- 1Department of Plant Sciences, University of the Free State, Bloemfontein, South Africa
- 2Agricultural Research Council, Vegetable, Industrial and Medicinal Plants, Pretoria, South Africa
Grain yield in South Africa cowpea remains low compared to other cowpea producing countries, due largely to a lack of breeding efforts. The objectives of this study were to cross diverse parental genotypes and to measure yield and yield components in the parents and F1 hybrid progeny. Ten cowpea parental genotypes selected for diverse characteristics were assessed for genetic diversity using 20 simple sequence repeat (SSR) markers. Parents were crossed in a half diallel mating design which produced 45 F1 hybrids, and parents and the hybrids were evaluated for grain yield and yield components in four environments (two locations in two seasons). The genetic diversity analysis identified five highly informative markers (SSR6265, SSR6217, SSR6451, SSR6277, and SSR6436, PIC ≥ 0.5) which indicated their usefulness in determining the genetic structure of cowpea. High broad-sense heritability (>0.80) was observed for all traits except for pod width, indicating that a good response to selection can be expected. Although cowpea is a self-pollinating crop, high levels of heterosis were evident for yield and yield components in this study. Six hybrids (IT96D-602 × Glenda, IT96D-602 × Kisumi-mix, IT96D-602 × 98K-5301, ITOOK-1060 × TVU13953, TVU13953 × Glenda, and IT96D-602 × TVU13953) performed significantly better than their parental genotypes for grain yield, showing heterosis and potential for hybrid breeding. Parental genotypes TVU13953 and IT96D-602 were high yielding and could serve as a foundation for subsequent breeding efforts.
1 Introduction
Cowpea (Vigna unguiculata L. Walp) is an important grain legume that is widely grown in many parts of the world, particularly in sub-Sahara Africa (SSA), for its edible seeds and forage. Cowpea is highly nutritious and its production and consumption can contribute to healthier diets in populations with high levels of malnutrition (Gonçalves et al., 2016; Mbuma et al., 2021). The crop can tolerate drought, due to its deep rooting, early flowering, and early maturity (Gonçalves et al., 2016). Cowpea also can fix atmospheric nitrogen, thus contributing to increased soil fertility (Kyei-Boahen et al., 2017).
The global cowpea production amounted to 8.99 million ton in 2021, with Nigeria being the top producer (3.63 million ton) and Africa contributing 97% of production (FAOSTAT, 2021). Globally, the yield for 2021 was 6,026 kg/ha, but South Africa produced only 435 kg/ha contributing only 4,689.16 ton to the total global production (FAOSTAT, 2021). Cowpea production in Africa is hindered by biotic and abiotic stresses (Yirzagla et al., 2021; Abdou, 2022), and in addition to these constraints, the low grain yield in South Africa could be attributed to lack of improved cultivars and low soil fertility (Omomowo and Babalola, 2021) as well as a lack of good agronomic management practices.
The small-scale producers in the country grow cowpea landraces and other imported accessions. These accessions are poor yielding, unstable and susceptible to major pests and diseases. South Africa imported cowpea germplasm from the International Institute for Tropical Agriculture (IITA), Nigeria, which includes wild species, accessions, mutants, and cultivars developed through hybrid breeding. This imported cowpea germplasm is used mainly for pre-breeding purposes to evaluate adaptation and their performance in South African agro-ecological conditions (Mbuma et al., 2021; Gerrano et al., 2015, 2019; Mbuma et al., 2022; Gumede et al., 2022a).
Studies conducted in South Africa (Mbuma et al., 2021; Gerrano et al., 2019; Gumede et al., 2022b; Asiwe, 2009) reported significant variation among available cowpea genotypes for yield and yield components. Another study to assess the genetic diversity and population structure of 90 cowpea accessions in South Africa, using single nucleotide polymorphism (SNP) markers (Gumede et al., 2022a), concluded that the accessions evaluated showed genetic variation and a significant potential to contribute to breeding for new cultivars. Thus, it would be beneficial to create genetic variation through hybridization, in efforts to eventually improve the crop for grain yield, yield components and morphology (Kumari and Chauhan, 2018; Owusu et al., 2018, 2020). The observed yield is well below the yield of 1,500 to 2,500 kg/ha obtained in other countries, largely due to the lack of improved cultivars (Omomowo and Babalola, 2021; Gumede et al., 2022a). Hybridisation should be employed as intervention strategy to improve cowpea productivity for desired yield components and morphology through selection (Kumari and Chauhan, 2018; Owusu et al., 2018, 2020). There have been no cowpea breeding activities in South Africa before, due mainly to the fact that the crop was never considered important. A single academic study that produced dual-purpose second-generation (F2) cowpeas is the only evidence of cowpea breeding in South Africa thus far (Moalafi et al., 2010). This underlines the need for a breeding program for South Africa. As cowpea is a self-pollinating crop this can be done through hybridization and pedigree selection, or if heterosis is present, hybrid breeding could be an option.
The objectives this study were to cross selected diverse cowpea parental genotypes in a half-diallel design to determine the expression and heritability of grain yield and yield components in the parents and the hybrids in two locations and two seasons.
2 Materials and methods
2.1 Planting materials
Ten cowpea parental genotypes were obtained from the Agricultural Research Council Vegetable, Industrial, Medicinal Plants (ARC-VIMP) pre-breeding programme. They were selected based on high grain yield, drought tolerance/susceptibility, and high levels of Fe, Zn, and protein content (Table 1).
2.2 DNA isolation protocol
The ten parental genotypes (Table 1) were sown (two seeds per parent) in pots filled with sterile soil in the glasshouse at the University of the Free State, Bloemfontein, South Africa in June 2020. Two-week old leaves were sampled on ice and freeze-dried. DNA was isolated using a CTAB (hexadecyltrimethylammonium bromide) DNA isolation method (Saghai-Maroof et al., 1984). Samples were diluted with 1 × TE buffer (10 mM Tris-HCl, pH of 8.0; 1 mM EDTA) to a working concentration of 20 ng/μL for subsequent experiments.
2.3 SSR analysis
Twenty SSR primers (Table 2) that were previously identified (Danso et al., 2018) were used for genetic diversity assessment of the parental genotypes following the protocol described in the same paper. Each PCR amplification reaction contained 40 ng DNA, 2 mM MgCl2, 1 × KAPATaq ReadyMix DNA polymerase, 50 ng each of the forward and reverse primer (Integrated DNA technologies) and 0.10 mg/ml Bovine serum albumin (BSA) in a total reaction volume of 10 μl.
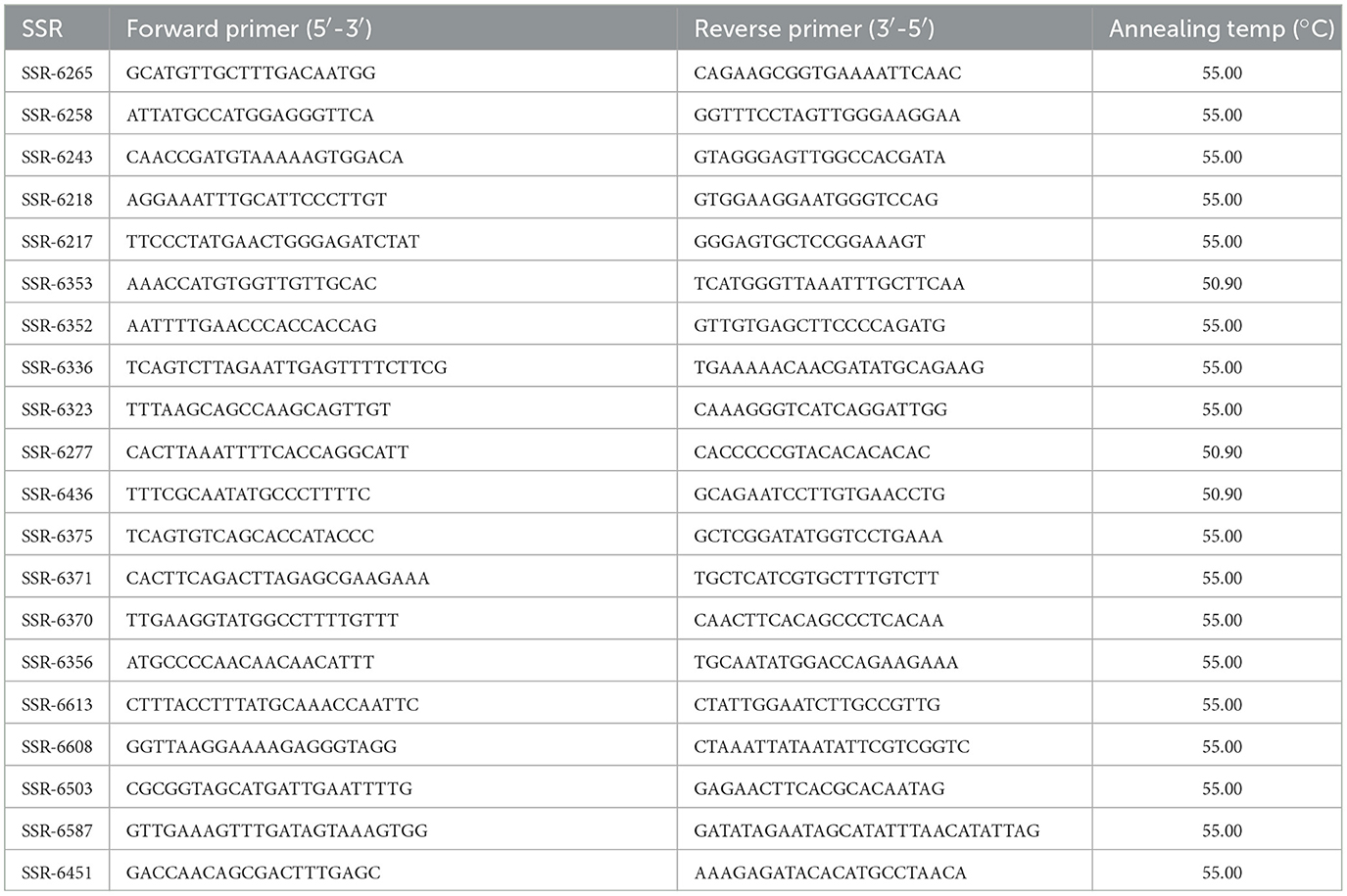
Table 2. List of molecular markers used to assess the genetic diversity of the cowpea parental genotypes.
The optimized cycling conditions for the primers were 1 cycle at 94°C for 2 min followed by 35 cycles of denaturation for 30 seconds at 94°C. The annealing process followed for 30 seconds at 55.00 or 50.90°C (depending on the primer), then initial extension for 1 min at 72°C followed by a final extension for 10 min at 72°C.
2.4 Diallel mating
Ten genetically different cowpea lines were used as parents for hybridization. The parents were selected based on yield, drought tolerance and specific nutritional attributes. Three seeds of the parental genotypes were planted per pot (filled with sterile soil) in three consecutive weeks to synchronize flowering. The half-diallel crosses were conducted in the greenhouse under controlled conditions which prevented insect-mediated cross pollination. A distance of 50 cm was maintained between plants. Just before flowering, the flower buds of the female parents were emasculated and covered with paper bags. Cross-pollination was done manually, where pollen from the male was transferred to the stigma of the female parent. The pollination was carried out between 8h00 and 10h00 am, when the flowers were most receptive. After pollination, the flowers were re-bagged to exclude foreign pollen. The pods that developed from the pollinated flowers indicated that the cross-pollination was successful. The seeds from the 45 successful F1 hybrids were collected and bulked for field evaluation.
2.5 Experimental locations
Field experiments were planted at the ARC research farms, at Loskop and Brits in 2021 and 2022 during the summer cropping season (Table 3). These locations represent current cowpea production areas in South Africa, which will also be targeted in future cowpea breeding efforts. Due to low amounts of seed generated from crosses, there was only enough seed for two environments and two seasons. Loskop is situated in the Limpopo Province and is characterized by a sandy loam soil and a pH value that ranged from 4.00 to 8.50. Brits is located in the Northwest Province and is characterized by clay loam soil with a pH ranging from 5.90 to 8.08.
2.6 Experimental design, trial establishment and management
The trial layout was a randomized complete block design with three replications, planted using one seed per hole of a 3 m plot spaced at 1 m between rows and 0.50 m between plants. Manual weeding and other normal cultural practices were used when needed to ensure optimal growing conditions. Supplementary irrigation was applied using sprinklers for 2 h to give an amount of 12.50 mm when needed to prevent drought stress.
2.7 Data collection
Quantitative traits were recorded according to published cowpea descriptors (Alves and Bettencourt, 2007). The data was collected on five randomly selected plants in each replication. The traits recorded were plant height (PH, cm), number of branches (NB), leaf length (LL, cm), leaf width (LW, cm), days to 50% flowering (D50F), number of pods per plant (NPP), hundred seed weight (HSWt, g), and grain yield (GY, t/ha). Pod length (PL, mm), pod width (PW, mm), and number of seeds per pod (NSPP) were measured from 10 randomly selected pods. Grain yield (GY) was converted to t/ha using the Equation 1.
Where mc was the moisture content of grain at harvest, 14% was the standard constant for legume moisture content, and 10,000 is the conversion factor used for hectare (Nkhoma, 2020).
2.8 Data analysis
2.8.1 Genetic diversity analysis
The alleles were scored as present (1) or absent (0) based on the size of the amplified product. Scored data were used to construct a binary data matrix for statistical analysis. Total allele number (Na) and average number of alleles at each locus were calculated manually. Allelic polymorphic information content (PIC) was calculated from the binary data of the 20 SSR markers using iMEC: online marker efficiency calculator developed by Amiryousefi et al. (2018). PIC evaluates polymorphism of a marker by characterizing the efficiency of each primer for detecting polymorphic loci (Shete et al., 2000). A PIC of >0.50 indicates high diversity, a PIC < 0.25 low diversity and a PIC between 0.25 and 0.50 intermediate diversity (Botstein et al., 1980). Cluster analysis based on the unweighted neighbor-joining (NJ) with 30 000 bootstrap repetitions was conducted with DARwin 6.0.19 (Perrier and Jacquemoud-Collet, 2006) to construct a rooted dendrogram. The dendrogram illustrates the relationship of the cowpea parental genotypes based on SSR allele variation. Genetic similarities between the parents were compared by using the Jaccard similarity coefficient (Jaccard, 1908).
2.8.2 Analysis of variance
Analysis of variance (ANOVA) was done on data of all yield components using Agrobase generation II SQL-version 38 (Agronomix Statistical Software Inc., 2019) statistical software. The least significant difference (LSD) at P ≤ 0.001 and P ≤ 0.05 was used for means separation. The following statistical linear mixed model was used for genotype analysis in multi-environments (Equation 2).
Where Yijk = Yield components in the kth replication of the ith genotype recorded at the lth location, Ll = Random effect of the lth location, Gi = Random effect of the ith genotype, Rk(Ei) = Effect of kth replication in the lth location, εljk = Residual error or random experimental error, μ = Grand mean. The combined ANOVA using the statistical linear mixed model (Equation 3).
Where, Yijkl = observation for ith genotype, in jth season, in kth replication nested within lth location, μ = overall mean, Gi = the random effect of the ith genotype, Ll = the random effect of the lth location, R(L)k(l) = the random effect of the kth replication nested within the lth location t and was the error term for the environment effect. Where GLil = random interaction effect between the ith genotype and the lth location, GR(L)ik(l) = the random interaction effect between the ith genotype and the kth replication nested in the lth location and was the error term for the genotype and genotype by environment interaction effect. Where Sj = random effect of the jth season, SLjl = the random interaction effect between the jth season and the lth location. Where SR(L)jk(l) = random interaction effect between the jth season and the kth replication nested within the lth location and was the error term for the season and location by season interaction effect. Where GSij = random interaction effect between the ith genotype and the jth season, GLSijl = random interaction effect between the ith genotype, lth location and jth season, and εijkl = residual error.
Heritability (broad from the ANOVAs for two locations and two seasons for parents and progeny combined rather than from the diallel analysis.
Genotypic () and phenotypic variance () were calculated using equations:
Genotypic variance across environments was determined by equation.
Genotypic variance for combined analysis was determined by equation.
The phenotypic variance for individual environments was determined by equation.
The phenotypic variance across environments was determined by equation.
The phenotypic variance for combined environments was determined by equation.
Where σ2g = genotypic variance, σ2p = phenotypic variance, σ2ge = genotype by environment variance, σ2gs = genotype by season variance, σ2gsl = genotype by location by season variance, environmental error (σ2ε) = error mean squares, MSg = mean squares for genotype, MSgsl = mean squares for genotype by season by location interaction, MSgl = mean squares for genotype by lth location, MSgs = mean squares for genotype by season, MSε = mean squares for error, r = number of replication, l = number of lth locations and s = number of seasons.
Broad-sense heritability (H2) was determined for each trait using equation
Where σ2g = Genotypic variance, σ2p = Phenotypic variance.
3 Results
3.1 SSR marker analysis
Three of the markers, SSR6336, SSR6370, and SSR6603, were excluded because they were monomorphic for all alleles produced at those loci. In total 56 alleles were detected in the 10 parents. The number of alleles per locus ranged from one to six, with an average of 2.80. The loci SSR6451 and SSR6436 had the most alleles (six each), while three markers, SSR6336, SSR6370, and SSR6603, had only one allele each (Table 4). The PIC ranged from 0.16 to 0.79 with an average value of 0.43. Out of 17 polymorphic markers, five (29.40%) markers were highly informative (PIC ≥ 0.50), ten markers (58.80%) were intermediately informative (PIC = 0.25–0.50), and two markers (11.80%) had the lowest informativeness (PIC ≤ 0.25). The parental genotypes had a mean gene diversity of 0.49, with individual markers ranging from 0.18 (SSR6323 and SSR6356) to 0.81 (SSR6451) (Table 4).
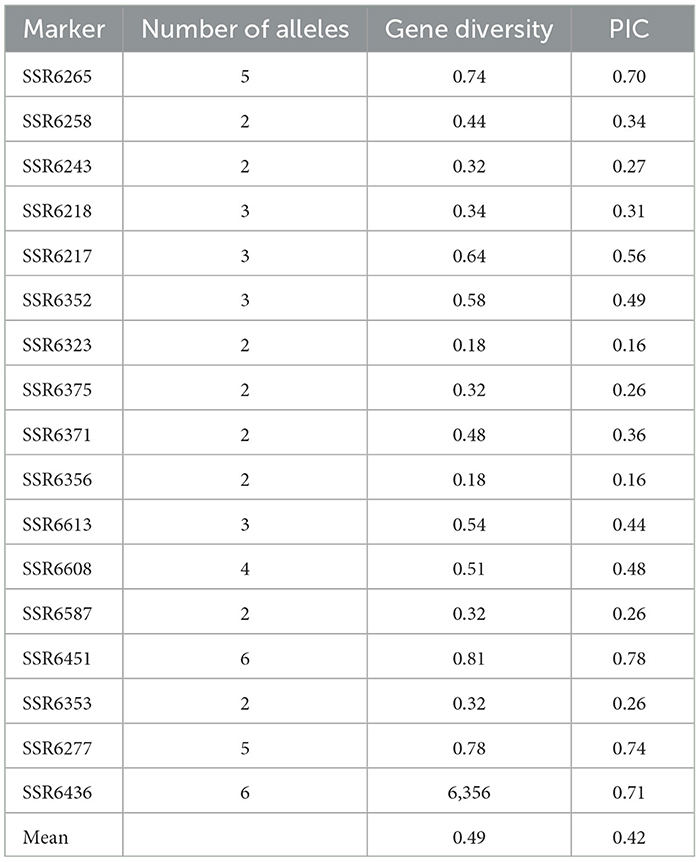
Table 4. SSR marker analysis showing the number of alleles detected, gene diversity and the polymorphic information content (PIC) values.
3.2 Genetic relationships amongst cowpea parental genotypes
The ten cowpea parental genotypes were divided into three main clusters (Figure 1). Cluster I had two genotypes (TVU-14196 and IT96D-602) from Nigeria, and they showed 50% genetic similarity. Cluster II contained three genotypes (IT845-2246, TVU13953, and Kisumi-mix), each with a different shade of brown seed color. Cluster III included five genotypes (Glenda, ITOOK-1060, 98K-5301, TVU7778, and IT93K-129-4). Two of the genotypes within the cluster were cream-white and one was tan. The other two genotypes were speckled, possibly indicating a cross between the cream white and tan genotypes. Three genotypes were from Nigeria, and two from South Africa. Table 5 shows the genetic distances between parental genotypes, ranging from 0.29 (for ITOOK-1060 and TVU7778) to 0.61 (for TVU13953 and Glenda, TVU-14196 and Glenda).
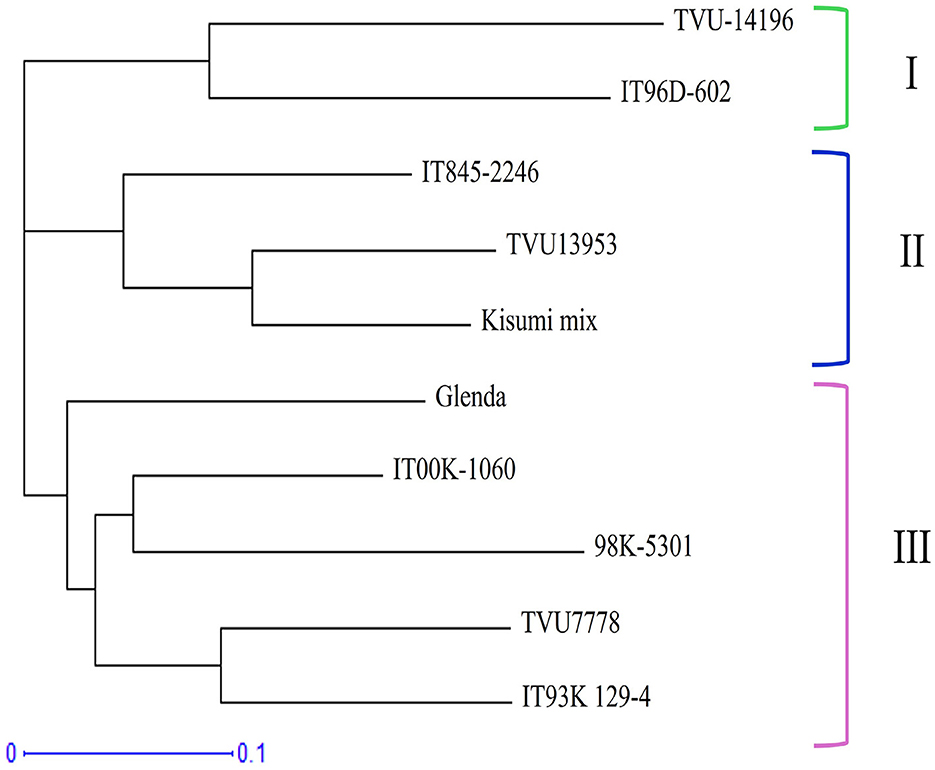
Figure 1. Dendrogram constructed based on genetic distance from simple sequence repeat molecular markers of the 10 selected cowpea parental genotypes.
3.3 Analysis of variance for grain yield and yield components
Significant genotype effects (P ≤ 0.05) were evident for all the traits measured at all locations (Table 6). The effect of environment (Brits and Loskop in 2021 and 2022) was significant (P ≤ 0.05) for GY, NPP, NSPP, HSWt, and PH. For Brits and Loskop in 2021 and 2022, the location effect was highly significant (P ≤ 0.001) for GY, NSPP, HSWt, and PH. Genotype by location interaction effect was significant (P ≤ 0.05) for all traits, except for season 2021 (HSWt, LL, and LW) and season 2022 (NPP, PW, LL, LW, and NB).
From combined ANOVA, the genotype effect was significant (P ≤ 0.05) for all traits (Table 7). The location effect was significant (P ≤ 0.05) for GY, NPP, NSPP, and HSWt. Season effect was significant (P ≤ 0.05) for NPP, PW, PH, and D50F. Genotype by location interaction effect was significant (P ≤ 0.05) for all the traits measured, except PL, LL, and LW. Genotype by season and genotype by location by season interaction effects were significant (P ≤ 0.05) for GY, NPP, NB, PW, PH, and D50F. Season by location interaction effect was significant (P ≤ 0.05) for GY, NPP, and PW.
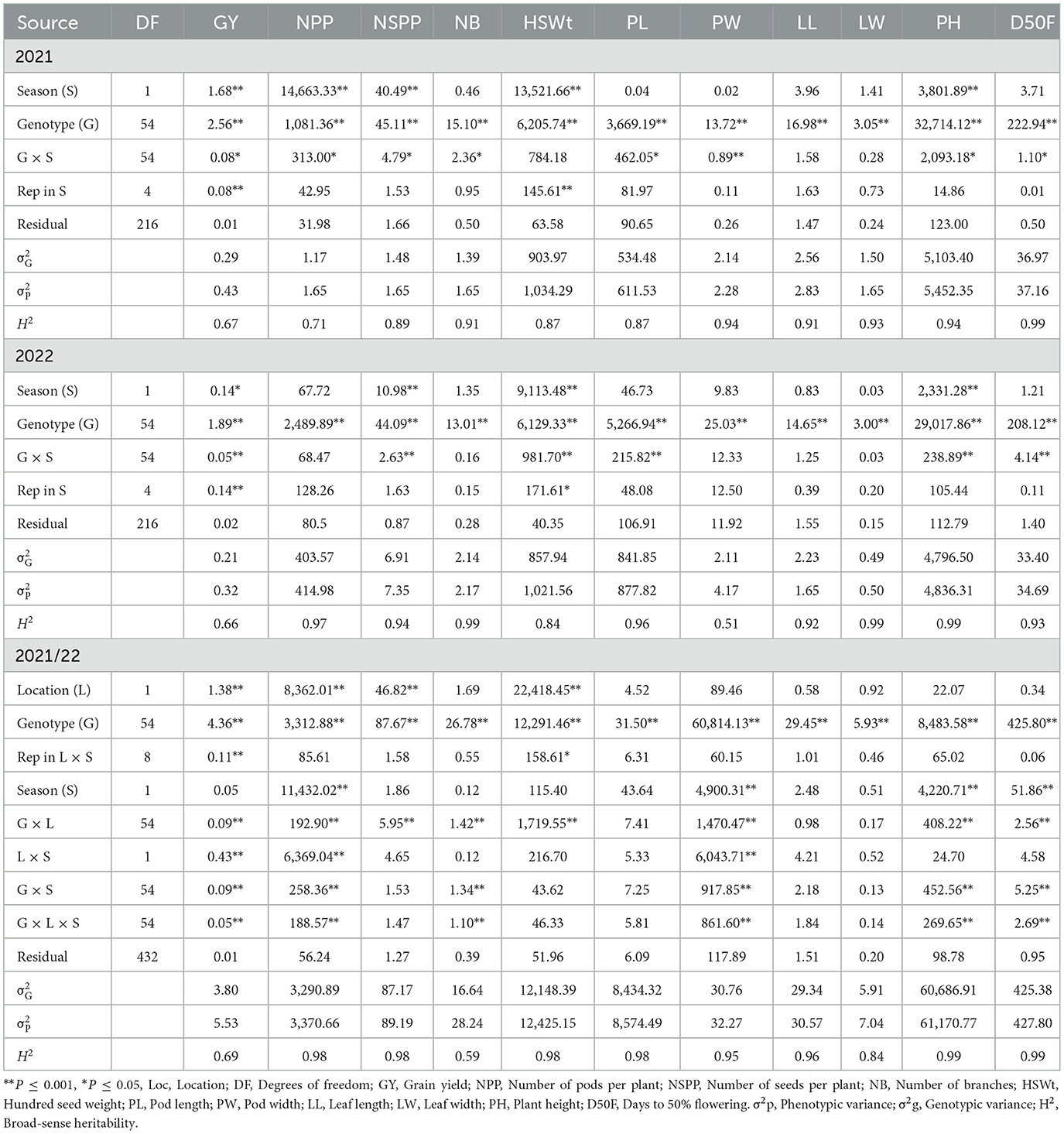
Table 7. Mean squares for grain yield and yield components, evaluated in the 2021 and 2022 seasons, and combined for all trials.
The broad sense heritability (H2) ranged from 0.61 (GY) to 0.98 (PH) at Brits 2021 (Table 6) whereas it ranged between 0.07 (PL) to 0.97 (HSWt and PH) at Brits 2022. The H2 ranged from 0.43 (GY) to 0.98 (PH) at Loskop 2021, whereas it ranged from 0.57 (GY) to 0.98 (PH) at Loskop 2022. The H2 ranged from 0.61 (NPP) to 0.99 (D50F) in season 2021 (Table 7), whereas it ranged from 0.53 (NSPP) to 0.98 (LL, NB, and PH) in season 2022. The H2 ranged from 0.59 (NB) to 0.99 (PH and D50F), for combined analysis (Table 7).
3.4 Mean performance of parental genotypes and their F1 hybrids from combined analysis
Mean performance for GY from combined ANOVA ranged from 0.27 to 2.95 t/ha, with an overall mean of 1.34 t/ha (Table 8). The six hybrids that were superior for GY (2.70 to 2.95 t/ha) had either Glenda, TVU13953 or IT96D-602 as a common parent. The three hybrids which were inferior for GY (0.27 to 0.55 t/ha) had TVU7778 as a common parent. The yield of the hybrids was significantly higher than for the parents (Table 8).
The mean value of NPP ranged from 19.08 to 87.08, with an overall mean of 44.57 (Table 8). The seven hybrids with the highest NPP (70.00 to 91.00) had either TVU13953, Glenda or IT96D-602 as a common parent. The hybrids had far more pods per plant than the parents. The mean value of NSPP ranged from 9.11 to 21.19, with an overall mean of 14.20 (Table 8). The 10 hybrids with the highest NSPP (17.00 to 21.00) had either TVU13953 or IT96D-602 as a common parent. The hybrids had significantly higher NSPP values than the parents.
The mean of NB for combined analysis ranged from 4.37 to 10.33, with an overall mean of 6.54 (Table 8). The three hybrids with the highest NB (9.00 to 10.50) had Glenda as a common parent. Mean performance for HSWt ranged from 11.22 to 38.87 g, and an overall mean of 26.96 g (Table 8). The eight hybrids with the highest HSWt (35.29 to 39.00 g) had either IT96D-602, TVU13953, Glenda or ITOOK-1060 as a common parent. The mean value of PL from combined analysis ranged from 110.01 to 214.03 mm (Table 8), with an overall mean of 167.99 mm. The eight hybrids with the highest PL (207.00 to 230.00 mm) had either TVU13953 or IT96D-602 as a common parent. The pod lengths of the hybrids were much higher than that of the parents.
The mean value of PW from combined analysis ranged from 5.83 to 12.27 mm, with an overall mean of 8.64 mm (Table 8). The 14 hybrids that had the highest PW (10.00 to 12.27 mm) had either Glenda, ITOOK-1060 or Kisumi-mix as a common parent. The mean value of LL from combined analysis ranged from 6.27 to 12.14 cm, with an overall mean of 9.08 cm (Table 8). The 10 hybrids with the highest LL (10.00 to 12.65 cm) had either TVU7778, IT93K-129-4 or Glenda as a common parent. LL was 21% higher in the hybrids than the parents.
The mean of LW ranged from 1.71 to 4.94 cm, with an overall mean of 3.18 cm (Table 8). The five hybrids with the highest LW (4.00 to 4.94 cm) had Glenda as a common parent. The mean of PH from combined analysis ranged from 37.65 to 215.39 cm, with an overall mean of 108.62 cm (Table 8). The 10 hybrids with the highest PH (130.00 to 216.00 cm) had either IT96D-602, TVU13953, IT93K-129-4, and 98K-5301 as a common parent. The hybrids grew significantly taller than the parents (44%). The mean of D50F from combined analysis ranged from 34.75 to 54.50 days, and an overall mean of 44.20 days (Table 8). The seven hybrids with the lowest D50F (34.00 to 36.50 days) had either IT93K-129-4, IT845-2246, or TVU13953 as a common parent.
4 Discussion
4.1 Genetic diversity analysis of ten selected cowpea parental genotypes
Genetic diversity in a population is essential for selecting diverse genotypes and broadening a population's genetic basis. When analyzing the genetic diversity, the PIC value represents the relative informativeness of each marker and in the current study, 29.4% of the markers (SSR6265, SSR6217, SSR6451, SSR6277, and SSR6436) were highly informative (PIC ≥ 0.50), indicating that they could be useful genetic tools for determining the genetic structure of cowpea. Other studies also reported the informativeness of SSR markers in discrimination of cowpea genotypes for analysis of genetic diversity (Ali et al., 2015; Dagnon et al., 2022; Sarr et al., 2021). The study observed one to six alleles per locus which corroborated diversity studies conducted in Nigeria and in Ghana (Adetiloye et al., 2013; Diouf and Hilu, 2005). The number of markers and parental genotypes used in the current study could have resulted in fewer alleles observed.
The expected heterogeneity (0.10 to 0.81, with an average of 0.49) observed, indicated a wide genetic diversity between the cowpea parents. The average heterogeneity observed in the current study corroborated a study on Sudanese cowpea germplasm which reported heterogeneity average of 0.49 (Ali et al., 2015). The findings are in contrast with a lower heterogeneity average (0.23) and a higher heterogeneity average (0.54) previously reported on cowpea (Dagnon et al., 2022; Seo et al., 2020).
In general, the genetic distances between genotypes in each cluster showed close relationships or similarities. It would be preferable to avoid crossing individual genotypes within a cluster. However, cluster I and III had genotypes with the least similarity, therefore crosses between these genotypes should be considered. The genotypes with the highest genetic diversity, particularly among the three different clusters, may serve as sources of novel alleles for cowpea breeding.
4.2 Analysis of variance and variance components for grain yield and yield components
Differences in genetic makeup of parental genotypes is necessary to generate variability in hybrids (Seo et al., 2020). The genetic diversity between the cowpea parental genotypes confirmed the presence of genetic variation, which, when combined through hybridization, provides an opportunity for selection. These findings agree with that of a previous study (Mbuma et al., 2021), which evaluated the Southern African cowpea germplasm collection for grain yield and yield components and reported highly significant genotype effects for seed yield, number of pods, and number of seeds per plant. The results are also in line with findings reported previously (Stoilova and Pereira, 2013), when genetic diversity of 48 cowpea accessions consisting of 18 landraces from Bulgaria and Portugal and 30 advanced breeding genotypes of different origins were evaluated, showing significant differences in number of pods and seeds per plant. Another study (Mofokeng et al., 2020), conducted on 100 cowpea genotypes, also reported significant differences among genotypes for grain yield, number of branches per plant, number of pods per plant, pod weight per plant and 100 seed weight. Thus, the presence of variation within a population allows selection efficiency for crop improvement.
When parents and the hybrid progeny were evaluated at two locations in two seasons, significant genotype by location interaction was observed for all the yield components, except for leaf length and leaf width, indicating that the parents and hybrids did not rank consistently for these traits at the different locations. The significant genotype by season and genotype by season by location interaction for grain yield, number of pods per plant, pod width, number of branches, plant height and days to 50% flowering, indicated changes in genotype ranking across seasons and locations.
Previous studies also reported significant genotype by location interaction for grain yield and yield components (Mbuma et al., 2021; Gerrano et al., 2020; Ishiyaku et al., 2017; Odeseye et al., 2018). The location affects the genotype performance, thus reducing the relationship between the genotype and the corresponding phenotype. The significant interaction between genotype and the location indicates that the selection for superior yielding ability in one location might not lead to good yielding ability in another due to instability of the genotype. Thus, breeding for stable yielding genotypes for the targeted production areas is important. Evaluation and selection for yield stability should be a part of future breeding efforts. Phenotypic variances were consistently only slightly higher than the genotypic variances for all the traits measured, showing that the phenotype was largely representative of the genotype. This indicates that traits were largely determined genetically. High genetic variance is necessary to obtain a good response to selection, as this indicates a limited effect of location on the desired traits.
4.3 Broad-sense heritability estimates for grain yield and yield components
The broad-sense heritability was higher than 0.80 for all traits measured, except for pod width, across seasons and locations. These findings indicated a considerable proportion of genetic variance to the total variance in the population and could result in good response to selection during breeding. The results corroborated a previous study (Mofokeng et al., 2020), on 100 cowpea genotypes where high heritability (0.97) was reported for 100 seed weight. A study of grain yield and yield components in cowpea also gave high estimates of broad-sense heritability (0.91) for grain size (Aliyu and Makinde, 2016). Another study (Ajayi et al., 2014) also reported high broad-sense heritability (≤ 0.99) for seed length and seed weight. In contrast, other studies reported < 0.50 of H2 for seed yield and related traits (Mbuma et al., 2021; Aliyu and Makinde, 2016). The high broad-sense heritability observed in this study suggests the potential for a good response to selection.
4.4 Performance of parental genotypes and their F1 hybrids
A number of hybrids were high yielding (above average, ≥2.50 t/ha), which indicated that they can already be considered for production at the test locations. The results are in agreement with findings of other authors (Iseki et al., 2021; Owusu et al., 2021) that reported grain yield performance of more than 2 t/ha for parental genotypes and hybrids across environments. It is recommended that the best genotypes be further evaluated at more locations and seasons to confirm their yield potential and stability.
Several hybrids yielded significantly higher than their parents, suggesting the presence of heterosis. The hybrids that had higher than average grain yield had either parents TVU13953, IT96D-602, or Glenda in common, suggesting that these parental genotypes had contributed significantly to grain yield increases observed in the hybrids. The finding suggests the need for combining ability studies to determine the nature of gene action involved in expression of grain yield and yield components. The parental genotypes TVU13953 and IT96D-602 had above average mean performance for grain yield. However, the parental line Glenda had lower grain yield than the average of the population studied (Gerrano et al., 2019).
The high yield of specific hybrids (IT96D-602 × Glenda, IT96D-602 × Kisumi-mix, IT96D-602 × 98K-5301, ITOOK-1060 × TVU13953, TVU13953 × Glenda, and IT96D-602 × TVU13953) suggests the presence of heterosis. This was confirmed by the 25% higher yield in the hybrids than the parents. The parental genotypes require further evaluation for general and specific combining ability, for better use in strategic breeding for grain yield enhancement. Superior hybrids over parental genotypes were also reported in other studies (Kumari and Chauhan, 2018; Owusu et al., 2018, 2020). This emphasizes the potential of hybrid cowpea breeding in South Africa.
The parental genotypes generally had below average (≤ 1.34 t/ha) mean grain yield compared to hybrids, with only two parental genotypes (TVU13953 and IT96D-602) having above average (≥1.34 t/ha) grain yield. TVU13953 and IT96D-602 were also superior for 100 seed weight, number of pods per plant and height compared to the other parental genotypes, and their superiority was evident in their progeny that had the highest mean for the traits. Previous studies reported a positive correlation of grain yield with number of seeds per pod and plant height (Mbuma et al., 2021; Walle et al., 2018). Parental line TVU7778 generally performed poorly and produced inferior hybrids for most of the important traits, which could be because of poor breeding value.
Two hybrids (ITOOK-1060 × TVU13953 and IT96D-602 × TVU13953) had above average values for grain yield, number of pods per plant, number of seeds per pod, plant height, pod length, and pod width. The improvement of cowpea for grain yield can be done through indirect selection of yield components, as grain yield is a polygenic trait (Mofokeng et al., 2020; Aliyu and Makinde, 2016). Previous studies reported cowpea genotypes with above average plant height (Owusu et al., 2021) and pod length (Gerrano et al., 2013). Studies on other legumes reported strong correlation between grain yield and number of branches per stem, number of leaves and plant height (Jayasingha and Fernando, 2020; Upadhyaya et al., 2012; Gerrano et al., 2013; Siwale et al., 2022). In the current study the hybrids had significantly more pods per plant (28%), longer pods (21%) and wider pods (17%), and wider leaves (21%), and significantly taller plants (44%) than the parents. Therefore, although cowpea is a self-pollinating crop, there clearly is heterosis which can be exploited through hybrid breeding.
Earliness in flowering is also important, particularly in the ever-changing climatic conditions, hence resource poor farmers will benefit from early flowering to mitigate the short rainy seasons. In the current study four hybrids (IT96D-602 × TVU13953, IT96D-602 × 98K-5301, TVU-14196 × IT845-2246, and TVU13953 × Glenda) were early flowering (34 to 35 days). However, early flowering does not translate to above average grain yield for the current study, as the genotypes that flowered early had both average and above average grain yield. The current study corroborated findings of the negative correlation of days to 50% flowering with seed yield (Shanko et al., 2014; Thorat and Gadewar, 2013). The advantages of early flowering should therefore be weighed against possible reduced yield compared to later flowering genotypes.
5 Conclusions
This study showed significant genetic variation amongst the F1 hybrids and their parental genotypes which can be explored for improvement of grain yield and yield components in cowpea. Two cowpea parental genotypes (TVU13953 and IT96D-602) displayed superior performance compared to other parental genotypes for grain yield, thus could be selected for future breeding activities, if they also have good breeding values. Although cowpea is a self-pollinating crop, there was significant heterosis for yield and yield components, which can be exploited in hybrid breeding. Six hybrids (IT96D-602 × Glenda, IT96D-602 × Kisumi-mix, IT96D-602 × 98K-5301, ITOOK-1060 × TVU13953, TVU13953 × Glenda, and IT96D-602 × TVU13953) with above-average grain yield should be evaluated for potential hybrid production.
Data availability statement
Publicly available datasets were analyzed in this study. Data can be accessed at: https://doi.org/10.38140/ufs.28929431.
Author contributions
MM: Data curation, Formal analysis, Writing – review & editing, Investigation, Validation, Writing – original draft. ML: Conceptualization, Data curation, Formal analysis, Funding acquisition, Methodology, Supervision, Writing – review & editing, Project administration, Resources. AG: Project administration, Resources, Supervision, Writing – review & editing. AM-O: Resources, Supervision, Writing – review & editing. NM: Conceptualization, Data curation, Formal analysis, Investigation, Project administration, Resources, Supervision, Writing – review & editing.
Funding
The author(s) declare that financial support was received for the research and/or publication of this article. The funding for the research was provided by the National Research Foundation of South Africa through the SARChI chair initiative (UID 84647) and the Agricultural Research Council -Vegetable, Industrial and Medicinal Plants.
Acknowledgments
The authors would like to thank the plant breeding team of the Agricultural Research Council - Vegetable, Industrial and Medicinal Plants for providing technical support.
Conflict of interest
The authors declare that the research was conducted in the absence of any commercial or financial relationships that could be construed as a potential conflict of interest.
The author(s) declared that they were an editorial board member of Frontiers, at the time of submission. This had no impact on the peer review process and the final decision.
Generative AI statement
The author(s) declare that no Gen AI was used in the creation of this manuscript.
Publisher's note
All claims expressed in this article are solely those of the authors and do not necessarily represent those of their affiliated organizations, or those of the publisher, the editors and the reviewers. Any product that may be evaluated in this article, or claim that may be made by its manufacturer, is not guaranteed or endorsed by the publisher.
References
Abdou, S. (2022). Evaluation of cowpea (Vigna unguiculata L. Walp) genotypes for high grain and fodder yields in the dry season of Niger republic. Heliyon. 8, e09147.x. doi: 10.1016/j.heliyon.2022.e09147
Adetiloye, I. S., Ariyo, O. J., Alake, C. O., Oduwaye, O. O., and Osewa, S. O. (2013). Genetic diversity of some selected Nigeria cowpea using simple sequence repeats (SSR) marker. Afr. J. Agric. Res. 8, 586–590. doi: 10.5897/AJAR11.811
Agronomix Statistical Software Inc. (2019). Agrobase Generation II version 38. Available online at: https://agronomix.com
Ajayi, A. T., Adekola, M. O., Taiwo, B. H., and Azuh, V. O. (2014). Character expression and differences in yield potential of ten genotypes of cowpea (Vigna unguiculata L. Walp). Int. J. Plant Res. 4, 63–71. doi: 10.5923/j.plant.20140403.01
Ali, Z. B., Yao, K. N., Odeny, D. A., Kyalo, M., Skilton, R., Eltahir, I. M., et al. (2015). Assessing the genetic diversity of cowpea (Vigna unguiculata L. Walp) accessions from Sudan using simple sequence repeat (SSR) markers. Afr. J. Plant Sci. 9, 293–304. doi: 10.5897/AJPS2015.1313
Aliyu, O. M., and Makinde, B. O. (2016). Phenotypic analysis of seed yield and yield components in cowpea (Vigna unguiculata L. Walp). Plant Breed. Biotech. 4, 252–261. doi: 10.9787/PBB.2016.4.2.252
Alves, P. A., and Bettencourt, E. (2007). Descriptors for cowpea (Vigna unguiculata L. Walp.). Rome, Italy: Biodivers. Int., 1–25.
Amiryousefi, A., Hyvönen, J., and Poczai, P. (2018). iMEC: Online marker efficiency calculator. Appl. Plant Sci. 6:e01159. doi: 10.1002/aps3.1159
Asiwe, J. A. N. (2009). Needs assessment of cowpea production practices, constraints and utilization in South Africa. Afr. J. Biotechnol. 8, 5383–5388. doi: 10.5897/AJB09.1293
Botstein, D., White, R. L., Skolnick, M., and Davis, R. W. (1980). “Construction of a genetic linkage map in man using restriction fragment length polymorphisms,” in Landmarks in medical genetics: Classic papers with commentaries, ed. P.S. Harper (New York: Oxford University Press), 142–151.
Dagnon, Y. D., Palanga, K. K., Bammite, D., Bodian, A., Akabassi, G. C., Foncéka, D., et al. (2022). Genetic diversity and population structure of cowpea (Vigna unguiculata L. Walp) accessions from Togo using SSR markers. PLoS ONE 17:e0252362. doi: 10.1371/journal.pone.0252362
Danso, B. A., Dzidzienyo, D. K., Prempeh, R. N., and Quain, M. D. (2018). DNA fingerprinting and assessment of genetic diversity among 22 cowpea (Vigna unguiculata L. Walp) varieties grown in Ghana. Open Access J. Sci. 5, 1–4. doi: 10.4236/oalib.1104938
Diouf, D., and Hilu, K. W. (2005). Microsatellites and RAPD markers to study genetic relationships among cowpea breeding lines and local varieties in Senegal. Gen. Res. Crop E52, 1057–1067. doi: 10.1007/s10722-004-6107-z
FAOSTAT (2021). Statistics of cowpea production. Available online at: https://www.fao.org/faostat/en/#data/QCL/2021 (accessed August 23, 2023).
Gerrano, A. S., Adebola, P. O., Jansen, W., van Rensburg, S., and Laurie, S. L. (2015).Genetic variability in cowpea (Vigna unguiculata L. Walp) genotypes. S. Afr. J. Plant Soil 32, 165–174. doi: 10.1080/02571862.2015.1014435
Gerrano, A. S., Jansen, W., van Rensburg, S., and Adebola, P. O. (2013). Assessment of genetic variability in bambara groundnut (Vigna subterranea L. Verdc.) using morphological quantitative traits. AJAR 1, 45–51. doi: 10.15413/ajar.2012.0129
Gerrano, A. S., Jansen, W., van Rensburg, S., and Kutu, F. R. (2019). Agronomic evaluation and identification of potential cowpea (Vigna unguiculata L. Walp) genotypes in South Africa. Acta Agric. Scand. B Soil Plant Sci. 69, 295–303. doi: 10.1080/09064710.2018.1562564
Gerrano, A. S., Jansen, W., van Rensburg, S., Mathew, I., Shayanowako, A. I., Bairu, M. W., et al. (2020). Genotype and genotype by environment interaction effects on the grain yield performance of cowpea genotypes in dryland farming system in South Africa. Euphytica 216, 1–11. doi: 10.1007/s10681-020-02611-z
Gonçalves, A., Goufo, P., Barros, A., Domínguez-Perles, R., Trindade, H., Rosa, E. A., et al. (2016). Cowpea (Vigna unguiculata L. Walp), a renewed multipurpose crop for a more sustainable agri-food system: nutritional advantages and constraints. J. Sci. Food Agric. 96, 2941–2951. doi: 10.1002/jsfa.7644
Gumede, M. T., Gerrano, A. S., Amelework, A. B., and Modi, A. T. (2022a). Analysis of genetic diversity and population structure of cowpea (Vigna unguiculata L. Walp) genotypes using single nucleotide polymorphism markers. Plants 11:3480. doi: 10.3390/plants11243480
Gumede, M. T., Gerrano, A. S., Modi, A. T., and Thungo, Z. (2022b). Influence of genotype and environment on grain yield among cowpea (Vigna unguiculata L. Walp) genotypes under dry land farming system. Acta Agric. Scand. B Soil Plant Sci. 72, 709–719. doi: 10.1080/09064710.2022.2069593
Iseki, K., Ikazaki, K., and Batieno, J. B. (2021). Cowpea yield variation in three dominant soil types in the Sudan Savanna of West Africa. Field Crops Res. 261:108012. doi: 10.1016/j.fcr.2020.108012
Ishiyaku, M. F., Yilwa, V. M., Singh, B. B., Olufajo, O. O., and Zaria, A. A. (2017). Phenotypic stability for selected traits of some cowpea lines in Nigerian agro-ecologies. Plant Breed. Biotech. 5, 67–77. doi: 10.9787/PBB.2017.5.2.67
Jaccard, P. (1908). Nouvelles recherches sur la distribution florale. Bulletin de la Société. Vaudoise des Sci. Natur. 44, 223–270.
Jayasingha, D. G. M. A., and Fernando, K. M. (2020). Identification of seedling root morphological traits associated with economic yield and yield components of cowpea (Vigna unguiculata L. Walp) AGRIEAST. J. Agric. Sci. 14, 17–30. doi: 10.4038/agrieast.v14i2.94
Kumari, J., and Chauhan, D. A. (2018). Genetic analysis for yield and contributing characters in cowpea (Vigna unguiculata L. Walp). J. Pharmacogn. Phytochem. 7, 2453–2458. doi: 10.20546/ijcmas.2018.707.395
Kyei-Boahen, S., Savala, C. E., Chikoye, D., and Abaidoo, R. (2017). Growth and yield responses of cowpea to inoculation and phosphorus fertilization in different environments. Front. Plant Sci. 8:646. doi: 10.3389/fpls.2017.00646
Mbuma, N. W., Gerrano, A. S., Lebaka, N., and Labuschagne, M. (2022). Interrelationship between grain yield components and nutritional quality traits in cowpea genotypes. S Afr J Bot. 150, 34–43. doi: 10.1016/j.sajb.2022.07.006
Mbuma, N. W., Gerrano, A. S., Lebaka, N., Mofokeng, A., and Labuschagne, M. (2021). The evaluation of a southern African cowpea germplasm collection for seed yield and yield components. Crop Sci. 61, 466–489. doi: 10.1002/csc2.20336
Moalafi, A. L., Asiwe, J. A., and Funnah, S. M. (2010). Germplasm evaluation and enhancement for the development of cowpea (Vigna unguiculata L. Walp) dual-purpose F2 genotypes. Afr. J. Agric. Res. 7, 573–579. doi: 10.5897/AJAR10.059
Mofokeng, M. A., Mashilo, J., Rantso, P., and Shimelis, H. (2020). Genetic variation and genetic advance in cowpea based on yield and yield-related traits. Acta Agric. Scand. B Soil Plant Sci. 70, 381–391. doi: 10.1080/09064710.2020.1749295
Nkhoma, N. (2020). Genetic improvement of cowpea (Vigna unguiculata L. Walp.) for grain yield and yield component components in Zambia (PhD thesis). University of Kwa-Zulu Natal, South Africa pp.1–183.
Odeseye, A. O., Amusa, N. A., Ijagbone, I. F., Aladele, S. E., and Ogunkanmi, L. A. (2018). Genotype by environment interactions of twenty accessions of cowpea (Vigna unguiculata L. Walp) across two locations in Nigeria. Ann. Agrar. Sci. 16, 481–489. doi: 10.1016/j.aasci.2018.03.001
Omomowo, O. I., and Babalola, O. O. (2021). Constraints and prospects of improving cowpea productivity to ensure food, nutritional security and environmental sustainability. Front. Plant Sci. 12:751731. doi: 10.3389/fpls.2021.751731
Owusu, E. Y., Amegbor, I. K., Darkwa, K., Oteng-Frimpong, R., and Sie, E. K. (2018). Gene action and combining ability studies for grain yield and its related traits in cowpea (Vigna unguiculata L. Walp). Cogent Food Agric. 4:1519973. doi: 10.1080/23311932.2018.1519973
Owusu, E. Y., Karikari, B., Kusi, F., Haruna, M., Amoah, R. A., Attamah, P., et al. (2021). Genetic variability, heritability and correlation analysis among maturity and yield traits in Cowpea (Vigna unguiculata L. Walp) in Northern Ghana. Heliyon 7:e07890. doi: 10.1016/j.heliyon.2021.e07890
Owusu, E. Y., Mohammed, H., Manigben, K. A., Adjebeng-Danquah, J., Kusi, F., Karikari, B., et al. (2020). Diallel analysis and heritability of grain yield, yield components, and maturity traits in cowpea (Vigna unguiculata L. Walp). Sci. World J. 1:9390287. doi: 10.1155/2020/9390287
Perrier, X., and Jacquemoud-Collet, J. (2006). DARwin software. Available online at: http://darwin.cirad.fr/darwin (accessed September 20, 2024).
Saghai-Maroof, M. A., Soliman, K. M., Jorgensen, R. A., and Allard, R. (1984). Ribosomal DNA spacer-length polymorphisms in barley: Mendelian inheritance, chromosomal location, and population dynamics. PNAS 81, 8014–8018. doi: 10.1073/pnas.81.24.8014
Sarr, A., Bodian, A., Gbedevi, K. M., Ndir, K. N., Ajewole, O. O., Gueye, B., et al. (2021). Genetic diversity and population structure analyses of wild relatives and cultivated cowpea (Vigna unguiculata L. Walp) from Senegal using simple sequence repeat markers. Plant Mol. Biol. Rep. 39, 112–124. doi: 10.1007/s11105-020-01232-z
Seo, E., Kim, K., Jun, T. H., Choi, J., Kim, S. H., Muñoz-Amatriaín, M., et al. (2020). Population structure and genetic diversity in Korean cowpea germplasm based on SNP markers. Plants 9:1190. doi: 10.3390/plants9091190
Shanko, D., Andargie, M., and Zelleke, H. (2014). Interrelationship and path coefficient analysis of some growth and yield traits in cowpea (Vigna unguiculata L. Walp) genotypes. J. Plant Sci. 2, 97–101. doi: 10.11648/j.jps.20140202.13
Shete, S., Tiwari, H., and Elston, R. C. (2000). On estimating the heterozygosity and polymorphism information content value. Theor. Popul. Biol. 57, 265–271. doi: 10.1006/tpbi.2000.1452
Siwale, J., Labuschagne, M., Gerrano, A. S., and Mbuma, N. W. (2022). Phenotypic diversity and characterization of the Southern African Bambara groundnut germplasm collection for grain yield and yield components. Agronomy 12:1811. doi: 10.3390/agronomy12081811
Stoilova, T., and Pereira, G. (2013). Assessment of the genetic diversity in a germplasm collection of cowpea (Vigna unguiculata L. Walp) using morphological traits. Afr. J. Agric. Res. 8, 208–215. doi: 10.5897/AJAR12.1633
Thorat, A., and Gadewar, R. D. (2013). Variability and correlation studies in cowpea (Vigna unguiculata L. Walp). ESSENCE Int. J. Env. 4, 44–49.
Upadhyaya, H. D., Kashiwagi, J., Varshney, R. K., Gaur, P. M., Saxena, K. B., Krishnamurthy, L., et al. (2012). Phenotyping chickpeas and pigeon peas for adaptation to drought. Front. Physiol. 3:179. doi: 10.3389/fphys.2012.00179
Walle, T., Mekbib, F., Amsalu, B., and Gedil, M. (2018). Correlation and path coefficient analyses of cowpea (Vigna unguiculata L. Walp) landraces in Ethiopia. Am. J. Plant Sci. 9, 2794–2812. doi: 10.4236/ajps.2018.913202
Yirzagla, J., Atokple, I. K., Haruna, M., Mohammed, A. R., Adobaba, D., Haruna, B., et al. (2021). “Impacts of cowpea innovation platforms in sustaining TL III project gains in Ghana,” in Enhancing smallholder farmers' access to seed of improved legume cultivars through multi-stakeholder platforms, Learning from the TLIII project experiences in sub-Saharan Africa and South Asia, eds. E. Akpo, C.O. Ojiewo, I. Kapran, L.O. Omoigui, A. Diama R.K. Varshney (Singapore: Springer), 171–183. doi: 10.1007/978-981-15-8014-7_12
Keywords: heritability, cowpea, genetic diversity, grain yield, breeding
Citation: Matjeke MB, Labuschagne MT, Gerrano AS, Minnaar-Ontong A and Mbuma NW (2025) Heritability and expression of yield and yield components in cowpea, an underutilized crop in Africa. Front. Sustain. Food Syst. 9:1588245. doi: 10.3389/fsufs.2025.1588245
Received: 05 March 2025; Accepted: 16 April 2025;
Published: 15 May 2025.
Edited by:
Henry Awika, University of the Virgin Islands, US Virgin IslandsReviewed by:
Mazharul Islam, University of the Virgin Islands, US Virgin IslandsDevi Kandel, Langston University, United States
Copyright © 2025 Matjeke, Labuschagne, Gerrano, Minnaar-Ontong and Mbuma. This is an open-access article distributed under the terms of the Creative Commons Attribution License (CC BY). The use, distribution or reproduction in other forums is permitted, provided the original author(s) and the copyright owner(s) are credited and that the original publication in this journal is cited, in accordance with accepted academic practice. No use, distribution or reproduction is permitted which does not comply with these terms.
*Correspondence: Maryke Tine Labuschagne, bGFidXNjbUB1ZnMuYWMuemE=