokinins that are important for cell division and elongation induction (Siemens et al., 2006; Ludwig-Müller, 2008). Overexpression of these hormones enhances abnormal lateral root growth to produce pathological galls. Our experiments identified a similar phenomenon in that Pb stress significantly increased the TTC content in cabbage roots. Devos et al. (2005) studied Chinese cabbage and found that the zeatin content increased earlier in infected plants during clubroot formation. Additionally, zeatin has been previously reported to promote chloroplast development and increase the chlorophyll content (Criado et al., 2009; Zulfiqar et al., 2020). This is probably the reason why the chlorophyll in Pb-stressed cabbage was higher than that of control plants in our study. Interestingly, inoculation with Juj3 improved root vigor and chlorophyll content of cabbage in normal settings; however, the two physiological indicators of cabbage treated with Juj3 were lower than control treatments under Pb stress. Thus, our results indicate that Juj3 can help cabbage reduce the Pb stress effects and maintain regular metabolic activity. But the specific mechanism mediating these effects remains to be determined.
Apart from their ability to promote plant growth, PGPRs have also been recognized to improve the health of plants by enhancing their defense system by the mechanism of ISR (Beneduzi et al., 2012; van de Mortel et al., 2012; Li and Chen, 2019). For instance, three rhizobacteria, Burkholderia territorii strain A63, B. metallica strain A53, and Pseudomonas geniculata strain 95, were found to effectively activate plant defense and significantly reduce symptom development in leaves challenged with Xanthomonas citri subsp. citri (Riera et al., 2018). Treatment of plant roots with the cyclic lipopeptides secreted from the strain Bacillus UCMB5113 resulted in disease suppression when challenged with Alternaria, indicating primed systemic defense as ISR (Asari et al., 2017). Additionally, the application PGPRs to the seeds or seedlings of certain plants have resulted in increased efficiency of ISR against several pathogens (Ramamoorthy et al., 2001). Regardless of the method of its application (foliar spraying or soil drenching), the 100-fold-diluted fermentation broth of Streptomyces sp. JCK-6131 suppressed the development of tomato bacterial wilt and cucumber Fusarium wilt by ~50%, suggesting that strain JCK-6131 could enhance plant defense resistance (Le et al., 2021). Our study demonstrated that strain Juj3 reduces the clubroot disease index and provides a higher biocontrol efficacy in the greenhouse than in the field. Many studies have also found that PGPR elicits greater greenhouse than field effectiveness (Boregowda et al., 2019). The complex field environment likely contributes to this reduced effectiveness.
Emerging evidence has shown that phytohormones, especially SA and jasmonic acid (JA), and ethylene (ET) signaling pathways regulate plant defense against various pathogens (Zhao et al., 2009). The gene PR2 induction in plants provides them resistance against biotrophic fungi and is regulated by SA to activate systemic acquired resistance (SAR) (Ali et al., 2017). Researchers have indicated that an exogenous application of SA to broccoli resulted in up-regulation of PR1 and PR2 genes and a moderate reduction in clubroot severity (Lovelock et al., 2012). Heteroconium chaetospira (Hc) plus Pb up-regulated the expression of PR2 relative to Pb inoculation alone (Lahlali et al., 2014). The strong expression of PR2 in this study, coupled with the evidence above, suggests the possible involvement of PR2 in Juj3-mediated plant defense against clubroot. PAL is involved in the biosynthesis of SA through the PAL pathway, and it is a critical enzyme catalyzing the first step of phenylpropanoid biosynthesis controlled by a multi-gene family (Zhao et al., 2009). A previous study reported that the genes BnCCR and BnOPCL, encoding phenylpropanoid pathways enzymes, were up-regulated in strain Clonostachys rosea treated plants. In another work, strain Hc treatments generally increased PAL activity, but the expression of the BnCCR and BnOPCL genes was not up-regulated (Lahlali et al., 2014). In the current study, gene PAL was not up-regulated by Juj3 plus Pb when compared to Pb inoculation alone. In the process, whether Juj3 affected the PAL synthetic pathway needs further to be investigated. The systemic resistance response induced in plants by beneficial rhizobacteria is in many cases regulated by the phytohormones JA and ET (Zamioudis and Pieterse, 2012). EIN3s encode key transcriptional factors of ET signaling and they function downstream of ET receptors (Jin et al., 2022). The strong expression of EIN3 in the current study also contrasts with the general lack of ET-related gene up-regulation in mycorrhiza-mediated ISR, where elevated ET levels may have a negative impact on mycorrhizal development (Martin-Rodriguez et al., 2011; Fracetto et al., 2013). Galindo-Gonzalez et al. (2020) showed that JA-mediated responses seemed to be mostly inhibited following Pb inoculation, especially in the resistant genotype. PR3 is known to impart resistance in response to necrotrophs and is regulated by JA to activate ISR (Chandrashekar et al., 2018). In our research, compared to the cabbages inoculated with the Pb alone, cabbages inoculated with Juj3 plus Pb showed a much lower PR3 gene expression level in leaves. Juj3 may likely participate in disease resistance by down-regulating the expression of PR3.
In conclusion, our work demonstrates that A. faecalis Juj3 has a positive role in resistance to clubroot and is a promising biocontrol agent for promotion of the growth and quality of brassica plants.
Data availability statement
The original contributions presented in the study are included in the article/Supplementary Material, further inquiries can be directed to the corresponding author/s.
Author contributions
YW and RJ designed the study and wrote the manuscript. RJ, JC, LH, and XL performed the experiments. RJ, JC, KX, and YW analyzed the data. All authors contributed to the article and approved the submitted version.
Funding
This research was supported by the National Key R&D Program of the Ministry of Science and Technology (2018YFD0201205) and the Science and Technology Innovation and Achievement Transformation Project of the Northwest Agriculture and Forestry University Experimental Demonstration Station.
Acknowledgments
The authors thank Professor Andrew Jackson and Professor John Richard Schrock for proofreading our paper.
Conflict of interest
The authors declare that the research was conducted in the absence of any commercial or financial relationships that could be construed as a potential conflict of interest.
Publisher's note
All claims expressed in this article are solely those of the authors and do not necessarily represent those of their affiliated organizations, or those of the publisher, the editors and the reviewers. Any product that may be evaluated in this article, or claim that may be made by its manufacturer, is not guaranteed or endorsed by the publisher.
Supplementary material
The Supplementary Material for this article can be found online at: https://www.frontiersin.org/articles/10.3389/fsufs.2022.942409/full#supplementary-material
References
Agrillo, B., Mirino, S., Tate, R., Gratino, L., Gogliettino, M., Cocca, E., et al. (2019). An alternative biocontrol agent of soil-borne phytopathogens: a new antifungal compound produced by a plant growth promoting bacterium isolated from North Algeria. Microbiol. Res. 221, 60–69. doi: 10.1016/j.micres.2019.02.004
Alexander, A., Singh, V. K., and Mishra, A. (2021). Overexpression of differentially expressed AhCytb6 gene during plant-microbe interaction improves tolerance to N2 deficit and salt stress in transgenic tobacco. Sci. Rep. 11, 13435. doi: 10.1038/s41598-021-92424-4
Ali, S., Mir, Z. A., Tyagi, A., Mehari, H., Meena, R. P., Bhat, J. A., et al. (2017). Overexpression of NPR1 in Brassica juncea confers broad spectrum resistance to fungal pathogens. Front. Plant Sci. 8, 1693. doi: 10.3389/fpls.2017.01693
Asari, S., Ongena, M., Debois, D., De Pauw, E., Chen, K., Bejai, S., et al. (2017). Insights into the molecular basis of biocontrol of Brassica pathogens by Bacillus amyloliquefaciens UCMB5113 lipopeptides. Ann. Bot. 120, 551–562. doi: 10.1093/aob/mcx089
Awan, S. A., Ilyas, N., Khan, I., Raza, M. A., Rehman, A. U., Rizwan, M., et al. (2020). Bacillus siamensis reduces cadmium accumulation and improves growth and antioxidant defense system in two wheat (Triticum aestivum L.) varieties. Plants 9, 878. doi: 10.3390/plants9070878
Bendaly, A., Messedi, D., Smaoui, A., Ksouri, R., Bouchereau, A., and Abdelly, C. (2016). Physiological and leaf metabolome changes in the xerohalophyte species Atriplex halimus induced by salinity. Plant Physiol. Biochem. 103, 208–218. doi: 10.1016/j.plaphy.2016.02.037
Beneduzi, A., Ambrosini, A., and Passaglia, L. M. (2012). Plant growth-promoting rhizobacteria (PGPR): their potential as antagonists and biocontrol agents. Genet. Mol. Biol. 35, 1044–1051. doi: 10.1590/S1415-47572012000600020
Bonaldi, M., Chen, X., Kunova, A., Pizzatti, C., Saracchi, M., and Cortesi, P. (2015). Colonization of lettuce rhizosphere and roots by tagged Streptomyces. Front. Microbiol. 6, 25. doi: 10.3389/fmicb.2015.00025
Boregowda, R. S., Murali, N., Udayashankar, A. C., Niranjana, S. R., Lund, O. S., and Prakash, H. S. (2019). Antifungal activity of Eclipta alba metabolites against sorghum pathogens. Plants 8, 72. doi: 10.3390/plants8030072
Chandrashekar, N., Ali, S., and Grover, A. (2018). Exploring expression patterns of PR-1, PR-2, PR-3, and PR-12 like genes in Arabidopsis thaliana upon Alternaria brassicae inoculation. 3 Biotech 8, 230. doi: 10.1007/s13205-018-1259-2
Comas, L. H., Eissenstat, D. M., and Lakso, A. N. (2000). Assessing root death and root system dynamics in a study of grape canopy pruning. New Phytol. 147, 171–178. doi: 10.1046/j.1469-8137.2000.00679.x
Cook, R. J., Thomashow, L. S., Weller, D. M., Fujimoto, D., Mazzola, M., Bangera, G., et al. (1995). Molecular mechanisms of defense by rhizobacteria against root disease. P. Natl. Acad. Sci. U. S. A. 92, 4197–4201. doi: 10.1073/pnas.92.10.4197
Cordovez, V., Schop, S., Hordijk, K., Dupre de Boulois, H., Coppens, F., Hanssen, I., et al. (2018). Priming of plant growth promotion by volatiles of root-associated Microbacterium spp. Appl. Environ. Microbiol. 84, e01865–e01818. doi: 10.1128/AEM.01865-18
Criado, M. V., Caputo, C., Roberts, I. N., Castro, M. A., and Barneix, A. J. (2009). Cytokinin-induced changes of nitrogen remobilization and chloroplast ultrastructure in wheat (Triticum aestivum). J. Plant Physiol. 166, 1775–1785. doi: 10.1016/j.jplph.2009.05.007
Devos, S., Vissenberg, K., Verbelen, J. P., and Prinsen, E. (2005). Infection of Chinese cabbage by Plasmodiophora brassicae leads to a stimulation of plant growth: impacts on cell wall metabolism and hormone balance. New Phytol. 166, 241–250. doi: 10.1111/j.1469-8137.2004.01304.x
Etesami, H., and Maheshwari, D. K. (2018). Use of plant growth promoting rhizobacteria (PGPRs) with multiple plant growth promoting traits in stress agriculture: action mechanisms and future prospects. Ecotoxicol. Environ. Saf. 156, 225–246. doi: 10.1016/j.ecoenv.2018.03.013
Fedeila, M., Hachaichi-Sadouk, Z., Bautista, L. F., Simarro, R., and Nateche, F. (2018). Biodegradation of anionic surfactants by Alcaligenes faecalis, Enterobacter cloacae and Serratia marcescens strains isolated from industrial wastewater. Ecotoxicol. Environ. Saf. 163, 629–635. doi: 10.1016/j.ecoenv.2018.07.123
Fracetto, G. G. M., Peres, L. E. P., Mehdy, M. C., and Lambais, M. R. (2013). Tomato ethylene mutants exhibit differences in arbuscular mycorrhiza development and levels of plant defense-related transcripts. Symbiosis 60, 155–167. doi: 10.1007/s13199-013-0251-1
Franche, C., Lindström, K., and Elmerich, C. (2008). Nitrogen-fixing bacteria associated with leguminous and non-leguminous plants. Plant Soil 321, 35–59. doi: 10.1007/s11104-008-9833-8
Galindo-Gonzalez, L., Manolii, V., Hwang, S. F., and Strelkov, S. E. (2020). Response of Brassica napus to Plasmodiophora brassicae involves salicylic acid-mediated immunity: an RNA-seq-based study. Front. Plant Sci. 11, 1025. doi: 10.3389/fpls.2020.01025
Guo, D., Yuan, C., Luo, Y., Chen, Y., Lu, M., Chen, G., et al. (2020). Biocontrol of tobacco black shank disease (Phytophthora nicotianae) by Bacillus velezensis Ba168. Pestic. Biochem. Physiol. 165, 104523. doi: 10.1016/j.pestbp.2020.01.004
Honda, N., Hirai, M., Ano, T., and Shoda, M. (1999). Control of tomato damping-off caused by Rhizoctonia solani by the heterotrophic nitrifier Alcaligenes faecalis and its product, hydroxylamine. Ann. Phytopathol Soc. Jpn. 65, 153–162. doi: 10.3186/jjphytopath.65.153
Huang, M., Bulut, A., Shrestha, B., Matera, C., Grundler, F. M. W., and Schleker, A. S. S. (2021). Bacillus firmus I-1582 promotes plant growth and impairs infection and development of the cyst nematode Heterodera schachtii over two generations. Sci. Rep. 11, 14114. doi: 10.1038/s41598-021-93567-0
Jin, J., Essemine, J., Xu, Z., Duan, J., Shan, C., Mei, Z., et al. (2022). Arabidopsis ETHYLENE INSENSITIVE 3 directly regulates the expression of PG1beta-like family genes in response to aluminum stress. J. Exp. Bot. erac161. doi: 10.1093/jxb/erac161
Kageyama, K., and Asano, T. (2009). Life Cycle of Plasmodiophora brassicae. J. Plant Growth Regul. 28, 203–211. doi: 10.1007/s00344-009-9101-z
Karamat, U., Sun, X., Li, N., and Zhao, J. (2021). Genetic regulators of leaf size in Brassica crops. Hortic. Res. 8, 91. doi: 10.1038/s41438-021-00526-x
Kasuga, T., Hayden, K. J., Eyre, C. A., Croucher, P. J. P., Schechter, S., Wright, J. W., et al. (2021). Innate resistance and phosphite treatment affect both the pathogen's and host's transcriptomes in the tanoak-Phytophthora ramorum pathosystem. J. Fungi 7, 198. doi: 10.3390/jof7030198
Kenneth, J. L., and Thomas, D. S. (2001). Analysis of relative gene expression data using real-time quantitative PCR and the 2−ΔΔCT method. Methods 25, 402–408. doi: 10.1006/meth.2001.1262
Khan, N., Bano, A., Rahman, M. A., Guo, J., Kang, Z., and Babar, M. A. (2019). Comparative physiological and metabolic analysis reveals a complex mechanism involved in drought tolerance in chickpea (Cicer arietinum L.) induced by PGPR and PGRs. Sci. Rep. 9, 2097. doi: 10.1038/s41598-019-38702-8
Klewer Luerssen, Graf, and Siemens. (2009). Restriction fragment length polymorphism markers to characterize Plasmodiophora brassicae single-spore isolates with different virulence patterns. J. Phytopathol. 149, 121–127. doi: 10.1046/j.1439-0434.2001.00595.x
Kumar, A., Singh, R., Yadav, A., Giri, D. D., Singh, P. K., and Pandey, K. D. (2016a). Isolation and characterization of bacterial endophytes of Curcuma longa L. 3 Biotech 6, 60. doi: 10.1007/s13205-016-0393-y
Kumar, P., Pahal, V., Gupta, A., Vadhan, R., Chandra, H., and Dubey, R. C. (2020). Effect of silver nanoparticles and Bacillus cereus LPR2 on the growth of Zea mays. Sci. Rep. 10, 20409. doi: 10.1038/s41598-020-77460-w
Kumar, S., Stecher, G., and Tamura, K. (2016b). MEGA7: Molecular Evolutionary Genetics Analysis version 7.0 for bigger datasets. Mol. Biol. Evol. 33, 1870–1874. doi: 10.1093/molbev/msw054
Kusstatscher, P., Wicaksono, W. A., Thenappan, D. P., Adam, E., Muller, H., and Berg, G. (2020). Microbiome management by biological and chemical treatments in maize is linked to plant health. Microorganisms 8, 1–14. doi: 10.3390/microorganisms8101506
Lahlali, R., McGregor, L., Song, T., Gossen, B. D., Narisawa, K., and Peng, G. (2014). Heteroconium chaetospira induces resistance to clubroot via upregulation of host genes involved in jasmonic acid, ethylene, and auxin biosynthesis. PLoS ONE 9, e94144. doi: 10.1371/journal.pone.0094144
Le, K. D., Kim, J., Nguyen, H. T., Yu, N. H., Park, A. R., Lee, C. W., et al. (2021). Streptomyces sp. JCK-6131 protects plants against bacterial and fungal diseases via two mechanisms. Front. Plant Sci. 12, 726266. doi: 10.3389/fpls.2021.726266
Li, J. (2011). Growth-promotion and disease-control effects of biocontrol strain BC2007 to tomato. J. Anhui Agri. Sci. 39, 22365–22367. doi: 10.13989/j.cnki.0517-6611.2011.36.207
Li, Y., and Chen, S. (2019). Fusaricidin produced by Paenibacillus polymyxa WLY78 induces systemic resistance against Fusarium wilt of cucumber. Int. J. Mol. Sci. 20, 5240. doi: 10.3390/ijms20205240
Liu, X., Chen, Z., Gao, Y., Liu, Q., Zhou, W., Zhao, T., et al. (2019). Combinative effects of Azospirillum brasilense inoculation and chemical priming on germination behavior and seedling growth in aged grass seeds. PLoS ONE 14, e0210453. doi: 10.1371/journal.pone.0210453
Lorenzini, G., Guidi, L., Nali, C., Ciompi, S., and Soldatini, G. F. (1997). Photosynthetic response of tomato plants to vascular wilt diseases. Plant Sci. 124, 143–152. doi: 10.1016/S0168-9452(97)04600-1
Lovelock, D. A., Donald, C. E., Conlan, X. A., and Cahill, D. M. (2012). Salicylic acid suppression of clubroot in broccoli (Brassicae oleracea var. italica) caused by the obligate biotroph Plasmodiophora brassicae. Australas. Plant Path. 42, 141–153. doi: 10.1007/s13313-012-0167-x
Ludwig-Müller, J. (2008). Glucosinolates and the clubroot disease: defense compounds or auxin precursors? Phytochem. Rev. 8, 135–148. doi: 10.1007/s11101-008-9096-2
Ludwig-Müller, J., Prinsen, E., Rolfe, S. A., and Scholes, J. D. (2009). Metabolism and plant hormone action during clubroot disease. J. Plant Growth Regul. 28, 229–244. doi: 10.1007/s00344-009-9089-4
Ludwig-Müller, J., and Schuller, A. (2008). What can we learn from clubroots: alterations in host roots and hormone homeostasis caused by Plasmodiophora brassicae. Eur. J. Plant Pathol. 121, 291–302. doi: 10.1007/s10658-007-9237-2
Malinowski, R., Smith, J. A., Fleming, A. J., Scholes, J. D., and Rolfe, S. A. (2012). Gall formation in clubroot-infected Arabidopsis results from an increase in existing meristematic activities of the host but is not essential for the completion of the pathogen life cycle. Plant J. 71, 226–238. doi: 10.1111/j.1365-313X.2012.04983.x
Martin-Rodriguez, J. A., Leon-Morcillo, R., Vierheilig, H., Ocampo, J. A., Ludwig-Muller, J., and Garcia-Garrido, J. M. (2011). Ethylene-dependent/ethylene-independent ABA regulation of tomato plants colonized by arbuscular mycorrhiza fungi. New Phytol. 190, 193–205. doi: 10.1111/j.1469-8137.2010.03610.x
Miller, G. L. (1959). Use of dinitrosalicylic acid reagent for determination of reducing sugar. Anal. Chem. 31, 426–428. doi: 10.1021/ac60147a030
Peng, G., Lahlali, R., Hwang, S.-F., Pageau, D., Hynes, R. K., McDonald, M. R., et al. (2014). Crop rotation, cultivar resistance, and fungicides/biofungicides for managing clubroot (Plasmodiophora brassicae) on canola. Can. J. Plant Pathol. 36, 99–112. doi: 10.1080/07060661.2013.860398
Pinto, L., Azevedo, J. L., Pereira, J. O., and Labate, V. (2000). Symptomless infection of banana and maize by endophytic fungi impairs photosynthetic efficiency. New Phytol. 147, 609–615. doi: 10.1046/j.1469-8137.2000.00722.x
Pozo, M. J., Van Der Ent, S., Van Loon, L. C., and Pieterse, C. M. J. (2008). Transcription factor MYC2 is involved in priming for enhanced defense during rhizobacteria-induced systemic resistance in Arabidopsis thaliana. New Phytol. 180, 511–523. doi: 10.1111/j.1469-8137.2008.02578.x
Qessaoui, R., Bouharroud, R., Furze, J. N., El Aalaoui, M., Akroud, H., Amarraque, A., et al. (2019). Applications of new rhizobacteria Pseudomonas isolates in agroecology via fundamental processes complementing plant growth. Sci. Rep. 9, 12832. doi: 10.1038/s41598-019-49216-8
Ramamoorthy, V., Viswanathan, R., Raguchander, T., Prakasam, V., and Samiyappan, R. (2001). Induction of systemic resistance by plant growth promoting rhizobacteria in crop plants against pests and diseases. Crop Prot. 20, 1–11. doi: 10.1016/S0261-2194(00)00056-9
Riera, N., Wang, H., Li, Y., Li, J., Pelz-Stelinski, K., and Wang, N. (2018). Induced systemic resistance against citrus canker disease by rhizobacteria. Phytopathology 108, 1038–1045. doi: 10.1094/PHYTO-07-17-0244-R
Ruf, M., and Brunner, I. (2003). Vitality of tree fine roots: reevaluation of the tetrazolium test. Tree Physiol. 23, 257–263. doi: 10.1093/treephys/23.4.257
Sachdev, D. P., Chaudhari, H. G., Kasture, V. M., Dhavale, D. D., and Chopade, B. A. (2009). Isolation and characterization of indole acetic acid (IAA) producing Klebsiella pneumoniae strains from rhizosphere of wheat (Triticum aestivum) and their effect on plant growth. Indian J. Exp. Biol. 47, 993–1000.
Santoa, S., Oliveira, L. K. X., de Melo, I. S., Velozo, E, and M. R. (2011). Antifungal activity of bacterial strains from the rhizosphere of Stachytarpheta crassifolia. Afr. J. Biotechnol. 10, 4996–5000. doi: 10.5897/AJB10.2312
Sarma, R. K., and Saikia, R. (2013). Alleviation of drought stress in mung bean by strain Pseudomonas aeruginosa GGRJ21. Plant Soil 377, 111–126. doi: 10.1007/s11104-013-1981-9
Sayyed, R. Z., and Chincholkar, S. B. (2009). Siderophore-producing Alcaligenes feacalis exhibited more biocontrol potential vis-à-vis chemical fungicide. Curr. Microbiol. 58, 47–51. doi: 10.1007/s00284-008-9264-z
Sayyed, R. Z., and Patel, P. R. (2011). Biocontrol potential of siderophore producing heavy metal resistant Alcaligenes sp. and Pseudomonas aeruginosa RZS3 vis-a-vis organophosphorus fungicide. Indian J. Microbiol. 51, 266–1272. doi: 10.1007/s12088-011-0170-x
Siemens, J., Keller, I., Sarx, J., Kunz, S., Schuller, A., Nagel, W., et al. (2006). Transcriptome analysis of Arabidopsis clubroots indicate a key role for cytokinins in disease development. Mol. Plant Microbe. Interact. 19, 480–494. doi: 10.1094/MPMI-19-0480
Singh, P., Singh, R. K., Li, H. B., Guo, D. J., Sharma, A., Lakshmanan, P., et al. (2020). Diazotrophic bacteria Pantoea dispersa and Enterobacter asburiae promote sugarcane growth by inducing nitrogen uptake and defense-related gene expression. Front. Microbiol. 11, 600417. doi: 10.3389/fmicb.2020.600417
Stevens, R., Buret, M., Garchery, C., Carretero, Y., and Causse, M. (2006). Technique for rapid, small-scale analysis of vitamin C levels in fruit and application to a tomato mutant collection. J. Agric. Food Chem. 54, 6159–6165. doi: 10.1021/jf061241e
Strelkov, S. E., and Hwang, S. F. (2014). Clubroot in the Canadian canola crop: 10 years into the outbreak. Can. J. Plant Pathol. 36, 27–36. doi: 10.1080/07060661.2013.863807
van de Mortel, J. E., de Vos, R. C., Dekkers, E., Pineda, A., Guillod, L., Bouwmeester, K., et al. (2012). Metabolic and transcriptomic changes induced in Arabidopsis by the rhizobacterium Pseudomonas fluorescens SS101. Plant Physiol. 160, 2173–2188. doi: 10.1104/pp.112.207324
Van Loon, L. C. (2007). Plant responses to plant growth-promoting rhizobacteria. Eur. J. Plant Pathol. 119, 243–254. doi: 10.1007/s10658-007-9165-1
Vejan, P., Abdullah, R., Khadiran, T., Ismail, S., and Nasrulhaq Boyce, A. (2016). Role of plant growth promoting rhizobacteria in agricultural sustainability-a review. Molecules 21, 1–17. doi: 10.3390/molecules21050573
Vessey, J. K. (2003). Plant growth promoting rhizobacteria as biofertilizers. Plant Soil 255, 571–586. doi: 10.1023/A:1026037216893
Walters, D. R., McRoberts, N., and Fitt, B. D. (2008). Are green islands red herrings? Significance of green islands in plant interactions with pathogens and pests. Biol. Rev. Camb. Philos. Soc. 83, 79–102. doi: 10.1111/j.1469-185X.2007.00033.x
Wei, Y., Shi, A., Jia, X., Zhang, Z., Ma, X., Gu, M., et al. (2018). Nitrogen supply and leaf age affect the expression of TaGS1 or TaGS2 driven by a constitutive promoter in transgenic tobacco. Genes 9, 406. doi: 10.3390/genes9080406
Whipps, J. M. (2001). Microbial interactions and biocontrol in the rhizosphere. J. Exp. Bot. 52, 487–511. doi: 10.1093/jxb/52.suppl_1.487
Yang, L., and Yang, K. (2020). Biological function of Klebsiella variicola and its effect on the rhizosphere soil of maize seedlings. Peer J. 8, e9894. doi: 10.7717/peerj.9894
Zamioudis, C., and Pieterse, C. M. (2012). Modulation of host immunity by beneficial microbes. Mol. Plant Microbe. Interact. 25, 139–150. doi: 10.1094/MPMI-06-11-0179
Zhang, B., Zhao, H., Wu, X., and Zhang, L. Q. (2020). The Oxidoreductase DsbA1 negatively influences 2,4-diacetylphloroglucinol biosynthesis by interfering the function of Gcd in Pseudomonas fluorescens 2P24. BMC Microbiol. 20, 39. doi: 10.1186/s12866-020-1714-1
Zhang, J., Chen, J., Hu, L., Jia, R., Ma, Q., Tang, J., et al. (2021). Antagonistic action of Streptomyces pratensis S10 on Fusarium graminearum and its complete genome sequence. Environ. Microbiol. 23, 1925–1940. doi: 10.1111/1462-2920.15282
Zhang, W., Wang, S., Yu, F., Tang, J., Yu, L., Wang, H., et al. (2019). Genome-wide identification and expression profiling of sugar transporter protein (STP) family genes in cabbage (Brassica oleracea var. capitata L.) reveals their involvement in clubroot disease responses. Genes 10, 71. doi: 10.3390/genes10010071
Zhang, Y. Z., Chen, Q., Liu, C. H., Liu, Y. B., Yi, P., Niu, K. X., et al. (2016). Chitin synthase gene FgCHS8 affects virulence and fungal cell wall sensitivity to environmental stress in Fusarium graminearum. Fungal Biol. 120, 764–774. doi: 10.1016/j.funbio.2016.02.002
Zhao, J., Buchwaldt, L., Rimmer, S. R., Sharpe, A., McGregor, L., Bekkaoui, D., et al. (2009). Patterns of differential gene expression in Brassica napus cultivars infected with Sclerotinia sclerotiorum. Mol. Plant Pathol. 10, 635–649. doi: 10.1111/j.1364-3703.2009.00558.x
Zhou, H., Ren, Z. H., Zu, X., Yu, X. Y., Zhu, H. J., Li, X. J., et al. (2021). Efficacy of plant growth-promoting bacteria Bacillus cereus YN917 for biocontrol of rice blast. Front. Microbiol. 12, 684888. doi: 10.3389/fmicb.2021.684888
Zhu, M., He, Y., Li, Y., Ren, T., Liu, H., Huang, J., et al. (2019). Two new biocontrol agents against clubroot caused by Plasmodiophora brassicae. Front. Microbiol. 10, 3099. doi: 10.3389/fmicb.2019.03099
Keywords: PGPR, Plasmodiophora brassicae, biocontrol efficacy, photosynthesis, induced disease resistance
Citation: Jia R, Chen J, Hu L, Liu X, Xiao K and Wang Y (2022) Alcaligenes faecalis Juj3 alleviates Plasmodiophora brassicae stress to cabbage via promoting growth and inducing resistance. Front. Sustain. Food Syst. 6:942409. doi: 10.3389/fsufs.2022.942409
Received: 12 May 2022; Accepted: 27 June 2022;
Published: 15 July 2022.
Edited by:
Zhen He, Yangzhou University, ChinaReviewed by:
Bei Bei Ge, Institute of Plant Protection (CAAS), ChinaManoj Kumar Solanki, University of Silesia in Katowice, Poland
Wenming Zheng, Henan Agricultural University, China
Copyright © 2022 Jia, Chen, Hu, Liu, Xiao and Wang. This is an open-access article distributed under the terms of the Creative Commons Attribution License (CC BY). The use, distribution or reproduction in other forums is permitted, provided the original author(s) and the copyright owner(s) are credited and that the original publication in this journal is cited, in accordance with accepted academic practice. No use, distribution or reproduction is permitted which does not comply with these terms.
*Correspondence: Yang Wang, d2FuZ3lhbmcyMDA2QG53YWZ1LmVkdS5jbg==