- 1Laboratory of the Engineering and Applied Technologies, Higher School of Technology, Sultan Moulay Slimane University, Beni Mellal, Morocco
- 2Regional Center of Agricultural Research of Tadla, National Institute of Agricultural Research (INRA), Rabat, Morocco
- 3Laboratoires TBC, Laboratory of Pharmacology, Pharmacokinetics, and Clinical Pharmacy, Faculty of Pharmaceutical and Biological Sciences, Lille, France
- 4Department of Pharmacognosy, College of Pharmacy, King Saud University, Riyadh, Saudi Arabia
Introduction: Goat milk (GM) is highly valued for its rich nutritional content, easy digestibilityand low allergenic potential, making it an excellent alternative in the dairy industry.Quinoa, an Andean pseudo cereal, offers significant nutritional benefits, including high protein, fiber, and antioxidant properties. This study aims to assess the impact of adding quinoa flour (QF) on the fermentation, physicochemical and sensory properties of goat milk yogurt (GMY), addressing a research gap regarding the use of roasted quinoa flour (RoQF) in GMY production.
Methodologie: White and red quinoa flours, both roasted and unroasted, were incorporated at 0.5, 1, and 1.5% in the yogurt. The analysis focused on fermentation time, lactic acid production, pH, macronutrient composition, product stability, and sensory evaluation.
Results and discussion: The inclusion of 1% quinoa flour (QF) significantly increased the yogurt’s macronutrient content (p ≤ 0.05) compared to the control test, where protein content was 4.01 and fat content was 3.94 (g/100 g). Protein content increased by 0.1 to 0.17 (g/100 g), reaching 4.18; 4.15; 4.15 and 4.11 (g/100 g) for goat milk yogurt with white quinoa flour (GMYWQF), goat milk yogurt with red quinoa flour (GMYRQF), goat milk yogurt with roasted white quinoa flour (GMYRoWQF) and goat milk yogurt with roasted red quinoa flour (GMYRoRQF), respectively. Similarly, fat content increased by 0.03 to 0.09 (g/100 g) reaching 3.98, 4.03, 3.97, and 3.98 (g/100 g) for GMYWQF, GMYRQF, GMYRoWQF, and GMYRoRQF, respectively. These changes led to reduced fermentation time, minimizing it to 4 h, by promoting faster lactic acid production and lowering the pH more efficiently compared to the control test, indicating more efficient fermentation. Sensory analysis revealed that QF significantly improved the texture and flavor of the yogurt (p ≤ 0.05), with roasted quinoa flour (RoQF) further significantly enhancing consumer acceptability (p ≤ 0.05), by reducing the strong flavor of GM. Additionally, QF significantly improved yogurt stability, enhancing texture and shelf life (p ≤ 0.05). These findings emphasize the value of QF as an ingredient in GMY: significantly (p ≤ 0.05) increasing the protein content and improving texture, resulting in a more stable product with reduced Syneresis; Roasting the QF further significantly (p ≤ 0.05) enhances its sensory qualities, effectively decreasing the goat flavor, which is often less favored by some consumers, thus increasing overall acceptability. These advantages have important implications for refining dairy product formulations.
1 Introduction
Quinoa (Chenopodium quinoa) is an annual plant of the Amaranthaceae family, has been cultivated as a staple food for many years in the Andean region (Guardianelli et al., 2022). Known for its ability to thrive in a wide range of soil and climatic conditions, quinoa has earned its place as a “pseudo-cereal” due to its grain-like properties, despite being botanically unrelated to true cereals (Zhang et al., 2022). In recent years, quinoa has gained global recognition for its exceptional nutritional profile, making it a valuable addition to a wide variety of food products. It is rich in high-quality proteins, essential fatty acids, dietary fiber, vitamins, and minerals, as well as a remarkable balance of all nine essential amino acids (Navruz-Varli and Sanlier, 2016). In addition, quinoa itself is gluten-free, making it an ideal source of protein for those who suffer from celiac disease or follow a gluten-free diet (Zhang et al., 2024). Beyond its nutritional value, quinoa also boasts several functional properties that contribute to its growing utilization in the food industry (Adetunji et al., 2021). These include its high water retention capacity, gelation ability, and emulsifying properties, which have opened new avenues for its incorporation into value-added food products such as gluten-free baked goods, beverages, and dairy alternatives (Valcárcel-Yamani et al., 2012). Yoghurt is an important dairy product that is very popular all over the world (Pappa et al., 2024). It is rich in many nutrients, including various minerals, vitamins, fatty acids and high-quality protein (Paszczyk and Tońska, 2025). Unlike milk, yogurt offers additional benefits due to the fermentation process, which not only enhances digestibility but also provides probiotics that support gut health (Fernández-García et al., 2023). Over the years, there has been increasing interest in fortifying yogurt with functional ingredients to improve its nutritional profile and appeal to health-conscious consumers. Ingredients such as fruit powders, vegetable oils, antioxidants, dietary fibers, minerals, probiotics, and vitamins have been successfully incorporated into yogurt to boost its health benefits (Ahmad et al., 2022). Many different ingredients are added to yogurts, including popular fruits, fruit seed extracts, vegetables, nuts, muesli and spices, in order to attract consumer attention, reduce the sour taste and enhance the health-promoting effect (Cais-Sokolińska and Walkowiak-Tomczak, 2021). Among the various types of milk used in yogurt production, goat milk has garnered particular attention due to its unique nutritional properties, which some consumers find more appealing than those of cow’s milk (Nayik et al., 2022). Goat milk is lower in lactose, higher in certain minerals, and contains a different protein structure, which may offer potential health benefits, particularly for individuals with lactose sensitivity or digestive issues (El-Shafei et al., 2020). However, the use of goat milk in yogurt production presents certain challenges. Goat milk is known to have lower viscosity, increased sensitivity to Syneresis, and reduced gel strength compared to cow’s milk, which can affect the texture and overall quality of the yogurt (El-Shafei et al., 2020; Zine-eddine et al., 2021a,b).
The consumption of goat milk (GM) and goat milk products remains limited in many regions, despite their significant nutritional benefits (Ningtyas and Haskito, 2020). The use of GM in the human diet is less widespread, which could be attributed to its distinct organoleptic characteristics, including its strong odor, pronounced flavor, and slightly salty taste. The fatty acid composition of goat milk contains a higher proportion of medium-chain fatty acids, such as caproic (C6:0), caprylic (C8:0), and capric (C10:0), which are partly responsible for its characteristic goaty flavor. This distinctive flavor and aroma limit the market opportunities for this milk (Yang et al., 2023; Zine-eddine et al., 2021a,b). Furthermore, GM has lower viscosity, increased sensitivity to Syneresis, and reduced gel strength compared to cow’s milk, which can influence the texture and stability of yogurt (Costa et al., 2022).
In this context, the addition of QF to GMY holds great potential to counteract these specific challenges. Quinoa flour’s high water retention capacity, emulsifying properties, and gelation ability are expected to enhance the viscosity, reduce syneresis, and improve both the texture and overall sensory characteristics of GMY (Casarotti et al., 2014; Elslmawy et al., 2023). Furthermore, the incorporation of roasted quinoa flour (RoQF) may further boost sensory properties by enhancing flavor and reducing antinutritional compounds, contributing to better consumer acceptance of GMY.
Several studies have shown that incorporating QF into various food products improves their nutritional properties while offering health benefits (Abd Elmontaleb and Abbas, 2022; Balakrishnan and Schneider, 2022; Casarotti et al., 2014; Codină et al., 2016; Ismail and Rayan, 2022; Yang et al., 2023). Additionally, research on the roasting process of quinoa has demonstrated that roasting reduces antinutritional compounds while enhancing the sensory properties of products, such as taste and texture (Castro-Alba et al., 2019; Dong et al., 2021; Kheto et al., 2022; Repo-Carrasco-Valencia et al., 2010; Sharma et al., 2022).
However, despite these promising results regarding the benefits of roasted quinoa flour (RoQF), most studies have focused on its use in products like cakes, cupcakes, and other baked goods, with limited application in dairy products (Dong et al., 2021; Kheto et al., 2022; Sharma et al., 2022; Younis et al., 2024). There have been some studies examining the use of cow’s milk yogurt with QF (Akkoyun and Arslan, 2020; Alkobeisi et al., 2022; El-Menawy et al., 2023; Elslmawy et al., 2023; Ismail and Rayan, 2022; Rafiq, 2021), as well as another exploring GMY with extract of quinoa(El-Shafei et al., 2020), but none have utilized RoQF in their formulations, and especially not in goat milk yogurt. This highlights a significant gap in the research, as the use of QF in GMY has not been widely explored.
Considering the potential benefits of supplementing GMY with QF, we hypothesized that the addition of both roasted and unroasted QF would positively affect the physicochemical properties (such as chemical composition, texture, acidification, syneresis, during storage and sensory characteristics of the final product). Therefore, the aim of this study is to evaluate the effects of incorporating QF (Unroasted and roasted; White and red) at different concentrations (0.5, 1, and 1.5 g per 100 mL), on fermentation, physicochemical and sensory properties of GMY.
2 Materials and methods
2.1 Goat milk
Fresh GM from an Alpine breed, obtained from a farm in Béni Mellal, Morocco, was used for yogurt production. The milk samples were stored at 4°C until analysis.
2.2 Physicochemical analysis of raw goat milk
100 mL of fresh GM at 16°C was used to determine the studied physico-chemical parameters. Milko-Scan (Foss 5000 combi, Foss Electric, Hillerod, Denmark) was used for the milk composition analysis. The milk acidity was determined by a method described by Guiraud (2003), which involves acid–base titration using sodium hydroxide (NaOH) and phenolphthalein as a color indicator. The milk acidity results are reported in lactic acid %. Additionally, the pH of the milk was measured with a HI 2211 pH/ORP pH meter (Alia et al., 2023).
2.3 Quinoa flour production
The white quinoa seeds (WQS) cultivated in the regional center of agricultural research of Tadla, national institute of agricultural research (INRA), and the red quinoa seed (RQS) commercialized were washed with cold water to remove saponins, one portion of WQS and RQS was dried at room temperature for 24 h, and the other was roasted in an oven in a temperature at a temperature of 120°C for 5 min. The dried Quinoa seeds (QS) and roasted quinoa seeds (RoQS) were ground in a blender to obtain QF. The flour was then packed and stored until further use in goat milk yogurt (GMY) production. Using the AOAC approach (Paez et al., 2016), QF was analyzed for moisture and macronutrient composition (fat, ash, protein).
2.4 Preparation of yogurt
The GM milk was subjected to heat treatment at 60°C for 30 min in a water bath. After cooling to 45°C, different concentrations of QF were added: white quinoa flour (WQF), red quinoa flour (RQF), roasted white quinoa flour (RoWQF), and roasted red quinoa flour (RoRQF), at concentrations of 0.5, 1, and 1.5%, respectively. Several preliminary trials were conducted to determine the most suitable concentrations for optimal physicochemical and sensory properties. These trials included percentages ranging from 0.5% to 5%. Based on a sensory analysis checklist, we eliminated the percentages that exhibited a strong taste and color, retaining the concentrations of 0.5, 1, and 1.5%. A control test was performed in which 8% sugar was added to the milk to enhance the sweetness of the yogurt. The mixture was then blended for 2 min to ensure proper homogenization. The milk was subsequently inoculated with a starter culture containing Lactobacillus delbrueckii subsp. bulgaricus and Streptococcus thermophilus at a concentration of 1.2%. The inoculation levels were adjusted to 107 CFU/mL for each strain to ensure optimal fermentation without affecting the texture or taste of the yogurt. The inoculated milk was incubated at 45°C, and fermentation was considered complete when the pH dropped below 4.5, indicating the production of lactic acid. The resulting yogurts were transferred to airtight 50 mL transparent plastic cups, labeled according to the QF concentration, and stored at 4°C. These containers were chosen for their ability to minimize moisture loss and prevent contamination, ensuring proper storage conditions for the yogurt. Finally, physicochemical and sensory analyses were conducted on the products to evaluate their characteristics.
2.5 Physicochemical properties of yogurt
2.5.1 Determination of pH and acidity titratable during fermentation
pH and acidity were measured every hour (T0, T1, T2… T7) during the fermentation of the prepared samples. This monitoring was performed for all the samples: GMYWQF, GMYRQF, GMYRoWQF, and GMYRoRQF with concentrations of 0.5, 1, and 1.5% until a pH of 4.6 was reached. A blank sample was prepared to compare the results obtained.
pH was measured using a digital pH meter (Ins Mask Model number: IS12BL) following the method described by AOAC (2005). The pH meter was standardized with buffer solutions having pH 4.00 and pH 7.00 before use. To check the pH of the sample, it was transferred to a beaker, mixed thoroughly, and the electrode of the pH meter was dipped into the sample. The pH was then noted (Saeed et al., 2021).
Acidity was determined by the method mentioned in AOAC (2005). A well-mixed homogeneous 10 mL yogurt sample was taken in a beaker and diluted with 20 mL distilled water. Then, 2–3 drops of phenolphthalein were added as an indicator into the beaker and titrated against N/10 sodium hydroxide (0.1 N NaOH) solution until a pink color appeared as the endpoint and was retained for 30 s (Alia et al., 2023).
2.5.2 Approximate composition
The approximate composition of the final products was analyzed following the methods established by the Association of Official Analytical Chemists (Paez et al., 2016): The protein content was determined using the micro-Kjeldahl method, while fat content was assessed by the Gerber method. Moisture and ash content were measured by drying the samples at 105°C and by incineration at 550°C, respectively.
2.5.3 Viscosity apparent
The rheological measurements were performed using a Brookfield rotational viscometer (model DV-II+Pro) (Brookfield Lab., Middleboro, MA, United States). The measurements were carried out at 81°C with the RV spindle (No. 4) rotating at 100 rpm. For each yogurt sample, three readings were taken. The rheological behavior of the yogurt samples was characterized with the help of the power law (Ostwald-de-Wael) model, as developed from the data obtained in the viscometer (Codină et al., 2016; Zine-Eddine et al., 2022).
2.5.4 Stability of yogurts during refrigerated storage
The pH and total acidity of yogurt samples were recorded on the 1st, 7th, and 14th days during storage at 5°C (Curti et al., 2017).
2.5.5 Syneresis
After storing the yogurt samples for 24 h at 46°C, Syneresis was assessed using the centrifugation technique. A 10 mL glass tube containing about 5 g of yogurt was centrifuged for 15 min at 3500 rpm. Syneresis was determined as the proportion of the released whey over the initial gel weight (Codină et al., 2016).
2.6 Sensory analysis
2.6.1 Sensory evaluation
The yogurt samples were subjected to sensory evaluation. This evaluation was conducted by trained panelists, specifically trained to identify a set of characteristics: color homogeneity, creaminess, gel firmness, fatty layer, quinoa flavor, artificial flavor, goat milk (GM) flavor, cream flavor, unpleasant flavor, legume taste, sour taste, sweet taste, and bitter taste. The sensory evaluation was carried out on a scale of 1–9, where the intensity increased with the number (Alia et al., 2023).
The sensory panel training followed the guidelines outlined in ISO 8586:2012: “Sensory analysis: General guidelines for the selection, training, and monitoring of assessors.”
The sensory panel consisted of 10 assessors, primarily students and researchers from the Higher School of Technology, Sultan Moulay Slimane University, aged between 25 and 45 years, with a balanced gender representation (5 males, 5 females). All panelists were selected based on their previous experience in sensory evaluation, ensuring that they had the necessary expertise to perceive and describe the sensory attributes relevant to yogurt. The selection method was based on identifying candidates who demonstrated the ability to detect and differentiate sensory nuances in food products, with preference given to individuals who had previously participated in sensory evaluations as part of their studies or research. Prior to the evaluation, the panel underwent two training sessions. The first session, lasting 2 h, focused on familiarizing the panelists with key sensory attributes such as texture, flavor, and appearance. The second session, also 2 h in duration, involved practical exercises where the panelists evaluated a range of yogurt samples to practice identifying and describing sensory characteristics. This approach helped ensure that the panelists had a solid understanding and consistency in their evaluations. The panel calibration was conducted through regular meetings throughout the study, during which panelists compared samples and discussed differences in sensory characteristics. Comparative tastings of yogurt samples with varying levels of quinoa flour (QF) and goat milk (GM) were performed to refine the panelists’ understanding of each attribute and align their evaluations. Sensory attributes, including “quinoa flavor” and “GM flavor,” were clearly defined for the panelists at the beginning of the training, and standardized reference materials were used to help distinguish subtle differences in flavor, texture, and appearance. To ensure the reliability of the results, the consistency of the panel was monitored by assessing inter-panelist variability during the training and calibration sessions, where ratings for the same sample were compared. This process helped identify and minimize inconsistencies, and statistical analysis was conducted to evaluate the level of agreement among the panelists and ensure the reproducibility of the sensory evaluations (Höhl and Busch-Stockfisch, 2015).
The results were expressed as an average score for each characteristic. The samples were evaluated: GMYWQF, GMYRoWQF, GMYRQF and GMYRoRQF for different percentages of added QF: 0, 0.5, 1, and 1.5%. To remove any aftertaste, mineral water was supplied in between samples.
2.6.2 Global acceptance
For the acceptance test, civil servants, teachers, researchers, and students from the Béni Mellal Higher School of Technology (Sultan Moulay Slimane University, Morocco) took part in the consumer test. These participants evaluated the samples and gave them a mark on a scale of 1–9.
2.7 Statistical analysis
The data analysis involved conducting a one-way multivariate ANOVA and a Tukey test at a significance level of 0.05, using Minitab’s statistical package, version 18 (Minitab, Inc.).
3 Results and discussion
3.1 Chemical composition of fresh goat milk and quinoa flour
The physicochemical characteristics of the fresh GM used in yogurt production are shown in Table 1.
The physicochemical characteristics of the fresh GM used in yogurt production in this study are consistent with those reported in the literature (Zine-eddine et al., 2021a,b). Numerous studies have highlighted the potential of GM for yogurt production (Costa et al., 2022; Ningtyas and Haskito, 2020). Moreover, incorporating QF with GM can improve both the nutritional profile and sensory properties of the resulting yogurt.
The proximate physicochemical composition of WQS cultivated (unroasted and roasted), RQS commercialized (unroasted and roasted) and GM samples is shown in Table 2.
The data in the table reveal significant differences (p ≤ 0.05) in the nutritional composition of quinoa samples based on their variety (white, red) and processing method (roasted, unroasted). The moisture content is highest in the commercialized RQF (11.34%), followed by the WQF (9.34%), while the RoQF varieties have lower moisture levels (5.34% for RoWQF and 7.34% for RoRQF). This decrease in moisture after roasting is typical, as roasting leads to water evaporation. Ash content, which reflects mineral concentration, is highest in the WQF (6.44%) and lowest in RoRQF (4.66%). In terms of fat, the RQF contains the highest fat content (6.4 g/100 g), WRoQF varieties, especially ROWQF, have lower fat levels (2.65 g/100 g). The fat content in RoRQF (4.53 g/100 g) remains relatively high compared to RoWQF. Protein content is highest in WQF (18.91 g/100 g), with roasted quinoa samples showing a reduction in protein levels, particularly in RoRQF, which has the lowest protein content (10.3 g/100 g). These variations suggest that WQF is a better source of protein, while RQF offers higher fat content. Roasting appears to reduce the moisture, fat, and protein content of quinoa, especially in RQF, which may affect its texture and nutritional profile when used in food products.
Studies have highlighted the nutritional value of quinoa, whose composition can vary from one study to another due to several factors, such as the specific variety of quinoa cultivated, climatic conditions, and storage practices, as noted in previous research (El-Hakim et al., 2022; Li et al., 2021; Sekhavatizadeh et al., 2021). Furthermore Kheto et al. (2022), Sharma et al. (2022), Younis et al. (2024) reported that roasting influences the macronutrient composition, particularly proteins and lipids, which could explain the results obtained in our study.
3.2 Physicochemical analysis
3.2.1 Effect of adding QF on fermentation parameters
The evolution of pH (Figure 1) and acidity (Figure 2) was tracked throughout the fermentation process in all of the samples examined in order to demonstrate the impact of QF addition on the metabolism of lactic acid bacteria. When the control sample’s pH hit 4.6, the lactic fermentation process was terminated.
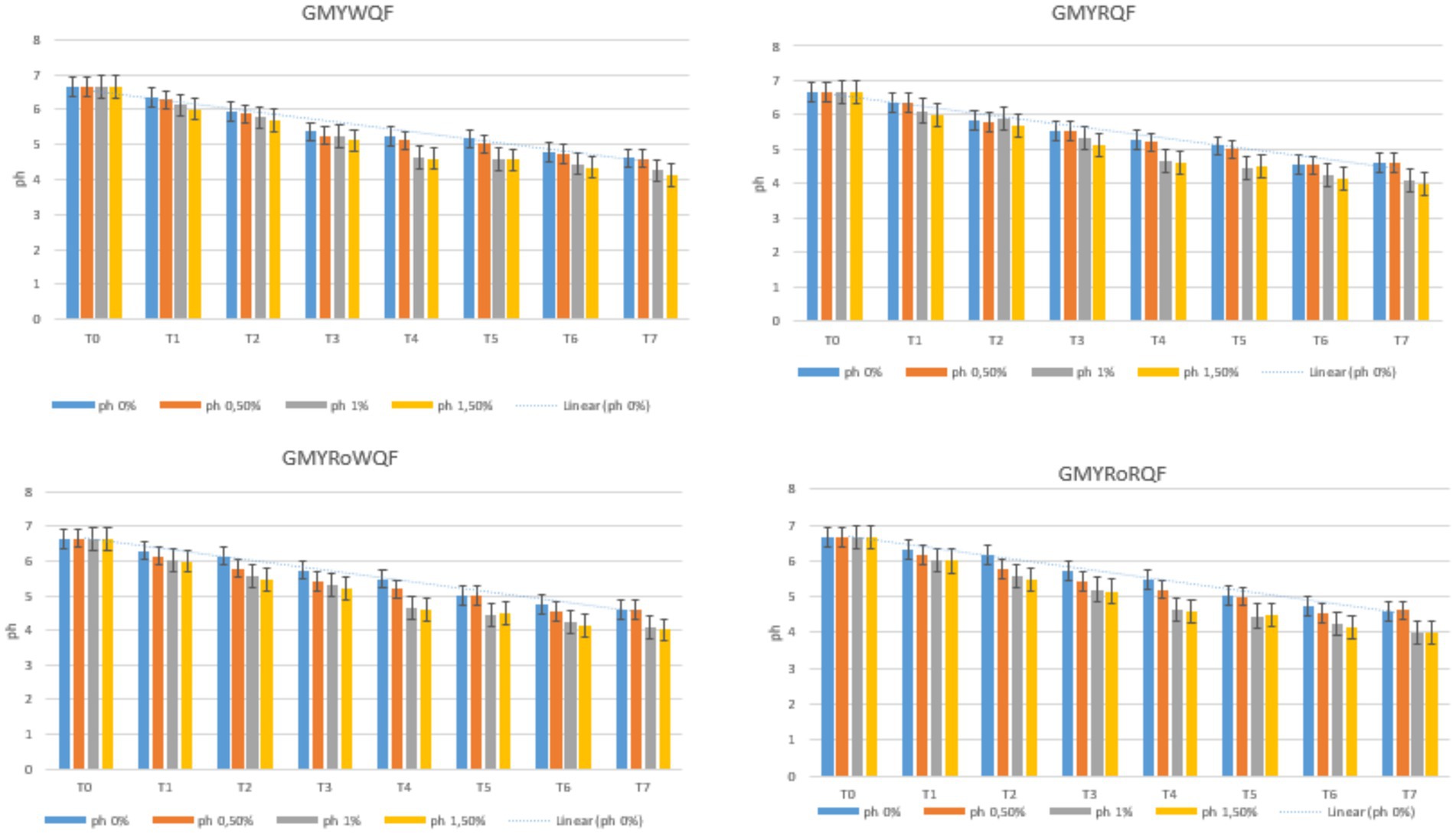
Figure 1. pH changing during fermentation time (every hour) in yogurt samples with different level (0.5, 1, and 1.5%) of: white quinoa flour (GMYWQF), red quinoa flour (GMYRQF), roasted white quinoa flour (GMYRoWQF) and roasted red quinoa flour (GMYRoRQF). The trend line, based on the control (0%), shows a gradual decrease in pH, with a notable drop observed in the samples enriched with 1.5%QF, followed by those with 1%.
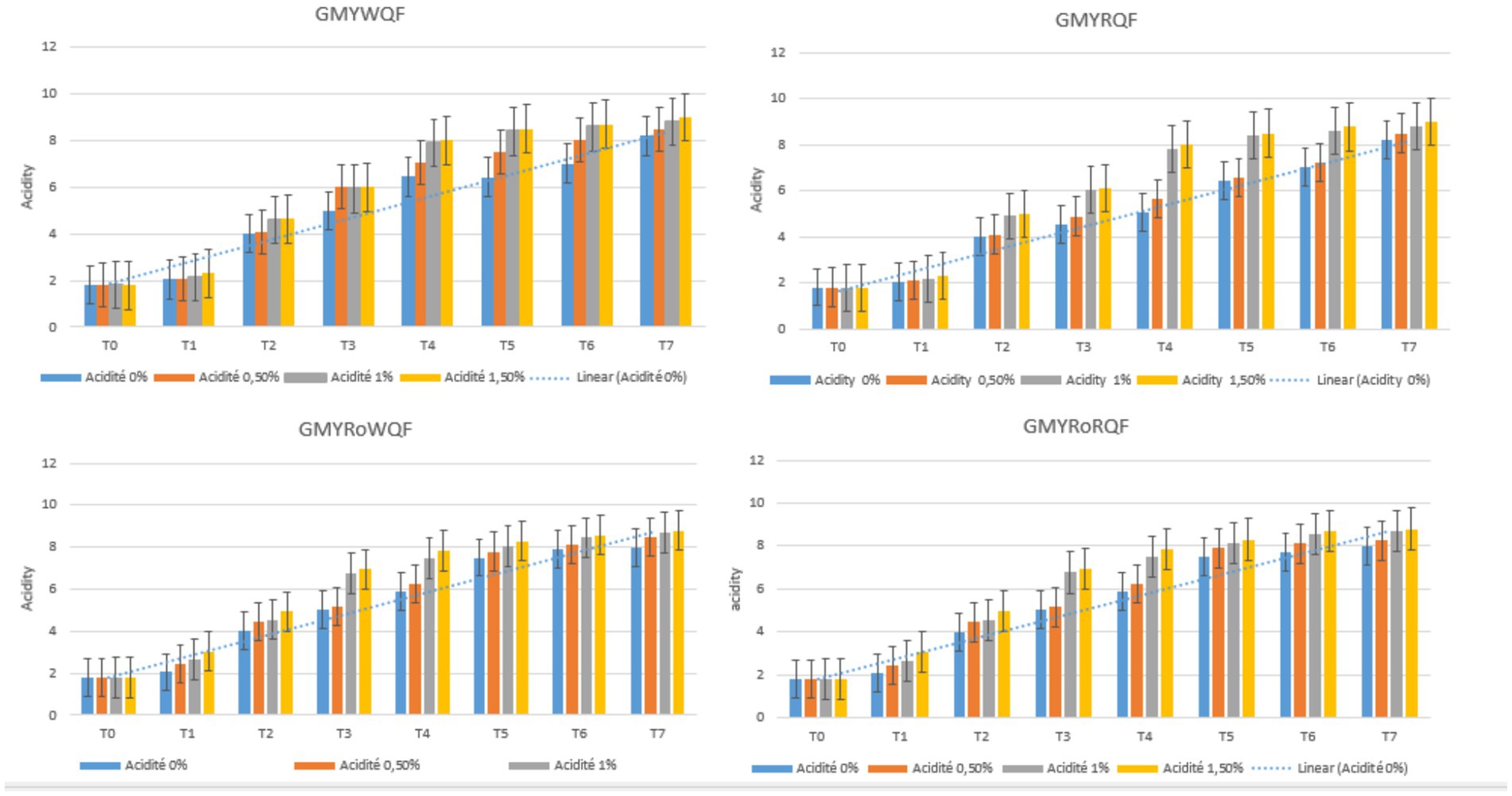
Figure 2. Changing in titratable acidity during fermentation time (every hour) in yogurt samples with different level (0.5, 1, and 1.5%) of: white quinoa flour (GMYWQF), red quinoa flour (GMYRQF), roasted white quinoa flour (GMYRoWQF) and roasted red quinoa flour (GMYRoRQF). The trend line, based on the control (0%), shows a gradual increase in acidity, with a notable rise observed in the samples enriched with 1.5%QF, followed by those with 1%.
The data presented, illustrating the variations in pH and titratable acidity of yogurt enriched with QF, roasted or unroasted, at different concentrations (0.5, 1, and 1.5%) compared to a control sample without any quinoa addition, show significant differences in the fermentation profiles of the samples. As the QF concentration increased, the fermentation time gradually decreased. After 1 h of incubation, the pH of the 1.5% QF-enriched samples (GMYWQF, GMYRQF, GMYRoWQF, and GMYRoRQF) was lower than that of the control (pH = 6.65), with values of 6.02, 6.01, 6.00, and 6.01, respectively. After 2 h of fermentation, the pH of the 1% QF-enriched samples (GMYWQF, GMYRQF, GMYRoWQF, and GMYRoRQF) was also lower than the control (pH = 6.15), with values of 5.78, 5.88, 5.58, and 5.20, respectively. After 4 h of fermentation, the yogurt samples enriched with 1.5 and 1% QF reached a pH of 4.6 and an acidity of 80°D, while the samples with lower quinoa concentrations (0.5%) and the control required approximately 5 h or more to reach similar levels of pH reduction and acidity increase.
Interestingly, the results show similar trends between both roasted and unroasted quinoa samples, as well as between white and red quinoa varieties, suggesting that the variety of quinoa and its roasting process have little to no significant impact on the fermentation dynamics in yogurt production. These results indicate that quinoa has a notable impact on the activity of the yogurt starter culture, accelerating the fermentation process and promoting faster acidification. These findings are consistent with previous studies (Akkoyun and Arslan, 2020; Alkobeisi et al., 2022; Casarotti et al., 2014; Codină et al., 2016; Elslmawy et al., 2023) who suggested that quinoa-enriched samples exhibited a greater decrease in pH and a higher increase in acidity compared to the control sample during the fermentation process. This accelerated acid production observed in the samples can likely be attributed to the beneficial nutritional properties of quinoa, particularly its high content of amino acids and essential minerals, which are known to support the growth and metabolic activity of lactic acid bacteria. Amino acids serve as energy sources for the bacteria, while minerals act as cofactors for enzymes involved in lactose degradation, thus enhancing bacterial efficiency in producing lactic acid. As a result, this leads to a faster decrease in pH and a more rapid increase in acidity, optimizing the fermentation process (Akkoyun and Arslan, 2020; Elslmawy et al., 2023). Additionally Elslmawy et al. (2023) demonstrated that modifying the carbohydrate composition of milk enhances the acidification rate of yogurt starters.
Therefore, the addition of QF (unroasted and roasted), regardless of its variety or roasting process, could serve as an effective means of optimizing the fermentation process in yogurt production, as hypothesized in this study, potentially offering a more efficient way to control the acidity and texture of the final product.
3.2.2 Approximate composition of yogurt samples
The table below presents the physicochemical composition of various prepared products. The results reveal a significant difference between the samples (p ≤ 0.05).
In our study, we examined the effects of incorporating QF at three concentrations: 0.5, 1, and 1.5%. At the 0.5% level, yogurt enriched with WQF contained 0.74% ash, 3.96% protein, 4.1% lipids, while the yogurt with RQF showed 0.73% ash, 3.97% protein, and 4.08% lipids. Notably, the RoQF exhibited slightly lower values: the RoWQF had 0.73% ash, 3.95% protein, and 4.03% lipids, while the RoRQF contained 0.73% ash, 3.96% protein, and 4.06% lipids.
Increasing the QF content to 1% resulted in further nutritional improvements. The yogurt with WQF showed 0.77% ash, 3.98% protein, and 4.19% fat, while the yogurt with RQF contained 0.76% ash, 4% protein, and 4.16% lipids. Once again, the roasted varieties presented lower values: the RoWQF produced 0.76% ash, 3.96% protein, and 4.15% fat, while the RoRQF showed 0.75% ash, 3.98% protein, and 4.11% fat.
At the 1.5% level, the yogurt demonstrated further improvements, with the WQF yielding 0.8% ash, 4% protein and 4.21% fat. The yogurt with RQF contained 0.79% ash, 4.03% protein and 4.24% fat. However, as observed at lower concentrations, the RoQF varieties exhibited slightly lower nutritional values: the RoWQF had 0.78% ash, 3.97% protein, and 4.22% fat, while the RoRQF yielded 0.77% ash, 4% protein, and 4.16% fat.
These results confirm that the addition of QF significantly improves the nutritional composition of yogurt (p ≤ 0.05), as reported by many studies (Akkoyun and Arslan, 2020; Balakrishnan and Schneider, 2022; El-Shafei et al., 2020; Elslmawy et al., 2023; Ismail and Rayan, 2022; Mabrouk and Effat, 2020). This improvement is mainly attributed to quinoa’s composition, particularly its high protein and fat content, as discussed in the previous section. However, roasting quinoa leads to a slight decrease in some nutritional qualities, which aligns with other research indicating that roasting can reduce quinoa’s nutritional value (Dong et al., 2021; Kheto et al., 2022; Sharma et al., 2022; Younis et al., 2024). Overall, our findings support the positive impact of QF on yogurt’s nutritional profile and validate our hypothesis that quinoa supplementation enhances yogurt’s nutritional composition, with roasting having a minor but noticeable effect on nutrient retention.
The information shown in Table 3, details the apparent viscosity of GMY enriched with different concentrations of QF.
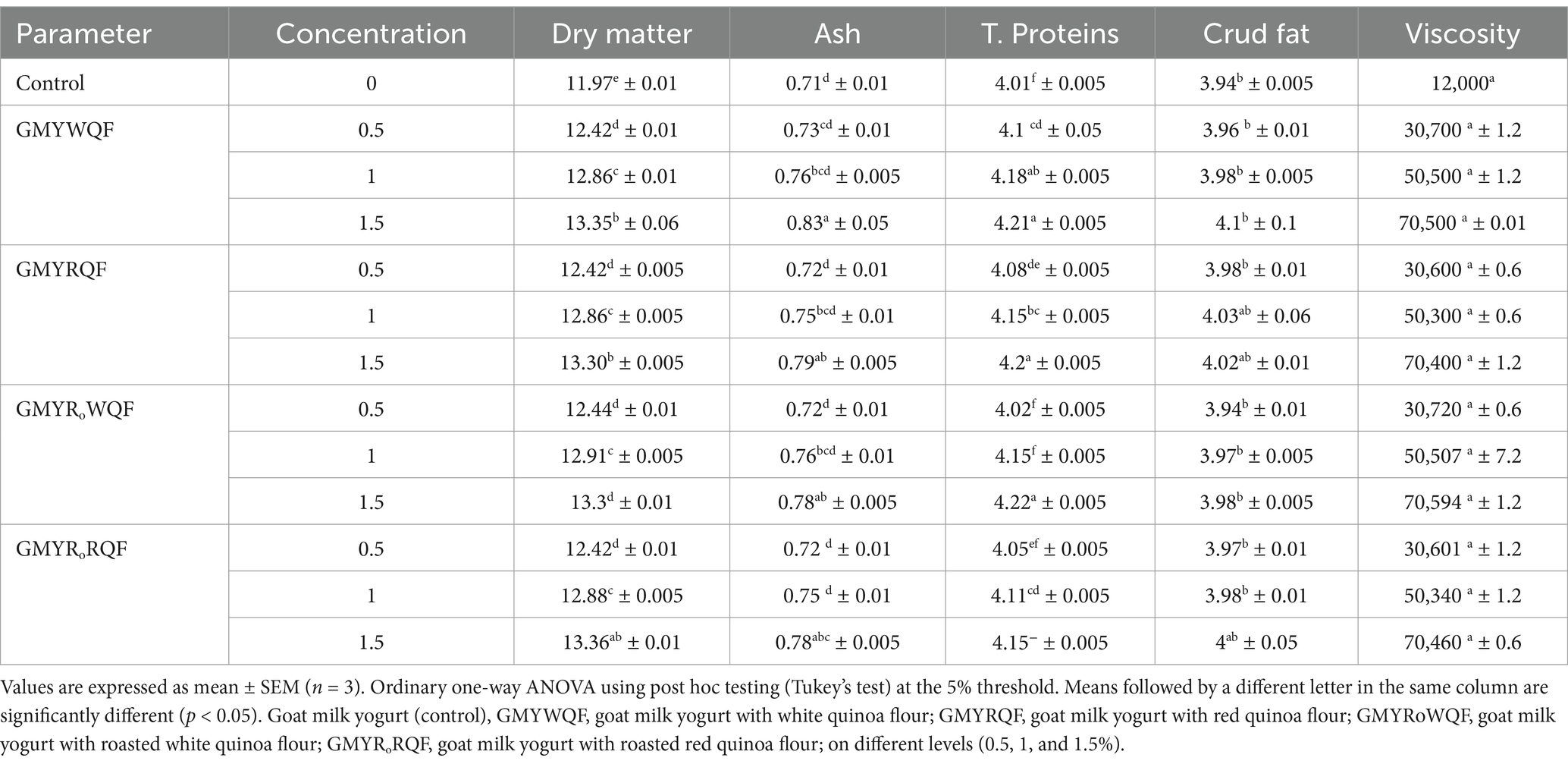
Table 3. Chemical composition and viscosity characteristics of goat milk yogurts (GMY) enriched with quinoa flour (QF).
As hypothesized, higher viscosities were recorded in the yogurt samples supplemented with QF. Furthermore, our findings indicate that increasing the QF content leads to a rise in the yogurt’s viscosity. The treatments GMYWQF, GMYRQF, GMYRoWQF and GMYRoRQF; at 1 and 1.5%, exhibited the highest viscosity values. These results are consistent with other studies (El-Shafei et al., 2020; Elslmawy et al., 2023; Mabrouk and Effat, 2020), which attributed this increase to several factors: the rise in apparent viscosity is associated with the excellent binding characteristics of QF and the presence of starch granules rich in amylopectin, which serve as thickeners in frozen and fermented product. Heating starch above its gelatinization temperature in the presence of water makes granules absorb and bind water, swell and disrupt their structure, and thus change their rheological properties. The gelatinization temperature of quinoa starch ranges from 57 to 64°C and heating both milk and quinoa that contain starch at 85°C for 20 min prior to the starter inoculation was adequate for starch gelatinization to occur in milk. In this mechanism, the swelling of starch granules and leaching of amylose and amylopectin in the continuous phase might have increased the viscosity of yoghurts. Moreover Codină et al. (2016) stated that due to its high content of fiber, QF up to 1.0% addition contributes to an increase in apparent viscosity of yogurt samples, probably due to the fact that QF can bond water in samples and consequently increase apparent viscosity, this may be a benefit, as yogurt may retain its stability for a longer period of time.
3.2.3 Stability of yogurts during refrigerated storage
Figures 3, 4 presented illustrate the changes in pH and acidity of yogurt over a 15 day storage period, with varying concentrations of QF added (0, 0.5, 1, and 1.5%) and different types of QF (white, red, roasted, and unroasted).
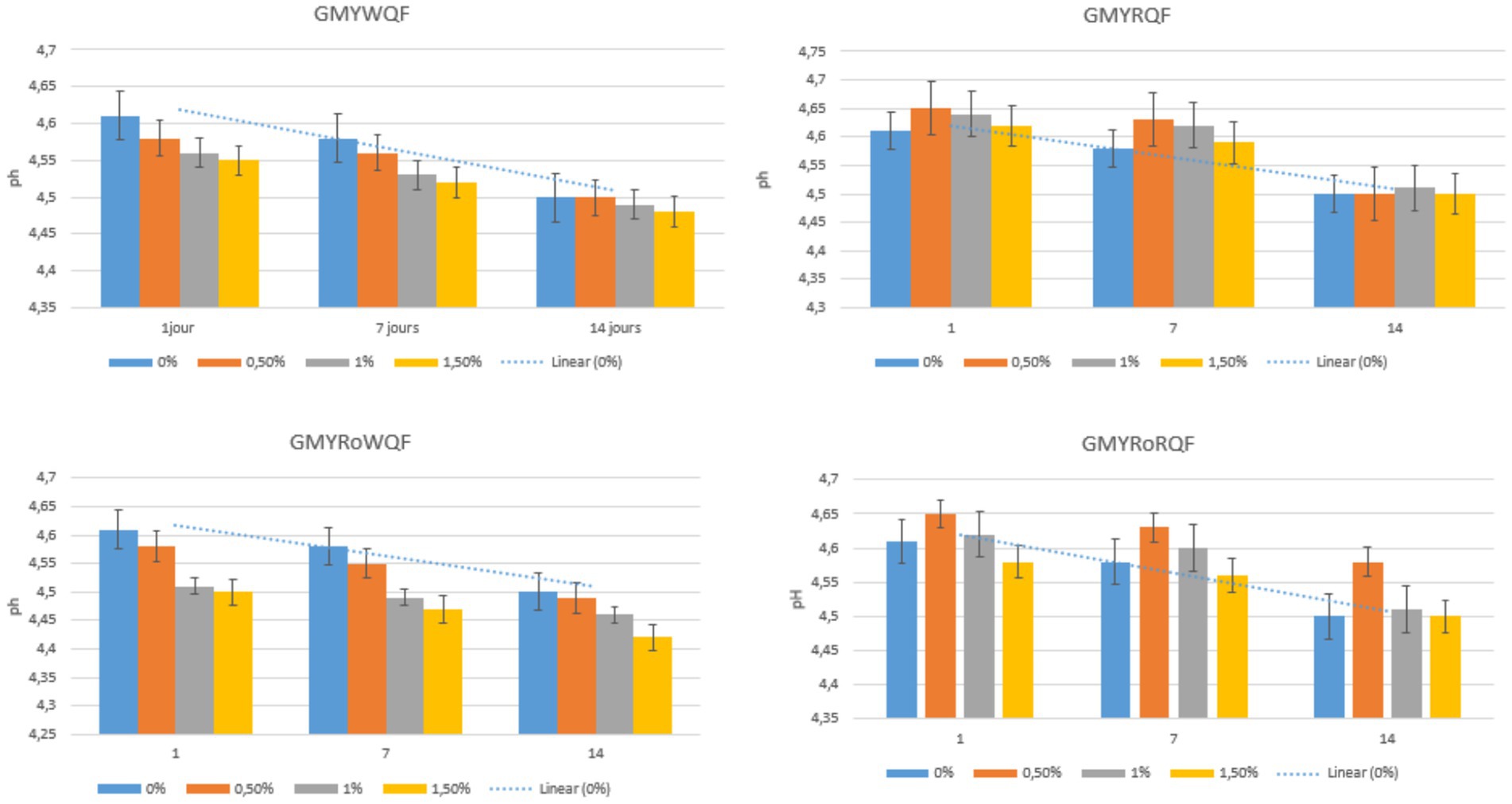
Figure 3. pH changing goat milk yogurt enriched with different percentages (0.5, 1, and 1.5%) of: white quinoa flour (GMYWQF), red quinoa flour (GMYRQF), roasted white quinoa flour (GMYRoWQF) and roasted red quinoa flour (GMYRoRQF) during storage (1, 7, and 14 days) at 4°C. The trend line, based on the control (0%), shows a gradual decrease in pH, with a slight decrease observed in the 1.5% samples compared to the lower concentrations.
The yogurts enriched with QF at concentrations of 0.5, 1, and 1.5% (both with white and red quinoa, whether roasted or unroasted) exhibited a notable decrease in pH and an increase in acidity at the 1 and 1.5% quinoa levels, with the changes being more pronounced compared to the 0.5% concentration. However, no significant differences were found between these higher concentrations, indicating that adding quinoa up to 1.5% remains acceptable in terms of product stability. This decrease in pH and the corresponding increase in total acidity during storage can be attributed to the increased consumption of residual lactose by lactic acid bacteria, as the lactic acid bacteria continue to consume the residual lactose during storage, the acidity increases gradually over time, which explains the continued decrease in pH, as suggested by El-Shafei et al. (2020).
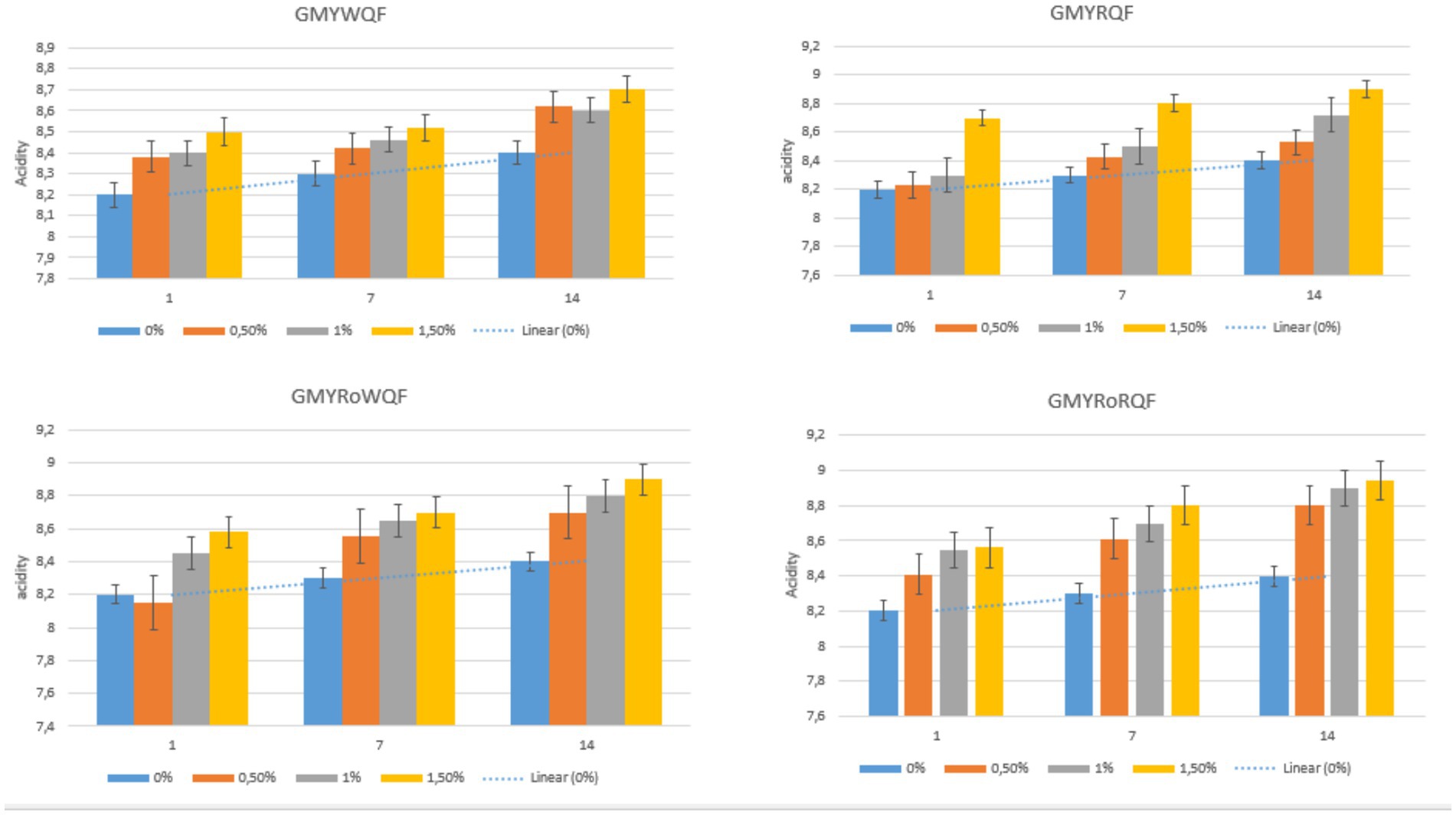
Figure 4. Acidity changing of goat milk yogurt enriched with different percentages (0.5, 1, and 1.5%) of white quinoa flour (GMYWQF), red quinoa flour (GMYRQF), roasted white quinoa flour (GMYRoWQF) and roasted red quinoa flour (GMYRoRQF) during storage(1, 7, and 14 days) at 4°C. The trend line, based on the control (0%), shows a progressive increase in acidity, with a slight increase observed in the 1.5% samples compared to the other concentrations.
According to Casarotti et al. (2014) adding up to 3% QF to fermented milk had no effect on the fermentation kinetics during the 28 day storage period. However, research of Curti et al. (2017) suggested that while a 1% quinoa addition enhances product stability, higher concentrations, such as 5% and beyond, may cause syneresis, which adversely impacts the yogurt’s texture and stability.
Importantly, the results were consistent for both WQ and RQ, as well as for roasted and unroasted varieties, indicating that the type and treatment of quinoa (roasted or unroasted) do not significantly influence the overall stability of the product.
3.2.4 Syneresis
Syneresis is a physical defect that is observed after keeping yoghurt for a particular time, it is undesirable, affecting consumers’ acceptance, as it may result in water leakage it (Pappa et al., 2024). Figure 5 illustrates the Syneresis observed in various yogurt samples prepared with different quinoa percentages and types, including raw, white, roasted, and unroasted quinoa.
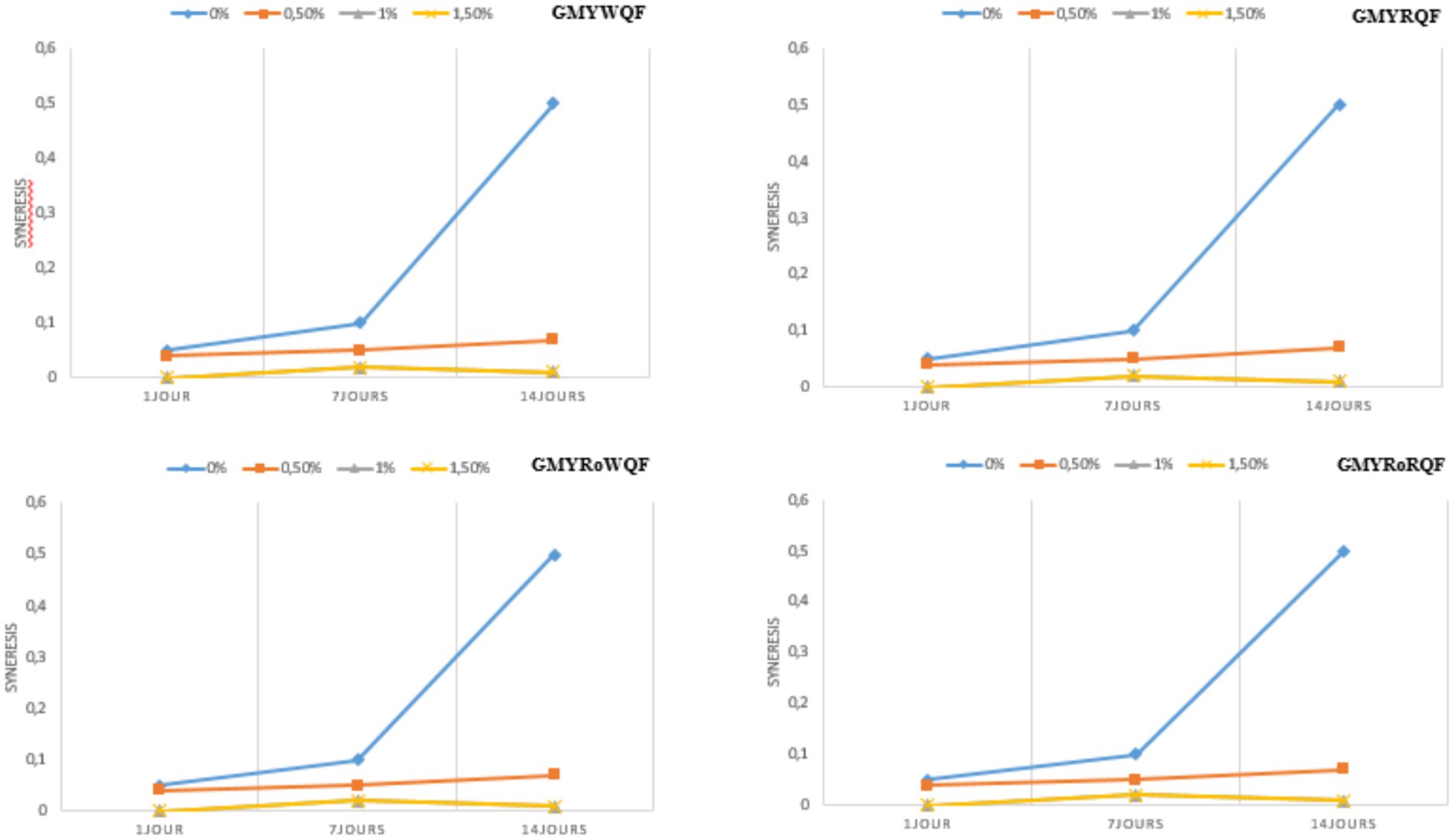
Figure 5. Syneresis changing of goat milk yogurt enriched with different percentages of: white quinoa flour (GMYWQF), red quinoa flour (GMYRQF), roasted white quinoa flour (GMYRoWQF) and roasted red quinoa flour (GMYRoRQF) during storage (1, 7, and 14 days). Syneresis was higher in the control sample compared to the products with QF.
In this study, yogurts enriched with QF at concentrations of 1 and 1.5%, whether made with white or RQ and either roasted or unroasted, showed no whey separation. This indicates a significant improvement in product stability compared to the control sample and the 0.5% quinoa enriched samples. The absence of syneresis suggests that the presence of starch and fiber in QF could reduce free water molecules due to their water binding properties (Abugoch James, 2009). In contrast, the 0.5% quinoa enriched samples, regardless of whether they were roasted or unroasted, exhibited some degree of whey separation. This suggests that lower concentrations of QF may not provide sufficient structural reinforcement to fully prevent syneresis. This finding is in agreement with Alkobeisi et al. (2022), who observed the lowest Syneresis values in the control, 100% QF and 75% QF samples. Furthermore, it aligns with studies Codină et al. (2016) showing that higher concentrations of QF (such as 3 and 5%) lead to syneresis formation. The differences between our results and those of previous studies may be partly attributed to the type of milk used. While previous studies used cow’s milk, which has a different protein composition, our study used GM. GM may interact differently with quinoa components, potentially enhancing yogurt stability at lower quinoa concentrations. These results support the idea that QF enhances yogurt stability, and syneresis can be minimized at higher concentrations. However, as the shelf stability of yogurt is also influenced by other factors such as microbial growth and texture changes over time, further research could explore how quinoa-enriched yogurt maintains its texture and overall quality during extended storage periods. This would provide more detailed insights into the long-term shelf life of quinoa-enriched yogurt and help evaluate its potential for commercial production.
3.3 Sensorial analysis
3.3.1 Sensory evaluation
A useful criterion for assessing the acceptability and quality of yogurt is sensory (Barrantes et al., 1996). Table 4 shows the application of the Tukey method for data regrouping and a 95% confidence level to an ANOVA analysis of response to sensory characteristics for 16 different types of yogurt based on GM and QF.
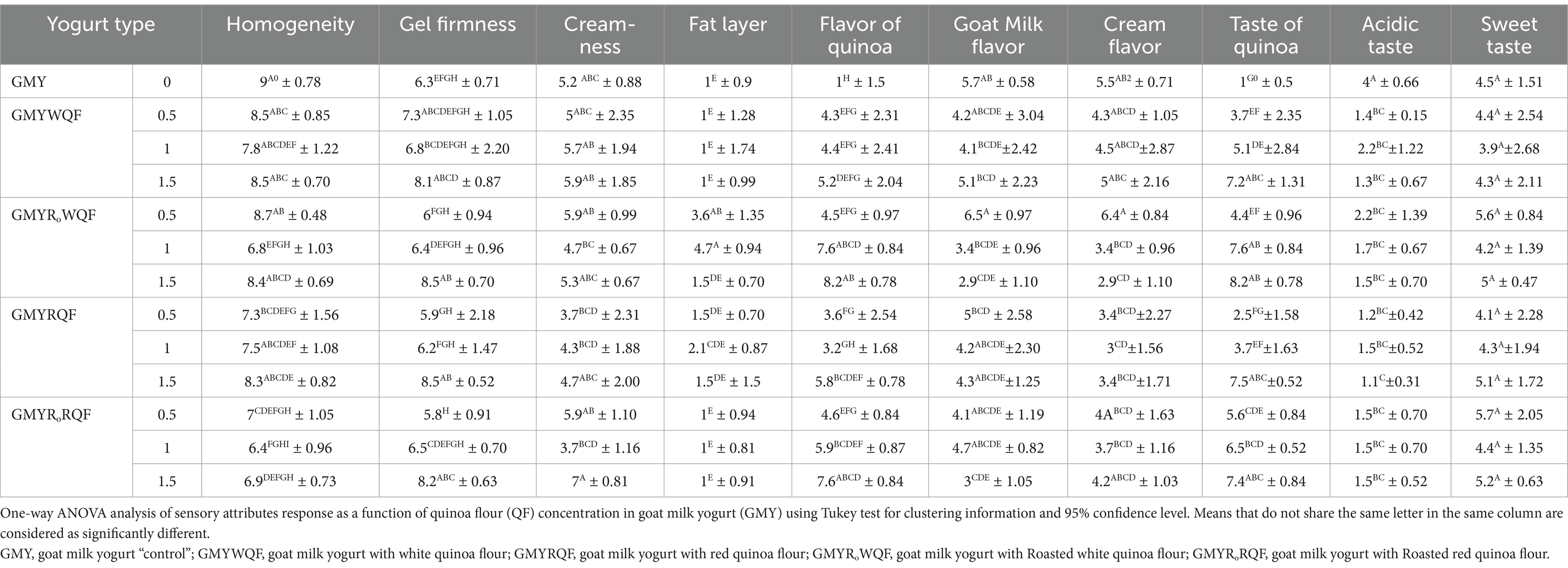
Table 4. Sensory analysis results of goat milk yogurt enriched with white and red quinoa (roasted and unroasted) at different concentrations.
The sensory evaluation results revealed significant differences (p ≤ 0.05) across the various yogurt samples fortified with QF, particularly in terms of homogeneity, firmness, creaminess, texture, flavor and taste. These differences were influenced by the concentration of quinoa added (0.5, 1, and 1.5%) and whether the quinoa was roasted or unroasted. The sensory attributes of the yogurt were closely related to the changes in physical properties like viscosity, which in turn were affected by QF addition.
3.3.2 Homogeneity
In terms of homogeneity, the yogurt samples containing 1.5% quinoa (both roasted and unroasted white and red) were the least homogeneous, while samples containing 0.5% QF and the control samples (without quinoa) showed the highest levels of homogeneity. This suggests that adding higher concentrations of QF, particularly 1.5%, may disrupt the uniform texture of the yogurt, while lower concentrations or the absence of quinoa allow for a smoother, more uniform product. The addition of higher quinoa levels may pose challenges for effective homogenization, likely due to the larger particle sizes or varying properties of quinoa flour at higher concentrations which could also affect other sensory attributes such as firmness and creaminess.
3.3.3 Firmness and texture
Regarding firmness, yogurts with 1.5% QF (whether roasted or unroasted) were firmer than those with 1% quinoa, while samples with 0.5% quinoa and the control samples had lower firmness scores. The increase in firmness can likely be attributed to the higher protein content in quinoa it helps to strengthen the gel matrix of the yogurt, improving its overall texture and firmness compared to the control samples or those with lower quinoa concentration. This, in turn, affects the creaminess of the yogurt, as a firmer texture may alter the creamy sensation in the mouth, making it feel denser and less smooth (Alkobeisi et al., 2022).
3.3.4 Creaminess and viscosity
When it comes to creaminess, control samples and those with 0.5% RoWQF and RQF were found to be the creamiest, while samples with 1 and 1.5% quinoa were less creamy. This trend was also observed with both roasted and unroasted quinoa. The decrease in creaminess at higher quinoa concentrations can likely be explained by the viscosity changes observed in the previous results. As quinoa concentration increases, its effect on the viscosity of the yogurt becomes more pronounced, leading to a denser, less creamy texture. Higher quinoa levels (1 and 1.5%) may increase the viscosity of the liquid phase, altering the mouth feel and resulting in a less creamy product. In contrast, lower quinoa concentrations (0.5%) allow for a softer, creamier texture due to the more fluid consistency.
3.3.5 Flavor and taste
The quinoa flavor varied significantly between samples. The yogurt samples with 0.5% quinoa, had the least pronounced quinoa flavor, while those with 1.5% RoWQF and RoRQF had a much stronger quinoa flavor. Yogurts containing 1% quinoa displayed a moderate quinoa flavor, falling between the 0.5 and 1.5% samples. Compared to control samples, those with higher quinoa concentrations (1 and 1.5%) exhibited less goat milk flavor and cream flavor. The increase in quinoa flavor at higher concentrations is likely due to the more pronounced flavor profile of quinoa at these levels. In particular, roasting quinoa intensifies its flavor, likely through the Maillard reaction and the caramelization of sugars during roasting (Castro-Alba et al., 2019; Fayle and Gerrard, 2002). The lower quinoa concentration (0.5%) results in a more subtle flavor, which might be less detectable to consumers, especially those who are sensitive to quinoa’s characteristic taste.
The taste of quinoa varies depending on the concentration in the samples. Yogurts containing 0.5% quinoa (white and red; unroasted and roasted) have a more subtle quinoa taste, while those with 1.5% roasted (RoWQF and RoRQF) quinoa have a much stronger quinoa taste. Yogurts with 1% quinoa display an intermediate taste, falling between the 0.5 and 1.5% samples. The addition of roasted quinoa not only masks the acidity but also imparts a subtle sweetness to the yogurt, which is reflected in the increased sweetness scores, with higher values for certain formulations 1 and 1.5%. This sweetness enhances the overall enjoyment of the tasting, making the yogurt even more pleasant to the palate.
In correlation with the physicochemical results, these findings are likely due to the enhancement of starter culture growth, which increases the production of lactic acid and other flavor compounds, as well as the high protein content of quinoa, which positively influences the texture of the final product. The addition of QF to GMY overall improved the sensory characteristics in terms of firmness, texture, and creaminess. Furthermore, the roasting process significantly enhanced the yogurt’s acceptability, especially in terms of taste and flavor, by masking the often undesirable goat milk flavor. These findings are consistent with those reported by Alkobeisi et al. (2022), Castro-Alba et al. (2019), Curti et al. (2017), Elslmawy et al. (2023), and Mabrouk and Effat (2020) who also found that the addition of QF improved yogurt stability and increased its consumer acceptability. Roasting QF before adding it to yogurt not only enhances the taste but also improves its overall sensory appeal, making the yogurt more palatable to a broader range of consumers, including those who might find the goat milk flavor too strong. Thus, the hypothesis that the addition of QF to GMY would improve its sensory attributes, stability, and overall quality is confirmed. The inclusion of QF at higher concentrations (1%) leads to improved texture, firmness, and overall acceptability, while the roasting process enhances the yogurt’s flavor and reduces undesirable goat milk flavor.
3.3.6 Acceptance global of final product
The results presented in Figure 6 illustrate the levels of acceptance of participants towards GMY fortified with varying concentrations of QF, with the mean values represented by a 95% confidence interval.
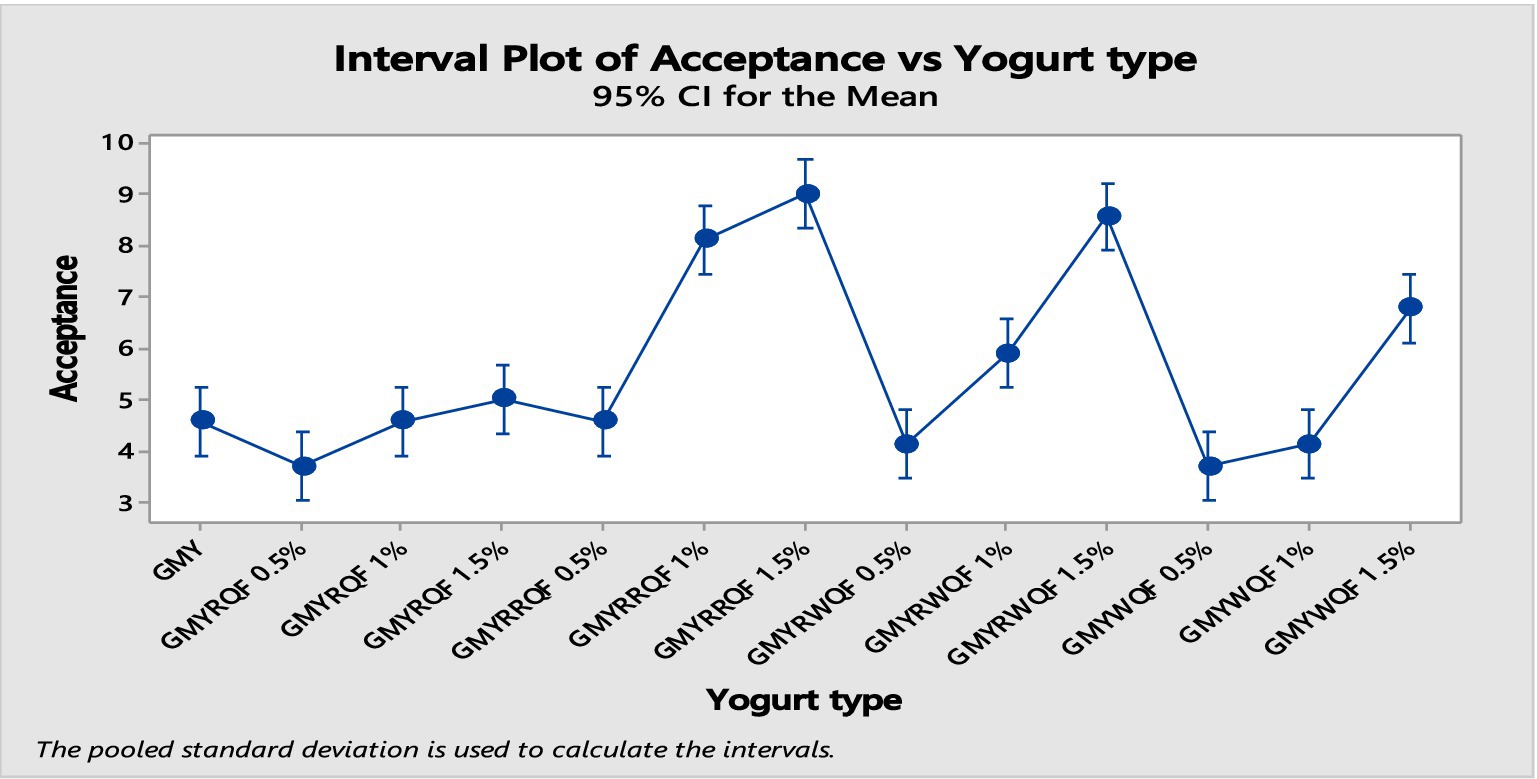
Figure 6. Interval plot of participants’ acceptance of GMY enriched with QF (95% CI of the mean). The plot highlights trends in consumer acceptance, with the highest acceptance observed in GMYRoWQF and GMYRoRQF both containing 1.5% QF, followed by those with a 1% concentration. GMY: goat milk yogurt “control”; GMYWQF: goat milk yogurt with white quinoa flour; GMYRQF: goat milk yogurt with red quinoa flour; GMYRoWQF: goat milk yogurt with Roasted white quinoa flour; GMYRoRQF: goat milk yogurt with Roasted red quinoa flour.
Statistical analysis revealed a significant difference (p < 0.05) in the participants’ responses. Among the different types of fortified yogurt, the one containing 1.5% RoRQF was the most preferred by the participants, followed by yogurt enriched with 1.5% RoWQF. Yogurt fortified with 1% RoRQF and yogurt enriched with WQF were also favored, though to a lesser extent. Yogurt with 1% RoWQF and 0.5% RoRQF came next in terms of preference, WQF and RQF, as well as the control sample.
Previous studies have suggested that adding QF to yogurt, whether made from goat or cow milk, can enhance its organoleptic properties. For instance, research by El-Shafei et al. (2020) demonstrated that products containing quinoa permeate extract received high overall acceptability across all levels of supplementation. Similarly, Mabrouk and Effat (2020) found that the incorporation of QF into set yogurt improved the product’s sensory quality, organoleptic profile, and nutritional value, which could increase consumer appeal. These findings are consistent with studies by Akkoyun and Arslan (2020), Alkobeisi et al. (2022), and Elslmawy et al. (2023), which indicated that the addition of QF positively affected yogurt stability and consumer acceptability. Furthermore, the inclusion of quinoa malt or powder in milk makes the beverage more nutritious and enhances its acceptability among a broader range of consumers.
Others studies examined the effect of dry roasting on the quality of QS found that dry roasting improved the sensory attributes and overall acceptability of the final product (Kheto et al., 2022; Sharma et al., 2022; Younis et al., 2024). This increased acceptability is likely due to the degradation of ant-nutrients such as phytates and saponins that remain in QS during the roasting process, as they are broken down under heat (Castro-Alba et al., 2019). This aligns with our hypothesis, which posited that roasting quinoa would enhance the sensory properties of the yogurt by reducing the undesirable goat milk flavor, improving the overall texture, and increasing consumer acceptability. This improvement in sensory properties opens up interesting prospects for the dairy industry by offering an innovation that meets the growing consumer demand for products that are both tasty, nutritious, and functional, benefiting from the richness of quinoa and the characteristics of GM while masking the undesirable goaty flavor.
3.4 Limitations of this study
Several limitations should be considered in this study, including potential variability in QF composition, which can affect the consistency and nutritional profile of the product. Additionally, fermentation conditions, such as temperature and time, can influence the final outcome, and any variations in these factors could lead to inconsistent results. Consumer acceptance testing is also subject to subjective preferences, with different demographics possibly reacting differently to the sensory characteristics of the product. Lastly, scaling up production could introduce new challenges that were not addressed in the study’s controlled conditions. Further research is needed to overcome these limitations.
4 Conclusion
In this study, we assessed the impact of adding QF to GMY production. The results showed that incorporating 1% QF significantly enhanced the yogurt’s macronutrient content, increasing protein content by 0.17 g/100 g and fat content by 0.09 g/100 g, compared to the control sample. This addition also led to a reduction in fermentation time to 4 h, indicating a more efficient fermentation process. Sensory evaluations revealed that QF improved yogurt texture and flavor, with RoQF further enhancing consumer acceptability by reducing the strong goat milk flavor. Additionally, QF improved the yogurt’s stability, reducing syneresis and enhancing both texture and shelf life. These findings fill a significant gap in the literature by demonstrating that QF, especially when roasted, can improve both the nutritional and sensory qualities of GMY, offering an innovative solution for the dairy industry to meet consumer demands for functional, clean-label products. This innovation presents new opportunities for the dairy sector by offering a nutritious, stable, and consumer-preferred product, strengthening its position in the evolving functional food market.
Data availability statement
The original contributions presented in the study are included in the article/supplementary material, further inquiries can be directed to the corresponding authors.
Author contributions
NA: Conceptualization, Methodology, Software, Writing – original draft. YZ-e: Conceptualization, Data curation, Methodology, Writing – original draft. SL: Data curation, Formal analysis, Methodology, Writing – original draft. OA: Data curation, Methodology, Writing – original draft. KE: Supervision, Validation, Visualization, Writing – review & editing. MBo: Data curation, Formal analysis, Investigation, Methodology, Writing – review & editing. RH: Formal analysis, Funding acquisition, Validation, Visualization, Writing – review & editing. AS: Funding acquisition, Investigation, Validation, Visualization, Writing – review & editing. MBe: Data curation, Software, Validation, Visualization, Writing – review & editing. FK: Supervision, Validation, Visualization, Writing – review & editing. KB: Investigation, Project administration, Supervision, Validation, Writing – review & editing.
Funding
The author(s) declare that financial support was received for the research and/or publication of this article. This research was funded by the Researchers Supporting Project number (RSPD2025R1057) at King Saud University, Riyadh, Saudi Arabia.
Acknowledgments
The authors are grateful to the Researchers for supporting Project Number (RSPD2025R1057) at King Saud University, Riyadh, Saudi Arabia. They also wish to express their sincere appreciation to the entire staff of the Agri-Food Technologies and Quality Laboratory, located at the ‘Qualipôle Alimentation de Béni-Mellal’ and affiliated with the National Institute of Agricultural Research (INRA).
Conflict of interest
The authors declare that the research was conducted in the absence of any commercial or financial relationships that could be construed as a potential conflict of interest.
Generative AI statement
The authors declare that no Gen AI was used in the creation of this manuscript.
Publisher’s note
All claims expressed in this article are solely those of the authors and do not necessarily represent those of their affiliated organizations, or those of the publisher, the editors and the reviewers. Any product that may be evaluated in this article, or claim that may be made by its manufacturer, is not guaranteed or endorsed by the publisher.
References
Abd Elmontaleb, H., and Abbas, K. (2022). The influence of quinoa flour addition on the physicochemical, antioxidant activity, textural, and sensory characteristics of UF-soft cheese during refrigerated storage. J. Food Process. Preserv. 45, 1–10. doi: 10.1111/jfpp.15494
Abugoch James, L. E. (2009). “Chapter 1 Quinoa (Chenopodium quinoa Willd.)” in Advances in food and nutrition research (Elsevier), 1–31. Available at: https://www.sciencedirect.com/science/article/abs/pii/S1043452609580011
Adetunji, C. O., Michael, O. S., Kadiri, O., Varma, A., Akram, M., Oloke, J. K., et al. (2021). “Quinoa: from farm to traditional healing, food application, and phytopharmacology” in Biology and biotechnology of quinoa (Singapore: Springer), 439–466. Available at: https://link.springer.com/
Ahmad, I., Hao, M., Li, Y., Zhang, J., Ding, Y., and Lyu, F. (2022). Fortification of yogurt with bioactive functional foods and ingredients and associated challenges – a review. Trends Food Sci. Technol. 129, 558–580. doi: 10.1016/j.tifs.2022.11.003
Akkoyun, Y., and Arslan, S. (2020). The impact of quinoa flour on some properties of ayran. Food Sci. Nutr. 8, 5410–5418. doi: 10.1002/fsn3.1832
Alia, O. A. E., Zine-Eddine, Y., Chaji, S., Souhassou, S., Kzaiber, F., Oussama, A., et al. (2023). Physico-chemical and sensory characterization of camel milk yogurt enriched with persimmon (Diospyros kaki) fruit. Acta Sci. Pol. Technol. Aliment. 22, 267–278. doi: 10.17306/J.AFS.1152
Alkobeisi, F., Varidi, M. J., Varidi, M., and Nooshkam, M. (2022). Quinoa flour as a skim milk powder replacer in concentrated yogurts: effect on their physicochemical, technological, and sensory properties. Food Sci. Nutr. 10, 1113–1125. doi: 10.1002/fsn3.2771
Balakrishnan, G., and Schneider, R. G. (2022). The role of Amaranth, quinoa, and millets for the development of healthy, sustainable food products—a concise review. Food Secur. 11:2442. doi: 10.3390/foods11162442
Barrantes, E., Tamime, A. Y., Sword, A. M., Muir, D. D., and Kaláb, M. (1996). The manufacture of set-type natural yoghurt containing different oils — 1. Compositional quality, microbiological evaluation and sensory properties. Int. Dairy J. 6, 811–826. doi: 10.1016/0958-6946(96)00009-X
Cais-Sokolińska, D., and Walkowiak-Tomczak, D. (2021). Consumer-perception, nutritional, and functional studies of a yogurt with restructured elderberry juice. J. Dairy Sci. 104, 1318–1335. doi: 10.3168/jds.2020-18770
Casarotti, S. N., Carneiro, B. M., and Penna, A. L. B. (2014). Evaluation of the effect of supplementing fermented milk with quinoa flour on probiotic activity. J. Dairy Sci. 97, 6027–6035. doi: 10.3168/jds.2014-8197
Castro-Alba, V., Lazarte, C. E., Perez-Rea, D., Sandberg, A.-S., Carlsson, N.-G., Almgren, A., et al. (2019). Effect of fermentation and dry roasting on the nutritional quality and sensory attributes of quinoa. Food Sci. Nutr. 7, 3902–3911. doi: 10.1002/fsn3.1247
Codină, G. G., Franciuc, S. G., and Mironeasa, S. (2016). Rheological characteristics and microstructure of milk yogurt as influenced by quinoa flour addition. J. Food Qual. 39, 559–566. doi: 10.1111/jfq.12210
Costa, M. P., Rosario, A. I. L. S., Silva, V. L. M., Vieira, C. P., and Conte-Junior, C. A. (2022). Rheological, physical and sensory evaluation of low-fat Cupuassu goat milk yogurts supplemented with fat replacer. Food Sci. Anim. Resour. 42, 210–224. doi: 10.5851/kosfa.2021.e64
Curti, C. A., Vidal, P. M., Curti, R. N., and Ramón, A. N. (2017). Chemical characterization, texture and consumer acceptability of yogurts supplemented with quinoa flour. Food Sci. Technol 37, 627–631. doi: 10.1590/1678-457x.27716
Dong, G., Dong, J., Zhu, Y., Shen, R., and Qu, L. (2021). Development of weaning food with prebiotic effects based on roasted or extruded quinoa and millet flour. J. Food Sci. 86, 1089–1096. doi: 10.1111/1750-3841.15616
El-Hakim, A., Ahmed, F., Mady, E., Abou Tahoun, A. M., Ghaly, M. S., and Eissa, M. A. (2022). Seed quality and protein classification of some quinoa varieties. J. Ecol. Eng. 23, 24–33. doi: 10.12911/22998993/143866
El-Menawy, R. K., Mohamed, D. M., Ismail, M. M., and Hassan, A. M. (2023). Optimal combination of cow and quinoa milk for manufacturing of functional fermented milk with high levels of antioxidant, essential amino acids and probiotics. Sci. Rep. 13:20638. doi: 10.1038/s41598-023-47839-6
El-Shafei, S. M. S., Sakr, S. S., and Abou-Soliman, N. H. I. (2020). The impact of supplementing goats’ milk with quinoa extract on some properties of yoghurt. Int. J. Dairy Tech. 73, 126–133. doi: 10.1111/1471-0307.12628
Elslmawy, K. K., Hassanin, A. M., and Omar, M. A. (2023). Production of Greek yoghurt with quinoa flour. Al-Azhar J. Agric. Res. 48, 551–563.
Fernández-García, N., Román-García, I., and Olmos, E. (2023). The outlook for Latin-American crops. Latin-American Seeds: Agronomic, Processing and Health Aspects, 91–118. doi: 10.1201/9781003088424-3
Guardianelli, L. M., Salinas, M. V., Brites, C., and Puppo, M. C. (2022). Germination of white and red quinoa seeds: improvement of nutritional and functional quality of flours. Food Secur. 11:3272. doi: 10.3390/foods11203272
Höhl, K., and Busch-Stockfisch, M. (2015). The influence of sensory training on taste sensitivity. Effects on sweet and bitter perception over a half-year period. Ernahrungs Umschau 62, 208–215. doi: 10.4455/eu.2015.035
Ismail, H. A., and Rayan, A. M. (2022). Preparation and evaluation of quinoa-Kishk as a novel functional fermented dairy product. J. Food Sci. Technol. 59, 1063–1074. doi: 10.1007/s13197-021-05110-8
Kheto, A., Joseph, D., Islam, M., Dhua, S., Das, R., Kumar, Y., et al. (2022). Microwave roasting induced structural, morphological, antioxidant and functional attributes of quinoa (Chenopodium quinoa Willd). J. Food Process. Preserv. 46:e16595. doi: 10.1111/jfpp.16595
Li, L., Lietz, G., and Seal, C. J. (2021). Phenolic, apparent antioxidant and nutritional composition of quinoa (Chenopodium quinoa Willd.) seeds. Int. J. Food Sci. Technol. 56, 3245–3254. doi: 10.1111/ijfs.14962
Mabrouk, A., and Effat, B. (2020). Production of high nutritional set yoghurt fortified with quinoa flour and probiotics. doi: 10.22161/ijeab.56.16
Navruz-Varli, S., and Sanlier, N. (2016). Nutritional and health benefits of quinoa (Chenopodium quinoa Willd.). J. Cereal Sci. 69, 371–376. doi: 10.1016/j.jcs.2016.05.004
Nayik, G. A., Jagdale, Y. D., Gaikwad, S. A., Devkatte, A. N., Dar, A. H., and Ansari, M. J. (2022). Nutritional profile, processing and potential products: a comparative review of goat Milk. Dairy 3, 622–647. doi: 10.3390/dairy3030044
Ningtyas, C. K., and Haskito, A. E. P. (2020). Comparison of acceptability analysis of goat milk yogurt fortification with various rice bran flour, in: IOP conference series: Earth and environmental science. Institute of Physics Publishing. doi: 10.1088/1755-1315/465/1/012012, 465, 012012
Paez, V., Barrett, W. B., Deng, X., Diaz-Amigo, C., Fiedler, K., Fuerer, C., et al. (2016). AOAC SMPR® 2016.002. J. AOAC Int. 99, 1122–1124. doi: 10.5740/jaoacint.SMPR2016.002
Pappa, E. C., Kondyli, E., Pappas, A. C., Kyriakaki, P., Zoidis, E., Papalamprou, L., et al. (2024). Physicochemical characteristics of commercially available Greek yoghurts. Dairy 5, 436–450. doi: 10.3390/dairy5030034
Paszczyk, B., and Tońska, E. (2025). Influence of plant additives on changes in the composition of fatty acids, lipid quality indices and minerals of fermented dairy products from cow’s milk. Molecules 30:235. doi: 10.3390/molecules30020235
Rafiq, Z. (2021). Potential use of quinoa for yoghurt preparation. Agric. Sci. J. 2, 9–18. doi: 10.56520/asj.v2i2.46
Repo-Carrasco-Valencia, R. A., Encina, C. R., Binaghi, M. J., Greco, C. B., and Ronayne De Ferrer, P. A. (2010). Effects of roasting and boiling of quinoa, kiwicha and kañiwa on composition and availability of minerals in vitro. J. Sci. Food Agric. 90, 2068–2073. doi: 10.1002/jsfa.4053
Saeed, M., Ali, S. W., and Ramzan, S. (2021). Physicochemical analysis of mango flavored yogurt supplemented with moringa oleifera leaf powder. J. Food Sci. Technol. 58, 4805–4814. doi: 10.1007/s13197-021-05146-w
Sekhavatizadeh, S. S., Hosseinzadeh, S., and Mohebbi, G. (2021). Nutritional, antioxidant properties and polyphenol content of quinoa (Chenopodium quinoa willd) cultivated in Iran. Future Food J. Food Agric. Soc. 9:20210514665. doi: 10.17170/kobra-202102163256
Sharma, S., Kataria, A., and Singh, B. (2022). Effect of thermal processing on the bioactive compounds, antioxidative, antinutritional and functional characteristics of quinoa (Chenopodium quinoa). LWT 160:113256. doi: 10.1016/j.lwt.2022.113256
Valcárcel-Yamani, B., Lannes, S., and Da, S. (2012). Applications of quinoa (Chenopodium quinoa Willd.) and amaranth (Amaranthus spp.) and their influence in the nutritional value of cereal based foods.
Yang, Y., Zhang, R., Zhang, F., Wang, B., and Liu, Y. (2023). Storage stability of texture, organoleptic, and biological properties of goat milk yogurt fermented with probiotic bacteria. Front. Nutr. 9:1093654. doi: 10.3389/fnut.2022.1093654
Younis, M., Ahmed, I. A. M., AlJuhaimi, F., Wang, D., Uslu, N., and Özcan, M. M. (2024). Effect of heating and germination on bioactive properties, phenolic compounds, fatty acid and mineral contents of quinoa (Chenopodium quinoa Willd.) seeds. Int. J. Food Sci. Technol. 59, 4765–4773. doi: 10.1111/ijfs.17204
Zhang, Y., Bai, B., Yan, Y., Liang, J., and Guan, X. (2022). Bound polyphenols from red quinoa prevailed over free polyphenols in reducing postprandial blood glucose rises by inhibiting α-glucosidase activity and starch digestion. Nutrients 14:728. doi: 10.3390/nu14040728
Zhang, X., Ding, Y., Zhou, J., Xu, Q., Li, T., and Wang, L. (2024). The influence of protein components on quinoa protein-xanthan gum complex gels at different pH levels. Gels 10:840. doi: 10.3390/gels10120840
Zine-Eddine, Y., Kzaiber, F., Oussama, A., and Boutoial, K. (2022). Physicochemical and sensorial characterisation of marinated white cheese made from goat milk with olive oil and thyme. Acta Sci. Pol. Technol. Aliment. 21, 401–409. doi: 10.17306/J.AFS.1086
Zine-eddine, Y., Zinelabidine, L. H., Kzaiber, F., Oussama, A., and Boutoial, K. (2021a). The use of paired comparison test on the goat’s milk adulteration detection (whole and semiskimmed). J. Sens. Stud. 36:e12690. doi: 10.1111/joss.12690
Keywords: physicochemical properties, goat milk, quinoa flour, sensory evaluation, yogurt
Citation: Ajbli N, Zine-eddine Y, Laaraj S, Ait El Alia O, Elfazazi K, Bouhrim M, Herqash RN, Shahat AA, Benbati M, Kzaiber F and Boutoial K (2025) Effect of quinoa flour on fermentation, physicochemical and sensory properties of goat milk yogurt. Front. Sustain. Food Syst. 9:1561991. doi: 10.3389/fsufs.2025.1561991
Edited by:
Kathleen L. Hefferon, Cornell University, United StatesReviewed by:
Muthukumar Serva Peddha, Central Food Technological Research Institute (CSIR), IndiaMalgorzata Ziarno, Warsaw University of Life Sciences, Poland
Copyright © 2025 Ajbli, Zine-eddine, Laaraj, Ait El Alia, Elfazazi, Bouhrim, Herqash, Shahat, Benbati, Kzaiber and Boutoial. This is an open-access article distributed under the terms of the Creative Commons Attribution License (CC BY). The use, distribution or reproduction in other forums is permitted, provided the original author(s) and the copyright owner(s) are credited and that the original publication in this journal is cited, in accordance with accepted academic practice. No use, distribution or reproduction is permitted which does not comply with these terms.
*Correspondence: Khalid Boutoial, a2hhbGlkLmJvdXRvaWFsQHVzbXMubWE=; Salah Laaraj, c2FsYWgubGFhcmFqQHVzbXMubWE=