- 1Department of Chemistry, Birzeit University, Birzeit, Ramallah, Palestine
- 2Department of Biology, University of Antwerp, Antwerp, Belgium
- 3Department of Public Health, Faculty of Medicine and Health Sciences, An-Najah National University, Nablus, Palestine
Introduction: Fish consumption can be a primary pathway for human exposure to toxic metals such as, Cd, As, Hg, and Pb. Even at relatively low concentrations, long-term exposure to these metals might result in accumulation levels leading to toxicity. Therefore, monitoring their concentrations in fish regularly is crucial to ensure consumer safety. We aimed to measure the concentrations of As, Cd, Hg, and Pb in different fish and canned fish samples and to compare the results with permissible exposure limits to assess potential human health hazards.
Methods: Thirteen different fish species and eight canned fish samples were collected from local markets at Ramallah city, Palestine. Following microwave-assisted digestion, a high-resolution inductively coupled plasma-mass spectrometer (HR-ICP-MS) was used to measure the concentrations of these metals in the digested samples.
Results and discussion: The ranges of average metal concentrations [expressed in μg/g dry weight (dw)] in the 13 tested fish species were as follows: As (0.03–54.27), Cd (<limit of quantitation (LOQ)-0.11), Hg (<LOQ-0.18), and Pb (0.02–0.07). In canned fish samples, the average concentrations were as follows: As (1.68–5.65), Cd (0.02–0.12), Hg (<LOQ–0.20), and Pb (0.02–0.07). Health risk assessment based on estimated daily intake (EDI), target hazard quotient (THQ), and carcinogenic risk (CR), indicated no cancer and non-carcinogenic health risk. Although, our results pointed to no possible health risk associated with these metals from fish and canned fish intake, environmental and human exposure assessment should be sustained for long-term food security.
Introduction
Living organisms need trace amounts of some metals, such as cobalt, copper, iron, manganese, molybdenum, vanadium, strontium, and zinc, but excess amounts are usually detrimental. Although many metals are non-toxic to living organisms under certain conditions, they can be toxic in certain forms at high doses (Barakat, 2011). Measuring metal concentrations in representative samples is crucial prior to assessing their toxicity. Metals are non-biodegradable, environmentally persistent, and can bio-accumulate and bind to short carbon chains (Edelstein and Ben-Hur, 2018).
Metals can disrupt cellular activities including growth, proliferation, differentiation, damage–repair processes, and apoptosis. When ingested in excessive amounts, they can combine with biomolecules, such as proteins and enzymes, to form stable bio-toxic compounds, thereby mutilating their structures; consequently, this may prevent their biological functions (Duruibe et al., 2007).
Seafood and processed seafood are considered rich sources of healthy vitamins, proteins, omega-3 fatty acids, selenium, calcium, and minerals, all of which are important for human health (Kalantzi et al., 2016; Yasmeen et al., 2016; Zhang et al., 2017). Almost all elements that are considered essential for the maintenance of normal physiological functions are found in seafood (Tanaskovski et al., 2016). The American Heart Association recommends two servings of fish per week as part of a healthy diet (Neff et al., 2014). Therefore, monitoring the levels of metals in commercial fish and canned fish regularly is crucial to ensure consumer safety.
This study is of crucial importance to human health, both at the national and international levels as it evaluates exposure to a complex mixture of metals in a common food source worldwide. Health authorities should be notified of such results to regulate human exposure to these metals.
Materials and methods
Chemicals and reagents
All reagents and solutions were prepared using water obtained from a Milli-Q water purification system (Millipore). All chemicals used for sample preparation were of analytical reagent grade or higher purity. Nitric acid (HNO3, VWR, electronic purposes “e.p.”) was further purified by sub-boiling distillation. Prior to use, all reagent containers underwent a strict cleaning procedure as described by Qadah et al. (2023) to prevent unnecessary contamination. They were filled with a 50% “1:1” mixture of concentrated HNO3 and hydrochloric acid (HCl, VWR, pro-analyse “p.a.”) and left for at least 48 h, subsequently rinsed with Milli-Q water, and placed in a 20–30% (v/v) HNO3 bath. After 24 h, they were thoroughly washed with Milli-Q water and stored in a clean plastic box at room temperature.
Sample collection
A total of 13 fish species and eight canned fish (six tuna and two sardine cans) samples were collected from local markets in Ramallah city (Table 1, Supplementary File 1) on 10 February 2022. These samples were selected following the recommendations of experts at the Palestinian Ministry of Agriculture in Ramallah city. Based on their experience, these fish and canned fish are the most popular for consumption among local Palestinian residents. For all fish samples, three specimens of average size available in the market at the time of sampling were collected, except for salmon fish, for which ~1.0 kg of filet was collected because it is “mostly” sold as a pre-cut filet rather than a whole fish. The fresh fish samples were placed immediately in polyethylene (PE) bags, kept in an insulated box filled with ice, and transported to the Chemistry laboratory at Birzeit University, where they were washed with deionized water and stored at −20°C until needed. For canned fish samples, three replicates of each type were collected and stored in a clean, dry place at room temperature until needed for treatment.
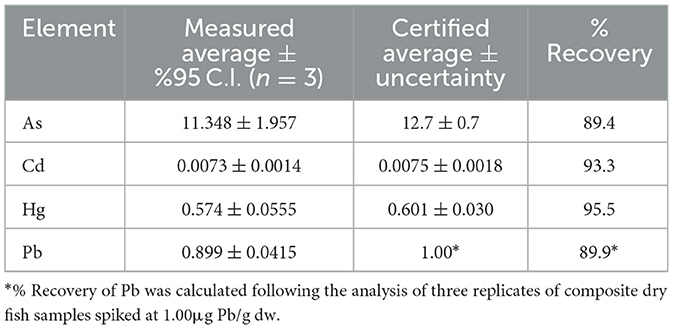
Table 1. Average concentrations (expressed in μg/g dw ± 95% C.I., n = 3), and percent recoveries of As, Cd, and Hg measured in the certified reference material (ERM-BB422, fish muscle), and percent recovery of Pb obtained for three replicates of composite dry fish tissue samples spiked at 1.00 μg/g dw.
Sample pretreatment
The fish samples were washed with deionized water to remove adsorbed salts from the skin before cutting. The skin and bones were separated from the muscle tissue. Fish cans were opened, and excess oil was manually drained (as effectively as possible by squeezing the oil through a piece of porous fabric) from the fish tissues. Composite tissue samples were taken from the whole fish and canned fish, then homogenized using an electric food processor equipped with hard-plastic blades. Each homogenized sample was then divided into three portions and stored in pre-cleaned polyethylene plastic vials at −20°C: (i) ~15 g portion for the determination of moisture and dry mass contents; (ii) ~100 g portion for ICP-MS analysis; and (iii) the remaining portion stored as backup. After freezing at −20°C for at least 48 h, the 100 g portion samples were shipped frozen to the laboratories of the Biology Department at An-Najah University (Nablus city), where they were freeze-dried using a lyophilizer. The dried tissue samples were then shipped to the laboratories of the SPHERE group (Systemic Physiological and Ecotoxicological Research) at the University of Antwerp, Belgium, for further analysis.
Moisture and dry mass contents
Moisture and dry mass contents in fish and canned fish were determined following the standard hot air method of the Association of Official Analytical Chemists (AOAC, 2019). In short, three replicates of wet samples were dried at 105°C to a constant weight using a hot air oven. The weight loss was used to calculate the moisture content of the samples, and the dry mass content was then calculated as 100 – %moisture.
Sample digestion and analysis
In the SPHERE lab, fish samples were transferred to pre-cleaned 80 mL microwave digestion vessels. From each sample, three replicates of approximately 0.20 g were weighed using a Mettler AT261 DeltaRange® (±0.001 g) sensitive balance and placed in the digestion vessels. To each vessel, 4 mL of ultra-pure concentrated HNO3 (69%, ICP-MS reagent grade) was added. The samples were then allowed to digest at room temperature for 24 h under a fume hood, and then 400 μL of high-purity grade hydrogen peroxide (H2O2, 29 %) was added to each vessel. The samples were left open for 30 min, and then closed. Subsequently, the samples were digested using a SP-Discover Microwave (CEM, USA) following a method slightly modified from the standard method described by Mataba et al. (2016). In this regard, digestion was accomplished by heating the vessels from room temperature to 200°C at a ramping rate of 5.0°C/min, held isothermal at 200°C for 5 min. Maximum microwave power was set at 300 Watts, and the pressure inside the digestion vessels increased steadily; the fish tissues were considered fully digested when the pressure in the vessels reached a plateau (typically below 400 psi). When digestion was completed, the samples were diluted to 50 mL with highly purified water obtained from Millipore Milli-Q® (Integral 3 Water Purification System, Merck KGaA, Darmstadt, Germany). The system is fitted with a Q-POD® Element unit, which is an additional point-of-delivery purifier designed to deliver ultrapure water, specifically dedicated to HR-ICP-MS analysis. Prior to metal analysis, the sample solutions were further diluted to bring the acid concentrations to 2–3% (v/v). For quality assurance/quality control (QA/QC) and method validation purposes, three replicates of standard reference fish material (ERM-BB422, European Union Joint Research Center, JRC-IRMM, Geel, Belgium), and three method blanks were subjected to the same microwave digestion procedure and processed for analysis alongside the fish samples. Measured metal concentrations were compared with the corresponding values reported in the certificate of analysis (COA).
Toxic metal analysis
Analysis of As, Cd, Hg, and Pb in the sample solutions was performed using HR-ICP-MS in cold plasma mode (Thermo Scientific Finnigan Element 2, Waltham, MA, USA), with an instrumental detection limit of 0.001 μg/L, as described by Rodriguez-Levy et al. (2022). For quantification of metal concentrations, a series of calibration standards were prepared by diluting high-purity ICP-MS multi-element standard solutions obtained from Agilent Technologies (Santa Clara, CA, USA). For additional QA/QC purposes, an external water sample Standard Reference Material (SRM-1643f, National Institute of Standards and Technology “NIST”, Gaithersburg, MD, USA) was also analyzed at the beginning, middle, and end of the analysis. The use of a NIST standard in this case was to validate the accuracy and precision of the instrument during metals analysis. In the results section, concentrations were first calculated and reported in μg/g dry weight (dw), and then in μg/g wet weight (ww) to calculate the various health risk assessment parameters described in the following subsections.
Human health risk assessment of toxic metals
Under conditions of regular consumption, it is important to assess the metal pollution index, estimated daily intake (Elnabris et al., 2013), target hazard quotients (THQ), and hazard index (HI) of toxic metals from fish consumption (Bogdanović et al., 2014; Zhelyazkov et al., 2018), and compare these with the values of the recommended daily dietary allowance (RDA) and the provisional tolerable daily intake (PTDI) suggested by some international food safety organizations, such as the Food and Agriculture Organization (FAO) of the United Nations (UN) and the World Health Organization (WHO) (FAO/WHO, 1999, 2003, 2009).
Metal pollution index
The metal pollution index (MPI) was calculated to assess metal pollution using Equation 1 below as described by other workers (Usero et al., 2005; Abdel-Khalek et al., 2016):
where CM1 is the concentration of the first metal, CM2 is the concentration of the second metal, CM3 is the concentration of the third metal, and CMn is the concentration of the nth metal, all expressed in μg/g wet weight (ww) in the tissue sample of a particular species.
Estimated daily intake
Estimated daily intake (EDI) was calculated based on the metal concentrations in fish and daily fish consumption. Equation 2 was used to calculate EDI as described by the USEPA (2000a, 2010) and other workers (Wei et al., 2014; Varol et al., 2017; Sarker et al., 2020):
where Cn is the concentration of metal in the selected fish muscle tissues (μg/g ww), IGr is the expected ingestion rate (8.22 g/day according to the Palestinian Ministry of Agriculture in the West Bank, and 11.66 g/day according to the Directorate General of Fisheries at the Palestinian Ministry of Agriculture in the Gaza Strip), and Bw is the body weight (70 kg was used) as reported by USEPA (2008).
Target hazard quotient
Target hazard quotient (THQ) was estimated by the ratio of EDI to the oral reference dose (RfD). A ratio value < 1 implies non-significant risk effects (Baki et al., 2018). Equation 3 was used to calculate THQ as described in other studies (Baki et al., 2018; Traina et al., 2019; Sarker et al., 2020):
where Ed is the exposure duration (65 years) as described by USEPA (2008), Ep is exposure frequency (365 days/year), RfDs are the reference doses in mg element per kg per day, based on 0.0003, 0.001,0.0005, and 0.004 mg/kg/day for As, Cd, Hg, and Pb, respectively, as followed in other studies (Wang et al., 2005; Baki et al., 2018), and At is the average time for non-carcinogenic elements (Ed × Ep).
Hazard index
Hazard index (HI) values in the tested fish samples were calculated using Equation 4 shown below as described by other workers (Yi et al., 2011; Orajiaka-Uchegbu et al., 2020):
where THQ is the estimated risk value for individual metals. When the HI value is > 1, the non-carcinogenic risk effect is considered high for “potentially” exposed consumers (Dadar et al., 2017; Fakhri et al., 2017).
Carcinogenic risk
Carcinogenic risk (CR) was calculated to assess potential cancer cell development in fish or canned fish consumer's body over a lifetime due to long-term exposure to “potentially toxic” metals (Zhong et al., 2018). The acceptable range of the CR limit is 10−6 to 10−4 as reported in other studies (Li et al., 2013). Equation 5, followed by other workers, was used to assess CR (Cao et al., 2014; Vu et al., 2017):
where CSF is the oral slope factor of a particular carcinogen (mg/kg/day); CSF values are available only for As (1.5), Pb (0.0085), and Cd (6.3) (USEPA, 2000a,b, 2010).
Data analysis
Descriptive statistics to calculate average, range, standard deviation, median, and 95% confidence interval (C.I.) were performed using Microsoft Excel (Microsoft Office, 2019). No other statistical analyses were conducted. Chart plots (shown in Figures 1, 2) were prepared using GraphPad Prism 9 software.
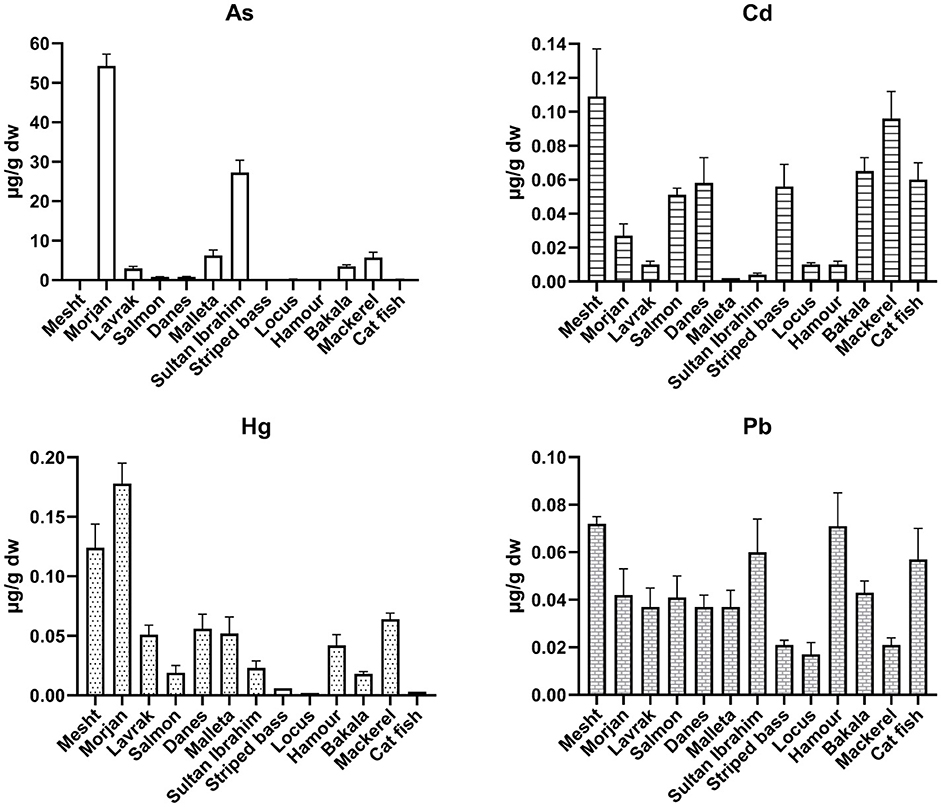
Figure 1. Average concentrations of toxic metals in 13 fish species expressed in μg/g dw (± 95% confidence interval “CI”, n = 3).
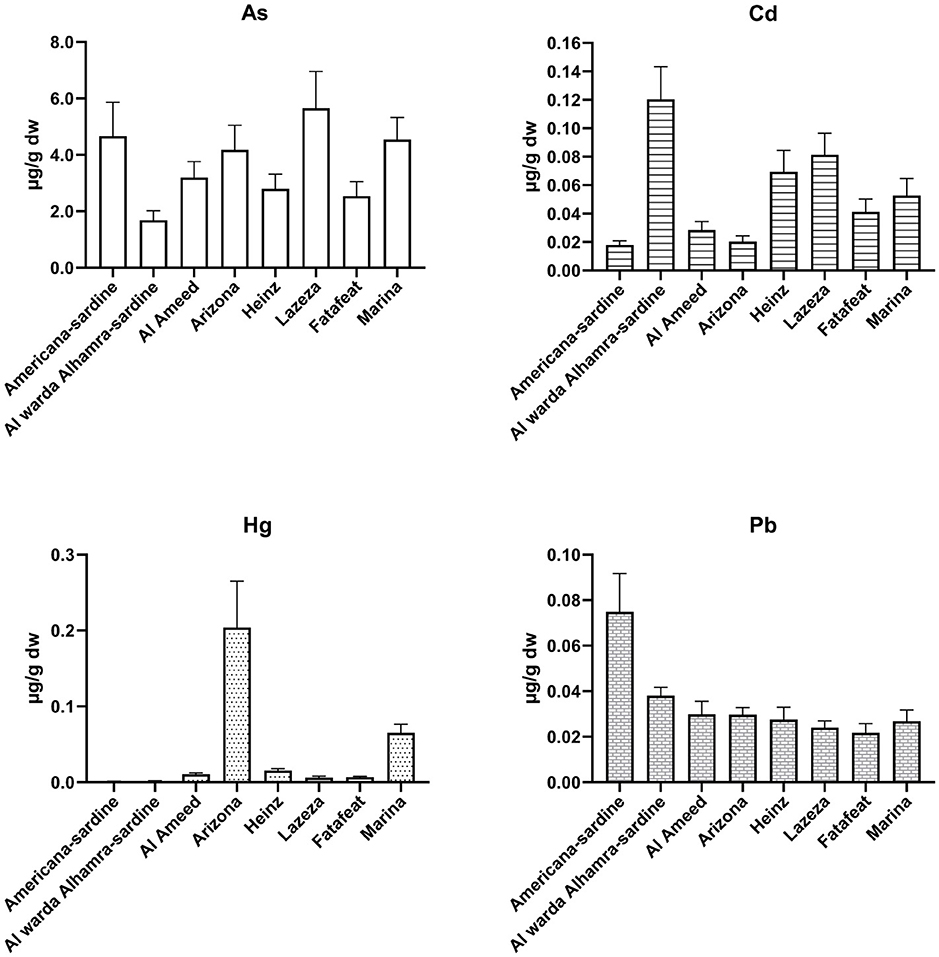
Figure 2. Average concentrations of toxic metals in eight canned fish samples expressed in μg/g dw (± 95% CI, n = 3).
Results and discussion
Method validation and quality assurance
The average concentrations of the four toxic metals with their corresponding 95% confidence interval (C.I.). and the certified values with their uncertainties are shown in Table 1. The calculated percent recoveries (% recovery = (measured concentration/certified concentration) × 100) were within acceptable values, i.e, 89%, 93%, 96%, and 90% for As, Cd, Hg, and Pb, respectively. It should be noted that the certificate of analysis (COA) did not include a certified value for Pb; therefore, three replicates of composite dry fish tissue samples spiked at 1.00 μg Pb/g dw were processed and measured following the same method to calculate the % recovery of Pb.
Concentrations of toxic metals in fish
The average concentrations of toxic metals in 13 fish species (expressed in μg/g dw) are shown in Table 2, Figure 1. Average As ranged from 0.03 to 54.27 (mean of 0.83), Cd from < LOQ to 0.11 (0.05), Hg from < LOQ to 0.18 (0.04), and Pb ranged from 0.02 to 0.07 (0.04). The highest mean concentrations of As and Hg were measured in morjan fish (54.3 and 0.18 μg/g dw, respectively), the highest level of Cd was found in mesht fish (0.11 μg/g dw), and the highest values of Pb were detected in mesht and hamour fish samples (both were 0.07 μg/g dw). The lowest concentration of As was measured in striped bass fish (0.03 μg/g dw), the lowest levels of Cd were found in malleta and sultan ibrahim fish samples (both were 0.002 μg/g dw), the lowest Hg contents were those detected in locus and catfish samples (both were 0.002 μg/g dw), and the lowest Pb concentrations were found in striped bass, locus, and mackerel fish samples (all were 0.02 μg/g dw). The high concentrations of Cd and Pb in mesht fish were quite surprising because mesht fish is raised in local farms at Jericho city or imported from fish farms in neighboring countries. Careful investigation is needed to determine the source of potential metal contamination in mesht fish from these farms.
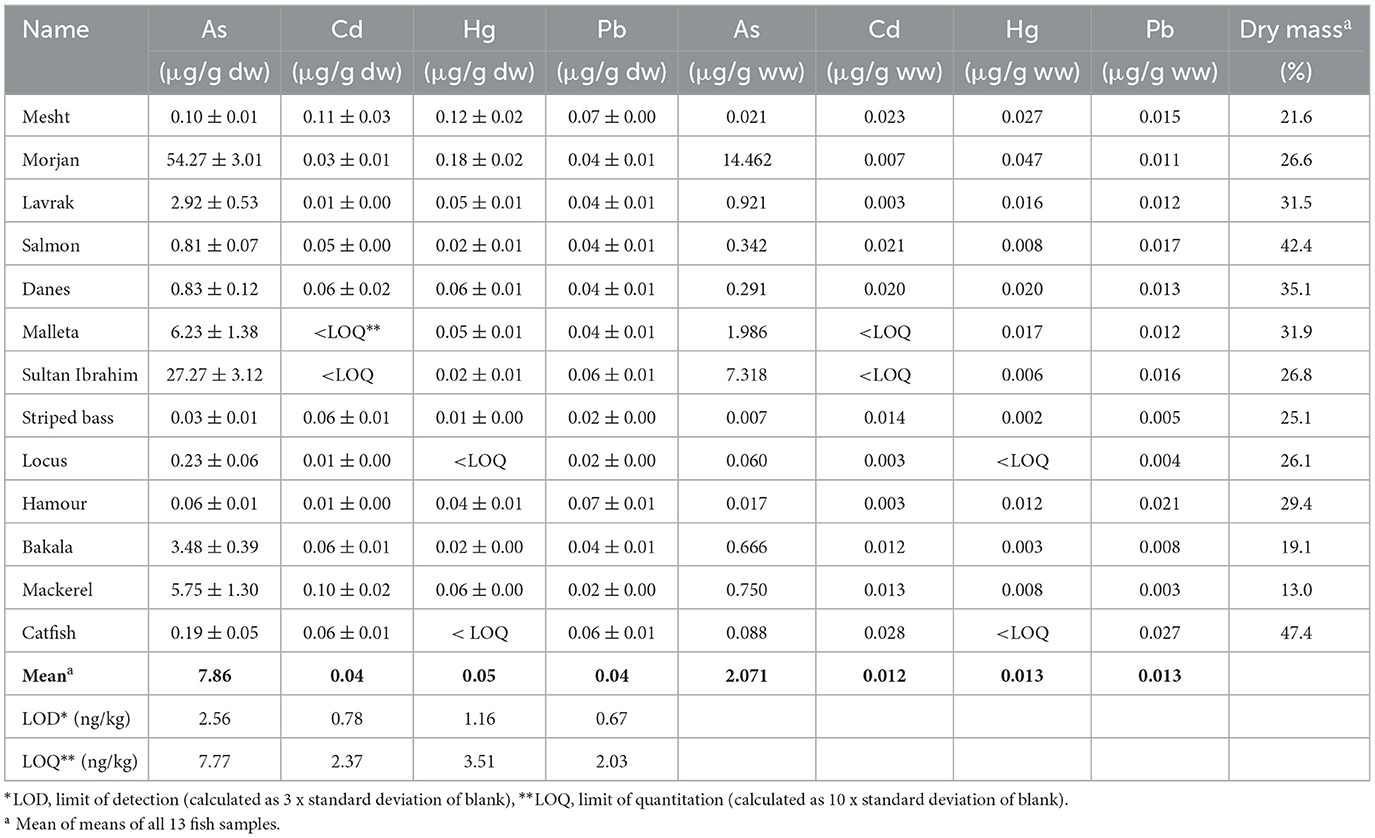
Table 2. Average concentrations of toxic metals expressed in dry weight (μg/g dw ± 95% C.I., n =3) and wet weight (μg/g ww) bases along with average percentage of dry mass in the 13 fish species.
As concentrations (expressed in μg/g dw, Table 2) in the fish samples of our study were much higher than those reported by Belivermiş et al. (2016) in a study conducted in Türkiye, where As concentrations ranged from 5.30 to 18.30 μg/g dw (mean of 9.30 μg/g dw, n = 20). Ustaoglu and Yüksel (2024) reported an average As concentration of 0.163 μg/g ww in four different fish species in a recent study conducted in Türkiye. The mean As content in the 13 tested fish samples (2.071 μg/g ww, Table 2) was about 13 times higher than that of Ustaoglu and Yüksel. The California Environmental Protection Agency (CEPA, 1995–97) set 1.0 μg/g ww as the maximum permissible concentration (MPC) for As in edible fish; thus, As levels (when expressed in μg/g ww, Table 2) in 3 out of the 13 tested fish samples exceeded the MPC of California state and also exceeded the FAO (1983) MPC for As (1.0 μg/g ww). Namely, those were morjan fish (14.46 μg/g ww), malleta fish (1.99 μg/g ww), and sultan ibrahim fish (7.32 μg/g ww). However, because As concentrations reported herein represent total arsenic, one should be cautious when interpreting these results. Recent studies suggested that the majority of As in fish is present as arsenobetaine (AsB), which is considered non-toxic to humans (Hoy et al., 2023). For example, Krishnakumar et al. (2016) reported total As content ranging from 11 to 134 μg/g dw in Arabian Gulf seafood, with ~70% of which being AsB, while the inorganic arsenic (iAs) fraction was < 2%. Kalantzi et al. (2017) studied As speciation in 12 different fish species collected from Greek coastal zone, where the total As levels varied between 11.8 and 62.6 μg/g dw with the AsB fraction accounting for 67–95% of it. Moreover, Ustaoglu et al. (2024) estimated that iAs makes up to 10% of total As concentration. Therefore, measuring the concentrations of all possible As species, organic and inorganic, is a crucial step in health risk assessment associated with potential As contamination. Conducting such a speciation analysis was beyond the scope of our study simply because we lack the necessary instrumentation and infrastructure to do so at Birzeit University.
The maximum permissible concentration (MPC) of Cd in the muscle meat of fish ranges from 0.05 to 0.25 μg/g ww as specified by the EU Commission Regulation (2023), and 0.50 μg/g ww as described by FAO/WHO (2011). In our study, the concentrations (expressed on a wet weight basis, μg/g ww) of Cd detected in all 13 fish samples (Table 2) were below the threshold limits set by the EU and FAO/WHO. Two similar studies conducted in Saudi Arabia on similar fish species, the first was conducted by Alturiqi and Albedair (2012), where Cd concentrations ranged from 1.17 to 4.25 μg/g dw; while the second was conducted by Younis et al. (2021), where Cd concentrations ranged from 3.00 to 5.10 μg/g dw for five different fish species collected from Jeddah coast. The Cd concentrations measured in the 13 fish species of this study were lower compared to those reported by Alturiqi and Albedair (2012) and those reported by Younis et al. (2021). Moreover, Al-Weher (2008) measured the concentrations of Cd, Cu, and Zn in three fish species (Oreochromis aureus, Cyprinus carpio, and Clarias lazera) collected from the Northern Jordan Valley. Mean Cd concentrations ranged between 0.02 and 0.24 (median of 0.14 μg/g dw); Cd concentrations in fish measured in our study were within the range reported by Al-Weher (2008).
Concentrations of Hg varied among the 13 tested species, with the highest level in morjan and the lowest in locus (Table 2, Figure 1). These Hg concentrations were comparable to those reported by Alturiqi and Albedair (2012), where Hg concentrations measured in farmed fish samples collected from Saudi Arabian markets ranged from 0.014 to 0.055 μg/g dw. It should be noted, however, that Hg concentrations (when expressed in μg/g ww, Table 2) in the 13 tested fish samples were below the maximum permissible concentrations of Hg in fish (0.50 and 0.5-1.0 μg/g ww) described by FAO/WHO (2009, 2011) and the EU Commission Regulation (2023), respectively.
The concentrations of Pb in the 13 tested fish samples also varied, with the highest level in mesht and the lowest in locus (Table 2, Figure 1). The average concentrations of Pb in this study (0.02–0.07, mean of 0.04 μg/g dw) were lower than those reported by: (i) Younis et al. (2021), where Pb concentrations ranged from 0.70 to 0.80 μg/g dw; (ii) Aytekin et al. (2019), where Pb levels varied between 22.18 and 62.75 μg/g dw in different tissues of Penaeus semiculatus collected from the coast of Iskenderun Gulf (Türkiye); and (iii) Abarshi et al. (2017), who reported Pb contents of 0.20 to 0.50 μg/g dw in some organs of fish samples collected from Bonny River, Nigeria. It should be noted that Pb concentrations (when expressed in μg/g ww, Table 2) measured in all fish samples of the present study were lower than the maximum acceptable levels of Pb (0.3 and 0.5 μg/g ww) set by the EU Commission Regulation (2023) and WHO (1996), respectively.
Concentrations of toxic metals in canned fish
The average concentrations of toxic metals in eight canned fish samples (expressed in μg/g dw) are shown in Table 3, Figure 2, and were as follows: As concentrations ranged from 1.68 to 5.65 (mean of 3.69), Cd levels from 0.02 to 0.12 (0.05), Hg concentrations varied from < LOQ-0.20 (0.01), and Pb contents from 0.02 to 0.07 (0.03). The highest concentrations were those of As, while concentrations of Cd, Hg, and Pb were “relatively” low and comparable in the eight canned fish samples under investigation. The highest concentrations (in μg/g dw) of the four tested toxic metals were as follows: As in Lazeza tuna cans (5.65), Cd in Al warda Al hamra sardine cans (0.12), Hg in Arizona tuna cans (0.20), and Pb in Americana sardine cans (0.075). The lowest levels of these toxic metals were as follows: As in Al warda Al hamra sardine cans (1.68), Cd in Arizona tuna and Americana sardine cans (0.02), Hg in Americana sardine (0.001) and Al warda Al hamra sardine cans (0.002), and Pb found in Lazeza tuna (0.024) and Fatafeat tuna cans (0.022).
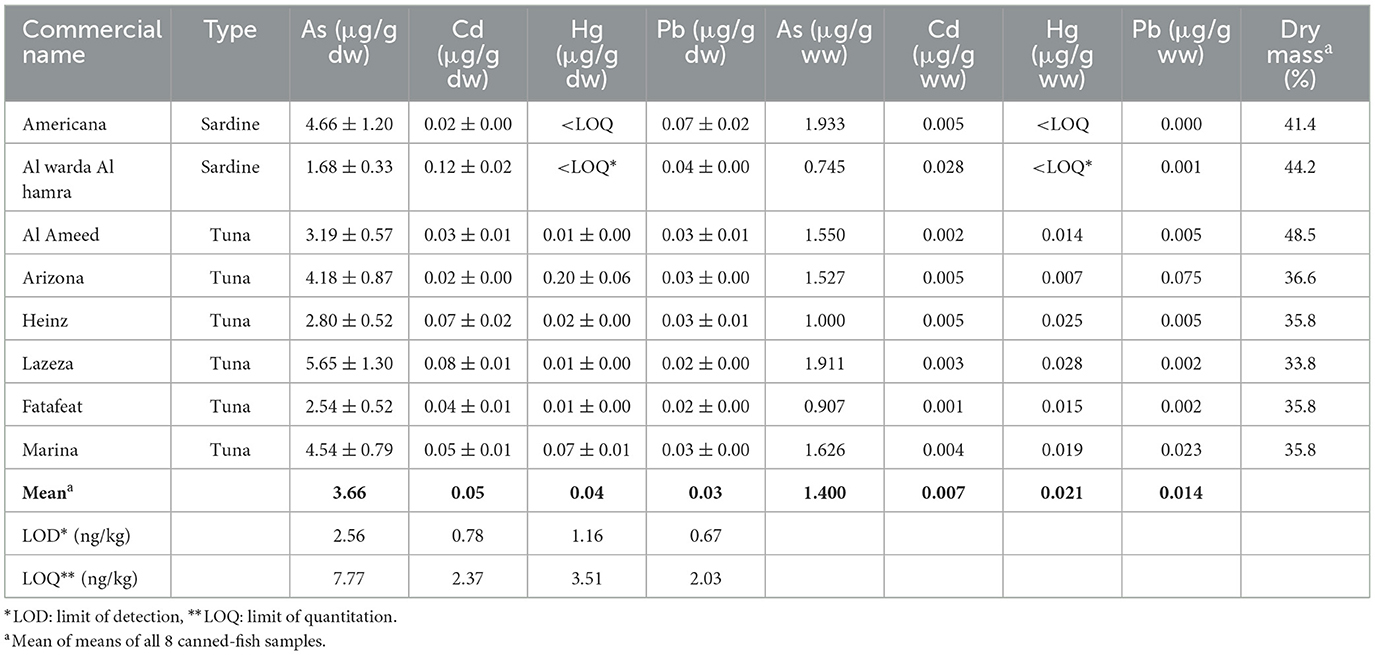
Table 3. Average concentrations of toxic metals expressed in dry weight (μg/g dw ± 95% C.I., n =3) and wet weight (μg/g ww) bases along with average percentage of dry mass in the eight canned fish samples.
The highest concentrations of Cd and Pb were detected in Al warda Al hamra sardine and Americana sardine cans. These high Cd and Pb concentrations were also quite surprising because sardines are supposed to be “relatively” much less contaminated with metals or organic pollutants, especially when compared to tuna canned fish due to several reasons. First, the size of sardine fish is much smaller than that of tuna fish and all other fish species investigated; second, the lifespan of sardine fish is much shorter compared to that of all examined fish species; third, consequently, the weight and fat content of sardine fish are much less than those of all fish species tested in this study. We expected to find higher metal accumulations in the six tuna canned samples compared to those accumulated in the two sardine canned samples. Since the opposite trend was observed, further investigations are needed to identify the sources of metal contamination in sardine cans marketed in local markets in Ramallah city.
On a wet weight basis, concentrations of As in the eight canned fish samples ranged from 0.745 to 1.933 μg/g ww (Table 3). Concentrations in six of the eight tested canned fish samples exceeded the MPC of As (1.0 μg/g ww) described by CEPA (1995–97) and FAO (1983), suggesting that based on total As content, only two of the eight tested canned fish brands are safe for human consumption. However, this conclusion might be misleading due to the discussion presented earlier regarding As speciation. Only a small fraction ( ≤ 10%) of total As content in fish is inorganic, as mentioned earlier, while most of it is present in different organic arsenical compounds, which are “non-toxic” (Krishnakumar et al., 2016; Kalantzi et al., 2017; Hoy et al., 2023; Ustaoglu et al., 2024). Therefore, upon calculating the fraction of iAs as 10% of the total As concentrations shown in Table 3, one might conclude that all eight tested canned fish are safe for consumption.
In two similar studies conducted on canned fish samples collected from Lebanese and Iranian markets, Korfali and Abou Hamdan (2013) reported Cd concentrations ranging from 0.021 to 0.645 μg/g dw (n = 14), while Hosseini et al. (2015) reported Cd concentrations ranging from 0.00 to 0.37 μg/g dw (n = 30). We observed that the Cd concentrations measured in canned fish in the present study were generally lower than those reported by the two studies mentioned above. In another study performed on canned fish samples in Türkiye, Tuzen and Soylak (2007) reported Cd concentrations ranging from 0.06 to 0.25 μg/g dw (n = 4); the Cd concentrations measured in canned fish in the present study were also lower than those reported by Tuzen and Soylak.
The concentrations of Hg measured in our canned fish samples were relatively lower than those reported by Korfali and Abou Hamdan (2013), where Hg concentrations ranged from 0.025 to 0.395 μg/g dw (n = 14) in a study conducted in Lebanon, which was mentioned earlier.
A comparison of the concentrations of the four toxic metals measured in the fish and canned fish samples of the present study, as well as those reported by similar studies conducted in other neighboring and non-neighboring countries, is shown in Supplementary Files 2 and 3 (Tuzen and Soylak, 2007; Al-Weher, 2008; Hilal and Ismail, 2008; Islam et al., 2010; Obeid et al., 2011; Alturiqi and Albedair, 2012; Mahalakshmi et al., 2012; Korfali and Abou Hamdan, 2013; Hosseini et al., 2015; Belivermiş et al., 2016; Zohra and Habib, 2016; Abarshi et al., 2017; Al-Qadasy et al., 2018; Keshavarzi et al., 2018; Popovic et al., 2018; Aytekin et al., 2019; Kowalska et al., 2020; Younis et al., 2021). The differences in the concentrations of some toxic metals observed when comparing our results with those of some of these studies, as we described and discussed earlier, could be attributed to various reasons, such as: (i) the history of contamination of the water, suspended particulate matter (SPM), and sediments where the tested fish species lived; (ii) possible variations in the age, weight, length, and fat content among the fish and canned fish samples investigated in these studies; and (iii) variations in environmental factors, such as water pH, dissolved oxygen, dissolved carbon, total organic carbon in SPM and sediments, and redox potential of sediments. These factors could lead to significant differences in the physical and chemical behaviors of the studied metals, thus affecting their potential uptake, bioaccumulation, and biomagnification by the fish species under consideration. It is important to note that investigating the variations of these factors to assess their impact on the concentrations of toxic metals in fish and canned fish samples was beyond the scope of this study.
Comparison with international dietary standards and guidelines
The mean concentrations of the four toxic metals measured in the fish samples of the present study, along with maximum permissible concentrations (MPC) described by several international organizations such as WHO, EU, and FAO, are shown in Table 4. The results revealed that the concentrations of the analyzed toxic metals were lower than the MPC established by all three international agencies, except for As. Estimated daily intake (EDI), target hazard quotient (THQ), and carcinogenic risk (CR) were analyzed to interpret potential effects on public health.
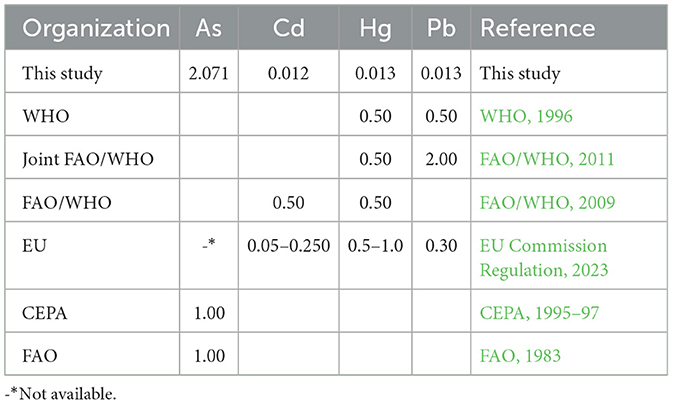
Table 4. Comparison of the average concentrations of toxic metals (expressed in μg/g ww) measured in fish samples from this study with the maximum permissible concentrations (MPC) in fish muscles (μg/g ww) set by various international organizations.
Health risk assessment (consumption safety)
Metal pollution index (MPI)
The Metal Pollution Index (MPI), which compares total metal contents in the muscles of the examined fish or canned fish, was used to assess the degree of metal contamination in the fish or canned fish samples under investigation. As shown in Supplementary File 4, calculated MPI values (based on concentrations in μg/g ww) for the 13 tested fish species ranged from 0.004 to 0.086 (median of 0.021, n = 13). Although the MPI calculated for bakala fish was the third highest among the other fish species, the potential risk associated with consuming this particular fish species is much higher compared to that associated with consuming any of the other studied species, simply because bakala fish is the cheapest among all fish types available at Ramallah markets, making it affordable for most local consumers.
For the canned fish samples, as shown in Supplementary File 5, calculated MPI values (based on concentrations in μg/g ww) ranged from 0.012 to 0.045 (median of 0.027, n = 8). The lowest MPI values were found in the two sardine canned fish samples (Al warda Al hamra and Americana), suggesting that sardine canned fish might be safer than tuna canned fish. Generally speaking, tuna canned fish (regardless of the brand) is very popular in the markets of Ramallah (and other Palestinian cities); it is consumed regularly by most families. Thus, the potential health hazards associated with the consumption of tuna canned are likely greater than those resulting from sardine canned fish consumption.
Estimated daily intake
As mentioned earlier, the average quantity of fish consumed by a 70 kg person per day is 8.22 g in the West Bank and 11.66 g in the Gaza Strip. Therefore, the mean of both averages (i.e., that of the West Bank and that of the Gaza Strip) was calculated (9.94 g per day per person) and used to determine the EDI values using Equation 2. As shown in Table 5, EDI values (expressed in μg/kg bw/day) were first estimated for the most commonly consumed fish species in Ramallah city markets, particularly bakala fish, Danes fish, catfish, and salmon. EDI values for the four analyzed metals were as follows: As 0.0103–0.0782, with the highest value found in bakala fish; Cd 0.0015–0.0033, with the highest value found in catfish; Hg 0.0001–0.0023, with the highest value found in Danes fish; and Pb 0.001–0.0032, with the highest value found in catfish. The EDI values for Cd, Hg, and Pb were all below the provisional tolerable daily intake (PTDI) (FAO/WHO, 1999, 2003, 2011). However, these EDI values should be interpreted with caution. While it is permissible to use any fish or canned fish sample with an EDI value lower than PTDI for edible purposes, this does not imply that no negative health effects may arise from their consumption.
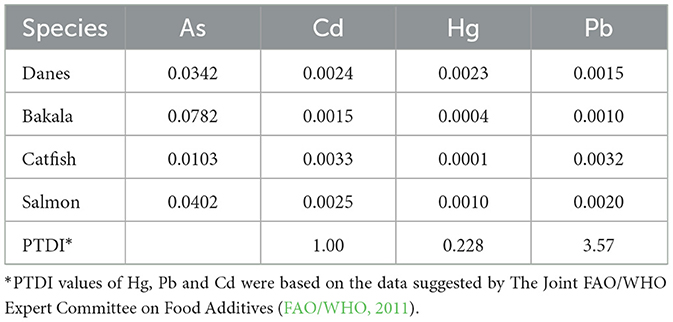
Table 5. Estimated daily intake (EDI, μg/kg bw/day) of toxic metals calculated to asses potential hazard effects in local fish or canned fish consumers at the city of Ramallah, Palestine.
For As, we could not find any PTDI value in the literature, but it is well documented that exposure to potentially toxic metals, such as, arsenic through the ingestion of food or drinks, could result in acute or long-term intoxication (Yüksel et al., 2023). It is important to note that EDI values for As were calculated using total concentrations rather than those of iAs. To achieve a better risk assessment of exposure to As through food, one should differentiate between the levels of organic and inorganic forms present. Thus, without confirming the fraction of iAs in the samples under investigation through a speciation study, the actual risk associated with potential As contamination cannot be assessed.
As shown in Table 6, EDI values were also calculated, but this time using the average concentration of each metal in all studied samples (13 fish and eight canned fish). EDI values ranged from 0.001 for Cd to 0.258 μg/kg bw/day for As, and ascended in the following order: As> Hg> = Pb>Cd. While EDI values of Cd, Hg, and Pb did not increase when the data from all 21 samples were pooled, the As EDI value increased by a factor of six (from an average of 0.041 to 0.258). It should be noted that EDI values of Cd, Hg, and Pb were lower than both recommended daily dietary allowance (RDA) and provisional tolerable daily intake (PTDI) values of the Joint FAO and WHO Expert Committee for Food Additives (FAO/WHO, 1999, 2003, 2011). Once again, because the EDI of As was calculated based on total concentrations, the actual risk associated with potential As contamination could not be assessed without conducting a speciation study. Unfortunately, the necessary instrumentation and infrastructure to do so are not available in the Palestinian territories.
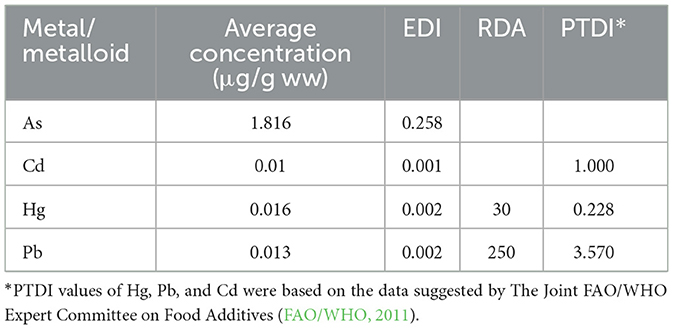
Table 6. Estimated daily intake (EDI, μg/kg bw/day) calculated using the average concentrations of toxic metals in all 21 studied samples, along with the provisional tolerable daily intake (PTDI) and the recommended daily dietary allowance (RDA) as suggested by WHO and joint FAO/WHO (in μg/kg bw/day).
Target hazard quotient (THQ)
The target hazard quotient (THQ) is considered one of the reasonable parameters for the risk assessment of metal contamination and accumulation that might result from consuming contaminated fish (Cao et al., 2014). The threshold limit of THQ is 1.0, as suggested by USEPA (2008, 2010), while a THQ value < 1.0 implies non-significant risk effects (Baki et al., 2018).
The THQ values of As, Cd, Hg, and Pb shown in Supplementary File 6 were calculated for all 21 samples (i.e., fish and canned fish). All of them were below 1.0, except for two values of As (6.85 for morjan fish and 3.46 for sultan ibrahim fish). These values might be lower if much of the As is present in non-toxic organic forms.
Hazard index (HI)
For the risk assessment of multiple metals contamination in fish, the total hazard index (HI) was employed; this approach takes all calculated THQ values into account. While THQ values were calculated for each of the four toxic metals (As, Cd, Hg, and Pb) separately, HI values were calculated for all of them collectively. Any HI value higher than 1.0 suggests that the corresponding fish species or canned fish might not be safe for human consumption. All HI values (Supplementary File 6) were lower than 1.0 for all fish and canned fish samples, except for morjan fish (HI = 6.86) and sultan ibrahim fish (HI = 3.466). This was because their corresponding As THQ values were also high (6.85 and 3.46, respectively). These two high HI values might be lower if much of the As is present in non-toxic organic forms. Therefore, based on these THQ and HI results, no non-carcinogenic health risk effects should be expected.
Carcinogenic risk (CR)
Generally, a CR value above 10−4 is considered unacceptable, whereas a CR ranging from 10−4 to 10−6 is regarded as an acceptable carcinogenic risk, and a CR value below 10−6 is considered negligible (Li et al., 2013). As shown in Supplementary File 7, the CR values of As, Cd, and Pb were below 10−6 for all 21 samples, suggesting no cancer health risk effects should be expected.
Conclusions and recommendations
Concentrations of all four toxic metals measured in this study, except for As, were below the MPC for fish consumption as proposed by the WHO in 1996 and the EU in 2023. As concentrations in three fish species (out of 13 studied species) and in six canned fish samples exceeded the MPCs approved by the FAO in 1983 and by CEPA between 1995 and 1997. Although As levels in these nine samples exceeded the permissible limits, the actual risk to public health might be lower if much of the As is present in non-toxic organic forms. Thus, a follow-up As speciation study is needed in future research.
Careful investigation is needed to determine the source of potential metals contamination in locally raised mesht fish. Further investigations are needed to identify the sources of metal contamination in sardine cans marketed in local markets in Ramallah city. Although the concentrations of most tested toxic metals were lower than the MPCs of WHO, FAO, and EU, this does not mean that all canned fish products available to the public in Ramallah city are safe for human consumption.
The calculated EDI values were lower than the RDA and PTDI levels, suggesting no lifetime health risk. In addition, the THQ, HI, and CR data indicated that the introduction of the toxic metals under investigation through fish and canned fish would not pose a significant health risk.
Future human biomonitoring studies are needed to better correlate environmental exposure with human biological assessment and to link this exposure with acute and chronic adverse effect biomarkers that could represent this complex mixture of exposure in the human food chain.
Data availability statement
The original contributions presented in the study are included in the article/Supplementary material, further inquiries can be directed to the corresponding author.
Author contributions
BG: Data curation, Investigation, Writing – original draft. DQ: Conceptualization, Data curation, Formal analysis, Funding acquisition, Investigation, Methodology, Project administration, Resources, Software, Supervision, Validation, Visualization, Writing – original draft, Writing – review & editing. VM: Data curation, Methodology, Writing – review & editing. LB: Writing – review & editing. HA: Writing – review & editing. RB: Funding acquisition, Writing – review & editing.
Funding
The author(s) declare that no financial support was received for the research and/or publication of this article.
Acknowledgments
We would like to thank Asem Mubarak at the Chemistry Department of Birzeit University and Nour Halayqa at the Laboratories of the Ministry of Health at Ramallah for their technical support and assistance. We would also like to thank our colleagues at the Laboratories of the Biology Department of An-Najah University for freeze-drying the samples. Last, but not least, we are thankful to Angham Baniowda at the Ministry of Agriculture for her help in samples selection.
Conflict of interest
The authors declare that the research was conducted in the absence of any commercial or financial relationships that could be construed as a potential conflict of interest.
Generative AI statement
The author(s) declare that no Gen AI was used in the creation of this manuscript.
Publisher's note
All claims expressed in this article are solely those of the authors and do not necessarily represent those of their affiliated organizations, or those of the publisher, the editors and the reviewers. Any product that may be evaluated in this article, or claim that may be made by its manufacturer, is not guaranteed or endorsed by the publisher.
Supplementary material
The Supplementary Material for this article can be found online at: https://www.frontiersin.org/articles/10.3389/fsufs.2025.1591035/full#supplementary-material
References
Abarshi, M. M., Dantala, E. O., and Mada, S. B. (2017). Bioaccumulation of heavy metals in some tissues of croaker fish from oil spilled rivers of Niger Delta region, Nigeria. Asian Pac. J. Trop. Biomed. 7, 563–568. doi: 10.1016/j.apjtb.2017.05.008
Abdel-Khalek, A. A., Elhaddad, E., Mamdouh, S., and Marie, M.-S. (2016). Assessment of metal pollution around sabal drainage in River Nile and its impacts on bioaccumulation level, metals correlation and human risk hazard using Oreochromis niloticus as a bioindicator. Turkish J. Fish. Aquat. Sci. 16, 227–239. doi: 10.4194/1303-2712-v16_2_02
Al-Qadasy, M., Babaqi, A., Al-Abyadh, M., and Al-Kaf, A. (2018). The effects of lead, cadmium, mercury and arsenic on fish and seawater in Red Sea and the Gulf of Aden at three different locations in Yemen. SF J. Pharm. Anal. Chem. 1:1002.
Alturiqi, A. S., and Albedair, L. A. (2012). Evaluation of some heavy metals in certain fish, meat and meat products in Saudi Arabian markets. Egypt. J. Aquatic Res. 38, 45–49. doi: 10.1016/j.ejar.2012.08.003
Al-Weher, S. (2008). Levels of heavy metal Cd, Cu and Zn in three fish species collected from the Northern Jordan Valley, Jordan. Jordan J. Biol. Sci. 1, 41–46.
AOAC (2019) Official Methods of Analysis of the Association of Official Analytical Chemists: Official Methods of Analysis of AOAC International (21st Edition). (Washington DC: AOAC).
Aytekin, T., Kargin, D., Çogun, H. Y., Temiz, Ö., Varkal, H. S., and Kargin, F. (2019). Accumulation and health risk assessment of heavy metals in tissues of the shrimp and fish species from the Yumurtalik coast of Iskenderun Gulf, Türkiye. Heliyon 5:e02131. doi: 10.1016/j.heliyon.2019.e02131
Baki, M. A., Hossain, M. M., Akter, J., Quraishi, S. B., Shojib, M. F. H., Ullah, A. A., et al. (2018). Concentration of heavy metals in seafood (fishes, shrimp, lobster and crabs) and human health assessment in Saint Martin Island, Bangladesh. Ecotoxicol. Environ. Saf. 159, 153–163. doi: 10.1016/j.ecoenv.2018.04.035
Barakat, M. (2011). New trends in removing heavy metals from industrial wastewater. Arab. J. Chemist. 4, 361–377. doi: 10.1016/j.arabjc.2010.07.019
Belivermiş, M., Kiliç, Ö., and Çotuk, Y. (2016). Assessment of metal concentrations in indigenous and caged mussels (Mytilus galloprovincialis) on entire Turkish coastline. Chemosphere 144, 1980–1987. doi: 10.1016/j.chemosphere.2015.10.098
Bogdanović, T., Ujević, I., Sedak, M., Listeš, E., Šimat, V., Petričević, S., et al. (2014). As, Cd, Hg and Pb in four edible shellfish species from breeding and harvesting areas along the eastern Adriatic Coast, Croatia. Food Chem. 146, 197–203. doi: 10.1016/j.foodchem.2013.09.045
Cao, S., Duan, X., Zhao, X., Ma, J., Dong, T., Huang, N., et al. (2014). Health risks from the exposure of children to As, Se, Pb and other heavy metals near the largest coking plant in China. Sci. Total Environ. 472, 1001–1009. doi: 10.1016/j.scitotenv.2013.11.124
CEPA (1995–97). California Environmental Protection Agency. State Water Resources Control Board. Available online at: https://calepa.ca.gov/ (accessed October 18, 2022).
Dadar, M., Adel, M., Nasrollahzadeh Saravi, H., and Fakhri, Y. (2017). Trace element concentration and its risk assessment in common kilka (Clupeonella cultriventris caspia Bordin, 1904) from southern basin of Caspian Sea. Toxin Rev. 36, 222–227. doi: 10.1080/15569543.2016.1274762
Duruibe, J.O., Ogwuegbu, M., and Egwurugwu, J. (2007). Heavy metal pollution and human biotoxic effects. Int. J. Phys. Sci. 2, 112–118. Available online at: http://www.academicjournals.org/IJPS
Edelstein, M., and Ben-Hur, M. (2018). Heavy metals and metalloids: Sources, risks and strategies to reduce their accumulation in horticultural crops. Sci. Hortic. 234. doi: 10.1016/j.scienta.2017.12.039
Elnabris, K.J., Muzyed, S. K., and El-Ashgar, N. M. (2013). Heavy metal concentrations in some commercially important fishes and their contribution to heavy metals exposure in Palestinian people of Gaza Strip (Palestine). J. Assoc. Arab Universit. Basic Appl. Sci. 13, 44–51. doi: 10.1016/j.jaubas.2012.06.001
EU Commission Regulation (2023). Commission Regulation (EU) 2023/915 of 25 April 2023 on maximum levels for certain contaminants in food and repealing Regulation (EC) No 1881/2006. Off. J. Eur. Union 119, 103–157.
Fakhri, Y., Mohseni-Bandpei, A., Oliveri Conti, G., Keramati, H., Zandsalimi, Y., Amanidaz, N., et al. (2017). Health risk assessment induced by chloroform content of the drinking water in Iran: systematic review. Toxin Rev. 36, 342–351. doi: 10.1080/15569543.2017.1370601
FAO (1983) Compilation of Legal Limits for Hazardous Substances in Fish and Fishery Product. London: FAO.
FAO/WHO (1999). Summary and Conclusions of the Fifty-third meeting of the Joint FAO/WHO Expert Committee on Food Additives (JECFA). Rome: FAO/WHO.
FAO/WHO (2003). Summary and Conclusions of the 61st meeting of the Joint FAO/WHO Expert Committee on Food Additives (JECFA). Rome: FAO/WHO
FAO/WHO (2009). Evaluation of Certain Food Additives: Sixty-Ninth Report of the Joint FAO/WHO Expert Committee on Food Additives. Geneva: World Health Organization.
FAO/WHO (2011). Evaluation of Certain Contaminants in Food: Seventy-Second [72nd] Report of the Joint FAO/WHO Expert Committee on Food Additives. Geneva: World Health Organization.
Hilal, A., and Ismail, N. S. (2008). Heavy metals in eleven common species of fish from the Gulf of Aqaba, Red Sea. Jordan J. Biol. Sci. 1, 13–18.
Hosseini, S. V., Sobhanardakani, S., Miandare, H.K., Harsij, M., and Regenstein, J. M. (2015). Determination of toxic (Pb, Cd) and essential (Zn, Mn) metals in canned tuna fish produced in Iran. J. Environm. Health Sci. Eng. 13, 1–6. doi: 10.1186/s40201-015-0215-x
Hoy, K. S., Davydiuk, T., Chen, X., Lau, C., Schofield, J. R., Lu, X., et al. (2023). Arsenic speciation in freshwater fish: challenges and research needs. Food Qual. Safety 7:fyad032. doi: 10.1093/fqsafe/fyad032
Islam, M., Bang, S., Kim, K.-W., Ahmed, M., and Jannat, M. (2010). Heavy metals in frozen and canned marine fish of Korea. J. Sci. Res. 2, 549–549. doi: 10.3329/jsr.v2i3.4667
Kalantzi, I., Mylona, K., Sofoulaki, K., Tsapakis, M., and Pergantis, S. A. (2017). Arsenic speciation in fish from Greek coastal areas. J. Environm. Sci. 56, 300–312. doi: 10.1016/j.jes.2017.03.033
Kalantzi, I., Pergantis, S., Black, K., Shimmield, T., Papageorgiou, N., Tsapakis, M., et al. (2016). Metals in tissues of seabass and seabream reared in sites with oxic and anoxic substrata and risk assessment for consumers. Food Chem. 194, 659–670. doi: 10.1016/j.foodchem.2015.08.072
Keshavarzi, B., Hassanaghaei, M., Moore, F., Mehr, M. R., Soltanian, S., Lahijanzadeh, A. R., et al. (2018). Heavy metal contamination and health risk assessment in three commercial fish species in the Persian Gulf. Mar. Pollut. Bull. 129, 245–252. doi: 10.1016/j.marpolbul.2018.02.032
Korfali, S. I., and Abou Hamdan, W. (2013). Essentail and toxic metals in lebanese marketed canned food: impact of metal cans. J. Food Res. 2, 19–30. doi: 10.5539/jfr.v2n1p19
Kowalska, G., Pankiewicz, U., and Kowalski, R. (2020). Determination of the level of selected elements in canned meat and fish and risk assessment for consumer health. J. Anal. Methods Chem. 2020, 2148794–2148806. doi: 10.1155/2020/2148794
Krishnakumar, P. K., Qurban, M. A., Stiboller, M., Nachman, K. E., Joydas, T. V., Manikandan, K. P., et al. (2016). Arsenic and arsenic species in shellfish and finfish from the western Arabian Gulf and consumer health risk assessment. Sci. Total Environm. 566, 1235–1244. doi: 10.1016/j.scitotenv.2016.05.180
Li, J., Huang, Z., Hu, Y., and Yang, H. (2013). Potential risk assessment of heavy metals by consuming shellfish collected from Xiamen, China. Environ. Sci. Pollut. Res. Int. 20, 2937–2947. doi: 10.1007/s11356-012-1207-3
Mahalakshmi, M., Balakrishnan, S., Indira, K., and Srinivasan, M. (2012). Characteristic levels of heavy metals in canned tuna fish. J. Toxicol. Environ. Health Sci. 4, 43–45. doi: 10.5897/JTEHS11.079
Mataba, G. R., Verhaert, V., Blust, R., and Bervoets, L. (2016). Distribution of trace elements in the aquatic ecosystem of the Thigithe river and the fish Labeo victorianus in Tanzania and possible risks for human consumption. Sci. Total Environm.547, 48–59. doi: 10.1016/j.scitotenv.2015.12.123
Microsoft Office (2019) Microsoft Corporation [Computer software]. Retrieved from: https://officecdn.microsoft.com/db/492350F6-3A01-4F97-B9C0-C7C6DDF67D60/media/en-US/ProPlus2019Retail.img
Neff, M. R., Bhavsar, S. P., Ni, F. J., Carpenter, D. O., Drouillard, K., Fisk, A. T., et al. (2014). Risk-benefit of consuming Lake Erie fish. Environ. Res. 134, 57–65. doi: 10.1016/j.envres.2014.05.025
Obeid, P. J., El-Khoury, B., Burger, J., Aouad, S., Younis, M., Aoun, A., et al. (2011). Determination and assessment of total mercury levels in local, frozen and canned fish in Lebanon. J. Environm. Sci. 23, 1564–1569. doi: 10.1016/S1001-0742(10)60546-3
Orajiaka-Uchegbu, C., Patrick-Iwuanyanwu, K., Ogbo, A., and Egbuna, C. (2020). Bioaccumulation of heavy metals and potential health risk through consumption of seafoods from selected creeks in Rivers State, Nigeria. Egypt. J. Aquatic Biol. Fisher. 24, 1033–1053. doi: 10.21608/ejabf.2020.133858
Popovic, A. R., Djinovic-Stojanovic, J. M., Djordjevic, D. S., Relic, D. J., Vranic, D. V., Milijasevic, M. P., et al. (2018). Levels of toxic elements in canned fish from the Serbian markets and their health risks assessment. J. Food Composit. Analy. 67, 70–76. doi: 10.1016/j.jfca.2018.01.003
Qadah, D., Bervoets, L., and Blust, R. (2023). Effect of incubation time of three single extraction procedures on trace element extraction from sediment and soil. Environ. Monit. Assess. 195:342. doi: 10.1007/s10661-022-10890-3
Rodriguez-Levy, I. E., Van Damme, P. A., Carvajal-Vallejos, F. M., and Bervoets, L. (2022). Trace element accumulation in different edible fish species from the Bolivian Amazon and the risk for human consumption. Heliyon 8:e11649. doi: 10.1016/j.heliyon.2022.e11649
Sarker, M. J., Polash, A. U., Islam, M. A., Rima, N. N., and Farhana, T. (2020). Heavy metals concentration in native edible fish at upper Meghna River and its associated tributaries in Bangladesh: a prospective human health concern. SN Appl. Sci. 2, 1667–1676. doi: 10.1007/s42452-020-03445-z
Tanaskovski, B., Jović, M., Mandić, M., Pezo, L., Degetto, S., and Stanković, S. (2016). Elemental analysis of mussels and possible health risks arising from their consumption as a food: the case of Boka Kotorska Bay, Adriatic Sea. Ecotoxicol. Environ. Saf. 130, 65–73. doi: 10.1016/j.ecoenv.2016.04.007
Traina, A., Bono, G., Bonsignore, M., Falco, F., Giuga, M., Quinci, E. M., et al. (2019). Heavy metals concentrations in some commercially key species from Sicilian coasts (Mediterranean Sea): Potential human health risk estimation. Ecotoxicol. Environ. Saf. 168, 466–478. doi: 10.1016/j.ecoenv.2018.10.056
Tuzen, M., and Soylak, M. (2007). Determination of trace metals in canned fish marketed in Türkiye. Food Chem. 101, 1378–1382. doi: 10.1016/j.foodchem.2006.03.044
USEPA (2000a) Guideline for Assessing Chemical Contaminant Data for use in Fish Advisories vol. I: Fish Sampling and Analysis (3 edn). Washington, DC: Office of Water. U.S. Environmental Protection Agency, (Document No. EPA 823-B-00007. November 2000).
USEPA (2000b) Methodology for Deriving Ambient Water Quality Criteria for the Protection of Human Health (EPA-822-B-00-004). Washington DC: USEPA.
USEPA (2008) Integrated Risk Information System. Washington, DC: United States Environmental Protection Agency. Available online at: https://www.epa.gov/iris (accessed 5 May, 2020).
USEPA (2010) Risk-Based Concentration Table. Available online at: http://www.epa.gov/reg3hwmd/risk/human/index.htm (accessed October 18 2022).
Usero, J., Morillo, J., and Gracia, I. (2005). Heavy metal concentrations in molluscs from the Atlantic coast of southern Spain. Chemosphere 59, 1175–1181. doi: 10.1016/j.chemosphere.2004.11.089
Ustaoglu, F., Kabir, M. H., Kormoker, T., Ismail, Z., Islam, M. S., Taş, B., et al. (2024). Appraisal of macro elements and trace metals in the edible fish from the Black Sea connecting coastal river, Türkiye: A preliminary study for health risk assessment. Region. Stud. Marine Sci. 71:103406. doi: 10.1016/j.rsma.2024.103406
Ustaoglu, F., and Yüksel, B. (2024). Bioaccumulation of metals in muscle tissues of economically important fish species from black sea lagoon lakes in Türkiye: consumer health risk and nutritional value assessment. Microchem. J. 205:111337. doi: 10.1016/j.microc.2024.111337
Varol, M., Kaya, G. K., and Alp, A. (2017). Heavy metal and arsenic concentrations in rainbow trout (Oncorhynchus mykiss) farmed in a dam reservoir on the Firat (Euphrates) River: Risk-based consumption advisories. Sci. Total Environm. 599, 1288–1296. doi: 10.1016/j.scitotenv.2017.05.052
Vu, C. T., Lin, C., Yeh, G., and Villanueva, M. C. (2017). Bioaccumulation and potential sources of heavy metal contamination in fish species in Taiwan: assessment and possible human health implications. Environ Sci Pollut Res 24, 19422–19434. doi: 10.1007/s11356-017-9590-4
Wang, X., Sato, T., Xing, B., and Tao, S. (2005). Health risks of heavy metals to the general public in Tianjin, China via consumption of vegetables and fish. Sci. Total Environm. 350, 28–37. doi: 10.1016/j.scitotenv.2004.09.044
Wei, Y., Zhang, J., Zhang, D., Tu, T., and Luo, L. (2014). Metal concentrations in various fish organs of different fish species from Poyang Lake, China. Ecotoxicol. Environ. Saf. 104, 182–188. doi: 10.1016/j.ecoenv.2014.03.001
WHO (1996). Health criteria other supporting information, Guidelines for Drinking Water Quality. Geneva: WHO.
Yasmeen, K., Mirza, M. A., Khan, N. A., Kausar, N., Rehman, A.-U., and Hanif, M. (2016). Trace metals health risk appraisal in fish species of Arabian Sea. Springerplus 5, 1–7. doi: 10.1186/s40064-016-2436-6
Yi, Y., Yang, Z., and Zhang, S. (2011). Ecological risk assessment of heavy metals in sediment and human health risk assessment of heavy metals in fishes in the middle and lower reaches of the Yangtze River basin. Environm. Pollut. 159, 2575–2585. doi: 10.1016/j.envpol.2011.06.011
Younis, E. M., Abdel-Warith, A.-W. A., Al-Asgah, N. A., Elthebite, S. A., and Rahman, M. M. (2021). Nutritional value and bioaccumulation of heavy metals in muscle tissues of five commercially important marine fish species from the Red Sea. Saudi J. Biol. Sci. 28, 1860–1866. doi: 10.1016/j.sjbs.2020.12.038
Yüksel, B., Ustaoglu, F., Yazman, M. M., Seker, M. E., and Öncü, T. (2023). Exposure to potentially toxic elements through ingestion of canned non-alcoholic drinks sold in Istanbul, Türkiye: a health risk assessment study. J. Food Composit. Analy. 121:105361. doi: 10.1016/j.jfca.2023.105361
Zhang, W., Xu, Y., Tahir, H. E., Zou, X., and Wang, P. (2017). Rapid and wide-range determination of Cd(II), Pb(II), Cu(II) and Hg(II) in fish tissues using light addressable potentiometric sensor. Food Chem. 221, 541–547. doi: 10.1016/j.foodchem.2016.11.141
Zhelyazkov, G., Yankovska-Stefanova, T., Mineva, E., Stratev, D., Vashin, I., Dospatliev, L., et al. (2018). Risk assessment of some heavy metals in mussels (Mytilus galloprovincialis) and veined rapa whelks (Rapana venosa) for human health. Mar. Pollut. Bull. 128, 197–201. doi: 10.1016/j.marpolbul.2018.01.024
Zhong, W., Zhang, Y., Wu, Z., Yang, R., Chen, X., Yang, J., et al. (2018). Health risk assessment of heavy metals in freshwater fish in the central and eastern North China. Ecotoxicol. Environ. Saf. 157, 343–349. doi: 10.1016/j.ecoenv.2018.03.048
Keywords: toxic, metals, microwave assisted digestion, environmental exposure, risk assessment
Citation: Gazzawi B, Qadah D, Mubiana VK, Bervoets L, Al Zabadi H and Blust R (2025) Exposure assessment of As, Cd, Hg, and Pb in fish and canned fish collected from local markets at Ramallah city using high-resolution inductively coupled plasma mass spectrometry. Front. Sustain. Food Syst. 9:1591035. doi: 10.3389/fsufs.2025.1591035
Received: 11 March 2025; Accepted: 22 April 2025;
Published: 21 May 2025.
Edited by:
Roberto Anedda, Porto Conte Ricerche, Parco Scientifico e Tecnologico della Sardegna, ItalyReviewed by:
Levent Bat, Sinop University, TürkiyeOana Cadar, INCDO INOE 2000, Research Institute for Analytical Instrumentation, Romania
Bayram Yuksel, Giresun University, Türkiye
Copyright © 2025 Gazzawi, Qadah, Mubiana, Bervoets, Al Zabadi and Blust. This is an open-access article distributed under the terms of the Creative Commons Attribution License (CC BY). The use, distribution or reproduction in other forums is permitted, provided the original author(s) and the copyright owner(s) are credited and that the original publication in this journal is cited, in accordance with accepted academic practice. No use, distribution or reproduction is permitted which does not comply with these terms.
*Correspondence: Diab Qadah, ZHFhZGFoQGJpcnplaXQuZWR1
†ORCID: Diab Qadah orcid.org/0000-0002-6498-6433