- 1Institute of Environment and Sustainable Development in Agriculture, Chinese Academy of Agricultural Sciences, Beijing, China
- 2Institute of Agri-Food Processing and Nutrition, Beijing Academy of Agriculture and Forestry Sciences, Beijing, China
- 3Division of Horticultural Sciences, Institute of Plant Sciences and Resource Conservation, University of Bonn, Bonn, Germany
There is a growing interest in cultivating pakchoi under controlled environment agriculture. However, research on establishing the ideal environmental conditions for growing pakchoi in plant factories remains insufficient. Therefore, in this study, we investigated the optimal red-to-blue light ratio for enhancing pakchoi plants’ nutritional quality and growth. Five light treatments (B, R2B1, R4B1, R8B1, and R) were employed in our study, while white light (W) provided by fluorescent lamps was served as the control. The cultivars used were ‘Jingguan No. 1′, a green pakchoi, and ‘Ziguan No. 1′, a red pakchoi. After 20 days of treatment application, we observed significant improvements in dry weight and leaf area in green and red pakchoi plants under treatment R. Specifically, dry weight increased by 14.8% in green pakchoi and 26.7% in red pakchoi, while leaf area increased by 41.8% in green pakchoi and 81.1% in red pakchoi compared to the control treatment. Additionally, treatment R2B1 promoted net photosynthetic rate (Pn) in red pakchoi plants and enhanced stomatal conductance (Gs), intercellular CO2 concentration (Ci), and transpiration rate (Tr) in both pakchoi varieties. Treatment R4B1 facilitated the accumulation of photosynthetic pigments in pakchoi cultivars. On the other hand, the control treatment was found to be more conducive to the accumulation of glucosinolate concentration (GSc) in both red and green pakchoi cultivars. Notably, the concentrations of vitamin C (Vc) and soluble sugar in green pakchoi plants under treatment R4B1 increased by 78.5 and 31.4%, respectively, compared with those under control treatment. Similarly, the concentrations of Vc, soluble sugar, and anthocyanin in red pakchoi plants under treatment R2B1 were increased by 31.6, 177.8, and 114.4%, respectively, compared with those under the control treatment. These findings indicate that different pakchoi varieties exhibit distinct responses to different light-quality combinations.
1 Introduction
Pakchoi, also known as bok choy, is a nutrient-rich leafy vegetable widely consumed in China and other parts of the world. It belongs to the Brassica family and is highly valued for its health benefits, including a rich content of vitamins, minerals, and antioxidants (Kaleri et al., 2020; Dong et al., 2024). In China, pakchoi accounts for 30 to 40% of the total vegetable production area, making it a staple in many households (Wang Y. et al., 2024). Its nutrient profile includes dietary fibers, vitamin C, carotenoids, and glucosinolates (GSc), which contribute to its popularity. GS, are key bioactive compounds in pakchoi and other Brassica vegetables. These sulfur-containing compounds, found in the Brassicaceae family, contribute to the pungent flavors of these vegetables and offer health benefits, including potential anti-cancer and antimicrobial properties (Maina et al., 2020). Additionally, glucosinolates and their derivatives play essential roles in plant defense and human health protection. Their biological activities, such as anticancer properties, bacteriostasis, and biological control, make them significant subjects of research in botany, medicine, and food science (Connolly et al., 2021). Pakchoi can be harvested at various growth stages, from young seedlings to mature shoots, allowing for a prolonged harvest period (Ding et al., 2022). The flavor of pakchoi is influenced by glucosinolates (GS), such as 2-hydroxy-3-butenyl GS and 3-indole methyl G (Yao et al., 2018). In previous studies, different Brassica species have shown varying concentrations of GSs, with kale exhibiting the highest concentration and cabbage the lowest (Liping et al., 2015). This variation is important to consider when studying the impact of environmental factors, such as light on GS accumulation in pakchoi, as it may help guide the optimization of growing conditions for improving its nutritional quality. Furthermore, it is worth noting that the concentration and composition of GSs, and generally healthy ingredients, can vary significantly based on the cultivars, plant organs, environmental factors, and cultivation conditions of the vegetables (Podsędek, 2007; Kim et al., 2023).
Pakchoi is mainly produced in the open field and only a tiny part in greenhouses. These cultivation methods are influenced by the external natural environment conditions, leading to long growth periods, quality variation, and frequent diseases and pests in pakchoi plants (Guirrou et al., 2023). As the demand for high-quality vegetables continues to rise, there is increasing interest in growing pakchoi in controlled environment agriculture. The closed plant production systems include plant factories with artificial light (PFAL) and vertical farming. However, the optimal environmental parameters required for cultivating pakchoi plants in a PFAL, especially those related to the light environment, have not yet been studied and remain unclear.
The rapid development of light-emitting diode (LED) technology makes it possible to study the photobiology of plants’ response to different light parameters. In addition to light quantity and period, many studies have focused on the biological response of plants to the light spectral composition in recent years. Light quality and spectrum have much more complex effects on plant morphology and physiology (Li et al., 2000; Wang et al., 2016).
Typically, red light (620–700 nm) and blue light (400–500 nm) are widely utilized for promoting plant growth, primarily due to their higher absorption rates by photosynthetic pigments than other wavelengths (Olle and Viršile, 2013). As a result, these specific wavelengths have been recognized as primary contributors to the light spectrum required for plant photosynthesis and development under controlled environmental conditions (Samuolienė et al., 2013). For instance, Li et al. (2013) studied the effects of different light qualities on the growth and morphogenesis of rapeseed (Brassica napus L.) plantlets in vitro. The use of a red-to-blue LED light ratio of 1:3 was found to be a favorable combination for cultivating rapeseed plantlets, as indicated by the observed differentiation rate, proliferation rate, growth rate, and transplantation survival rate. Previous studies have shown that red light benefits specific leaf areas and the intercellular CO2 concentration in tomato seedlings (Zhang et al., 2022). It also promotes pepper seedlings’ stomatal conductance, transpiration rate and photosynthetic capacity (Li et al., 2023). Moreover, red light also benefits the increase of soluble sugar, crude fiber and acid concentration of vegetables (Chen et al., 2009; Ilić and Fallik, 2017; Anum et al., 2024a). While blue light can control plant growth, leaf expansion, stomatal opening, photosynthesis and pigment accumulation (Ramalho et al., 2002). Studies also showed that blue light could reduce nitrate content and nitrite reductase activity and increase Vc and crude protein concentrations (Bian et al., 2018; Fan et al., 2019). A combination of red and blue light can harness the benefits of both wavelengths to fulfill the growth requirements of plants. Researches indicate that different plant species exhibit varying responses to red and blue light combinations (Cervantes et al., 2019; Cammarisano and Körner, 2022; Liu and van Iersel, 2022). For instance, pepper plants showed increased yields under a combination of red and blue LEDs, while lettuce demonstrated reduced height with higher blue light percentages (Naznin et al., 2019). According to Kaiser et al. (2019) and Izzo et al. (2021), adding proper red to blue light was beneficial to enhance biomass, leaf area, root activity and chlorophyll content in lettuce and tomatoes. Red to blue light can also significantly improve the nutritional quality of plants. Zhang et al. (2020) showed that red to blue lights could improve soluble sugar, protein, total glucosinolates, anthocyanins, flavonoids and antioxidant activity in kale.
At present, most studies focused on the effects of red and blue light ratios on the plant growth morphology, and photosynthetic characteristics of leafy vegetables, including spinach (Gao et al., 2020), water spinach (Shuo et al., 2019) and lettuce (Gao et al., 2018) in PFAL. However, scant attention has been paid to the effects of different red and blue light ratios on the nutritional quality and growth of pakchoi plants. Only a limited number of studies have been conducted to date, despite the growing interest in optimizing plant growth and nutrition through light manipulation (Huang et al., 2021; Mao et al., 2021; Choi and Choi, 2023). Moreover, it is vital to select a suitable light source for improving plant development and nutritional quality by reducing the operating costs of PFAL.
The primary objective of this study was to identify the optimal light combination for cultivating green and red pakchoi plants in PFAL. Specifically, the study aims to investigate the effects of different light spectra on the growth, photosynthetic characteristics, and nutritional quality of pakchoi plants in a controlled environment. The selection of these two cultivars (‘Jingguan No. 1’, a green pakchoi, and ‘Ziguan No. 1’ a red pakchoi) is important for understanding how different red-to-blue light ratios affect them differently under varying light conditions.
2 Materials and methods
2.1 Plant materials
Green pakchoi ‘Jingguan No. 1’ and red pakchoi ‘Ziguan No. 1’ (Brassica napus L.) were selected as the experimental plant materials provided by the Vegetable Institute of Beijing Academy of Agriculture and Forestry Sciences. Pakchoi seeds were sown in a substrate consisting of a mixture of peat, vermiculite, and perlite with a volume ratio of 2:1:1 using trays with 72 holes. Thirty-six trays were used for pakchoi plant cultivation in a plastic greenhouse for 14 days. After the second true leaf was fully expanded, the seedlings were randomly divided into six groups and transferred to PFAL. The day/night temperature was 23/17°C, relative humidity was 60–70%, and the concentration of CO2 was 500- 700 μmol mol−1. The LED panels (Datang New Energy Technology Co., Ltd., Shenzhen, China) were installed on cultivation shelves. The distance between the LED panels and the crops was 0.3 m. The modified Yamasaki nutrient solution (pH = 5.9; EC = 1.5 mS·cm−1) was applied for plant growth during the experiment. Seedlings of pakchoi were irrigated every other day with the Yamasaki nutrient solution to prevent moisture loss during germination, ensuring optimal growth conditions (Figure 1).
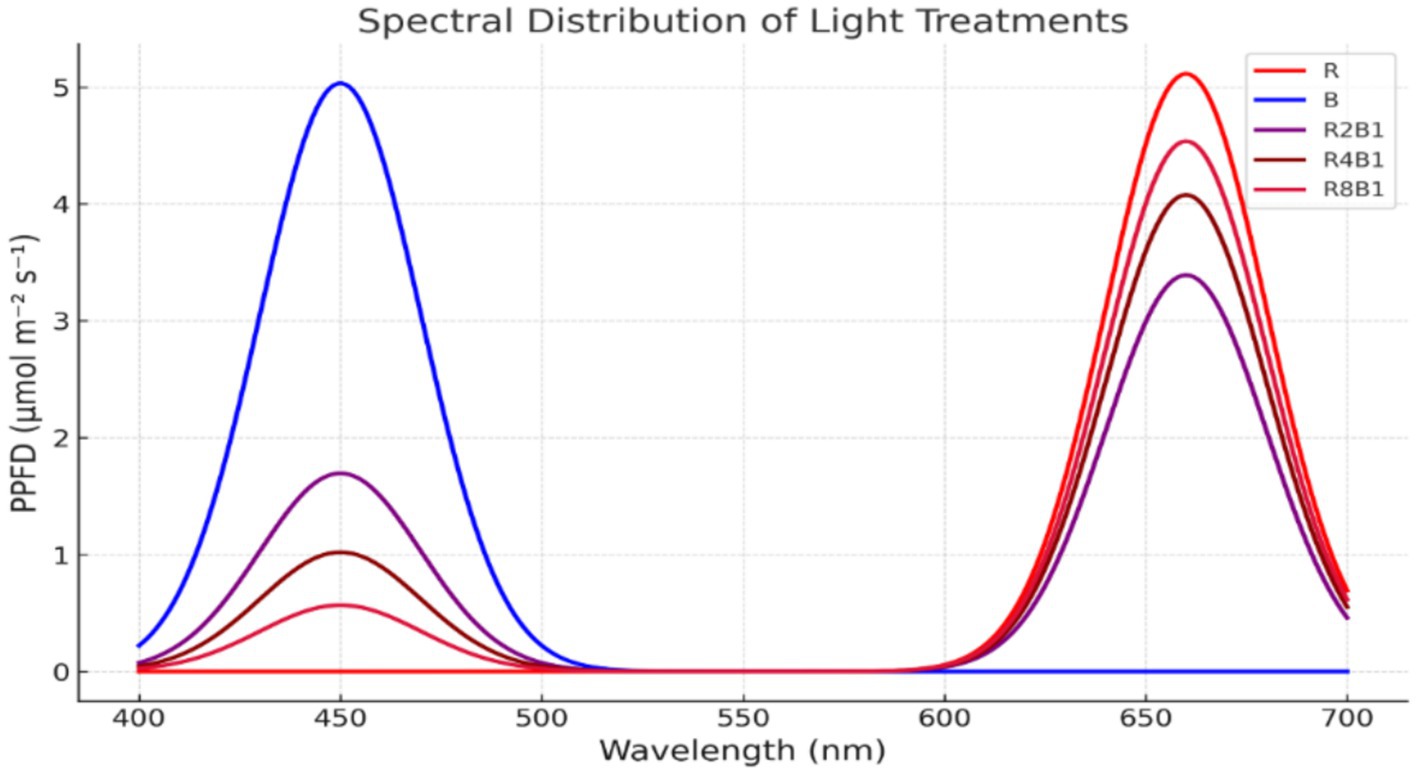
Figure 1. Differentiation in spectral properties of five treatments with varying red-blue light ratios.
2.2 Experimental design
This experiment involved five treatments with varying red-to-blue light ratios, as detailed in Table 1. Each treatment (B, R2:B1, R4:B1, R8:B1, and R) was subjected to a total PPFD of 250 μmol m−2 s−1, delivered using LED panels (Datang New Energy Technology Co., Ltd., Shenzhen, China). As a control, white light (W) was provided by fluorescent lamps (TL-D56W; Osram), offering a broad spectrum between 380 and 750 nm. A spectrometer (Avaspec-2048CI; Avantes, Apeldoorn, the Netherlands) was used to measure the PPFD (μmol m−2 s−1) levels for red and blue lights. The spectral composition, including the red-to-blue (R:B) ratios, was determined by analyzing the intensity of wavelengths within the red (600–700 nm) and blue (400–500 nm) regions. The R:B ratio was calculated based on the relative photon flux density in these wavelength ranges for each treatment. Each treatment was repeated three times to ensure data reliability. Plants were harvested and analyzed 20 days post-transplantation. Figure 2 presents the light spectrum corresponding to each treatment.
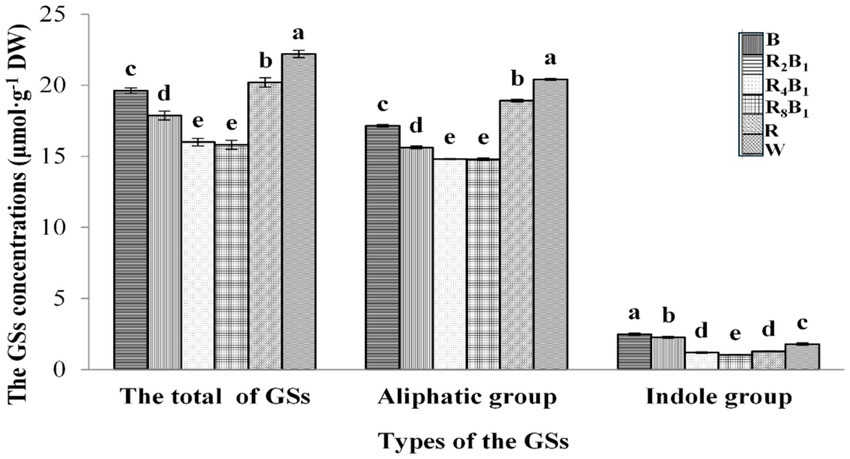
Figure 2. The concentrations (μmol·g−1 DW) of total glucosinolates (GSs), the aliphatic GS group, and the indole GS group in Green Pakchoi (Jingguan No. 1) assessed on day 20 after treatment application under varying red-blue light ratios in a controlled environment. Different lowercase letters indicate significant differences at the 0.05 level between treatments.
2.3 Measurements
2.3.1 Plant growth
After 20 days of transplanting, 15 plants were randomly selected from each treatment. Total fresh weight was measured using an electronic weighing balance. A vernier caliper was used to measure the stem diameter and root length. The total dry weights of the plants were measured using an electronic balance (Si-234; Denver Instrument, Bohemia, NY, USA) after drying the fresh samples in an oven at 80°C to a constant weight. Moreover, the total leaf area was measured using a leaf area meter (LI-3100C, LI-COR Biosciences, Lincoln, NE, USA).
2.3.2 Photosynthetic characteristics
The net photosynthetic rate (Pn), stomatal conductance (Gs), intercellular CO2 concentration (Ci), and transpiration rate (Tr) of the second true leaf of 15 pakchoi plants were measured using a portable photosynthetic instrument (LI-6400XT, LI-COR Biosciences, Lincoln, NE, USA). The measurements were taken under the actual light conditions of plant growth with a transparent leaf chamber (6400–08, LI-COR Biosciences, Lincoln, NE, USA). The environmental conditions of the leaf chamber were maintained at 24°C, with a CO2 level of 700 μmol m−2 s−1 and relative humidity of 60–70%. The order of measurements was arranged randomly for each replication, and each treatment was repeated three times.
The chlorophyll a (Chl a), chlorophyll b (Chl b) and carotenoid content were measured on day 20 after transplanting. Three fresh leaf disks (approximately 3.74 cm2) were taken from the same point on the leaf using a leaf perforator and then placed into 10 mL centrifuge tubes. These samples were soaked overnight with 7 mL of 95% ethanol and stored in a dark, cool place at 4°C. The absorbance of the liquid extract was measured using an ultraviolet spectrophotometer (UV-1800, Shimadzu Corp., Kyoto, Japan) at wavelengths of 665 nm (Chl a), 649 nm (Chl b), and 470 nm (carotenoid), respectively. The readings were taken immediately after extraction using cuvettes. Chlorophyll and carotenoid contents were determined using the equations reported by Lichtenthaler and Wellburn (1983). Chlorophyll a + b (Chl a + b) was calculated by adding Chl a to Chl b, while chlorophyll a/b (Chl a/b) was the ratio of Chl a to Chl b.
2.3.3 Nutritional quality and active components analysis
Leaf samples were collected on day 20 after treatment application, quickly frozen with liquid nitrogen, and then stored at −80°C prior to measurement to preserve pigment integrity. The concentrations of Vc, soluble sugars, crude protein, anthocyanins, and glucosinolates (GSs) were measured. The concentration of Vc was determined following national food safety standards GB 14754 (Fecka et al., 2021). The crude protein concentration was assessed according to the Association of Official Analytical Chemists method 976.05 (AOAC, 2000). Anthocyanins were quantified using NYT 2640 (Lee et al., 2005), while soluble sugar content was determined based on Zhang et al. (2021) and GSs were evaluated using the HPLC method as described by Nieto et al. (2023).
2.4 Data analysis
All tests were carried out in triplicate, and the data were exhibited as mean ± standard deviation (SD). The statistical software SPSS 25.0 was used to analyze variance (ANOVA). Significant differences between treatments were determined using Tukey’s post hoc test at p ≤ 0.05. All figures were generated using Origin 8.0 software.
3 Results
3.1 Plant growth and morphology
As shown in Table 2, the plant height of green pakchoi (Jingguan No. 1) under treatment R4B1 was the largest, measuring 1.3 times greater than that under the control treatment. Plant height under treatment R increased by 13.0% compared to the control treatment. Significantly higher stem diameters were observed under treatments R2B1 and R compared to those under the other treatments. No significant difference in root length was found between treatment R4B1 and the control, but it was higher than that under the other treatments. Compared to the control, leaf area increased by 17.7 and 14.8%, respectively, under treatments R2B1 and R. The highest dry weight of pakchoi plants was recorded under treatment R.
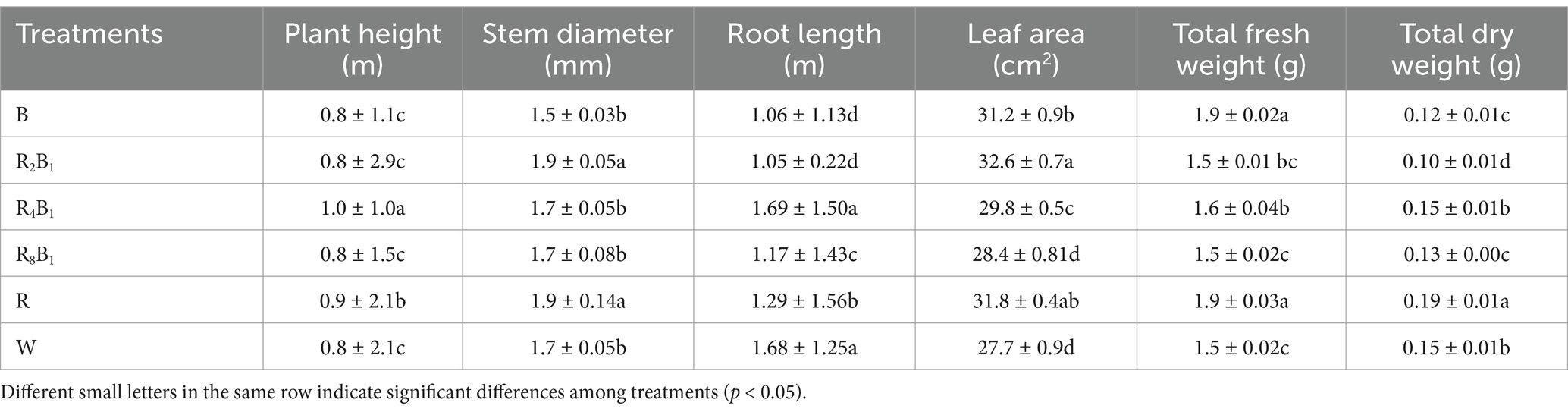
Table 2. The growth and morphology of Green Pakchoi (Jingguan No. 1) were assessed on day 20 after treatment application under varying red-blue light ratios in a controlled environment.
Different from the response of various red-to-blue (R/B) ratios in red pakchoi, the highest plant height was observed under treatment B, which increased by 27.3% compared to the control treatment (Table 3). The stem diameter under different red-to-blue ratios was significantly greater than that under the control treatment. Except for treatments R2B1 and R4B1, the root lengths under treatments B, R8B1, and R were 1.0, 1.3, and 1.2 times higher than that under the control treatment, respectively. The pakchoi plants’ leaf area and total dry weight were highest under treatment R, increasing by 41.8 and 81.1% compared to the control treatment, respectively. No significant difference in total dry weight was found among treatments B, R2B1, R4B1, R8B1, and W.
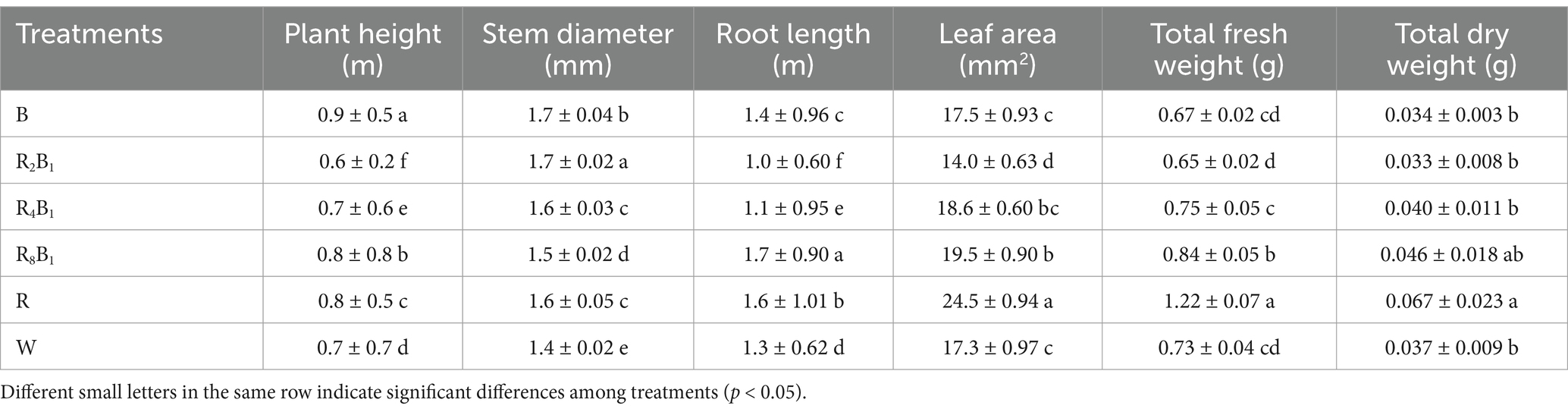
Table 3. The growth and morphology of Red Pakchoi (Ziguan No. 1) were assessed on day 20 after treatment application under varying red-blue light ratios in a controlled environment.
3.2 Leaf photosynthetic characteristics
3.2.1 Leaf photosynthesis
According to Table 4, the Pn of green pakchoi leaf was the highest under treatment R8B1, followed by that under treatment W. Moreover, the Pn under treatment R and B showed the lowest data. The Gs, Ci and Tr under treatment R2B1 were the highest, which were 2.4, 1.3 and 2.0 times higher than that under control treatment, respectively. On the other hand, Gs, Ci, and Tr under treatment R4B1 were the lowest, decreasing by 66.7, 18.3, and 65.2%, respectively, compared with those under control treatment.
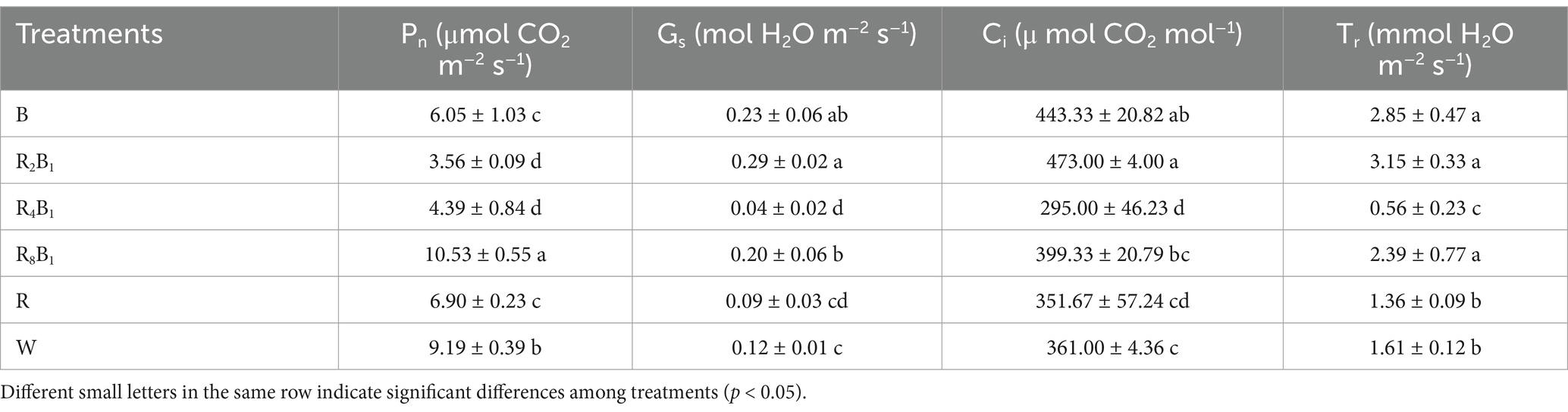
Table 4. Photosynthetic parameters, including net photosynthetic rate (Pn), stomatal conductance (Gs), internal carbon dioxide concentration (Ci), and transpiration rate (Tr) of Green Pakchoi (Jingguan No. 1), assessed on day 20 after treatment application under varying red-blue light ratios in a controlled environment.
As shown in Table 5, the photosynthetic rate (Pn) of red pakchoi leaves was highest under treatment W, followed by treatment R2B1. There were no significant differences among treatments B, R4B1, R8B1, and R. Compared to treatment W, the Pn under treatments R4B1 and R8B1 decreased by 38.6 and 42.0%, respectively. The stomatal conductance (Gs), intercellular CO2 concentration (Ci), and transpiration rate (Tr) were highest under treatment R2B1, with no significant differences observed between treatments B and W. The Gs, Ci, and Tr under treatment R8B1 decreased by 75.0, 22.3, and 73.3%, respectively, compared to those under the control treatment.
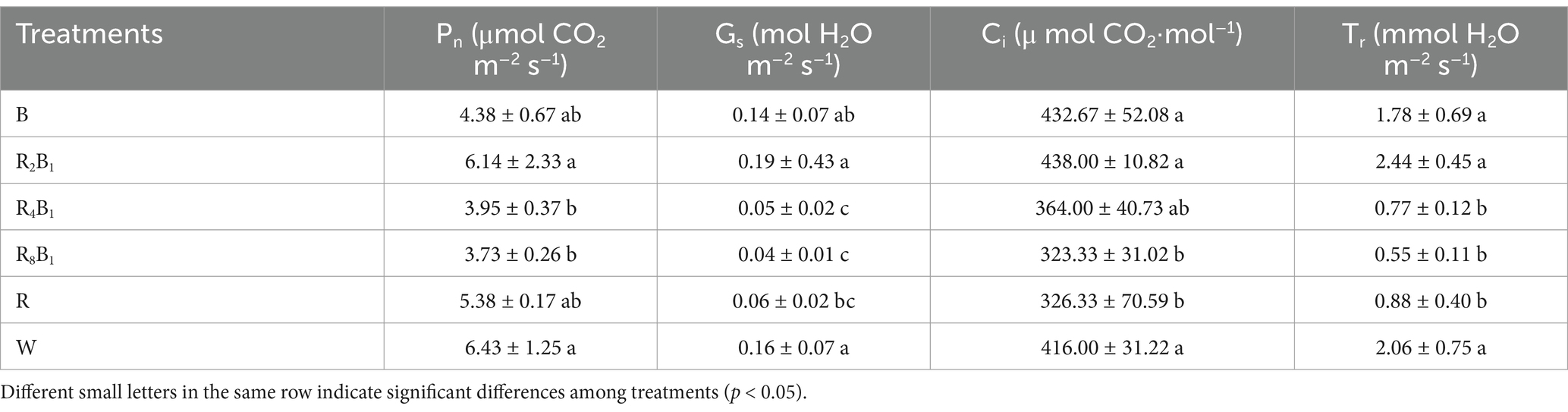
Table 5. Photosynthetic parameters, including net photosynthetic rate (Pn), stomatal conductance (Gs), internal carbon dioxide concentration (Ci), and transpiration rate (Tr) of Red pakchoi (Ziguan No. 1), assessed on day 20 after treatment application under varying red-blue light ratios in a controlled environment.
3.2.2 Photosynthetic pigments
Table 6 shows the photosynthetic pigments of green pakchoi as affected by different red-to-blue ratios. An increasing trend was observed for the Chl a and Chl a + b content of pakchoi leaves, with the highest chlorophyll content found under treatments R2B1 and R4B1. No significant differences were detected between treatments R2B1, R4B1, and W. The lowest Chl a and Chl a + b content were recorded under treatment B, which were 21.4 and 35.7% lower than those under the control treatment, respectively. Compared to the control treatment, the Chl b concentration under treatment B decreased by 40.0%, with no significant differences observed among the other treatments. The Chl a/b ratio was unaffected by different red-to-blue ratios. Different red-to-blue ratios significantly affected the carotenoid concentration in pakchoi leaves. The highest carotenoid content was observed under treatment R4B1, which increased by 33.3% compared to treatment W. There were no significant differences between the other treatments and the control treatment.
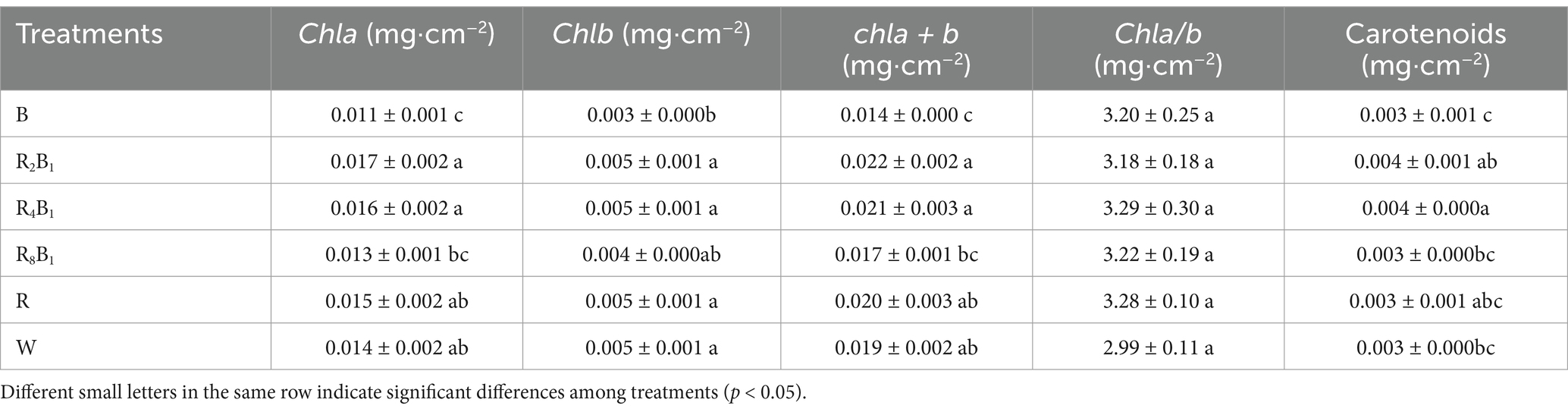
Table 6. Photosynthetic pigments, including chlorophyll a (Chl a), chlorophyll b (Chl b), and carotenoids of Green Pakchoi (Jingguan No. 1), assessed on day 20 after treatment application under varying red-blue light ratios in a controlled environment.
Compared to the response of green pakchoi, a different trend was observed in red pakchoi for the Chl a and Chl a + b content. Specifically, under treatment R2B1, Chl a and Chl a + b content in red pakchoi leaves decreased by 29.4 and 34.8%, respectively, compared to treatment W (Table 7). Unlike green pakchoi, the concentrations of Chl a, Chl b, Chl a + b, and carotenoids in red pakchoi were highest under treatment W. There were no significant differences in Chl b, Chl a/b, and carotenoid content under different red-to-blue ratios.
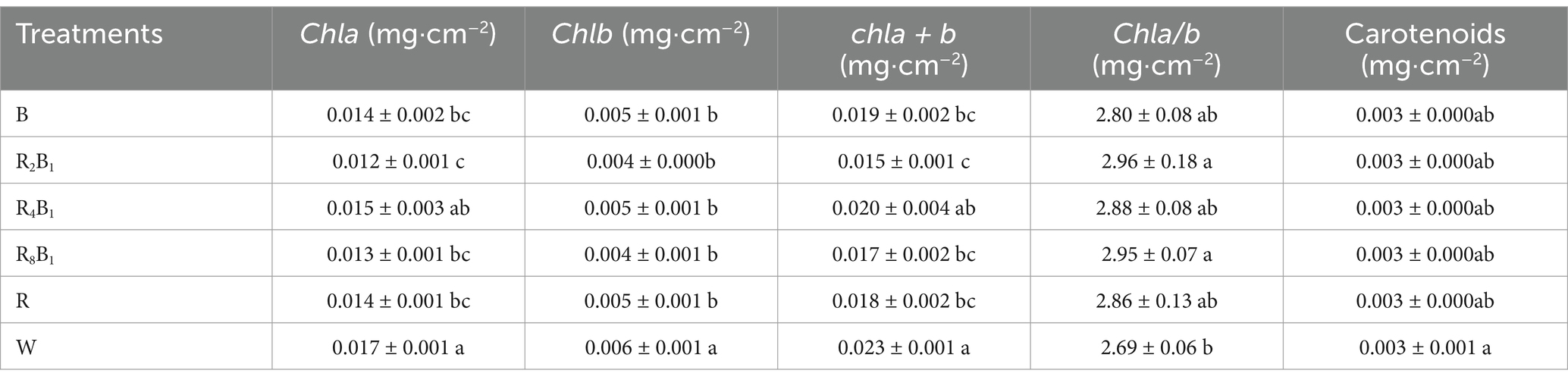
Table 7. Photosynthetic pigments, including chlorophyll a (Chl a), chlorophyll b (Chl b), and carotenoids of Red Pakchoi (Ziguan No. 1), assessed on day 20 after treatment application under varying red-blue light ratios in a controlled environment.
3.3 Nutritional quality
3.3.1 GSs concentration of ‘Jingguan no. 1’
As shown in Table 8, a total of 9 glucosinolate (GS) types were detected in green pakchoi, including 5 aliphatic GSs: progoitrin (PRO), sinigrin (SIN), glucoraphanin (RAA), gluconapin (NAP), and glucobrassicanapin (GBN); and 4 indole GSs: 4-hydroxyglucobrassicin (4OH), glucobrassicin (GBC), 4-methoxyglucobrassicin (4ME), and neoglucobrassicin (NEO). Different GS types exhibited varying responses to different red-to-blue ratios. The concentrations of PRO, SIN, and NEO under treatment B were the highest, being 2.0, 2.3, and 1.4 times higher than those under the control treatment, respectively. Treatment R2B1 increased indole GS concentrations while treatment R significantly promoted RAA concentration, which was 4.95 times higher than that under the control treatment. However, the control treatment was more conducive to accumulating NAP and GBN.
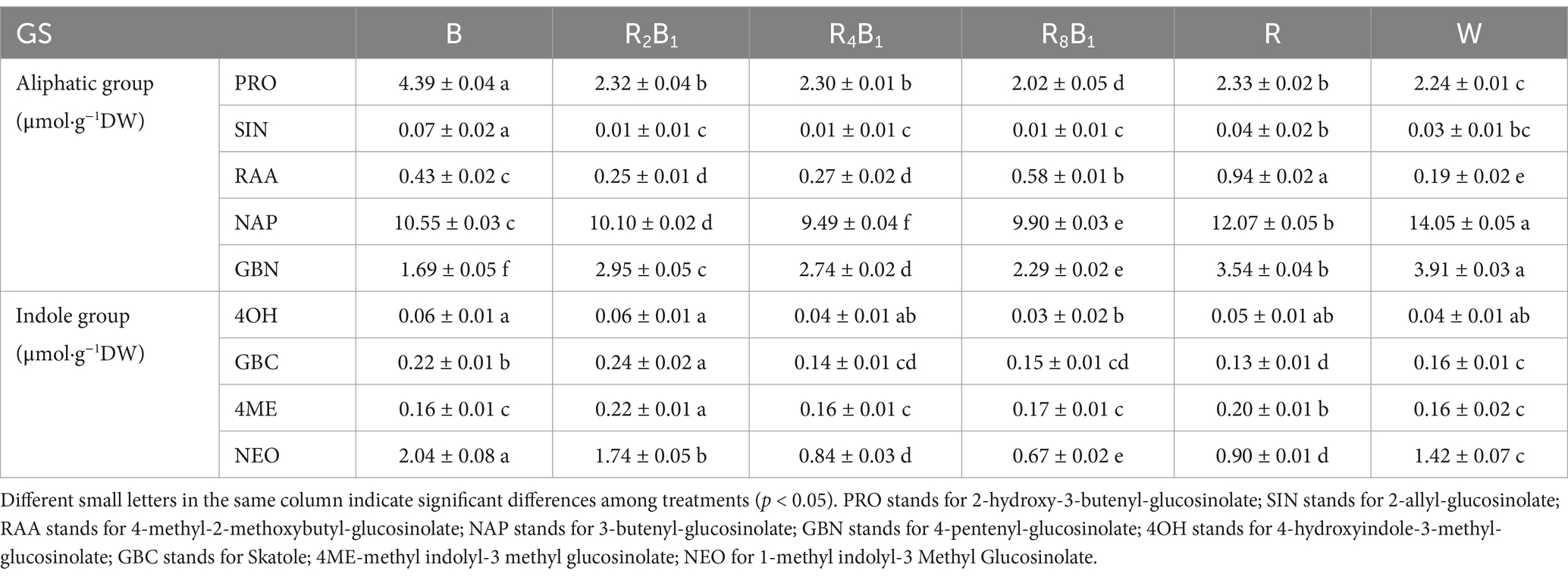
Table 8. GS concentration of the aliphatic group (μmol·g−1 DW) and indole group (μmol·g−1 DW) in Green Pakchoi (Jingguan No. 1) assessed on day 20 after treatment application under varying red-blue light ratios in a controlled environment.
Furthermore, Figure 2 shows the concentrations of total glucosinolates (GSs), the aliphatic GS group, and the indole GS group as affected by different red-to-blue ratios. The concentrations of total GSs, the aliphatic GS group, and the indole GS group initially decreased and then increased with the reduction in blue light proportion. The concentration of aliphatic GSs was significantly higher than that of indole GSs. The concentrations of total GSs and aliphatic GSs were highest under treatment W, measuring 22.19 μmol·g−1 and 20.41 μmol·g−1, respectively. There was no significant difference in total GSs and aliphatic GSs between treatments R4B1 and R8B1. However, both blue light and red light alone promoted the concentrations of total GSs and aliphatic GSs. Furthermore, indole GS concentrations under treatments B and R2B1 increased by 39.3 and 27.0%, respectively, while those under treatments R4B1, R8B1, and R decreased by 33.2, 42.7, and 28.1%, respectively, when compared with the control treatment.
3.3.2 GSs concentration of ‘Ziguan no. 1’
Similar with ‘Jingguan No. 1’, nine glucosinolate (GS) types were also detected in red pakchoi, including five aliphatic GSs and four indole GSs (Table 9). Except for sinigrin (SIN) and the aliphatic glucosinolate RAA, the concentrations of the other GS types generally increased with the increasing red-to-blue light ratio. Treatment W was more conducive to the accumulation of GSs in red pakchoi and had the highest concentrations, except for RAA. Treatment B significantly increased the concentration of RAA, which rose by 290.0% compared to that under treatment W. However, no significant differences in the concentrations of SIN under different red-to-blue ratios were observed.
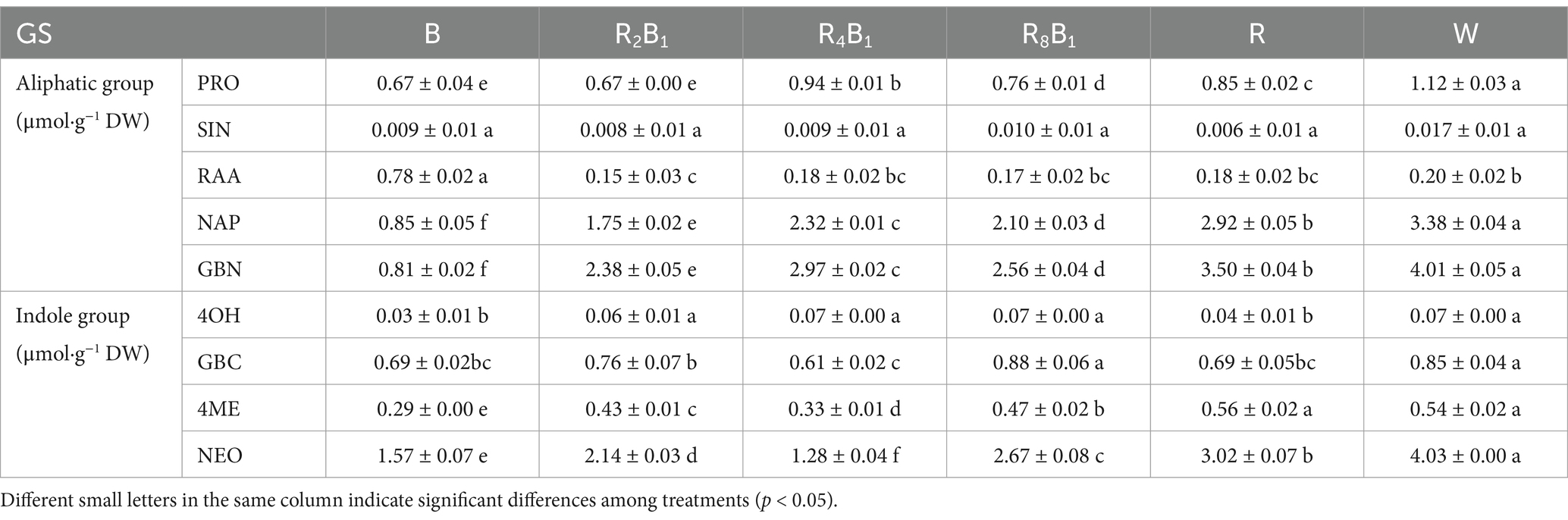
Table 9. GS concentration of the aliphatic group (μmol·g−1 DW) and indole group (μmol·g−1 DW) in Red Pakchoi (Ziguan No. 1) assessed on day 20 after treatment application under varying red-blue light ratios in a controlled environment.
As shown in Figure 3, the concentrations of total glucosinolates (GSs), aliphatic GSs, and indole GSs in red pakchoi generally increased with the rise in the red-light ratio. The highest concentrations were observed in the control group, measuring 14.21 μmol·g−1, 8.71 μmol·g−1, and 5.50 μmol·g−1, respectively, followed by those under treatment R. This indicates that red and white light were conducive to the accumulation of GSs in red pakchoi.
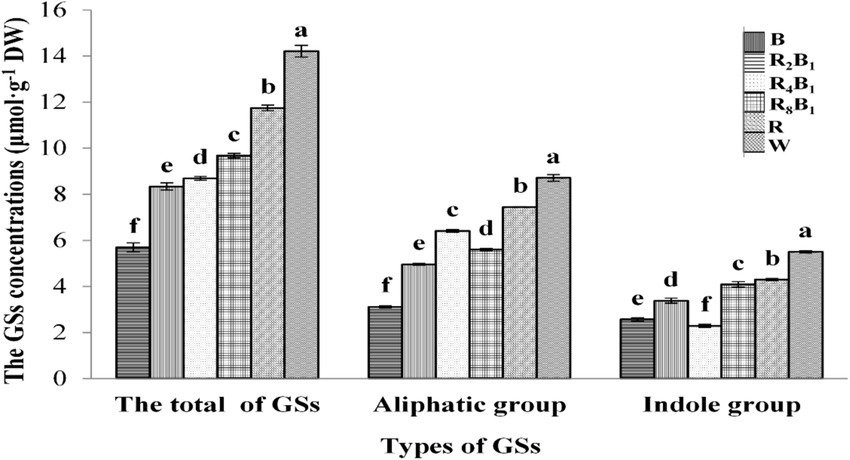
Figure 3. The concentrations (μmol·g−1 DW) of total glucosinolates (GSs), the aliphatic GS group, and the indole GS group in Red Pakchoi (Ziguan No. 1) assessed on day 20 after treatment application under varying red-blue light ratios in a controlled environment. Different lowercase letters indicate significant differences at the 0.05 level between treatments same as below.
3.3.3 Anthocyanin concentrations of ‘Ziguan no. 1’
Figure 4 demonstrates that different red-blue light ratios significantly influenced the anthocyanin concentration in red pakchoi. At higher blue light ratios, anthocyanin levels increased; however, these levels decreased as the blue light ratio was reduced. Despite this decline, the concentration remained significantly higher than that of the control treatment, reaching a maximum of 735.39 mg·kg−1 under treatment R2B1. The increases in anthocyanin concentrations under treatments B, R2B1, R4B1, R8B1, and R were 92.3, 114.4, 63.0, 77.5, and 39.4%, respectively, when compared to the control treatment. In contrast, for green pakchoi, anthocyanin levels were either negligible or undetectable under the experimental conditions. As a result, we did not include anthocyanin data. The absence of significant anthocyanin accumulation in green pakchoi, irrespective of the light treatments, contrasts with the notable anthocyanin responses observed in red pakchoi.
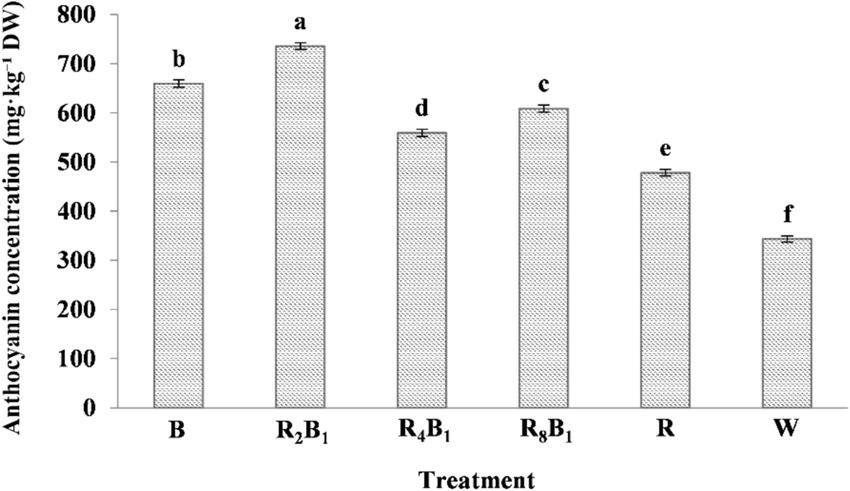
Figure 4. The anthocyanin concentrations (μmol·g−1 DW) of Red pakchoi (Ziguan No. 1) assessed on day 20 after treatment application under varying red-blue light ratios in a controlled environment. Different lowercase letters indicate significant differences at the 0.05 level between treatments same as below.
3.3.4 Soluble sugar concentration
A similar variation trend in soluble sugar concentration was observed in both green and red pakchoi during the experiment (Figure 5). The concentration increased at lower red-light ratios but decreased as the red-light ratio continued to rise. The soluble sugar concentration in green pakchoi was highest under treatment R4B1, which showed an increase of 31.4% compared to treatment W. There was no significant difference between treatments R and W. However, the soluble sugar concentrations under treatments B and R2B1 decreased by 57.3 and 13.1%, respectively, compared to treatment W. These results indicate that pure blue light and red-blue combination light with a low red-light proportion are not conducive to the accumulation of soluble sugars in green pakchoi. In contrast, for red pakchoi, the soluble sugar concentration decreased as the red-light proportion increased, with treatment W showing the lowest concentration. The soluble sugar concentration under treatment R2B1 was 2.78 times higher than that under the control treatment. Additionally, the soluble sugar concentrations under treatments B, R4B1, R8B1, and R increased by 146.1, 101.7, 85.0, and 28.3%, respectively, compared to those under the control treatment.
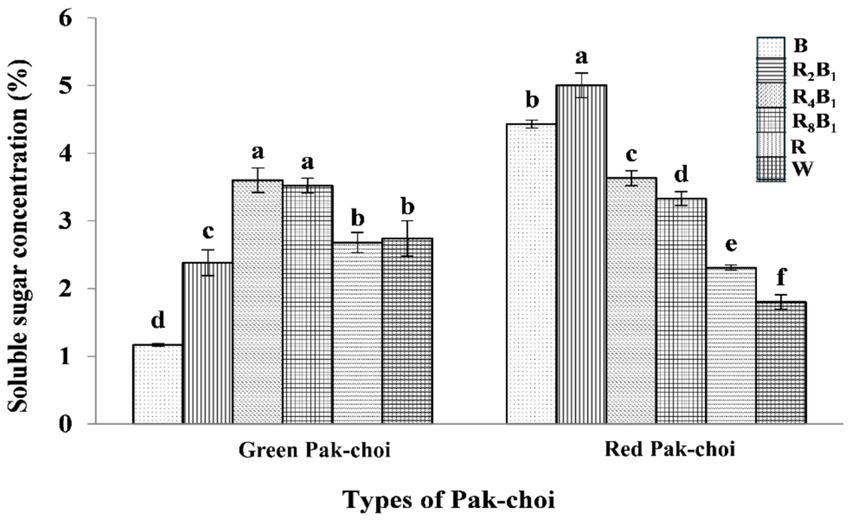
Figure 5. The soluble sugar concentration of both Green and Red pakchoi assessed on day 20 after treatment application under varying red-blue light ratios in a controlled environment. Different lowercase letters indicate significant differences at the 0.05 level between treatments.
3.3.5 Vitamin C (Vc) concentration
As shown in Figure 6, the concentration of Vc in green pakchoi increased at lower ratios of red light but began to decrease as the ratio of red light increased. The maximum concentration of Vc reached 65.91 mg·100 g−1 under treatment R4B1. The Vc level under treatment R8B1 was 34.3% higher than that under treatment W. Conversely, treatments B and R resulted in decreases of 28.6 and 16.1%, respectively, compared to treatment W. These results indicate that pure red and blue lights are not effective for accumulating Vc in green pak-choi, while incorporating red light into blue light significantly improves Vc levels.
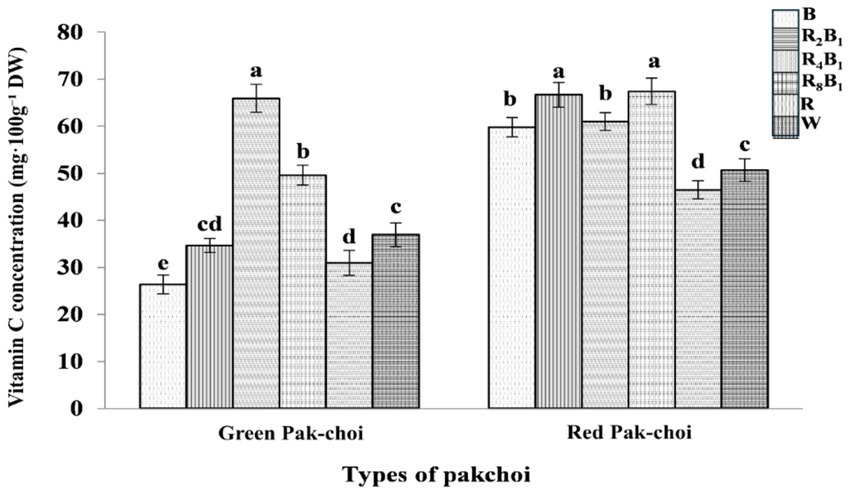
Figure 6. The vitamin C (Vc) concentration of Green and Red pakchoi assessed on day 20 after treatment application under varying red-blue light ratios in a controlled environment. Different lowercase letters indicate significant differences at the 0.05 level between treatments.
For red pak-choi, the Vc concentrations under treatments B, R2B1, R4B1, and R8B1 were 18.0, 31.6, 20.3, and 33.0% higher than that under treatment W. Unlike in green pak-choi, the Vc concentrations under treatments B and R2B1 also promoted the accumulation of Vc in red pakchoi.
3.3.6 Crude protein concentration
The crude protein concentration was significantly higher in red pakchoi than in green pakchoi (Figure 7). In green pakchoi, crude protein concentration initially increased under all treatments but later decreased as the blue light ratio declined. The highest concentration was observed under treatment R2B1, which showed a 65.0% increase compared to treatment W. In contrast, the crude protein concentration in red pakchoi decreased at first but increased again as the blue light ratio declined. It reached its peak under treatment B, which was 2.1 times higher than the control treatment.
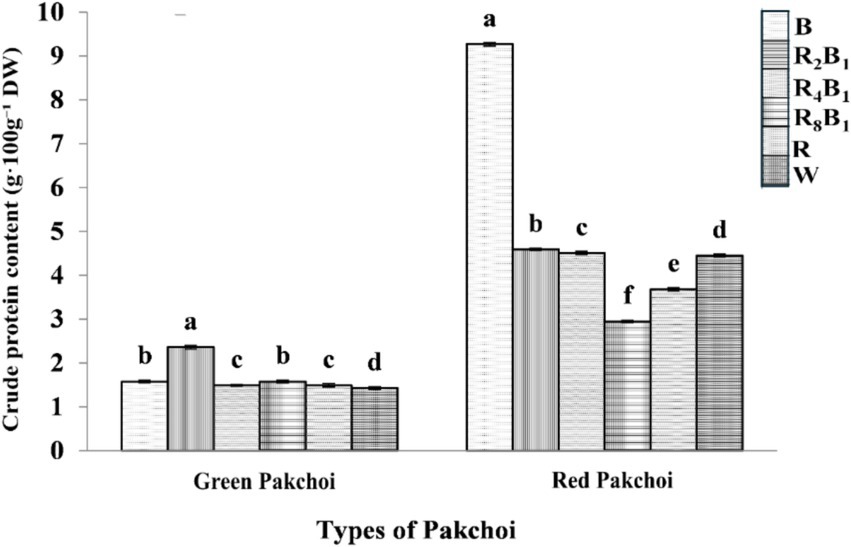
Figure 7. The crude protein concentration of Green and Red pakchoi assessed on day 20 after treatment application under varying red-blue light ratios in a controlled environment. Different lowercase letters indicate significant differences at the 0.05 level between treatments same as below.
Unlike green pakchoi, the crude protein concentration in red pakchoi significantly increased under treatment B. This suggests that red pakchoi may be more responsive to blue light than green pakchoi. Compared to treatment W, the crude protein concentrations under treatments R8B1 and R decreased by 33.7 and 17.3%, respectively. These findings indicate that the accumulation of crude protein in red pakchoi is significantly inhibited under treatments featuring pure red light and/or a high red-to-blue light ratio.
4 Discussion
4.1 Photosynthetic characteristics and growth of pakchoi plants as affected by different red-blue ratios
Light is an indispensable factor in the process of plant growth, and it is also the source of energy for plant photosynthesis (Trivellini et al., 2023). Plants show significant improvements in height, root length, leaf area, and fresh weight when treated with a combination of red and blue light (Luo et al., 2024). Research indicates that red light promotes stem elongation, while blue light helps regulate this growth, leading to optimal plant height (Chan et al., 2024). Our results align with previous studies on cucumber seedlings, which demonstrate that the combination of red and blue light spectrums enhances root development, resulting in improved root length and overall root system health, both of which are crucial for nutrient uptake (Wang T. et al., 2024). Additionally, both red and blue light contribute to increased leaf area; red light encourages leaf expansion while blue light enhances chlorophyll production, resulting in larger and healthier leaves that can capture more sunlight for photosynthesis (Wang et al., 2022). The synergistic effect of red and blue light treatments also leads to greater biomass accumulation in pakchoi, as previous studies have shown that plants grown under these conditions exhibit higher fresh weight due to enhanced photosynthetic activity and improved nutrient absorption (Trivellini et al., 2023). A combination of red and blue lights has been reported to be effective in driving photosynthesis (Wang et al., 2016; Liu and Van Iersel, 2021). In our study, both Pn and Chla were promoted under the combination treatments of red and blue lights, especially in red pakchoi plants (Tables 4–7). Chlorophyll a is the primary pigment responsible for absorbing light energy in photosynthesis. It plays a central role in the light-dependent reactions by capturing light in the blue and red parts of the visible spectrum, with a peak absorption at approximately 430 nm (blue light) and 665 nm (red light) (Chu et al., 2023). Our study found that the different ratios of red-to-blue light significantly affected the photosynthetic pigments in plants, with Chla being particularly sensitive to such changes (Tables 6, 7). In addition, blue light under the background of red light was a benefit for stomatal opening and transpiration rate (Shimazaki et al., 2007; Wang et al., 2016). Furthermore, chlorophyll plays a crucial role in regulating plant development by influencing physiological processes such as stomatal opening and gas exchange, which are essential for maintaining optimal photosynthetic conditions (Harrison et al., 2020). Moreover, the ability of plants to adjust their chlorophyll content in response to environmental factors like light quality and availability highlights its significance in growth and development (Driesen et al., 2020). In our study, Gs, Ci, and Tr of both green and red pakchoi plants were observed under treatment R2B1. These results, align with previous studies, which have shown that different ratios of red to blue light can significantly impact growth parameters such as leaf area, fresh weight, and photosynthetic efficiency. For example, a red-to-blue ratio of R8:B2 has been found to enhance lettuce growth and quality compared to other ratios (Li et al., 2020).
The relative quantum efficiency of red light for photosynthesis is higher than that of blue light, as indicated by previous research (Liu and Van Iersel, 2021). Our study confirms the superiority of red light in promoting photosynthesis, as demonstrated by the highest net photosynthetic rate (Pn) observed in green pakchoi in treatment R8B1. Distinct trends were observed for the net photosynthetic rate (Pn) of plants under different light qualities in the tested cultivars, with the Pn of red pakchoi plants being lower than that of green pakchoi plants. This phenomenon is consistent with the findings of Dou et al. (2019) who observed a lower photosynthetic effective radiation quantum efficiency in red basil compared to green basil, resulting in differences between the two varieties. The observed differences in Pn between green and red pakchoi plants in this study may also be attributed to differences in the photosynthetic pigments and their associated light absorption spectra and differences in other physiological processes in the two plant varieties. Furthermore, red basil had higher anthocyanin content and more vital light absorption ability. Therefore, it reduced the absorption of light energy by chloroplast, which reduced the photochemical energy transferred to the reaction centers (Anum et al., 2024b).
This experiment showed inconsistent Pn and growth responses in the green and red pakchoi plants (Tables 2, 5). The stem diameter, Chla, Chl a + b, stomatal conductance, intercellular carbon dioxide concentration and transpiration rate of pakchoi plants under treatment R2B1 were significantly increased, while the plant height, root length and photosynthetic pigment under treatment R4B1 were promoted. For red pakchoi plants, the R2B1 treatment significantly increased stem diameter and Chla/b compared to the green pakchoi, while the net photosynthetic rate, stomatal conductance, intercellular carbon dioxide concentration, and transpiration rate also increased, but to a lesser extent. It is important to note that leaf area can affect yield, and treatment R increased the leaf area of both cultivars of pakchoi, thereby contributing to the increases in fresh and dry weights of the plants. However, the chlorophyll, carotenoid content, and net photosynthetic rate of red pakchoi were highest when treated with W light, aligning with the findings of Zhou et al. (2020). Their study on pakchoi reported that white light with low light intensity significantly enhanced chlorophyll content. This suggests that the response to light quality varies between different leafy vegetables. Other studies have also investigated the effects of light quality on pakchoi and found that white light increased both chlorophyll content and the photosynthetic rate. These studies emphasized that light intensity, when combined with the appropriate light spectrum, plays a crucial role in enhancing the growth and physiological responses of leafy vegetables like pakchoi (Zhu et al., 2017; Zheng, 2018; Frede et al., 2019).
4.2 Nutritional quality of pakchoi
Vc (ascorbic acid), soluble sugar, crude protein, anthocyanin, and glucosinolates (GSs), as plant metabolites, have protective effects on vegetables and are essential components of plant nutritional quality (Sharma et al., 2024). In this experiment, Vc and soluble sugar content in green pakchoi were highest under the R4B1 treatment, while the R2B1 treatment significantly increased the crude protein content. Previous studies, such as those by Trivellini et al. (2023) and Lingwan et al. (2023) demonstrated that using a combined light source not only increases production efficiency but also enhances the nutritional composition of plants by promoting the synthesis of essential metabolites. This suggests that optimizing light quality can improve both the yield and nutritional value of leafy vegetables. Comparable outcomes were observed in red pakchoi, where treatment with R8B1 led to an increase in Vc content. However, the difference in Vc content between R8B1 and R2B1 was not statistically significant. Previous studies have shown that blue light is the most effective in regulating anthocyanin biosynthesis among various wavelengths in tomatoes (Li et al., 2021; He et al., 2022). Furthermore, blue light promotes the expression of genes that regulate the anthocyanin pathway (Anum et al., 2024b). In the present study, R2B1 treatment significantly increased the concentration of soluble sugar and anthocyanins, indicating that a higher proportion of blue light can promote the accumulation of anthocyanins in red pakchoi, which is also directly related to the increase of sugar concentration. Solfanelli et al. (2006) pointed out that the expression of most structural and regulatory genes of anthocyanins is regulated by sugar; at the same time, treatment B was also beneficial to the accumulation of crude protein concentration. Previous studies have reported that blue light, as well as a suitable combination of red and blue lights, can enhance the concentration of crude protein, soluble sugar, and vitamin C, while reducing nitrate concentration in lettuce and cucumber plants (Wendong et al., 2015; Xiaoxue et al., 2015). The variations in the light environment in each study could also have played a role, as plants exhibit different adaptabilities to varying light conditions. Thus, to establish optimal light conditions for improving the nutritional quality of crops, it is essential to consider the specific plant species and growing conditions (Ouzounis et al., 2015).
4.3 GSs concentration in pakchoi
Plant GSs concentration is significantly affected by light environment. Previous researches have indicated that plants covered with blue plastic film had higher GSs concentration than those covered with other colored plastic films (Antonious et al., 1996). Moreover, ultraviolet irradiation has increased broccoli’s total and aliphatic GSs concentration (Moreira-Rodríguez et al., 2017).
SIN, RAA and GBC are considered beneficial GSs, while PRO is considered harmful. Excessive intake of PRO by a human will lead to goiter (Padilla et al., 2007; Verkerk et al., 2009). In this experiment, PRO, SIN, NEO and 4OH of green pakchoi were the highest under treatment B. However, the treatment B significantly reduced PRO concentration and increased RAA concentration in red pakchoi, which agrees with the findings of (Zheng, 2018; Park et al., 2019) demonstrating that blue light treatment positively influenced the GSs concentration and phenolic accumulation in pakchoi shoots. Similarly, (Zheng, 2018) found that a higher blue light supplement intensity significantly enhanced the GSs concentration in green and red leaf pakchoi.
In our study, treatment B significantly decreased the concentrations of total GSs and aliphatic GSs in red pakchoi. This result contradicts the findings of (Qian et al., 2016), who found that blue light increased GSs concentrations in Chinese kale. Furthermore, in our study, the concentrations of total GSs and aliphatic GSs in green pakchoi, as well as the concentrations of total, aliphatic, and indole GSs in red pakchoi, were highest under treatment W. Metallo et al. (2018) conducted a study comparing the effects of white light and a combination of 5% blue light and 95% red light on the GSs concentration of cabbage. They found that the red and blue light combination increased the total, indole, and aliphatic GSs concentration in cabbage. However, our study obtained different results, which may be attributed to variations in cultivars, cultivation techniques, and the duration of the light treatments. It should be noted that the growth, development, and nutritional quality of plants are influenced not only by light quality but also light intensity and duration, as evidenced by changes in photosynthetic pigments, phenolic compounds, Vc, soluble sugar, and GSs concentration (Steindal et al., 2013; Brazaitytė et al., 2015).
5 Conclusion
To improve the nutritional quality and growth of pakchoi plants, the optimum red-to-blue light ratios were investigated by using Green pakchoi of “Jingguan No.1” and red pakchoi of “Ziguan No.1” in this experiment. This study demonstrates that different light spectral qualities significantly impact the growth, photosynthesis and nutritional quality of pakchoi. Treatment R was the best for the growth of green and red pakchoi, resulting in a significant increase in leaf area and dry and fresh weights. Regarding photosynthetic characteristics, treatment R4B1 showed beneficial effects on the accumulation of photosynthetic pigments of both pakchoi varieties. Additionally, R2B1 treatment increased the Pn, Gs, Ci, and Tr of both pakchoi varieties compared with those under the control. Finally, regarding nutritional quality, treatment R4B1 showed a significant increase in the concentrations of Vc and soluble sugar of green pakchoi plants. In contrast, treatment R2B1 resulted in a higher accumulation of Vc, soluble sugar, and anthocyanin concentrations in red pakchoi. Notably, both pakchoi varieties had the highest glucosinolate concentration under control. Therefore, the selection of suitable light quality according to the specific cultivar is crucial for the successful cultivation of pakchoi plants.
Data availability statement
The raw data supporting the conclusions of this article will be made available by the authors, without undue reservation.
Author contributions
HA: Data curation, Investigation, Writing – original draft, Writing – review & editing. YW: Conceptualization, Investigation, Writing – original draft, Writing – review & editing. YL: Conceptualization, Data curation, Resources, Validation, Writing – original draft. GS: Data curation, Formal analysis, Writing – review & editing. JL: Formal analysis, Writing – review & editing. NG: Conceptualization, Data curation, Investigation, Writing – review & editing. GL: Funding acquisition, Supervision, Writing – review & editing. YT: Writing – original draft, Writing – review & editing.
Funding
The author(s) declare that financial support was received for the research and/or publication of this article. This research was funded by the Innovation and Capacity Building Project of Beijing Academy of Agriculture and Forestry Sciences (KJCX20230309, KJCX20230212, KJCX20240405) and Beijing Innovation Consortium of Agriculture Research System (BAIC01-2024-22).
Conflict of interest
The authors declare that the research was conducted in the absence of any commercial or financial relationships that could be construed as a potential conflict of interest.
Generative AI statement
The author(s) declare that no Gen AI was used in the creation of this manuscript.
Publisher’s note
All claims expressed in this article are solely those of the authors and do not necessarily represent those of their affiliated organizations, or those of the publisher, the editors and the reviewers. Any product that may be evaluated in this article, or claim that may be made by its manufacturer, is not guaranteed or endorsed by the publisher.
References
Antonious, G. F., Kasperbauer, M. J., and Byers, M. E. (1996). Light reflected from colored mulches to growing turnip leaves affects glucosinolate and sugar contents of edible roots. Photochem. Photobiol. 64, 605–610. doi: 10.1111/j.1751-1097.1996.tb03112.x
Anum, H., Cheng, R.-F., and Tong, Y.-X. (2024a). Improving plant growth, anthocyanin production and oxidative status of red lettuce (Lactuca sativa cv. Lolla Rossa) by optimizing red to blue light ratio with a constant green light fraction in a plant factory. Sci. Hortic. 338:113832. doi: 10.1016/j.scienta.2024.113832
Anum, H., Li, K., Tabusam, J., Saleh, S. A. A., Cheng, R.-F., and Tong, Y.-X. (2024b). Regulation of anthocyanin synthesis in red lettuce in plant factory conditions: A review. Food Chem. 458:140111. doi: 10.1016/j.foodchem.2024.140111
AOAC (2000). “Coffee and tea” in Official methods of analysis. 17th edition ed (Gaithersburg, MD: Association of Official Analytical Chemists).
Bian, Z., Cheng, R., Wang, Y., Yang, Q., and Lu, C. (2018). Effect of green light on nitrate reduction and edible quality of hydroponically grown lettuce (Lactuca sativa L.) under short-term continuous light from red and blue light-emitting diodes. Environ. Exp. Bot. 153, 63–71. doi: 10.1016/j.envexpbot.2018.05.010
Brazaitytė, A., Sakalauskienė, S., Samuolienė, G., Jankauskienė, J., Viršilė, A., Novičkovas, A., et al. (2015). The effects of LED illumination spectra and intensity on carotenoid content in Brassicaceae microgreens. Food Chem. 173, 600–606. doi: 10.1016/j.foodchem.2014.10.077
Cammarisano, L., and Körner, O. (2022). Response of cyanic and Acyanic lettuce cultivars to an increased proportion of blue light. Biology 11:959. doi: 10.3390/biology11070959
Cervantes, L., Ariza, M., Gómez-Mora, J., Miranda, L., Medina, J., Soria, C., et al. (2019). Light exposure affects fruit quality in different strawberry cultivars under field conditions. Sci. Hortic. 252, 291–297. doi: 10.1016/j.scienta.2019.03.058
Chan, A. M., Pay, M. L., Christensen, J., He, F., Roden, L. C., Ahmed, H., et al. (2024). Red, blue or mix: choice of optimal light qualities for enhanced plant growth and development through in silico analysis. in silico Plants 6:8. doi: 10.1093/insilicoplants/diae008
Chen, Q., Liu, S., Zhang, Z., Cui, H., Hao, S., and Liu, Z. (2009). Effect of different light emitting diode sources on tomato fruit quality during color-changed period. Trans. Chin. Soc. Agric. Eng. 25, 156–161.
Choi, J.-H., and Choi, H.-Y. (2023). Effect of pretreatment conditions on quality characteristics and antioxidant activity in pak choi (Brassica rapa L. ssp. chinensis). Food Preserv. Sci. 30, 969–982. doi: 10.11002/kjfp.2023.30.6.969
Chu, Q., Qin, Y., Li, C., Cheng, S., Su, L., He, Z., et al. (2023). Effects of different photoperiods on the growth and nutritional characteristics of two celery cultivars in plant factory. Agronomy 13:3039. doi: 10.3390/agronomy13123039
Connolly, E. L., Sim, M., Travica, N., Marx, W., Beasy, G., Lynch, G. S., et al. (2021). Glucosinolates from cruciferous vegetables and their potential role in chronic disease: investigating the preclinical and clinical evidence. Front. Pharmacol. 12:767975. doi: 10.3389/fphar.2021.767975
Ding, X., Zhang, H., He, L., Jin, H., Zhou, Q., Yang, J., et al. (2022). Changes in leaf and growth of pak choi in a semi-closed greenhouse in eastern China. HortScience 57, 643–651. doi: 10.21273/HORTSCI16523-22
Dong, S., Fang, S., Li, J., Zheng, W., Wang, Z., Hu, J., et al. (2024). Comparative metabolic profiling of different pakchoi cultivars reveals nutritional diversity via widely targeted metabolomics. Food Chem. X 22:101379. doi: 10.1016/j.fochx.2024.101379
Dou, H., Niu, G., and Gu, M. (2019). Pre-harvest UV-B radiation and photosynthetic photon flux density interactively affect plant photosynthesis, growth, and secondary metabolites accumulation in basil (Ocimum basilicum) plants. Agronomy 9:434. doi: 10.3390/agronomy9080434
Driesen, E., Van den Ende, W., De Proft, M., and Saeys, W. (2020). Influence of environmental factors light, CO2, temperature, and relative humidity on stomatal opening and development: A review. Agronomy 10:1975. doi: 10.3390/agronomy10121975
Fan, X.-X., Xue, F., Song, B., Chen, L.-Z., Xu, G., and Xu, H. (2019). Effects of blue and red light on growth and nitrate metabolism in pakchoi. Open Chem. 17, 456–464. doi: 10.1515/chem-2019-0038
Fecka, I., Nowicka, A., Kucharska, A. Z., and Sokół-Łętowska, A. (2021). The effect of strawberry ripeness on the content of polyphenols, cinnamates, L-ascorbic and carboxylic acids. J. Food Compos. Anal. 95:103669. doi: 10.1016/j.jfca.2020.103669
Frede, K., Schreiner, M., and Baldermann, S. (2019). Light quality-induced changes of carotenoid composition in pak choi Brassica rapa ssp. chinensis. J. Photochem. Photobiol. B Biol. 193, 18–30. doi: 10.1016/j.jphotobiol.2019.02.001
Gao, W., He, D., Ji, F., Zhang, S., and Zheng, J. (2020). Effects of daily light integral and LED spectrum on growth and nutritional quality of hydroponic spinach. Agronomy 10:1082. doi: 10.3390/agronomy10081082
Gao, Y., Li, Q. M., Liu, B. B., Li, S. H., Ai, X. Z., Wei, M., et al. (2018). Effects of light quality ratio on photosynthetic characteristics and quality of red lettuce. Chin. J. Appl. Ecol. 29, 3649–3657. doi: 10.13287/j.1001-9332.201811.024
Guirrou, I., El Harrak, A., El Antari, A., Hssaini, L., Hanine, H., El Fechtali, M., et al. (2023). Bioactive compounds assessment in six Moroccan rapeseed (Brassica napus L.) varieties grown in two contrasting environments. Agronomy 13:460. doi: 10.3390/agronomy13020460
Harrison, E. L., Arce Cubas, L., Gray, J. E., and Hepworth, C. (2020). The influence of stomatal morphology and distribution on photosynthetic gas exchange. Plant J. 101, 768–779. doi: 10.1111/tpj.14560
He, R., Wei, J., Zhang, J., Tan, X., Li, Y., Gao, M., et al. (2022). Supplemental blue light frequencies improve ripening and nutritional qualities of tomato fruits. Front. Plant Sci. 13:888976. doi: 10.3389/fpls.2022.888976
Huang, J., Xu, Y.-I., Duan, F.-M., Du, X., Yang, Q.-C., and Zheng, Y.-J. (2021). Improvement of the growth and nutritional quality of two-leaf-color pak choi by supplemental alternating red and blue light. HortScience 56, 118–125. doi: 10.21273/HORTSCI15180-20
Ilić, Z. S., and Fallik, E. (2017). Light quality manipulation improves vegetable quality at harvest and postharvest: A review. Environ. Exp. Bot. 139, 79–90. doi: 10.1016/j.envexpbot.2017.04.006
Izzo, L. G., Mickens, M. A., Aronne, G., and Gómez, C. (2021). Spectral effects of blue and red light on growth, anatomy, and physiology of lettuce. Physiol. Plant. 172, 2191–2202. doi: 10.1111/ppl.13395
Kaiser, E., Ouzounis, T., Giday, H., Schipper, R., Heuvelink, E., and Marcelis, L. F. (2019). Adding blue to red supplemental light increases biomass and yield of greenhouse-grown tomatoes, but only to an optimum. Front. Plant Sci. 9:2002. doi: 10.3389/fpls.2018.02002
Kaleri, A. R., Ma, J., Jakhar, A. M., Hakeem, A., Ahmed, A., Napar, W. P. F., et al. (2020). Effects of dung beetle-amended soil on growth, physiology, and metabolite contents of bok choy and improvement in soil conditions. J. Soil Sci. Plant Nutr. 20, 2671–2683. doi: 10.1007/s42729-020-00333-8
Kim, S.-H., Ochar, K., Hwang, A., Lee, Y.-J., and Kang, H. J. (2023). Variability of glucosinolates in Pak choy (Brassica rapa subsp. chinensis) germplasm. Plan. Theory 13:9. doi: 10.3390/plants13010009
Lee, J., Durst, R. W., Wrolstad, R. E., Kupina, C., et al. (2005). Determination of total monomeric anthocyanin pigment content of fruit juices, beverages, natural colorants, and wines by the pH differential method: collaborative study. J. AOAC Int. 88, 1269–1278. doi: 10.1093/jaoac/88.5.1269
Li, Y., Liu, C., Shi, Q., Yang, F., and Wei, M. (2021). Mixed red and blue light promotes ripening and improves quality of tomato fruit by influencing melatonin content. Environ. Exp. Bot. 185:104407. doi: 10.1016/j.envexpbot.2021.104407
Li, H., Sun, Q., Zhao, S., and Zhang, W. (2000). Principles and techniques of plant physiological biochemical experiment. Beijing: Higher Education Press, 195–197.
Li, H., Tang, C., and Xu, Z. (2013). The effects of different light qualities on rapeseed (Brassica napus L.) plantlet growth and morphogenesis in vitro. Sci. Hortic. 150, 117–124. doi: 10.1016/j.scienta.2012.10.009
Li, L., Tong, Y.-X., Lu, J.-I., Li, Y.-M., and Yang, Q.-C. (2020). Lettuce growth, nutritional quality, and energy use efficiency as affected by red–blue light combined with different monochromatic wavelengths. HortScience 55, 613–620.
Li, Y., Xin, G., Shi, Q., Yang, F., and Wei, M. (2023). Response of photomorphogenesis and photosynthetic properties of sweet pepper seedlings exposed to mixed red and blue light. Front. Plant Sci. 13:984051. doi: 10.3389/fpls.2022.984051
Lichtenthaler, H. K., and Wellburn, A. R. (1983). Determinations of total carotenoids and chlorophylls a and b of leaf extracts in different solvents. Biochem. Soc. Trans. 11, 591–592. doi: 10.1042/bst0110591
Lingwan, M., Pradhan, A. A., Kushwaha, A. K., Dar, M. A., Bhagavatula, L., and Datta, S. (2023). Photoprotective role of plant secondary metabolites: biosynthesis, photoregulation, and prospects of metabolic engineering for enhanced protection under excessive light. Environ. Exp. Bot. 209:105300. doi: 10.1016/j.envexpbot.2023.105300
Liping, H., Guangmin, L., Jungeng, K., Xuezhi, Z., Yue, M., and Hongju, H. (2015). Composition and content analysis of glucosinolates in different cabbage varieties. Chin. Veg. 6, 42–47.
Liu, J., and Van Iersel, M. W. (2021). Photosynthetic physiology of blue, green, and red light: light intensity effects and underlying mechanisms. Front. Plant Sci. 12:619987. doi: 10.3389/fpls.2021.619987
Liu, J., and van Iersel, M. W. (2022). Far-red light effects on lettuce growth and morphology in indoor production are cultivar specific. Plants 11:2714. doi: 10.3390/plants11202714
Luo, S., Zou, J., Shi, M., Lin, S., Wang, D., Liu, W., et al. (2024). Effects of red-blue light spectrum on growth, yield, and photo-synthetic efficiency of lettuce in a uniformly illumination environment. Plant Soil Environ. 70, 305–316. doi: 10.17221/480/2023-PSE
Maina, S., Misinzo, G., Bakari, G., and Kim, H.-Y. (2020). Human, animal and plant health benefits of glucosinolates and strategies for enhanced bioactivity: A systematic review. Molecules 25:3682. doi: 10.3390/molecules25163682
Mao, P., Duan, F., Zheng, Y., and Yang, Q. (2021). Blue and UV-A light wavelengths positively affected accumulation profiles of healthy compounds in pak-choi. J. Sci. Food Agric. 101, 1676–1684. doi: 10.1002/jsfa.10788
Metallo, R. M., Kopsell, D. A., Sams, C. E., and Bumgarner, N. R. (2018). Influence of blue/red vs. white LED light treatments on biomass, shoot morphology, and quality parameters of hydroponically grown kale. Sci. Hortic. 235, 189–197. doi: 10.1016/j.scienta.2018.02.061
Moreira-Rodríguez, M., Nair, V., Benavides, J., Cisneros-Zevallos, L., and Jacobo-Velázquez, D. A. (2017). UVA, UVB light doses and harvesting time differentially tailor Glucosinolate and phenolic profiles in broccoli sprouts. Molecules 22:1065. doi: 10.3390/molecules22071065
Naznin, M. T., Lefsrud, M., Gravel, V., and Azad, M. O. K. (2019). Blue light added with red LEDs enhance growth characteristics, pigments content, and antioxidant capacity in lettuce, spinach, kale, basil, and sweet pepper in a controlled environment. Plan. Theory 8:93. doi: 10.3390/plants8040093
Nieto, J. A., Hellín, P., Pérez, B., Viadel, B., Alapont, A., and Agudelo, A. (2023). Fresh Brassicaceae sprouting broccoli (Bimi®) glucosinolates profile characterization and bioaccessibility through an in vitro dynamic digestion study. J. Food Compos. Anal. 115:104941. doi: 10.1016/j.jfca.2022.104941
Olle, M., and Viršile, A. (2013). The effects of light-emitting diode lighting on greenhouse plant growth and quality. Agric. Food Sci. 22, 223–234. doi: 10.23986/afsci.7897
Ouzounis, T., Rosenqvist, E., and Ottosen, C.-O. (2015). Spectral effects of artificial light on plant physiology and secondary metabolism: A review. HortScience 50, 1128–1135. doi: 10.21273/HORTSCI.50.8.1128
Padilla, G., Cartea, M. E., Velasco, P., de Haro, A., and Ordás, A. (2007). Variation of glucosinolates in vegetable crops of Brassica rapa. Phytochemistry 68, 536–545. doi: 10.1016/j.phytochem.2006.11.017
Park, C. H., Kim, N. S., Park, J. S., Lee, S. Y., Lee, J.-W., and Park, S. U. (2019). Effects of light-emitting diodes on the accumulation of Glucosinolates and phenolic compounds in sprouting canola (Brassica napus L.). Food Secur. 8:76. doi: 10.3390/foods8020076
Podsędek, A. (2007). Natural antioxidants and antioxidant capacity of Brassica vegetables: A review. LWT 40, 1–11. doi: 10.1016/j.lwt.2005.07.023
Qian, H., Liu, T., Deng, M., Miao, H., Cai, C., Shen, W., et al. (2016). Effects of light quality on main health-promoting compounds and antioxidant capacity of Chinese kale sprouts. Food Chem. 196, 1232–1238. doi: 10.1016/j.foodchem.2015.10.055
Ramalho, J., Marques, N., Semedo, J., Matos, M., and Quartin, V. (2002). Photosynthetic performance and pigment composition of leaves from two tropical species is determined by light quality. Plant Biol. 4, 112–120. doi: 10.1055/s-2002-20443
Samuolienė, G., Brazaitytė, A., Jankauskienė, J., Viršilė, A., Sirtautas, R., Novičkovas, A., et al. (2013). LED irradiance level affects growth and nutritional quality of Brassica microgreens. Cent. Eur. J. Bio. 8, 1241–1249. doi: 10.2478/s11535-013-0246-1
Sharma, M., Bithel, N., Sharma, K. K., and Sharma, M. (2024). “Potential of vegetables and plant metabolites in healthcare” in Plant metabolites and vegetables as nutraceuticals. ed. M. Sharma (Florida: Apple Academic Press), 3–31.
Shimazaki, K.-I., Doi, M., Assmann, S. M., and Kinoshita, T. (2007). Light regulation of stomatal movement. Annu. Rev. Plant Biol. 58, 219–247. doi: 10.1146/annurev.arplant.57.032905.105434
Shuo, Z., Kehan, Z., Ziyi, C., Jingjin, Z., and Danfeng, H. (2019). Growth response of Ipomoea aquatica sprouts to LED light intensity and light quality. Chin. Veg. 6:7.
Solfanelli, C., Poggi, A., Loreti, E., Alpi, A., and Perata, P. (2006). Sucrose-specific induction of the anthocyanin biosynthetic pathway in Arabidopsis. Plant Physiol. 140, 637–646. doi: 10.1104/pp.105.072579
Steindal, A. L., Mølmann, J., Bengtsson, G. B., and Johansen, T. J. (2013). Influence of day length and temperature on the content of health-related compounds in broccoli (Brassica oleracea L. var. italica). J. Agric. Food Chem. 61, 10779–10786. doi: 10.1021/jf403466r
Trivellini, A., Toscano, S., Romano, D., and Ferrante, A. (2023). The role of blue and red light in the orchestration of secondary metabolites, nutrient transport and plant quality. Plan. Theory 12:2026. doi: 10.3390/plants12102026
Verkerk, R., Schreiner, M., Krumbein, A., Ciska, E., Holst, B., Rowland, I., et al. (2009). Glucosinolates in Brassica vegetables: the influence of the food supply chain on intake, bioavailability and human health. Mol. Nutr. Food Res. 53 Suppl 2:S219. doi: 10.1002/mnfr.200800065
Wang, Y., Li, L., Zhang, X., and Ji, M. (2024). Pesticide residues in greenhouse leafy vegetables in cold seasons and dietary exposure assessment for consumers in Liaoning Province, Northeast China. Agronomy 14:322. doi: 10.3390/agronomy14020322
Wang, S., Liu, X., Liu, X., Xue, J., Ren, X., Zhai, Y., et al. (2022). The red/blue light ratios from light-emitting diodes affect growth and flower quality of Hippeastrum hybridum ‘red lion’. Front. Plant Sci. 13:1048770. doi: 10.3389/fpls.2022.1048770
Wang, J., Lu, W., Tong, Y., and Yang, Q. (2016). Leaf morphology, photosynthetic performance, chlorophyll fluorescence, stomatal development of lettuce (Lactuca sativa L.) exposed to different ratios of red light to blue light. Front. Plant Sci. 7:250. doi: 10.3389/fpls.2016.00250
Wang, T., Sun, Q., Zheng, Y., Xu, Y., Liu, B., and Li, Q. (2024). Effects of red and blue light on the growth, photosynthesis, and subsequent growth under fluctuating light of cucumber seedlings. Plan. Theory 13:1668. doi: 10.3390/plants13121668
Wendong, X., Xiaoying, L., Xuelei, J., and Zhigang, X. (2015). LED light with different red and blue ratios regulates the growth of cucumber seedlings. Acta. Physiol. Sin. 51, 1273–1279.
Xiaoxue, F., Bo, S., Hai, X., Longzheng, C., Zhigang, X., and Xihan, Y. (2015). Effects of different LED light sources on lettuce growth and quality. J. Hubei Univ. Nat. 33, 330–333. doi: 10.13592/j.cnki.ppj.2015.0241
Yao, L., Xian, S., Fan, J., Sun, X., Geng, Z., and Du, C. (2018). The role of glucosinolates and study on anabolism in Brassica napus L. Biotechnology 28, 397–402.
Zhang, R., Cao, H., Sun, C., and Martin, J. J. J. (2021). Characterization of morphological and fruit quality traits of coconut (Cocos nucifera L.) germplasm. HortScience 56, 961–969. doi: 10.21273/HORTSCI15887-21
Zhang, Y., Ji, J., Song, S., Su, W., and Liu, H. (2020). Growth, nutritional quality and health-promoting compounds in Chinese kale grown under different ratios of red: blue LED lights. Agronomy 10:1248. doi: 10.3390/agronomy10091248
Zhang, G., Li, Z., Cheng, J., Cai, X., Cheng, F., Yang, Y., et al. (2022). Morphological and physiological traits of greenhouse-grown tomato seedlings as influenced by supplemental white plus red versus red plus blue LEDs. Agronomy 12:2450. doi: 10.3390/agronomy12102450
Zheng, Y. (2018). Study on the mechanism of light regulation of pakchoi quality and its light quality construction. Guangzhou, China: South China Agricultural University.
Zhou, F., Zuo, J., Xu, D., Gao, L., Wang, Q., and Jiang, A. (2020). Low intensity white light-emitting diodes (LED) application to delay senescence and maintain quality of postharvest pakchoi (Brassica campestris L. ssp. chinensis (L.) Makino var. communis Tsen et Lee). Sci. Hortic. 262:109060. doi: 10.1016/j.scienta.2019.109060
Keywords: glucosinolates, light quality, pakchoi, growth, nutritional quality, plant factory
Citation: Anum H, Wang Y, Li Y, Sun G, Luo J, Gruda NS, Liu G and Tong Y (2025) Physiological and nutritional responses of two pakchoi (Brassica chinensis) cultivars to different red-blue light ratios in controlled environment. Front. Sustain. Food Syst. 9:1561118. doi: 10.3389/fsufs.2025.1561118
Edited by:
Maria Inês Dias, Centro de Investigação de Montanha (CIMO), PortugalReviewed by:
Marta R. M. Lima, Virginia Tech, United StatesLaura Cammarisano, University of California, Davis, United States
Copyright © 2025 Anum, Wang, Li, Sun, Luo, Gruda, Liu and Tong. This is an open-access article distributed under the terms of the Creative Commons Attribution License (CC BY). The use, distribution or reproduction in other forums is permitted, provided the original author(s) and the copyright owner(s) are credited and that the original publication in this journal is cited, in accordance with accepted academic practice. No use, distribution or reproduction is permitted which does not comply with these terms.
*Correspondence: Guangmin Liu, TGl1Z3VhbmdtaW5AaWFwbi5vcmcuY24=; Yuxin Tong, dG9uZ3l1eGluQGNhYXMuY24=
†These authors have contributed equally to this work and share first authorship