- 1Mariculture Division, Indian Council of Agricultural Research-Central Marine Fisheries Research Institute (ICAR-CMFRI), Veraval, Gujarat, India
- 2Department of Agricultural Science, Texas State University, San Marcos, TX, United States
- 3Fisheries Resource Assessment, Economics and Extension Division, Indian Council of Agricultural Research-Central Marine Fisheries Research Institute (ICAR-CMFRI), Veraval, Gujarat, India
- 4Finfish Fisheries Division, Visakhapatnam Regional Centre, Indian Council of Agricultural Research-Central Marine Fisheries Research Institute (ICAR-CMFRI), Visakhapatnam, Andhra Pradesh, India
- 5Fisheries Resource Assessment, Economics and Extension Division, Indian Council of Agricultural Research-Central Marine Fisheries Research Institute (ICAR-CMFRI), Kochi, Kerala, India
- 6Department of Computer Science, KL University, Vijayawada, Andhra Pradesh, India
- 7Fisheries Resource Assessment, Economics and Extension Division, Vizhinjam Research Centre, Indian Council of Agricultural Research-Central Marine Fisheries Research Institute (ICAR-CMFRI), Thiruvananthapuram, Kerala, India
- 8Mariculture Division, Indian Council of Agricultural Research-Central Marine Fisheries Research Institute (ICAR-CMFRI), Kochi, Kerala, India
- 9Indian Council of Agricultural Research-Central Marine Fisheries Research Institute (ICAR-CMFRI), Kochi, Kerala, India
Mariculture, a sustainable and rapidly growing aquaculture sub-sector, meets global seafood demand while reducing strain on wild fish stocks and continues to expand worldwide. However, rising feed and seed costs pose significant challenges, overshadowing other farming expenses. Climate change exacerbates the profitability of sea cage farming, increasing volatility. The surge in fishmeal costs has a detrimental impact on operational expenditure (Opex) and continues to be a threat. Consequently, sea cage farming in India is at a critical juncture, needing a balance between technological advancements and stakeholders’ fiscal needs. In this context, this study examines peer-reviewed synthesized data by employing metrics to evaluate the implications of feed and seed costs in Asian Seabass (Lates calcarifer) sea cage farming over a 10-year horizon. The total sales revenue over the 10-year period was US $100,848, with a net profit of US $55,198.89. A 30% increase in feed and seed prices significantly altered the economic dynamics of the enterprise, leading to an 8.8 and 9.2% rise in the respective break-even points. There was also a significant effect on the Benefit–Cost Ratio (BCR), with a 30% increase in each factor resulting in a 10 and 18% change in the projected BCR, respectively. Therefore, the significance of feed and seed cost has been established, necessitating an inevitable shift from low-value fish to formulated feed adaption for sustainable mariculture development. The study suggests improvements to existing practices to maximize efficiency and minimize production costs.
1 Introduction
In the wake of the impending Ocean 4.0 blue economy era, sea farming has been identified as a potential marine business and an investment opportunity for all maritime nations. Among them, India is the third largest fish producer, accounting for 8% of global production and with an export value of up to US $8.09 billion in the year 2022–2023 (MPEDA, 2022). Due to the non-availability of cultivable land for aquaculture, driven by the growing population, open sea mariculture is acquiring significance as a potential and innovative solution to improve global food security (Ye et al., 2022). Asian seabass, Lates calcarifer, is a finfish species with considerable economic significance and importance with its widespread area of distribution and popularity on a global scale (FAO, 2023). The expansive occurrence of this particular species extends across tropical and sub-tropical regions, especially within the Indo-West Pacific, spanning from the eastern edge of the Persian Gulf to China, Taiwan, and southern Japan, and further southward to include southern Papua New Guinea and northern Australia (FAO-FAD, 2020; Fish Base, 2023). Owing to the technological advancements in seed production standardization and hatchery production, Asian seabass has emerged as a prominent marine finfish variety (Arasu et al., 2008; Thirunavukkarasu et al., 2009), cultured in marine ecosystems, ocean nursery brackish estuaries, and fresh waters as monoculture or polyculture (Sorphea et al., 2019). Globally, seabass has a vast domestic and international market demand for its products (Hassan et al., 2021; Future Market Insights, 2021). These major factors have incentivized the fishermen and stakeholders to engage in Asian seabass mariculture in sea cages along the territorial waters. However, as a high-value mariculture species, seabass requires high-protein diets, which have traditionally relied on fishmeal and fish oil derived from wild pelagic fish resources. Edwards et al. (2004) have duly reported that conventional aquaculture methods that rely on low value as a direct feed are unlikely to grow considerably in the future. This places the fishermen in a difficult predicament and can lead to financial losses. In addition, economic projections centered around seabass cage culture, especially on implications of feed and seed costs in the face of climate change are still relatively scarce.
Feed is an integral component of production economics (Bakİ and Yücel, 2017), with low-value fish making up the majority of the feed in the marine aquaculture (Bunlipatanon et al., 2014). Nevertheless, the same scenario (low-value fish as feed) has prevailed from the genesis of the indigenous sea cage farming activities since 2007. Of the total aquaculture production, 20 million tons are used for non-human consumption, mainly for fishmeal and fish oil production (16 million tons, 81%). The global fishmeal market is expected to reach US $10.31 billion by 2027, with an increasing CAGR of 4.1% during 2021–2027 (Fish Meal Market: Global Industry Trends, Share, Size, Growth, Opportunity and Forecast 2022-2027, 2024). Despite all these statistical assessments, the use of low-value fish is exponentially found in other feed industries (Allen, 2004; Funge-Smith et al., 2005). Hence, relying on low value for a long time in commercial aquaculture may not lead to positive revenues. Species such as anchovy, capelin, sand eel, and sardines, used for commercial fishmeal, are fast-growing, short-lived pelagic species. The productivity of these species experienced a 50% decline in the North Atlantic Ocean and a 20% reduction globally. This decrease resulted in a diminished supply of raw materials essential for fishmeal production (Schmittner et al., 2003). Moreover, the short-term (El Niño, ENSO) climate variability patterns cause significant reductions in Humboldt anchovy production (Chavez et al., 2003). The production of fishmeal from wild-catch sources is gradually decreasing, leading to the development of low-cost, eco-friendly feed for various species (Baruah et al., 2017). As a direct consequence of all these factors, there have been reports of decreased worldwide fishmeal production in the first 7 months of 2022 (IFFO Reports, 2024). This has caused a demand–supply disruption in the fishmeal industry, which has subsequently led to higher prices. According to the Global Animal Protein Outlook 2023 report, the price of fish meal in 2022 reached US $1,600 per ton, which was significantly higher than the prices seen in recent years (Animal Feed Protein Market Share Statistics Report 2023-2032, 2024).
Climate change significantly challenges marine cage farming by causing extreme weather events that disrupt the ocean’s biophysical environment and mariculture operations. These changes negatively affect the growth, activities, survival, and overall production of marine cage culture species, impacting the industry’s economic and social aspects (Chang et al., 2013; Parsons and Lear, 2001; Takasuka et al., 2007). Instances of severe mortalities in marine sea cages due to extreme weather conditions have been reported, resulting in substantial economic losses. For instance, Hsieh et al. (2008) and Chang et al. (2009) documented cases of mass mortalities in the Penghu Archipelago, Taiwan Strait, which led to a loss exceeding US$10 million. Such incidents demonstrate the vulnerability of cage mariculture to the intensifying effects of climate change (Callaway et al., 2012; Chang et al., 2013; Rosa et al., 2012).
Another major challenge in Asian seabass aquaculture is the limited availability of productive genetic lines and high-quality seeds, hindering commercial mariculture expansion (Pham, 2019). Effective aquaculture practices and prevention of wild fishery depletion require specific seed production management strategies (Shah et al., 2020). It is beyond one to successfully grow marine fish throughout their whole life cycles due to the complexity of their eggs and the tiny size of their larvae when they are first fed (de Jesus-Ayson and Ayson, 2013). In India, the culture of marine fish has taken off as a result of the development of indigenous open sea cage farming of Asian seabass by the Indian Council of Agricultural Research (ICAR)–Central Marine Fisheries Research Institute (CMFRI) and the advancement of hatchery methods by Rajiv Gandhi Centre for Aquaculture (RGCA), which has allowed the production of fry at a regulated price (Kandan, 2009).
Currently, seed dissemination is not well organized and requires a substantial overhaul (Idris et al., 2022). Finding private hatcheries capable of effectively managing and supplying improved brood stock for mass-scale breeding is challenging. Additionally, climate change poses long-term consequences, including the limited availability of wild stock due to reduced growth and survival rates (Barange et al., 2018). The same scenario is reflected majorly across the country coast with capture-based mariculture of seabass in cages. The cost and availability of fishmeal are critical limiting factors for the production of formulated feed required in Asian Seabass culture. Due to climate change, the availability of low-cost fish species (Barange et al., 2009) used for fishmeal production has decreased, leading to increased fishmeal costs. This rise in fishmeal costs subsequently increases feed costs, ultimately affecting the productivity and economic feasibility of seabass culture. This demonstrates a clear connection between feed availability, seed costs, and the broader impacts of climate change on the aquaculture industry. To meet the rising demand for stockable size seeds, central and state administrations have initiated efforts. However, gaps in the consistent supply of stockable size seeds and seasonal integration for sea farming in India need resolution.
Given these considerations, we have analyzed time series data over a decade (2009–2020) to elucidate the dynamics of economic efficiency in seabass cage farming in India and to project the future economic efficiency of this farming innovation directly or indirectly under changing climate conditions. The main objective of this study was to provide an economic analysis of Asian seabass cage farming, focusing on the input costs of feed and seed, and to develop a climate-resilient and sustainable mariculture system in India.
2 Methodology
2.1 Data and cost assessment framework
For the purpose of optimizing investment choices and anticipating the interaction of economic elements in aquaculture enterprise building, we base our projections on data that has been spatio-temporally validated and gathered over a period of 10 years (2009–2020) from peer-reviewed data extraction from the publications. We utilize credible, peer-reviewed data sources that are technically reliable and consistent with the expanding sea cage farming industry in India. The collected data were then segregated into categories, with a specific focus on the farming system (sea cages), biological production outputs (harvests/produce), dimension (size of the cages/production volumes), and input cost structures (Capex and Opex). By evaluating the changes over time and across different locations along the coastline, insights into the spatio-temporal dynamics of the production system along with production capacity were considered. The costs associated with seed and feed were the central part of the analysis. Changes in production were then compared using relevant metrics and developed a framework for authentic data collection as shown in Figure 1.
In view of the importance of baseline in reliable assessment, we have conducted a baseline economic assessment of Asian Seabass (L. calcarifer) in order to have a better understanding of how the cost of feed and seed contributes to the overall cost of production under climate changes. Operational costs such as labor charges, capacity building costs, maintenance expenses, administrative overhead, and depreciation were excluded during the analysis as their implication is insignificant to the total production cost while feed and seed costs contribute the maximum production cost of the facilities (Bakİ and Yücel, 2017; Aswathy and Imelda, 2018). Market data were gathered through primary surveys and secondary sources from the coastal states of India. By accounting for both long-term trends and short-term fluctuations, these data support a comprehensive evaluation of market dynamics and aid in making well-informed judgments within the model. Furthermore, real-time data were collected by team members and validated using available peer-reviewed literature in the public domain and from institutional research and development repositories, including Mojjada et al. (2013), Mojjada et al. (2012a), Rao et al. (2013), Mojjada (2015), and Aswathy and Imelda (2018, 2020) for Asian seabass cage farming. These data were considered in the analysis to arrive at reliable fiscal projections and input decision optimization (Table 1). The enterprise budget of sea cage culture is represented in Table 2.
2.2 Production model and profit impact evaluation
The baseline economic, biological, and technical data from Mojjada et al. (2013), Mojjada et al. (2012a), Rao et al. (2013), Mojjada (2015), and Aswathy and Imelda (2018, 2020) have been integrated, and compared in the evaluation process to increase the validity. We used an extensively practiced and locally developed floating sea cage farming system to study the dynamics and interactions of economic factors. Specifically, we focused on a floating sea cage with a 6-m diameter and a production volume ranging from 150 to 170 m3, actively promoted under national schemes and recognized as a farmer-scale model, for data acquisition and analysis. Table 3 illustrates the anticipated cost structure and life expectancy of the capital items utilized for the culture of this species through which the results depicted in Table 4 were obtained. To enhance the business model and improve fiscal decision-making, we developed a baseline by integrating peer-reviewed studies and data on the mariculture of Asian seabass (Lates calcarifer). This comprehensive approach utilized findings from Mojjada et al. (2013) and Mojjada (2015), ensuring a robust foundation for optimizing economic strategies in mariculture operations. To address the variability in culture system sizes, production volumes were standardized, and production quantities were calculated per unit of output for rationalization and easy understanding. The culture species, growth performance, and culture duration (harvest time) were the biological components of the study. The important biological and production variables were considered, including increases in length and weight (%), increment in weight per day (grams), the specific growth rate (SGR), and survival rate (%), as shown in the following equations (Equations 1–5) (Table 4):
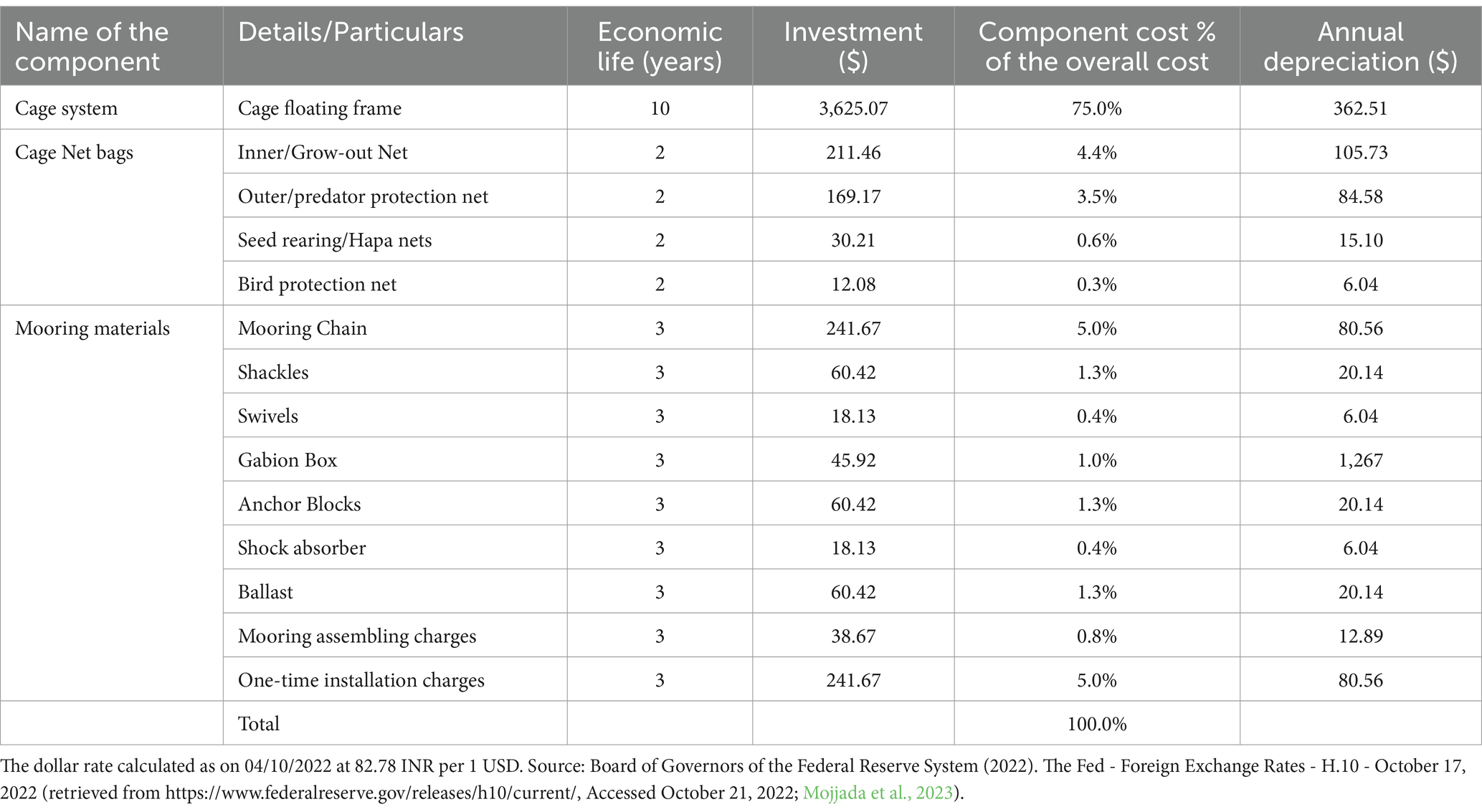
Table 3. The cost structure of the indigenous cage farming system and life expectancy of components.
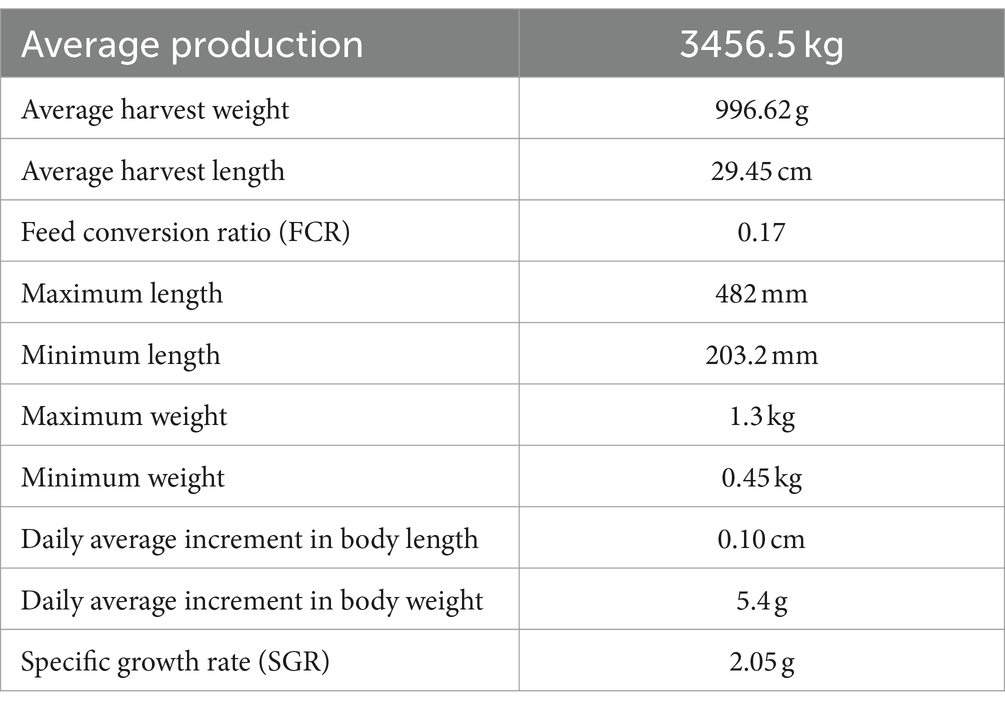
Table 4. Growth parameters of Asian Seabass and production based on Mojjada et al. (2012b) and Mojjada (2015).
The financial and production data that were synthesized from the baseline were employed in order to realize the consequences of feed and seed costs on the production cost. The economic feasibility of the project was then assessed using a variety of important economic metrics, including the Net Present Value of the Project (NPV), Internal Rate of Return (IRR), Benefit–Cost Ratio (BCR), Repay Back Period, Net Profit Margin, and Breakeven point analysis (BEP) represented by the equations (Equations 6–11) below:
where INV = initial investment, NCF = net cash flow, r = weighted average cost of capital for discounting, and t = time period of the cash flow.
where r = discounted rate and i = time;
where i = discount rate, n = number of periods, t = period that the cash flow occurs.
Sensitivity analysis has been performed to assess the future point of fiscal risks of the enterprise and to understand the magnitude of price reaction to changes in underlying factors. Another important element in the assessment of projects is the sensitivity to production costs, which will directly reflect the operational components such as feed and seed. The potential threat to revenue from rising input costs was thoroughly analyzed. Net profit sensitivity, BCR sensitivity, and net present value (NPV) sensitivity were conducted. Therefore, the present research measured the profit sensitivity with respect to the changes in the feed and seed costs, and the sensitivity test suggested positive or negative factor modifications.
3 Results
The economic analysis could examine how the market price and input cost, specifically seed and feed cost in the present study, affect the cage aquaculture profitability in particular to Asian seabass (L. calcarifer), which is a highly sought-after mariculture species in cages (Supplementary Table S1). Evaluation of the net profit margin demonstrated favorable returns from the first year of farming (Figure 2). The total sales revenue was recorded to be US $100,848 from the data analysis over the 10-year horizon, with a net profit of US $55,198.89. With an average net profit margin of 56.6%, it was clearly established that the business is quite profitable after sales. The findings suggest that even a relatively modest increase in feed and seed prices can have a substantial impact on the economic viability of the enterprise. An 8.8–9.2% increase in break-even points indicates that the business would need to generate significantly more revenue to cover its costs, highlighting the importance of managing input costs effectively. The results indicated that shifts in the feed and seeds had a significant effect on BCR, with an increase of 30% in each of these factors resulting in a 10 and 18% change in the projected BCR, respectively (Figure 3). Furthermore, the farming operation could make the indicated profits if the capacity utilization was to the fullest extent and since fixed cost structures remain the same across different levels of capacity utilization, higher capacity utilization results in a higher net profit (Figure 4). Overall, the benefits from this investment were calculated using the BCR and were found to be twice the cost. Figure 5 illustrates the sensitivity of the net present value with regard to the capacity utilization and the direct cost. According to the results, the net present value (NPV) dropped to US $14,998.82 for the most hostile instance of the capacity utilization scenario (a decline in capacity utilization by 25%), while it was reduced to US $20,849.09 when the capacity utilization was reduced to 15%.
4 Discussion
The adverse effects of climate change on agricultural production pose significant challenges to the quantity and quality of global food security (Fatima et al., 2023). Over 50% of the world’s population relies on staple commodities such as wheat, rice, maize, vegetables, legumes, and fish to ensure their food security (Tripathi et al., 2016). However, these essential dietary sources are under considerable pressure as a result of climate change. In India, significant techno-scientific endeavors spanning more than a decade have given rise to the establishment of sea cage culture enterprises (Vijayakumaran et al., 2009; Mohamed et al., 2010; Rao et al., 2010, 2013; Mojjada et al., 2012a,b; Philipose, 2013). The stakeholders optimize the available marine resources because of the exceptional durability and resilience of sea cages, and the technology has been adopted with relative ease because of the higher economic returns (Swathilekshmi et al., 2018; Vipinkumar et al., 2021; Divu et al., 2023b; Divu et al., 2023a). Significant technological advancements in breeding, seed production, and grow-out of marine finfish and shellfish species in artificial enclosures have facilitated profitable farming in open sea, coastal, and estuarine waters (Parappurathu et al., 2023). Grow-out technologies for several commercially important marine species have also been demonstrated in sea cages especially Asian Seabass (Shingare et al., 2020; Daet, 2019).
Feed and seed are the key components in influencing overall farming system profitability (Neori et al., 2004; Bakİ and Yücel, 2017). The economic and environmental sustainability of aquaculture is considerably impacted by the nature and quality of the fish feed, which contributes to a majority share (40–60%) in the operational cost (Suloma and Ogata, 2006). However, marine resources used for fish feed cannot sustain the rising global aquaculture demand indefinitely. While fish meal demand is projected to grow, global fishmeal output has recently declined (Fish Meal Market: Global Industry Trends, Share, Size, Growth, Opportunity and Forecast 2022-2027, 2024). This decline is partly due to climate change affecting ocean circulation and reducing the productivity of key fish stocks such as Peruvian anchovy and sardines (Pike and Barlow, 2003). The average use of pelagic fishmeal in aquaculture feed has significantly decreased over the past decade (Olsen and Hasan, 2012), increasing feed vulnerability and impacting farming profitability (Rana et al., 2009; Maulu et al., 2021). Additionally, the lack of improved seabass strains and high-quality seeds remains a major barrier to sustainable aquaculture development (Pham, 2019). Due to the intricacies of these diverse ecological and socioeconomic factors, stakeholders encounter challenges in understanding the growing cost structure of feed and seed for commercially significant finfish. Therefore, we carried out a comprehensive economic analysis to optimize investment decisions and foresee the interplay of economic factors, such as feed and seed costs of Asian seabass farming. This analysis will assist stakeholders in addressing crucial aspects of sea cage farming ventures, such as increased climate vulnerability of feed and seed and their cost structure, and ultimately improving the return on revenue by assessing the risk appetite of the uptaken components (Bakİ and Yücel, 2017).
Various studies have examined the economic aspects of several aquaculture species in different countries, including Egypt, Nigeria, Korea, and the Philippines (Soliman and Gaber, 1988; Olagunju et al., 2007; Lipton and Kim, 2007; Hurtado et al., 2001). The crucial deliverable of this study is to understand how the major influential factors in operational cost impacted over a period of 10-year horizon. Furthermore, the study examines future vulnerabilities associated with using low-value fish as feed during the grow-out cycle. By consolidating data and examining the correlation between feed and seed costs, the study enables aquaculture industries to understand the economic implications impacting production cost and viability (Adwan, 2017). We have revisited the existing techno-scientific and economic information and subsequently spatio-temporally reassessed the economic performance of the seabass cage operation and feasibility over the course of a decade, comparing our results with legitimately published data from the Indian subcontinent (Vijayakumaran et al., 2009; Mohamed et al., 2010; Rao et al., 2010, 2013; Mojjada et al., 2012a,b; Philipose et al., 2013). The insights derived from our study are transferrable and applicable to regions where seabass culture has been implemented. The adaptability of our findings extends beyond the specific context of the Indian subcontinent, providing valuable implications for areas globally engaged in seabass aquaculture. We stress the essential alignment of economic sustainability with environmental considerations, a prerequisite for Goal 14 of the Sustainable Development Goals (SDGs) for 2030 (Arru et al., 2019). Furthermore, this is the first empirical research in this sector to assess the economic implications of fish feed and seed into the input production cost, thereby facilitating the development of climate-resilient and sustainable Asian seabass cage farming in the Indian subcontinent.
Both the demand for fish feed and seed, along with their prices, are expected to escalate. This escalation will make it more challenging, particularly for smaller-scale producers, to continue their mariculture enterprises. Without anticipating the input cost escalations and the intricacies of Capex, stakeholders may find themselves facing money-losing ventures. Furthermore, the operational cost structure changes compel the Indian aquaculture sector to raise the cost of raw materials used for fish feed. Hence, the immediate need of the hour is to explore scenarios that could help reduce the feed and seed costs in cage farming systems. Several researchers have looked at alternative raw materials, such as insect-based or plant-based sources for fish feed to counter the problem of growing feed costs and to enhance the long-term viability of mariculture systems in a sustainable way (Van Huis, 2013; Arru et al., 2019; Jannathulla et al., 2019). Skretting, being one of the international aquafeed companies, has also recently revealed an R&D breakthrough with their micro-balanced feed formulation, producing a fishmeal-free feed (Fishmeal-Free Breakthrough for Skretting, 2023). However, despite these technological advancements, there is still a pressing need for an economic analysis of seabass cage aquaculture. We require a thorough and diligent examination to obtain the necessary social or entrepreneurial approval.
Numerous countries, such as Singapore (Liu et al., 2024; Yue et al., 2017), Vietnam (Khang et al., 2018), Thailand (Joerakate, et al., 2018; Senanan et al., 2015), and Malaysia (Idris et al., 2022) have initiated breeding programs for the Asian seabass to improve its growth. The Asian seabass culture in open sea cages proved to be a successful and futuristic mariculture business due to its exceptional market demand across the globe. In our study, the evaluation indicated that the venture’s cumulative average return on revenue (RoR) was 52% at 67.8% capacity utilization and could reach 63% at 100% capacity utilization. Therefore, sea cage cultivation is a significantly profitable venture under the present circumstances, and input costs can be recovered speedily. The scheme provisions of the Government of India would further boost these technology ventures. Moreover, data show that the average production from the farmer-scale model was 3.45 tons, yielding a net profit of US $2,024.64, based on a total input cost of US $4,789.91 (with an annual fixed cost of US $1,713.65 and total operational costs of US $3,076.27). The NPV of the investment was US $23,564.71, with the BCR of the investment of 0.5 for the first year and 1.5 for 10 cumulative years. The IRR or RoR was 29.7% for 1 year and 34.3% for 10 cumulative years.
The present study indicated that the fish cost was US $1.45 per kg, but their selling price was US $2.28 per kg. Aswathy et al. (2020) recorded that the cost/kg of the fish was US $2.13, while the selling price was US $4.83 per kg. This difference may be attributed to the regional and seasonal demand of the species as the average selling price per kg of seabass in India was US $1.81 in 2010 (Ravisankar and Thirunavukkarasu, 2010), representing a 2.67-fold increase in cost over 10 years. The cage-reared seabass in the brackish waters of Vennangapattu and Mammalapuram, Tamil Nadu (East coast), was priced at US $4.83 in 2017 (Kumaran et al., 2021), which is approximately 2.6 times the selling price in 2010. Therefore, the variances clearly indicate the geographical (Eastern and Western coastal states of India) demand for the species, demonstrating that seabass has achieved remarkable incremental price realization in nearly all situations. In addition, this suggests that the net profit from seabass aquaculture has the potential to increase.
The contribution of low-value fish to the total price of production was recorded to be 34% when the seabass market price was US $1.81 per kg. In contrast, if the computed production cost uses current market prices with the expected feed conversion ratio (FCR) (1:1.5), the feed cost amounts to US $12,203.23, which is expensive and may lead to a loss-making enterprise. However, a subsequent increase in the market price of the seabass has also been documented, and at present, it ranges from US $6.95 to 9.15 per kg (Sea Bass Wholesalers and Wholesale Dealers in India, 2024; Connect2india, 2024). This has helped to maintain the breakeven threshold and equalize the input costs. This demonstrated the association between the cost of feed and the cost of production, as well as how seabass farming may still prove to be a lucrative endeavor despite all these factors. In addition, one might argue that it will continue to generate a profit up to the point when the ratio of the cost of feed to the price of seabass on the market is maintained at a linear level, which is very well depicted in Figure 3. Considering these intricacies and after recognizing that seabass farming has a significant potential for growth, several international companies have only recently entered the Indian market for seabass cultivation.
Over the past decade, feed costs have risen, leading farmers to adopt formulated feed to combat this trend. The FCR significantly impacts total feed costs (Bai et al., 2022). Initially, FCRs were high with fish meal feed, escalating production costs, as shown in Figure 1. This elevated FCR had a direct impact on production costs, causing a noticeable escalation in profits. It is important to note that these findings are based on data derived from institutional demonstration experiences. However, by the end of the decade, the switch to formulated feed led to reduced FCR and overall production costs. Formulated feed has proven to be superior to fish meal, with an increased focus on alternative protein sources (Arru et al., 2019; Jannathulla et al., 2019; Hodar et al., 2020). The lower FCRs with formulated feeds are due to species-specific diet formulations with the required protein content. In light of climate change, replacing fishmeal protein is crucial for cost-effective feeds. Skretting, a multinational company, has been working on the formulation and manufacturing of a feed that does not include fishmeal (Fishmeal-Free Breakthrough for Skretting, 2023). Growel Feeds Ltd., Apex Frozen Foods Ltd., Avanti Feeds Ltd., Coastal Corporation Limited, and Adinath Bio Labs are some of the leading Indian aquafeed companies with environmental agenda and innovative approaches to develop cost-effective species-specific fish feeds (INDmoney, 2024).
Another significant factor that contributes an average of 17% to the overall production cost of seabass farming in cages is the cost of the seeds. Seed quality and quantity are regularly central concerns in the growth of the marine aquaculture enterprise. Ravisankar and Thirunavukkarasu (2010) recorded the seabass seed price to be US $0.12 for each piece for the year 2010, contributing 10.67% to the total production cost. However, at present, the market prices are US $0.37–0.49 (Vimala et al., 2021). Concerning this, there has not been a lot of research conducted on the economic viability of hatcheries and seed rearing in order to determine whether it would be possible for the activity to be expanded in terms of economics and business. According to Kumaran et al. (2021), the BCR was 2.76 and 1.9, respectively, while the IRR was 300 and 130%, suggesting the economic feasibility of nursery rearing under differing salinity regimes. In the present study, when the seed price increased by 30%, the project’s BCR decreased by 18%, and the break-even point increased to 9.2%. More dependency on natural seeds would be a risk in terms of input cost structure flux and sustainability. The results of these studies explicitly indicated that nursery rearing serves solely as a livelihood development activity for coastal fisher families, involving active engagement from coastal communities. Thus, mass-scale seed production is an imminent need for sector expansion. The establishment of finfish hatcheries to ensure a steady supply of seabass seeds is a critical aspect in supporting the widespread acceptance of nursery rearing as a sustainable sea cage farming activity.
The free cash flow demonstrated an increase from year one, eventually reaching a peak during year eight, and is expected to follow a similiar trend throughout years 9 and 10 of the farming activity, according to the cash flow study. By analyzing offshore rock bream culture in Korea, Lipton and Kim (2007) proposed a remunerative production method with a positive NPV of US $3,151,402 for a 10-year cash flow and an 8% discount rate. The IRR was calculated to be 29.7% for a single year and 34.3% for a cumulative 10 years. Here, we take into consideration the projected value of cash flows along with the time value of money. The results of sensitivity analysis showed that net profits are sensitive to increases in feed and seed prices. Therefore, if seed costs rise by 30%, the project net profits fall by 13.1%, whereas if feed prices rise by 30%, the project net profits rise by 17.8%. It showed that a lowered selling price with an FCR of 1.5 would make both production processes commercially feasible (de Bezerra et al., 2016). The results of sensitivity analysis in two types of aquaculture systems (offshore and inshore) in Italy divulged that offshore farming practices fetched greater commercial feasibility than coastal farming practices by varying input and production costs in combination with optimized FCR and stocking density. Inshore aquaculture systems were found to have lower economic viability than offshore aquaculture systems. This showed that prudent market selection decisions and effective farm business strategies would aid in enhancing the venture’s lucrative valuation and cashflow.
India is currently adopting a planned approach to mariculture development. Divu et al. (2021), Divu et al. (2023b), and Divu et al. (2023a) have developed a mapping model that assesses various factors including environmental, socio-economic, infrastructural, and regulatory aspects to determine the suitability of sites for mariculture. This approach ensures logical and practical developmental activities, promoting sustainable and efficient mariculture practices. Due to the increasing demand for seabass in the global marketplace, cage farming of this species is a confirmed lucrative enterprise, and this trend may continue, as predicted by several reports with a rising CAGR at above 5%. Furthermore, the market value of sole seabass was projected to reach US $960 million in 2023 and is expected to reach US $1,492 million by 2033 (Future Market Insights, 2021). This substantial demand opens the opportunities to tap the forex earnings and also catalyzes the expansion of seabass farming, and the most deserved farming system would be in sea cages due to its high production possibilities. However, there is an impending threat to the availability of feed and seeds as a result of climate change-induced reductions in raw material availability and rising prices (Jannathulla et al., 2019; Maulu et al., 2021), which is the greatest obstacle to mariculture enterprises and other stakeholders. Climate change can create rapid alterations in marine environments and the dynamics of marine organisms. It can elevate seawater temperatures, alter hydrological patterns, cause rare and extreme oceanic events, and reduce marine fish populations (Yatsu et al., 2005; Lloret et al., 2008; Knutson et al., 2010). Additionally, variations in ocean conditions such as ocean currents and reduction in coastal upwelling also adversely affect marine productivity (IPCC, 2007). The global sea surface temperature (SST) has risen by 0.7°C over recent decades but in the tropical Indian Ocean, this increase is more pronounced at 1.0°C. The northern Arabian Sea has shown the most accelerated warming, with a rate of 0.18°C per decade from 1976 to 2005 (Roxy et al., 2020; Krishnan et al., 2020). Climate change has also affected species richness within marine assemblages by altering their habitat quality (Wilson et al., 2008). In Asian seabass aquaculture, climate change poses a significant challenge by impacting both productivity and economic sustainability. For example, most fishmeal plants are located on the Southern coast of India where a traditionally high abundance of low-cost fishmeal species is available. Indian oil sardine is extensively utilized in the preparation of fish meal for aquaculture feed (Alder et al., 2008; Barange et al., 2014) in the southern regions of India (Ponnusamy et al., 2012). Investigations into the climatic impacts on the Indian Ocean fishery have indicated a latitudinal extension of Indian oil sardine populations toward the Northern Arabian Sea habitats. This shift is attributed to the increase in SST in the southern region and the resulting changes in oceanic circulation patterns (Vivekanandan et al., 2009; BoBP, Chennai, 2008; Bharti et al., 2019). Consequently, the availability of species for fishmeal production in the southern region of India has been diminishing, leading to an increase in costs.
Therefore, there is a need to build resilience and sustain the production system in a changing climate, and the aquaculture producers must adapt to the available options in the short term while mitigating the effects by making necessary adjustments in their production practices in the long term, which is being recommended as climate change mitigation strategy by the FAO (Barange et al., 2018). Considering the significance of feed and seed variables to the aquaculture enterprise and the fact that it is highly vulnerable to the effects of climate change, the operational production costs are likely to rise. Therefore, the performed economic analysis assists the entrepreneur/stakeholder’s investment in addressing the key attributes where the farmer is expected to optimize the input cost, investigate the risk profile of uptaken components, and develop mitigation measures while venturing into seabass cage farming in open seas. Suitable species for cage farming can be selected based on market demand, location suitability, rapid growth, and availability of specialized feed, seed, and nutritional requirements (Rao et al., 2013). Moreover, India is emphasizing the expansion of mariculture, the promotion of sea cage farming, and the doubling of fish production (Pradhan Mantri Matsya Sampada Yojana, 2020). Therefore, in the context of doubling seabass production, feed and seed are the primary market segments where this technological disparity exists. Hence, we recommend cost-effective species-specific fish feed formulations and climate-resilient hatcheries development with the help of the present investigation of the vast market potential of Asian seabass. Moreover, it is proposed that policymakers and entrepreneurs undertake bigger dimension production models at industrial scales in the Indian context with seasonal resource assimilation in order to tap into the full extent of the seabass market. This is crucial for the mariculture sector’s long-term viability, given the expected challenges such as increased feed and seed costs, heightened climate vulnerability, and economic implications.
5 Conclusion
This research has shed light on the challenges posed by the vulnerability of feed and seed, the escalation in the market cost, and the factors that impact the economics of farming ventures in the mariculture sector. It is anticipated that the impact of climate change is directly or indirectly affecting the profitability of the aquaculture ventures discussed in this article, most importantly in terms of the feed cost. Therefore, there is an urgent need for an economic analysis of sea cage farming for economically important finfish species. A comprehensive fiscal evaluation that is capable of investigating the productivity of farming Asian Seabass (L. calcarifer) in cage farming systems over the course of 10 years has been carried out. This research in an Indian context culminated in the development of a baseline economic study that offers critical output insights into making efficient use of significant resources such as feed and seed, along with other input expenses. Farmers and stakeholders will be able to make better input cost decisions as a result of this, which will allow them to circumvent the challenges of an increased input cost structure stemming from the feed and seed aspect of the marine cage operation. With the enormous flow of funds from various Government schemes such as “Pradhan Mantri Matsya Sampada Yojana” as well as the recommendations for geospatial planning for earmarking critical mariculture zones along the Indian coastline (Divu et al., 2021), the wagers for the expansion of offshore cage farming of Asian seabass in Indian waters is imminent. We emphasize that the economic aspect of sustainability must go hand in hand with the environmental one, which is a necessary prerequisite in compliance with Goal 14 of the Sustainable Development Goals for 2030. Therefore, additional research in this field is required to improve knowledge on this topic in order to better respond to future challenges, support the aquaculture sector, and assist policymakers in making wise and prudent decisions. With the newfound knowledge, we will be able to assist stakeholders in making more informed decisions, and the government will be in a better position to subsidize aquafeed enterprises in the development of cost-effective species-specific fish food formulations.
We propose several recommendations for addressing climate change impacts on feed and seed vulnerability, along with risk mitigation strategies for seabass mariculture:
1. To bolster the resilience of the aquafeed sector, climate economics and technological innovations should be integrated to formulate cost-effective feeds that substitute for fishmeal. This approach, coupled with technological development, will enable stakeholders to better prepare for and adapt to future climate change.
2. In light of the declining supply of fishmeal and the growing demand for it, the use of alternate protein sources should be explored for the use of cost-effective feed formulation, indicating the sector’s resilience for fishmeal replacement to a certain level. Future research should evaluate the economic effects more robustly on the aquaculture cost structure of using other eco-friendly fish feed (e.g., insect species and poultry meal) for seabass production and other fishes, evaluating the impacts of accounting for different FCRs.
3. The economic feasibility of farming seabass may easily be equivalent to that of farming shrimp provided new technological interventions can minimize the cost of feed and seed as well as the duration needed for pond rearing. The problem of an inadequate supply of seeds for stocking may be circumvented by the expansion of existing hatcheries and nurseries into more sophisticated facilities. In addition, filleting, convenience processing, and live fish marketing have the potential to provide Asian seabass farming in India the boast needed for rapid expansion.
Data availability statement
The original contributions presented in the study are included in the article/Supplementary material, further inquiries can be directed to the corresponding author.
Author contributions
DD: Conceptualization, Methodology, Investigation, Project administration, Supervision, Writing – original draft, Writing – review & editing, Data curation, Funding acquisition, Resources. SM: Conceptualization, Investigation, Methodology, Project administration, Supervision, Writing – original draft, Writing – review & editing, Formal analysis, Software, Validation, Visualization. PS: Data curation, Formal analysis, Software, Validation, Writing – original draft, Writing – review & editing. SL: Formal analysis, Writing – original draft, Writing – review & editing. MM: Resources, Writing – original draft, Writing – review & editing. GG: Resources, Writing – original draft, Writing – review & editing. MT: Data curation, Formal analysis, Methodology, Resources, Validation, Writing – review & editing. RM: Formal analysis, Methodology, Software, Writing – review & editing. KR: Data curation, Investigation, Resources, Validation, Writing – review & editing. VV: Resources, Writing – original draft, Writing – review & editing. JS: Writing – review & editing. AS: Writing – review & editing. BI: Resources, Writing – original draft, Writing – review & editing. SR: Project administration, Resources, Writing – review & editing. AG: Funding acquisition, Project administration, Resources, Writing – review & editing.
Funding
The author(s) declare that financial support was received for the research, authorship, and/or publication of this article. The authors gratefully acknowledged the financial support obtained from the Indian Council of Agriculture Research (ICAR) through the National Initiative on Climate Resilient Agriculture (NICRA) and the All India Network Project on Mariculture (AINP-M). This research was supported by the Indian Council of Agricultural Research (ICAR), New Delhi, Government of India, and we duly acknowledge their funding support.
Acknowledgments
We express our sincere thanks to the Director, ICAR-Central Marine Fisheries Research Institute (ICAR-CMFRI), Coordinator, All India Network Project on Mariculture (AINP-M), and National Initiative on Climate Resilient Agriculture (NICRA), former and present Head-In-Charge of Mariculture Division of the institute, for their constant support and necessary facilitation. We duly appreciate and acknowledge the support rendered by the staff of Veraval Regional Station who have been involved and contributed to this study.
Conflict of interest
The authors declare that the research was conducted in the absence of any commercial or financial relationships that could be construed as a potential conflict of interest.
Generative AI statement
The authors declare that Generative AI was used in the creation of this manuscript. During the preparation of this work, the author(s) used AI-assisted technologies to improve readability and language of the manuscript during the writing process. After using this tool/service, the author(s) reviewed and edited the content as needed and take(s) full responsibility for the content of the publication.
Publisher’s note
All claims expressed in this article are solely those of the authors and do not necessarily represent those of their affiliated organizations, or those of the publisher, the editors and the reviewers. Any product that may be evaluated in this article, or claim that may be made by its manufacturer, is not guaranteed or endorsed by the publisher.
Supplementary material
The Supplementary material for this article can be found online at: https://www.frontiersin.org/articles/10.3389/fsufs.2024.1352131/full#supplementary-material
References
Adwan, O. M. (2017). Analyzing fish farming system in the Jordan valley comparative study. J. Soc. Sci. 6, 827–832. doi: 10.25255/jss.2017.6.4.827-832
Alder, J., Campbell, B., Karpouzi, V., Kaschner, K., and Pauly, D. (2008). Forage fish: from ecosystems to markets. Annu. Rev. Environ. Resour. 33, 153–166. doi: 10.1146/annurev.environ.33.020807.143204
Allen, G. (2004). Fish, aquaculture and food security: sustaining fish as food supply, in Livestock production/industries resource/energy economics and policy. Australia. Available at: https://ageconsearch.umn.edu/record/124068/?v=pdf (Accessed 19 May 2024).
Animal Feed Protein Market Share Statistics Report 2023-2032. (2024). Global market insights Inc. Available at: https://www.gminsights.com/industry-analysis/global-protein-market-for-animal-feed-application (Accessed April 13, 2023).
Arasu, A. R. T., Kailasam, M., Sundaray, J. K., Subburaj, R., Thiagarajan, G., and Karaiyan, K., (2008). Improved hatchery technology for Asian seabass Lates calcarifer (Bloch).
Arru, B., Furesi, R., Gasco, L., Madau, F. A., and Pulina, P. (2019). The introduction of insect meal into fish diet: the first economic analysis on European sea bass farming. Sustain. For. 11:1697. doi: 10.3390/su11061697
Aswathy, N., and Imelda, J. (2018). Economic viability of cage farming of Asian seabass in the coastal waters of Kerala. Int. J. Fish. Aquat. Stud. 6, 368–371.
Aswathy, N., and Imelda, J. (2020). Logit analysis of the factors affecting cage fish farming adoption decisions in the southwest coast of India. Curr. J. Appl. Sci. Technol. 39, 29–34. doi: 10.9734/cjast/2020/v39i4031109
Aswathy, N., Imelda, J., Ignatius, B., and Joseph, S. (2020). Economic viability of cage fish farming in India. Kochi: Central Marine Fisheries Research Institute (CMFRI) Special Publication, Central Marine Fisheries Research Institute. 36.
Bai, S. C., Hardy, R. W., and Hamidoghli, A. (2022). “Diet analysis and evaluation,” in Fish nutrition. eds. Hardy R. W. and Kaushik S. J. (San Diego, CA: Academic Press), 709–743.
Bakİ, B., and Yücel, S. (2017). Feed cost/production income analysis of seabass (Dicentrarchus labrax) aquaculture. Int. J. Ecosyst. Ecol. Sci. 7, 859–864.
Barange, M., Bahri, T., Beveridge, M. C., Cochrane, K. L., Funge-Smith, S., and Poulain, F. (2018). Impacts of climate change on fisheries and aquaculture. Unit. Nat. Food Agric. Organ. 12, 628–635.
Barange, M., Coetzee, J., Takasuka, A., Hill, K., Gutierrez, M., Oozeki, Y., et al. (2009). Habitat expansion and contraction in anchovy and sardine populations. Prog. Oceanogr. 83, 251–260. doi: 10.1016/j.pocean.2009.07.027
Barange, M., Merino, G., Blanchard, J. L., Scholtens, J., Harle, J., Allison, E. H., et al. (2014). Impacts of climate change on marine ecosystem production in societies dependent on fisheries. Nat. Clim. Chang. 4, 211–216. doi: 10.1038/nclimate2119
Baruah, K., Norouzitallab, P., and Pal, A. K. (2017). “Development of low cost and eco-friendly feed for various candidate species for the sustainability of commercial aquaculture and reduction of aquatic pollution,” in Developing new functional food and nutraceutical products. eds. D. Bagchi and S. Nair (US: Academic Press), 441–453.
Bharti, V., Jayasankar, J., Shukla, S. P., Varghese, E., Sathianandan, T. V., George, G., et al. (2019). Distribution of Indian oil sardine Sardinella longiceps along south-west coast of India. Indian J. Anim. Sci. 89, 912–917. doi: 10.56093/ijans.v89i8.93070
BoBP, Chennai (2008). Impact of climate change on Indian marine fisheries. Bay of Bengal News, 32–37.
Bunlipatanon, P., Songseechan, N., Kongkeo, H., Abery, N. W., and De Silva, S. S. (2014). Comparative efficacy of trash fish versus compounded commercial feeds in cage aquaculture of Asian seabass (Lates calcarifer) (Bloch) and tiger grouper (Epinephelus fuscoguttatus) (Forsskål). Aquac. Res. 45, 373–388. doi: 10.1111/j.1365-2109.2012.03234.x
Callaway, R., Shinn, A. P., Grenfell, S. E., Bron, J. E., Burnell, G., Cook, E. J., et al. (2012). Review of climate change impacts on marine aquaculture in the UK and Ireland. Aquat. Conserv. Mar. Freshwat. Ecosyst. 22, 389–421. doi: 10.1002/aqc.2247
Chang, Y., Lee, K. T., Lee, M. A., and Lan, K. W. (2009). Satellite observation on the exceptional intrusion of cold water in the Taiwan Strait. Terrestr. Atmospher. Ocean. Sci. 20:661. doi: 10.3319/TAO.2008.08.07.01(Oc)
Chang, Y., Lee, M. A., Lee, K. T., and Shao, K. T. (2013). Adaptation of fisheries and mariculture management to extreme oceanic environmental changes and climate variability in Taiwan. Mar. Policy 38, 476–482. doi: 10.1016/j.marpol.2012.08.002
Chavez, F. P., Ryan, J., Lluch-Cota, S. E., and Niquen, C. M. (2003). From anchovies to sardines and back: multidecadal change in the Pacific Ocean. Science 299, 217–221. doi: 10.1126/science.1075880
Connect2india (2024) Sea bass buyers, suppliers, importers, exporters and manufacturers - latest price and trends. Available at: https://connect2india.com/Sea-Bass#:~:text=The%20HS%20code%20for%20Sea,India%20is%20Rs%20600%2F20Kilogram (Accessed April 18, 2023).
Daet, I. (2019). “February. Study on culture of sea bass (Latescalcarifer, Bloch 1790) inhapa-in-pond environment” in IOP Conference Series: Earth and Environmental Science (IOP Publishing), 012115.
de Bezerra, T. R. Q., Domingues, E. C., Maia Filho, L. F. A., Rombenso, A. N., Hamilton, S., and Cavalli, R. O. (2016). Economic analysis of cobia (Rachycentron canadum) cage culture in large-and small-scale production systems in Brazil. Aquac. Int. 24, 609–622. doi: 10.1007/s10499-015-9951-2
de Jesus-Ayson, E. G., and Ayson, F. G. (2013). “Reproductive biology of the Asian seabass, Lates calcarifer,” in Biology and culture of Asian seabass Lates calcarifer. ed. D. R. Jerry (Boca Raton: CRC Press), 67–76.
Divu, D. N., Mojjada, S. K., Dash, G., Sundaram, S. L. P., Menon, M., Tade, M. S., et al. (2023a). A novel decision-support system for augmenting sustainable lobster production through comparison of mariculture systems at increasing stages of technological development. J. Clean. Prod. 401:136759. doi: 10.1016/j.jclepro.2023.136759
Divu, D., Mojjada, S. K., Muktha, M., Azeez, P. A., Tade, M. S., Subramanian, A., et al. (2023b). Mapping of potential sea-cage farming sites through spatial modelling: preliminary operative suggestions to aid sustainable mariculture expansion in India. Indian J. Fish. 70, 1–17. doi: 10.21077/ijf.2023.70.4.132866-01
Divu, D. N., Mojjada, S. K., Pokkathappada, A. A., Sukhdhane, K., Menon, M., Mojjada, R. K., et al. (2021). Decision-making framework for identifying best suitable mariculture sites along north east coast of Arabian Sea, India: a preliminary GIS-MCE based modelling approach. J. Clean. Prod. 284:124760. doi: 10.1016/j.jclepro.2020.124760
Edwards, P., Tuan, L. A., and Allan, G. L. (2004). A survey of marine trash fish and fish meal as aquaculture feed ingredients in Vietnam (no. 437-2016-33834).
FAO (2023). Lates calcarifer, Cultured aquatic species information Programme. Text by Rimmer, M.A. Rome: Fisheries and Aquaculture Division. Available at: https://www.fao.org/fishery/en/culturedspecies/lates_calcarifer?lang=en (Accessed October 26, 2024).
Fatima, A., Singh, V. K., Babu, S., Singh, R. K., Upadhyay, P. K., Rathore, S. S., et al. (2023). Food production potential and environmental sustainability of different integrated farming system models in Northwest India. Front. Sustain. Food Syst. 7:959464. doi: 10.3389/fsufs.2023.959464
Fish Base (2023). Global fish meal market, size, forecast 2022-2027, industry trends, growth, impact of covid-19, opportunity company analysis, research and markets - market research reports - welcome. Available at: https://www.researchandmarkets.com/reports/5546200/global-fish-meal-market-size-forecast2022?utm_source=GNOM&utm_medium=PressRelease&utm_code=6fsm3m&utm_campaign=1740482%2B%2BGlobal%2BFish%2BMeal%2BMarket%2BReport%2B2022%3A%2BIndustry%2Bto%2BGrow%2Bat%2B4.1%25%2BAnnually%2BThrough%2B2027&utm_exec=como322prd (Accessed: April 12, 2023).
Fish Meal Market: Global Industry Trends, Share, Size, Growth, Opportunity and Forecast 2022-2027 (2024). Size, share, growth and forecast 2022-2027. Available at: https://www.imarcgroup.com/fish-meal-market (Accessed November 16, 2022).
Fishmeal-Free Breakthrough for Skretting (2023) Skretting. Available at: https://www.skretting.com/en/news-and-stories/fishmeal-free-breakthrough-for-skretting/ (Accessed April 12, 2023).
Funge-Smith, S., Lindebo, E., and Staples, D. (2005). Asian fisheries today: the production and use of low value/trash fish from marine fisheries in the Asia-Pacific region. Food and Agriculture Organization of the United Nations Regional Office for Asia and the Pacific, Bangkok. Rap Publication 2005/16. Asia-Pacific Fishery Commission. Available at: http://www.fao.org/3/a-ae934e.pdf
Future Market Insights (2021). Asian Sea bass market by source, form and region for 2021 – 2031 | global sales analysis and opportunity - 2031 FMI. Available at: https://www.futuremarketinsights.com/reports/sea-bass-market (Accessed April 17, 2023).
Hassan, H. U., Ali, Q. M., Khan, W., Masood, Z., Abdel-Aziz, M. A., Shah, M. I. A., et al. (2021). Effect of feeding frequency as a rearing system on biological performance, survival, body chemical composition, and economic efficiency of Asian seabass Lates calcarifer (Bloch, 1790) reared under controlled environmental conditions. Saudi J. Biol. Sci. 28, 7360–7366. doi: 10.1016/j.sjbs.2021.08.031
Hodar, A. R., Vasava, R. J., Mahavadiya, D. R., and Joshi, N. H. (2020). Fish meal and fish oil replacement for aqua feed formulation by using alternative sources: a review. J. Exp. Zool. India 23, 13–21.
Hsieh, H. J., Hsien, Y. L., Jeng, M. S., Tsai, W. S., Su, W. C., and Chen, C. A. (2008). Tropical fishes killed by the cold. Coral Reefs 27:599. doi: 10.1007/s00338-008-0378-3
Hurtado, A. Q., Agbayani, R. F., Sanares, R., and de Castro-Mallare, M. T. R. (2001). The seasonality and economic feasibility of cultivating Kappaphycus alvarezii in Panagatan cays, Caluya, antique. Aquaculture 199, 295–310. doi: 10.1016/S0044-8486(00)00553-6
Idris, S. M., Noordin, W. N. M., Manah, F. O., and Hamzah, A. (2022). Toward systematic breeding of Asian Sea bass, Lates calcarifer (Bloch, 1790), in Malaysia: status, challenges and prospects for future development. Asian Fish. Sci. 35, 1–12. doi: 10.33997/j.afs.2022.35.1.001
IFFO Reports. IFFO reports lower fishmeal production in the first seven months of 2022 (2024). Available at: https://www.aquafeed.com/newsroom/reports/iffo-reports-lower-fishmeal-production-in-the-first-seven-months-of-2022/ (Accessed: April 13, 2023).
INDmoney. Aquaculture companies in India: is their niche market a boon or a bane? INDmoney. (2024). Available at: https://www.indmoney.com/articles/stocks/listed-aquaculture-companies-in-India (Accessed: April 17, 2023).
IPCC. (2007). Climate change 2007: impacts, adaptation and vulnerability. Contribution of working group II to the fourth assessment report of the intergovernmental panel on climate change, M. L. Parry, O. F. Canziani, J. P. Palutikof, P. J. Linden van der, and C. E. Hanson, Eds., Cambridge University Press, Cambridge
Jannathulla, R., Rajaram, V., Kalanjiam, R., Ambasankar, K., Muralidhar, M., and Dayal, J. S. (2019). Fishmeal availability in the scenarios of climate change: inevitability of fishmeal replacement in aquafeeds and approaches for the utilization of plant protein sources. Aquac. Res. 50, 3493–3506. doi: 10.1111/are.14324
Joerakate, W., Yenmak, S., Senanan, W., Tunkijjanukij, S., Koonawootrittriron, S., and Poompuang, S. (2018). Growth performance and genetic diversity in four strains of Asian sea bass, Lates calcarifer (Bloch, 1790) cultivated in Thailand. Agric. Nat. Resour. 52, 93–98. doi: 10.1016/j.anres.2018.05.015
Khang, P. V., Phuong, T. H., Dat, N. K., Knibb, W., and Nguyen, N. H. (2018). An 8-year breeding program for Asian seabass Lates calcarifer: Genetic evaluation, experiences, and challenges. Front. Genet. 9:191. doi: 10.3389/fgene.2018.00191
Kandan, S. (2009). “Commercial seed production and farming of Asian seabass Lates calcarifer,” in Course manual: Winter school on recent advances in breeding and larviculture of Marine finfish and shellfish. eds. K. Madhu and R. Madhu (Kochi, India: ICAR-Central Marine Fisheries Research Institute). Available at: https://eprints.cmfri.org.in/5332/1/9.pdf
Knutson, T. R., McBride, J. L., Chan, J., Emanuel, K., Holland, G., Landsea, C., et al. (2010). Tropical cyclones and climate change. Nat. Geosci. 3, 157–163. doi: 10.1038/ngeo779
Krishnan, R., Gnanaseelan, C., Sanjay, J., Swapna, P., Dhara, C., Sabin, T. P., et al. (2020). “Introduction to climate change over the Indian region,” in Assessment of climate change over the Indian region. eds. D. Krishnan, J. Sanjay, C. Gnanaseelan, M. Mujumdar, A. Kulkarni, and S. Chakraborty (Springer: Nature Singapore Pte Ltd).
Kumaran, M., Geetha, R., Antony, J., Vasagam, K. K., Anand, P. R., Ravisankar, T., et al. (2021). Prospective impact of Corona virus disease (COVID-19) related lockdown on shrimp aquaculture sector in India–a sectoral assessment. Aquaculture 531:735922. doi: 10.1016/j.aquaculture.2020.735922
Lipton, D. W., and Kim, D. H. (2007). Assessing the economic viability of offshore aquaculture in Korea: an evaluation based on rock bream, Oplegnathus fasciatus, production. J. World Aquacult. Soc. 38, 506–515. doi: 10.1111/j.1749-7345.2007.00124.x
Liu, P., Xia, J. H., Sun, F., Wang, L., Yang, Z., Lee, M., et al. (2024). Breeding Asian seabass to increase survival against big belly disease and growth. Aquac. 9, 731–738. doi: 10.1016/j.aaf.2022.08.004
Lloret, J., Marín, A., and Marín-Guirao, L. (2008). Is coastal lagoon eutrophication likely to be aggravated by global climate change? Estuar. Coast. Shelf Sci. 78, 403–412. doi: 10.1016/j.ecss.2008.01.003
Maulu, S., Hasimuna, O. J., Haambiya, L. H., Monde, C., Musuka, C. G., Makorwa, T. H., et al. (2021). Climate change effects on aquaculture production: sustainability implications, mitigation, and adaptations. Front. Sustain. Food Syst. 5:609097. doi: 10.3389/fsufs.2021.609097
Mohamed, G., Rao, G. S., and Ghosh, S. (2010). Aquaculture of spiny lobsters in sea cages in Gujarat, India. J. Mar. Biol. Assoc. India 52, 316–319.
Mojjada, S. K. (2015). Studies on technological biological innovations in Open Sea cage farming. PhD Thesis. Mangalore, India: Mangalore University.
Mojjada, S. K., Divu, D. N., Sudhakaran, P. O., Sundaram, S. L. P., Menon, M., Mojjada, R. K., et al. (2023). An integrated techno-economic decision-support fiscal forecast model for sea cage mariculture enterprises for Asian seabass production in Indian territorial waters. Aquaculture 580:740351. doi: 10.1016/j.aquaculture.2023.740351
Mojjada, S. K., Imelda, J., Koya, M., Sreenath, K. R., Dash, G., Dash, S. S., et al. (2012a). Capture based aquaculture of mud spiny lobster, Panulirus polyphagus (Herbst, 1793) in open sea floating net cages off Veraval, north-west coast of India. Indian J. Fish. 59, 29–33.
Mojjada, S. K., Imelda, J., Maheswarudu, G., Ranjan, R., Dash, B., Ghosh, S., et al. (2012b). Open Sea mariculture of Asian seabass Lates calcarifer (Bloch, 1790) in marine floating cage at Balasore, Odisha, north-east coast of India. Indian J. Fish. 59, 89–93.
Mojjada, S. K., Imelda, J., Rao, P. S., Mukherjee, C. K., Ghosh, S., and Rao, G. S. (2013). Design, development and construction of open sea floating cage device for breeding and farming marine fish in Indian waters. Indian J. Fish. 60, 61–65.
MPEDA. MPEDA press note export performance 2022-23, 25 May 22, v2 1. (2022). Available at: https://mpeda.gov.in/wp-content/uploads/2023/06/Marine-products-export-performance-2022-23-english.pdf (Accessed December 6, 2023).
Neori, A., Chopin, T., Troell, M., Buschmann, A. H., Kraemer, G. P., Halling, C., et al. (2004). Integrated aquaculture: rationale, evolution and state of the art emphasizing seaweed biofiltration in modern mariculture. Aquaculture 231, 361–391. doi: 10.1016/j.aquaculture.2003.11.015
Olagunju, F. I., Adesiyan, I. O., and Ezekiel, A. A. (2007). Economic viability of cat fish production in Oyo state, Nigeria. J. Hum. Ecol. 21, 121–124. doi: 10.1080/09709274.2007.11905961
Olsen, R. L., and Hasan, M. R. (2012). A limited supply of fishmeal: impact on future increases in global aquaculture production. Trends Food Sci. Technol. 27, 120–128. doi: 10.1016/j.tifs.2012.06.003
Parappurathu, S., Menon, M., Jeeva, C., Belevendran, J., Anirudhan, A., Lekshmi, P. S., et al. (2023). Sustainable intensification of small-scale mariculture systems: farm-level insights from the coastal regions of India. Front. Sustain. Food Syst. 7:1078314. doi: 10.3389/fsufs.2023.1078314
Parsons, L. S., and Lear, W. H. (2001). Climate variability and marine ecosystem impacts: a North Atlantic perspective. Prog. Oceanogr. 49, 167–188. doi: 10.1016/S0079-6611(01)00021-0
Pham, K. V. (2019). Improving seed production and genetic improvement of Asian seabass Lates calcarifer (Doctoral dissertation,: University of the Sunshine Coast.
Philipose, K. K. (2013). “Development of innovative low-cost cages for promoting open sea cage culture along the Indian coast,” in Central Marine Fisheries Research Institute (CMFRI) Publication. Karwar, Karnataka, India: Karwar Research Centre of CMFRI. Available at: http://eprints.cmfri.org.in/id/eprint/9717
Philipose, K. K., Sharma, S. R., Loka, J., Vaidya, N. G., Divu, D., Sadhu, N., et al. (2013). Culture of Asian seabass (Lates calcarifer, Bloch) in open sea floating net cages off Karwar, South India. Indian J. Fish. 60, 67–70.
Pike, I. H., and Barlow, S. M. (2003). Impact of fish farming on fish stocks. Fish Farmer 26, 14–16.
Ponnusamy, K., Ambasankar, K., and Ponniah, A. G. (2012). Production and marketing of fish meal in India-a study. Indian J. Fish. 59, 147–149.
Pradhan Mantri Matsya Sampada Yojana (2020) Pradhan Mantri Matsya Sampada Yojana | Department of Fisheries, MoCIT, GoI. Available at: http://pmmsy.dof.gov.in/ (Accessed April 12, 2023).
Rana, K. J., Siriwardena, S., and Hasan, M. R. (2009). Impact of rising feed ingredient prices on aquafeeds and aquaculture production (no. 541) : Food and Agriculture Organization of the United Nations (FAO).
Rao, G. S., George, R. M., Anil, M. K., Saleela, K. N., Jasmine, S., Kingsly, H. J., et al. (2010). Cage culture of the spiny lobster Panulirus homarus (Linnaeus) at Vizhinjam, Trivandrum along the south-west coast of India. Indian J. Fish. 57, 23–29.
Rao, G. S., Imelda, J., Philipose, K. K., and Mojjada, S. K. (2013). Cage aquaculture in India : Central Marine Fisheries Research Institute.
Ravisankar, T., and Thirunavukkarasu, A. R. (2010). Market prospects of farmed Asian seabass Lates calcarifer (Bloch). Indian J Fish 57, 49–53.
Rosa, R., Marques, A., and Nunes, M. L. (2012). Impact of climate change in Mediterranean aquaculture. Rev. Aquac. 4, 163–177. doi: 10.1111/j.1753-5131.2012.01071.x
Roxy, M. K., Gnanaseelan, C., Parekh, A., Chowdary, J. S., Singh, S., Modi, A., et al. (2020). “Indian ocean warming,” in Assessment of climate change over the Indian region. eds. D. Krishnan, J. Sanjay, C. Gnanaseelan, M. Mujumdar, A. Kulkarni, and S. Chakraborty (Springer: Nature Singapore Pte Ltd).
Senanan, W., Pechsiri, J., Sonkaew, S., Na-Nakorn, U., Sean-In, N., and Yashiro, R. (2015). Genetic relatedness and differentiation of hatchery populations of Asian seabass (Lates calcarifer) broodstock in Thailand inferred from microsatellite genetic markers. Aquac. Res. 46, 2897–2912. doi: 10.1111/are.12442
Schmittner, A., Saenko, O. A., and Weaver, A. J. (2003). Coupling of the hemispheres in observations and simulations of glacial climate change. Quat. Sci. Rev. 22, 659–671. doi: 10.1016/S0277-3791(02)00184-1
Sea Bass Wholesalers and Wholesale Dealers in India (2024). IndiaMART. Available at: https://dir.indiamart.com/impcat/sea-bass.html?biz=30 (Accessed: April 17, 2023).
Shah, S. B. H., Yongtong, M., Narejo, N. T., Jarwarc, A., Oadd, S., Alia, M., et al. (2020). Economic analysis of barramundi (Lates calcarifer) (Bloch 1790), in Pakistan and opportunities for its aquaculture development. Indian J. GeoMar. Sci. 49, 1425–1434.
Shingare, P. E., Pagarkar, A. U., Chaudhari, K. J., Dhaker, H. S., Meshram, S. J., Sawant, N. H., et al. (2020). Eco-friendly and sustainable Asian seabass culture system: an alternate candidate species other than shrimp for brackish water aquaculture. J. Exp. Zool. 23, 983–985.
Soliman, I., and Gaber, M. (1988). An economic study of existing aquaculture systems in Egypt. In Proceedings of the 13th International Congress for Statistics, Computer Science, Social and Demographic Research (pp. 26–31).
Sorphea, S., Terai, A., Sreyrum, P., Lundh, T., Barnes, A. C., Da, C. T., et al. (2019). Growth performance of fry and fingerling Asian seabass Lates calcarife from Cambodian brood stock reared at different salinities. Livestock Res. Rural Dev. 31, 1–8.
Suloma, A., and Ogata, H. Y. (2006). Future of rice-fish culture, desert aquaculture and feed development in Africa: the case of Egypt as the leading country in Africa. Jpn Agric. Res. Q. 40, 351–360. doi: 10.6090/jarq.40.351
Swathilekshmi, P. S., Narayanakumar, R., and Ramachandran, C. (2018). A study on the socio-economic status and level of cognitive, psychomotor and affective domains of Sidi tribes in Open Sea cage culture. Indian J. Ext. Educ. 54, 195–200.
Takasuka, A., Oozeki, Y., and Aoki, I. (2007). Optimal growth temperature hypothesis: why do anchovy flourish and sardine collapse or vice versa under the same ocean regime? Can. J. Fish. Aquat. Sci. 64, 768–776. doi: 10.1139/f07-052
Thirunavukkarasu, A. R., Kailasam, M., and Sundaray, J. K. (2009). “Success in hatchery development of seabass and its potential for commercial cage culture in India,” in Course manual: National training on cage culture of seabass. Kochi: ICAR- Central Marine Fisheries Research Institute (CMFRI) & Hyderabad, India: National Fisheries Development Board (NFDB), 71–80. Available at: https://eprints.cmfri.org.in/6078/1/13.Thi.pdf
Tripathi, A., Tripathi, D. K., Chauhan, D. K., Kumar, N., and Singh, G. S. (2016). Paradigms of climate change impacts on some major food sources of the world: a review on current knowledge and future prospects. Agric. Ecosyst. Environ. 216, 356–373. doi: 10.1016/j.agee.2015.09.034
Van Huis, A. (2013). Potential of insects as food and feed in assuring food security. Annu. Rev. Entomol. 58, 563–583. doi: 10.1146/annurev-ento-120811-153704
Vijayakumaran, M., Venkatesan, R., Murugan, T. S., Kumar, T. S., Jha, D. K., Remany, M. C., et al. (2009). Farming of spiny lobsters in sea cages in India. N. Z. J. Mar. Freshw. Res. 43, 623–634. doi: 10.1080/00288330909510028
Vimala, D. D., Kailasam, M., Ambasankar, K., Kumaran, M., Vasagam, K. K., Ravisankar, T., et al. (2021). Frequently asked questions on Asian seabass (Lates calcarifer) seed production and farming. Chennai: ICAR-Central Institute of Brackishwater Aquaculture (CIBA) Special Publication, 87.
Vipinkumar, V. P., Subramanniyan, S., Aswani, N., Vikas, P. A., Sreeletha, P., Athira, P. V., et al. (2021). Cage farming of fish-a success story of SHGs. Mar. Fish. Inf. Serv. Tech. Ext. Ser. 250, 28–29.
Vivekanandan, E., Rajagopalan, M., and Pillai, N. G. K. (2009). “Recent trends in sea surface temperature and its impact on oil sardine” in Impact, adaptation and vulnerability of Indian agriculture to climate change. ed. P. K. Aggarwal (Indian Council of Agricultural Research: New Delhi).
Wilson, S. K., Burgess, S. C., Cheal, A. J., Emslie, M., Fisher, R., Miller, I., et al. (2008). Habitat utilization by coral reef fish: implications for specialists vs. generalists in a changing environment. J. Anim. Ecol. 77, 220–228.
Yatsu, A., Watanabe, T., Ishida, M., Sugisaki, H., and Jacobson, L. D. (2005). Environmental effects on recruitment and productivity of Japanese sardine Sardinops melanostictus and chub mackerel Scomber japonicus with recommendations for management. Fish. Oceanogr. 14, 263–278. doi: 10.1111/j.1365-2419.2005.00335.x
Ye, W., Sun, H., Li, Y., Zhang, J., Zhang, M., Gao, Z., et al. (2022). Greenhouse gas emissions from fed mollusk mariculture: a case study of a Sinonovacula constricta farming system. Agric. Ecosyst. Environ. 336:108029. doi: 10.1016/j.agee.2022.108029
Keywords: mariculture, Asian seabass, Opex, economic analysis, climate change, sustainability, production costs
Citation: Divu DN, Mojjada SK, Sudhakaran PO, Sundaram SLP, Menon M, George G, Tade MS, Mojjada RK, Radhakrishnan K, Vishwambharan VS, Shree J, Subramanian A, Ignatius B, Raghavan SV and Gopalakrishnan A (2024) Implications of feed and seed costs in Asian seabass mariculture in the face of climate change. Front. Sustain. Food Syst. 8:1352131. doi: 10.3389/fsufs.2024.1352131
Edited by:
Sanzidur Rahman, University of Reading, United KingdomReviewed by:
Franklin Isaac Ormaza-González, ESPOL Polytechnic University, EcuadorJosé Alberto Zepeda Domínguez, Autonomous University of Baja California, Mexico
Copyright © 2024 Divu, Mojjada, Sudhakaran, Sundaram, Menon, George, Tade, Mojjada, Radhakrishnan, Vishwambharan, Shree, Subramanian, Ignatius, Raghavan and Gopalakrishnan. This is an open-access article distributed under the terms of the Creative Commons Attribution License (CC BY). The use, distribution or reproduction in other forums is permitted, provided the original author(s) and the copyright owner(s) are credited and that the original publication in this journal is cited, in accordance with accepted academic practice. No use, distribution or reproduction is permitted which does not comply with these terms.
*Correspondence: Suresh Kumar Mojjada, c3VyZXNoa3VtYXIubWpkQGdtYWlsLmNvbQ==; c3VyZXNoLm1vamphZGFAaWNhci5nb3YuaW4=
†These authors have contributed equally to this work and share first authorship