- 1Department of Microbiology and Immunology, Loyola University Chicago, Maywood, IL, United States
- 2Department of Obstetrics, Gynecology and Reproductive Sciences, University of California, San Diego, La Jolla, CA, United States
Polymicrobial or mixed urine cultures of more than one predominant microbe confound clinical urinary tract infection diagnosis. The current College of American Pathologists clinical laboratory standard states that a urine sample cultured with more than two isolates with >10,000 colony forming units/ml is to be considered contaminated. However, the presence of urinary sample bacteria in individuals without urinary symptoms (referred to as asymptomatic bacteriuria) is common especially in older people and in pregnant individuals. Furthermore, the discovery of an indigenous urinary microbiome (urobiome) in healthy humans throughout life from shortly after birth to death conflicts with the long-standing notion that urine derived from sterile filtered blood should be sterile above the urethral sphincter. Polymicrobial infections are not consistent with Koch’s postulates that a single pathogen is causal for disease. In this review, we will discuss current standards of contamination, how to reconcile the sterility of urine with the existence of the urobiome, a history of polymicrobial infections, and why re-examining current practices is essential for the practice of medicine, improving quality of life, and potentially saving lives.
1 Introduction
The College of American Pathologists guidance states “a contaminated urine culture was defined as the presence of more than 2 isolates at greater than or equal to 10–000 CFU/mL” (Valenstein and Meier, 1998; Bekeris et al., 2008). This includes commensal microbes thought to be of skin origin or, in adult females, vulvo-vaginal contaminants (Brubaker et al., 2021a). Standard practice is to resample although often the same result is obtained. Then, the predominant microbe is reported (often E. coli), while the other detected microbes are often ignored (Sfeir and Hooton, 2018). Examples include reports of “contamination” or “mixed flora” in asymptomatic bacteriuria of pregnant individuals (46.7%) (O'Leary et al., 2020), in general practice (54.9%) (Hansen et al., 2022), and in outpatient clinics (46.2%) (Whelan et al., 2022). This is comparable to results obtained with a multiplex polymerase chain reaction (M-PCR) panel, where 56.1% of an older cohort (≥65 years old) with diagnosed urinary tract infection (UTI) had polymicrobial (more than one microbe) results (Vollstedt et al., 2020).
While the method of urine collection can sample different aspects of the urinary tract (e.g., suprapubic aspirate and transurethral catheter: bladder and upper urinary tract; midstream void: entire urinary tract including urethra and peri-urethral skin plus urogenital regions), there are subtle differences in microbes detected depending on sampling technique (Chen et al., 2020; Pohl et al., 2020; Brubaker et al., 2021a; Wang et al., 2023; Du et al., 2024; Shafik et al., 2005; McFadyen and Eykyn, 1968; Wolfe et al., 2012) Table 1. Nevertheless, midstream void or the so-called “clean catch” is most often used in clinical practice (LaRocco et al., 2015; Moreland et al., 2024). A recent meta-analysis of urine collection methods and contamination concluded that methods to decrease contamination (e.g. cleansing, boric acid preservative, and refrigerating urine sample to prevent nonspecific growth) were of limited value (LaRocco et al., 2015). The possibility remains that many microbes reported as contamination or mixed flora could represent potential polymicrobial infection that, in some cases, may breach the renal urine blood barrier and progress to urosepsis (Siegman-Igra, 1994; Siegman-IgraY, 1994; Peach et al., 2016; Akhtar et al., 2021; Collaborators, GBD 2021 Antimicrobial Resistance, 2024). Distinguishing contamination from the presence of clinically relevant microbes is essential to test interpretation and reporting. It is helpful to understand the history of urine culture contamination.
2 Origins of urine sample contamination
The belief that urine is sterile above the urethral sphincter is attributed to Pasteur and his studies proposing germ theory in the 1860s (Asscher et al., 1966; Roll-Hansen, 1979; Brubaker et al., 2023). The seminal fact omitted in many contemporary accounts is that Pasteur boiled the urine, vacuum sealed the flasks and observed no growth. Indeed, Pasteur considered urine alone to be an excellent bacterial growth media (Asscher et al., 1966). The point of his famous experiment was to demonstrate that the growth he observed in urine open to the environment resulted from microbes and not spontaneous generation from miasma (Roll-Hansen, 1979). Later publications by Roberts reported no detectable microbes in fresh urine of healthy subjects using the techniques of the day (e.g., microscopy) (Roberts, 1881). However, all samples left open to the air and at room temperature developed cloudiness and an ammonia odor in two to three days. This was attributed to microbes that could metabolize urea (Roberts, 1881) and later shown to be due to urease expressing facultative anaerobes such as Proteus (Armbruster et al., 2017).
The success of Koch’s postulates in identifying single microbes as causes of mortal diseases led to a quantum advance in the diagnosis of infectious disease in the late nineteenth century (Blevins and Bronze, 2010). As culture methods evolved for the detection of UTI-associated pathogens, protocols that detected the most common pathogen, Bacterium coli commune (later Escherichia coli), became standard clinical laboratory practice (Friedmann, 2014). Although multiple publications during the twentieth century reported microbes in the “sterile” urine of healthy individuals without symptoms (Hort, 1914; Marple, 1941; Philpot, 1956; McFadyen and Eykyn, 1968; Maskell, 1986; Maskell, 1988), special methods were necessary to culture these presumably “uncultivatable” microbes (Maskell, 1988; Khasriya et al., 2013; Hilt et al., 2014; Price et al., 2016; Legaria et al., 2022), and the dogma remained that urine was sterile in the absence of clinical conditions, such as UTI. Thus, contamination as it is currently defined is thought to arise from extravesicular (outside the bladder) sources and be unrelated to the cause of symptoms or infection; contamination is an artifact of urine sample collection (Valenstein and Meier, 1998; Bekeris et al., 2008; LaRocco et al., 2015). The prevailing view is that urine specimens can easily become contaminated with periurethral, epidermal, perianal, and vaginal flora (LaRocco et al., 2015). One report defined contamination as “bacteria that are found in normal vaginal or skin flora and do not cause UTI” (Blake and Doherty, 2006).
Urine culture contaminants attributed to dermal origin (skin) are usually identified as Gram-positive diphtheroids (Corynebacteria, club-like), Gram-positive clustered cocci (Staphylococcus), and Gram-positive cocci (Streptococcus, Micrococcus). A survey of the human skin microbiome identifying the ten most abundant taxa in four different regions of skin (dry, moist, sebaceous, and feet) found 22 different microbes. Of these, 21 are Gram-positive aerobes, microaerophiles or facultative anaerobes (Byrd et al., 2018) (Supplementary Table 1). Three are within the Viridians group streptococci (VGS) (Doern and Burnham, 2010). Only one is Gram-negative; the anaerobic coccus Veillonella parvula (found only in the dry region sample). Thus, skin contamination would be consistent with Gram-positive commensals.
The vaginal microbiome changes with age and reproductive status (menarche, reproductive age and post-menopausal) (Ravel et al., 2011; Nunn and Forney, 2016; Saraf et al., 2021; Park et al., 2023). Consequently, vaginal microbiota can vary with the patient. In general, the healthy vaginal microbiome is predominated by Lactobacilli, although other taxa have been observed (Ravel et al., 2011; Nunn and Forney, 2016; Saraf et al., 2021) (Supplementary Table 2). Vaginal contaminants are usually identified as Gram-positive bacilli and are attributed to commensal flora. These include L. iners, L. crispatus, L. gasseri and L. jensenii but can also include Gardnerella vaginalis (Gram-variable) and Gram-positive anaerobe Atopobium vaginae (now known as Fannyhessea vaginae) (Nouioui et al., 2018).
The vulvar microbiome has recently been investigated and includes representative taxa from both skin and vagina, including the genera Corynebacterium, Lactobacillus, Staphylococcus, Prevotella, Propionibacterium (Cutibacterium) and Finegoldia (Pagan et al., 2021). These microbes are Gram-positive diphtheroids, rods, and cocci except for the Gram-negative anaerobe Prevotella. Assessing gastrointestinal contamination including perianal and perineal regions becomes problematic as these microbes are facultative anaerobes that have also been identified as uropathogens. These include the genera Escherichia, Pseudomonas, Klebsiella, Proteus, and Gram positive Enterococcus commonly reported in UTI and are the microbes most often detected using current diagnostic techniques (urine dipstick and SUC) (Flores-Mireles et al., 2015; Moreland et al., 2023) Tables 2 and 3. Consequently, they are diagnosed as uropathogens rather than perineal contaminants.
Guidelines for common microbial contaminants in blood culture are available (Palavecino et al., 2024) and the Centers for Disease Control maintains a list of microbes that have been detected as commensals in UTI and blood infections (CDC, 2022). However, a list of urinary tract microbial contaminants is much more nebulous beyond listing niches (periurethral, epidermal, perianal, and vaginal), or assuming that microbes that are commensals in one niche are commensals in another (Blake and Doherty, 2006; LaRocco et al., 2015) (Supplementary Tables 1, 2).
Thus, in current clinical diagnostic practice, if a sample contains mixed flora, it is the number of different microbes (≥ 2 or more at 104 CFU/ml) that determines contamination and not the actual microbe unless it is a commonly recognized urinary pathogen (Bekeris et al., 2008; LaRocco et al., 2015; Sfeir and Hooton, 2018; Mancuso et al., 2023).
3 Limitations of diagnostic standard urine culture
Today, diagnosis of UTIs typically relies on patient symptoms and urinalysis. The latter uses urine dipstick testing and, in some cases, the standard urine culture (SUC) method (Chambliss and Van, 2022; Moreland et al., 2024; Werneburg et al., 2023) Table 2. Urine dipstick testing, frequently used to determine further testing, such as urine cultures is reviewed elsewhere (Moreland et al., 2024; Chambliss and Van, 2022). The limitations of SUC have been identified and are starting to impact current diagnostics (Price et al., 2017; Dixon et al., 2020; Wojno et al., 2020; Brubaker et al., 2023; Gleicher et al., 2024; Werneburg and Hsieh, 2024).
It is now well recognized that SUC under aerobic conditions (Gillespie et al., 1960), or even under 5% CO2, detects a limited number of microbes, almost all facultative anaerobes (Price et al., 2016, 2017; Wojno et al., 2020; Brubaker et al., 2023; Festa et al., 2023). As a result, reports based on SUC, including almost all literature to date, repeatedly document a constellation of the same microbes from the genera Escherichia, Pseudomonas, Klebsiella, Proteus, Staphylococcus, and Enterococcus, with Escherichia coli by far considered the predominant cause of UTI (Table 3) (Flores-Mireles et al., 2015; Kline and Lewis, 2016; Mancuso et al., 2023; Moreland et al., 2023; Werneburg et al., 2023; Timm et al., 2025). However, these results have been obtained because SUC was designed to detect fast growing, non-fastidious, facultative anaerobes, and thus fails to detect many other microbes. For example, a recent study directly compared SUC results to those of a multiplex polymerase chain reaction (M-PCR) panel for a cohort of 1,132 diagnosed UTI patients. M-PCR detected microbes in 823 of these patients, who also exhibited elevated infection-associated urine biomarkers (Haley et al., 2024). Of the 10 microbes most detected by M-PCR, only 4 were detected by SUC with 2 of those often not detected (Haley et al., 2024). Most striking was the failure of SUC to detect 3 of the 5 microbes most detected by M-PCR. These were the genera Aerococcus and Actinotignum and the Viridians group Streptococcus (including S. anginosus, S. oralis, and S. gallolyticus subsp. pasteurianus (formerly known as Streptococcus pasteurianus) (Doern and Burnham, 2010). Thus, except for E. coli, all known uropathogens (“the usual suspects”) represented only 13% or less of UTIs diagnosed with symptoms (Haley et al., 2024) (Table 3).
One outstanding issue with SUC has been the diagnosis of sterile pyuria, defined as positive for white blood cells but negative urine cultures in patients that report UTI symptoms (Wise and Schlegel, 2015; Horton et al., 2018; Cohen et al., 2019; Xu et al., 2024). Yet, a recent report suggests that Actinotignum (which SUC cannot detect but M-PCR diagnostics finds to be quite common) may be an underlying cause of sterile pyuria (Horton et al., 2018). Until recently, however, few had questioned the standard diagnostic method as flawed. As this standard method supports the established dogma that UTIs are caused by microbes arising from the gastrointestinal tract and, in females, vulvo-vagina reservoirs, it has, for the most part, gone unchallenged (Timm et al., 2025).
4 The urobiome “complication”
With the advent of DNA-based techniques (metagenomics) and enhanced culture methods (metaculturomics), the existence of female urethral and bladder microbiota has been confirmed with subtle differences existing between the two (Chen et al., 2020; Wang et al., 2023). Thus, we now know that the typical human urinary tract above the urinary sphincter is not sterile; instead, it contains an indigenous urinary microbiome (also known as the urobiome) (Price et al., 2020; Brubaker et al., 2021b; Du et al., 2024). We also know that the urobiome can have multiple healthy states (Pearce et al., 2014; Price et al., 2020; Jayalath and Magana-Arachchi, 2022; Joos et al., 2024). Moreover, many adult males and most adult females have a detectable urobiome without experiencing relevant urinary symptoms. Clearly, this does not mean we all have a UTI (Finucane, 2017). Consistent with clinical diagnosis of UTI, in the absence of relevant urinary symptoms, there is no “infection.” Diagnosis of a UTI requires that the patient exhibits at host response, and typically experiences symptoms (including urgency, frequency, urinary incontinence, and/or pain) (Anger et al., 2019).
Within any microbiome, microbes can be classified into 6 categories: non-pathogen (i.e., those that do not cause disease), pathogen (i.e., those that cause disease), commensal (i.e., those resident within the tissue and benefiting the host), symbiont (i.e., those resident within the tissue and benefiting both the host and the microbe), colonizer (i.e. resident within the tissue and may or may not cause disease), and pathobiont (i.e., resident within the tissue and generally beneficial but disease-causing under certain conditions) (Dey and Ray Chaudhuri, 2022). The urobiome has the full range of the 6 categories described above, including pathogens and pathobionts (Thomas-White et al., 2018; Du et al., 2024). Yet, most human beings do not have a clinical infection (i.e., UTI) even though pathogens or pathobionts are “citizens” of their urobiome community. An informative study enrolled heathy volunteers ≥ 65 years old without urinary symptoms as a comparison group for patients diagnosed with UTIs (Akhlaghpour et al., 2024). In that study, an M-PCR panel consistently detected the known uropathogens E. coli and Enterococcus faecalis, as well as the emerging uropathogens Aerococcus urinae, Actinotignum schaalli, and members of the Viridians group Streptococcus in healthy volunteers without urinary tract symptoms. In addition, few of these volunteers experienced an increase in infection-related immune markers, a hallmark of infection (Akhlaghpour et al., 2024). Furthermore, many adult females do not get UTIs. Finally, it is well known that UTIs can resolve spontaneously (Hoffmann et al., 2020; Barnes et al., 2021; Midby and Miesner, 2024). This implies that the indigenous urobiome together with both innate and adaptive immune responses can often restore urinary health and resolve infection.
Biomass influences microbial communities. The gastrointestinal tract is the best-known example of a high biomass microbial niche. In contrast, the urobiome has relatively low microbial biomass and thus, in some cases, urine samples yield culture-negative and DNA-based-negative test results (Hilt et al., 2014; Pearce et al., 2015; Neugent et al., 2020). An analogy would be a city block in the Bronx with 35,000 inhabitants versus a high plains plateau in Wyoming with sparse settlements. Within both types of communities, however, interactions occur among the residents. The same is true for ecological microcosms within the human microbiome.
If most contamination arises from periurethral, epidermal, perianal, and vaginal flora as suggested (LaRocco et al., 2015), it would be helpful to compare the bladder urobiome to these other niches. A comparison of gastrointestinal, vaginal and bladder microbiomes revealed that while all three niches were distinct from each other, there were similarities between the vagina and bladder microbiomes (Thomas-White et al., 2018; Du et al., 2024) (Figure 1). Furthermore, a recent survey of the skin microbiome lists the top ten taxa from four different niches (Byrd et al., 2018). Of the 22 different microbes identified among the ten most abundant taxa in four different regions of skin (dry, moist, sebaceous, and feet), 18 (82%) are also found in urine obtained directly from the bladder by transurethral catheterization (Thomas-White et al., 2018; Du et al., 2024) (Supplementary Table 1). Therefore, mere taxonomic identity (via Gram stain, oxygen tolerance, metabolic panel, and/or MALDI-TOF MS) cannot distinguish bladder residents from skin periurethral, perianal, and vaginal residents. To make such a distinction requires genome sequencing and complex bioinformatic analysis that determines whether two isolates are of the same lineage or not.
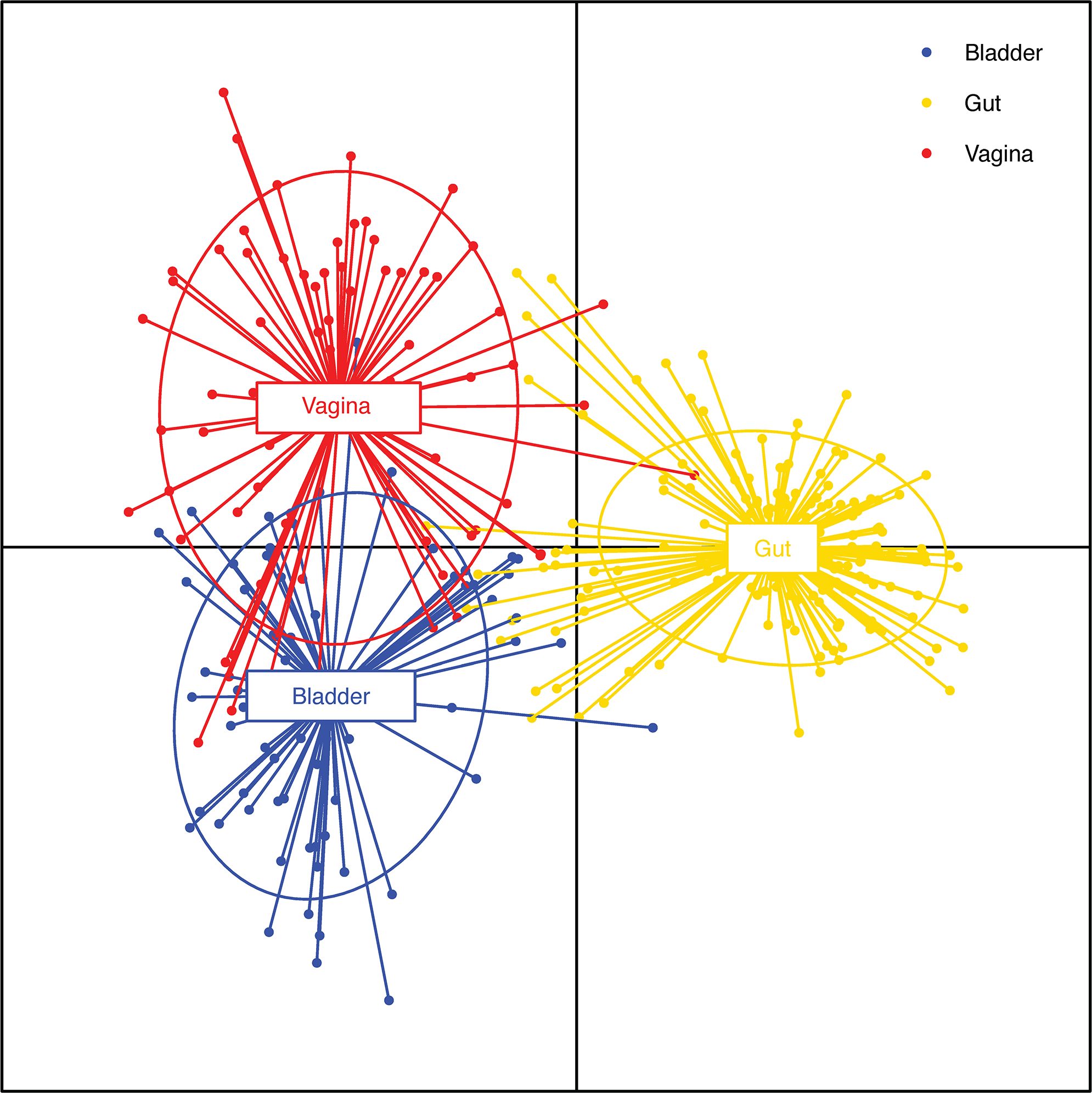
Figure 1. Comparison of bladder, vaginal and gut isolate functions. Discriminant analysis of principal components analysis of the functions of bacterial species isolated from asymptomatic individuals shared among 3 different niches: bladder (blue; n=68), vagina (red; n=74) and gut (yellow; n=175). The dots represent individual functions. The lines show the extent of those functions. Thus, a line that begins in the bladder centroid and ends in the vaginal centroid represents a function that is expressed by species that inhabit both the bladder and the vagina. Note the extensive overlap of functions in the bladder and vagina in contrast to the limited overlap between functions characteristic of the bladder or vagina and those of the gut. Adapted from (Du et al., 2024) (published under a Creative Commons license).
5 Polymicrobial infections
The history of polymicrobial infections dates to the late nineteenth century and W.D. Miller, a microbiologist and dentist who had worked in Robert Koch’s lab (Murray et al., 2014; Sedgley, 2004). By microscopy and characterization of pus from oral abscesses, he reported that the application of Koch’s postulates was not consistent with cultured microbes isolated from murine models. In fact, Miller obtained a more virulent response from the abscess pus than he did the cultured, recovered microbes. Armed with only culture conditions, a microscope and staining, Miller concluded that uncultivatable microbes worsened the infection (Murray et al., 2014). It was not until the availability of DNA sequence-based methods in the late twentieth and early twenty-first century that microbial ecology and polymicrobial infections were confirmed and appreciated in some human niches.
Thus, the concept of polymicrobial UTIs is not new but their existence has been confirmed with many examples reported in the late twentieth century (Siegman-Igra, 1994; Siegman-Igra et al., 1988, 1993; Siegman-IgraY, 1994). Yet, it may be useful to divide the studies on mixed cultures/polymicrobial infections into two groups: before and after the advent of DNA molecular techniques. The former was limited to urine sediment, urine culture, microscopy, Gram staining, and metabolic panels. Urine culture limited to SUC (most often aerobic) identified mostly facultative anaerobes (Tables 3, 4). Consequently, this literature identified mostly Gram-negative rods as pathogens and excluded most Gram-positive rods and cocci as contaminants (with a few exceptions such as Enterococcus and coagulase negative Staphylococcus (CoNS), especially S. saprophyticus. Early studies on urosepsis and mixed (polymicrobial) cultures from patients revealed that matching cultures from urine and blood of the same microbe suggested that upper urinary tract infection had transitioned into the bloodstream. In one study, 716 bacteremic episodes were observed in 692 patients out of 52,012 admissions over 5 years (Siegman-Igra, 1994). Of these, 307 episodes in 303 patients were due to UTI. In this group, 198 had at least one microbe that was detected in both blood and urine culture (Table 4). These 198 urosepsis cases represented 194 patients (96 male, 98 female) with a mean age of 68 years. The most common microbe in monomicrobial infections was Escherichia coli; however, in polymicrobial infections, Pseudomonas aeruginosa was most predominant. P. aeruginosa also was among the microbes associated with fatal outcomes. In an earlier study of polymicrobial bacteremia, of 67 cases across multiple organ systems and causes, 46 percent were diagnosed with UTI (Siegman-Igra et al., 1988). Of the 67 cases, 28 died, with 42 percent diagnosed with UTI. While urosepsis is considered rare among the population, in neonates and patients 65 and older, it is a significant morbidity (Peach et al., 2016; Akhtar et al., 2021; Collaborators, GBD 2021 Antimicrobial Resistance, 2024).
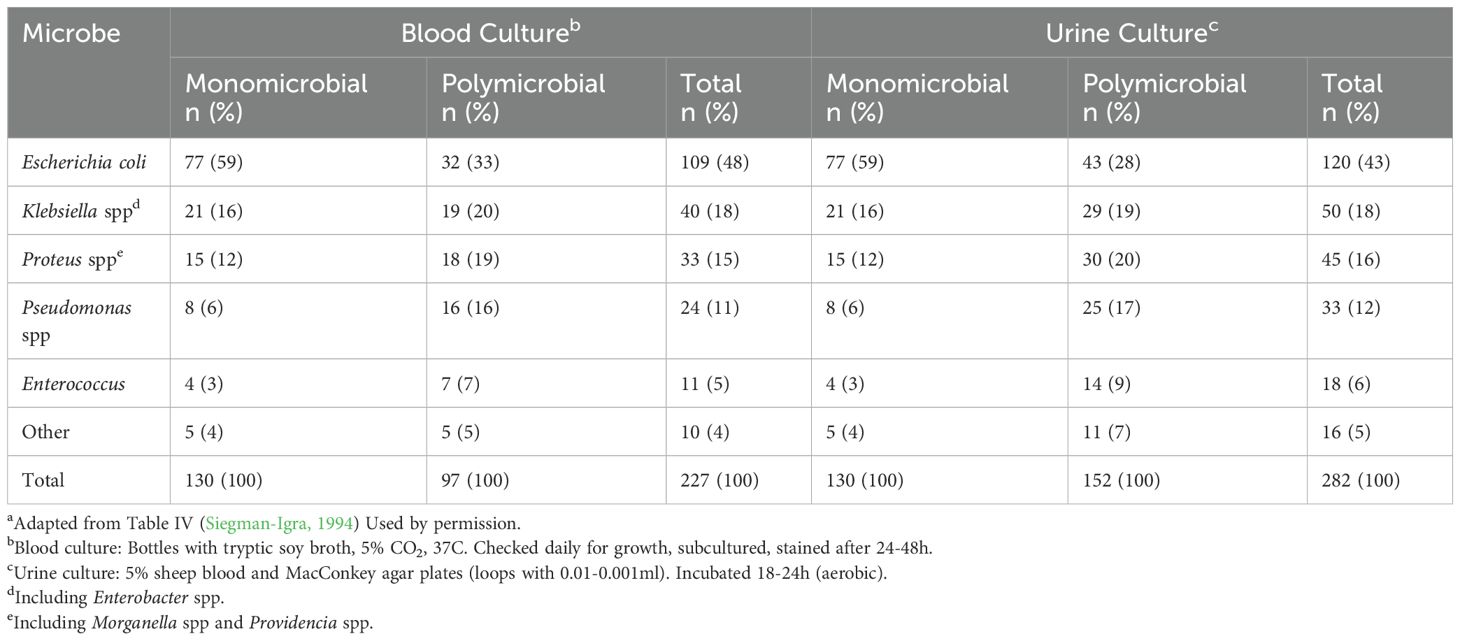
Table 4. Characteristics of microbes from UTI with matching blood infectionsa .
While traditional urinary pathogens are predominantly Gram-negative facultative anaerobes, Gram-positive microbes are also detected in patients diagnosed with UTI, particularly in polymicrobial infections (Kline and Lewis, 2016). While usually regarded as commensals, Gram-positive microbes’ pathogenic potential have been questioned (Clarke et al., 2010; Kline and Lewis, 2016; Leal et al., 2016). Yet, Gram-positive CoNS and Enterococcus, as well as emerging urinary pathogens such as Aerococcus, Actinotignum, Gardnerella, and Corynebacteria are found prevalently and abundantly in patients with UTI symptoms (Kline and Lewis, 2016; Moreland et al., 2023). Microaerophiles and anaerobes also have been observed (Legaria et al., 2022; Maskell, 1986) and cultures to rule out these microbes was suggested as part of diagnosis seventy years ago (Jawetz, 1953).
With the advent of metaculturomic methods (approaches designed to permit growth of typically uncultivated microbes) and metagenomic approaches (DNA-dependent methods that do not require growth), attempts (e.g., the human microbiome project) have sought to define the microbiota of various human niches, notably skin, respiratory tract, and the gastrointestinal tract, as well as the urogenital and reproductive tracts (Lloyd-Price et al., 2016; Joos et al., 2024). Lessons learned from the last two decades of research have taught us that there are multiple healthy states within niches that vary with sex, age and reproductive status (Lloyd-Price et al., 2016; Joos et al., 2024). Also, disease states are more complicated than originally anticipated by Koch and his postulated approach (Blevins and Bronze, 2010; Murray et al., 2014). A recent opinion paper questioned whether the urobiome has any impact on UTI, in part because Koch’s postulates have not been performed to determine whether any of the members of the newly identified urobiome cause disease symptoms (Werneburg and Southgate, 2024). Yet, Koch’s postulates (one organism, one infection, one cause of disease) cannot be applied to polymicrobial infections including UTIs (Ronald et al., 2008; Murray et al., 2014; Short et al., 2014). The request to use Koch’s postulates to validate the role of the urobiome (Werneburg and Southgate, 2024) is further complicated by the currently accepted tools that may no longer apply: a detection system (urine dipsticks, SUC) that misses many uropathogens (Moreland et al., 2024) and standards that define polymicrobial infections as contamination (Siegman-Igra et al., 1993; Siegman-IgraY, 1994; Bekeris et al., 2008).
One DNA-dependent method, multiplex PCR (M-PCR), allows quantitative, real-time detection of microbial DNA as long as a primer set is present to detect them (Moreland et al., 2024). Using M-PCR, a surprisingly high rate of polymicrobial specimens have been detected in older patients (≥ 65) with diagnosed UTI symptoms, ranging from 45 to 65 percent depending on simple or complex UTI, sex, and comorbidities (Vollstedt et al., 2020; Wojno et al., 2020; Korman et al., 2023; Wang et al., 2023; Akhlaghpour et al., 2024; Haley et al., 2024; Kardjadj et al., 2025). For example, in one study examining the differences of microbes based on catheterized (bladder) urine versus midstream void (entire urinary tract including urogenital areas), more polymicrobial infections were detected in midstream voided compared to catheter-collected samples (64.4% vs 45.7%, p < 0.0001) in females but the opposite in males (35.6% vs 47.0%, p = 0.002 (Wang et al., 2023). While M-PCR detected microbes that are not detected by SUC, the use of inflammatory markers of infection allowed the distinction of volunteers without relevant clinical symptoms from symptom-diagnosed UTI patients and stratifying those microbes into tiers based on abundance of occurrence (Akhlaghpour et al., 2024; Haley et al., 2024).
In the laboratory, specific microbes are studied in isolation using a reductionist approach. In the real world, communities of microbes make up an ecosystem that changes based on the predominating species and interactions between them (Murray et al., 2014; Short et al., 2014; Jayalath and Magana-Arachchi, 2022). These communities interact in synergy with residents by providing nutrients, soluble signaling factors, cell contact through adhesins, or in some cases facilitating antibiotic resistance (Ryan and Dow, 2008; Short et al., 2014; Murray et al., 2014; Gaston et al., 2021). Likewise, some microbes secrete molecules that eliminate their competition such as Pseudomonas (Gaston et al., 2021) or kill uropathogens such as commensal Lactobacillus (Abdul-Rahim et al., 2021; Johnson et al., 2022; Szczerbiec et al., 2022).
While polymicrobial results in the studies described above were reported as a group, the individual microbes within the subsets of polymicrobial infections were not identified. Instead, individual microbes were reported by taxon (Vollstedt et al., 2020; Wojno et al., 2020; Korman et al., 2023; Wang et al., 2023; Akhlaghpour et al., 2024; Haley et al., 2024). A reanalysis of the data from these studies could reveal associations of specific microbes that as a group or community are more pathogenic than as single isolates. In an effort to understand microbial ecology of polymicrobial isolates, one study examined 72 bacteria isolates collected from 23 patients diagnosed with polymicrobial UTI (de Vos et al., 2017). An ecological network analysis was developed, finding that most interactions clustered based on evolutionary relatedness. Eight complete communities of four different microbes were found, four of which were predicted to be stable and four not stable (de Vos et al., 2017). The isolates used for this study were originally collected using standard culture techniques (Croxall et al., 2011), which may explain the predominance of classical uropathogens. Expanding and extending this concept, another study examined pathogens and bladder commensal isolates grown under urine-like conditions and the effects different microbes had on the community (Zandbergen et al., 2021). Artificial urine media conditioned by commensals had effects on growth of uropathogens and vice versa. This early attempt at gaining insights into the complexities of urobiome ecology shows that while a microbe in isolation may have certain growth characteristics, the community - through direct physical interaction, direct interaction with the host, or secretion/excretion of signaling molecules or metabolites, - may exhibit a different response than a single microbe in monoculture (Heidrich et al., 2022; Short et al., 2014; Murray et al., 2014).
6 Future directions: a call to action
To advance diagnosis, we suggest the following:
1. Reexamine standards for urine collection/culture contamination to ensure accurate sampling, taking into consideration the new knowledge concerning the urobiome. A recent scoping review highlights the issues, especially the lack of consensus among guidelines concerning urine culture thresholds for UTI and the reliance upon dated and sparse evidence for current standards (Hilt et al., 2023). To achieve accuracy, we should recognize that urine samples must be processed immediately or be stored under conditions that do not permit microbial growth (LaRocco et al., 2015).
2. Recognize the shortcomings of SUC. There is now sufficient data in the literature to adjudicate the accuracy of this method. It should be examined, weighing its strengths and acknowledging its weaknesses (Price et al., 2017; Wojno et al., 2020; Xu et al., 2021; Festa et al., 2023; Brubaker et al., 2023; Gleicher et al., 2024). Besides its ability to detect only a limited group of facultative anaerobes, time is also a consideration as re-sampling and re-culturing requires time that may allow an infection to progress.
3. Recognize the presence and importance of polymicrobial UTI. The literature provides evidence that these infections are more prevalent than previously believed and misdiagnosis as contamination should be considered and re-evaluated. Ultimately, clinical diagnostic pathways might be modified to diagnose these infections before they can progress to upper tract UTI and potentially urosepsis (Siegman-Igra, 1994; Peach et al., 2016; Akhtar et al., 2021).
4. Recognize the importance of redefining contamination, acknowledging the existence of polymicrobial infection. Critical for antibiotic stewardship is the development of accurate and rapid diagnostics that not only detect microbes but also determine if they are involved in a host immune response. The increase in antibiotic resistance and future predictions are sobering (Collaborators, GBD 2021 Antimicrobial Resistance, 2024). Proper and appropriate use of antibiotics to treat UTI not only affects the health of patients but preserves critical antibiotics for those who need them (Simoni et al., 2024).
5. Take a lesson from other organ systems and diseases (Murray et al., 2014; Short et al., 2014), and ask how diagnostic tools can be improved and new tools developed or implemented to characterize microbial communities in polymicrobial infections/mixed cultures. One way to accomplish this would be to reanalyze data from polymicrobial samples to identify interaction networks and determine if any groups of microbes are more commonly associated with symptoms or the lack thereof (Vollstedt et al., 2020; Wang et al., 2023; Akhlaghpour et al., 2024; Haley et al., 2024).
6. Finally, the concept of contamination must be reevaluated in the context of the new knowledge that the urobiome exists. Urine is not sterile above the urethral sphincter and the existence of communities containing both commensal, non-pathogenic microbes and potential uropathogens should be acknowledged. While dysbiosis is generally a concept foreign to the UTI literature, it needs to be recognized, and diagnostics updated to reflect the current science (Price et al., 2017; Jayalath and Magana-Arachchi, 2022; Simoni et al., 2024). Koch’s postulates are invalid in polymicrobial systems (Murray et al., 2014; Short et al., 2014) but unfortunately continue to persist as a consequence of the dogma that single microbes are causative of disease (Blevins and Bronze, 2010; Werneburg and Southgate, 2024).
The danger of dismissing species considered to be routine contaminants, such as members of the genus Corynebacterium, can lead to dismissing pathogens like C. urealyticium, which is both nitrate negative by urine dipstick and grows slowly under SUC conditions. Yet, while PCR testing is much more rapid than culture (Dixon et al., 2020; Gleicher et al., 2024; Zering and Stohs, 2024), without some reference to host immune response (indicative of infection), the practicing clinician is left with 10–20 names on a page with little clue what to do next (Zering and Stohs, 2024; Xu et al., 2021). Fortunately, one test correlates an M-PCR panel of 30 microbes with biomarkers of inflammation (Akhlaghpour et al., 2024; Haley et al., 2024). While this is but one study with older patients, it opens the opportunity for researchers to examine other groups (e.g., different age groups, males versus females, or pregnant versus non-pregnant individuals.It is likely the differences encountered will require tailored treatments depending on the type of patient.
So, what should we do with this additional information? We foresee both scientific and clinical paths. As scientists broaden the view from “identify the pathogen and kill it” to “understand the ecology,” new insights into therapies should arise that parallel those of the oncological research and clinical community that has spent decades working to better understand, diagnose, and treat cancers. Their recognition that the old diagnostics and treatment algorithms were insufficient has led to precision diagnostics, targeted treatments, and better outcomes for patients. We should do the same for our patients. Teams of scientists and clinicians have begun this process; others should join the effort.
Where does this leave clinical microbiologists and clinicians who deal daily with the quandary of accurate diagnosis balanced by microbial stewardship? Even though the human microbiome project began two decades ago, the translation of that research into clinical practice is just beginning (Gilbert et al., 2025). In other niches, where the research is more advanced, there have been three stages of translations into the clinic. The first stage involves identification of microbiota and normal (healthy) microbial ecology. This process leads to understanding the mechanisms underlying dysbiosis and symptoms/disease. The second stage develops metrics associating taxa, host response, and disease characteristics that lead to biomarkers and new diagnostics. Finally, armed with this information, strategies are developed to modify the microbiota to restore a normal, healthy state (Gilbert et al., 2025).
Our community is still in the earlier stages of this process, having shown that the urobiome exists and obtained evidence that it is associated with health and disease. Progress on the second stage has begun but there is much to do. To be consistent with developing science, clinical microbiologists must challenge current standards concerning urine culture contamination (Bekeris et al., 2008). Also, the incidence of polymicrobial infection must be established across diverse populations (e.g. age, sex, nosocomial versus outpatient status). For their part, clinicians should be aware of the older diagnostics flaws and help progress new diagnostic developments with the goal of a rapid, accurate test that identifies both microbes and host response. For diagnosis of UTI, the host response could be monitored by inflammatory biomarkers (Akhlaghpour et al., 2024). In the meantime, clinicians can be open to emerging new knowledge and new diagnostics. Their intellectual preparation to use that knowledge to make their best clinical decision will be critical for improved patient care. Finally, all should recognize that better diagnostics and better understanding of their results are key to antibiotic stewardship as the convenience of empirical treatment, or the use of flawed culture methods ultimately will deprive those who need antibiotics to survive in an increasingly antibiotic-resistant clinical environment (Collaborators, GBD 2021 Antimicrobial Resistance, 2024; Simoni et al., 2024).
7 Conclusions
In this review, we have presented the current diagnostic state of urine contamination and discussed the limitations of current diagnostic techniques, such as SUC. We have reviewed the evidence for polymicrobial UTI, raising doubt concerning the appropriateness of applying Koch’s postulates and recognizing the consequences of potential missed diagnosis. The challenges for clinical microbiologists, clinicians and research scientists are to question the current dogmas, address polymicrobial infections, and work to define the microbial ecology of the urinary tract. The future is bright as multidisciplinary collaboration offers cross-pollinating efforts to improve patient care and quality of life.
Author contributions
RM: Investigation, Writing – original draft, Writing – review & editing. LB: Writing – review & editing. AW: Conceptualization, Supervision, Writing – original draft, Writing – review & editing.
Funding
The author(s) declare that no financial support was received for the research and/or publication of this article.
Acknowledgments
We thank Amanda Harrington, PhD, D (ABMM), for thought-provoking and helpful discussions. We also thank Christopher Beger, Gail Hendler, and the staff of the Loyola Health Sciences Library, Loyola University Chicago, for assistance with interlibrary loans and obtaining articles and reviews.
Conflict of interest
AW declares membership on the Scientific Advisory Boards of Astek, Carrillo, Pathnostics, and Urobiome Therapeutics. LB declares editorial stipends from UpToDate and JAMA.
The remaining author declares that the research was conducted in the absence of any commercial or financial relationships that could be construed as a potential conflict of interest.
Generative AI statement
The author(s) declare that no Generative AI was used in the creation of this manuscript.
Publisher’s note
All claims expressed in this article are solely those of the authors and do not necessarily represent those of their affiliated organizations, or those of the publisher, the editors and the reviewers. Any product that may be evaluated in this article, or claim that may be made by its manufacturer, is not guaranteed or endorsed by the publisher.
Supplementary material
The Supplementary Material for this article can be found online at: https://www.frontiersin.org/articles/10.3389/fcimb.2025.1562687/full#supplementary-material
Supplementary Table 1 | Most abundant skin microbes by region/nichea and incidence in urobiomeb. a From the top ten most abundant microbes in four different skin regions (Byrd et al., 2018). Note that some microbes are abundant in multiple regions (for ex., Corynebacterium tuberculostearicum and Staphylococcus epidermidis). Across four regions with ten microbes each, there are 22 unique taxa. b Microbes in bold type were found in the human female urobiome (Du et al., 2024) as determined by sequencing of genomes from isolates from catheterized urinary bladder urine. c Skin regions/niches are defined as follows: Dry: Hypothenar palm and volar forearm. Moist: Nare, antecubital fossa, inguinal crease, interdigital web, and popliteal fossa. Sebaceous: Alar crease, cheek, glabella, external auditory canal, manubrium, retroauricular crease, occiput, back. Feet:|Toe web space, toenail, andplantar heel. d Bacterial Diversity Database. See (Schober et al., 2025). e Also known as Cutibacterium acnes.
Supplementary Table 2 | Most abundant vaginal microbesa and incidence in urobiomeb. a Ravel et al., 2011 evaluated vaginal microflora and vaginal pH in 396 asymptomatic sexually active women representing four ethnic groups (white, black, Hispanic, and Asian) by pyrosequencing of 16S rRNA genes. Lactobacillus species dominated four groups, but one group accounting for 27% of women (termed as group IV community state type) lacked significant lactobacilli and was heterogeneous with higher proportion of strictly anaerobic bacteria including genera Gardnerella, Prevotella, Atopobium, as well as Dialister, Megasphaera, Peptoniphilus, Sneathia, Eggerthella, Aerococcus, Finegoldia, and Mobiluncus. This latter group was over-represented in black and Hispanic women (Ravel et al., 2011). b Microbes in bold type were found in the human female urobiome (Du et al., 2024) as determined by sequencing of genomes from isolates from catheterized urinary bladder urine. c For bacterial vaginosis, see (Saraf et al., 2021) d Bacterial Diversity Database. See (Schober et al., 2025). e Atopobium vaginae is now known as Fannyhessea vaginae (Nouioui et al., 2018).
References
Abdul-Rahim, O., Wu, Q., Pistone G Price, T. K., Diebel, K., and Wolfe AJ Bugni, T. S. (2021). Phenyl-lactic acid is an active ingredient in bactericidal supernatants of lactobacillus crispatus. J. Bacteriol. 203, e0036021. doi: 10.1128/JB.00360-21
Akhlaghpour, M., Haley E Parnell, L., Luke, N., Mathur, M., Festa, R. A., Percaccio, M., et al. (2024). Urine biomarkers individually and as a consensus model show high sensitivity and specificity for detecting UTIs. BMC Infect. Dis. 24, 153. doi: 10.1186/s12879-024-09044-2
Akhtar, A., Ahmad Hassali, M. A., Zainal, H., Ali, I., and Khan, A. H. (2021). A cross-sectional assessment of urinary tract infections among geriatric patients: prevalence, medication regimen complexity, and factors associated with treatment outcomes. Front. Public Health 9, 657199. doi: 10.3389/fpubh.2021.657199
Anger, J., Lee, U., Ackerman, A. L., Chou, R., Chughtai, B., Clemens, J. Q., et al. (2019). Recurrent uncomplicated urinary tract infections in women: AUA/CUA/SUFU guideline. J. Urol. 202, 282–289. doi: 10.1097/JU.0000000000000296
Armbruster, C. E., Smith, S. N., Johnson, A. O., DeOrnellas, V., Eaton, K. A., Yep, A., et al. (2017). The Pathogenic Potential of Proteus mirabilis Is Enhanced by Other Uropathogens during Polymicrobial Urinary Tract Infection. Infect. Immun. 85, e00808–e00816. doi: 10.1128/IAI.00808-16
Asscher, A. W., Sussman, M., Waters, W. E., Davis, R. H., and Chick, S. (1966). Urine as a medium for bacterial growth. Lancet 2, 1037–1041. doi: 10.1016/S0140-6736(66)92023-X
Barnes, H. C., Wolff, B., Abdul-Rahim, O., Harrington, A., Hilt, E. E., Price, T. K., et al. (2021). A randomized clinical trial of standard versus expanded cultures to diagnose urinary tract infections in women. J. Urol. 206, 1212–1221. doi: 10.1097/JU.0000000000001949
Bekeris, L. G., Jones, B. A., Walsh, M. K., and Wagar, E. A. (2008). Urine culture contamination: a College of American Pathologists Q-Probes study of 127 laboratories. Arch. Pathol. Lab. Med. 132, 913–917. doi: 10.5858/2008-132-913-UCCACO
Blake, D. R. and Doherty, L. F. (2006). Effect of perineal cleansing on contamination rate of mid-stream urine culture. J. Pediatr. Adolesc. Gynecol. 19, 31–34. doi: 10.1016/j.jpag.2005.11.003
Blevins, S. M. and Bronze, M. S. (2010). 'Robert Koch and the 'golden age' of bacteriology. Int. J. Infect. Dis. 14, e744–e751. doi: 10.1016/j.ijid.2009.12.003
Brubaker, L., Chai, T. C., Horsley, H., Moreland, R. B., Khasriya, R., and Wolfe, A. J. (2023). 'Tarnished gold—the “standard” urine culture: reassessing the characteristics of a criterion standard for detecting urinary microbes. Front. Urol. 3. doi: 10.3389/fruro.2023.1206046
Brubaker, L., Gourdine, J. F., Siddiqui, N. Y., Holland, A., Halverson, T., Limeria, R., et al. (2021a). Forming consensus to advance urobiome research. mSystems 6, e0137120. doi: 10.1128/msystems.01371-20
Brubaker, L., Putonti, C., Dong, Q., and Wolfe, A. J. (2021b). The human urobiome. Mamm. Genome 32, 232–238. doi: 10.1007/s00335-021-09862-8
Byrd, A. L., Belkaid, Y., and Segre, J. A. (2018). The human skin microbiome. Nat. Rev. Microbiol. 16, 143–155. doi: 10.1038/nrmicro.2017.157
CDC (2022) National healthcare safety network master organism list. (Online database). Available at: https://www.cdc.gov/nhsn/xls/master-organism-com-commensals-lists.xlsx.
Chambliss, A. B. and Van, T. T. (2022). Revisiting approaches to and considerations for urinalysis and urine culture reflexive testing. Crit. Rev. Clin. Lab. Sci. 59, 112–124. doi: 10.1080/10408363.2021.1988048
Chang, Z., Deng, J., Zhang, J., Wu, H., Wu, Y., Bin, L., et al. (2025). Rapid and accurate diagnosis of urinary tract infections using targeted next-generation sequencing: A multicenter comparative study with metagenomic sequencing and traditional culture methods. J. Infect. 90, 106459. doi: 10.1016/j.jinf.2025.106459
Chen, Y. B., Hochstedler, B., Pham, T. T., Acevedo-Alvarez, M., Mueller, E. R., and Wolfe, A. J. (2020). The urethral microbiota: A missing link in the female urinary microbiota. J. Urol. 204, 303–309. doi: 10.1097/JU.0000000000000910
Clarke, T. M., Citron, D. M., and Towfigh, S. (2010). The conundrum of the gram-positive rod: are we missing important pathogens in complicated skin and soft-tissue infections? A case report and review of the literature. Surg. Infect. (Larchmt). 11, 65–72. doi: 10.1089/sur.2008.085
Cohen, J. E., Yura, E. M., Chen, L., and Schaeffer, A. J. (2019). Predictive utility of prior negative urine cultures in women with suspected recurrent uncomplicated urinary tract infections. J. Urol. 202, 979–985. doi: 10.1097/JU.0000000000000325
Collaborators, GBD 2021 Antimicrobial Resistance (2024). Global burden of bacterial antimicrobial resistance 1990–2021: a systematic analysis with forecasts to 2050. Lancet 404 (10459), 1199–1226. doi: 10.1016/S0140-6736(24)01867-1
Croxall, G., Weston, V., Joseph, S., Manning, G., Cheetham, P., and McNally, A. (2011). Increased human pathogenic potential of Escherichia coli from polymicrobial urinary tract infections in comparison to isolates from monomicrobial culture samples. J. Med. Microbiol. 60, 102–109. doi: 10.1099/jmm.0.020602-0
Deen, N. S., Ahmed, A., Tasnim, N. T., and Khan, N. (2023). Clinical relevance of expanded quantitative urine culture in health and disease. Front. Cell Infect. Microbiol. 13, 1210161. doi: 10.3389/fcimb.2023.1210161
de Vos, M. G. J., Zagorski, M., McNally, A., and Bollenbach, T. (2017). Interaction networks, ecological stability, and collective antibiotic tolerance in polymicrobial infections. Proc. Natl. Acad. Sci. U. S. A 114, 10666–10671. doi: 10.1073/pnas.1713372114
Dey, P. and Ray Chaudhuri, S. (2022). The opportunistic nature of gut commensal microbiota. Crit. Rev. Microbiol. 49, 739–763. doi: 10.1080/1040841X.2022.2133987
Dixon, M., Sha, S., Stefil, M., and McDonald, M. (2020). Is it time to say goodbye to culture and sensitivity? The case for culture-independent urology. Urology 136, 112–118. doi: 10.1016/j.urology.2019.11.030
Doern, C. D. and Burnham, C. A. (2010). It's not easy being green: the viridans group streptococci, with a focus on pediatric clinical manifestations. J. Clin. Microbiol. 48, 3829–3835. doi: 10.1128/JCM.01563-10
Du, J., Khemmani, M., Halverson, T., Ene, A., Limeira, R., Tinawi, L., et al. (2024). Cataloging the phylogenetic diversity of human bladder bacterial isolates. Genome Biol. 25, 75. doi: 10.1186/s13059-024-03216-8
Festa, R. A., Luke, N., Mathur, M., Parnell, L., Wang, D., Zhao, X., et al. (2023). A test combining multiplex-PCR with pooled antibiotic susceptibility testing has high correlation with expanded urine culture for detection of live bacteria in urine samples of suspected UTI patients. Diagn. Microbiol. Infect. Dis. 107, 116015. doi: 10.1016/j.diagmicrobio.2023.116015
Finucane, T. E. (2017). Urinary tract infection"-requiem for a heavyweight. J. Am. Geriatr. Soc. 65, 1650–1655. doi: 10.1111/jgs.2017.65.issue-8
Flores-Mireles, A. L., Walker, J. N., Caparon, M., and Hultgren, S. J. (2015). Urinary tract infections: epidemiology, mechanisms of infection and treatment options. Nat. Rev. Microbiol. 13, 269–284. doi: 10.1038/nrmicro3432
Friedmann, H. C. (2014). Escherich and escherichia. EcoSal. Plus. 6, 133–196. doi: 10.1128/ecosalplus.esp-0025-2013
Gaston, J. R., Johnson, A. O., Bair, K. L., White, A. N., and Armbruster, C. E. (2021). Polymicrobial interactions in the urinary tract: is the enemy of my enemy my friend? Infect. Immun. 89. doi: 10.1128/IAI.00652-20
Gilbert, J. A., Azad, M. B., Bäckhed, F., Blaser, M. J., Byndloss, M., Chiu, C. Y., et al. (2025). Clinical translation of microbiome research. Nat. Med. 31, 1099–1113. doi: 10.1038/s41591-025-03615-9
Gillespie, W. A., Linton, K. B., Miller, A., and Slade, N. (1960). The diagnosis, epidemiology and control of urinary infection in urology and gynaecology. J. Clin. Pathol. 13, 187–194. doi: 10.1136/jcp.13.3.187
Gleicher, S., Karram, M., Wein, A. J., and Dmochowski, R. R. (2024). Recurrent and complicated urinary tract infections in women: Utility of advanced testing to enhance care. Neurourol. Urodyn. 43, 161–166. doi: 10.1002/nau.25280
Haley, E., Luke, N., Mathur, M., Festa, R. A., Wang, J., Jiang, Y., et al. (2024). The prevalence and association of different uropathogens detected by M-PCR with infection-associated urine biomarkers in urinary tract infections. Res. Rep. Urol. 16, 19–29. doi: 10.2147/RRU.S443361
Hansen, M. A., Valentine-King, M., Zoorob, R., Schlueter, M., Matas, J. L., Willis, S. E., et al. (2022). Prevalence and predictors of urine culture contamination in primary care: A cross-sectional study. Int. J. Nurs. Stud. 134, 104325. doi: 10.1016/j.ijnurstu.2022.104325
Heidrich, V., Inoue, L. T., Asprino, P. F., Bettoni, F., Mariotti, A. C. H., Bastos, D. A., et al. (2022). Choice of 16S ribosomal RNA primers impacts male urinary microbiota profiling. Front. Cell Infect. Microbiol. 12, 862338. doi: 10.3389/fcimb.2022.862338
Hilt, E. E. and Ferrieri, P. (2022). Next generation and other sequencing technologies in diagnostic microbiology and infectious diseases. Genes (Basel). 13, 1566–1590. doi: 10.3390/genes13091566
Hilt, E. E., McKinley, K., Pearce, M. M., Rosenfeld, A. B., Zilliox, M. J., Mueller, E. R., et al. (2014). Urine is not sterile: use of enhanced urine culture techniques to detect resident bacterial flora in the adult female bladder. J. Clin. Microbiol. 52, 871–876. doi: 10.1128/JCM.02876-13
Hilt, E. E., Parnell, L. K., Wang, D., Stapleton, A. E., and Lukacz, E. S. (2023). Microbial threshold guidelines for UTI diagnosis: A scoping systematic review. Pathol. Lab. Med. Int. 15, 43–63. doi: 10.2147/PLMI.S409488
Hoffmann, T., Peiris, R., Mar, C. D., Cleo, G., and Glasziou, P. (2020). Natural history of uncomplicated urinary tract infection without antibiotics: a systematic review. Br. J. Gen. Pract. 70, e714–ee22. doi: 10.3399/bjgp20X712781
Hort, E. C. (1914). The sterility of normal urine in man. J. Hyg. (Lond). 14, 509–516. doi: 10.1017/S0022172400006021
Horton, L. E., Mehta, S. R., Aganovic, L., and Fierer, J. (2018). Actinotignum schaalii infection: A clandestine cause of sterile pyuria? Open Forum Infect. Dis. 5, ofy015. doi: 10.1093/ofid/ofy015
Jawetz, E. (1953). Urinary tract infections; problems in medical management. California. Med. 79, 99–102.
Jayalath, S. and Magana-Arachchi, D. (2022). 'Dysbiosis of the human urinary microbiome and its association to diseases affecting the urinary system. Indian J. Microbiol. 62, 133–166. doi: 10.1007/s12088-021-00991-x
Johnson, J. A., Delaney, L. F., Ojha, V., Rudraraju, M., Hintze, K. R., Siddiqui, N. Y., et al. (2022). Commensal urinary lactobacilli inhibit major uropathogens in vitro with heterogeneity at species and strain level. Front. Cell Infect. Microbio. 12, 870603. doi: 10.3389/fcimb.2022.870603
Joos, R., Boucher, K., Lavelle, A., Arumugam, M., Blaser, M. J., Claesson, M. J., et al. (2024). Examining the healthy human microbiome concept. Nat. Rev. Microbiol. 23 (3), 192–205. doi: 10.1038/s41579-024-01107-0
Kardjadj, M., Chang, T. W., Chavez, R., Derrick, D., Spangler, F. L., Priestly, I. P., et al. (2025). The clinical validity and utility of PCR compared to conventional culture and sensitivity testing for the management of complicated urinary tract infections in adults: A secondary (Ad hoc) analysis of pathogen detection, resistance profiles, and impact on clinical outcome. Microorganisms 13, 949. doi: 10.3390/microorganisms13040949
Kavuru, V., Vu, T., Karageorge, L., Choudhury, D., Senger, R., and Robertson, J. (2020). Dipstick analysis of urine chemistry: benefits and limitations of dry chemistry-based assays. Postgrad. Med. 132, 225–233. doi: 10.1080/00325481.2019.1679540
Khasriya, R., Sathiananthamoorthy, S., Ismail, S., Kelsey, M., Wilson, M., Rohn, J. L., et al. (2013). Spectrum of bacterial colonization associated with urothelial cells from patients with chronic lower urinary tract symptoms. J. Clin. Microbiol. 51, 2054–2062. doi: 10.1128/JCM.03314-12
Kline, K. A. and Lewis, A. L. (2016). Gram-positive uropathogens, polymicrobial urinary tract infection, and the emerging microbiota of the urinary tract. Microbiol. Spect. 4, 10.1128. doi: 10.1128/microbiolspec.UTI-0012-2012
Korman, H. J., Baunoch, D., Luke, N., Wang, D., Zhao, X., Levin, M., et al. (2023). 'A diagnostic test combining molecular testing with phenotypic pooled antibiotic susceptibility improved the clinical outcomes of patients with non-E. coli or polymicrobial complicated urinary tract infections. Res. Rep. Urol. 15, 141–147. doi: 10.2147/RRU.S404260
LaRocco, M. T., Franek, J., Leibach, E. K., Weissfeld, A. S., Kraft, C. S., Sautter, R. L., et al. (2015). Effectiveness of preanalytic practices on contamination and diagnostic accuracy of urine cultures: a laboratory medicine best practices systematic review and meta-analysis. Clin. Microbiol. Rev. 29, 105–147. doi: 10.1128/CMR.00030-15
Leal, S. M., Jr, Jones, M., and Gilligan, P. H. (2016). Clinical significance of commensal gram-positive rods routinely isolated from patient samples. J. Clin. Microbiol. 54, 2928–2936. doi: 10.1128/JCM.01393-16
Legaria, M. C., Barberis, C., Famiglietti, A., De Gregorio, S., Stecher, D., Rodriguez, C. H., et al. (2022). Urinary tract infections caused by anaerobic bacteria. Utility of anaerobic urine culture. Anaerobe 78, 102636. doi: 10.1016/j.anaerobe.2022.102636
Lloyd-Price, J., Abu-Ali, G., and Huttenhower, C. (2016). The healthy human microbiome. Genome Med. 8, 51. doi: 10.1186/s13073-016-0307-y
Mancuso, G., Midiri, A., Gerace, E., Marra, M., Zummo, S., and Biondo, C. (2023). Urinary tract infections: the current scenario and future prospects. Pathogens 12, 623–640. doi: 10.3390/pathogens12040623
Marple, C. D. (1941). The frequency and character of urinary tract infections in a group of unselected women. Ann. Internal Med. 14, 2220–2239. doi: 10.7326/0003-4819-14-12-2220
Maskell, R. (1986). Are fastidious organisms an important cause for dysuria and frequency- the case for (London, UK: John Wiley and Sons).
Maskell, R. A. (1988). Pathogenesis, consequences and natural history of urinary tract infection (London, UK: Edward Arnold).
McFadyen, I. R. and Eykyn, S. J. (1968). Suprapubic aspiration of urine in pregnancy. Lancet 1, 1112–1114. doi: 10.1016/S0140-6736(68)90185-2
Midby, J. S. and Miesner, A. R. (2024). Delayed and non-antibiotic therapy for urinary tract infections: A literature review. J. Pharm. Pract. 37, 212–224. doi: 10.1177/08971900221128851
Moreland, R. B., Brubaker, L., Tinawi, L., and Wolfe, A. J. (2024). Rapid and accurate testing for urinary tract infection: new clothes for the emperor. Clin. Microbiol. Rev. 38, e0012924. doi: 10.1128/cmr.00129-24
Moreland, R. B., Choi, B. I., Geaman, W., Hochstedler-Kramer, B. R., Gonzalez, C., John, J., et al. (2023). Beyond the usual suspects: emerging uropathogens in the microbiome age. Front. Urol. 3. doi: 10.3389/fruro.2023.1212590
Mundt, L. A. and Shanahan, K. (2010). Chapter 4. Chemical Analysis of Urine (Philadelphia, PA: Wolters-Kluwer/Lippincott Williams & Wiliams).
Murray, J. L., Connell, J. L., Stacy, A., Turner, K. H., and Whiteley, M. (2014). Mechanisms of synergy in polymicrobial infections. J. Microbiol. 52, 188–199. doi: 10.1007/s12275-014-4067-3
Neugent, M. L., Hulyalkar, N. V., Nguyen, V. H., Zimmern, P. E., and De Nisco, N. J. (2020). Advances in understanding the human urinary microbiome and its potential role in urinary tract infection. mBio 11, 100753–100770. doi: 10.1128/mBio.00218-20
Neugent, M. L., Kumar, A., Hulyalkar, N. V., Lutz, K. C., Nguyen, V. H., Fuentes, J. L., et al. (2022). Recurrent urinary tract infection and estrogen shape the taxonomic ecology and function of the postmenopausal urogenital microbiome. Cell Rep. Med. 3, 100753. doi: 10.1016/j.xcrm.2022.100753
Nouioui, I., Carro, L., García-López, M., Meier-Kolthoff, J. P., Woyke, T., Kyrpides, N. C., et al. (2018). Genome-based taxonomic classification of the phylum actinobacteria. Front. Microbiol. 9, 2007. doi: 10.3389/fmicb.2018.02007
Nunn, K. L. and Forney, L. J. (2016). Unraveling the dynamics of the human vaginal microbiome'. Yale J. Biol. Med. 89, 331–337.
O'Leary, B. D., Armstrong, F. M., Byrne, S., Talento, A. F., and O'Coigligh, S. (2020). The prevalence of positive urine dipstick testing and urine culture in the asymptomatic pregnant woman: A cross-sectional study. Eur. J. Obstet. Gynecol. Reprod. Biol. 253, 103–107. doi: 10.1016/j.ejogrb.2020.08.004
Pagan, L., Ederveen, R. A. M., Huisman, B. W., Schoones, J. W., Zwittink, R. D., Schuren, F. H. J., et al. (2021). The human vulvar microbiome: A systematic review. Microorganisms. 9 (12), 2568. doi: 10.3390/microorganisms91225
Palavecino, E. L., Campodónico, V. L., and She, R. C. (2024). Laboratory approaches to determining blood culture contamination rates: an ASM laboratory practices subcommittee report. J. Clin. Microbiol. 262 (2), e0102823. doi: 10.1128/jcm.01028-23
Park, M. G., Cho, S., and Oh, M. M. (2023). Menopausal changes in the microbiome-A review focused on the genitourinary microbiome. Diagn. (Basel). 13, 1193. doi: 10.3390/diagnostics13061193
Peach, B. C., Garvan, G. J., Garvan, C. S., and Cimiotti, J. P. (2016). Risk factors for urosepsis in older adults: A systematic review. Gerontol. Geriatr. Med. 2, 2333721416638980. doi: 10.1177/2333721416638980
Pearce, M. M., Hilt, E. E., Rosenfeld, A. B., Zilliox, M. J., Thomas-White, K., Fok, C., et al. (2014). The female urinary microbiome: a comparison of women with and without urgency urinary incontinence. mBio 5, e01283–e01214. doi: 10.1128/mBio.01283-14
Pearce, M. M., Zilliox, M. J., Rosenfeld, A. B., Thomas-White, K. J., Richter, H. E., Nager, C. W., et al. (2015). The female urinary microbiome in urgency urinary incontinence. Am. J. Obstet. Gynecol. 213, 347 e1–347 11. doi: 10.1016/j.ajog.2015.07.009
Philpot, V. B. (1956). The bacterial flora of urine specimens from normal adults. J. Urol. 75, 562–568. doi: 10.1016/S0022-5347(17)66848-4
Pohl, H. G., Groah, S. L., Pérez-Losada, M., Ljungberg, I., Sprague, B. M., Chandal, N., et al. (2020). 'The urine microbiome of healthy men and women differs by urine collection method. Int. Neurourol. J. 24, 41–51. doi: 10.5213/inj.1938244.122
Price, T. K., Dune, T., Hilt, E. E., Thomas-White, K. J., Kliethermes, S., Brincat, C., et al. (2016). The clinical urine culture: enhanced techniques improve detection of clinically relevant microorganisms. J. Clin. Microbiol. 54, 1216–1222. doi: 10.1128/JCM.00044-16
Price, T. K., Hilt, E. E., Dune, T. J., Mueller, E. R., Wolfe, A. J., and Brubaker, L. (2017). Urine trouble: should we think differently about UTI? Int. Urogynecol. J. 29, 205–210. doi: 10.1007/s00192-017-3528-8
Price, T. K., Lin, H., Gao, X., Thomas-White, K. J., Hilt, E. E., Mueller, E. R., et al. (2020). Bladder bacterial diversity differs in continent and incontinent women: a cross-sectional study. Am. J. Obstet. Gynecol. 223, 729.e1–29.e10. doi: 10.1016/j.ajog.2020.04.033
Ravel, J., Gajer, P., Abdo, Z., Schneider, G. M., Koenig, S. S., McCulle, S. L., et al. (2011). Vaginal microbiome of reproductive-age women. Proc. Natl. Acad. Sci. U. S. A 108, 4680–4687. doi: 10.1073/pnas.1002611107
Roberts, W. (1881). On the occurrence of micro-organisms in fresh urine. Br. Med. J. 2, 623–625. doi: 10.1136/bmj.2.1085.623
Roll-Hansen, N. (1979). Experimental method and spontaneous generation: the controversy between Pasteur and Pouchet 1859–64. J. Hist. Med. Allied Sci. 34, 273–292. doi: 10.1093/jhmas/XXXIV.3.273
Ronald, L. S., Yakovenko, O., Yazvenko, N., Chattopadhyay, S., Aprikian, P., Thomas, W. E., et al. (2008). Adaptive mutations in the signal peptide of the type 1 fimbrial adhesin of uropathogenic Escherichia coli. Proc. Natl. Acad. Sci. U. S. A 105, 10937–10942. doi: 10.1073/pnas.0803158105
Ryan, R. P. and Dow, J. M. (2008). Diffusible signals and interspecies communication in bacteria. Microbiol. (Reading). 154, 1845–1858. doi: 10.1099/mic.0.2008/017871-0
Saraf, V. S., Sheikh, S. A., Ahmad, A., Gillevet, P. M., Bokhari, H., and Javed, S. (2021). Vaginal microbiome: normalcy vs dysbiosis. Arch. Microbiol. 203, 3793–3802. doi: 10.1007/s00203-021-02414-3
Schober, I., Koblitz, J., Sardà Carbasse, J., Ebeling, C., Schmidt, M. L., Podstawka, A., et al. (2025). Bac Dive in 2025: the core database for prokaryotic strain data. Nucleic Acids Res. 53, D748–DD56. doi: 10.1093/nar/gkae959
Sfeir, M. M. and Hooton, T. M. (2018). Practices of clinical microbiology laboratories in reporting voided urine culture results. Clin. Microbiol. Infect. 24, 669–670. doi: 10.1016/j.cmi.2017.12.023
Shafik, A., Shafik, I., El Sibai, O., and Shafik, A. (2005). Changes in the urine composition during its passage through the ureter. A concept of urothelial function. Urol. Res. 33, 426–428. doi: 10.1007/s00240-005-0499-x
Short, F. L., Murdoch, S. L., and Ryan, R. P. (2014). Polybacterial human disease: the ills of social networking. Trends Microbiol. 22, 508–516. doi: 10.1016/j.tim.2014.05.007
Siegman-Igra, Y. (1994). Polymicrobial and monomicrobial bacteraemic urinary tract infection. J. Hosp. Infect. 28, 49–56. doi: 10.1016/0195-6701(94)90152-X
Siegman-Igra, Y., Kulka, T., Schwartz, D., and Konforti, N. (1993). The significance of polymicrobial growth in urine: contamination or true infection. Scand. J. Infect. Dis. 25, 85–91. doi: 10.1080/00365549309169675
Siegman-Igra, Y., Schwartz, D., and Konforti, N. (1988). Polymicrobial bacteremia. Med. Microbiol. Immunol. 177, 169–179. doi: 10.1007/BF00232896
Siegman-IgraY (1994). The significance of urine culture with mixed flora. Curr. Opin. Nephrol. Hypertens. 3, 656–659. doi: 10.1097/00041552-199411000-00017
Simoni, A., Schwartz, L., Junquera, G. Y., Ching, C. B., and Spencer, J. D. (2024). Current and emerging strategies to curb antibiotic-resistant urinary tract infections. Nat. Rev. Urol. 21, 707–722. doi: 10.1038/s41585-024-00877-9
Szczerbiec, D., Piechocka, J., Głowacki, R., and Torzewska, A. (2022). Organic Acids Secreted by Lactobacillus spp. Isolated from Urine and Their Antimicrobial Activity against Uropathogenic Proteus mirabilis. Molecules 27, 5557. doi: 10.3390/molecules27175557
Thomas-White, K., Forster, S. C., Kumar, N., Van Kuiken, M., Putonti, C., Stares, M. D., et al. (2018). Culturing of female bladder bacteria reveals an interconnected urogenital microbiota. Nat. Commun. 9, 1557. doi: 10.1038/s41467-018-03968-5
Timm, M. R., Russell, S. K., and Hultgren, S. J. (2025). Urinary tract infections: pathogenesis, host susceptibility and emerging therapeutics. Nat. Rev. Microbiol. 23, 72–86. doi: 10.1038/s41579-024-01092-4
Valenstein, P. and Meier, F. (1998). Urine culture contamination: a College of American Pathologists Q-Probes study of contaminated urine cultures in 906 institutions. Arch. Pathol. Lab. Med. 122, 123–129.
Vollstedt, A., Baunoch, D., Wolfe, A., Luke, N., Wojno, K. J., Cline, K., et al. (2020). Bacterial interactions as detected by pooled antibiotic susceptibility testing (P-AST) in polymicrobial urine specimens. J. Surg. Urol. 1, 101.
Wang, D., Haley, E., Luke, N., Festa, R. A., Zhao X Anderson, L. A., Allison, L. L., et al. (2023). Emerging and fastidious uropathogens were detected by M-PCR with similar prevalence and cell density in catheter and midstream voided urine indicating the importance of these microbes in causing UTIs. Infect. Drug Resist. 16, 7775–7795. doi: 10.2147/IDR.S429990
Werneburg, G. T. and Hsieh, M. H. (2024). Clinical microbiome testing for urology. Urol. Clin. North Am. 51, 493–504. doi: 10.1016/j.ucl.2024.06.007
Werneburg, G. T., Lewis, K. C., Vasavada, S. P., Wood, H. M., Goldman, H. B., Shoskes, D. A., et al. (2023). Urinalysis exhibits excellent predictive capacity for the absence of urinary tract infection. Urology 175, 101–106. doi: 10.1016/j.urology.2023.02.028
Werneburg, G. T. and Southgate, J. (2024). Urinary microbiome research has not established a conclusive influence: revisiting koch's postulates. Eur. Urol. Focus 10, 686–687. doi: 10.1016/j.euf.2024.07.005
Whelan, P., Nelson, A., Kim, C. J., Tabib, C., Preminger, G., Turner, N. A., et al. (2022). Investigating risk factors for urine culture contamination in outpatient clinics: A new avenue for diagnostic stewardship. Antimicrob. Steward. Healthc. Epidemiol. 2, e29. doi: 10.1017/ash.2021.260
Wise, G. J. and Schlegel, P. N. (2015). Sterile pyuria. N. Engl. J. Med. 372, 1048–1054. doi: 10.1056/NEJMra1410052
Wojno, K. J., Baunoch, D., Luke, N., Opel, M., Korman, H., Kelly, C., et al. (2020). Multiplex PCR based urinary tract infection (UTI) analysis compared to traditional urine culture in identifying significant pathogens in symptomatic patients. Urol 136, 119–126. doi: 10.1016/j.urology.2019.10.018
Wolfe, A. J., Toh, E., Shibata, N., Rong, R., Kenton, K., Fitzgerald, M., et al. (2012). Evidence of uncultivated bacteria in the adult female bladder. J. Clin. Microbiol. 50, 1376–1383. doi: 10.1128/JCM.05852-11
Xu, R., Deebel, N., Casals, R., Dutta, R., and Mirzazadeh, M. (2021). A new gold rush: A review of current and developing diagnostic tools for urinary tract infections. Diagnostics 11, 479. doi: 10.3390/diagnostics11030479
Xu, R., Ritts, R., and Badlani, G. (2024). Unresolved pyuria. Curr. Bladder. Dysfunct. Rep. 19, 1–9. doi: 10.1007/s11884-023-00730-6
Zandbergen, L. E., Halverson, T., Brons, J. K., Wolfe, A. J., and de Vos, M. G. J. (2021). The good and the bad: ecological interaction measurements between the urinary microbiota and uropathogens. Front. Microbiol. 12, 659450. doi: 10.3389/fmicb.2021.659450
Keywords: contamination, mixed culture, polymicrobial, urine culture, urinary tract infection, urogynecology, urology
Citation: Moreland RB, Brubaker L and Wolfe AJ (2025) Polymicrobial urine cultures: reconciling contamination with the urobiome while recognizing the pathogens. Front. Cell. Infect. Microbiol. 15:1562687. doi: 10.3389/fcimb.2025.1562687
Received: 18 January 2025; Accepted: 24 April 2025;
Published: 19 May 2025.
Edited by:
Ramadhani Chambuso, Harvard University, United StatesReviewed by:
Kylie Mansfield, University of Wollongong, AustraliaJay Prakash Kumar, National Institutes of Health (NIH), United States
Copyright © 2025 Moreland, Brubaker and Wolfe. This is an open-access article distributed under the terms of the Creative Commons Attribution License (CC BY). The use, distribution or reproduction in other forums is permitted, provided the original author(s) and the copyright owner(s) are credited and that the original publication in this journal is cited, in accordance with accepted academic practice. No use, distribution or reproduction is permitted which does not comply with these terms.
*Correspondence: Alan J. Wolfe, YXdvbGZlQGx1Yy5lZHU=