- 1Department of Biotechnology and Food Engineering, Technion-Israel Institute of Technology, Haifa, Israel
- 2Department of Biotechnology and Food Engineering, Guangdong Technion-Israel Institute of Technology, Shantou, China
- 3Guangdong Provincial Key Laboratory of Materials and Technologies for Energy Conversion, Guangdong Technion-Israel Institute of Technology, Shantou, China
Introduction: The global generation of large amounts of eggshell waste (ESW) is a persistent annual issue, requiring sustainable disposal solutions. Soil biosolarization (SBS), which combines organic amendments with solar heating, is a sustainable agricultural practice that serves as an alternative to chemical soil fumigation for effective pest control. This study evaluates the feasibility of using ESW as a soil amendment in SBS.
Methods: A bioreactor-based simulation system was used to conduct SBS experiments under aerobic and anaerobic conditions. Assessments included soil health, microbial activity, soil microbiome, and phytotoxicity.
Results and discussion: The results demonstrate that ESW significantly altered the pH of acidic soils, shifting them toward neutral and alkaline conditions. CO₂ emissions from ESW-amended soils increased in both aerobic (up to 7.0 mg CO₂/g soil) and anaerobic conditions (0.62 mg CO₂/g soil), indicating efficient microbial utilization of ESW and enhanced microbial activity. Additionally, ESW promoted the release of functional volatile organic compounds such as dimethyl sulfide, methanethiol, ammonia, and formic acid, which are known to suppress soilborne pathogens. Bacterial community analysis showed that ESW amendments promoted beneficial genera (e.g., Bacillus, Brevibacillus, Paenibacillus) associated with pest suppression and plant growth, while inhibiting harmful genera like Alicyclobacillus. Residual phytotoxicity on lettuce seedlings remained low, and weed seed inactivation of Bidens pilosa was effective in all SBS simulations with ESW.
Conclusion: These findings highlight the potential of ESW to enhance SBS efficiency, suppress pathogens, and promote sustainable waste recycling.
1 Introduction
In recent decades, the demand for eggs in human diets has steadily risen. Global egg production in 2018 was 76.7 million tons, a 14.95% increase over the past decade (FAO, 2020). For the past 30 years, China has been the world’s largest egg producer, with a total production of 26.9 million tons in 2018 (FAO, 2020). By 2030, global egg production is projected to reach 90 million tons (Ferraz et al., 2018). Given that the eggshell (ES) makes up about 10% of an egg’s weight (Laca et al., 2017), approximately 7.67 million tons of ES are generated worldwide annually. Among them, ES produced in the egg processing and food industry accounts for a large proportion, about 2.3 million tons in 2018 (Ahmed et al., 2021).
As a type of food waste, ES may cause environmental pollution when it is not properly disposed of in the environment (Ngayakamo and Onwualu, 2022). Eggshell waste (ESW) is usually discarded in landfills with minimal or no pre-treatment (Oliveira et al., 2013), and thus becomes a source of environmental pollution with odor production, microbial growth, and greenhouse gas (GHG) production (Mignardi et al., 2020). Because its calcified shell contains more than 94% calcium carbonate and its membrane contains various proteins, ES should be considered a valuable natural material rather than a waste product (Baláž et al., 2021). Possible applications of ES include low-investment processes such as the manufacture of fertilizers, animal feed, and limestone, as well as high-investment processes for transforming as materials for human consumption, heavy metals absorption, paper treatment, catalysts for biodiesel production, protein production, etc. (Oliveira et al., 2013). In environmental remediation, ES has proven effective in neutralizing acid mine drainage and removing heavy metals like Pb, Cd, and Cu (Kalyoncu Ergüler, 2015; Zhang et al., 2017), with smaller particles demonstrating enhanced efficiency in these processes (López-Díaz et al., 2024). These findings highlight the potential of ES for both waste recycling and environmental protection.
More recently, the European Union has been working to promote the valorization of ESW through soil applications (Quina et al., 2017). The reuse of ESW in agriculture is a practical approach to meet the need for soil calcium supplementation and acidic pH adjustment, contributing to the development of a circular economy and environmental sustainability (Oliveira et al., 2013). In addition, ES can also be used for the immobilization of heavy metals in soil. Soares et al. reported that adding industrial ESW to a co-compost mixture was suitable for soil remediation to immobilize lead and zinc (Soares et al., 2015).
Another promising approach to reuse ESW for soil improvement and value-added crop production is its application as a soil amendment in soil biosolarization (SBS), a use that has not been previously reported. SBS is a sustainable soil pest control practice that combines the addition of organic amendments into soil with solarization (Achmon et al., 2016). Solarization is an environmentally friendly alternative to soil fumigation (Stapleton, 2000). This process involves mulching moistened soil in open fields or greenhouses with transparent plastic film and applying solar heating to create a greenhouse effect, raising the soil surface temperature to levels lethal or near-lethal to pathogens (Katan and DeVay, 1991). However, there are still some limitations to solarization, including long treatment times, reliance on sunny weather, and limited effectiveness in controlling all pathogens (Achmon et al., 2017).
To address these challenges, SBS has emerged as an enhanced method of solarization, gaining significant attention in recent years (Fernández-Bayo et al., 2017; Fernandez-Bayo et al., 2020; Shea et al., 2022). By incorporating organic amendments, SBS creates reductive conditions and produces biopesticidal compounds in the soil, effectively inactivating soilborne pests (Achmon et al., 2017; Fernandez-Bayo et al., 2020). Studies have demonstrated that SBS can rapidly increase soil microbial abundance and activity (Randall et al., 2020), produce organic acids with bio-pesticidal effects (Hestmark et al., 2019), and raise topsoil temperatures above 50°C, intensifying stress on soilborne plant pathogens (Stapleton, 2000).
Soil respiration (i.e., CO₂ release) is widely used as an indicator of soil microbial activity in SBS, as the CO₂ emitted primarily originates from microbial respiration. It provides insight into microbial responses to thermal stress and substrate utilization (Achmon et al., 2016; Fernández-Bayo et al., 2018; Liang et al., 2022; Zou et al., 2023). Soil volatile organic compound (VOC) release profiles have recently been proposed as a tool to characterize soil microbial metabolism, including the production of antimicrobial substances during SBS (Liang et al., 2022; Zou et al., 2023). The presence of microbial VOCs (mVOCs) in this process could be linked to specific metabolic pathways that contribute to the effectiveness of SBS (Liang et al., 2022). Notably, some mVOCs have shown potential for inhibiting plant pathogens (de Boer et al., 2019), making their production considered one of the key factors in the success of SBS. Therefore, measuring soil respiration and VOC release is crucial for evaluating microbial activity and understanding the metabolic processes involved in SBS, providing valuable insights into its effectiveness for pathogen suppression.
Soil microbiota, including both endogenous microorganisms and those introduced through organic amendments, play a crucial role in the SBS process (Fernández-Bayo et al., 2017; Fernández-Bayo et al., 2019). These microorganisms not only facilitate fermentation but also significantly influence subsequent soil health (Chaparro et al., 2012). A previous SBS study using tomato pomace and green waste compost has shown an increase in bacterial genera such as Clostridium, Weissella, and Acetobacter, which promote the accumulation of volatile fatty acids (Achmon et al., 2020). Similarly, an SBS study with almond residues as amendments has demonstrated an increased abundance of Streptomyces, which facilitates biopesticide formation, and Clostridium, which promotes the production of organic acids (Shea et al., 2022). The enrichment of bacteria that produce bacteriostatic substances during the SBS process contributes to the suppression of soilborne plant pathogens. Given their critical role in SBS and subsequent agricultural outcomes, understanding soil microbiota restructuring and community dynamics is essential.
Previous studies have shown that using tomato pomace and olive pomace in SBS improves the health and quality of crops in consecutive cropping systems (Achmon et al., 2018; Domínguez et al., 2014). Agricultural wastes such as grape, tomato, and fish processing waste have also been found to effectively suppress weed seeds during SBS, but they also leave residual phytotoxicity that may adversely affect subsequent crops (Achmon et al., 2016, 2017; Liang et al., 2022). While phytotoxicity is useful for weed control, its persistence in soil can negatively impact crop growth, making its management crucial for SBS effectiveness.
In this study, the feasibility of using ESW as a soil amendment in the SBS process was investigated through a lab-scale simulation system. The evaluation of SBS with ESW was conducted under both aerobic and anaerobic environments, focusing on four key parameters: (1) soil properties (pH, volatile solids, electrical conductivity, and nutrient content), (2) gas emissions (soil respiration and VOC release), (3) alterations in bacterial community structure (diversity and composition) and (4) residual phytotoxicity and weed seed inactivation. These findings offer valuable insights into the potential application of ESW in field-based SBS and other soil-related applications, contributing to the advancement of sustainable agricultural systems.
2 Materials and methods
2.1 Soil and eggshell waste preparation and characterization
The soil was collected from the field experiment station of the Guangdong Technion Israel Institute of Technology (GTIIT), in Shantou, Guangdong, China (23°31′4″N, 116°45′6″E). Approximately 100 kg of soil was excavated from the top 20 cm of the surface layer using a shovel and placed into a container. The collected soil was then transported to the laboratory, where it was sieved through 2 mm sifters and then dried in an oven (Bluepard Instrument DZF- 6000 Vacuum Drier) at 45°C until the mass remained constant. Once dried, the soil was stored at room temperature for further use in the experiments.
Collection of ESW took place in the kitchen of the GTIIT canteen, where the canteen personnel separated it from other food waste to avoid contamination. It was obtained during the common process of using fresh eggs, where the contents (yolk and white) were poured out, and the shells were discarded. After collection, ESW was first sun-dried until its mass remained constant, then ground into particles smaller than 1 mm using a laboratory blender to ensure it could later mix uniformly with the soil. The dried powder of ESW was stored in sealed boxes at 4°C to preserve its stability and prevent potential degradation or microbial contamination, ensuring it remained in optimal condition for future use in experiments.
The moisture content of soil and ESW was determined gravimetrically by comparing the mass of samples before and after drying in an oven (Bluepard Instrument DZF- 6000 Vacuum Drier) at 105°C until a constant weight was achieved. The volatile solids (VS) content was determined by measuring the mass loss of samples after 6 h of incineration in a muffle furnace. (SAFTherm Furnace, STM-12-12) at 550°C and was expressed as a fraction of the dry weight of the sample (Achmon et al., 2016). The pH and electrical conductivity (EC) were measured on the supernatant of a mixture composed of sample (g) and distilled water (mL) at a 1:5 ratio using a multi-parameter measuring instrument (Thermo Scientific Orion Versa Star Pro). The characterization of physicochemical parameters of nutrient element content in both soil and ESW was carried out by Nanjing Convinced-Test Technology Co., Ltd., China, as previously done (Zou et al., 2023). The properties of the dried soil and ESW powder are presented in Table 1.
2.2 Soil biosolarization simulation system assembly
A laboratory-level SBS simulation system was established, which consisted of a temperature-programmable incubator, a multi-channel Micro-Oxymax respirometer, and a Proton-Transfer-Reaction Time-of-Flight Mass Spectrometry (PTR-TOF-MS) (the details of each section will be described below). Similar simulation systems have been successfully used to study SBS using residues of tomato, grape, fish, and tea waste as soil amendments (Achmon et al., 2016; Liang et al., 2022; Zou et al., 2023). In this study, an SBS simulation system with two settings was used: (1) an aerobic setting that simulates soil conditions near the soil surface or in sandier soils, and (2) an anaerobic setting that simulates soil conditions in deeper layers of the soils or those soils that have high clay and/or moisture contents (Achmon et al., 2016). For an overview of the research design, experimental system setup, and the experiments conducted in this study, refer to Supplementary Figure S1.
In the system, 100 mL or 250 mL glass bottles were used as bioreactors, loaded with mixtures of soil and ESW, and they were placed in the temperature-programmable incubator (Lab Companion, Low Temp. Incubator, Multi-Chamber, 1 L-11-4C) operated under a periodic temperature program that simulates the diurnal temperature changes during an actual SBS. The incubator’s temperature program was based on the average near-surface temperature observed in previous field studies (for the specific program, refer to Supplementary Table S1) (Achmon et al., 2017, 2018; Hestmark et al., 2019). In this study, soil mixtures with 0.5, 2.5, 5, and 8% ESW (on a dry weight basis) were examined. These soil mixtures were then wetted with 30% wt. of distilled water before being loaded into the bioreactors. During 30 days of SBS simulation, soil samples from each bioreactor were taken to measure pH, VS, and EC every six days using the method mentioned above. The nutrient contents of carbon (C), nitrogen (N), phosphorous (P), magnesium (Mg), potassium (K), and calcium (Ca) were determined on day 0 and day 30 of the SBS simulation.
2.3 Soil respiration and volatile organic compound emissions measurement
A Micro-Oxymax respirometer (Columbus Instruments International) was used to measure soil microbial respiration during SBS simulation. The Micro-Oxymax respirometer was equipped with a paramagnetic O2 sensor (Columbus Instruments International Serial 200,135–3), a non-dispersive infrared CO2 sensor (Columbus Instruments International Serial 200,135–4) with a 0–3% detection range, and a CH4 sensor with a 0–5% detection range (Columbus Instruments International Serial 200,135–5). Inside the incubator, bioreactors were connected to gas collectors and then connected to the Micro-Oxymax respirometer. Through auto-sampling by a sampling pump (Columbus Instruments International Serial 200,135–1) and auto-measurement by gas sensors, the Micro-Oxymax respirometer obtained the production rate and the accumulation of O2, CO2, and CH4 in the bioreactors. The consecutive sampling interval for each bioreactor was about 4 h, and the total sampling duration lasted 30 days.
A Proton-Transfer-Reaction Time-of-Flight Mass Spectrometry (PTR-TOF-MS) (Ionicon Analytik Ges.m.b.H, Innsbruck, Austria) was used to detect VOCs that were emitted from the soil in the bioreactors. The reagent ion of the PTR-TOF-MS was H3O+. The mass scale of the PTR-TOF-MS was calibrated using the signals at 21.022 m/z and 59.049 m/z (H3O+-isotope and protonated acetone). The bioreactors were taken out of the incubators to measure soil VOC emissions. By connecting the sample tube of the PTR-TOF-MS to the air outlet on the bioreactor lid, the PTR-TOF-MS automatically sampled the gas from the headspace of the bioreactors and transferred it into a drift pump for ionization. As previously done (Liang et al., 2022; Yu et al., 2024), the actual working conditions of the drift pump were set as follows: drift pressure 2.30 mbar, drift temperature 80°C, and drift voltage 630 V. The ratio of electric field intensity to number density (E/N) was 142 Td (1 Td = 10–17 V·cm2). VOC emissions in each bioreactor were detected once a day by PTR-TOF-MS for 120 s with one spectrum per second, and the total detection duration lasted 30 days.
The soil VOC data were analyzed using PTRMS_Viewer 3.4 software. Signals at 21.022 m/z (H3O + -isotope) and 59.049 m/z (protonated acetone) were calibrated for mass accuracy. The stable signal region of each bioreactor was selected and used to calculate the average intensity of the signal. To analyze VOC emission profiles across all soil treatments over 30 days, principal component analysis (PCA) was applied. The ESW-enriched VOCs were determined by comparing the average intensity of all VOCs in the ESW-amended soils to the corresponding control soils over 30 days. The threshold for significant ESW-enriched VOC emission was set to >3 for the aerobic system and >15 for the anaerobic system. The heatmaps of the relative intensity of ESW-enriched VOCs under different soil treatments were generated using Origin software (version 2021b).
2.4 16S rRNA gene sequencing and data analysis
The soil samples from different days of the SBS simulation (day 0/6/12/18/24/30) were collected in triplicate and sent to Majorbio Bio-Pharm Technology Co. Ltd. (Shanghai, China) for 16S rRNA gene sequencing. Sequences corresponding to the V3-V4 region of the bacteria 16S rRNA genes were amplified by PCR reactions with the primer pairs 338F (5’-ACTCCTACGGGAGGCAGCAG-3′) and 806R (5′- GGACTACHVGGGTWTCTAAT-3′). Amplicons were extracted from 2% agarose gels and purified using the AxyPrep DNA Gel Extraction Kit (Axygen Biosciences, Union City, CA, USA). Purified amplicons were then paired-end sequenced on an Illumina MiSeq PE300 platform (Illumina, San Diego, USA) and quantified with Quantus Fluorometer (Promega, USA). The sequencing data generated for this study have been deposited in the NCBI Sequence Read Archive (SRA) under the accession number PRJNA1190941.
The resulting raw data were obtained from the Majorbio and then demultiplexed, quality-filtered, and analyzed using the Quantitative Insights into Microbial Ecology II (QIIME II) software package (version 2023.5). The SILVA database (version 132) was used to phylogenetically bin the operational taxonomic units (OTUs). Relative abundances of bacterial phyla and genera, along with feature richness, Pielou’s evenness, and Shannon index, were calculated using RStudio (version 2023.06.1). The Vegan package was used to generate principal co-ordinates analysis (PCoA) plots from the Bray–Curtis dissimilarity matrix calculated from OTU abundance values.
2.5 Residual phytotoxicity and weed seed inactivation
The residual phytotoxicity of ESW-amended soils was evaluated using lettuce (Lactuca sativa L. var. capitata L) (Qingxianchunfeng Vegetable Breeding Cooperation, Hebei, China) growth assay in a similar way to previous studies (Achmon et al., 2016; Liang et al., 2022; Zou et al., 2023). Soils amended with 0, 2.5, 5, and 8% (w/w) of ESW were prepared in aerobic and anaerobic SBS simulation systems and incubated for 14 days. Immediately after incubation, equal amounts of soil mixtures (approximately 52 g) from each bioreactor were transferred into plastic seedling trays (6 *6 *5.3 cm3) and placed in a greenhouse. The trays were divided into three groups, with seeds sown on days 0, 3, and 7 after the simulated SBS closure. Six lettuce seeds were seeded equidistantly in each tray.
Only on day 0, fresh soils amended with 0, 2.5, 5, and 8% (w/w) of ESW, which had not undergone the simulated SBS process, were prepared as freshly prepared samples. Freshly prepared samples were also transferred into plastic seedling trays, placed in the greenhouse, and seeded with lettuce seeds in the same way. The greenhouse maintained an average daily temperature of around 25°C, and soil moisture was managed by adding distilled water twice daily at 9 am and 4 pm. Distilled water was used to ensure consistent water quality and eliminate potential variability in mineral content that could influence seedling growth. After 25 days of planting, the trays were returned to the laboratory, and the lettuce seedlings were harvested. The germination rate was calculated by counting the number of seedlings that emerged in each tray. Harvested seedlings were placed on a sheet of white paper for photography, and their shoot and root lengths were measured using ImageJ (Version 1.53, National Institutes of Health, Bethesda, MD). The average dry biomass of the seedlings was determined by weighing the seedlings after drying at 105°C for one day.
The efficacy of ESW-amended soils in inactivating unwanted weed seeds was assessed using a germination assay with Bidens pilosa in a similar way to previous studies (Achmon et al., 2016; Liang et al., 2022; Zou et al., 2023). Weed seeds were collected from the field where the soil samples were obtained. Six weed seeds were placed on the upper layer (approximately 5 cm below the soil surface) of ESW-amended soils (0, 2.5, 5, or 8% w/w) in each bioreactor within the aerobic and anaerobic SBS simulation systems. The experimental setup was designed to simulate conditions in which dormant Bidens pilosa seeds were already buried in the soil prior to field SBS treatment.
Following a 14-day incubation, the soil and weed seeds from each bioreactor were transferred into a plastic seedling tray and placed in the greenhouse. Freshly prepared samples, not subjected to the simulated SBS process, were also prepared accordingly on the same day. Over the 25-day greenhouse period, the emergence of weed seedlings was recorded daily, along with their sprouting days.
2.6 Data analysis
ANOVA analysis with post hoc Tukey’s Honest Significant Difference test was used to compare mean response values across soil groups. The familywise error rate equaled 0.05 for all comparisons. Statistical analyses were performed using SPSS (IBM SPSS Statistics 26).
3 Results and discussion
3.1 Properties of soil and eggshell waste before soil biosolarization treatment
The properties of the dried soil and ESW powder before the SBS treatment are presented in Table 1. The basic field soil was acidic with pH = 5.00 ± 0.07, containing a low amount of organic matter with VS = 9.6 ± 0.2% of dry solids (DS), and a low Ca concentration of about 1.17 mg/g DS.
In contrast, the ESW collected from the university canteen was alkaline (pH = 9.67 ± 0.07) and rich in nitrogen (C/N = 2.15 ± 0.08). The low C/N ratio is likely due to nitrogen-rich organic residues, primarily egg yolk and white, remaining on the ESW during collection. Additionally, the ESW had a high Ca content (approximately 43.09 mg/g DS) and a low percentage of organic matter (VS = 7.9 ± 0.3 %DS). The carbon-to-nitrogen ratio (C/N) of ESW was significantly lower than that of other reported soil amendments (C/N ≥ 30), suggesting that ESW may have a potential role in enriching soils that require nitrogen supplementation (Liang et al., 2022).
High acidity and low Ca concentration in the soil would lead to impaired bio-accessibility and hinder the normal development of plants (Quina et al., 2017). Many studies have demonstrated that ES is effective in regulating the pH of acidic soils and supplying the calcium necessary for crop growth (Elwakeel and Yousif, 2010; Cordeiro and Hincke, 2011). Although ESW has not previously been reported for use in SBS, its characteristics suggest it could be a valuable amendment.
3.2 Description of soil properties
Soil pH is an important factor for pest inactivation and is influenced by fermentation products, which significantly affect the efficacy of the SBS process (Momma et al., 2006). The results showed that SBS with ESW significantly increased soil pH in both aerobic and anaerobic conditions (p < 0.05). In aerobic conditions, all treated soils exhibited a significant increase in pH after the SBS process (Figure 1A). The pH of the control soil increased from 4.95 to 5.67, remaining within the acidic range. In contrast, the soil amended with 0.5% ESW showed the largest increase, rising from 6.00 to 7.62, reaching a neutral to slightly alkaline pH. The soil with 2.5% ESW exhibited the smallest increase, with pH rising from 7.46 to 8.41, becoming more alkaline. This is likely due to the higher initial pH of the soil amended with 2.5% ESW, resulting in a smaller net increase but a higher final pH.
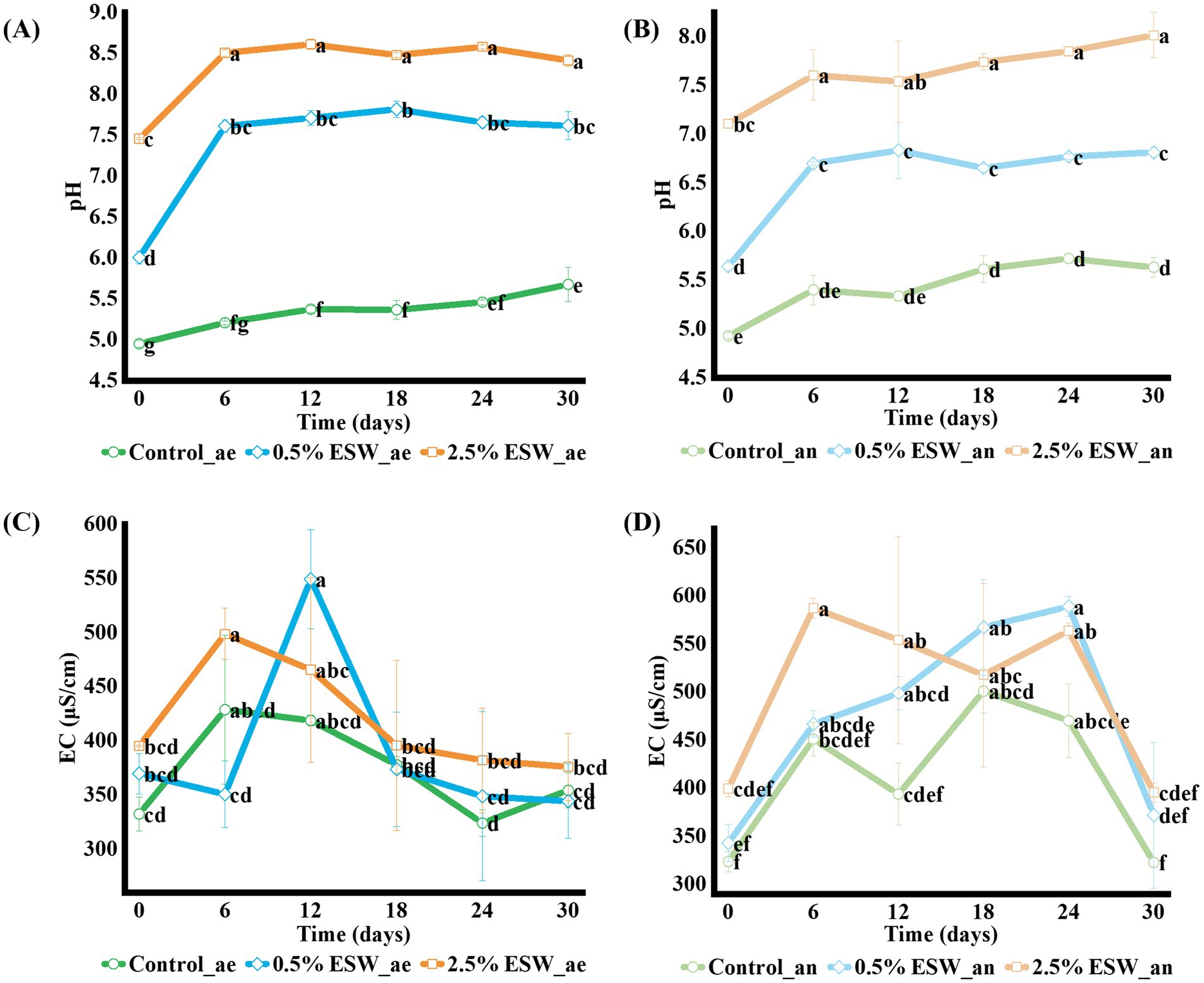
Figure 1. Changes in soil pH and electrical conductivity (EC) during simulated SBS processes. (A) Soil pH under aerobic conditions. (B) Soil pH under anaerobic conditions. (C) Soil EC under aerobic conditions. (D) Soil EC under anaerobic conditions. Control, control soil without ESW; 0.5% ESW, soil with 0.5% (w/w) of ESW; 2.5% ESW, soil with 2.5% (w/w) of ESW; ae, aerobic setting; an, anaerobic setting. Data represent the means of triplicates with a standard deviation. Data not connected by the same letter are significantly different (α = 0.05).
In anaerobic conditions, pH also increased significantly for all treated soils, mirroring the trends observed in aerobic conditions (p < 0.05) (Figure 1B). The pH of the control soil increased from 4.93 to 5.63, remaining in the acidic range. The soil amended with 0.5% ESW showed the greatest increase, with pH rising from 5.64 to 6.81, approaching neutral. In contrast, the soil with 2.5% ESW exhibited the smallest increase, with pH rising from 7.11 to 8.01, becoming more alkaline. As observed in aerobic conditions, the higher final pH at 2.5% ESW can be attributed to the initial alkalinity of the soil amended with 2.5% ESW, which resulted in a smaller net increase but a higher final pH. Studies have indicated that the higher pH resulting from increased Ca concentration in the soil can inhibit soil-borne plant pathogens associated with plant root diseases (He et al., 2014; Niwa et al., 2007). Thus, the application of Ca-rich ESW in the SBS process holds great potential for suppressing soil-borne plant pathogens and controlling plant root diseases. The addition of ESW did not significantly affect the VS content, likely due to the low organic matter in the ES. Detailed changes in VS content under both aerobic and anaerobic conditions are shown in Supplementary Figure S2.
Soil EC indicates the salinity levels and the ability of soil to conduct electrical current, which is closely related to the concentration of soluble salts. Optimum soil EC is crucial for plant growth, as it affects nutrient availability, water uptake, microbial activity, and more (Mishra et al., 2023). The results demonstrated that the addition of ESW did not significantly increase the soil EC value (Figures 1C,D). After 30 days of SBS treatment, the EC value of the amended soil remained unchanged. The addition of ESW did not lead to salinity in the soil, as the EC values in both aerobic and anaerobic conditions remained below 600 μS/cm, well below the threshold for saline soil (EC1:5 of 1,000 μS/cm or higher) (Rengasamy, 2010).
The contents of six nutrient elements in the soil were measured before and after 30 days of aerobic and anaerobic SBS simulations. The ESW amendment did not have a significant effect on the contents of C, N, P, and Mg in the soil (Figures 2A–D). Post-treatment, there were no significant differences in the levels of these four nutrients between the control soils and the amended soils. The addition of ESW led to a slight decrease in soil K content (Figure 2E). Following the aerobic SBS, soil K level decreased further, whereas, after anaerobic SBS treatment, soil K content showed a slight increase. The addition of 0.5% (w/w) of ESW did not significantly increase of Ca level in soil, while the addition of 2.5% (w/w) of ESW did result in a significant increase (p < 0.05) (Figure 2F). The results showed that 30 days of aerobic and anaerobic SBS treatment had little effect on Ca content across all soil groups.
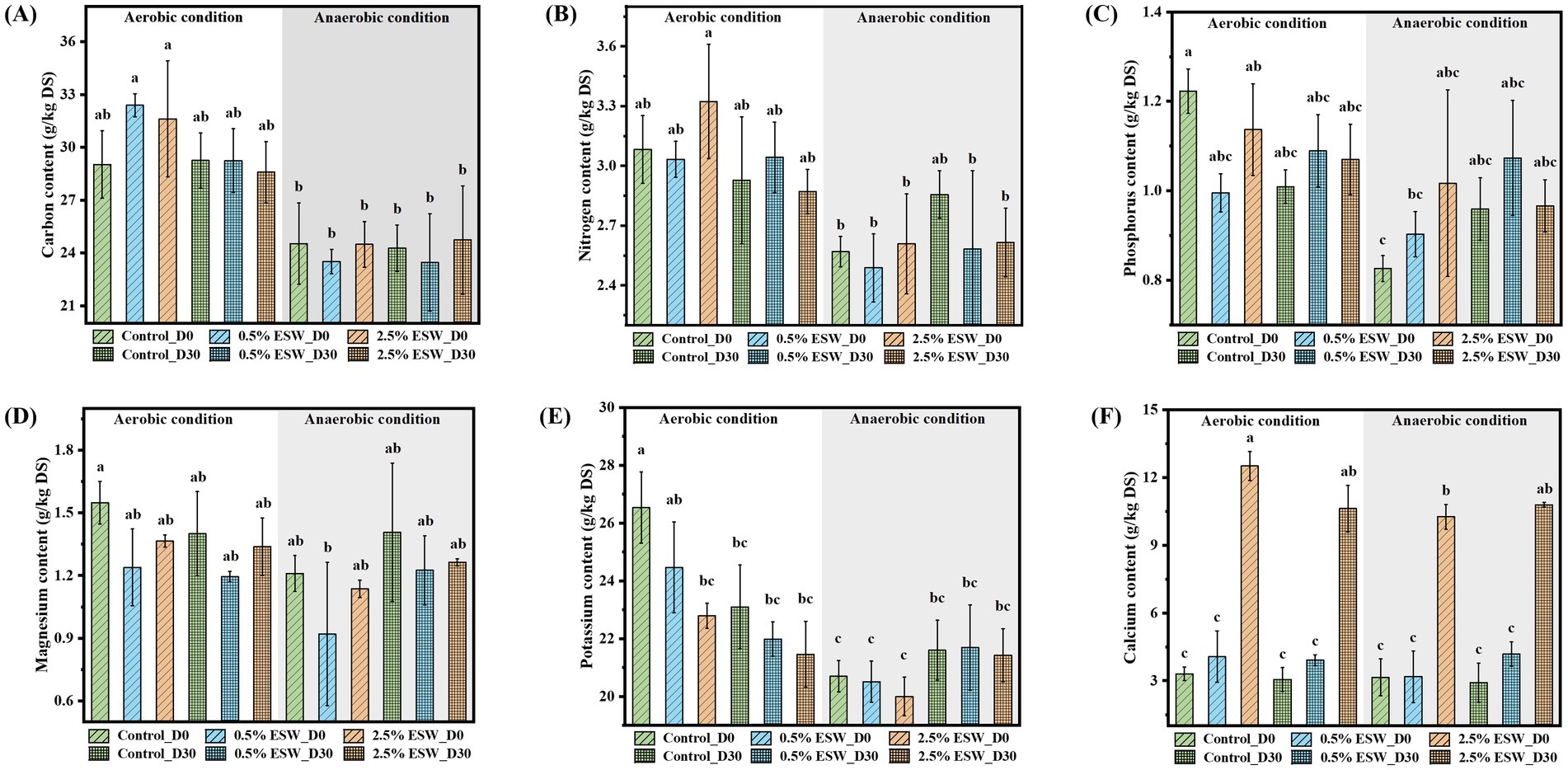
Figure 2. Six nutrient contents in the soil before and after aerobic and anaerobic SBS simulations: carbon (A), nitrogen (B), phosphorus (C), magnesium (D), potassium (E), and calcium (F). DS, dry solids; Control, control soil without ESW; 0.5% ESW, soil with 0.5% (w/w) of ESW; 2.5% ESW, soil with 2.5% (w/w) of ESW; D0, before SBS simulation; D30, after SBS simulation. Data represent the means of triplicates with a standard deviation. Data not connected by the same letter are significantly different (α = 0.05).
3.3 Evolution of gas emissions
3.3.1 Soil respiration
Soil respiration serves as an indicator of microbial activity during SBS, reflecting the microorganisms’ capacity to decompose organic amendment and the overall intensity of their activity (Achmon et al., 2016; Yazdanpanah et al., 2016). To assess soil respiration, the evolution of CO2, O2, and CH4 was constantly monitored over 30 days of simulated SBS in both aerobic and anaerobic conditions (Figure 3).
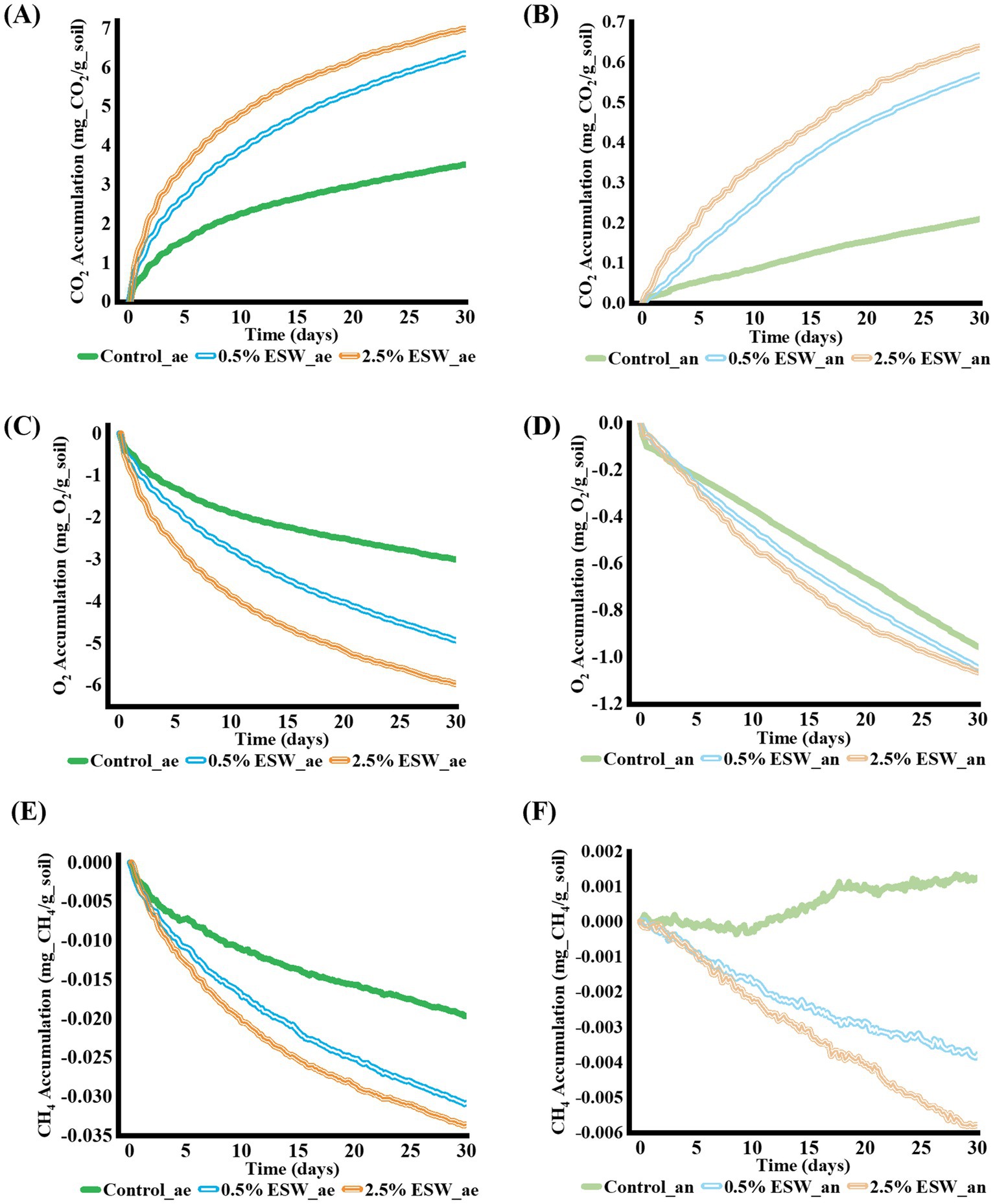
Figure 3. Soil respiration during simulated SBS processes. (A) CO₂ accumulation in soils under aerobic conditions. (B) CO₂ accumulation in soils under anaerobic conditions. (C) O₂ accumulation in soils under aerobic conditions. (D) O₂ accumulation in soils under anaerobic conditions. (E) CH₄ accumulation in soils under aerobic conditions. (F) CH₄ accumulation in soils under anaerobic conditions. Control, control soil without ESW; 0.5% ESW, soil with 0.5% (w/w) of ESW; 2.5% ESW, soil with 2.5% (w/w) of ESW; ae, aerobic setting; an, anaerobic setting. Data represent the means of triplicates.
The addition of ESW significantly increased soil respiration during both aerobic and anaerobic simulated SBS processes (p < 0.05). In aerobic conditions, soil amended with 0.5 and 2.5% ESW showed 1.8-fold and 2-fold increases in CO2 accumulation compared to the control, reaching 6.4 mg CO2/g soil and 7.0 mg CO2/g soil, respectively (Figure 3A). These levels were considerably lower than the 17 mg CO2/g soil reported for soils with 5% red wine grape waste (Achmon et al., 2016) and the 25 mg CO2/g soil observed in soils with 2% fish waste (Liang et al., 2022), potentially due to the lower organic content in ESW.
In anaerobic conditions, soil amended with 0.5 and 2.5% ESW showed 2.7-fold and 3-fold increases in CO2 accumulation compared to the control, reaching 0.57 mg CO2/g soil and 0.62 mg CO2/g soil, respectively (Figure 3B). These levels were also lower than the 3 mg CO2/g soil reported for soil with 2% fish waste (Liang et al., 2022). These results suggest that soil microbes efficiently utilized the added ESW, as evidenced by the significant increase in CO2 accumulation observed during both aerobic and anaerobic SBS simulations. Although the dissolution of calcium carbonate in ESW under acidic conditions may contribute to CO2 release (Tamir et al., 2011), the observed emissions were likely dominated by microbial activity. This conclusion is supported by the sustained CO2 evolution over the 30-day period, the parallel trends in O2 consumption under aerobic conditions, and continued CO2 accumulation under anaerobic conditions—none of which would be expected from a purely abiotic reaction. These findings collectively suggest active microbial respiration in response to the organic residues in ESW.
O2 consumption due to microbial activity in the soil was also monitored. As expected, during the simulated SBS processes, O2 consumption was positively correlated with CO2 production, with similar trends observed for both variables. In aerobic systems, soil amended with 2.5% ESW consumed nearly 6.0 mg of O2/g soil by the end of the 30-day test, while soil amended with 0.5% ESW consumed 4.9 mg of O2/g soil (Figure 3C). In anaerobic systems, soils amended with both concentrations of ESW consumed approximately 1.0 mg O2/g soil by the end of the 30-day test (Figure 3D).
When conducting waste management, it is crucial to consider potential negative environmental effects. The release of gases from soil is often associated with greenhouse gases (GHGs). Since CH4 is a potent GHG, its release could undermine the feasibility of SBS application (Liang et al., 2022). In soils amended with ESW, no positive CH4 accumulation was observed during either the aerobic or anaerobic SBS processes, which is a favorable outcome for SBS (Figures 3E,F).
3.3.2 Volatile organic compound emissions
Soils are sources of volatile organic compounds (VOCs), many of which are produced by microorganisms and referred to as microbial VOCs (mVOCs) (Insam and Seewald, 2010). These compounds have been demonstrated to be rapid, sensitive, and functional indicators of biological soil quality (Brown et al., 2021). Previous SBS studies suggest that the VOC profiles can act as distinctive fingerprints for characterizing the simulated SBS process (Liang et al., 2022; Zou et al., 2023).
Principal component analysis (PCA) revealed that the greatest differences, under both aerobic and anaerobic conditions, were observed between the control soil and the soil amended with 2.5% ESW (Figures 4A,B). By comparing the VOC intensities between the two groups, the top VOCs significantly enriched by the 2.5% ESW amendment were identified under both aerobic and anaerobic conditions (Figures 4C,D). The tentative identities of these compounds were determined through further analysis using PTRMS_Viewer software, as shown in Table 2. In both aerobic and anaerobic conditions, 2.5% ESW amendment significantly enhanced the release of ammonia, methanethiol, and dimethyl sulfide (DMS). Notably, methanethiol and DMS were also significantly increased in a previous SBS simulation with fish waste (Liang et al., 2022). This is an important finding because DMS is a mVOC known to have inhibitory effects on nematodes and pathogenic fungi (de Boer et al., 2019). Methanethiol, an intermediate in the formation of DMS, can be catalyzed into DMS, potentially amplifying its suppressive effects (Abis et al., 2016). Additionally, ammonia is known to exert inhibitory effects on plant-parasitic nematodes and fungi for a relatively short duration (days to weeks, depending on the soil environment) (Oka, 2010).
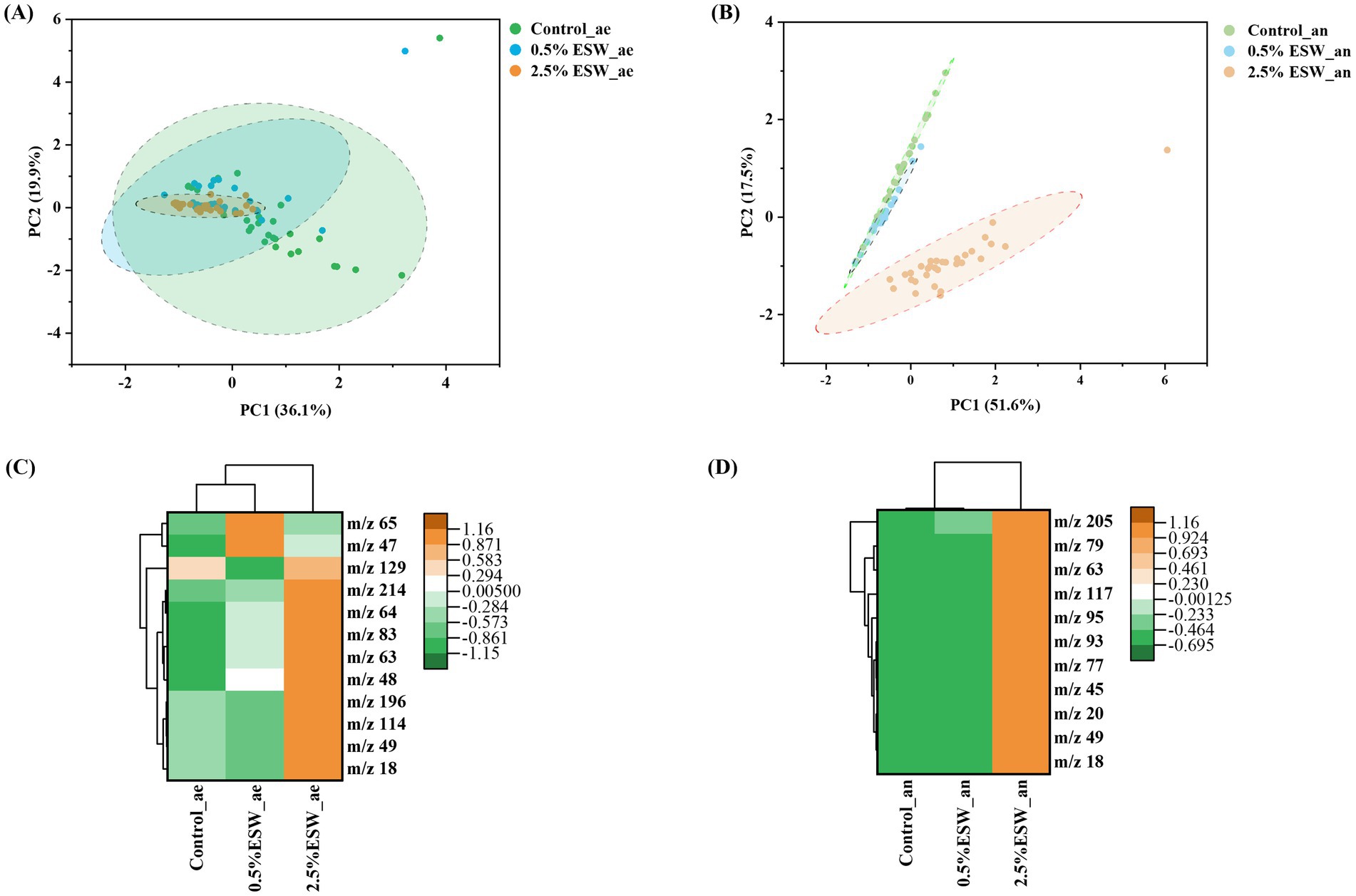
Figure 4. Soil VOC emission profiles of simulated SBS systems. (A) PCA plot of VOCs accumulation from soils under aerobic conditions. (B) PCA plot of VOCs accumulation from soils under anaerobic conditions. (C) Heatmap of the top 12 ESW enriched-VOCs in aerobic simulated SBS systems. (D) Heatmap of the top 11 ESW enriched-VOCs in anaerobic simulated SBS systems. Control, control soil without ESW; 0.5% ESW, soil with 0.5% (w/w) of ESW; 2.5% ESW, soil with 2.5% (w/w) of ESW; ae, aerobic setting; an, anaerobic setting. Ellipses represent 95% confidence intervals for the centroids associated with each cluster.
In aerobic conditions, the 2.5% ESW amendment also significantly increased the emissions of formic acid and octanal, with intensity ratios of 4.8 and 7.3, respectively (Table 2). Formic acid is a nematicidal compound, typically generated as part of the group of short-chain fatty acids during the decomposition of amendments in saturated soils (Oka, 2010). Similarly, octanal, an aldehyde, is recognized for its fungicidal activities. It has been shown to inhibit the growth of Aspergillus flavus (Li et al., 2021) and Guignardia citri-auranti (Zhou et al., 2014), pathogens that are responsible for significant crop damage.
In anaerobic conditions, the 2.5% ESW amendment also significantly increased the emissions of acetaldehyde, with intensity ratios of 25.6 (Table 2). Acetaldehyde has been reported to exhibit antimicrobial activity, effectively inactivating fungi, bacteria, and various yeast species commonly found in fruits and vegetables (Abd-Alla et al., 2013). It should also be noted that the concentration of benzene and toluene, both recognized soil pollutants (García Pinto et al., 2011), is also more evident in soil with 2.5% ESW compared to the control soil. Further study is needed to ensure that their levels are kept within an acceptable range.
3.4 Changes in bacterial community composition and diversity
3.4.1 Alpha and beta diversities
The alpha diversity metrics, including observed features, Pielou’s evenness, and the Shannon index, were calculated to assess the richness and evenness of bacterial species in each soil treatment (Control, 0.5% ESW, and 2.5% ESW) at different time points (day 0, 6, 12, 18, 24, and 30). On day 0, immediately following amendment, the three alpha diversity indices showed higher levels in the soils before the aerobic SBS simulation. However, these differences were not statistically significant (Figures 5A–C). After 6 days of aerobic SBS simulation, a significant decrease in alpha diversity was observed across all treatments (p < 0.05), as expected due to the selective pressures imposed by elevated soil temperatures on microbial communities (Haber et al., 2022; Riah-Anglet et al., 2015). Interestingly, soils amended with ESW maintained significantly higher alpha diversity indices compared to the control soils (p < 0.05), suggesting that ESW provided a buffering effect. This observation diverges from previous studies, where SBS processes introduced additional selective pressures due to the high concentrations of VOCs, primarily volatile fatty acids, which intensified microbial community shifts and led to more pronounced declines in diversity (Haber et al., 2022; Shea et al., 2022). From day 6 to day 30, the alpha diversity levels remained stable across all treatments, suggesting that the bacterial community had likely reached a stable state after the initial disturbance caused by the aerobic SBS simulation with ESW. This stabilization is commonly observed in systems subjected to stress perturbations, where microbial communities initially shift but later stabilize as they adapt to the new conditions (Shade et al., 2012).
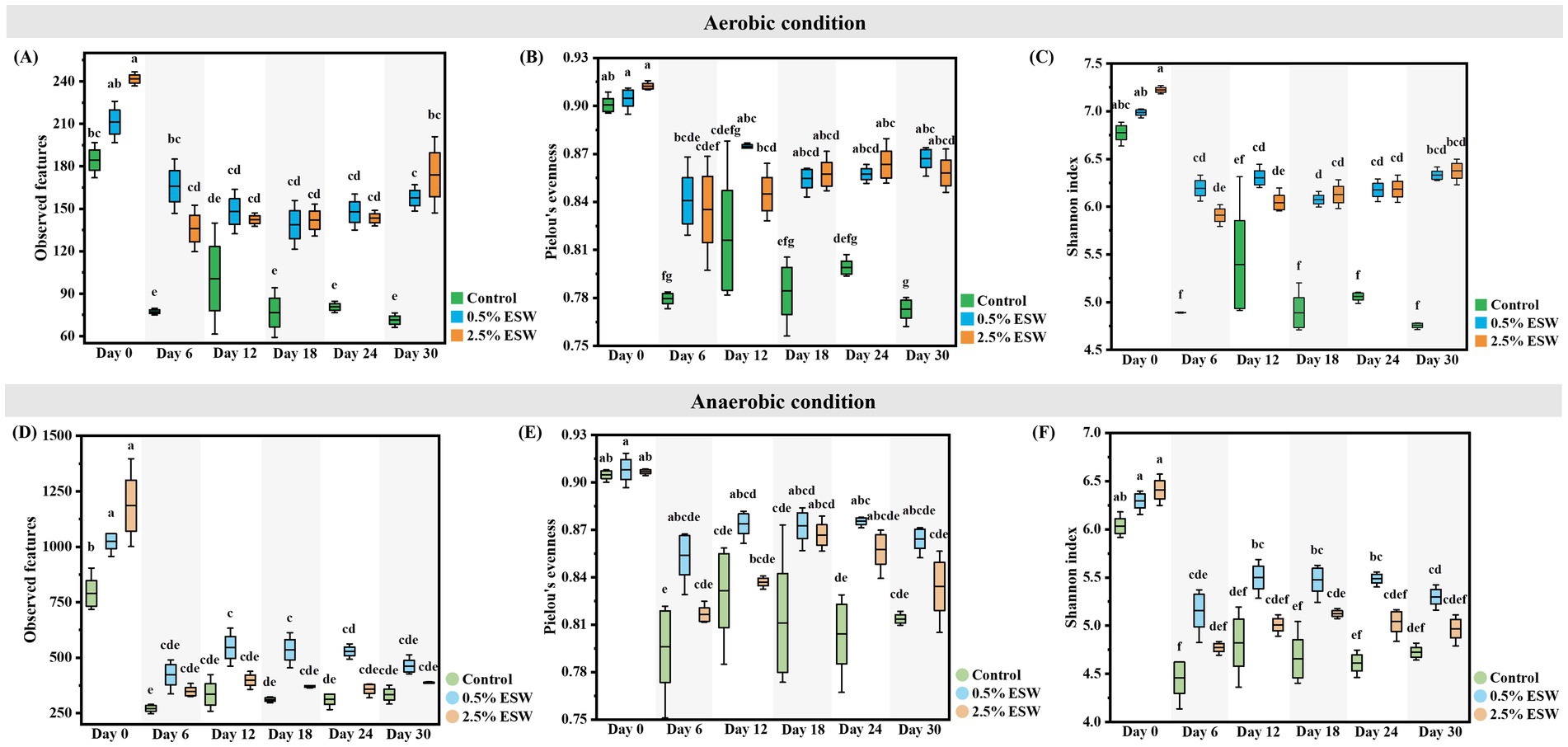
Figure 5. Alpha diversity metrics for soil bacterial communities during the (A–C) aerobic SBS simulation and (D–F) anaerobic SBS simulation. Control, control soil without ESW; 0.5% ESW, soil with 0.5% (w/w) of ESW; 2.5% ESW, soil with 2.5% (w/w) of ESW; Day 0/6/12/18/24/30, different soil sampling time points from the beginning of SBS simulation. Data represent the means of triplicates with a standard deviation. Data not connected by the same letter are significantly different (α = 0.05).
In contrast, soils immediately after amendment but before anaerobic SBS simulation (day 0) exhibited significantly higher observed features compared to the control (p < 0.05). However, there were no significant differences in Pielou’s evenness or Shannon index (Figures 5D–F). After 6 days of anaerobic SBS simulation, these alpha diversity metrics were also significantly reduced in all soils, consistent with expectations due to the elevated temperatures (Haber et al., 2022; Riah-Anglet et al., 2015). Notably, no significant differences were observed between control and amended soils, indicating that the presence of ESW neither exacerbated nor mitigated the loss in alpha diversity. From day 6 to day 30, alpha diversity levels remained stable across all treatments, indicating that the bacterial community had likely reached a steady state after the initial disturbance caused by the anaerobic simulated SBS process (Shade et al., 2012).
Principal Coordinates Analysis (PCoA) using Bray–Curtis dissimilarity indices was conducted to evaluate changes in bacterial community composition across all soil treatments and time points based on the relative abundance of taxa (Figure 6). On day 0, before the SBS simulations, bacterial communities across all treatments clustered tightly, indicating similar initial community structures. However, separations were observed between day 0 and subsequent time points for all treatments, reflecting the pronounced effect of soil heating on the microbiome (Shea et al., 2022). Furthermore, the PCoA plot revealed significant differences between ESW-amended soils and control soils under both aerobic and anaerobic conditions, confirming that the ESW amendment significantly altered bacterial community composition (p < 0.05) during the simulated SBS process, consistent with previous studies (Achmon et al., 2020; Haber et al., 2022; Shea et al., 2022).
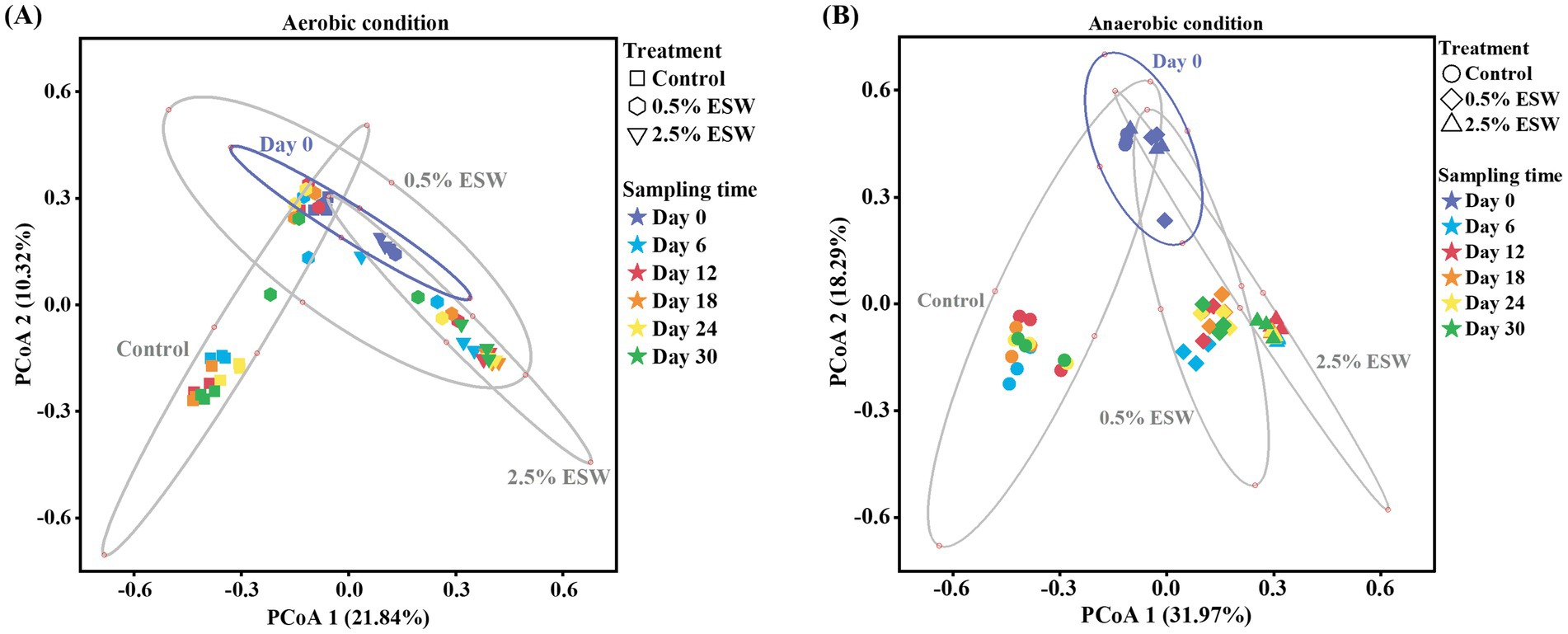
Figure 6. Principal coordinate analysis (PCoA) plots based on Bray-Curtis’s dissimilarity of OTU counts between treatments and time points during the (A) aerobic SBS simulation and (B) anaerobic SBS simulation. Control, control soil without ESW; 0.5% ESW, soil with 0.5% (w/w) of ESW; 2.5% ESW, soil with 2.5% (w/w) of ESW; Day 0/6/12/18/24/30, different soil sampling time points from the beginning of SBS simulation. Ellipses represent 95% confidence intervals for centroids associated with each cluster: all soil treatments at day 0, control soil at all time points, soil with 0.5% ESW at all time points, and soil with 2.5% ESW at all time points.
3.4.2 Relative abundance
The relative abundance of dominant bacterial phyla and genera across treatments and time points during aerobic and anaerobic SBS simulations with ESW is shown in Figure 7. During the aerobic SBS simulation process, on day 0, the dominant bacterial phyla identified in both control and ESW-amended soils included Firmicutes, Actinobacteriota, Proteobacteria, and Chloroflexi. Together with Planctomycetota and Acidobacteriota, these phyla accounted for approximately 94% of the bacterial community (Figure 7A). At the genus level, the most abundant taxa was Bacillus (Figure 7B). These findings are consistent with previous studies on SBS using tomato pomace (Achmon et al., 2020) and nonpareil almond residue (Shea et al., 2022) as organic amendments, which similarly reported an abundance of Firmicutes and Actinobacteriota in the initial soil microbiome.
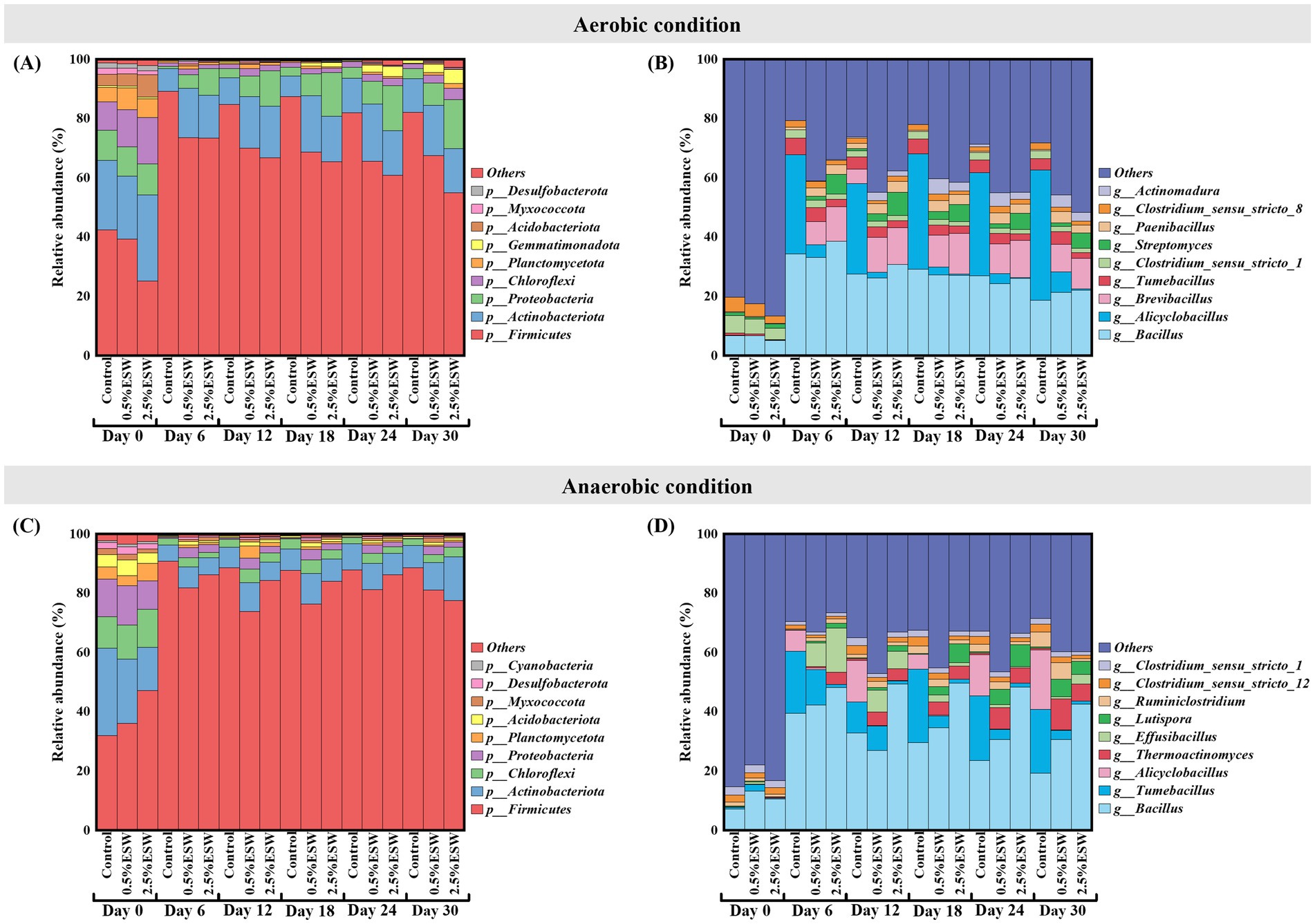
Figure 7. Relative abundance of dominant bacterial phyla and genera during SBS simulations. (A) Dominant bacterial phyla in aerobic SBS simulation. (B) Dominant bacterial genera in aerobic SBS simulation. (C) Dominant bacterial phyla in anaerobic SBS simulation. (D) Dominant bacterial genera in anaerobic SBS simulation. Control, control soil without ESW; 0.5% ESW, soil with 0.5% (w/w) of ESW; 2.5% ESW, soil with 2.5% (w/w) of ESW; Day 0/6/12/18/24/30, different soil sampling time points from the start of SBS simulation. Data represent the means of triplicates.
After 6 days of aerobic SBS simulation, the bacterial community structure of all treated soils changed. Firmicutes were notably enriched, comprising about 89% of all OTUs in control soil and 73% in ESW-amended soils. Previous studies suggest that Firmicutes play a key role in SBS efficacy due to their ability to degrade polysaccharides, ferment, and produce organic acids (Fernández-Bayo et al., 2019; Shea et al., 2022). The increase in Firmicutes was primarily driven by Bacillus, which rose from around 6 to 35% of the bacterial community in all treated soils. Additionally, Alicyclobacillus showed a significant increase in control soil, rising from near-zero to a relative abundance of 33%. This acidophilic and thermophilic bacterium, often linked to fruit juice spoilage, can contaminate fresh fruit before harvest and is difficult to eliminate during processing (Groenewald et al., 2009). In contrast, its relative abundance in ESW-amended soils remained below 4%, which may suggest that the ESW amendment effectively suppressed this bacterium, possibly by raising the soil pH to an alkaline level. Furthermore, ESW amendments increased the relative abundance of Brevibacillus and Paenibacillus, both from the Paenibacillaceae family. These bacteria promote crop growth and provide protection against insect herbivores and phytopathogens, including bacteria, fungi, nematodes, and viruses, through the production of various antimicrobials and insecticides (Grady et al., 2016; Ray et al., 2020). Additionally, Streptomyces and Actinomadura, members of the Actinomycetia class, were more abundant in ESW-amended soils compared to control soil. These bacteria are also active producers of antibiotics and volatile organic compounds, contributing to the biological control of pests and plant diseases, as well as promoting plant growth (Silva et al., 2022; Vurukonda et al., 2018). From day 6 to day 30, the abundance of dominant bacterial phyla/genus gradually decreased, while others increased, suggesting a shift toward a new equilibrium in the bacterial community.
On day 0 of the anaerobic SBS simulation, the dominant bacterial phyla in both control and ESW-amended soils were Firmicutes, Actinobacteriota, Chloroflexi, and Proteobacteria, with Planctomycetota and Acidobacteriota comprising approximately 91% of the bacterial community (Figure 7C). At the genus level, Bacillus was the most abundant taxa (Figure 7D). These patterns were similar to those observed in the soils before the start of the aerobic SBS simulation.
After 6 days of anaerobic SBS simulation, the microbial community structure of all treated soils shifted, with Firmicutes becoming more abundant while other phyla decreased. At the genus level, Bacillus was the most abundant in all treated soils. A significant increase in Alicyclobacillus was observed in the control soil, but its abundance was minimal in ESW-amended soils, likely due to the increase in soil pH. These changes were similar to those observed in the aerobic SBS simulation. Tumebacillus, a mesophilic bacterium related to Alicyclobacillus, also increased significantly in control soil (from 1 to 21%), but to a lesser extent in ESW-amended soils (from 2 to 12% in soil with 0.5% ESW, and from 0 to 1% in soil with 2.5% ESW). Tumebacillus, discovered in 2008, has an unclear relationship with soil health and plants, though recent research highlights its potential for producing novel natural products (Kikuchi et al., 2023). Effusibacillus, a thermophilic bacterium in the Alicyclobacillaceae family (Konishi et al., 2021), showed an increase in ESW-amended soils (from 1 to 8% in soil with 0.5% ESW; from 0 to 15% in soil with 2.5% ESW), while it was virtually absent in control soils. Two additional thermophilic bacteria, Thermoactinomyces and Lutispora, both of which are efficient at decomposing organic matter in high-temperature composting environments (Liu et al., 2018; Oumaima et al., 2024; Wang et al., 2023), also showed increased abundance in ESW-amended soils compared to control soil. These findings suggest that ESW amendment may enhance soil heating during anaerobic SBS simulation, potentially aiding in the control of soilborne pathogens through thermo-inactivation (Stapleton, 2000).
From day 6 to day 30, the bacterial community structure at the phylum level remained relatively stable. At the genus level, Bacillus declined more sharply in control soil (from 40 to 19%) compared to ESW-amended soils (from 42 to 31% in soil with 0.5% ESW; from 48 to 43% in soil with 2.5% ESW). Alicyclobacillus continued to increase in control soil (from 7 to 20%) but remained negligible in ESW-amended soils. Three thermophilic genera, Effusibacillus, Thermoactinomyces, and Lutispora, were unable to reestablish in control soil, remaining nearly absent in their relative abundance. In contrast, Effusibacillus slightly declined in ESW-amended soils, while Thermoactinomyces and Lutispora showed slight increases. These trends suggest that ESW amendments may selectively promote the growth of Thermoactinomyces and Lutispora, likely due to their ability to metabolize ESW substrates and adapt to conditions such as elevated pH and nutrient availability.
3.5 Residual phytotoxicity and weed seed inactivation
Soil phytotoxicity was evaluated through lettuce germination tests, which measured germination rate, shoot length, root length, and dry biomass (Figure 8). The germination rate of lettuce seeds showed no significant differences across all tested soils and seeding days (Figure 8A), indicating that soils amended with ESW did not exhibit high levels of phytotoxicity before or after SBS simulations. Freshly prepared amended soils and those subjected to aerobic SBS simulation did not significantly affect seedling biomass. In contrast, soils treated under anaerobic SBS conditions resulted in significantly lower seedling biomass compared to non-amended soil (p < 0.05) (Figure 8B), likely due to the accumulation of phytotoxic compounds. The reduction in biomass diminished with longer aeration time (or seeding days), suggesting that the phytotoxic compounds may be volatile, as reported in previous studies (Achmon et al., 2018; Shea et al., 2021). Therefore, aerating the soil before sowing can help mitigate the impact of volatile phytotoxic substances on crop growth. Shoot lengths of lettuce seedlings were minimally affected by all soil treatments, whereas root elongation was significantly inhibited by both aerobic and anaerobic SBS simulations (p < 0.05) (Figures 8C,D). Similar observations have been reported in SBS tests using tomato and grape residues, where residual phytotoxicity primarily affected root development rather than shoot growth (Achmon et al., 2016). Root growth in amended soils gradually recovered with extended aeration, showing no significant difference from the control soil after seven days of aeration before seeding. This supports the idea that phytotoxic compounds may be volatile and can disperse from the soil over time with adequate aeration.
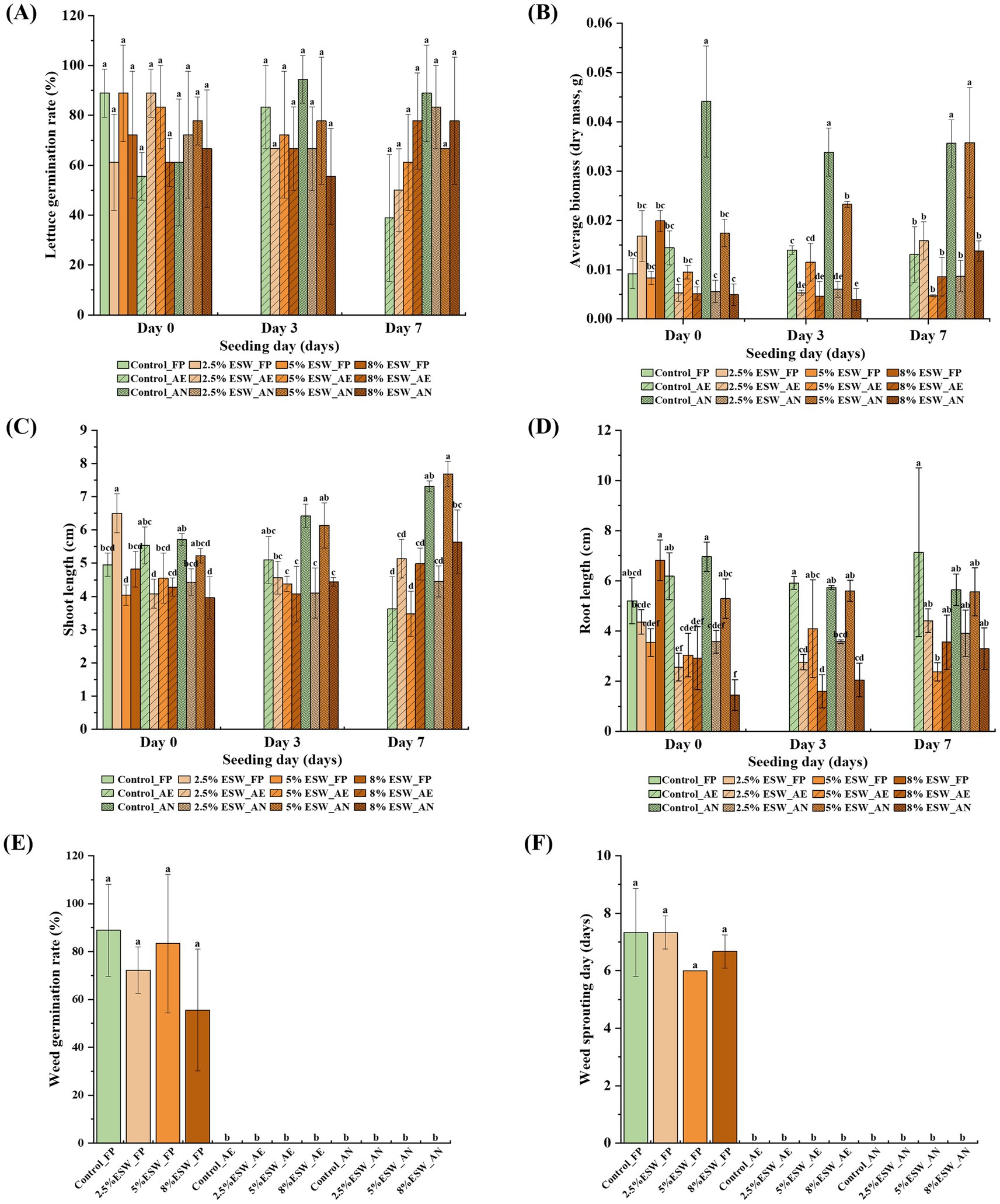
Figure 8. Residual phytotoxicity and weed seed inactivation in soils amended with ESW. Lettuce seedling growth was evaluated by measuring four parameters of phytotoxicity: (A) germination rate (%), (B) dry biomass (g), (C) shoot length (cm), and (D) root length (cm). Weed seed inactivation was assessed by two parameters: (E) weed germination rate (%) and (F) sprouting day (the day the first Bidens sprout was observed). Control, control soil without ESW; 0.5% ESW, soil with 0.5% (w/w) of ESW; 2.5% ESW, soil with 2.5% (w/w) of ESW; 5% ESW, soil with 5% (w/w) of ESW; 8% ESW, soil with 8% (w/w) of ESW; FR, freshly prepared soils; AE, aerobic SBS-treated soils; AN, anaerobic SBS-treated soils; Day 0/3/7, days after the closure of SBS simulation to seeding. Data represent the means of triplicates with a standard deviation. Data not connected by the same letter are significantly different (α = 0.05).
Weed seed inactivation tests were conducted to evaluate the inhibitory potential of SBS with ESW on weed seed germination, specifically to determine whether the addition of ESW interfered with or enhanced the herbicidal effect of SBS. The weed species Bidens pilosa, a cosmopolitan annual herb known for its hardiness and explosive reproductive potential (Arthur et al., 2012), was used in this study, as in previous SBS studies, to examine its response to weed inactivation (Liang et al., 2022; Zou et al., 2023). In freshly prepared soils, the addition of ESW had no significant effect on weed germination or sprouting days. However, in all soils treated with simulated SBS, weed germination was completely inhibited, with a germination rate of zero, significantly lower than in freshly prepared soils (p < 0.05). This suggests that the high temperatures during the simulated SBS process were sufficient to effectively inactivate weed seeds, regardless of the presence of ESW. Therefore, while this study could not determine whether ESW contributed to weed inhibition, it did not interfere with the weed control efficacy of simulated SBS, which yielded satisfactory results. Future studies should explore different weed species to further assess the role of SBS and ESW in weed suppression.
4 Conclusion
This study is the first to evaluate the feasibility of using ESW as a soil amendment in SBS, presenting a sustainable agricultural strategy for managing organic waste. The findings demonstrate that ESW amendments effectively adjusted soil pH, stimulated microbial activity, and promoted the emission of functional VOCs linked to pest suppression, without contributing to GHG effects. Additionally, ESW supported the growth of beneficial bacteria associated with soilborne pest suppression and plant growth promotion. Furthermore, it effectively inactivated weed seeds and maintained low residual phytotoxicity. These findings highlight ESW’s potential to enhance SBS efficiency while promoting sustainable waste recycling in agriculture. Future research, including field trials, is needed to validate these findings and evaluate ESW’s real-world applicability in SBS.
Data availability statement
The datasets presented in this study can be found in online repositories. The names of the repository/repositories and accession number(s) can be found in the article/Supplementary material.
Author contributions
CL: Conceptualization, Formal analysis, Investigation, Supervision, Writing – original draft, Writing – review & editing. PY: Data curation, Writing – review & editing. YZ: Data curation, Writing – review & editing. BQ: Data curation, Writing – review & editing. MC: Investigation, Writing – review & editing. DC: Investigation, Writing – review & editing. ZZ: Investigation, Writing – review & editing. ZC: Investigation, Writing – review & editing. SY: Methodology, Resources, Supervision, Writing – review & editing. YA: Conceptualization, Methodology, Project administration, Resources, Supervision, Writing – review & editing.
Funding
The author(s) declare that financial support was received for the research and/or publication of this article. This research was supported by the 2024 Li Ka Shing Foundation STU-GTIIT joint research grants (2024LKSFG01). It was also supported by the seed grant provided by the China-Israel Changzhou Innovation Park (CICP) (GCII-Seed-202401). This research was also partially supported by the “Climbing Plan” project funded by the Guangdong Provincial Science and Technology Innovation Strategy Special Fund. The authors acknowledge the support of the Guangdong Provincial Key Laboratory of Materials and Technologies for Energy Conversion.
Acknowledgments
Parts of this work were previously published in preprint form on SSRN 4561982 (Li et al., 2023b) and SSRN 4611906 (Li et al., 2023a). The authors acknowledge the contribution of these preprints in providing an initial framework for the current study.
Conflict of interest
The authors declare that the research was conducted in the absence of any commercial or financial relationships that could be construed as a potential conflict of interest.
Generative AI statement
The author(s) declare that no Gen AI was used in the creation of this manuscript.
Publisher’s note
All claims expressed in this article are solely those of the authors and do not necessarily represent those of their affiliated organizations, or those of the publisher, the editors and the reviewers. Any product that may be evaluated in this article, or claim that may be made by its manufacturer, is not guaranteed or endorsed by the publisher.
Supplementary material
The Supplementary material for this article can be found online at: https://www.frontiersin.org/articles/10.3389/fsufs.2025.1543216/full#supplementary-material
References
Abd-Alla, M. A., El-Mougy, N. S., Abd-El-K Ade, M. M., Abd-El-Karee, F., El-Gamal, N. G., and El-Mohame Dy, R. S. (2013). Aldehydes compounds for controlling black scurf disease of potato (Solanum Tubrosum L.) under field conditions. International. J. Agric. For. 2013, 34–39. doi: 10.5923/j.ijaf.20130302.02
Abis, L., Ciuraru, R., Lafouge, F., Loubet, B., Maron, P.-A., and Sadet-Bourgeteau, S. (2016). Review: microbial volatile organic compounds (mVOCs) emissions by soil. SOMmic.
Achmon, Y., Claypool, J. T., Fernández-Bayo, J. D., Hernandez, K., McCurry, D. G., Harrold, D. R., et al. (2020). Structural changes in bacterial and fungal soil microbiome components during biosolarization as related to volatile fatty acid accumulation. Appl. Soil Ecol. 153:103602. doi: 10.1016/j.apsoil.2020.103602
Achmon, Y., Fernández-Bayo, J. D., Hernandez, K., McCurry, D. G., Harrold, D. R., Su, J., et al. (2017). Weed seed inactivation in soil mesocosms via biosolarization with mature compost and tomato processing waste amendments. Pest Manag. Sci. 73, 862–873. doi: 10.1002/ps.4354
Achmon, Y., Harrold, D. R., Claypool, J. T., Stapleton, J. J., VanderGheynst, J. S., and Simmons, C. W. (2016). Assessment of tomato and wine processing solid wastes as soil amendments for biosolarization. Waste Manag. 48, 156–164. doi: 10.1016/j.wasman.2015.10.022
Achmon, Y., Sade, N., Fernández-Bayo, J. D., Harrold, D. R., Stapleton, J. J., VanderGheynst, J. S., et al. (2018). Effects of short-term biosolarization using mature compost and industrial tomato waste amendments on the generation and persistence of biocidal soil conditions and subsequent tomato growth. J. Agric. Food Chem. 66, 5451–5461. doi: 10.1021/acs.jafc.8b00424
Ahmed, T. A. E., Wu, L., Younes, M., and Hincke, M. (2021). Biotechnological applications of eggshell: recent advances. Front. Bioeng. Biotechnol. 9:675364. doi: 10.3389/fbioe.2021.675364
Arthur, G. D., Naidoo, K. K., and Coopoosamy, R. M. (2012). Bidens pilosa L.: Agricultural and pharmaceutical importance. J. Med. Plants Res. 6, 3282–3287. doi: 10.5897/JMPR012.195
Baláž, M., Boldyreva, E. V., Rybin, D., Pavlović, S., Rodríguez-Padrón, D., Mudrinić, T., et al. (2021). State-of-the-art of eggshell waste in materials science: recent advances in catalysis, pharmaceutical applications, and mechanochemistry. Front. Bioeng. Biotechnol. 8:1522. doi: 10.3389/FBIOE.2020.612567/BIBTEX
Brown, R. W., Bull, I. D., Journeaux, T., Chadwick, D. R., and Jones, D. L. (2021). Volatile organic compounds (VOCs) allow sensitive differentiation of biological soil quality. Soil Biol. Biochem. 156:108187. doi: 10.1016/j.soilbio.2021.108187
Chaparro, J. M., Sheflin, A. M., Manter, D. K., and Vivanco, J. M. (2012). Manipulating the soil microbiome to increase soil health and plant fertility. Biol. Fertil. Soils 48, 489–499. doi: 10.1007/s00374-012-0691-4
Cordeiro, C. M. M., and Hincke, M. T. (2011). Recent patents on eggshell: shell and membrane applications. Recent Patents Food Nutr. Agricult. 3, 1–8. doi: 10.2174/2212798411103010001
de Boer, W., Li, X., Meisner, A., and Garbeva, P. (2019). Pathogen suppression by microbial volatile organic compounds in soils. FEMS Microbiol. Ecol. 95:fiz105. doi: 10.1093/femsec/fiz105
Domínguez, P., Miranda, L., Soria, C., De Los Santos, B., Chamorro, M., Romero, F., et al. (2014). Soil biosolarization for sustainable strawberry production. Agron. Sustain. Dev. 34, 821–829. doi: 10.1007/s13593-014-0211-z
Elwakeel, K. Z., and Yousif, A. M. (2010). Adsorption of malathion on thermally treated egg shell material. Water Sci. Technol. 61, 1035–1041. doi: 10.2166/wst.2010.005
Fernández-Bayo, J. D., Achmon, Y., Harrold, D. R., McCurry, D. G., Hernandez, K., Dahlquist-Willard, R. M., et al. (2017). Assessment of two solid anaerobic digestate soil amendments for effects on soil quality and biosolarization efficacy. J. Agric. Food Chem. 65, 3434–3442. doi: 10.1021/acs.jafc.6b04816
Fernández-Bayo, J. D., Hestmark, K. V., Claypool, J. T., Harrold, D. R., Randall, T. E., Achmon, Y., et al. (2019). The initial soil microbiota impacts the potential for lignocellulose degradation during soil solarization. J. Appl. Microbiol. 126, 1729–1741. doi: 10.1111/jam.14258
Fernández-Bayo, J. D., Randall, T. E., Harrold, D. R., Achmon, Y., Hestmark, K. V., Su, J., et al. (2018). Effect of management of organic wastes on inactivation of Brassica nigra and fusarium oxysporum f.sp. lactucae using soil biosolarization. Pest Manag. Sci. 74, 1892–1902. doi: 10.1002/ps.4891
Fernandez-Bayo, J. D., Shea, E. A., Parr, A. E., Achmon, Y., Stapleton, J. J., VanderGheynst, J. S., et al. (2020). Almond processing residues as a source of organic acid biopesticides during biosolarization. Waste Manag. 101, 74–82. doi: 10.1016/j.wasman.2019.09.028
Ferraz, E., Gamelas, J. A. F., Coroado, J., Monteiro, C., and Rocha, F. (2018). Eggshell waste to produce building lime: calcium oxide reactivity, industrial, environmental and economic implications. Mater. Struct. 51:115. doi: 10.1617/s11527-018-1243-7
García Pinto, C., Herrero Martín, S., Pérez Pavón, J. L., and Moreno Cordero, B. (2011). A simplified quick, easy, cheap, effective, rugged and safe approach for the determination of trihalomethanes and benzene, toluene, ethylbenzene and xylenes in soil matrices by fast gas chromatography with mass spectrometry detection. Anal. Chim. Acta 689, 129–136. doi: 10.1016/j.aca.2011.01.023
Grady, E. N., MacDonald, J., Liu, L., Richman, A., and Yuan, Z. C. (2016). Current knowledge and perspectives of Paenibacillus: a review. Microb. Cell Factories 15:203. doi: 10.1186/s12934-016-0603-7
Groenewald, W. H., Gouws, P. A., and Witthuhn, R. C. (2009). Isolation, identification and typification of Alicyclobacillus acidoterrestris and Alicyclobacillus acidocaldarius strains from orchard soil and the fruit processing environment in South Africa. Food Microbiol. 26, 71–76. doi: 10.1016/j.fm.2008.07.008
Haber, Z., Wilhelmi, M. D. M. R., Fernández-Bayo, J. D., Harrold, D. R., Stapleton, J. J., Toubiana, D., et al. (2022). The effect of circular soil biosolarization treatment on the physiology, metabolomics, and microbiome of tomato plants under certain abiotic stresses. Front. Plant Sci. 13:1009956. doi: 10.3389/fpls.2022.1009956
He, K., Yang, S. Y., Li, H., Wang, H., and Li, Z. L. (2014). Effects of calcium carbonate on the survival of Ralstonia solanacearum in soil and control of tobacco bacterial wilt. Eur. J. Plant Pathol. 140, 665–675. doi: 10.1007/s10658-014-0496-4
Hestmark, K. V., Fernández-Bayo, J. D., Harrold, D. R., Randall, T. E., Achmon, Y., Stapleton, J. J., et al. (2019). Compost induces the accumulation of biopesticidal organic acids during soil biosolarization. Resour. Conserv. Recycl. 143, 27–35. doi: 10.1016/j.resconrec.2018.12.009
Insam, H., and Seewald, M. S. A. (2010). Volatile organic compounds (VOCs) in soils. Biol. Fertil. Soils 46, 199–213. doi: 10.1007/s00374-010-0442-3
Kalyoncu Ergüler, G. (2015). Investigation the applicability of eggshell for the treatment of a contaminated mining site. Miner. Eng. 76, 10–19. doi: 10.1016/j.mineng.2015.02.002
Katan, J., and DeVay, J. E. (1991). Soil solarization: historical perspectives, principles, and uses. Soil Solarization, CRC Press. 23–37.
Kikuchi, Y., Kawashima, M., Iwatsuki, M., Kimishima, A., Tsutsumi, H., Asami, Y., et al. (2023). Comprehensive analysis of biosynthetic gene clusters in bacteria and discovery of Tumebacillus as a potential producer of natural products. J. Antibiot. 76, 316–323. doi: 10.1038/s41429-023-00609-y
Konishi, T., Tamura, T., Tobita, T., Sakai, S., Matsuda, N., and Kawasaki, H. (2021). Effusibacillus dendaii sp. nov. isolated from farm soil. Arch. Microbiol. 203, 4859–4865. doi: 10.1007/s00203-021-02470-9
Laca, A., Laca, A., and Díaz, M. (2017). Eggshell waste as catalyst: a review. J. Environ. Manag. 197, 351–359. doi: 10.1016/j.jenvman.2017.03.088
Li, C., Yu, P., Zeng, Y., Qiu, B., Chen, M., Cai, D., et al. (2023a). Assessment of reusing municipal food waste and eggshells as soil amendments for biosolarization. doi: 10.2139/ssrn.4611906
Li, C., Yu, P., Zeng, Y., Qiu, B., Chen, M., Cai, D., et al. (2023b). Chemical and microbial risk assessment of municipal food waste and eggshells residues as soil amendments in a simulated soil biosolarization. doi: 10.2139/ssrn.4561982
Li, Q., Zhu, X., Xie, Y., and Liang, J. (2021). Antifungal properties and mechanisms of three volatile aldehydes (octanal, nonanal and decanal) on aspergillus flavus. Grain Oil Sci. Technol. 4, 131–140. doi: 10.1016/j.gaost.2021.07.002
Liang, Y., Li, Y., Lin, Y., Liu, X., Zou, Y., Yu, P., et al. (2022). Assessment of using solid residues of fish for treating soil by the biosolarization technique as an alternative to soil fumigation. J. Clean. Prod. 357:131886. doi: 10.1016/j.jclepro.2022.131886
Liu, L., Wang, S., Guo, X., Zhao, T., and Zhang, B. (2018). Succession and diversity of microorganisms and their association with physicochemical properties during green waste thermophilic composting. Waste Manag. 73, 101–112. doi: 10.1016/j.wasman.2017.12.026
López-Díaz, J. A., Talavera-Mendoza, O., Juárez, K., Esparza-Soto, M., Violante-González, J., Ocampo-Díaz, Y. Z. E., et al. (2024). Acid mine drainage neutralization rate using biogenic calcite. Revista Internac. de Contamin. Ambiental. 40, 115–135. doi: 10.20937/RICA.55194
Mignardi, S., Archilletti, L., Medeghini, L., and De Vito, C. (2020). Valorization of eggshell biowaste for sustainable environmental remediation. Sci. Rep. 10:2436. doi: 10.1038/s41598-020-59324-5
Mishra, A. K., Das, R., George Kerry, R., Biswal, B., Sinha, T., Sharma, S., et al. (2023). Promising management strategies to improve crop sustainability and to amend soil salinity. Front. Environ. Sci. 10:962581. doi: 10.3389/fenvs.2022.962581
Momma, N., Yamamoto, K., Simandi, P., and Shishido, M. (2006). Role of organic acids in the mechanisms of biological soil disinfestation (BSD). J. Gen. Plant Pathol. 72, 247–252. doi: 10.1007/s10327-006-0274-z
Ngayakamo, B., and Onwualu, A. P. (2022). Recent advances in green processing technologies for valorisation of eggshell waste for sustainable construction materials. Heliyon 8:e09649. doi: 10.1016/j.heliyon.2022.e09649
Niwa, R., Kumei, T., Nomura, Y., Yoshida, S., Osaki, M., and Ezawa, T. (2007). Increase in soil pH due to ca-rich organic matter application causes suppression of the clubroot disease of crucifers. Soil Biol. Biochem. 39, 778–785. doi: 10.1016/j.soilbio.2006.09.027
Oka, Y. (2010). Mechanisms of nematode suppression by organic soil amendments—a review. Appl. Soil Ecol. 44, 101–115. doi: 10.1016/j.apsoil.2009.11.003
Oliveira, D. A., Benelli, P., and Amante, E. R. (2013). A literature review on adding value to solid residues: egg shells. J. Clean. Prod. 46, 42–47. doi: 10.1016/j.jclepro.2012.09.045
Oumaima, B., Nour-Eddine, C., Mohammed, R. S., Ouammou, A., Oussama, C., and Faouzi, E. (2024). Thermoactinomyces sacchari competent strain: isolation from compost, selection and characterization for biotechnological use. Scientific African 23:e02121. doi: 10.1016/j.sciaf.2024.e02121
Quina, M. J., Soares, M. A. R., and Quinta-Ferreira, R. (2017). Applications of industrial eggshell as a valuable anthropogenic resource. Resour. Conserv. Recycl. 123, 176–186. doi: 10.1016/j.resconrec.2016.09.027
Randall, T. E., Fernandez-Bayo, J. D., Harrold, D. R., Achmon, Y., Hestmark, K. V., Gordon, T. R., et al. (2020). Changes of fusarium oxysporum f.sp. lactucae levels and soil microbial community during soil biosolarization using chitin as soil amendment. PLoS One 15:e0232662. doi: 10.1371/journal.pone.0232662
Ray, S., Patel, N., and Amin, D. (2020). “Brevibacillus” in Beneficial microbes in agro-ecology. eds. N. Amaresan, M. Senthil Kumar, K. Annapurna, and A. Krishna Kumar Sankaranarayanan (Netherlands: Elsevier), 149–167.
Rengasamy, P. (2010). Soil processes affecting crop production in salt-affected soils. Funct. Plant Biol. 37:613. doi: 10.1071/FP09249
Riah-Anglet, W., Trinsoutrot-Gattin, I., Martin-Laurent, F., Laroche-Ajzenberg, E., Norini, M.-P., Latour, X., et al. (2015). Soil microbial community structure and function relationships: a heat stress experiment. Appl. Soil Ecol. 86, 121–130. doi: 10.1016/j.apsoil.2014.10.001
Shade, A., Peter, H., Allison, S. D., Baho, D. L., Berga, M., Bürgmann, H., et al. (2012). Fundamentals of microbial community resistance and resilience. Front. Microbiol. 3:417. doi: 10.3389/fmicb.2012.00417
Shea, E. A., Fernández-Bayo, J. D., Hodson, A. K., Parr, A. E., Lopez, E., Achmon, Y., et al. (2022). Biosolarization restructures soil bacterial communities and decreases parasitic nematode populations. Appl. Soil Ecol. 172:104343. doi: 10.1016/j.apsoil.2021.104343
Shea, E. A., Wang, Z., Allison, B., and Simmons, C. W. (2021). Alleviating phytotoxicity of soils biosolarized with almond processing residues. Environ. Technol. Innov. 23:101662. doi: 10.1016/j.eti.2021.101662
Silva, G. D. C., Kitano, I. T., Ribeiro, I. A. D. F., and Lacava, P. T. (2022). The potential use of Actinomycetes as microbial inoculants and biopesticides in agriculture. Front. Soil Sci. 2:833181. doi: 10.3389/fsoil.2022.833181
Soares, M. A. R., Quina, M. J., and Quinta-Ferreira, R. M. (2015). Immobilisation of lead and zinc in contaminated soil using compost derived from industrial eggshell. J. Environ. Manag. 164, 137–145. doi: 10.1016/j.jenvman.2015.08.042
Stapleton, J. J. (2000). Soil solarization in various agricultural production systems. Crop Prot. 19, 837–841. doi: 10.1016/S0261-2194(00)00111-3
Tamir, G., Shenker, M., Heller, H., Bloom, P. R., Fine, P., and Bar-Tal, A. (2011). Can soil carbonate dissolution lead to overestimation of soil respiration? Soil Sci. Soc. Am. J. 75, 1414–1422. doi: 10.2136/sssaj2010.0396
Vurukonda, S. S. K. P., Giovanardi, D., and Stefani, E. (2018). Plant growth promoting and biocontrol activity of Streptomyces spp. as endophytes. Int. J. Mol. Sci. 19:952. doi: 10.3390/ijms19040952
Wang, P., Wang, Z., Zhu, M., Zhu, C., Feng, W., Duan, G., et al. (2023). Di-n-butyl phthalate stress hampers compost multifunctionality by reducing microbial biomass, diversity and network complexity. Bioresour. Technol. 376:128889. doi: 10.1016/j.biortech.2023.128889
Yazdanpanah, N., Mahmoodabadi, M., and Cerdà, A. (2016). The impact of organic amendments on soil hydrology, structure and microbial respiration in semiarid lands. Geoderma 266, 58–65. doi: 10.1016/j.geoderma.2015.11.032
Yu, P., Zeng, Y., Li, C., Qiu, B., Shi, Y., He, Q., et al. (2024). Quality change of Citri reticulatae pericarpium (pericarps of Citrus reticulata ‘Chachi’) during storage and its sex-based in vitro digestive performance. Food Secur. 13:3671. doi: 10.3390/foods13223671
Zhang, T., Tu, Z., Lu, G., Duan, X., Yi, X., Guo, C., et al. (2017). Removal of heavy metals from acid mine drainage using chicken eggshells in column mode. J. Environ. Manag. 188, 1–8. doi: 10.1016/j.jenvman.2016.11.076
Zhou, H., Tao, N., and Jia, L. (2014). Antifungal activity of citral, octanal and α-terpineol against Geotrichum citri-aurantii. Food Control 37, 277–283. doi: 10.1016/j.foodcont.2013.09.057
Zou, Y., Qiu, B., Lin, F., Wu, W., Guo, R., Xing, J., et al. (2023). Assessment of the influence of using green tea waste and fish waste as soil amendments for biosolarization on the growth of lettuce (Lactuca sativa L. var. ramosa Hort.). Front. Sustain. Food Syst. 7:1174528. doi: 10.3389/fsufs.2023.1174528
Keywords: sustainable agriculture, eggshell waste, soil biosolarization, volatile organic compounds, microbial community
Citation: Li C, Yu P, Zeng Y, Qiu B, Chen M, Cai D, Zhao Z, Chen Z, Yaron S and Achmon Y (2025) Impacts of soil biosolarization with eggshell waste on soil health, microbial communities, and gas emissions. Front. Sustain. Food Syst. 9:1543216. doi: 10.3389/fsufs.2025.1543216
Edited by:
Shalini Gaur Rudra, Indian Agricultural Research Institute, IndiaReviewed by:
Patricia D. Millner, Agricultural Research Service (USDA), United StatesGiovanni Hernández-Flores, National Council of Science and Technology (CONACYT), Mexico
Copyright © 2025 Li, Yu, Zeng, Qiu, Chen, Cai, Zhao, Chen, Yaron and Achmon. This is an open-access article distributed under the terms of the Creative Commons Attribution License (CC BY). The use, distribution or reproduction in other forums is permitted, provided the original author(s) and the copyright owner(s) are credited and that the original publication in this journal is cited, in accordance with accepted academic practice. No use, distribution or reproduction is permitted which does not comply with these terms.
*Correspondence: Sima Yaron, c2ltYXlAYmZlLnRlY2huaW9uLmFjLmls; Yigal Achmon, eWlnYWwuYWNobW9uQGd0aWl0LmVkdS5jbg==