- 1Laboratorio de Anatomía Comparada y Evolución de los Vertebrados, Museo Argentino de Ciencias Naturales Bernardino Rivadavia, Buenos Aires, Argentina
- 2Fundación de Historia Natural Félix de Azara, Universidad Maimónides, Buenos Aires, Argentina
- 3Consejo Nacional de Investigaciones Científicas y Técnicas, Buenos Aires, Argentina
Recent years witnessed the discovery of a great diversity of early birds as well as closely related non-avian theropods, which modified previous conceptions about the origin of birds and their flight. We here present a review of the taxonomic composition and main anatomical characteristics of those theropod families closely related with early birds, with the aim of analyzing and discussing the main competing hypotheses pertaining to avian origins. We reject the postulated troodontid affinities of anchiornithines, and the dromaeosaurid affinities of microraptorians and unenlagiids, and instead place these groups as successive sister taxa to Avialae. Aiming to evaluate previous phylogenetic analyses, we recoded unenlagiids in the traditional TWiG data matrix, which resulted in a large polytomy at the base of Pennaraptora. This indicates that the TWiG phylogenetic scheme needs a deep revision. Regarding character evolution, we found that: (1) the presence of an ossified sternum goes hand in hand with that of ossified uncinate processes; (2) the presence of foldable forelimbs in basal archosaurs indicates widespread distribution of this trait among reptiles, contradicting previous proposals that forelimb folding driven by propatagial and associated tendons was exclusive to the avian lineage; (3) in basal paravians and avialans (e.g., Archaeopteryx, Anchiornis) the wings are relatively large and wide, with relatively short rectricial feathers, a rounded alar contour, and a convex leading margin. These taxa exhibit restricted forelimb folding capability with respect to more derived birds, their hands being preserved at angles of flexion (with respect to the radius/ulna) of no less than 90°. In more derived birds, however, the rectrices are notably elongate and the angle between the hand and forearm is much less than 90°, indicating not only increased forelimb folding capability but also an increased variety of wingbeat movements during flight. Because of the strong similarities in pectoral girdle configuration between ratites and basal avialans and paravians, it is possible to infer that the main forelimb movements were similar in all these taxa, lacking the complex dorsoventral wing excursion characteristic of living neognathans.
Introduction
The origin and early evolution of paravian theropods is one of the most hotly debated topics in vertebrate paleontology. The hypothesis that birds are nested within theropod dinosaurs is accepted by most paleontologists.
Over the past decades numerous remarkable discoveries, mainly from the Early Cretaceous of China (Figure 1), as well as innovative studies of living bird behavior, have enriched and deeply modified our understanding of early paravian evolution and flight origins [see integrative summaries by Xu et al. (2014), Brusatte et al. (2015), and Cau (2018)]. Such novel discoveries are important and unprecedented in that they include fossils with preserved soft tissues, mainly a diversity of feather types across different non-avian dinosaurs and basal birds. A view that the evolutionary transition to birds was considerably more complex than previously thought is now emerging (Brusatte et al., 2015; Cau, 2018). Recent paleontological studies of these fossils have considered temporal, morphological, and functional issues, as well as neontological evidence coming from embryology, feather development, aerodynamic studies, endothermic physiology, the reproductive biology of living taxa, growth rates, and analysis of respiratory systems.
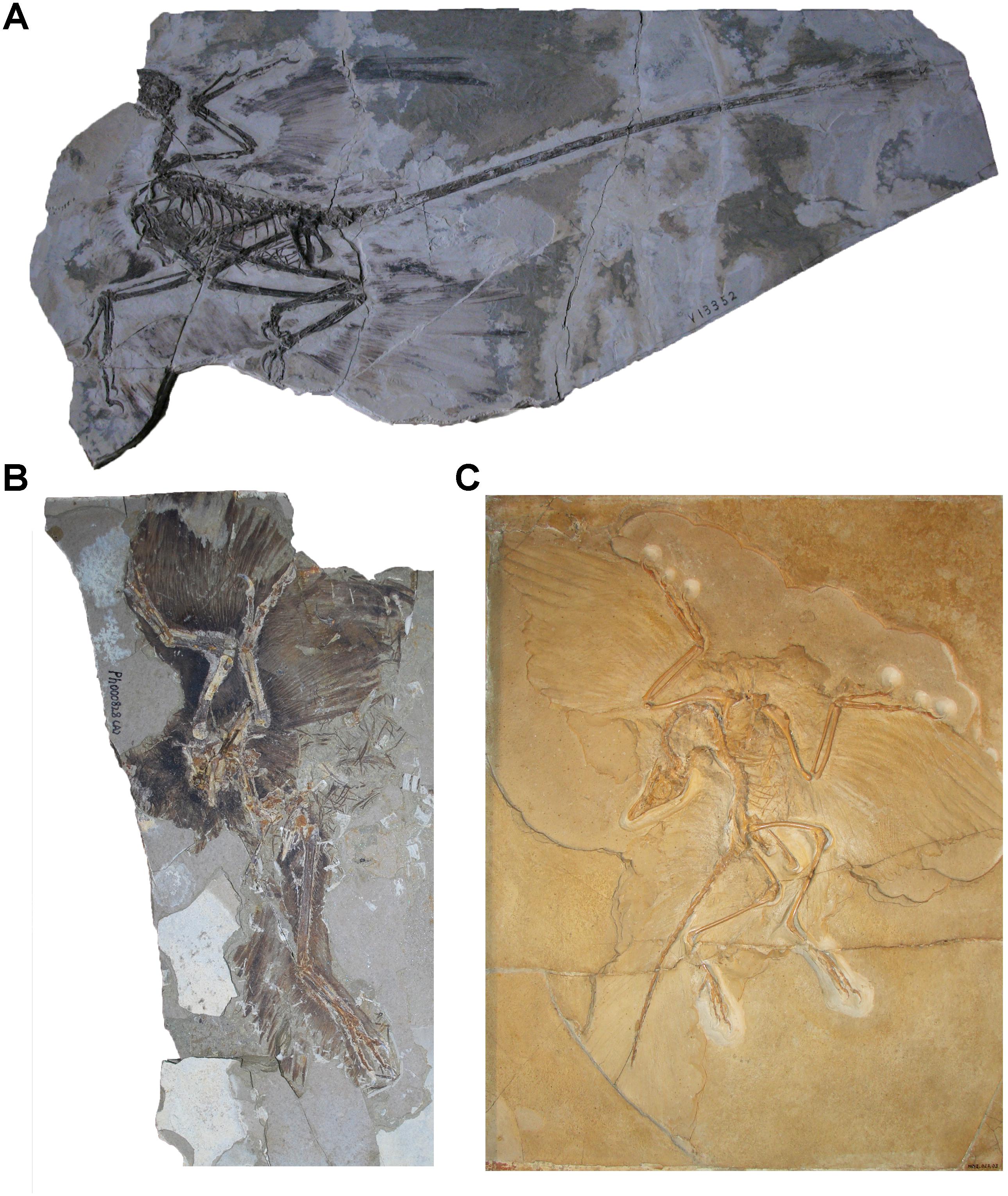
Figure 1. (A) Microraptor zhaoianus (IVPP V123352); (B) Anchiornis huxleyi (BMNHC PH 828); (C), Archaeopteryx lithographica (MB.AV.100). Not to scale.
Available information shows that the acquisition of powered flight as it is known in living neornithines was preceded in the course of pennaraptoran/paravian evolution by a complex sequence of anatomical and functional innovations, and that many characters previously thought to be exclusively avian are widespread among non-avian theropods even though their prevalence is largely obscured by the mosaic distribution of anatomical traits across the theropod phylogenetic tree (Xu et al., 2017). For this reason, a refined and robust phylogeny is imperative in order to elucidate the sequence of evolutionary stages that resulted in the acquisition of major avian traits. Unfortunately, the paleontological community is far from reaching such a phylogenetic consensus, as evidenced by the diverse hypotheses on paravian phylogeny that have been published to date (e.g., Agnolin and Novas, 2013; Godefroit et al., 2013b; Cau et al., 2017; Gianechini et al., 2017; Hu et al., 2018; see Figure 2).
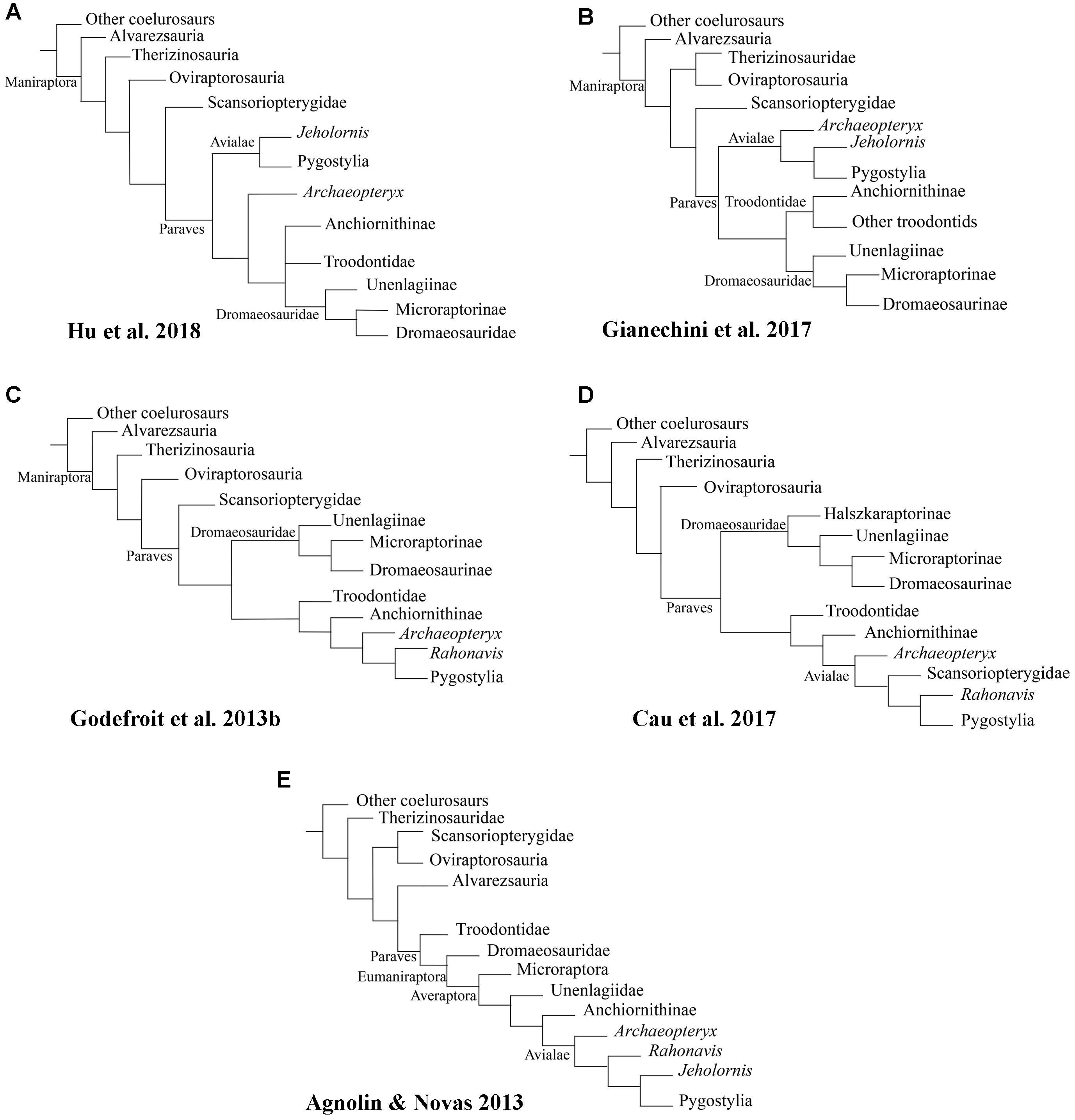
Figure 2. Simplified results of recent paravian phylogenetical analyses: (A) Gianechini et al., (2017); (B) Hu et al., (2018); (C) Godefroit et al., (2013b); (D) Cau et al., (2017); (E) Agnolin and Novas, (2013).
We offer below a summary review of the main competing hypotheses, including discussion of the following special topics. Are anchiornithids basal troodontids? Are scansoriopterygids basal birds, or basal pennaraptorans? Are unenlagiids and microraptorians dromaeosaurids? Two interconnected questions that involve the relationships of all these clades are whether Deinonychosauria is a monophyletic clade, and which paravian clades are most closely related to Avialae. Here we point out inconsistencies in previous efforts to address these topics, and offer our own views about the phylogenetic relationships of pennaraptorans and the evolutionary processes that affected the early history of this group.
Setting Up the Phylogenetic Problem
The clade Deinonychosauria was erected in 1969 by Colbert and Russell to include the small predatory theropods Dromaeosaurus, Velociraptor, and Deinonychus. Later, Gauthier (1986) employed the name Deinonychosauria for a monophyletic clade comprising Troodontidae plus Dromaeosauridae, with the presence of a raptorial ungual on pedal digit II as the most distinctive diagnostic feature. Subsequently, Sereno (1997, 1999) re-defined Deinonychosauria as the clade including “Troodon, Velociraptor, their most common ancestor and all descendants,” interpreting this clade as the sister group of Avialae (i.e., the theropod group that includes Archaeopteryx lithographica and Passer domesticus, their most common ancestor and all of its descendants; Gauthier, 1986). The monophyly of Deinonychosauria was widely accepted by most authors during the 1990’s and 2000’s (e.g., Sereno, 1997, 1999; Norell et al., 2001, 2006; Xu et al., 2002, 2011; Makovicky et al., 2003, 2005; Xu and Norell, 2004; Senter, 2007; Turner et al., 2007a,b, 2012; Gianechini et al., 2017; Lefèvre et al., 2017; Figure 2). Nevertheless, some authors questioned its monophyly and relationship with Avialae (Osmólska, 1981; Barsbold, 1983; Currie, 1987, 1995; Osmólska and Barsbold, 1990; Currie and Zhao, 1993; Holtz, 1994, 1998, 2001; Novas and Puerta, 1997; Padian et al., 1997).
Norell and co-workers (identified as “the Theropod Working Group” or “TWiG”; see Norell et al., 2001, 2006; Makovicky et al., 2003, 2005; Turner et al., 2012) defended the concept of a monophyletic Deinonychosauria including Troodontidae and Dromaeosauridae sensu lato, and therefore containing a large number of morphologically disparate taxa (see also Senter, 2007; Turner et al., 2007a,b; Gianechini et al., 2011b, 2017).
Recent discoveries, mainly from China and South America, resulted in new phylogenetic hypotheses that challenged the traditional phylogenetic scheme (e.g., Xu et al., 2009, 2011; Agnolin and Novas, 2011, 2013; Godefroit et al., 2013a,b; Foth et al., 2014; Cau et al., 2017; Foth and Rauhut, 2017; Gianechini et al., 2018; Figure 2). These discoveries led to the recognition of novel paravian clades that further complicated our understanding of paravian evolution, leading some authors to propose that Deinonychosauria was a paraphyletic or even polyphyletic assemblage (Agnolin and Novas, 2011, 2013; Cau et al., 2017; Foth and Rauhut, 2017).
Novas and Puerta (1997) described the Patagonian paravian Unenlagia comahuensis and recovered it as the sister taxon of Avialae, a hypothesis recently supported by Agnolin and Novas (2011, 2013). Agnolin and Novas (2013) coined the name Averaptora for a clade grouping together a number of basal paravians but excluding Eudromaeosauria and Troodontidae. In this scheme Troodontidae, Dromaeosauridae, Microraptoria, Unenlagiidae, and Anchiornis are interpreted as successively closer outgroups to Avialae.
In a ground-breaking proposal, Xu et al. (2011) hypothesized that Archaeopteryx was more nearly related to deinonychosaurians than to birds and that deinonychosaurs become secondarily flightless, a hypothesis previously envisaged by Paul (2002). This hypothesis was supported by a variety of more recent analyses (Godefroit et al., 2013a; Xu et al., 2015; Hu et al., 2018). In this phylogenetic scheme, Paraves includes the clades Avialae (including Scansoriopterygidae) and Archaeopterygidae + Deinonychosauria.
Godefroit et al. (2013b) proposed that Paraves consisted of Scansoriopterygidae plus Eumaniraptora (Figure 2). In this hypothesis Deinonychosauria was regarded as paraphyletic, with Troodontidae (including Anchiornis) as the sister group to Avialae (see also Lefèvre et al., 2017). Foth et al. (2014), Cau et al. (2017), Foth and Rauhut (2017), Cau (2018), and Gianechini et al. (2018) proposed similar phylogenetic hypotheses.
More recently, Cau et al. (2017; Figure 2) recognized the basal dromaeosaurid clade Halszkaraptorinae, represented by the species Halszkaraptor escuilliei, Mahakala omnogovae, and Hulsanpes perlei (Osmólska, 1982; Turner et al., 2007b; Cau et al., 2017; Cau and Madzia, 2018). Halszkaraptor possesses a unique mosaic of features, indicating a probably amphibious mode of life (Cau et al., 2017). Further, the synapomorphies of Dromaeosauridae seen in Halszkaraptor were questioned previously by other authors (Agnolin and Novas, 2011, 2013; Xu et al., 2011), and are more widely distributed than previously thought. Halszkaraptor and its kin demonstrate the great morphological disparity among purported dromaeosaurids. In concert with the inconclusive positions of other clades previously regarded as dromaeosaurids (i.e., Microraptoria, Unenlagiidae), they weaken the interpretation that halszkaraptorines belong to Dromaeosauridae.
In sum, the various hypotheses mainly differ with regard to the monophyly or paraphyly of Deinonychosauria and the phylogenetic position of Anchiornithidae within Coelurosauria. Paravian phylogeny is far from being well understood, and a consensus among authors is still wanting. In the following section we present a brief overview of the taxonomic composition of selected paravian clades whose affiliations are problematic or controversial.
Taxonomy of Basal Paravian Clades
We will discuss first the taxonomic composition and definition of each of the main paravian groups, namely Troodontidae, Microraptoria, Unenlagiidae, Anchiornithidae, Scansoriopterygidae.
Troodontidae
The clade Troodontidae includes several genera and species of gracile-limbed theropods, exclusively from North America and Asia (Makovicky and Norell, 2004; Tsuihiji et al., 2014; Pei et al., 2017b; Shen et al., 2017; van der Reest and Currie, 2017; Xu et al., 2017). Isolated troodontid teeth have been reported from Europe (see Vullo et al., 2007) and India (Goswami et al., 2013). However, the assignment of isolated teeth to specific theropod clades is difficult. For example, the single tooth described by Goswami et al. (2013) lacks the globose crown and hypertrophied denticles diagnostic of derived troodontids (see Averianov and Sues, 2007). We consider that the presence of troodontids in India is far from being certain.
Typical troodontids such as Troodon, Gobivenator, Sinornithosaurus, and Saurornithoides share apomorphic features including braincase with expanded and deep subotic recess, large lateral depression on the prootic, strong otosphenoidal crest, long anterior lacrimal process extending rostrally beyond the anterior border of the antorbital fenestra, row of foramina along the lateral edge of the nasal, triangular dentary in side view, relatively large number of heterodont teeth (25 dentary teeth), and transversely broad and flat pubic apron (Currie, 1985; Makovicky and Norell, 2004; Xu et al., 2017; Figure 3).
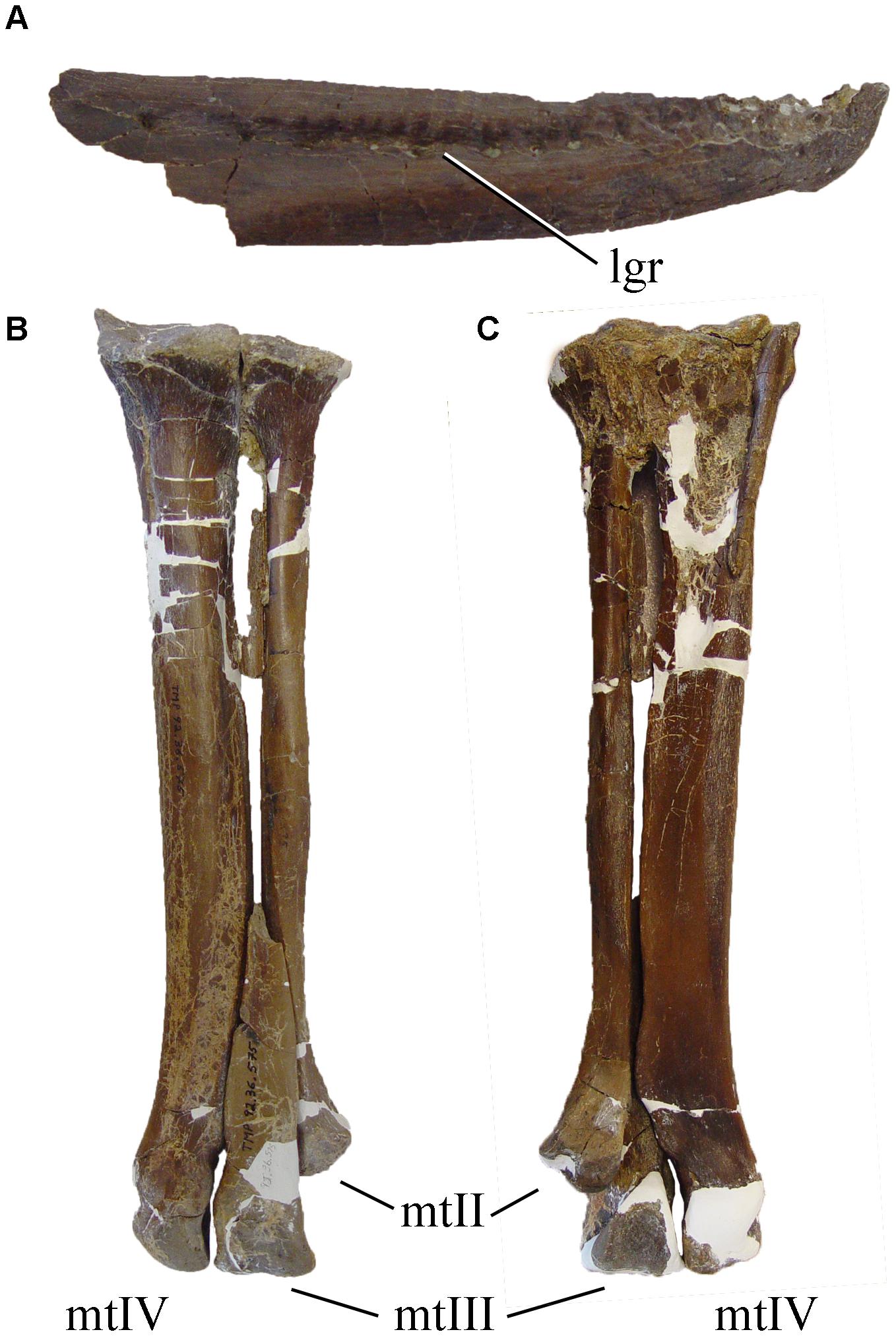
Figure 3. Selected troodontid traits: (A) right dentary (CMN 12340) of Latenivenatrix mcmasterae in lateral view, showing the longitudinal dentary groove; (B,C) left metatarsus of Latenivenatrix mcmasterae (CMN 12340) in (B) dorsal, and (C) lateral views, showing the size difference between metatarsal IV and metatarsal II. Not to scale.
Several authors have agreed that Anchiornis huxleyi, Jinfengopteryx elegans, Mei long, and Sinovenator changii (Xu et al., 2002; Ji et al., 2005; Hu et al., 2009) constitute basal troodontids (see Anchiornithinae, below). However, most troodontid apomorphic traits are absent in Jinfengopteryx, Mei, and Sinovenator, which has prompted some authors to doubt the troodontid affinities of these genera (Senter et al., 2004; Agnolin and Novas, 2013). Further, these basal forms share with birds (and not with derived troodontids) a very small ilium (iliac length less than two and half times total femoral length), a well-developed supratrochanteric process on the ilium, a laterally prominent iliac antitrochanter that is posterodorsally placed, and teeth with reduced serrations (Shen et al., 2017). These features were previously thought to be apomorphies of the clade formed by Troodontidae + Avialae but are, in fact, absent in unambiguous troodontids (see details in Cau, 2018). Furthermore, recent detailed analysis of the Sinovenator skull (Yin et al., 2018) resulted in the recognition of important autapomorphic features that are absent in derived troodontids, and of several traits that are “intermediate” between those seen in derived troodontids and non-troodontid paravians, but shared with basal birds such as Archaeopteryx (e.g., reduced subotic recess, absence of lateral depression, poorly developed otosphenoidal crest, deep basisphenoidal recess, posterior contact between pterygoids, anterior and posterior palatine depressions separated by a transverse crest; Yin et al., 2018). Shen et al. (2017) coined the name Sinovenatorinae for a clade including these sharply distinct taxa (i.e., Jinfengopteryx, Mei, Daliansaurus, and Sinovenator). Sinovenatorines apomorphically share a very small premaxilla, unfused zygapophyses on the sacral vertebrae, and contact between premaxilla and nasal, excluding the maxilla from the nasal fossa (Shen et al., 2017).
Because of the small number of features shared between Sinovenatorinae and Trodontidae, it seems probable that sinovenatorines do not belong to Troodontidae, but instead form a separate clade of derived paravians, in agreement with the hypothesis originally proposed by Senter et al. (2004).
Microraptoria
Microraptorians compose a monophyletic assemblage of small to medium-sized basal paravians, mostly documented from Early Cretaceous beds in Liaoning Province, NE China (Senter et al., 2004; Xu and Wang, 2004; Makovicky et al., 2005; Senter, 2007; Turner et al., 2007a; Zheng X. et al., 2009, Lü and Brusatte, 2015). Members of Microraptoria are Sinornithosaurus millenii (Xu et al., 1999b; including its junior synonym S. haoiana), Microraptor zhaoianus (Xu et al., 2000; including several previously described species; see Turner et al., 2007a, 2012), Graciliraptor lujiatunensis (Xu and Wang, 2004), Hesperonychus elizabethae (Longrich and Currie, 2009), Changyuraptor yangi (Han et al., 2014), Zhongjianosaurus yangi Xu and Qin (2017), and probably Tianyuraptor ostromi (Zheng X. et al., 2009) and Zhenyuanlong suni (Lü and Brusatte, 2015). Longrich and Currie (2009) recovered the Late Cretaceous Mongolian Shanag ashile as a microraptorian, but this result was criticized by Agnolin and Novas (2013). In fact, the phylogenetic position of Shanag is far from certain and this taxon is here regarded as a paravian of uncertain phylogenetic relationships.
Microraptorians have been considered as a monophyletic group by several authors (e.g., Xu and Wang, 2004; Makovicky et al., 2005; Norell et al., 2006; Senter, 2007; Longrich and Currie, 2009; Zheng X. et al., 2009; Zanno, 2010; Turner et al., 2012; Han et al., 2014; Pittman et al., 2015), but as a paraphyletic one by others (e.g., Hwang et al., 2002; Novas et al., 2009; Choiniere et al., 2010; Lü and Brusatte, 2015. They share a number of derived features, including ornamented maxilla, tear-drop shaped coracoid fenestra, short manual digit II, slender tibiotarsus and pedal phalanges, lateral process on the pubis, pubic shaft with strong posterior curvature, spatulate pubic foot, and extremely long caudal vertebrae, among other anatomical details (see Longrich and Currie, 2009; Figure 4). The feathers seen in microraptorians are morphologically diverse and distributed over the entire body. Some forms have expansive wings on the hind- and forelimbs (Xu et al., 2003), a feature that led to the hypothesis of flying capabilities in these taxa (Xu et al., 2003; Senter et al., 2004; Chatterjee and Templin, 2007; Agnolin and Novas, 2013; Han et al., 2014). Interrelationships within Microraptoria are still far from being well understood.
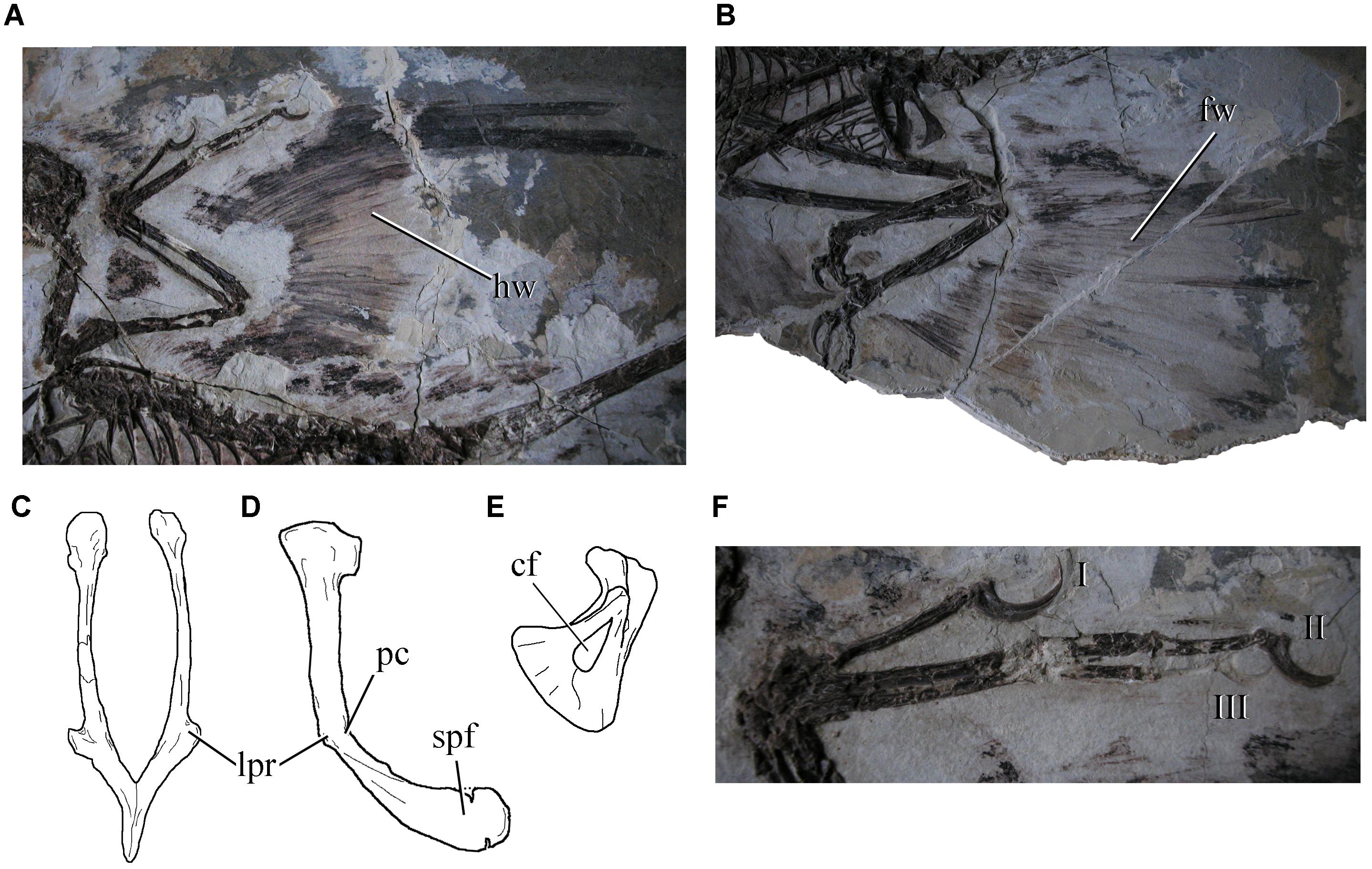
Figure 4. Selected microraptorian traits: (A) feathered hindlimb and (B) feathered forelimbs of Microraptor zhaoianus (IVPP V123352); pubis in anterior (C) and lateral (D) views of Hesperornychus elizabethae (redrawn from Longrich and Currie, 2009) showing the lateral process, posterior flexure of shaft and spatulate pubic foot; (E) coracoid of Sinornithosaurus millenii showing the coracoid fenestra (modified from Xu, 2002); and (F) left manus of Microraptor zhaoianus (IVPP V123352). cf, coracoid fenestra; fw, forelimb wing; hw, hindlimb wing; lpr, lateral process of pubis; pc, posterior concavity; spf, spatulate pubic foot. Not to scale.
Sinornithosaurus millenii was the first microraptorian to be described (Xu et al., 1999b; see Turner et al., 2012), and comes from the Early Cretaceous of China. Its postcranial anatomy shows how remarkably bird-like basal dromaeosaurids are (Xu et al., 1999b). A specimen nicknamed “Dave” (NGMC 91) was subsequently referred to Sinornithosaurus sp. (Ji et al., 2001), but a more precise taxonomic assignment is prevented by the poor state of preservation of the bones and the sub-adult nature of the specimen (Ji et al., 2001). Interestingly, Senter et al. (2004) recovered this specimen as more closely related to Microraptor than to Sinornithosaurus.
Microraptor zhaoianus was the second microraptorian to be described. It is among the smallest known non-avian theropods and comes from the Early Cretaceous of China (Xu et al., 2000, 2003; see Turner et al., 2012; Figure 1A; Lee et al., 2014). It was the first paravian to show not only winged arms but also hind-wings, indicating that the “tetrapteryx” condition (proposed as a speculative possibility by Beebe, 1915) was present in taxa closely related to Aves. This four-winged condition has since been reported in many other basal paravians (see Xu et al., 2017). Microraptor is known from hundreds of specimens (Alexander et al., 2010), and many aspects of its anatomy and paleobiology are relatively well understood including plumage coloration (Li et al., 2012), and diet (Larrson et al., 2010; O’Connor et al., 2011a; Xing et al., 2013).
More recently, Hesperonychus elizabethae (Longrich and Currie, 2009) from Canada became the first non-Asian microraptorian to be described. Moreover, it also extended the temporal range of microraptorians from the Early Cretaceous to the Late Cretaceous (middle Late Campanian). Furthermore, Senter et al. (2004; see also Senter, 2007) proposed that the avian-like Late Cretaceous North American dromaeosaurid Bambiraptor feinbergi (Burnham et al., 2000; Burnham, 2004) belongs to Microraptora. In fact, Bambiraptor shares with microraptorians the presence of slender limb elements and elongate pedal phalanges, premaxillary teeth much smaller than maxillary teeth, posteriorly bowed distal pubis, strongly ventrally oriented post-acetabular blade of the ilium, and large biceps tubercle on coracoid, among other anatomical details (see Senter, 2007). However, the putative microraptorian affinities of Bambiraptor were disputed by Longrich and Currie (2009) and later by Turner et al. (2012), who suggested derived dromaeosaurid affinities for Bambiraptor. In sum, the phylogenetic position of Bambiraptor is still uncertain, but microraptorian affinities should not be dismissed.
Two other members of Microraptoria from the Early Cretaceous of China are Tianyuraptor ostromi (Zheng X. et al., 2009) and Zhenyuanlong suni (Lü and Brusatte, 2015). Both taxa are short-armed and relatively large (approximately 2 m long). They exhibit a combination of characters shared by disparate paravians. For example, Tianyuraptor shows characters present in Microraptoria, including sculptured maxilla, significantly shortened manual phalanx III-2, and spatulate distal end of the pubis, but also lacks many of the synapomorphic characters diagnosing this clade (i.e., large oval fenestra on coracoid, shortened penultimate manual phalanges, and lateral projection on the mid-length of the pubic shaft). This combination of derived and plesiomorphic features led Zheng X. et al. (2009) to suggest that Tianyuraptor might be the basalmost microraptorian, or a basal dromaeosaurid not closely related to microraptorians. If Tianyuraptor is a basal microraptorian, this could indicate that the more derived, long-armed microraptorians convergently acquired forelimb proportions similar to those of birds (Zheng X. et al., 2009). Curiously, Tianyuraptor possess several features that are present in unenlagiids and basal avialans, including a significantly elongated iliac preacetabular process and strongly concave ischial posterior margin (Zheng X. et al., 2009). Similarly, Zhenyuanlong suni (Lü and Brusatte, 2015) lacks many characters shared by most microraptorians (i.e., lateral tubercle on pubic shaft, elongate forelimbs, low number of sacral vertebrae). These characters cast some doubt on the affinities of those two taxa, and their inclusion among microraptorians is not certain.
Zhongjianosaurus yangi (Xu and Qin, 2017) from the Yixian Formation is the most recently named microraptorian. It is one of the smallest non-avian dinosaurs (mass: 0,31 kg), and its unique combination of characters led Xu and Qin (2017) to suggest that it may have played a different ecological role than other Jehol “dromaeosaurids.” Zhongjianosaurus was referred to Microraptoria on the basis of several derived characters (e.g., tear-drop shaped coracoid fenestra, manual digit II short), including a caudotheca, but differs from other microraptorians in diverse anatomical features. Most notably, Zhongjianosaurus shares several derived characters with the early avialans Archaeopteryx, Rahonavis, Jeholornis, Confuciusornis, and Sapeornis (Zhou and Zhang, 2003a,b; Wellnhofer, 2009; Foth and Rauhut, 2017; Rauhut et al., 2018), including forelimbs as robust as hindlimbs, humerus with a strongly medially offset proximal end, large fenestra perforating the deltopectoral crest (a condition shared by basal birds such as Confuciusornis and Sapeornis; Xu and Qin, 2017), ulna longer than humerus, distal end of ulna transversely expanded and ball-like, arctometatarsalian pes, fused tarsometatarsus, and non-gynglimoid metatarsal II. These features are suggestive of avialan relationships for Zhongjianosaurus. However, a comprehensive phylogenetic analysis is needed in order to clarify the affinities of this taxon.
Unenlagiidae
The family Unenlagiidae was erected by Bonaparte (1999) to include Unenlagia comahuensis and Rahonavis ostromi, on the basis of general similarities in scapular and pelvic anatomy. However, the suggestion that Rahonavis is a unenlagiid has been severely criticized by recent authors (O’Connor et al., 2012; Novas et al., 2018; see above).
Currently, the only taxa widely accepted as belonging to Unenlagiidae are South American forms, namely Unenlagia comahuensis, Buitreraptor gonzalezorum, and Austroraptor cabazai (Novas and Puerta, 1997; Makovicky et al., 2005; Novas et al., 2009, 2018; Gianechini et al., 2011b, 2017, 2018; Currie and Paulina Carabajal, 2012) together with less completely known taxa such as Neuquenraptor argentinus, “Unenlagia” paynemili, and Pamparaptor micros (Calvo et al., 2004; Novas and Pol, 2005; Gianechini and Apesteguía, 2011; Porfiri et al., 2011; Brissón Egli et al., 2017). Agnolin et al. (2010), following Bonaparte (1999), proposed that Timimus hermani from the Early Cretaceous of Australia may belong to Unenlagiidae. However, this was recently questioned by Benson et al. (2012), so we think that Timimus should be considered a coelurosaur of uncertain affinities.
Recent analyses (Brissón Egli et al., 2017; Novas et al., 2018; Figure 5) resulted in the recognition of several synapomorphies that support the monophyly of unenlagiids, including scapula with poorly developed glenoid lips, proximally positioned scar-like fourth trochanter on the femur, metatarsal III with a sublaminar and anteriorly excavated proximal portion, metatarsal II with an expanded tongue-shaped flange that overlaps most of the posterior surface of metatarsal III, metatarsal IV with notably convex posterior flange, pedal phalanx III-1 strongly ventrally arched, and penultimate pedal phalanges with dorsally displaced collateral ligamental pits that almost converge dorsally. These features are absent in most basal paravians, including Dromaeosauridae.
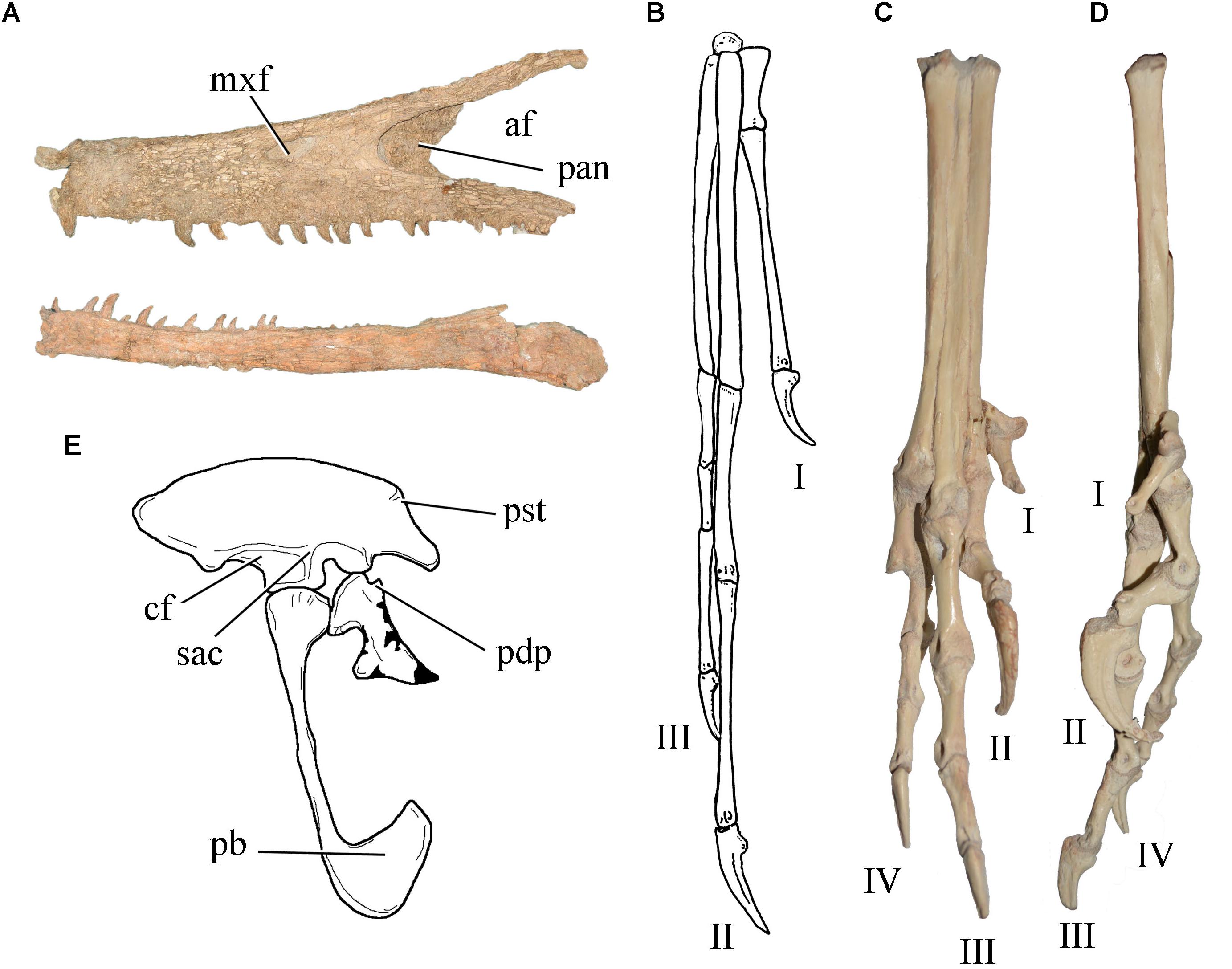
Figure 5. Selected unenlagiid traits: (A) maxilla and dentary of Austroraptor cabazai showing the rostral process of the maxilla, the anteroposteriorly elongated postantral wall, and the sub-parallel dorsal and ventral margins of the dentary; (B) elongate left manus of Buitreraptor gonzalezorum (modified from Novas et al., 2018); dorsal (C) and medial (D) views of Buitreraptor gonzalezorum pes showing slender and gracile metatarsals, sub-equal lengths of metatarsals II and IV, and modified digit II (MPCA-PV-598); (E) reconstruction of Unenlagia comahuensis pelvic girdle (modified from Novas and Puerta, 1997) indicating the proximodorsal process, pronounced supracetabular crest, and sub-triangular pubic boot. af, antorbital fenestra; cf, fossa for M. cuppedicus; mxf, maxillary fenestra; pan, postantral wall; pb, pubic boot; pdp, proximodorsal process; pst, processus supratrochantericus; rpmx, rostral process of maxilla; sac, supracetabular crest. Not to scale.
Rahonavis ostromi, a species assigned to Unenlagiidae by some authors, comes from the Upper Cretaceous of northwestern Madagascar (Forster et al., 1998). It is known from a partial skeleton and several isolated specimens, and shows a striking combination of avian-like and dromaeosaurid-like features (Forster et al., 1998; O’Connor and Forster, 2010). This combination of traits has resulted in a lack of consensus about the phylogenetic affinities of Rahonavis.
Bonaparte (1999), Makovicky et al. (2005) and several recent authors (e.g., Hu et al., 2009, 2018; Xu et al., 2011; Foth et al., 2014; Gianechini et al., 2017, 2018) included Rahonavis within Unenlagiidae, mainly based on the presence of a prominent supracetabular crest, a concave dorsocaudal edge of the ilium, and a preacetabular process of the ilium that is markedly longer than the postacetabular process. Nevertheless, these traits are widespread among basal avialans such as Archaeopteryx, Sapeornis, Jeholornis, and Confuciusornis (Agnolin and Novas, 2013). In agreement with the conclusions reached by Forster et al. (1998) in their original description of Rahonavis, many authors have regarded this Malagasy taxon as closely allied to Avialae (e.g., Chiappe, 2001; Hwang et al., 2002; Zhou and Zhang, 2003b; Novas and Pol, 2005; Xu et al., 2008; Yuan, 2008; O’Connor et al., 2011b; Agnolin and Novas, 2013; Godefroit et al., 2013b; Cau et al., 2017; Novas et al., 2018). Features shared by Rahonavis and avialans, but not by dromaeosaurids or unenlagiids, include large and prominent acromion on the scapula, twisted distal end of the ulna, and several pelvic and pedal features (Novas et al., 2018). These features indicate a close relationship between Rahonavis and basal, long-tailed birds more derived than Archaeopteryx (see also O’Connor et al., 2012), and support the original phylogenetic hypothesis proposed by Forster et al. (1998).
Anchiornithinae
Anchiornis huxleyi (Xu et al., 2008; Hu et al., 2009) is one of the best-known Jurassic Chinese paravians, represented by more than 200 specimens from the Upper Jurassic (Oxfordian) Tiaojishan Formation of Liaoning Province (Zheng et al., 2014). The excellent preservation of many of these specimens allows evaluation of details of feather morphology (Longrich et al., 2012; Saitta et al., 2018; Figure 1B), the shape of the body outline (Wang et al., 2017), intraspecific differences (Pei et al., 2017a), and also feather color (Li et al., 2010). Moreover, several new taxa closely related to Anchiornis have recently been described, including Xiaotingia, Pedopenna, Aurornis, Eosinopteryx, Serikornis, Liaoningvenator, and Caihong (Xu and Zhang, 2005; Xu et al., 2011; Godefroit et al., 2013a,b; Lefèvre et al., 2017; Shen et al., 2017; Hu et al., 2018).
Despite lack of consensus regarding their phylogenetic position, Anchiornis and Xiaotingia are usually regarded as forming a monophyletic group (see Foth and Rauhut, 2017) for which Xu (2016) coined the name Anchiornithinae. The latter group of authors defined this subfamily as the most inclusive clade including Anchiornis but not Archaeopteryx, Gallus, Troodon, Dromaeosaurus, Unenlagia, or Epidexipteryx. At nearly the same time, Foth and Rauhut (2017) erected the clade Anchiornithidae in order to include Xiaotingia, Pedopenna, Eosinopteryx, Anchiornis, and Ostromia, designating Anchiornis huxleyi as the type genus of this group. Furthermore, Hu et al. (2018) coined the name Anchiorninae to gather together nearly the same list of taxa. However, priority belongs to those who originally erected the clade, namely Xu (2016). We therefore recommend use of Anchiornithinae to avoid future taxonomic and nomenclatural mistakes.
Anchiornithinae was diagnosed by Foth and Rauhut (2017) on the basis of several synapomorphies, most of them shared with troodontids (for example, nutrient foramina on dentary placed in a deep groove, dentary teeth numerous, anterior dentary teeth smal, and more closely appressed than those in the middle of the tooth row) or avialans (for example, anterior edge of acromial margin of scapula laterally everted or hooked, medial surface of proximal fibula flat, and fan-shaped posterior dorsal neural spines) (Figure 6). Synapomorphies possibly exclusive of Anchiornithinae among paravians are dorsal vertebrae with posteriorly expanded neural spines, straight nasal process of the premaxilla, very short deltopectoral crest of the humerus, and straight ulna and radius, among others [see Foth et al. (2014) and Pei et al. (2017a) for details] (Figure 6).
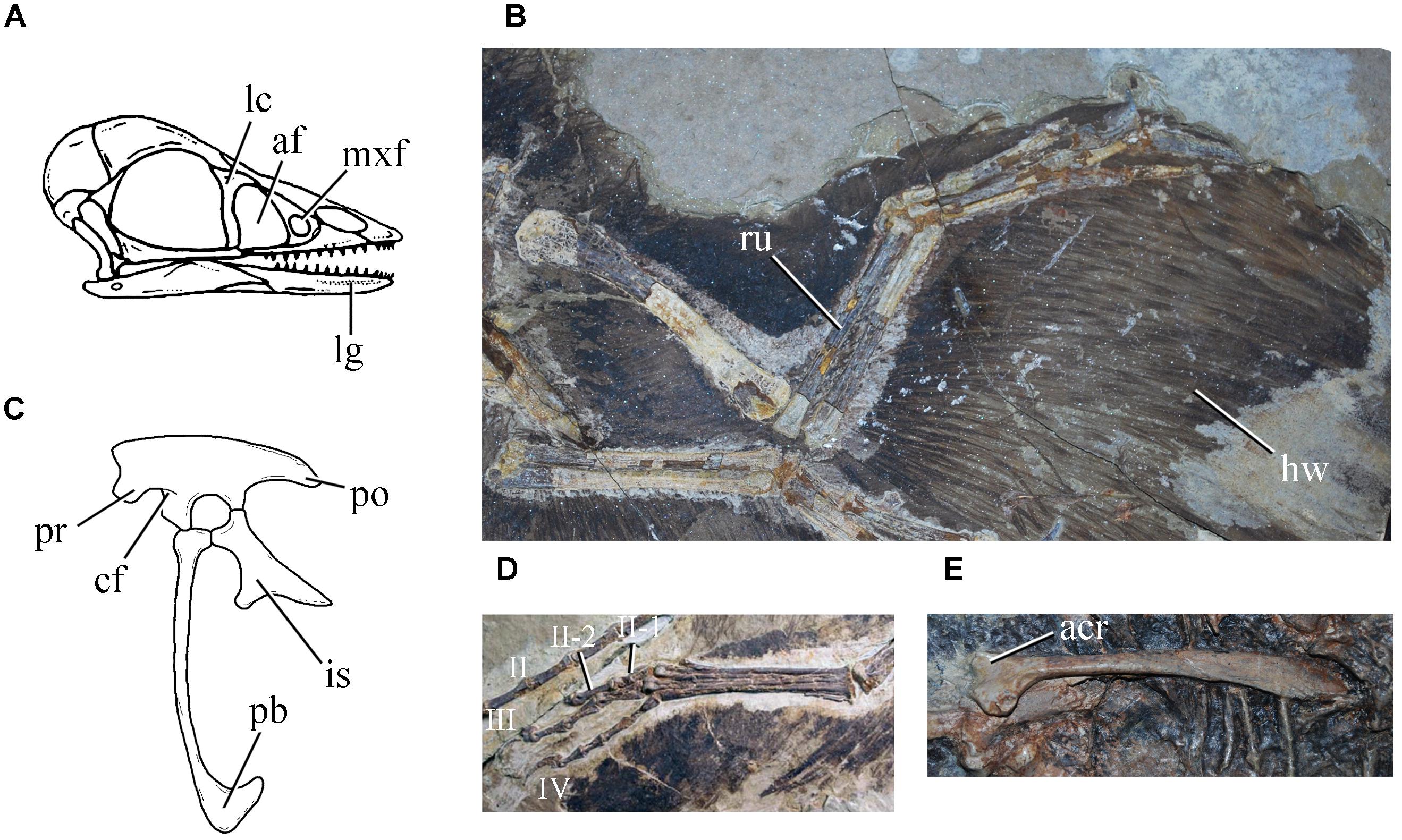
Figure 6. Selected anchiornithine traits: (A) reconstruction of Anchiornis huxleyi (modified from Agnolin and Novas, 2013) skull, showing nutrient foramina on dentary placed in a deep groove, small anterior dentary teeth, maxillary fenestra ventrally placed, and antorbital fenestra dorsoventrally expanded; (B) Anchiornis huxleyi left forelimb showing straight radius and ulna and long covert feathers; (C) reconstruction of Anchiornis huxleyi pelvic girdle showing anteriorly long postacetabular blade and ventrally oriented preacetabular blade (modified from Xu et al., 2011); (D) right foot of Anchiornis huxleyi (BMNHC PH 828); (E) Xiaotingia zhengi (STM 27-2) left scapula. acr, acromion; af, antorbital fenestra; cf, cuppedicus fossa; hw, wing feathers; is, ischium; mxf, maxillary fenestra; lc, lacrimal; lg, lateral groove of dentary; pb, pubic boot; po, postacetabular blade; pr, preacetabular blade; ru, radius. Not to scale.
Taxa commonly interpreted as members of Anchiornithinae include Anchiornis, Xiaotingia, Pedopenna, Aurornis, Eosinopteryx, Serikornis, Caihong, and Ostromia (Xu and Zhang, 2005; Xu et al., 2011; Godefroit et al., 2013a,b; Foth and Rauhut, 2017; Lefèvre et al., 2017; Hu et al., 2018). However, the following comments are required.
Eosinopteryx, Aurornis, and Serikornis are from the same beds and in the same area as the holotype of Anchiornis (Middle-Late Jurassic Tiaojishan Formation, Godefroit et al., 2013a,b; Lefèvre et al., 2017). All of these taxa closely resemble Anchiornis huxleyi in most anatomical details, and were mainly distinguished from that species by subtle osteological differences and proportions (see Foth et al., 2014; Pei et al., 2017a for details). Moreover, it is worthy of note that Eosinopteryx, Aurornis, and Serikornis are each based on a single specimen, while Anchiornis is represented by more than 200 specimens that show important intraspecific variation (Pei et al., 2017a). Further, the two-dimensional nature of Liaoning fossils renders identification of some anatomical features difficult, and in some cases deformation can obscure anatomical details. We agree with Pei et al. (2017a) in considering Aurornis xui to be a junior synonym of Anchiornis huxleyi, based on the absence of unambiguous characters distinguishing the two species. Pei et al. (2017a) also indicated that Eosinopteryx is almost identical to Anchiornis, except in the shorter length of the tail. However, Hu et al. (2018) pointed out that the tail of the only known specimen of Eosinopteryx brevipenna is not complete, and thus, tail length cannot be used as a point of distinction from Anchiornis. Further, Pei et al. (2017a) and Hu et al. (2018) noticed a high level of variation in plumage morphology and preservation among Anchiornis specimens, and this calls into question the use of plumage characters to differentiate closely related taxa. Thus, we uphold the view that Eosinopteryx brevipenna is a junior synonym of Anchiornis huxleyi.
Lefèvre et al. (2017) proposed two unambiguous autapomorphies of Serikornis that clearly distinguish it from Anchiornis and related taxa: the anteriormost four maxillary teeth are twice as large as the others; and the ventrodistal process of the ischium is narrow, hook-like, strongly deflected caudodorsally and placed at the distal end of the ischium. Nevertheless, figures of the holotype specimen of Serikornis (Lefèvre et al., 2017; Supplementary Figure S4) show that the four anteriormost maxillary teeth are somewhat loose and the partially exposed roots show the typical longitudinal groove commonly seen in other paravian specimens (see Gianechini et al., 2011a). Furthermore, tooth size is variable within Anchiornis and Caihong, as demonstrated by recent investigations (see Pei et al., 2017a; Hu et al., 2018; Figure 7).
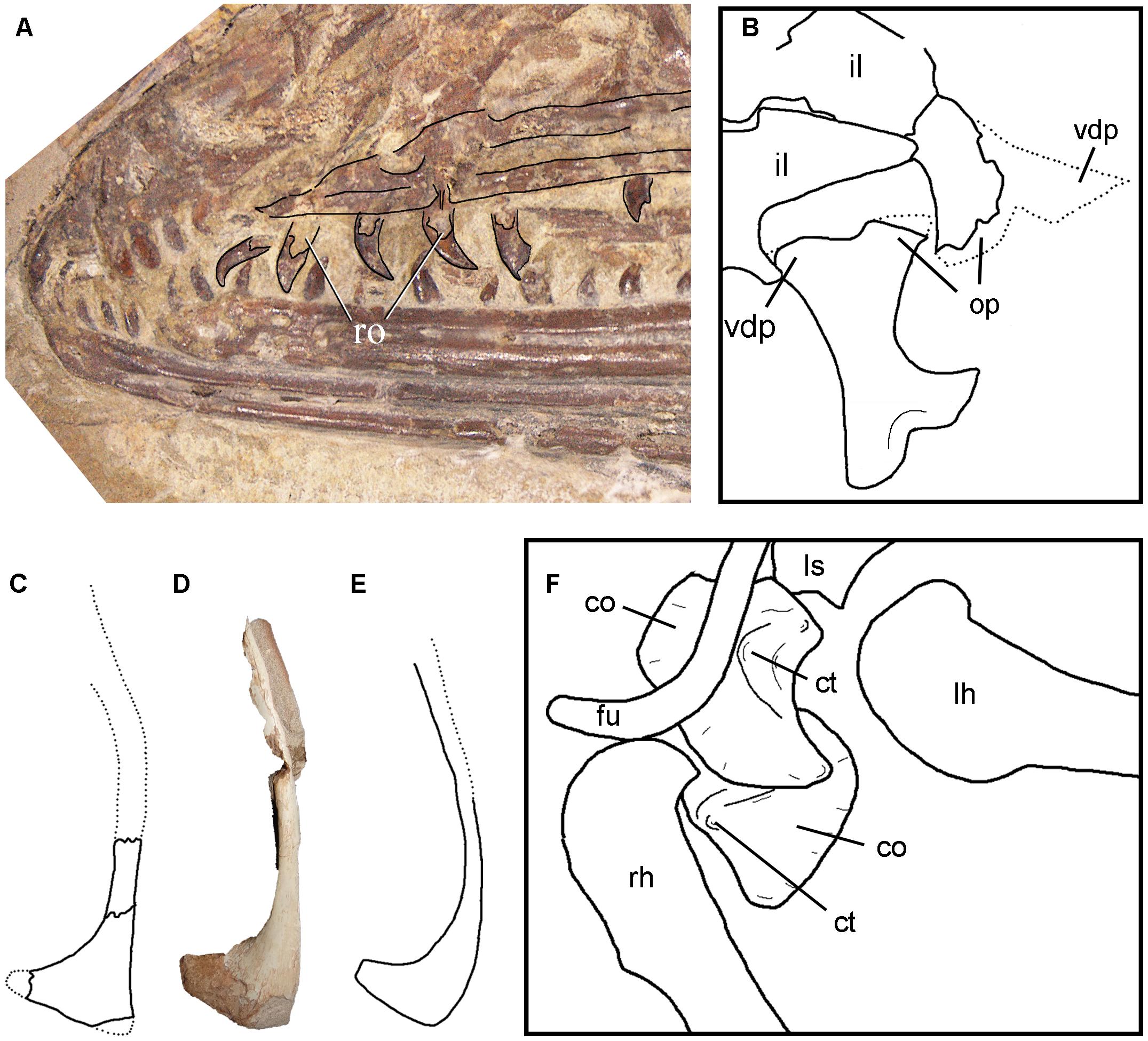
Figure 7. Re-evaluation of Anchiornis-like paravians: (A) Serikornis sungei maxillary teeth indicating the inner root and external displacement (modified from Lefèvre et al., 2017); (B) re-interpretation of the right coracoid of Serikornis sungei indicating the position of the coracoid tubercle (modified from Lefèvre et al., 2017); (B–E) right pubes in lateral view of Ostromia crassipes (C) (modified from Foth and Rauhut, 2017); Buitreraptor gonzalezorum (D) (MPCA-PV-598); and Anchiornis huxleyi (E) (BMNHC PH 828) showing the posterior flexure of the pubic shaft, sub-triangular shape of the pubic boot and flat ventral margin of the pubic boot; (F) re-interpretation of ischium of Anchiornis huxleyi (modified from Lefèvre et al., 2017). Not to scale. co, coracoids; ct, coracoid tuber; cv, caudal vertebrae; f, femur; fu, furcula; il, ilium; lh, left humerus; lpr, lateral process of the coracoid; ls, left scapula; op, obturator process; rh, right humerus; ro, inner root of teeth; rs, right scapula sac, sacral vertebrae, vdp, ventrodistal process.
Lefèvre et al. (2017) also indicated that the ischium of Serikornis is very different from that of other paravians, including Anchiornis. However, we suggest that the Anchiornis left ischium illustrated by these authors (Lefèvre et al., 2017, Figure 3C), is displaced from its original position, and rotated upside down. Thus, the processes originally interpreted as the “obturator process” and the “ventrodistal process” correspond, in fact, to the ischiadic and iliac processes, respectively (Figure 7B). In the context of this new interpretation, the concavity that separates the two processes represents the acetabular margin, being well-delimited ventrolaterally by a concave crest that constitutes the ventral acetabular margin.
The presence of a ventrodistal process of the ischium that is relatively narrow, hook-like (caudodorsally deflected) and located at the distal end of the bone is described by Lefèvre et al. (2017) as an autapomorphy of Serikornis. Nevertheless it is possible to observe in Figure 3 and Supplementary Figure S6 of Lefèvre et al. (2017) that the ischia of Serikornis are highly taphonomically compressed and damaged. Further, details of ischium morphology are intraspecifically variable among Anchiornis specimens (Xu et al., 2008; Hu et al., 2009; Pei et al., 2017a). For all these reason, we are not certain about the distinctive ischial features of Serikornis identified by Lefèvre et al. (2017). We think that some such features may be encompassed within the intraspecific variation of Anchiornis, while others may be the result of postmortem deformation of the element.
Another feature listed by Lefèvre et al. (2017) in support of the validity of Serikornis, is the absence of numerous small pits sculpturing the ventral surface of coracoid, a condition regarded as autapomorphic for Anchiornis (Xu et al., 2009). Nevertheless, the illustrated right coracoid of Serikornis (Lefèvre et al., 2017, Figure 4A) appears to have only its anterodorsal surface exposed (as suggested in the present contribution, Figure 7F), so that the condition of the outer surface of the coracoid in the holotype specimen of Serikornis remains unknown.
In sum, because of the absence of strong autapomorphic characters that allow Serikornis to be distinguished from Anchiornis, we propose that Serikornis sungei is a junior synonym of Anchiornis huxleyi.
Shen et al. (2017) published a new, exquisitely preserved theropod named Liaoningvenator curriei, from the Early Cretaceous Yixian Formation of Liaoning Province in China. The authors recovered Liaoningvenator curriei as a basal troodontid, forming a clade with Anchiornis and its kin (=Anchiornithinae auct). Its inclusion among anchiornithines was based on the presence of a laterally everted acromial margin on the scapula and a posterior flange on manual phalanx II-1 (Shen et al., 2017). Additionally, Liaoningvenator shares with anchiornithines the following characters: straight radio-ulna, dorsal vertebrae with posteriorly expanded neural spines, and transition point anteriorly located within the caudal vertebrae series. Nevertheless, Liaoningvenator shows strong differences from anchiornithines, including dorsal centra that retain pneumatic foramina and a humerus that is relatively short when compared with the femur. The ischium is also different from that of Anchiornis, lacking a long and finger-like posterodistal process, whereas the obturator process is longer than in Anchiornis, resembling the condition in the troodontid Almas (Pei et al., 2017b). The unique combination of characters shown by Liaoningvenator casts some doubt on the affinities of this taxon, and its inclusion among anchiornithines is not certain.
Recently, Foth and Rauhut (2017) re-described the partial, poorly preserved Haarlem Archaeopteryx specimen from the Late Jurassic of Germany as belonging to the new taxon Ostromia crassipes, and referred this species to Anchiornithidae. The authors supported the inclusion of Ostromia among anchiornithines on the basis of pubis morphology and the presence of longitudinal furrows on both sides of all preserved manual phalanges, and of metacarpal III. Although Foth and Rauhut (2017) discuss the longitudinal furrows in depth, we are not confident that they constitute a true anatomical feature. In fact, longitudinal grooves may form along manual and pedal elements as the result of taphonomic compression during fossilization. Furrows along the long axes of long bones are common in two-dimensionally preserved fossils, and indeed are also observed in the femur of Ostromia (see Foth and Rauhut, 2017, Figure 6A). The furrows are probably the result of the collapse of the cortical bone into the wide medullary cavity, resulting in furrow-like longitudinal breakage (Figure 7).
Another trait that Foth and Rauhut (2017) indicate as shared between Ostromia and Anchiornis is a pubic shaft that is strongly curved, being anteriorly convex at mid-length. This condition contrasts with the slender and straight pubis exhibited by other Archaeopteryx specimens (Wellnhofer, 2009). Furthermore, the pubic boot of Ostromia is sub-triangular in outline with an almost straight distal margin (Foth and Rauhut, 2017), contrasting with other Archaeopteryx specimens in which the pubic boot is distally convex and spoon-shaped (Wellnhofer, 2009; Rauhut et al., 2018). Nevertheless, the type of pubic morphology seen in Ostromia is also present in Buitreraptor, in which the pubic boot is likewise sub-triangular in outline, with a straight distal margin and a shallow, anterior ridge-like expansion of the boot (Novas et al., 2018). The presence of such a combination of characters in the unenlagiid Buitreraptor and also probably in Unenlagia (Novas and Puerta, 1997; Figure 7) weakens the case that Ostromia has anchiornithine affinities.
We agree with Foth and Rauhut (2017) in that the Haarlem specimen should not be referred to Archaeopteryx, but the inclusion of Ostromia among Anchiornithinae is dubious at best, and we propose that it should instead be considered an indeterminate paravian pending inclusion of the only known specimen in a detailed phylogenetic analysis. In sum, our evidence and observations lead us to restrict the taxonomic composition of Anchiornithinae to the genera Anchiornis, Xiaotingia, Pedopenna, and Caihong.
Scansoriopterygidae
Zhang et al. (2002) described the new genus and species Epidendrosaurus ningchengensis, on the basis of a partial articulated skeleton from the Middle Jurassic Haifanggou Formation at Ningcheng County, China. The specimen was interpreted as a juvenile individual with a unique combination of characters. Because of its bizarre anatomy, Zhang et al. (2002) were not able to narrow down its phylogenetic position beyond Maniraptora. Nearly at the same time, Czerkas and Yuan (2002) described an incomplete maniraptoran skeleton from the same beds under the name Scansoriopteryx heilmanni, and due to its unique features coined the monotypic clade Scansoriopterygidae. Later, Wang et al. (2005) showed that both skeletons pertain to the same taxon, and synonymized both under the species name Epidendrosaurus ningchengensis based on priority. More recently, Zhang et al. (2008) described a well-preserved skeleton with feathers from the same beds and named it Epidexipteryx hui. This new find allowed Zhang et al. (2008) to recognize that Scansoriopterygidae included at least two different genera. Recently, Xu et al. (2015) reported the new scansoriopterygid Yi qi based on a partial skeleton from the Middle–Upper Jurassic Tiaojishan Formation of Hebei Province, China. This specimen provides valuable anatomical information, including data regarding soft tissues and the unique presence of membranous wings and propatagia. Scansoriopterygids are small taxa (approximately 26 cm long from tip of bony tail to premaxilla In the case of Epidexipteryx) characterized by manual digit III considerably exceeding the lengths of the remaining digits, propubic pelvis with pubis lacking distal boot and elongate ischium dorsally curved, and several additional features (see Zhang et al., 2008; Xu et al., 2015). Further, Yi shows a unique neomorphic wrist bone (“styliform element”) that supported a wing membrane (Xu et al., 2015). Scansoriopterygids also preserve impressions of soft tissue, including a covering of filamentous protofeathers (in Epidendrosaurus, Epidexipteryx, and Yi), elongate ribbon-like tail feathers (in Epidexipteryx), and patches of a membrane extending between succesive manual digits (in Yi). Notably enough, the membranous surface present in Yi is aerodynamically quite different from the feathered wings characterizing birds and their closest relatives (Xu et al., 2015).
O’Connor and Sullivan (2014) proposed that the Early Cretaceous basal avialan Zhongornis haoae belonged to the Scansoriopterygidae. However, this species shows strong differences from Epidexipteryx and Yi, including edentate rostrum, elongate distally acute scapula, strut-like coracoid (but see O’Connor and Sullivan, 2014), large and robust furcula (a furcula appears to be absent in scansoriopterygids), and caudal vertebrae with short zygapophyses and small chevrons, among other anatomical details. These features, taken together, are present in birds as originally proposed by Gao et al. (2008), suggesting that the phylogenetic position of Zhongornis is at least dubious.
In an innovative contribution, Xu et al. (2011) proposed that archaeopterygids were not directly related to birds but were basal deinonychosaurians. In the same paper, Xu et al. (2011) indicated that scansoriopterygids and oviraptorosaurians probably represented the morphotype ancestral to birds. Members of both groups resemble basal avialans such as Jeholornis and Sapeornis in having an anteroposteriorly short and dorsoventrally tall skull with small orbits and a very deep premaxilla and dentary, and in possibly being herbivorous. However, Agnolin and Novas (2013) noted that the condition in some basal birds was uncertain due to poor preservation, and also pointed out that the skull shape of several basal birds, such as Ichthyornis and members of Enantiornithes, is similar to that of Archaeopteryx and “deinonychosaurs.” This contradicts the evolutionary scenario proposed by Xu et al. (2011) and suggests that deep and robust skulls, as well as herbivorous habits, were homoplastically distributed among paravians and that these traits were probably convergently acquired by Scansoriopterygidae plus Oviraptorosauria, and some basal birds (see also Lee and Worthy, 2012).
Paravian Phylogeny: Comments and Proposals
A virtual consensus exists among authors to the effect that Dromaeosauridae, Troodontidae, Unenlagiidae, Anchiornithidae, Microraptoria, and Aves belong to a monophyletic clade named Paraves (see Norell et al., 2001; Cau, 2018). Nevertheless, there exists a wide array of competing interpretations as to the interrelationships among the above-mentioned subclades.
As a step toward the general aim of evaluating previous hypotheses of the phylogenetic relationships of basal paravians, we decided to test the robustness and accuracy of the cladograms produced by the TWiG data matrix from Gianechini et al. (2017). We chose this matrix because it provided the basis for the phylogenetic proposals that were affirmed by a relatively strong consensus in the 1990’s and 2000’s, and drove most explanations and hypotheses regarding evolutionary changes at the dinosaur-bird transition.
Very recently, detailed studies of Buitreraptor and its kin (Brissón Egli et al., 2017; Gianechini et al., 2017, 2018; Novas et al., 2018) improved our knowledge of the anatomy of unenlagiids in general. With the new information afforded by Buitreraptor, we also recode into the TWiG matrix, based on first hand examination, all relatively informative unenlagiids, including Unenlagia comahuensis and Austroraptor cabazai (see Supplementary Material). The new codings strongly affected tree topology, resulting in an arrangement sharply different from that produced by analysis of previous versions of the TWiG matrix (Makovicky et al., 2005; Norell et al., 2006; Turner et al., 2012; Gianechini et al., 2017, 2018). The consensus tree (see Supplementary Material) included a large polytomy at the base of Pennaraptora, encompassing Oviraptorosauria, Unenlagiidae, Microraptoria, Eudromaeosauria, Troodontidae, and Avialae (Figure 8). The clades Oviraptorosauria, Troodontidae, and Avialae are recovered as monophyletic while Dromaeosauridae sensu lato is not recovered. The results from our test of the TWiG matrix agree with those of some previous phylogenetic analyses (Xu et al., 2011; Agnolin and Novas, 2013; Lefèvre et al., 2017; Cau, 2018) in suggesting that the traditional concepts of Deinonychosauria and Dromaeosauridae rest on weak evidence and lack strong anatomical support. Because of the poor resolution obtained from analysis of the TWiG matrix, we opt not to follow these phylogenetic results, and instead present below our own interpretations of the possible phylogenetic affinities of each of these paravian subclades based on a review of the available morphological evidence.
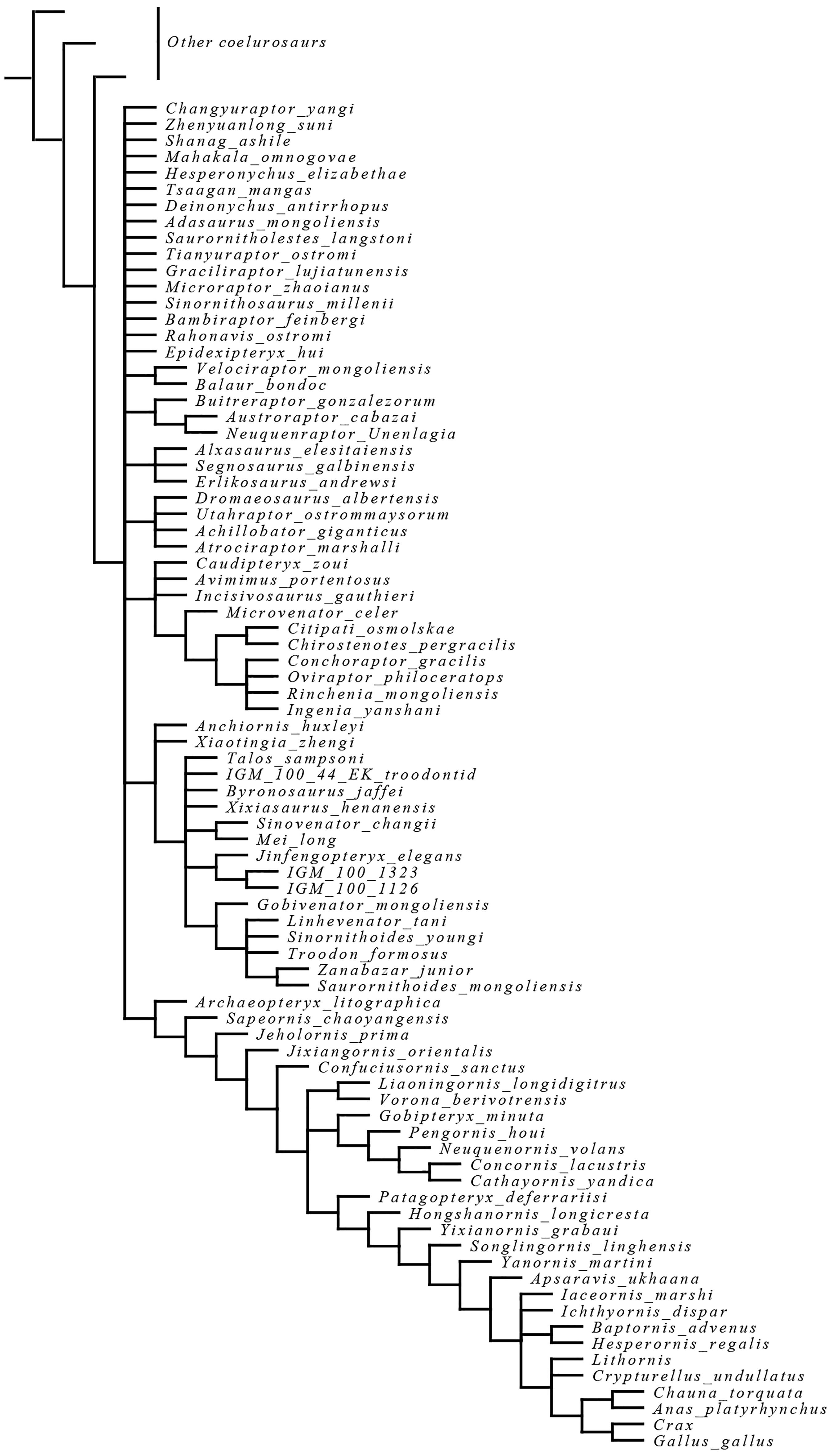
Figure 8. Strict consensus tree based on modified TWiG data matrix. Using this modified version of the matrix, the phylogenetic relationships of basal paravians are not resolved.
Phylogenetic Relationships of Unenlagiidae Among Paraves
Unenlagia was originally interpreted as more closely related to birds than to dromaeosaurids (e.g., Novas and Puerta, 1997; Forster et al., 1998; Xu et al., 1999b; Rauhut, 2003; Novas, 2004). Subsequently, Makovicky et al. (2005) nested Unenlagia and its kin within Dromaeosauridae under the name Unenlagiinae, an interpretation followed by most later cladistic analyses (e.g., Novas and Pol, 2005; Senter, 2007; Hu et al., 2009, 2018; Novas et al., 2009; Xu et al., 2011; Turner et al., 2012; Godefroit et al., 2013a,b; Cau et al., 2017; Foth and Rauhut, 2017; Gianechini et al., 2017, 2018; Lefèvre et al., 2017). In contrast, Agnolin and Novas (2011, 2013; see also Novas et al., 2018) regarded unenlagiids as stem Avialae, in agreement with the original description of Unenlagia (Novas and Puerta, 1997). In this connection, Agnolin and Novas (2011) analyzed the unenlagiid traits that had been considered as synapomorphies of Deinonychosauria by previous authors (e.g., Turner et al., 2007b; Xu et al., 2008; Hu et al., 2009), and concluded that most of them were widespread among coelurosaurs (for details see Agnolin and Novas, 2011, 2013). The reanalysis of the TWiG data matrix presented above helps to confirm that the inclusion of unenlagiids within deinonychosaurs or dromaeosaurids lacks support.
Agnolin and Novas (2011, 2013) also discussed the features shared with Avialae that support the exclusion of Unenlagiidae from Dromaeosauridae. As such, Agnolin and Novas (2013) emphasized the multiple avian-like traits present in unenlagiids, including: elongate and subtriangular acromion on scapula, extremely elongate and gracile metacarpals and manual phalanges, and ilium with large lobular preacetabular blade and thick, well-defined supratrochanteric process, among other anatomical details (Novas, 2004; Novas et al., 2018).
In sum, we follow the original proposal by Novas and Puerta (1997) that unenlagiids constitute the immediate sister-group of Avialae.
Phylogenetic Relationships of Troodontidae Among Paraves
Troodontidae was regarded as the sister group of Dromaeosauridae by Gauthier (1986), an interpretation that has been followed by many later authors (e.g., Norell et al., 2001; Xu et al., 2011). However, based on detailed analysis of the braincase, Currie (1985, 1987) and Currie and Zhao (1993) concluded that troodontids share with birds many derived features that are absent among dromaeosaurids. The hypothesis that troodontids are more closely related to birds than to dromaeosaurids has gained support in recent years (Godefroit et al., 2013a; Cau et al., 2017; Foth and Rauhut, 2017; Lefèvre et al., 2017; Cau, 2018; Gianechini et al., 2018). Some of the most relevant features supporting this include premaxillary teeth round in cross-section, anterodorsally inclined lacrimal with a medially inset ventral ramus, vaulted frontals and parietals, reduced supratemporal fossae not extending onto the frontals, depressed crista interfenestralis in the middle ear, dorsoventrally elongate foramen magnum, absence of a fossa housing cranial nerves X and XII, vestigial coronoid, marked reduction of middle caudal postzygapophyses, relatively short ilium, and proximally narrowing femoral diaphysis, among other anatomical details. However, most of these features are also documented in unenlagiid theropods (e.g., Austroraptor, Buitreraptor), including the anterodorsally inclined lacrimal with inset ventral ramus, reduced supratemporal fossae, vestigial coronoid, and proximally narrowing femoral shaft (Agnolin and Novas, 2011; Gianechini et al., 2017; Novas et al., 2018). Furthermore, several of the above mentioned braincase features are also shared by selected oviraptorids and dromaeosaurids (Currie, 1985; Chatterjee, 1991; Makovicky et al., 2003; Norell et al., 2004; Rauhut, 2004), showing that several features proposed to unite birds and troodontids are probably more widespread than suspected. Nevertheless, some characters stand as potential synapomorphies uniting birds and troodontids, namely a vaulted skull and a marked reduction of the middle caudal postzygapophyses, features not seen in dromaeosaurids, microraptorians, and unenlagiids.
In sum, we agree with Currie’s (1985) original assessment that the affinities of troodontids among paravians are far from being well understood.
Phylogenetic Relationships of Microraptoria Among Paraves
Traditionally, microraptorians were considered to be nested within Dromaeosauridae, as basal members of this clade (e.g., Longrich and Currie, 2009; Turner et al., 2012; Lü and Brusatte, 2015). However, Agnolin and Novas (2011, 2013) criticized previous lists of dromaeosaurid features thought to be present in microraptorians, suggesting that most of these characters were more widespread than previously thought. In spite of this, microraptorians share with dromaeosaurids such as Deinonychus and Velociraptor a rigid caudal sheath, known as a caudotheca and formed of notably elongate prezygapophyses and chevrons (Senter, 2011). This condition may stand as the only unambiguous synapomorphy uniting Microraptoria with Dromaeosauridae.
Moreover, some authors have recovered Microraptoria in different positions, alternatively forming a polytomy with Troodontidae, Unenlagiidae, and Dromaeosauridae (Xu et al., 2008), or as stem Avialae (Agnolin and Novas, 2011, 2013). In fact, microraptorians such as Sinornithosaurus and Microraptor share with unenlagiids and with basal avialans such as Archaeopteryx some anatomical details, including ventrally bowed manual phalanges (especially phalanx I-1), manual phalanx II-1 with a posteromedial flange that probably anchored feathers (Paul, 2002), posterodorsal process on ischium, distally placed obturator process on ischium, proximally pinched metatarsal III (subarctometatarsalian condition), and posterior flange on metatarsal IV. These features, as detailed by Agnolin and Novas (2013), suggest that microraptorians may be more nearly related to avialans than to dromaeosaurids.
Phylogenetic Relationships of Anchiornithinae Among Paraves
The position of Anchiornis and its kin among paravians is far from being settled (Hu et al., 2009, 2018; Xu et al., 2009; Agnolin and Novas, 2011, 2013; Turner et al., 2012; Cau et al., 2017; Foth and Rauhut, 2017; Pei et al., 2017a). Xu et al. (2009) originally proposed that Anchiornis was the basalmost known avialan, a suggestion followed by some other authors (Agnolin and Novas, 2011, 2013; Godefroit et al., 2013b; Pei et al., 2017a). Later, Hu et al. (2009) argued that Anchiornis might be the basalmost troodontid, the interpretation followed by most authors (e.g., Gao et al., 2012; Lee and Worthy, 2012; Senter et al., 2012; Turner et al., 2012; Xu et al., 2012; Godefroit et al., 2013a; Tsuihiji et al., 2014, 2016; Brusatte et al., 2015; Averianov and Sues, 2016; Gianechini et al., 2017). Later, Xu et al. (2011) included Archaeopteryx, Anchiornis, and Xiaotingia within the clade Archaeopterygidae, which was in turn nested within Deinonychosauria (Troodontidae plus Dromaeosauridae and Archaeopterygidae) and far from Avialae (Xu et al., 2011, 2015). Finally, Lefèvre et al. (2017) proposed that Xiaotingia was more related to Scansoriopterygidae than to Anchiornis, and that Pedopenna, Aurornis, Eosinopteryx, and Serikornis formed a monophyletic sister clade to Eumaniraptora, far from Anchiornis.
Hu et al. (2009) included Anchiornis within Troodontidae, forming part of a monophyletic Deinonychosauria. Xu et al. (2011) considered that Archaeopteryx, Anchiornis, and Xiaotingia shared with traditionally recognized deinonychosaurs some unique features unknown in any other theropod group, including: (1) large promaxillary fenestra, (2) T-shaped lacrimal, (3) lateral, posteriorly expanding longitudinal groove on the dentary, (4) manual phalanx IV-2 shorter than IV-1, (5) short ischium, (6) ischium with distally located obturator process and posterodistal process, and (7) extensible pedal phalanx II-2. However, Agnolin and Novas (2013) claimed that these traits are diagnostic of Paraves, and that some of them (e.g., characters 1, 2, 4, 6) are shared with basal birds (e.g., Confuciusornis, Sapeornis, Jeholornis; Chiappe et al., 1999; Zhou and Zhang, 2002, 2003a,b; O’Connor et al., 2011b; Lee and Worthy, 2012). In agreement with this interpretation, Xu et al. (2011) reported a large number of typical deinonychosaurian features that are wanting in Archaeopteryx, Anchiornis, and Xiaotingia, including lateral exposure of the splenial, muscle scar on the deltopectoral crest, and enlarged, raptorial ungual on pedal digit II. Thus, deinonychosaurian affinities for Anchiornis are not supported by available evidence.
Regarding inclusion of Anchiornis within Troodontidae, Hu et al. (2009) listed the following characteristics: large maxillary fenestra separated from the antorbital fenestra by a narrow interfenestral bar, dorsoventrally flattened internarial bar, posteriorly widening groove on dentary, and closely packed premaxillary and dentary teeth in the symphyseal region. Agnolin and Novas (2013), however, discussed these characters and concluded that most of them are widespread among derived paravians (e.g., the presence on the dentary of a lateral groove carrying a series of neurovascular foramina), have an uncertain distribution among Paraves (e.g., closely packed dentary and premaxillary teeth), or are synapomorphies of the clade Anchiornis + Xiaotingia plus Avialae (e.g., a flat internarial bar).
Phylogenetic Relationships of Archaeopterygidae and Scansoriopterygidae Among Paraves
Based on their phylogenetic analysis, Xu et al. (2011) grouped Archaeopteryx, Anchiornis, and Xiaotingia within Archaeopterygidae on the basis of several features, including manual phalanx III-1 more than twice as long as IV-1, manual phalanx IV-3 markedly longer than IV-1 plus IV-2 combined, and ventral notch between distal portion of obturator process and ischial shaft. However, this arrangement was strongly criticized, and most authors agree that Archaeopteryx and Anchiornis do not conform a monophyletic clade (see Turner et al., 2012). It is possible to interpret the combination of characters highlighted by Xu et al. (2011) as diagnostic of basal avialans, rather than exclusive to Archaeopterygidae, in accordance with the phylogenetic scheme of Agnolin and Novas (2013).
Because of their unique anatomy, and because most of the available skeletons are of juvenile individuals preserved two-dimensionally on slabs, the phylogenetic position of scansoriopterygids remains debatable. In their original description of Scansoriopteryx, Czerkas and Yuan (2002) argued that this genus was a non-dinosaurian maniraptoran descended from Middle Triassic basal saurischians, and that it was some kind of “living fossil” that had persisted into Late Jurassic or Early Cretaceous times. Furthermore, Czerkas and Feduccia (2014) “agreed with Czerkas and Yuan (2002) that it was not even a dinosaur, but rather could have been derived from earlier arboreal archosaurs. However, these hypotheses rest on weak evidence, and most authors do not consider them to be viable proposals regarding scansoriopterygid affinities. On the other hand, because scansoriopterygids share a large number of features with maniraptoran theropods, authors agree that scansoriopterygids belong to Coelurosauria, and probably Maniraptora. Zhang et al. (2002) regarded scansoriopterygids as maniraptorans of uncertain relationships, whereas Turner et al. (2012), Godefroit et al. (2013a,b) and Xu et al. (2015) suggested that they were basal paravians. Zhang et al. (2008) were the first authors to suggest that scansoriopterygids formed the sister clade to Avialae. Because of their bizarre anatomy, the suggestion that scansoriopterygids are stem-avialans has a profound impact on reconstructions of the sequence of acquisition of typical avian traits. This issue was considered in some detail by several authors that supported avialan affinities for scansoriopterygids (Xu and Zhang, 2005; Hu et al., 2009; Choiniere et al., 2010; Xu et al., 2011; Foth et al., 2014; Cau et al., 2017; Foth and Rauhut, 2017). On the other hand, Xu et al. (2010) suggested that scansoriopterygids might be related to oviraptorosaurs, a hypothesis cladistically supported by Agnolin and Novas (2013) and later endorsed by O’Connor and Sullivan (2014).
Zhang et al. (2008) and Foth et al. (2014) defended the putative avialan affinities of scansoriopterygids based on a combination of characters that is also shared with oviraptorosaurs, and especially with caenagnathids and avimimids (Kurzanov, 1981; Osmólska et al., 2004; Funston et al., 2017; see Agnolin and Novas, 2013), including: dentary and maxillary teeth devoid of serrations and with roots that are subcircular in cross-section, relatively few caudal vertebrae, fused metatarsal cap, and pedal phalanx II-2 longer than phalanx II-1.
Furthermore, Zhang et al. (2008) also suggested that a reversed hallux with a well-developed ungual phalanx is a feature shared between birds and scansoriopterygids. However, as indicated by Agnolin and Novas (2013), in Epidendrosaurus (the only known scansoriopterygid in which the hallux is preserved) the hallux is laterally oriented and its plantar surface is posteriorly directed, a plesiomorphic feature that occurs in most theropods (Zhang et al., 2002). Zhang et al. (2008) also maintained that an elongate preacetabular process of the ilium with a strongly convex anterior margin was a character shared with birds and scansoriopterygids. However, the ilium in scansoriopterygids is strongly apomorphic, being dorsoventrally low and having a finger-like preacetabular blade. We interpret this anatomy as unique to scansoriopterygids (Zhang et al., 2008) and different from the avialan condition (Novas, 2004). Finally, Zhang et al. (2008) reported one feature that seems to be shared by avialans and scansoriopterygids, but that is not present in oviraptorosaurs: humerus longer than femur. This may stand as a potential synapomorphy uniting the first two clades.
On the other hand, as stated by Agnolin and Novas (2013), scansoriopterygids share an important number of synapomorphic features with oviraptorosaurs, including short and high skull with reduced nuchal and parietal crests and enlarged fenestrae, small and subtriangular maxilla, external nares positioned high on the skull and with a sub-vertical main axis, dorsally convex dentary with a prominent chin, and teeth cylindrical, procumbent and lacking serrations. Furthermore, scansoriopterygids share with oviraptorosaurs some anatomical traits that are uncommon among theropods. For example, Epidexipteryx and such oviraptorosaurs as Nomingia share a distally tapering pygostyle-like structure formed by unfused caudals at the end of the tail (Barsbold et al., 2000; Funston et al., 2017; also present in therizinosaurs, see Xu et al., 2003).
It is important to note that scansoriopterygids retained striking plesiomorphic traits in their pectoral girdle, including a caudoventrally oriented scapulocoracoid glenoid, a subrectangular coracoid with a reduced biceps tubercle, and a distally expanded scapular blade. These features also shared by oviraptorosaurs as Avimimus and Heyuannia (Lü et al., 2005; Funston et al., 2017), among other non-paravian/pennaraptoran theropods.
The Dinosaur to Bird Transition: Selected Anatomical Topics
Recent studies have recognized that acquisition of bird flight involved a large number of anatomical changes related to coracoid shape, increasing muscle volume, supracoracoideus muscle development, the length of both the forelimb feathers and the forelimb itself, and remige geometry, among others (e.g., Ostrom, 1978; Baier et al., 2007; Longrich et al., 2012; Xu et al., 2014; Dececchi et al., 2016; Wang et al., 2017; Voeten et al., 2018). Anatomical and functional information provided by new basal maniraptorans, including specimens from both China and Argentina, needs to be incorporated into scenarios for the early steps in the evolution of powered bird flight. Below, we make some brief comments about selected anatomical topics related to the origin of flight.
Sternum, Uncinate Processes and Gastralia: The Paravian Thoracic Cage
Traditionally, an ossified sternum has been among the derived anatomical features considered to represent the most important prerequisites for avian flight (Ostrom, 1976; Feduccia, 1996). In extant volant birds the sternum shows a well-developed longitudinal keel anchoring important muscles (i.e., M. pectoralis and M. supracoracoideus), which drive the upstroke and downstroke during flight performance (Ostrom, 1976). Because of their importance, sternal morphology and evolution in the early stages of avian history are hotly debated topics (Zheng et al., 2012, 2014; Foth, 2014).
Paired ossified sternal plates have been reported in several dinosaurs, including some theropods (Galton and Upchurch, 2004; Norman et al., 2004). Among basal coelurosaurs, the ornithomimosaur Pelecanimimus polyodon (Perez-Moreno et al., 1994) has a pair of ossified sternal plates, but these elements are unknown in other members of the Ornithomimidae or in more basal theropods. However, well-preserved and articulated specimens of other ornithomimids reveal an empty space between the cranialmost gastralia and the coracoids, suggesting the presence of a cartilaginous sternum (Sternberg, 1933; Osmólska et al., 1972; Nicholls and Russell, 1985; Kobayashi et al., 1999). On this basis, Sereno et al. (2004) considered ornithomimosaurs to represent an initial step among basal coelurosaurs toward the avian condition, having paired, fully ossified, and anteroposteriorly extensive sternal plates (reported sternal plates for more basal theropods, including Baryonyx and Carnotaurus are dubious, at best; Paul, 2002). Unambiguous sternal ossification occurs among basal maniraptoriforms in the clade Alvarezsauridae, in which the sternal plates are fused at the midline and bear a longitudinal keel (Perle et al., 1994; Xu et al., 2011). A fully ossified sternum is present in oviraptorosaurs (Zhou and Wang, 2000), but some interesting differences exist regarding sternal morphology, degree of fusion, and development of a sternal keel. For example, the sternal plates are small, rounded and separate in Caudipteryx and the probable oviraptorosaur Epidexipteryx (Zhou and Wang, 2000; Zhang et al., 2008). They are rectangular in Oviraptor and Ingenia (Barsbold, 1983), whereas in Heyuannia (Barsbold, 1983) the sternal plates are fused to each other along the midline and bear a low midline keel. This variation in shape and morphology of the sternal plates within a single pennaraptoran subclade constitutes a cautionary note for evolutionary inferences regarding the presence or absence, as well as the general morphology, of the sternum in the various taxa involved in the dinosaur-bird transition (Figure 9).
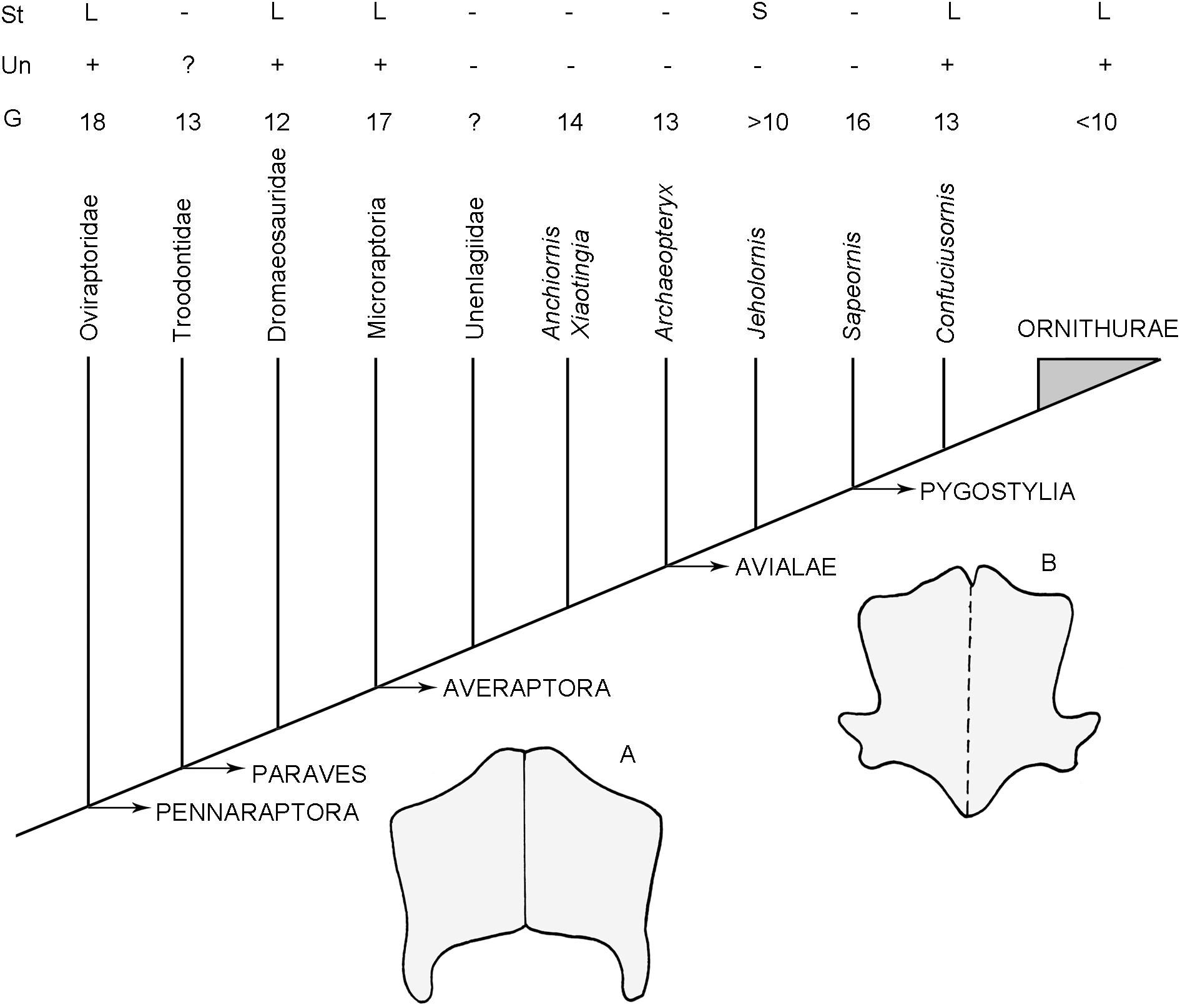
Figure 9. Simplified cladogram showing the phylogenetic relationships of derived coelurosaurs following the proposal of Agnolin and Novas (2013). The figure indicates the number of gastral pairs (G), presence or absence of uncinate process (Un) and relative development of the sternum (St) in avian-line theropods. (A,B) Gross morphology of the sternum of: (A) the dromaeosaurid Linheraptor exquisitus, (B) the pygostylian Eoconfuciusornis zhengi (modified from Zheng et al., 2012). G, number of pairs of gastralia, L, present and large, S, present but small; St, Sternum; Un, Uncinate process; ?, uncertain condition; +, presence; –, absence.
Among eudromaeosaurids the large sternal plates may be separate (Barsbold, 1983; Norell and Makovicky, 1997, 1999; Xu et al., 1999b, 2000; Burnham et al., 2000; Godfrey and Currie, 2004) or fused (Xu et al., 2010). In Microraptor the sternal plates are firmly fused to each other (Xu et al., 2003), and in one unpublished microraptorian specimen the sternum has what appears to be a incipient midline keel (Agnolin and Novas, 2013). In troodontids and anchiornithines, on the other hand, an ossified sternum appears to be absent (Xu and Norell, 2004; Zheng et al., 2014; Xu et al., 2017).
In basal birds the situation is puzzling. Zheng et al. (2014; see also O’Connor et al., 2014) proposed that in Archaeopteryx and Sapeornis the sternum was totally absent, as in anchiornithines and troodontids. However, Foth (2014; see also Lambertz and Perry, 2015) dismissed such an interpretation, suggesting that the apparent absence of the sternum in the above mentioned taxa was probably due to taphonomic bias and emphasizing that in Archaeopteryx the third to the eight thoracic ribs show distally expanded ends for articulation with the sternum. Furthermore, in specimens of Jeholornis and Microraptor the presence of sternal ossifications is variable (Xu et al., 2001, 2003; Zheng et al., 2014). However, some microraptorian specimens, including individuals of Sinornithosaurus and Microraptor, possess an ossified sternum that shows strong morphological similarities to that of avialans including Jeholornis and ornithothoracine birds on one hand, and to that of oviraptorosaurs and dromaeosaurids on the other. This observation suggests that a sternum was likely present in Archaeopteryx, Sapeornis, and Anchiornis, and probably also in troodontids, thus supporting Foth’s (2014) proposal. Because the sternum is one of the last elements to ossify, its absence in fossils may be explicable in many cases on the basis of ontogenetic and taphonomic biases (Foth, 2014).
The sternal keel, together with the surrounding parts of the sternum, anchors the Mm. supracoracoideus and pectoralis (Olson and Feduccia, 1979). The former attaches at the base of the keel and is connected to the humerus through the triosseal canal, passing over a pulley-like structure and constituting the main muscle driving the upstroke of the wing (Ostrom, 1976). The ventral adductor muscle M. pectoralis drives the downstroke, and originates from the most external portion of the sternal carina (Ostrom, 1976). Because Archaeopteryx (and also Anchiornis) lacked an ossified sternum and triosseal foramen, some authors have concluded that the wing musculature was reduced in these taxa, and in particular that the supracoracoideus must have been poorly developed due to the absence of a sternal keel (e.g., Ostrom, 1976; Ruben, 1991; Feduccia, 1996). This led some authors to assume that Archaeopteryx and non-avian theropods were unable to sustain flapping flight or take off from the ground, a hypothesis sustained by proponents of the arboreal theory of avian flight (Olson and Feduccia, 1979; Tarsitano, 1985; Vazquez, 1994). However, it is worth mentioning that the absence of an ossified sternum does not necessarily imply that the musculature was poorly developed. In this connection, extant tinamids and galliforms show reduced ossified sternum surfaces (Feduccia, 1996), and the main wing muscles anchor on the membrane that unites the ossified lateral trabeculae with the ossified midline keel of the sternum.
Recent studies by Claessens (2004) and Codd et al. (2005) indicate that structures such as the gastral basket and uncinate processes of the axial skeleton, in addition to the sternum, played a key role in the origin and evolution of the bird breathing system. It has been demonstrated that uncinate processes are ventilatory structures in birds, associated with inspiratory and expiratory movements; changes in thoracic volume, resulting from movements of the sternum and ribs, create pressure differences that drive lung ventilation (Zhang et al., 2014). Codd et al. (2005) pointed out that contraction of the M. appendicocostalis muscle, which attaches each uncinate process to the following rib during inspiration, assists the action of rotating the ribs cranially and the sternum ventrally. The uncinate processes are the insertion site of muscles that assist during inspiration and pull the sternum dorsally during expiration.
As indicated above, oviraptorids, dromaeosaurids, and microraptorians have large and wide sternal plates, and they also possess well-developed, ossified uncinate processes on their dorsal ribs (Paul, 2002; Xu, 2002). However, in more derived taxa, including unenlagiids, Anchiornis, Xiaotingia, and basal avialans such as Archaeopteryx, Jeholornis, and Sapeornis, the sternum is small (as is the case in Jeholornis) or not ossified, and the uncinate processes are totally absent (probably not ossified; Zhou and Zhang, 2002, 2003b; Mayr et al., 2007). This contrasts with the condition in more derived pygostylians (e.g., Confuciusornis, Enantiornithes, and Aves) in which the sternum is large and fully ossified, and uncinate processes are well developed on the dorsal ribs (Zhang et al., 2014). Thus, based on the distribution of the relevant features on phylogenetic trees, a single genetic switch probably controls the tandem ossification, or lack thereof, of the sternum and uncinate processes in pennaraptorans (Figure 9).
In all the above-mentioned taxa (including the avialan Archaeopteryx), the capability for active flight is debatable and far from certain, and flightlessness cannot be excluded (Nudds and Dyke, 2010; Novas and Agnolin, 2014; Feo et al., 2015; Lambertz and Perry, 2015). Thus, poor ossification and reduction of the uncinate processes and sternum may support an inference of poor flying capabilities for at least some paravians, in comparison to highly specialized modern birds.
The detailed study of Claessens (2004) demonstrated that the gastral basket played a dynamic role in respiration in the archosaur line (see also Perry, 1983; Carrier and Farmer, 2000). It has been hypothesized that in theropods active retraction and protraction of the gastralia produced ventilation of the abdominal air sac complex (Claessens, 2004), an important aspect of airflow pathways in the avian lung (Butler et al., 1988; Kuethe, 1988; Boggs et al., 1997; Codd et al., 2005). Furthermore, gastral elements may have also played a role in respiration by supporting the belly wall against inward collapse (Perry, 1983; Claessens, 2004).
A high number of gastral elements has been reported in non-pygostylian theropods. In most taxa, including oviraptorids, there are 18 pairs of gastral elements (Dal Sasso and Maganuco, 2011; Lambertz and Perry, 2015). In contrast, in paravians this number is variable, with 17 gastral pairs in microraptorians, 12 in dromaeosaurids, 13–14 in Anchiornis and Archaeopteryx, and 16 in Sapeornis. Pygostylians more derived than Sapeornis consistently have fewer than 14 pairs of gastral elements (Lambertz and Perry, 2015; Figure 9).
We concur with Lambertz and Perry (2015) that the number of gastral elements in derived pygostylian birds became reduced in combination with an increase in the caudal extent of the sternum. However, we are not able to find a clear correlation between the number of gastralia and sternal development in more basal taxa. As indicated above, a large number of gastral elements is present both in basal paravians in which an enlarged sternum is clearly present (e.g., Microraptor) and in those having poorly ossified sternal elements such as Archaeopteryx and Jeholornis. Thus, we think that reduction in the number of gastral elements may not be entirely correlated with the size of the sternum, and may instead correlate at least partially with some other, still unknown, anatomical features.
Forelimb Posture and Soft Tissues
Several authors suggested that the acquisition of typical avian traits in the theropod forelimb skeleton can be correlated with changes in associated soft structures, such as patagial skin and muscles (Sereno and Chenggang, 1992; Xu et al., 2014; Wang et al., 2017). For example, the capacity for coordinated flexion of the wrist and elbow was considered unique to living birds by Vazquez (1992, 1994). This author concluded that this type of flexion depends mainly on the action of a large number of tendons located within the propatagium, and consequently should be considered unique to modern birds and intimately related to powered flight (Vazquez, 1994). As indicated by Sereno and Chenggang (1992), in birds the wing most often assumes this retracted (flexed) position upon death, apparently due to passive contraction of forelimb ligaments. Because the propatagium participates in controlling the flexion of the wrist and elbow, the presence of a flexed forelimb in a fossil theropod may indicate the existence of a propatagium. Maniraptorans and some other basal tetanurans display a relatively large capacity of forelimb flexion, though most of them were clearly non-volant, and in some cases (e.g., Caudipteryx) specimens with preserved propatagia are known (see Agnolin and Novas, 2013; Feduccia and Czerkas, 2015; White et al., 2015; Wang et al., 2017). Xu et al. (2014) mentioned that lateral folding capability may initially have been a synapomorphy of Tetanurae (Figure 10). However, the presence of folded forelimbs in the Triassic basal dinosaur Guaibasaurus (Agnolin and Martinelli, 2012), as well as in pterosaurs (Kellner et al., 2009), indicates that automatic flexion was a widespread condition among ornithodirans. Moreover, Hutson and Hutson (2014) have shown that living crocodiles also exhibit automatic wrist folding guided mainly by soft tissues (see also Walker, 1972). This raises the interesting question of whether co-joined flexion of the wrist and elbow may represent an ancestral archosaurian mechanism that became strongly enhanced in theropods on the line to birds. It is possible that automatic folding and the propatagium evolved before the origin of birds/wings/flight.
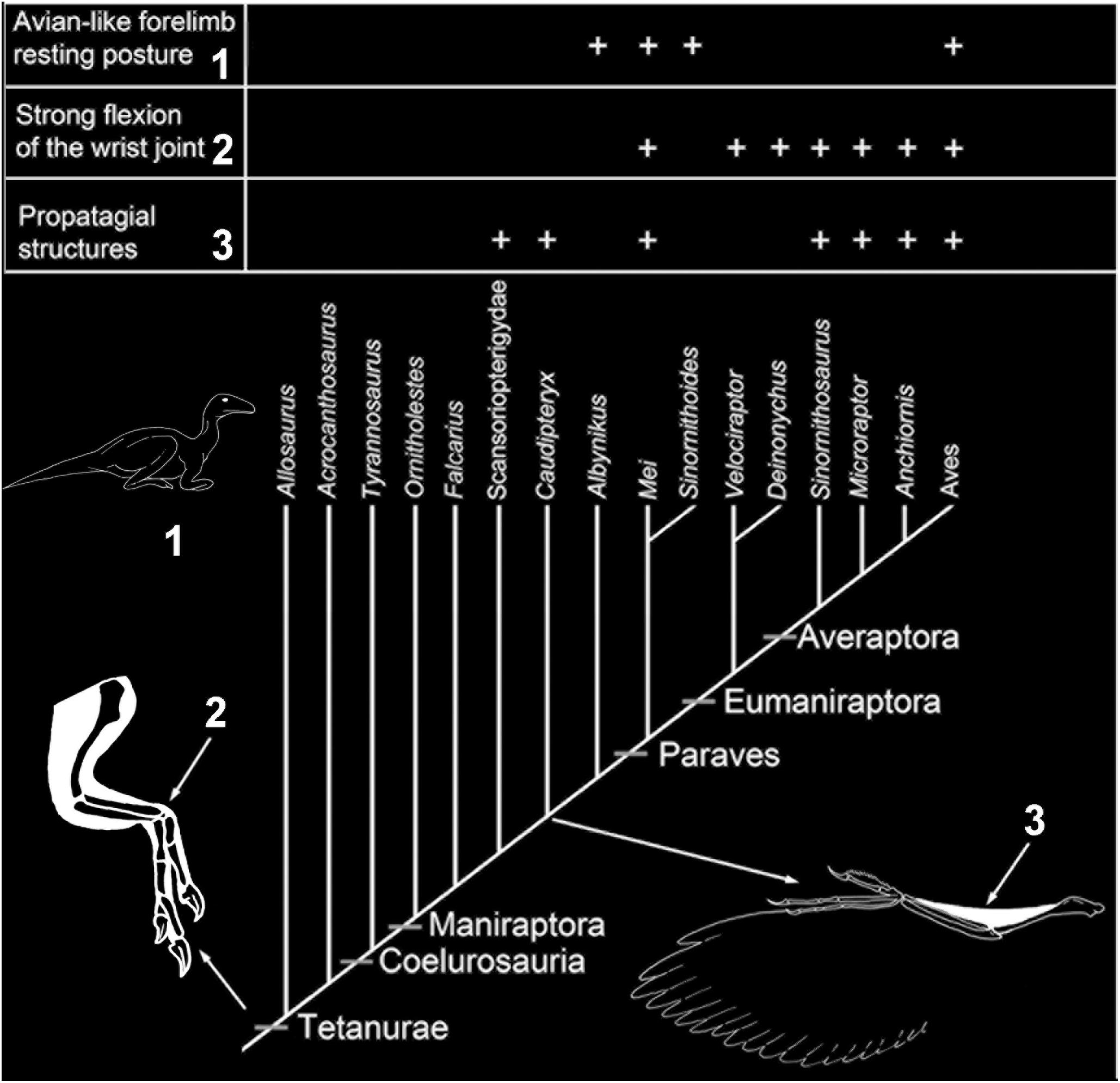
Figure 10. Simplified phylogenetic scheme for Tetanurae, showing the distribution of flexion of the wrist joint and propatagial structures among theropods (modified from Chimento et al., 2017).
Nevertheless, it is well-known that maniraptorans possess a larger degree of forelimb flexion than basal tetanurans, and that the capacity for flexion has been further enhanced in birds having powered flight (see Xu et al., 2014; White et al., 2015). Hopp and Orsen (2004) proposed that diversity in the range of forelimb flexion among maniraptorans may be a consequence of managing forelimb feathers used in brooding, and Chatterjee and Templin (2004) related the swivel wrist joint to climbing behavior (but see Dececchi and Larsson, 2011). The amount of possible forelimb flexion is not homogeneous among derived paravians and basal avialans (Sereno and Chenggang, 1992), and some distinctive patterns can be inferred on the basis of the fossil record.
Longrich et al. (2012; see also Foth et al., 2014; Nudds, 2014) explored wing shape in Anchiornis, Archaeopteryx and modern birds. These authors proposed that a profound change in wing shape took place in the course of early avialan evolution, prior to the origin of pygostylians. Although some of the anatomical interpretations originally proposed by Longrich et al. (2012) were later questioned (Foth et al., 2014; Nudds, 2014), we observe that the wing shape seen in Anchiornis and Archaeopteryx is different from that of more derived birds (e.g., Confuciusornis, Sapeornis, Archaeornithura, Jeholornis). In basal paravians and avialans such as Archaeopteryx, Anchiornis, and Zhenyuanlong Lü and Brusatte, 2015 the wings are relatively large and wide, with relatively short rectrices, a rounded alar contour, and a convex leading margin. Fossils of these taxa (e.g., Archaeopteryx, Anchiornis) consistently exhibit forelimbs with a restricted capacity for folding, having the main axis of the hand located at approximately 90° with respect to that of the forelimb (Sereno and Chenggang, 1992). In more derived birds, including Jeholornis and enantiornithines (Sereno and Chenggang, 1992), the remigial feathers become notably elongate (especially the outer ones), and the hands are folded at an acute angle over the forelimbs. In available specimens of Confuciusornis, Sapeornis, and Jeholornis, in fact, the angle between the main axes of the hand and forelimb is always lower than 90°, indicating that the forelimbs were capable of folding to a greater degree than was possible in more basal avialans. It is possible that the wing shape seen in more derived avialans may be a consequence of having forelimbs with a capacity for hyperflexion. We are not certain about the exact implications of this important change in the degree of wing folding, but it is possible that enhanced folding capabilities mirrored improvements in the wing stroke and in locomotor performance in the air.
Feathers: Fossil Record and Phylogenetic Distribution
Fossils with exquisitely preserved plumage from the Late Jurassic and Early Cretaceous of northeastern China provide valuable information about feather evolution (Ji et al., 1998, 2001; Xu et al., 1999b, 2000, 2001; Zhang et al., 2010). Diverse four-winged dinosaurs from Liaoning have recently been described, providing new information about the morphology and structure of the feathered forelimbs, hindlimbs and tail (e.g., Xu et al., 2003; Hu et al., 2009; Han et al., 2014).
Filamentous integumentary structures (probably plesiomorphic feathers or protofeathers; see Xu et al., 2001) and contour feathers are widespread among theropods. Filamentous contour feathers have been documented in multiple theropod taxa, including the basal tetanuran Sciurumimus (Rauhut et al., 2012), Compsognathidae (Ji and Ji, 1996), Tyrannosauroidea (Xu et al., 2004, 2012), Ornithomimidae (Zelenitsky et al., 2012; van der Reest et al., 2016), Therizinosauroidea (Xu et al., 1999a, 2009), Troodontidae (Xu et al., 2017), Microraptora (Xu et al., 2000), and Anchiornithinae (Hu et al., 2009). Due to their low preservation potential, it is possible that feathers were also in fact present in more basal theropod dinosaurs, as they have been reported in ornithischians (Mayr et al., 2002; Zheng X.T. et al., 2009; Godefroit et al., 2014) and also probably pterosaurs (known as pycnofibers; Kellner et al., 2009).
With the aim of discussing the evolution of feather types among basal paravians, we have mapped feather traits on the phylogeny of Agnolin and Novas (2013), and on the latest version of the TWiG phylogeny (Gianechini et al., 2017). We have also analyzed the patterns of feather evolution implied by additional tree topologies, including those of Godefroit et al. (2013a), Foth and Rauhut (2014), Lefèvre et al. (2017), and Xu et al. (2017). It becomes obvious that the inferred sequence of acquisition of feather characteristics depends profoundly on the choice of phylogenetic hypothesis (Figure 11).
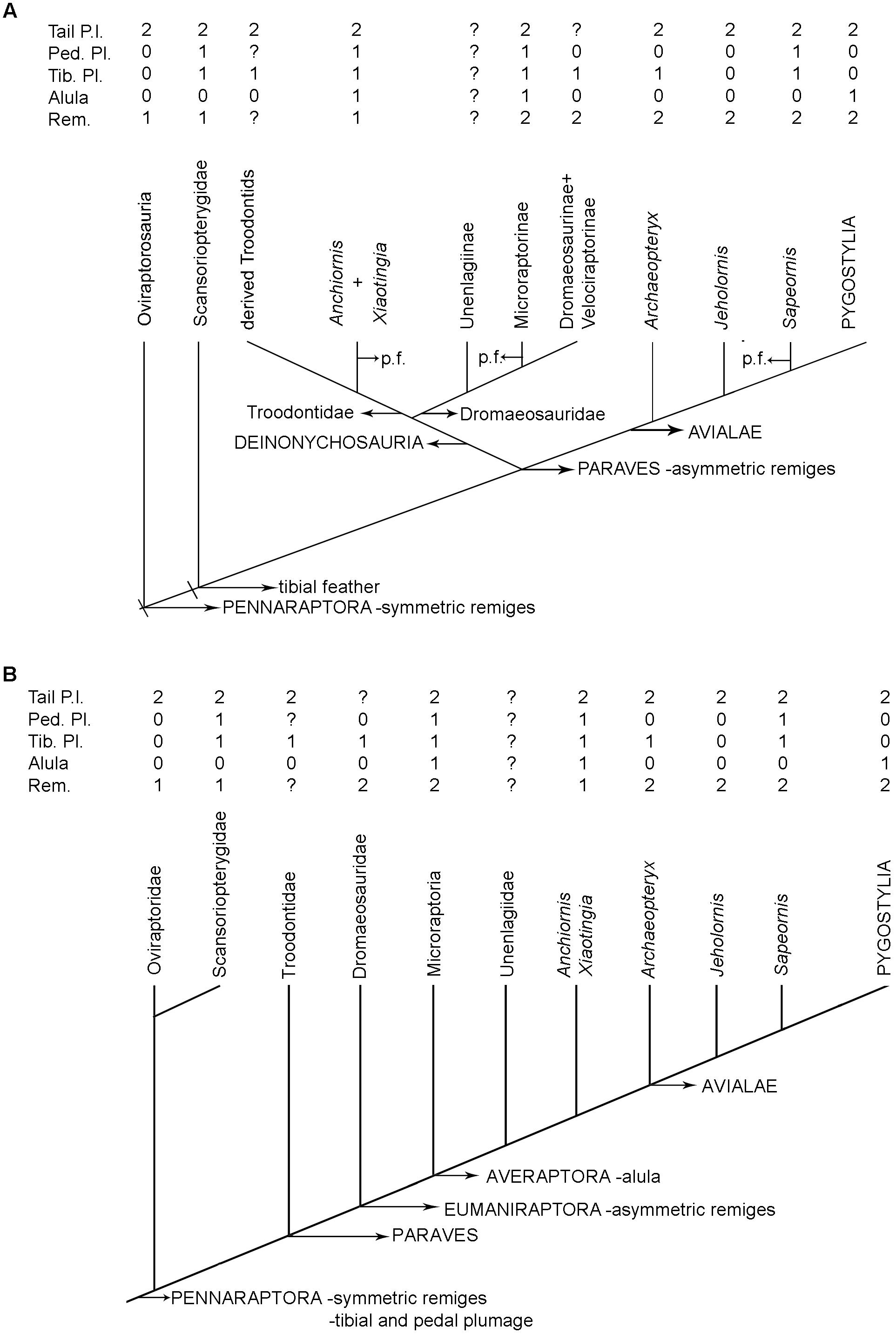
Figure 11. Simplified cladograms showing the distribution of feathers among derived coelurosaurs following the proposals of: (A) Gianechini et al. (2017); and (B) Agnolin and Novas (2013). Tail Pl., tail plumage; Ped. Pl., pedal or metatarsal plumage; Tib. Pl., tibial plumage; Rem., forelimb remiges; ?, uncertain condition; 0, absence; 1, presence of plumulaceous feathers; 2, presence of pennaceous feathers.
Preserved remigial feathers are known not only among short and long-armed pennaraptorans (e.g., Agnolin and Novas, 2013; Foth et al., 2014; Lefèvre et al., 2017), but also in ornithomimids (Zelenitsky et al., 2012), suggesting that elongate remiges have a single origin at the base of the coelurosaurian tree (Agnolin and Novas, 2013) (Figure 11).
Foth et al. (2014) proposed that asymmetrical remiges evolved convergently in Dromaeosauridae and Avialae. This interpretation is supported by the phylogenetic hypothesis defended by Turner et al. (2012), but not by the cladogram obtained by Agnolin and Novas (2013). The latter phylogeny implies that asymmetrical remiges evolved only once, at the base of the clade Eumaniraptora, as also proposed by Lefèvre et al. (2017) (Figure 11).
The presence of an alula in both Microraptor and Avialae has been proposed to be a result of convergent evolution (Senter et al., 2004). Nevertheless, the recent discovery of an alula in anchiornithines (Hu et al., 2018) supports the proposal of Agnolin and Novas (2013) that this character may be widespread among Averaptorans (Figure 11).
In sum, it is possible that forelimb feathers, including a functional alula, may be more widespread than previously thought, including in taxa such as unenlagiids in which they have never been reported. It is also possible that this combination of traits was present in non-avialan theropods (or at least, taxa that were not capable of sustained flight). Accordingly, the origin and early evolution of forelimb plumage is far from being well-understood (Figure 11).
Elongated tibial feathers were first described in Microraptor (Xu et al., 2003), but more recently have been found in a large variety of basal paravians and basal birds (Zheng et al., 2013) including Archaeopteryx (Foth et al., 2014). The presence of hindlimb plumage has been proposed as synapomorphic for Eumaniraptora (Foth et al., 2014), Paraves (Lefèvre et al., 2017), or Averaptora (Agnolin and Novas, 2013). Foth et al. (2014; see Turner et al., 2012) proposed that pedal or metatarsal plumage was convergently acquired in three paravian clades, namely Microraptora, Anchiornithinae, Sapeornis, and more derived birds. Contrarily, Lefèvre et al. (2017) proposed that pennaceous metatarsal feathers appeared only once, at the base of Paraves. Based on Agnolin and Novas’s (2013) phylogenetic scheme, metatarsal plumage may have appeared two times among theropods, once in Scansoriopterygidae and once in Averaptora. Nevertheless, it is possible to infer that this trait was widespread among theropods, and may have been acquired in concert with tibial plumage at the base of the coelurosaurian tree (Figure 11).
Tail plumage is highly variable among coelurosaurs. Oviraptorosaurs exhibit elongated symmetrical rectrices along the distal half of the tail (Ji et al., 1998; Xu et al., 2010), and asymmetric pennaceous feathers have recently been documented in a troodontid tail (Xu et al., 2017) and at the lateral margins of the tail in Archaeopteryx (Xu et al., 2017). Elongate rectrices attached to the distal half of the tail have been reported in the microraptorians Microraptor and Changyuraptor (Li et al., 2012; Han et al., 2014).
The plumulaceous feathers on the tail of “Eosinopteryx” are short, in contrast with the pennaceous rectrices present in other Anchiornithinae including Anchiornis, “Aurornis,” and “Serikornis,” whereas in Caihong large rectrices are attached to either side of the caudal series to form a frond-shaped tail similar to that of Archaeopteryx (Hu et al., 2018). It is possible that the differences in tail feathering among Anchiornis specimens may represent variation across the molting stages of a single taxon.
Large rectrices attached to either side of the caudal series, as in Archaeopteryx, may be hypothesized to have been present in the unenlagiid Buitreraptor, based on some anatomical details of the caudal vertebrae (see details in Motta et al., 2018). Among Avialae the tail plumage is even more variable, being fan-shaped, frond-like or composed at least partly of “rachis-dominated” feathers (see O’Connor et al., 2012, 2016).
The origin and function of tail plumage in basal birds is still debated. Some authors (Gatesy and Dial, 1996) proposed that tail feathers had a locomotor role, acting as lift-producing surfaces during flight. Others interpreted tail feathers as having assisted with maintaining body balance when perching (Chuong et al., 2013; Agnolin et al., 2017), or with stabilizing the trunk when climbing (Hu et al., 2015). Still other authors suggested that elongate tail feathers might have been associated with sexual display or visual communication (Chiappe et al., 1999, 2008; Zhang and Zhou, 2000; Clarke et al., 2006; Li et al., 2006, 2012; Zheng et al., 2007; O’Connor et al., 2011b, 2012, 2013; Padian and Horner, 2011). These possibilities are not mutually exclusive, and it seems reasonable to suppose that different tail feather functions complemented and interacted with one another in the lives of these animals. Based on available information it is difficult to define a clear pattern of tail feather evolution along the avialan lineage, but the evidence indicates that morphologically disparate feather types (i.e., plumaceous, symmetric pennaceous, asymmetric pennaceous, and “rachis-dominated”) evolved before the origin of birds, and were eventually co-opted for aerodynamic functions in volant forms (Figure 11).
In sum, some of the diverse phylogenetic analyses put forward by different authors indicate that each feather type may have evolved convergently two or three different times, whereas other analyses indicate a single origin for flight feathers. For this reason, the origin and early evolution of the different feather types is far from being well-known and largely depends on the phylogenetic scheme adopted.
Glenoid Cavity: Implications for the Origin of Powered Flight
The pectoral girdle of extant birds shows a unique combination of characters that are crucial for flight. Among these main characteristics are the strut-like scapula and coracoid, the narrow angle between the scapula and coracoid, the large size of the acrocoracoid process, the presence of a triosseal canal, the development of a sternal keel, and modifications in the furcula, supporting the powerful muscles that facilitate the wing stroke (see Ostrom, 1974, 1976; Olson and Feduccia, 1979).
Because there is virtually complete agreement that the shape and orientation of the glenoid are features that play a key role in determining the range of forelimb movements (e.g., Ostrom, 1976; Jenkins, 1993; Baier et al., 2007), we focus below on the anatomy of this region of the pectoral girdle in both living birds and basal paravians.
The glenoid cavity is formed by the scapula and coracoid in birds and basal paravians. This cavity is relevant to avian flight because it is the site where the humerus pivots, allowing a wide repertoire of forelimb movements. In volant neognathans the glenoid is an ovoid cavity oriented mostly in a latero-dorsal direction (Feduccia, 1993). Moreover, the cavity is predominantly aligned horizontally, such that the angle formed between the main axis of the cavity and the horizontal is less than 10°. The glenoid cavity has two different anatomical sections: one coracoidal, and one scapular. These sections differ in size and orientation. The scapular section is small, faces laterally and slightly posterodorsally, and has a dorsoventrally oriented major axis; the coracoidal section is much larger, faces dorsolaterally and slightly anteriorly, and has a predominantly horizontal major axis (Figure 12). The coracoidal section of the glenoid receives most of the humeral head. This configuration allows the wing a wide dorsoventral range of movement, but a restricted anteroposterior range (Dial et al., 1991; Jenkins, 1993; Poore et al., 1997).
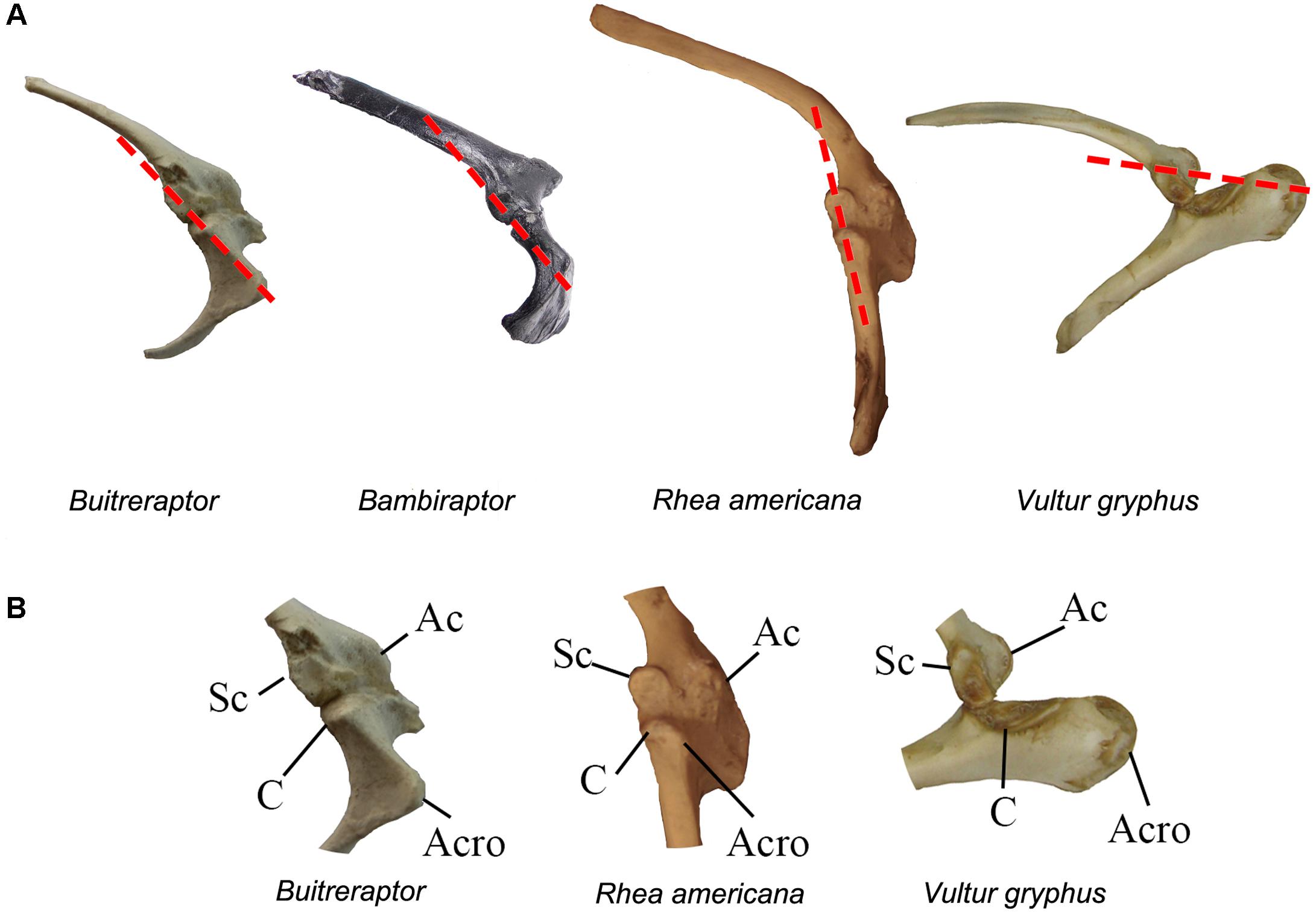
Figure 12. (A) Lateral view of the right scapulocoracoid in selected basal and derived paravians. The dotted lines indicate the inclination of the major axis of the glenoid cavity. (B) Detail of the glenoid of the right scapulocoracoid of selected paravians in lateral view. Not to scale. C, coracoidal portion of the glenoid; Sc, scapular portion of the glenoid, Ac, acromion; Acro, acrocoracoid or biceps tubercle.
The glenoid cavity of flightless ratites (e.g., Rhea, Struthio) differs notably from those of volant neornithines in being ovoidal or subcircular in contour, being mostly laterally oriented, and having a main axis that is subvertical (i.e., oriented at approximately 30° with respect to the vertical plane). Furthermore, the coracoidal and scapular sections of the glenoid are sub-equal in size and shape (Figure 12).
In basal avialans (e.g., Archaeopteryx, Anchiornis) and basal paravians (e.g., Buitreraptor) the glenoid facet is laterally oriented. Furthermore, the scapular and coracoidal sections of the glenoid are subequal in size and aligned in the same vertical plane, as in ratite birds (Figure 12). This corroborates Feduccia’s (1986) assertion that ratites are the best living analogs for understanding the morphology and function of the pectoral girdle in non-avian theropods.
Furthermore, ratite birds resemble most basal paravians, including Archaeopteryx, Anchiornis, Bambiraptor, and Buitreraptor, in that the acrocoracoid process is represented by a small bump or process known as the biceps tubercle, being notably underdeveloped with respect to neognathan birds (see Ostrom, 1976). The morphology of this tubercle largely dictates the course of the tendon of the main humerus protractor (and rotator), the M. supracoracoideus, whose action is essential to the wing beat of flying birds (Ostrom, 1976; Dial et al., 1991; Baier et al., 2007).
Based on anatomical traits of the pectoral girdle, as well as personal observations of ratite behavior in the field (Novas et al., 2016; Lo Coco et al., 2018), the wings of ratites move predominantly in an anteroposterior direction, lacking the complex and largely dorsoventral wing excursion seen in living neognathans (see Chin et al., 2017; Stowers et al., 2017). Because of the strong similarities in pectoral girdle structure between ratites on the one hand and basal avialans and paravians such as Archaeopteryx and Buitreraptor on the other, it can be inferred that the main forelimb movements were from anterodorsal to posteroventral in the latter group of taxa. Accordingly, their forelimb movements differed from those of flying neognathans, and basal avialans including Archaeopteryx were very probably not capable of powered flight. This is in agreement with other lines of evidence, including feather morphology and bone geometry (Feo et al., 2015; Voeten et al., 2018), that suggest flight capability was low or absent in basal avialans.
Conclusion
As indicated throughout the text, a consensus about the phylogenetic relationships of paravians is far from being reached. For this reason, knowledge of the steps toward the acquisition of anatomical and behavioral traits contributing to the origin of avian flight is still uncertain and in a state of flux. We were not able to find a clear sequence of evolutionary novelties. Also, the fossil record of the dinosaur-bird transition is sparse, and new fossils are needed to bridge the gaps among the various paravian clades.
It has long been known that much experimentation and convergence occurs when a new “adaptive zone” is being crossed into (Gould, 1989). It is possible that the adaptive breakthrough of evolving from a non-avian dinosaur into a bird was accompanied by much convergence in closely related lineages on bird-like features (Feduccia, 2013). The degree of evolutionary experimentation and convergence on “bird-ness” demonstrated by recent discoveries, especially those from China, may ensure that a consensus on phylogenetic relationships remains elusive.
Along similar lines, the nature of the early paravian and avialan radiation is totally uncertain, as are its center of origin and dispersal routes. Although some authors propose Laurasia, and more precisely eastern Asia, as the possible primal area of paravian diversification (Xu and Zhang, 2005; Foth and Rauhut, 2017), the evidence is so patchy and inconclusive that any proposal is highly speculative. As recognized early on by Darwin (1859), the fossil record is notably incomplete, and is particularly biased in the case of paravian theropods. For example, recent finds in Brazil indicate that ribbon-like feathers were geographically widespread during the Mesozoic (Carvalho et al., 2015), and the identification of ribbon-like feathers in the Middle Jurassic (Callovian) of western Asia (Agnolin et al., 2017) reinforces the idea of a still unknown radiation of early feathered paravians. Furthermore, the intermingled sequence of Asiatic, North American, South American, European, and Malagasy taxa in the steps toward Avialae reinforces the current impossibility of identifying a center of origin for paravians.
The aim of the present contribution was to analyze some anatomical points and details that possibly have significant functional implications. We think that future studies will need to examine morphology in a holistic, functional manner to draw inferences regarding flight, rather than focusing on just one anatomical element/region such as the wing. Such integrative analyses will be urgently needed to understand the early steps in the evolution of bird flight.
Finally, there is a strong need for anatomical and functional studies of living archosaurs, in order to better understand basal paravians. For example, the recent discovery by Hutson and Hutson (2014) that crocodiles exhibit automatic wrist folding guided mainly by soft tissues, a feature previously thought to be exclusive to birds, represents an important benchmark in the progress of detailed anatomical studies on extant archosaurs. Furthermore, the strong similarities between ratites and early paravians in pectoral girdle morphology invite extensive and detailed studies of the anatomy of this region in cassowaries, emus, and ostriches. We hope such studies will shed light on the early steps in avian origins and the acquisition of flight.
Author Contributions
All authors listed have made a substantial, direct and intellectual contribution to the work, and approved it for publication.
Conflict of Interest Statement
The authors declare that the research was conducted in the absence of any commercial or financial relationships that could be construed as a potential conflict of interest.
Acknowledgments
We thank S. Perry for sharing important bibliographic material. We thank M. Ezcurra and D. Pol for sharing information on specimens. We thank N. Chimento, G. Lio, S. Rozadilla, M. Aranciaga, J. D’Angelo, A. Martinelli, and A. Gentil for discussion and comments on the ideas expressed on present manuscript, and N. Cazzaniga for helping us with diverse nomenclatorial and taxonomic issues. Special thanks to the Editor CS, an anonymous reviewer, and SGL for their enlightening comments on the manuscript.
Supplementary Material
The Supplementary Material for this article can be found online at: https://www.frontiersin.org/articles/10.3389/feart.2018.00252/full#supplementary-material
References
Agnolin, F., and Novas, F. E. (2013). Avian Ancestors: A Review of the Phylogenetic Relationships of the Theropods Unenlagiidae, Microraptoria, Anchiornis and Scansoriopterygidae. Berlin: Springer Science & Business Media. doi: 10.1007/978-94-007-5637-3
Agnolin, F. L., Ezcurra, M. D., Pais, D. F., and Salisbury, S. W. (2010). A reappraisal of the cretaceous non-avian dinosaur faunas from Australia and New Zealand: evidence for their gondwanan affinities. J. Syst. Palaeontol. 8, 257–300. doi: 10.1080/14772011003594870
Agnolin, F. L., and Martinelli, A. G. (2012). Guaibasaurus candelariensis (Dinosauria, Saurischia) and the early origin of avian-like resting posture. Alcheringa 36, 263–267. doi: 10.1111/j.1469-185X.2009.00094.x
Agnolin, F. L., and Novas, F. E. (2011). Unenlagiid theropods: are they members of the Dromaeosauridae (Theropoda, Maniraptora)? An. Acad. Bras. Ciênc. 83, 117–162. doi: 10.1590/S0001-37652011000100008
Agnolin, F. L., Rozadilla, S., and de Souza Carvalho, I. (2017). Praeornis sharovi Rautian, 1978 a fossil feather from the early Late Jurassic of Kazakhstan. Hist. Biol. 1–5. doi: 10.1080/08912963.2017.1413102
Alexander, D. E., Gong, E., Martin, L. D., Burnham, D. A., and Falk, A. R. (2010). Model tests of gliding with different hindwing configurations in the four-winged dromaeosaurid Microraptor gui. Proc. Natl. Acad. Sci. U.S.A. 107, 2972–2976. doi: 10.1073/pnas.0911852107
Averianov, A., and Sues, H. D. (2016). Troodontidae (Dinosauria: Theropoda) from the upper cretaceous of uzbekistan. Cretac. Res. 59, 98–110. doi: 10.1016/j.cretres.2015.11.005
Averianov, A. O., and Sues, H. D. (2007). A new troodontid (Dinosauria: Theropoda) from the cenomanian of uzbekistan, with a review of troodontid records from the territories of the former Soviet Union. J. Vertebr. Paleontol. 27, 87–98. doi: 10.1671/0272-4634(2007)27[87:ANTDTF]2.0.CO;2
Baier, D. B., Gatesy, S. M., and Jenkins, F. A. (2007). A critical ligamentous mechanism in the evolution of avian flight. Nature 445, 307–310. doi: 10.1038/nature05435
Barsbold, R., Osmólska, H., Watabe, M., Currie, P. J., and Tsogtbaatar, K. (2000). A new oviraptorosaur [Dinosauria, Theropoda] from mongolia: the first dinosaur with a pygostyle. Acta Palaeontol. Pol. 45, 97–106.
Benson, R. B., Rich, T. H., Vickers-Rich, P., and Hall, M. (2012). Theropod fauna from southern Australia indicates high polar diversity and climate-driven dinosaur provinciality. PLoS One 7:e37122. doi: 10.1371/journal.pone.0037122
Boggs, D. F., Jenkins, F. A. Jr., and Dial, K. P. (1997). The effects of the wingbeat cycle on respiration in black-billed magpies (Pica pica). J. Exp. Biol. 200, 1403–1412.
Bonaparte, J. F. (1999). Tetrapod faunas from South America and India: a palaeobiogeographic interpretation. Proc. Natl. Acad. Sci. U.S.A. 65, 427–437.
Brissón Egli, F., Aranciaga Rolando, A. M., Agnolín, F. L., and Novas, F. E. (2017). Osteology of the unenlagiid theropod Neuquenraptor argentinus from the late cretaceous of patagonia. Acta Palaeontol. Pol. 62, 549–562. doi: 10.4202/app.00348.2017
Brusatte, S. L., O’Connor, J. K., and Jarvis, E. D. (2015). The origin and diversification of birds. Curr. Biol. 25, R888–R898. doi: 10.1016/j.cub.2015.08.003
Burnham, D. A. (2004). “New information on Bambiraptor feinbergi (Theropoda: Dromaeosauridae) from the late cretaceous of Montana,” in Feathered Dragons: Studies on the Transition from Dinosaurs to Birds, eds P. J. Currie, E. B. Koppelhus, M. A. Shugar, and J. L. Wright (Bloomington: Indiana University Press), 67–111.
Burnham, D. A., Derstler, K. L., Currie, P. J., Bakker, R. T., Zhou, Z., and Ostrom, J. H. (2000). Remarkable New Birdlike Dinosaur (Theropoda: Maniraptora) From the Upper Cretaceous of Montana. Lawrence, KS: The University of Kansas Paleontological Contributions, 13.
Butler, J. P., Banzett, R. B., and Fredberg, J. J. (1988). Inspiratory valving in avian bronchi: aerodynamic considerations. Respir. Physiol. 72, 241–256. doi: 10.1016/0034-5687(88)90010-2
Calvo, J. O., Porfiri, J. D., and Kellner, A. W. (2004). On a new maniraptoran dinosaur (Theropoda) from the Upper Cretaceous of Neuquén, Patagonia, Argentina. Arquivos Museu Nac. 62, 549–566.
Carrier, D. R., and Farmer, C. G. (2000). The evolution of pelvic aspiration in archosaurs. Paleobiology 26, 271–293. doi: 10.1666/0094-8373(2000)026<0271:TEOPAI>2.0.CO;2
Carvalho, I., Novas, F. E., Agnolín, F. L., Isasi, M. P., Freitas, F. I., and Andrade, J. A. (2015). A mesozoic bird from gondwana preserving feathers. Nat. Commun. 6:7141. doi: 10.1038/ncomms8141
Cau, A. (2018). The assembly of the avian body plan: a 160-million-year long process. Boll. Soc. Paleontol. Ital. 57, 1–25.
Cau, A., Beyrand, V., Voeten, D. F., Fernandez, V., Tafforeau, P., Stein, K., et al. (2017). Synchrotron scanning reveals amphibious ecomorphology in a new clade of bird-like dinosaurs. Nature 552, 395–399. doi: 10.1038/nature24679
Cau, A., and Madzia, D. (2018). Redescription and affinities of Hulsanpes perlei (Dinosauria, Theropoda) from the Upper Cretaceous of Mongolia. PeerJ 6:e4868. doi: 10.7717/peerj.4868
Chatterjee, S. (1991). Cranial anatomy and relationships of a new triassic bird from texas. Philos. Trans. R. Soc. Lond. B 332, 277–346. doi: 10.1098/rstb.1991.0056
Chatterjee, S., and Templin, R. J. (2004). “Feathered coelurosaurs from China: new light on the arboreal origin of avian flight,” in Feathered Dragons: Studies on the Transition from Dinosaurs to Birds, eds P. J. Currie, E. B. Koppelhus, M. A. Shugar, and J. L. Wright (Bloomington: Indiana Univ Press), 251–281.
Chatterjee, S., and Templin, R. J. (2007). Biplane wing planform and flight performance of the feathered dinosaur Microraptor gui. Proc.0 Natl. Acad. Sci. U.S.A. 104, 1576–1580. doi: 10.1073/pnas.0609975104
Chiappe, L. M. (2001). “Phylogenetic relationships among basal birds,” in New Perspectives on the Origin and Evolution of Birds, eds J. Gauthier and L. F. Gall (New Haven, CT: Yale University), 125–139.
Chiappe, L. M., Ji, S., Ji, Q., and Norell, M. A. (1999). Anatomy and systematics of the Confuciusornithidae (Theropoda: Aves) from the Late Mesozoic of northeastern China. Bull. Am. Museum Nat. Hist. 242, 1–89.
Chiappe, L. M., Marugán-Lobón, J., Ji, S. A., and Zhou, Z. (2008). Life history of a basal bird: morphometrics of the early cretaceous confuciusornis. Biol. Lett. 4, 719–723. doi: 10.1098/rsbl.2008.0409
Chimento, N. R., Agnolin, F. L., Novas, F. E., Ezcurra, M. D., Salgado, L., Isasi, M. P., et al. (2017). Forelimb posture in Chilesaurus diegosuarezi (Dinosauria, Theropoda) and its behavioral and phylogenetic implications. Ameghiniana 54, 567–575. doi: 10.5710/AMGH.11.06.2017.3088
Chin, D. D., Matloff, L. Y., Stowers, A. K., Tucci, E. R., and Lentink, D. (2017). Inspiration for wing design: how forelimb specialization enables active flight in modern vertebrates. J. R. Soc. Interface 14:20170240. doi: 10.1098/rsif.2017.0240
Choiniere, J. N., Xu, X., Clark, J. M., Forster, C. A., Guo, Y., and Han, F. (2010). A basal alvarezsauroid theropod from the early late jurassic of Xinjiang, China. Science 327, 571–574. doi: 10.1126/science.1182143
Chuong, C.-M., Yeh, C.-Y., Ting-Xin, J., and Widelitz, R. (2013). Module-based complexity formation: periodic patterning in feathers and hairs. Wiley Interdiscip. Rev. Dev. Biol. 2, 97–112. doi: 10.1002/wdev.74
Claessens, L. P. (2004). Dinosaur gastralia; origin, morphology, and function. J. Vertebrate Paleontol. 24, 89–106. doi: 10.1371/journal.pone.0003303
Clarke, J. A., Zhou, Z., and Zhang, F. (2006). Insight into the evolution of avian flight from a new clade of early cretaceous ornithurines from China and the morphology of Yixianornis grabaui. J. Anat. 208, 287–308. doi: 10.1111/j.1469-7580.2006.00534.x
Codd, J. R., Boggs, D. F., Perry, S. F., and Carrier, D. R. (2005). Activity of three muscles associated with the uncinate processes of the giant Canada goose Branta canadensis maximus. J. Exp. Biol. 208, 849–857. doi: 10.1242/jeb.01489
Currie, P. J. (1985). Cranial anatomy of Stenonychosaurus inequalis (Saurischia, Theropoda) and its bearing on the origin of birds. Can. J. Earth Sci. 22, 1643–1658. doi: 10.1139/e85-173
Currie, P. J. (1987). Bird-like characteristics of the jaws and teeth of troodontid theropod (Dinosauria, Saurischia). J. Vertebrate Paleontol. 7, 72–81. doi: 10.1080/02724634.1987.10011638
Currie, P. J. (1995). New information on the anatomy and relationships of Dromaeosaurus albertensis (Dinosauria: Theropoda). J. Vertebrate Paleontol. 15, 576–591. doi: 10.1080/02724634.1995.10011250
Currie, P. J., and Dong, Z. (2001). New information on Cretaceous troodontids (Dinosauria, Theropoda) from the people’s republic of China. Can. J. Earth Sci. 38, 1753–1766. doi: 10.1007/s00114-014-1143-9
Currie, P. J., and Paulina Carabajal, A. (2012). A new specimen of Austroraptor cabazai Novas, Pol, Canale, Porfiri and Calvo, 2008 (Dinosauria, Theropoda, Unenlagiidae) from the latest Cretaceous (Maastrichtian) of Rio Negro, Argentina. Ameghiniana 49, 662–667. doi: 10.5710/AMGH.30.8.2012.574
Currie, P. J., and Zhao, X. (1993). A new carnosaur (Dinosauria, Theropoda) from the upper jurassic of Xinjiang, People’s Republic of China. Can. J. Earth Sci. 30, 2037–2081. doi: 10.1139/e93-179
Czerkas, S. A., and Feduccia, A. (2014). Jurassic archosaur is a non-dinosaurian bird. J. Ornithol. 155, 841–851. doi: 10.1007/s10336-014-1098-9
Czerkas, S. A., and Yuan, C. (2002). An arboreal maniraptoran from northeast China. Feathered Dinosaurs Origin Flight 1, 63–95. doi: 10.1007/s00114-017-1496-y
Dal Sasso, C., and Maganuco, S. (2011). Scipionyx samniticus (Theropoda: Compsognathidae) from the lower cretaceous of italy: osteology, ontogenetic assessment, phylogeny, soft tissue anatomy, taphonomy and palaeobiology. Mem. Della Soc. Ital. Sci. Nat. Museo Civ. Storia Nat. Milano 37, 1–504.
Darwin, C. (1859). On the Origins of Species by Means of Natural Selection. or the Preservation of Favoured Races in the Struggle for Life. London: John Murray,
Dececchi, T. A., Larsson, H. C., and Habib, M. B. (2016). The wings before the bird: an evaluation of flapping-based locomotory hypotheses in bird antecedents. PeerJ 4:e2159. doi: 10.7717/peerj.2159
Dececchi, T. A., and Larsson, H. C. E. (2011). Assessing arboreal adaptations of bird antecedents: testing the ecological setting of the origin of the avian flight stroke. PLoS One 6:e22292. doi: 10.1371/journal.pone.0022292
Dial, K. P., Goslow, G. E. Jr., and Jenkins, F. A. Jr. (1991). The functional anatomy of the shoulder in the European starling (Sturnus vulgaris). J. Morphol. 207, 327–344. doi: 10.1002/jmor.1052070309
Feduccia, A. (1993). Evidence from claw geometry indicating arboreal habits of Archaeopteryx. Science 259, 790–793. doi: 10.1126/science.259.5096.790
Feduccia, A., and Czerkas, S. A. (2015). Testing the neoflightless hypothesis: propatagium reveals flying ancestry of oviraptorosaurs. J. Ornithol. 156, 1067–1074. doi: 10.1007/s10336-015-1190-9
Feo, T. J., Field, D. J., and Prum, R. O. (2015). Barb geometry of asymmetrical feathers reveals a transitional morphology in the evolution of avian flight. Proc. R. Soc. B 282:20142864. doi: 10.1098/rspb.2014.2864
Forster, C. A., Sampson, S. D., Chiappe, L. M., and Krause, D. W. (1998). The theropod ancestry of birds: new evidence from the late cretaceous of madagascar. Science 279, 1915–1919. doi: 10.1126/science.279.5358.1915
Foth, C. (2014). Comment on the absence of ossified sternal elements in basal paravian dinosaurs. Proc. Natl. Acad. Sci. U.S.A. 111, E5334–E5334. doi: 10.1073/pnas.1419023111
Foth, C., and Rauhut, O. W. (2017). Re-evaluation of the Haarlem Archaeopteryx and the radiation of maniraptoran theropod dinosaurs. BMC Evol. Biol. 17:236. doi: 10.1186/s12862-017-1076-y
Foth, C., Tischlinger, H., and Rauhut, O. W. (2014). New specimen of Archaeopteryx provides insights into the evolution of pennaceous feathers. Nature 511, 79–82. doi: 10.1038/nature13467
Funston, G. F., Mendonca, S. E., Currie, P. J., and Barsbold, R. (2017). Oviraptorosaur anatomy, diversity and ecology in the Nemegt Basin. Palaeogeogr. Palaeoclimatol. Palaeoecol. 494, 101–120. doi: 10.1016/j.dib.2017.11.086
Galton, P. M., and Upchurch, P. (2004). Prosauropoda. Berkeley, CA:University of California Press. doi: 10.1525/california/9780520242098.003.0014
Gao, C., Chiappe, L. M., Meng, Q., O’Connor, J. K., Wang, X., Cheng, X., et al. (2008). A new basal lineage of early cretaceous birds from China and its implications on the evolution of the avian tail. Palaeontology 51, 775–791. doi: 10.1111/j.1475-4983.2008.00793.x
Gao, C., Morschhauser, E. M., Varricchio, D. J., Liu, J., and Zhao, B. (2012). A second soundly sleeping dragon: new anatomical details of the Chinese troodontid Mei long with implications for phylogeny and taphonomy. PLoS One 7:e45203. doi: 10.1371/journal.pone.0045203
Gatesy, S. M., and Dial, K. P. (1996). From frond to fan: Archaeopteryx and the evolution of short-tailed birds. Evolution 50, 2037–2048. doi: 10.1111/j.1558-5646.1996.tb03590.x
Gianechini, F. A., Agnolin, F. L., and Ezcurra, M. D. (2011a). A reassessment of the purported venom delivery system of the bird-raptor Sinornithosaurus. Paläontol. Z. 85, 103–107.
Gianechini, F. A., Makovicky, P. J., and Apesteguía, S. (2011b). The teeth of the unenlagiine theropod Buitreraptor from the cretaceous of patagonia, argentina, and the unusual dentition of the gondwanan dromaeosaurids. Acta Palaeontol. Pol. 56, 279–290. doi: 10.4202/app.2009.0127
Gianechini, F. A., and Apesteguía, S. (2011). Unenlagiinae revisited: dromaeosaurid theropods from South America. Ana. Acad. Brasil. Ciências 83, 163–195. doi: 10.1590/S0001-37652011000100009
Gianechini, F. A., Makovicky, P. J., and Apesteguía, S. (2017). The cranial osteology of Buitreraptor gonzalezorum makovicky, apesteguía, and agnolín, 2005 (Theropoda, Dromaeosauridae), from the Late Cretaceous of Patagonia, Argentina. J. Vertebrate Paleontol. 37:e1255639. doi: 10.1080/02724634.2017.1255639
Gianechini, F. A., Makovicky, P. J., Apesteguía, S., and Cerda, I. (2018). Postcranial skeletal anatomy of the holotype and referred specimens of Buitreraptor gonzalezorum makovicky, apesteguía and agnolín 2005 (Theropoda, Dromaeosauridae), from the Late Cretaceous of Patagonia. PeerJ 6:e4558. doi: 10.7717/peerj.4558
Godefroit, P., Cau, A., Dong-Yu, H., Escuillié, F., Wenhao, W., and Dyke, G. (2013a). A Jurassic avialan dinosaur from China resolves the early phylogenetic history of birds. Nature 498, 359–362. doi: 10.1038/nature12168
Godefroit, P., Demuynck, H., Dyke, G., Hu, D., Escuillié, F., and Claeys, P. (2013b). Reduced plumage and flight ability of a new Jurassic paravian theropod from China. Nat. Commun. 4:1394. doi: 10.1038/ncomms2389
Godefroit, P., Sinitsa, S. M., Dhouailly, D., Bolotsky, Y. L., Sizov, A. V., McNamara, M. E., et al. (2014). A Jurassic ornithischian dinosaur from Siberia with both feathers and scales. Science 345, 451–455. doi: 10.1126/science.1253351
Godfrey, S. J., and Currie, P. J. (2004). “A theropod (Dromaeosauridae, Dinosauria) sternal plate from the Dinosaur Park Formation (Campanian, Upper Cretaceous) of Alberta, Canada,” in Feathered Dragons: Studies on the Transition from Dinosaurs to Birds, eds P. J. Currie, E. B. Koppelhus, M. A. Shugar, and J. L. Wright (Bloomington: Indiana University Press), 67–111.
Goswami, A., Prasad, G. V. R., Verma, O., Flynn, J. J., and Benson, R. B. J. (2013). A troodontid dinosaur from the latest Cretaceous of India. Nat. Commun. 4:1703. doi: 10.1038/ncomms2716
Han, G., Chiappe, L. M., Ji, S. A., Habib, M., Turner, A. H., Chinsamy, A., et al. (2014). A new raptorial dinosaur with exceptionally long feathering provides insights into dromaeosaurid flight performance. Nat. Commun. 5:4382. doi: 10.1038/ncomms5382
Holtz, T. R. Jr. (2001). Arctometatarsalia Revisited: the Problem of Homoplasy in Reconstructing Theropod Phylogeny. New Haven, CT: Peabody Museum of Natural History, 99–121.
Holtz, T. R. Jr. (1994). The phylogenetic position of the Tyrannosauridae: implications for theropod systematics. J. Vertebrate Paleontol. 65, 1100–1117. doi: 10.1017/S0022336000026706
Hopp, T. P., and Orsen, M. J. (2004). “Dinosaur brooding behavior and the origin of flight feathers,” in Feathered Dinosaurs: Studies on the Transition from Dinosaurs to Birds, eds E. J. Currie, E. B. Koppelhus, M. A. Shugar, and J. L. Wright (Bloomington: University Press), 234–250.
Hu, D., Clarke, J. A., Eliason, C. M., Qiu, R., Li, Q., Shawkey, M. D., et al. (2018). A bony-crested Jurassic dinosaur with evidence of iridescent plumage highlights complexity in early paravian evolution. Nat. Commun. 9:217. doi: 10.1038/s41467-017-02515-y
Hu, D., Hou, L., Zhang, L., and Xu, X. (2009). A pre-Archaeopteryx troodontid theropod from China with long feathers on the metatarsus. Nature 461, 640–643. doi: 10.1038/nature08322
Hu, H., O’Connor, J. K., and Zhou, Z. (2015). A new species of Pengornithidae (Aves: Enantiornithes) from the Lower Cretaceous of China suggests a specialized scansorial habitat previously unknown in early birds. PLoS One 10:e0126791. doi: 10.1371/journal.pone.0126791
Hutson, J. D., and Hutson, K. N. (2014). A repeated-measures analysis of the effects of soft tissues on wrist range of motion in the extant phylogenetic bracket of dinosaurs: implications for the functional origins of an automatic wrist folding mechanism in crocodilia. Anat. Rec. 297, 1249–1228. doi: 10.1002/ar.22903
Hwang, S. H., Norell, M. A., Ji, Q., and Gao, K.-Q. (2002). New specimens of Microraptor zhaoianus (Theropoda: Dromaeosauridae) from northeastern China. Am. Mus. Novit. 3381, 1–44. doi: 10.1206/0003-0082(2002)381<0001:NSOMZT>2.0.CO;2
Jenkins, F. A. (1993). The evolution of the avian shoulder joint. Am. J. Sci. 293, 253–253. doi: 10.2475/ajs.293.A.253
Ji, Q., Currie, P. J., Norell, M. A., and Ji, S. A. (1998). Two feathered dinosaurs from northeastern China. Nature 393, 753–761. doi: 10.1038/nature10906
Ji, Q., Ji, S., Lu, J., You, H., Chen, W., Liu, Y., et al. (2005). First avialan bird from China (Jinfengopteryx elegans gen. et sp. nov.). Geol. Bull. China 24, 197–205.
Ji, Q., and Ji, S. A. (1996). On discovery of the earliest bird fossil in China and the origin of birds. Chin. Geol. 233, 30–33.
Ji, Q., Norell, M. A., Gao, K. Q., Ji, S. A., and Ren, D. (2001). The distribution of integumentary structures in a feathered dinosaur. Nature 410, 1084–1088. doi: 10.1038/35074079
Kellner, A. W. A., Wang, X., Tischlinger, H., Campos, D. A., Hone, D. W. E., and Meng, X. (2009). The soft tissue of Jeholopterus (Pterosauria, Anurognathidae, Batrachognathinae) and the structure of the pterosaur wing membrane. Proc. R. Soc. Lond. B 277, 321–329. doi: 10.1098/rspb.2009.0846
Kobayashi, Y., Lu, J.-C., Dong, Z.-M., Barsbold, R., Azuma, Y., and Tomida, Y. (1999). Herbivorous diet in an ornithomimid dinosaur. Nature 402, 480–481. doi: 10.1038/44999
Kurzanov, S. M. (1981). An unusual theropod from the Upper Cretaceous of Mongolia. Joint Soviet Mongolian Paleontol. Expedition 15, 39–49. doi: 10.1371/journal.pone.0022916
Lambertz, M., and Perry, S. F. (2015). Remarks on the evolution of the avian sternum, dinosaur gastralia, and their functional significance for the respiratory apparatus. Zool. Anzeiger A J. Comp. Zool. 255, 80–84. doi: 10.1016/j.jcz.2015.02.008
Larrson, H. C. E., Hone, D. W., Dececchi, T. A., Sullivan, C., and Xu, X. (2010). The winged non-avian dinosaur microraptor fed on mammals: implications for the Jehol Biota ecosystem. J. Vert. Paleont. 30:114A.
Lee, M. S., and Worthy, T. H. (2012). Likelihood reinstates Archaeopteryx as a primitive bird. Biol. Lett. 8, 299–303. doi: 10.1098/rsbl.2011.0884
Lee, M. S. Y., Cau, A., Naish, D., and Dyke, G. (2014). Sustained miniaturization and anatomical innovation in the dinosaurian ancestors of birds. Science 345, 562–566. doi: 10.1126/science.1252243
Lefèvre, U., Cau, A., Cincotta, A., Hu, D., Chinsamy, A., Escuillié, F., et al. (2017). A new Jurassic theropod from China documents a transitional step in the macrostructure of feathers. Sci. Nat. 104:74. doi: 10.1007/s00114-017-1496-y
Li, L., Ye, D., Dongyu, H., Li, W., Shaoli, C., and Lianhai, H. (2006). New eoenantiornithid bird from the early cretaceous Jiufotang formation of Western Liaoning, China. Acta Geol. Sin. English Edition 80, 38–41. doi: 10.1111/j.1755-6724.2006.tb00792.x
Li, Q., Gao, K. Q., Meng, Q., Clarke, J. A., Shawkey, M. D., D’Alba, L., et al. (2012). Reconstruction of Microraptor and the evolution of iridescent plumage. Science 335, 1215–1219. doi: 10.1126/science.1213780
Li, Q., Gao, K. Q., Vinther, J., Shawkey, M. D., Clarke, J. A., D’alba, L., et al. (2010). Plumage color patterns of an extinct dinosaur. Science 327, 1369–1372. doi: 10.1126/science.1186290
Lo Coco, G. E., Motta, M. J., Agnolín, F. L., Brissón Egli, F., and Novas, F. E. (2018). “Implicancias en las modificaciones del hueso coracoides en terópodos paravianos,” in Proceedings of the XXXII Jornadas Argentina de Paleontología de Vertebrados Libro de Resúmenes, Argentina 39.
Longrich, N. R., and Currie, P. J. (2009). A microraptorine (Dinosauria-Dromaeosauridae) from the late Cretaceous of North America. Proc. Natl. Acad. Sci. U.S.A. 106, 5002–5007. doi: 10.1073/pnas.0811664106
Longrich, N. R., Vinther, J., Meng, Q., Li, Q., and Russell, A. P. (2012). Primitive wing feather arrangement in Archaeopteryx lithographica and Anchiornis huxleyi. Curr. Biol. 22, 2262–2267. doi: 10.1016/j.cub.2012.09.052
Lü, J., and Brusatte, S. L. (2015). A large, short-armed, winged dromaeosaurid (Dinosauria: Theropoda) from the early cretaceous of china and its implications for feather evolution. Sci. Rep. 5:11775. doi: 10.1038/srep11775
Lü, J.-C., Huang, D., and Qui, L. (2005). “The pectoral girdle and the forelimb of Heyuannia (Dinosauria: Oviraptorosauria),” in The Carnivorous Dinosaurs, ed. K. Carpenter (Indianapolis: University of Indiana Press), 257–273.
Makovicky, P., Apesteguía, S., and Agnolín, F. L. (2005). The earliest dromaeosaurid theropod from South America. Nature 437, 1007–1011. doi: 10.1038/nature03996
Makovicky, P. J., and Norell, M. A. (2004). “Troodontidae,” in The Dinosauria, eds D. B. Weishampel, P. Dodson, and H. Osmólska (Berkeley,CA: University of California Press), 184–195. doi: 10.1525/california/9780520242098.003.0011
Makovicky, P. J., Norell, M. A., Clark, J. M., and Rowe, T. (2003). Osteology and relationships of Byronosaurus jaffei (Theropoda: Troodontidae). Am. Museum Novitates 3402, 1–32. doi: 10.1206/0003-0082(2003)402<0001:OAROBJ>2.0.CO;2
Mayr, G., Peters, S. D., Plodowski, G., and Vogel, O. (2002). Bristle-like integumentary structures at the tail of the horned dinosaur Psittacosaurus. Naturwissenschaften 89, 361–365. doi: 10.1007/s00114-002-0339-6
Mayr, G., Pohl, B., Hartman, S., and Peters, D. S. (2007). The tenth skeletal specimen of Archaeopteryx. Zool. J. Linnean Soc. 149, 97–116. doi: 10.1111/j.1096-3642.2006.00245.x
Motta, M. J., Aranciaga Rolando, A. M., Rozadilla, S., Agnolín, F. E., Chimento, N. R., Brissón Egli, F., et al. (2018). “New theropod fauna from the upper cretaceous (huincul formation) of northwestern patagonia, argentina. N. Mexico Museum Nat. Hist. Sci. Bull. 71, 231–253.
Nicholls, E. L., and Russell, A. P. (1985). Structure and function of the pectoral girdle and forelimb of Struthiomimus altus (Theropoda: Ornithomimidae). Palaeontology 28, 643–677.
Norell, M. A., Clark, J. M., and Makovicky, P. J. (2001). “Phylogenetic relationships among coelurosaurian theropods,” in New Perspectives on the Origin and Early Evolution of Birds. New Haven: Peabody Museum of Natural History, eds J. Gauthier and L. F. Gall (New Haven: Yale University), 49–67.
Norell, M. A., Clark, J. M., Turner, A. H., Makovicky, P. J., Barsbold, R., and Rowe, T. (2006). A new dromaeosaurid theropod from UkhaaTolgod (Omnogov, Mongolia). Am. Museum Novitates 1–51.
Norell, M. A., and Makovicky, P. J. (1997). Important features of the dromaeosaur skeleton: information from a new specimen. Am. Museum Novitates 3215, 1–28.
Norell, M. A., and Makovicky, P. J. (1999). Important features of the dromaeosaur skeleton II: information from newly collected specimens of Velociraptor mongoliensis. Am. Museum Novitates 3282, 1–45.
Norell, M. A., Makovicky, P. J., and Clark, J. M. (2004). “The braincase of Velociraptor,” in Feathered Dragons: Studies on the Transition From Dinosaurs to Birds, eds P. J. Currie, E. B. Koppelhus, M. A. Shugar, and J. L. Wright (Bloomington: Indiana University Press), 133.
Norman, D. B., Sues, H.-D., Witmer, L. M., and Coria, R. A. (2004). “Basal Ornithopoda,” in The Dinosauria, 2nd Edn, eds D. B. Weishampel, P. Dodson, and H. Osmólska (Berkeley: University of California Press), 393–412.
Novas, F. E. (2004). “Avian traits in the ilium of Unenlagia comahuensis (Maniraptora, Avialae),” in Feathered Dragons: Studies on the Transition From Dinosaurs to Birds, eds P. J. Currie, E. B. Koppelhus, M. A. Shugar, and J. L. Wright (Bloomington: Indiana University Press), 150–168.
Novas, F. E., and Agnolin, F. L. (2014). “Scapular girdle morphology in paravians and implications for the origin of flight,” in Proceedings of the Abstract Volume, 4th International Palaeontological Congress, Mendoza, 145.
Novas, F. E., Agnolin, F. L., and Lo Coco, G. (2016). Morfología de la cintura escapular de los paravianos basales y aves Ratites: implicancias en el origen del vuelo aleteado Abstracts XXX Jornadas Argentinas de Paleontologia de Vertebrados. Ameghiniana 53, 63–64.
Novas, F. E., Brissón Egli, F., Agnolín, F. L., Gianechini, F. A., and Cerda, I. (2018). Postcranial osteology of a new specimen of Buitreraptor gonzalezorum (Theropoda, Unenlagiidae). Cretac. Res. 83, 127–167. doi: 10.1016/j.cretres.2017.06.003
Novas, F. E., and Pol, D. (2005). New evidence on deinonychosaurian dinosaurs from the late cretaceous of patagonia. Nature 433, 858. doi: 10.1038/nature03285
Novas, F. E., Pol, D., Canale, J. I., Porfiri, J. D., and Calvo, J. O. (2009). A bizarre Cretaceous theropod dinosaur from Patagonia and the evolution of Gondwanan dromaeosaurids. Proc. R. Soc. Lond. B 276, 1101–1107. doi: 10.1098/rspb.2008.1554
Novas, F. E., and Puerta, P. F. (1997). New evidence concerning avian origins from the Late Cretaceous of Patagonia. Nature 387, 390–392. doi: 10.1038/387390a0
Nudds, R. L. (2014). Reassessment of the wing feathers of Archaeopteryx lithographica suggests no robust evidence for the presence of elongated dorsal wing coverts. PLoS One 9:e93963. doi: 10.1371/journal.pone.0093963
Nudds, R. L., and Dyke, G. J. (2010). Narrow primary feather rachises in Confuciusornis and Archaeopteryx suggest poor flight ability. Science 328, 887–889. doi: 10.1126/science.1188895
O’Connor, J. K., Chiappe, L. M., Chuong, C. M., Bottjer, D. J., and You, H. (2012). Homology and potential cellular and molecular mechanisms for the development of unique feather morphologies in early birds. Geosciences 2, 157–177. doi: 10.3390/geosciences2030157
O’Connor, J. K., and Sullivan, C. (2014). Reinterpretation of the Early Cretaceous maniraptoran (Dinosauria: Theropoda) Zhongornis haoae as a scansoriopterygid-like non-avian, and morphological resemblances between scansoriopterygids and basal oviraptorosaurs. Vertebr. Palasiat. 52, 3–30.
O’Connor, J. K., Wang, M., Zheng, X., and Zhou, Z. (2014). Reply to Foth: preserved cartilage is rare but not absent: troodontid sternal plates are absent, not rare. Proc. Natl. Acad. Sci. U.S.A. 111, E5335–E5335. doi: 10.1073/pnas.1419403111
O’Connor, J. K., Wang, X., Zheng, X., Hu, H., Zhang, X., and Zhou, Z. (2016). An enantiornithine with a fan-shaped tail, and the evolution of the rectricial complex in early birds. Curr. Biol. 26, 114–119. doi: 10.1016/j.cub.2015.11.036
O’Connor, J. K., Zhang, Y., Chiappe, L. M., Meng, Q., Quanguo, L., and Di, L. (2013). A new enantiornithine from the Yixian Formation with the first recognized avian enamel specialization. J. Vertebr. Paleontol. 33, 1–12. doi: 10.1080/02724634.2012.719176
O’Connor, J. K., Zhou, Z., and Xu, X. (2011a). Additional specimen of Microraptor provides unique evidence of dinosaurs preying on birds. Proc. Natl. Acad. Sci. U.S.A. 108, 19662–19665. doi: 10.1073/pnas.1117727108
O’Connor, J. K., Zhou, Z., and Zhang, F. (2011b). A reappraisal of Boluochia zhengi (Aves: Enantiornithes) and a discussion of intraclade diversity in the Jehol avifauna, China. J. Syst. Palaeontol. 9, 51–63. doi: 10.1080/14772019.2010.512614
O’Connor, P. M., and Forster, C. A. (2010). A late cretaceous (Maastrichtian) avifauna from the maevarano formation, madagascar. J. Verteb. Paleontol. 30, 1178–1201. doi: 10.1080/02724634.2010.483544
Olson, S. L., and Feduccia, A. (1979). Flight capability and the pectoral girdle of Archaeopteryx. Nature 278, 247–248. doi: 10.1038/278247a0
Osmólska, H. (1981). Coossified tarsometatarsi in theropod dinosaurs and their bearing on the problem of bird origins. Palaeontologia Polonica 42, 79–95.
Osmólska, H. (1982). Hulsanpes perlei ng n sp. (Deinonychosauria, Saurischia, Dinosauria) from the upper cretaceous barun goyot formation of mongolia. Neues Jahrb. Geol. Paläontol. Monatshef 7, 440–448.
Osmólska, H., Currie, P. J., and Barsbold, R. (2004). “Oviraptorosauria,” in The Dinosauria, 2nd Edn, eds D. B. Weishampel, P. Dodson, and H. Osmólska (University of California Press), 165–183. doi: 10.1525/california/9780520242098.003.0010
Osmólska, H., Roniewicz, E., and Barsbold, R. (1972). A new dinosaur, Gallimimus bullatus n. gen., n. sp. (Ornithomimidae) from the upper cretaceous of mongolia. Palaeontol. Pol. 27, 103–143.
Ostrom, J. H. (1974). Archaeopteryx and the origin of flight. Q. Rev. Biol. 49, 27–47. doi: 10.1086/407902
Ostrom, J. H. (1976). Archaeopteryx and the origin of birds. Biol. J. Linnean Soc. 8, 91–182. doi: 10.1111/j.1095-8312.1976.tb00244.x
Padian, K., and Horner, J. R. (2011). The evolution of ‘bizarre structures’ in dinosaurs: biomechanics, sexual selection, social selection or species recognition? J. Zool. 283, 3–17. doi: 10.1111/j.1469-7998.2010.00719.x
Padian, K., Hutchinson, J. R., and Holtz, T. R. Jr. (1997). Phylogenetic definitions and nomenclature of the major taxonomic categories of the theropod dinosaurs. J. Verteb. Paleontol. 17(Suppl. 3):68A.
Pei, R., Li, Q., Meng, Q., Norell, M. A., and Gao, K. Q. (2017a). New specimens of Anchiornis huxleyi (Theropoda: Paraves) from the Late Jurassic of northeastern China. Bull. Am. Museum Nat. Hist. 2017, 1–67. doi: 10.1206/0003-0090-411.1.1
Pei, R., Norell, M. A., Barta, D. E., Bever, G. S., Pittman, M., and Xu, X. (2017b). Osteology of a new late cretaceous troodontid specimen from ukhaa tolgod, ömnögovi aimag, Mongolia. Am. Museum Novitates 3889, 1–47. doi: 10.1206/3889.1
Perez-Moreno, B. P., Sanz, J. L., Buscalioni, A. D., Moratalla, J. J., Ortega, F., and Raskin-Gutman, D. (1994). A unique multitoothed ornithomimosaur from the Lower Cretaceous of Spain. Nature 30, 363–367. doi: 10.1038/370363a0
Perle, A., Chiappe, L. M., Rinchen, B., Clark, J. M., and Norell, M. (1994). Skeletal morphology of Mononykus olecranus (Theropoda, Avialae) from the late cretaceous of mongolia. Am. Museum Novitates 3105, 1–34.
Perry, S. F. (1983). Reptilian lungs. functional anatomy and evolution. Adv. Anat. Embryol. Cell Biol. 79, 1–81. doi: 10.1007/978-3-642-68964-2_1
Pittman, M., Pei, R., Tan, Q., and Xu, X. (2015). The first dromaeosaurid (Dinosauria: Theropoda) from the lower cretaceous bayan gobi formation of nei mongol, China. PeerJ 3:e1480. doi: 10.7717/peerj.1480
Poore, S. O., Sanchez-Haiman, A., and Goslow, G. E. Jr. (1997). Wing upstroke and the evolution of flapping flight. Nature 387:799. doi: 10.1038/42930
Porfiri, J. D., Calvo, J. O., and dos Santos, D. (2011). A new small deinonychosaur (Dinosauria: Theropoda) from the Late Cretaceous of Patagonia, Argentina. Ana. Acad. Brasil. Ciências 83, 109–116. doi: 10.1590/S0001-37652011000100007
Rauhut, O. W. (2004). Braincase structure of the middle jurassic theropod dinosaur Piatnitzkysaurus. Can. J. Earth Sci. 41, 1109–1122. doi: 10.1139/e04-053
Rauhut, O. W., Foth, C., and Tischlinger, H. (2018). The oldest Archaeopteryx (Theropoda: Avialiae): a new specimen from the Kimmeridgian/Tithonian boundary of Schamhaupten, Bavaria. PeerJ 6:e4191. doi: 10.7717/peerj.4191
Rauhut, O. W., Foth, C., Tischlinger, H., and Norell, M. A. (2012). Exceptionally preserved juvenile megalosauroid theropod dinosaur with filamentous integument from the Late Jurassic of Germany. Proc. Natl. Acad. Sci. 109, 11746–11751. doi: 10.1073/pnas.1203238109
Rauhut, O. W. M. (2003). Special Papers in Palaeontology, The Interrelationships and Evolution of Basal Theropod Dinosaurs (No. 69). Hoboken, NJ: Blackwell Publishing.
Ruben, J. (1991). Reptilian physiology and the flight capacity of Archaeopteryx. Evolution 45, 1–17. doi: 10.1111/j.1558-5646.1991.tb05261.x
Saitta, E. T., Gelernter, R., and Vinther, J. (2018). Additional information on the primitive contour and wing feathering of paravian dinosaurs. Palaeontology 61, 273–288. doi: 10.1111/pala.12342
Senter, P. (2007). A new look at the phylogeny of Coelurosauria (Dinosauria: Theropoda). J. Syst. Palaeont 5, 429–463. doi: 10.1017/S1477201907002143
Senter, P. (2011). Using creation science to demonstrate evolution 2: morphological continuity within Dinosauria. J. Evol. Biol. 24, 2197–2216. doi: 10.1111/j.1420-9101.2011.02349.x
Senter, P., Barsbold, R., Britt, B. B., and Burnham, D. A. (2004). Systematics and evolution of Dromaeosauridae (Dinosauria, theropoda). Bull. Gunma Museum Nat. Hist. 8, 1–20.
Senter, P., Kirkland, J. I., DeBlieux, D. D., Madsen, S., and Toth, N. (2012). Correction: new dromaeosaurids (Dinosauria: Theropoda) from the lower cretaceous of utah, and the evolution of the dromaeosaurid tail. PLoS One 7:36790, doi: 10.1371/journal.pone.0036790
Sereno, P. C. (1997). The origin and evolution of dinosaurs. Annu. Rev. Earth Planet. Sci. 25, 435–489. doi: 10.1146/annurev.earth.25.1.435
Sereno, P. C. (1999). The evolution of dinosaurs. Science 284, 2137–2147. doi: 10.1126/science.284.5423.2137
Sereno, P. C., and Chenggang, R. (1992). Early evolution of avian flight and perching: new evidence from the lower cretaceous of China. Science 255, 845–848. doi: 10.1126/science.255.5046.845
Sereno, P. C., Wilson, J. A., and Conrad, J. L. (2004). New dinosaurs link southern landmasses in mid Cretaceous. Proc. R. Soc. Lond. B 271, 1325–1330. doi: 10.1098/rspb.2004.2692
Shen, C., Zhao, B., Gao, C. L., Lü, J., and Kundrát, M. (2017). A new troodontid dinosaur, Liaoningvenator curriei gen. et sp. nov., from the Early cretaceous yixian formation of western Liaoning Province, China. Acta Geosci. Sin. 38, 1–13.
Sternberg, C. M. (1933). A new Ornithomimus with complete abdominal cuirass. Can. Field Nat. 47, 79–83.
Stowers, A. K., Matloff, L. Y., and Lentink, D. (2017). How pigeons couple three-dimensional elbow and wrist motion to morph their wings. J. Royal Soc. Interface 14:224. doi: 10.1098/rsif.2017.0224
Tarsitano, S. F. (1985). “The morphological and aerodynamic constraints on the origin of avian flight,” in The Beginnings of Birds, Proceedings of the International Archaeopteryx Conference, eds M. K. Hecht, J. H. Ostrom, G. Viohl, and P. Wellnhofer (Eichstatt: Freunde des Jura-Museums Eichstatt), 319–332.
Tsuihiji, T., Barsbold, R., Watabe, M., Tsogtbaatar, K., Chinzorig, T., Fujiyama, Y., et al. (2014). An exquisitely preserved troodontid theropod with new information on the palatal structure from the Upper Cretaceous of Mongolia. Naturwissenschaften 101, 131–142. doi: 10.1007/s00114-014-1143-9
Tsuihiji, T., Barsbold, R., Watabe, M., Tsogtbaatar, K., Suzuki, S., and Hattori, S. (2016). New material of a troodontid theropod (Dinosauria: Saurischia) from the Lower Cretaceous of Mongolia. Hist. Biol. 28, 128–138. doi: 10.1080/08912963.2015.1005086
Turner, A. H., Makovicky, P. J., and Norell, M. A. (2012). A review of dromaeosaurid systematics and paravian phylogeny. Bull. Am. Museum Nat. Hist. 20, 1–206. doi: 10.1206/748.1
Turner, A. H., Hwang, S. H., and Norell, M. A. (2007a). A small derived theropod from oösh, early cretaceous, Baykhangor Mongolia. Am. Mus Novit 3557, 1–27. doi: 10.1206/0003-0082(2007)3557[1:ASDTFS]2.0.CO;2
Turner, A. H., Pol, D., Clarke, J. A., Erickson, G. M., and Norell, M. A. (2007b). A basal dromaeosaurid and size evolution preceding avian flight. Science 317, 1378–1381.
van der Reest, A. J., and Currie, P. J. (2017). Troodontids (Theropoda) from the dinosaur park formation, alberta, with a description of a unique new taxon: implications for deinonychosaur diversity in North America. Can. J. Earth Sci. 54, 919–935. doi: 10.1139/cjes-2017-0031
van der Reest, A. J., Wolfe, A. P., and Currie, P. J. (2016). A densely feathered ornithomimid (Dinosauria: Theropoda) from the Upper Cretaceous Dinosaur Park Formation, Alberta, Canada. Cretaceous Res. 58, 108–117. doi: 10.1016/j.cretres.2015.10.004
Vazquez, R. J. (1992). Functional osteology of the avian wrist and the evolution of flapping flight. J. Morphol. 211, 259–268. doi: 10.1002/jmor.1052110303
Vazquez, R. J. (1994). Functional osteology of avian wrist and evolution of flapping flight. J. Morphol. 211, 259–268. doi: 10.1002/jmor.1052110303
Voeten, D. F., Cubo, J., Margerie, E., Röper, M., Beyrand, V., Bureš, S., et al. (2018). Wing bone geometry reveals active flight in Archaeopteryx. Nat. Commun. 9:923. doi: 10.1038/s41467-018-03296-8
Vullo, R., Neraudeau, D., and Lenglet, T. (2007). Dinosaur teeth from the Cenomanian of Charentes, western France: evidence for a mixed Laurasian-Gondwanan assemblage. J. Verteb. Paleontol. 27, 931–943. doi: 10.1671/0272-4634(2007)27[931:DTFTCO]2.0.CO;2
Walker, A. D. (1972). New light on the origin of birds and crocodiles. Nature 237, 257–263. doi: 10.1038/237257a0
Wang, X., Pittman, M., Zheng, X., Kaye, T. G., Falk, A. R., Hartman, S. A., et al. (2017). Basal paravian functional anatomy illuminated by high-detail body outline. Nat. Commun. 8:14576. doi: 10.1038/ncomms14576
Wang, X., Zhou, Z., He, H., Jin, F., Wang, Y., Zhang, J., et al. (2005). Stratigraphy and age of the Daohugou bed in Ningcheng, Inner Mongolia. Chin. Sci. Bull. 50, 2369–2376. doi: 10.1007/BF03183749
White, M. A., Bell, P. R., Cook, A. G., Barnes, D. G., Tischler, T. R., Bassam, B. J., et al. (2015). Forearm range of motion in Australovenator wintonensis (Theropoda, Megaraptoridae). PLoS One 10:e0137709. doi: 10.1371/journal.pone.0137709
Xing, L., Persons, W. S. I. V., Bell, P. R., Xu, X., Zhang, J., Miyashita, T., et al. (2013). Piscivory in the feathered dinosaur Microraptor. Evolution 67, 2441–2445. doi: 10.1111/evo.12119
Xu, X. (2002). Deinonychosaurian Fossils from the Jehol Group of Western Liaoning and the Coelurosaurian Evolution. Ph.D. thesis, Chinese Academy of Sciences, Beijing, 1–322.
Xu, X. (2016). Phylogenetic relationships of basal Pennaraptora. Abstracts XXX Jornadas Argentinas de Paleontología de Vertebrados. Ameghiniana 53:64.
Xu, X., Choinere, J., Pittman, M., Tan, Q., Xiao, D., Li, Z., et al. (2010). A new dromaeosaurid (Dinosauria: Theropoda) from the Upper Cretaceous Wulansuhai Formation of Inner Mongolia, China. Zootaxa 2403, 1–9. doi: 10.11646/zootaxa.2403.1.1
Xu, X., Currie, P., Pittman, M., Xing, L., Meng, Q., Lü, J., et al. (2017). Mosaic evolution in an asymmetrically feathered troodontid dinosaur with transitional features. Nat. Commun. 8:14972. doi: 10.1038/ncomms14972
Xu, X., and Norell, M. A. (2004). A new troodontid dinosaur from China with avian-like sleeping posture. Nature 431, 838–841. doi: 10.1038/nature02898
Xu, X., Norell, M. A., Kuang, X., Wang, X., Zhao, Q., and Jia, C. (2004). Basal tyrannosauroids from China and evidence for protofeathers in tyrannosauroids. Nature 431, 680–684. doi: 10.1038/nature02855
Xu, X., Norell, M. A., Wang, X. L., Makovicky, P. J., and Wu, X. C. (2002). A basal troodontid from the early cretaceous of China. Nature 415, 780–784. doi: 10.1038/415780a
Xu, X., and Qin, Z. C. (2017). A new tiny dromaeosaurid dinosaur from the lower cretaceous Jehol Group of western Liaoning and niche differentiation among the Jehol dromaeosaurids. Verteb. Palasiatica 55, 129–144.
Xu, X., Tang, Z. L., and Wang, X. L. (1999a). A therizinosauroid dinosaur with integumentary structures from China. Nature 399, 350–354. doi: 10.1038/20670
Xu, X., Wang, X. L., and Wu, X. C. (1999b). A dromaeosaurid dinosaur with a filamentous integument from the Yixian Formation of China. Nature 401, 262–266. doi: 10.1038/45769
Xu, X., Wang, K., Zhang, K., Ma, Q., Xing, L., Sullivan, C., et al. (2012). A gigantic feathered dinosaur from the Lower Cretaceous of China. Nature 484, 92–95. doi: 10.1038/nature10906
Xu, X., and Wang, X. (2004). A new dromaeosaur (Dinosauria: Theropoda) from the Early Cretaceous Yixian Formation of Western Liaoning. Vertebrat. Palasiatica 42, 111–119.
Xu, X., You, H., Du, K., and Han, F. (2011). An Archaeopteryx-like theropod from China and the origin of Avialae. Nature 475, 465–470. doi: 10.1038/nature10288
Xu, X., and Zhang, F. (2005). A new maniraptoran dinosaur from China with long feathers on the metatarsus. Naturwissenschaften 92, 173–177. doi: 10.1007/s00114-004-0604-y
Xu, X., Zhao, Q., Norell, M., Sullivan, C., Hone, D., Erickson, G., et al. (2008). A new feathered maniraptoran dinosaur fossil that fills a morphological gap in avian origin. Chin. Sci. Bull. 54, 430–435.
Xu, X., Zheng, X., Sullivan, C., Wang, X., Xing, L., Wang, Y., et al. (2015). A bizarre Jurassic maniraptoran theropod with preserved evidence of membranous wings. Nature 521, 70–73. doi: 10.1038/nature14423
Xu, X., Zheng, X., and You, H. (2009). A new feather type in a nonavian theropod and the early evolution of feathers. Proc. Natl. Acad. Sci. U.S.A. 106, 832–834. doi: 10.1073/pnas.0810055106
Xu, X., Zhonghe, Z., and Xiaolin, W. (2000). The smallest known non-avian theropod dinosaur. Nature 408, 705–708. doi: 10.1038/35047056
Xu, X., Zhou, Z., Dudley, R., Mackem, S., Chuong, C. M., Erickson, G. M., et al. (2014). An integrative approach to understanding bird origins. Science 346:1253293. doi: 10.1126/science.1253293
Xu, X., Zhou, Z., Wang, X., Kuang, X., Zhang, F., and Du, X. (2003). Four-winged dinosaurs from China. Nature 421, 335–340. doi: 10.1038/nature01342
Xu, X., Zhou, Z. H., and Prum, R. O. (2001). Branched integumental structures in Sinornithosaurus and the origin of feathers. Nature 410, 200–204. doi: 10.1038/35065589
Yin, Y., Pei, R., and Zhou, C. (2018). Cranial morphology of Sinovenator changii (Theropoda: Troodontidae) on the new material from the Yixian Formation of western Liaoning, China. PeerJ 6:e4977. doi: 10.7717/peerj.4977
Yuan, C. (2008). A new genus and species of Sapeornithidae from Lower Cretaceous in Western Liaoning, China. Acta Geol. Sin. 82, 48–55. doi: 10.1371/journal.pone.0069723
Zanno, L. E. (2010). A taxonomic and phylogenetic review of Therizinosauria. J. Syst. Palaeontol. 8, 503–543. doi: 10.1080/14772019.2010.488045
Zelenitsky, D. K., Therrien, F., Erickson, G. M., DeBuhr, C. L., Kobayashi, Y., Eberth, D. A., et al. (2012). Feathered non-avian dinosaurs from North America provide insight into wing origins. Science 338, 510–514. doi: 10.1126/science.1225376
Zhang, F., Kearns, S. L., Orr, P. J., Benton, M. J., Zhou, Z., Johnson, D., et al. (2010). Fossilized melanosomes and the colour of Cretaceous dinosaurs and birds. Nature 463, 1075–1078. doi: 10.1038/nature08740
Zhang, F., and Zhou, Z. (2000). A primitive enantiornithine bird and the origin of feathers. Science 290, 1955–1959. doi: 10.1126/science.290.5498.1955
Zhang, F., Zhou, Z., Xu, X., and Wang, X. (2002). A juvenile coelurosaurian theropod from China indicates arboreal habits. Naturwissenschaften 89, 394–398. doi: 10.1007/s00114-002-0353-8
Zhang, F., Zhou, Z., Xu, X., Wang, X., and Sullivan, C. (2008). A bizarre Jurassic maniraptoran from China with elongate ribbon-like feathers. Nature 455, 1105–1108. doi: 10.1038/nature07447
Zhang, Z., Chen, D., Zhang, H., and Hou, L. (2014). A large enantiornithine bird from the Lower Cretaceous of China and its implication for lung ventilation. Biol. J. Linn. Soc. 113, 820–827. doi: 10.1111/bij.12330
Zheng, X., O’Connor, J., Wang, X., Wang, M., Zhang, X., and Zhou, Z. (2014). On the absence of sternal elements in Anchiornis (Paraves) and Sapeornis (Aves) and the complex early evolution of the avian sternum. Proc. Natl. Acad. Sci. U.S.A. 111, 13900–13905. doi: 10.1073/pnas.1411070111
Zheng, X., Wang, X., O’Connor, J., and Zhou, Z. (2012). Insight into the early evolution of the avian sternum from juvenile enantiornithines. Nat. Commun. 3:1116. doi: 10.1038/ncomms2104
Zheng, X., Xu, X., You, H., Zhao, Q., and Dong, Z. (2009). A short-armed dromaeosaurid from the Jehol Group of China with implications for early dromaeosaurid evolution. Proc. R. Soc. Lond. B Biol. Sci. 277, 211–217. doi: 10.1098/rspb.2009.1178
Zheng, X. T., You, H. L., Xu, X., and Dong, Z. M. (2009). An Early Cretaceous heterodontosaurid dinosaur with filamentous integumentary structures. Nature 458, 333–336. doi: 10.1038/nature07856
Zheng, X., Zhang, Z., and Hou, L. (2007). A new enantiornitine bird with four long rectrices from the Early Cretaceous of northern Hebei, China. Acta Geol. Sin. 81, 703–708. doi: 10.1111/j.1755-6724.2007.tb00995.x
Zheng, X., Zhou, Z., Wang, X., Zhang, F., Zhang, X., Wang, Y., et al. (2013). Hind wings in basal birds and the evolution of leg feathers. Science 339, 1309–1312. doi: 10.1126/science.1228753
Zhou, Z., and Wang, X. (2000). A new species of Caudipteryx from the Yixian Formation of Liaoning, northeast China. Vertebrata Palasiatica 38, 113–130.
Zhou, Z., and Zhang, F. (2002). A long-tailed, seed-eating bird from the Early Cretaceous of China. Nature 418, 405–409. doi: 10.1038/nature00930
Zhou, Z., and Zhang, F. (2003a). Anatomy of the primitive bird Sapeornis chaoyangensis from the Early Cretaceous of Liaoning, China. Can. J. Earth Sci. 40, 731–747. doi: 10.1139/e03-011
Keywords: Paraves, Deinonychosauria, Dromaeosauridae, Microraptoria, Anchiornithinae, Unenlagiidae, Avialae
Citation: Agnolin FL, Motta MJ, Brissón Egli F, Lo Coco G and Novas FE (2019) Paravian Phylogeny and the Dinosaur-Bird Transition: An Overview. Front. Earth Sci. 6:252. doi: 10.3389/feart.2018.00252
Received: 07 September 2018; Accepted: 28 December 2018;
Published: 12 February 2019.
Edited by:
Corwin Sullivan, University of Alberta, CanadaReviewed by:
Thomas Alexander Dececchi, University of Pittsburgh, United StatesSpencer G. Lucas, New Mexico Museum of Natural History & Science, United States
Copyright © 2019 Agnolin, Motta, Brissón Egli, Lo Coco and Novas. This is an open-access article distributed under the terms of the Creative Commons Attribution License (CC BY). The use, distribution or reproduction in other forums is permitted, provided the original author(s) and the copyright owner(s) are credited and that the original publication in this journal is cited, in accordance with accepted academic practice. No use, distribution or reproduction is permitted which does not comply with these terms.
*Correspondence: Federico L. Agnolin, ZmVkZWFnbm9saW5AeWFob28uY29tLmFy