- 1ICAR-Central Institute of Brackishwater Aquaculture, Chennai, Tamil Nadu, India
- 2ICAR-Central Institute of Freshwater Aquaculture, Bhubaneshwar, Odisha, India
- 3Faculty of Fishery Sciences, West Bengal University of Animal and Fishery Sciences, Kolkata, India
- 4College of Fisheries Sciences, Kamdhenu University, Gandhinagar, Gujarat, India
- 5ICAR-Central Marine Fisheries Research Institute, Kochi, Kerala, India
- 6ICAR-National Bureau of Fish Genetic Resources, Lucknow, Uttar Pradesh, India
- 7ICAR-Central Inland Fisheries Research Institute, Barrackpore, West Bengal, India
- 8Agricultural Development and Rural Transformation Centre, Institute for Social and Economic Change, Bengaluru, India
- 9ICAR-Central Institute of Fisheries Education, Mumbai, Maharashtra, India
- 10Regional Center of Central Institute of Freshwater Aquaculture, Vijayawada, Andhra Pradesh, India
- 11Mandapam Regional Centre, ICAR-Central Marine Fisheries Research Institute, Mandapam, Tamil Nadu, India
- 12Mangalore Regional Centre, ICAR-Central Marine Fisheries Research Institute, Mangalore, Karnataka, India
- 13Fisheries Science Division, Indian Council of Agricultural Research, New Delhi, India
Economic burden of diseases on Indian aquaculture sector was estimated to be US$ 2.48 B, 14.95% of annual aquaculture production value. Analysis revealed a higher cost of disease (US$ t−1) in shrimp (1,224.82) followed by marine fish (815.87), IMC+ (364.89), tilapia (260.34), IMC (200.70), and pangasius (pond 198.92; cage 168.36). The major contributors to the disease burden included production loss (23.90%), expenses on prophylactics (50.31%) and therapeutics (17.26%). The economic loss was dominated by diseases of multiple etiology (US$ 468.27 M), bacterial hemorrhagic septicemia (US$ 326.47 M), and epizootic ulcerative syndrome (US$ 88.12 M) in finfish and by Enterocytozoon hepatopenaei infection (US$ 571.24 M) in shrimp. Multinomial logit regression identified farm size, water source and exchange, stocking biomass and feed type as the main determinants of disease. The study findings would assist in prioritizing resource allocation and developing intervention strategies at the national level for effective and targeted disease management.
Introduction
Foods of animal origin are critical for the nutritional security, health, and livelihoods of the growing world population. A recent EAT-Lancet Commission report identifies fish as a crucial source of essential micronutrients (Willett et al., 2019) and suggests its inclusion in food security programs to harness health benefits (Golden et al., 2021). Specifically, the report acknowledged that fish consumption could help to meet the nutritional requirements of populations in regions with limited access to other animal protein sources. Aquaculture is expected to fill the ever-increasing demand for aquatic animal proteins as global capture fisheries production is declining (FAO, 2016). Aquaculture is a major economic driver in many parts of the world, generating social and economic benefits for the producer and offering safe and high-quality animal protein for consumers. Indian fish production has grown over 8% during the last decade and stands second globally, with an annual production of 16.25 M tons contributing 1.1% to the national GDP (Figure 1) (FAO, 2022; Ministry of Fisheries, Animal Husbandry and Dairying, Government of India, 2022). Indian major carps (IMC), exotic and minor carps, tilapia and pangasius in freshwater and penaeid shrimp in brackishwater aquaculture were the major species farmed. Most of the freshwater produce is consumed domestically, while 0.71 M tons of farmed shrimp worth US$ 5.48 B is exported, contributing to 25% of the global shrimp trade (MPEDA, 2023). Aquatic animals are increasingly integrated into the global food supply system due to their popularity as healthy food, technological transformations in farm management, value chains and rising income in emerging economies (Miao and Lal, 2016). However, a recent report on global aquaculture developments over the past two decades identified diseases, suboptimal feed efficiency and climate change as major challenges to the sector's sustainable growth (Naylor et al., 2021). Diseases are expected to influence the future of global aquaculture production and supply, which will affect nutritional security and livelihood (Stentiford et al., 2012).
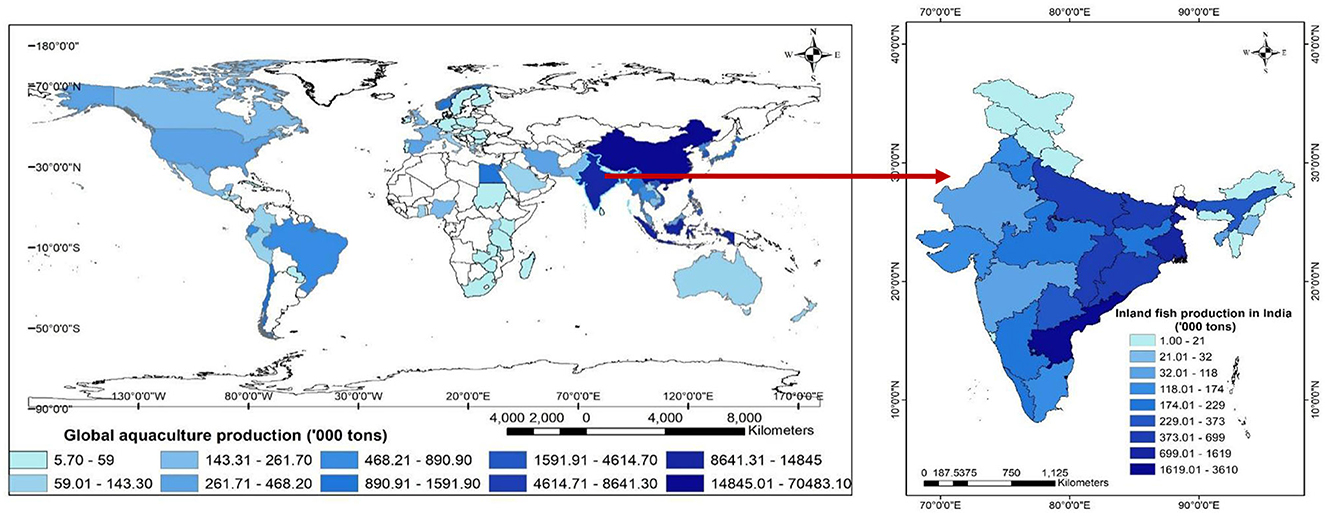
Figure 1. Global aquaculture production (in '000 tons). Aquaculture contributes nearly half of the global fish production worth US$ 263.6 B, dominated by Asian countries, China (66.13 Mt), Indonesia (14.77 Mt), India (7.07 Mt) and Vietnam (4.15 Mt) with a share of 89%. Global aquaculture production (122.6 Mt, 2020) is projected to increase by 21.7% by 2030 and is likely to double by 2050 (FAO, 2022).
Animal diseases cause a considerable burden on global nutritional security and economic sustainability, yet the availability of information on its influence on the socio-economic impact is limited (GBAD, 2022). The bio-economic methodologies of Global Burden of Animal Diseases (GBAD) program, an initiative of the World Organization for Animal Health (WOAH), suggested changing the allocation of resources depending on the socio-economic impact of given disease (GBAD, 2022) to improve economic sustainability. Despite ongoing efforts in farming practice and management to prevent and control diseases, they continue to cause substantial economic loss to the aquaculture industry globally (Naylor et al., 2021). Earlier studies have estimated the economic effects of diseases such as foot and mouth disease in terrestrial livestock sector at US$ 6 B annually (Knight-Jones and Rushton, 2013) and white spot syndrome virus exceeding the 40% of the global capacity for shrimp industry. Similar economic research is required in Indian aquaculture to assess the magnitude of disease problems and economically efficient policies and regulations appropriate to reduce the losses. Limited studies have published using of bio-economic models to investigate the impact of diseases on national production, market price and international trade of fish and fishery products. Early studies have estimated loss of CAD 0.56 kg−1 salmon due to sea lice infestation to Canadian (Mustafa et al., 2001) and USD 436 M to the Norwegian salmon industry (Abolofia et al., 2017), annual loss of USD 84 M to the Brazilian aquaculture (Tavares-Dias and Martins, 2017) and USD 16.9 M to the Mississippi catfish industry (Peterman and Posadas, 2019). Similar studies estimated the loss of USD 800 M to Vietnamese shrimp industry in 2011 and drastic reduction of production in Taiwan (Brummett et al., 2014) due to AHPND alone. In India, foot and mouth disease in domestic animals caused an estimated annual loss of US$ 2.05–2.39 B during 2006–2010. The loss due to morbidity was 97.64% which included 49.83% due to loss of milk while 16.15% was the opportunity cost and 12.20% due to growth reduction (Singh et al., 2013). Similarly, a study reported the estimated economic loss due to brucellosis (US$ 3.4 B) mainly in cattle and buffalo (95.6%) (Singh et al., 2015). Further loss at level of individual animal for cattle (US$ 6.8), buffalo (US$18.2), sheep (US$ 0.7), goat (US$ 0.5) and pig (US$ 0.6) suggested the need for prioritizing the control of these diseases. The limited investigations have been reported using bio-economic models to assess the impact of diseases on national production, market price and international trade of fish and fishery products to different countries. Previous reports indicated that Argulosis alone caused a loss of US$ 615 ha−1 to Indian carp farms (Sahoo et al., 2013). Also, economic loss due to diseases in pond-based fish farming of North East India totalled US$ 437 ha−1 (Debnath et al., 2019). In shrimp, the estimated loss due to diseases stands at US$ 1.02 B (Patil et al., 2021) of which US$ 813 t−1 was caused by Enterocytozoon hepatopenaei (EHP) alone (Geetha et al., 2022). A nationwide investigation is required to assess the magnitude of the disease burden to the Indian aquaculture sector which will assist in formulating efficient policies and regulations to reduce economic losses promoting sustainable production.
The direct cost of disease includes loss of stock and expenditure toward prophylactic and therapeutic measures, while indirect cost may include employment lost in upper (hatcheries, nursery, feed mills, chemical and healthcare manufacture, etc.) and lower (processing, marketing, exports, etc.) production lines, contributing significant loss to the exchequer. This study aims to assess direct cost of diseases in Indian aquaculture. For the purpose of this study economically important aquatic animal species farmed in freshwater ponds (Indian major carps and other minor carps, tilapia, pangasius, etc.), reservoir cages (pangasius), marine cages and brackishwater ponds (peneaid shrimp) were included. Generally, the sample size for each species were proportional to their contribution to national fish production (Figure 2). The information from the econometric analysis ensures improved fish production and supply through development of intervention strategies, policies, and resource allocation.
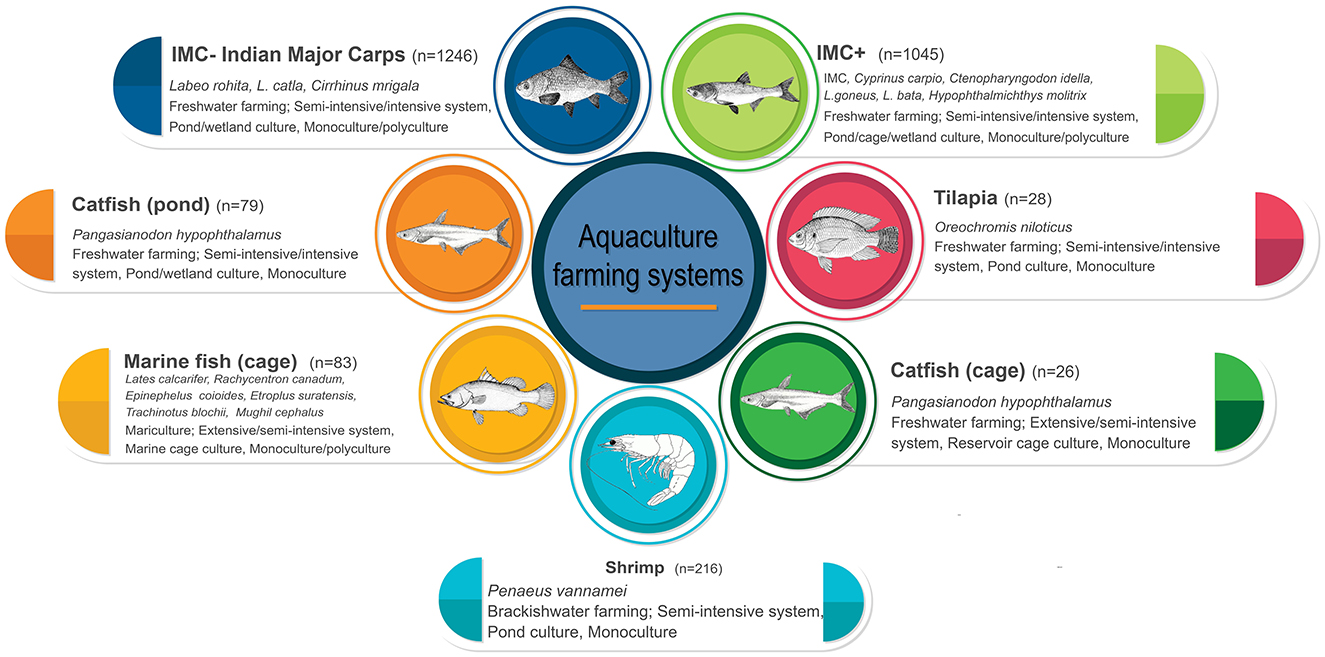
Figure 2. Schematic representation showing the classification of farm groups and farm types. Values in parenthesis indicate number of farms.
Methods
Source of data
A questionnaire-based survey was conducted from April 2021 to March 2022, through personal interviews of 2,723 farmers/farm managers in major fish farming states of India, which contribute 80.62% to the national aquaculture production. Based on the farming system and the species cultured, the farms were categorized as IMC (n = 1,246), IMC+ (n = 1,045), pangasius pond (n = 79), pangasius cage (n = 26 batteries), tilapia (n = 28), marine fish cages (n = 83 batteries) and shrimp (n = 216) (Figure 2). Each farm had more than one production unit, such as ponds, tanks or batteries of cages owned/operated by a farmer. IMC and IMC+ have been categorized together as IMC & IMC+ for loss estimation at the national level. These interviews were intended to collect key information on farming practices, disease occurrence, health management practices and cost of production, including the expenditures on account of prophylactic and therapeutic measures. Also, socio-economic, farm and production characteristics were collected using the triangulation approach (simple random sampling, multistage stratified random sampling and purposive sampling) as it incorporates multiple data sources, enhancing the validity and reliability of collected data (Carter et al., 2014; Sciberras and Dingli, 2023). Importantly, qualified researchers recorded the occurrence of a specific disease(s) based on the symptoms described by farmers and laboratory investigations. Data from the farms located within the disease affected region but not experiencing the disease were used to set baseline production data for healthy farms. Additionally, the average farm gate price for the fish/shrimp species in the region was used to estimate the total income from the culture operation.
Economic loss due to diseases in aquaculture (ELDA)
A model framework ELDA was developed following stakeholder consultation, farming systems practiced and economically important species of fish and their major diseases as the basic requirements (Figure 3A). A survey was conducted as described in the previous section to collect the data. The collected data was processed and verified for possible anomalies. Variables were categorized from the metadata and subjected to descriptive and econometric analysis. The frequency of disease and economic burden at the farm and national level were calculated using a spreadsheet method. Stochastic frontier model and multinomial logit regression (Figure 3B) were used to identify technical inefficiency determinants and relative risk ratio, respectively. The output from the analysis was computed and a final report on economic loss estimation was prepared based on the farming system and disease information pertaining to the region/country. The employment loss was calculated based on the reduction in duration of culture, and the proportionate man-days lost and the average daily wages in the concerned region.
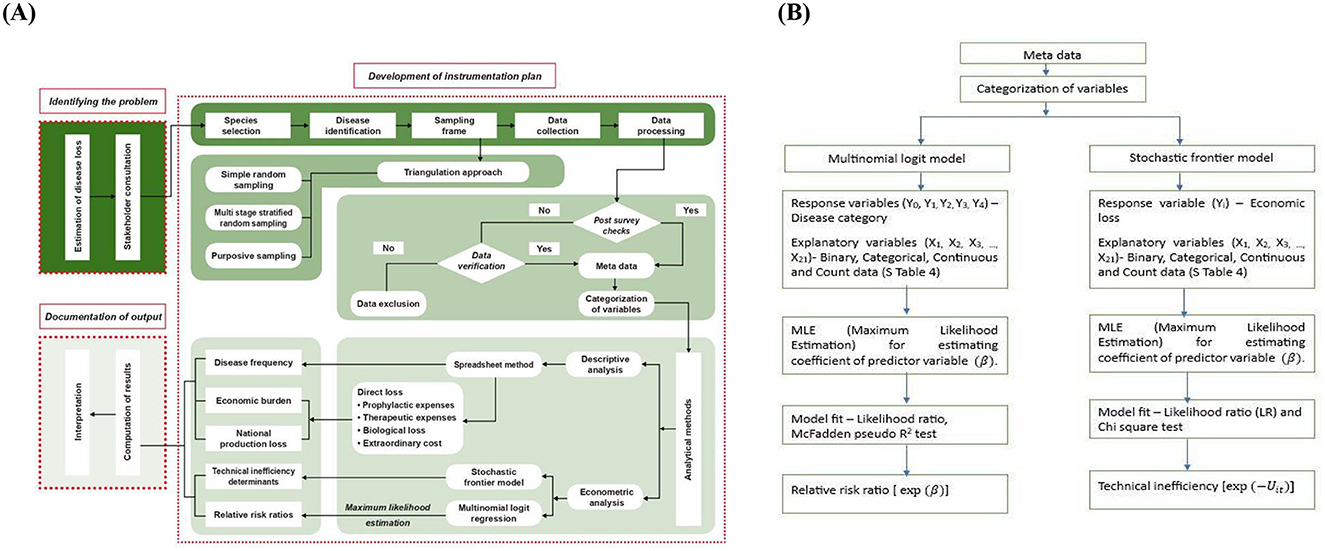
Figure 3. (A) Schematic representation of the proposed ELDA (Economic Loss due to Diseases in Aquaculture) framework. ELDA was used to estimate the economic burden of aquatic animal diseases at regional and national levels. STAGE 1: Identifying the problem through stakeholder consultation to understand their perspectives, needs, and interests. STAGE 2: Instrumentation plan consists of species selection, disease identification, sampling frame, data collection, processing, and post-survey verification, followed by statistical analysis. The model summarizes key characteristics using a spreadsheet approach to identify trends and patterns. (B) The framework assesses the economic burden based on the disease frequency and the estimated direct loss. Further, relative risk ratios for each disease are ascertained, and the source of technical inefficiency is identified. STAGE 3: The documented results determine the significance and relevance of the research suggesting strategic policies to improve farm efficiency by reducing disease incidence.
Disease frequency
Fish showing abnormal swimming and feeding behavior was suspected of having disease and, a presumptive diagnosis was made based on antemortem examination of the specimens. Infectious diseases were suspected when fish were lethargic, anorectic with signs of hemorrhage on body surface and mortality. Similarly, parasitic infestation was suspected when fish showed scratching behavior, surfacing or erratic swimming and poor growth rate. Water quality parameters were considered for categorizing the environmental conditions (Supplementary Table S1). Tissue samples from representative cases from different farming groups were confirmed by specific laboratory tests. Based on the etiology, the diseases reported in the study were classified as bacterial, fungal, parasitic, viral, and altered environmental conditions.
Estimation of direct loss
The cost of production (US$ t−1) was estimated as a function of expenses on pond preparation, seed, feed, labor, consultation, power, and cost of disease. Here, loss incurred in affected farms due to reduced production, expenses on prophylactics and therapeutics and the additional manpower were considered for estimating the cost of disease. Healthcare products used by farmers for prevention and control of diseases were categorized as disinfectants, environmental modifiers, probiotics, immunostimulants, nutritional supplements, natural antimicrobial agents, antibiotics, antifungal, anti-parasitic agents, and traditional formulations (Supplementary Table S2), and their proportional expenses to total expenditure was calculated. The biological loss was calculated based on the difference in the biomass harvested in healthy and affected farms. The extraordinary cost (compensatory stocking, laboratory, and consultancy charges) and additional labor cost were also included while estimating the loss.
The loss of production for the disease categories at the national level was estimated using disease frequency and national area under farming and production data for a given species of fish (FAO, 2022; Ministry of Fisheries, Animal Husbandry and Dairying, Government of India, 2022; MPEDA, 2023). In addition, percentage production loss due to a specific disease at national level was estimated. Total loss due to the disease under different cost categories for each of the species coupled with its correction factor (2.5 crops per year for shrimp) was used to calculate total economic loss due to aquatic animal diseases to Indian aquaculture.
Estimation of relative risk ratios
The relative risk ratio of disease occurrence and the relationship between the cause-and-effect variables were analyzed using a multinomial logit regression model. The dependent variable is categorized and indexed as occurrence of bacterial (1), fungal, viral, or parasitic (2), environmental (3), multiple etiology diseases (4), and healthy farms (0) (Supplementary Table S3). For analysis, healthy farms were used as a reference category. Fitness of the model was assessed by information criterion, likelihood ratio tests and McFadden pseudo R2 using IBM SPSS 22.0. The relative risk ratios [exp (β)] and the likelihood of the farms to be in certain disease category was estimated using probabilistic equations obtained from the final model (Supplementary Information 1).
Estimation of technical inefficiency
Maximum likelihood estimation (MLE) from the stochastic frontier approach (SFA) was used to assess the factors like diseases that cause economic loss, which affects the efficiency of the farm.
where, Yi is the economic loss ( t−1); Xi are the independent variables; εi is the error term of the model (ε = v – u) “u” signifies a technical inefficiency error and “v” represents random error; β0 is the intercept of the model; and βi are the parameters (Supplementary Information 2).
Statistical methods and software packages
All statistical analyses were performed with SPSS 22.0 (IBM, New York, USA) and Stata 16 (Texas, USA). The relative risk ratios through multinomial logit model were estimated using the STATA, and technical inefficiency through stochastic frontier approach using SPSS. The descriptive statistics were performed on farm characteristics, production parameters, and frequency distribution of diseases among different species and their impact on production. To assess the impact of farm characteristics on the health of cultured organisms, Chi-square (χ2) test of independence was used to determine the relationship between pairs of categorical variables. The relationship between major quantitative variables was analyzed using Pearson's correlation coefficient. Data on disease frequency, expenditure, economic loss and distribution of technical inefficiency were visualized using Datawrapper (2023). Similarly, the heatmaps depicting disease loss percentage to the cost and national aquaculture production were prepared using Displayr (Pyrmont, Australia) and Proportionate economic loss using Flourish (London, UK). Correlation plots and heatmaps for economic loss due to individual diseases were prepared using R studio (version 2022.07) packages such as corrplot and seriation.
Verification of the projected economic burden due to diseases and data availability
The results obtained from the ELDA framework underwent a thorough examination and were reviewed through stakeholder consultation to ensure their fineness and dependability. Stakeholder involvement not only adds depth and context to the findings but also serves as a vital mechanism to cross-reference the data and interpretations. The datasets generated and/or analyzed during the study are available from corresponding author on request.
Results
Farm and socio-economic characteristics and health status
The current comprehensive study surveyed a variety of aquaculture farms (ponds, tanks, lakes, and marine and freshwater cages) across the country that cultivate diverse finfish species and shrimp through monoculture or polyculture. Farm size significantly (p < 0.05) influenced the health status of IMC+ farms, where majority of the affected farms were small. Practice of bottom soil drying during pond preparation, regular water exchange, and aeration during farming significantly reduced the disease occurrence in most of the farm groups studied. Perennial farms and farms that sourced canal water were more prone to diseases (Supplementary Tables S4, S5). Further, a strong correlation was observed between the health status and farm characteristics such as stocking biomass, culture period, FCR in IMC and IMC+ farms, productivity in IMC, pangasius and tilapia farms, and FCR in marine fish (cage). For instance, short culture period, higher FCR, and lower farm gate prices were reported from affected shrimp farms (Supplementary Table S6), demonstrating the stronger link between these factors. The educational qualification of the farmers was found to be significantly effective in disease management in most of the farm groups. However, significantly higher number of the farmers of affected farms seems to avail technical help. Additionally, Pearson correlation analysis revealed a positive association of biological loss (finfish 86%, shrimp 95%), cost of treatment (finfish 50%, shrimp 30%), and FCR (shrimp 25%) with economic loss (Supplementary Figure S1, Supplementary Table S5).
Types and frequency of disease occurrence
Among the farming systems, bacterial infections were dominant in pangasius (cage) (38.46%), followed by marine fishes in cages (28.92%), IMC (25.84%), IMC+ (10.43%) and tilapia (7.14%) farms. Notably, 39.35% of shrimp farms were affected by Enterocytozoon hepatopenaei (EHP), while 33.7 % and 18.07% marine fish cage were infested with parasites and altered environmental conditions, respectively. However, co-infections were prominently recorded in the IMC & IMC+ farms (Figure 4A).
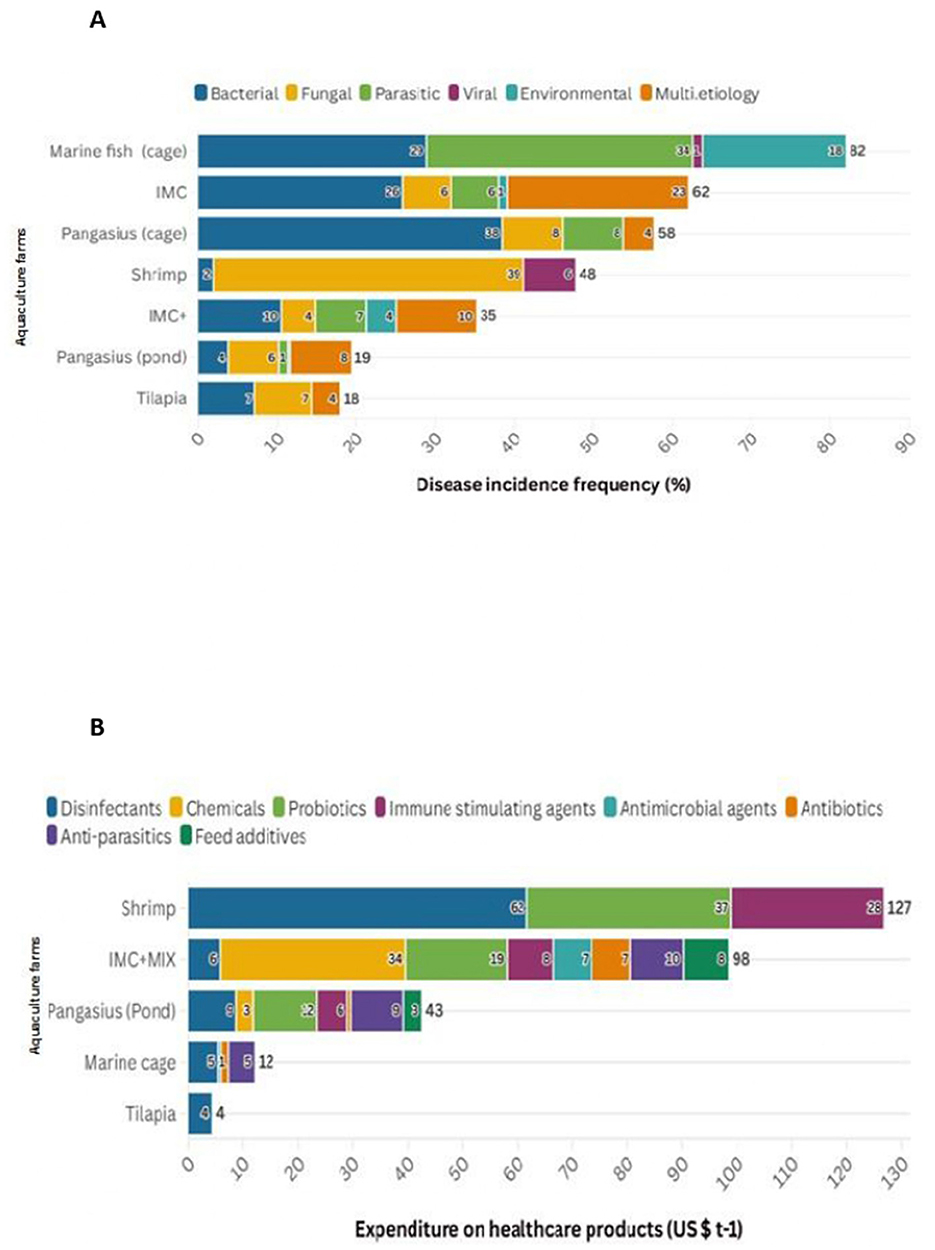
Figure 4. Estimated disease frequency (%) and estimated costs to manage multiple diseases (US$ t−1) across different farms. (A) The study observed a higher incidence of disease conditions in marine fish (cage) (81.89%), followed by IMC (62.03%), pangasius (cage) (54.55%), shrimp farms (47.68%) and IMC+ farms (35.13%), whereas lower incidences were recorded in pangasius (pond) (19.26%) and tilapia (17.85%) farms. (B) Major expenditures on healthcare products in different farms. IMC farms, probiotics (28.16%) followed by nutritional supplements (20.11%), and anti-parasitic agents (16.76%); Shrimp farms, disinfectants (48.69%), probiotics (29.29%), and immunostimulants (22.06%); pangasius (pond) farms, probiotics (27.39%), anti-parasitic agents (22.21%), disinfectants (20.71%), immunostimulants (13.01%); marine fish (cage), disinfectants (44.94%), anti-parasitic agents (40.11%), and antibiotics (10.91%).
Determinants of disease occurrence
A set of explanatory variables (finfish 18, shrimp 15) (Supplementary Table S3) were used to project the likelihood of a farm experiencing bacterial (finfish 18.2%, shrimp 1.9%), fungal, viral and parasitic (finfish 11.3%, shrimp 39.4%), environmental (finfish 2.2%, shrimp 6.5%), and multiple etiology (finfish 16.6%) diseases. The model-fitting results confirm the significant influence of independent variables on the occurrence of diseases (p < 0.05). The values of AIC (Akaike Information Criterion), BIC (Bayesian Information Criterion), likelihood tests, and McFadden R2 (finfish 0.264, shrimp 0.338) indicate the goodness-of-fit of the developed model. The likelihood ratio tests identified the significantly influencing variables on disease occurrence (p ≤ 0.05) (Supplementary Information 1).
The relative risk ratios [with 95% Confidence interval] revealed that the feed type 1.67 [1.10–2.52] times, excessive feeding 6.40 [4.47–9.16] times, farms not practicing water exchange 3.67 [2.21–6.09] times, and not using aerators 2.67 [1.01–7.01] times significantly increase the chances of bacterial infection in finfish farms. Further, the incidence of bacterial infection reduced with farm size (medium, 30.5%) and source of water (borewell, 82.1%; rain, 68.5%; and river, 43.9%). The chances of outbreak of fungal, viral and parasitic disease are increased by 59.9% when wet feed is used. Similarly, excessive feeding 4.14 [2.45–6.24] times and lack of aeration 4.84 [1.13–20.66] times increased the probabilities. Contrastingly, water from well and river reduce the fungal, viral and parasitic disease occurrence by 70% and 61.5%, respectively. Moreover, excessive feeding multiplies the chances of disease occurrence due to environmental causes by 3.42 [1.33–8.76] times. Large farm size 1.73 [0.46–2.44] times, lack of water exchange 1.86 [0.89–3.84] times, feed types- wet 3.82 [2.01–5.59] times; wet and pellet 2.08 [1.37–3.14] times, and natural 3.18 [1.33–7.59] times, and excessive feeding 7.98 [5.56–11.44] times increased disease occurrence caused by multiple etiological factors, whereas source of water (borewell, 77.6% and rain, 65.1%) reduced the disease occurrence caused by multiple etiological factors (Supplementary Table S7A). Analysis of data from shrimp farms revealed that an increase in stocking density multiplies the incidence of EHP and WSSV by 9.34 [0.95–92] times. Similarly, large farms 52.92 [3.36–833.18] times and lack of water exchange 7.22 [0.81–64.23] times contributes to poor water quality parameters (Supplementary Table S7B).
Expenditure on prophylactics and therapeutics
Prophylactic application of environmental modifiers amounting to US$ 13.23 t−1 in IMC and immunostimulants amounting to US$ 26.63 t−1 in IMC+ were effective in reducing the incidence of diseases. In pangasius (pond), environmental modifiers worth US$ 5.41 t−1, antiparasitic drug worth US$ 5.41 t−1, and nutritional supplements worth US$ 7.63 t−1) was helpful in managing disease occurrence. Similarly, environmental modifiers worth US$ 17.93 t−1, probiotics worth US$ 7.33 t−1, and nutritional supplements worth US$ 9.05 t−1 is spent to control disease outbreak in tilapia farms. Notably, in shrimp farms, higher expenditure on environmental modifiers (US$ 129.79 t−1) (Supplementary Figure S2) is useful in reducing the occurrence of than other products. Among the affected farms, IMC+ farmers spent (US$ 142.78 t−1) the most on therapeutic usage of healthcare products, followed by shrimp (US$ 126.8 t−1), IMC (US$ 53.94 t−1), pangasius (US$ 42.52 t−1), marine cages (US$ 12.08 t−1), and tilapia (US$ 4.29 t−1) farmers (Figure 4B). The expenditure on therapeutics to the production cost was higher in IMC+ farms (8.04%), followed by pangasius (pond) (3.25%), IMC (3.16%), shrimp (2.94%), marine cage (0.22%), and tilapia (0.27%) farmers. Further, the use of healthcare products in freshwater and marine cage farming for prophylactic or therapeutic purposes was negligible.
Disease loss to total cost of production
In any culture systems, including aquaculture, losses due to disease significantly increase cost of production. The biological loss was the highest in shrimp (15.83%), followed by tilapia (15.72%), pangasius (cage) (13.54%), and pangasius (pond) (9.45%) whereas prophylactic expenses were major cost in IMC+ (9.63%) and IMC (4.06%) farms. Interestingly, extraordinary cost (9.26%) in terms of compensatory stocking constituted a major expense in marine cage (Figure 5A). Additionally, biological loss in IMC (3.40%) and marine cage (4.50%); cost of disease management in IMC+ (8.04%) and pangasius (pond) (3.25%); prophylactic expenses in shrimp (9.02%) and pangasius (cage) (0.24%) also constituted substantial portion in their cost of production.
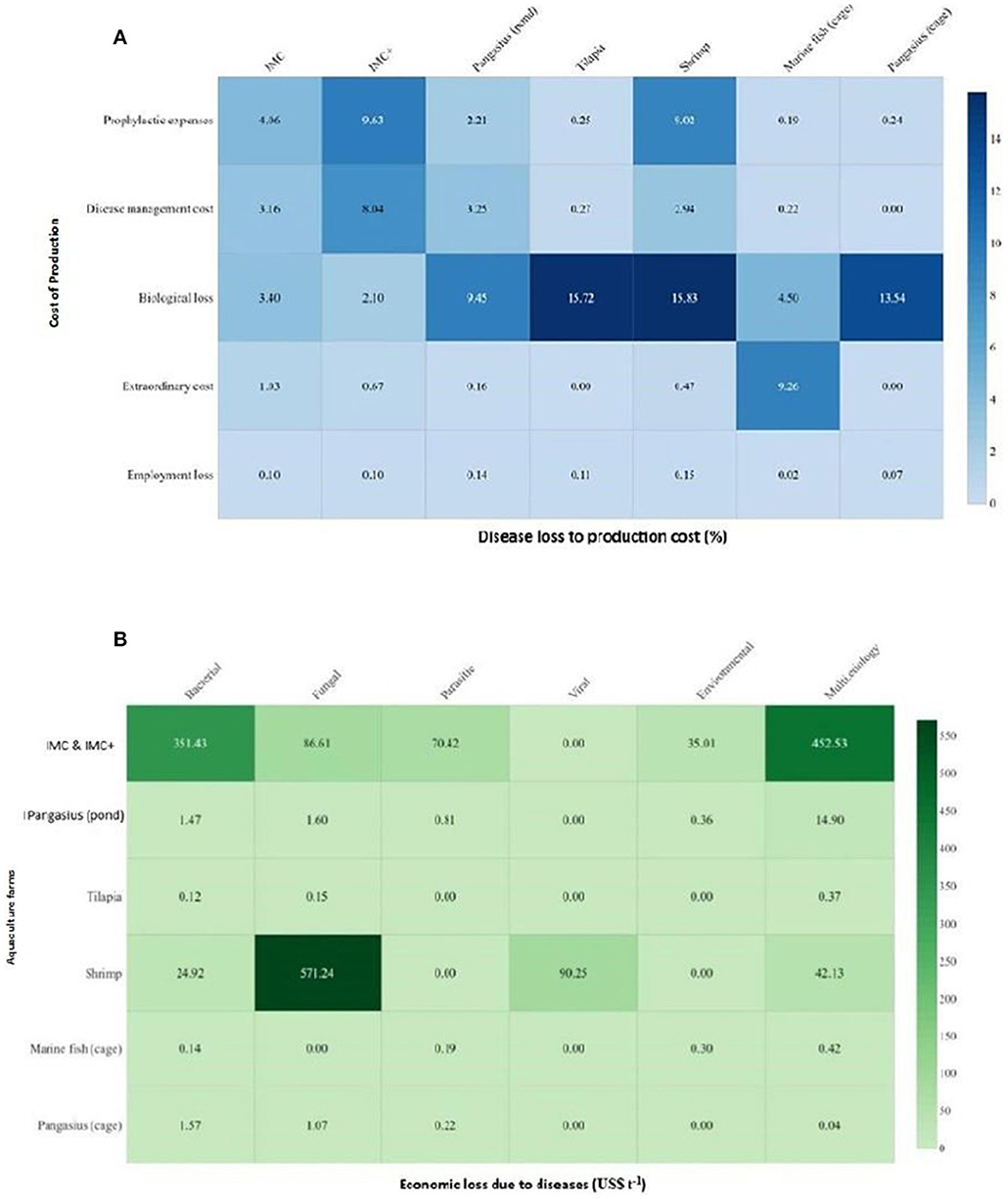
Figure 5. Estimation of economic loss in various farms based on disease type and production costs. (A) Estimated disease loss to production cost (%). The major components considered for estimation of cost of production include expenses on seed, feed, prophylactic and therapeutic measures, labor, and electricity in addition to pond preparation. Marine fish (cage) exhibit a higher cost of production (US$ 5,375.46 t−1) followed by shrimp (US$ 4,310.60 t−1), US$ 1,200–1,800 t−1 for carps, tilapia and pangasius (pond and cage) culture. (B) Estimated economic loss due to different diseases in commercially important fish species (US$ t−1). In carp farms, bacterial (92.48%), parasitic (98.05%), environmental (98.15%), and multiple etiology diseases (88.63%). In shrimp farms, EHP (86.6%) and WSSV (99.99%).
Technical inefficiency in disease management
The stochastic frontier cost function analysis in fish farms revealed large-sized farm reduces the economic loss by 0.37% in comparison to small sized farms; increasing the productivity reduces the economic loss by 0.22%; fishes having higher average body weight during disease resulted in 0.15% reduction in economic loss. Technical inefficiency scores with 95% confidence interval for the factors are given below. Increasing the stocking biomass 0.05% [0.01–0.09], higher cost of production 0.15% [−0.02–0.32], expenses on disinfectant 0.05% [0.03–0.06], probiotics 0.02% [0.03–0.15], immunostimulants 0.09% [0.06–0.11], higher biological loss 0.74% [0.68–0.81], higher therapeutic usage of disinfectant 0.10% [0.08–0.12], environmental modifiers 0.17% [0.15–0.18], probiotics 0.09% [0.06–0.11], natural antimicrobial agents 0.07% [0.05–0.07], antibiotics 0.07% [0.05–0.10], nutritional supplements 0.05% [0.02–0.08], and higher compensatory stocking 0.26% [0.22–0.3] results in increasing the economic loss (Supplementary Table S8). Majority of the pangasius (pond) (47%), IMC+ (40%) and IMC (34%) farms had low technical inefficiency. Nearly half of the IMC (57%) and tilapia (50%) farms had moderate technical inefficiency in disease management (Supplementary Figure S3). Interestingly, farm size seems to have little association with inefficiency scores across the farm groups.
Aquatic animal diseases of economic importance
The direct economic loss due to diseases in affected IMC & IMC+ farms is US$ 996.01 M, which includes loss from diseases of multiple etiology (US$ 452.53 M, 45.43%), followed by bacterial (US$ 351.43 M, 35.28%), fungal (US$ 86.61 M, 8.70%) infections, parasitic infestations (US$ 70.42 M, 7.07%) and altered environmental conditions (US$ 35.01 M, 3.52%). Similarly, the loss in affected pangasius (pond) farms is US$ 19.15 M, which includes diseases of multiple etiology (US$ 14.90 M, 77.82%), followed by fungal (US$ 1.60 M, 8.38%) and bacterial infections (US$ 1.47 M, 7.70%). In tilapia farms, bacterial (US$ 0.12 M, 19.59%) and fungal infections (US$ 0.15 M, 22.79%) were prominent. Economic loss due to diseases in marine fish cage farms loss stands at US$ 1.06 M, which includes loss from mixed infections (US$ 0.42 M, 39.58%), environmental alterations (US$ 0.30 M, 28.26%), parasitic (US$ 0.19 M, 18.18%), and bacterial infections (US$ 0.14 M, 13.55%) while the major contributors of disease loss in freshwater cage (US$ 2.90 M) were bacterial (US$ 1.57 M, 54.13%), fungal (US$ 1.07 M, 36.90%) and parasitic (US$ 0.22 M, 7.43%) infections. Economic loss in shrimp farms is US$ 728.55 M due to EHP, which contributes 78.41% worth US$ 571.24 M and WSSV contributes 12.39% worth US$ 90.25 M, 12.39%, (Figure 5B). The economic impact of the different disease conditions varied among the farm groups. Here, Bacterial Haemorrhagic Septicaemia (BHS) in IMC & IMC+ (US$ 326.47 M, 92.89%), EHP in shrimp (US$ 571.24 M, 78.41%), Edwardsiellosis (US$ 1.57 M, 0.45%) in pangasius (cage), and altered environmental conditions in marine fish (cage) (US$ 0.30 M, 0.84%) were the serious disease conditions causing higher economic loss (Figure 6A, Table 1, and Supplementary Figure S4).
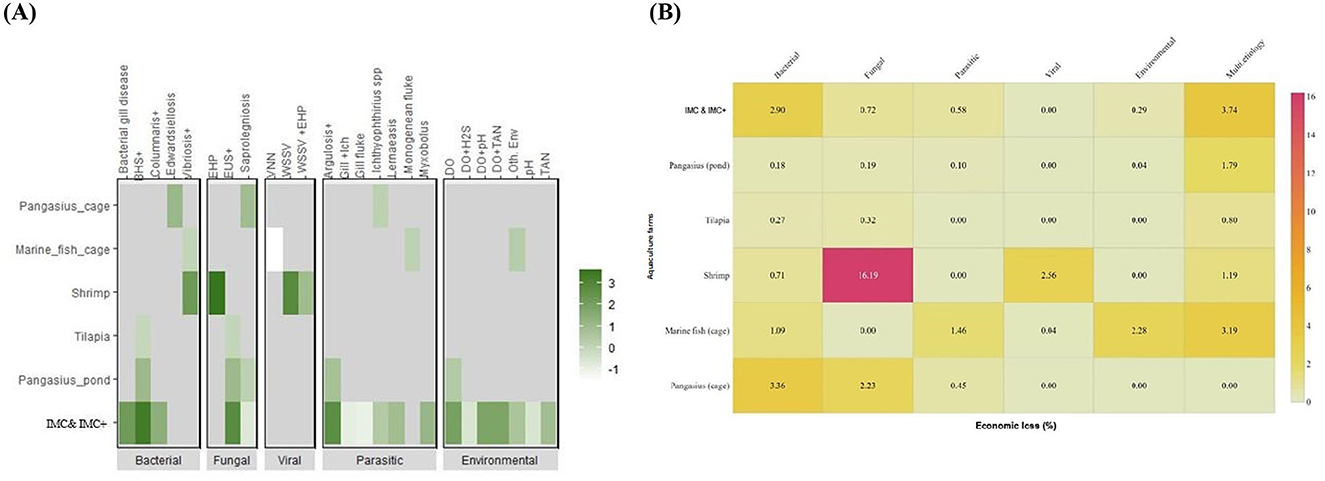
Figure 6. Contribution of different diseases in different farm groups to economic loss and joint estimation of loss to national aquaculture production. (A) The economic loss at the national level individual disease in the specified farm group. Frequency of disease occurrence, production and expenditures in affected farms were considered for the estimation. Log values of economic loss for each disease condition were plotted against the respective farm groups. (B) Estimated economic loss (%) to the aquaculture sector in India. National production data for IMC and IMC+, pangasius (pond and cage), tilapia, shrimp, and marine fish (cage) were considered for the estimation. Farm gate prices were used to calculate values at a national level.
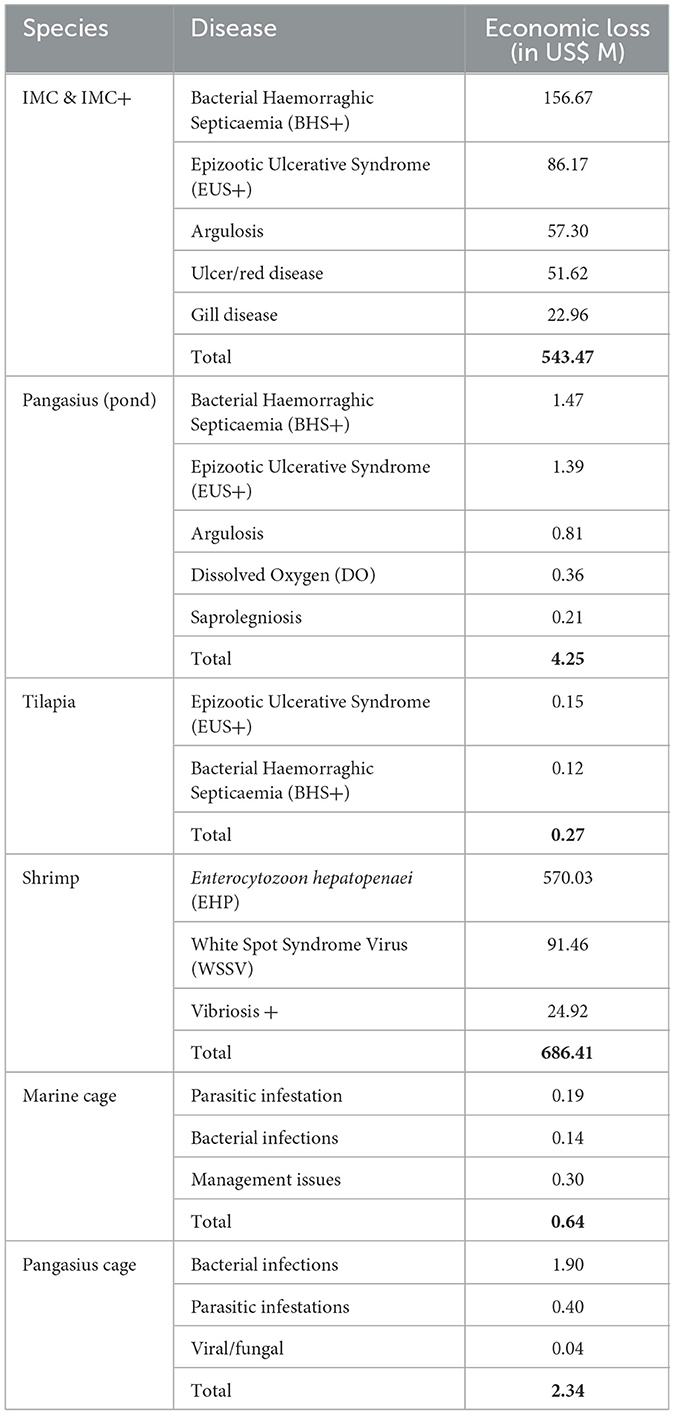
Table 1. Contribution of different major diseases in different farm groups to economic loss (US$ M).
Economic burden of diseases to Indian aquaculture
The IMC & IMC+ (US$ 12102.65 M), shrimp (US$ 3527.83 M), tilapia (US$ 46 M), pangasius (pond) (US$ 830.20 M), pangasius (cage) (US$ 48.01 M) and marine fish cage (US$ 13.12 M) largely contribute to the estimated national aquaculture fish production value (US$ 16.57 B) during April, 2019–March, 2020. Bacterial disease in IMC & IMC+ farms (2.90%) and pangasius (cage) (3.36%) and altered environmental conditions in marine fish cage (2.28%) caused major losses relative to the national production value, whereas individual diseases like EHP (16.19%) and WSSV (2.56%) were the major contributors of loss to the national shrimp production (Figure 6B).
Estimated overall loss due to disease per ton of production was highest in shrimp farms (US$ 1,224.82), followed by marine cage (US$ 815.87), IMC+ (US$ 364.89), tilapia (US$ 260.34), IMC (US$ 200.70), pangasius (pond) (US$ 198.92), and pangasius (cage) (US$ 168.36) (Figure 7A). Among the major cultured species, IMC & IMC+ farms incurred the highest loss of US$ 1.51 B (61.14%) followed by US$ 0.89 B (35.94%) in shrimp farms. Interestingly, farmers in healthy farms spent US$ 586.00 M for disease prevention and US$ 142.65 M as an extraordinary cost, which contributed to the overall burden of diseases to Indian aquaculture. The share of prophylactic expense in the cost of disease was major in IMC & IMC+ and shrimp farms (42–60%), followed by extraordinary cost in marine fish and biological loss in pangasius cage culture (Supplementary Figure S5). The overall direct loss due to aquatic animal diseases to Indian aquaculture is estimated to be US$ 2.48 B ( 183.04 B) (Figure 7B), which represent 14.95% of the national aquaculture production value.
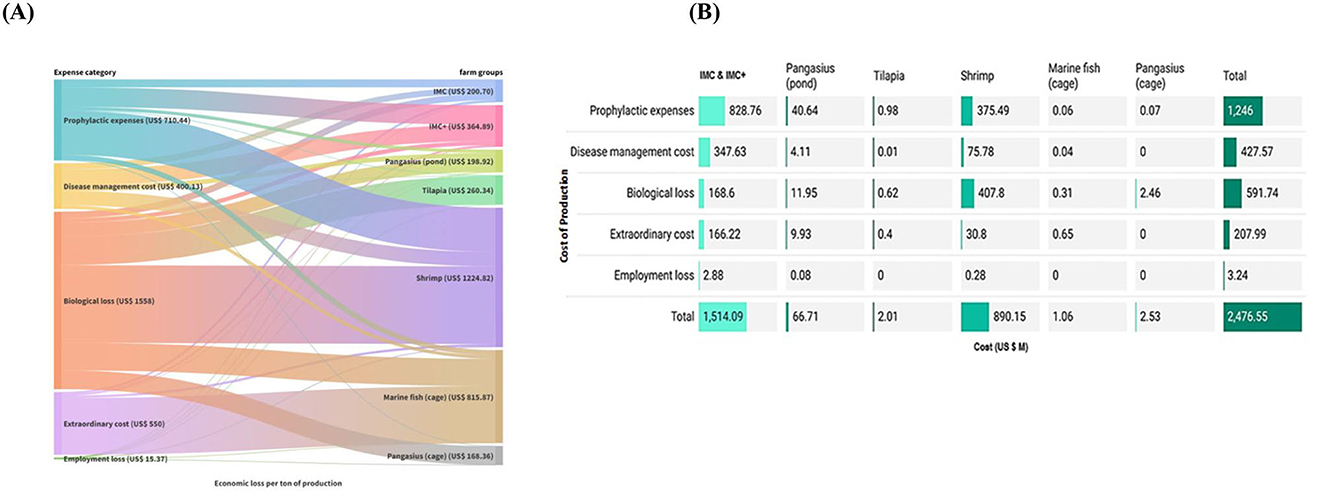
Figure 7. Economic burden of diseases and their influence on loss in different farms. (A) Economic loss per ton of production. Comparative analysis revealed that biological loss and prophylactic expenses dominated most farms, including shrimp farms. (B) Economic burden of diseases to Indian aquaculture (US$ M) was estimated using the prevalence of the disease in a particular region and preventive costs in healthy farms.
Projected economic burden and its alignment with the actual economic impact due to diseases
The estimated economic burden, arrived through meticulous analysis and modeling, provides valuable insights into the potential financial implications in multiple scenarios. The stakeholders discussion conducted following the analysis revealed commendable alignment between the estimated economic burden and the actual economic loss. In most cases, we observed a correlation up to 0.8, while the lowest observed figure was 0.55. This observation signifies the effectiveness of the ELDA framework, demonstrating its ability to capture the complex interplay of factors influencing the economic landscape. However, the data is not shown as these findings were exclusively derived from stakeholder discussions.
Discussion
Economic sustainability of food production involves adopting practices that assure long-term profitability and economic viability while minimizing negative environmental and social impacts. It is crucial to supply quality nutrients to the growing population in addition to employment generation, enhancing livelihoods, poverty alleviation and economic gains. Diseases impose yield-limiting effects and continue to be the primary cause of production and economic loss, adversely impacting the income of the producers and supply chain operators. Disease outbreaks have significant economic impacts on the aquaculture industry. Outbreaks led to direct losses from mortality and reduced productivity, as well as indirect losses due to trade restrictions and increased production costs. Thus, disease control measures, surveillance, and timely interventions are essential to minimize economic losses and maintain production sustainability.
GBAD, a comprehensive framework to characterize the animal population, the value of the investment and the models to capture net losses in production and expenditure on animal health management, was developed (Huntington et al., 2021). In line with Animal Health Loss Envelope (AHLE) (Torgerson and Shaw, 2021) concepts, the proposed ELDA framework evaluates the economic impact of diseases relative to the healthy population in the respective production systems. ELDA contemplates the loss of biomass, farm gate price and expenses on disease prevention and control, in addition to the cost of restocking, professional help and loss of employment. The methodology could be utilized in the GBAD programs and is expected to help the researchers to produce standardized and comparable information on the economic impact on various animal production sectors across different levels.
Farm characteristics and culture practices greatly influence the susceptibility and outcome of a disease outbreak. In this study, the water source, farm size, water exchange, and aeration significantly influenced the incidence of various disease conditions. Higher relative risk ratio in bacterial (6.40 times), fungal, viral and parasitic (4.14 times), environmental (3.42 times), and diseases of multiple etiology (7.98 times) demonstrated a strong relationship between disease occurrence and multiple farming factors, including overfeeding, frequency of water exchange, and duration of aeration, and showed that amendments in the farming practices help in reducing economic losses. Further, ELDA's effectiveness in projecting disease occurrence in new farms was demonstrated by its accurate classification of finfish (67.4%) and shrimp (68.1%) farms, showcasing its practical utility.
Diseases like BHS, columnaris, EUS, argulosis, dactylogyrus (Gill fluke) and caligus infestation in finfish farming and WSSV, EHP and vibriosis in shrimp farming threaten the economic sustainability of the Indian aquaculture sector (Patil et al., 2021). Frequent occurrence of bacterial diseases (BHS, edwardsiellosis, bacterial gill disease), parasitic infestations (Argulus spp., Ergasilus spp., Lernaea spp., monogenetic trematodes, Myxoosporeans), and fungal infections (Saprolegnia spp., EUS) lead to growth retardation, extensive mortalities, increased expenses on disease management. Collectively, these factors inflict adverse economic consequences on Indian fish farming sector (Kamilya and Baruah, 2014; Mishra et al., 2017; Mohanty and Sahoo, 2007; Swain et al., 2007). A recent survey reported the frequent occurrence of parasitic infections (53.03%) followed by bacterial diseases (27.27%), poor nutrition and environmental fluctuations (10.61%), and fungal diseases (9.09%) in Moyna area of West Bengal, India (Hoque et al., 2023). Similarly, present study recorded a higher incidence of bacterial, parasitic and infections of mixed origins nationally. These findings demonstrate the applicability of ELDA in projecting parameters that significantly contribute to economic loss over a large area.
Expenditure incurred to contain the infectious agents were identified as a major financial burden (Lafferty et al., 2015) on farming activity in addition to its social impact. In support, study results revealed higher prophylactic expenses, especially environmental modifiers, probiotics and nutritional supplements. Moreover, the majority of the non-drainable IMC farms continually accumulate organic waste which possibly led to poor environmental quality, resulting in higher use of disinfectants, environmental modifiers and herbicides (Patil et al., 2022a). Generally, aqua farms experience substantial economic losses primarily due to the higher prevalence of diseases. Confirming these facts, ELDA predicted that diseases like BHS, EUS, argulosis, and bacterial gill disease, cost US $ 990 M to the sector.
Globally, shrimp farms encounter serious challenges like reduced growth caused by EHP, acute mass mortalities by WSSV, mortalities at the early growth phase due to bacterial infections and water-soil quality issues (Thitamadee et al., 2015). Though no economic models were used, several previous reports over different period have predicted a loss of US$ 1 B due to diseases in Asian shrimp farming (Briggs et al., 2004), US$ 15 B worldwide over two decades (Lightner et al., 2012a), US$ 20 B over a decade in Asian countries (Dierberg and Kiattisimkul, 2018), US$ 11.58 B (2010–2016) in Thailand (Shinn et al., 2015), and US$ 6 B loss to global shrimp sector (Lightner et al., 2012b). Similarly, earlier studies from India estimated an annual disease loss of US$ 250 M (Kalaimani et al., 2013) (2006–2008) and US$ 1.02 B (Patil et al., 2021) (2018–2019) to Indian shrimp industry. Unlike previous reports, the proposed novel statistical framework, ELDA estimated the economic loss based on the primary data obtained from different aquaculture systems.
Previous studies reported a higher economic loss due to EHP despite the lower biological loss compared to other diseases (Geetha et al., 2022). Farms affected by EHP experience extended for feed and manpower, while acute mortality due to WSSV leads to the complete suspension crop duration due to growth retardation, resulting in increased expenditures of farming activity (Geetha et al., 2022). However, the economic loss due to EHP remained similar to our previous report (US$ 567 M) (Patil et al., 2021) despite its increase in frequency from 17% to 39%. This could be attributed to the effective management of disease through increased awareness among the farmers, amendments in farming practices (improved pond preparation, reduced stocking density, feed and harvest management) in addition to the availability of improved diagnostic services and commercial healthcare formulations containing phytochemicals, probiotics and other microbial products which offered considerable success. However, a significant drop in losses due to WSSV from US$ 238 M (Geetha et al., 2022) to US$ 90.25 M in the present study could be attributed to a decline in disease frequency from 25% to 6.48%. The National Surveillance Program on Aquatic Animal Disease (NSPAAD) of India reported a reduction in the prevalence of WSSV and an increased EHP occurrence (CIBA, 2022).
Farming of high value fish species like cobia, pompano and Asian seabass in marine cages is gaining popularity due to the increasing demand for seafood. The commonly reported conditions in Indian marine cage farming is associated with parasites like monogenetic flukes and lice (Ramudu et al., 2020), and vibriosis (Sharma et al., 2013). Recent report suggests similar observations indicating the dominance of parasitic (55%) and bacterial (31%) diseases in marine cages of East and Southeast Asian countries (Jahangiri et al., 2022). Similar to these observations, the study observed the dominance of parasitic infections in three-fourths of the marine cages. Though the most frequent disease conditions were parasitic infestations, farmers incurred the highest economic loss due to altered environmental parameters. Earlier studies reported a 30% reduction in production with an increased production cost of NOK 6 kg−1 due to pancreatic disease in Norwegian salmon (Aunsmo et al., 2009). Attributes like high stocking density, frequent introduction of naive fish hosts, homogenous host populations, rapid growth, and behavior of aggregation (Nowak, 2007) in addition to limitations in controlling exposure to planktonic organisms or net fouler favors parasite evolution in marine cages.
Changes in water quality play a significant role in the marine cage farming. The observed higher economic loss due to altered environmental conditions in the study could be attributed to higher incidences of opportunistic vibrio pathogens in warmer months. These observations were similar to the previous reports on cage cultured large yellow croaker (Liu et al., 2016) and Asian seabass (Sharma et al., 2013). Further, most Indian marine fish cages are located in the coastal waters and are subjected to sudden changes in physicochemical parameters like temperature, salinity, dissolved oxygen, and suspended particles due to changes in the weather conditions, greatly influencing the severity of disease conditions and consequent economic loss (Sobhana, 2009).
India, an agrarian country with several major and minor water projects, has close to 500 medium and large reservoirs. These reservoirs have a combined water spread area of 2.04 M ha of water spread area with potential to house 0.7 million cages for fish production. This enormous area has the capacity to produce substantial quantities of fish, generate employment, and promote rural economic activity (Ministry of Fisheries, Animal Husbandry and Dairying, Government of India, 2022). Similar to previous reports, over 50% of the surveyed reservoir cages reported the occurrence of diseases, including edwardsiellosis, saprolegniasis and ichthyophthiriasis. This emphasizes the necessity of continued efforts in promoting scientific disease management. Since cages are operated in medium and large reservoirs with pristine environments, suboptimal water quality was not found to be a cause of biological loss in the surveyed farms. Considering 18,000 cages in operation in the reservoirs, the overall loss due to diseases was estimated to be US$ 2.61 M to the freshwater cage culture sector of India.
Tilapia, the second major farmed fish globally, is an important source of income and nutrition for many, particularly in low-income food-deficit countries. Due to the inherent hardiness of the species, the disease incidence was relatively lower. However, several bacterial diseases have been reported globally in recent intensification activities, including India (Adikesavalu et al., 2017; Basri et al., 2020; Behera et al., 2022; Dong et al., 2015). Tilapia farmers mostly rely on disinfectants to manage the diseases, but the culture practice lacks effective therapeutic measures resulting in observed higher biological loss. Previous reports indicated an annual loss of US$ 150 M in 2000 due to S. iniae and S. agalactiae infections and US$ 250 M in 2008, representing ~5.7% and 6.7% of the total global value of tilapia, respectively (Klesius et al., 2008).
Stripped catfish P. hypophthalmus, introduced from Southeast Asia, is the most widely and intensively cultured pangasius in both seasonal and perennial ponds and tanks of India. Similar to other freshwater fish farms, BHS, EUS, argulosis and low dissolved oxygen were the economically important disease conditions. However, the intensity of saprolegniasis was higher in pond reared pangasius compared to the cages. Further, economic loss per ton of production was also low in pond reared pangasius compared to their cage culture. Environment issue was a minor contributor to disease loss, likely due to moderate tolerance of the species to suboptimal water quality conditions (Anka et al., 2014). The observed higher expenditures on prophylactic measures indicate awareness among the catfish farmers about disease prevention.
Stunted growth and reduced biomass due to EHP, mass mortality due to WSSV in shrimp and biological loss due to BHS, EUS, argulosis and oxygen depletion in IMC, pangasius (both in pond and cage) and tilapia were the major limiting factors observed in the study. The estimated loss per ton of production due to disease was higher for shrimp farms and marine fish cages due to high valued species than freshwater finfishes. Previous investigations estimated an annual loss of US$ 615.0 ha−1 in IMC & IMC+ farms due to argulosis which contributed to reduced growth (82%), mortality (8%) and cost of drug (10%) (Sahoo et al., 2013). Similarly, early reports indicated an economic loss of US$ 120 M due to bacterial septicemia (Qi, 2002) and US$ 1.73 M due to pancreatic disease (Aunsmo et al., 2009), while the sea lice infestation caused a loss of US$ 300 M to the global salmon industry (Costello, 2009) and US$ 436 M to the Norwegian aquaculture industry (Abolofia et al., 2017). Later studies have estimated an overall annual loss of US$ 84 M due to diseases in the Brazilian aquaculture sector (Tavares-Dias and Martins, 2017) and US$ 0.72 M to the Egyptian tilapia industry (Ali et al., 2020). In a similar study, the computed cost of salmon lice in the Norwegian salmon industry indicated that the loss in terms of forgone growth was higher than the cost of treatment (Abolofia et al., 2017). The present study for the first time estimated economic loss due to diseases in Indian aquaculture using statistical framework (ELDA) based on nationwide farm data.
In general, the occurrence of diseases could be controlled through the implementation of comprehensive disease control activities, including better management practices, stocking quality (PCR-tested negative seeds (especially in shrimp), reducing the stress by adjusting stocking densities and maintaining the farm environmental parameters within optimum ranges, and use of quality feed. The exponential growth of aquaculture could partially attribute to application of veterinary medicinal products and other chemicals for managing the health of cultured animals and the farm environment (Patil et al., 2022b). The study observed that the application of chemicals, in-feed administration of immunostimulants, natural antimicrobial agents, and nutritional supplements effectively reduced the disease incidence despite significant variations in their efficacy. The beneficial effects of probiotics in improving overall fish health and immunity have been extensively reviewed (Kumar et al., 2022; Nayak, 2020). Earlier, we reported the higher use of chemicals for maintaining optimal environmental quality and in-feed administration of probiotics, disinfectants, and nutritional supplements in Indian shrimp farming operations (Patil et al., 2022a,b). Additionally, stocking density, survival, and productivity play an important role in the occurrence of diseases (Patil et al., 2022a). These suggested guidelines help farmers in making decisions regarding production strategies, resource allocation, and market planning. Also, accurate predictions and prediction-based guidelines enhance farm efficiency, financial management on the use of inputs, risk assessment on disease loss and biological loss, increasing the profitability of farming.
Cage farming is done in natural waters, and released chemical substances might adversely affect the biodiversity of the surrounding environments. Thus, limited application of healthcare products is encouraged in Indian cage farming operations. Higher dependence on disinfectants and anti-parasitic agents in marine cages indicates a need for more awareness among the farmers on scientific disease management. Further, in the absence of specific treatment, shrimp farmers spent most on effective disease management through application of probiotics, disinfectants and immune stimulating agents (Chaijarasphong et al., 2020; Dey et al., 2019). However, the observed high variability between the expenditures on disease management and disease occurrence suggests the need for information on the scientific application of healthcare products across farming systems. Traditional knowledge, along with farmers experience, is of significant help in reducing disease occurrence.
Based on the national production data and the average farm gate price reported during the study period, the national aquaculture production could be estimated at US$ 16.57 B ( 1,224.53 B). The loss due to bacterial (2.29%), fungal (3.98%), viral (0.54%) and parasitic (0.43%) diseases in affected farms were estimated to be US$ 1.74 B, which is 10.55% of the India's aquaculture produce. Considering the cost of disease prevention in healthy farms (US$ 0.74 B), the overall disease burden to Indian aquaculture was estimated to be US$ 2.48 B. A similar study estimated an annual loss of US$ 6 B globally due to aquaculture diseases (World Bank, 2014), of which pancreatic diseases cost US$ 2.45 M in Norway (Aunsmo et al., 2009). A study from Bangladesh reported the loss due to mortality (11%), chemicals (11%) and reduction in growth (65%) in parasite infested IMC farms (Monir et al., 2015). Further, an average disease loss to the aquaculture was estimated to be US $ 0.3 kg−1, similar to the earlier estimate of US$ 0.46 kg−1 in sea lice infested Atlantic salmon farm (Abolofia et al., 2017). Shrimp farms incurred a major production cost loss of 28.41%, while the average loss in finfish farms was 15.93%. Earlier reports suggested a total loss of 40% of global capacity in shrimp (Israngkura and Sae-Hae, 2002) and 15–20% of Nepal fish production (Gurung, 2011) due to infectious diseases, and 5.8–16.5% of UK aquaculture due to parasitic infestations (Shinn et al., 2015) and 15% of global shrimp production due to WSSV (Stentiford et al., 2012). Further, financial burden due to disease outbreaks could extend to future crops as farmers are likely to reduce stocking densities, especially in shrimp farms and spend more on pond preparation and disease prevention in the subsequent farming cycles.
Transboundary movement of pathogens, veterinary drug residues and antimicrobial resistance have become the major non-trade barriers in international trade of animal foods. OIE Aquatic animal health code mandates the exporting country to provide the aquatic animal health certificate, information on aquatic animal health situation, and the efforts put to prevent and control the diseases (WOAH, 2022). Since India is one of the major exporters of fish and fishery products, restricted international market access could lead to significant indirect losses in addition to the estimated direct loss. Current efforts at the national level through enhanced funding for research and capacity building through training and dissemination of scientific health management practices would help reduce disease prevalence and the consequent economic loss. Further, institutional strengthening and capacity building, implementation of science-based risk analysis, epidemiology, surveillance, comprehensive disease management strategies, and planning for emergency response need to be emphasized to reduce disease burden. The country has a National Aquatic Animal Disease Surveillance Program (NSPAAD) (Bondad-Reantaso et al., 2021; Sood et al., 2021) implemented in 22 states in Phase I (2013–2022) and followed by Phase II as Pan-India plan (2022–2025). A recent proposal for a national policy through “Aquatic Animal Disease and Health Management Bill” is set to develop guidelines for effective containment of disease spread. Crop insurance is another area of development where the policies not only compensate the farmers for their losses but also will create an enabling environment for improved compliance with best aquaculture practices. Since IMC contribute the bulk of the domestic fish consumption and shrimp, a major export commodity, prioritized efforts are required to reduce the losses in farming these species. Using the data generated in the study, a comprehensive policy at the national level covering the major farming systems and economically important species of fish farmed in the country may be proposed. The policy interventions across the complete value chain at the appropriate stages will help in reducing the burden of diseases in aquaculture. Standard operating procedures could be established and implemented for site selection, quality seed production, scientific feeding practices, healthcare management and training the farmers and farm workers. Such policies with system and region-specific customization could be developed for other major fish production countries. In addition to policy making, the data generated would help in the advancement of the One Health Concept and the targeted UN Sustainable Development Goals (SDGs) of health, environment, hunger and poverty.
The guidelines for clinical diagnosis of the diseases at the farm level have been established by various research institutes for different cultured species of fishes. The farmers and farm workers are routinely trained and provided with atlas written in regional languages for identification of the symptoms to arrive at the preliminary diagnosis with reasonable accuracy. Since the diseases reported in the study are well established and distinctly identifiable, it is expected that farm level diagnosis could generally be considered in agreement with the laboratory confirmation to a great extent. Further, the estimated economic burden demonstrates a correlation of 0.55–0.8 with the actual economic loss. A correlation ranging from 0.55 to 0.8 is often considered more suitable for practical applicability due to its balance between strength and flexibility. This range signifies a moderate to strong relationship, providing valuable predictive power without being overly deterministic. Moreover, verbal communication with individuals for scientific data collection carries inherent limitations. Reliability of the proposed framework could be improved provided the availability of more accurate farm account statements. Thus, we believe that a correlation of ~0.7 is the best fit to estimate the economic burden due to diseases through the ELDA framework.
Conclusion
Diseases of multiple etiologies, BHS and EUS in finfish, and EHP in shrimp were the major economic constraints, while expenses on prophylactics, therapeutics, and biological loss of crop were the key components of economic burden to the Indian aquaculture. A novel framework, ELDA, is proposed to estimate the economic loss due to diseases in aquaculture systems. Based on predictions, reorienting the expenditure on prophylactic and therapeutic agents, awareness programs, quality diagnostic services, developing guidelines for the scientific use of healthcare products, and implementing targeted disease control programs could help reduce the losses. Farmers can address the specifically identified factors which have the maximum influence on the disease losses. Further efforts are necessary to address the identified determinants of the diseases to improve production, revenue, and profit from aquaculture, thereby strengthening food security.
Data availability statement
The original contributions presented in the study are included in the article/Supplementary material, further inquiries can be directed to the corresponding author.
Ethics statement
Ethical review and approval was not required for the study on human participants in accordance with the local legislation and institutional requirements. Written informed consent from the [patients/ participants OR patients/participants legal guardian/next of kin] was not required to participate in this study in accordance with the national legislation and the institutional requirements.
Author contributions
PKP: Conceptualization, Funding acquisition, Investigation, Writing – original draft. RG: Formal analysis, Methodology, Writing – review & editing. SSM: Resources, Writing – review & editing. TJA: Investigation, Resources, Writing – review & editing. HGS: Data curation, Resources, Writing – review & editing. SRKS: Resources, Writing – review & editing. PKPr: Investigation, Resources, Writing – review & editing. SKM: Investigation, Resources, Writing – review & editing. SA: Data curation, Validation, Writing – review & editing. DA: Formal analysis, Software, Writing – review & editing. KTF: Formal analysis, Software, Writing – review & editing. TNV: Funding acquisition, Visualization, Writing – review & editing. KP: Investigation, Resources, Writing – review & editing. AP: Investigation, Resources, Writing – review & editing. SAR: Investigation, Writing – review & editing. RS: Resources, Writing – review & editing. SNS: Resources, Writing – review & editing. RR: Investigation, Writing – review & editing. PR: Investigation, Writing – review & editing. RB: Investigation, Writing – review & editing. ST: Investigation, Writing – review & editing. AKD: Visualization, Writing – review & editing. MJ: Visualization, Writing – review & editing. PS: Methodology, Writing – review & editing. NKS: Resources, Writing – review & editing. JKJ: Funding acquisition, Writing – review & editing.
Funding
The author(s) declare that financial support was received for the research and/or publication of this article. This work was funded by Indian Council of Agriculture Research (ICAR) through All India Network Project on Fish Health (AINP_FH) Project Number: 1006916.
Acknowledgments
The authors acknowledge the All-Indian Network Project on Fish Health funded by the Indian Council of Agriculture Research (ICAR), Ministry of Agriculture and Farmer's Welfare, Government of India. The support and facilities provided by Directors of ICAR-CIBA, ICAR-CIFA, ICAR-CIFE, ICAR-CIFRI, ICAR-CMFRI, ICAR-NBFGR, Dean, College of Fisheries, KU, and Dean, Faculty of Fisheries, WBUAFS, was acknowledged.
Conflict of interest
The authors declare that the research was conducted in the absence of any commercial or financial relationships that could be construed as a potential conflict of interest.
The reviewer AS declared a shared affiliation with no collaboration, with the authors PKP, AP to the handling editor.
Publisher's note
All claims expressed in this article are solely those of the authors and do not necessarily represent those of their affiliated organizations, or those of the publisher, the editors and the reviewers. Any product that may be evaluated in this article, or claim that may be made by its manufacturer, is not guaranteed or endorsed by the publisher.
Supplementary material
The Supplementary Material for this article can be found online at: https://www.frontiersin.org/articles/10.3389/fsufs.2025.1480094/full#supplementary-material
References
Abolofia, J., Asche, F., and Wilen, J. E. (2017). The cost of lice: quantifying the impacts of parasitic sea lice on farmed salmon. Mar. Res. Econ. 32, 329–349. doi: 10.1086/691981
Adikesavalu, H., Banerjee, S., Patra, A., and Abraham, T. J. (2017). Meningoencephalitis in farmed monosex Nile tilapia (Oreochromis niloticus L.) caused by Streptococcus agalactiae. Arch. Pol. Fisher. 25, 187–200. doi: 10.1515/aopf-2017-0018
Ali, S. E., Jansen, M. D., Mohan, C. V., Delamare-Deboutteville, J., and Charo-Karisa, H. (2020). Key risk factors, farming practices and economic losses associated with tilapia mortality in Egypt. Aquaculture 527:735438. doi: 10.1016/j.aquaculture.2020.735438
Anka, I. Z., Faruk, M., Hasan, M., and Azad, M. (2014). Environmental Issues of Emerging Pangas (Pangasianodon hypophthalmus) Farming in Bangladesh. Progress. Agricult. 24, 159–170. doi: 10.3329/pa.v24i1-2.-2.19118
Aunsmo, A., Valle, P., Sandberg, M., Midtlyng, P. J., and Bruheim, T. (2009). Stochastic modelling of direct costs of pancreas disease (PD) in Norwegian farmed Atlantic salmon (Salmo salar L.). Prev. Vet. Med. 93, 233–241. doi: 10.1016/j.prevetmed.2009.10.001
Basri, L., Nor, R. M., Salleh, A., Yasin, I. S. M., Saad, M. Z., Rahaman, N. Y. A., et al. (2020). Co-Infections of Tilapia Lake Virus, Aeromonas hydrophila and Streptococcus agalactiae in Farmed Red Hybrid Tilapia. Animals (Basel) 10:2141. doi: 10.3390/ani10112141
Behera, B. K., Dhar, S., Kumar, V., Parida, P. K., Hoque, F., Parida, S. N., et al. (2022). Molecular approach and techniques used in the diagnosis of fish parasites. AATCC Rev. 34–54. doi: 10.58321/AATCCReview.2022.10.02.39
Bondad-Reantaso, M. G., Fejzic, N., MacKinnon, B., Huchzermeyer, D., Seric-Haracic, S., Mardones, F. O., et al. (2021). A 12-point checklist for surveillance of diseases of aquatic organisms: a novel approach to assist multidisciplinary teams in developing countries. Rev. Aquacult. 13, 1469–1487. doi: 10.1111/raq.12530
Briggs, M., Funge-Smith, S., Subasinghe, R., and Phillips, M. (2004). Introductions and movement of Penaeus vannamei and Penaeus stylirostris in Asia and the Pacific. RAP Publ. 10, 88.
Brummett, R. E., Alvial, A., Kibenge, F., Forster, J., Burgos, J. M., Ibarra, R., et al. (2014). Reducing Disease Risk in Aquaculture. Agriculture and Environmental Services Discussion Paper 9. World BANK REPORT NUMBER 88257-GLB (Washington, D.C.: The World Bank), 119.
Carter, N., Bryant-Lukosius, D., DiCenso, A., Blythe, J., and Neville, A. J. (2014). The use of triangulation in qualitative research. Oncol. Nurs. Forum 41, 545–547. doi: 10.1188/14.ONF.545-547
Chaijarasphong, T., Munkongwongsiri, N., Stentiford, G. D., Aldama-Cano, D. J., Thansa, K., Flegel, T. W., et al. (2020). The shrimp microsporidian Enterocytozoon hepatopenaei (EHP): Biology, pathology, diagnostics and control. J. Invertebr. Pathol. 186:107458. doi: 10.1016/j.jip.2020.107458
CIBA (2022). Annual Report 2021. Central Institute of Brackishwater Aquaculture annual Report. New Delhi: ICAR - Indian Agricultural Research Institute.
Costello, M. J. (2009). The global economic cost of sea lice to the salmonid farming industry. J. Fish Dis. 32, 115–118. doi: 10.1111/j.1365-2761.2008.01011.x
Datawrapper. (2023). Datawrapper - Create charts, maps, and tables. Datawrapper GmbH, Germany. Available online at : https://www.datawrapper.de/
Debnath, D., Manna, S. K., Devnath, D. J., Payeng, L. K., Das, N., Bandyopadhyay, C., et al. (2019). Assessment of economic loss due to fish diseases in Assam, India: Implications of farming practices. KRISHI Public. Data Inven. Reposit. 51, 146–154. doi: 10.47780/JIFSI.51.2.2019.106499
Dey, B., Dugassa, G., Hinzano, S., and Bossier, P. (2019). Causative agent, diagnosis and management of white spot disease in shrimp: A review. Rev. Aquacult. 12, 822–865. doi: 10.1111/raq.12352
Dierberg, F., and Kiattisimkul, W. (2018). Asian shrimp production and the economic costs of disease. Asian Fish. Sci. 31, 29–58. doi: 10.33997/j.afs.2018.31.S1.003
Dong, H. T., Nguyen, V. V., Le, H. D., Sangsuriya, P., Jitrakorn, S., Saksmerprome, V., et al. (2015). Naturally concurrent infections of bacterial and viral pathogens in disease outbreaks in cultured Nile tilapia (Oreochromis niloticus) farms. Aquaculture 448, 427–435. doi: 10.1016/j.aquaculture.2015.06.027
FAO (2022). The State of World Fisheries and Aquaculture 2022. FAOannual Reports 2022 (Rome: FAO), 266.
Geetha, R., Avunje, S., Solanki, H. G., Priyadharshini, R., Vinoth, S., Anand, P. R., et al. (2022). Farm-level economic cost of Enterocytozoon hepatopenaei (EHP) to Indian Penaeus vannamei shrimp farming. Aquaculture 548:737685. doi: 10.1016/j.aquaculture.2021.737685
Golden, C. D., Koehn, J. Z., Shepon, A., Passarelli, S., Free, C. M., Viana, D. F., et al. (2021). Aquatic foods to nourish nations. Nature 598, 315–320. doi: 10.1038/s41586-021-03917-1
Gurung, T. (2011). Proceedings of the 8th National Workshop on Livestock and Fisheries Research. Kathmandu: Nepal Agricultural Research Council, 1–9.
Hoque, F., Adhikari, S., Mandal, R. N., Hussan, A., Chattopadhyay, D., Paul, B. N., et al. (2023). A retrospective study on the incidence of fish diseases and use of therapeutants in aquaculture farms of Moyna, the ‘fisheries hub' of West Bengal, India. Explor. Anim. Med. Res. 12, 195–204. doi: 10.52635/eamr/12.2.195-204
Huntington, B., Bernardo, T. M., Bondad-Reantaso, M., Bruce, M., Devleesschauwer, B., Gilbert, W., et al. (2021). Global Burden of Animal Diseases: a novel approach to understanding and managing disease in livestock and aquaculture. Rev. Sci. Tech. 40, 567–584. doi: 10.20506/rst.40.2.3246
Israngkura, A., and Sae-Hae, S. A. (2002). Review of the Economic Impacts of Aquatic Animal Disease. FAO Fisheries Tech. Paper 2002, 53–286.
Jahangiri, L., MacKinnon, B., and St-Hilaire, S. (2022). Infectious diseases reported in warm-water marine fish cage culture in East and Southeast Asia—A systematic review. Aquac. Res. 53, 2081–2108. doi: 10.1111/are.15769
Kalaimani, N., Thiagarajan, R., Chakravarthy, N., Raja, S., and Ponniah, A. G. (2013). Economic Losses due to Disease Incidences in Shrimp Farms of India. Fisher. Technol. 50, 80–86.
Kamilya, D., and Baruah, A. (2014). Epizootic ulcerative syndrome (EUS) in fish: History and current status of understanding. Rev. Fish Biol. Fish 24, 369–380. doi: 10.1007/s11160-013-9335-5
Klesius, P. H., Shoemaker, C. A., and Evans, J. J. (2008). “Streptococcus: A Worldwide Fish Health Problem,” in International Symposium on Talipia in Aquaculture. Egypt: Ag. Press Unit Abbassa.
Knight-Jones, T. J., and Rushton, J. (2013). The economic impacts of foot and mouth disease - what are they, how big are they and where do they occur? Prev. Vet. Med. 112, 161–173. doi: 10.1016/j.prevetmed.2013.07.013
Kumar, S., Choubey, A. K., and Srivastava, P. K. (2022). The effects of dietary immunostimulants on the innate immune response of Indian major carp: a review. Fish Shellfish Immunol. 123, 36–49. doi: 10.1016/j.fsi.2022.02.039
Lafferty, K. D., Harvell, C. D., Conrad, J. M., Friedman, C. S., Kent, M. L., Kuris, A. M., et al. (2015). Infectious diseases affect marine fisheries and aquaculture economics. Ann. Rev. Mar. Sci. 7, 471–496. doi: 10.1146/annurev-marine-010814-015646
Lightner, D. V., Redman, R. M., Pantoja, C. R., Noble, B. L., and Tran, L. H. (2012b). Early mortality syndrome affects shrimp in Asia. Glob. Aquacult. Advocate 15, 40.
Lightner, D. V., Redman, R. M., Pantoja, C. R., Tang, K. F. J., Noble, B. L., Schofield, P., et al. (2012a). Historic emergence, impact and current status of shrimp pathogens in the Americas. J. Invertebr. Pathol. 110, 174–183. doi: 10.1016/j.jip.2012.03.006
Liu, L., Ge, M., Zheng, X., Tao, Z., Zhou, S., and Wang, G. (2016). Investigation of Vibrio alginolyticus, V. harveyi, and V. parahaemolyticus in large yellow croaker, Pseudosciaena crocea (Richardson) reared in Xiangshan Bay, China. Aquacult. Rep. 3, 220–224. doi: 10.1016/j.aqrep.2016.04.004
Miao, W., and Lal, K. K (2016). Sustainable intensification of aquaculture in the Asia-Pacific region. Rome: FAO publication.
Ministry of Fisheries Animal Husbandry and Dairying, Government of India. (2022). Handbook on Fisheries Statistics. Poranki: Fisheries Statistics Division, Department of Fisheries.
Mishra, S. S., Das, R., Dhiman, M., Choudhary, P., Debbarma, J., and Sahoo, S. N. (2017). Present status of fish disease management in freshwater aquaculture in India: State-of the-Art-Review. J. Aquac. Fish. 1:003. doi: 10.24966/AAF-5523/100003
Mohanty, B. R., and Sahoo, P. K. (2007). Edwardsiellosis in fish: a brief review. J. Biosci. 32, 1331–1344. doi: 10.1007/s12038-007-0143-8
Monir, S., Bagum, N., Rahman, S., Ashaf-Ud-Doulah, M., Bhadra, A., and Borty, S. C. (2015). Parasitic diseases and estimation of loss due to infestation of parasites in Indian major carp culture ponds in Bangladesh. Int. J. Fisher. Aquat. Stud. 2, 118–122.
Mustafa, A., Rankaduwa, W., and Campbell, P. (2001). Estimating the cost of sea lice to salmon aquaculture in eastern Canada. The Canadian veterinary journal. La revue vétérinaire canadienne. 42, 54–6.
Nayak, S. K. (2020). Current prospects and challenges in fish vaccine development in India with special reference to Aeromonas hydrophila vaccine. Fish Shellfish Immunol. 100, 283–299. doi: 10.1016/j.fsi.2020.01.064
Naylor, R. L., Hardy, R. W., Buschmann, A. H., Bush, S. R., Cao, L., Klinger, D. H., et al. (2021). A 20-year retrospective review of global aquaculture. Nature 591, 551–563. doi: 10.1038/s41586-021-03308-6
Nowak, B. F. (2007). Parasitic diseases in marine cage culture – An example of experimental evolution of parasites? Int. J. Parasitol. 37, 581–588. doi: 10.1016/j.ijpara.2007.01.003
Patil, P. K., Geetha, R., Bhuvaneswari, T., Saraswathi, R., Raja, R. A., Avunje, S., et al. (2022a). Use of chemicals and veterinary medicinal products (VMPs) in Pacific whiteleg shrimp, P. vannamei farming in India. Aquaculture 546:737285. doi: 10.1016/j.aquaculture.2021.737285
Patil, P. K., Geetha, R., Ravisankar, T., Avunje, S., Solanki, H. G., Abraham, T. J., et al. (2021). Economic loss due to diseases in Indian shrimp farming with special reference to Enterocytozoon hepatopenaei (EHP) and white spot syndrome virus (WSSV). Aquaculture 533:736231. doi: 10.1016/j.aquaculture.2020.736231
Patil, P. K., Mishra, S. S., Pradhan, P. K., Manna, S. K., Abraham, J. T., Solanki, H. G., et al. (2022b). Usage pattern of chemicals, biologicals and veterinary medicinal products in Indian aquaculture. Rev. Aquacult. 14, 2038–2063. doi: 10.1111/raq.12688
Peterman, M. A., and Posadas, B. C. (2019). Direct economic impact of fish diseases on the east Mississippi catfish industry. N. Am. J. Aquac. 81, 222–229. doi: 10.1002/naaq.10090
Qi, W. (2002). Social and economic impacts of aquatic animal health problems in aquaculture in China (Rome: FAO), 55–61.
Ramudu, K. R., Shivam, S., Sanil, N. K., Sharma, S. R., Vijayagopal, P., Suresh Babu, P. P., et al. (2020). Histopathological Changes in the Gills Associated with Dactylogyrus spp. Infestation in Orange-spotted Grouper, Epinephelus coioides reared in sea cages. Int. J. Curr. Microbiol. Appl. Sci. 9, 1–8. doi: 10.20546/ijcmas.2020.908.001
Sahoo, P. K., Mohanty, J., Garnayak, S. K., Mohanty, B. R., Kar, B., Prasanth, H., et al. (2013). Estimation of loss due to argulosis in carp culture ponds in India. Indian J. Fish. 60, 99–102.
Sciberras, M., and Dingli, A. (2023). Investigating AI Readiness in the Maltese Public Administration (Berlin: Springer), 31–32.
Sharma, S. R., Rathore, K., Verma, G., Sadhu, D. N., and Philipose, K. (2013). Vibrio alginolyticus infection in Asian seabass (Lates calcarifer, Bloch) reared in open sea floating cages in India. Aquac. Res. 44, 86–92. doi: 10.1111/j.1365-2109.2011.03013.x
Shinn, A. P., Pratoomyot, J., Bron, J. E., Paladini, G., Brooker, E. E., Brooker, A. J., et al. (2015). Economic costs of protistan and metazoan parasites to global mariculture. Parasitology 142, 196–270. doi: 10.1017/S0031182014001437
Singh, B., Prasad, S., Sinha, D., and Verma, M. R. (2013). Estimation of economic losses due to foot and mouth disease in India. Indian J. Anim. Sci. 81, 964–970.
Singh, B. B., Dhand, N. K., and Gill, J. P. (2015). Economic losses occurring due to brucellosis in Indian livestock populations. Prev. Vet. Med. 119, 211–215. doi: 10.1016/j.prevetmed.2015.03.013
Sobhana, K. S. (2009). Diseases of seabass in cage culture and control measures. Kochi: CMFRI and NFDB.
Sood, N., Pradhan, P. K., Swaminathan, T. R., Rathore, G., Jena, J. K., and Lal, K. K. (2021). National Surveillance Programme for Aquatic Animal Diseases – a stepping stone for establishing disease governance system in India. Curr. Sci. 120, 273–277. doi: 10.18520/cs/v120/i2/273-277
Stentiford, G. D., Neil, D. M., Peeler, E. J., Shields, J. D., Small, H. J., Flegel, T. W., et al. (2012). Disease will limit future food supply from the global crustacean fishery and aquaculture sectors. J. Invertebr. Pathol. 110, 141–157. doi: 10.1016/j.jip.2012.03.013
Swain, P., Mishra, S., Dash, S., Nayak, S. K., Mishra, B. K., Pani, K. C., et al. (2007). Association of Flavobacterium branchiophilum in bacterial gill disease of Indian major carps. Indian J. Anim. Sci. 77, 646–649.
Tavares-Dias, M., and Martins, M. (2017). An overall estimation of losses caused by diseases in the Brazilian fish farms. J. Parasit. Dis. 41, 913–8. doi: 10.1007/s12639-017-0938-y
Thitamadee, S., Prachumwat, A., Srisala, J., Jaroenlak, P., Salachan, P. V., Sritunyalucksana, K., et al. (2015). Review of current disease threats for cultivated penaeid shrimp in Asia. Aquaculture 452, 69–87. doi: 10.1016/j.aquaculture.2015.10.028
Torgerson, P. R., and Shaw, A. P. M. (2021). A simple metric to capture losses. The concept of an animal health loss envelope. OIE Bull. 2021, 2–5. doi: 10.20506/bull.2021.1.3259
Willett, W., Rockström, J., Loken, B., Springmann, M., Lang, T., Vermeulen, S., et al. (2019). Food in the anthropocene: the EAT-Lancet Commission on healthy diets from sustainable food systems. Lancet 393, 447–492. doi: 10.1016/S0140-6736(18)31788-4
WOAH (2022). Aquatic Animal Health Code. General Obligations Related to Certification. Paris: WOAH Press.
Keywords: economic loss in aquaculture, fish, shrimp, ELDA framework, epizootic ulcerative syndrome, white spot syndrome virus, Enterocytozoon hepatopenaei, bacterial hemmorhagic septicemia
Citation: Patil PK, Geetha R, Mishra SS, Abraham TJ, Solanki HG, Krupesha Sharma SR, Pradhan PK, Manna SK, Avunje S, Abhinaya D, Felix KT, Vinay TN, Paniprasad K, Paria A, Raja SA, Saraswathy R, Sahoo SN, Rathod R, Rameshkumar P, Baitha R, Thomas S, Dev AK, Jayanthi M, Swain P, Sanil NK and Jena JK (2025) Unveiling the economic burden of diseases in aquatic animal food production in India. Front. Sustain. Food Syst. 9:1480094. doi: 10.3389/fsufs.2025.1480094
Received: 13 August 2024; Accepted: 25 March 2025;
Published: 17 April 2025.
Edited by:
Neaz A. Hasan, Bangabandhu Sheikh Mujibur Rahman Science and Technology University, BangladeshReviewed by:
Salah Mesalhy Aly, Suez Canal University, EgyptEkemini Okon, Ghent University, Belgium
Arun Sudhagar, ICAR-National Bureau of Fish Genetic Resources, India
Copyright © 2025 Patil, Geetha, Mishra, Abraham, Solanki, Krupesha Sharma, Pradhan, Manna, Avunje, Abhinaya, Felix, Vinay, Paniprasad, Paria, Raja, Saraswathy, Sahoo, Rathod, Rameshkumar, Baitha, Thomas, Dev, Jayanthi, Swain, Sanil and Jena. This is an open-access article distributed under the terms of the Creative Commons Attribution License (CC BY). The use, distribution or reproduction in other forums is permitted, provided the original author(s) and the copyright owner(s) are credited and that the original publication in this journal is cited, in accordance with accepted academic practice. No use, distribution or reproduction is permitted which does not comply with these terms.
*Correspondence: P. K. Patil, cGsucGF0aWxAaWNhci5nb3YuaW4=