- 1Department of Environmental Studies, California State University, Sacramento, CA, United States
- 2Office of Sustainability, California State University, Sacramento, CA, United States
- 3Three Sisters Gardens, West Sacramento, CA, United States
Urban agriculture is crucial for enhancing food security in densely populated areas, but maintaining soil health in these settings is key for long-term productivity. This study investigates the effects of locally produced compost and biochar amendments on soil health and plant nutrients at two urban farms in Sacramento, California, USA. Regionally sourced biochar was produced from pistachio shells, and the compost was made from on-site green waste, creating a closed-loop system that recycles organic materials back into urban soils. We hypothesized that the combined application of biochar and compost would increase soil water holding capacity and nutrient retention, leading to enhanced microbial activity and higher nutrient concentrations in corn compared to individual amendments. Field trials were established at both sites using the randomized complete block design with four treatments: control, compost applied at approximately 25 t ha−1 (equivalent to 10 Mg C ha−1), biochar at 12.5 t ha−1 (equivalent to 10 Mg C ha−1), and a compost-biochar mix (20 Mg C ha−1). Soil samples were collected during mid- and late-season, and analyzed for key physicochemical and microbial properties. Corn kernel nutrient concentrations were also measured. Over one growing season, results showed that biochar and compost-biochar mix treatments significantly increased soil water holding capacity, organic matter content, and cation exchange capacity, leading to better soil structure and nutrient retention. Soil nitrate (NO3−–N) and bioavailable phosphorus (P) were significantly higher in biochar-amended soils. The compost-biochar mix enhanced microbial metabolic activity and microbial community evenness, though overall microbial diversity remained stable. Corn kernels in biochar and compost-biochar treatments exhibited increased nutrient concentrations, particularly for P, Mg, Ca, and Fe. Overall, the compost-biochar combination treatment had the most significant impact on improving soil physicochemical properties, microbial responses, and the plant nutrient concentrations, demonstrating their cumulative benefits for urban soil health and crop nutrition. By utilizing locally sourced materials and recycling organic waste, this study demonstrates a resource-efficient approach to improving soil fertility at resource-limited urban farms. These practices have the potential to play a vital role in addressing food security challenges in urban communities, particularly those experiencing food insecurity, while promoting sustainable resource management.
1 Introduction
Urban agriculture has emerged as a critical component of sustainable food production systems, particularly in densely populated areas where access to fresh, nutritious food is often limited (Nogeire-McRae et al., 2018). In Sacramento, California, USA, urban farms and gardens like Three Sisters Gardens and the Capital Radio Garden serve as vital resources for food security.1,2 These organizations and initiatives address food insecurity by growing and donating fresh produce to local low-income communities, highlighting the social and environmental benefits of urban agriculture in promoting a more sustainable and equitable food system (Joyner et al., 2025). However, maintaining healthy and productive soils remains a key challenge for urban farmers, as many urban soils suffer from low organic matter, poor nutrient retention, and limited water availability (Wortman and Lovell, 2013). Developing soil management strategies that enhance soil fertility, water retention, and microbial activity is essential for ensuring the long-term productivity and resilience of urban farms (Salomon and Cavagnaro, 2022).
Soil health, defined as the continued capacity of a soil to function as a vital living system, is essential for agricultural productivity and environmental quality (Lehmann et al., 2020). One promising strategy for enhancing soil health involves the sequestration of carbon (C) in soils through the application of organic amendments, such as compost and biochar. These C-rich materials have the potential to improve soil fertility, enhance soil structure, and mitigate greenhouse gas emissions (Favoino and Hogg, 2008; Gao et al., 2017; Villagra-Mendoza and Horn, 2018). Compost is widely recognized as a valuable organic amendment for urban farms due to its ability to improve soil structure and provide readily available nutrients (Eldridge et al., 2018; Rashid and Shahzad, 2021). In recent years, the use of on-site composting systems, such as three-bin composting or windrow composting, has gained popularity in urban farms as a way to recycle food scraps and green waste while reducing reliance on external soil amendments (Carvalho et al., 2022; Torrijos et al., 2021). Both Three Sisters Gardens and the Capital Radio Garden demonstrate a holistic approach to waste management and resource utilization by utilizing compost generated on-site from organic waste (Harrison et al., 2024a; Joyner et al., 2025; Sacramento State Sustainability, 2022). This approach promotes a circular economy, closing the loop between organic waste generation and food production (Mohan et al., 2020). Several studies have demonstrated that compost produced from urban organic waste and green waste can significantly increase soil organic matter, improve nutrient availability, and enhance plant productivity (Heckman et al., 2022; Richardville et al., 2022). However, compost decomposition rates can be relatively fast, leading to nutrient losses through leaching and volatilization, particularly in Mediterranean climates where organic matter mineralization is accelerated (Kurganova et al., 2012).
Biochar, a carbon-rich byproduct of biomass pyrolysis or gasification, has been extensively studied for its long-term soil carbon sequestration potential and ability to improve nutrient and water retention in agricultural systems (Lehmann and Joseph, 2024). Unlike compost, biochar is highly stable, with a low decomposition rate and high porosity, making it particularly valuable in semi-arid environments (Cen et al., 2021). Studies have shown that biochar can increase soil cation exchange capacity (CEC), enhance microbial habitat quality, and reduce nutrient leaching losses in arid and semi-arid agroecosystems (Blanco-Canqui et al., 2024; Diatta et al., 2020). However, biochar alone is often nutrient-poor and does not provide an immediate source of plant-available macronutrients like nitrogen (N) and phosphorus (P) (DeLuca et al., 2024). By harnessing the combined effects of compost and biochar amendments, urban farmers and gardeners can potentially revitalize degraded urban soils, increase agricultural productivity, and mitigate environmental impacts associated with conventional waste disposal practices (Hu et al., 2021; Kharola et al., 2022).
Despite the recognized benefits of compost and biochar amendments in improving soil health, studies examining their combined effects have reported variable outcomes due differences in soil conditions, amendment composition, and environmental factors (Agegnehu et al., 2017; Qian et al., 2023). Moreover, few studies have evaluated the combined use of biochar with compost derived from urban food and green waste in community farms, despite the increasing interest in urban circular economy practices (Adhikari et al., 2010; Malone et al., 2023; Morrow and Davies, 2022). In particular, studies assessing their co-application in urban agricultural systems remain limited, especially in Mediterranean climates with semi-arid growing seasons like Sacramento, California. This study aims to evaluate how compost, biochar, and their combination influence soil health and crop nutrient uptake in two semi-arid urban farms in Sacramento, California. Specifically, we hypothesize that the combined application of biochar and compost will enhance soil water retention and nutrient availability, leading to greater microbial activity and enhanced corn kernel nutrient concentrations. Given the regional context of Sacramento’s Mediterranean climate, this study provides empirical, field-based evidence on whether the co-application of biochar and compost leads to cumulative benefits that persist in soil and translate into improved crop nutrition. By focusing on urban farm-specific challenges and locally produced organic amendments, this research offers practical insights for urban farmers seeking to optimize soil fertility and maximize nutrient availability in resource-limited urban agricultural systems.
2 Materials and methods
2.1 Study sites and field experimental design
The field trial was conducted at two urban agricultural sites around Sacramento, California, USA: the Capital Radio Garden and Three Sisters Gardens. These sites were selected due to similar environmental conditions and management practices, as well as their suitability for growing natural sweet corn. Both locations are community-run urban farms that implement organic soil management practices and produce fresh produce for local food-insecure communities. Both sites exhibit a Mediterranean climate with a mean annual temperature of 18.2°C and a mean annual precipitation of 311 mm. Summers are hot and dry, with a July average temperature of 29.3°C and no recorded rainfall. Winters are cooler and wetter, with a January average temperature of 8.5°C and 55 mm of monthly average precipitation. Climate data, including temperature and precipitation trends for the study period, are provided in Supplementary Table 1.
The soils at both sites are classified as mixed, thermic Typic Psammaquents (soil order: Entisols) and are somewhat poorly drained (Soil Survey Staff), with a sandy loam texture at the surface (0–30 cm). This consistency between the two sites allows for a robust comparison of soil health responses to compost and biochar amendments in urban agricultural settings. Specifically, soil at the Capital Radio Garden has a pH of 6.7, bulk density of 0.58 g cm−3, cation exchange capacity (CEC) of 20.9 meg 100 g−1, and 41.2 g kg−1 total C and 2.98 g kg−1 total nitrogen (N). The soil at the Three Sisters Gardens has a pH of 6.8, bulk density of 0.42 g cm−3, CEC of 15.6 meg 100 g−1, and 40.5 g kg−1 soil total C, and 2.48 g kg−1 total N. By conducting the study at two urban agricultural sites, we can better assess the generalizability of our findings and provide valuable insights into the broader applicability of these soil management practices across urban farming contexts in Sacramento.
A randomized complete block design was employed at both sites, with four treatments and three replicates per treatment, totaling 12 plots per site. Each plot measured 1 m by 1 m, with a 1-meter buffer zone between plots to minimize edge effects. The treatments included: (1) control (no amendment), (2) compost applied at 25 t ha−1 (equivalent to 10 Mg C ha−1), (3) biochar applied at 12.5 t ha−1 (equivalent to 10 Mg C ha−1), and (4) a compost-biochar mix applied at 25 t ha−1 of compost + 12.5 t ha−1 of biochar. Treatments were incorporated into the surface soil (0–15 cm) using a rake and pitchfork in late April 2024. Control plots were similarly raked to ensure consistent soil disturbance. This field experimental design, applied consistently across both study sites, allows for a detailed comparison of treatment effects while accounting for potential site-specific differences. Each farm site can be considered a stand-alone study while also providing broader insights into the combined effects of compost and biochar on soil health in urban agricultural systems.
Natural sweet corn (Zea mays L. Var. Saccharata) was sown on May 1st 2024, following standard management practices used at both farms. Corn was direct-seeded with 30 cm within-row spacing, and 90 cm between-row spacing to optimize plant growth. Drip irrigation was used at both sites, with water application managed based on soil moisture levels and standard farm irrigation schedules. Manual weeding was performed as needed, with no synthetic herbicides used. Integrated pest management was implemented at both farms, relying on biological control and row covers to minimize damage. No chemical pesticide were applied. No synthetic fertilizers were used; and nutrient inputs were solely derived from compost and biochar amendments. Corn was harvested on July 27th 2024, with the entire experiment spanning approximately 3 months.
2.2 Biochar and compost characterization
The biochar used in the field trials at both study sites was sourced from VGrid Energy Systems in Camarillo, California, and produced through a high-temperature gasification process (900–1,200°C) using 100% pistachio shells grown in California. Gasification biochar is produced nder limited oxygen conditions, generating syngas as a primary energy product, with biochar as a byproduct. Gasification biochar is distinct from pyrolysis biochar in its characteristics, especially its higher C stability, lower volatile matter content, and improved potential in nutrient retention (Tan, 2023). The biochar used in our study has a H:Corg ratio of 0.18, volatile matter of 18.8%, and ash content of 6.5% (summarized in Table 1). The biochar had a particle size range of 1–3 mm. Additional details regarding the production and properties of the biochar can be found at https://persistproducts.com/persist-biochar/.
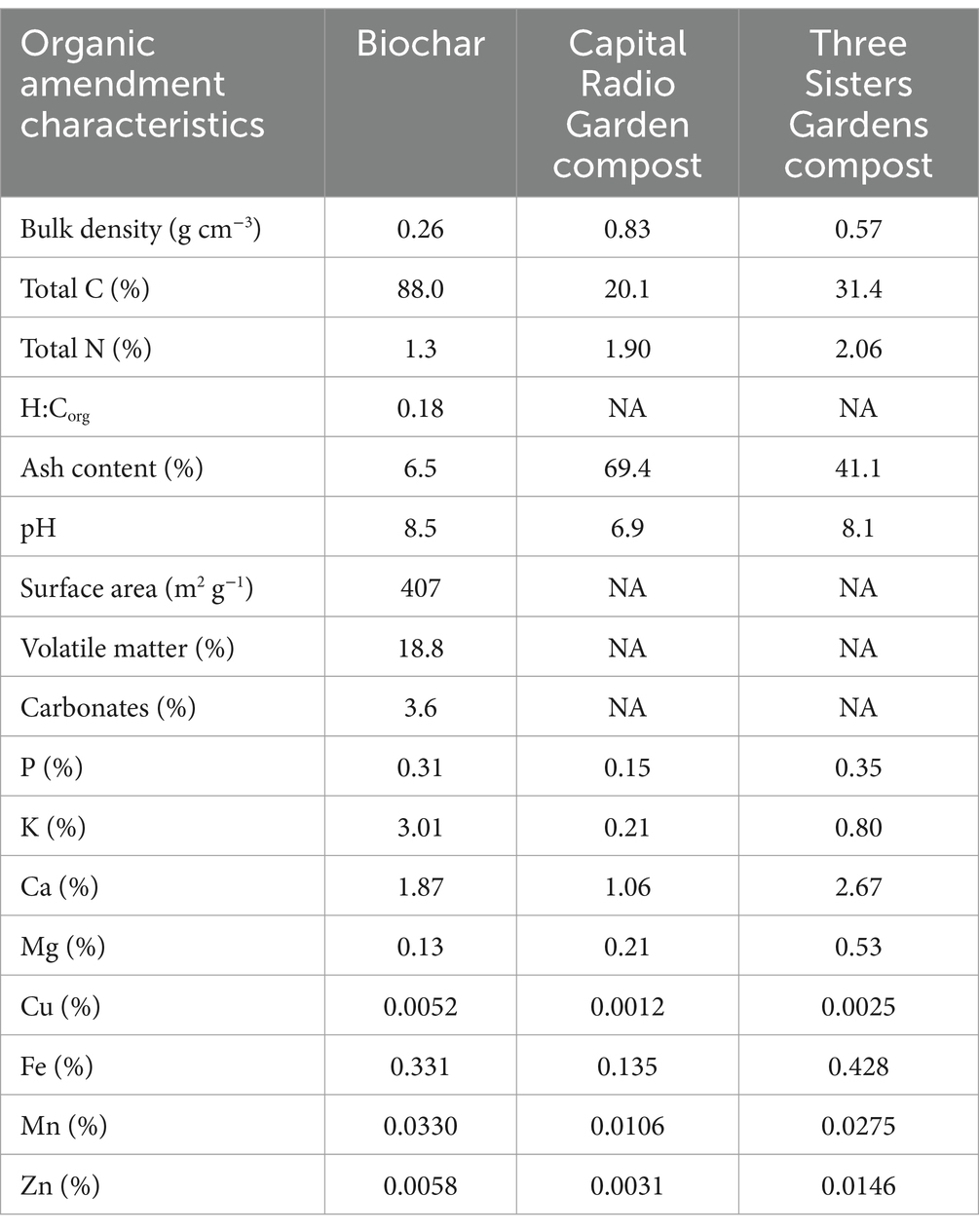
Table 1. Characteristics of organic amendments used in field trials at the Capital Radio Garden and Three Sisters Gardens, Sacramento, CA, USA.
The compost used in the trials was locally produced at each site as part of a closed-loop waste recycling system, reflecting urban farm-scale composting practices. At the Capital Radio Garden, compost was generated using a windrow composting system from green waste collected at Sacramento State University Campus. This feedstock primarily consisted of grass clippings, vegetable and fruit scraps, and tree leaves, supplemented with straw to balance the carbon-to-nitrogen ratio. The windrows were turned every 2 weeks to ensure adequate aeration, support microbial activity, and maintain proper decomposition rates. The composting cycle lasted around 12 weeks before reaching maturity. At Three Sisters Gardens, compost was produced using a three-bin composting system, which allowed for staged decomposition and effective nutrient cycling. The feedstock primarily included food scraps and plant residues sourced directly from the farm, along with shredded paper, cardboard, dried leaves, and small wood chips to balance carbon and nitrogen inputs. The composting process involved regular turning (every 1–2 weeks) to ensure adequate oxygenation, with moisture levels adjusted as needed to optimize microbial activity. Compost at this site typically reached maturity within 12 weeks. Table 1 lists the relevant physical and chemical characteristics of both the biochar and compost used in this study. Due to differences in feedstock composition and processing methods, the Capital Radio Garden compost had a slightly lower total P and K content compared to that from the Three Sisters Gardens (Table 1; Supplementary Table 2). These differences in compost composition contributed to site-specific soil responses observed in this study.
2.3 Soil sampling and analysis
Soil sampling was carried out at both sites at two time points throughout the growing season: During the mid-season at mid-June 2024, and at the end of the growing season at late July 2024. Sampling at both mid-season and late-season allowed us to track treatment effects over time, capturing both immediate changes in nutrient availability and longer-term soil responses. Mid-season sampling was conducted to assess initial mineralization dynamics and microbial activity in response to organic amendments, while late-season sampling provided insights into cumulative treatment effects on soil fertility and plant-available nutrients at the crop maturity stage. Soil was sampled to a depth of approximately 0–30 cm, allowing us to account for potential vertical movement of nutrients due to drip irrigation and biological mixing, as well as root zone interactions where plant uptake and microbial activity are most active. Each soil sample was a composite of six subsamples collected from each plot using a soil probe. Soil samples were analyzed for various parameters, including moisture content, bulk density, water holding capacity, pH, electrical conductivity (EC), cation exchange capacity (CEC), organic matter content, total C, extractable N, net N mineralization rate, bioavailable phosphorus (P), as well as other macro and micronutrients. Soil microbial activity and diversity indices were also analyzed using community level physiological profiling.
Briefly, bulk density was determined using a bulk density corer (7 cm diameter). Water holding capacity was determined by gravimetry (Loveday, 1974). Soil pH and EC were determined on fresh soil (1:2 v/v soil to DI water) on a pH/EC meter (Mettler Toledo). For CEC, soil samples were extracted using an ammonium acetate solution at pH 7.0. The exchangeable cations (i.e., Ca, Mg, K, and Na) were displaced by ammonium ions, and the concentrations of these cations were quantified using an inductively coupled plasma optical emission spectrometer (ICP-OES). The CEC value was then calculated based on the sum of the exchangeable cations and expressed as milliequivalents per 100 grams of soil (meq 100 g−1). Organic matter content was determined using the Loss-on-Ignition (LOI) method, and oven dried (65°C) samples were ground and analyzed for total C on an elemental analyzer (Costech ECS 4010 CHNS-O).
Fresh soil samples were weighed, shaken in 2 M KCl for 1 h, filtered through Whatman #1 filter papers, and the extractants were analyzed for extractable NO3−, NH4+ by microplate-colorimetric techniques using the vanadium-chloride method and the salicylate-nitroprusside method, respectively (Mulvaney et al., 1996). Soil net N mineralization rates were determined using the 28-d aerobic incubation method and were calculated by subtracting the initial extractable N (day 0) from that determined at the end of the incubation (day 28) (Hart et al., 1994). Soil bioavailable P status was determined using the biologically based P method described in DeLuca et al. (2015) to assess a suite of four plant P acquisition strategies. Briefly, 0.01 M CaCl2, 10 mM citric acid, 0.2 enzyme unit mL−1 phosphatase enzyme (derived from wheat germs), and 1 M HCl were used as extractants to emulate free soluble P, active inorganic P (weakly sorbed or bounded in inorganic precipitates), active or labile organic P (readily attacked by phosphatase enzymes), and moderately stable inorganic P (present in P-precipitates). Extracts were analyzed for orthophosphate using the Malachite green method (Ohno and Zibilske, 1991). We also measured soil macro and micronutrients on soils collected from the end of the growing season. The macro and micronutrients were measured on ICP-OES following a dry-ash and acid digestion procedure (Górecka et al., 2006; Munter et al., 1984).
Soil microbial metabolic activity and functional diversity indices were evaluated on late-season soils using the Biolog EcoPlates method (Kiersztyn et al., 2019), which assesses microbial utilization of various C sources. Since microbial communities take time to fully respond to organic amendments, late-season sampling provided a more representative assessment of longer-term microbial shifts in the agroecosystem. Briefly, 3 g soil was mixed with 27 mL sterile 0.85% NaCl solution, shaken at 220 rpm for 30 min. The supernatant was obtained after 10-min standing. The supernatant was serially diluted to create a 1:1,000 soil solution. One hundred and fifty microliters of this solution were added to each well of the Biolog EcoPlate, and plates were incubated at 25°C in the dark. The optical density at 590 nm (OD590) in each well was determined every 24 h on a microplate reader (ACCURIS Smart Reader, Edison, NJ, USA). OD590 values measured at 96 h provided the best differentiation between treatments, and these were used to calculate the average well color development (AWCD) and the diversity indices, including Shannon, Simpson, and McIntosh following equations described in Du et al. (2022).
2.4 Corn kernel nutrient analysis
At the end of the growing season, corn kernels were collected from each treatment plot, dried at 65°C for 24 h, and ball-milled for nutrient analysis. Total N was measured using an elemental analyzer (Costech ECS 4010 CHNS-O), and macro- and micronutrients (P, K, Mg, Ca, Zn, Mn, Fe, and Cu) were analyzed via ICP-OES using the same methods described for soil nutrient analysis. Corn kernels were selected for nutrient analysis due to their representation of the primary harvested product. Farmers prioritize nutrient composition of kernels for human consumption and donation, making it the most relevant component for analysis. Leaves and stems were returned to composting systems on-site, contributing to long-term nutrient cycling, but were not included in this study’s nutrient assessment.
2.5 Data analysis
Before performing statistical analyses, data were screened for outliers, and tests for homogeneity of variance and normality of residuals were conducted using the Shapiro–Wilk test. When necessary, data were log-transformed to meet the assumptions of normality and homogeneity of variance. Analysis of variance (ANOVA) was conducted to assess the effects of treatments on individual soil and plant variables, followed by post-hoc tests (Tukey’s HSD) to identify significant differences among treatments. Statistical significance was determined at an alpha level of 0.05. Pearson correlation analyses were used to evaluate the relationships between key soil and plant metrics. Mixed linear regression (MLR) models were also used to identify the dominant factors contributing to variations in soil microbial properties. All statistical analyses were performed in R (R Core Team, 2020).
3 Results
3.1 Soil physicochemical properties
The compost, biochar, and compost-biochar mix treatments significantly influenced soil physicochemical properties across both study sites (Figure 1 and Table 2). The water holding capacity (WHC) increased significantly in biochar and compost-biochar mix treatments. Soil pH and bulk density remained relatively stable across all treatments. Soil electrical conductivity (EC) values were significantly higher in the compost and compost-biochar mix treatments at Three Sisters Gardens. The cation exchange capacity (CEC), which represents the soil’s ability to retain and exchange essential nutrients, was the highest in the compost-biochar mix treatment at both sites. Both organic matter content and total C were significantly higher in biochar and compost-biochar mix treatments.
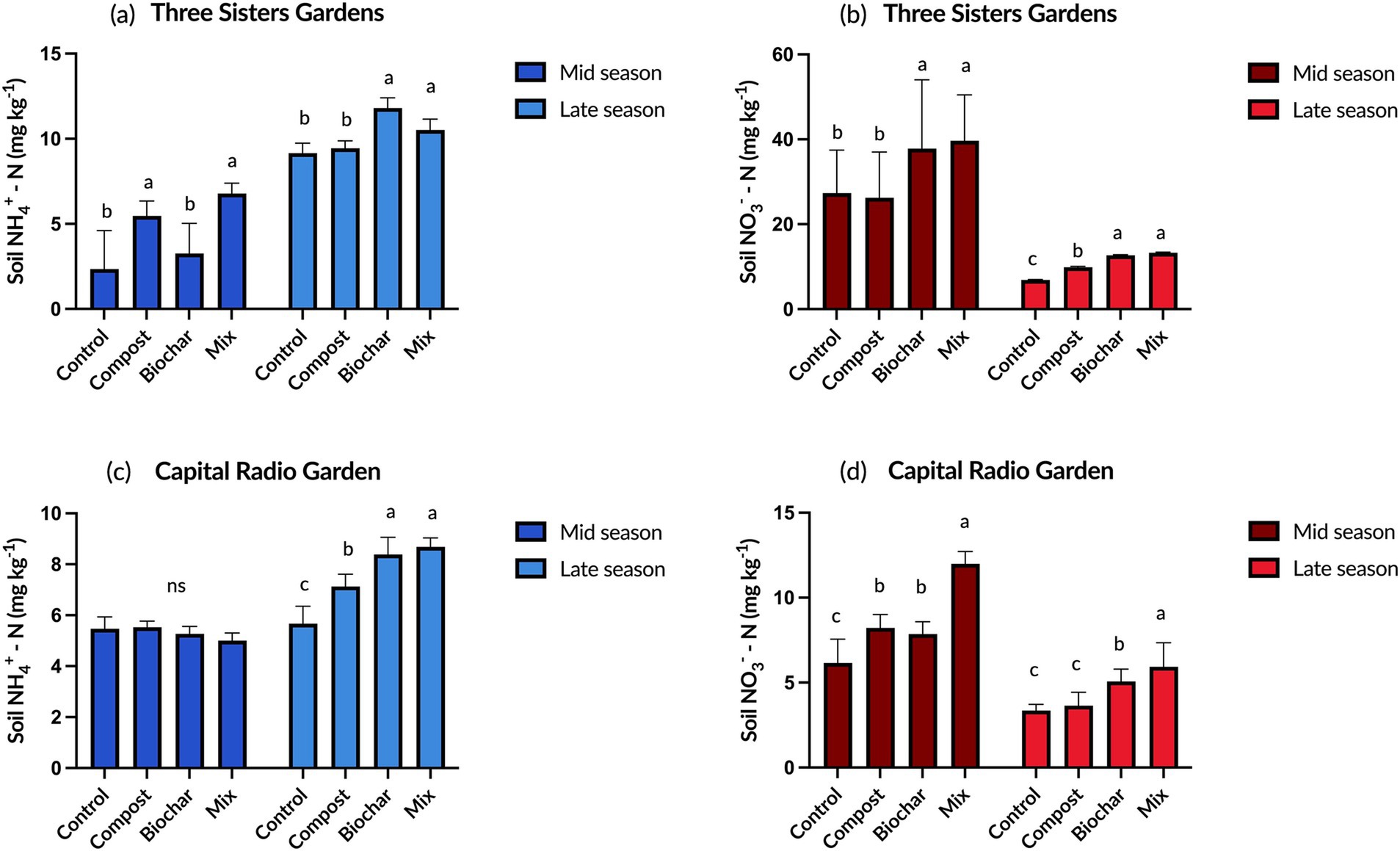
Figure 1. Soil extractable (a) NH₄+–N and (b) NO₃−–N at mid- and late-season at Three Sisters Gardens, and (c) NH₄+–N and (d) NO₃−–N at mid- and late-season at Capital Radio Garden in response to compost, biochar, and compost-biochar mix treatments during field trials in Sacramento, CA, USA. Error bars represent ±1 standard error.
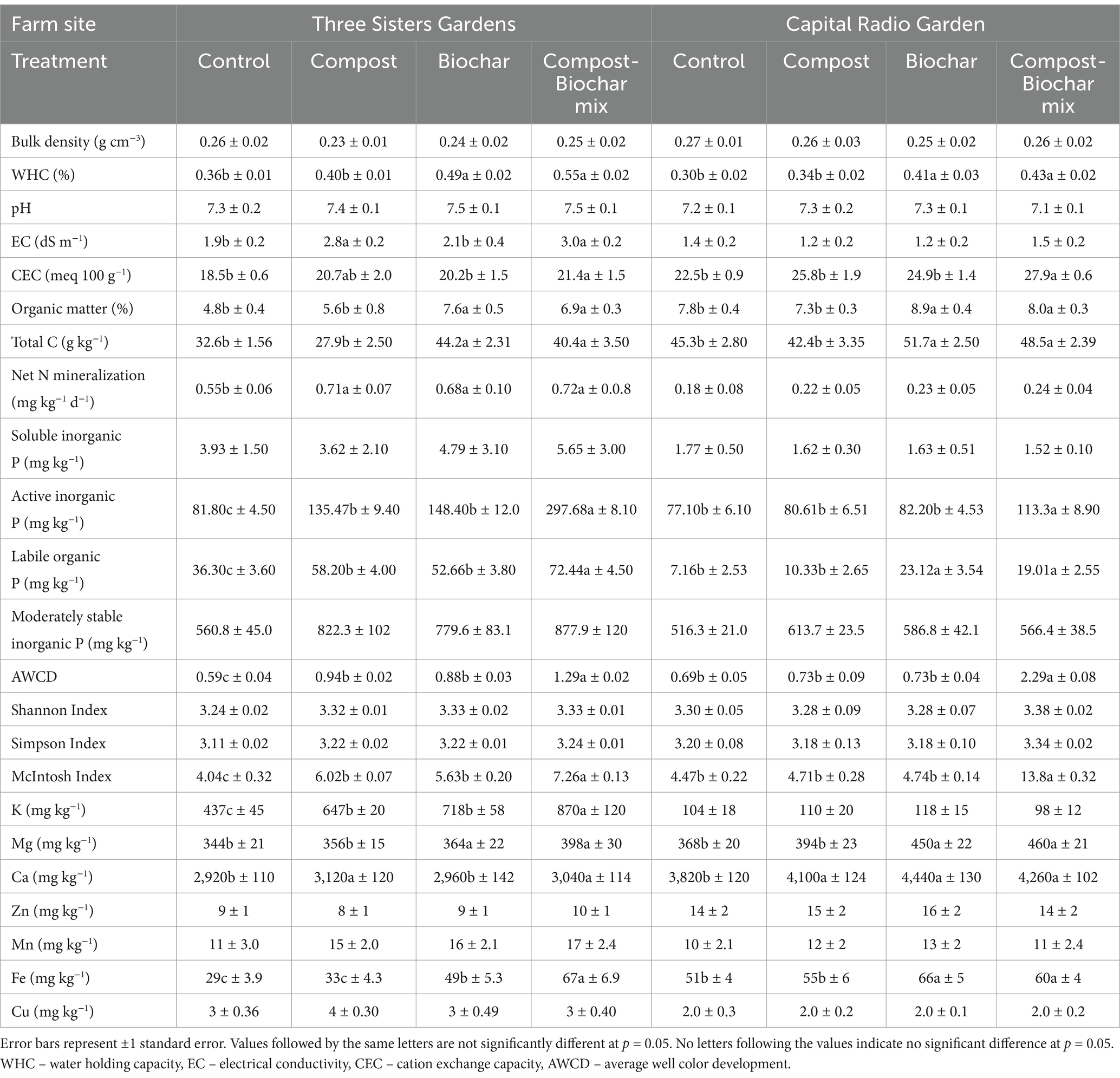
Table 2. Soil physicochemical properties, microbial metrics, and nutrient concentrations at late-season in response to compost, biochar, or compost-biochar mix amendments in the field trials at the Capital Radio Garden and Three Sisters Gardens, Sacramento, CA, USA.
Soil extractable NH₄+–N did not significantly respond to the addition of biochar at either site during the mid growing season, but the effect of biochar on soil NH₄+–N was statistically significant at the late growing season at both sites (Figure 1). In contrast, the biochar effect on soil NO₃−–N was positive and statistically significant at both mid- and late-season at both sites (Figure 1). Compared to control soils or the compost treatment, biochar and compost-biochar mix treatments both significantly increased soil extractable NH₄+–N and NO₃−–N at late-season at both study sites, and soils under the compost-biochar mix treatment exhibited the highest N availability throughout the season (Figure 1). For soil P bioavailability, the treatments had significant effects across most P fractions (Table 2). The active inorganic P fraction, which was extracted by 0.01 M citrate acid and included P that is readily available for plant uptake, was significantly higher in soils with all three organic amendment treatments compared to control at Three Sisters Gardens; and the compost-biochar mix treatment resulted in the highest active inorganic P concentrations at both study sites (Table 2). Similarly, labile organic P, extracted by phosphatase enzyme and an indicator of biologically active organic P, was also significantly higher in the compost-biochar mix treatment at both sites. Readily available inorganic P (extracted by 0.01 M CaCl2), and the moderately stable inorganic P (extracted by 1 M HCl) that represents P that is more tightly bound in soil minerals, both remained largely unaffected by the treatments. Biochar and compost-biochar mix treatments led to increased concentrations of Mg, Ca, and Fe at both sites (Table 2).
3.2 Soil microbial activity and diversity
Soil microbial metabolic activity, as measured by the average well color development (AWCD), was the highest in the compost-biochar mix treatment compared to control, compost alone, or biochar alone at both field sites (Figure 2). Though no compost effect or biochar effect was being observed on soil AWCD at the Capital Radio Garden site, both compost and biochar additions significantly increased soil AWCD compared to control at the Three Sisters Gardens site (Figure 2). The MLR analysis (Table 3) showed that microbial activity was significantly driven by soil parameters such as WHC, CEC, NO₃−–N availability, and the availability of active inorganic and organic P. Additionally, the McIntosh index, which highlights microbial community evenness, was the highest in the compost-biochar mix treatment (Figure 2 and Table 3). However, Shannon and Simpson indices of overall microbial diversity showed no significant differences across treatments (Table 3).
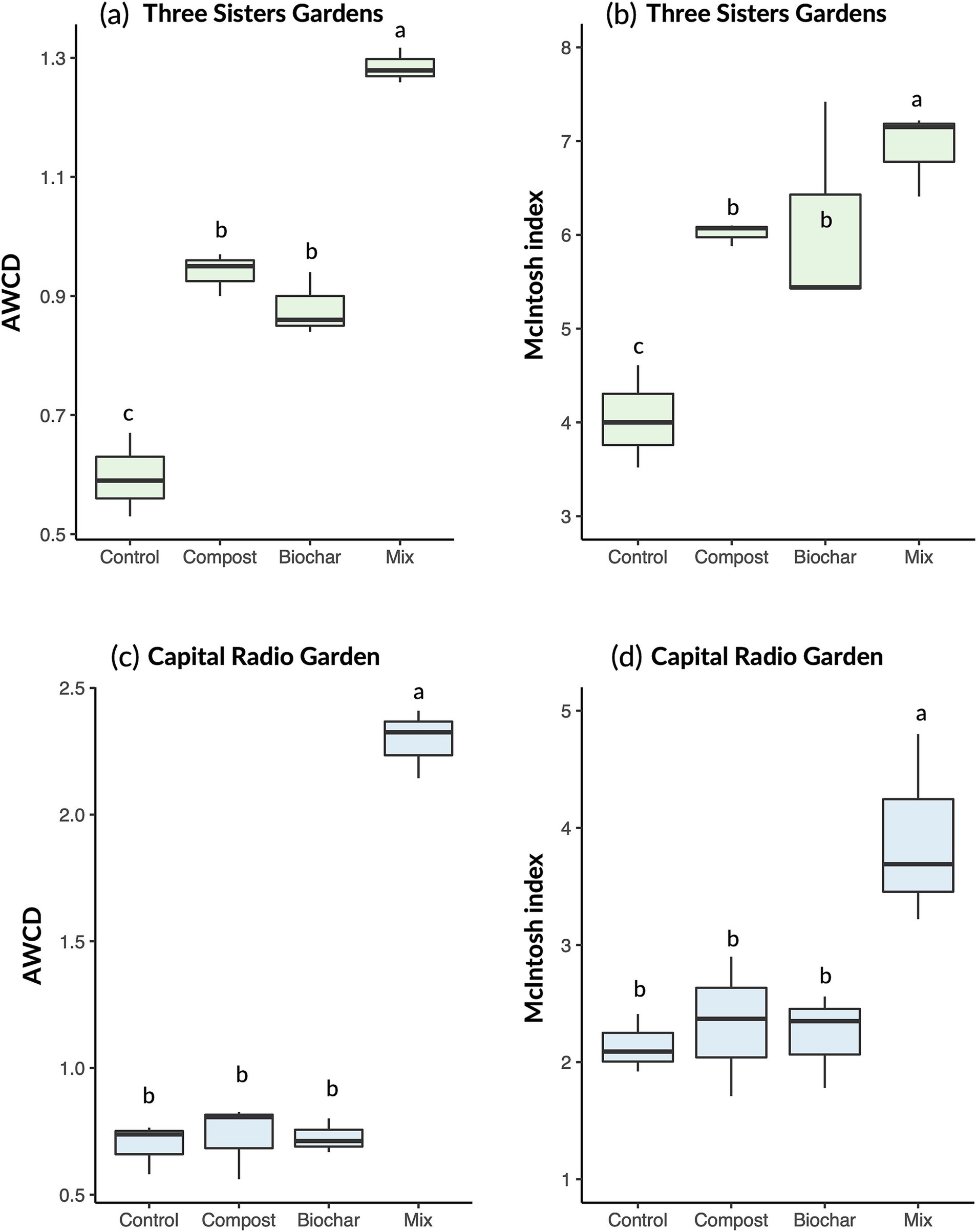
Figure 2. Average well color development (AWCD) as an indicator of soil microbial metabolic activity and McIntosh diversity index as an indicator of soil microbial species evenness in response to compost, biochar, and compost-biochar mix treatments in the field trials at the Three Sisters Gardens (a,b) and Capital Radio Garden (c,d) in Sacramento, CA, USA. Error bars represent ±1 standard error.
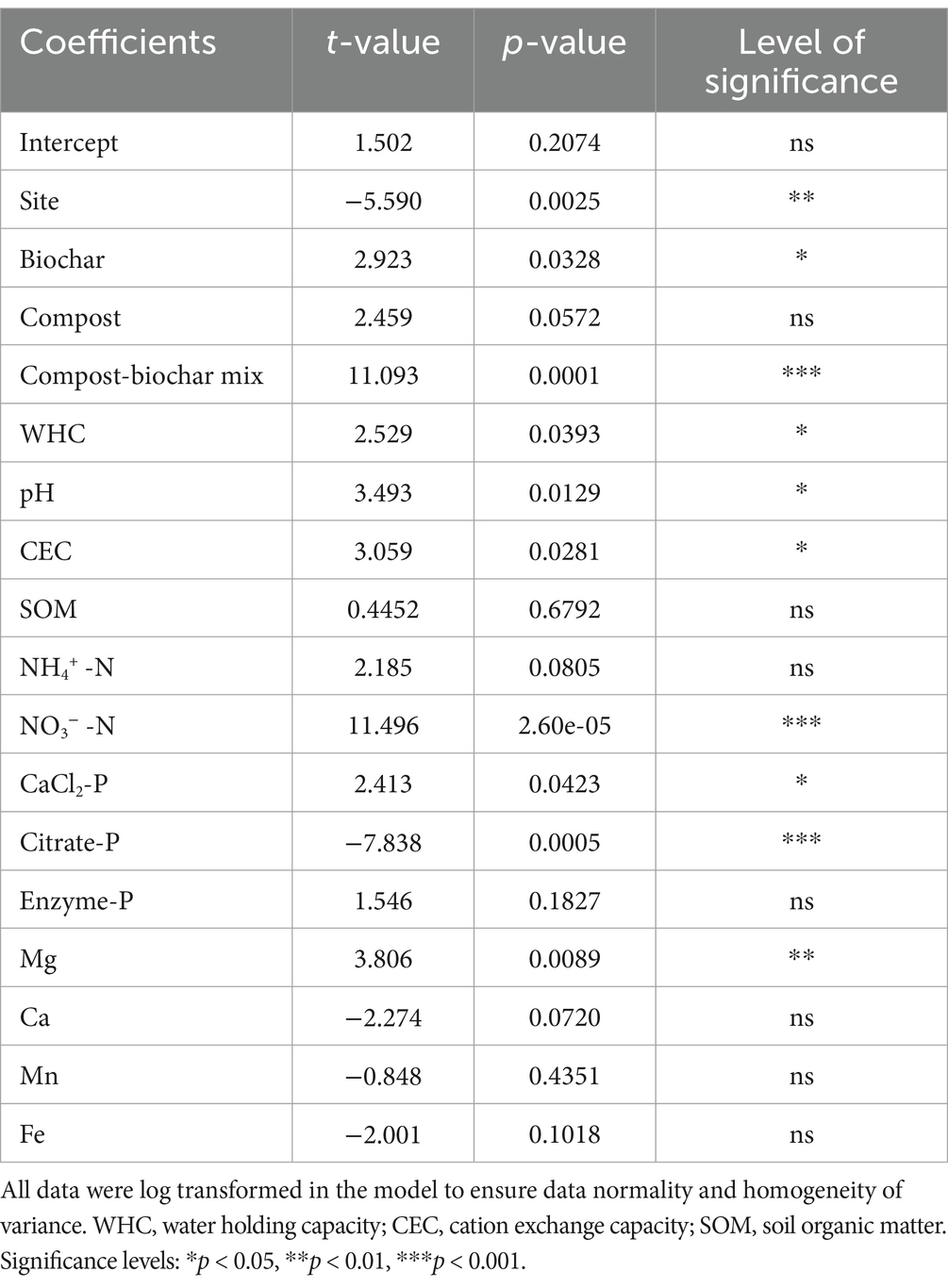
Table 3. Statistical summary of the multiple linear regression models analyzing soil metabolic activity (AWCD) as a function of soil nutrient availability and treatment types (compost, biochar, compost-biochar mix) at the Capital Radio Garden and Three Sisters Gardens, Sacramento, CA, USA.
3.3 Corn nutrient responses
The nutrient concentration in corn kernels was significantly influenced by biochar and compost-biochar treatments over one growing season (Table 4). The compost-biochar mix led to the highest concentrations of P, K, Mg, and Fe in corn kernels at both field sites, compared to control and individual amendment treatments. For example, P concentration in corn kernels was significantly higher in the biochar-compost mix plots, reaching 3.9 g kg−1 at Three Sisters Gardens and 4.0 g kg−1 at Capital Radio Garden. Correlation analysis further revealed strong positive correlations between soil nutrient availability and crop nutrient uptake. Specifically, soil active organic P, Mg, Ca, and Fe showed significant correlations with corresponding nutrient levels in corn kernels (Figure 3), highlighting the role of improved soil conditions under biochar-compost amendments in enhancing nutrient availability for crop uptake and better plant nutrition.
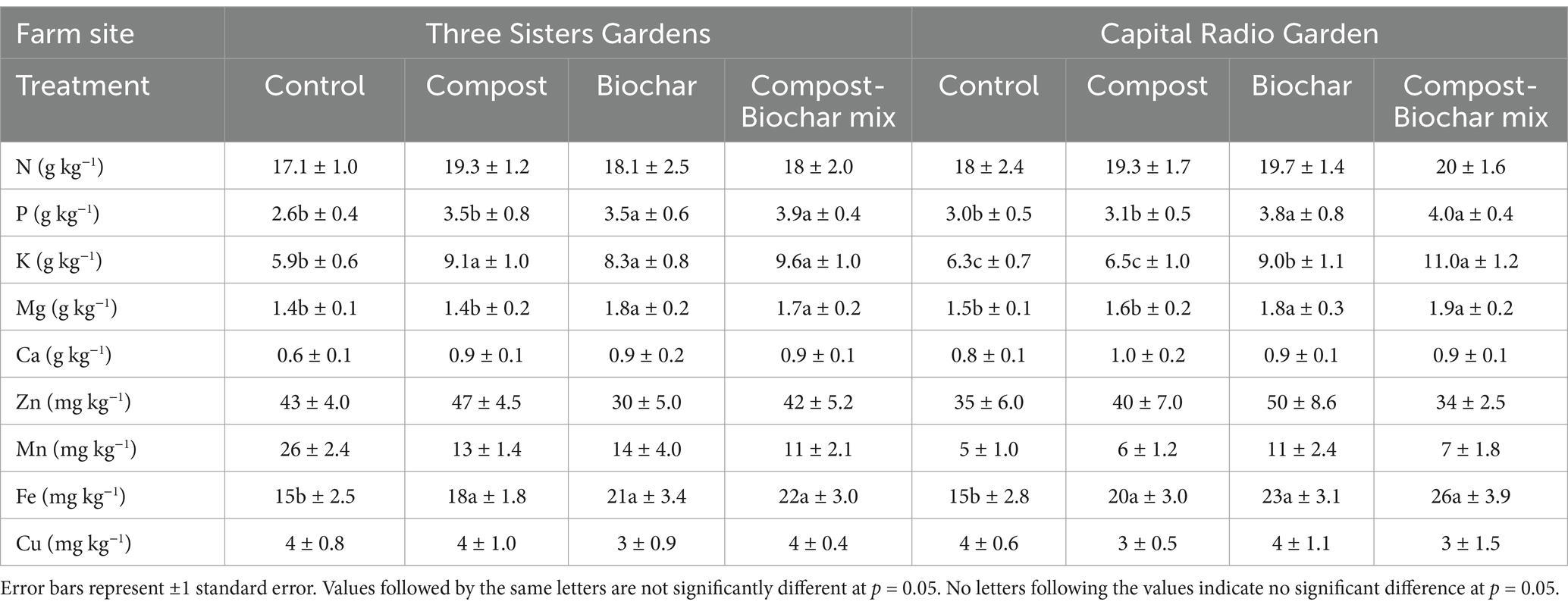
Table 4. Effects of compost, biochar, and a compost-biochar mix on corn kernel nutrient concentrations over one growing season in field trials at the Capital Radio Garden and Three Sisters Gardens, Sacramento, CA, USA.
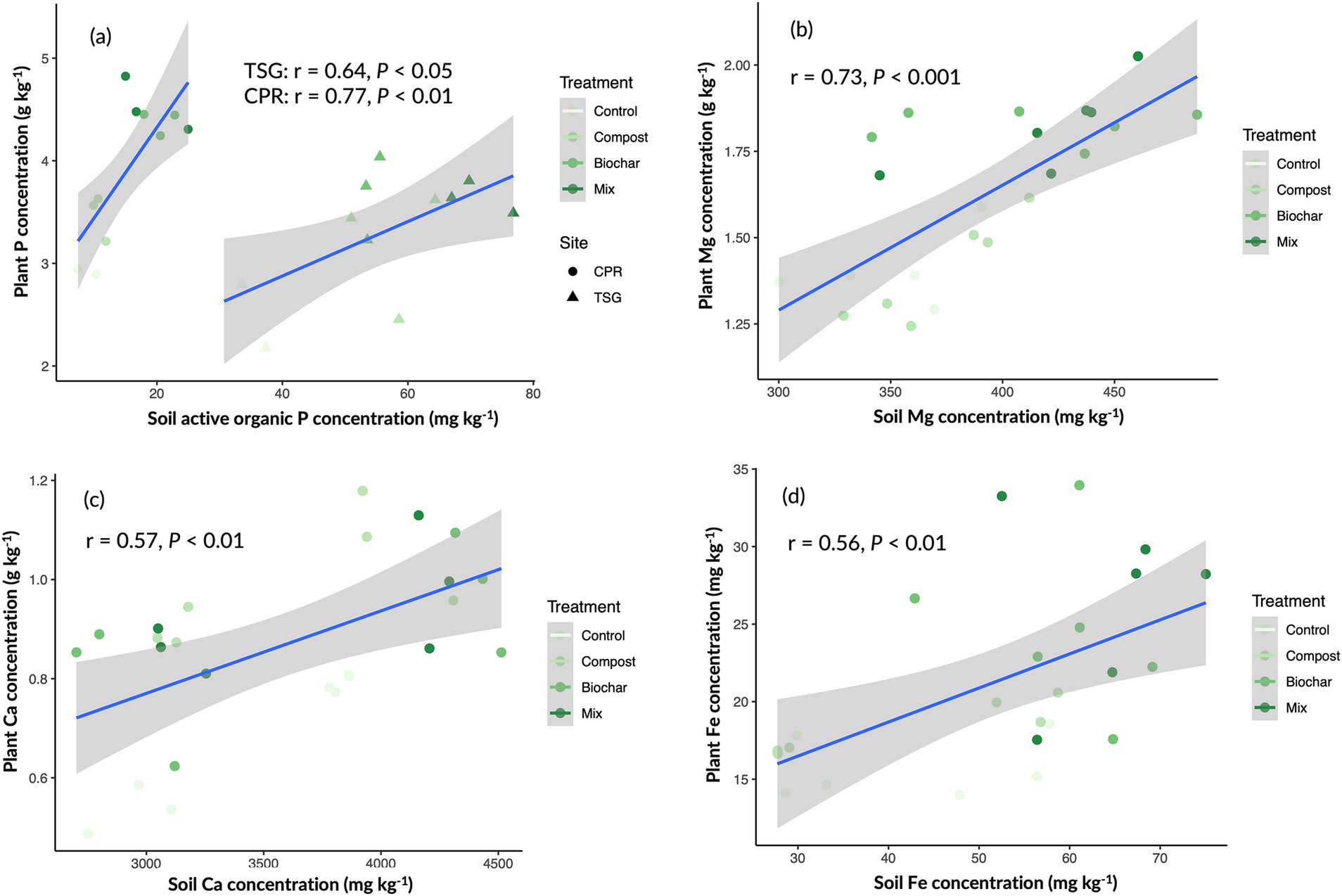
Figure 3. Pearson correlation coefficients (r) and statistical significance between selected soil nutrients (a) active organic P, (b) Mg, (c) Ca, and (d) Fe and corn kernel nutrient concentrations across the field trials at the Capital Radio Garden (CPR) and Three Sisters Gardens (TSG), Sacramento, CA, USA.
4 Discussion
4.1 Soil physicochemical properties
Soil WHC increased significantly with the addition of biochar but not compost. This results aligns with previous findings that biochar’s porous structure enhances soil water retention by increasing microporosity and aggregation (Razzaghi et al., 2020). Unlike biochar, compost provides readily decomposable organic matter that enhances soil structure, but usually does not have the same porosity or surface area as biochar (Seyedsadr et al., 2022; Thao et al., 2023). Electrical conductivity (EC) only responded to compost application, particularly at the Three Sisters Gardens site (Table 2). This response could be attributed to the salts and nutrients introduced directly through compost decomposition, where the compost produced at the Three Sisters Gardens site has slightly higher nutrient content (e.g., P, K, Mg, and Ca) compared to the compost at Capital Radio Garden (Table 1 and Supplementary Table 2). The elevated EC response in compost-treated plots at Three Sisters Gardens suggests that site-specific differences in compost composition and nutrient inputs played a role in influencing soil nutrient availability. In contrast, biochar alone did not significantly affect soil EC, possibly due to its lower capacity to directly introduce nutrients (Table 1). Soil CEC was the highest in the compost-biochar mix treatment (Table 1), which likely reflects the cumulative effect of both amendments (Frimpong et al., 2021; Gao et al., 2023).
For soil N availability, biochar alone had a significant positive effect on soil nitrate (NO3−–N) at both mid and late seasons (Figure 1). This finding aligns with previous studies showing that biochar can stimulate nitrification and increase the abundance of nitrifying bacteria and archaea (DeLuca and Sala, 2006; Gao and DeLuca, 2020) particularly when the ammonium (NH₄+–N) substrate is sufficient under an optimal soil pH for nitrifiers (Table 2). Additionally, biochar may help retain NO₃−–N in the soil by reducing leaching and keeping them available for plant uptake over time (Gao et al., 2016). The delayed increase in NH₄+–N in response to biochar, observed only at the late growing season, suggests early microbial or plant uptake, as well as biochar’s gradual facilitation of NH₄+ sorption onto soil exchange sites (Weldon et al., 2022; Wu et al., 2023). Additionally, biochar’s influence on the retention and slow release of labile organic nitrogen might require more time to manifest, as suggested by the pattern observed in net N mineralization (Table 2). Regardless, the compost-biochar mix exhibited the highest extractable N availability throughout the season considering both NH₄+ and NO₃−, compared to control, compost alone, or biochar alone, indicating its ability to accelerate N cycling or retain extractable N, thereby potentially supporting sustained plant growth over the season.
The treatments had significant effects on various soil phosphorus (P) fractions, with the compost-biochar mix significantly increasing active inorganic P, particularly the citric acid-extractable fraction, at both sites (Table 2). This result aligns with previous studies on sandy soils (Gao et al., 2017; Gao and DeLuca, 2018), suggesting that biochar can play a role in enhancing P bioavailability by interacting with metallic ions through its organic functional groups. Biochar is known to alter soil P dynamics by binding with soil minerals that would otherwise fix P into non-available forms, making P more bioavailable (DeLuca et al., 2024; Ghodszad et al., 2021). Moreover, the increase in labile organic P observed in the compost-biochar mix treatment points to enhanced microbial-mediated P cycling. Biochar can influence microbial communities, particularly those involved in organic matter decomposition and P mineralization, such as the abundance and structure of phoC- and phoD-harboring microbial communities (Gao and DeLuca, 2018; Tian et al., 2021). The compost used at both sites supplied organic P sources (Supplementary Table 2) that are more readily accessed by plants and microbes through enzyme hydrolysis, while biochar helped to retain these nutrients and release them gradually. The combined amendment results in higher overall N and P availability (Table 2), providing a sustained supply of nutrients essential for plant growth and soil fertility.
Increased concentrations of other nutrients like Mg, Ca, and Fe were also observed in biochar and compost-biochar treatments. This could be due to the direct contribution of these nutrients from compost and biochar, and biochar’s ability to adsorb and retain metal cations, which helps prevent leaching and ensures these nutrients remain available to plants over time (Gao et al., 2017; Zemanová et al., 2017). The compost-biochar mix provided the highest total Mg, Ca, and Fe inputs compared to individual amendment alone (Supplementary Table 2), which translated into higher soil concentrations of these nutrients at late-season sampling (Table 2). Additionally, biochar may interact with root exudates, particularly carboxylic acids, that act as natural chelating agents. These exudates facilitate the mobilization of Mg, Ca, and Fe from soil minerals by forming soluble complexes, enhancing their availability to plants (Bornø et al., 2018, 2022). However, this mechanism of nutrient mobilization through root exudates and biochar interaction was not directly evidenced in our study and requires further investigation to better understand its role in nutrient cycling under compost-biochar treatments.
The compost-biochar mix treatment consistently resulted in the highest SOC and total C content, highlighting its potential for enhancing long-term carbon sequestration. While compost was applied at the same rate as biochar (10 Mg C ha−1), compost alone did not significantly increase total C or SOC compared to the control by the end of the growing season at either site (Table 2). This could be attributed to the rapid decomposition of compost’s labile organic matter, which releases CO₂ back into the atmosphere more quickly, limiting its effectiveness in long-term C storage (Gao et al., 2023; Harrison et al., 2024b). In contrast, biochar, with its more recalcitrant C structure, offers a stable form of C that can persist in soils over extended periods, thus contributing more effectively to soil C sequestration (Joseph et al., 2021). The stability of the biochar used in our study is also supported by its low H:Corg ratio (Table 1). A H:Corg ratio below 0.3 is reported to be indicative of high aromatic stability, meaning the biochar used in our study has a strong resistance to microbial degradation (Lehmann et al., 2024). While biochar alone demonstrated a clear advantage in sequestering carbon, its effects on available N and P were not as pronounced as the compost-biochar mix. Compost alone increased soil available N and P, but the mix treatment showed greater nutrient retention and gradual release, highlighting the combined effect of biochar and compost in supporting nutrient cycling. The compost-biochar mix leverages both the stable and labile C pools, enhancing soil fertility and providing a pathway for gradual C accumulation while maintaining soil productivity. These findings suggest that integrating biochar into urban agricultural systems not only plays a crucial role in mitigating climate change through carbon sequestration but, when combined with compost, offers additional benefits by improving nutrient availability and cycling.
4.2 Soil microbial activity and diversity
The compost-biochar mix treatment resulted in the highest microbial metabolic activity compared to control or compost alone or biochar alone at both sites (Figure 2). This suggests an enhanced microbial C utilization and overall metabolic function, where biochar-compost combination provided a more favorable microenvironment for microbial growth and activity. Biochar’s porous structure supported microsites that retained stable moisture and nutrient conditions, while the labile organic C in compost provided an accessible energy source for microbial metabolism (Tables 1, 3), and this synergy between biochar and compost may have contributed to the observed increase in microbial activity through diverse C substrates that support a range of microbial groups, as shown in the increased average well color development (AWCD) (Khan et al., 2023). The multiple linear regression (MLR) analysis (Table 3) also showed that soil properties, including WHC, CEC, and the availability of NO₃−–N and P, were significant predictors of microbial activity, which showed significantly response to the compost-biochar mix treatment. These findings emphasize the importance of both soil moisture and nutrient retention for sustaining active and resilient microbial communities, particularly in semi-arid regions like the study site (Cui et al., 2020). By enhancing these key soil properties simultaneously, the biochar-compost combination supported a functionally active microbial community capable of contributing to nutrient cycling and soil health.
Our results indicated that while the organic amendment treatments significantly increased microbial metabolic activity compared to control (Figure 2), there were no substantial changes in overall microbial diversity, as measured by the Shannon and Simpson indices (Table 2). This suggests that the organic amendments did not lead to major shifts in species richness or dominance in the microbial community (Chernov et al., 2015). In other words, neither rare species nor dominant species responded significantly to the organic amendments. Interestingly, the McIntosh index increased under the compost-biochar mix treatment (Figure 2), indicating an increase in the evenness within the microbial community, where the population distribution across species became more balanced (Kitikidou et al., 2024). This suggests that the compost-biochar mix supports a more even distribution of microbial species that contribute equally to soil processes, without necessarily increasing species richness or altering the dominance of existing species. Biochar’s porous structure creates microsites that offer a stable habitat for a variety of microorganisms, while compost provides a rich supply of organic substrates. Together, these amendments supported a broader range of microbial functional groups or reduced microbial competition for nutrient acquisition, which led to a more balanced microbial community (Ren et al., 2022). This reduction in competition helps maintain evenness in the microbial community by allowing more microbial groups to coexist without one group dominating. Higher evenness is also be able to provide insurance and promote resilience through the complementarity effect (Wang et al., 2021), allowing the community to maintain key ecosystem functions under environmental stress.
4.3 Corn nutrient responses
The results demonstrated that soil active organic P extracted by phosphatase was significantly correlated with corn kernel P concentrations at both study sites (Figure 3). This suggests that bioavailable organic P in the soil, which is accessible to plants through enzyme hydrolysis, directly contributes to plant P uptake. Similarly, soil and plant concentrations of Mg, Ca, and Fe were positively correlated, indicating that soil amendments directly influence nutrient availability to crops. The compost-biochar mix treatment consistently resulted in the highest levels of these essential plant nutrients, pointing to the cumulative effect of biochar and compost on supplying nutrient inputs and their efficacy in enhancing nutrient cycling and plant nutrition (Figure 3). One potential mechanism for these findings is the enhanced nutrient retention and gradual release provided by biochar and compost together. Biochar’s porous structure increases soil’s capacity to retain nutrients like P, Mg, Ca, and Fe, preventing them from leaching while making them more available for plant uptake over time (Jílková and Angst, 2022). Compost, on the other hand, provides a rich source of organic matter and microbial activity that can promote the mineralization of organic forms of nutrients into forms accessible by plants. This pattern is consistent with the amount of nutrient inputs from amendments (Supplementary Table 2), which shows that the compost-biochar mix resulted in the highest total nutrient inputs across multiple essential elements.
As discussed above, the increased concentrations of soil active organic P, Mg, Ca, and Fe in biochar-amended soils could be attributed to biochar’s ability to adsorb and retain nutrients, which can then gradually become available to plants through microbial processes (Novak et al., 2021). This is particularly relevant for P, which can be bound to organic matter or soil minerals and becomes available through extracellular enzymatic activity facilitated by a thriving microbial community (Baigorri et al., 2020). The higher soil microbial activity observed in the compost-biochar mix treatment also enhanced the enzyme-mediated release of P, thus supporting greater plant nutrient uptake (Hu et al., 2023). Moreover, biochar appears to improve the electrochemical properties of plant roots, which can further enhance nutrient uptake. Studies have shown that biochar increases the negative charge on root surfaces, there enhancing their ability to absorb nutrients such as Ca, Mg, and Fe (Farhangi-Abriz and Ghassemi-Golezani, 2023). For instance, biochar application was found to increase the number of functional groups (such as hydroxycinnamic acid and carboxyl groups) on root cell walls, which play a key role in nutrient sorption (Farhangi-Abriz and Ghassemi-Golezani, 2023). Biochar also can retain root exudates like carboxylic acids, which act as chelating agents to solubilize P and Fe from soil minerals, enhancing their bioavailability (Yuan et al., 2024). These mechanisms collectively explain the higher concentrations of these nutrients in corn kernels observed in the biochar-compost mix treatment. These findings highlight the practical benefits of co-applying compost and biochar, particularly for urban farmers seeking to optimize soil fertility and plant nutrition in resource-limited environments.
While these findings highlight the benefits of combined biochar and compost applications, it is important to acknowledge the limitations of our nutrient analysis. This study focused only on kernel nutrient concentrations rather than total biomass nutrient uptake (kg ha−1). Measuring nutrient content in both kernels and whole-plant biomass (stems, leaves, husks) would provide a more comprehensive assessment of total nutrient uptake. Given that leaves and stems were returned to on-site composting systems, understanding their nutrient retention and recycling potential is another key area for future research. Additionally, yield data (kg ha−1) was not systematically recorded during this study, limiting our ability to evaluate whether nutrient concentration differences were associated with changes in total crop productivity. Future research should incorporate biomass yield measurements to assess whether higher kernel nutrient concentrations correspond to increased total nutrient removal per unit area. Long-term trials monitoring nutrient cycling between crop biomass and composted residues would further clarify the sustainability of biochar-compost integration in urban farming systems.
5 Conclusion
This study highlights the significant potential of locally sourced biochar and compost amendments in enhancing soil health and crop nutrient uptake in urban agricultural systems. Conducted at two urban farms in Sacramento, California, the field trial revealed that the compost-biochar mix treatment led to the greatest improvements in soil physicochemical properties, including increased water holding capacity, organic matter content, and cation exchange capacity, along with greater retention of essential nutrients like available nitrogen and phosphorus. These enhancements in soil nutrient availability were reflected in increased nutrient uptake by corn, particularly for phosphorus, magnesium, calcium, and iron. While biochar alone contributed significantly to carbon sequestration, the compost-biochar combination was more effective at enhancing both nutrient retention and microbial activity, promoting a more balanced microbial community. The use of locally sourced biochar from pistachio shells and compost from local organic waste exemplifies a closed-loop system, providing a sustainable solution for urban farmers by recycling organic waste and improving soil fertility. These findings are especially relevant for urban areas facing food insecurity, as the combined application of biochar and compost not only boosts soil health but also supports resilient crop production. Although the results from this single growing season are promising, further long-term research is necessary to evaluate the lasting impacts of these amendments in urban agricultural systems. Nevertheless, this study offers a practical model for integrating resource-efficient soil management practices into urban agriculture, presenting viable solutions for sustainable food production in cities like Sacramento.
Data availability statement
The raw data supporting the conclusions of this article will be made available by the authors without undue reservation.
Author contributions
SG: Conceptualization, Data curation, Formal Analysis, Funding acquisition, Investigation, Methodology, Project administration, Visualization, Writing – original draft, Writing – review & editing. MM: Data curation, Investigation, Writing – review & editing. LG-O: Investigation, Resources, Writing – review & editing. KaB: Investigation, Resources, Writing – review & editing. KrB: Investigation, Resources, Writing – review & editing. AM: Conceptualization, Methodology, Resources, Supervision, Writing – review & editing.
Funding
The author(s) declare that financial support was received for the research and/or publication of this article. This research was supported by the Research Enhanced Support Grant from California State University, Sacramento.
Acknowledgments
We gratefully acknowledge the financial support provided by the Research Enhanced Support Grant awarded to S. Gao at California State University Sacramento. We also extend our thanks to the staff and student volunteers at Sacramento State Sustainability and Three Sisters Gardens for their assistance in maintaining the field trials, including planting, irrigation, and other essential management activities at the Capital Radio Garden and Three Sisters Gardens.
Conflict of interest
The authors declare that the research was conducted in the absence of any commercial or financial relationships that could be construed as a potential conflict of interest.
Generative AI statement
The authors declare that no Gen AI was used in the creation of this manuscript.
Publisher’s note
All claims expressed in this article are solely those of the authors and do not necessarily represent those of their affiliated organizations, or those of the publisher, the editors and the reviewers. Any product that may be evaluated in this article, or claim that may be made by its manufacturer, is not guaranteed or endorsed by the publisher.
Supplementary material
The Supplementary material for this article can be found online at: https://www.frontiersin.org/articles/10.3389/fsufs.2025.1546426/full#supplementary-material
Footnotes
References
Adhikari, B. K., Trémier, A., Martinez, J., and Barrington, S. (2010). Home and community composting for on-site treatment of urban organic waste: perspective for Europe and Canada. Waste Manag. Res. J. Sustain. Circ. Econ. 28, 1039–1053. doi: 10.1177/0734242X10373801
Agegnehu, G., Srivastava, A. K., and Bird, M. I. (2017). The role of biochar and biochar-compost in improving soil quality and crop performance: a review. Appl. Soil Ecol. 119, 156–170. doi: 10.1016/j.apsoil.2017.06.008
Baigorri, R., Francisco, S. S., Urrutia, Ó., and García-Mina, J. M. (2020). Biochar-ca and biochar-Al/-Fe-mediated phosphate exchange capacity are main drivers of the different biochar effects on plants in acidic and alkaline soils. Agronomy 10:968. doi: 10.3390/agronomy10070968
Blanco-Canqui, H., Creech, C. F., and Easterly, A. C. (2024). How does biochar impact soils and crops in a semi-arid environment? A 5-yr assessment. F. Crop. Res. 310:109340. doi: 10.1016/j.fcr.2024.109340
Bornø, M. L., Müller-Stöver, D. S., and Liu, F. (2018). Contrasting effects of biochar on phosphorus dynamics and bioavailability in different soil types. Sci. Total Environ. 627, 963–974. doi: 10.1016/j.scitotenv.2018.01.283
Bornø, M. L., Müller-Stöver, D. S., and Liu, F. (2022). Biochar modifies the content of primary metabolites in the rhizosphere of well-watered and drought-stressed Zea mays L. (maize). Biol. Fertil. Soils 58, 633–647. doi: 10.1007/s00374-022-01649-6
Carvalho, C. A., Más-Rosa, S., and Ventura, A. C. (2022). “Urban gardens and composting: effective government for strengthening urban resilience and community waste management,” In Water-Energy-Food Nexus and Climate Change in Cities. eds. L. L. B. Lazaro, L. L. Giatti, and L. S. V. Macedo (Cham: Springer International Publishing). pp. 217–241.
Cen, R., Feng, W., Yang, F., Wu, W., Liao, H., and Qu, Z. (2021). Effect mechanism of biochar application on soil structure and organic matter in semi-arid areas. J. Environ. Manag. 286:112198. doi: 10.1016/j.jenvman.2021.112198
Chernov, T. I., Tkhakakhova, A. K., and Kutovaya, O. V. (2015). Assessment of diversity indices for the characterization of the soil prokaryotic community by metagenomic analysis. Eurasian Soil Sci. 48, 410–415. doi: 10.1134/S1064229315040031
Cui, Y., Wang, X., Zhang, X., Ju, W., Duan, C., Guo, X., et al. (2020). Soil moisture mediates microbial carbon and phosphorus metabolism during vegetation succession in a semiarid region. Soil Biol. Biochem. 147:107814. doi: 10.1016/j.soilbio.2020.107814
DeLuca, T. H., Glanville, H. C., Harris, M., Emmett, B. A., Pingree, M. R. A., de Sosa, L. L., et al. (2015). A novel biologically-based approach to evaluating soil phosphorus availability across complex landscapes. Soil Biol. Biochem. 88, 110–119. doi: 10.1016/j.soilbio.2015.05.016
DeLuca, T. H., Gundale, M. J., MacKenzie, M. D., Gao, S., and Jones, D. L. (2024). “Biochar effects on soil nutrient transformations” in Biochar for environmental management. eds. J. Lehmann and S. Joseph (New York, NY, USA: Routledge), 401–440.
DeLuca, T. H., and Sala, A. (2006). Frequent fire alters nitrogen transformations in ponderosa pine stands of the inland northwest. Ecology 87, 2511–2522. doi: 10.1890/0012-9658(2006)87[2511:ffanti]2.0.co;2
Diatta, A. A., Fike, J. H., Battaglia, M. L., Galbraith, J. M., and Baig, M. B. (2020). Effects of biochar on soil fertility and crop productivity in arid regions: a review. Arab. J. Geosci. 13, 1–17. doi: 10.1007/s12517-020-05586-2
Du, Z., Zhang, J., Cheng, C., Shi, B., Zhu, L., Wang, J., et al. (2022). Effects of 3,6-Dibromocarbazole on soil health—based on soil enzymes and the biolog-ECO test. Water Air Soil Pollut. 233, 1–11. doi: 10.1007/S11270-022-05736-0/TABLES/2
Eldridge, S. M., Yin Chan, K., Donovan, N. J., Saleh, F., Orr, L., and Barchia, I. (2018). Agronomic and economic benefits of green-waste compost for peri-urban vegetable production: implications for food security. Nutr. Cycl. Agroecosyst. 111, 155–173. doi: 10.1007/s10705-018-9931-9
Farhangi-Abriz, S., and Ghassemi-Golezani, K. (2023). Improving electrochemical characteristics of plant roots by biochar is an efficient mechanism in increasing cations uptake by plants. Chemosphere 313:137365. doi: 10.1016/j.chemosphere.2022.137365
Favoino, E., and Hogg, D. (2008). The potential role of compost in reducing greenhouse gases. Waste Manag. Res. 26, 61–69. doi: 10.1177/0734242X08088584
Frimpong, K. A., Abban-Baidoo, E., and Marschner, B. (2021). Can combined compost and biochar application improve the quality of a highly weathered coastal savanna soil? Heliyon 7:e07089. doi: 10.1016/j.heliyon.2021.e07089
Gao, S., and DeLuca, T. H. (2018). Wood biochar impacts soil phosphorus dynamics and microbial communities in organically-managed croplands. Soil Biol. Biochem. 126, 144–150. doi: 10.1016/j.soilbio.2018.09.002
Gao, S., and DeLuca, T. H. (2020). Biochar alters nitrogen and phosphorus dynamics in a western rangeland ecosystem. Soil Biol. Biochem. 148:107868. doi: 10.1016/j.soilbio.2020.107868
Gao, S., Harrison, B. P., Thao, T., Gonzales, M. L., An, D., Ghezzehei, T. A., et al. (2023). Biochar co-compost improves nitrogen retention and reduces carbon emissions in a winter wheat cropping system. GCB Bioenergy 15, 462–477. doi: 10.1111/gcbb.13028
Gao, S., Hoffman-Krull, K., Bidwell, A. L., and DeLuca, T. H. (2016). Locally produced wood biochar increases nutrient retention and availability in agricultural soils of the San Juan Islands, USA. Agric. Ecosyst. Environ. 233, 43–54. doi: 10.1016/j.agee.2016.08.028
Gao, S., Hoffman-Krull, K., and DeLuca, T. H. (2017). Soil biochemical properties and crop productivity following application of locally produced biochar at organic farms on Waldron Island, WA. Biogeochemistry 136, 31–46. doi: 10.1007/s10533-017-0379-9
Ghodszad, L., Reyhanitabar, A., Maghsoodi, M. R., Asgari Lajayer, B., and Chang, S. X. (2021). Biochar affects the fate of phosphorus in soil and water: a critical review. Chemosphere 283:131176. doi: 10.1016/j.chemosphere.2021.131176
Górecka, H., Chojnacka, K., and Górecki, H. (2006). The application of ICP-MS and ICP-OES in determination of micronutrients in wood ashes used as soil conditioners. Talanta 70, 950–956. doi: 10.1016/j.talanta.2006.05.061
Harrison, B. P., McNeil, W. H., Dai, T., Campbell, J. E., and Scown, C. D. (2024a). Site suitability and air pollution impacts of composting infrastructure for California’s organic waste diversion law. Environ. Sci. Technol. 58, 19913–19924. doi: 10.1021/acs.est.4c06371
Harrison, B. P., Moo, Z., Perez-Agredano, E., Gao, S., Zhang, X., and Ryals, R. (2024b). Biochar-composting substantially reduces methane and air pollutant emissions from dairy manure. Environ. Res. Lett. 19:014081. doi: 10.1088/1748-9326/ad1ad2
Hart, S. C., Stark, J. M., Davidson, E. A., and Firestone, M. K. (1994). “Nitrogen mineralization, immobilization, and nitrification” in Methods of soil analysis. Madison, Wisconsin, USA: Part 2- microbiological and biochemical properties.
Heckman, J. R., Krogmann, U., and Wyenandt, C. A. (2022). Community shade tree leaves: beneficial uses for agriculture. HortTechnology 32, 479–484. doi: 10.21273/HORTTECH05095-22
Hu, Q., Jung, J., Chen, D., Leong, K., Song, S., Li, F., et al. (2021). Biochar industry to circular economy. Sci. Total Environ. 757:143820. doi: 10.1016/J.SCITOTENV.2020.143820
Hu, W., Zhang, Y., Xiangmin, R., Fei, J., Peng, J., and Luo, G. (2023). Coupling amendment of biochar and organic fertilizers increases maize yield and phosphorus uptake by regulating soil phosphatase activity and phosphorus-acquiring microbiota. Agric. Ecosyst. Environ. 355:108582. doi: 10.1016/j.agee.2023.108582
Jílková, V., and Angst, G. (2022). Biochar and compost amendments to a coarse-textured temperate agricultural soil lead to nutrient leaching. Appl. Soil Ecol. 173:104393. doi: 10.1016/j.apsoil.2022.104393
Joseph, S., Cowie, A. L., Van Zwieten, L., Bolan, N., Budai, A., Buss, W., et al. (2021). How biochar works, and when it doesn’t: a review of mechanisms controlling soil and plant responses to biochar. GCB Bioenergy 13, 1731–1764. doi: 10.1111/GCBB.12885
Joyner, L., Gonzales, G., Gao, S., and Cachelin, A. (2025). “Understanding environmental and food (in) justice through community-based research: stories from Sacramento community coalitions” in Equity in the urban built environment. ed. B. Bereitschaft (New York, NY, USA: Routledge), 64–78.
Khan, N., Bolan, N., Jospeh, S., Anh, M. T. L., Meier, S., Kookana, R., et al. (2023). Complementing compost with biochar for agriculture, soil remediation and climate mitigation. Adv. Agron. 179, 1–90. doi: 10.1016/bs.agron.2023.01.001
Kharola, S., Ram, M., Kumar Mangla, S., Goyal, N., Nautiyal, O. P., Pant, D., et al. (2022). Exploring the green waste management problem in food supply chains: a circular economy context. J. Clean. Prod. 351:131355. doi: 10.1016/J.JCLEPRO.2022.131355
Kiersztyn, B., Chróst, R., Kaliński, T., Siuda, W., Bukowska, A., Kowalczyk, G., et al. (2019). Structural and functional microbial diversity along a eutrophication gradient of interconnected lakes undergoing anthropopressure. Sci. Rep. 9, 1–14. doi: 10.1038/s41598-019-47577-8
Kitikidou, K., Milios, E., Stampoulidis, A., Pipinis, E., and Radoglou, K. (2024). Using biodiversity indices effectively: considerations for forest management. Ecologies 5, 42–51. doi: 10.3390/ecologies5010003
Kurganova, I. N., de Gerenyu, V. O. L., Lancho, J. F. G., and Oehm, C. T. (2012). Evaluation of the rates of soil organic matter mineralization in forest ecosystems of temperate continental, mediterranean, and tropical monsoon climates. Eurasian Soil Sci. 45, 68–79. doi: 10.1134/S1064229312010085
Lehmann, J., Abiven, S., Azzi, E., Fang, Y., Singh, B. P., Sahi, S., et al. (2024). “Persistence of biochar: mechanisms, measurements, predictions” in Biochar for environmental management. eds. J. Lehmann and S. Joseph (New York, NY, USA: Routledge), 277–311.
Lehmann, J., Bossio, D. A., Kögel-Knabner, I., and Rillig, M. C. (2020). The concept and future prospects of soil health. Nat. Rev. Earth Environ. 1, 544–553. doi: 10.1038/s43017-020-0080-8
Lehmann, J., and Joseph, S. (2024). Biochar for environmental management science, technology and implementation. 3rd Edn. New York, NY, USA: Routledge.
Loveday, J. (1974). Methods for analysis of irrigated soils. Wallingford, Oxfordshire, UK: Commonwealth Agricultural Bureaux.
Malone, Z., Berhe, A. A., and Ryals, R. (2023). Impacts of organic matter amendments on urban soil carbon and soil quality: a meta-analysis. J. Clean. Prod. 419:138148. doi: 10.1016/j.jclepro.2023.138148
Mohan, S. V., Hemalatha, M., Amulya, K., Velvizhi, G., Chiranjeevi, P., Sarkar, O., et al. (2020). Decentralized urban farming through keyhole garden: a case study with circular economy and regenerative perspective. Mater. Circ. Econ. 2, 1–12. doi: 10.1007/s42824-020-00011-1
Morrow, O., and Davies, A. (2022). Creating careful circularities: community composting in new York City. Trans. Inst. Br. Geogr. 47, 529–546. doi: 10.1111/tran.12523
Mulvaney, R. L., Sparks, D. L., Page, A. L., Helmke, P. A., Loeppert, R. H., Soltanpour, P. N., et al. (1996). “Nitrogen-inorganic forms” in Methods soil anal. Madison, Wisconsin, USA: Part 3-chemical methods, 1123–1184.
Munter, R. C., Halverson, T. L., and Anderson, R. D. (1984). Quality assurance for plant tissue analysis by ICP-AES. Commun. Soil Sci. Plant Anal. 15, 1285–1322. doi: 10.1080/00103628409367559
Nogeire-McRae, T., Ryan, E. P., Jablonski, B. B. R., Carolan, M., Arathi, H. S., Brown, C. S., et al. (2018). The role of urban agriculture in a secure, healthy, and sustainable food system. Bioscience 68, 748–759. doi: 10.1093/BIOSCI/BIY071
Novak, J. M., Watts, D. W., Sigua, G. C., and Ducey, T. F. (2021). Corn grain and Stover nutrient uptake responses from sandy soil treated with designer biochars and compost. Agronomy 11:942. doi: 10.3390/agronomy11050942
Ohno, T., and Zibilske, L. M. (1991). Determination of low concentrations of phosphorus in soil extracts using malachite green. Soil Sci. Soc. Am. J. 55, 892–895. doi: 10.2136/sssaj1991.03615995005500030046x
Qian, S., Zhou, X., Fu, Y., Song, B., Yan, H., Chen, Z., et al. (2023). Biochar-compost as a new option for soil improvement: application in various problem soils. Sci. Total Environ. 870:162024. doi: 10.1016/j.scitotenv.2023.162024
R Core Team (2020). R: a language and environment for statistical computing. Vienna, Austria: R Found. Stat. Comput.
Rashid, M. I., and Shahzad, K. (2021). Food waste recycling for compost production and its economic and environmental assessment as circular economy indicators of solid waste management. J. Clean. Prod. 317:128467. doi: 10.1016/j.jclepro.2021.128467
Razzaghi, F., Obour, P. B., and Arthur, E. (2020). Does biochar improve soil water retention? A systematic review and meta-analysis. Geoderma 361:114055. doi: 10.1016/j.geoderma.2019.114055
Ren, H., Li, Z., Chen, H., Zhou, J., and Lv, C. (2022). Effects of biochar and plant growth-promoting Rhizobacteria on plant performance and soil environmental stability. Sustain. For. 14:10922. doi: 10.3390/su141710922
Richardville, K., Egel, D., Flachs, A., Jaiswal, A., Perkins, D., Thompson, A., et al. (2022). Leaf mold compost reduces waste, improves soil and microbial properties, and increases tomato productivity. Urban Agric. Reg. Food Syst. 7:e20022. doi: 10.1002/uar2.20022
Sacramento State Sustainability (2022). Sacramento State campus sustainability report. Sacramento. Available online at: https://www.csus.edu/experience/innovation-creativity/sustainability/_internal/_documents/sustainability-report-21-22.pdf (Accessed March 9, 2025).
Salomon, M. J., and Cavagnaro, T. R. (2022). Healthy soils: the backbone of productive, safe and sustainable urban agriculture. J. Clean. Prod. 341:130808. doi: 10.1016/j.jclepro.2022.130808
Seyedsadr, S., Šípek, V., Jačka, L., Sněhota, M., Beesley, L., Pohořelý, M., et al. (2022). Biochar considerably increases the easily available water and nutrient content in low-organic soils amended with compost and manure. Chemosphere 293:133586. doi: 10.1016/j.chemosphere.2022.133586
Tan, E. C. D. (2023). Sustainability engineering: challenges, technologies, and applications. Boca Raton, FL, USA: CRC Press.
Thao, T., Harrison, B. P., Gao, S., Ryals, R., Dahlquist-Willard, R., Diaz, G. C., et al. (2023). The effects of different biochar-dairy manure co-composts on soil moisture and nutrients retention, greenhouse gas emissions, and tomato productivity: observations from a soil column experiment. Agrosyst. Geosci. Environ. 6, 1–14. doi: 10.1002/agg2.20408
Tian, J., Kuang, X., Tang, M., Chen, X., Huang, F., Cai, Y., et al. (2021). Biochar application under low phosphorus input promotes soil organic phosphorus mineralization by shifting bacterial phoD gene community composition. Sci. Total Environ. 779:146556. doi: 10.1016/J.SCITOTENV.2021.146556
Torrijos, V., Calvo Dopico, D., and Soto, M. (2021). Integration of food waste composting and vegetable gardens in a university campus. J. Clean. Prod. 315:128175. doi: 10.1016/j.jclepro.2021.128175
Villagra-Mendoza, K., and Horn, R. (2018). Effect of biochar addition on hydraulic functions of two textural soils. Geoderma 326, 88–95. doi: 10.1016/j.geoderma.2018.03.021
Wang, X. Y., Ge, Y., Gao, S., Chen, T., Wang, J., and Yu, F. H. (2021). Evenness alters the positive effect of species richness on community drought resistance via changing complementarity. Ecol. Indic. 133:108464. doi: 10.1016/j.ecolind.2021.108464
Weldon, S., van der Veen, B., Farkas, E., Kocatürk-Schumacher, N. P., Dieguez-Alonso, A., Budai, A., et al. (2022). A re-analysis of NH4+ sorption on biochar: have expectations been too high? Chemosphere 301:134662. doi: 10.1016/j.chemosphere.2022.134662
Wortman, S. E., and Lovell, S. T. (2013). Environmental challenges threatening the growth of urban agriculture in the United States. J. Environ. Qual. 42, 1283–1294. doi: 10.2134/jeq2013.01.0031
Wu, X., Ye, M., Wang, J., Wu, F., Liu, C., Li, Z., et al. (2023). Adsorption characteristics and mechanism of ammonia nitrogen and phosphate from biogas slurry by Ca2+−modified soybean straw biochar. PLoS One 18:e0290714. doi: 10.1371/journal.pone.0290714
Yuan, J., Chen, H., Chen, G., Pokharel, P., Chang, S. X., Wang, Y., et al. (2024). Long-term biochar application influences phosphorus and associated iron and sulfur transformations in the rhizosphere. Carbon Res. 3:25. doi: 10.1007/s44246-024-00109-0
Keywords: biochar, compost, urban agriculture, agroecosystem nutrient management, circular economy
Citation: Gao S, Medina M, Gonzalez-Ospina L, Burce K, Burce K and Melbourne A (2025) Boosting soil health and crop nutrients with locally sourced biochar and compost in Sacramento urban agriculture. Front. Sustain. Food Syst. 9:1546426. doi: 10.3389/fsufs.2025.1546426
Edited by:
Kiril Manevski, Aarhus University, DenmarkCopyright © 2025 Gao, Medina, Gonzalez-Ospina, Burce, Burce and Melbourne. This is an open-access article distributed under the terms of the Creative Commons Attribution License (CC BY). The use, distribution or reproduction in other forums is permitted, provided the original author(s) and the copyright owner(s) are credited and that the original publication in this journal is cited, in accordance with accepted academic practice. No use, distribution or reproduction is permitted which does not comply with these terms.
*Correspondence: Si Gao, cy5nYW9AY3N1cy5lZHU=