- 1Research Institute for Global Change, Japan Agency for Marine-Earth Science and Technology, Yokohama-Shi, Kanagawa, Japan
- 2Center for Earth Surface System, Atmosphere and Ocean Research Institute, The University of Tokyo, Kashiwa-Shi, Chiba, Japan
- 3Computational Climate Science Research Team, Advanced Institute for Computational Science/RIKEN, Kobe, Hyogo, Japan
The eastward shift of the enhanced activity of tropical cyclone to the central Pacific is a robust projection result for a future warmer climate, and is shared by most of the state-of-the-art climate models. The shift has been argued to originate from the underlying El-Ñino like sea surface temperature (SST) forcing. This study explores the possibility that the change of the activity of the Madden–Julian Oscillation (MJO) can be an additional, if not alternative, contributor to the shift, using the dataset of Yamada et al. (2010) from a global non-hydrostatic 14-km grid mesh time-slice experiment for a boreal-summer case. Within the case-study framework, we develop the hypothesis that an eastward shift of the high-activity area of the MJO, as manifested itself as the significant intra-seasonal modulation of the enhanced precipitation, is associated with the increased tropical cyclogenesis potential over the North central Pacific by regulating cyclonic relative vorticity and vertical shear. In contrast, the North Indian Ocean and maritime continent undergo relatively diminished genesis potential. An implication is that uncertainty in the future tropical cyclogenesis in some part of the Pacific and other ocean basins could be reduced if projection of the MJO and its connection with the underlying SST environment can be better understood and constrained by the improvement of climate models.
Introduction
The regional change of tropical cyclones in future warmer climate has been a highly debated area of research. Even the sign of change in cyclone frequency differs from one model to another based on well-coordinated recent model datasets from Coupled Model Intercomparison Project (CMIP) 3 and 5 (Knutson et al., 2010; Camargo, 2013; Emanuel, 2013; Tory et al., 2013). However, the state-of-the art hydrostatic general circulation models all project an overall increase of genesis in the central Pacific, compared to present-day climatic conditions (Li et al., 2010; Murakami et al., 2012; Zhao and Held, 2012). This change was mostly ascribed to the underlying El-Ñino-type sea surface temperature (SST) forcing which was specified as a warmer climate condition. Notably, a time-slice experiment with the global non-hydrostatic model also projected a similar change of tropical cyclone activity (Yamada et al., 2010; Y2010). It remains unclear however how the change of the tropical cyclogenesis would be influenced by the associated changes of atmospheric phenomena such as the Madden–Julian Oscillation (MJO, Madden and Julian, 1971, 1972). Li et al. (2010) indicated that the enhanced tropical cyclone activity over the central Pacific region can be related to an increased variance of tropical synoptic-scale perturbations. This short article sheds a new light on this problem by focusing on a link between the change in the role and horizontal distribution of the MJO and the tropical cyclogenesis over the North central Pacific under a warmer climate state based on the experiment reported by Yamada et al. (2010). Note that the boreal-summer season is the focus of this research.
The model used is the Non-hydrostatic ICosahedral Atmospheric Model (NICAM, Tomita and Satoh, 2004; Satoh et al., 2008), a global model that is capable of calculating meso-scale convection, which is an essential building block of tropical convection, but a most elusive element in traditional hydrostatic models. With these benefits, NICAM simulations captured boreal winter- (Miura et al., 2007; Fudeyasu et al., 2008), spring- (Taniguchi et al., 2010), and summer-time (Oouchi et al., 2009, 2012; Satoh et al., 2012) MJO events and associated tropical cyclogenesis as well. The application of NICAM to research on the future change of the MJO and tropical cyclogenesis draws strongly upon these successful case studies. This study does not go beyond the case-study framework. As the model needs large computational resources, we were required to make some compromises with respect to various aspects, e.g., temporal duration and the size of ensembles in order to perform the integration necessary to obtain a greater statistical validity for intra-seasonal phenomenon such as MJO. The time integration is 5 months each of present and future experiments. Among various issues of MJO, this study focuses on the geographical change of the MJO activity that can be related to tropical cyclogenesis. The design of the experiment is explained in section Experimental Design, which is followed by the presentation of the results in section Results. Section Summary and Remarks concludes with a summary and further remarks.
Experimental Design
The NICAM experiments are performed using a grid spacing of around 14-km. The method used is a time-slice experiment (Bengtsson et al., 1996), and details are explained in Yamada et al. (2010). To be brief, the model is integrated over the five (JJASO) and six (MJJASO)—months period for present-climate (PRESENT) and future (FUTURE) experiment, respectively. The SST in PRESENT is derived from the NOAA Optimum Interporation (OI) SST V2 dataset for 2004 (Reynolds et al., 2002). In FUTURE, the model was spun up during all of May, which is excluded from the analysis. The FUTURE SST forcing is created by adding the differences between PRESENT (1979–2003) and FUTURE (2075–2099) onto the PRESENT SST with the dataset of the World Climate Research Program Coupled Model Inter-comparison Project phase 3 (CMIP3), following the method of Mizuta et al. (2008). The forcing has an El-Ñino like horizontal pattern (Y2010, Figure 1) which, as argued later, affects the interpretation of the results. The projection of tropical cyclone change in the same suite of data is reported in Y2010 along with its comparison with a downscaling method (Emanuel et al., 2010). The tracking methodology of tropical cyclone follows Yamada et al. (2010). The observational dataset is from the Unisys Corporation (http://weather.unisys.com/hurricane/).
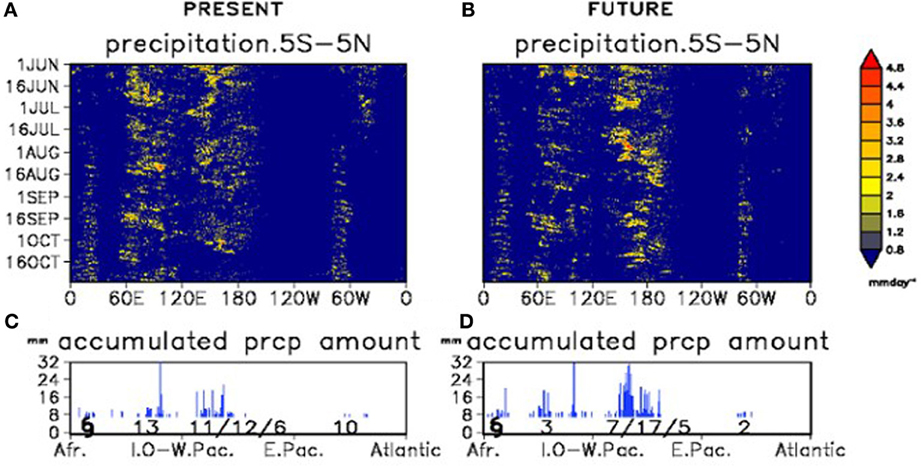
Figure 1. (A) Hovmoller plots of precipitation averaged over 5° S–5° N [mm day−1] for PRESENT. (B) Same as (A) except for FUTURE. (C) Blue bars: accumulated precipitation amount [mm] summed over the date with higher precipitation rate (more than 8 mm day−1) during JJASO for PRESENT; bottom numerals: tropical cyclone counts for western Pacific, Indian Ocean, Pacific, and Atlantic. Pacific area are divided as 100°–150° E, 150° E–160° W, and 160°–90° W with slash marks, (D) Same as (C).
Results
We first focus on a comparison of the time series of equatorial precipitation, zonal distribution of its accumulated amount, and zonal-vertical circulation in the tropical region between PRESENT and FUTURE (Figure 1). A significant future change in precipitation is the increased precipitation near the dateline, a location which closely coincides with the underlying largest SST forcing (Figures S1A,B). The precipitation undergoes a marked intra-seasonal modulation in FUTURE. Consequently, the main area of upwelling for JJASO is located more eastwardly in FUTURE, and is characterized by the intensified vertical shear in the western and eastern branches with respect to its center (Figures S1C,D).
The change in the zonal circulation pattern is also confirmed by both the Walker Circulation Index (WCI, Wang, 2002) and also the tropospheric (from the surface to 150 hPa) moisture convergence flux (MFlx) averaged over the Indian ocean (60–100°E) (Figure S2). The Walker circulation is driven by the temperature difference in the underlying SST along the equatorial Pacific, and the WCI measures the vertical velocity anomaly difference between the eastern and western Pacific region at the 500-hPa level (See Wang, 2002 for a detailed definition).
An overall comparison reveals that throughout the simulation periods, the sign of the temporal WCI variation is almost in phase with that of MFlx in PRESENT, and out of phase in FUTURE. In other words, the zonal circulation pattern over the Indian-Ocean to the entire Pacific sector changes in FUTURE from bimodal to unimodal consisting respectively, of upward/downward motions in the central Pacific and downward/upward motions in the western Pacific and the Indian Ocean.
Figures 1C,D is annotated with tropical cyclone counts during JJASO. Each of the three numbers separated by slash marks are the Pacific counts for 100–150° E, 150o E–160o W, and 160–90o W, respectively. The total number of tropical cyclones decreases in FUTURE, as previously shown by Y2010, and consistent with other previous studies. However, an increase in the FUTURE count is evident over the central Pacific (150o E–160o W), in contrast with a decrease over the western side (100–150o E), showing an eastward shift of tropical cyclogenesis in FUTURE. Figure 1B shows the intra-seasonal variation of precipitation over the central Pacific. This implies that the increase in tropical cyclones over the North central Pacific is related not solely to the change in stationary flows associated with the ENSO-type SST response, but also to changes in intra-seasonal oscillations, i.e., the MJO. It is unclear whether any intra-seasonal variability of precipitation over the central Pacific is associated with the MJO. The next question is in what way the active areas of the MJO and tropical cyclogenesis change in association with these changes in climate state.
The change in MJO activity can be seen in Figure 2 displaying the Hovmoller plots of zonal velocity anomaly at 200 hPa (A,B), and Real-time Multivariate (RMM) MJO indices (C,D) based on Wheeler and Hendon (2004) (WH04). The indices elucidate the behaviors of MJO over the different longitudes. They are based on extended empirical orthogonal function analysis of zonal velocity anomalies at 200 and 850 hPa in addition to the outgoing longwave radiation (OLR) anomaly derived from the daily outputs. To create anomalies, the climatological mean of the reanalysis dataset for the period 1979–2001 is subtracted from the raw output using NCEP/NCAR daily reanalysis (Kalnay et al., 1996) for velocities and NOAA for OLR. Each anomaly is then divided by its longitudinally-averaged normalization factor (as computed in WH04 to be 15.1 Wm−2 for OLR, 1.81 ms−1 for 850 hPa zonal wind, and 4.81 ms−1 for 200 hPa zonal wind), and then these are projected onto the WH04 EOFs. Estimating the applicability of the indices to the future climate dataset is an important subject for future studies.
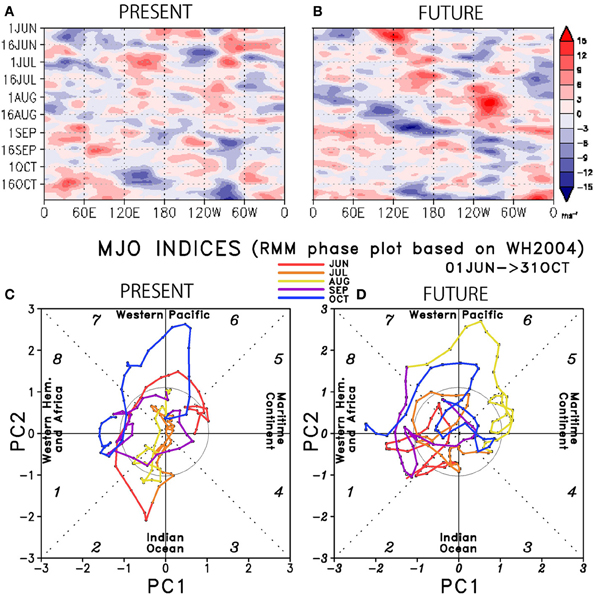
Figure 2. (A) Hovmoller plots of zonal velocity anomaly at 200 hPa from the time average over JJASO [ms−1] for PRESENT. (B) Same as (A) except for FUTURE. (C) PC1–PC2 phase-space points of JJASO MJO activity for PRESENT based on the method of Wheeler and Hendon (2004). For each of eight equal-angled phase-space categories, the approximate locations of the enhanced convective signal of MJO are labeled. (D) Same as (C) except for FUTURE.
There are eight phases for categorizing the convectively active signal of the MJO (PH1–8), each of which roughly corresponds to the area of active MJO center. Overall, the eastward movement of the velocity is seen both in PRESENT and FUTURE (Figures 2A,B), and clear amplifications of its eastward movement are detected in FUTURE (e.g., earlier in August over 120–60o W, and in late August over 120o E–180o). On the RMM phase space, the eastward movement of the MJO can be seen through the traces moving counter-clockwise across the corresponding geographical location. Figure 2C indicates the presence of an eastward movement from early June to early July which corresponds to the MJO case discussed by Oouchi et al. (2009). During July and August, no clear eastward movement is present compared to the observations (URL:http://cawcr.gov.au/staff/mwheeler/maproom/RMM/phasediag/pd.2004.6.1.gif), but it is followed by a stronger signal in October across PH6 and 7, being almost comparable to a western Pacific MJO signal in the early October (Nakazawa, 2006). As we do not expect the model to be skillful at simulating the behavior of the MJO beyond 20 days or so (Vitart, 2009), the apparent similarity of the signals later than mid-July is not examined further here. A comparison between PRESENT and FUTURE reveals a series of suppressed signals during June and July, while a stronger signal is present in August corresponding to the one mentioned above. Among PH1–8, the amplitude in FUTURE increases relatively in PH6 and 7, compared to PH2–4. These results are consistent with the intuitive impressions obtained by the Hovmoller diagrams shown by Figure 1.
To see a rough linkage between the change of the MJO and the environment of tropical cyclogenesis, Figures 3A,B illustrates the large RMM phase (LRP, eastward propagation only) which we define as the date when the difference of the maximum amplitude of MJO index between the consecutive 6-days period is larger than 1.2 (red triangles) or between 0.8 and 1.2 (blue triangles); (C,D) are the meridional-time plot of genesis potential density (GPD) defined as the ratio of genesis potential index (GPI; Emanuel and Nolan, 2004) with respect to the entire period and PH regions. In this study, GPI is calculated for each of the following areas for major ocean basins to facilitate discussion: (Indian Ocean, 45–90° E; maritime continent, 90–110° E; Western Pacific, 100–150° E; Central Pacific, 150° E–160° W; Eastern Pacific and Atlantic, 160–20° W. In PRESENT, we cannot see close correspondence between the LRP in excess of 1.2 and a higher GPD. In other words, GPD is not necessarily contributed to by the growth of RMM in PRESENT. On the other hand, in FUTURE the correspondence becomes stronger over the central Pacific (PH6 and 7), and to some degree over the Eastern Pacific and Atlantic, suggesting a closer link between the MJO's amplification, and higher GPD over the central Pacific. Interestingly, the higher GPD is more dominant over the central Pacific and the western hemisphere than it is elsewhere in FUTURE. This may be associated with the bimodal/unimodal circulation patterns in PRESENT/FUTURE (Figure S1).
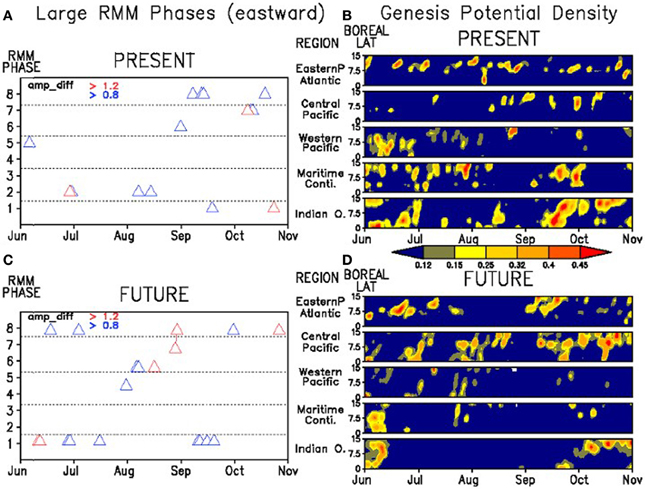
Figure 3. (A) The large RMM phases defined as the date when the difference of the maximum RMM amplitude between the consecutive 6-days period is larger than 1.2 (red) and 0.8 (blue) for PRESENT. (B) Same as (A) except for FUTURE. (C) Time series of genesis potential index (Emanuel and Nolan, 2004) density [%] for PRESENT for the following regions: Indian Ocean, 45–90° E; maritime continent, 90–110° E; Western Pacific, 100–150° E; Central Pacific, 150°E–160°W; Eastern Pacific and Atlantic, 160–20° W. The density is with respect to whole defined regions and period (JJASO). (D) Same as (C) except for FUTURE.
To investigate the link between MJO and tropical cyclogenesis potential in the North central Pacific, the meridional-time plot of relative vorticity anomaly at 850 hPa over 150o E–160o W is shown in the upper panel of Figure 4A for PRESENT and (B) for FUTURE. The “central” Pacific region is selected, as defined in the tropical cyclone count in the slash-marked values in Figures 1A,B. The bottom sub-panels (A,B) plot the time series of the relative vorticity, RMM amplitude (larger than 0.8), and vertical shear averaged over 0–10° N and the longitudinal region. A comparison between PRESENT and FUTURE reveals that increased cylonic vorticity is more notable in FUTURE. The increase closely coincides with that of the RMM amplitude and also the decrease of the vertical shear, and these features become more pronounced in FUTURE. This suggests that the propagation of the MJO and associated enhancement of vorticity and weakening of vertical shear can contribute positively to tropical cyclogenesis over the North central Pacific region in FUTURE. The tropical cyclogenesis locations are plotted in the upper panels of Figures 4A,B. We can see that the genesis in FUTURE occurs at the timing of the larger RMM phase, and it is associated with larger values of cyclonic vorticity and negative anomaly of vertical shear more clearly than PRESENT. A clear case occurs during first August–mid September. These results suggest a possible connection between the eastward shift of the MJO location and that of tropical cyclogenesis over the North central Pacific in FUTURE.
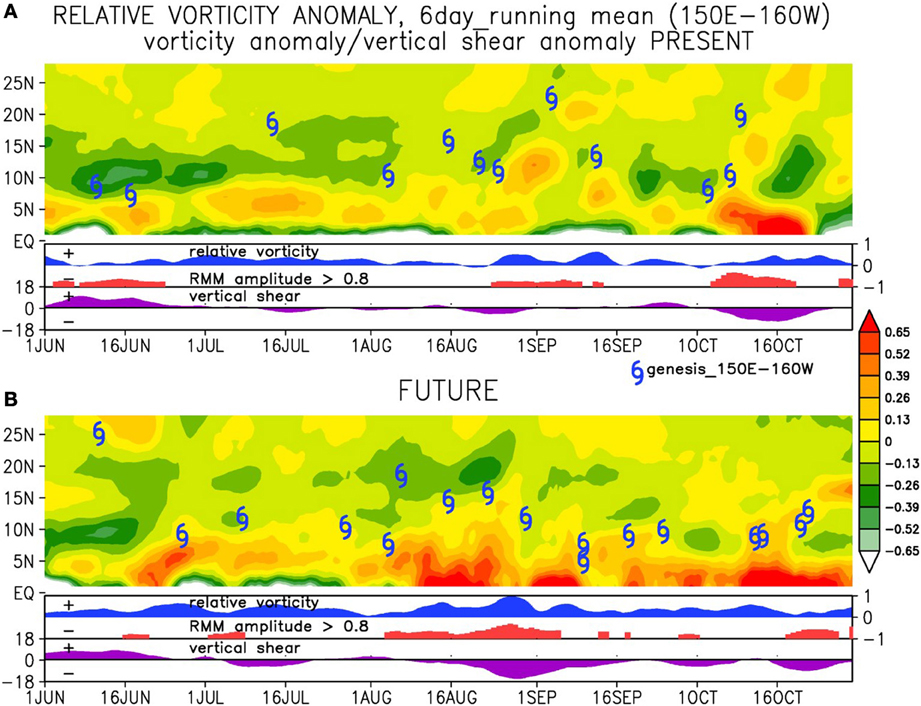
Figure 4. (A) Top; Meridional-time plot of relative vorticity at 850 hPa averaged over 150° E–160° W (the “central” Pacific region as defined in the tropical cyclone count in the slash-marked values in Figures 1C,D) for PRESENT, Bottom; the time series of relative vorticity (multiplied by arbitrary factor), RMM amplitude (larger than 0.8), and vertical shear averaged over the above longitudinal region and 0–10° N. The tropical cyclone marks indicate the genesis points in the region. (B) Same as (A) except for FUTURE.
Summary and Remarks
The eastward shift of the enhanced Pacific tropical cyclone activity—from the western Pacific to the central Pacific—under a future warmer climate has been a widely projected result in the global climate models, including the global non-hydrostatic model (Y2010). However, no previous studies have discussed its association with the change of the MJO. This study proposes a hypothesis for this question within a boreal summer case-study framework of Y2010 using the global non-hydrostatic model NICAM. Although the simulation is too short to consider inter-annual variability, numerical results show a common signal of decrease in the total number of tropical cyclones in FUTURE (Y2010). In our result, the increase in tropical cyclones is seen over the North central Pacific; this motivates us to further investigate what causes the enhancement of tropical cyclone activity over the North central Pacific in FUTURE. We found that the simulated MJO becomes active in August in FUTURE, and tropical cyclones are more generated associated with this active MJO.
Our study has the following implications: (1) an eastward shift of the higher activity area of the MJO reaching the central Pacific with its likely association with the underlying SST forcing, enhanced precipitation and Walker circulation; (2) relatively weakened activity over the tropical warm pool across the Indian Ocean to maritime continent; (3) an increase in tropical cyclogenesis potential over the North central Pacific associated to some extent with the propagation of MJO that favorably controls the vertical shear and relative vorticity, and (4) relatively suppressed tropical cyclogenesis potential over the North Indian Ocean and maritime continent, whose association with MJO activity relative to other factors remains to be substantiated. The finding (1) supports the prevailing notion that the warmer SST condition helps sustain or enhance the development of MJO, and is consistent with the observational evidence that convective activity and the atmospheric response to SST propagates more eastwardly into the central Pacific in El-Ñino years (Dunkerton and Crum, 1995; Hendon et al., 1999; Kessler, 2001). It remains to be completely clarified whether TC genesis under such a condition would be more likely to appear under either or both of the effects of MJO and SST, which may not be competing depending on the time scale of interest.
In the context of the present climate, there is a large body of observational studies on the possible modulation of tropical cyclones by the MJO over the global basins (e.g., Camargo et al., 2009), the western Pacific (e.g., Nakazawa, 1986; Liebmann et al., 1994; Kim et al., 2008), and the Atlantic (Klotzbach, 2010). This paper focuses on the potential increase of tropical cyclones through the change of MJO-associated synoptic-scale environmental fields including the parameters relevant to the modulation associated with the MJO (e.g., intensified relative vorticity, and moderated vertical shear, Camargo et al., 2009) over the central Pacific. Clarification of the mechanism behind the causal relationship between the activation of the MJO and the net increase of TC awaits further study. One might argue that such a straightforward extrapolation of “present-climate” analogy to future condition needs to be carefully considered. There can be other factors responsible for increase of the total number of TCs that may differ from one basin to another depending on the different background states between future and present climate conditions, and this question is left for future investigation. It should also be noted that much longer-term simulation is necessary to clarify how TC modulation is affected by different MJO-phases (both active- and inactive- MJO periods), in addition to the MJO-active case presented here. These concerns need to be addressed in future.
While our results suggest that our understanding of changes in Pacific tropical cyclogenesis in a future warmer climate may be enhanced by further research on the link between MJO and associated tropical cyclogenesis, much work remains to be done. As only a very few MJO events are simulated in our study, the generality of the results cannot be proven in any climatological sense, and this needs to be investigated in future with longer-period simulations. This study does not consider future changes of the MJO (and evaluation of the MJO index) per se, which would need a larger sample size with extended simulation period, and which could bring new challenges of its own. Additionally, some cautions need to be kept in mind to ensure progress in a suitable direction. Pacific tropical cyclogenesis is known to be substantially affected by other tropical disturbances in addition to the MJO, including equatorial waves (e.g., Li, 2012). Even when the MJO apparently controls cyclogenesis, other disturbances can play roles in some way or another, associated with or independent of the presence of the MJO. In this article, the effects of the other disturbances are ignored in favor of the MJO, assuming that the MJO would exert the largest scale first-order control. Identifying the relative effects of the other disturbances will be a priority for future investigation. We also did not address the exact role of the MJO in controlling the frequency: whether it generates or modulates the tropical cyclone activity, and what sub-synoptic pathway is present to the emergent tropical cyclone. Future work should explore this issue. Given the shortness of the time integration spanning 5 months, this study is viewed as a case study for a boreal summer season. We plan to extend the experiment across the seasons to evaluate the statistical reliability of the findings. In the near future, we also plan to expand the temporal boundaries of the research as we get access to greater computing resources via the K-computer (Yokokawa et al., 2011).
Conflict of Interest Statement
The authors declare that the research was conducted in the absence of any commercial or financial relationships that could be construed as a potential conflict of interest.
Acknowledgments
The author thanks development members of NICAM team. The numerical experiments were performed on the Earth Simulator of JAMSTEC under the framework of KAKUSHIN project funded by the Ministry of Education, Culture, Sports, Science, and Technology, Japan. The CMIP3 sea surface temperature and sea ice concentration dataset was provided by the Meteorological Research Institute. The comments from reviewers helped improve the original manuscript.
Supplementary Material
The Supplementary Material for this article can be found online at: http://www.frontiersin.org/journal/10.3389/feart.2014.00001/abstract
References
Bengtsson, L., Botzet, M., and Esch, M. (1996). Will greenhouse-induced warming over the next 50 years lead to higher frequency and greater intensity of hurricanes? Tellus A 48, 57–73. doi: 10.1034/j.1600-0870.1996.00004.x
Camargo, S. J. (2013). Global and regional aspects of tropical cyclone activity in the CMIP5 models. J. Clim. 26, 9880–9902. doi: 10.1175/JCLI-D-12-00549.1
Camargo, S. J., Wheeler, M. C., and Sobel, A. H. (2009). Diagnosis of the MJO modulation of tropical cyclogenesis using an empirical index. J. Atmos. Sci. 66, 3061–3074. doi: 10.1175/2009JAS3101.1
Dunkerton, T. J., and Crum, F. X. (1995). Eastward propagating 2- to 15-day equatorial convection and its relation to the tropical intraseasonal oscillation. J. Geophys. Res. 100, 25781–25790. doi: 10.1029/95JD02678
Emanuel, K., and Nolan, D. S. (2004). “Tropical cyclone activity and the global climate system,” in Paper Presented at the 26th Conference on Hurricanes and Tropical Meteorology (Am. Meteorol. Soc) (Miami: Fla).
Emanuel, K. A. (2013). Downscaling CMIP5 climate models shows increased tropical cyclone activity over the 21st century. Proc. Natl. Acad. Sci. U.S.A. 110, 12219–12224. doi: 10.1073/pnas.1301293110
Emanuel, K. A., Oouchi, K., Satoh, M., Tomita, H., and Yamada, Y. (2010). Comparison of explicitly simulated and downscaled tropical cyclone activity in a high-resolution global climate model. J. Adv. Model. Earth Syst. 2, 9. doi: 10.3894/JAMES.2010.2.9
Fudeyasu, H., Wang, Y., Satoh, M., Nasuno, T., Miura, H., and Yanase, W. (2008). The global cloud-system-resolving model NICAM successfully simulated the life cycles of two real tropical cyclones. Geophys. Res. Lett. 35, L22808. doi: 10.1029/2008GL036003
Hendon, H. H., Zhang, C., and Glick, J. D. (1999). Interannual variation of the Madden-Julian osclliation during austral summer. J. Clim. 12, 2538–2550. doi: 10.1175/1520-0442(1999)012<2538:IVOTMJ>2.0.CO;2
Kalnay, E., Kanamitsu, M., Kistler, R., Collins, W., Deaven, D., Gandin, L., et al. (1996). The NCEP/NCAR 40-year reanalysis project. Bull. Amer. Meteor. Soc. 77, 437–471. doi: 10.1175/1520-0477(1996)077%3C0437:TNYRP%3E2.0.CO;2
Kessler, W. S. (2001). EOF representation of the Madden-Julian oscillation and its connection with ENSO. J. Clim. 14, 3055–3061. doi: 10.1175/1520-0442(2001)014%3C3055:EROTMJ%3E2.0.CO;2
Kim, J., Ho, C., Kim, H., Sui, C., and Park, S. K. (2008). Systematic variation of summertime tropical cyclone activity in the western North Pacic in relation to the Madden-Julian oscillation. J. Clim. 21, 1171–1191. doi: 10.1175/2007JCLI1493.1
Klotzbach, P. J. (2010). On the Madden-Julian oscillation-Atlantic hurricane relationship. J. Clim. 23, 282–293. doi: 10.1175/2009JCLI2978.1
Knutson, T. R., McBride, J. L., Chan, J., Emanuel, K., Holland, G., Landsea, C., et al. (2010). Tropical cyclones and climate change. Nat. Geosci. 3, 157–163. doi: 10.1038/ngeo779
Li, T. (2012). “Synoptic and climatic aspects of tropical cyclogenesis in western north Pacific,” in Cyclones: Formation, Triggers, and Control, Chapter 3, eds K. Oouchi and H. Fudeyasu (New York, NY: Nova Science Publishers), 61–94.
Li, T., Kwon, M., Zhao, M., Kug, J.-S., Luo, J.-J., and Yu, W. (2010). Global warming shifts Pacific tropical cyclone location, Geophys. Res. Lett. 37, L21804. doi: 10.1029/2010GL045124
Liebmann, B., Hendon, H. H., and Glick, J. D. (1994). The relationship between tropical cyclones of the western Pacific and Indian Oceans and the Madden-Julian oscillation. J. Meteorol. Soc. Japan 72, 401–411.
Madden, R., and Julian, P. (1971). Detection of a 40-50 day oscillation in the zonal wind in the tropical Pacific. J. Atmos. Sci. 28, 702–708. doi: 10.1175/1520-0469(1971)028%3C0702:DOADOI%3E2.0.CO;2
Madden, R., and Julian, P. (1972). Description of global scale circulation cells in the tropics with a 40–50 day period. J. Atmos. Sci. 29, 1109–1123. doi: 10.1175/1520-0469(1972)029%3C1109:DOGSCC%3E2.0.CO;2
Miura, H., Satoh, M., Nasuno, T., Noda, A. T., and Oouchi, K. (2007). A Madden-Julian oscillation event simulated using a global cloud-resolving model. Science 318, 1763–1765. doi: 10.1126/science.1148443
Mizuta, R., Adachi, Y., Yukimoto, S., and Kusunoki, S. (2008). Estimation of the Future Distribution of sea Surface Temperature and Sea Ice Using the CMIP3 Multi-Model Ensemble Mean. Technical Report 56, Meteorol Research Institute, Tsukuba.
Murakami, H., Wang, Y., Yoshimura, H., Mizuta, R., Sugi, M., Shindo, E., et al. (2012). Future changes in tropical cyclone activity projected by the new high-resolution MRI-AGCM. J. Clim. 25, 3237–3260. doi: 10.1175/JCLI-D-11-00415.1
Nakazawa, T. (1986). Intraseasonal variation of OLR in the tropics during the FGGE year. J. Meteor. Soc. Japan 64, 17–34; 72, 401–412.
Nakazawa, T. (2006). Madden-Julian Oscillation activity and typhoon landfall on Japan in 2004. SOLA 2, 136–139. doi: 10.2151/sola.2006-035
Oouchi, K., Noda, A. T., Satoh, M., Miura, H., Tomita, H., Nasuno, T., et al. (2009). A simulated preconditioning of typhoon genesis controlled by a boreal summer Madden-Julian Oscillation event in a global cloud-system-resolving model. SOLA 5, 65–68. doi: 10.2151/sola.2009-017
Oouchi, K., Taniguchi, H., Nasuno, T., Satoh, M., Tomita, H., Yamada, Y., et al. (2012). “A prototype quasi real-time intra-seasonal forecasting of tropical convection over the warm pool region: a new challenge of global cloud-system-resolving model for a field campaign,” in Cyclones: Formation, Triggers, and Control, Chapter 11, eds K. Oouchi and H. Fudeyasu (New York, NY: Nova Science Publishers Inc.), 233–248.
Reynolds, R. W., Rayner, N. A., Smith, T. M., Stokes, D. C., and Wang, W. (2002). An improved in situ and satellite SST analysis for climate. J. Clim. 15, 1609–1625. doi: 10.1175/1520-0442(2002)015%3C1609:AIISAS%3E2.0.CO;2
Satoh, M., Matsuno, T., Tomita, H., Miura, H., Nasuno, T., and Iga, S. (2008). Nonhydrostatic icosahedral atmospheric model (NICAM) for global cloud resolving simulations. J. Comput. Phys. 227, 3486–3514. doi: 10.1016/j.jcp.2007.02.006
Satoh, M., Oouchi, K., Nasuno, T., Taniguchi, H., Yamada, Y., Tomita, H., et al. (2012). The Intra-Seasonal Oscillation and its control of tropical cyclones simulated by high-resolution global atmospheric models. Clim. Dyn. 39, 2185–2206. doi: 10.1007/s00382-011-1235-6
Taniguchi, H., Yanase, W., and Satoh, M. (2010). Ensemble simulation of cyclone Nargis by a global cloud-system-resolving model – modulation of cyclogenesis by the Madden-Julian Oscillation. J. Meteor. Soc. Japan 88, 571–591. doi: 10.2151/jmsj.2010-317
Tomita, H., and Satoh, M. (2004). A new dynamical framework of nonhydrostatic global model using the icosahedral grid. Fluid Dyn. Res. 34, 357–400. doi: 10.1016/j.fluiddyn.2004.03.003
Tory, K. J., Chand, S. S., McBride, J. L., Ye, H., and Dare, R. A. (2013). Projected changes in late-twenty-first-century tropical cyclone frequency in 13 coupled climate models from phase 5 of the coupled model intercomparison project. J. Clim. 26, 9946–9959. doi: 10.1175/JCLI-D-13-00010.1
Vitart, F. (2009). Impact of the Madden Julian Oscillation on tropical storms and risk of landfall in the ECMWF forecast system. Geophys. Res. Lett. 36, L15802. doi: 10.1029/2009GL039089
Wang, C. (2002). Atmospheric circulation cells associated with the El Ñino-Southern Oscillation. J.Clim. 15, 399–419. doi: 10.1175/1520-0442(2002)015<0399:ACCAWT>2.0.CO;2
Wheeler, M., and Hendon, H. H. (2004). An all-season real-time multivariate MJO index: development of an index for monitoring and prediction. Mon. Wea. Rev. 132, 1917–1932. doi: 10.1175/1520-0493(2004)132%3C1917:AARMMI%3E2.0.CO;2
Yamada, Y., Oouchi, K., Satoh, M., Tomita, H., and Yanase, W. (2010). Projection of changes in tropical cyclone activity and cloud height due to green-house warming: global cloud-system-resolving approach. Geophys. Res. Lett. 37, L07709. doi: 10.1029/2010GL042518
Yokokawa, M., Shoji, F., Uno, A., Kurokawa, M., and Watanabe, T. (2011). “The K computer: Japanese next-generation supercomputer development project,” in Proceedings of 17 IEEE/ACM International Symposium on Low-Power Electronics and Design (Kobe), 371–372. doi: 10.1109/ISLPED.2011.5993668
Keywords: central-Pacific tropical cyclone, future MJO, global non-hydrostatic projection, model-derived hypothesis, seamless weather projection
Citation: Oouchi K, Satoh M, Yamada Y, Tomita H and Sugi M (2014) A hypothesis and a case-study projection of an influence of MJO modulation on boreal-summer tropical cyclogenesis in a warmer climate with a global non-hydrostatic model: a transition toward the central Pacific? Front. Earth Sci. 2:1. doi: 10.3389/feart.2014.00001
Received: 04 December 2013; Accepted: 28 January 2014;
Published online: 18 February 2014.
Edited by:
Jing-Jia Luo, Centre for Australian Weather and Climate Research, AustraliaReviewed by:
Matthew C. Wheeler, Bureau of Meteorology, AustraliaHoward J. Diamond, NOAA's National Climatic Data Center, USA
Copyright © 2014 Oouchi, Satoh, Yamada, Tomita and Sugi. This is an open-access article distributed under the terms of the Creative Commons Attribution License (CC BY). The use, distribution or reproduction in other forums is permitted, provided the original author(s) or licensor are credited and that the original publication in this journal is cited, in accordance with accepted academic practice. No use, distribution or reproduction is permitted which does not comply with these terms.
*Correspondence: Kazuyoshi Oouchi, Research Institute for Global Change, Japan Agency for Marine-Earth Science and Technology, 3173-25 Showamachi, Kanazawa-ku, Yokohama-Shi, Kanagawa 236-0001, Japan e-mail:ay1vdWNoaUBqYW1zdGVjLmdvLmpw