- 1School of Chemistry Engineering, Zhengzhou University, Zhengzhou, China
- 2Henan Key Laboratory of Tea Plant Biology, College of Life Science, Xinyang Normal University, Xinyang, China
- 3School of Life Science, Zhengzhou University, Zhengzhou, China
- 4College of Biotechnology and Pharmaceutical Engineering, Nanjing Tech University, Nanjing, China
Essential trace minerals play vital roles in maintaining human and animal health. However, an overdose of the existing inorganic trace minerals is prone to induce detrimental effects that outweigh positive benefits. In this study, an extracellular polymeric substances (EPS)-producing bacterium, identified as Bacillus licheniformis CCTCC M2020298, was isolated from marine using glutinous rice processing wastewater as enrichment medium. The EPS yield of Bacillus licheniformis CCTCC M2020298 could reach 8.62 g/L by using glutinous rice-processing wastewater containing medium. Furthermore, the potential of the EPS as a carrier for synthesizing EPS-iron (Fe) and EPS-copper (Cu) complex was explored. The results showed that the optimum condition for the synthesis EPS-Fe were the reaction temperature 70°C, pH 8.5–9.0 and mass ratio of EPS to trisodium citrate 2:1. The iron content of EPS-Fe reached 77.4 mg/g. Under the same condition, the copper content of EPS-Cu reached 90.7 mg/g. The elemental composition, functional groups and valence state of the mineral elements of EPS-Fe and EPS-Cu were well characterized. The EPS-Fe and EPS-Cu exhibited antioxidant activity in scavenging ·OH, DPPH and ·O2− free radicals, thereby leading to reduced oxidative stress and apoptosis levels in human colonic epithelial cells in vitro. They also inhibited the proliferation of mouse hepatocellular carcinoma H22 and the growth of intestinal pathogens in vitro. This study provided an effective avenue for EPS production from glutinous rice processing wastewater and proved the potential of EPS-Fe and EPS-Cu complexes as a new-type comprehensive essential trace mineral supplement.
1 Introduction
Trace elements also known as trace minerals, are important micronutrients in very minute quantities (<0.01%) of the mass of mammals (Freeland-Graves et al., 2015; Zemrani and Bines, 2020). Adults usually require 1–100 mg of trace elements per day. The recognized essential trace elements in humans include copper, iron, zinc, selenium, iodine, manganese, chromium, cobalt, molybdenum and fluoride. They play an irreplaceable role in the growth and development of humans and animals by participating in physiological and biochemical activities such as immune regulation, blood transport, and protein formation (Zoroddu et al., 2019). For example, iron is required for the synthesis of oxygen transport proteins, in particular hemoglobin and myoglobin, and for the formation of heme enzymes in the body (Jing et al., 2022). Iron and copper are essential cofactors for oxidase enzymes related to electron transfer and oxidation-reductions in various metabolic pathways (Tavsan and Ayar Kayali, 2013). Moreover, the various trace elements in the body do not work independently and can therefore have antagonistic or synergistically effects (Wang et al., 2022b). Copper may enhance iron absorption in the intestinal of patients with anemia (Myint et al., 2018).
Despite of the importance functions of the trace elements, there is a U-shaped relationship between trace elements status (deficiencies and toxicities) and disease risk. Most cases of anemia worldwide are caused by low iron intake and/or bioavailability (Miller, 2013). Copper deficiency may cause hypochromic anemia not responsive to iron supplements, and neutropenia (Myint et al., 2018). Traditionally, the solution is the enhancement of corresponding inorganic metal salt intake, such as ferrous sulfate and copper sulphate. However, the first generation of inorganic metal salts faces the problem of low absorptivity and high side effects. In addition, the excessive intake of inorganic trace elements may lead to instances of acute or chronic liver injury and neurological damage (Mehri, 2020). The second generation of trace elements are soluble small molecular organic metal salts, such as ferrous lactate and cupric citrate. Although the absorption rate and bioavailability of the small molecular organic metal salts are improved, they have quality issues, including instability (Jing et al., 2022).
Different from the previous two generations of metal supplements, a new generation of organic metal complexes, called the third generation of trace metal supplements, has aroused a great deal of interest (Kostova, 2022). The organic metal complexes mainly include metal complexes, polypeptide metal chelate, metal-rich microbes, etc., (Krüger and Baumgartner, 2010; Wu et al., 2017). They possess smaller toxicity and side effects than the first and second generations. It has been reported that the organic metal complex can be synthesized from various organic substrates, such as polysaccharides, polypeptides, proteins, amino acids and microbes (Megías et al., 2008; Mitić et al., 2011; Lu et al., 2016; Gao et al., 2018; Bai et al., 2022; Wang et al., 2023). Moreover, the organic metal complexes, especially for the polysaccharide metal complex, always have specific biological activity (e.g., antimicrobial, antioxidant and immunity-enhancing properties) because of the polysaccharide component itself (Li et al., 2017; Harvey et al., 2021; Yuan et al., 2022). The polysaccharide used in the synthesis of metal complexes can be derived from plants, microbes or the market (Mitić et al., 2011; Tang et al., 2013; Escárcega-González et al., 2018). However, commercial production of polysaccharide metal complexes from these polysaccharides on a large scale is still lacking due to the high cost of the extraction procedure. In addition, the polysaccharide metal complexes can also be extracted from the microbes cultivated with metal supplemented, which is difficult to industrialize the production due to the low metal tolerance of the strains.
Microbial extracellular polymeric substances (EPS) are the secondary metabolites secreted by microorganisms into the environment, which mainly composed of organic compounds such as polysaccharides, proteins, and nucleic acid (Strieth et al., 2021). As the EPS are rich in active groups such as hydroxyl, amino, and negative oxygen groups, they have been applied in wastewater treatment and heavy metal adsorption (Harish et al., 2012; Wang Z et al., 2015). Some Bacillus licheniformis species have been found to be able to synthesize extracellular polymers by using waste biomass as substrates (Zhuang et al., 2012). Moreover, B. licheniformis is used as a probiotic in fermented food and feed to adjust the intestinal health of humans and animals (Muras et al., 2021). Thus, synthesis of organic trace metals using B. licheniformis EPS fermented from the waste biomass should be safer and lower-cost than that using polysaccharides from the market or extracted from plants or microbes.
In this study, high EPS producing strain B. licheniformis CCTCC M2020298 was isolated from seawater by using glutinous rice-processing wastewater containing medium. Then, organic EPS-Fe and EPS-Cu was successfully synthesized with the B. licheniformis EPS, and the optimum synthesis conditions were explored. The physicochemical properties of EPS-Fe and EPS-Cu, including selemental composition, functional groups and valence state of the mineral elements, were characterized by energy dispersive spectroscopy (EDS), ultraviolet–visible (UV–vis) spectrophotometry, Fourier transform-infrared (FT-IR) spectroscopy and X-ray photoelectron spectroscopy (XPS). Furthermore, the anti- oxidant, bacterial pathogenic activities and tumor cell proliferation were investigated in vitro. To the best of our knowledge, the EPS-Fe and EPS-Cu are the first organic Fe and Cu synthesized by using secreted bacterial EPS other than plant/fungus extracts or microbes.
2 Materials and methods
2.1 Isolation of EPS producing strain
EPS producing strains were isolated from the seawater collected from Shapowei, Xiamen (111.9°E, 21.7°N). Diluted seawater was plated on the solid screening medium. Then, the single colony was picked and inoculated in a flask containing seed medium at 37°C for 12 h with shaking at 200 rpm. The strain in the seed medium was inoculated in a flask containing glutinous rice-processing wastewater based fermentation medium for 48 h at 37°C with shaking at 200 rpm. The fermentation process was repeated for each colony and the faster growing strain with the highest viscosity was selected for EPS production.
The solid screening medium contained glucose 10 g/L, yeast paste 1.5 g/L, urea 1.0 g/L, KH2PO4 0.3 g/L, K2HPO4 0.4 g/L, MgSO4 0.4 g/L, NaCl 0.5 g/L, agar 1.5 g/L (pH 7.0–8.0). The seed medium contained glucose 10 g/L, yeast paste 1.5 g/L, urea 1.0 g/L, KH2PO4 0.3 g/L, K2HPO4 0.4 g/L, MgSO4 0.4 g/L, NaCl 0.5 g/L (pH 7.0 to 8.0). The glutinous rice-processing wastewater based fermentation medium contained glucose 10 g, urea 2.0 g, yeast paste 1.0 g, with glutinous rice processing wastewater volume to 1 L (pH 7.0 ~ 8.0).
The EPS-producing strain was identified on the basis of the 16S rRNA gene according to the method in a previous study (Huang et al., 2018). The genomic DNA of strain was extracted using an E.Z.N.A. Bacterial DNA Kit (OMEGA, Norcross, GA). The 16S rRNA gene was sequenced by Shenggong Biotech (China) and analyzed by blast in the National Center for Biotechnology Information (NCBI) Database.
2.2 Purification of EPS
The B. licheniformis CCTCC M2020298 preserved in glycerol tubes was cultured in slant medium at 37°C for 12 h. The colonies on the slant were inoculated to the seed medium and cultured at 200 rpm at 37°C for 12 h. The seed liquid was taken 4% of the inoculum (v/v) to the fermentation medium of glutinous rice-processing wastewater and cultured at 200 rpm at 37°C for 48 h. The fermentation liquid was centrifuged at 5000 rpm for 15 min, and the supernatant was collected. The supernatant was mixed with anhydrous ethanol at a volume ratio of 1:4. Then, the mixture was stored at 4°C for 12 h. After that, the mixture was centrifuged at 6000 rpm for 10 min, and the precipitates was collected. The precipitate was dissolved in water and dialyzed with a 6,000 Da dialysis bag at 4°C for 12 h. Anhydrous ethanol was added to the dialyzed solution, which was then stored at 4°C for 12 h before centrifugation. The precipitate was freeze-dried to obtain EPS (Labconco Corporation, America).
2.3 Synthesis of EPS-Fe and EPS-Cu and optimization of the synthesis condition
EPS-Fe was synthesized as follows: 1.0 g of EPS and trisodium citrate were dissolved in 60 mL of ultrapure water with stirring at 70°C. Subsequently, 10% sodium hydroxide solution was added to the solution to adjust the pH of the solution to alkaline. Add 2 mol/L ferric chloride solution to the above solution. The reaction was maintained at a constant heating and stirring rate of 200 rpm, and the alkaline environment was maintained by using 10% sodium hydroxide solution. The reaction was terminated when a brown precipitate appeared in the solution, which was then left to be cool at room temperature. After that, the supernatant was centrifuged at 8000 rpm for 10 min to obtain the supernatant. Three times of the volume of anhydrous ethanol was added to the supernatant, and the supernatant was stored at 4°C for 12 h. Precipitation was retained, washed with anhydrous ethanol for 2–3 times and freeze-dried for further research.
The effects of different factors (amount of trisodium citrate, reaction temperature and pH) on the iron content in EPS-Fe were investigated. Firstly, the effect of amount of trisodium citrate on the content of iron ions in EPS-Fe was investigated. 1 g EPS with different concentrations of trisodium citrate (0.1 g, 0.3 g, 0.5 g, 0.8 g and 1.0 g) were dissolved in 60 mL water. During the reaction, the temperature of the reaction solution was maintained at 70°C and the pH was maintained between 8.5 and 9.0. Then the effect of reaction temperature on the content of iron ions was explored. 1.0 g of EPS and 0.5 g of trisodium citrate were dissolved in 60 mL water and pH was between 8.5 and 9.0. The reaction temperature was controlled at 60°C, 70°C and 80°C. Finally, changing pH to study changes in iron content. 1.0 g of EPS and 0.5 g of trisodium citrate were dissolved in 60 mL water and reaction temperature was 70°C. The pH of the reaction solution was maintained between 7.5–8.0, 8.5–9.0, 9.5–10.0, 10.5–11.0 and 11.5–12. EPS-Fe was prepared according to the above synthesis process. EPS-Cu was prepared by the same optimal condition.
2.4 Characterization of EPS, EPS-Fe and EPS-Cu
The EDS (Helios G4 CX, Czech Republic) was used to analyze the elemental distribution in EPS-Fe/EPS-Cu. The EPS-Fe/EPS-Cu was dissolved in ultrapure water to form the corresponding solutions, which were scanned at 200–800 nm with a UV–vis spectrophotometer (Agilent Cary 60, America). The FT-IR spectra of EPS-Fe/EPS-Cu ranging from 500 to 4,000 cm−1 was obtained using a FTIR spectrometer (STA8000-Frontier, Netherlands). EPS-Fe/EPS-Cu was scanned by XPS (AXIS Supra, Britain) to analyze the valence states of Fe/Cu. The content of Fe/Cu was measured by ICP-MS (ICP-9820, Japan). At the same time EPS was used as a control. The total sugar content of the EPS was measured by the phenol-sulfuric acid method using glucose as the standard solution and the total protein content was measured by the Lowery method using bovine serum albumin as the standard solution as previously described (Xiong et al., 2010).
2.5 Determination of anti-oxidant activity of EPS-Fe/Cu
2.5.1 ·OH free radical scavenging activity
The ·OH free radical scavenging activity of EPS and EPS-Fe/EPS-Cu was evaluated according to previously reported methods with minor modifications (Jin et al., 2011). Shortly, 1 mL of EPS or EPS-Fe/EPS-Cu solution at different concentrations (0.25, 0.5, 1, 2, 3 and 4 g/L) was added to the reaction system which was consisted of 1 mL of 9 mmol/L salicylic acid solution, 1 mL of 9 mmol/L FeSO4 solution, 1 mL of 9 mmol/L H2O2 solution. The reaction was carried out at 37°C for 20 min, and the reaction solution was measured at 510 nm. Ascorbic acid (Vc), was used as a positive control.
·OH free radical scavenging activity was calculated as the equation:
·OH free radical scavenging activity (%) = (A0 − (A1 − A2))/A0*100%.
A0 – the absorbance of the mixture with water, A1 – the absorbance of the mixture with EPS or EPS-Fe/Cu, A2 – the absorbance of the EPS or EPS or EPS-Fe/Cu.
2.5.2 DPPH free radical scavenging activity
The 1,1-diphenyl-2-picryl-hydrazyl (DPPH) free radical scavenging activity of EPS and EPS-Fe/EPS-Cu was evaluated according to previously reported method with minor modifications (Ye et al., 2012). Shortly, 1 mL of EPS or EPS-Fe/EPS-Cu solution at different concentrations (0.25, 0.5, 1, 2, 3 and 4 g/L) was mixed with 2 mL of 0.1 mmol/L DPPH solution. The reaction was protected from light for 30 min and the absorbance was measured at 517 nm. Ascorbic acid (Vc), was used as a positive control.
DPPH free radical scavenging activity was calculated as the equation:
DPPH free radical scavenging activity (%) = (A0 − (A1 − A2))/A0*100%.
A0 – the absorbance of the mixture with water, A1 – the absorbance of the mixture with EPS or EPS-Fe/Cu, A2 – the absorbance of the EPS or EPS or EPS-Fe/Cu.
2.5.3 ·O2− free radical scavenging activity
The superoxide (·O2−) free radical scavenging activity of EPS and EPS-Fe/EPS-Cu was evaluated according to previously reported method with minor modifications (Cheng et al., 2017). Shortly, 1 mL of EPS or EPS-Fe/EPS-Cu solution at different concentrations (0.25, 0.5, 1, 2, 3 and 4 g/L) was added to the reaction system which was consisted of 2 mL of Tris–HCl buffer (50 mmol/L, pH 8.2), 0.1 mL of 1 mmol/L pyrogallol solution. The absorbance of the reaction solution at 315 nm was measured at 30 s intervals from the beginning of the reaction up to 5 min. Ascorbic acid (Vc), was used as a positive control.
·O2− free radical scavenging activity was calculated as the equation:
·O2−free radical scavenging activity (%) = [1 − (ΔA0 − ΔA1)/ΔA0]*100%.
A0 – the change in absorbance of the mixture with water during the period of 30–300 s, A1 – the change in absorbance of the mixture with EPS or EPS-Fe/EPS-Cu during the period of 30–300 s.
2.5.4 Protective effect against H2O2 oxidative stress-mediated cells
Human colonic epithelial cells (HCoEpiCs) were cultured in DMEM high glucose medium supplemented with 10% fetal bovine serum (FBS) and 1% penicillin–streptomycin solution at 37°C under a humidified atmosphere of 5% CO2 and 95% air. The cultured HCoEpiCs were then added to 96-well plates with a density of 6.7 × 104 cells/mL and incubated for 4 h. Next, H2O2 with the concentration ranging from 0.05 mM to 3 mM was added to the 96-well plates and the cells were cultured for another 4 h to screen the appropriate concentration of H2O2 and establish the H2O2-induced cellular injury model. The group treated with fresh complete medium without H2O2 for another 4 h was taken as a control. Cell viability of HCoEpiCs was calculated according to the equation:
Cell viability (%) = Asample/Ablank × 100.
Where Asample is the absorbance of the mixture with EPS-Fe/EPS-Cu, and Ablank is the absorbance of the mixture without EPS-Fe/EPS-Cu.
To evaluate the protective effect of EPS-Fe/EPS-Cu against H2O2-induced cells, HCoEpiCs were first treated with EPS-Fe/EPS-Cu for 24 h and then exposed to H2O2 for another 4 h. The concentration of H2O2 was obtained by H2O2-induced cellular injury model with the cell viability <50%. For the control group, H2O2-induced cells were cultivated without EPS-Fe/EPS-Cu for 24 h and treated with H2O2 for another 4 h. The cell viability was determined using a CCK-8 kit according to the manufacturer’s protocol.
2.6 Determination of anti-proliferation activity
The cytotoxicity of EPS-Fe/EPS-Cu and Na2SeO3 for HCoEpiCs was determined. First, HCoEpiCs were incubated for 4 h in the 96-well plate with an initial density of 5.8 × 104 cells/mL. Then, the cell cultures were mixed with EPS-Fe/EPS-Cu or Na2SeO3 solution with concentration ranging from 1.07 μg/mL to 85.92 μg/mL and incubated for 24 h. Finally, cell viability was determined by a CCK-8 kit according to the manufacturer’s protocols. The anti-proliferation activities of EPS and EPS-Fe/EPS-Cu were tested against mouse hepatocellular carcinoma H22 in vitro. The H22 cells were cultured in 1640 medium supplemented with 10% FBS and 1% penicillin–streptomycin solution at 37°C under a humidified atmosphere of 5% CO2 and 95% air. The cultured H22 were transferred into 96-well plates containing EPS or EPS-Fe/EPS-Cu solution with concentration ranging from 10 μg/mL to 800 μg/mL and incubated for 24 h. Cell viability was determined by a CCK-8 kit according to the manufacturer’s protocols.
2.7 Determination of anti-bacterial activity of EPS-Fe/EPS-Cu
The anti-bacterial activity of EPS-Fe/EPS-Cu were tested using two common pathogenic bacteria, Staphylococcus aureus ATCC 6538, Staphylococcus aureus ATCC 29213, Staphylococcus aureus ATCC 12600 (Gram-positive bacteria), Salmonella enterica ATCC 13076 and Salmonella enterica ATCC 13311 (Gram-negative bacteria), respectively. The above strains conserved in glycerol tubes were activated by scribing on LB plates at 37°C for 12 h, and seed solutions were prepared by selecting single spots into LB liquid at 37°C, 200 rpm for 12 h. The pre-cultured strains were then incubated on 96-well culture plates with an initial optical density (OD600 nm) of 0.05 at 600 nm, with each well containing LB liquid medium supplemented containing EPS-Fe/EPS-Cu. The absorbance was measured by a microplate reader (SpectraMax Paradigm, Salzburg, Austria) at 600 nm. The inhibition rates of EPS-Fe/EPS-Cu on different pathogens were calculated according to the equation:
Inhibition rate (%) = [1 − (A2 − A0)/(A1 − A0)] × 100%.
A0 – the absorbance of the mixture without the EPS-Fe/EPS-Cu and tested strains, A1 – the absorbance of the mixture without EPS-Fe/EPS-Cu but with tested strains, A2 – the absorbance of the mixture with EPS-Fe/EPS-Cu and tested strains.
2.8 Statistical analysis
All experiments were repeated three times and statistically analyzed using the software Origin (V9.8.0.200, OriginLab). Values are presented as mean ± standard deviation (SD). The level of difference between treatments was assessed using the Statistical Package for the Social Sciences (SPSS, V1. SPSS, V24.0, International Business Machines Corporation, United States) using the Tukey method. Differences were considered significant when p ≤ 0.05.
3 Results and discussions
3.1 Isolation and identification of EPS producing bacteria
Four bacterial isolates were screened from the seawater sample for EPS production by using the glutinous rice-processing wastewater based medium. After continuous cultivation for three generations, the faster-growing strain (named SPW) with the highest viscosity in glutinous rice-processing wastewater based medium was selected for further study. The 16S rRNA gene sequence of the strain SPW confirmed it to be Bacillus sp. The strain is currently preserved at the China Center for Type Culture Collection (registration number is CCTCC M 2020298). Subsequently, the effect of glutinous rice-processing wastewater on the yield of EPS from B. licheniformis SPW was investigated. As shown in Figure 1A, the highest yield of EPS reached 8.62 g/L with the sterilized glutinous rice-processing wastewater based medium. However, the yield of EPS in the unsterilized glutinous rice-processing wastewater based medium was decreased to 7.46 g/L. The yield of EPS was 45% of the highest yield when replacing the glutinous rice-processing wastewater with deionized water in the medium. The promotion of the yield of EPS by the glutinous rice-processing wastewater might be attributed by the large amounts of valuable active ingredients, (e.g., carbohydrates, organic nitrogen) in the wastewater (Wang et al., 2022a), which could be beneficial for the strain growth and EPS synthesis. Meanwhile, various microorganisms existed in the wastewater could suppress the growth of B. licheniformis. Thus, sterilization of the glutinous rice-processing wastewater is necessary when it is used in the medium for EPS production. pH and temperature are significant factors that influence the growth rate of bacteria, enzymatic activity, and subsequently, the production of EPS. In order to examine the impact of pH variation within the range of 3–11 and temperature on EPS production, an investigation was conducted on B. licheniformis SPW (Figures 1B,C). Notably, a substantial EPS yield was observed at an initial pH of 7.2 and 9, suggesting that EPS production is most favorable under neutral to moderately alkaline conditions. Furthermore, the optimal temperature for EPS production by B. licheniformis SPW was determined to be 40°C, resulting in the highest recorded EPS yield of 10.2 g/L. The EPS yield exhibited a decrease to 7.12 g/L when subjected to a temperature of 50°C. This decline in EPS yield at elevated temperatures can be attributed to a reduction in both enzyme activity and biomass. In addition, sea salt serves a dual purpose by creating a salt-rich environment that enables marine bacteria to maintain osmotic pressure, while also providing trace elements that promote microbial growth and the EPS production. Notably, the maximum yield of EPS (13.12 g/L) was achieved at a sea salt concentration of 30 g/L (Figure 1D), while higher concentrations led to substantial reductions in EPS yield, potentially due to the inhibitory effects of hypersaline conditions on microbial activity.
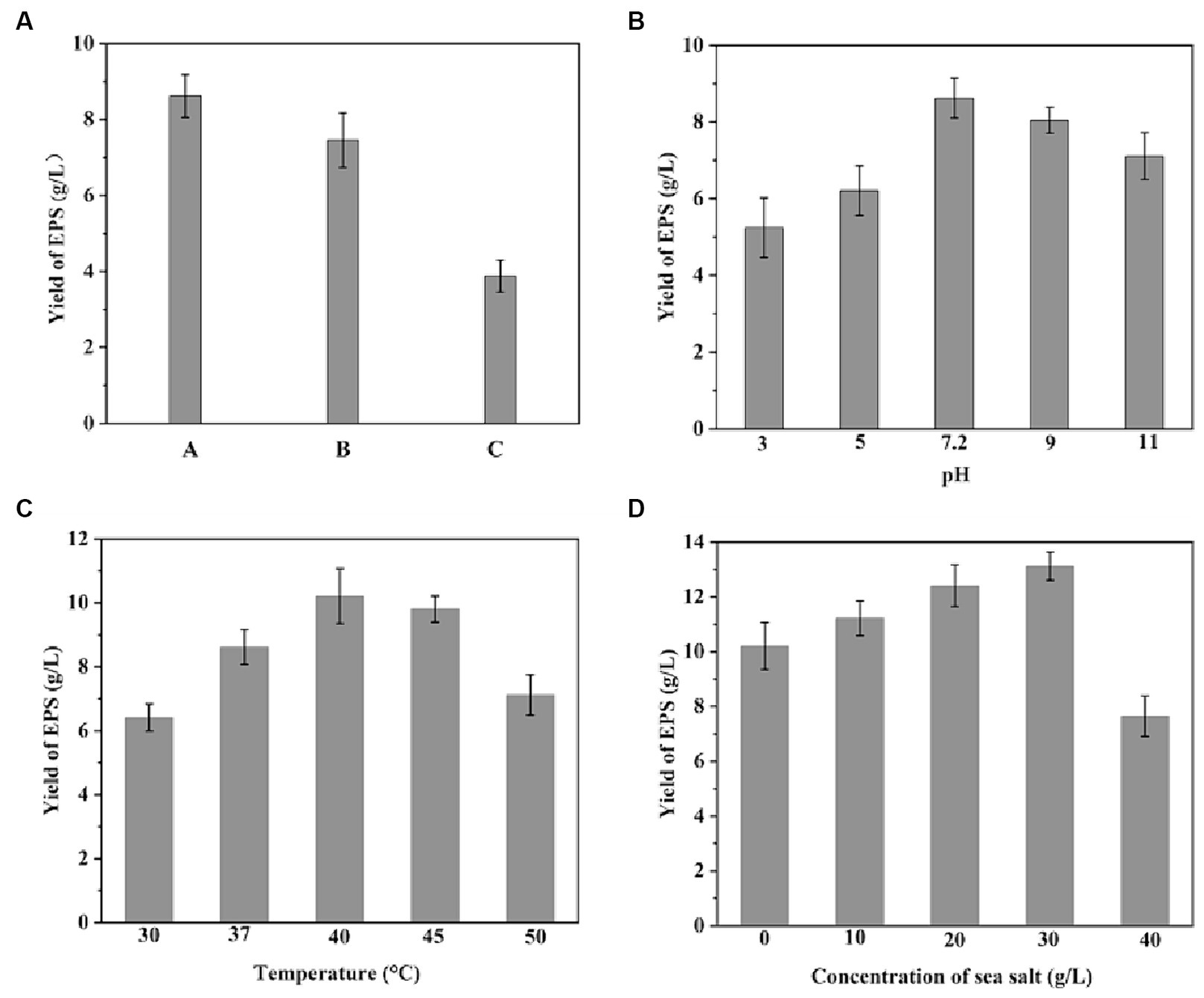
Figure 1. Production of EPS by Bacillus licheniformis CCTCC M2020298 under various conditions. (A) Production of EPS with different fermentation medium: sterilized glutinous rice-processing wastewater based medium (A), unsterilized glutinous rice-processing wastewater based medium (B), sterilized medium with deionized water replacing glutinous rice-processing wastewater (C). (B) Production of EPS at different pH conditions. (C) Production of EPS at different temperatures. (D) Production of EPS at different sodium chloride concentrations.
3.2 Synthesis of EPS-Fe and EPS-Cu, and optimization of the synthesis conditions
The EPS-Fe and EPS-Cu were synthesized with EPS as a template under an alkaline environment. The color of EPS was changed from white to brown and blue when the Fe and Cu bind to the EPS, respectively (Figure 2). In the complexation process, the effects of the dosage of stabilizer trisodium citrate, temperature and pH were studied. Through the single-factor optimization experiment. The Fe content of EPS-Fe could reach 77.4 mg/g when the mass ratio of EPS to trisodium citrate was 2:1, the reaction temperature was at 70°C, and the reaction pH was 8.5–9 (Figure 3). The content of Cu in EPS-Cu could reach 90.7 mg/g under the best conditions of EPS-Fe synthesis. For adults, the requirements for Fe and Cu are approximately 15 and 0.8 mg per day, respectively (Cannas et al., 2020), which are no more than 200 mg of EPS-Fe and 10 mg of EPS-Cu.
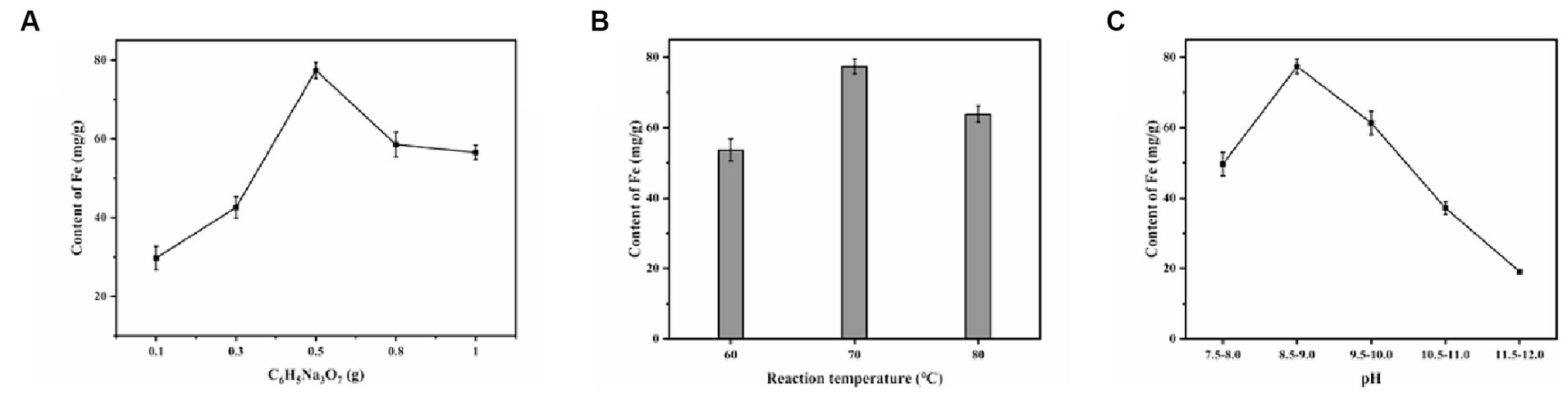
Figure 3. Effect of different factors on the iron content of the synthesized EPS-Fe: (A) C6H5NaO7 dosage, (B) reaction temperature and (C) pH.
3.3 Characterization of EPS, EPS-Fe and EPS-Cu
The element composition, functional groups and valence of the EPS-Fe and EPS-Cu were characterized to confirm the synthesis of complexes and to understand their bioactivity and synthesis mechanism. From the EDS images (Figure 4A), atoms C, O, N, P and S were detected on the surface of EPS, suggesting that the EPS may be composed of polysaccharides, nucleic acids, proteins and lips. The EPS produced by B. licheniformis SPW was composed of 88.63% polysacchrides and 10.21% protein (wt/wt). Additional Fe and Cu signals were found on the surface of EPS-Fe (Figure 4B) and EPS-Cu (Figure 4C), respectively, which indicates the successful synthesis of organic Fe and Cu complexes in this study.
Compared with the UV–vis spectrum of the EPS, the UV–vis spectra were absence of the peak at around the wavelength of 270 nm (Wang Y et al., 2015), illustrating the glycoprotein component of the EPS was involved in the complexation reaction with Fe (Figure 5A) and Cu (Figure 5D). It was also previously reported that the selenium could also interact with O- and N- containing groups on the glycoprotein component of the EPS. The functional groups of the EPS were identified using FT-IR spectroscopy. The result of FT-IR is shown in Figures 5B,E, which indicated the presence of hydroxyl, amide, and carboxyl groups in the EPS. FT-IR spectroscopy further demonstrated the varying of functional groups among EPS, EPS-Fe (Figure 5B) and EPS-Cu (Figure 5E). The infrared spectrum of EPS showed a broad and strong C-O-H absorption peak at 3220.96 cm−1, and structural characteristic peaks of carbohydrates at 2824.95 cm−1. and 1077.94 cm-1. This indicated that the EPS contains polysaccharides and abundant active radicals (Zhang et al., 2020). In the spectrum of the synthesized EPS-Fe, the blueshifted of the peak of the hydroxyl group to 3436.83 cm−1 was observed. Moreover, the absorption peaks of the carboxyl group symmetric and antisymmetric stretching vibration were present at 1396.75 and 1589.75 cm−1 (Govarthanan et al., 2020). These differences in the spectrum between EPS and EPS-Fe suggested that the iron might be able to bind with the EPS through Fe-OOH or Fe-O bond. Similarly, the infrared spectrum of EPS-Cu has undergone some changes compared with that of EPS. The peak of the hydroxyl group blueshifted (3436.83 cm−1). The characteristic absorption peak of the carboxyl group appeared at 1601 19 and 1,398 18 cm−1 (Pagliaccia et al., 2022). Most importantly, a new absorption peak at 613.31 cm−1 appeared in the spectrum of EPS-Cu, which was assigned to a stretching vibration of Cu–O.
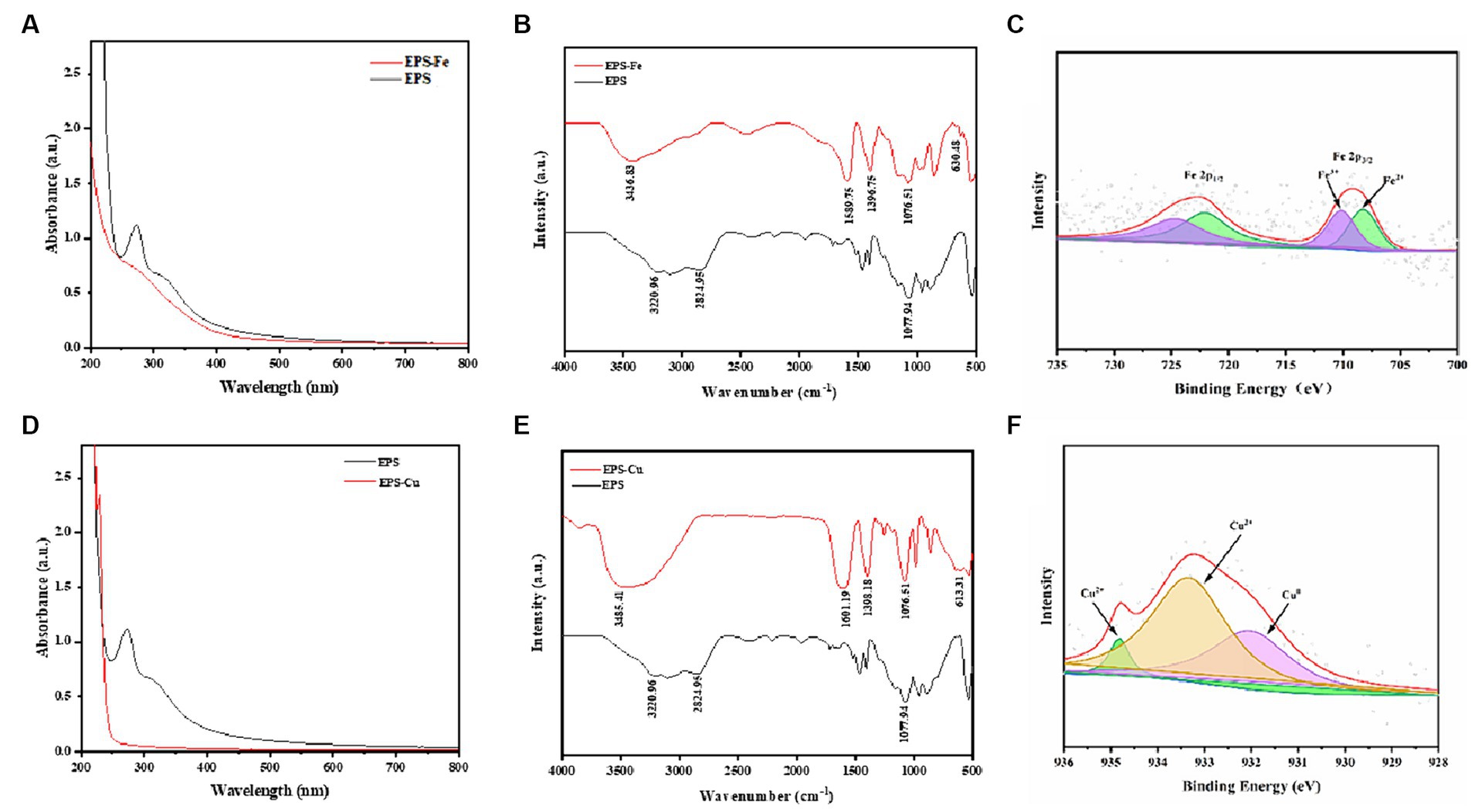
Figure 5. Chemical characteristics of the EPS-Fe and EPS-Cu. UV–vis spectra of EPS-Fe (A) and EPS-Cu (D), FTIR spectra of EPS-Fe (B) and EPS-Cu (E), and Fe 2p (C) and Cu 2p (F) XPS patterns.
Finally, XPS was conducted to analyze the valence state of the Fe (Figure 5C) and Cu (Figure 5F) in the complexes. Typical Fe 2p3/2 and 2p1/2 peaks were detected at 723 and 709 eV, respectively, confirming that the valence state of Fe in EPS-Fe was divalent and trivalent. The satellite peaks (710 and 708 eV) of the Fe 2p3/2 indicate different chemical environments, i.e., Fe—OOH and Fe—O. A typical Cu 2p peak was detected at 932 eV, which includes three satellite peaks at 934.28, 933.33 and 932.03 eV. This indicated the valence state of Cu in EPS-Cu was zero and divalent, in which most of Cu was divalent.
In addition, the cost of the organic Fe or Cu was calculated thoroughly by combing the energy and material consumption, which are about 500 dollars per kilogram organic Fe or Cu. If the glutinous rice processing wastewater treatment cost was deducted, the cost could be lower.
3.4 Biological activity of EPS-Fe and EPS-Cu
3.4.1 Anti-oxidant activity
To determine the antioxidant activity of EPS-Fe and EPS-Cu, the scavenging effects of them on -OH, DPPH and -O2− free radicals were investigated. It can be seen from Figure 6 that the free radical scavenging ability of EPS, EPS-Fe and EPS-Cu shown in a concentration-dependent manner were dosage dependent. Compared with EPS, the antioxidant activity of EPS-Fe and EPS-Cu did not change significantly, indicating that the complexation of EPS with Fe or Cu did not enhance or weaken its antioxidant activity. Their anti-oxidant activities were not as strong as that of Vc. The scavenging activities on ·OH and ·O2− were relatively higher than that on DPPH for the EPS-Fe and EPS-Cu in the range of 0.25–4 g/L. When the concentration of EPS-Fe and EPS-Cu reached 4 g/L, the scavenging rates of -OH and -O2− free radicals were higher than 60%.
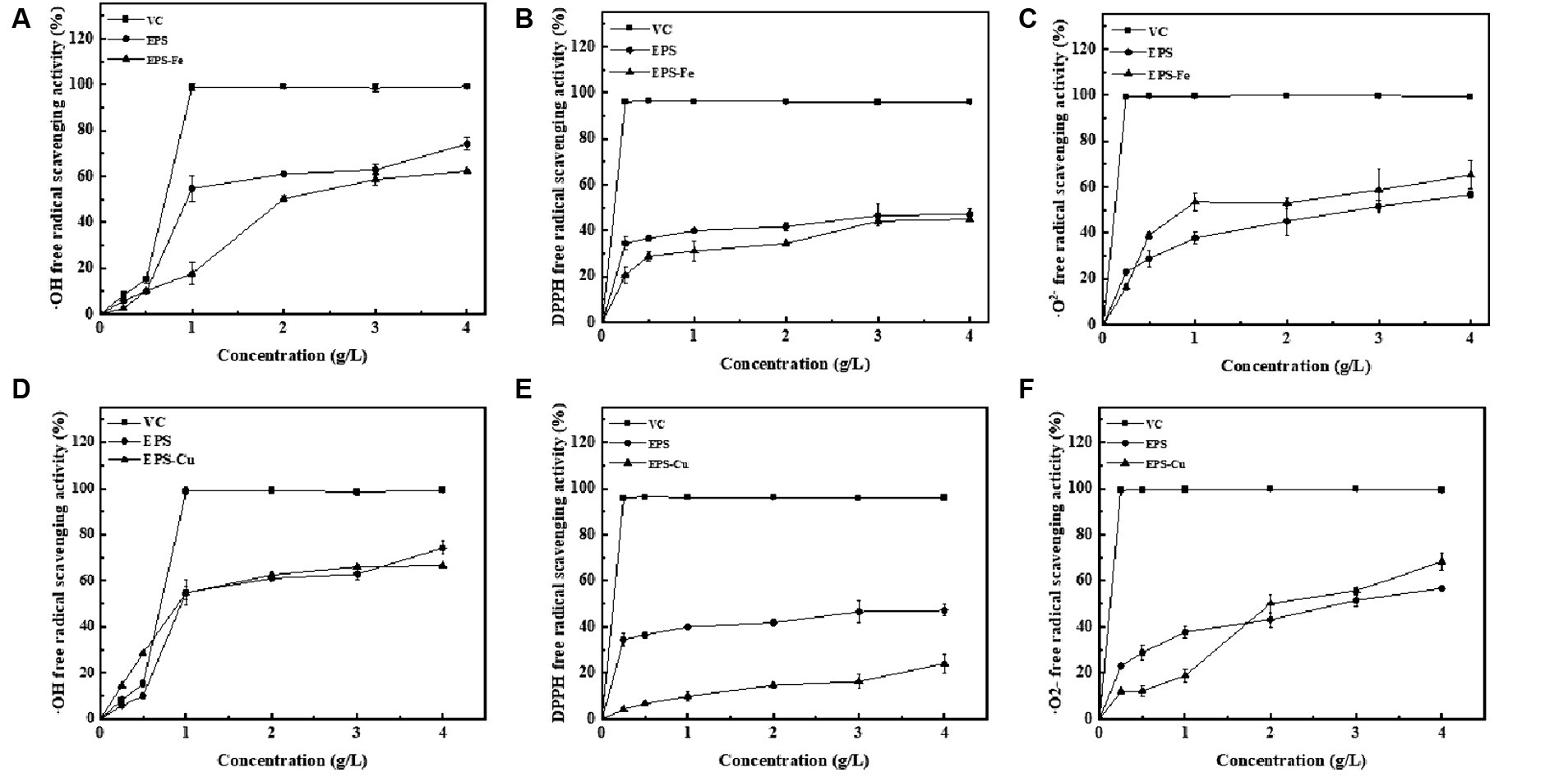
Figure 6. Evaluation of the antioxidant of the EPS-Fe and EPS-Cu. Scavenging activity of ·OH free radicals by various concentration of EPS-Fe (A) and EPS-Cu (D), scavenging activity of DPPH free radicals by various concentration of EPS-Fe (B) and EPS-Cu (E), and scavenging activity of ·O2− free radicals by various concentration of EPS-Fe (C) and EPS-Cu (F). VC was used as a reference compound.
The long-term effect of subcytotoxic stress on cells can induce premature senescence (Dasari et al., 2006). As the present biosynthesized EPS-Fe and EPS-Cu exhibited an antioxidant property, their protective effect against H2O2 oxidative stress-mediated cells was determined. First, an H2O2-induced cellular injury model was established by measuring the damaging effects of H2O2 at different concentrations on HCoEpiCs. As shown in Figure 7A, H2O2 showed a concentration-dependent (0.05–3 mM) potency for HCoEpiCs. Cell viability was reduced to 47.30% (<50%) when HCoEpiCs were incubated with 2.5 mM H2O2. Therefore, 2.5 mM H2O2 was selected to stimulate the HCoEpiCs. The HCoEpiCs were first treated with EPS-Fe or EPS-Cu for 24 h and then exposed to 2.5 mM H2O2 for another 4 h. The HCoEpiCs showed increased cell viability compared with the cells without prior treatment with EPS-Fe or EPS-Cu (Figures 7B,C). Moreover, the cell viability of the HCoEpiCs could be restored from 47.30 to 58.2% when the concentration of EPS-Fe was increased from 0 to 500 μg/mL. Based on H2O2 stimulation, the EPS-Fe intervention can reduce the oxidative stress and apoptosis levels of HCoEpiCs, and the protective ability of the EPS-Fe for H2O2-induced HCoEpiCs is dose-dependent. The cell viability of the HCoEpiCs was not increased obviously with the concentration of EPS-Cu increased from 0 to 500 μg/mL. The cell HCoEpiCs were even destroyed by EPS-Cu with a concentration higher than 50 μg/mL, which might be due to the low tolerance of cells for Cu.
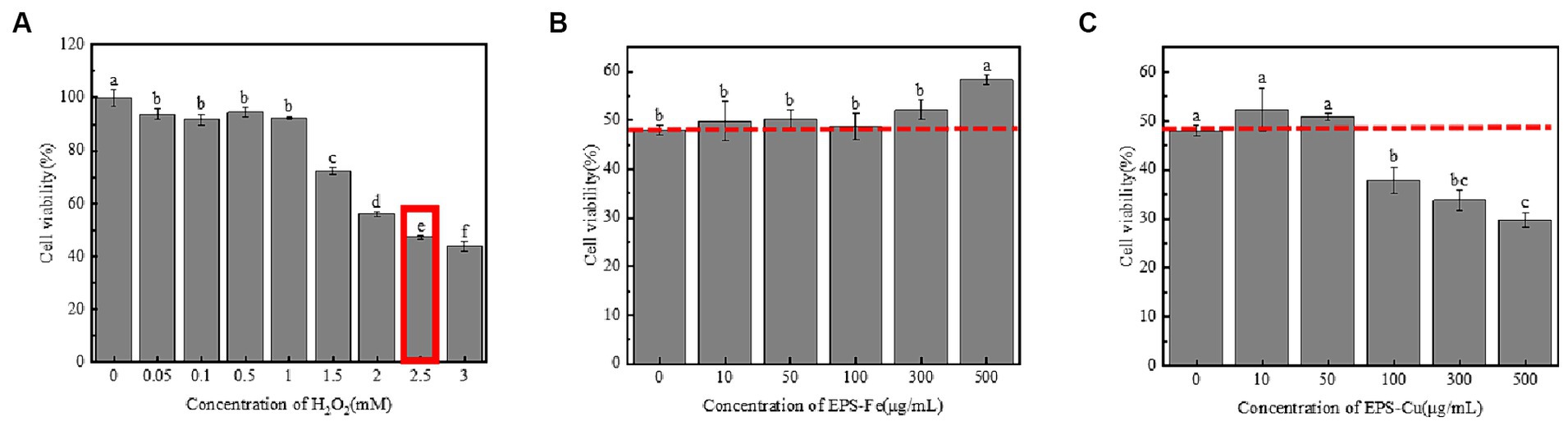
Figure 7. Protective effect of EPS-Fe and EPS-Cu on damaged cells. (A) Establishment of a H2O2-induced cellular injury model. Protectivity of (B) EPS-Fe and (C) EPS-Cu for the H2O2 injured HCoEpiCs. Different letters (a, b, c, d, e and f) on bars in the same group indicate a significant difference between the different concentrations of H2O2/EPS-Fe/Cu on the cell viability of HCoEpiCs.
3.4.2 Anti-proliferation activity
The EPS-Fe and EPS-Cu in this study contained EPS of bacterial origin. Thus, their damage to human cells needs to be determined. Here, HCoEpiC cells located on the surface of the human colonic mucosa were selected to evaluate the cytotoxicity of the EPS-Fe, EPS-Cu and inorganic Fe and Cu. It can be seen from the Figure 8A,B show that the toxic effect of the EPS-Fe and EPS-Cu was much smaller than that of the corresponding inorganic format for HCoEpiC cells under the same concentration of metal atom. These results indicate that the EPS-Fe and EPS-Cu are much safer than the inorganic metal at the same amount of metal supplementation for the human body.
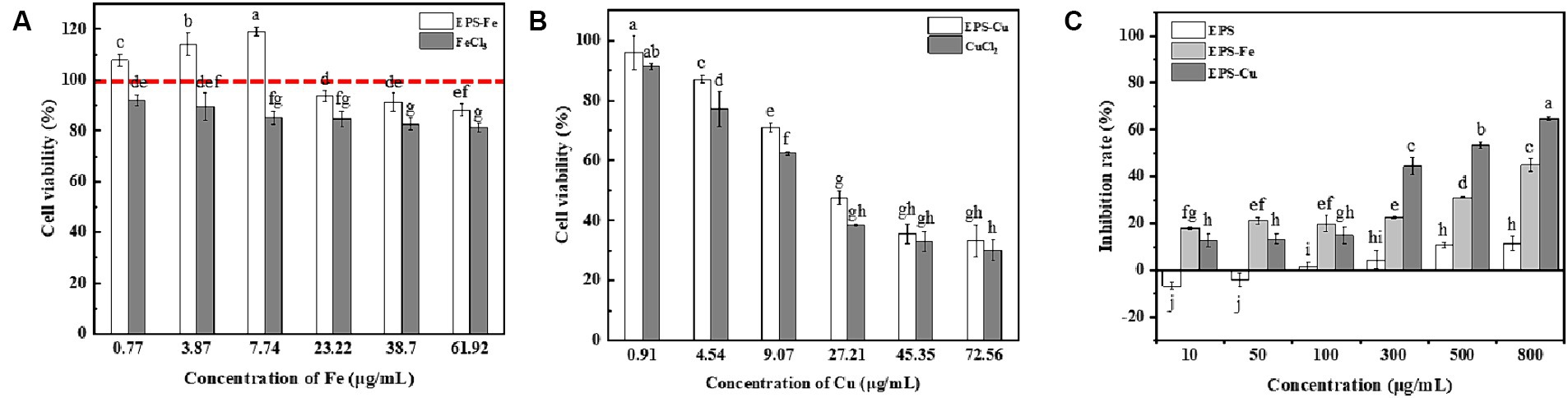
Figure 8. Determination of the cytotoxicity and anti-tumour activity of EPS-Fe and EPS Cu. (A) Cytotoxicity of EPS-Fe and FeCl3 on HCoEpiCs. (B) Cytotoxicity of EPS-Cu and CuCl2 on HCoEpiCs. (C) Anti-proliferation of EPS-Fe and EPS-Cu against mouse hepatoma cells H22. Different letters (a, b, c, d, e, f, g, h, i) on bars in the same group indicate a significant difference between different concentrations of /FeCl3/CuCl2/EPS-Fe/EPS-Cu on HCoEpiCs or mouse hepatoma cells H22.
It has been reported that the antitumor activity of EPS could be enhanced by chemical modification (i.e., sulfation and phosphorylation) (Li et al., 2021). In this study, the antiproliferation activity of the EPS-Fe and EPS-Cu against mouse hepatocellular carcinoma H22 was tested and compared with that of EPS. As illustrated in Figure 8C, the EPS-Fe and EPS-Cu exhibited a dose-dependent inhibition of the proliferation of H22 cells, and the antiproliferation activity of the EPS-Fe and EPS-Cu was remarkably higher than that of EPS. This result indicates that the antitumor activity of EPS could be enhanced by the interacted Fe and Cu. Moreover, the EPS-Fe and EPS-Cu synthesized in this study had higher toxicity and selectivity toward cancer cells than normal cells, suggesting their cancer-fighting potential.
3.4.3 Anti-bacterial activity
The mammalian gut harbors trillions of microorganisms, and the balance of the gut microbiome plays a central role in host health infection. However, the colonization of pathogenic bacteria or opportunistic pathogens in the gut can lead to serious infection and disease, such as inflammatory bowel disease (IBD), appendicitis and cancer (Venkova et al., 2018). The inhibitory effect of EPS-Fe on the proliferation of Staphylococcus aureus and Salmonella was evaluated. As shown in Table 1, when 1,000 μg/mL of EPS-Fe or EPS-Cu was added to the medium, the growth of six tested strains could be inhibited in varying degrees. The anti-bacterial activity of EPS-Fe was lower than that of EPS-Cu. In addition, the EPS-Cu has a better inhibitory effect on the proliferation of the Gram-positive strain Staphylococcus aureus than the Gram-negative strain Salmonella enterica. The highest inhibition rate for Staphylococcus aureus ATCC 12600 could reach 57.6% when the concentration of EPS-Cu was 1,000 μg/mL.
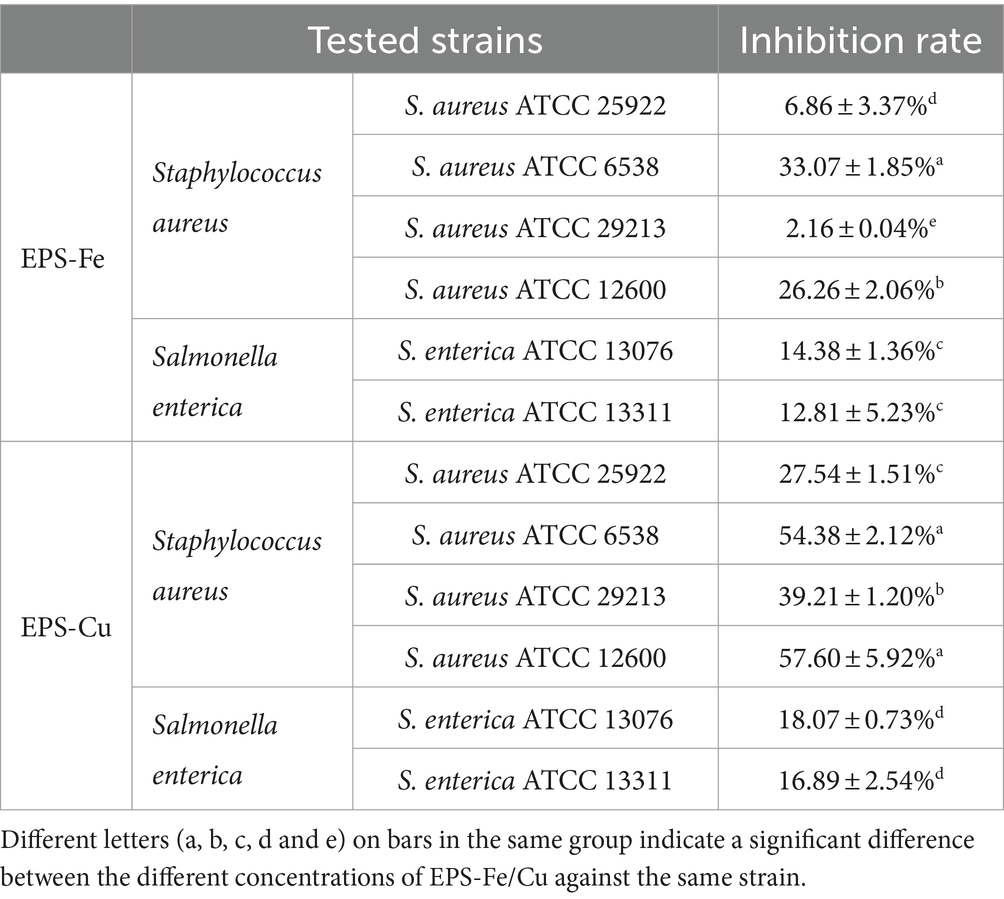
Table 1. Evaluation of the antibacterial activity of the EPS-SeNPs and EPS-Cu at the concentration of 1,000 μg/mL.
4 Conclusion
This study presents a sustainable avenue to synthesize EPS-Fe and EPS-Cu with B. licheniformis EPS fermented from glutinous rice processing wastewater. In particular, the EPS-Fe and EPS-Cu are much safer than the corresponding inorganic metal. Moreover, the EPS-Fe and EPS-Cu possess antioxidant, antitumor and antibacterial activities depending on the combination of EPS and inorganic metal. Overall, this work provides a potential way to reduce the environmental pollution of the glutinous rice processing wastewater for producing valuable and to reduce the production cost of organic metal complexes, facilitating large-scale production and practical application of organic metal complexes as dietary supplements and feed or fertilizer additives.
Data availability statement
The nucletide data presented in the study are deposited in the GenBase repository (https://ngdc.cncb.ac.cn/genbase/), accession number 0002950.
Author contributions
ZC: Data curation, Formal analysis, Investigation, Writing – original draft. SW: Conceptualization, Methodology, Writing – review & editing. ZH: Data curation, Formal analysis, Writing – original draft. FW: Methodology, Validation, Writing – review & editing. YY: Formal analysis, Investigation, Writing – review & editing. YH: Methodology, Writing – review & editing. YL: Formal analysis, Investigation, Methodology, Writing – original draft. ZY: Methodology, Software, Writing – review & editing. YC: Formal analysis, Visualization, Writing – review & editing. WZ: Writing – review & editing. DL: Methodology, Writing – review & editing. ZW: Data curation, Funding acquisition, Methodology, Project administration, Supervision, Writing – review & editing, Writing – original draft. HY: Project administration, Resources, Supervision, Writing – review & editing.
Funding
The author(s) declare financial support was received for the research, authorship, and/or publication of this article. This research was funded by the National Natural Science Foundation of China (22308338, 52200178, 22208276), Key Program for Collaborative and Innovation of Nanyang, Henan Province, China (21XTCX21001), the Henan Provincial Key Technology Research and Development Program (222102310142 and 12102310864), Key Research Project of the Higher Education Institutions of Henan Province, China (22A530010) and National Engineering Research Center of Solid-state Brewing (GFGS-2023000501).
Conflict of interest
The authors declare that the research was conducted in the absence of any commercial or financial relationships that could be construed as a potential conflict of interest.
Publisher’s note
All claims expressed in this article are solely those of the authors and do not necessarily represent those of their affiliated organizations, or those of the publisher, the editors and the reviewers. Any product that may be evaluated in this article, or claim that may be made by its manufacturer, is not guaranteed or endorsed by the publisher.
References
Bai, X., Qiu, Z., Zheng, Z., Song, S., Zhao, R., Lu, X., et al. (2022). Preparation and characterization of garlic polysaccharide-Zn (II) complexes and their bioactivities as a zinc supplement in Zn-deficient mice. Food Chem. X 15:100361. doi: 10.1016/j.fochx.2022.100361
Cannas, D., Loi, E., Serra, M., Firinu, D., Valera, P., and Zavattari, P. (2020). Relevance of essential trace elements in nutrition and drinking water for human health and autoimmune disease risk. Nutrients 12, 1–22. doi: 10.3390/nu12072074
Cheng, Y., Xiao, X., Li, X., Song, D., Lu, Z., Wang, F., et al. (2017). Characterization, antioxidant property and cytoprotection of exopolysaccharide-capped elemental selenium particles synthesized by Bacillus paralicheniformis SR14. Carbohydr. Polym. 178, 18–26. doi: 10.1016/j.carbpol.2017.08.124
Dasari, A., Bartholomew, J. N., Volonte, D., and Galbiati, F. (2006). Oxidative stress induces premature senescence by stimulating caveolin-1 gene transcription through p 38 mitogen-activated protein kinase/Sp1-mediated activation of two GC-rich promoter elements. Cancer Res. 66, 10805–10814. doi: 10.1158/0008-5472.CAN-06-1236
Escárcega-González, C. E., Garza-Cervantes, J. A., Vázquez-Rodríguez, A., and Morones-Ramírez, J. R. (2018). Bacterial exopolysaccharides as reducing and/or stabilizing agents during synthesis of metal nanoparticles with biomedical applications. Int. J. Polym. Sci. 2018, 1–15. doi: 10.1155/2018/7045852
Freeland-Graves, J. H., Sanjeevi, N., and Lee, J. J. (2015). Global perspectives on trace element requirements. J. Trace Elem. Med. Biol. 31, 135–141. doi: 10.1016/j.jtemb.2014.04.006
Gao, W., Huang, Y., He, R., and Zeng, X. (2018). Synthesis and characterization of a new soluble soybean polysaccharide-iron (III) complex using ion exchange column. Int. J. Biol. Macromol. 108, 1242–1247. doi: 10.1016/j.ijbiomac.2017.11.038
Govarthanan, M., Jeon, C. H., Jeon, Y. H., Kwon, J. H., Bae, H., and Kim, W. (2020). Non-toxic nano approach for wastewater treatment using Chlorella vulgaris exopolysaccharides immobilized in iron-magnetic nanoparticles. Int. J. Biol. Macromol. 162, 1241–1249. doi: 10.1016/j.ijbiomac.2020.06.227
Harish, R., Samuel, J., Mishra, R., Chandrasekaran, N., and Mukherjee, A. (2012). Bio-reduction of Cr (VI) by exopolysaccharides (EPS) from indigenous bacterial species of Sukinda chromite mine, India. Biodegradation 23, 487–496. doi: 10.1007/s10532-011-9527-4
Harvey, K. M., Cooke, R. F., Colombo, E. A., Rett, B., De Sousa, O. A., Harvey, L. M., et al. (2021). Supplementing organic-complexed or inorganic co, cu, Mn, and Zn to beef cows during gestation: Postweaning responses of offspring reared as replacement heifers or feeder cattle. J. Anim. Sci. 99, 1–11. doi: 10.1093/jas/skab082
Huang, X., Ni, J., Yang, C., Feng, M., Li, Z., and Xie, D. (2018). Efficient ammonium removal by bacteria rhodopseudomonas isolated from natural landscape water: China case study. Water (Switzerland) 10:1107. doi: 10.3390/w10081107
Jin, M., Lu, Z., Huang, M., Wang, Y., and Wang, Y. (2011). Sulfated modification and antioxidant activity of exopolysaccahrides produced by Enterobacter cloacae Z 0206. Int. J. Biol. Macromol. 48, 607–612. doi: 10.1016/j.ijbiomac.2011.01.023
Jing, Y., Zhang, S., Li, M., Zhang, R., Zhang, H., Zheng, Y., et al. (2022). Structural characterization and biological activities of polysaccharide iron complex synthesized by plant polysaccharides: a review. Front. Nutr. 9:1013067. doi: 10.3389/fnut.2022.1013067
Kostova, I. (2022). Editorial to the special issue: “synthesis of organic ligands and their metal complexes in medicinal chemistry.”. Molecules 27:3644. doi: 10.3390/molecules27113644
Krüger, R. A., and Baumgartner, T. (2010). Metal-rich organometallics. Dalton (Cambridge, England) 39, 5759–5767. doi: 10.1039/b925709h
Li, Y., Lin, Z., Guo, M., Xia, Y., Zhao, M., Wang, C., et al. (2017). Inhibitory activity of selenium nanoparticles functionalized with oseltamivir on H1N1 influenza virus. Int. J. Nanomedicine 12, 5733–5743. doi: 10.2147/IJN.S140939
Li, Y., Lv, J., Wang, J., Zhou, S., Zhang, G., Wei, B., et al. (2021). Changes in carbohydrate composition in fermented Total mixed ration and its effects on in vitro methane production and microbiome. Front. Microbiol. 12, 1–10. doi: 10.3389/fmicb.2021.738334
Lu, Q., Xu, L., Meng, Y., Liu, Y., Li, J., Zu, Y., et al. (2016). Preparation and characterization of a novel Astragalus membranaceus polysaccharide-iron (III) complex. Int. J. Biol. Macromol. 93, 208–216. doi: 10.1016/j.ijbiomac.2016.08.049
Megías, C., Pedroche, J., Yust, M. M., Girón-Calle, J., Alaiz, M., Millán, F., et al. (2008). Production of copper-chelating peptides after hydrolysis of sunflower proteins with pepsin and pancreatin. LWT 41, 1973–1977. doi: 10.1016/j.lwt.2007.11.010
Mehri, A. (2020). Trace elements in human nutrition (II) – an update. Int. J. Prev. Med. 11:2. doi: 10.4103/ijpvm.IJPVM
Miller, J. L. (2013). Iron deficiency anemia: a common and curable disease. Cold Spring Harb. Perspect. Med. 3:a011866. doi: 10.1101/cshperspect.a011866
Mitić, Ž., Cakić, M., Nikolić, G. M., Nikolić, R., Nikolić, G. S., Pavlović, R., et al. (2011). Synthesis, physicochemical and spectroscopic characterization of copper (II)-polysaccharide pullulan complexes by UV-vis, ATR-FTIR, and EPR. Carbohydr. Res. 346, 434–441. doi: 10.1016/j.carres.2010.12.011
Muras, A., Romero, M., Mayer, C., and Otero, A. (2021). Biotechnological applications of Bacillus licheniformis. Crit. Rev. Biotechnol. 41, 609–627. doi: 10.1080/07388551.2021.1873239
Myint, Z. W., Oo, T. H., Thein, K. Z., Tun, A. M., and Saeed, H. (2018). Copper deficiency anemia: review article. Ann. Hematol. 97, 1527–1534. doi: 10.1007/s00277-018-3407-5
Pagliaccia, B., Carretti, E., Severi, M., Berti, D., Lubello, C., and Lotti, T. (2022). Heavy metal biosorption by extracellular polymeric substances (EPS) recovered from anammox granular sludge. J. Hazard. Mater. 424:126661. doi: 10.1016/j.jhazmat.2021.126661
Strieth, D., Schwarz, A., Stiefelmaier, J., Erdmann, N., Muffler, K., and Ulber, R. (2021). New procedure for separation and analysis of the main components of cyanobacterial EPS. J. Biotechnol. 328, 78–86. doi: 10.1016/j.jbiotec.2021.01.007
Tang, M., Wang, D., Hou, Y., Buchili, P., and Sun, L. (2013). Preparation, characterization, bioavailability in vitro and in vivo of tea polysaccharides-iron complex. Eur. Food Res. Technol. 236, 341–350. doi: 10.1007/s00217-012-1891-8
Tavsan, Z., and Ayar Kayali, H. (2013). The effect of iron and copper as an essential nutrient on mitochondrial electron transport system and lipid peroxidation in Trichoderma harzianum. Appl. Biochem. Biotechnol. 170, 1665–1675. doi: 10.1007/s12010-013-0273-4
Venkova, T., Yeo, C. C., and Espinosa, M. (2018). Editorial: the good, the bad, and the ugly: multiple roles of bacteria in human life. Front. Microbiol. 9, 9:Article 1702. doi: 10.3389/fmicb.2018.01702
Wang, Z., Li, Y., Hui, Z., Liu, J., Guo, X., Chen, Z., et al. (2023). Biologically active selenium nanoparticles composited with Bacillus licheniformis extracellular polymeric substances fermented from cane molasses. LWT 187:115255. doi: 10.1016/j.lwt.2023.115255
Wang, Y., Li, Y., Liu, Y., Chen, X., and Wei, X. (2015). Extraction, characterization and antioxidant activities of se-enriched tea polysaccharides. Int. J. Biol. Macromol. 77, 76–84. doi: 10.1016/B978-0-323-88537-9.00012-X
Wang, X., Rungang, T., Huanyi, Y., Shen, C., and Han, X. (2022a). Effect of baking technique for rice wine production and the characteristics of baked rice wine. J. Sci. Food Agric. 102, 1498–1507. doi: 10.1002/jsfa.11484
Wang, Z., Shen, L., Zhuang, X., Shi, J., Wang, Y., He, N., et al. (2015). Flocculation characterization of a bioflocculant from Bacillus licheniformis. Ind. Eng. Chem. Res. 54, 2894–2901. doi: 10.1021/ie5050204
Wang, X., Zhao, Y., Wu, X., Cui, L., and Mao, S. (2022b). Editorial: trace element chemistry and health. Front. Nutr. 9:1034577. doi: 10.3389/fnut.2022.1034577
Wu, W., Li, B., Hou, H., Zhang, H., and Zhao, X. (2017). Isolation and identification of calcium-chelating peptides from Pacific cod skin gelatin and their binding properties with calcium. Food Funct. 8, 4441–4448. doi: 10.1039/C7FO01014A
Xiong, Y., Wang, Y., Yu, Y., Li, Q., Wang, H., Chen, R., et al. (2010). Production and characterization of a novel bioflocculant from Bacillus licheniformis. Appl. Environ. Microbiol. 76, 2778–2782. doi: 10.1128/AEM.02558-09
Ye, S., Liu, F., Wang, J., Wang, H., and Zhang, M. (2012). Antioxidant activities of an exopolysaccharide isolated and purified from marine Pseudomonas PF-6. Carbohydr. Polym. 87, 764–770. doi: 10.1016/j.carbpol.2011.08.057
Yuan, S., Dong, P. Y., Ma, H. H., Liang, S. L., Li, L., and Zhang, X. F. (2022). Antioxidant and biological activities of the Lotus root polysaccharide-Iron (III) complex. Molecules 27:7106. doi: 10.3390/molecules27207106
Zemrani, B., and Bines, J. E. (2020). Recent insights into trace element deficiencies: causes, recognition and correction. Curr. Opin. Gastroenterol. 36, 110–117. doi: 10.1097/MOG.0000000000000612
Zhang, X., Fan, W. Y., Yao, M. C., Yang, C. W., and Sheng, G. P. (2020). Redox state of microbial extracellular polymeric substances regulates reduction of selenite to elemental selenium accompanying with enhancing microbial detoxification in aquatic environments. Water Res. 172:115538. doi: 10.1016/j.watres.2020.115538
Zhuang, X., Wang, Y., Li, Q., Yan, S., and He, N. (2012). The production of bioflocculants by Bacillus licheniformis using molasses and its application in the sugarcane industry. Biotechnol. Bioprocess Eng. 17, 1041–1047. doi: 10.1007/s12257-012-0213-0
Keywords: extracellular polymer substance (EPS), trace element, Bacillus species, complexes, glutinous rice processing wastewater
Citation: Chen Z, Wang S, Hui Z, Wang F, Ye Y, He Y, Li Y, Yu Z, Cai Y, Zhuang W, Liu D, Wang Z and Ying H (2024) Sustainable production of extracellular polymeric substances and iron or copper complex from glutinous rice processing wastewater. Front. Sustain. Food Syst. 8:1347500. doi: 10.3389/fsufs.2024.1347500
Edited by:
Hui-Wen Gu, Yangtze University, ChinaReviewed by:
Kashif Ameer, Chonnam National University, Republic of KoreaPoonam Singh, LaserLeap Technologies, Portugal
Copyright © 2024 Chen, Wang, Hui, Wang, Ye, He, Li, Yu, Cai, Zhuang, Liu, Wang and Ying. This is an open-access article distributed under the terms of the Creative Commons Attribution License (CC BY). The use, distribution or reproduction in other forums is permitted, provided the original author(s) and the copyright owner(s) are credited and that the original publication in this journal is cited, in accordance with accepted academic practice. No use, distribution or reproduction is permitted which does not comply with these terms.
*Correspondence: Zhi Wang, emhpd2FuZ0B6enUuZWR1LmNu
†These authors have contributed equally to this work