- 1Naturalis Biodiversity Center, Leiden, Netherlands
- 2Institute of Biology, Leiden University, Leiden, Netherlands
- 3Lankester Botanical Garden, University of Costa Rica, Cartago, Costa Rica
- 4Royal Botanic Gardens, Kew, United Kingdom
- 5Hortus Botanicus, Leiden University, Leiden, Netherlands
- 6Biosystematics Group, Wageningen University, Wageningen, Netherlands
- 7Natural History Museum, University of Oslo, Oslo, Norway
- 8Radboud Institute for Biological and Environmental Sciences, Nijmegen, Netherlands
Introduction: Edible terrestrial orchids are endangered worldwide due to overharvesting and illegal trade. One method to distinguish illegally traded wild-collected orchids from sustainably harvested and legally traded cultivated ones is to artificially create hybrids that do not occur naturally and can be morphologically recognized. Creating artificial orchid hybrids is relatively simple. As a result, thousands of artificial orchid hybrids have already been registered with the Royal Horticultural Society, but predominantly for ornamental purposes. We identified a potential hybrid parental pool for edible orchids from terrestrial species that possess desirable traits from both the grower and consumer perspective.
Method: From the pool of candidate species, we evaluated the possibility of crosses producing viable seed based on phylogenetic distance metrics, based on matK and nrITS sequences of 435 species. Subsequently, we checked the accuracy of our prediction of hybrid compatibility against registered records of hybrids on the International Orchid Register and by experimental production of hybrids.
Results: Our results show that (1) phylogenetic distance can indeed be used as a proxy for predicting hybrid compatibility in orchids.
Discussion: Potential of creating edible orchid hybrids for sustainable trade has not yet been fully exploited.
Introduction
Containing approximately 8% of the world’s vascular plants, Orchidaceae, the Orchid family, is a notable assemblage of biodiversity (Willis, 2017). Orchids are a natural resource extensively used by humans, and wild harvesting poses the greatest threat to their survival (Nic Lughadha et al., 2020; Wraith and Pickering, 2018). Regional surveys report uses of orchids, including as foods, ornamentals, and medicines, in all continents where they grow (Fonge et al., 2019; Lawler and Slaytor, 1970; Moerman, 2021; Nurfadilah, 2020; Pieroni et al., 2017; Rahamtulla et al., 2020; Schultes, 1990).
Orchids uses are not confined to the regions in which they grow. They are commercially traded across international boundaries (Hinsley et al., 2018). The scale of annual trade for many species of orchid used in traditional medicine, food, and cosmetics is measured in tonnes. For example, in 2013, importer reports recorded by the Convention on International Trade in Endangered Species of Wild Fauna and Flora (CITES) of orchids used in Traditional Chinese Medicine included 715 tonnes of Dendrobium nobile, 908 tonnes of Gastrodia elata, 40 Bletilla striata, and 24 D. eriiflorum (UNEP-WCMC, 2013). Wild orchids attract higher prices on international markets than they do in domestic markets. While collectors of wild orchids in Nepal for medicinal trade reported being paid up to $2.5 per kilo, the international trade price for D. eriiflorum was $1300 in 2008–2009 (Subedi et al., 2013). At least 35 non-native orchid species, most likely sourced through import, are traded in Europe for cosmetics, food, and medicinal products (Brinckmann, 2014). Tubers of terrestrial orchids consumed as chikanda in Zambia are wild-collected, not only within Zambia but also from Angola, the Democratic Republic of the Congo, Malawi, Mozambique, and significant volumes from Tanzania (Veldman et al., 2017).
A total of 84% of the plant species listed by CITES are orchids (Willis, 2017). Global orchid trade is mostly in cultivated plants, however harvesting wild orchids for international trade is a significant conservation concern. Moreover, some species traded as cultivated may, in fact, be wild-collected, as suspected in the export of Dendrobium chrysotoxum from Laos (IUCN, 2017). Traceability is a suggested means of limiting illegal wild-collected orchid trade via authenticating legal trade, in which cultivated or sustainably harvested CITES-listed species are tracked on entry, progress, and exit through a regulated system (Lehr and Jaramillo, 2017).
Wild-collected orchids can be identified by looking at physical features such as presence of algae and lichens on leaves or bark held by roots, or damage caused by removing the plant from its growing location (CITES Secretariat, 2002). However, these indicators of wild origins can be removed by growing wild collected plants under cultivation for some time and are not present in dried or otherwise processed orchids traded for food, medicine, and cosmetics. CITES provides guides using morphological features to identify groups of plants in trade for Traditional Asian Medicine (Arcery and Briggs, 2006; Pendry et al., 2004; Variawa et al., 2023). There is no guide for orchid species in trade, for reasons that include the difficulty of identifying non-flowering orchids and the immense number of species in trade.
Forensic science methods, deployed in criminal justice systems to identify people who are perpetrators or victims of crime, have been considered for application in detecting wildlife trade. Stable isotope analysis is a means by which living conditions and geographical origin can be distinguished. In conjunction with other approaches to identification, such as wood anatomy and genetics, stable isotope analysis is being used to identify illegally harvested timber (Dormontt et al., 2015) and salep orchids (Bilgin et al., 2023). However, stable isotope analysis alone has not been adopted for use as a sole identification tool. DNA metabarcoding can be used to detect traces of orchids in processed products (de Boer et al., 2017), but this method alone cannot distinguish whether a plant is wild-collected or cultivated. Creating hybrids to meet market demand has the advantage that these are ultimately intended for commercial purposes rather than for reintroduction of endangered species into natural habitats.
In the case of salep, a product sold as tubers or powder used in the preparation of a drink and ice cream, at least 35 species of orchids from different genera that grow in the Mediterranean region, of which Anacamptis, Dactylorhiza and Orchis are most well-known, may be included in the mixture (Ghorbani et al., 2016; Kasparek and Grimm, 1999; Kreziou et al., 2016). Salep is currently illegally traded in contravention of CITES requirements for permits and for international orchid trade to comply with national legislation (Masters et al., 2022). As salep is already traded as a blended product, not as a single species of orchid, an artificial hybrid may both satisfy consumer requirements and be identifiable as having cultivated origins at CITES control points. Creating artificial hybrids is relatively simple as many orchids are prone to spontaneous hybridization in the wild (Cozzolino et al., 2006). As a result, 192,749 artificial orchid hybrids have already been registered with the Royal Horticultural Society but these were predominantly recorded for ornamental purposes (Royal Horticultural Society, 2024).
Alternative orchid species to supply the trade in edible terrestrial orchids for chikanda and salep have previously been sought. The German botanist Georg Rumphius, who was active in Indonesia during the 17th century, evaluated the tropical orchids he found, considering the properties of orchids used for salep in the Mediterranean region and Europe. He described eating the root of Habenaria rumphii, which he called Orchis Amboinica major, radice Raphanoide: “The taste comes close to that of Satyrium, not bitter, but cloying, and a little sharp,” and “…crumbles when chewed, almost melting in one’s mouth, and is considered to have the same effects as our European Satyria” (Beekman, 2003). Despite surveys for taste and texture as described above, actual salep cultivars have not yet been developed. In contrast to Erycina pusilla, an emerging orchid model organism because it is fast-growing (Dirks-Mulder et al., 2017), edible orchids take several years to progress from pollination to mature plants producing harvestable tubers (Pan et al., 2012). For expediency and economical use of resources, it is worth focusing hybridisation of orchids for sustainable production of edible products on genera and species that are most likely to have edible parts. Lack of cultivar development is likely caused by the relatively long developmental time of most salep orchids.
In this study, we sought to identify a potential hybrid parent pool for orchids that can be cultivated for salep from orchids that have edible tubers and possess desirable traits from both the grower and the consumer perspective to satisfy the demand for trade. Such traits include a high glucomannan content in tubers and individual plants that produce more than one new tuber annually. From the pool of candidates found we evaluated the possibility of crosses producing viable seed based on phylogenetic distance metrics. Using DNA sequence data, the distance between species can be represented and used to predict viability of interspecific and intergeneric crosses (Viruel et al., 2021). Subsequently, we checked the accuracy of this prediction of hybrid compatibility against registered records of hybrids on the International Orchid Register (Royal Horticultural Society, 2024), and results from hybrid crosses that we attempted to produce ourselves. We focused on crosses between species that do not naturally flower in close proximity in the wild due to geographic, pollinator, or temporal separation barriers, following the concept of reproductive isolation (Bateman, 2012; Scopece et al., 2007). Conservation action is propelled by legislation, economy, and technology. Here, we address the technical feasibility of a proposed solution to unsustainable collection of wild orchids for salep.
Materials and methods
Selection of edible terrestrial orchids
We inventoried ‘Medicinal Orchids of Asia and Orchids as Aphrodisiac, Medicine, or Food’ (Teoh, 2019; Teoh, 2016), and selected orchids of which the tubers, or other parts such as pseudobulbs, were described as edible and having uses or properties that were similar to salep, for example, to soothe coughs. This was supplemented by references focused on smaller regions or specific countries (Challe and Price, 2009; Fuchs, 1542; Ghorbani et al., 2014; Gras et al., 2017; Hamisy, 2010; Kasparek and Grimm, 1999; Kasulo et al., 2009; Kreziou et al., 2016; Lee et al., 2008; Low, 1987; Moerman, 2021; Tekinşen and Güner, 2010; Uprety et al., 2016; Veldman et al., 2017, 2018). Reference source, name in reference, and use were recorded alongside currently accepted name and, when documented, chromosome number (Cozzolino et al., 2006; Leitch et al., 2019; POWO, 2024; Rice et al., 2015). This resulted in a list of 203 taxa (See Supplementary Data Appendix I).
Hybridisation records
For each orchid selected, we checked records of cross-compatibility by searching parentage records, as pollen parent and seed parent, against all other orchids within our selection in The International Orchid Register (Royal Horticultural Society, 2024). This was used to generate a matrix of known hybrids (Supplementary Data Appendix II).
Phylogenetic framework and heatmap analyses
Multispecies sequence alignments were made with DNA sequences of nrITS and matK markers generated by Jin et al. (2017) downloaded from NCBI GenBank, and aligned using MAFFT (Katoh and Standley, 2013). We followed the methods described in Viruel et al. (2021) to reconstruct an ultrametric phylogenic tree and calculate pairwise phylogenetic distances. Phylogenetic trees were reconstructed using IQ-TREE applying the GTR + G model of nucleotide evolution and 1,000 replicates of bootstrapping searches (Nguyen et al., 2015). An ultrametric tree was produced using treePL by assigning the value of ‘1.0’ to the root of the tree (Smith and O’Meara, 2012). The ultrametric tree was used to calculate pairwise phylogenetic distances using the patristic method as implemented in the function distRoot from the R package adephylo (Jombart et al., 2010; Pavoine et al., 2008). Species from Cranichideae and Diurideae were used as outgroups. Both Cranichideae and Diurideae are predominantly terrestrial tribes of orchids, of which no species are used as salep (Salazar et al., 2003; Zhang et al., 2023). Phylogenetic trees were visualized with iTOL v6 (Letunic and Bork, 2024). For the heat map analyses, a total of 17 intergeneric hybrids registered with the Royal Horticultural Society (Table 1), were scored for whether both, or one of the two parental species were present in the alignments. If no parental species was present in the dataset, a score of 0 was assigned, if one of the parents was sequenced a score of 1 was given, and if both parental species were sequenced a 2 was assigned. The consensus tree was visualized using the ape package (Paradis and Schleip, 2019) and the ggtree package (Yu et al., 2017), with the heatmap generated by the gheatmap function in R (R Core Team, 2023) within RStudio (RStudio Team, 2022). This approach included an integrated heatmap representing the relationships between nothogenera and their parental species as tree tip labels. The same method was employed to visualize a heatmap of patristic distances derived from the phylogenetic analyses.
Experimental hybrid crossing
Hybrid crossing attempts were conducted in the Netherlands in collaboration with horticultural hobbyist growers from the Vereniging Orchideeën Vermeerdering (VOV) Working Group on salep. Pollinia were removed from the paternal parent using tweezers and placed on the stigma of the maternal parent, whose pollinia had also been removed and discarded. Pollinia packages from 1 to 4 fresh flowers from 6 father plants of a total of 4 species were hand-collected and transferred to the stigma of flowers on 6 mother plants in 2020 and 2023 (Table 2). This resulted in the formation of 6 sets of fruits in 2020, and 2 sets of fruits in 2023 (see Table 2).
Choice of parentage of the hybrid was based on having both parent plants verifiably cultivated and legally available for use, and having desirable tuber characteristics such as fragrant tubers (Dactylorhiza maculata subsp fuchsii).
In 2020, for two crosses, we used Dactylorhiza x foliorella, a hybrid that is readily available in cultivation, as the maternal and paternal parent, respectively. This hybrid cannot occur spontaneously in the wild due to geographical separation of its parental species: Dactylorhiza foliosa is endemic to Madeira, and D. purpurella is native to Denmark, Great Britain, the Netherlands, and Norway (POWO, 2024). We crossed D. × foliorella with Dactylorhiza fuchsii as the maternal and paternal parent, respectively, one of the orchids used in salep that has particularly fragrant tubers and the characteristic palmate tubers of Dactylorhiza.
Serapias is a genus of orchids with known capacity to produce more than one new tuber per year, individual plants have 2 to 5 tubers (Delforge, 2005). Additional crosses were attempted between Serapias cordigera and Dactylorhiza fuchsii. Tuber production by Anacamptis morio has been documented in horticulture (Cribb and Bailes, 1989). In 2023, we crossed A. morio with two species of Serapias: S. strictiflora and S. lingua. A. morio tolerates a range of growth conditions from dry to damp and acidic to alkaline soils, and is found from North Africa to the boreal (Plant Atlas 2020, 2024; POWO, 2024). S. strictiflora and S. lingua form more than one new tuber, and have not been reported as being traded for salep although other species of this genus are traded for salep (Masters et al., 2022; Veltman et al., 2023).
Hybrid cultivation and validation
Pod ripening was assessed visually. Nearly ripe (still closed) pods were sent to Phytesia SA, an orchid growing company, in 2020 and members of the Vereniging Orchideeen Vermeerdering in 2023. Seeds were sown on nutrient medium. DNA was extracted from fresh leaves of both parental plants and hybrids following the CTAB protocol of Doyle and Doyle (1990) with overnight incubation in CTAB buffer for 1–3 days. PCR on a Westburg T3 Biometra thermocycler was used to amplify plastid trnL-trnF with a and b, c and d, e and f primers (Taberlet et al., 1991) and nrITS1 and nrITS2 DNA barcoding markers using 17SE and 26SE primers (Sun et al., 1994). NucleoSpin Gel and PCR clean-up kits (Sigma-Aldrich) were used to purify all resulting amplicons. A total of 5 microliter of PCR product was loaded on an 1.5% agarose gel, visualized by staining with Ethidium bromide, and sized by visual comparison with a standard size ladder. PCR products of the correct size were sent to be Sanger sequenced on an ABI 3730 XL (ThermoFisher) at Radboudumc Technology Center Genomics in Nijmegen. (See Supplementary Data Appendix III gel electrophoresis images of hybrid amplicons). The DNA sequences generated for hybrid validation were not included in the phylogenetic framework.
Results
Phylogenetic analysis and heatmap
Pairwise phylogenetic distances were calculated (see Supplementary Data Appendix IV) and a consensus phylogenetic tree of orchids with known or potential use as edible was generated (see Supplementary Data Appendix V). Inter-generic hybrids registered on the International Orchid Register were combined with the phylogenetic tree to generate a heatmap of crosses between species, and crosses between genera for registered inter-generic crosses. The combined phylogenetic tree and heatmap generated is depicted in Figure 1.
The combined phylogenetic tree and heat map show that phylogenetic distance can be used as a proxy for predicting hybrid compatibility. Aggregation is visible and already registered, or potential hybrids are not spread all over the phylogeny but clearly confined to specific clades of closely related species with low phylogenetic distances. Intergeneric compatibility seems to be restricted to the genera Anacamptis, Cynorkis, Dactylorhiza, Gymnadenia, Habenaria, Himantoglossum, Neotinea, Orchis, Ophrys, Pecteilis, Platanthera, Pseudorchis, and Serapias. There are several intergeneric hybrids with underexploited potential. Amantoglossum (Anacamptis x Himantoglossum) and Orchimantoglossum (Himantoglossum x Orchis) may be particularly desirable for cultivation because Himantoglossum is a genus known for large tubers offering the possibility of high yield. There was no Serapirhiza (Serapias x Dactylorhiza) hybrid registered for the species within our phylogenetic tree, however the two genera are compatible. So, attempting to hybridize species of Dactylorhiza and Serapias known to be traded as salep is likely to succeed.
New experimental hybrid
Validation via DNA barcoding rejected successful hybridisation for 5 out of the 6 crosses attempted but confirmed that a hybrid was successfully created between Dactylorhiza × foliorella and D. maculata subsp. fuchsia, i.e., the seeds harvested from the pods were not the result of self-pollination. This newly created hybrid was registered with the International Orchid Register (Royal Horticultural Society, 2024) under P.34144. It is also documented via botanical illustrations and photographs (see Figure 2). Botanical illustration of hybrid ‘Sarah’ and Figure 3 photograph of flowers of hybrid ‘Sarah’. A description of this newly created hybrid and the three species of its parentage, highlighting morphological differences between the hybrid and its parents, was created (Supplementary Data Appendix VI).
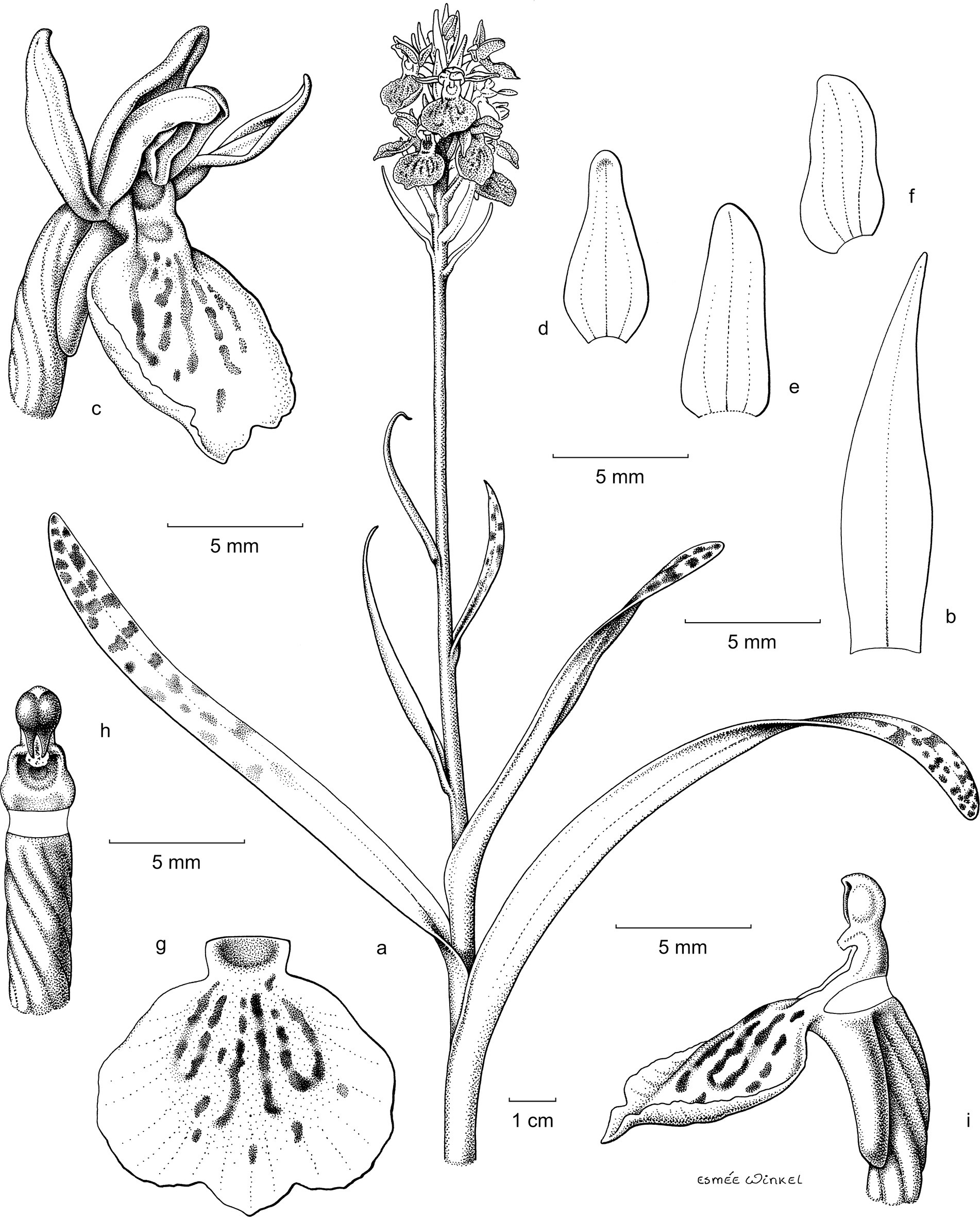
Figure 2. Illustrations for different parts of experimentally created hubrid ‘Sarah’. (a) Habitus. (b) Sterile bract. (c) Flower in frontal view. (d) Median sepal. (e) Lateral sepal. (f) Lateral petal. (g) Median petal (lip). (h) Column. (i) Spur in lateral view. Drawings by Esmée Winkel.
Discussion
Phylogenetic distance metrics help predict viable crosses
Known and likely intergeneric hybrids clustered within close proximity on the phylogenetic tree. This confirms that intergeneric hybrids can occur but are more likely between closely related genera. Our integrated heatmap and phylogenetic tree show the territory of known hybrids and highlight species with edible tubers that are likely to successfully hybridize. This predictive power is useful for focusing hybridisation efforts where they are most likely to succeed. This is particularly important when the time scale for hybridisation is both constrained by seasonal flower production, and long due to the length of time from flowering to seed capsule formation, and then germination, followed by growth to maturity.
Novel hybrid
Our successful hybridisation of D. x foliorella crossed with Dactylorhiza maculata subsp. fuchsii, combined a species known to have particularly fragrant tubers with a hybrid containing the germplasm of an endemic species not currently in salep trade. This created a hybrid with distinct morphology, that cannot occur as a wild plant and is also predisposed to meeting requirements for use as salep.
To prevent the risk that our hybrid, or others, out-compete or back cross with wild species, additional steps need to be taken, such as developing infertile plants with peloric flowers, in which the inner two floral whorls with stamen and stigma are replaced by tepals, so-called non-perianth transitions (Bateman and Rudall, 2006). Such sterile ‘double flowers’ have been developed for many horticultural varieties, for instance, rose, peony, and carnation (Meyerowitz et al., 1989), and are sporadically found in wild orchids (Figure 4). A potential additional benefit from sterile flowers is that resources may be directed to tuber growth instead of seed production.
Our hybridisation experiment was restricted to using orchids that were already in cultivation with known provenance, i.e., they were not wild-collected. The next step is to expand hybridisation to a wider range of parents by collecting pollinia from wild orchids. This would provide greater scope for integrating more desirable traits, such as selecting parents on the basis of their volatile chemical profile, vigorous above ground growth, or proliferation of their underground tubers (Şavşatlı, 2023).
Hybrids as tools of conservation and items for trade
Both wild and artificial plant hybrids have demonstrated utility in conservation and sustainable trade. For example, in conservation of genetic diversity, Butternut (Juglans cinerea) has declined across its native range in eastern North America due to butternut canker (Ophiognomonia clavigignenti-juglandacaerum), a fungal pathogen. Buartnut (J. x bixbyi) is a naturally occurring hybrid with introduced Japanese walnut (J. ailantifolia), which tolerates this infection. A framework for conserving butternut proposes backcrossing buartnut with butternut to enhance butternut canker-resistance while retaining native germplasm (Pike et al., 2021). An example of sustainable trade is Tahitian vanilla (Vanilla × tahitensis), an edible orchid hybrid with high value, noted for its floral aroma, due to the anisyl alcohol content of its seeds. Its parents are V. planifolia and V. odorata (Lubinsky et al., 2008; Nielsen-Massey, 2024; Pérez-Silva et al., 2021).
Previously, attempts were undertaken to make salep trade more sustainable by creating synthetic products packaged in sachets made with corn starch and artificial sweeteners to thicken milk and give it a smooth, creamy texture just like real salep. Such artificial salep products are much cheaper to produce than collecting, drying, and grinding real orchid tubers into powder and, therefore, also much more affordable. It was hoped that artificial salep would reduce the pressure on wild-collected orchids because of its low costs for both producers and consumers. This did not happen, however, because of rising global demand for authentic food products. We developed a method to make salep trade more sustainable by using real orchids. Although rather than collecting them from the wild, we propose to create and grow artificially made sterile hybrids.
As previously noted, the higher cost of orchid tubers from cultivated plants compared to wild collected plants is a barrier (Masters et al., 2022). Analysis of economic viability is a stage for subsequent investigation. Using tissue cultivation for propagation could reduce the cost of purchasing juvenile plants for cultivation. Similarly hybrids producing 2 or more new tubers would sustain replanting from cultivated stock and having tubers to sell. To outcompete harvesting of wild orchids, additional costs of growing such hybrids and harvesting the tubers should be kept at a minimum as well. This could, for instance, be done by combining outdoor cultivation with other crops in mixed farming where local wild orchid populations have already gone extinct.
Focusing on technical feasibility of an identifiably cultivated orchid, in this case via hybridisation, was the purpose of this research. However, we recognize that collecting tubers from wild orchids for salep trade is a component of rural income (Ertuğ, 2000). Cultivation schemes can ensure that income received benefits community members and is not only accessible to landowners. An example of returning benefits to the community from formerly wild collected orchids is the cultivation of Cymbidium orchids supported by the government of Bhutan (Masters, 2015). One of the resolutions passed at the International Orchid Conservation Congress 2016 was that propagation of threatened orchids by small and local enterprises should be supported for sustainable production (Gale et al., 2018).
Conclusion
There is viable opportunity to develop hybrid orchids that can meet consumer demand for salep from verifiably cultivated supply. On a global scale, there is value in supporting wildlife trade to be legitimate as legal wildlife trade has an average worth of $220 billion per annum. In contrast, from 1997 to 2016, illegal wildlife trade was estimated to be worth $7–23 billion per annum (Andersson et al., 2021). An identifiable hybrid orchid suitable for salep offers a pathway from illegal trade in wild-harvested orchids to verifiably cultivated tubers.
Data availability statement
The original contributions presented in the study are included in the article/Supplementary material, further inquiries can be directed to the corresponding author.
Author contributions
SM: Conceptualization, Data curation, Formal analysis, Investigation, Methodology, Writing – original draft, Writing – review & editing. DB: Formal analysis, Software, Visualization, Writing – review & editing. JV: Conceptualization, Software, Visualization, Writing – review & editing. RV: Methodology, Validation, Writing – review & editing. TA: Conceptualization, Formal analysis, Supervision, Writing – original draft, Writing – review & editing. HB: Conceptualization, Formal analysis, Investigation, Methodology, Supervision, Writing – original draft, Writing – review & editing. BG: Conceptualization, Formal analysis, Funding acquisition, Investigation, Methodology, Project administration, Resources, Supervision, Writing – original draft, Writing – review & editing.
Funding
The author(s) declare that no financial support was received for the research and/or publication of this article.
Conflict of interest
The authors declare that the research was conducted in the absence of any commercial or financial relationships that could be construed as a potential conflict of interest.
Generative AI statement
The author(s) declare that no Gen AI was used in the creation of this manuscript.
Publisher’s note
All claims expressed in this article are solely those of the authors and do not necessarily represent those of their affiliated organizations, or those of the publisher, the editors and the reviewers. Any product that may be evaluated in this article, or claim that may be made by its manufacturer, is not guaranteed or endorsed by the publisher.
Supplementary material
The Supplementary material for this article can be found online at: https://www.frontiersin.org/articles/10.3389/fsufs.2025.1526533/full#supplementary-material
References
Andersson, A. A., Tilley, H. B., Lau, W., Dudgeon, D., Bonebrake, T. C., and Dingle, C. (2021). CITES and beyond: illuminating 20 years of global, legal wildlife trade. Global Ecol. Conserv. 26:e01455. doi: 10.1016/j.gecco.2021.e01455
Bateman, R. (2012). Circumscribing species in the European orchid flora: multiple datasets interpreted in the context of speciation mechanisms. Ber. Arbeitskrs. Heim. Orchid 8, 160–212.
Bateman, R. M., and Rudall, P. J. (2006). “The good, the bad, the ugly: using naturally occurring Terata to distinguish the possible from the impossible in orchid floral evolution” in Aliso: a journal of systematic and floristic botany, vol. 22.
Beekman, E. M. (2003). Rumphius’ orchids orchid texts from the Ambonese herbal by Georgius Everhardus Rumphius translated, edited, annotated, and with an introduction by E.M. Beekman : Yale University Press.
Bilgin, A. K., Cengiz, M. F., Karakaş-Budak, B., Gümüş, C., Kılıç, S. A., Perinçek, F., et al. (2023). Elemental compositions and stable isotope signatures for determining the geographical origin of salep orchids collected from different regions of Turkey. J. Appl. Res. Med. Aromatic Plants 37:100505. doi: 10.1016/j.jarmap.2023.100505
Brinckmann, J. A. (2014). Quick scan of Orchidaceae species in European commerce as components of cosmetic, food and medicinal products. CITES Management Authority Switzerland Lichtenstein, 1–111.
Challe, J. F. X., and Price, L. L. (2009). Endangered edible orchids and vulnerable gatherers in the context of HIV/AIDS in the southern highlands of Tanzania. J. Ethnobiol. Ethnomed. 5. doi: 10.1186/1746-4269-5-41
CITES Secretariat (2002). CITES World, Official Newsletter of the Parties. Available online at: https://cites.org/eng/news/world/9.pdf (Accessed February 10, 2023).
Cozzolino, S., Nardella, A. M., Impagliazzo, S., Widmer, A., and Lexer, C. (2006). Hybridization and conservation of Mediterranean orchids: should we protect the orchid hybrids or the orchid hybrid zones? Biol. Conserv. 129, 14–23. doi: 10.1016/j.biocon.2005.09.043
Cribb, P., and Bailes, C. (1989). Hardy orchids: orchids for the garden and frost-free greenhouse. London, UK: Christopher Helm.
de Boer, H. J., Ghorbani, A., Manzanilla, V., Raclariu, A. C., Kreziou, A., Ounjai, S., et al. (2017). DNA metabarcoding of orchid-derived products reveals widespread illegal orchid trade. Proc. R. Soc. B Biol. Sci. 284:20171182. doi: 10.1098/rspb.2017.1182
Delforge, P. (2005). Orchids of Europe, North Africa, and the Middle East. London, UK: A & C Black Publishers.
Dirks-Mulder, A., Butôt, R., van Schaik, P., Willem, J., Wijnands, P. M., van den Berg, R., et al. (2017). Exploring the evolutionary origin of floral organs of Erycina pusilla, an emerging orchid model system. BMC Evol. Biol. 17:89. doi: 10.1186/s12862-017-0938-7
Dormontt, E. E., Boner, M., Braun, B., Breulmann, G., Degen, B., Espinoza, E., et al. (2015). Forensic timber identification: It’s time to integrate disciplines to combat illegal logging. Biol. Conserv. 191, 790–798. doi: 10.1016/j.biocon.2015.06.038
Ertuğ, F. (2000). Rely to Kasparek and Grimm’s article published in EB 53(4): 396-406 orchid trade for Salep. Econ. Bot. 54:421422.
Fonge, B. A., Essomo, S. E., Bechem, T. E., Tabot, P. T., Arrey, B. D., Afanga, Y., et al. (2019). Market trends and ethnobotany of orchids of Mount Cameroon. J. Ethnobiol. Ethnomed. 15:29. doi: 10.1186/s13002-019-0308-1
Gale, S. W., Fischer, G. A., Cribb, P. J., and Fay, M. F. (2018). Orchid conservation: bridging the gap between science and practice. Bot. J. Linn. Soc. 186, 425–434. doi: 10.1093/botlinnean/boy003
Ghorbani, A., Gravendeel, B., Selliah, S., Zarré, S., and de Boer, H. (2016). DNA barcoding of tuberous Orchidoideae: a resource for identification of orchids used in Salep. Mol. Ecol.Resources 5:4171. doi: 10.1111/1755-0998.12615
Ghorbani, A., Gravendeel, B., Zarre, S., and de Boer, H. (2014). Illegal wild collection and international trade of CITES-listed terrestrial orchid tubers in Iran. TRAFFIC Bulletin.
Gras, A., Garnatje, T., Ibáñez, N., López-Pujol, J., Nualart, N., and Vallès, J. (2017). Medicinal plant uses and names from the herbarium of Francesc Bolòs (1773–1844). J. Ethnopharmacol. 204, 142–168. doi: 10.1016/j.jep.2017.04.002
Hamisy, W. C. (2010). Orchid conservation project (REF: 58.09.08) development of conservation strategies for the wild edible orchids in Tanzania. The Rufford Foundation, London UK.
Hinsley, A., De Boer, H. J., Fay, M. F., Gale, S. W., Gardiner, L. M., Gunasekara, R. S., et al. (2018). A review of the trade in orchids and its implications for conservation. Bot. J. Linn. Soc. 186, 435–455. doi: 10.1093/botlinnean/box083
Jin, W. T., Schuiteman, A., Chase, M. W., Li, J. W., Chung, S. W., Hsu, T. C., et al. (2017). Phylogenetics of subtribe Orchidinae s.l. (Orchidaceae; Orchidoideae) based on seven markers (plastid matK, psaB, rbcL, trnL-F, trnH-psba, and nuclear nrITS, Xdh): implications for generic delimitation. BMC Plant Biol. 17:222. doi: 10.1186/s12870-017-1160-x
Jombart, T., Balloux, F., and Dray, S. (2010). Adephylo: new tools for investigating the phylogenetic signal in biological traits. Bioinformatics 26, 1907–1909. doi: 10.1093/bioinformatics/btq292
Kasparek, M., and Grimm, U. (1999). European trade in Turkish salep with special reference to Germany. Econ. Bot. 53, 396–406. doi: 10.1007/BF02866718
Kasulo, V., Mwabumba, L., and Cry, M. (2009). A review of edible orchids in Malawi. J. Hortic. For. 1, 133–139.
Katoh, K., and Standley, D. M. (2013). MAFFT multiple sequence alignment software version 7: improvements in performance and usability. Mol. Biol. Evol. 30, 772–780. doi: 10.1093/molbev/mst010
Kreziou, A., De Boer, H., and Gravendeel, B. (2016). Harvesting of salep orchids in North-Western Greece continues to threaten natural populations. Oryx 50, 393–396. doi: 10.1017/S0030605315000265
Lawler, L. J., and Slaytor, M. (1970). Uses of Australian orchids by aborigines and early settlers. Med. J. Aust. 2, 1259–1261. doi: 10.5694/j.1326-5377.1970.tb63459.x
Lee, S., Xiao, C., and Pei, S. (2008). Ethnobotanical survey of medicinal plants at periodic markets of Honghe prefecture in Yunnan Province, SW China. J. Ethnopharmacol. 117, 362–377. doi: 10.1016/j.jep.2008.02.001
Lehr, H., and Jaramillo, L. (2017). Applicability of traceability systems for CITES-listed medicinal and ornamental plants (appendices II and III) preliminary assessment key findings. United Nations Publication. Available online at: http://www.elsevier.com/locate/scp (Accessed February 10, 2021).
Leitch, I., Johnston, E., Pellicer, J., Hidalgo, O., and Bennett, M. (2019). Plant DNA C-values database. Available online at: https://cvalues.science.kew.org (Accessed January 11, 2023).
Letunic, I., and Bork, P. (2024). Interactive tree of life (iTOL) v6: recent updates to the phylogenetic tree display and annotation tool. Nucleic Acids Res. 52, W78–W82. doi: 10.1093/nar/gkae268
Lubinsky, P., Cameron, K. M., Molina, M. C., Wong, M., Lepers-Andrzejewski, S., Gómez-Pompa, A., et al. (2008). Neotropical roots of a Polynesian spice: the hybrid origin of Tahitian vanilla, vanilla tahitensis (Orchidaceae). Am. J. Bot. 95, 1040–1047. doi: 10.3732/ajb.0800067
Masters, S., Anthoons, B., Madesis, P., Saroja, S. G., Schermer, M., Gerritsen, W., et al. (2022). Quantifying an online wildlife trade using a web crawler. Biodivers. Conserv. 31, 855–869. doi: 10.1007/s10531-022-02367-z
Meyerowitz, E. M., Smuth, D. R., and Bowman, J. L. (1989). Abnormal flowers and pattern formation in floral development. Development 106, 209–217. doi: 10.1242/dev.106.2.209
Moerman, D. (2021). Native American ethnobotany database. Available online at: http://naeb.brit.org/ (Accessed February 10, 2021).
Nguyen, L. T., Schmidt, H. A., Von Haeseler, A., and Minh, B. Q. (2015). IQ-TREE: a fast and effective stochastic algorithm for estimating maximum-likelihood phylogenies. Mol. Biol. Evol. 32, 268–274. doi: 10.1093/molbev/msu300
Nic Lughadha, E., Bachman, S. P., Leão, T. C. C., Forest, F., Halley, J. M., Moat, J., et al. (2020). Extinction risk and threats to plants and fungi. Plants People Planet 2, 389–408. doi: 10.1002/ppp3.10146
Nielsen-Massey. (2024). Tahitian pure vanilla extract. Available online at: https://nielsenmassey.com/products/tahitian-pure-vanilla-extract/ (Accessed August 18, 2024).
Nurfadilah, S. (2020). Utilization of orchids of Wallacea region and implication for conservation. IOP Conf. Ser. Earth Environ. Sci. 473:012063. doi: 10.1088/1755-1315/473/1/012063
Pan, I. C., Liao, D. C., Wu, F. H., Daniell, H., Singh, N. D., Chang, C., et al. (2012). Complete chloroplast genome sequence of an orchid model plant candidate: Erycina pusilla apply in tropical oncidium breeding. PLoS One 7:e34738. doi: 10.1371/journal.pone.0034738
Paradis, E., and Schleip, K. (2019). Ape 5.0 an environment for modern phylogenetics and evolutionary analyses in R. Bioinformatics 35, 526–528. doi: 10.1093/bioinformatics/bty633
Pavoine, S., Ollier, S., Pontier, D., and Chessel, D. (2008). Testing for phylogenetic signal in phenotypic traits: new matrices of phylogenetic proximities. Theor. Popul. Biol. 73, 79–91. doi: 10.1016/j.tpb.2007.10.001
Pendry, S., Allan, C., Wu, J., and Cameron, G. (2004). Traditional Asian medicine identification guide for law enforcers TRAFFIC International, Cambridge UK: Version II.
Pérez-Silva, A., Nicolás-García, M., Petit, T., Dijoux, J. B., De Los Ángeles Vivar-Vera, M., Besse, P., et al. (2021). Quantification of the aromatic potential of ripe fruit of Vanilla planifolia (Orchidaceae) and several of its closely and distantly related species and hybrids. Eur. Food Res. Technol. 247, 1489–1499. doi: 10.1007/s00217-021-03726-w
Pieroni, A., Sõukand, R., Quave, C. L., Hajdari, A., and Mustafa, B. (2017). Traditional food uses of wild plants among the Gorani of South Kosovo. Appetite 108, 83–92. doi: 10.1016/j.appet.2016.09.024
Pike, C. C., Williams, M., Brennan, A., Woeste, K., Jacobs, J., Hoban, S., et al. (2021). Save our species: a blueprint for restoring butternut (Juglans cinerea) across eastern North America. J. For. 119, 196–206. doi: 10.1093/jofore/fvaa053
Plant Atlas 2020 (2024). Available at: https://plantatlas2020.org/atlas (Accessed August 18, 2024).
POWO (2024). Plants of the world online. Facilitated by the royal the royal botanic gardens. Kew: Published on the Internet.
R Core Team (2023). R: A language and environment for statistical computing. Vienna, Austria: R Foundation for Statistical Computing.
Rahamtulla, M., Pradhan, U. C., Roy, A. K., Rampilla, V., and Khasim, S. M. (2020). “Ethnomedicinal aspects of some orchids from Darjeeling Himalaya, India” in Orchid biology: recent trends & challenges. S. M. Khasim et al. (eds.).
Rice, A., Glick, L., Abadi, S., Einhorn, M., Kopelman, N. M., Salman-Minkov, A., et al. (2015). “The chromosome counts database (CCDB) - a community resource of plant chromosome numbers” in New Phytologist, vol. 206, 19–26.
Royal Horticultural Society (2024). The international orchid register. Available online at: https://apps.rhs.org.uk/horticulturaldatabase/orchidregister/orchidregister.asp (Accessed July 30, 2024).
Salazar, G. A., Chase, M. W., Soto Arenas, M. A., and Ingrouille, M. (2003). Phylogenetics of Cranichideae with emphasis on Spiranthinae (Orchidaceae, Orchidoideae): evidence from plastid and nuclear DNA sequences. Am. J. Bot. 90, 777–795. doi: 10.3732/ajb.90.5.777
Şavşatlı, Y. (2023). Identification of volatile compounds in salep (Serapias vomeracea) tubers and effects of harvest time and drying method on composition variation. Ciencia e Agrotecnologia 47, 1–9. doi: 10.1590/1413-7054202347002223
Schultes, R. (1990). Medicinal orchids of the Indians of the Colombian Amazon. Miami, USA: American Orchid Society Bulletin.
Scopece, G., Musacchio, A., Widmer, A., and Cozzolino, S. (2007). Patterns of reproductive isolation in Mediterranean deceptive orchids. Evolution 61, 2623–2642. doi: 10.1111/j.1558-5646.2007.00231.x
Smith, S. A., and O’Meara, B. C. (2012). TreePL: divergence time estimation using penalized likelihood for large phylogenies. Bioinformatics 28, 2689–2690. doi: 10.1093/bioinformatics/bts492
Subedi, A., Kunwar, B., Choi, Y., Dai, Y., van Andel, T., Chaudhary, R. P., et al. (2013). Collection and trade of wild-harvested orchids in Nepal. J. Ethnobiol. Ethnomed. 9. doi: 10.1186/1746-4269-9-64
Sun, Y., Skinner, D. Z., Liang, G. H., and Hulbert, S. H. (1994). Phylogenetic analysis of Sorghum and related taxa using internal transcribed spacers of nuclear ribosomal DNA. Theor. Appl. Genet. 89, 26–32. doi: 10.1007/BF00226978
Taberlet, P., Gielly, L., Pautou, G., and Bouvet, J. (1991). Universal primers for amplification of three non-coding regions of chloroplast DNA. Plant Mol. Biol. 17, 1105–1109. doi: 10.1007/BF00037152
Tekinşen, K. K., and Güner, A. (2010). Chemical composition and physicochemical properties of tubera salep produced from some Orchidaceae species. Food Chem. 121, 468–471. doi: 10.1016/J.FOODCHEM.2009.12.066
Teoh, E. S. (2019). Orchids as aphrodisiac, medicine or food. 1st Edn. Switzerland: Springer International Publishing.
Uprety, Y., Poudel, R. C., Gurung, J., Chettri, N., and Chaudhary, R. P. (2016). “Traditional use and management of NTFPs in Kangchenjunga landscape: implications for conservation and livelihoods” in Journal of ethnobiology and Ethnomedicine, vol. 12 (BioMed Central Ltd) Available at: https://trade.cites.org/ (Accessed July, 7, 2023).
Variawa, T., Pfab, M. F., and Jabar, L. (2023). Guide to the identification of succulent plant species included by South Africa in appendix III of the convention on international trade in endangered species of wild Fauna and Flora (CITES). South African National Biodiversity Institute, Pretoria, South Africa.
Veldman, S., Gravendeel, B., Otieno, J. N., Lammers, Y., Duijm, E., Nieman, A., et al. (2017). High-throughput sequencing of African chikanda cake highlights conservation challenges in orchids. Biodivers. Conserv. 26, 2029–2046. doi: 10.1007/s10531-017-1343-7
Veldman, S., Kim, S.-J., Van Andel, T. R., Font, M. B., Bone, R. E., Bytebier, B., et al. (2018). Trade in Zambian edible orchids-DNA barcoding reveals the use of unexpected orchid taxa for Chikanda. Genes 9:595. doi: 10.3390/genes9120595
Veltman, M. A., Anthoons, B., Schrøder-Nielsen, A., Chimal Ballesteros, J. A., Ghorbani, A., Karahan, A., et al. (2023). “Geographic shifts and increasing species diversity of wild orchid harvesting threatens survival of natural populations” in Hitting a moving target: novel genomic resources to identify and trace traded orchids. Veltman, M. A (ed.) (Oslo, Norway: University of Oslo).
Viruel, J., Kantar, M. B., Gargiulo, R., Hesketh-Prichard, P., Leong, N., Cockel, C., et al. (2021). Crop wild phylorelatives (CWPs): phylogenetic distance, cytogenetic compatibility and breeding system data enable estimation of crop wild relative gene pool classification. Available online at: https://academic.oup.com/botlinnean/article/195/1/1/5903667 (Accessed September 12, 2024).
Wraith, J., and Pickering, C. (2018). Quantifying anthropogenic threats to orchids using the IUCN red list. Ambio 47, 307–317. doi: 10.1007/s13280-017-0964-0
Yu, G., Smith, D. K., Zhu, H., Guan, Y., and Lam, T. T. (2017). GGTREE: an R package for visualization and annotation of phylogenetic trees with their covariates and other associated data. Methods Ecol. Evolution 8, 28–36. doi: 10.1111/2041-210X.12628
Keywords: CITES, chikanda, salep, wildlife trade, orchids (Orchidaceae)
Citation: Masters S, Bogarín D, Viruel J, van Vugt R, van Andel T, de Boer HJ and Gravendeel B (2025) Horticultural hybrid development of edible terrestrial orchids for verifiable sustainable trade. Front. Sustain. Food Syst. 9:1526533. doi: 10.3389/fsufs.2025.1526533
Edited by:
Matteo Balderacchi, Independent Researcher, Piacenza, ItalyReviewed by:
Endang Semiarti, Gadjah Mada University, IndonesiaBrandon Eduardo Gutiérrez Rodríguez, Instituto de Ecología (INECOL), Mexico
Copyright © 2025 Masters, Bogarín, Viruel, van Vugt, van Andel, de Boer and Gravendeel. This is an open-access article distributed under the terms of the Creative Commons Attribution License (CC BY). The use, distribution or reproduction in other forums is permitted, provided the original author(s) and the copyright owner(s) are credited and that the original publication in this journal is cited, in accordance with accepted academic practice. No use, distribution or reproduction is permitted which does not comply with these terms.
*Correspondence: Susanne Masters, c3VzYW5uZS5tYXN0ZXJzQG5hdHVyYWxpcy5ubA==