- 1Water and Land Resource Centre (WLRC), Addis Ababa, Ethiopia
- 2School of Earth Sciences, College of Natural and Computational Sciences, Addis Ababa University, Addis Ababa, Ethiopia
- 3College of Development Studies, Addis Ababa University, Addis Ababa, Ethiopia
- 4ICRISAT · Resilient Farm and Food System – Landscapes Soil Fertility and Water Management, Addis Ababa, Ethiopia
- 5Department of Geography and Environmental Studies, College of Social Science, Addis Ababa University, Addis Ababa, Ethiopia
- 6Wondo Genet College of Forestry and Natural Resources, Hawassa University, Hawassa, Ethiopia
- 7School of Engineering, Centre of Water, Newcastle University, Newcastle upon Tyne, United Kingdom
Knowledge-based evidence about potential and existing rainfed cropping is crucial for decision-making for sustainable land use and food security. Using multi-criteria spatial analysis techniques, this study assessed the current status of cropland availability and projected impacts on future crop production in Ethiopia. The study primarily defined the extent of the Rainfed Cropping Area (RCA) and assessed the performances of different cropping practices. After precisely mapping cultivated area, cropping intensification potentials were estimated. Subsequently, disregarding the existing cultivated area, completely unsuitable areas, and protected and intact forest areas, the potentially available arable land using suitability analysis techniques was determined. In addition, the performance of existing crop production systems was evaluated against the natural potential. The findings reveal that RCA covers ~60% of the country’s landmass, of which cropping is practiced in only 33%. The coverage of Potentially Available Cropland (yet uncultivated) accounts for 16% of the country’s RCA. This is dominantly located in sparsely populated western and southwestern parts of the country. This study confirms that Horizontal Cropping Intensification (HCI) in the RCA of Ethiopia reaches only 33%. On the other hand, Vertical Cropping Intensification (VCI) practices cover only 10%, while about 1/3 of the RCA is suitable for VCI strategies at various levels of suitability. The performance of existing VCI-oriented cropping (which covers only 10% of the RCA) is very poor. Challenges to the use of the available cropland and ways of addressing land shortage for needy farmers are highlighted to inform efforts to readdress landlessness and food insecurity in Ethiopia.
1 Introduction
Agriculture in Ethiopia is age-old and renowned for its diverse farming landscapes (Hurni, 1985) and accommodates over 80% of 120 million people relying on rainfed systems (Abdollahzadeh et al., 2023; Asfaw et al., 2021). Some argue there’s no more land left to expand grain production, while others believe there are still uncharted and untapped landscapes, possibly larger than what’s currently used (Hurni, 1983). Considering polarized scientific views over rainfed farming, the authors sought to address four research gaps that could lead to invent strategies to unlock the RCA’s potential and address landlessness and food insecurity problems of Ethiopia’s smallholder farmers: (i) research focus, (ii) data and knowledge, (iii) methodology, and (iv) policy gaps. Previous research has exhaustively covered the challenges of rainfed farming to intensify food production faced by Ethiopia’s smallholder farmers, including land degradation, poor cropping methods, land fragmentation, low input use, traditional practices, and single-crop systems, resulting in low yields. With average landholdings below 1 hectare per household, food security for a family of 4–5 is unattainable without intensification strategies (Endalew et al., 2015). Forthcoming efforts to increase yields are further constrained by diminishing farm sizes (Hurni et al., 2015), climate change, and the land tenure system (Rahmato, 2003). Enhancing grain yields and resolving land scarcity is challenging due to high input costs, limited technology, and undulating terrain (Teshome, 2014). Additionally, limited knowledge, weak institutions, poor land use policies, and population pressure make rainfed agriculture an inefficient and unreliable farming system (Hurni, 1988). The majority of reports from such research pronounce recurrent socio-ecological crises like drought, famine, migration, and displacement are peculiar characteristics of rainfed systems (Taddese, 2001). Contrarily, a different scientific perspective like the book “Sufferings in God’s Environment” by McCann (1995) highlights Ethiopia’s RCA potential and argues that many Ethiopians suffer due to a lack of understanding of this potential. Another key study is the highland reclamation research from the early 1980 (Hurni, 1983), which comprehensively evaluated Ethiopia’s rainfed farming and reported that by applying existing traditional cropping practices, the RCA can support approximately 250 million people (Hurni, 1985). Indeed, while much research has addressed the challenges of rainfed agriculture, less attention has been given to its potential for improving food security and addressing landlessness (Lambin and Meyfroidt, 2011; Pretty, 1999; Nkwasa et al., 2023). As a result, biased research emphases have led to generate inconsistent facts about the opportunities and potentials of Ethiopia’s RCA. Given these scientific insights, this study targets a synoptic assessment of the potential and performance/efficiency of Ethiopia’s rainfed farming systems.
In developing countries like Ethiopia, a lack of inclusive studies has hindered the creation of reliable knowledge. Efforts to ensure food security have been weakened by the absence of evidence-based spatial information on suitable and usable extra land for crop production (Pretty, 1999; Lotze-Campen et al., 2010; Lambin, 2012; Wirsenius et al., 2010). Though data about natural capitals, like cultivated land size within Rainfed Cropping Area (RCA) and Potentially Available Cropland (PAC) are crucial, they are often lacking in the necessary scale and accuracy (Mandryk et al., 2015).
Data and knowledge gap about Ethiopia’s rainfed farming is partly due to definition limitations (agriculture or cropland/cultivated land). For instance, there is no agreed definition of Rainfed Agricultural Area (RAA) or RCA and highland region of the country. RAA is widely used to characterize varying types of agricultural practices that rely on rainfall (Wani et al., 2009). Since it includes diverse practices like forestry, livestock farming, and crop production, its meaning is always contextual. Under Ethiopia’s complex agricultural practices, some studies have used the definition and boundaries of highland, RAA, and RCA interchangeably. Therefore, such interchangeable use of terms has led to inconsistent estimates of agricultural or cultivated areas (Hurni et al., 2015). Moreover, the boundary and extents of RCA are not yet clearly defined spatially, nor updated and improved in a timely manner. Such methodological issues in research have led to growing doubts on the potentials and opportunities of Ethiopia’s RCA, which clearly justifies the third research gap authors have identified. Therefore, in the quest for cropping intensification (CI) strategies, as cropping factors vary over time and space, not only properly delimiting the RCA but also regularly updating both definitions and boundaries as per the context is essential. In defining RCA, mapping the potential, suitability, and availability of landscapes for crop production, and characterizing the spatial distributions of croplands across the various altitudinal gradients and agro-climatic zones is crucial (Hurni, 1998). In delimiting the spatial boundary of RCA, theoretically, altitude, which controls precipitation and temperature, is the most important indicator (Hurni, 1998). Factors such as soil type and slope are also important to further classify and elaborate the level of suitability of the landscape for specific cropping.
When aiming to provide policy evidence to unlock rainfed-based intensification potentials of Ethiopia, local-scale comprehensive research and primary data covering the whole RCA are essential, though challenging (Kassawmar et al., 2018c). Because the national-level datasets provide generic information and are therefore of limited use for assessing national- and regional-level natural capitals and ecosystem services, including intensification (Nachtergaele, 2008). In Ethiopia, most of the available spatial evidence about RCA are either from specific case studies only, which are sparse (Muluneh, 2010), or at the national level, which is outdated and varied due to differences in approach, extent, and purpose (Kassawmar et al., 2018a). This highlights the importance of the second research gap identified by the authors: the need for national-scale, high-resolution, and accurate spatial data on natural capitals, such as detailed Land Use and Land Cover information (LULC), land use, farming, livelihoods, and cropping systems. In developing countries, where resource allocation for knowledge generation is limited, generating comprehensive evidence about the natural capital of Ethiopia’s RCA is not a trivial task. In Ethiopia, on top of limited investment for data generation, methodological and technological challenges contribute to the lack of reliable evidence for complex smallholder farming systems over large areas. In the pursuit of national-level spatial evidence for decision-making (Muluneh, 2010; Tadesse et al., 2014), researchers face not only financial limitations but also technical, technological, and infrastructural challenges. Consequently, researchers often choose to conduct their studies on a smaller spatial scale. Experiences have shown that, to produce and provide comprehensive data in large, complex areas like Ethiopia’s RCA, researchers recommend applying advanced technology and substantial resources (Liu L. et al., 2020). To generate reliable and synoptic spatial evidence, advancements in remote sensing and geoinformatics privileged cost-effective and accurate methods compared to ground survey (Qiu et al., 2023). However, the intrinsic challenges of generating spatial evidence and knowledge over large and complex areas cannot be fully resolved by high-resolution imageries and advanced techniques like machine learning alone (Kassawmar et al., 2018a,c). Experiences indicate that local knowledge and secondary data are crucial for selecting relevant imagery and designing effective, context-specific mapping approaches.
Authors have theorized that the primary key to unlocking Ethiopia’s RCA intensification potential lies in effectively understanding and utilizing the two relatively neglected natural capitals: agricultural space (land) and green water (rainfall). This would need to apply remote sensing technologies, ground-truthing methods, and participatory approaches to engage with smallholder farmers. By building a comprehensive datasets and robust analytical frameworks, we can better assess the relationship between farming practices and natural capital, leading to more informed decisions and policies (Lambin, 2012; FAO, 2007). However, the issue of unlocking intensification potential of Ethiopia’s RCA goes beyond definition, delineating its extent and mapping and assessing its natural capitals. The success of any effort to intensify grain production will depend on the availability of an enabling policy environment and commitment in implementing it. In Ethiopia, much of the studies condemn existing land tenure systems and land use policies as never encourage intensification (Praveen et al., 2023; Zhang et al., 2021). This justifies the fourth category of research gap authors identified. Thus, in the quest for intensive food production, spatial evidence need to be generated, aiming to design and formulate context-specific and appropriate policy frameworks that allow to develop a sustainable strategy to alleviate food insecurity in the country (Endalew et al., 2015).
To this end, this study aimed to fill knowledge and information gaps in: clarifying the extent of the RCA and defining its boundaries with relevant criteria, and estimating the suitability, availability (PAC), and usability of land for horizontal (spatial expansion) and vertical (temporal cropping frequency) intensifications, as well as evaluating the performance of existing crop production systems. Specific objectives of the study are (i) to assess the vertical/spatiotemporal cropping intensification potentials of Ethiopia’s RCA, (ii) to explore and propose solutions to address landlessness and food insecurity among smallholder farmers in Ethiopia, and (iii) thereby to explore and generate policy-relevant knowledge to design intensification strategies. The high-quality national-scale spatial evidence generated will bridge knowledge gaps, enhance cropping intensification potential, and provide a foundation for understanding the RAA’s natural capital and regular monitoring of crop production. This research will help advocate for agricultural space-water management as a key solution to landlessness and food insecurity for smallholder farmers relying on rainfed systems.
2 Study area and data
2.1 Study area
The present assessment considered the entire Ethiopian boundary to delimit the extent of the Rainfed Cropping Area (RCA); detailed assessments of agricultural suitability were also made on the RCA, the vast proportion of which is often referred to as ‘highland.’ The highlands, which account for about 45% of the country’s total land area, are home to 90% of the total population and about 75% of the 33 million livestock population (Hurni, 1998). The larger part of the highland areas of the country, where smallholder traditional agricultural system has been practiced for thousands of years (Zeleza and McCann, 1997; McCann, 1997), has agro-climatic zones that are favorable to rainfed agriculture (Figure 1). Ethiopia is an agrarian country where the economy of the majority (>80%) of the population is exclusively dependent on rainfed-based farming. According to Zeleza and McCann (1997), the Ethiopian highlands (with elevation range > 1,500 m a.s.l.) have been inhabited by humans far longer than most places in the world. As a result, the highland areas face many environmental degradation problems due to long-standing agricultural activities, intensified by population pressure (Hurni, 1985).
Given that about 80% of Ethiopia’s population is dependent on agriculture, high population growth (2.5% in 2016) affects the RCA’s capacity in providing required ecosystem services (Teshome, 2014). According to the Central Statistical Agency of Ethiopia (CSA) (CSA, 2019), the country’s population was 22 million in the first National Sample Survey in 1967. It reached an estimated 100 million by 2014 (Teshome, 2014; CSA, 2019) and is expected to be 125 million in 2025. It is Africa’s second-most populous country. The growing population size has led to steady increase in the extent of cultivated land over the last half century but a decline in per capita cultivated land area. In 1950, on average 35 people shared 1 km2 of cultivated land; in 2014, 1 km2 of cultivated land was shared among 270 people (Teshome, 2014). According to projections, the Ethiopian population will double every 20–30 years (Teshome, 2014). This population growth could lead to acute land scarcity for crop production, with serious repercussions for food security (Hurni et al., 2005). The current household-level landholdings of the majority of Ethiopian highlanders is very small—46% of households possessed <1 ha in 2014 (CSA, 2019) and about 10% of the highland household is landless.
The impacts of climate change and extreme environmental degradation resulting from undesirable land use and land cover change (LULCC), as well as population pressures, would hinder sustainable development in Ethiopia (Wondie et al., 2016). For example, land degradation contributes to a decline in agricultural productivity and persistent food insecurity (Taddese, 2001; Tadesse et al., 2014). Continued population growth (Hurni et al., 2005) and fragile institutional frameworks (Pretty, 1999) further expose the highlands to continuous land degradation and conversion (Taddese, 2001; Zeleke and Hurni, 2001). As a result, the small-scale farming system in the highlands has become less profitable, and the carrying capacity of the land has extremely declined. In the current situation, increasing yields per hectare alone cannot ensure food security at the household level (average 4.5 family members) (Teshome, 2014). In the country’s highlands, grain production to feed the growing population is a concern that requires the implementation of alternative remedies, taking into account the realities of individual agricultural plots (Tadesse et al., 2014). Effective remediation measures on highly fragmented plots and patchy landscapes require detailed spatial information. Therefore, research that determines the extent of RCA (land currently cultivated and unused but suitable for growing rainfed crops) is important to support efforts to reshape Ethiopia’s development policy in the right direction.
2.2 Data types and sources
This study required the integration of various spatial datasets at the national level. The main datasets used include LULC, climate, topography, soil, and surface rockiness maps (Figure 2). For 2016, a detailed LULC map was created from Landsat-8 images with a pixel resolution of 30 m. A classification approach explained in Kassawmar et al. (2018a) was used, which takes the heterogeneity of the landscape into account. In addition to the LULC maps, complementary geospatial datasets were used including data on institutionally bounded areas (e.g., protected areas and settlements) obtained from government agencies and sources identified in Young et al. (2020) and the UNESCO database (IUCN, UNEP-WCMC, 2015). Other geospatial datasets used include: agroecological zones (Hurni, 1998); datasets on topographic variables such as slope and elevation ranges (SRTM at 30 m resolution from NASA (EarthExplorer1); datasets on climatic variables such as precipitation and temperature from the Ethiopian Meteorological Institute (EMI) and WorldClim data (WorldClim); soil data from the Water and Land Resource Center (WLRC2); and socio-economic data such as the Agricultural Area Survey Report, population census, and landholding sizes (CSA, 2019)). Improved versions of many of these geospatial datasets are available from WLRC as a series of geospatial database packages known as EthioGIS.II.3
3 Materials and methods
The data were analyzed in five main steps: (Hurni, 1985) defining the RCA (Abdollahzadeh et al., 2023) assessing the overall LULC composition in the RCA (Asfaw et al., 2021) estimating the currently cultivated and available (but uncultivated) cropland using sustainability analysis techniques (Hurni, 1983) mapping and estimating VCI indices by integrating primary and secondary data, and (Endalew et al., 2015) assessing the opportunities, challenges, and gaps of VCI and HCI.
3.1 Defining the extent of RCA
To redefine the RCA, the following steps were followed. First, we reclassified four important determinant factors (precipitation, temperature, altitude, and slope) into different value ranges (eight classes). Taking into account the agro-climatic suitability, a multi-criteria spatial analysis of the main factors of rain-based crop production was carried out (Lambin, 2012; Hurni, 1998). To assess and estimate the cultivated and uncultivated areas within the RCA, we constructed a detailed LULC map as described in Kassawmar et al. (2018c), which applies a second-level classification scheme with approximately 40 classes. Since the focus of the study was on the cultivated landscape, a special classification technique was used to create the LULC map. To obtain a reasonable and accurate estimate of cropland area from Landsat images, we followed a context-oriented, practical, and applicable approach for Ethiopia (Kassawmar et al., 2018a). To know the upper and lower limits of existing crop production practices, we reclassified the LULC data into two classes: cultivated and uncultivated, so that potentially available cropland in the currently uncultivated landscape could be assessed. Subsequently, we performed iterative spatial analyses by overlaying cultivated pixels over the maps of the reclassified major determinant factors. Then, the presence of pixels representing cultivated areas in each newly classified area of determining factors was examined and statistically analyzed to determine the presence and absence of cropland in each class as well as the relationship between the determining factors and cultivation practices. A recent map (2020) showing the cultivated areas was used to determine the currently cultivated area and later iteratively validate the boundary of the RCA. Based on spatial association rules and overlay analysis techniques, the lower and upper limits of the determinant factors for crop production under rainfed systems were determined. Later, the boundary of RCA was determined using the upper and lower limits of major factor relationships that exist with crop production systems.
3.2 Mapping and assessing farming, livelihood, and land use systems in the RCA
Recent and improved farming, livelihood, and land use systems for the RCA were produced by updating previous datasets available from the FUSNET database of FAO (Medhin, 2011) employing several geospatial data integration and analysis complemented by ground survey and secondary data sources. The production of detailed LULC maps in this study (Level II) enabled us to significantly improve both the content and accuracy of these maps (Supplementary Figure S1 and Supplementary Table S3).
3.3 Rainfed crop production potential and performance assessment
With the aim of demonstrating the multi-faceted challenges of smallholder farmers of Ethiopia, whose livelihood is largely dependent on rainfed-based food production systems, such as food insecurity and landlessness, initially, two categories of solutions were anticipated: (i) land use systems and land management-oriented solutions and (ii) institutional/policy solutions. For the former case, detailed natural capital, land quality, and ecological suitability/environmental sustainability analyses were carried out to test two CI options that could address the prevailing challenges: (1) HCI option (Hurni, 1985) HCI options, i.e., how much extra cultivable land does exist on currently uncultivated portion of the RCA; and (2) VCI option (Abdollahzadeh et al., 2023) VCI, i.e., to what extent Multiple Cropping System (MCS) can be implemented practically without adding more space on the existing cultivated landscape of the RCA. To explore options and solutions of the first category, a detailed crop production suitability assessment on currently uncultivated landscapes of the RCA was performed and PAC was estimated. In the latter case, the performance of existing CPS was evaluated against the potential of RCA to apply MCS. Eventually, the findings were narrated in relation to the potential and possibility of addressing food security and landlessness challenges of inhabitants of the RCA of Ethiopia. For the latter solution resettlement, outmigration, and land policy (such as land redistribution, land consolidation, land allocation/reallocation, population control, and land tenure) were assessed and discussed. Supplementary Tables S2, S3 present the identified indicators with their formula/equation and basic description of the indices used to assess the performance of different CPS.
3.4 Cropping intensification potential and performance assessment
The potential and performance/efficiency of LUS and CPS can be assessed from varying dimensions, but scholars have widely used the three sustainability pillars, namely ecological, economical, and social dimensions (Wirsenius et al., 2010; Biswas et al., 2006; Liu J. et al., 2020). This study adopted a new holistic performance/efficiency framework that allows us to perform a multidimensional land use performance and efficiency developed by Liu J. et al. (2020). The potential of the RCA and existing CPSs were assessed using three categories of performance/efficiency evaluation measures: Natural Capital, Land Use Performance, Production, and Economic Performance. In the first category, Cropping Density Index (CDI), Agricultural Density Index (ADI); in the second category, Cultivated Land Utilization Index (CLUI), Multiple Cropping Index (MCI), and Cropping Intensity Index (CII); and in the third category, Area Diversity or Crop Diversity Index (CDI), Relative Yield/Productivity Index (RYI), and Unit Productivity Index (UPI) were used.
3.4.1 VCI potential, performance, and gap assessment
According to the present assessment, VCI is meant to produce food/grain applying multiple times (>2 times) crop production strategies over a single year in the same land, such as multiple cropping systems (MCS) and agroforestry systems using only rain water. A list of MCS, CPS, relevant CI indicators/measures, indices with formula, equations, used to assess the performance of CPS, and descriptions of indicators are provided as Supplementary Tables S2, S3.
3.4.2 Potential of HCI and gaps assessment
According to Lambin (2012), suitability of potentially available cropland (PAC)—sometimes referred to as land reserve, underutilized land, or spare land—is a category used to distinguish land areas considered moderately to highly suitable for cropping, which could be brought under cultivation in the near future. Two main approaches are often used to estimate PAC: residual and categorical approaches (Lambin, 2012). The residual approach involves simply excluding currently cultivated areas from the entire agroecologically suitable region (Ramankutty et al., 2002). The residual approach is used when spatially explicit, detailed land requirements information is not available and/or when the scope of the analysis is very wide, e.g., global or continental (Campbell et al., 2008). The use of the categorical approach requires detailed land quality requirements that allow the integration of various biophysical determinants of crop production (see Supplementary Table S1). The output can be categorized into different levels of suitability classes. The categorical approach is therefore best suited for spatially explicit and interlinked analysis made at the local/regional level (Lambin, 2012; Campbell et al., 2008). To assess the present and project the PAC in the RCA, we followed the FAO Land Suitability Assessment Framework (FAO, 2007). The following steps were performed: (Hurni, 1985) First, we took the 2016 LULC map of the RCA and defined the Actually Available Cropland (AAC), which is the currently cultivated landscape of the RCA. Next, the total uncultivated area of the entire RCA that is considered environmentally sustainable and theoretically usable for crop production was determined by excluding the AAC pixels from the RCA (Abdollahzadeh et al., 2023). Then we identified completely unsuitable (N) and theoretically suitable (S) landscapes within the RCA using the detailed land requirement factor maps (see Supplementary Table S1). From the LULC datasets, the N landscape includes settlement, water bodies, exposed rocks, afro-alpine, infrastructures, and river courses (Asfaw et al., 2021). Then, we identified institutionally constrained areas, such as parks, sanctuaries, reserves, conservation priority areas, ritual sites (churches and mosques), priority forests, and hunting areas. To map the PAC within the RCA, we need to determine the degree of suitability of the uncultivated area based on various crop production factors (slope, rainfall, temperature, land cover, soil depth, and altitude) (Hurni, 1983). Therefore, in the last step, we determined the degree of suitability of S-pixels within the RCA. This means that S has expanded the suitability classes according to both biophysical and socio-economic aspects. To further map and estimate the suitability level of S landscapes, which could indicate the temporal dimension of conversion (likelihood of being used for crop production), a multi-criteria spatial analysis technique was carried out, covering the entire rainfed agricultural area. This was done after excluding currently cultivated areas, completely unsuitable areas, protected areas, and intact forest areas; later evaluating the biophysical suitability of the remaining areas using multi-criteria decision rule techniques. Finally, the usability of the PAC was transcribed using a systematic assessment of the PAC and its availability from a socio-political perspective, such as population density, settlement, landholding size, landlessness, agricultural/livelihood system, policies, and history in the country.
3.5 Overall potential and performance assessment and implications
The overall performance of existing CPS against the inherent potential of the RCA was evaluated taking the average values of each performance index from all categories (with the assumption of having equal weights of each factor). The overall implication of the existing grain production performances of the various CPS was assessed by comparing food supply potential of the rainfed landscape against the existing food/grain demand of the society residing at 1 km2 grid. Taking the overall average performance values of each CPS, gaps and future policy directions were identified by comparing the existing performance against the inherent natural capital or potential of the RCA to practice any cropping intensification options (CI).
4 Results
4.1 Extent of the RCA and current state
As identified by the newly defined RCA of Ethiopia (Figure 3), crop growing altitudinal limits of Ethiopia’s RCA vary between 500 and 3,800 m a.s.l. depending on the latitudinal and longitudinal influence on crop production. The RCA covers 667,094 km2, making up about 60% of the country’s land mass. The upper and lower elevation limits of the RCA include both currently cultivated land cover (221,653 km2 or 33% of the RCA); 67% (445,441 km2) of the RCA is uncultivated.
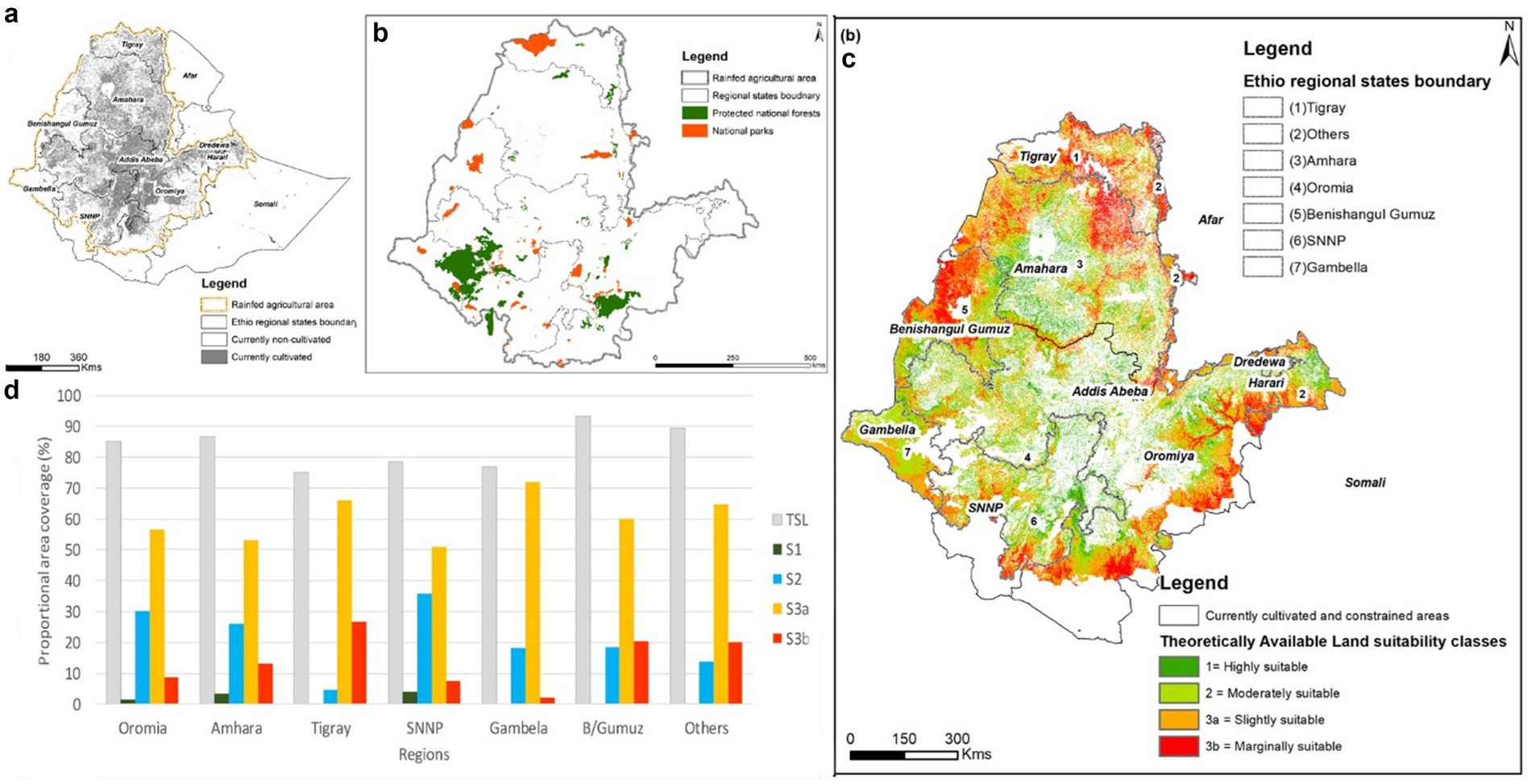
Figure 3. The redefined RCA supper imposed on the currently cultivated area (top-left-A) and the PAC within the RCA (right-C), institutionally restricted areas for cropping (top-middle-B), and proportion of theoretically suitable land (sum of S1, S2, S3a, and S3b) with different suitability classes in the RCA summarized according to administrative regions of Ethiopia (bottom-left-D).
The RCA landscape features very complex LULC and LUS types (Supplementary Figure S1 and Table 1). As per the facts generated from the LULC maps, currently, the cultivated landscape covers about a third of the RCA. The uncultivated part of the RCA is dominantly covered by woody vegetation (37%), forest (12%), grassland (12%), and non-woody vegetation (7%).
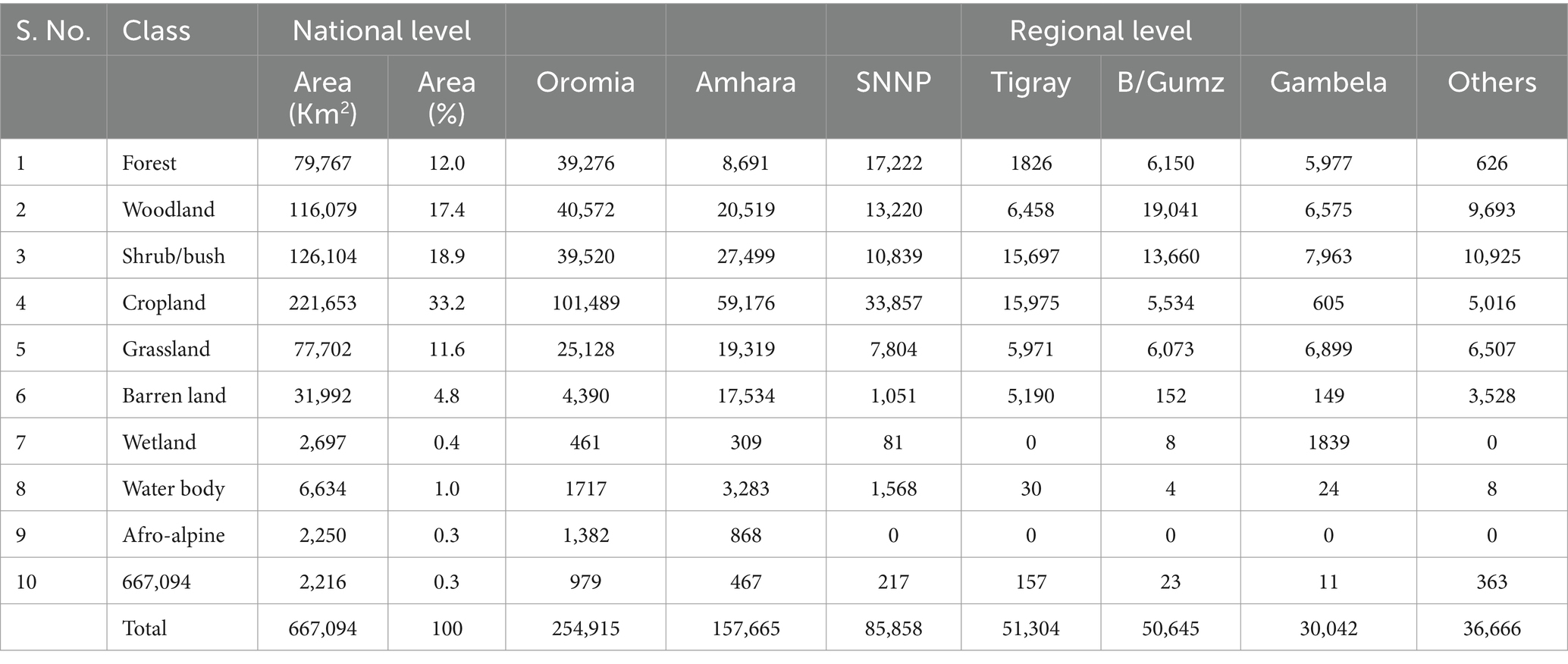
Table 1. Composition of major LULC types and land use systems in the RCA (Kassawmar et al., 2018a) by administrative regions.
4.2 Rainfed-based crop/food production systems in the RCA
The type, spatial distributions, and coverage of major growing seasons are provided as a Supplementary Figure S2. The location, coverage, and nature of crop growing season within the RCA vary considerably, being dependent on the rainfall pattern. Spatio-temporal variations in the amount, intensity, onset, and offset times of rainfall seasons are the main cause for diversity in crop production systems. Statistical facts generated from Supplementary Figure S2 confirm that about 38% of Ethiopia’s RCA is used for food production, applying only one main rainfall season (locally called Meher season; June–September).
In some parts of the RCA, a second rainfed-based crop production system, known locally as the Belg rainfall season, allows a significant portion of the population to produce grain 1–3 times in a year. Additionally, a third growing season—residual soil moisture-based crop production—exists but lacks sufficient attention from the government and scientific community. This system is typically practiced after the main rainy season ends, with farmers also utilizing residual moisture left in the soil after the Belg rainy season.
Although the widely known residual moisture-based CPS represents a growing season that starts from the end of the main rainy season, in some areas, farmers also use residual moisture left in the soil after the end of the short rainy season (Belg). Thus, a fourth category of CPS exists in some places where overlapping of the first, second, and third growing seasons happens. Indeed, farmers rarely use such growing season for a complete crop production; instead, farmers use it to support the growth of crops planted either during the main growing season and/or during short rainy seasons. Such practices are commonly observed in high-altitude areas where evaporation is minimal. Supplementary Figures S1, S2 present major rainfed-based growing seasons and major CPS.
As depicted in Supplementary Figures S1, S2, the start and end of the second growing season (Belg or short rainy season) are extremely variable, which makes the spatial and temporal boundary fuzzy. As a result, in areas where there is a high synergy between these rainy seasons, the total length of growing periods (LGP) is higher, which allows farmers to grow perennial and permanent crops like coffee, false banana (locally called Enset), mango, avocado, banana, papaya, and several types of root crops. According to the facts generated from the maps depicted in Figure 4, in the majority of the RCA, which accounts for 38%, crop production is practiced using the main rainfall growing season (Mehere), followed by Meher-Residual-Belg complex synergy (12%), Meher-Residual (~10%), and Meher-Residual-Belg overlapping (5%).
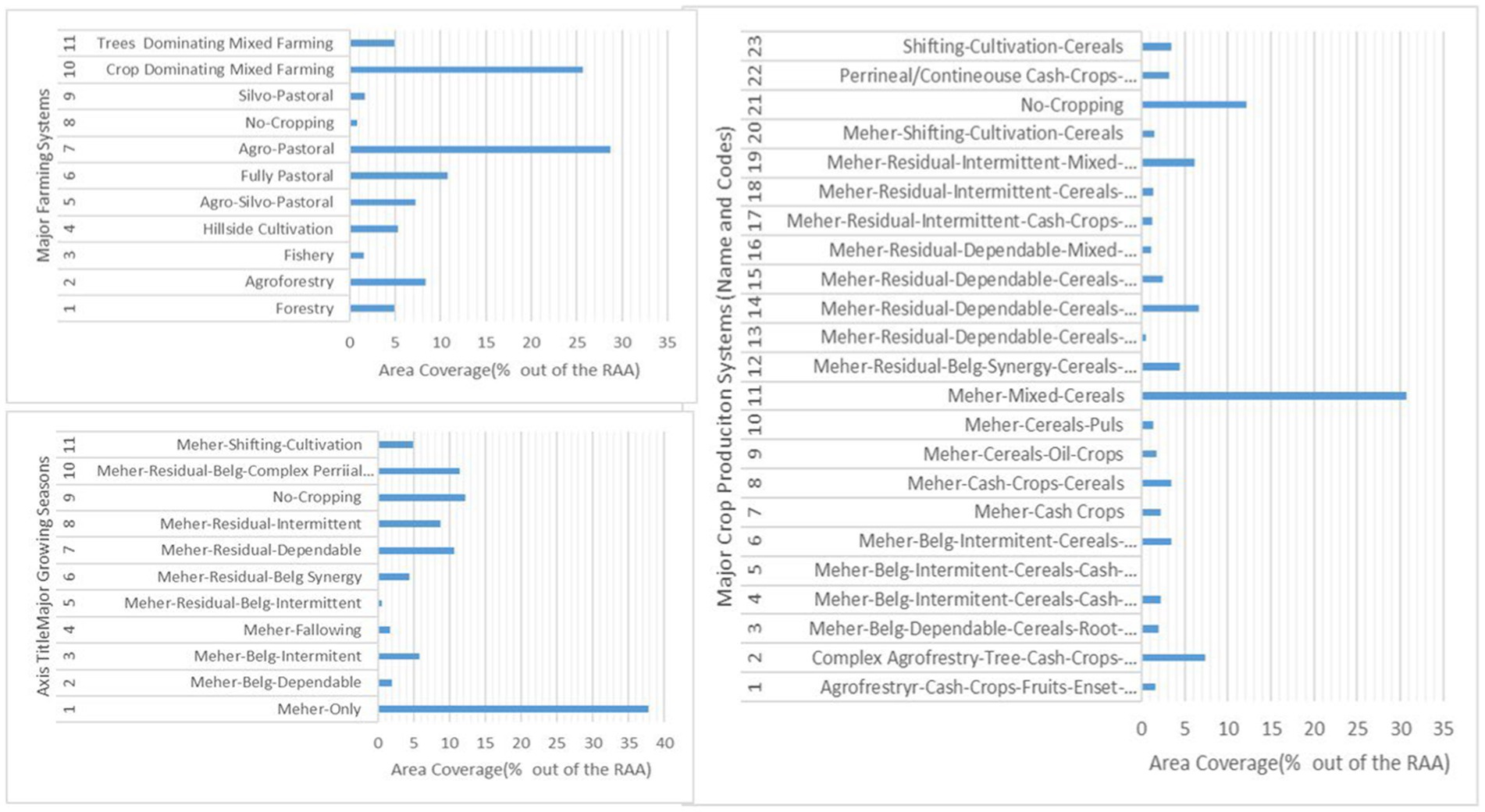
Figure 4. Major farming systems (top left), major growing seasons (bottom left), and major crop production systems (right).
4.3 Determinants of rainfed-based food production in Ethiopia
This study identified major determinant factors and evaluated several biophysical and socio-economical constraining factors that could hinder expansion of the extent of existing crop production on uncultivated landscapes of the RCA. The type and spatial distribution of most important and direct constraining factors of rainfed-based crop production were identified from the detailed LULC map. Before assessing the level of suitability, which was decided based on the identified determinant factors, non-cultivable areas were excluded from the analysis.
According to the definition given by this study, parts of the RCA landscapes that are biophysically constrained are areas technically infeasible, economically not viable, or ecologically unsustainable to produce grain or food. In that case, these landscapes represent areas where crop production remains infeasible and unprofitable when applying existing knowledge, technology, and efforts. These landscapes are easily discernable from our detailed LULC map and include classes such as exposed rocks, extremely degraded hills, exposed sand surface, river course, water bodies, settlements, and permanent wetlands, as well as landscapes having slope > 50%. Moreover, the entire currently uncultivated RCA is not usable for crop production, even if theoretically and biophysically suitable. Unless we checked the institutional constraints. Institutionally constrained areas identified by this study include parks, sanctuaries, reserves, conservation priority areas, ritual sites (churches and mosques), priority forests, and hunting areas (Supplementary Figure S2 and Supplementary Table S4). These classes in total account for about 6% of the RCA.
4.4 Cropping intensification potential
4.4.1 Potential of the RCA for HCI
4.4.1.1 Theoretically available cropland (TAC) in the RCA
The current cultivated landscape is taken as actually available cropland (AAC), which implies a landscape has never been constrained by any biophysical and socio-political factors for grain production (Table 2). Theoretically available area (TAC) which was estimated by subtracting the completely unsuitable portion of the RCA from the sum of the currently uncultivated area (CUA) and the currently cultivated area (CCA) (Table 2). This implies that the portion of existing uncultivated landscapes, less cultivated landscapes, and the constrained areas (Section 4.3) gives theoretically available area to expand crop production in the RCA of Ethiopia.
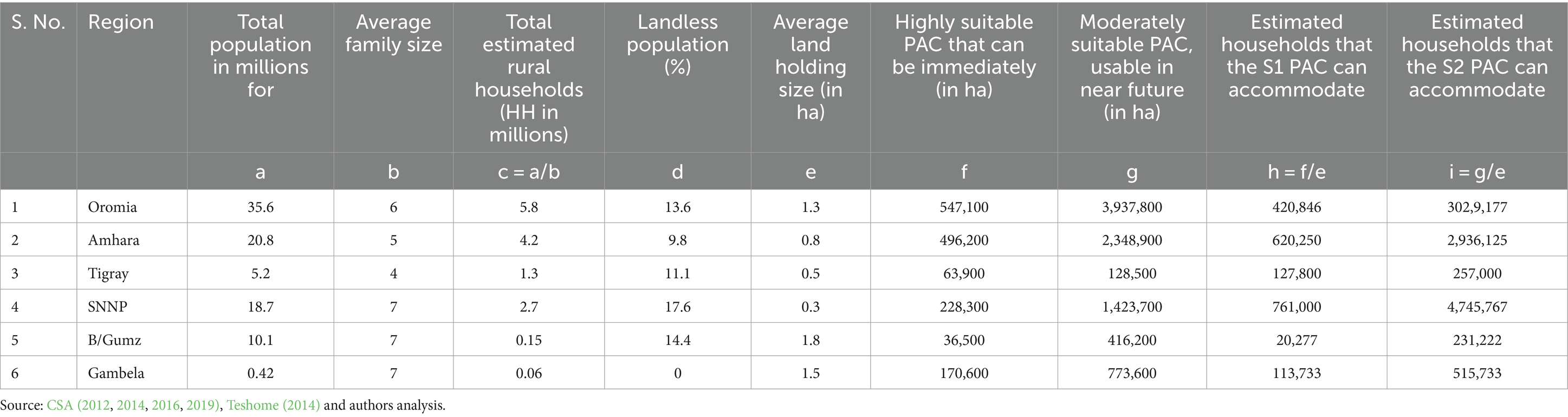
Table 2. Political administrative level statistical summary of PAC and its implications for addressing landlessness.
4.4.1.2 Potentially available cropland (PAC) in the RCA of Ethiopia
The TAC is a portion of the RCA that cannot be fully used in the short term due to several constraints, such as knowledge, technology, and resources. Thus, only a portion of TAC on which the society can utilize the land with the available knowledge and technology is PAC. The availability of more space for grain production in the RCA were estimated at four different suitability classes (S1, S2, S3a, and S3b), showing the degree of conversion to cropland. The suitability classes S1 and S2 (from which we have adopted PAC) together represent areas to be exploited in the near future. According to the estimates, about 15,797 km2 are highly suitable, while the moderately suitable landscape covers 94,051 km2, accounting for about 4 and 21% of the RCA’s currently uncultivated area, respectively. In total, both account for approximately a quarter (25%) of the RCA’s currently uncultivated area and 16% of the total RCA, respectively. The remaining, currently uncultivated area, however, is assessed as slightly suitable (56%) and hardly suitable (12%) of the RCA. The spatial distribution and the administrative regional proportional coverage of the CCA and the PAC are shown in Figure 3.
About 67% of the RCA in Ethiopia is currently not cultivated, and over half of the RCA in each region is non-cultivated (Table 3). Considerable proportions of non-cultivated RCA are found in Gambela and Benishangul-Gumuz regional states. The theoretically suitable land (S) of the RCA is about 419,338 km2 (63%). S assumes that all such areas could be converted from their current land use systems to crop cultivation. However, landscapes are subject to constraints from a variety of biophysical, socio-economic, and institutional factors. Excluding those areas that refer to completely unsuitable (N) areas from a biophysical perspective, the theoretically suitable land is reduced by 4% (i.e., from 67% [column “ci”] to 63% [column “ei”]) (Table 3). According to our assessment, about 6% of Ethiopia’s RCA is institutionally constrained in terms of possible land use. Broken down into administrative regional states (see Figure 3), more land in Gambela is institutionally restricted (20% of the RCA of the region), followed by SNNP (15%), Tigray (15%), and Oromia (9%). Amhara region has the smallest (2%) percentage of institutionally restricted land. However, this analysis only refers to landscapes subject to state-level institutional restrictions. If local-level institutional restrictions were considered (e.g., community enclosures), the estimated PAC area would likely shrink significantly.
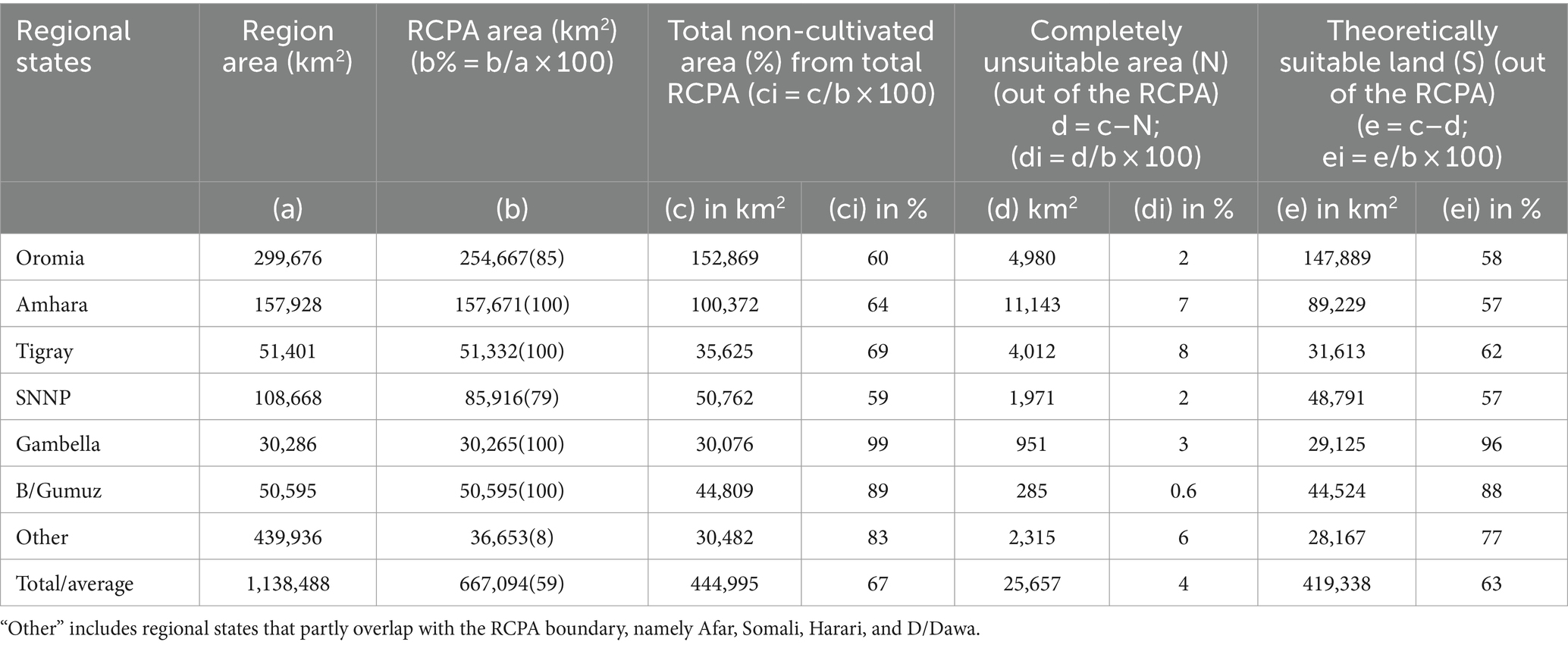
Table 3. Currently non-cultivated, permanently unsuitable, and theoretically suitable areas of the RCPA.
4.4.2 Vertical cropping intensification (VCI) potentials and gaps
4.4.2.1 VCI potentials of the RCA
The VCI potential of the RCA, assessed by comparing existing practices against the potential, is presented in Figure 5, expressing the suitability of the RCA to intensify crop production using existing knowledge and technologies. The assessment was made on both CCA and PAC parts of the RCA. According to the potential assessment results, about 50% of the RCA of Ethiopia can support rainfed-based Multiple Cropping Systems (MCS) with a varying level of suitability. Specifically, around 14 and 25% of the RCA features moderately and slightly suitable landscapes for double cropping. The CI potential and the existing CI practices are presented in Figure 5.
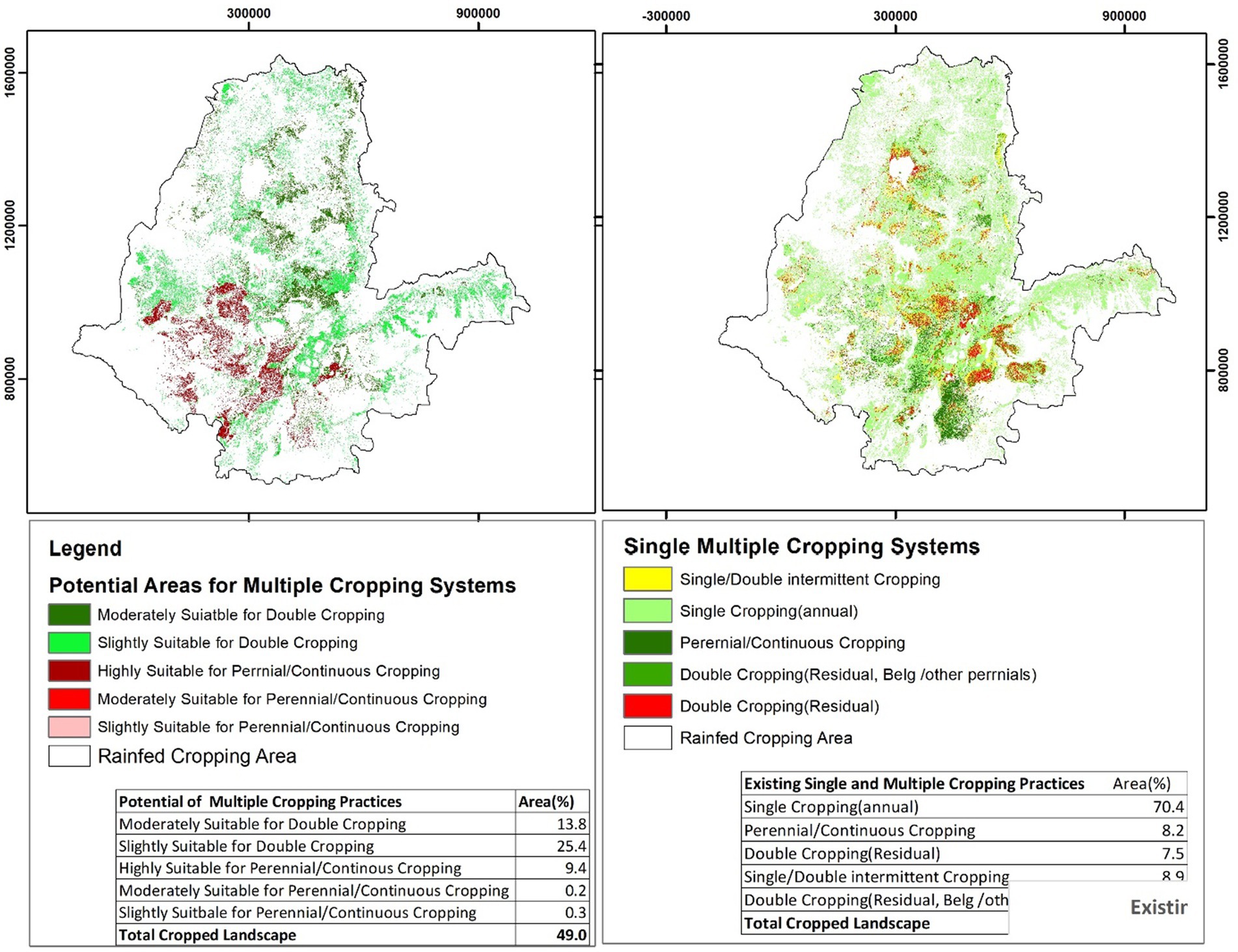
Figure 5. Multiple cropping system (MCS)-based CI (potential left) and existing (right) in the RCA of Ethiopia and statistical comparisons (bottom).
In contrast, only 9, 0.2, and 0.3% of the RCA are highly, moderately, and slightly suitable for perennial/continuous cropping, respectively, indicating that 10% of the RCA can support such systems without significant land use changes or investments. Despite this potential, single cropping remains dominant in 70% of the RCA. Double cropping is consistently practiced in 7% of the CCA, intermittently practiced in 9%, and both double and perennial cropping are intermittently practiced in 6% of the RCA.
4.5 Potential and performance of existing CI practices
The performance assessment focused solely on currently cultivated landscapes, using a multidimensional approach to evaluate CI potential and the performance of existing CPS. Figure 6 displays the spatially explicit performance assessment results, organized and summarized by major growing seasons.
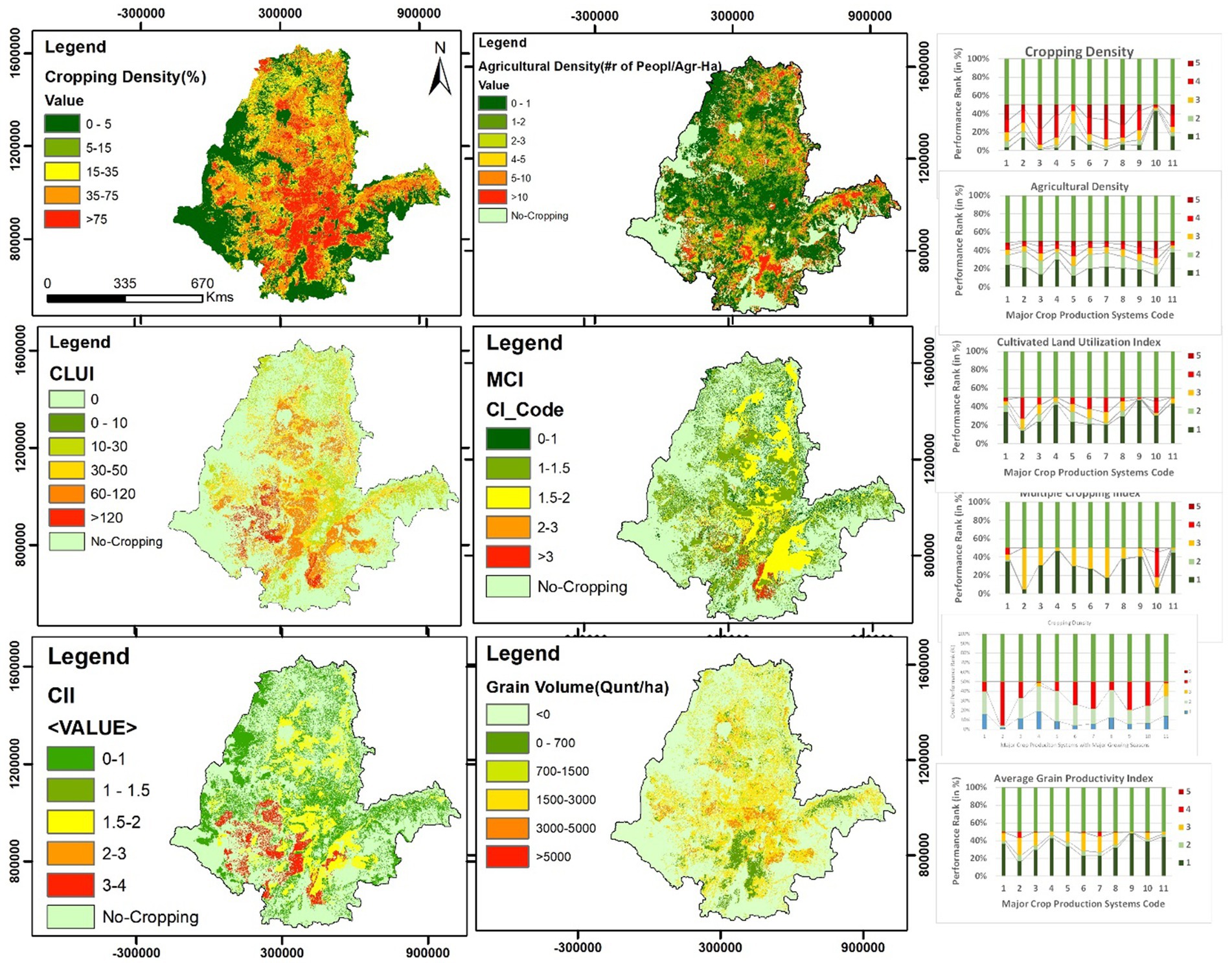
Figure 6. Performance of different CPS: CDI (top left), AGDI (top middle), CLUI (left middle), MCI (middle middle), CII (bottom left), AYI (right bottom).
The CDI represents the percentage of cropped pixels within a 1 km2 area of the PAC, reflecting how well existing CPS enhances HCI. There is significant spatial variation in CDI values across the RCA, with the central and northeastern regions showing relatively higher CDI values (>50%). In contrast, the peripheries have low CDI values, indicating substantial areas remain uncultivated. As shown in Figure 6, regions with intermittent Meher-Residual-Belg seasons are the most densified. Figure 6 illustrates that the CDI values closely resemble the AGD values, though not uniformly.
A higher CLUI indicates that farmers use CPS involving either multiple cropping methods, resulting in more frequent grain production, or single-season crops grown over extended periods. Landscapes managed with the former approach have higher CII values due to increased grain yield within a single production season. In other words, extensively cultivated land is covered by crops for longer periods or cultivate the land multiple times in a year.
Cultivated landscapes in the southern, southwestern, and central parts of the RCA, with CLUI values above 50, cover a considerable area. Areas managed under perennial/continuous CPS, residual-based CPS, and Meher-Residual and Meher-Residual-Belg-based CPS also exhibit high CLUI values. The MCI assesses CPS performance by comparing the total area used for grain production to the total available cropland per year. Typically, if the available land is used for a single-crop production annually, the MCI value is 1.
In the northern, western, northwestern, and partly southern parts of the RCA, where there is typically only one growing season and the available land is used for cropping a maximum of once per year, the MCI value is <1. However, in areas with intermittent double-growing seasons and double cropping practices, the MCI value can exceed 1. For example, in regions where Belg rainfall and residual moisture-based CPS are practiced intermittently, MCI values are influenced by the success or failure of these moisture sources. Figure 7 displays the overall potential and performance assessment results, which were obtained by applying PCA to each index for every dimension.
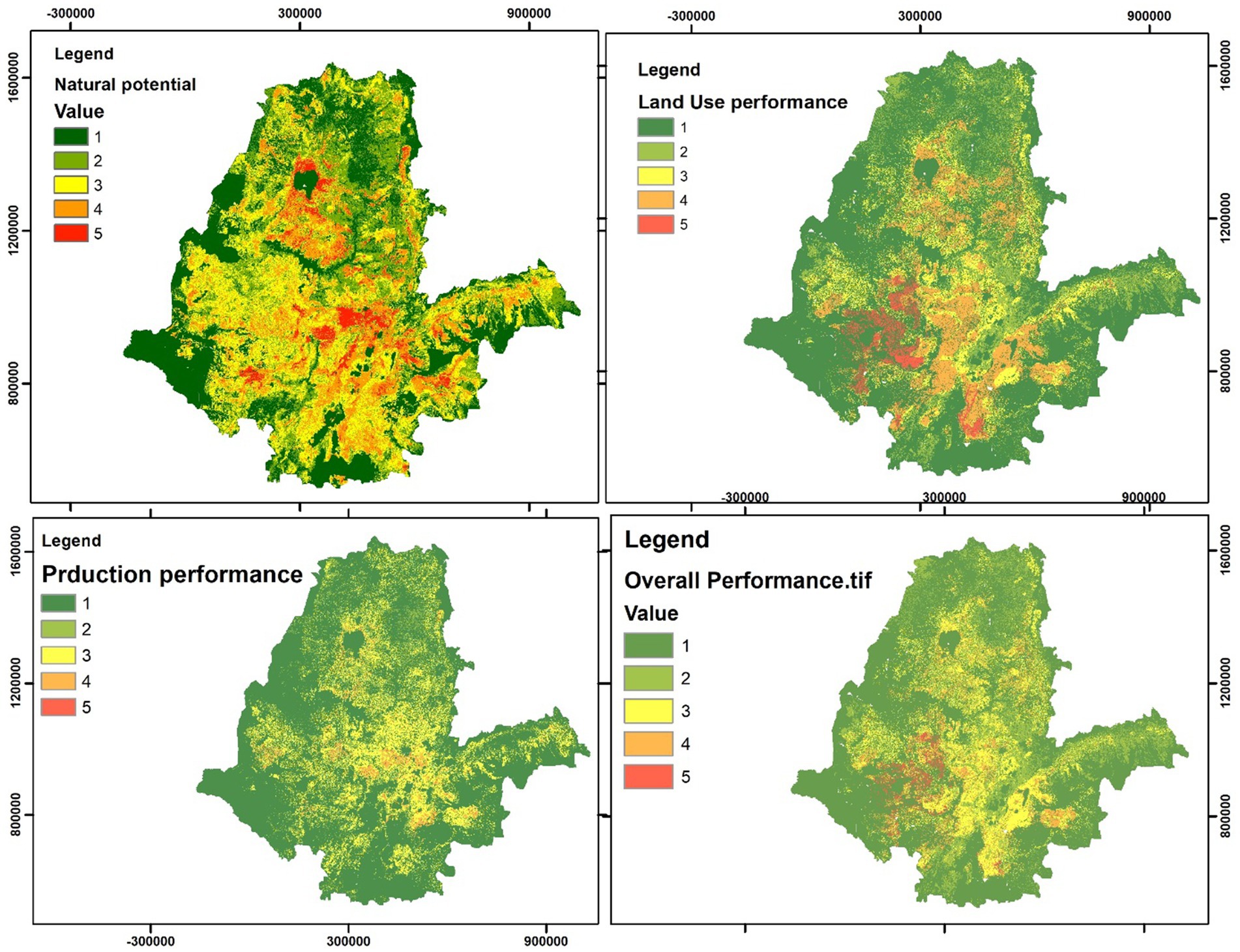
Figure 7. Overall average natural capital/land potential of the RCA for cropping intensification (top left), average land use performance (right top), average production performance (left bottom), and average overall performance (bottom right) of CPS in RCA.
Performance comparisons in Figure 7 also reveal that the central part of the RCA generally performs better than the southern and southwestern regions.
4.5.1 Cropping intensifications gaps and implications
As discussed, the overall performance of existing CPS varies significantly based on the RCA’s potential and land use and production performances of the applied CPS. To better understand the performance of LUS and CPS and its implications, a spatially explicit evaluation was conducted, comparing food supply potential with existing food/grain demand at a 1 km2 scale. Figure 8 illustrates the food gap and the total number of food-needy people at a 1 km2 grid scale. The map shows that a significant portion of the central and part of the north-central RCA has no food gap, indicating these areas produce a surplus of grain relative to the food demand. However, contrary to our by CPS performance evaluation, the southern and southwestern parts of the RCA show a higher food demand and gap. Indeed, our food demand and gap analysis did not account for non-grain crops, permanent tree crops like False Banana, other forest products, or livestock products. As shown by official data on long-term food insecurity and food aid (Figure 8, bottom right), regular food aid has been provided by the government to people in high-potential districts in the southern parts of the RCA.
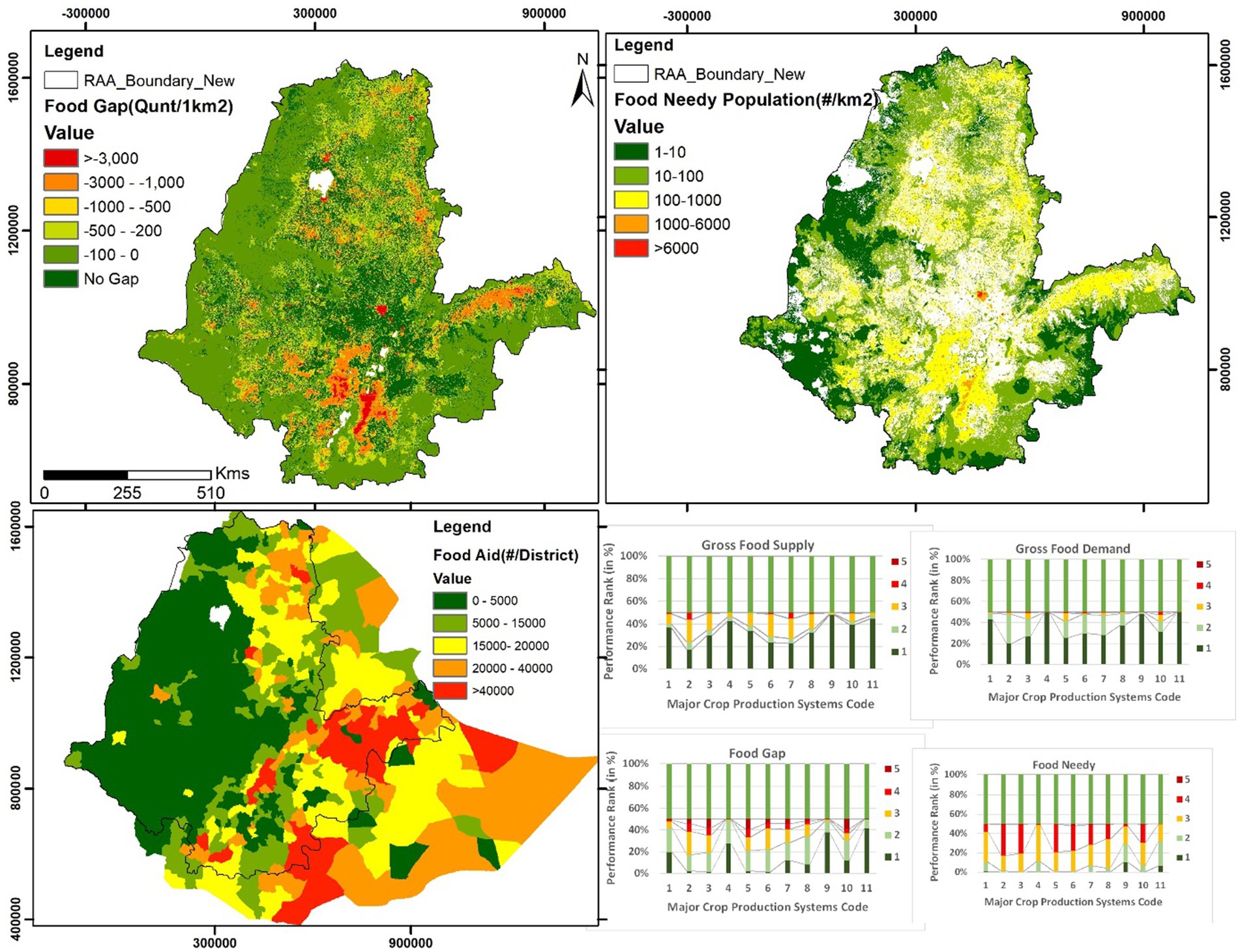
Figure 8. Socio-economic implications of existing CPS: GGI (top left), G/FDPI (top right), food aided population (bottom left), and statistical facts about each indicator (bottom right).
5 Discussion
5.1 State of Ethiopia’s RCA
In Ethiopia, there have been few attempts to delineate the boundary and estimate the extent of RCA, e.g., Hurni (1998) and Hedberg (1970). According to Hurni (1998), 3,800 m a.s.l. is the uppermost altitude limit of cropping, while the lower altitude limit varies depending on the climate, mainly the relationship between rainfall and evaporation. As indicated by research findings of Hurni (1998) the limit varies spatially; in the western side of the country, it can reach up to 800 m a.s.l.; in the eastern side of the country, it reaches up to 1,200 m a.s.l. Researchers in the field also identify clear temporal trends of land use practices related to change in agro-climatic factors, which shift the lower and upper altitudinal limits of crop production (Hurni, 1998; Ramankutty et al., 2002; Harvey and Pilgrim, 2011). Moreover, ecological and technological adaptations also push the temporal boundaries of CPS (what can be cultivated and where). This study highlights not only the importance of continually redefining the physical boundaries of Ethiopia’s RCA but also presents the techniques for regularly defining it in a manner that is spatially consistent and temporally fitting with existing cropping practices in relation to the dynamic determinant factors of rainfed-based crop production, like climate. Moreover, establishing such boundaries alone does not help to know the suitability level or the potential of the RCA for food production, as several biophysical, socio-economic, and institutional factors could control food production system.
5.1.1 Options and alternative solutions of HCI
The pixel-level HCI assessment determined the PAC with various levels of suitability. The PAC estimates indicate the highly (S1) and moderately (S2) suitable portions of the RCA that remain uncultivated. Figure 9 shows the cropping eligibility ranges after accounting for biophysical, institutional, and socio-economic constraints. According to our assessment, Ethiopia’s PAC is the sum of highly (S1) and moderately (S2) suitable areas, which is about 109,848 km2 and accounts for 25% of the currently uncultivated area of the RCA. This means that an additional 16% of Ethiopia’s RCA could be used for rainfed-based crop production in the future. This value appears small when compared to the percentage of marginally suitable areas (S3a and S3b). Theoretically suitable (S) and potentially available (PAC) RCA for crop production compared to landless populations varies significantly among regional states in Ethiopia (Figures 7, 9b and Table 2). For example, less populated regions such as Gambela and Benishangul-Gumuz regions have relatively large proportions of uncultivated landscape. However, most of these landscapes in the region are not particularly suitable compared to the highland landscape (Figure 9). This limits the usability of the uncultivated landscape in these regions. In contrast, populous regions such as SNNP and Amhara have large numbers of landless people and a low proportion of PAC, making it difficult to address landlessness through agricultural livelihood options. The spatial variation in land availability within PAC and the population-to-land ratio vary widely across regional states. That implies, while reducing pressure on the environment and other ecosystem goods and services, expanding acreage within each regional state and attaining the required area per capita to achieve equitable economic benefits may not be possible. Under such circumstances, the implementation of alternative policies (e.g., resettlement/reallocation and intensification) largely depends on the existence of an enabling policy environment (Pretty, 1999; Lotze-Campen et al., 2010; Harvey and Pilgrim, 2011). Therefore, how to utilize the larger area (67% of the currently uncultivated RCA) to address landlessness and food insecurity is an important strategic challenge that needs to be resolved. Some of the possible solutions and pertinent challenges to effectively utilize the PAC are discussed below.
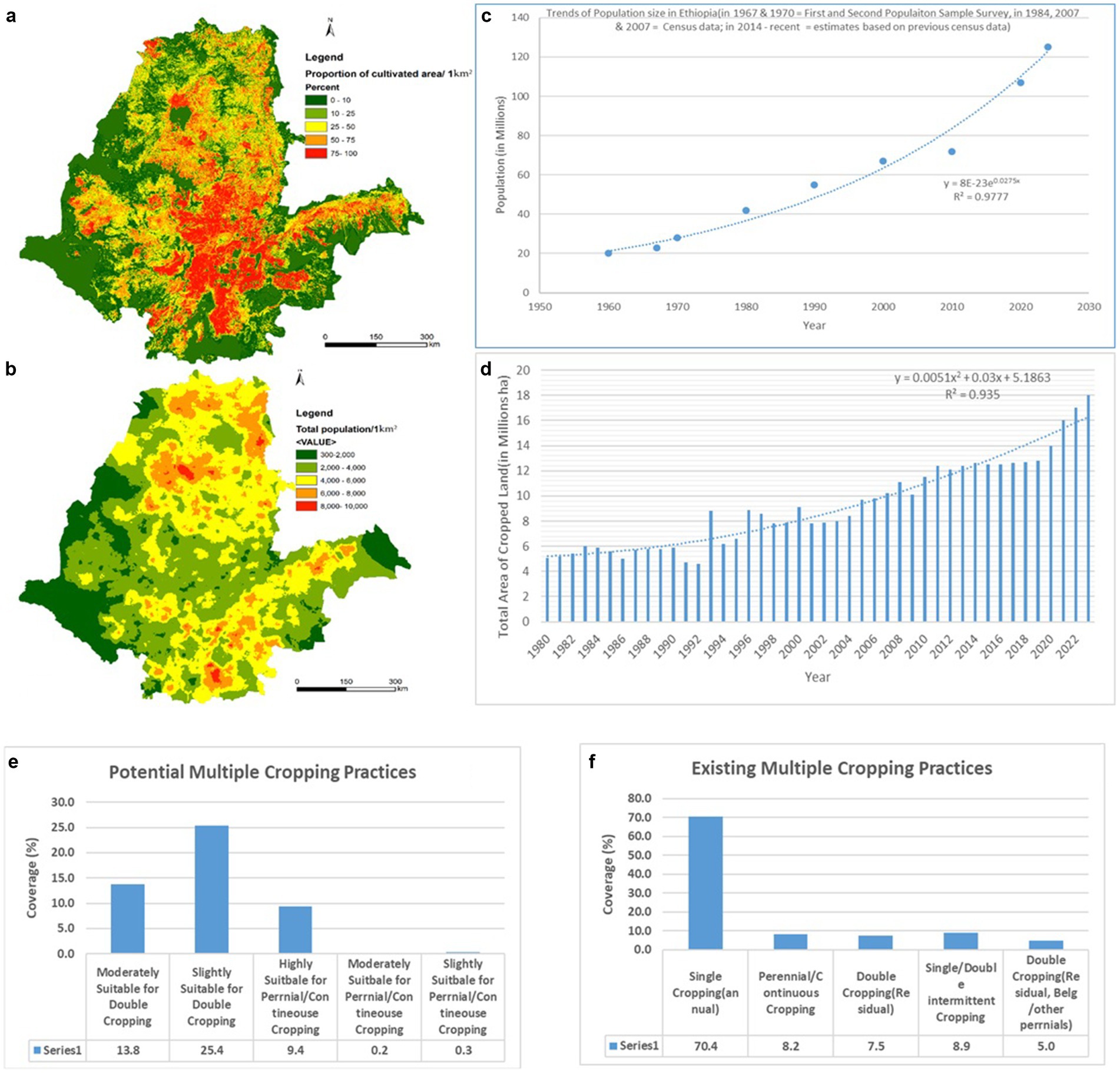
Figure 9. (a) Cultivated/Cropland density index (currently cultivated area per km2) (source: present study) and (b) comparison of rural population density, (c) national population growth, and (d) cropland expansion (source: CSA, 2019, 2014, 2016), potential MCP (e) and existing MCP (f).
5.1.2 The challenges of HCI
5.1.2.1 Outmigration and resettlement options
In the highly degraded, densely populated, and food insecure highland areas of Ethiopia, migration and/or resettlement of smallholder households have been considered as alternative policy options to address the problems of drought, famine, landlessness, and food insecurity (Rahmato, 2003). For example, Ethiopia’s 2002 food security strategy presents resettlement as one of the pillars of its’ approach. Apart from the directed flows and forms of migration, these measures focused primarily on planned and large-scale rural-to-rural migration (Rahmato, 2003). However, in our view, strategies to combat landlessness that emphasize rural-to-rural migration must first examine two critical factors, namely: (Hurni, 1985) the availability (area) and spatial distribution of PAC; and (Abdollahzadeh et al., 2023) the existence of an enabling policy environment for implementation. Regarding point one, the spatial distribution of PAC in Ethiopia varies significantly from region to region. Rural–rural outmigration or resettlement (sporadic or planned) could be seen as a major option for regions such as Tigray, Eastern Amhara, and Eastern Harerghe in view of the lack of PAC (Rahmato, 2003). These regions have already utilized most of their suitable areas for crop cultivation (Figure 6 and Table 2). Their remaining areas are subject to institutional bans (see Rural Land Administration and Use Proclamation, Proc. No. 456/2005). These imply that resettlement and rural–rural migration are right measures in view of factor 1.
However, the second critical factor—the need for an enabling policy environment—reveals complications: Ethiopia’s population policy, adopted in 1993 and described in strategy papers (Abesha et al., 2022), discourages rural–rural migration (small or large resettlements). In effect, inter- and intra-regional planned resettlement is prohibited after 2005. Furthermore, resettlement programs aiming to move people from degraded, densely populated areas to supposedly fertile, sparsely populated areas are highly contested from a variety of ecological, political, and socio-cultural perspectives (Lemenih et al., 2004; Hammond, 2008). In recent years, the political face of the issue is more complicated by ethnic-based resource use and protection, which is leading to ‘ethnophobia.’ There are also criticisms that administrative and executive efforts are weak to resolve those problems. There is also ample evidence that large-scale resettlement programs in Ethiopia have failed to achieve their goals (Rahmato, 2003). Thus, intra-regional resettlement and rural–rural migration are not supported by enabling institutional, administrative, and political climates.
Overall, programs of rural–rural migration and/or planned resettlement of landless rural households appear problematic and unsustainable both in the short term and the long term (Hammond, 2008). The prospects of using such strategies to address landlessness and food insecurity in Ethiopia were further diminished with the adoption of a decentralized land administration policy. The evidence generated on PAC in the different regional states can be utilized to revisit and analyze the intra-regional resettlement policy options and help to steer again resettlement and migration policy making.
5.1.2.2 Land distribution/redistribution, allocation/reallocation options
Efforts toward addressing landlessness may require land distribution/redistribution and allocation/reallocation. Following the overthrow of Ethiopia’s imperial regime in 1974, the land use right was transferred from the former landlords to the poor peasants under the motto “land to the tiller” by Proclamation No. 31/1975. Since then and until the late 1990s, allocation and reallocation (redistribution) of land were undertaken as important means of addressing landlessness (Rahmato, 2003). However, until 2005, the land use arrangements (especially cropland expansion) and legal land redistribution were made untenable (Hurni et al., 2005; Zeleke and Hurni, 2001). This is mainly because the redistribution considers addressing landlessness, non-land suitability, and productivity. As a result, major portions of RCA landscapes gradually deteriorated due to inappropriate land use conversion, improper crop production practices, and a lack of entitlement or ownership within a functioning tenure system. Land redistribution can only work where the plots of land are large enough to support individual households under any possible strategy of CI or sustainable use of land (Teshome, 2014). Some researchers have criticized previous repeated land redistribution efforts for transforming Ethiopian agriculture from small-scale agriculture to micro-agriculture, hampering food security at the national level (Teshome, 2014). It may be feasible to redistribute land in some regions or areas of Ethiopia, for example, in Oromia, Somalia, and Gambella regional states where redistribution was not implemented. However, considering that efforts to redistribute currently cultivated land would negatively impact Ethiopia’s food security and thwart its Green Economy development strategy, land redistribution was banned (Hammond, 2008).
Concerning the second option (land allocation and reallocation), land reserve is required to support a growing future population, especially for youths who have no option to inherit land from their family. Given that about 10% of the highland rural households in Ethiopia are currently landless (Table 2), such options are very important. According to projections, Ethiopia’s population will grow to 120 million by 2025 and 150 million by 2050 (based on the 2007 national census; see Figure 9) (Teshome, 2014). This implies a doubling of Ethiopia’s current landless households (from 10% to 20%) and serious shortages of land for cultivation. Under such conditions, land allocation/reallocation could be an important option to utilize the available uncultivated land for crop production (Benin and Pender, 2001). In this regard, the 67% of currently non-cultivated land within Ethiopia’s RCA would be a huge resource to address landlessness and related food insecurity. Viewed nationally, disregarding the currently cultivated land areas, a maximum of 25% of the currently non-cultivated portion of the RCA is available (i.e., S1 and S2) for near-term use. At the sub-national level, the potentially available land could accommodate about 10% of the current landless population in Oromia, 25% in Amhara, 19% in Tigray, 37% in SNNP, and 15% in Benishangul Gumz regional states (see Table 2). In the long term, there is also the possibility of using marginally suitable (S3a and S3b) land and landscapes, as these areas could be made suitable for cultivation through massive land rehabilitation and management measures, use of improved crop varieties, and agronomic practices. The former option, however, has a much longer time horizon, as major efforts to rehabilitate degraded lands have already been underway for two decades (Kassawmar et al., 2018b). Given that existing policies do not favor redistribution of currently cultivated land, allocation of PAC to the landless and producing grain shall be seen as an important option (Wubneh, 2018). However, it is well understood that, while rural land use and administration policy does foresee expansion of cultivable land, there are conflicts with environmental protection goals and development strategies of the country, such as investment policies, that need much of the PAC. Therefore, this option can be useful only if appropriate land use and sustainable land management measures are implemented as part of land allocation programs and the country’s agricultural development strategies (Haregeweyn et al., 2012).
5.2 The potentials of VCI in Ethiopia’s RCA: options and alternative solutions
Present trends in global agricultural development are oriented toward VCI of agriculture through technology improvement and knowledge transfer. In Ethiopia, some observers view VCI as a means to meet future food demand with less environmental impact and to prevent inappropriate expansion of cropland (Harvey and Pilgrim, 2011). As shown in Supplementary Figure S1 and Figures 3, 9, Ethiopia’s RCA has immense potential for practicing diverse farming systems (FS) and land use systems (LUS) and for supporting various livelihood systems. Comparisons between existing and potential rainfed MCSs in the RCA (Figure 5) show that, with few exceptions, there is only one main rainy season in the northern and central parts. In contrast, the southern and southwestern regions benefit from an extended main rainy season (over 6 months) and a shorter rainy season. Although there is minimal rainfall in the northern and northwestern parts, farmers in these areas often adopt effective cropping systems that make optimal use of the limited rainfall available in a short period of time (approximately 1 month). In the eastern and central-eastern parts of the RCA, a bimodal rainfall pattern in some areas supports MCS, such as Meher-Residual-Belg. However, rugged terrain and inappropriate land use have led to severe land degradation, reducing the effectiveness of these MCP systems (Zhang et al., 2021). Conversely, the southern and southwestern parts of the RCA, with their extended rainfall season (over 6 months), are well suited for permanent and perennial/continuous cropping, allowing for double and triple food production per year.
According to the assessment results, about one-third of the CCA in the RCA could benefit from VCI through MCP. However, the level of suitability for intensification varies significantly over time and space. For example, only about 10% of the RCA is highly suitable for MCS, where complex CPS have integrated complex and mixed annual and perennial crops, including fruits, tree crops, and root crops, within the same field. Contrarily, the majority of the RCA is currently underutilized, as 70% of the landscape is managed by single cropping practices. The Cropped Area Diversity Index (CADI) value-based CPS performance shows that most of the RCA has values above 10, indicating that approximately 10 different crops can grow in a 1 km2 area. This represents an exceptional potential for food and nutrition security. However, according to the AGDI, the rainfed-based livelihood system supports an average of about five people per a hectare of cropland. Although explicit data (Figure 9) reveal that only 33% of the TAC or suitable landscapes in the RCA are used for crop production the average CDI for the RCA is below 50%, meaning that only 50% of each 1 km2 of suitable land is effectively utilized for grain or crop production. This indicates that Ethiopia has substantial HCI potential for increasing and intensifying food production, but achieving this will require more efficient land use and cropping systems and enabling policy (Benin and Pender, 2001).
In some areas, such as the western and northwestern regions and the peripheries of the RCA, AGDI values can reach up to 10, indicating a person-to-cropland ratio of 1:10. CLUI values confirm that while some landscapes are overused, many parts of the RCA remain underutilized. CLUI estimates show that about 45% of the RCA have CLUI values below 50%, indicating that the current CPS only utilize about half of the year for food production. Indeed, some areas have CLUI values reaching up to 200, indicating the practice of perennial or continuous cropping systems. Low to moderate MCI and CII values are observed in the western, central, northern, northwest, and northeastern parts of the RCA.
The moderate to high MCI and CII values in these RCA areas are primarily due to CPS based on Residual Soil Moisture and Belg Rainfall. However, there is significant room for improvement in enhancing VCI in these regions. Despite having moderate to high MCI and CII values, their performance in terms of AYI is low to very low. In approximately 35 and 45% of the RCA with such CPS, the RYI was found to be low and very low, respectively. Higher RYI values are observed in the southern, southwestern, central, and some northern parts of the RCA, where MCI and CII are relatively higher. The VCI assessment indicates that only 1% of the RCA is managed under CPS with very high CI performance. In the remaining parts, cultivated landscape managed under CPS with poor, moderate, and high CI performance cover 19, 18, and 7% of the RCA, respectively (see Figure 10).
5.3 Implications and policy direction
A comparative analysis of the food security index across different CPS shows a significant food gap in the North, Northeast, Central, Central East, East, and parts of the Central RCA. The spatial distribution of GGD and GFDP confirms that the largest nutritional gaps exist in the best-performing landscapes with efficient CPS (see Figure 7 for index values on the map). An important question that needs thorough explanation is why the overall performance of the southern regions is lower compared to other RCA regions, despite the southern and southwest regions having higher MCI and CII values or greater CI potential. There are three main reasons for this discrepancy: (i) The CI analysis weighted all indices equally, resulting in higher values for areas with higher CDI and lower AGD values. (ii) The indicators used in this study did not account for other food sources, such as non-grain foods, or False Banana and fruit crops, which are significant for many populations with different feeding cultures. (iii) These regions are solidly populated, with a high ratio of people to cropland, sometimes exceeding 10 people per hectare. Comparing food supplies to the food-needy population at the district level using secondary sources could help clarify some of the ambiguities (Figure 8).
The performance assessment results suggest that a more efficient CPS alone may not meet food demand or solve problems of landlessness and food security. Consequently, our analysis is not sufficient to fully assess food security and its relationship to CPS. However, the study concludes that high-performing LUS and CPS alone do not ensure food security. In systems with multiple annual harvests, such as perennial or continuous cropping and complex agroforestry systems the MCI is not a good indicator as it only takes into account the area covered and does not differentiate between different crops in a year. In comparison, the CII is a better indicator because it takes into account both the area covered per production season and the frequency of annual grain harvest per unit area. This is evidenced by high CII values in complex agroforestry and perennial/continuous cropping systems where continuous production occurs throughout the year.
In addition to knowledge, technology, and land use/management strategies beyond VCI, effective policy solutions are needed to balance natural capital with human needs. In fact, careful interpretation of the data is crucial, as some results of the implemented framework may contradict real-world conditions.
6 Conclusion
This study redefined Ethiopia’s Rainfed Cropping Area (RCA) and found it comprises about 60% of the country’s land mass. It also assessed the suitability of the uncultivated portion of the RCA for rainfed-based grain production and feed its nation. The results indicate that Ethiopia is currently using only 33% of its RCA for crop cultivation. Out of the remaining 67% of the RCA, only 16% is highly and moderately suitable, which is considered as Potentially Available Cropland (PAC). This study highlights the possible options to use this portion of the RCA. This study confirms that, against the perceived HCI potentials, there is less remaining land in the RCA which is highly suitable (4% of the currently uncultivated portion of the RCA) and can be cultivated without investment than is usually assumed. If land allocation is chosen as a policy option, the highly suitable PAC cannot fully accommodate the current landless households (10% of the total rural household population). However, inclusion of both highly suitable and moderately suitable PAC (109,848 km2) makes it possible to fully address current landlessness. However, implementation of the identified policy options—land distribution/redistribution, resettlement, and intensification—will face challenges; there is considerable incongruity between densely populated areas, high landlessness, and PAC throughout Ethiopia. Ethiopia’s existing highly and moderately suitable (PAC) landscapes are located in sparsely populated areas. In theory, this points to resettlement or rural–rural outmigration as possible options. This implies that land use planners could consider resettling landless people from highly populated regions to the less populated regions. However, policymakers could find these options very difficult to implement under the current ethnic-based political administration; resettling people could continue to foment unrest or ethnic tensions unless handled very carefully. Implementation of such alternative measures also depends on the existence of enabling policy environment across and within the regional governments. Moreover, PAC is largely found in relatively less hospitable environments (particularly regarding temperatures) compared to the cold, humid highlands of the RCA. Considering the options of VCI and/or HCI solutions to landlessness and food insecurity problems, both currently non-cultivated (67%) and cultivated portions (33%) of the RAA have huge potential for non-crop production/farming systems such as Silviculture farming (e.g., timber, wild honey, wild coffee), livestock production (e.g., dairy farming, beef farming, apiary, poultry), eco-tourism, and others. However, the assessments within this study did not consider these farming systems, which is a limitation, and it is recommended that more research is needed in this regard.
The assessment findings revealed that the efforts exerted towards HCI in Ethiopia reach only 33% of the RCA. On the other hand, existing VCI practices cover only 10% of RCA, while in nearly 30% of the RCA, it can be practiced at various levels of suitability. In addition, the performance of existing VCI-oriented CPS is below average, as in only 7% of the practices an acceptable or higher performance is recorded. This confirms that existing efforts made to enhance both HCI and VCI are both insufficient and inefficient.
The study’s findings suggest that to fully realize the potential of both intensification practices, it is essential to enhance productivity in marginal areas through sustainable land management practices. Integrating land rehabilitation with intensive agriculture not only benefits farmers to maximize yield, but also to enhance a wide range of benefits that could be obtained from conserved ecosystems. Experiences from the regions of Tigray and eastern Amhara show that investments in marginally suitable lands can bear fruit by generating ecosystem services and creating extra cropland. At the same time, successfully integrating land rehabilitation and VCI requires innovative technologies, improved inputs, effective agronomic practices, and enabling policy options. Various land rehabilitation activities are already being implemented in many parts of the RCA landscapes. In utilizing the full potential of the RCA, the introduction of better land management interventions can help to boost food production and, in turn, contribute to addressing landlessness and food insecurity in Ethiopia. Ensuring sustainable land management and enhancing productivity call for prohibiting undesirable land use shift and enforcing proper land use practices, including making sure any crop cultivation on steep slopes embeds in its soil and water conservation measures. Above all, agricultural research must also be intensified to enable better technological options, such as improved crop varieties, and scaling mechanisms, such as adaptation and upscaling of different crop varieties and cropping practices.
In summary, available options for tacking efforts to address the food insecurity and landlessness issues require: (i) applying efficient and effective land use and land management practices; (ii) developing and implementing strong policy options and strategies that accommodate and/or support sustainable cropping intensifications (resettlement, land distribution, and land use adjustment require spatially explicit datasets and information). We strongly suggest incorporating spatial information in decision-making processes, including land use planning and land administration. Such studies can provide both knowledge and information on the availability of land and alternative options for these lands in addressing landlessness. Therefore, the presented approach and outputs play a considerable role to support scientific and evidence-based decision-making.
Data availability statement
The raw data supporting the conclusions of this article will be made available by the authors, without undue reservation.
Author contributions
TK: Conceptualization, Data curation, Formal analysis, Funding acquisition, Investigation, Methodology, Project administration, Resources, Software, Supervision, Validation, Visualization, Writing – original draft, Writing – review & editing. MT: Supervision, Validation, Visualization, Writing – review & editing. GD: Resources, Supervision, Validation, Writing – review & editing. AB: Supervision, Validation, Visualization, Writing – review & editing. ET: Data curation, Validation, Visualization, Writing – review & editing. WB: Funding acquisition, Investigation, Project administration, Resources, Supervision, Visualization, Writing – original draft, Writing – review & editing. LA: Data curation, Resources, Software, Writing – review & editing. GZ: Funding acquisition, Project administration, Resources, Supervision, Writing – review & editing. CW: Funding acquisition, Project administration, Resources, Supervision, Writing – review & editing. GO’D: Resources, Software, Supervision, Validation, Visualization, Writing – review & editing.
Funding
The author(s) declare that financial support was received for the research, authorship, and/or publication of this article. This study was supported by the Water Security and Sustainable Development Hub, which is funded by the UK Research and Innovation’s Global Challenges Research Fund (GCRF), grant number: ES/S008179/1.
Acknowledgments
The authors express their sincere gratitude to the Water and Land Resource Centre of Addis Ababa University (WLRC-AAU) for the facilitation of the data collection and provision of other required resources to undertake this study.
Conflict of interest
The authors declare that the research was conducted in the absence of any commercial or financial relationships that could be construed as a potential conflict of interest.
Publisher’s note
All claims expressed in this article are solely those of the authors and do not necessarily represent those of their affiliated organizations, or those of the publisher, the editors and the reviewers. Any product that may be evaluated in this article, or claim that may be made by its manufacturer, is not guaranteed or endorsed by the publisher.
Supplementary material
The Supplementary material for this article can be found online at: https://www.frontiersin.org/articles/10.3389/fsufs.2024.1393124/full#supplementary-material
Footnotes
References
Abdollahzadeh, G., Sharifzadeh, M. S., Sklenička, P., and Azadi, H. (2023). Adaptive capacity of farming systems to climate change in Iran: application of composite index approach. Agric. Syst. 204:103537. doi: 10.1016/j.agsy.2022.103537
Abesha, N., Assefa, E., and Petrova, M. A. (2022). Large-scale agricultural investment in Ethiopia: development, challenges and policy responses. Land Use Policy 117. doi: 10.1016/j.landusepol.2022.106091
Asfaw, A., Bantider, A., Simane, B., and Hassen, A. (2021). Smallholder farmers’ livelihood vulnerability to climate change-induced hazards: agroecology-based comparative analysis in northcentral Ethiopia (Woleka sub-basin). Heliyon. 7. doi: 10.1016/j.heliyon.2021.e06761
Benin, S., and Pender, J. (2001). Impacts of land redistribution on land management and productivity in the Ethiopian highlands. Land Degrad. Rehabil. 12, 555–568. doi: 10.1002/ldr.473
Biswas, B., Ghosh, D. C., Dasgupta, M. K., Trivedi, N., Timsina, J., and Dobermann, A. (2006). Integrated assessment of cropping systems in the eastern indo-Gangetic plain. Field Crops Res. 99, 35–47. doi: 10.1016/j.fcr.2006.03.002
Campbell, J. E., Lobell, D. B., Genova, R. C., and Field, C. B. (2008). The global potential of bioenergy on abandoned agriculture lands. Environ. Sci. Technol. 42, 5791–5794. doi: 10.1021/es800052w
CSA (2014). The Federal Democratic Republic of Ethiopia Central Statistical Report Agency statistical. Eur. J. Clin. Pharmacol. 73.
CSA (2016). The Federal Democratic Republic of Ethiopia Central Statistical Agency report on area and production of major. Stat Bull. 584.
CSA. (2019). The Federal Democratic Republic of Ethiopia Central Statistical Agency agricultural sample survey. Report Area Prod Major Crop. p. 1.
Endalew, B., Muche, M., and Tadesse, S. (2015). Assessment of food security situation in Ethiopia: a review. J. Agric. Res. 9, 55–68. doi: 10.3923/ajar.2015.55.68
Hammond, L. (2008). Strategies of invisibilization: how Ethiopia’s resettlement programme hides the poorest of the poor. J. Refug. Stud. 21, 517–536. doi: 10.1093/jrs/fen041
Haregeweyn, N., Berhe, A., Tsunekawa, A., Tsubo, M., and Meshesha, D. T. (2012). Integrated watershed management as an effective approach to curb land degradation: a case study of the enabered watershed in northern Ethiopia. Environ. Manag. 50, 1219–1233. doi: 10.1007/s00267-012-9952-0
Harvey, M., and Pilgrim, S. (2011). The new competition for land: food, energy, and climate change. Food Policy 36, S40–S51. doi: 10.1016/j.foodpol.2010.11.009
Hurni, H. (1985) Erosion–productivity–conservation systems in Ethiopia. In: IV international conference on soil conservation November 3–9, 1985, Maracay, Venezuela.
Hurni, H. (1988). Degradation and conservation of the resources in the Ethiopian highlands. Mt. Res. Dev. :8(2–3).
Hurni, H. (1998) Agroecologial belts of Ethiopia: Explanatory notes on three maps at a scale of 1:1,000,000. Research Report, Soil conservaton research program.
Hurni, H., Tato, K., and Zeleke, G. (2005). The implications of changes in population, land use, and land management for surface runoff in the upper Nile Basin area of Ethiopia. Mt. Res. Dev. 25, 1–14.
Hurni, K., Zeleke, G., Kassie, M., Tegegne, B., Kassawmar, T., Teferi, E., et al. (2015) The economics of land degradation. Ethiopia case study. Soil degradation and sustainable land Management in the Rainfed Agricultural Areas of Ethiopia: an assessment of the economic implications. Report for the Economics of land degradation initiative.
IUCN, UNEP-WCMC. (2015) The world database on protected areas [online], November. Available at: www.protectedplanet.com (accessed January 2015).
Kassawmar, T., Eckert, S., Hurni, K., Zeleke, G., and Hurni, H. (2018a). Reducing landscape heterogeneity for improved land use and land cover (LULC) classification across the large and complex Ethiopian highlands. Geocarto Int. 33, 53–69. doi: 10.1080/10106049.2016.1222637
Kassawmar, T., Gessesse, G. D., Zeleke, G., and Subhatu, A. (2018b). Assessing the soil erosion control efficiency of land management practices implemented through free community labor mobilization in Ethiopia. Int. Soil Water Conserv. Res. 6, 87–98. doi: 10.1016/j.iswcr.2018.02.001
Kassawmar, T., Zeleke, G., Bantider, A., Gessesse, G. D., and Abraha, L. (2018c). A synoptic land change assessment of Ethiopia’s rainfed agricultural area for evidence-based agricultural ecosystem management. Heliyon. 4, 1–21. doi: 10.1016/j.heliyon.2018.e00914
Lambin, E. F. (2012). Global land availability: Malthus versus Ricardo. Glob. Food Sec. 1, 83–87. doi: 10.1016/j.gfs.2012.11.002
Lambin, E. F., and Meyfroidt, P. (2011). Global land use change, economic globalization, and the looming land scarcity. Proc. Natl. Acad. Sci. USA 108, 3465–3472. doi: 10.1073/pnas.1100480108
Lemenih, M., Olsson, M., and Karltun, E. (2004). Comparison of soil attributes under Cupressus lusitanica and Eucalyptus saligna established on abandoned farmlands with continuously cropped farmlands and natural forest in Ethiopia. For. Ecol. Manag. 195:57. doi: 10.1016/j.foreco.2004.02.055
Liu, J., Jin, X., Xu, W., Gu, Z., Yang, X., Ren, J., et al. (2020). A new framework of land use efficiency for the coordination among food, economy and ecology in regional development. Sci. Total Environ. 710:135670. doi: 10.1016/j.scitotenv.2019.135670
Liu, L., Xiao, X., Qin, Y., Wang, J., Xu, X., Hu, Y., et al. (2020). Mapping cropping intensity in China using time series Landsat and Sentinel-2 images and Google earth engine. Remote Sens. Environ. 239, 1–18. doi: 10.1016/j.rse.2019.111624
Lotze-Campen, H., Popp, A., Beringer, T., Müller, C., Bondeau, A., Rost, S., et al. (2010). Scenarios of global bioenergy production: the trade-offs between agricultural expansion, intensification and trade. Ecol. Model. 221, 2188–2196. doi: 10.1016/j.ecolmodel.2009.10.002
Mandryk, M., Doelman, J., and Stehfest, E. (2015). Assessment of global land availability: land supply for agriculture. FOODSECURE Technical papers.
McCann, J. C. (1995). Suffering under God’s environment: a vertical study of the predicament of peasants in north-Central Ethiopia (review). Northeast. Afr. Stud. 2, 209–215. doi: 10.1353/nas.1995.0027
McCann, J. C. (1997). The plow and the forest: narratives of deforestation in Ethiopia, 1840-1992. Environ. Hist. 2, 138–159.
Medhin, G. (2011) Livelihood zones analysis. Improved livelihoods for smallholder farmers: A tool for planning agricultural water management investments, Ethiopia. Faowater.
Nachtergaele, F. (2008) Mapping land use systems at global and regional scales for land degradation assessment Analysis version 1.0. LADA Land Degradation Assessment in Drylands.
Nkwasa, A., Waha, K., and Van, G. A. (2023). Can the cropping systems of the Nile basin be adapted to climate change? Reg. Environ. Chang. 23, 1–14. doi: 10.1007/s10113-022-02008-9
Praveen, A., Jeganathan, C., and Mondal, S. (2023). Mapping annual cropping pattern from time-series MODIS EVI using parameter-tuned random Forest classifier. J. Indian Soc. Remote Sens. 51, 983–1000. doi: 10.1007/s12524-023-01676-2
Pretty, J. (1999). Can sustainable agriculture feed Africa? New evidence on progress, processes and impacts. Environ. Dev. Sustain. 1, 253–274. doi: 10.1023/A:1010039224868
Qiu, B., Hu, X., Yang, P., Tang, Z., Wu, W., and Li, Z. (2023). A robust approach for large-scale cropping intensity mapping in smallholder farms from vegetation, brownness indices and SAR time series. ISPRS J. Photogramm. Remote Sens. :203.
Rahmato, D. (2003). Resettlement in Ethiopia. The tragedy of population relocation in the 1980s. Forum Soc. Stud. 22, 66–75.
Ramankutty, N., Foley, J. A., Norman, J., and McSweeney, K. (2002). The global distribution of cultivable lands: current patterns and sensitivity to possible climate change. Glob. Ecol. Biogeogr. 11, 377–392. doi: 10.1046/j.1466-822x.2002.00294.x
Taddese, G. (2001). Land degradation: a challenge to Ethiopia. Environ. Manag. 27, 815–824. doi: 10.1007/s002670010190
Tadesse, G., Zavaleta, E., Shennan, C., and FitzSimmons, M. (2014). Policy and demographic factors shape deforestation patterns and socio-ecological processes in southwest Ethiopian coffee agroecosystems. Appl. Geogr. 54, 149–159. doi: 10.1016/j.apgeog.2014.08.001
Teshome, M. (2014). Population growth and cultivated land in rural Ethiopia: land use dynamics, access, farm size, and fragmentation. Resour. Environ. 4, 148–161. doi: 10.5923/j.re.20140403.03
Wirsenius, S., Azar, C., and Berndes, G. (2010). How much land is needed for global food production under scenarios of dietary changes and livestock productivity increases in 2030? Agric. Syst. 103, 621–638. doi: 10.1016/j.agsy.2010.07.005
Wondie, M., Schneider, W., Katzensteiner, K., Mansberger, R., and Teketay, D. (2016). Modelling the dynamics of landscape transformations and population growth in the highlands of Ethiopia using remote-sensing data. Int. J. Remote Sens. 37. doi: 10.1080/01431161.2016.1246773
Wubneh, M. (2018). Policies and praxis of land acquisition, use, and development in Ethiopia. Land Use Policy 73, 170–183. doi: 10.1016/j.landusepol.2018.01.017
Young, N. E., Evangelista, P. H., Mengitsu, T., and Leisz, S. (2020). Twenty-three years of forest cover change in protected areas under different governance strategies: a case study from Ethiopia’s southern highlands. Land Use Policy. 91.
Zeleke, G., and Hurni, H. (2001). Implications of land use and land cover dynamics for mountain resource degradation in the Northwestern Ethiopian highlands. Mt. Res. Dev. 21, 184–191. doi: 10.1659/0276-4741(2001)021[0184:IOLUAL]2.0.CO;2
Zeleza, P. T., and McCann, J. C. (1997). People of the Plow: an agricultural history of Ethiopia, 1800-1990. Technol. Cult. 38, 135–137.
Keywords: cropland, potential cropland, intensification, land suitability, rainfed agriculture, landlessness, food insecurity, Ethiopia
Citation: Kassawmar T, Tadesse M, Desta G, Bantider A, Teferi E, Bewket W, Abraha L, Zeleke G, Walsh CL and O’Donnell G (2024) Unlocking horizontal and vertical cropping intensification potentials to address landlessness and food security challenges of rainfed crop production systems in Ethiopia: potential, performance, and gap assessment. Front. Sustain. Food Syst. 8:1393124. doi: 10.3389/fsufs.2024.1393124
Edited by:
Vishal Tripathi, Graphic Era University, IndiaReviewed by:
Amanuel Zenebe Abraha, Mekelle University, EthiopiaVijay Laxmi Trivedi, Hemwati Nandan Bahuguna Garhwal University, India
Copyright © 2024 Kassawmar, Tadesse, Desta, Bantider, Teferi, Bewket, Abraha, Zeleke, Walsh and O’Donnell. This is an open-access article distributed under the terms of the Creative Commons Attribution License (CC BY). The use, distribution or reproduction in other forums is permitted, provided the original author(s) and the copyright owner(s) are credited and that the original publication in this journal is cited, in accordance with accepted academic practice. No use, distribution or reproduction is permitted which does not comply with these terms.
*Correspondence: Tibebu Kassawmar, dGliZWJ1Lmthc3Nhd21hckBhYXUuZWR1LmV0; Claire L. Walsh, Q2xhaXJlLldhbHNoQG5jbC5hYy51aw==