- Department of Agricultural, Food and Environmental Sciences (D3A), Università Politecnica delle Marche (UNIVPM), Ancona, Italy
Objective: This paper assesses the economic profitability of vertical farms producing microgreens. The study aims to estimate the financial sustainability of this class of farming systems, by classifying the most impacting costs for this sector, and if they are suitable in the Italian territory.
Methods: The study is performed by comparing key performance indicators of two vertical farms in the North of Italy. Data was obtained through semi structured interviews. Key factors considered included variable costs such as energy consumption, raw materials and labor cost, and fixed costs such as amortization and initial investment. The adopted indicators were the operational margin and the cost revenue ratio.
Results and discussion: Findings indicate that, under current conditions in Italy, microgreens vertical farming systems are economically profitable. From the results, it emerges that the real energy consumption is not an impacting factor as stated in literature, along with the important water efficiency of the systems and the capability to digitalize the workflow, supporting the need for technical experts to manage efficiently all procedures. The economic outcome shows a positive operational margin for both companies. The low-cost revenue ratio confirms the financial feasibility of the systems. The framework provides valuable guidance for future research and investment strategies, emphasizing the importance of fundings for agronomic strategies suited to combine profitability and sustainability.
1 Introduction
As a consequence of urban population growth, the need for more sustainable and healthy food has never been more urgent (Salisu et al., 2024). The agricultural sector should find a way to feed an ever-growing world that faces global challenges, like natural resource scarcity (i.e., agricultural area and water) and climate change. To do so, innovative technologies are becoming essential to develop efficient and sustainable solutions (Hajyzadeh and Egi, 2023). Among different alternatives, soilless farming, also called vertical farming or controlled environment agriculture (CEA) has recently garnered attention as a good approach to ensure food for everyone (Benke and Tomkins, 2017). These systems refer to the cultivation of vegetables without traditional soil as a rooting medium (Putra and Yuliando, 2015; Appicciutoli et al., 2025). Hydroponics delivers nutrients via a water-based solution (Swain et al., 2021; Ikrang et al., 2022), aeroponics relies on nutrient mist sprayed directly onto roots without a growing medium (Lakhiar et al., 2018; Kumari and Kumar, 2019), while aquaponics integrates plant cultivation with aquatic species, reducing the need for chemical fertilizers through symbiosis (Goddek et al., 2015; Ibrahim et al., 2023). Vertical farming applies these techniques within vertically oriented structures, optimizing space and resource efficiency (Appicciutoli et al., 2025). These systems offer several significant advantages, including optimized water usage, minimal or no pesticide application, and higher yields. However, it is important to note that vertical agricultural technologies have still limited application in agriculture, but at the urban level, they have qualified as a means for urban farming (Despommier, 2013; Bhargaw and Chauhan, 2020). It is worth noting that worldwide, 800 million people are involved in urban and peri-urban agriculture (Food and Agriculture Organization, 2022). As urban populations grow and rural-to-urban migration increases, urban agriculture will gain recognition for its benefits and services (Orsini et al., 2013). In this context, the revitalization of peripheral areas and the repurpose of abandoned industrial buildings present valuable opportunities for expanding vertical farming. These spaces, often overlooked, can be transformed into productive agricultural hubs, helping to address the growing demand for food while reducing urban brownfield (Boganini and Casazza, 2016; Zhang et al., 2021).
Although vertical farms are frequently acknowledged for their efficiency and sustainability, they also present significant obstacles as identified in the literature (Srivani and Manjula, 2019; Bihari et al., 2023; Martinez, 2024; Kumar and Verma, 2024; Sharma et al., 2024). The main disadvantages of vertical systems are:
i. High level of technical expertise: operators need specialized knowledge to manage systems and maintain optimal conditions for plant growth.
ii. High initial investment: setting up vertical systems is expensive due to the need for equipment, infrastructure, and technology.
iii. Complex monitoring requirements: factors such as pH, electrical conductivity, and nutrient concentration must be closely monitored and adjusted regularly in accordance with the vegetable species.
iv. Energy dependency: vertical systems entirely need electricity for lighting, pumps, and climate control.
However, while research efforts have explored those technical and agronomic aspects, studies on its economic implications remain scarce and recently investigated.
Banerjee and Adenaeuer (2014) aimed to construct a profitable vertical farm and investigate its economic feasibility. Avgoustaki and Xydis (2020) compare traditional farming with greenhouses and indoor vertical farming, focusing on the challenges and opportunities for each category. Most recently, the work of Souza et al. (2023) builds upon these studies by focusing on small rural properties in southern Brazil and conducting an economic analysis of lettuce production, advancing the research in this field. According to a sensitivity analysis and Monte Carlo simulation, the paper shows that even when exposed to risk and uncertainty, the hydroponic project proposed remains attractive and economically feasible. The study also highlights the potential for financing through funds from the National Program for Strengthening Family Agriculture. This program provides access to funding options such as subsidized loans, grants, and rural credit at favorable interest rates for Brazilian family farms.
A different approach is taken in the paper by Michalis et al. (2023), which explores the feasibility of hydroponic tomato greenhouse farms situated in Greece. The work demonstrates that, despite the high implementation and operational costs, the investment proves beneficial under the current market circumstances and prices, in accordance with three hypothetical scenarios. The three situations explored variable costs and pricing changes for hydroponic tomatoes. Scenario 1 featured a 100% increase in variable costs and a 10% rise in producer prices. Scenario 2 involved an 80% increase in variable costs with a 40% state subsidy for installation costs, based on Greece’s Rural Development Program. Scenario 3 combined an 80% rise in variable costs, a 20% increase in installation costs, and a 5% increase in producer prices. Another model is explored by Mishra et al. (2024), through a comparison between hydroponic and conventional farms in India. The study analyzed initial set-up costs, operating costs and yields of real farms. Apart from the higher initial investment for hydroponic compared to traditional farming, hydroponics has lower annual operating costs because of reduced labor and water requirements. Additionally, hydroponic systems demonstrate higher yields for crops such as tomatoes (15 kg/m2) and lettuce (30 kg/m2) compared to conventional farming. While the first two papers explore hypothetical scenarios, this is the only paper that evaluates economic profitability of soilless systems in real farms.
The findings from previous studies reveal significant gaps in the existing literature, particularly concerning the economic profitability of soilless agriculture. The insufficient focus on this component is a critical issue required to transcend theoretical assumptions and evaluate the actual performance of real-world farms. Therefore, the objective of this study is to deliver a thorough empirical evaluation of the profitability of hydroponic production, utilizing data gathered from semi-structured interviews in two Italian case studies and considering both variable and fixed costs to establish Key Performance Indicators (KPIs) for assessing the economic viability of this innovative systems.
Among other countries, Italy is one of the most active in microgreens research, a field that is emerging and rapidly growing (Ferreira et al., 2024). Europe’s vertical farming market is projected to grow at an annual average rate of 22% over the next 5 years, reaching approximately $5.11 billion by 2029 (Vgreens, 2025). Given that cost remains a primary challenge, this research represents a significant advancement in the field. It provides critical insights for the scientific community, fosters greater interest in vertical agriculture, and offers essential guidance for policymakers and farmers to make informed decisions. This study is not merely theoretical; it presents real data, underscoring its relevance in the broader context of the ongoing green transformation.
2 Materials and methods
In this section, the selected case study selection and data collection (Section 2.1), case study description (Section 2.2) and the data analysis (Section 2.3) will be described.
2.1 Case selection and data collection
Figure 1 provides a summary of the research framework, including sampling acquiring, theoretical sampling, data collection, case construction and data analysis.
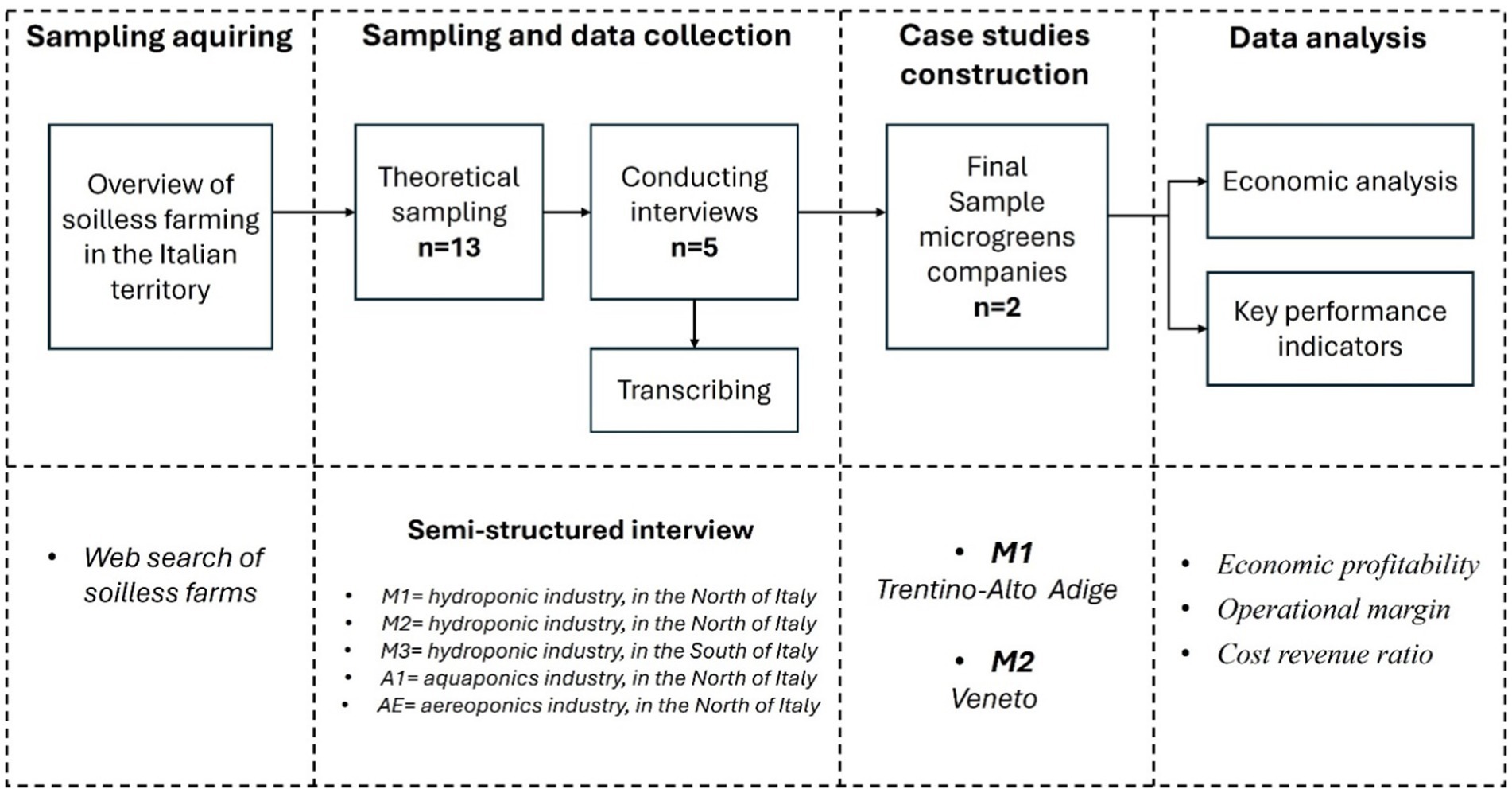
Figure 1. Overview of the four steps of the study, starting from the left: sampling acquiring, sampling and data collection, case studies construction and data analysis. Own source.
This study is based on Yin’s case study methodology. This methodology provides a structured framework for investigating contemporary phenomena within real-life contexts, making it particularly suitable for analyzing complex, context-dependent issues like the economic profitability of vertical farming. The sample selection aligns with this approach, as it focuses on a current issue defined by a small number of early adopters of vertical farming (Yin, 2018). The current issue is defining the economic profitability of vertical farming systems in Italy. In this context, a quantitative analysis alone would not be sufficient. The case study method is the most appropriate because the issue is context-dependent and requires detailed exploration of specific real-world cases to capture the complexities and challenges unique to each farm. The applied methodology encompasses the adoption of semi-structured interviews. A semi-structured interview is a qualitative research method that combines predefined questions with open-ended discussions. Semi-structured interviews fit perfectly for these cases, as they allow for flexibility in exploring the perspectives of farm operators while ensuring that key topics relevant to the research are covered. Additionally, this method enabled the acquisition of a wide variety of data covering several soilless systems, without being restricted to a single technology (e.g., not only hydroponics or aquaponics, but other systems and their adaptation to the farm) (Kallio et al., 2016).
Since the aim of this study is to evaluate the economic profitability of vertical farms in Italy, it is important to find businesses that can explain their reality. A web search for any vegetable producers using soilless technology was done to identify potential candidates for the sampling acquisition. Thirteen Italian businesses that use soilless technology were identified based on this. Potential subjects were contacted via email, with one follow-up email sent a week later if no response was received. However, only five of the 13 companies agreed to participate to the analysis (interview response rate: 38%). As stated, technique used to gather data include semi-structured interviews based on an ad hoc protocol.
The accuracy of the protocol was ensured by the approval of six researchers prior to data collection. In detail, the interview protocol was divided into 9 sections, arranged as follows. The first section explored the general aspects of the company, and the role of the person interviewed. Section 2 investigated the adopted technology. The third section explored the economic profitability of vertical farming through questions about:
• Growth area (including all vertically stacked growth layers).
• Initial investment costs (land, infrastructure, and technology)
• Initial funding (private investors, public funds, etc.)
• Main operational costs (maintenance, insurance, licenses, energy and water consumption, raw materials, external consulting, and staff costs)
• Depreciation of vertical systems
• Current annual revenue.
Sections 4 and 5 analyzed the product and financing aspects, respectively, by investigating:
• Average selling prices of products
• Annual production volume (tray) and total production data
• Product margin
• Initial funding.
Section 6 assessed the level of the sales market, while the seventh section evaluated the general company’s performance. The concluding sections, 8 and 9, focused on sustainability and environmental impact and the corporate legal framework.
The interviews were conducted in October 2024 and lasted between 65 and 158 min. Four farms had in-person interviews, and one company used video communication software (Google Meet). Each interviewee provided the informed consent form. To preserve all the subjects’ information, interviews were transcribed verbatim. Transcripts were subsequently subjected to further analysis to build the case study in alignment with the research objectives. Starting from a transcribed sample of five companies (n = 5), and following the methodology proposed by Flick (2015), the final sample included two farms (n = 2) that were suitable for the analysis since they provided viable economic indicators. According to this author, this phase of the analysis pathway is the case construction, which refers to the process of selecting, defining, and structuring the cases to be studied in qualitative research. It involves choosing cases based on theoretical assumptions or empirical observations to ensure they align with the research objectives. In this study, the selected companies were chosen for their comparability, as both produce the same vegetable (microgreens) using the same vertical farming technology (hydroponic), making them suited to answer the research question.
Microgreens have become one of the most widely adopted crops in vertical farming due to the ease of their cultivation using hydroponic systems (Zhang et al., 2021). Despite their growing popularity, it is important to note that, to date, limited research on the economic feasibility of microgreens as a vertically produced vegetable is present.
2.2 Case study description
To clarify the case study and ensure complete comprehension of these new products, it is important to provide further details about microgreens. Microgreens can be defined as immature edible greens, characterized by their peculiar texture and their intense flavor (Singh et al., 2024). Depending on the species, microgreens are generally collected from 7 to 21 days after germination. The edible part comprises stem and cotyledons, usually harvested when they are 5 to 10 cm long.
The term “microgreens” is not properly scientific, it is used for marketing purposes (Wojdyło et al., 2020). They should not be confused with similar products such as sprouts or baby greens that have different characteristics (D’Imperio et al., 2016; Ansah et al., 2018; Peng and Simko, 2023; Rouphael et al., 2021; Ebert, 2022). A detailed classification of microgreens and other products is illustrated in Figure 2.
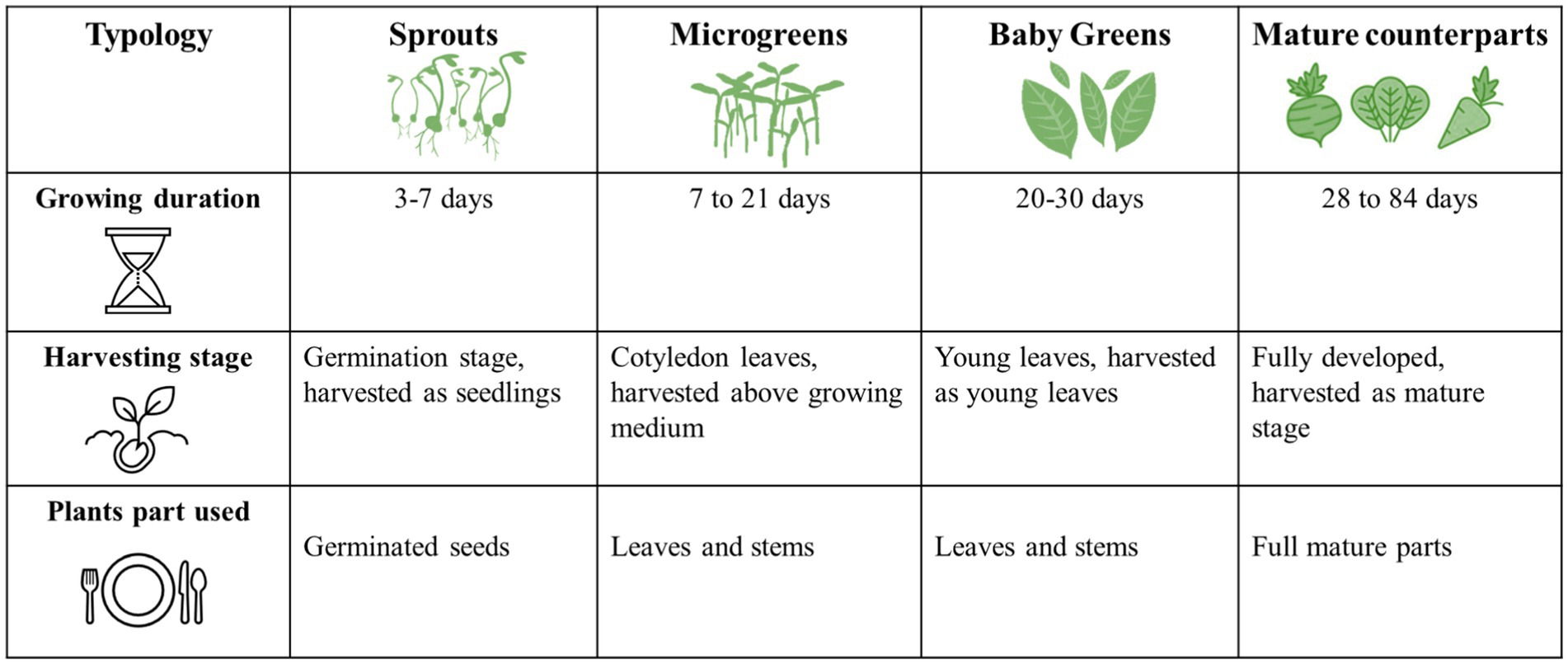
Figure 2. Sprouts-microgreens-baby greens and mature counterparts’ classification. Days of growing, harvesting stage, and vegetative part used are ascribed for each category. Own elaboration adapted from Gunjal et al. (2024).
Common varieties of microgreens include amaranth, mustard, parsley, radish, cabbage, celery, chard, chervil, coriander, cress, fennel, kale, rocket, beetroot, basil and sorrel. Sometimes cereals such as rice, oats, wheat, maize and barley are also cultivated, as well as legumes such as chickpeas, beans and lentils (Puente et al., 2024). Microgreens are rich in bioactive compounds, especially secondary metabolites or phytochemicals, providing antioxidants, anti-inflammatory, and potential anticancer benefits, making them a valuable dietary addition (Galieni et al., 2020). Due to their numerous advantageous attributes, microgreens are increasingly integrated into the current market.
They are usually cultivated in small enterprises due to the fact they do not need high-tech equipment. This shows how possible it is to grow these crops in small spaces using vertical farming technology. Other articles have already explored the possibility of growing microgreens in small places like containers or garages in cities (Michelon et al., 2019; Astapova et al., 2021). Thanks to this spatial flexibility, microgreens are often sold close to where they are produced, creating sustainable short supply chains. The potential of distributing goods in a short supply chain is associated with reduced distance to distribution, less quality losses during handling and transportation, and closer manufacturing to the target market (Puente et al., 2024). Microgreens’ segment corresponds mainly to high-cuisine restaurants. However, in developed countries, the population is willing to pay a high price to obtain good quality, local and nutritious products, so the market segments are expanded to organic supermarkets and local markets (Yanes-Molina et al., 2019). Considering commercial distribution, the study of Yanes-Molina et al. (2019) localizes three distribution pathways represented in Figure 3.
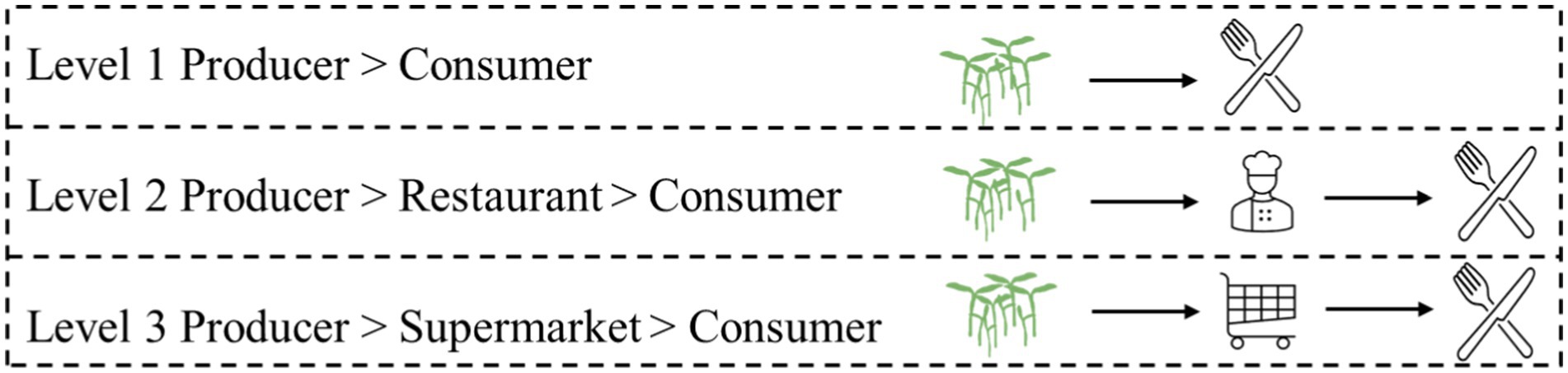
Figure 3. Representation of the three levels of microgreens distribution pathways in accordance with the actor of the food supply chain. Adapted from Yanes-Molina et al. (2019).
The Italian scenario encompasses only the first two levels, excluding the presence of microgreens in supermarkets. They are sold directly from producers to consumers and/or restaurants.
Considering the interest from the scientific community, United States, Italy and India represent the most active countries on this subject (Ferreira et al., 2024). Driven by their high revenue potential, among the variety of indoor crops, in United States microgreens stand out with a recorded profitability of 60% (State of Indoor Farming Report, 2017). The microgreens market is globally spread in regions including the United States, Canada, Asia, and Australia. This growth was driven by increased demand from chefs and the cosmetics industry. As a result, the market is becoming increasingly competitive on a global scale (Paraschivu et al., 2021). Figure 4 represents the microgreens market size by region in 2020.
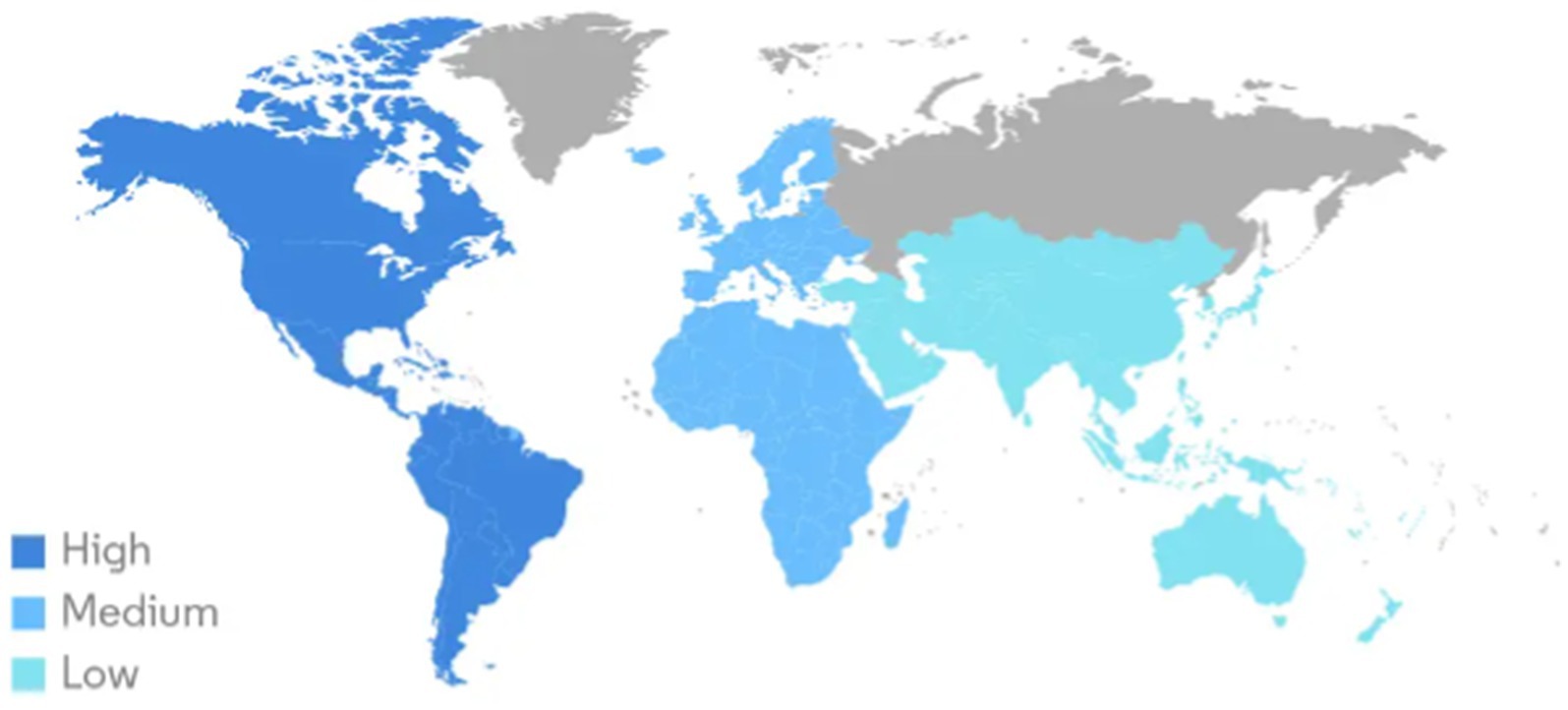
Figure 4. Global microgreens market—market size by region in 2020. The darkest color represents the highest market relevance, while the lightest represents the lowest. Source: Paraschivu et al. (2021).
After this complete overview of the production and microgreens, the two case studies can be described. The two microgreens’ farms considered are indicated as M1 and M2 throughout the text.
M1, located in Trentino Alto Adige (northern Italy), specializes in producing organic-certified sprouts and microgreens using vertical farming techniques. Their products are distributed through the Ho.Re.Ca. channels (Hotels, Restaurants, and Catering). With a real cultivation area of 150 m2, in accordance with the total shelf surface within the growth chamber, they achieve a production capacity of 300,000 microgreens trays per year. The company employs 8 workers, with an initial investment of 320,000 € in 2020. Among the two farms analyzed, M1 is the only company to have received regional funding to support its operations.
M2 is situated in Veneto, northern Italy, and specializes in hydroponic microgreens production. This small family-run business employs 2 workers, and it was born in 2020 with an initial investment of 300,000 €. Their products are distributed exclusively through the Ho.Re.Ca. channels. Operating with a real cultivation area of 64 m2, they produce up to 46,000 microgreens trays annually.
The work presented contains certain peculiarities; thus, a prior clarification is necessary for the comparison between the two companies. An initial elucidation should be made regarding the selling unit. While it is common to use kilograms or individual units for other vegetables products, applying these units to microgreens may not be a suitable approach. The microgreens analyzed in this study are sold live with their growing medium, which helps to extend their shelf life when kept under optimal conditions. The average dimensions of the trays in which they are sold are similar for both companies, measuring 13.5 × 7 cm. The edible portion per tray typically weighs 35 grams (slight variations can occur). Based on this, the paper will refer to trays (microgreens bowls) as the production unit throughout the article. This approach allows the comparison between the two farms. If needed, knowing the grams per tray, it is possible to calculate economic indicators per kilogram. However, the choice to express data per tray arises from the need to protect the privacy of the two farms.
A second aspect that must be highlighted is the nature of production throughout the year. In traditional farms, production is generally limited to seasonal cycles. This is not the case with vertical farming, where plants can grow over an extended-continual period (Martinez, 2024). With microgreens, production remains constant throughout the year. Additionally, production is continuous thanks to the stability of the local demand (microgreens are distributed to the Ho.Re.Ca. sector locally).
This leads to a third point: due to the continuity of production, the labor needed for harvesting and other farming operations is also constant. For this reason, through the paper, labor costs will be considered as fixed costs rather than variable (as usually intended in traditional farms).
Moreover, as the growing time is relatively short, usually microgreens do not need pesticides. Due to the fact neither M1 nor M2 uses pesticides, they are not part of variable costs. The absence of pesticides adoption cannot be awarded by the organic certification; as current regulations require organic crops to be grown in soil to qualify for the organic label. However, by selling microgreens as sprouts (<7 days after germination), M1 overcome regulation boundaries gaining the organic certification for their microgreens.
A last assumption to be underlined is the strong differences of the microgreens’ farms under analysis. If from one side we have strong-rooted companies with 8 workers such as M1, on the other side M2 is a small family business that comprises two members and a relatively small production. These differences were deeply investigated in the results section using the KPIs. A description summary is represented in Table 1.
2.3 Data analysis
The economic analysis aims to evaluate the economic profitability of microgreens produced in Italian vertical farms. According to the case study construction, the economic analysis attempts to examine farms profitability by comparing two case studies: farm M1 and farm M2. Focusing on the economic sphere, collected data concerning the economic profitability of microgreens refers to 2023 production. This economic performance was evaluated in accordance with the method proposed by other similar articles considering fixed and variable costs and some indexes (Lazo and Gonzabay, 2020; Finco et al., 2021; Bentivoglio et al., 2022; Staffolani et al., 2024). All production costs were gathered through in-depth semi-structured interviews. Based on this data, comparisons were made, and observations were drawn. To assess the economic performance and profitability of the two farming models the study categorizes costs into fixed and variable. The first evaluation focused on variable costs, which include the expenses incurred annually for cultivation operations in a vertical farming system.
The fixed costs include labor (salaries and wages), amortization and structure maintenance, insurance and rental. Amortization costs refer to partially depreciable goods, the only cost considered in this case is the amortization of vertical systems. The detail of variable and fixed costs is listed and descripted in Table 2.
Total cost refers to the sum of all expenses incurred by the two farms including both variable and fixed costs. Equation 1 shows the total cost formula:
To calculate total costs, the annual costs for each category were initially considered. The annual percentage of variable costs was then determined by dividing the total annual variable costs by the total annual costs and multiplying by one hundred. For comparison, the total annual costs were subsequently converted into per-tray costs. This approach provides a comprehensive understanding of cost distribution while maintaining privacy for both farms. The other factor in this economic sphere is represented by revenues, described as the total income generated by a business from selling its goods or services. It is calculated as the price per unit (tray) multiplied by the quantity sold in 1 year (Equation 2). In this case, trays of microgreens are sold to the Ho.Re.Ca. sector, which represents businesses in the food service and hospitality industry.
The difference between revenues and total costs results in a third important economic component: profit. It represents the financial gain a business makes after covering all its expenses (Equation 3). If profit is positive, the business is making money; if negative, it is incurring a loss.
Knowing the initial investment of both farms and their annual net profit, the payback period can be calculated. The payback period represents the time required for an investment to generate enough net profit to recover its initial cost. It is a key financial metric for assessing the risk and efficiency of an investment, with shorter payback periods indicating quicker returns and lower financial risk. It is calculated following the formula (Equation 4):
All those factors were considered during the assessment of the economic profitability of the farms. Economic profitability refers to the ability of a business or investment to generate financial returns (revenues) that exceed their total economic costs.
Moreover, the economic efficiency of the case studies was calculated through two KPIs: the operating profit margins and the cost-revenue ratio. The first indicator is a profitability ratio that expresses operating profit as a percentage of total revenue. It indicates how efficiently a company can generate profit through its core operations, and is represented by Equation 5:
where the operating profit corresponds to Earnings Before Interest and Taxes (EBIT). High operating profit margins indicate effective management of operating costs from a company (Marr, 2012). The second KPI is a measure of the efficiency that compares company’s expenses to its earnings (Equation 6):
A lower cost-revenue ratio means that a company can produce more using fewer resources.
Based on the analyzed data, it was decided to return to the interviewees after processing the interviews to add any missing information and allow them to review the content. This approach aims to prevent misunderstandings and enhance the reliability of the study’s methodology.
3 Results and discussion
In this section the results of the analysis were discussed. Table 3 provides production costs, total costs, and revenues of the farms considered in the study.
3.1 Variable costs
Following Table 3 flow, the first impacting variable costs are consumables. This expenditure includes seeds, growing medium, and packaging materials. Packaging represents the highest cost for both M1 and M2. Looking at the same table, those consumables accounts for the highest cost for both M1 (13%) and M2 (24%). To better understand the relevance of each variable cost, in Figure 5, the annual percentage of only variable costs impact is shown.
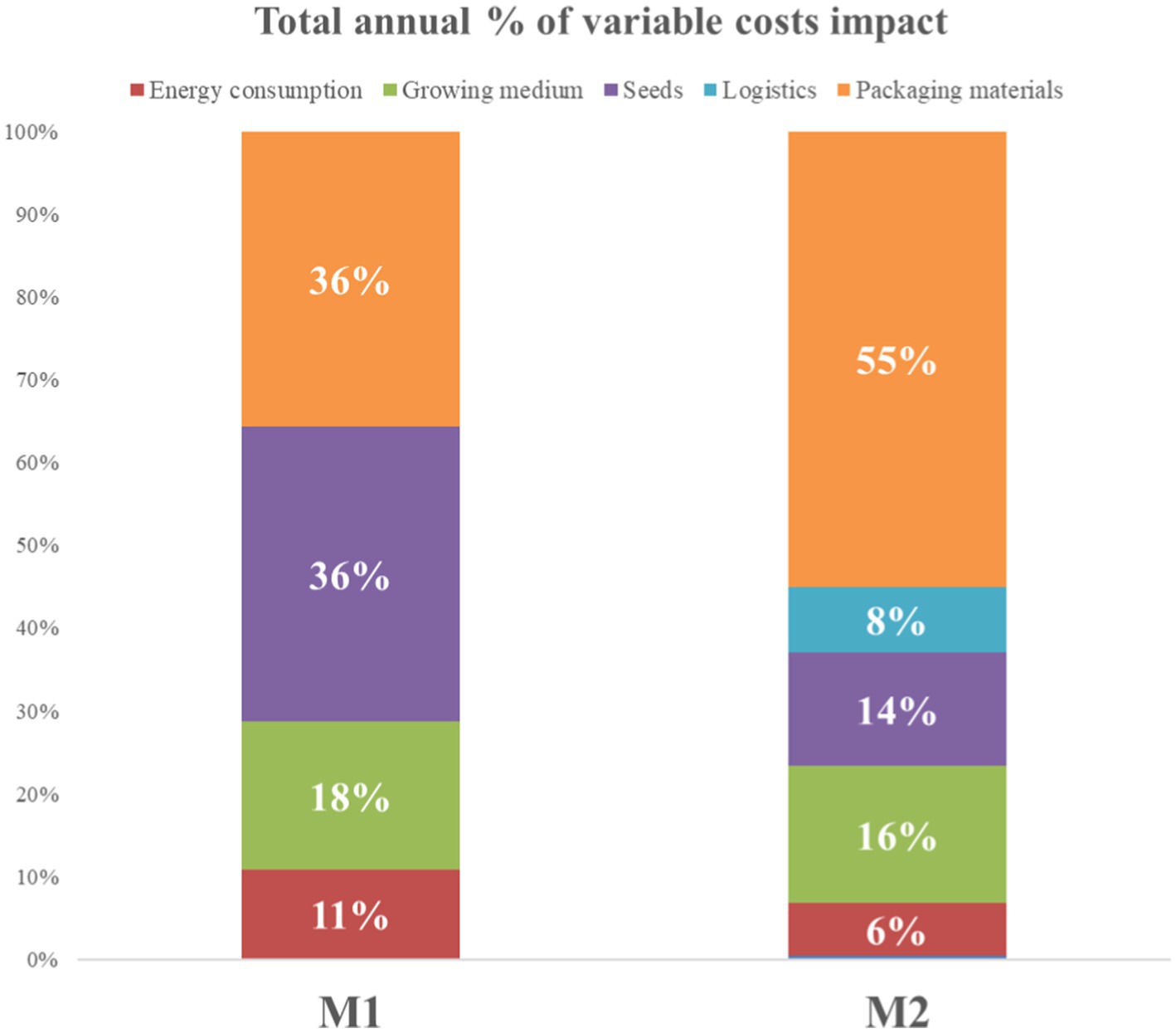
Figure 5. Total annual percentage of variable costs impacts for M1 on the left and M2 on the right. Our elaboration.
As shown, for M1, the cost distribution is more balanced, with packaging materials (36%) and seeds (36%) contributing similarly, indicating how raw material inputs are significant factors. The growing medium accounts for 18%, while energy consumption takes only 11%. Notably, water consumption is 0.14%, suggesting that water is a negligible cost and is managed very efficiently in hydroponic systems. Due to this minimal amount, in the order of 0.14% for M1 and 0.38% for M2, water consumption is not visually represented in Figure 5 for both farms.
In terms of the total annual percentage of variable costs, M2 shows a different profile. Packaging materials dominate at 55%, suggesting that a small farm could have issues accessing packaging materials suitable for microgreens production at affordable prices. Reflecting on this, it is important to consider the peculiarity of packaging materials gained from the semi-structured interview. Microgreens are sold in their growing trays, which means the materials must be suitable for prolonged water contact throughout the entire period. Given the size of the market, it seems reasonable to attribute this disparity to a lack in terms of specialty tray makers. Because microgreens are marketed with their moisture-retaining substrate, their packing materials differs from those for common vegetables. By employing recycled substrates created by different agri-food waste and trays made by paper, M1 adopts an eco-friendly strategy. In addition to cutting expenses, this also implies a stronger bond with the sellers of these specialty products. M2, on the other hand, employs traditional peat as a substrate, and its plastic trays are better suited to the wetter conditions that the peat creates.
Going on with the cost considerations, for M2 the share for seeds drops to 14%, implying that raw material input is less critical in M2 than in M1. The growing medium is similar at 16%, energy consumption is reduced to 6%, and water consumption is still at 0.38%, which might imply it is not a significant cost factor and is very efficiently used in both companies. Moreover, logistics represent an additional cost for the M2 farm, accounting for 8%, whereas this expense is borne by the buyers in M1. This extra service offered by M2 could be reasonably connected to the capability to sell the product at a higher price, given the fact they offer an additional service.
Scientific studies commonly highlight energy costs as a major expense in soilless cultivation (Barbosa Lages et al., 2015; Casey et al., 2022). For example, a comparison between soil-based and soilless systems shows that the former requires 29,808 kWh/ha of electricity compared to just 629 kWh/ha for soilless systems, representing approximately 4,637% more energy consumption (Maestre-Valero et al., 2018). According to the study by Cai et al. (2025), the energy consumption for soilless farming for the same crop is over 17 kWh/kg, while greenhouse farming consumes only about 3 kWh/kg, and open-field farming consumes around 1 kWh/kg. This highlights the significantly higher energy requirements of soilless farming compared to traditional farming methods. Although this comparison does not specifically focus on microgreens, it provides insight into the broader energy consumption patterns of soilless cultivation systems that reflect on costs. If it was reasonable to assume energy as critical costs, the data obtained in this study indicates that energy account for only 4% of the total costs for M1, where they represent the only significant operational expense as the company does not incur logistics expenses. In contrast, for M2, energy costs make up 3%, with logistics contributing an additional 4%, resulting in a combined operational cost of 7%. It can be hypothesized that these results are linked to the high level of digital progress in modern systems, which are now capable of consuming less energy thanks to technical advancements. Another contributing factor is that microgreens, as previously mentioned, have a short growth period, which reduces the operational time of energy-dependent systems. Compared to leafy vegetables, which usually need longer photoperiods, typically microgreens require less than 12 h. During the initial germination phase, which lasts around 2 to 5 days, the seeds only need a warm and moist environment, without the need for light. Considering that growth cycle lasts from 7 to 21 days, with a part of those days in darkness, this phase relies on the seeds’ stored energy, further reducing energy consumption in the early stages of growth (Peng and Simko, 2023; Amitrano et al., 2023). The need to clarify the specific energy consumption of microgreens further emphasizes the importance of such an analysis.
In summary, the primary variable costs for M1 and M2 are consumables, with packaging materials representing the highest expense (36% for M1 and 55% for M2), highlighting challenges in accessing affordable specialty trays for microgreens. Seeds are a significant cost for M1 (36%) but lower for M2 (14%), while energy costs are low (4% for M1 and 3% for M2), due to technological advancements and the short growth cycle of microgreens. Water consumption remains negligible (0.14% for M1 and 0.38% for M2), confirming the efficiency of hydroponic systems in managing this resource.
3.2 Fixed costs
Labor costs emerge as the most significant expense. Labor represents 46 and 41% of the M1 and M2 costs, respectively. Some consideration regarding labor cost should be made to clarify the impact of this item.
In vertical farms, labor is a fixed cost due to the lack of microgreens seasonality and continuous production. For M1, labor costs include the salaries of the two founders, who handle engineering tasks and coordination, along with six waged workers.
Additionally, costs related to digital technology are included, as an engineer is responsible for developing and maintaining software and managing digital systems. This engineer, contracted as part of the operational team, focuses on system optimization, troubleshooting, and seamless digital integration.
The team also includes an agricultural technician essential for crop management and adapting technologies to agronomic needs. While unskilled workers handle daily production, digital technology supports operations, reducing overall labor costs. However, the specialized technician remains crucial for optimizing production and ensuring system efficiency.
This aspect is not in line with the broader scientific consensus regarding soilless production. Numerous articles recognize vertical farming as an agronomy practice that requires a higher level of knowledge from the employees (in comparison with conventional soil farming practices) (Khatri et al., 2024). M1 addresses this issue with a higher degree of digitalization. By using tablets where the production process is entirely digitized, M1 enables employees to efficiently grow microgreens, even without strong agronomy training from the waged workers. This shift lowers labor costs, as specialized agronomy knowledge is partially supported by digital systems.
In M2, the distinction in family work is different, family members take on not only operational tasks but also managerial and logistical responsibilities, often working longer hours. This leads to higher labor costs compared to M1, where digitalization helps reduce labor expenses. The information was derived from the semi-structured interview. In this context, the entrepreneur of the farm receives a salary, while other family members receive wages, as their responsibilities are less expensive.
A comparison of the two companies reveals notable differences in amortization. M1 incurs vertical system amortization costs of 14% per tray, based on a five-year amortization period. M2, however, no longer considers amortization expenses, having fully settled this cost component after 1 year.
Even if M2 has already accounted for the amortization of its vertical structures the farm pays monthly rental costs for its buildings.
Structural costs include maintenance and insurance. M1 is responsible for both maintenance and insurance. For M1, these amount to 3% for maintenance and 1% for insurance, with a total of 4%. In contrast, M2’s structure costs consist solely of rent, which accounts for 16%, making it a more significant expense than amortization. In fact, M2’s rental costs exceed M1’s amortization expenses, underlining the importance of infrastructure in M2’s operations.
Table 3 reveals that, for M1, total costs amount to 1.55 €, with revenues of 1.95 €. For M2, total costs are higher at 2.54 €, but revenues also increased to 3.90 €. Both farms are profitable, but while M2 operates with higher costs, it generates a higher profit compared to M1.
As stated before, the two farms under analysis are completely different but profitable. Since M1 has a well-structured cost allocation, it could reasonably serve as a reference model for microgreens production in future research and analysis.
In summary, labor costs represent a major expense for both farms, with M1 reducing costs through digitalization, while M2 incurs higher labor costs due to family involvement in various roles. Additionally, M1 includes amortization and insurance as part of its structure quotas, whereas M2 does not have these cost components but instead allocates 16% to rental expenses, resulting in higher overall costs.
3.3 Microgreens farms economic performance
Table 4 provides a comparison of the cost and revenue between M1 and M2, highlighting total costs, revenues, operational profit, operational margin, and cost-revenue ratio.
With an operational margin of 34.87%, which is substantially greater (+70%) than M1’s value of 20.47%, M2 exhibits superior economic performance based on the KPIs displayed in Table 4. This difference is further reinforced by the cost revenue ratio. In fact, M2’s ratio of 0.65 indicates the requirement of less costs to generate its revenue. Despite these differences, both M1 and M2 can be considered financially healthy farms. A deeper analysis of M2’s higher operational margin must be done. As a family-run business, M2 reinvests part of its own profits back into production. The higher selling prices are dependent on M2’s strategic focus on a specific production area. The company benefits from an absence of competition and, thanks to the foresight of its management, has positioned itself within the high-quality Ho.Re.Ca. channels in its local area, offering a full-service model that includes weekly microgreens distribution. On the other hand, M1 is a larger, well-structured company with a well-defined marketing strategy. By adopting advanced digitalization to simplify production processes, the company operates effectively even with non-specialist employees. The extensive use of tablets and digitized workflows makes managing microgreens production more efficient, reflecting how M1’s structured approach supports its market position. Additionally, it is reasonable to think that the M1 scale economy also depends on access to specific regional funding programs.
M1 and M2 exhibit notable differences in both costs and profitability. M1 maintains lower production costs at €1.55 per tray, resulting in a limited profit of €0.40 based on its revenue of €1.95. In contrast, M2 incurs higher costs of €2.54 per tray but it generates substantially greater revenues of €3.90 per tray, yielding a much higher profit of €1.36. Considering the overall profitability, the net profit margin further demonstrates the economic profitability of the farms. M1 shows a net profit margin % of 20,47% while M2 31%. Considering the initial investment, M1, with an investment of €320,000, achieves a payback period of around two and half years, indicating a faster recovery in its investment compared to M2. In contrast, M2, with an investment of €300,000, has a payback period of around five and half years, suggesting a longer time to recover its initial costs and potentially higher financial risk.
In summary, M2’s higher operational margin results from its marketing strategy with a strong market positioning, by offerings high-quality product, and a full-service model, while M1’s financial success stems from its scale, digitalization, and streamlined processes. Both companies maintain strong financial health, but their business models differ significantly.
4 Conclusion and studies implications
This study demonstrates the growing importance of vertical farming systems as a real economic entity in the agrifood sector. Through the analysis of two case studies in Italy and a comparison with existing data, this work demonstrates the economic profitability of vertical farming systems involved into microgreens production. By focusing on microgreens, this study further contributes to the limited body of research evaluating the economic potential of such crops. Data obtained from the semi-structured interviews suggests that, while challenges such as high energy costs, the need for technical expertise, and constant monitoring are commonly cited in the literature, they may not pose the same level of critical barriers to the feasibility of these systems in the specific contexts of the two farms studied. However, as noted in the limitations section, these findings may not be broadly generalizable given the limited scope of the analysis. In accordance with our results, the challenge of technical expertise may be counteracted through the high level of digitalization of the systems. The complex monitoring requirements can also be efficiently addressed with advanced systems and sensors that reduce the burden on operators. Moreover, while energy dependency remains a consideration, it does not significantly impact the operation. In accordance with the strong M1 financial reality, it is reasonable to think that access to funding can result in well-structured farms. However, the high initial investment remains a major challenge representing the suggested focus for future research that could pose attention to fundings access at national or European level.
Also, certifications could play a cardinal role for the expansion of these farms considering that vertical farming products are not eligible for organic certification (current regulations require organic crops to be grown in soil to qualify for the organic label). Remarkably, these companies have achieved success at the national level without consistent support. To overcome this barrier, policymakers should consider providing targeted funding to enable farms to transition into these sustainable systems.
In accordance with the aspects discussed in this study, several theoretical, empirical and managerial implications could be identified.
Starting from policymakers, it is crucial to offer subsidies and financial support to mitigate the high initial investment costs of vertical farming systems, thereby promoting their growth and accessibility. Encouraging the integration of renewable energy sources into these systems could further lower operational costs while enhancing economic feasibility and environmental sustainability. One effective solution is the adoption of photovoltaics, which, despite requiring a significant initial investment, can lead to substantial long-term energy savings and should be carefully assessed.
Furthermore, clarity at the regulatory level is essential to include microgreens into effective policies and strategies for a sustainable, accessible, and low-impact agriculture of the future.
From a managerial aspect, the resilience of vertical farms that have survived without substantial funding highlights the adaptability of these systems. However, forming cooperatives among small-scale farmers could strengthen their ability to share resources, access bulk discounts, and adopt advanced technologies.
On a societal level, public awareness campaigns are essential to emphasize the benefits of vertical farming, increasing acceptance and encouraging greater consumption.
Moreover, urban farming integrated with soilless technologies presents significant opportunities, including the revitalization of unused urban spaces, the establishment of short supply chains, and reduced transportation costs, all of which contribute to sustainable urban development and environmental preservation. Defined as the practice of cultivating, processing, and distributing food within or around urban areas, urban cultivation aligns with the scientific community’s strong interest in soilless farming as a strategy that citizens could adopt in urban settings. As more people in cities become interested in growing food, technology makes it easier for them to try soilless farming, even without a background in agronomy. Soilless farming systems are automated and require less knowledge of soil health, while technology ensures precise control over water, nutrients, and environmental conditions, making farming more accessible.
This suggested improvements, would support the large-scale adoption of vertical farming technologies and contribute to more sustainable agricultural practices.
5 Limitations and future research
The study’s limitations are primarily constrained by the poor generalizability of the findings. These findings are contingent upon the limited sample size (n = 2), the economic feasibility being confined to hydroponic microgreens, and the study being restricted to a single country, Italy.
As a result, the results may not be easily transferable to different contexts without further research. These limitations also affect the strength of the conclusions drawn, especially regarding the elevated level of digitalization as a tool for improved workflow management. While the semi-structured interviews provided valuable insights that could not be captured through quantitative analysis, this approach must be considered within the context of the study’s limitations. The focus on microgreens trays could represent an additional limitation of the study, as it may limit broader generalizability.
Therefore, while the findings are reasonable for the case study analyzed, their broader validation in other contexts remains limited.
Based on this limitations, future studies should expand the sample size by improving generalization. Moreover, increasing sample size and comparing other crops could further demonstrate the economic feasibility of vertical farms. Lastly, it would be necessary to analyze the economic results of other vertical farms in the European area to verify economic profitability of those practices.
Regardless, by demonstrating the economic feasibility of hydroponic production, this work serves as a milestone for future initiatives, setting the foundation for continued progress in these areas.
Data availability statement
The raw data supporting the conclusions of this article will be made available by the authors, without undue reservation.
Ethics statement
Ethical review and approval was not required for the study on human participants in accordance with the local legislation and institutional requirements. Written informed consent was obtained from the [patients/ participants OR patients/participants legal guardian/next of kin] to participate in this study in accordance with the national legislation and the institutional requirements.
Author contributions
AA: Conceptualization, Data curation, Formal analysis, Investigation, Visualization, Writing – original draft. DA: Conceptualization, Data curation, Formal analysis, Investigation, Visualization, Writing – original draft. DB: Conceptualization, Funding acquisition, Methodology, Project administration, Supervision, Validation, Writing – review & editing. GS: Conceptualization, Formal analysis, Methodology, Writing – review & editing. GC: Conceptualization, Methodology, Writing – review & editing. MM: Conceptualization, Methodology, Writing – review & editing. AF: Conceptualization, Funding acquisition, Project administration, Supervision, Validation, Writing – review & editing.
Funding
The author(s) declare that financial support was received for the research and/or publication of this article. This research has received funding from the project Vitality-Project Code ECS00000041, CUP I33C22001330007-funded under the National Recovery and Resilience Plan (NRRP), Mission 4 Component 2 Investment 1.5—“Creation and strengthening of innovation ecosystems,” construction of “territorial leaders in R&D,” and Innovation Ecosystems-Project “Innovation, digitalization and sustainability for the diffused economy in Central Italy-VITALITY” Call for tender No. 3277 of 30/12/2021, and Concession Decree No. 0001057.23-06-2022 of Italian Ministry of University funded by the European Union-NextGenerationEU.
Acknowledgments
The authors wish to thank all the vertical farms for providing data and support to the research.
Conflict of interest
The authors declare that the research was conducted in the absence of any commercial or financial relationships that could be construed as a potential conflict of interest.
Generative AI statement
The authors declare that no Gen AI was used in the creation of this manuscript.
Publisher’s note
All claims expressed in this article are solely those of the authors and do not necessarily represent those of their affiliated organizations, or those of the publisher, the editors and the reviewers. Any product that may be evaluated in this article, or claim that may be made by its manufacturer, is not guaranteed or endorsed by the publisher.
References
Amitrano, C., Paglialunga, G., Battistelli, A., De Micco, V., Del Bianco, M., Liuzzi, G., et al. (2023). Defining growth requirements of microgreens in space cultivation via biomass production, morpho-anatomical and nutritional traits analysis. Front. Plant Sci. 14:1190945. doi: 10.3389/fpls.2023.1190945
Ansah, F. A., Amodio, M. L., and Colelli, G. (2018). Quality of fresh-cut products as affected by harvest and postharvest operations. J. Sci. Food Agric. 98, 3614–3626. doi: 10.1002/jsfa.8885
Appicciutoli, D., Amici, A. S., Bentivoglio, D., Chiaraluce, G., Staffolani, G., and Finco, A. (2025). Cultivating the future: a bibliometric review of emerging trends in soilless farming. Horticulturae 11:140. doi: 10.3390/horticulturae11020140
Astapova, M., Saveliev, A., and Markov, Y., (2021). Method for monitoring growth of microgreens in containers using computer vision in infrared and visible ranges. In Agriculture digitalization and organic production: Proceedings of the first international conference, ADOP 2021, St. Petersburg, Russia, June 7–9, 2021 (pp. 383–394). Singapore: Springer nature Singapore.
Avgoustaki, D. D., and Xydis, G. (2020). How energy innovation in indoor vertical farming can improve food security, sustainability, and food safety? Adv Food Secur Sustain 5, 1–51. doi: 10.1016/bs.af2s.2020.08.002
Banerjee, C., and Adenaeuer, L. (2014). Up, up and away! The economics of vertical farming. J Agricult Stud 2, 40–60. doi: 10.5296/jas.v2i1.4526
Barbosa Lages, G., Almeida Gadelha, F. D., Kublik, N., Proctor, A., Reichelm, L., Weissinger, E., et al. (2015). Comparison of land, water, and energy requirements of lettuce grown using hydroponic vs. conventional agricultural methods. Int. J. Environ. Res. Public Health 12, 6879–6891. doi: 10.3390/ijerph120606879
Benke, K., and Tomkins, B. (2017). Future food-production systems: vertical farming and controlled-environment agriculture. Sustainability 13, 13–26. doi: 10.1080/15487733.2017.1394054
Bentivoglio, D., Chiaraluce, G., and Finco, A. (2022). Economic assessment for vegetable waste valorization through the biogas-biomethane chain in Italy with a circular economy approach. Front Sustain Food Syst 6:1035357. doi: 10.3389/fsufs.2022.1035357
Bhargaw, A., and Chauhan, P. (2020). Analysis of soilless farming in urban agriculture. J Pharmacogn Phytochem 9, 239–242.
Bihari, C., Ahamad, S., Kumar, M., Kumar, A., Kamboj, A. D., Singh, S., et al. (2023). Innovative soilless culture techniques for horticultural crops: a comprehensive review. Int J Environ Climate Change 13, 4071–4084. doi: 10.9734/ijecc/2023/v13i103084
Boganini, L., and Casazza, C. (2016). “Soilless urban temporary agriculture as a strategy for brownfield site renewal” in Mediterranean green buildings and renewable energy: Selected papers from the world renewable energy Network’s med green forum Cham: Springer International Publishing. 729–740.
Cai, W., Bu, K., Zha, L., Zhang, J., Lai, D., and Bao, H. (2025). Energy consumption of plant factory with artificial light: challenges and opportunities. Renew. Sust. Energ. Rev. 210:115235. doi: 10.1016/j.rser.2024.115235
Casey, L., Freeman, B., Francis, K., Brychkova, G., McKeown, P., Spillane, C., et al. (2022). Comparative environmental footprints of lettuce supplied by hydroponic controlled-environment agriculture and field-based supply chains. J. Clean. Prod. 369:133214. doi: 10.1016/j.jclepro.2022.133214
D’Imperio, M., Renna, M., Cardinali, A., Buttaro, D., Serio, F., and Santamaria, P. (2016). Calcium biofortification and bioaccessibility in soilless “baby leaf” vegetable production. Food Chem. 213, 149–156. doi: 10.1016/j.foodchem.2016.06.071
Despommier, D. (2013). Farming up the city: the rise of urban vertical farms. Trends Biotechnol. 31, 388–389. doi: 10.1016/j.tibtech.2013.03.008
Ebert, A. W. (2022). Sprouts and microgreens—novel food sources for healthy diets. Plan. Theory 11:571. doi: 10.3390/plants11040571
Ferreira, M. E., Henschel, J. M., Olivoto, T., Batista, D. S., and Zeist, A. R. (2024). Research on microgreens: a bibliometric analysis. Vegetos 37, 1589–1601. doi: 10.1007/s42535-023-00699-x
Finco, A., Bucci, G., Belletti, M., and Bentivoglio, D. (2021). The economic results of investing in precision agriculture in durum wheat production: a case study in Central Italy. Agronomy 11:1520. doi: 10.3390/agronomy11081520
Flick, U. (2015). Introducing research methodology: A beginner's guide to doing a research project. (2nd ed.). London: SAGE Publications.
Food and Agriculture Organization (2022). Urban and Peri-urban agriculture: from production to food systems. Rome: FAO.
Galieni, A., Falcinelli, B., Stagnari, F., Datti, A., and Benincasa, P. (2020). Sprouts and microgreens: trends, opportunities, and horizons for novel research. Agronomy 10:1424. doi: 10.3390/agronomy10091424
Goddek, S., Delaide, B., Mankasingh, U., Ragnarsdottir, K. V., Jijakli, H., and Thorarinsdottir, R. (2015). Challenges of sustainable and commercial aquaponics. Sustain. For. 7, 4199–4224. doi: 10.3390/su7044199
Gunjal, M., Singh, J., Kaur, J., Kaur, S., Nanda, V., Sharma, A., et al. (2024). Microgreens: cultivation practices, bioactive potential, health benefits, and opportunities for its utilization as value-added food. Food Biosci. 62:105133. doi: 10.1016/j.fbio.2024.105133
Hajyzadeh, M., and Egi, Y., (2023). Innovative and groundbreaking technologies for sustainable agriculture. In Sustainable agriculture and food security. Ankara, Turkey: İksad Yayınevi. (pp. 247–266). doi: 10.5281/zenodo.8292204
Ibrahim, L. A., Shaghaleh, H., El-Kassar, G. M., Abu-Hashim, M., Elsadek, E. A., and Alhaj Hamoud, Y. (2023). Aquaponics: a sustainable path to food sovereignty and enhanced water use efficiency. Water 15:4310. doi: 10.3390/w15244310
Ikrang, E. G., Ehiomogue, P. O., and Udoumoh, U. I. (2022). Hydroponics in precision agriculture–a review. Annals Fac Engineer Hunedoara 20, 143–148.
Kallio, H., Pietilä, A. M., Johnson, M., and Kangasniemi, M. (2016). Systematic methodological review: developing a framework for a qualitative semi-structured interview guide. J. Adv. Nurs. 72, 2954–2965. doi: 10.1111/jan.13031
Khatri, L., Kunwar, A., and Bist, D. R. (2024). Hydroponics: advantages and challenges in soilless farming. Big Data Agric 6, 81–88. doi: 10.26480/bda.02.2024.81.88
Kumar, T. V., and Verma, R. (2024). A comprehensive review on soilless cultivation for sustainable agriculture. J Exp Agric International 46, 193–207. doi: 10.9734/jeai/2024/v46i62470
Kumari, R., and Kumar, R. (2019). Aeroponics: a review on modern agriculture technology. Indian Farmer 6, 286–292.
Lakhiar, I. A., Gao, J., Syed, T. N., Chandio, F. A., and Buttar, N. A. (2018). Modern plant cultivation technologies in agriculture under controlled environment: a review on aeroponics. J. Plant Interact. 13, 338–352. doi: 10.1080/17429145.2018.1472308
Lazo, R. P., and Gonzabay, J. Q. (2020). Economic analysis of hydroponic lettuce under floating root system in semi-arid climate. La Granja 31:118. doi: 10.17163/lgr.n31.2020.09
Maestre-Valero, J. F., Martin-Gorriz, B., Soto-García, M., Martinez-Mate, M. A., and Martinez-Alvarez, V. (2018). Producing lettuce in soil-based or in soilless outdoor systems. Which is more economically profitable? Agric. Water Manag. 206, 48–55. doi: 10.1016/j.agwat.2018.04.022
Marr, B. (2012). Key performance indicators (KPI): The 75 measures every manager needs to know. Pearson UK: Harlow, England.
Martinez, J. (2024). Controlled environment agriculture: A systematic review. Birmingham, MI: Food Safety Magazine, BNP Media. 30.
Michalis, E., Giatra, C. E., Skordos, D., and Ragkos, A. (2023). Assessing the different economic feasibility scenarios of a hydroponic tomato greenhouse farm: a case study from Western Greece. Sustain. For. 15:14233. doi: 10.3390/su151914233
Michelon, N., Pistillo, A., Paucek, I., Pennisi, G., Bazzocchi, G., Gianquinto, G., et al., (2019). From microgarden technologies to vertical farms: innovative growing solutions for multifunctional urban agriculture. In: International symposium on botanical gardens and landscapes 1298 (pp. 59–70).
Mishra, S. J., Rout, D., and Sahoo, D. (2024). Analysing the economic viability of hydroponic farming: a comparative cost-benefit analysis. Int J Progress Res Engineer Manage Sci 4, 1806–1811. doi: 10.58257/IJPREMS35128
Orsini, F., Kahane, R., Nono-Womdim, R., and Gianquinto, G. (2013). Urban agriculture in the developing world: a review. Agron. Sustain. Dev. 33, 695–720. doi: 10.1007/s13593-013-0143-z
Paraschivu, M., Cotuna, O., Sărățeanu, V., Durău, C. C., and Păunescu, R. A., (2021). Microgreens-current status, global market trends and forward statements. Scientific Papers. Series “Management, Economic Engineering in Agriculture and Rural Development”, University of Agronomic Sciences and Veterinary Medicine of Bucharest. 21, 633–640.
Peng, H., and Simko, I. (2023). Extending lettuce shelf life through integrated technologies. Curr. Opin. Biotechnol. 81:102951. doi: 10.1016/j.copbio.2023.102951
Puente, L., Char, C., Patel, D., Thilakarathna, M. S., and Roopesh, M. S. (2024). Research trends and development patterns in microgreens publications: a bibliometric study from 2004 to 2023. Sustain. For. 16:6645. doi: 10.3390/su16156645
Putra, P. A., and Yuliando, H. (2015). Soilless culture system to support water use efficiency and product quality: a review. Agric Agricult Sci Proc 3, 283–288. doi: 10.1016/j.aaspro.2015.01.054
Rouphael, Y., Colla, G., and De Pascale, S. (2021). Sprouts, microgreens and edible flowers as novel functional foods. Agronomy 11:2568. doi: 10.3390/agronomy11122568
Salisu, M. A., Oyebamiji, Y. O., Ahmed, O. K., Shamsudin, N. A., Fairuz, Y. S., Yusuff, O., et al. (2024). A systematic review of emerging trends in crop cultivation using soilless techniques for sustainable agriculture and food security in post-pandemic. AIMS Agric Food 9, 666–692. doi: 10.3934/agrfood.2024036
Sharma, A., Hazarika, M., Heisnam, P., Pandey, H., Devadas, V. S., and Wangsu, M. (2024). Controlled environment ecosystem: a plant growth system to combat climate change through soilless culture. Crop Design 3:100044. doi: 10.1016/j.cropd.2023.100044
Singh, A., Singh, J., Kaur, S., Gunjal, M., Kaur, J., Nanda, V., et al. (2024). Emergence of microgreens as a valuable food, current understanding of their market and consumer perception: a review. Food Chem. 23:101527. doi: 10.1016/j.fochx.2024.101527
Souza, V., Gimenes, R. M. T., de Almeida, M. G., Farinha, M. U. S., Bernardo, L. V. M., and Ruviaro, C. F. (2023). Economic feasibility of adopting a hydroponics system on substrate in small rural properties. Clean Techn. Environ. Policy 25, 2761–2775. doi: 10.1007/s10098-023-02529-9
Srivani, P., and Manjula, S. H., (2019) A controlled environment agriculture with hydroponics: variants, parameters, methodologies and challenges for smart farming. In 2019 fifteenth international conference on information processing (ICINPRO) (pp. 1–8). IEEE.
Staffolani, G., Bentivoglio, D., and Finco, A. (2024). Economic assessment of small-Scale Mountain dairy farms by using accounting data: evidence from an Italian case study. AGRIS 16, 109–120. doi: 10.7160/aol.2024.160308
State of Indoor Farming Report (2017) State of indoor farming report 2017. Available online at: https://www.cropscience.bayer.com/sites/cropscience/files/inline-files/stateofindoorfarming-report-2017.pdf(Accessed July 21, 2021).
Swain, A., Chatterjee, S., and Vishwanath, M. (2021). Hydroponics in vegetable crops: a review. Pharma Innov J 10, 629–634. doi: 10.1007/978-1-0716-3993-1_2
Vgreens, (2025). Vertical Farming in Europe: How to Balance Sustainability and Economic Viability. Available online at: https://v-greens.com/news/vertical-farming-in-europe-how-to-balance-sustainability-and-economic-viability/#:~:text=According%20to%20estimates%20by%20Market,approximately%20245.11%20billion%20by%202029 (Accessed March 24, 2025)
Wojdyło, A., Nowicka, P., Tkacz, K., and Turkiewicz, I. P. (2020). Sprouts vs. microgreens as novel functional foods: variation of nutritional and phytochemical profiles and their in vitro bioactive properties. Molecules 25:4648. doi: 10.3390/molecules25204648
Yanes-Molina, A. P., Jaime-Meuly, R., Andrade-Bustamante, G., Lucero-Flores, T. I., and Martínez-Ruíz, F. E. (2019). Microgreens-an alternative of horticultural production and market. Expert journal of marketing 7, 120–136.
Yin, R. K., (2018). Case study research and applications. Design and methods. (6th ed.). Thousand Oaks, CA: SAGE Publications.
Keywords: soilless agriculture, hydroponic, sustainability, economic profitability, microgreens, cost analysis, Italy
Citation: Amici AS, Appicciutoli D, Bentivoglio D, Staffolani G, Chiaraluce G, Mogetta M and Finco A (2025) From seed to profit: a comparative economic study of two Italian vertical farms. Front. Sustain. Food Syst. 9:1584778. doi: 10.3389/fsufs.2025.1584778
Edited by:
Michael Martin, Swedish Environmental Research Institute (IVL), SwedenReviewed by:
Laura Cammarisano, University of California, Davis, United StatesMaria J. Bustamante, Royal Institute of Technology, Sweden
Copyright © 2025 Amici, Appicciutoli, Bentivoglio, Staffolani, Chiaraluce, Mogetta and Finco. This is an open-access article distributed under the terms of the Creative Commons Attribution License (CC BY). The use, distribution or reproduction in other forums is permitted, provided the original author(s) and the copyright owner(s) are credited and that the original publication in this journal is cited, in accordance with accepted academic practice. No use, distribution or reproduction is permitted which does not comply with these terms.
*Correspondence: Deborah Bentivoglio, ZC5iZW50aXZvZ2xpb0BzdGFmZi51bml2cG0uaXQ=