- 1Facultad Barberi de Ingeniería, Diseño y Ciencias Aplicadas, Escuela de Ciencias Aplicadas e Industria Sostenible, Departamento de Ciencias Farmacéuticas y Químicas, Universidad Icesi, Cali, Valle del Cauca, Colombia
- 2Corporación Colombiana de Investigación Agropecuaria AGROSAVIA, C.I. La Selva, Rionegro, Antioquia, Colombia
- 3BioInc. Center for Biomass Valorization Research, Universidad Icesi, Cali, Valle del Cauca, Colombia
Capsicum species are widely cultivated yet their full industrial potential remains underexplored due to limited characterization of their physicochemical and phytochemical descriptors. In this study, we comprehensively evaluated 284 Capsicum accessions to address this gap and assess their suitability for industrial applications across food, pharmaceutical, and cosmetic sectors. Key quality descriptors—such as capsaicinoids, soluble solids, carotenoids, color index, pH, and texture—were measured and statistical clustering methods, including principal component analysis and k-means clustering, were employed to group accessions based on these traits. The analysis revealed four distinct genotype groups and identified nine standout genotypes with superior attributes; for instance, some accessions exhibited high capsaicinoid content (up to 6,062 mg/kg), elevated soluble solids (up to 17 °Brix), and remarkable carotenoid levels (up to 39.54 mg/g), meeting critical market demands. These findings provide a robust foundation for targeted breeding strategies and underscore the versatility of Capsicum as a valuable genetic resource for sustainable industrial innovation and conservation.
1 Introduction
The Capsicum genus, originating in the Amazon basin, is among the world’s oldest domesticated crops, with archeological evidence tracing its use back nearly 7,000 years. Despite this extensive history, much of its genetic diversity remains underutilized. While Capsicum annuum, Capsicum frutescens, and Capsicum chinense are the most widely cultivated species, numerous wild and underutilized genotypes continue to thrive across the diverse ecosystems of the Americas. The Andean region, particularly Colombia, stands out as a center of Capsicum diversity, where the complex topography of the Andes has driven the evolution of various species and ecotypes. Institutions such as Agrosavia have made significant strides in preserving this genetic legacy. Capsicum genus potential remains unexplored since the influence of Andean topography in shaping diversity as a key role in speciation events (Khoury et al., 2020).
Like its characteristic pungency, the nutritional profile of the Capsicum genus is remarkably diverse, providing essential vitamins, folates, proteins, minerals, lipids, and carbohydrates. It has even been proposed as a superfood due to its potential to address nutrient deficiencies by contributing significantly to the recommended daily intake (Kantar et al., 2016). Extracts from Capsicum fruits are widely consumed and consist of carotenoid pigments, neutral lipid, and other fatty compounds including tocopherols, and capsaicinoids responsible for their heat and widely recognized sensory properties. Capsanthin and capsorubin are the predominant carotenoids responsible for the intense red coloration, becoming esterified with fatty acids during the advanced stages of fruit ripening. However, smaller quantities of free carotenes and other xanthophylls can also be detected. The concentration of carotenoids, including free, mono-, and di-esterified forms, can vary significantly among red fruits (Penagos-Calvete et al., 2019).
The Capsicum genus harbors a rich diversity of species, from the fiery C. chinense to the sweet C. annuum, each exhibit genetic variability reflected in their chemical composition and organoleptic properties. Although chemotaxonomic differentiation within the genus has been challenging due to its great diversity, interest in its bioactive metabolites has grown significantly. These compounds, with antioxidant, anti-inflammatory, and antimicrobial properties, have opened doors to numerous applications in the food, pharma, and cosmetic industries providing natural alternatives to synthetic additives (Espichán et al., 2022; Hernández-Pérez et al., 2020; Sanati et al., 2018; Tripodi et al., 2021; Zewdie and Bosland, 2001).
Moreover, the industrial utilization of Capsicum is heavily influenced by social and cultural factors that shape consumer preferences and market demand. In regions with a tradition of spicy cuisines, there is a greater emphasis on hotter varieties, whereas areas favoring milder flavors tend to cultivate sweeter types. Understanding these socio-cultural dynamics is crucial for developing targeted breeding programs and effective marketing strategies (Aguilar-Meléndez et al., 2021; Catalfamo et al., 2022; Guevara et al., 2021; Rezazadeh et al., 2023; Sánchez Toledano et al., 2023).
In the quest to produce high-value foods and bioproducts for the global population, the Capsicum genus represents unprecedented opportunities in Andean countries to strengthen the economies of small-scale farmers through the biotransformation of differentiated materials with enhanced agronomic value. Plant breeding programs worldwide have generated extensive variation in fruit color, shape, size, and disease resistance, with increasing focus on phytochemical content as a quality descriptor (Lozada et al., 2022). This study explores the discovery process of the significant agronomic potential within publicly preserved Capsicum germplasm from Agrosavia-Colombia, using selected quality descriptors and physicochemical traits. Although Capsicum is a widely cultivated crop, certain wild pepper genotypes native to the Americas still exhibit highly valuable traits compared to those currently available on the market.
2 Materials and methods
2.1 Materials
HPLC-grade methanol, reagent-grade methanol and sodium hypochlorite were obtained from Merck (Darmstadt, Germany). Ethyl acetate, dichloromethane and formic acid was acquired from Sigma-Aldrich (St. Lous, MO, United States). Butylated hydroxytoluene (BHT) USP grade was obtained from Anmol chemicals (New York, United States).
2.2 Plant material
A total of 284 Capsicum accessions from the Agrosavia germplasm collection were evaluated. These accessions represent a diverse array of genetic backgrounds, preserved to maintain the full spectrum of Capsicum diversity for research and breeding purposes.
2.3 Pepper cultivation and fruit selection
The accessions were cultivated under controlled greenhouse conditions at the La Selva research center in Rionegro, Antioquia. For each genotype, seven plants were grown in a single row, with the terminal plants serving as microenvironmental controls to ensure representative sampling from the five central plants. Soil preparation included plowing, bed formation, amendment application, installation of a fertigation system, and agromulch placement, while monthly fertilization supplied essential macro- and micronutrients (see Figure 1). Daily drip irrigation for 15 min maintained optimal soil moisture. Fruits were harvested at their peak ripening stage during the first two production cycles and stored at −20°C until further analysis. Out of the 284 accessions, 233 produced fruits with an average weight exceeding 1 g. Additionally, accessions exhibiting atypical fruit characteristics, such as variations in color or shape, were classified as separate to account for intra-genotypic variability.
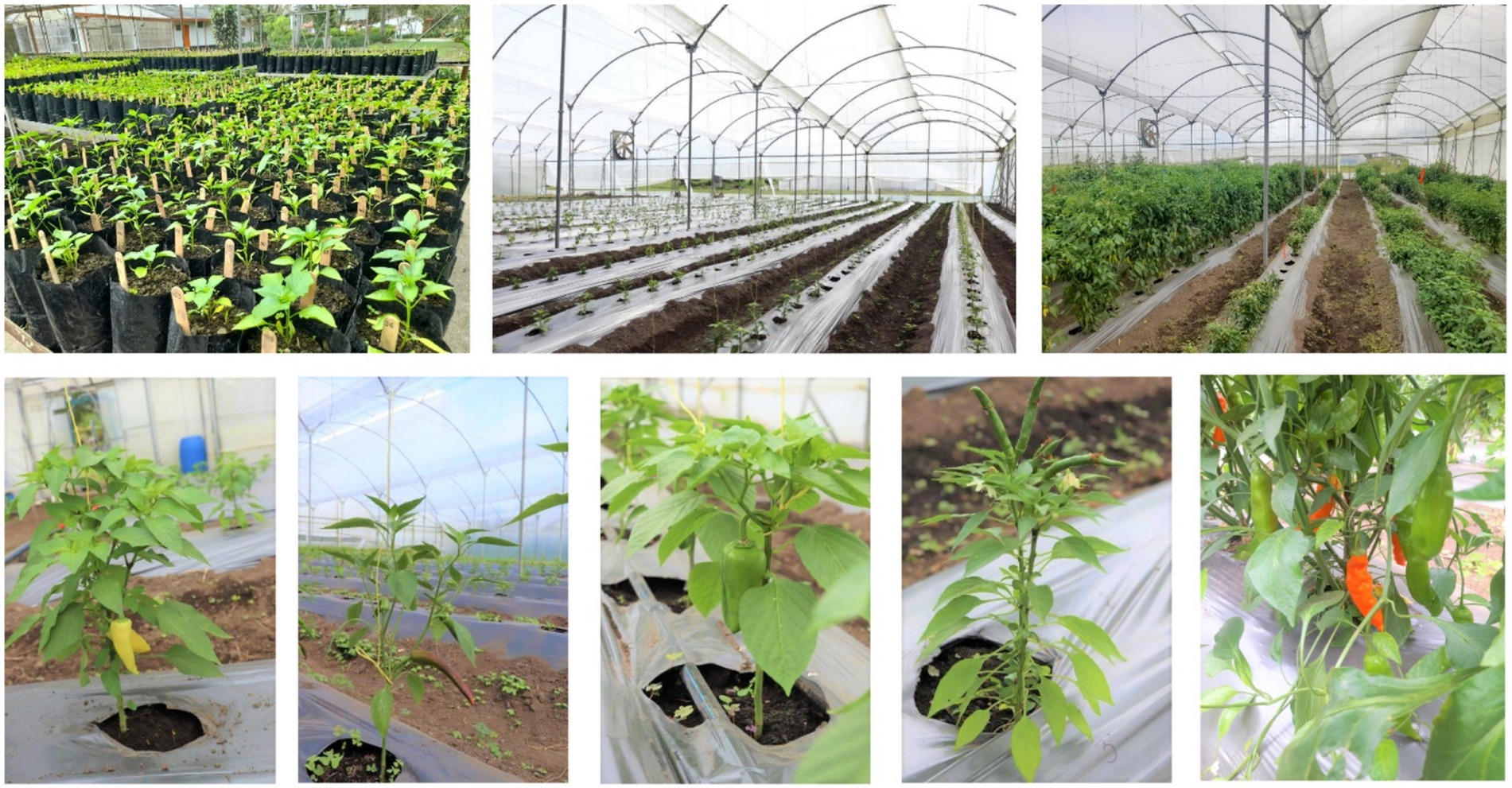
Figure 1. Greenhouse facilities for the cultivation and maintenance of Capsicum germplasm at Agrosavia, Rionegro-Antioquia. The collection is managed under stringent phytosanitary regulations to ensure its preservation and compliance with biosafety standards.
2.4 Fruit’s physicochemical analysis
For each evaluated accession, a minimum sample of 250 g was collected and disinfected using a 150-ppm sodium hypochlorite solution for 3 min. Pedicels were then removed, and the fruits were blended for 5 min to produce a chili paste. A calibrated pH meter was used with pH 4, 7, and 10 buffer solutions. A digital refractometer was used with distilled water as a reference blank for soluble solids (Brix). Total solids content was adapted from AOAC 934 method (AOAC, 1998) determined by measuring the weight loss of a 2-g sample after drying in a convection oven at 105°C for 2 h. Using the method proposed by Suttisansanee (Suttisansanee et al., 2023), a ColorFlex EZ spectrophotometer (Hunter Associates Laboratory, Resto, VA, USA) was employed to determine color spectrum analysis using the CIELab and CIELCh scales for lightness (L), red-green coordinates (a), yellow-blue coordinates (b), chroma (C), and hue (h) using black and white calibration standards. A TA-XT plus texture analyzer (Stable Micro Systems Ltd., UK) equipped with a 35 mm back-extrusion ring was used to determine firmness (Firm), consistency (Con), cohesiveness (Coh), and viscosity index (Wcoh). The test parameters included a trigger value of 5 g and speeds of 1, 5, and 10 mm/s for pre-test, test, and post-test, respectively, and a height of 20 mm, following the approach proposed by Sert et al. (2017).
2.5 Capsaicinoids-high-performance liquid chromatography (HPLC)
Two hundred milligrams of powder chili paste were extracted with reagent-grade methanol at a 1:10 (w/v) ratio. The mixture was vortexed for 3 min, sonicated at 45°C for 20 min, and subsequently allowed to settle for 3 min. The supernatant was filtered through a pre-activated Oasis HLB 1 mL solid-phase extraction cartridge (Waters Corporation, Milford, MA, USA) using methanol as the elution solvent. The resulting extract was stored under appropriate conditions until further analysis.
A Hitachi LaChrome Ultra high-performance liquid chromatography (HPLC) system was employed for analysis, featuring a binary pump, inline degasser, autosampler, and a thermostated column compartment. Chromatographic separation was performed on a Luna® C18 column (150 mm × 4.6 mm, 5 μm particle size, Phenomenex Inc., Torrance, CA, USA) at 40°C, with an injection volume of 10 μL. Type I water acidified with 0.1% formic acid was used as mobile phase A, and HPLC-grade MeOH was used as mobile phase B. The gradient for mobile phase A began at 23% at time 0, decreased to 18.5% by 7 min, further reduced to 10% at 8.5 min, then dropped to 7% at 12 min, and reached 2% at 14 min. Detection was carried out at 280 nm, and quantification was based on a standard curve of capsaicin and dihydrocapsaicin. The method was adapted and modified from Penagos-Calvete et al. (2019). Data processing was performed using EZ-Chrome Elite software.
2.6 Total carotenoid content analysis
For carotenoid extraction, 100 mg of red powder paste or 250 mg of yellow powder paste were extracted with 5 mL of an ethyl acetate:dichloromethane solution (8:2, v/v) containing 0.1% butylated hydroxytoluene (BHT). The mixture was vortexed for 3 min, followed by sonication for 30 min at 40°C. The resulting extract was filtered, evaporated to dryness, and reconstituted in 5 mL of HPLC-grade methanol. A 200 μL aliquot of the reconstituted solution was transferred to a microplate well, and carotenoid quantification was performed at 465 nm using a standard curve prepared with a work standard.
2.7 Desirability index calculation
Desirability indices were calculated to identify genotypes suitable for specific industrial applications, including the pharmaceutical, raw consumption, and food and cosmetics (F&C) sectors. Physicochemical and chemical variables were normalized to a 0–1 scale using min-max scaling, and weights were assigned to variables based on their relevance to each sector. Genotypes with the highest capsaicinoid content were prioritized for the pharmaceutical sector due to their bioactive properties. For raw consumption, genotypes with elevated soluble solids content were preferred, while for the F&C sector, genotypes exhibiting the highest carotenoid content and low capsaicinoid levels (Equation 1) were selected to align with industry demands and consumer preferences.
Desirability index for F&C sector where x is carotenoid content (mg/g) and y capsaicinoid content (mg/kg).
2.8 Statistical analysis
All data were processed and analyzed using R software (R Core Team, 2023) and EZChrom Elite version 3.3. Experiments were carried out in triplicate (n = 3), and the results were expressed as mean ± standard deviation (SD). To assess differences between species, a pairs wise Game-Howell test was conducted (p < 0.05). Spearman’s correlation analysis (p < 0.01) was used to identify relationships among traits. Furthermore, principal component analysis and k-means clustering were performed on pH, total solids, color index, firmness, consistency, carotenoids, and capsaicinoids to elucidate the underlying structure of the dataset and to identify homogeneous phenotypic groups. Finally, desirability indices were also evaluated using pairwise Game-Howell test (p < 0.05). Additionally, a heatmap was generated (using the heatmap package in R) to visualize cluster-level variations in desirability across industrial sectors, with color intensities reflecting the relative performance of each cluster in pharmaceutical, fresh consumption, and food and cosmetic applications.
3 Results
3.1 Pepper cultivation and fruit selection
A total of 233 Capsicum spp. accessions, representing five species (C. annuum, C. baccatum, C. chinense, C. frutescens, and C. pubescens), were evaluated, revealing significant phenotypic variability (Figures 2, 3). Typically, Capsicum crops initiate fruit production approximately 3 months after sowing, with harvests occurring at weekly or biweekly intervals. While some accessions exhibited continuous fruiting, others, such as accession 12, displayed intermittent production cycles. Notably, certain accessions achieved up to 11 harvests within a three-month period, highlighting the diversity in reproductive dynamics across the evaluated germplasm.
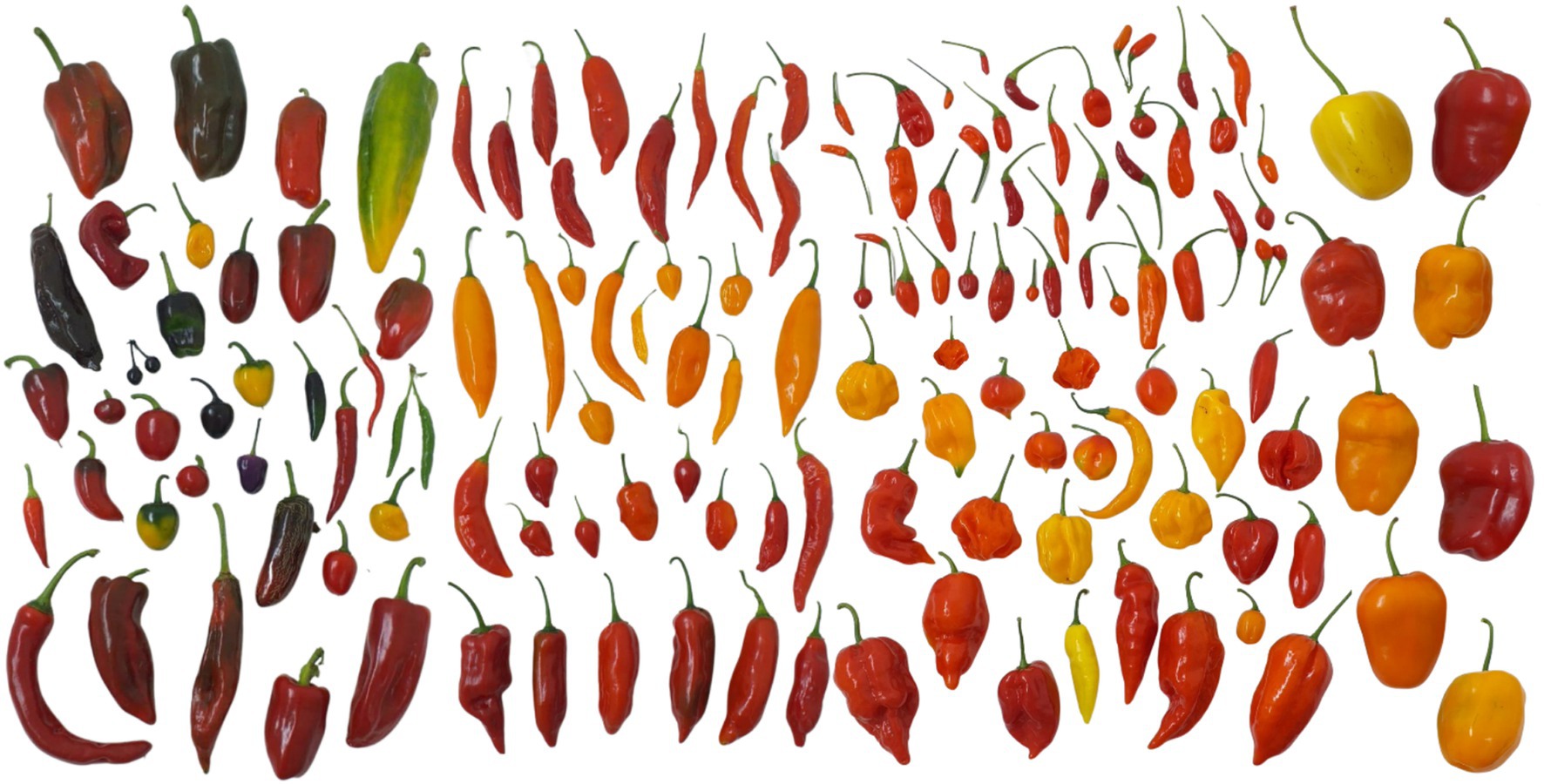
Figure 2. Representative fruits from the Colombian Capsicum germplasm collection preserved by Agrosavia at the La Selva research center (Rionegro, Antioquia). These original images represents a significant genetic resource developed through collaborative efforts under the Copyright Program for Bioeconomy (Contract 451-2021), involving Universidad Icesi, Hugo Restrepo & Cía., and Agrosavia.
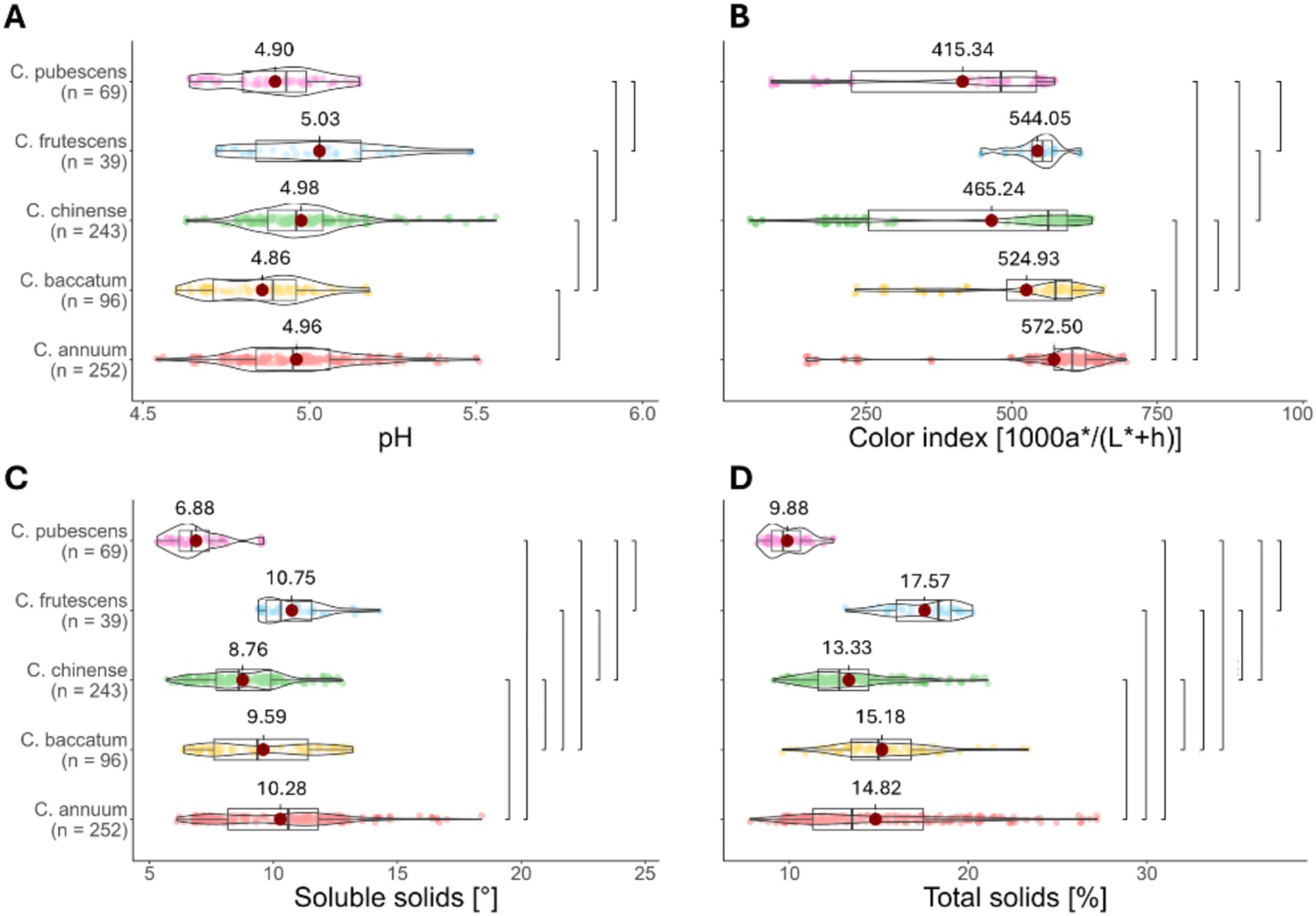
Figure 3. Distribution of physicochemical parameters across Capsicum species. pH (A). Color index (1000a/(L + h)) (B). Soluble solids (C). Total solids (D). Red dots represent median values, and significant comparisons are marked with brackets (p < 0.05).
3.2 Physicochemical and chemical traits
No clear differentiation in pH was observed among species, with median values ranging from 4.9 to 5.0. However, C. baccatum exhibited the lowest median, while certain C. annuum genotypes showed the lowest recorded pH values. For color index, C. pubescens had the lowest median (481), while C. annuum displayed the highest (603). In terms of soluble and total solids, C. pubescens exhibited the lowest median values (6.7 and 9.6, respectively), while C. frutescens showed the highest median for total solids (18.3) (Figure 3). Texture parameters followed a similar pattern, with C. pubescens showing the lowest values, and C. frutescens exhibiting the highest. Species ranked in terms of median texture values from lowest to highest as follows: C. pubescens, C. annuum, C. baccatum, C. chinense, and C. frutescens. Notably, significant outliers were observed among C. annuum, C. baccatum, and C. chinense (Figure 4).
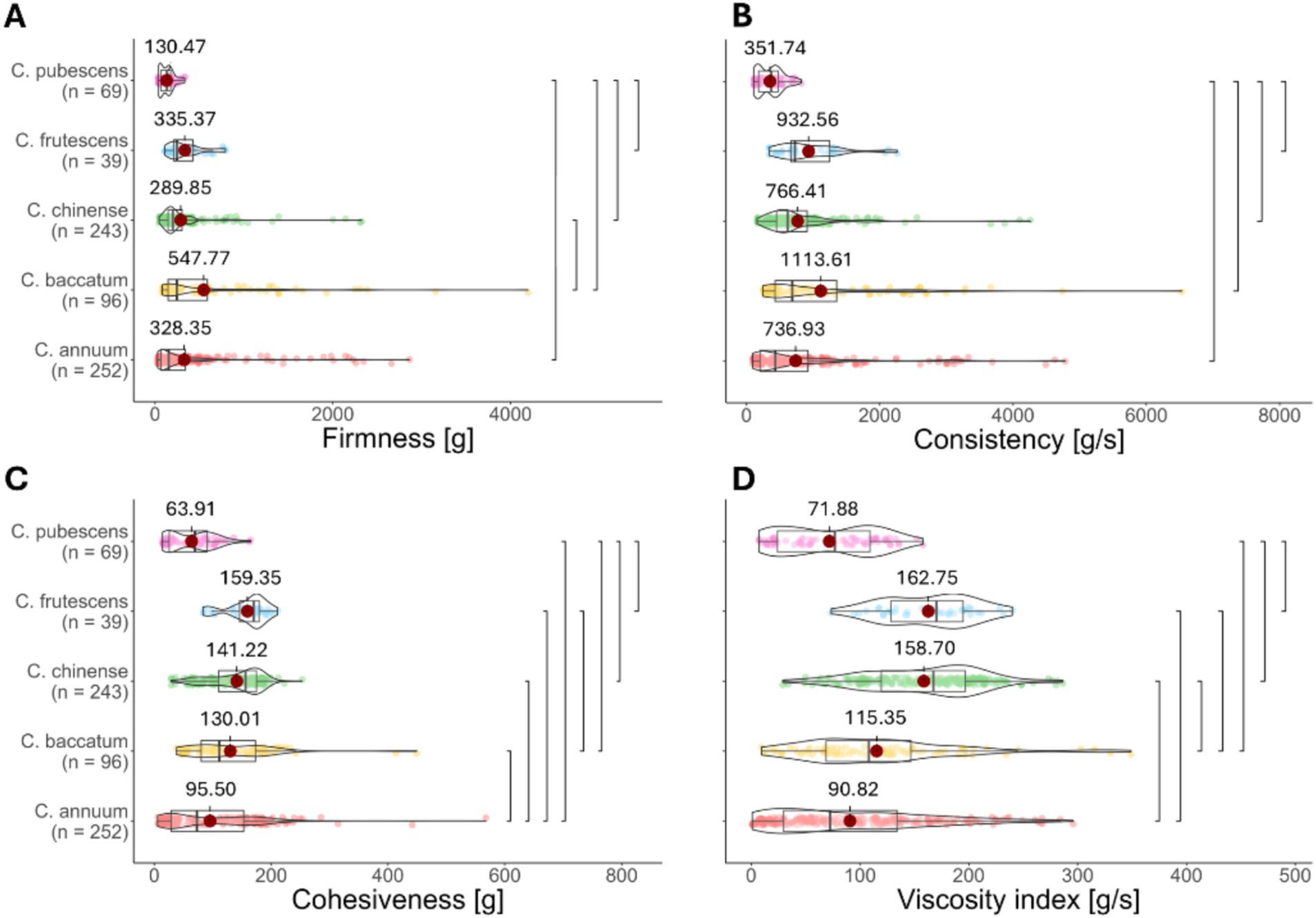
Figure 4. Distribution of texture parameters across Capsicum species. Firmness (A). Consistency (B). Cohesiveness (C). Viscosity index (D). Red dots represent median values, and significant comparisons are marked with brackets (p < 0.05).
Carotenoid content was lowest in C. pubescens, with a median of 3.34 mg/g, while C. annuum exhibited the highest median at 12.46 mg/g and a maximum of 44.72 mg/g, highlighting an exceptionally high-content accession. For capsaicinoids, C. annuum recorded the lowest median at 41.39 mg/kg, whereas C. frutescens displayed the highest median at 1173.98 mg/kg. The highest median capsaicin content was found in C. chinense (6117.96 mg/kg), while C. frutescens had the highest dihydrocapsaicin median (335.22 mg/kg), followed by C. pubescens (308.23 mg/kg), C. baccatum (100.38 mg/kg), C. chinense (83.45 mg/kg), and C. annuum (0 mg/kg). Notable outliers in capsaicinoid content were observed in C. chinense, with a peak of 6,897.59 mg/kg (Figure 5).
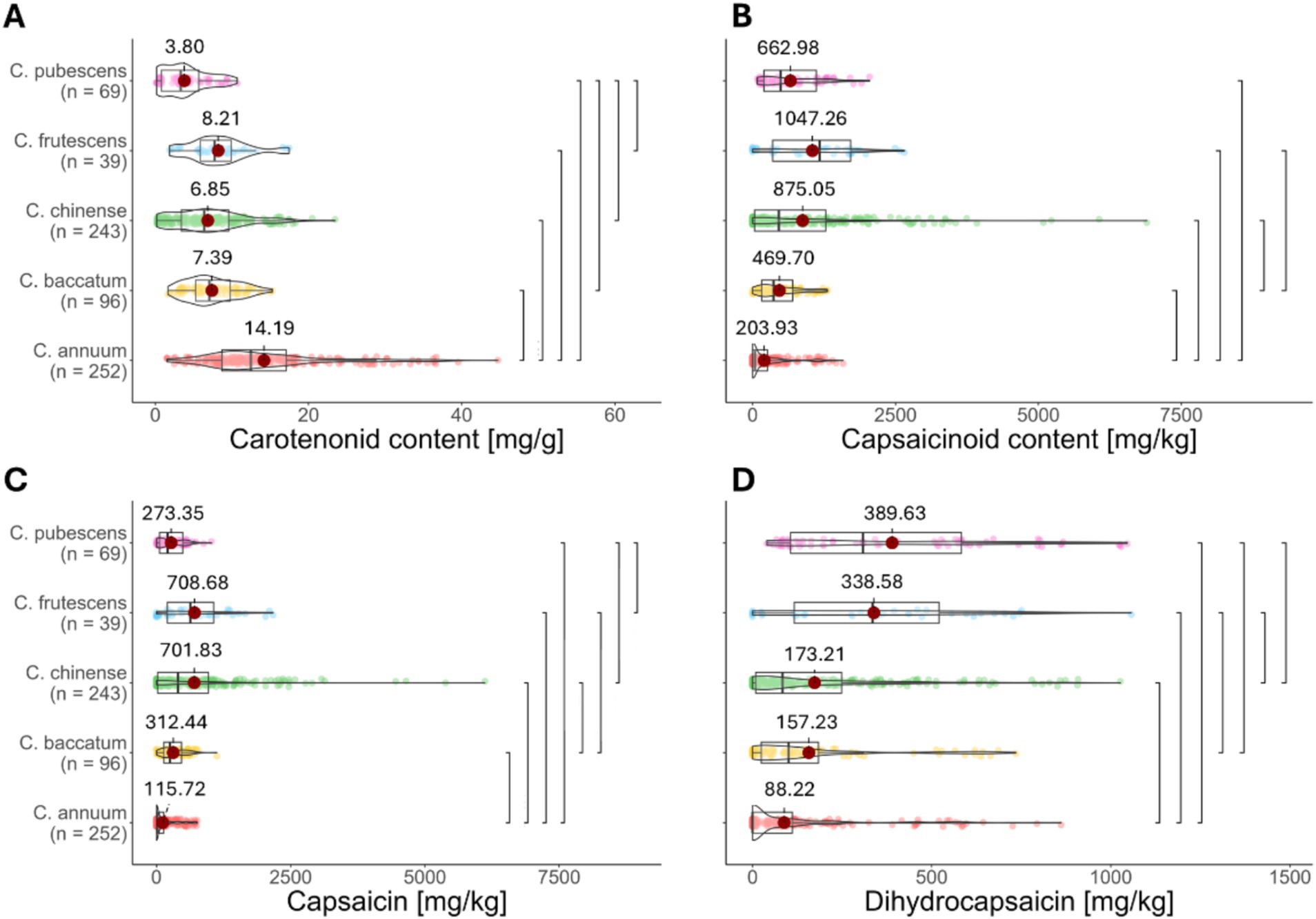
Figure 5. Distribution of chemical parameters across Capsicum species. Carotenoid content (A). Capsaicinoid content (B). Capsaicin (C). Dihydrocapsaicin (D). Red dots represent median values, and significant comparisons are marked with brackets (p < 0.05).
3.3 Multivariate analysis
Spearman’s correlation analysis (Figure 6) revealed strong correlations among color spectrum variables, with lightness showing significant relationships with texture parameters (r ≥ 0.15, p = 0.01) and capsaicin content (r = 0.3, p = 0.01). An inverse correlation was observed between carotenoid and capsaicinoid content (r = −0.27, p = 0.01), while carotenoid content correlated positively with soluble solids (r = 0.18, p = 0.01).
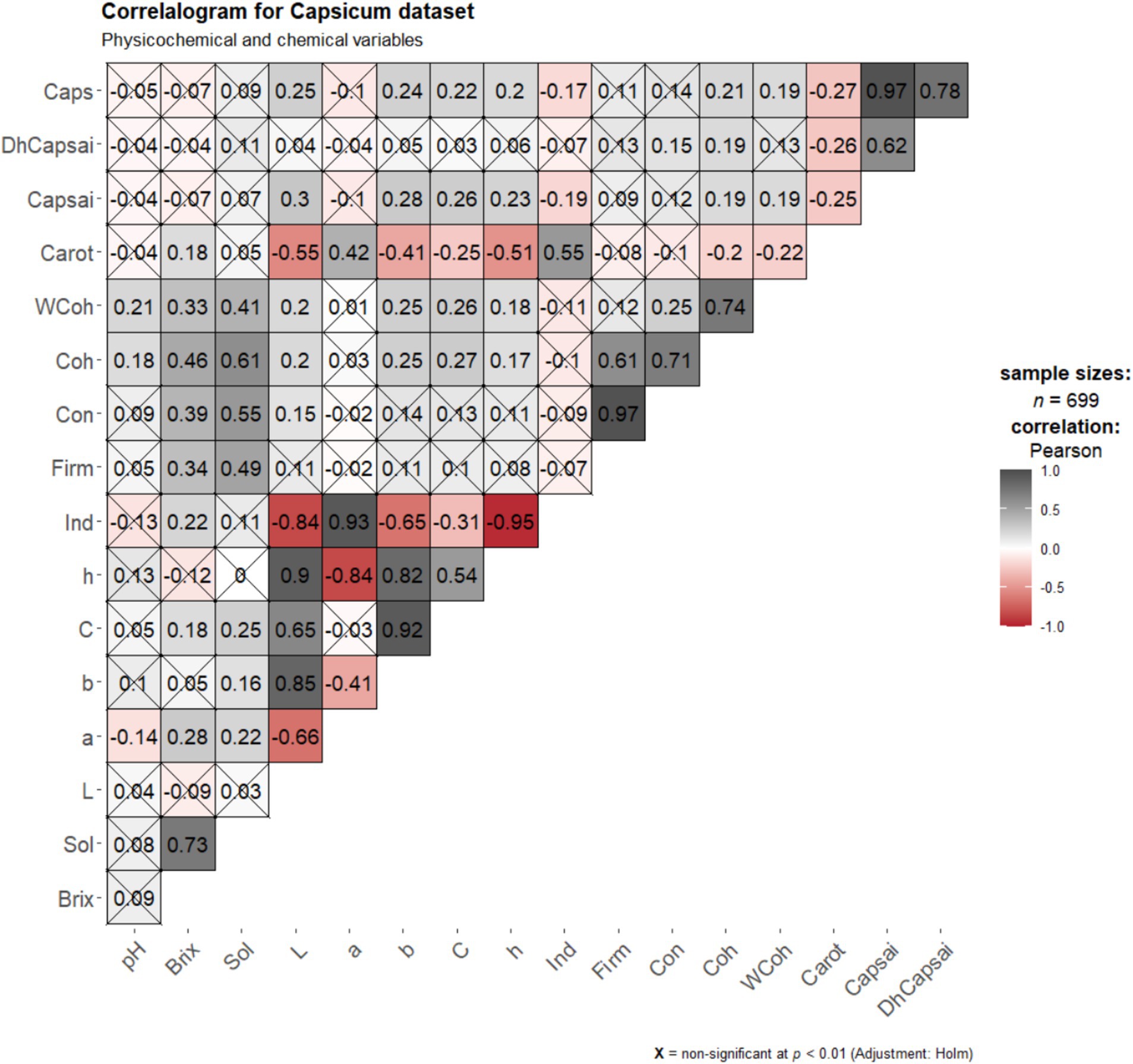
Figure 6. Pearson correlations among 14 variables related to Capsicum spp. traits. Significant correlations (p < 0.01, Holm adjustment) are displayed without a cross (×).
Due to these dependencies, PCA and k-means clustering were performed on individual values (not accession means) for pH, total solids, color index, firmness, consistency, carotenoids, and capsaicinoids to better understand the dataset’s underlying structure. Three principal components (PCs) with eigenvalues greater than 1 were identified (Table 1), accounting for 71.14% of the total phenotypic variance in the germplasm. In PC1, total solids, firmness, and cohesiveness explain 83.04% of the variation. The color index and carotenoids explain 74.1% of the variation in PC2, while pH and capsaicinoids explain 96.42% in PC3. PC1 explains a substantial portion of the variance, with 32.57%, driven primarily by cohesiveness and solids. PC2 accounts for 23.57% of the variation, with color index and carotenoids as main contributors. Finally, PC3 explains 15% of the variation, primarily associated with pH (Figure 7B).
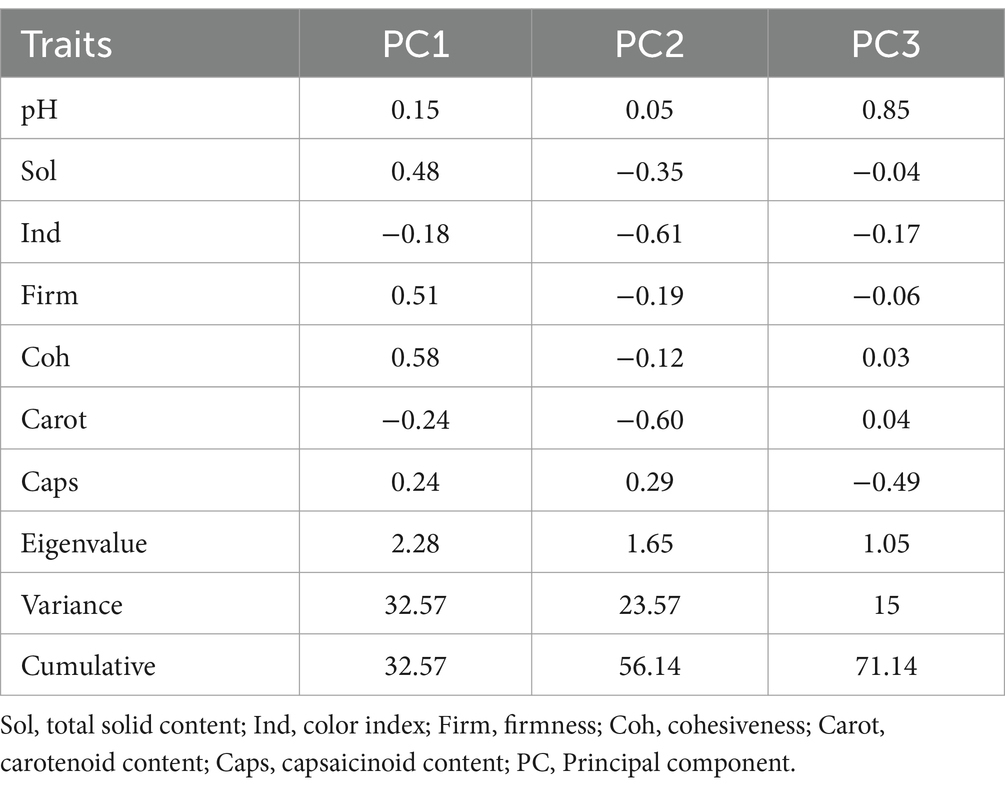
Table 1. Principal component analysis showing the contributions of each trait to the variation in the germplasm.
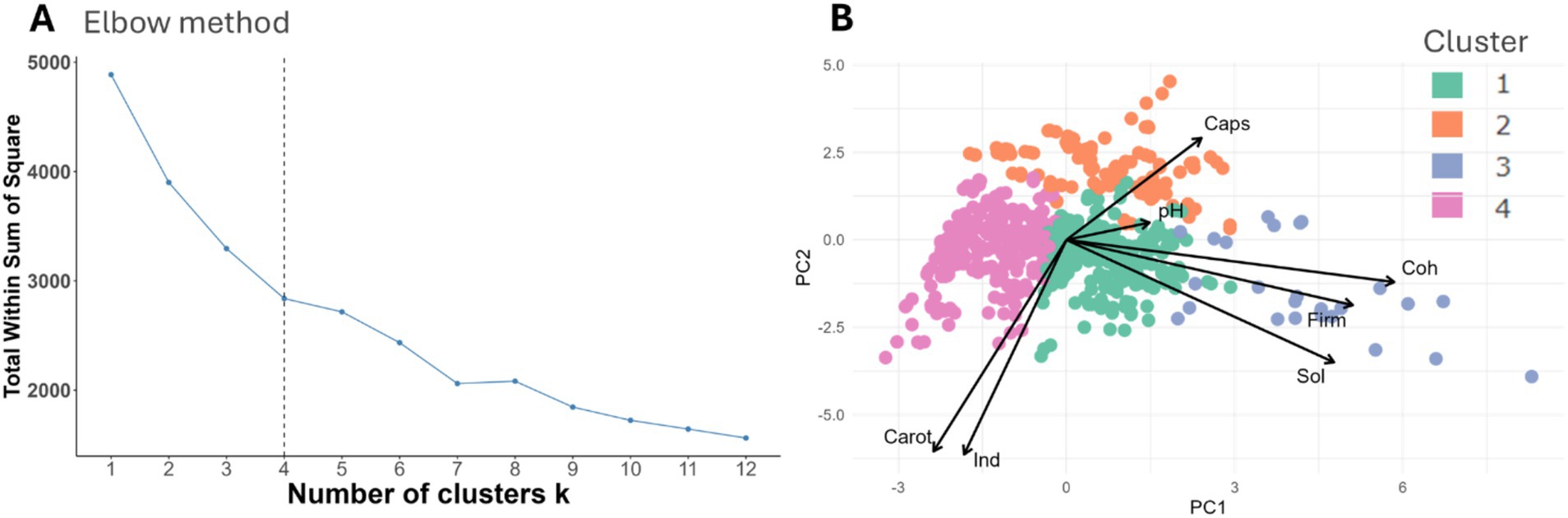
Figure 7. Clustering and PCA analysis of Capsicum spp. accessions. Optimal cluster by elbow method (A). PCA 2D plot showing clustering accessions with vectors representing the contributions of key traits in PC1 and PC2 (B).
Cluster analysis identified four distinct groups (Figure 7A) using the k-means algorithm by elbow method, an unsupervised machine learning technique for identifying patterns in multidimensional data. Cluster I, with 272 samples, was characterized by average values across most traits and consisted of 40% C. chinense, 30% C. annuum, 16% C. baccatum, and 13% C. frutescens. Cluster II, comprising 119 samples, was distinguished by a lower color index and reduced carotenoid content, with 57% C. chinense and 20% C. pubescens. Cluster III, the smallest with 26 samples, showed high total solids content and stronger textural properties, including 54% C. annuum, 31% C. baccatum, and 15% C. chinense. Lastly, Cluster IV, the largest group with 282 samples, exhibited low total solids and texture values but high carotenoid content, consisting of 49% C. annuum, 22% C. chinense, 16% C. pubescens, 12% C. baccatum, and 1% C. frutescens.
3.4 Desirability in cluster analysis
Categorical differentiation of the clusters based on desirability index by sector was visualized using a heatmap (Figure 8A), where the color intensity indicates relative suitability and darker shades represent higher desirability, while lighter shades correspond to lower desirability. For the pharmaceutical sector, no ideal candidates were identified except for one individual from Cluster II, which has the highest capsaicinoid content (Figure 8B). Conversely, Clusters I achieved the highest overall scores for the fresh consumption (Raw) sector (Figure 8C), with the highest soluble solids content. Lastly, Cluster IV predominantly contains accessions with high carotenoid and low capsaicinoid content, rendering them ideal for the food and cosmetics (F&C) sectors (Figure 8D).
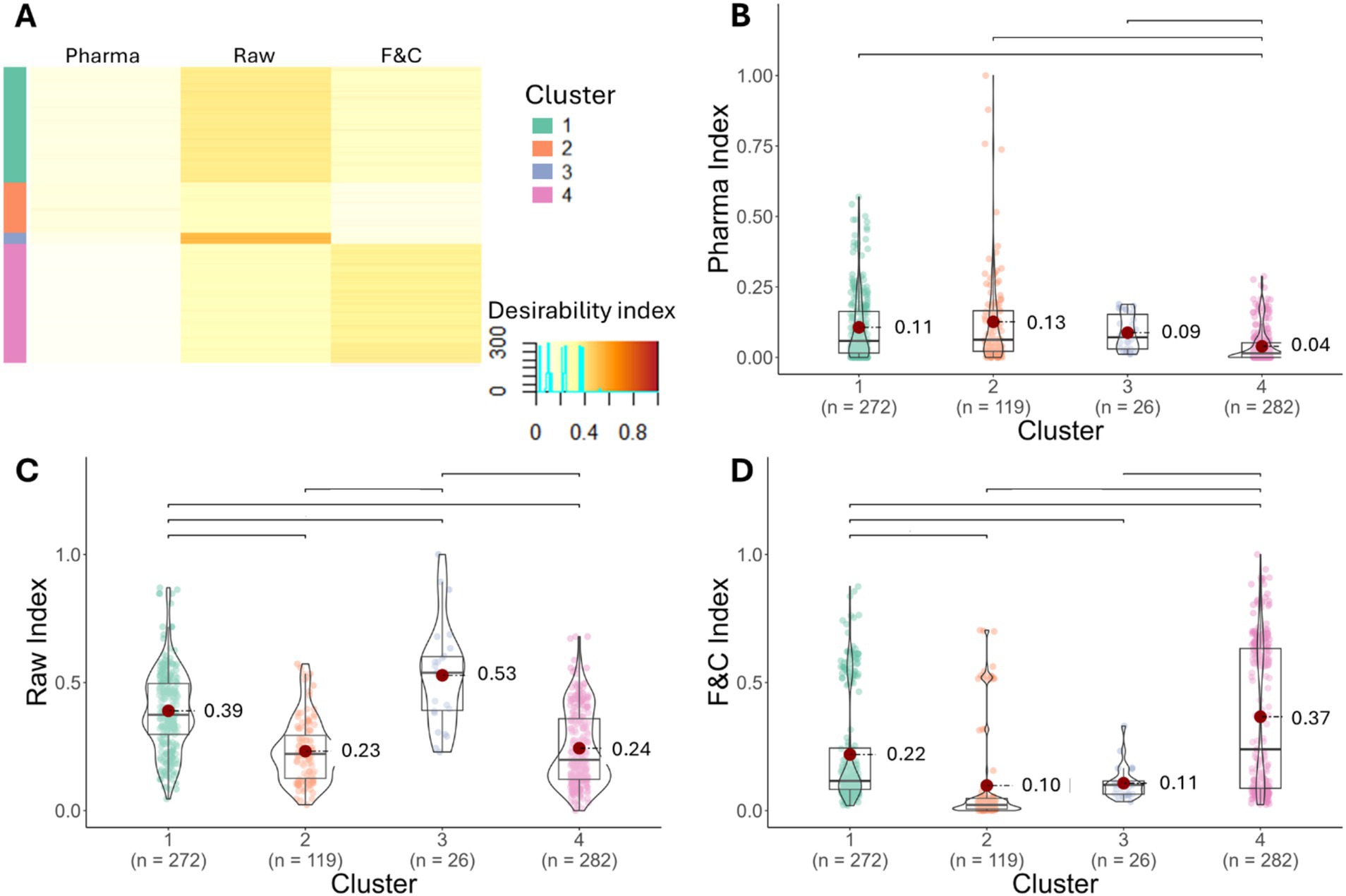
Figure 8. Distribution of desirability index across clusters. Heatmap of desirability index for industrial sectors between clusters (A). Distribution of desirability index in Pharma (B), Raw (C), Food and cosmetic (D) for each cluster. Red dots represent median values, and significant comparisons are marked with brackets (p < 0.05).
4 Discussion
Although Capsicum is a well-studied crop, wild varieties native to the Americas continue to exhibit high-value traits that surpass those of widely marketed cultivars (Figure 2). To better assess germplasm usability, this study employed a commercial chili paste preparation method that incorporates seeds, pericarp, and placenta. This integrative approach allows for a more comprehensive evaluation of both chemical and physical properties, offering a realistic representation of the product compared to conventional methods that focus solely on morphological traits or isolated fruit segments (Anani et al., 2024; Ramchiary and Chittaranjan, 2019).
By analyzing a diverse array of physicochemical and chemical characteristics, was demonstrated that these variables provide valuable insights for evaluating the potential applications of Capsicum genotypes in food, pharmaceutical, and cosmetic industries. Assessing genotypes based on these traits offer a versatile and accurate method to identify those best suited to meet specific industry demands, thereby enhancing our understanding of germplasm utility.
The evaluated germplasm exhibited extensive phenotypic variability, even within the same species (Figures 2–5). This diversity across the 233 accessions is highly relevant for breeding programs and industrial applications (Brilhante et al., 2021). While pH values remained relatively stable among species, C. baccatum and C. annuum genotypes (accessions 189 and 257) showed notably lower median pH levels. This trait could be advantageous for food processing, as lower pH enhances paste stability and fermentation conditions, promoting the development of desirable flavor compounds (Bozkurt and Erkmen, 2004; Liu et al., 2023). Moreover, a low pH may active enzymes that degrade pectin and modify fruit texture, serving as an indicator of both sweet and acid flavors (Baccichet et al., 2021). These accessions may therefore hold value for controlled fermentation applications.
In Capsicum fruits, soluble solids provide an indication of the water-soluble compounds mainly sugars, amino acids, and organic acids that are closely associated with flavor and maturity (Kim et al., 2021, 2019). Total solids represent the non-water portion of the fruit, encompassing carbohydrates, proteins, fats, and bioactive compounds, and are key to assessing nutritional quality, texture, shelf life, and food safety (Villaseñor-Aguilar et al., 2020). The color index reflects the intensity of extractable pigments, particularly carotenoids, which are valuable for natural colorants in food and cosmetic products. Texture attributes, such as firmness and cohesiveness, influence the processing characteristics and sensory quality of Capsicum products, impacting consumer acceptance and product stability (Hernández-Pérez et al., 2020; Konishi et al., 2022). Additionally, capsaicinoids determine the fruit’s pungency as critical trait for both flavor and pharmaceutical applications, while carotenoids not only impart vibrant hues but also provide antioxidant benefits and serve as precursors to vitamin A, thereby enhancing the overall functional value of Capsicum-based ingredients (Catalfamo et al., 2022; Guevara et al., 2021; Kaur et al., 2022; Rezazadeh et al., 2023; Suttisansanee et al., 2023).
Furthermore, C. pubescens consistently displayed low soluble and total solids but was the only species where dihydrocapsaicin content exceeded capsaicin (Figure 5), a distinctive trait previously reported (Zewdie and Bosland, 2001). In contrast, C. annuum showed high color index values, strongly correlated with extractable pigment content, making it a promising candidate for food and cosmetic applications (Nieto-Sandoval et al., 1999; Ramchiary and Chittaranjan, 2019).
Both genetic and environmental factors significantly influence the physicochemical properties of Capsicum fruits. Although environmental conditions—such as light intensity, temperature, and soil nutrient availability—can modulate traits like pH, soluble solids, and pigment composition (Brilhante et al., 2021; Kim et al., 2021), this study was conducted under uniform conditions in a single greenhouse. Consequently, the observed variability among the 233 accessions can be primarily attributed to genetic differences among species and genotypes. Importantly, the seeds used were not derived from controlled self-fertilization; rather, this germplasm bank preserves natural populations to maintain the full spectrum of genetic diversity, ensuring that the fruits analyzed reflect their natural reproductive behavior. Furthermore, our findings challenge the notion of fixed chemical stereotype among Capsicum species, as evidenced by the marked variability observed within species (Figure 7C) (Espichán et al., 2022; Zewdie and Bosland, 2001). Nevertheless, the presence of intra-cultivar variability in 12% of the accessions indicates that even under controlled conditions, minor environmental fluctuations or cross-pollination may influence the phenotypic expression of these traits (Ramchiary and Chittaranjan, 2019). To fully elucidate the contributions of genetic and environmental factors to the physicochemical characteristics of Capsicum fruits, future studies should incorporate multi-location trials and controlled environment experiments. Although it might be assumed that the physicochemical variability of Capsicum fruits is primarily species-dependent, the analysis reveals that multiple factors contribute to these traits. A high degree of variability was observed within each species (Figures 2–5), indicating that species alone is an inadequate basis for segregation. Therefore, the analysis was conducted at the accession level using a random-effects model that treated each accession as an independent unit within its respective species. Subsequently, an unsupervised multivariate clustering approach was applied to group accessions based on their phenotypic traits, providing a more accurate characterization of the inherent diversity within the population.
4.1 Sector-specific applications
By categorizing clusters based on their desirability indices across different sectors, each genotype was linked to its most suitable applications (Figure 8A). Although the pharmaceutical sector showed the lowest desirability index, the differences among clusters were not pronounced, Cluster II appears to be the most appropriate candidate for the pharmaceutical sector (Figure 8B). Notably, a cluster performing well in one sector (e.g., Cluster II for Pharma due to high capsaicinoid content) may not exhibit similar strength in others (e.g., Raw or F&C), underscoring the multidimensional nature of Capsicum trait variability. This finding highlights the importance of evaluating multiple parameters to identify cluster or individual genotypes that are best suited for specific industrial applications.
Capsaicin, the primary bioactive compound in several approved topical analgesics, is of particular interest to the pharmaceutical industry (Peppin and Pappagallo, 2014). Notably, genotype 160 from Cluster II exhibited the highest capsaicinoid concentration, reaching 6,479 mg/kg in dry weight (Table 2), equivalent to approximately 97.000 Scoville Heat Units (SHU), a pungency level comparable to commercial Habanero varieties (Hernández-Pérez et al., 2020). It is important to note that capsaicinoid content can increase significantly with fruit maturation, as observed in Cayenne varieties, where concentrations may rise up to fivefold over six harvest stages (Penagos-Calvete et al., 2019).
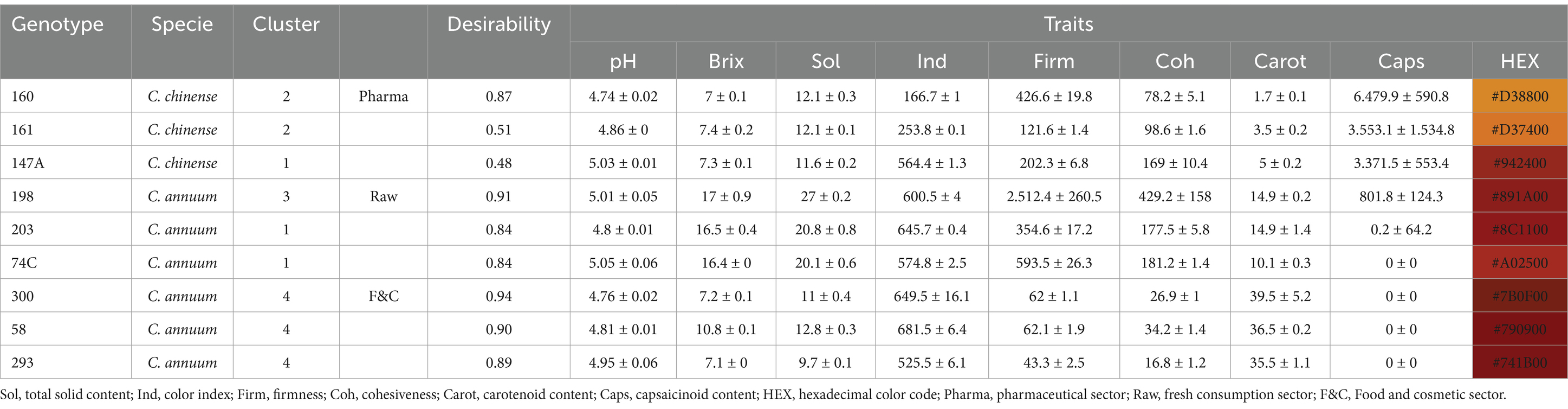
Table 2. Physicochemical and chemical traits of three selected Capsicum accessions for each industrial sector.
Although flavor and appearance are key traits for fresh consumption, they are often subjective and difficult to quantify objectively. To address this, soluble solids content was used as a proxy for amino acid and sugar concentrations, both closely linked to flavor perception (Kim et al., 2019; Liu et al., 2023). Clusters I and III, characterized by high soluble solids content, were identified as the most suitable for direct consumption (Figure 8C). Notably, genotype 198 from Cluster III exhibited the highest soluble solids content at 17° Brix (Table 2), significantly exceeding the typical range for C. annuum, which generally falls between 4 and 11° Brix (Cortés-Estrada et al., 2020; Moreno-Reséndez et al., 2016; Solís-Marroquín et al., 2017).
Capsicum is widely recognized for its rich content of carotenoids, vitamins, capsaicinoids, capsiates, and essential minerals, positioning it as a promising source to meet the increasing consumer demand for natural, bioactive phytocompounds for the food and cosmetic industries. To align with this market trend, desirability was linked to elevated carotenoid content while capsaicinoids were excluded due to their limited relevance in these sectors (Baenas et al., 2019). Cluster IV, characterized by high carotenoid and low capsaicinoid content, emerged as the most suitable group for such applications (Figure 8D). Notably, genotype 300 from this cluster displayed the highest carotenoid content at 39.54 mg/g (Table 2), significantly exceeding the reported average range of 16.20–24.66 mg/g for various C. annuum genotypes (Hassan et al., 2019; Hornero-Méndez et al., 2002; Mokhtar et al., 2016; Woldemariam et al., 2021), establishing it as a highly promising candidate for this niche market.
While the desirability index for the pharmaceutical sector primarily emphasizes capsaicinoid content, the potential applications of Capsicum extend beyond this single compound (Baenas et al., 2019). Therefore, the evaluated germplasm generally does not appear highly promising for this sector. However, further quantification and mapping of additional bioactive compounds are necessary to fully explore its pharmaceutical potential. In contrast, the food and cosmetic sector demonstrated a strong preference for genotypes with high carotenoid content, particularly within clusters IV and I. Several genotypes from these clusters exhibited remarkably high carotenoid concentrations, ranging from 35 to 39 mg/g during early maturation stages. This discovery is significant as it allows for the identification of promising materials for targeted breeding programs, maximizing the use of genetic resources while promoting biodiversity conservation. By addressing the rising consumer demand for natural and bioactive ingredients, this strategy effectively supports innovation in the food and cosmetic industries. Table 2 and Figure 9 present the top three genotypes for each sector based on the desirability index.
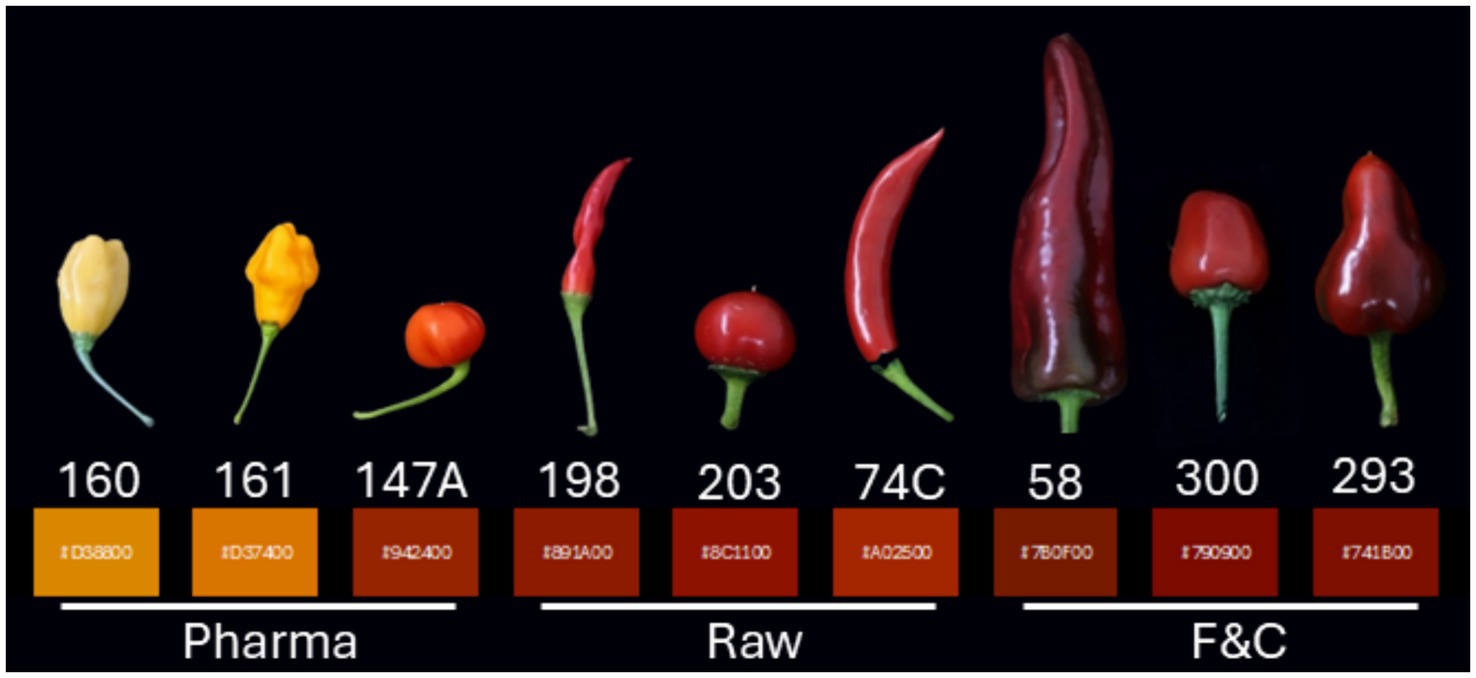
Figure 9. Representative fruits from selected Capsicum accessions, not shown to scale, highlighting the top three genotypes per sector based on their desirability index and key industrial applications (Original images).
5 Conclusion
This study comprehensively evaluated a diverse Capsicum germplasm collection to identify genotypes with high-value traits for targeted industrial applications. Through a detailed analysis of physicochemical and phytochemical descriptors, 233 accessions were clustered into distinct groups. Notably genotype 160 from C. chinense, exhibiting the highest but commonly within its gender, capsaicinoid content (6,062 mg/kg, approximately 91,000 SHU), emerges as acceptable candidate for pharmaceutical applications. Similarly, genotype 198 from C. annuum, with the highest soluble content (17 °Brix) is well-suited for fresh consumption and related uses, while genotype 300 from C. annuum stands out for its superior carotenoid content (39.54 mg/g), positioning it as ideal for food and cosmetic industries. These findings underscore the extensive phenotypic variability within Capsicum germplasm and provide robust foundation for targeted breeding programs aimed at sustainable valorization and conservation. Moreover, the observed correlations among key traits offer valuable insights into future research, highlighting the multifaceted potential of Capsicum as a source of high-value bioactive compounds.
Data availability statement
The raw data supporting the conclusions of this article will be made available by the authors without undue reservation.
Author contributions
EC: Writing – original draft, Formal analysis, Investigation. MV: Investigation, Writing – original draft. NG-V: Investigation, Writing – original draft. OL: Conceptualization, Project administration, Writing – original draft. JH-R: Conceptualization, Formal analysis, Funding acquisition, Supervision, Writing – review & editing. GM: Conceptualization, Funding acquisition, Supervision, Writing – review & editing, Project administration, Writing – original draft.
Funding
The author(s) declare that financial support was received for the research and/or publication of this article. This work was supported by the Ministry of Science, Technology, and Innovation of Colombian Government. Grants 80740-451-2021, Proyecto Jóvenes Investigadores BPIN 2022000100068, and 435-2021.
Acknowledgments
The authors wish to express their sincere gratitude to Laura María Restrepo Martinez for kindly providing the images included in this study. We extend our gratitude to the colleagues at the Agrosavia La Selva Research Center for facilitating access to essential resources and for their valuable insights and assistance. Additionally, the authors acknowledge the support and commitment of Hugo Restrepo & CIA, and we all appreciate the public funding from the subproject “Selección de materiales de Capsicum spp. con atributos diferenciales y ventajas competitivas agronómicas y agroindustriales” (code number 86924).
Conflict of interest
The authors declare that the research was conducted in the absence of any commercial or financial relationships that could be construed as a potential conflict of interest.
Generative AI statement
The authors declare that no Gen AI was used in the creation of this manuscript.
Publisher’s note
All claims expressed in this article are solely those of the authors and do not necessarily represent those of their affiliated organizations, or those of the publisher, the editors and the reviewers. Any product that may be evaluated in this article, or claim that may be made by its manufacturer, is not guaranteed or endorsed by the publisher.
References
Aguilar-Meléndez, A., Vásquez-Dávila, M. A., Manzanero-Medina, G. I., and Katz, E. (2021). Chile (Capsicum spp.) as food-medicine continuum in multiethnic Mexico. Food Secur. 10:2502. doi: 10.3390/foods10102502
Anani, P. Y., Bayor, H., Karikari, B., Amegbor, I. K., Nyarko, G., Hudu, A. R., et al. (2024). Exploring morphological variation and stability in hot pepper (Capsicum annuum) germplasm collection from the northern region of Ghana. Sci. Hortic. 337:113509. doi: 10.1016/j.scienta.2024.113509
AOAC (1998). Official method 934.01 loss on drying (moisture) at 95–100°C for feeds dry matter on oven drying at 95–100°C for feeds first. J. AOAC 577:6155.
Baccichet, I., Chiozzotto, R., Bassi, D., Gardana, C., Cirilli, M., and Spinardi, A. (2021). Characterization of fruit quality traits for organic acids content and profile in a large peach germplasm collection. Sci. Hortic. 278:109865. doi: 10.1016/j.scienta.2020.109865
Baenas, N., Belović, M., Ilic, N., Moreno, D. A., and García-Viguera, C. (2019). Industrial use of pepper (Capsicum annum L.) derived products: technological benefits and biological advantages. Food Chem. 274, 872–885. doi: 10.1016/j.foodchem.2018.09.047
Bozkurt, H., and Erkmen, O. (2004). Effects of production techniques on the quality of hot pepper paste. J. Food Eng. 64, 173–178. doi: 10.1016/j.jfoodeng.2003.09.028
Brilhante, B. D. G., Santos, T. D. O., Santos, P. H. A. D., Kamphorst, S. H., Neto, J. D. S., Rangel, L. H., et al. (2021). Phenotypic and molecular characterization of brazilian capsicum germplasm. Agronomy 11:854. doi: 10.3390/agronomy11050854
Catalfamo, L. M., Marrone, G., Basilicata, M., Vivarini, I., Paolino, V., Della-Morte, D., et al. (2022). The utility of Capsicum annuum L. in internal medicine and in dentistry: a comprehensive review. Int. J. Environ. Res. Public Health 19:11187. doi: 10.3390/ijerph191811187
Cortés-Estrada, C. E., Gallardo-Velázquez, T., Osorio-Revilla, G., Castañeda-Pérez, E., Meza-Márquez, O. G., López-Cortez, M. D. S., et al. (2020). Prediction of total phenolics, ascorbic acid, antioxidant capacities, and total soluble solids of Capsicum annuum L. (bell pepper) juice by FT-MIR and multivariate analysis. LWT 126:109285. doi: 10.1016/j.lwt.2020.109285
Espichán, F., Rojas, R., Quispe, F., Cabanac, G., and Marti, G. (2022). Metabolomic characterization of 5 native Peruvian chili peppers (Capsicum spp.) as a tool for species discrimination. Food Chem. 386:132704. doi: 10.1016/j.foodchem.2022.132704
Guevara, L., Domínguez-Anaya, M. Á., Ortigosa, A., González-Gordo, S., Díaz, C., Vicente, F., et al. (2021). Identification of compounds with potential therapeutic uses from sweet pepper (Capsicum annuum L.) fruits and their modulation by nitric oxide (no). Int. J. Mol. Sci. 22:4476. doi: 10.3390/ijms22094476
Hassan, N. M., Yusof, N. A., Yahaya, A. F., Rozali, N. N. M., and Othman, R. (2019). Carotenoids of capsicum fruits: pigment profile and health-promoting functional attributes. Antioxidants 8:469. doi: 10.3390/antiox8100469
Hernández-Pérez, T., Gómez-García, M. D. R., Valverde, M. E., and Paredes-López, O. (2020). Capsicum annuum (hot pepper): an ancient Latin-American crop with outstanding bioactive compounds and nutraceutical potential. A review. Compr. Rev. Food Sci. Food Saf. 19, 2972–2993. doi: 10.1111/1541-4337.12634
Hornero-Méndez, D., Costa-García, J., and Mínguez-Mosquera, M. I. (2002). Characterization of carotenoid high-producing Capsicum annuum cultivars selected for paprika production. J. Agric. Food Chem. 50, 5711–5716. doi: 10.1021/jf0256236
Kantar, M. B., Anderson, J. E., Lucht, S. A., Mercer, K., Bernau, V., Case, K. A., et al. (2016). Vitamin variation in capsicum spp. Provides opportunities to improve nutritional value of human diets. PLoS One 11:e0161464. doi: 10.1371/journal.pone.0161464
Kaur, A., Aggarwal, P., Kaur, N., and Kaur, S. (2022). Enhancement of biofunctional properties, sensory attributes, and shelf life of flatbread by incorporating red bell pepper (Capsicum annuum L.). J. Food Process. Preserv. 46:e17220. doi: 10.1111/jfpp.17220
Khoury, C. K., Carver, D., Barchenger, D. W., Barboza, G. E., van Zonneveld, M., Jarret, R., et al. (2020). Modelled distributions and conservation status of the wild relatives of Chile peppers (Capsicum L.). Divers. Distrib. 26, 209–225. doi: 10.1111/ddi.13008
Kim, E. H., Lee, S. Y., Baek, D. Y., Park, S. Y., Lee, S. G., Ryu, T. H., et al. (2019). A comparison of the nutrient composition and statistical profile in red pepper fruits (Capsicums annuum L.) based on genetic and environmental factors. Appl. Biol. Chem. 62, 1–13. doi: 10.1186/s13765-019-0456-y
Kim, E. H., Lee, K. M., Lee, S. Y., Kil, M., Kwon, O. H., Lee, S. G., et al. (2021). Influence of genetic and environmental factors on the contents of carotenoids and phenolic acids in red pepper fruits (Capsicum annuum L.). Appl. Biol. Chem. 64, 1–11. doi: 10.1186/s13765-021-00657-8
Konishi, A., Terabayashi, S., and Itai, A. (2022). Relationship of cuticle development with water loss and texture of pepper fruit. Can. J. Plant Sci. 102, 103–111. doi: 10.1139/cjps-2021-0031
Liu, Z., Cai, S., Zhang, S., Xiao, Y., Devahastin, S., Guo, C., et al. (2023). A systematic review on fermented chili pepper products: sensorial quality, health benefits, fermentation microbiomes, and metabolic pathways. Trends Food Sci. Technol. 141:104189. doi: 10.1016/j.tifs.2023.104189
Lozada, D. N., Bosland, P. W., Barchenger, D. W., Haghshenas-Jaryani, M., Sanogo, S., and Walker, S. (2022). Chile pepper (Capsicum) breeding and improvement in the “multi-omics” era. Front. Plant Sci. 13:879182. doi: 10.3389/fpls.2022.879182
Mokhtar, M., Russo, M., Cacciola, F., Donato, P., Giuffrida, D., Riazi, A., et al. (2016). Capsaicinoids and carotenoids in Capsicum annuum L.: optimization of the extraction method, analytical characterization, and evaluation of its biological properties. Food Anal. Methods 9, 1381–1390. doi: 10.1007/s12161-015-0311-7
Moreno-Reséndez, A., Parcero-Solano, R., Reyes-Carrillo, J. L., Salas-Pérez, L., Moncayo-Luján, M. D. R., Ramírez-Aragón, M. G., et al. (2016). Organic manures improved the phenolic content, antioxidant capacity and soluble solids in pepper. Food Nutr. Sci. 7, 1401–1413. doi: 10.4236/fns.2016.714127
Nieto-Sandoval, J. M., Ferná Ndez-Ló, J. A., Almela, L., and Muñ Oz, J. A. (1999). Dependence between apparent color and extractable color in paprika. Color. Res. Appl. 24, 93–97. doi: 10.1002/(SICI)1520-6378(199904)24:2<93::AID-COL4>3.0.CO;2-W
Penagos-Calvete, D., Guauque-Medina, J., Villegas-Torres, M. F., and Montoya, G. (2019). Analysis of triacylglycerides, carotenoids and capsaicinoids as disposable molecules from Capsicum agroindustry. Hortic. Environ. Biotechnol. 60, 227–238. doi: 10.1007/s13580-018-0111-2
Peppin, J. F., and Pappagallo, M. (2014). Capsaicinoids in the treatment of neuropathic pain: a review. Ther. Adv. Neurol. Disord. 7, 22–32. doi: 10.1177/1756285613501576
R Core Team. (2023). R: a language and environment for statistical computing. Vienna, Austria: R Foundation for Statistical Computing. Available at: https://www.R-project.org/
Ramchiary, N., and Chittaranjan, K. (Eds.) (2019). “Compendium of plant genomes the capsicum genome” in The capsicum genome (Switzerland: Springer Nature).
Rezazadeh, A., Hamishehkar, H., Ehsani, A., Ghasempour, Z., and Moghaddas Kia, E. (2023). Applications of capsaicin in food industry: functionality, utilization and stabilization. Crit. Rev. Food Sci. Nutr. 63, 4009–4025. doi: 10.1080/10408398.2021.1997904
Sanati, S., Razavi, B. M., and Hosseinzadeh, H. (2018). A review of the effects of Capsicum annuum L. and its constituent, capsaicin, in metabolic syndrome. Iran. J. Basic Med. Sci. 21, 439–448. doi: 10.22038/IJBMS.2018.25200.6238
Sánchez Toledano, B. I., Camarena Gómez, D. M. J., López Santiago, M. A., and Cuevas Reyes, V. (2023). Consumer preferences of Jalapeño pepper in the Mexican market. Horticulturae 9:684. doi: 10.3390/horticulturae9060684
Sert, D., Mercan, E., and Dertli, E. (2017). Characterization of lactic acid bacteria from yogurt-like product fermented with pine cone and determination of their role on physicochemical, textural and microbiological properties of product. Lwt 78, 70–76. doi: 10.1016/j.lwt.2016.12.023
Solís-Marroquín, D., Lecona-Guzmán, C. A., Ruiz-Lau, N., Ocampo, P., Rodas-Trejo, J., Gonzales-Santiago, C., et al. (2017). Bromatological analysis of “siete caldos” pepper fruits (Capsicum annuum) grown under outdoor and shade house conditions. Agroproductividad 10, 34–40.
Suttisansanee, U., Thiyajai, P., Inthachat, W., Pruesapan, K., Wongwathanarat, K., Charoenkiatkul, S., et al. (2023). Exploration of the nutritional and carotenoids profiles of vegetables in Thai cuisine as potential nutritious ingredients. Heliyon 9:e15951. doi: 10.1016/j.heliyon.2023.e15951
Tripodi, P., Rabanus-Wallace, M. T., Barchi, L., Kale, S., Esposito, S., Acquadro, A., et al. (2021). Global range expansion history of pepper (Capsicum spp.) revealed by over 10,000 genebank accessions. Proc. Natl. Acad. Sci. USA 118, 1–9. doi: 10.1073/pnas.2104315118
Villaseñor-Aguilar, M. J., Bravo-Sánchez, M. G., Padilla-Medina, J. A., Vázquez-Vera, J. L., Guevara-González, R. G., García-Rodríguez, F. J., et al. (2020). A maturity estimation of bell pepper (Capsicum annuum L.) by artificial vision system for quality control. Appl. Sci. (Switzerland) 10:5097. doi: 10.3390/app10155097
Woldemariam, H. W., Admassu Emire, S., Getachew Teshome, P., Toepfl, S., and Aganovic, K. (2021). Physicochemical, functional, oxidative stability and rheological properties of red pepper (Capsicum annuum L.) powder and paste. Int. J. Food Prop. 24, 1416–1437. doi: 10.1080/10942912.2021.1969945
Keywords: Capsicum, soluble solids, capsaicinoids, carotenoids, germplasm, bioprospecting, industrial applications
Citation: Castaño E, Vega-Muñoz MA, Grisales-Vásquez NY, Loaiza-Loaiza OA, Henao-Rojas JC and Montoya G (2025) Capsicum germplasm targeted valorization using physicochemical and phytochemical descriptors. Front. Sustain. Food Syst. 9:1571012. doi: 10.3389/fsufs.2025.1571012
Edited by:
Laurent Dufossé, Université de la Réunion, FranceReviewed by:
Miguel Ángel De León Zapata, Autonomous University of Coahuila, MexicoErlina Ambarwati, Gadjah Mada University, Indonesia
Copyright © 2025 Castaño, Vega-Muñoz, Grisales-Vásquez, Loaiza-Loaiza, Henao-Rojas and Montoya. This is an open-access article distributed under the terms of the Creative Commons Attribution License (CC BY). The use, distribution or reproduction in other forums is permitted, provided the original author(s) and the copyright owner(s) are credited and that the original publication in this journal is cited, in accordance with accepted academic practice. No use, distribution or reproduction is permitted which does not comply with these terms.
*Correspondence: Guillermo Montoya, Z2xtb250b3lhQGljZXNpLmVkdS5jbw==; Juan Camilo Henao-Rojas, amhlbmFvQGFncm9zYXZpYS5jbw==