- 1Chair of Ecosystem Management, Institute of Terrestrial Ecosystems, ETH Zurich, Zurich, Switzerland
- 2Department of Zoology, University of Oxford, Oxford, United Kingdom
- 3Center for Innovation Research and Consultancy (CIRC), Pune, India
- 4Department of Ecology and Evolutionary Biology, University of Connecticut Storrs, Storrs, CT, United States
Density-dependent interactions between plants and their natural enemies, including fungal pathogens and insect herbivores, help maintain plant species coexistence and diversity at local scales (α-diversity). However, turnover in plant species composition across space also contributes to biodiversity at larger spatial scales (β-diversity). Despite mounting evidence that enemies can maintain α-diversity, we know little about their contributions to β-diversity. Additionally, in the light of widespread habitat fragmentation and potentially modified insect and pathogen communities in forest fragments, the effects of fragment area on their diversity-maintaining roles are largely unknown. We carried out a field experiment to investigate how natural enemies in impact tree α and β-diversity in a fragmented rainforest landscape in the Western Ghats, India. In 21 rainforest fragments, we suppressed insects and fungi/oomycetes with pesticides, and examined changes in the diversity of tree seedlings. We found that fungicide had no effect on α-diversity, but significantly decreased β-diversity (species turnover among plots). The facilitative effects of fungi and oomycetes on β-diversity, however, weakened as fragments decreased in area, indicating that certain specialized plant-pathogen interactions may be lost when fragments become smaller. Insecticide, in contrast, increased α-diversity but tended to decrease β-diversity between distant plots. In summary, we found that interactions between plants and their natural enemies help maintain β-diversity in large forest fragments but not in small fragments. Small fragments are often viewed as future reservoirs of biodiversity in human-dominated landscapes, but our findings suggest that modified interactions with natural enemies may result in the erosion of this diversity over time.
Introduction
Throughout the tropics, contiguous rainforest is being replaced by fragments of forest isolated within agricultural and urban landscapes (Laurance et al., 2011; Haddad et al., 2015). The long-term effects of this large-scale habitat loss are particularly concerning because tropical rainforests harbor so much of the Earth's terrestrial biodiversity (Willig et al., 2003; Corlett, 2016). Although fragmented forests typically support less diversity per unit area than contiguous forests (Turner, 1996; Ewers and Didham, 2006; Laurance et al., 2011), they may still act as reservoirs of biological diversity in otherwise transformed landscapes (Wright and Muller-Landau, 2006; Dent and Wright, 2009; Anand et al., 2010). The long-term conservation value of forest fragments will depend on the viability of small, isolated populations of plants and animals that they contain, and their capacities to withstand altered microclimatic conditions (Ewers and Banks-Leite, 2013) and increased exposure to human influences (Debinski and Holt, 2000). Critically, biodiversity within small forest fragments will require the continued operation of the ecological processes that generate and maintain diversity in intact forests.
One such ecological process is described by the Janzen-Connell hypothesis (Connell, 1971; Janzen, 1971). Specialized natural enemies such as insect herbivores and pathogens (which include fungi, oomycetes, bacteria, nematodes, viruses, and others) cause elevated mortality on seedlings growing near conspecific adults or at high conspecific density (Augspurger, 1984; Bell et al., 2006; Freckleton and Lewis, 2006), facilitating the long-term persistence of locally-rare species. Attack by both fungal (Mangan, 2010; Bagchi et al., 2014) and oomycete (Augspurger, 1984; Augspurger and Wilkinson, 2007) pathogens has been linked to density dependent mortality of tropical plant seedlings. Negative density-dependence of this sort is prevalent in tropical forest plant communities, especially at early life stages (Comita et al., 2014), and several studies have demonstrated its importance in maintaining plant α-diversity, i.e., diversity at local spatial scales (Webb and Peart, 1999; Harms et al., 2000; Metz et al., 2010; Bagchi et al., 2014). Such interactions may also, however, play an important role in maintaining plant β-diversity at larger spatial scales because local compositions of natural enemies may vary considerably in space depending upon local microclimatic factors (Weber, 1973; Liu and He, 2019). Spatial turnover in insect herbivory and fungal/oomycete disease incidence (henceforth collectively referred to as pathogens is expected to cause turnover in plant species compositions through space, therefore maintaining β-diversity. Although the role of natural enemies in maintaining α-diversity is well-studied, little is known about their role in maintaining β-diversity.
When forests are fragmented, resultant shifts in microenvironments near edges, including increased ambient light and temperature (Arroyo-Rodríguez et al., 2017), and reduced humidity (Ewers and Banks-Leite, 2013), can cause shifts in pathogen and insect communities (Benitez-Malvido et al., 1999; Tscharntke and Brandl, 2004; Crockatt, 2012; Grilli et al., 2017; Ruete et al., 2017; Bagchi et al., 2018). This can in turn affect the maintenance of α-diversity (Krishnadas et al., 2018) and β-diversity. Such altered microclimatic conditions extend far into forest fragments and can affect insect herbivory and fungal and oomycete disease incidence (Benitez-Malvido et al., 1999; Wirth, 2008; Ruiz-Guerra et al., 2010; Krishnadas et al., 2018). Both theoretical work (Holt et al., 1999; Gravel et al., 2011) and empirical data (Rossetti et al., 2017; Ruete et al., 2017; Bagchi et al., 2018) indicate that communities of herbivores and pathogens also become less specialized when forests are fragmented. As the strength of the Janzen-Connell mechanism increases with host-specificity of natural enemies (Sedio and Ostling, 2013), the effectiveness of the mechanism for coexistence could be reduced in forest fragments. Such effects can manifest due to edge effects (Krishnadas et al., 2018) but also due to reduced fragment area (Viswanathan et al., 2019), with important implications for the future of diversity now contained within small forest fragments.
We may expect the weakening of density-dependent plant-enemy interactions in small forest fragments to lead to a decrease in α-diversity over time, but potential effects on β-diversity are unclear. Certain patterns observed in fragmented landscapes, like the consistent reduction of β-diversity (Arroyo-Rodríguez et al., 2013) and a shift toward more homogenized ecological communities (Lôbo et al., 2011; Arroyo-Rodríguez et al., 2013), may be explained by the loss of density-dependent interactions. Although abiotic shifts that favor a subset of the plant species pool may explain these patterns to a large extent, biotic mechanisms may play important roles. Modified plant-enemy interactions in fragmented landscapes may manifest as reduced variation in top-down control within and among small fragments (Rooney et al., 2004), leading to reductions in β-diversity.
In this study, we sought to understand links between plant enemies, woody plant diversity and forest fragment area during the plant life stage extending from seedling germination until establishment. We asked the following questions: (1) How do insects and fungal and oomycete pathogens impact plant diversity at multiple spatial scales (α-diversity and β-diversity)? (2) Does fragment area influence how insects and pathogens impact plant diversity? With the assumption that insect herbivores and fungal and oomycete pathogens suppress plants through specialized density-dependent interactions at fine spatial scales, we hypothesized that they increase diversity at local spatial scales (α-diversity) and at large spatial scales (β-diversity) due to a spatial turnover in fine-scale natural enemy compositions. We also hypothesized that their diversity-maintaining roles weaken with fragmentation because we expected fewer and weaker density-dependent interactions in fragmented forests.
Methods
Study Area
We conducted the study in Kadamane Tea Estate (12.8639–12.9389 N and 75.6361–75.6833 E) located on the western slopes of the Western Ghats in Karnataka, India. The Western Ghats region (combined with Sri Lanka) is considered one of the world's “Hottest Biodiversity Hotspots” (Myers et al., 2000) and harbors over 4,700 species of flowering plants (Myers et al., 2000) and a high diversity of herbivorous insect species (Fonseca, 2009). A study in the region identified 226 species of pathogenic fungi from a sample of 639 tree species (Mohanan and Yesodharan, 2005). Kadamane is a 30 km2 private estate that spans elevations of 800–1,300 m and contains fragments of evergreen forest interspersed within tea plantations and natural grassland. Fragments in the estate, although isolated, are in all cases <100 m from other fragments or contiguous forest. They have been isolated for roughly 50 years (clearing for tea fields and roads primarily occurred in the late 1960s and early 1970s).
Study Design
The experiment included 21 forest fragments (area range 1–149 ha, mean 27.8 ha, Figure 1, with elevation range 918–1,071 m). Our basic experimental unit consisted of five plots (initially cleared of all existing young vegetation to monitor seedlings) of 1 m2, the standard plot size used in Bagchi et al. (2014), Krishnadas et al. (2019) and other studies that detected impacts on α-diversity. Three of these experimental units were established at 37 locations across the 21 fragments, separated from each other by ca. 20 m and located at least 50 m from the forest edge wherever possible to reduce edge effects. Because fragments varied in size, we determined the number of replicate locations (each with three experimental units) per fragment based on its area, so that similar proportional areas were sampled within each one. We therefore established experimental units at one (fragment area < 10 ha), two (10–40 ha), three (>50 ha), or four (in the 149 ha site) randomly selected locations within each fragment. This design yielded 555 plots from 111 experimental units, grouped around 37 locations distributed across the 21 forest fragments.
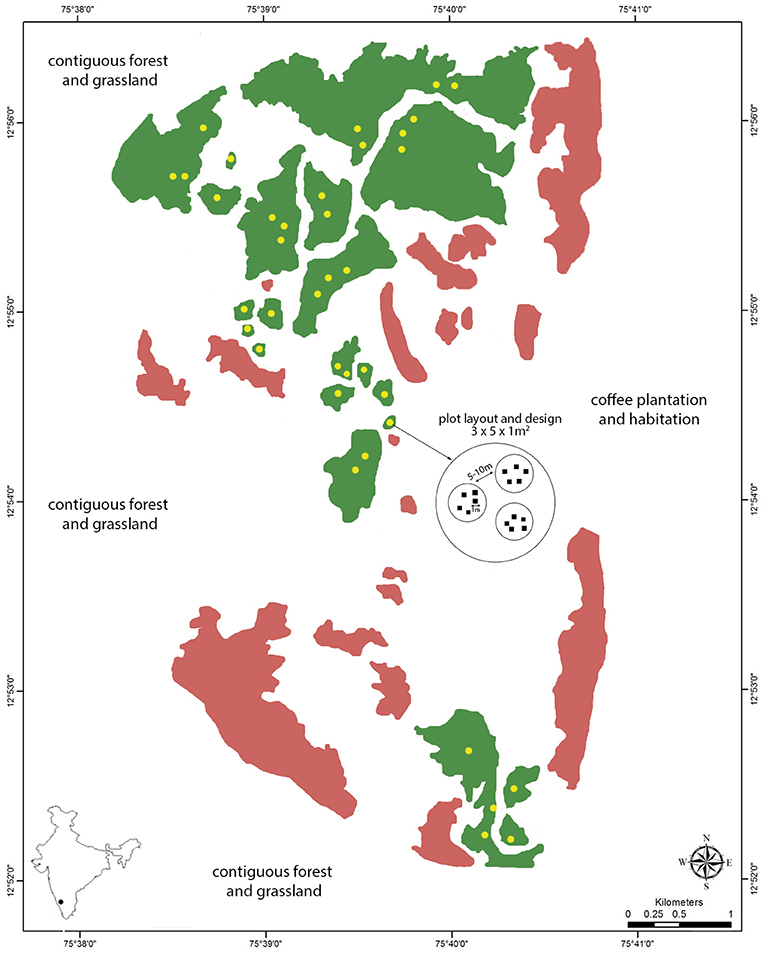
Figure 1. Map of the study site (Kadamane Estate) with focal fragments in green (N = 21), other (unsampled) forest fragments within the study site in pink, plot locations in yellow (N = 37), and the plot layout and design at each location (N = 555 plots). The study site is flanked by contiguous forest and high-altitude grassland to the north, south and west, and by coffee plantations and habitation to the east, as indicated by the text on the map.
The five seedling plots were randomly assigned to the following experimental treatments: (1) insect suppression, (2) fungal/oomycete suppression, (3) both insect and fungal/oomycete suppression, (4) water addition control, and (5) no-manipulation control. We suppressed insects by treating plots with a systemic insecticide, Thiamethoxam (Product name: Actara®). We suppressed fungal and oomycete pathogens by treating plots with a combination of a systemic fungicide Azoxystrobin (Product name: Amistar®), and a second fungicide that combines a contact fungicide, Mancozeb, and a systemic oomyticide, Metalxyl (Product name: Ridomil Gold®). These pesticides have been used widely in other ecological studies examining effects of pathogens and insects (Bell et al., 2006; Bagchi et al., 2014; Gripenberg et al., 2014; Gaviria and Engelbrecht, 2015; Krishnadas and Comita, 2018; Krishnadas et al., 2018). All three products were manufactured by Syngenta (Basel, Switzerland).
Insecticide and fungicide (any reference to fungicide henceforth includes oomyticide) treatments were started in July 2015 and ended in November 2016. Treatments were applied every 20 days during the dry season (November–May) and every 8 days during the wet season (June–October). They were applied more frequently in the wet season because heavy rain is likely to wash off pesticides sooner. To every insect suppression plot, we added 0.1 g of Actara dissolved in 50 ml of water (according to manufacturer specifications). To every fungal/oomycete suppression plot, we applied 0.02 ml of Amistar dissolved in 50 ml of water and 0.3 g of Ridomil Gold dissolved in 50 ml of water (both following manufacturer specifications, total of 100 ml of water). The insect and fungal/oomycete suppression plots received all three pesticides (total of 150 ml of water). Pesticide application added different amounts of water to each treatment so additional water was added (except in the combined fungicide and insecticide suppression plots) such that each plot, except the no-manipulation controls, received 150 ml of water at each pesticide application event. The no-manipulation control plots were completely unmanipulated.
We documented the species identity of each seedling in each plot during four censuses conducted in October–November 2015, April 2016, June–July 2016, and October 2016. During each census, we tagged each seedling that had established since the previous census with a uniquely numbered aluminum tag. We conducted a final census in November–December 2016 when we recorded the fate of tagged seedlings in all our plots.
We did not sample fungal, oomycete, and insect communities in our plots, so were unable to confirm the effectiveness of the biocides at suppressing their target organisms. However, the biocides used in this experiment have been found effective at reducing insect and pathogen related mortality and increasing plant recruitment and survival in other studies (Bagchi et al., 2010, 2014; Krishnadas et al., 2018).
Data Analysis
To test our hypothesis that insects and/or pathogens increase plant (seedling) diversity at local scales, we modeled the change in α-diversity (1 m2, Shannon Diversity Index) in each plot as a function of experimental treatment. To test our hypothesis that insects and/or pathogens increase plant diversity at large scales, we modeled the change in pairwise β-diversity (1 m2, Bray-Curtis dissimilarity) between each pair of plots in each experimental treatment (across all fragments) as a function of treatment, pairwise distance between plots and their interaction. In each of these analyses, we included fragment size as a covariate to test our hypothesis that larger fragments maintain greater diversity per unit area due to increased action by natural enemies. We checked and assumed Gaussian error distributions in all analyses of diversity.
We fitted linear mixed effects models using the lme4 package in R v3.5.0 (R Core Team, 2018). Models included nested random intercepts for seedling plot, experimental unit, and location to control for the dependence structure of the data. We used parametric bootstrapping with 1,000 iterations implemented with the bootMer function in the lme4 package (Bates et al., 2015) to obtain confidence intervals for parameter estimates and predictions from the models. We validated our models by checking the normality of residuals and unbiased predictors, and plotted absolute residuals against fitted values to test for homoscedasticity. We also used leverage plots to check whether any data points had disproportionately large effects on model results and we only report results that are robust to the exclusion of the most influential data points.
Direct Effects of Water, Fungicides, and Insecticides on Seedling Mortality/Growth
We did not find any significant effects of additional water on seedling mortality. We therefore treat the “water addition control” plots and the “no manipulation control” plots together in all analyses. Although we did not directly test for the effects of pesticides on plant growth, there is evidence that the effects of fungicides on mycorrhizal fungi are minimal (Maron et al., 2011). Maron et al. (2011) showed that these fungicides do not affect plant growth and survival, except through their action on pests. Although Thiamethoxam has been found to increase the vigor of certain crop species, especially in relation to environmental stressors like drought [for example in Almeida et al. (2014)], there is no evidence that it affects seedling survival.
Results
Overview of Seedling Dynamics
We recorded 7,404 newly germinated seedlings during the study period, of which 7,134 seedlings (12.85 seedlings m−2) of 106 species germinated during the 2016 monsoon (June–July and October censuses). We restricted all analyses to data from these last two censuses because they represented 96% of all seedlings recorded. Both fungicide and insecticide reduced overall seedling mortality from 65.8% in the control plots, to 59.2% in the fungicide plots, 48.3% in the insecticide plots, and 41.8% in plots with both pesticides. Two species, Syzygium rubicundum and Spatholobus purpureus, together accounted for 59.2% of the seedlings that germinated and 67.4% of the seedlings that died. Because seedlings of these two species were disproportionately abundant in our plots and masked patterns apparent in the rest of the community (104 species), we additionally reran all analyses after excluding these species.
α-Diversity
Seedling diversity within the 1 m2 plots (α-diversity) tended to decrease between germination and establishment (Table 1, Figure 2). Neither insecticide nor fungicide application significantly affected the change in α-diversity between germination and establishment (Table 1, Figure 2). When analyses focused on a reduced species assemblage by excluding seedlings of S. rubicundum and S. purpureus, decreases in α-diversity between germination and establishment were significantly greater in the control plots than in plots treated with insecticide. Fragment area had no noticeable effect on α-diversity or on the effects of biocides on α-diversity.
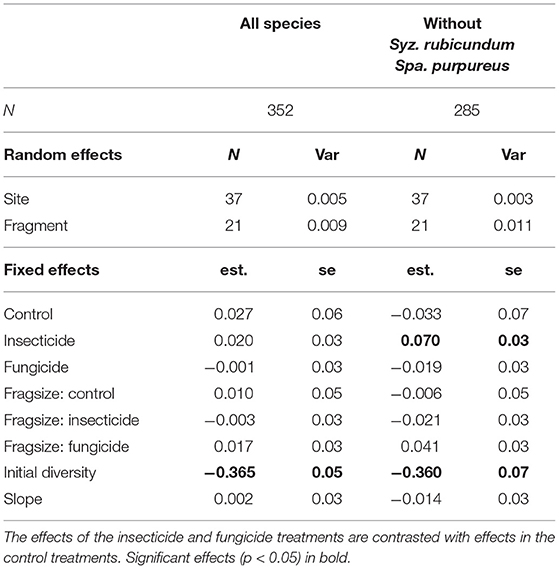
Table 1. Results of linear mixed effects models investigating the influence of experimental treatment and fragment size on changes in alpha diversity (Shannon's Index) of seedling assemblages from germination to establishment (1 m2 plots).
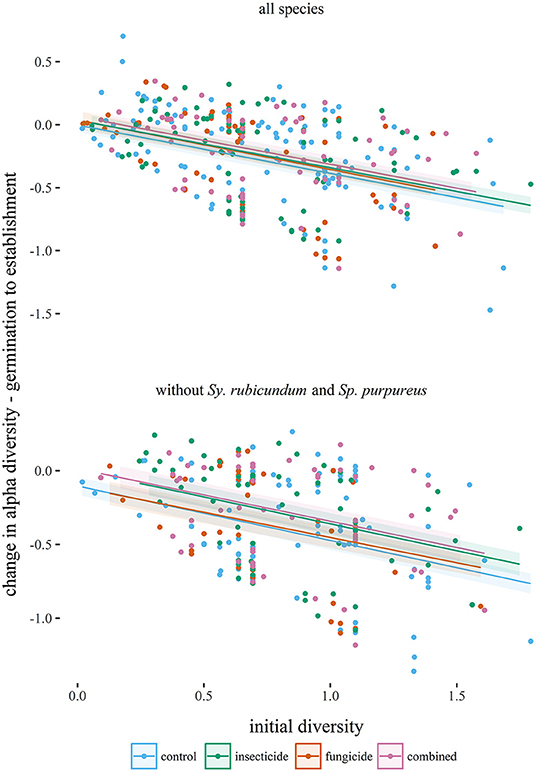
Figure 2. Changes in alpha diversity (Shannon index) of seedling assemblages (from germination to establishment) plotted against initial diversities of seedlings (1 m2 plots) in each experimental treatment. Lines are predicted from linear mixed effects models (Table 1) and are plotted with standard errors.
β-Diversity
Pairwise dissimilarity between 1 m2 plots (β-diversity) was higher for established seedlings than at germination in large fragments. β-diversity significantly increased with greater geographical distance between plots (Table 2, Figure 3), but that relationship decreased when plots were treated with fungicide or insecticide, and decreased significantly in the case of insecticide treatment. Increases in β-diversity between germination and establishment strengthened with fragment size (Table 2, Figure 3), but neither insecticide nor fungicide addition altered this effect significantly. However, when S. rubicundum and S. purpureus were excluded from this analysis and plots were treated with fungicide, the positive effect of fragment area was removed (Table 2, Figure 3).
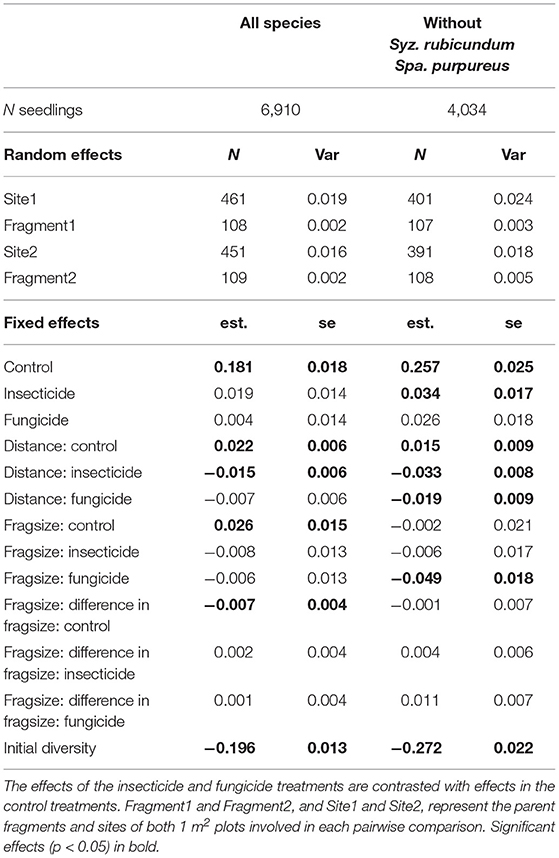
Table 2. Results of linear mixed effects models investigating the influence of experimental treatment, geographic distance between plots, mean pairwise fragment size, and the difference in pairwise fragment size, on changes in pairwise dissimilarity (standardized Bray-Curtis dissimilarities of seedling assemblages from germination to establishment in 1 m2 plots).
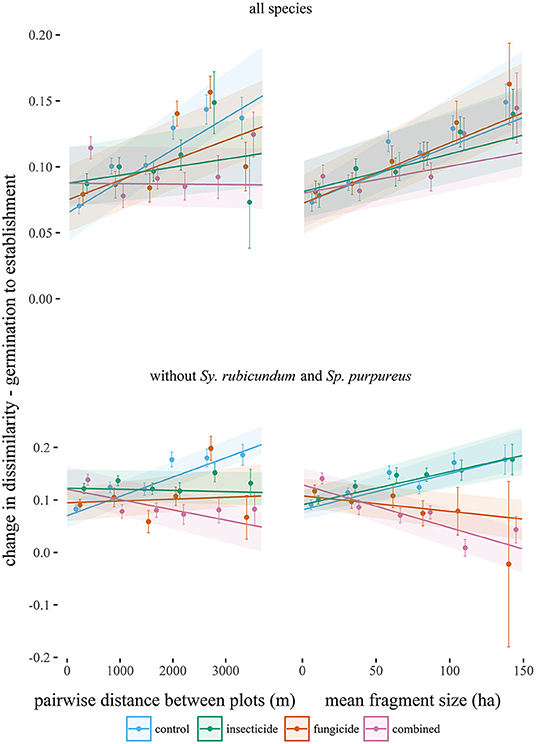
Figure 3. Changes in the pairwise Bray-Curtis dissimilarities (β-diversity) between seedling assemblages (from germination to establishment) plotted against the distance between plots, and against mean fragment size (from each pair of 1 m2 plots), in each experimental treatment. Points (standardized by the estimated random effects) equal mean changes in dissimilarity in each of seven binned distance or fragment size classes and are plotted with standard errors. Lines are predicted from linear mixed effects models (Table 2) and are plotted with standard errors.
Discussion
Enemy-Mediated Maintenance of Diversity at Multiple Spatial Scales
A focus on the Janzen-Connell hypothesis (and on associated density-dependent processes) as a mechanism for maintaining diversity in tropical forests has led to investigations of the effects of plant natural enemies on local diversity (α-diversity) (Comita et al., 2014). Very few studies have investigated the effects of natural enemies on large-scale and landscape level diversity (β-diversity), although it has been proposed that insect herbivores maintain plant β-diversity by driving trade-offs in resource allocation strategies (Fine et al., 2004; Lamarre et al., 2012). We found that insect herbivores and fungal and oomycete pathogens primarily affected β-diversity rather than α-diversity during the establishment of newly established seedlings in a rainforest in India.
Our original hypothesis was that the suppression of pathogens would influence α-diversity as observed in previous studies that focused on the seed-to-seedling transition (Bagchi et al., 2014; Krishnadas et al., 2018). The data presented here focus on a slightly later stage, extending from seedling germination until establishment during the 2016 monsoon. Our expectations hinged on the premise that natural enemies exert negative conspecific-density-dependent control of plant populations in our study, as has been observed during the seed-to-seedling transition, including at our study site (Bagchi et al., 2014; Krishnadas et al., 2018). Our results suggest neither a role for natural enemies, nor that α-diversity increases, during the germination to establishment transition, but instead indicates that this period does not involve community-wide specialized density-dependent interactions.
We instead found evidence that insect herbivores during this stage may be relatively generalist contrary to the narrative that they have community-wide specialized density-dependent effects (Comita et al., 2014). We found that insecticide significantly increased, rather than decreased, the seedling diversity of the assemblage without the two hyper-abundant species. Since the effects of generalist insects on plants will tend to be independent of plant conspecific densities, they are likely to homogenize plant species composition at local spatial scales by removing species intolerant to herbivores, thereby decreasing seedling diversity. Some studies (e.g., Bagchi et al., 2014; Gripenberg et al., 2014) have previously found evidence that insects suppress seed and seedling populations independent of fine-scale local densities.
Despite the lack of evidence that insects and pathogens increased plant α-diversity, our results suggest that primarily insects (but also, to a lesser extent, fungi, and oomycetes) enhanced dissimilarity between distant plots, and hence increased diversity at large spatial scales (β-diversity). One plausible explanation is that spatial variation in natural enemy communities generates heterogeneity in the amount of resources seedlings have to allocate to defense (Fine et al., 2004, 2006; Lamarre et al., 2012). Such trade-offs in resource allocation strategies have been linked to species turnover between different forest types (Fine et al., 2004); our results suggest that a similar pattern might manifest over more subtle environmental gradients.
Plant Diversity in Forest Fragments
Despite the relevance of fragmentation for the long-term conservation of biodiversity (Turner, 1996; Ewers and Didham, 2006; Laurance et al., 2011), effects of fragment area on young woody plant diversity (early life stages) have only rarely been investigated in tropical forests. Such effects of fragmentation can manifest differently at various spatial scales depending upon the underlying mechanisms that are modified. A study from the same study site found that edge effects (a driver closely associated with fragment area) manifest at fine spatial scales, and influence the maintenance of α-diversity but not of β-diversity (Krishnadas et al., 2019). Our results show that the detectable effects of fragment area are reversed, and manifest at large spatial scales (β-diversity) but not at fine spatial scales. Smaller fragments retain less β-diversity than large fragments during seedling establishment.
We show that the mechanism that underlies the low retention of β-diversity in small fragments may be the modification or weakening of biotic plant-enemy interactions. We demonstrate that the diversity-maintaining roles of the natural enemies are indeed influenced by fragment area, specifically that greater β-diversity is maintained in large fragments only when plots are not treated with fungicide (when plant-pathogen interactions are intact). A reduced role for fungi and oomycetes in maintaining β-diversity of small fragments may indicate that certain plant-pathogen interactions have been lost or weakened in these fragments as certain studies have found (Krishnadas and Comita, 2018; Krishnadas et al., 2018; Viswanathan et al., 2019).
We have assumed thus far that communities of herbivorous insects and pathogens, and their interactions with plants, are “natural” in this landscape. But given the history of recent fragmentation, proximity to intensive agriculture and increasing climatic fluctuations, natural processes in these forests may now be supplemented (or even suppressed) by those that involve alien pests (Jeger and Pautasso, 2008; Elad and Pertot, 2014; Hansen, 2015). Our results may therefore be representative of a new normal, or one that is dynamically changing with the emergence of new pests and novel interactions (Álvarez-Loayza et al., 2011; Velásquez et al., 2018) in this era of rapid global change.
Conclusions
As an increasingly large fraction of the Earth's biodiversity begins to be isolated within forest fragments (Haddad et al., 2015), it is important that we understand the long-term risks of this process, and strategize to minimize this risk. We found evidence that fungal and oomycete pathogens contributed less to β-diversity in smaller rainforest fragments than in large fragments, suggesting that the long-term stability of small forest fragments may be at risk. Our results have implications for the debate about whether several small fragments can serve the same purpose as a few large fragments. We suggest that diversity may be better preserved in large forest fragments, and that ensuring connectivity between smaller fragments may be important for the long-term conservation of tropical biodiversity.
Data Availability Statement
The datasets generated for this study are available on request to the corresponding author.
Author Contributions
RB, JG, OL, and AV conceptualized and designed the study. AV and GH conducted the experiments, collected, and entered data. AV and RB analyzed the data. AV and RB wrote the manuscript. JG and OL reviewed and revised the manuscript.
Funding
This research was funded by ETH Grant 42 13-1.
Conflict of Interest
The authors declare that the research was conducted in the absence of any commercial or financial relationships that could be construed as a potential conflict of interest.
Acknowledgments
We are especially grateful to Praveen Kumar and his family for help with logistics and data collection. Kadamane Estate provided us with an ideal set up for our study, a place to stay and people to help. We thank Mr. K. M. Cariappa, Senior Manager, Kadamane Estates Company, for his personal interest in the study. We are grateful to Vijay, Suresh, Netra, Kanjimalai and his family, Arun Kumar, Bhanu Sridharan, Ajith Ashokan, Akshay Surendra, Dayani Chakravarthy, Ansil Basheer, Vikrant Jathar, Santhosh Uthappa, Vinod Shanker, Bhuvanesh, and Nisarg Prakash for field assistance. We also thank Uma Shaanker and David Burslem for their comments.
References
Almeida, A. D. S., Deuner, C., Borges, C. T., Meneghello, G. E., Jauer, A., and Villela, F. A. (2014). Treatment of rice seeds with thiamethoxam: reflections on physiological performance. J. Seed Sci. 36, 392–398. doi: 10.1590/2317-1545v36n4980
Álvarez-Loayza, P., White, J. F. Jr., Torres, M. S., Balslev, H., Kristiansen, T., Svenning, J. C., et al. (2011). Light converts endosymbiotic fungus to pathogen, influencing seedling survival and niche-space filling of a common tropical tree, iriartea deltoidea. PLoS ONE 6:e16386. doi: 10.1371/journal.pone.0016386
Anand, M. O., Krishnaswamy, J., Kumar, A., and Archana, B. (2010). Sustaining biodiversity conservation in human-modified landscapes in the western ghats: remnant forests matter. Biol. Conserv. 143, 2363–2374. doi: 10.1016/j.biocon.2010.01.013
Arroyo-Rodríguez, V., Rös, M., Escobar, F., Melo, F. P., Santos, B. A., Tabarelli, M., et al. (2013). Plant β-diversity in fragmented rain forests: testing floristic homogenization and differentiation hypotheses. J. Ecol. 101, 1449–1458. doi: 10.1111/1365-2745.12153
Arroyo-Rodríguez, V., Saldaña-Vázquez, R. A., Fahrig, L., and Santos, B. A. (2017). Does forest fragmentation cause an increase in forest temperature? Ecol. Res. 32, 81–88. doi: 10.1007/s11284-016-1411-6
Augspurger, C. K. (1984). Seedling survival of tropical tree species: interactions of dispersal distance, light-gaps, and pathogens. Ecology 65, 1705–1712. doi: 10.2307/1937766
Augspurger, C. K., and Wilkinson, H. T. (2007). Host specificity of pathogenic pythium species: implications for tree species diversity. Biotropica 39, 702–708. doi: 10.1111/j.1744-7429.2007.00326.x
Bagchi, R., Brown, L. M., Elphick, C. S., Wagner, D. L., and Singer, M. S. (2018). Anthropogenic fragmentation of landscapes: mechanisms for eroding the specificity of plant–herbivore interactions. Oecologia 187, 521–533. doi: 10.1007/s00442-018-4115-5
Bagchi, R., Gallery, R. E., Gripenberg, S., Gurr, S. J., Narayan, L., Addis, C. E., et al. (2014). Pathogens and insect herbivores drive rainforest plant diversity and composition. Nature 506, 85–88. doi: 10.1038/nature12911
Bagchi, R., Swinfield, T., Gallery, R. E., Lewis, O. T., Gripenberg, S., Narayan, L., et al. (2010). Testing the Janzen-Connell mechanism: pathogens cause overcompensating density dependence in a tropical tree. Ecol. Lett. 13, 1262–1269. doi: 10.1111/j.1461-0248.2010.01520.x
Bates, D., Maechler, M., Bolker, B., and Walker, S. (2015). Fitting linear mixed-effects models using lme4. J. Stat. Softw. 67, 1–48. doi: 10.18637/jss.v067.i01
Bell, T., Freckleton, R. P., and Lewis, O. T. (2006). Plant pathogens drive density-dependent seedling mortality in a tropical tree. Ecol. Lett. 9, 569–574. doi: 10.1111/j.1461-0248.2006.00905.x
Benitez-Malvido, J., Garcia-Guzmán, G., and Kossmann-Ferraz, I. D. (1999). Leaf-fungal incidence and herbivory on tree seedlings in tropical rainforest fragments: an experimental study. Biol. Conserv. 91, 143–150. doi: 10.1016/S0006-3207(99)00090-7
Comita, L. S., Queenborough, S. A., Murphy, S. J., Eck, J. L., Xu, K., Krishnadas, M., et al. (2014). Testing predictions of the Janzen–Connell hypothesis: a meta-analysis of experimental evidence for distance- and density-dependent seed and seedling survival. J. Ecol. 102, 845–856. doi: 10.1111/1365-2745.12232
Connell, J. H. (1971). “On the role of natural enemies in preventing competitive exclusion in some marine animals and in rain forest trees” in Dynamics of Populations, eds P. J. den Boer and G. Gradwell (Wageningen: PUDOC), 298–312.
Corlett, R. T. (2016). Plant diversity in a changing world: status, trends, and conservation needs. Plant Divers. 38, 10–16. doi: 10.1016/j.pld.2016.01.001
Crockatt, M. E. (2012). Are there edge effects on forest fungi and if so do they matter? Fungal Biol. Rev. 26, 94–101. doi: 10.1016/j.fbr.2012.08.002
Debinski, D. M., and Holt, R. D. (2000). A survey and overview of habitat fragmentation experiments. Conserv. Biol. 14, 342–355. doi: 10.1046/j.1523-1739.2000.98081.x
Dent, D. H., and Wright, S. J. (2009). The future of tropical species in secondary forests: a quantitative review. Biol. conserv. 142, 2833–2843. doi: 10.1016/j.biocon.2009.05.035
Elad, Y., and Pertot, I. (2014). Climate change impacts on plant pathogens and plant diseases. J. Crop Improv. 28, 99–139. doi: 10.1080/15427528.2014.865412
Ewers, R. M., and Banks-Leite, C. (2013). Fragmentation impairs the microclimate buffering effect of tropical forests. PLoS ONE 8:e58093. doi: 10.1371/journal.pone.0058093
Ewers, R. M., and Didham, R. K. (2006). Confounding factors in the detection of species responses to habitat fragmentation. Biol. Rev. Camb. Philos. Soc. 81, 117–142. doi: 10.1017/S1464793105006949
Fine, P. V. A., Mesones, I., and Coley, P. D. (2004). Herbivores promote habitat specialization by trees in Amazonian forests. Science 305, 663–665. doi: 10.1126/science.1098982
Fine, P. V. A., Miller, Z. J., Mesones, I., Irazuzta, S., Appel, H. M., Stevens, M. H., et al. (2006). The growth-defense trade-off and habitat specialization by plants in amazonian forests. Ecology 87, S150–S162. doi: 10.1890/0012-9658(2006)87[150:tgtahs]2.0.co;2
Fonseca, C. R. (2009). The silent mass extinction of insect herbivores in biodiversity hotspots. Conserv. Biol. 23, 1507–1515. doi: 10.1111/j.1523-1739.2009.01327.x
Freckleton, R. P., and Lewis, O. T. (2006). Pathogens, density dependence and the coexistence of tropical trees. Proc. Royal Soc. B Biol. Sci. 273, 2909–2916. doi: 10.1098/rspb.2006.3660
Gaviria, J., and Engelbrecht, B. M. J. (2015). Effects of drought, pest pressure and light availability on seedling establishment and growth: their role for distribution of tree species across a tropical rainfall gradient. PLoS ONE 10:e0143955. doi: 10.1371/journal.pone.0143955
Gravel, D., Massol, F., Canard, E., Mouillot, D., and Mouquet, N. (2011). Trophic theory of island biogeography. Ecol. Lett. 14, 1010–1016. doi: 10.1111/j.1461-0248.2011.01667.x
Grilli, G., Longo, S., Huais, P. Y., Pereyra, M., Verga, E. G., Urcelay, C., et al. (2017). Fungal diversity at fragmented landscapes: synthesis and future perspectives. Curr. Opin. Microbiol. 37, 161–165. doi: 10.1016/j.mib.2017.09.003
Gripenberg, S., Bagchi, R., Gallery, R. E., Freckleton, R. P., Narayan, L., and Lewis, O. T. (2014). Testing for enemy-mediated density-dependence in the mortality of seedlings: field experiments with five neotropical tree species. Oikos 123, 185–193. doi: 10.1111/j.1600-0706.2013.00835.x
Haddad, N. M., Brudvig, L. A., Clobert, J., Davies, K. F., Gonzalez, A., Holt, R. D., et al. (2015). Habitat fragmentation and its lasting impact on earth's ecosystems. Sci. Adv. 1:e1500052. doi: 10.1126/sciadv.1500052
Hansen, E. M. (2015). Phytophthora species emerging as pathogens of forest trees. Curr. Forestry Rep. 1, 16–24. doi: 10.1007/s40725-015-0007-7
Harms, K. E., Wright, S. J., Calderon, O., Hernandez, A., and Herre, E. A. (2000). Pervasive density-dependent recruitment enhances seedling diversity in a tropical forest. Nature 404, 493–495. doi: 10.1038/35006630
Holt, R. D., Lawton, J. H., Polis, G. A., and Martinez, N. D. (1999). Trophic rank and the species-area relationship. Ecology 80, 1495–1504. doi: 10.1890/0012-9658(1999)080[1495:TRATSA]2.0.CO;2
Janzen, D. H. (1971). Seed predation by animals. Annu. Rev. Ecol. Syst. 2, 465–492. doi: 10.1146/annurev.es.02.110171.002341
Jeger, M. J., and Pautasso, M. (2008). Plant disease and global change – the importance of long-term data sets. New Phytol. 177, 8–11. doi: 10.1111/j.1469-8137.2007.02312.x
Krishnadas, M., Bagchi, R., Sridhara, S., and Comita, L. S. (2018). Weaker plant-enemy interactions decrease tree seedling diversity with edge-effects in a fragmented tropical forest. Nat. Commun. 9:4523. doi: 10.1038/s41467-018-06997-2
Krishnadas, M., and Comita, L. S. (2018). Influence of soil pathogens on early regeneration success of tropical trees varies between forest edge and interior. Oecologia 186, 259–268. doi: 10.1007/s00442-017-4006-1
Krishnadas, M., Kumar, A. N., and Comita, L. S. (2019). Edge effects reduce α-diversity but not β-diversity during community assembly in a human-modified tropical forest. Ecol. Appl. 29:e01996. doi: 10.1002/eap.1996
Lôbo, D., Leão, T., Melo, F. P. L., Santos, A. M., and Tabarelli, M. (2011). Forest fragmentation drives Atlantic forest of northeastern brazil to biotic homogenization. Divers. Distrib. 17, 287–296. doi: 10.1111/j.1472-4642.2010.00739.x
Lamarre, G. P. A., Baraloto, C., Fortunel, C., Dávila, N., Mesones, I., Rios, J. G., et al. (2012). Herbivory, growth rates, and habitat specialization in tropical tree lineages: implications for amazonian beta-diversity. Ecology 93, S195–S210. doi: 10.1890/11-0397.1
Laurance, W. F., Camargo, J. L., Luizão, R. C., Laurance, S. G., Pimm, S. L., Bruna, E. M., et al. (2011). The fate of amazonian forest fragments: a 32-year investigation. Biol. Conserv. 144, 56–67. doi: 10.1016/j.biocon.2010.09.021
Liu, Y., and He, F. (2019). Incorporating the disease triangle framework for testing the effect of soil-borne pathogens on tree species diversity. Funct. Ecol. 33, 1211–1222. doi: 10.1111/1365-2435.13345
Mangan, S. A., Schnitzer, S. A., Herre, E. A., Mack, K. M. L., Valencia, M. C., Sanchez, E. I., et al. (2010). Negative plant-soil feedback predicts tree-species relative abundance in a tropical forest. Nature 466, 752–755. doi: 10.1038/nature09273
Maron, J. L., Marler, M., Klironomos, J. N., and Cleveland, C. C. (2011). Soil fungal pathogens and the relationship between plant diversity and productivity. Ecol. Lett. 14, 36–41. doi: 10.1111/j.1461-0248.2010.01547.x
Metz, M. R., Sousa, W. P., and Valencia, R. (2010). Widespread density-dependent seedling mortality promotes species coexistence in a highly diverse amazonian rain forest. Ecology 91, 3675–3685. doi: 10.1890/08-2323.1
Mohanan, C., and Yesodharan, K. (2005). Biodiversity of Plant Pathogenic Fungi in the Kerala Part of the Western Ghats. Final Report Project No KFRI 375, Forest Research Institute, Kerala.
Myers, N., Mittermeier, R. A., Mittermeier, C. G., Da Fonseca, G. A., and Kent, J. (2000). Biodiversity hotspots for conservation priorities. Nature 403, 853–858. doi: 10.1038/35002501
R Core Team (2018). R: A Language and Environment for Statistical Computing. Vienna: R Foundation for Statistical Computing.
Rooney, T. P., Wiegmann, S. M., Rogers, D. A., and Waller, D. M. (2004). Biotic impoverishment and homogenization in unfragmented forest understory communities. Conserv. Biol. 18, 787–798. doi: 10.1111/j.1523-1739.2004.00515.x
Rossetti, M. R., Tscharntke, T., Aguilar, R., and Batáry, P. (2017). Responses of insect herbivores and herbivory to habitat fragmentation: a hierarchical meta-analysis. Ecol. Lett. 20, 264–272. doi: 10.1111/ele.12723
Ruete, A., Snäll, T., Jonsson, B. G., and Jönsson, M. (2017). Contrasting long-term effects of transient anthropogenic edges and forest fragment size on generalist and specialist deadwood-dwelling fungi. J. Appl. Ecol. 54, 1142–1151. doi: 10.1111/1365-2664.12835
Ruiz-Guerra, B., Guevara, R., Mariano, N. A., and Dirzo, R. (2010). Insect herbivory declines with forest fragmentation and covaries with plant regeneration mode: evidence from a Mexican tropical rain forest. Oikos 119, 317–325. doi: 10.1111/j.1600-0706.2009.17614.x
Sedio, B. E., and Ostling, A. M. (2013). How specialised must natural enemies be to facilitate coexistence among plants? Ecol. Lett. 16, 995–1003. doi: 10.1111/ele.12130
Tscharntke, T., and Brandl, R. (2004). Plant-insect interactions in fragmented landscapes. Annu. Rev. entomol. 49, 405–430. doi: 10.1146/annurev.ento.49.061802.123339
Turner, I. M. (1996). Species loss in fragments of tropical rain forest: a review of the evidence. J. Appl. Ecol. 33, 200–209. doi: 10.2307/2404743
Velásquez, A. C., Castroverde, C. D. M., and He, S. Y. (2018). Plant–pathogen warfare under changing climate conditions. Curr. Biol. 28, R619–R634. doi: 10.1016/j.cub.2018.03.054
Viswanathan, A., Ghazoul, J., Honwad, G., Arun Kumar, N., and Bagchi, R. (2019). The effects of rainforest fragment area on the strength of plant–pathogen interactions. Biol. Lett. 15:20180493. doi: 10.1098/rsbl.2018.0493
Webb, C. O., and Peart, D. R. (1999). Seedling density dependence promotes coexistence of Bornean rain forest trees. Ecology 80, 2006–2017. doi: 10.1890/0012-9658(1999)080[2006:SDDPCO]2.0.CO;2
Weber, G. F. (1973). Bacterial and Fungal Diseases of Plants in the Tropics. Gainesville, FL: University of Florida Press.
Willig, M. R., Kaufman, D. M., and Stevens, R. D. (2003). Latitudinal gradients of biodiversity: pattern, process, scale, and synthesis. Annu. Rev. Ecol. Evol. Syst. 34, 273–309. doi: 10.1146/annurev.ecolsys.34.012103.144032
Wirth, R., Meyer, S. T., Leal, I. R., and Tabarelli, M. (2008). “Plant herbivore interactions at the forest edge” in Progress in Botany, eds U. Lüttge, W. Beyschlag, and J. Murata (Berlin; Heidelberg: Springer), 423–448.
Keywords: insects, fungi, oomycetes, negative density-dependence (NDD), Janzen-Connell, coexistence
Citation: Viswanathan A, Ghazoul J, Lewis OT, Honwad G and Bagchi R (2020) Effects of Forest Fragment Area on Interactions Between Plants and Their Natural Enemies: Consequences for Plant Diversity at Multiple Spatial Scales. Front. For. Glob. Change 2:88. doi: 10.3389/ffgc.2019.00088
Received: 08 October 2019; Accepted: 09 December 2019;
Published: 08 January 2020.
Edited by:
Julieta Benitez-Malvido, National Autonomous University of Mexico, MexicoReviewed by:
Denita Hadziabdic, The University of Tennessee, Knoxville, United StatesPeter Matthew Scott, The New Zealand Institute for Plant and Food Research Ltd., New Zealand
Copyright © 2020 Viswanathan, Ghazoul, Lewis, Honwad and Bagchi. This is an open-access article distributed under the terms of the Creative Commons Attribution License (CC BY). The use, distribution or reproduction in other forums is permitted, provided the original author(s) and the copyright owner(s) are credited and that the original publication in this journal is cited, in accordance with accepted academic practice. No use, distribution or reproduction is permitted which does not comply with these terms.
*Correspondence: Ashwin Viswanathan, YXNod2ludjIwMDVAZ21haWwuY29t