- Pharmacology, Department of Biomedical Sciences, Veterinary Faculty, Institute of Biomedicine (IBIOMED), University of Leon, Leon, Spain
Organochlorine pesticides (OCP) are persistent pollutants well known for their ability to bioaccumulate. So, food contamination with these compounds is of concern for human health. The levels of nine OCP were determined in 60 samples of black tea and two herbal products (chamomile and linden) commercially available in Spain. The analysis was carried out by gas chromatography coupled with electron capture detector (GC-ECD), and confirmed by gas chromatography-mass spectrometry (GC-MS). The linearity, accuracy, precision, and limits of quantification and detection of the method were validated. OCP residues were detected in 66.7% of the samples at low levels, being always below the European maximum residue limits (MRL). No OCP was found in black tea samples, and only four pesticides were present in linden and chamomile products: 2,4′-DDD was the most frequently OCP detected, followed by aldrin, endrin, and 4,4′-DDD. The health risk assessment indicated that the presence of OCP in black tea and the two herbal products does not pose any risk to consumers.
1 Introduction
Tea and herbal products are popular worldwide beverages, and have been traditionally considered part of the human diet for both therapeutic and pleasure purposes. In fact, and together with water, tea is the most consumed beverage in the world. Their specific aromas and pleasant tastes, as well as their presumed benefits for human health, may explain their popularity among consumers. The global tea market (including herbal teas) was worth USD 63.5 billion in 2023, with an annual increase of 6.3% from 2024 to 2032 (Zion Market Research, 2024). In Europe, a total of 228869.10 t of tea (86% black tea) were imported into the European Union (EU) in 2018 (Tea & Herbal Infusions Europe, 2023a), and 64,493 t of herbal and fruit infusions were sold in 2020 in 10 EU countries (Tea & Herbal Infusions Europe, 2023b). Related to their potential health benefits, the World Health Organization (WHO) fostered to strengthen the role of traditional medicines, including herbal products, in its 2014–2023 strategy (World Health Organization, 2013). The Dutch dietary guidelines recommended some years later to daily drink three cups of green or black tea to reduce high blood pressure and the risk of stroke (Kromhout et al., 2016). Likewise, the Chinese Nutrition Society encouraged Chinese population to drink plain water or tea (Chinese Nutrition Society, 2017). This underlines the importance of these products among the population worldwide.
Nevertheless, consumption of tea and herbal products may eventually represent a potential source of human exposure to various hazardous chemicals such as organochlorine pesticides (OCP). These compounds have been used for more than 80 years to control pests in agriculture as well as prevent vector borne diseases. It has been estimated that about 7–10% of tea crops were lost by pests and disease attacks (Alnawaiseh et al., 2021). Despite their advantages, OCP tend to accumulate in living organisms due to their lipophilicity, low biodegradability, and high persistence in the environment, as well as their ability to transport far-off (Jayaraj et al., 2016; Ma et al., 2020; Olisah et al., 2019). Consequently, trace amounts of these substances may enter the food chain, and cause chronic health problems in humans.
According to the WHO, OCP pesticides are of concern for human health (World Health Organization, 2010), and the Stockholm Convention (United Nations Environment Programme, 2001) considered these compounds as priority hazardous pollutants. Moreover, the Agency for Toxic Substances and Disease Registry (ATSDR) indicated that these substances have developmental, endocrine, hepatic, neurological, and reproductive detrimental effects (Agency for Toxic Substances and Disease Registry, 2024). For all these reasons, most countries have banned or severely restricted their use, although they are still present in the environment, as well as in some foods and water sources (Ma et al., 2020; Ma et al., 2022). In addition, importing countries or institutions such as the EU have set maximum residue limits (MRL) in different foods, including tea or other herbal products, in order to protect consumers’ health. Restrictions set by regulatory agencies have made multi-residue analysis of OCP in teas and herbal products increasingly important. To monitor them, analysis is usually carried out in several steps, including target extraction from sample matrix, clean-up, pre-concentration, and chromatographic determination. Gas chromatography-mass spectrometry (GC-MS) has become very popular in pesticide residue analysis to identify and confirm the results obtained.
Therefore, a continuous assessment of the presence of OCP should be carried out in view of the high and increasing consumption rate of tea and herbal products, as well as the adverse effects of OCP residues on the health of consumers of these products, especially with regard to chronic health. Several studies have monitored the potential hazards of herbal products usually consumed as infusions related to the presence of OCP residues (Taha, 2020; Rai et al., 2008; Drumea et al., 2018; Siraj et al., 2021; Szpyrka and Słowik-Borowiec, 2019; Üner et al., 2018), as a way of minimizing their potential risk for human health and improving their safety. Nevertheless, in the EU these studies are scarce and sometimes outdated (Fernández et al., 1993; Fontcuberta et al., 2008; Witczak et al., 2018). In a previous survey carried out 30 years ago (Fernández et al., 1993), OCP concentrations were evaluated by this research group in the same type of products (black tea, chamomile, and linden). The study presented here may serve as comparison to assess the evolving trend in the occurrence of several OCP compounds, which is of great relevance from a public health point of view, even if the levels determined are below the established acceptable limits (Fattore, 2002). Thus, the objective of this study was to estimate the content of OCP residues in several commercial tea and herbal products usually consumed in Spain, and evaluate their potential health risks.
2 Materials and methods
2.1 Sample collection
A total of 60 black tea and herbal samples (chamomile and linden) were analyzed (20 of each type). They were obtained commercially in supermarkets from Leon (Spain), in boxes with 20–25 infusion bags containing 1–2 g of the product. Samples were collected among the most popular brands available in this country according to expiration date (2023 and 2024), acquiring two boxes with different batches for each year. After purchase, each sample was coded to blind recognition of the brand name. The boxes containing the bags were stored in their outer plastic wrapping at room temperature in a dry place until analysis.
2.2 Chemicals
Samples were screened for nine OCP: lindane (γ-hexachlorocyclohexane), heptachlor epoxide, aldrin, dieldrin, endrin, 4,4′-DDE, 2,4′-DDD, 4,4′-DDD, and 4,4′-DDT. These OCP compounds were selected as they had been also determined in a previous study carried out by this research group 30 years before (Fernández et al., 1993). Certified standards (purity > 96.5%) were purchased from Dr. Ehrenstorfer GmBH (Ausburg, Germany). Individual standard solutions of each compound were prepared in hexane at 1000 μg/mL. A stock solution containing 100 μg/mL of 4,4′-DDT and 10 μg/mL of the other eight OCP was then prepared in hexane. Working standard solutions were obtained by dilution in hexane to desired concentration levels. Solutions were stored in dark at 4°C. All reagents were pesticide residue quality grade, and acquired from VWR Chemicals (Leuven, Belgium). High-purity Florisil was obtained from Sigma-Aldrich (Steinheim, Germany).
2.3 Sample preparation
The content of three bags from the same box and batch were thoroughly combined, and an aliquot of 1 g taken. Extraction and clean-up were performed according to a method described previously (Stahr, 1977) with slight modifications. Briefly, 1 g sample was extracted with 2.5 mL acetonitrile, repeating extraction twice more. Subsequently, the collected supernatants were centrifuged at 1,500 rpm for 1 min, transferring acetonitrile to another glass tube. 7.5 mL of 2% aqueous sodium sulfate solution was then added, and mixed in a rotary mixer for 1 min. After triple extraction with 2 mL hexane each, the three organic phases were combined and concentrated to approximately 0.5 mL. Clean-up of the hexane extract was carried out with a glass column containing activated florisil and anhydrous sodium sulphate, by using 12 mL hexane and 24 mL 3% methanol in hexane. Extracts were first evaporated in a rotary evaporator at 60°C and then to dryness under a gentle stream of nitrogen. Finally, the dried residue was reconstituted with 1 mL hexane, and an aliquot transferred for GC analysis.
2.4 Chromatographic conditions
2.4.1 Gas chromatography-electron capture detector
Chromatographic separation and quantification of the nine OCP was performed according to conditions previously described (Fernandes et al., 2012), using a Shimadzu GC2010 gas chromatograph equipped with an electron capture detector (ECD) and an AOC-20 autoinjector. A TRB-5 fused capillary column (30 m × 0.25 mm i.d., 0.25 μm film thickness, Teknokroma) was employed as the analytical column. The carrier gas was helium at a 1.3 mL/min flow, and nitrogen was used as make-up gas (purity ≥99.999%) at a flow rate of 30 mL/min. A volume of 8 μL extract was injected in splitless mode. The column temperature was programmed from an initial temperature of 65°C (held for 2 min) to 160°C at 8°C/min, then to 235°C at 2°C/min, and finally to 250°C at 15°C/min. Injection port and detector temperatures were 250°C and 300°C, respectively. The total run time was 53 min. Each sample was injected into the gas chromatography-electron capture detector (GC-ECD) system in duplicate.
2.4.2 GC-MS/MS identification
Confirmation of OCP residues was carried out following a protocol established before (Fernandes et al., 2012). In this case, an Agilent 7010B triple quadrupole gas chromatograph mass spectrometer (GC-MS) was employed, equipped with a TR-5MS column (30 m × 0.25 mm i.d., 0.25 μm film thickness, Thermo Scientific). The mass spectrometer operated in the electron ionization mode, and the MS/MS option was used. The following mass spectrometric settings were established: electron ionization mode was set at 70 eV, 250°C ion source temperature, 250°C transfer line temperature, and 250°C injector temperature. The injector operated in the splitless mode, and helium was also used as a carrier gas at a flow rate of 1.3 mL/min. The column temperature was initially held at 40°C for 2 min, ramped to 220°C at a rate of 30°C/min (held for 5 min), and to 270°C at 10°C/min (held for 1 min). OCP were identified by comparing their mass spectra with those of the NIST library, and their corresponding retention times were established. Afterwards, the most appropriate precursor and product ions were selected to confirm each compound. Table 1 shows the precursor and product ions used to identify each compound. Total run time of GC-MS/MS analysis was 19 min.
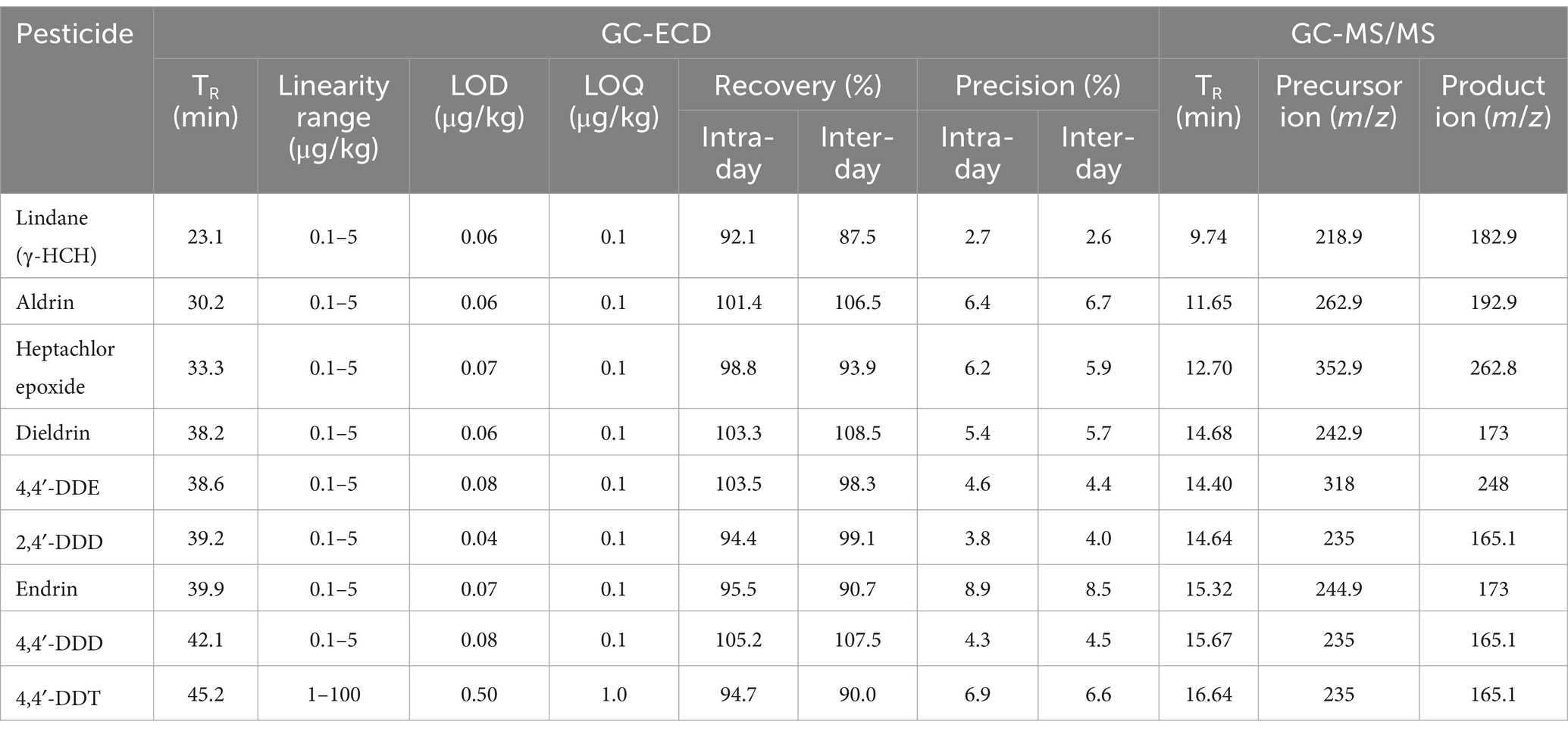
Table 1. List of target OCP, chromatographic retention times in GC-ECD and GC-MS/MS, analytical features of the GC-ECD method (linearity range, LOD, LOQ, and recovery), and selected m/z values for selective ion monitoring mode (GC-MS/MS).
2.5 Quality assessment of GC-ECD methodology
Working solutions were used to plot calibration curves as a function of peak area vs. concentrations of the nine selected OCP. The concentration range was 1–100 μg/kg for 4,4′-DDT and 0.1–5 μg/kg for the other eight compounds. The following parameters were studied to assess the validity of the methodology: linearity, precision, limits of detection (LOD) and quantification (LOQ), and recovery. The lowest detectable concentrations were defined as 3 times the standard deviation of the blank, whereas the lowest concentration level in calibration line was set as LOQ. Recoveries were established over LOQ (five replicates) on spiked blank matrices, which were analytically confirmed to have undetectable levels of pesticides. Precision was also assessed with 10 times LOQ. No matrix interference was detected in chromatograms for targeted OCP.
2.6 Estimated daily intake and risk assessment
Once residue concentrations have been established, it is necessary to assess if exposure to these compounds may involve a human health risk associated via tea and herbal product consumption. The most widely approach used is the estimation of daily intake (EDI), and its comparison with the acceptable daily intake (ADI), together with the estimation of carcinogenic and noncarcinogenic risks. EDI calculations were based on the OCP concentrations in the tea or herbal products consumed every day, following the equation:
where COCP is OCP concentration (mg/kg) in tea/herbal product, D means daily intake of the food (kg/day), and W refers average body weight (kg), which was considered 70 kg for adult people. To calculate the EDI, an average consumption of 0.1 cups of tea has been reported in Spain (Landais et al., 2018). A daily consumption of one tenth of a linden/chamomile bag (0.00011 kg/day for chamomile and 0.00012 kg/day for linden) has been considered.
Hazard Index (HI) was calculated as the ratio between EDI and acceptable daily intake (ADI) (mg/kg) as:
ADI values have been fixed by the WHO (World Health Organization, 2021), and if this quotient does not exceed 1, it is considered that the consumer would be adequately protected.
When possible, health risk of exposure was also evaluated according to the approaches described by US-EPA (Agency for Toxic Substances and Disease Registry, 2022). Noncarcinogenic risks were assessed by calculating hazard quotient (HQ) as:
being RfD the recommended dose of oral exposure (United States Environmental Protection Agency, 2024). Again, if HQ values are smaller than 1, no chronic risk is likely to occur from the exposure and consumption of these products. In those compounds for which there may be carcinogenic risks, the lifetime cancer risk (LCR) (Agency for Toxic Substances and Disease Registry, 2022) was calculated as equation:
where SF is the oral slope factor (United States Environmental Protection Agency, 2024), ED the exposure duration in years (a period of 60 years was considered, since the age of majority onwards), and LY the lifetime in years (78 years).
2.7 Statistical analysis
The results obtained from the chromatographic analysis were summarized using descriptive statistics (mean, standard deviation, and percentages). Differences between concentrations were assessed for each product and year, when possible. The Shapiro–Wilk test was used to assess the normality of the data. If normal, data were then compared using the unpaired t test; if not, the Mann–Whitney U test was applied. In all cases, a value of p ≤ 0.05 was considered significant.
3 Results and discussion
3.1 Linearity, LOD, LOQ, precision, and recovery
OCP concentrations were plotted against peak areas at 6–8 calibration levels, depending on the compound considered. Correlation coefficients (R2) were always greater than 0.96 for the nine compounds, indicating good linearity for the analyzed substances. The temperature GC-ECD program eluted the nine OCP between 23 and 45 min. Their retention times are shown in Table 1 and Figure 1. Table 1 also includes LOD and LOQ for all OCP residues, showing the high sensitivity of the analysis performed. LODs were in the range of 0.04–0.5 μg/kg, whereas LOQs ranged from 0.1 to 1 μg/kg. All LOD were also far lower the maximum residue limits (MRL) stipulated by the EU (European Commission, 2024) for these compounds, and they were low enough to accurately determine OCP concentrations in samples. OCP recoveries were within the analytical acceptable range (70–110%), and inter- and intra-day precision was always ≤ 15%. Figures 1, 2 show chromatograms after GC-ECD and GC-MS/MS analysis, respectively.
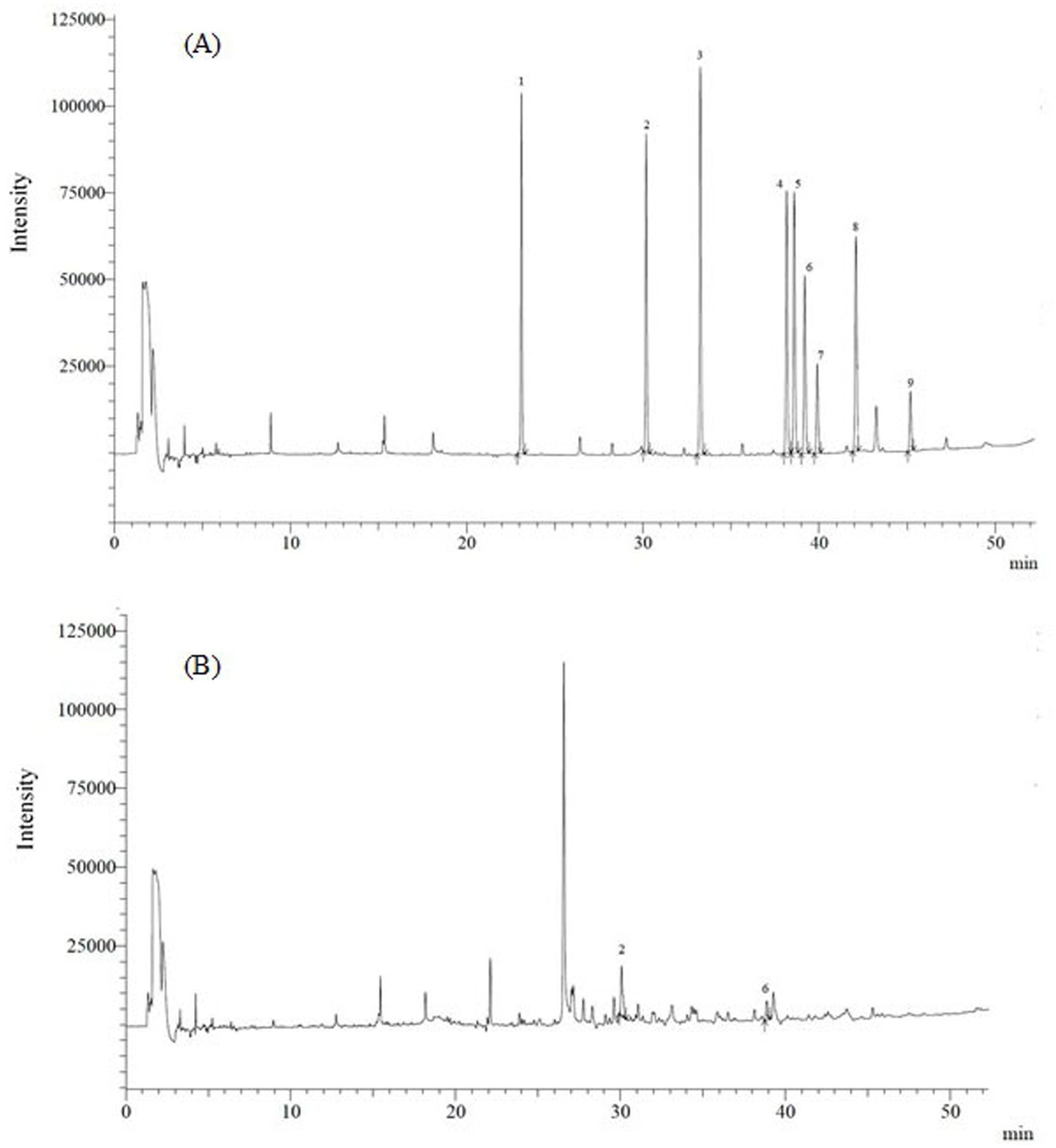
Figure 1. Representative GC-ECD chromatogram of (A) standard OCP mixture (5 μg/L) and (B) chamomile sample. Peak identification is in order of increasing retention time: (1) lindane; (2) aldrin; (3) heptachlor epoxide; (4) dieldrin; (5) 4,4′-DDE; (6) 2,4′-DDD; (7) endrin; (8) 4,4′-DDD; (9) 4,4′-DDT.
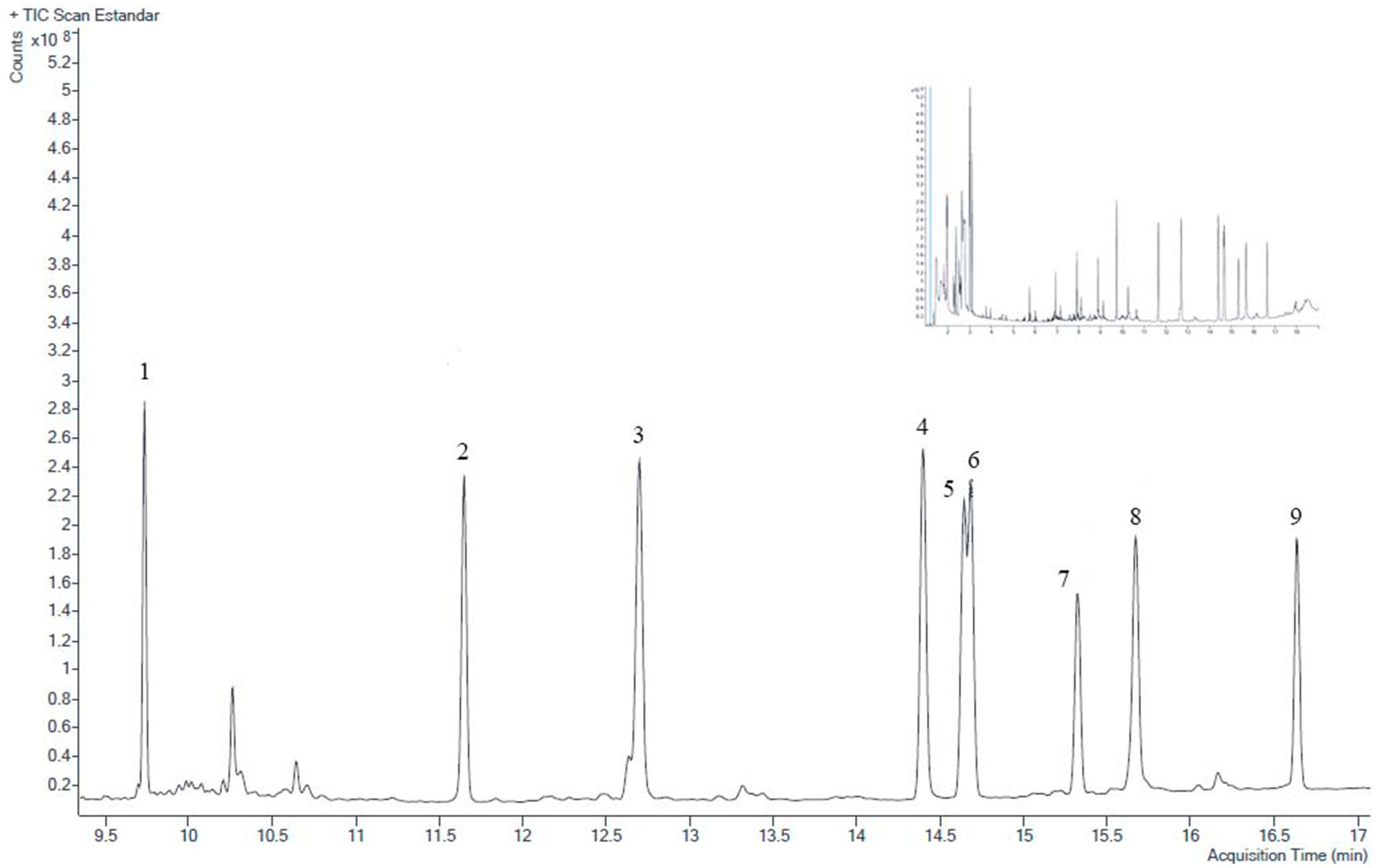
Figure 2. Representative GC-MS/MS chromatogram of standard OCP mixture (10 μg/L). A complete chromatogram is shown in small size at the top. Peak identification is in order of increasing retention time: (1) lindane; (2) aldrin; (3) heptachlor epoxide; (4) 4,4′-DDE; (5) 2,4′-DDD; (6) dieldrin; (7) endrin; (8) 4,4′-DDD; (9) 4,4′-DDT.
3.2 Occurrence of pesticide residues in samples
Results of the OCP residues analysis in tea and herbal products are summarized in Tables 2, 3. OCP residues were detected in 40 samples (66.7%). Only four of the nine compounds assessed were found in the samples (aldrin, endrin, 2,4′-DDD, and 4,4′-DDD). 2,4′-DDD was the most frequently compound detected. No pesticide was identified in any black tea sample, whereas co-occurrence of the four OCP previously described was observed in chamomile samples. Only two of these latter compounds (2,4′-DDD and 4,4′-DDD) were determined in linden samples. No significant differences were found between both expiration years for either chamomile (aldrin, endrin, and 2,4′-DDD) or linden (2,4′-DDD) (unpaired t test, p > 0.05). When 2,4′-DDD concentrations were compared between both herbal products (chamomile and linden), no significant differences were revealed (Mann–Whitney U test, p > 0.05).
Figure 3 shows mean values per year for each compound. The OCP with the highest mean concentration in all samples was endrin (4.58 μg/kg, ranging from 0.75 to 6.14 μg/kg), followed by aldrin (3.43 μg/kg, ranging from 0.80 to 6.14 μg/kg). These two compounds were detected only in chamomile samples. The two other OCP (2,4′-DDD and 4,4′-DDD) were determined in lower concentrations, which tended to be similar in both chamomile and linden samples: mean of 1.17 μg/kg (range 0.37–1.43 μg/kg) and 1.30 μg/kg (range from 0.32 to 4.27 μg/kg) for 2,4′-DDD in chamomile and linden samples, respectively; and 0.80 μg/kg (0.53–0.95 μg/kg) and 0.64 μg/kg (range from 0.13 to 1.05 μg/kg) for 4,4′-DDD, respectively.
On the other hand, half of the samples positive to the OCP screened contained only one compound. Two pesticides were noted in three samples (7.5%), three different OCP were found in eight samples (20%), and a combination of the four identified OCP were present in the other nine samples (22.5%). Those samples containing three or four different OCP residues were always chamomile ones. Moreover, residue patterns are different in the three types of products evaluated (black tea, chamomile, and linden): as mentioned above, no pesticide has been detected in black tea, whereas DDT metabolites are present in linden samples, and DDT metabolites, endrin, and aldrin were found in chamomile.
The results of the current study show that, despite their ban, residues of these compounds are still detected. Nevertheless, and compared with a similar study carried out 30 years before (Fernández et al., 1993), OCP levels were mostly lower, which shows the success of the legal measures taken. In this sense, the nine OCP were detected in those samples, whereas at present only four compounds were determined. On the other hand, as in the current study, chamomile and linden samples were also more contaminated than those of black tea.
Most recent studies have also reported their presence in tea and herbal products, with results quite variable, probably related with the country where the study was conducted. In Ethiopia (Siraj et al., 2021), OCP levels found were clearly higher than ours in domestic commercial teas (not imported) for aldrin (146.5 μg/kg), endrin (306.7 μg/kg) and DDT (204.4 μg/kg), whereas no dieldrin, 4,4′-DDE and 4,4′-DDT in rooibos samples were detected in South Africa (Areo et al., 2022). High concentrations of 4,4′-DDE (1.44–7.76 μg/L) were also reported in teas of Jordania (Alnawaiseh et al., 2021).
Low levels of lindane (36 μg/kg), 4,4′-DDE (1–18 μg/kg) and 4,4′-DDT (1–87 μg/kg) were also determined in commercial black tea in the United States (Hayward et al., 2015). As in our study, aldrin was detected in all samples assessed in Nigeria (Adenuga et al., 2022), but at higher levels than ours (10.6–96.5 μg/kg). Endrin was also found in this latter study, mostly at concentrations <0.8 μg/kg, but in two samples they raised to 8.5 and 24.6 μg/kg. Moreover, 4,4′-DDT (<0.06–647 μg/kg) and lindane (<0.4–69.8 μg/kg) were present. They also reported residues of 4,4′-DDE (<0.5–14.5 μg/kg) and 4,4′-DDD (<0.8–48 μg/kg). In Poland, as in our study, low levels were found in black tea and herbal leaves for lindane (3.68 and 6.27 ng/g, respectively), aldrin (2.29 and 2.11 ng/g), dieldrin (1.36 and 1.16 ng/g), endrin (0.16 ng/g in black tea), 2,4′-DDD (0.74 and 0.24 ng/g), and 4,4′-DDT (0.67 and 0.62 ng/g) (Witczak et al., 2018), whereas OCP levels were much higher than ours in linden flowers and leaves in Turkey (Üner et al., 2018). In view of the global restrictions on their use and the current evolution of agricultural practices, it is expected that OCP contamination in these and other edible products will tend to decrease. This reduction may be due to their substitution by other pesticides that are less persistent but not free of chronic health risks, or through the use of safer and more sustainable alternatives such as Integrated Pest Management or other sustainable agricultural practices.
As explained before, OCP residue levels are low in the samples assayed, and only four of the nine substances assessed were detected. Tables 2, 3 also shows the MRL set by the EU legislation in dried samples for the OCP evaluated (European Commission, 2024). All the analyzed samples were far below the MRL fixed by the EU, indicating a low-risk accumulation in tea and herbal products. It should be noted that no indication regarding the geographical origin (national, European or from third countries) of the plant samples was given on the labels of the commercial products acquired. At present, the companies that manufacture these products are usually international, and tend to import the raw material from various countries. Thus, it was not possible to establish their country of origin, but it is well known that China, India and Kenya are the world’s largest tea-producers (Chang, 2015; Food of Agriculture Organization of the United Nations, 2024). The low levels detected may reflect either a good agricultural practice in the country of origin, the effect of OCP ban worldwide, or the need to comply with the EU MRL to import these products. In the case of DDD isomers, their presence may indicate historical rather than recent use of DDT, which decomposes over time in the soil to DDE and DDD, and may be present in the environment for many years (Szpyrka and Słowik-Borowiec, 2019; Garrido et al., 1994).
As for the potential source of OCP, pesticides can reach the plants not only from soil, but also through the air, falling on leaves and flowers, from where they may penetrate or remain adsorbed on the surfaces (Üner et al., 2018). Thus, it is not impossible that, even if they have not been used in tea or herbal crops, their presence may be due to agricultural activities in neighbouring cultivation areas.
Regarding those differences observed in the OCP concentrations among the three products evaluated, they may be related to several reasons. In the case of black tea, leaves, leaf buds and tender stems are used, whereas in linden and chamomile mainly flowers are employed. Moreover, the essential oil present in chamomile flowers may help to explain concentrations detected in this herbal product. In the case of tea, and unlike other crops, leaves are harvested several times a year, which reduces the time between pesticide application and harvesting (Feng et al., 2015). Differences in post-harvest processing may also contribute, as chamomile and linden are picked and dried, whereas black tea is picked, withered, rolled, fermented and dried. Sood et al. (2004) pointed out that pesticide concentrations can be reduced in all steps involved in the conventional manufacture of black tea. Agricultural practices in cultivation areas can also change from one product to another.
On the other hand, the main route of human exposition to OCP is through food intake. Although in this study the presence of OCP has been detected at very low concentrations, long-term ingestion of low levels of these residues may accumulate in body tissues over time and pose a potential health risk. For this reason, and in order to understand the magnitude of exposure and potential health risk associated via tea and herbal product consumption, the EDI were calculated and noncarcinogenic and carcinogenic risks established. As shown in Table 4, the upper levels of EDI based on 95th percentile were far below both ADI and RfD values, being HI and HQ clearly lower than 1 for all the samples in which OCP residues were detected.
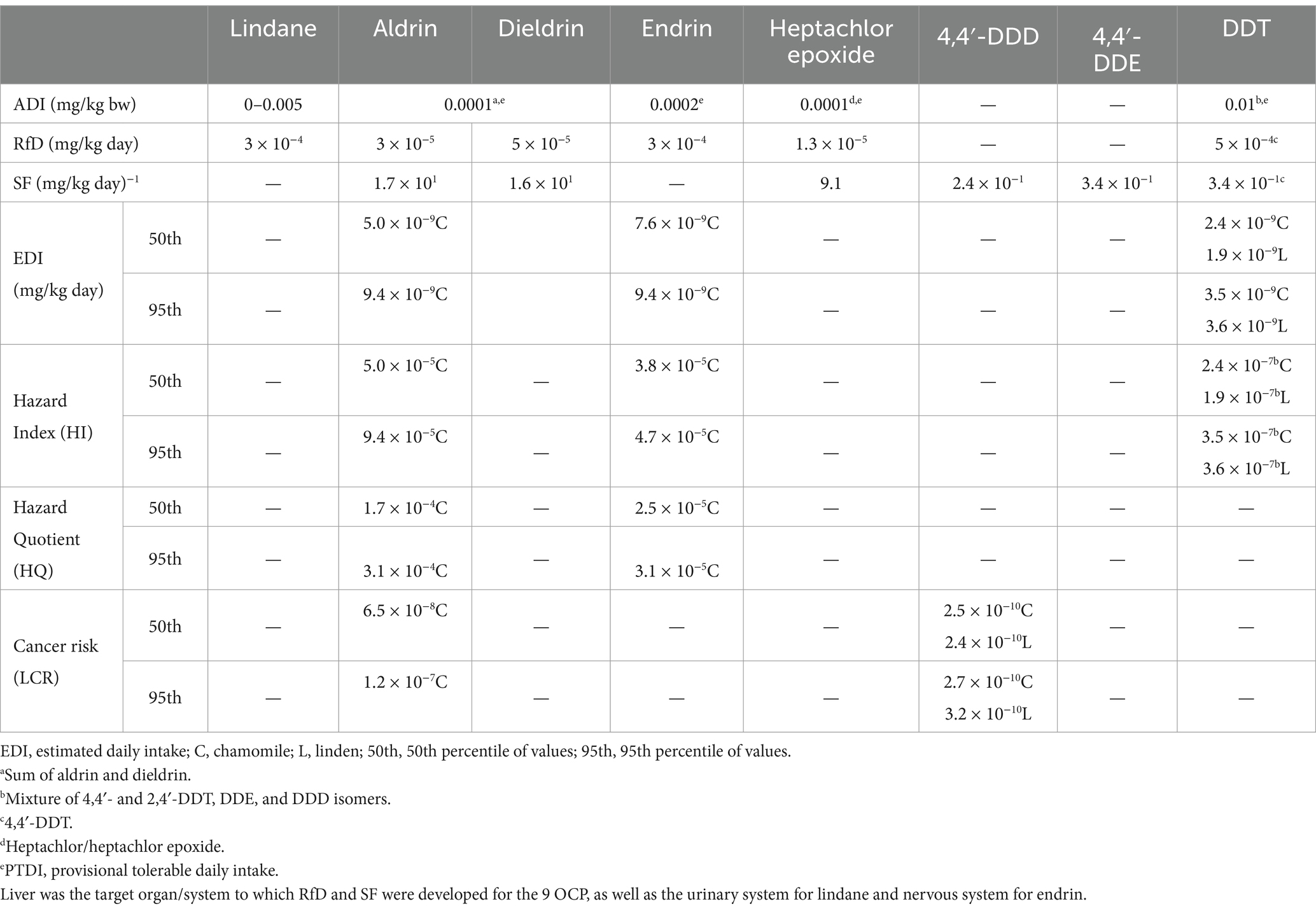
Table 4. Admissible daily intake (ADI) set by the Joint Meeting on Pesticide Residues (JMPR) of the Food and Agriculture Organization (FAO)/WHO (World Health Organization, 2021), reference dose for oral exposure (RfD) and oral slope factor (SF) fixed by the US-EPA (United States Environmental Protection Agency, 2024), and calculated health risk assessments due to OCP residues present in chamomile and linden products when consumed by adults.
For those OCP compounds with carcinogenic risk (aldrin and 4,4′-DDD), values calculated did not exceed the EPA thresholds of 10−4–10−6 (United States Environmental Protection Agency, 2009), indicating that these foods should not cause carcinogenic effects in the human body when chronically consumed. Nevertheless, it should be also borne in mind that these beverages contribute only with a small part to the potential daily exposure to pesticides, and they would be considered in addition to other foods and drinking water.
Finally, it should be also taken into account that OCP residues were assessed in this study in dried samples, in order to compare with the MRL established in the EU legislation, which are fixed in desecated product (European Commission, 2024). Thus, the residue transfer during brewing was not tested in this study. In the scientific literature different studies have evaluated OCP contamination in dried tea and herbal products (Siraj et al., 2021; Areo et al., 2022; Hayward et al., 2015; Adenuga et al., 2022), but others that did the same in brewed samples (Witczak et al., 2018; Lino and Silveira, 1997; Jeong et al., 2012; Jaggi et al., 2001), as actual exposure depends on the quantity of the residues leached into the brew finally consumed. In this latter case, OCP actual transfer were negligible (Jaggi et al., 2001), 10–30% (Lino and Silveira, 1997; Jeong et al., 2012) or 0–86% (Witczak et al., 2018). Moreover, health estimation was made assuming the total absorption of residues by the body, which may potentially overestimate OCP exposure. Another limitation is that we have tested only nine OCP in order to compare with a previous study carried out 30 years before. Thus, other potential contaminants with systemic or carcinogenic health risks (other OCP, organophosphate or pyrethroids pesticides, among others) have been excluded.
4 Conclusion
The occurrence of nine organochlorine pesticides in 60 samples of commercial black tea, chamomile and linden was assessed. Approximately two thirds of the analyzed samples showed low OCP concentrations, and no OCP compound was detected in black tea samples. Contamination pattern was slightly different in chamomile and linden samples. In chamomile, four compounds were determined, with aldrin, dieldrin and 2,4′-DDD present in almost all the samples and 4,4′-DDD in nearly the half, whereas in linden 2,4′-DDD was again present in most of them, and 4,4′-DDD only in a more sporadic way. In all cases concentrations were clearly below both EU MRL and the ADI established. Health-risk assessment also highlights that commercial black tea and herbal products consumed in Spain cannot be considered a serious threat to human health with respect to those OCP analyzed, and that OCP levels in these products have declined over time in this country. However, they should regularly monitor pesticides to ensure public health safety.
Data availability statement
The raw data supporting the conclusions of this article will be made available by the authors, without undue reservation.
Author contributions
RD: Conceptualization, Formal analysis, Investigation, Methodology, Project administration, Software, Supervision, Writing – review & editing, Validation. CL: Software, Writing – review & editing, Data curation, Resources. JMR: Data curation, Software, Writing – review & editing. RP: Resources, Software, Writing – review & editing. BR: Resources, Visualization, Writing – review & editing. EMV: Project administration, Resources, Visualization, Writing – review & editing. MJD: Conceptualization, Data curation, Formal analysis, Investigation, Methodology, Supervision, Validation, Writing – review & editing. NF: Data curation, Investigation, Software, Validation, Writing – review & editing. AMS: Conceptualization, Formal analysis, Investigation, Methodology, Project administration, Software, Supervision, Writing – original draft, Writing – review & editing.
Funding
The author(s) declare that no financial support was received for the research and/or publication of this article.
Acknowledgments
The authors thank Ms. F. Martínez Nuñez, from the Instrumental Techniques Laboratory (University of Leon), for providing technical support and assistance.
Conflict of interest
The authors declare that the research was conducted in the absence of any commercial or financial relationships that could be construed as a potential conflict of interest.
Generative AI statement
The authors declare that no Gen AI was used in the creation of this manuscript.
Publisher’s note
All claims expressed in this article are solely those of the authors and do not necessarily represent those of their affiliated organizations, or those of the publisher, the editors and the reviewers. Any product that may be evaluated in this article, or claim that may be made by its manufacturer, is not guaranteed or endorsed by the publisher.
References
Adenuga, A. A., Ore, O. T., Amos, O. D., Onibudo, A. O., Ayinuola, O., and Oyekunle, J. A. O. (2022). Organochlorine pesticides in therapeutic teas and human health risk assessment. Food Addit. Contam. B 15, 301–309. doi: 10.1080/19393210.2022.2127157
Agency for Toxic Substances and Disease Registry. (2022). Calculating hazard quotients and cancer risk estimates. Available online at: https://www.atsdr.cdc.gov/pha-guidance/conducting_scientific_evaluations/epcs_and_exposure_calculations/hazardquotients_cancerrisk.html#CommonNonCancerGuidelines. (Accessed October 25, 2024)
Agency for Toxic Substances and Disease Registry. (2024). Toxic substances portal. Available online at: https://wwwn.cdc.gov/TSP/substances/SubstanceAZ.aspx. (Accessed June 24, 2024)
Alnawaiseh, A., Arar, S., and Alawi, M. A. (2021). Health risk assessment of residual traces of organochlorine pesticides (OCPs) in infusions from different tea bags brands in Jordanian market: GC/ECD with GC-EI/MS confirmation. Fresenius Environ. Bull. 30, 2989–2997.
Areo, O. M., Olowoyo, J. O., Sethoga, L. S., Adebo, O. A., and Njobeh, P. B. (2022). Determination of pesticide residues in rooibos (Aspalathus linearis) teas in South Africa. Toxicol. Rep. 9, 852–857. doi: 10.1016/j.toxrep.2022.04.001
Chang, K. (2015). World tea production and trade. Current and future development. Rome: Food and Agriculture Organization of the United Nations.
Chinese Nutrition Society (2017). Chinese dietary guidelines. Summary 2016. Beijing: Chinese Nutrition Society.
Drumea, V., Olariu, L., Nita, R., Kamerzan, C., and Florea, M. (2018). The behavior of some pesticide residues in stored medicinal plants samples. Chem. Res. J. 3, 33–43. doi: 10.1055/s-0037-1608508
European Commission. (2024). EU Pesticides Database—MRLs. Available online at: https://ec.europa.eu/food/plant/pesticides/eu-pesticides-database/start/screen/mrls. (Accessed September 12, 2024)
Fattore, E. (2002). Persistent organic pollutants in food: public health implications. J. Epidemiol. Community Health 56, 831–832. doi: 10.1136/jech.56.11.831
Feng, J., Tang, H., Chen, D., and Li, L. (2015). Monitoring and risk assessment of pesticide residues in tea samples from China. Hum. Ecol. Risk Assess. 21, 169–183. doi: 10.1080/10807039.2014.894443
Fernandes, V. C., Domingues, V. F., Mateus, N., and Delerue-Matos, C. (2012). Analysing organochlorine pesticides in strawberry jams using GC-ECD, GC-MS/MS and QuEChERS sample preparation. Food Addit. Contam. A 29, 1074–1084. doi: 10.1080/19440049.2012.682319
Fernández, N., Sierra, M., García, J. J., Diez, M. J., and Terán, M. T. (1993). Organochlorine pesticide residues in black tea, camomile, and linden. Bull. Environ. Contam. Toxicol. 50, 479–485. doi: 10.1007/BF00191234
Fontcuberta, M., Arqués, J. F., Villalbí, J. R., Martínez, M., Centrich, F., Serrahima, E., et al. (2008). Chlorinated organic pesticides in marketed food: Barcelona, 2001–2006. Sci. Total Environ. 389, 52–57. doi: 10.1016/j.scitotenv.2007.08.043
Food of Agriculture Organization of the United Nations. (2024). Crops and livestock products. Available online at: https://www.fao.org/faostat/en/#data/QCL. (Accessed October 22, 2024)
Garrido, M. D., Jodral, M., and Pozo, R. (1994). Organochlorine pesticides in Spanish sterilized milk and associated health risks. J. Food Prot. 57, 249–252. doi: 10.4315/0362-028X-57.3.249
Hayward, D. G., Wong, J. W., and Park, H. Y. (2015). Determinations for pesticides on black, green, oolong, and white teas by gas chromatography triple-quadrupole mass spectrometry. J. Agric. Food Chem. 63, 8116–8124. doi: 10.1021/acs.jafc.5b02860
Jaggi, S., Sood, C., Kumar, V., Ravindranath, S. D., and Shanker, A. (2001). Leaching of pesticides in tea brew. J. Agric. Food Chem. 49, 5479–5483. doi: 10.1021/jf010436d
Jayaraj, R., Megha, P., and Sreedev, P. (2016). Organochlorine pesticides, their toxic effects on living organisms and their fate in the environment. Interdiscip. Toxicol. 9, 90–100. doi: 10.1515/intox-2016-0012
Jeong, H. R., Lim, S. J., and Cho, J. Y. (2012). Monitoring and risk assessment of pesticides in fresh omija (Schizandra chinensis Baillon) fruit and juice. Food Chem. Toxicol. 50, 385–389. doi: 10.1016/j.fct.2011.10.064
Kromhout, D., Spaaij, C. J. K., de Goede, J., and Weggemans, R. M. (2016). The 2015 Dutch food-based dietary guidelines. Eur. J. Clin. Nutr. 70, 869–878. doi: 10.1038/ejcn.2016.52
Landais, E., Moskal, A., Mullee, A., Nicolas, G., Gunter, M. J., Huybrechts, I., et al. (2018). Coffee and tea consumption and the contribution of their added ingredients to total energy and nutrient intakes in 10 European countries: benchmark data from the late 1990s. Nutrients 10:725. doi: 10.3390/nu10060725
Lino, C. M., and Silveira, M. I. N. (1997). Loss of organochlorine pesticide residues during the infusion processes of linden (Tilia cordata Mill.). J. Agric. Food Chem. 45, 2718–2722. doi: 10.1021/jf960941o
Ma, J., Li, X., Ma, S., Zhang, X., Li, G., and Yu, Y. (2020). Temporal trends of “old” and “new” persistent halogenated organic pollutants in fish from the third largest freshwater lake in China during 2011-2018 and the associated health risks. Environ. Pollut. 267:115497. doi: 10.1016/j.envpol.2020.115497
Ma, J., Yu, Z., Liu, S., Chen, Y., Lv, Y., Liu, Y., et al. (2022). Efficient extraction of trace organochlorine pesticides from environmental samples by a polyacrylonitrile electrospun nanofiber membrane modified with covalent organic framework. J. Hazard. Mater. 424:127455. doi: 10.1016/j.jhazmat.2021.127455
Olisah, C., Okoh, O. O., and Okoh, A. I. (2019). Global evolution of organochlorine pesticides research in biological and environmental matrices from 1992 to 2018: a bibliometric approach. Emerg. Contam. 5, 157–167. doi: 10.1016/j.emcon.2019.05.001
Rai, V., Kakkar, P., Singh, J., Misra, C., Kumar, S., and Mehrotra, S. (2008). Toxic metals and organochlorine pesticides residue in single herbal drugs used in important ayurvedic formulation—“Dashmoola”. Environ. Monit. Assess. 143, 273–277. doi: 10.1007/s10661-007-9976-8
Siraj, J., Mekonen, S., Astatkie, H., and Gure, A. (2021). Organochlorine pesticide residues in tea and their potential risks to consumers in Ethiopia. Heliyon 7:e07667. doi: 10.1016/j.heliyon.2021.e07667
Sood, C., Jaggi, S., Kumar, V., Ravindranath, S., and Shanker, A. (2004). How manufacturing processes affect the level of pesticide residues in tea. J. Sci. Food Agric. 84, 2123–2127. doi: 10.1002/jsfa.1774
Szpyrka, E., and Słowik-Borowiec, M. (2019). Consumer health risk to pesticide residues in Salvia officinalis L. and its infusions. J. Environ. Sci. Health B 54, 14–19. doi: 10.1080/03601234.2018.1501144
Taha, S. (2020). A rapid sensitive and selective GC-MS/MS method for multi residue analysis of a large number of pesticides in chamomile. Egypt. J. Chem. 64, 605–622. doi: 10.21608/ejchem.2020.51066.3046
Tea & Herbal Infusions Europe. (2023a). Tea market data. Available online at: https://thie-online.eu/market-data-tea.html. (Accessed September 10, 2024)
Tea & Herbal Infusions Europe. (2023b). Market data herbal and fruit infusions. Available online at: https://thie-online.eu/products/market-data-herbal-and-fruit-infusions.html. (Accessed September 10, 2024)
Üner, O., Torlak, C., and Özcan, C. (2018). Determination of heavy metals and organochlorine pesticides in the leaves and flowers from linden trees in Kırklareli province. Sustain. For. 1:789. doi: 10.24294/sf.v1i2.789
United Nations Environment Programme (2001). Final act of the conference of plenipotentiaries on the Stockholm convention on persistent organic pollutants. Stockholm: United Nations Environment Programme.
United States Environmental Protection Agency (2009). Risk assessment guidance for superfund. Volume I: Human health evaluation manual. EPA-540-R-070-022. Washington, DC: United States Environmental Protection Agency.
United States Environmental Protection Agency. (2024). IRIS assessments. Available online at: https://iris.epa.gov/AtoZ/?list_type=alpha. (Accessed October 24, 2024)
Witczak, A., Abdel-Gawad, H., Zalesak, M., and Pohoryło, A. (2018). Tracking residual organochlorine pesticides (OCPs) in green, herbal, and black tea leaves and infusions of commercially available tea products marketed in Poland. Food Addit. Contam. A 35, 479–486. doi: 10.1080/19440049.2017.1411614
World Health Organization (2010). The WHO recommended classification of pesticides by hazard and guidelines to classification 2009. Geneva: World Health Organization.
World Health Organization (2013). WHO traditional medicine strategy: 2014–2023. Geneva: World Health Organization.
World Health Organization. (2021). Inventory of evaluations performed by the joint meeting on pesticide residues (JMPR). Available online at: https://apps.who.int/pesticide-residues-jmpr-database/. (Accessed September 10, 2024)
Zion Market Research. (2024). Tea market, size, share, growth, trends, and forecast, 2032. Available online at: https://www.zionmarketresearch.com/report/tea-market. (Accessed September 25, 2024)
Keywords: black tea, chamomile, food contaminant, linden, organochlorine, pesticide, risk assessment, Spain
Citation: Diez R, Lopez C, Rodriguez JM, de la Puente R, Romero B, Vazquez EM, Diez MJ, Fernandez N and Sahagun AM (2025) Occurrence and risk assessment of organochlorine pesticide residues in tea and herbal products in Spain. Front. Sustain. Food Syst. 9:1575428. doi: 10.3389/fsufs.2025.1575428
Edited by:
Xiao-Li Yin, Yangtze University, ChinaReviewed by:
Adeoluwa Oluwaseyi Adeleye, Obafemi Awolowo University, NigeriaAreej Merhi, Lebanese American University, Lebanon
Mohsen Farahat, Central Metallurgical Research and Development Institute (CMRDI), Egypt
Copyright © 2025 Diez, Lopez, Rodriguez, de la Puente, Romero, Vazquez, Diez, Fernandez and Sahagun. This is an open-access article distributed under the terms of the Creative Commons Attribution License (CC BY). The use, distribution or reproduction in other forums is permitted, provided the original author(s) and the copyright owner(s) are credited and that the original publication in this journal is cited, in accordance with accepted academic practice. No use, distribution or reproduction is permitted which does not comply with these terms.
*Correspondence: Cristina Lopez, Y2xvcGNkQHVuaWxlb24uZXM=