- 1Independent Researcher, Munich, Germany
- 2School of Environment, The University of Auckland, Auckland, New Zealand
Unheralded “blue-sky” eruptions from dormant volcanoes cause serious fatalities, such as at Mt. Ontake (Japan) on 27 September 2014. Could these events result from magmatic gas being trapped within hydrothermal system aquifers by elemental sulfur (Se) clogging pores, due to sharp increases in its viscosity when heated above 159°C? This mechanism was thought to prime unheralded eruptions at Mt. Ruapehu in New Zealand. Impurities in sulfur (As, Te, Se) are known to modify S-viscosity and industry experiments showed that organic compounds, H2S, and halogens dramatically influence Se viscosity under typical hydrothermal heating/cooling rates and temperature thresholds. However, the effects of complex sulfur compositions are currently ignored at volcanoes, despite its near ubiquity in long-lived volcano-hydrothermal systems. Changes in S-viscosity, leading to system sealing and sudden failure, can potentially occur at other volcanoes worldwide, including calderas, not only during phreatic eruptions. Models of impure S behavior must be urgently formulated to detect pre-eruptive warning signs before the next “blue-sky” eruption.
Introduction
Despite technological advances in the ability to forecast volcanic eruptions over the last decades via geophysical methods, signals of volcanic unrest are often unnoticed at passively degassing volcanoes. This was tragically shown by the sudden 27 September 2014 Mt. Ontake eruption that killed at least 56 hikers (http://www.theguardian.com/science/2014/oct/01/terrawatch-mount-ontake-eruption-japan-steam; http://www.japantoday.com/category/national/view/2-more-bodies-found-on-mt-ontake-bringing-death-toll-to-56).
At Ruapehu volcano, New Zealand, a lake of hot acidic sulfate-chloride water (Crater Lake, at 2518 m elevation, Figure 1) covers its active vents. Eruptions in 1988, 2006, and 2007 occurred unheralded when the lake was cold with no signals of unrest (Hurst et al., 1991; Christenson and Wood, 1993; Christenson et al., 2010; Kilgour et al., 2010). The 25 September 2007 eruption followed 6 months of low lake temperatures, and geophysical disturbances were only recorded a few minutes before the explosive events (Christenson et al., 2010; Kilgour et al., 2010). Elemental S (Se) was the most abundant vug-filling material of blocks ejected, together with anhydrite, gypsum, pyrite, and natroalunite, showing that the eruption occurred from within a sealed hydrothermal system (Christenson et al., 2010; Kilgour et al., 2010). The causes for sealing were attributed to a reduction of system porosity by: (1) liquid Se blocking the pores due to its massive increase in viscosity, when heated above 159°C (Christenson and Wood, 1993), or (2) by gradual alteration minerals deposition within the host-rock (Christenson, 2000; Christenson et al., 2010).
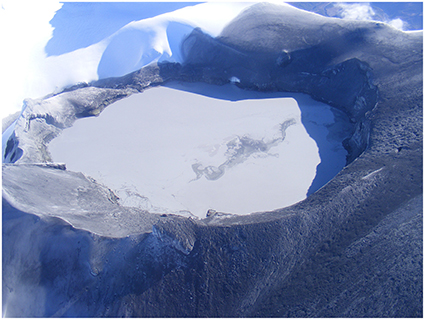
Figure 1. View of Ruapehu's Crater Lake (2518 m in elevation) after the unheralded 2007 explosive eruption, taken on 26 Sept 2007. The black deposits surrounding the lake are dominated by hydrothermally altered material exploded from the lake floor, including up to 5% elemental S.
Se accumulates over decadal quiescent intervals at Mt. Ruapehu and other similar vent-hosted hydrothermal systems by condensation of SO2 and H2S from shallow magmas. In Ruapehu's case, it forms a pool >50 m in diameter and >6 m deep below Crater Lake (Christenson and Wood, 1993). A similar process was invoked to explain the presence of a S-layer beneath Poás volcano (Costa Rica) (Oppenheimer and Stevenson, 1989). Transient S-layer(s) were also reported at Yugama crater-lake at Kusatsu Shirane (Japan) (Takano, 1987).
At ~160°C Se undergoes a four orders of magnitude increase in viscosity due to polymerization (Bacon and Fanelli, 1943; Meyer, 1976). This mechanism could temporarily seal a hydrothermal system, such as at Ruapehu, hindering passage of gas through it (Christenson and Wood, 1993). Pure sulfur viscosity is highly temperature dependent, but also extremely sensitive to the presence of impurities (Bacon and Fanelli, 1943; Meyer, 1976). This has been noted in volcanic settings (Takano, 1987; Rowe et al., 1992; Takano et al., 1994), but not followed up. Experiments carried out in the early 1940's (Bacon and Fanelli, 1943) showed that organic material, trivalent elements, ammonia, halogens, or the exposure to certain gases (e.g., H2S), almost always lead to lower viscosities on heating than for the pure Se case. Such modifications manifest only when certain temperature thresholds or heating rates are exceeded. These results require rethinking of how impure S behaves under the heating and gas influx regimes of volcanic systems.
Crater Lakes as Sensitive Indicators of S Viscosity
Re-examining, Crater Lake at Ruapehu shows it to be a good indicator of impure sulfur in priming explosive eruptions. It cycles between 10 and 60°C over periods of 4–16 months since 1945 (Hurst et al., 1991; Christenson and Wood, 1993; Hurst and McGinty, 1999; Christenson et al., 2010). Eruptions occurred near the peak of heating with seismicity, or, as described above with a cool lake without seismicity. A similar sudden eruption during declining levels of heat and seismicity occurred in the hydrothermal system at Mt. Tongariro (New Zealand) in 2012 (Procter et al., 2014). The waters of crater-lake at Poás volcano (Costa Rica) showed a similar “cyclic” behavior since 1978, attributed to the episodic release of heat and volatiles, either due to the fracturing of the upper margin of a shallow andesitic magma body, or to the upward migration of fresh magma (Rowe et al., 1992). At Ruapehu Hurst et al. (1991) explained the periodic variations in the lake temperature with S-pools following pure Se viscosity: temperature profiles that respond to changes in gas temperature/output from the underlying magma. The pure sulfur viscosity of 0.01 Pa·s between its melting point (at 116°C) and ~160°C, allows hot magmatic gases to escape (causing a hot lake). At >160 and up to ~200°C the sharp increase in viscosity (~100 Pa·s) causes trapping of the rising gas (lake cools). Over 300°C, Se viscosity drops to 3 Pa·s, releasing the gases again (lake heats). Unfortunately, this model based on pure Se is unlikely to be as simple as proposed, as reflected by more recent eruption cases (Christenson et al., 2010).
In a similar case, Kusatsu-Shirane volcano (Japan) ejected molten sulfur through the Yugama acidic crater-lake during several phreatic eruptions since 1805 (Takano et al., 1994). Takano (1987) noted that 2 months before an unheralded eruption on 26 October 1982, the concentration of polythionates ions (Sn) in the lake waters decreased, and that of sulfates increased. This was attributed to an increase in SO2/H2S ratio of the underlying fumaroles. A similar decrease in polythionate concentration in lake waters was reported at Poás volcano 3 months before an eruption in 1987, following a 7-year period of quiescence (Rowe et al., 1992). The increase in sulfate concentrations in the lake water was attributed to polythionate-breakdown reactions in the lake waters.
A decrease in polythionate (S4, S6O6) concentrations was observed also at Ruapehu's Crater Lake during very low temperatures for 3 months in 1988 prior to the Dec. 8, 1988 unheralded eruption (Takano et al., 1994). This apparently indicated that the liquid Se pool under the lake remained open to fumarolic degassing (H2S and SO2) even during periods of low lake temperatures, and therefore it could not be a barrier for magmatic heat transfer. Alternatively, Takano et al. (1994), suggest cyclic variation of the Ruapehu Crater Lake temperatures reflected variations in the deep P, T, and gas composition beneath the S-pool. This, however, is unable to explain the occurrence of unheralded eruptions.
Sulfur Properties
Up to 19 different melting points (i.e., 94–133°C) are reported for Se, due to the slow kinetics of the process, and the different reaction paths followed on cooling, which result in various metastable mixtures of different metastable allotropes (Meyer, 1964, 1976). Among allotropes, only orthorhombic (Sα) and monoclinic (Sβ) sulfur (both composed of packed S8 rings), are stable at low temperatures. According to Meyer (1976) Sα melts at 112.1°C, in some cases conversion into Sβ, drives the melting temperature up as far as 119°C. According to recent studies, this transition only occurs if the monoclinic allotrope is initially present in trace amounts and Cernosek et al. (2009) report a standard melting temperature at 116.3°C. This melting point is most commonly reported by volcanologists (e.g., Naranjo, 1985; Oppenheimer and Stevenson, 1989; Christenson et al., 2010), while 112.8°C is quoted in chemistry handbooks (e.g., Weast and Astle, 1978). Polymerization of Se begins at >159.4°C, and is most effective between 186 and 188°C, where viscosity reaches 93.2 Pa·s (four orders of magnitude higher than its initial 0.01 Pa·s). Viscosity decreases to ~3 Pa·s upon further heating to 300°C, until reaching ~0.1 Pa·s at its boiling point (444.6°C) as polymer chains break down (Bacon and Fanelli, 1943; Meyer, 1976).
The influence of impurities on S behavior was evaluated in the early 1940's (Bacon and Fanelli, 1942, 1943). Viscosity varied independently of the heating rates used for pure Se. Organic material in concentrations greater than 0.01% affected not only sulfur color, but also its friability, but this was not evident until heating to temperatures >200°C. Preheating S containing as little as 0.03 wt% organic material to 260°C drops its viscosity to ~4 Pa·s on cooling at 170–230°C (c.f., 93.2 Pa·s for pure S). This behavior was attributed to reactions between S and the organic material to form persulfides of hydrogen (H2S2 to H2Sx), which hinder polymerization (Bacon and Fanelli, 1943). The longer impure S was kept above 180–200°C, the higher the viscosities became on cooling, due to the breakdown or volatilization of hydrogen persulfides over time. Hydrogen persulfides also form by reactions of Se with H2S, H2Sx, hydrocarbons, and ammonia, thus reducing sulfur viscosity, under certain temperature thresholds, or heating rates. Even if unstable during heating (e.g., H2S2 boils at 75°C), hydrogen persulfides are stabilized by the presence of S, H2S, and organic compounds (Bacon and Fanelli, 1943).
Heating pure sulfur under a gas flux of H2S, at 140°C for 100 h, or heating of sulfur samples containing dissolved H2S between 180 and 210°C produced S viscosities of <10 Pa·s. Moreover, S never attained a viscous state and remained at 0.09 Pa·s when it was slowly heated under a H2S gas flux, between 125 and 190°C for 1.5 h. It decreased further (0.07 Pa·s) when the heating was prolonged for an additional 1.5 hrs. This low viscosity (compared to pure S) was also attributed to the formation of hydrogen persulfides due to reactions between H2S and liquid S.
Halogens, especially fluorine and chlorine (common in volcanic emissions), in concentrations of a few %, are also able to lower S-viscosity for indefinite periods in a closed system (Bacon and Fanelli, 1943). Heating S containing 1.8% Cl2 between 190 and 200°C in a closed system for ~200 h, led to S viscosity of <0.03 Pa·s, while 5% Cl2 produced the same result without extra heating.
In contrast, SO2 gas and H2SO4 have no effect on S viscosity (Bacon and Fanelli, 1943). Pure S-samples exposed to a gas flux of SO2 up to saturation at 136°C for 20 and 500 h, showed no change from pure S viscosities. The proportion of SO2 or H2S released from silicate melts depends on the oxidation state of the magma. Higher temperatures and lower f H2O (i.e., dry and mafic melts) promote higher SO2/H2S ratios, whereas felsic melts produce the opposite. Nevertheless, the degree of interaction between magmatic and hydrothermal fluids, the depth at which magma degasses (Oppenheimer, 2000), or gas reactions with cations in the host rocks also change the proportions of H2S and SO2 emitted (Rowe et al., 1992; Bernard et al., 2004).
How Variations in Impure S could Influence Eruptions
Sulfur in volcanic environments is far from pure, a fact largely overlooked by the most of the volcanological community. Previous studies (i.e., Takano, 1987; Rowe et al., 1992; Takano et al., 1994), noted the role of some types of impurities (H2S, Se, As, Te) as S-viscosity modifiers, but did not examine these further. Multiple distillations of sulfur containing organic material showed how hydrogen persulfides are responsible for maintaining low S viscosity for prolonged periods of heating, releasing H2S when hydrogen persulfides break up (Fanelli, 1942). The experimental findings imply that volcanic Se viscosity, when certain temperature thresholds (e.g., 200–260°C) are overcome during heating, will remain low for indefinite time when organic compounds are present, or under H2S or halogens inputs. However, a prolonged heating destroys or volatilizes viscosity-modifying substances, and higher viscosities and pore-blockage could be expected on S-cooling after a certain number of heating and cooling cycles.
Recognizing impure S behavior, unheralded eruptions at volcanoes like Ruapehu could thus be controlled by H2S and/or halogen gas inputs to volcanic S in the hydrothermal system. This is especially true if the S has experienced a complex heating and cooling history. At Ruapehu, high SO2 and CO2 accompanied five heating cycles of Crater Lake, between 2003 and 2008, whereas H2S was absent (Christenson et al., 2010). CO2 gas peaks likely reflect the escape of inert gas through low-viscosity liquid S, with this state being maintained by hydrogen persulfides forming in response to H2S gas supply, or reactions with organic impurities. High SO2 and CO2 emissions in the 7 months preceding the 2007 Ruapehu eruption show continuous degassing and prolonged heating. Experimentally, cooling of Se after prolonged heating with organic compounds, or dissolved H2S, produces sharp rises in viscosity (Bacon and Fanelli, 1943). Thus, cooling Se could trap gas release and prime an unheralded eruption, after long and intense heating episodes. The same process could also explain a phreatic event (June 29) before the major 1995 eruptive phase began at Ruapehu on 18 Sept. In this case, H2S was missing in fumarolic emissions 17 months before June 29, occurred when lake waters were cold, following two heating cycles (see Table 2 in Christenson, 2000).
Among the possible causes of unheralded eruptions at Ruapehu, the prolonged boiling of sulfur containing organic compounds is likely, considering the experimental findings during processes of sulfur purification (Bacon and Fanelli, 1942). During such process, organics separate as a “black mass,” during the first stages of boiling, accompanied by the production of hydrogen persulfides (H2Sx). Hydrogen persulfides are later disrupted with the emission of H2S (Bacon and Fanelli, 1942). The occurrence of hydrogen persulfides was observed also in chromatograms of sulfur slicks collected at various crater lakes. Takano et al. (1994) reported that 70% of the sulfur in sulfur-slicks samples collected in 1991 at Ruapehu was insoluble in CS2 used as a solvent to dissolve them. Nevertheless, experimental studies showed that sulfur reacts with most organic compounds, and the unsaturated organic compound may add to sulfur, polymerize, or both during multiple distillations processes. The residual insoluble black mass, obtained after boiling sulfur with a variety of organic substances, was found to be CS2 (Bacon and Fanelli, 1942).
At Kusatsu Shirane (Japan), Takano et al. (1994) reported that some S-slick samples were black and turned to yellow after some hours, while others remained black for years. These samples contained more than 10% iron sulfide as pyrite. They reported the occurrence of black S slicks containing pyrite also from Bannoe Lake (Kamchackta), and Kawah Ijen crater-lake (Indonesia). Based on the presence of pyrite and the absence of marcasite in diffraction pattern from the slicks, and the specific T-pH conditions related to the precipitation of the two sulfides with different crystal symmetry (i.e., T > 150°C and pH < 5 for pyrite and T < 150°C and pH > 5 for marcasite), Takano et al. (1994) inferred that the FeS2 content as pyrite attested the remobilization of deeper and hotter portions of the sulfur layers below crater lakes.
Instead we believe that black sulfur slicks can be better interpreted as organic residues as a result of a prolonged heating processes of sulfur containing organic impurities, as reported in processes of industrial purification of sulfur (Fanelli, 1942).
In volcanic environments, CH4, commonly observed in fumarolic emissions, can be reasonably considered a source of organic impurity in sulfur. The thermal degradation of old vegetative material, which occurrence is common in many volcano-hydrothermal systems, is considered responsible to produce hydrocarbons (Taran and Giggenbach, 2003). The presence of other types of organic impurities has been not reported in sulfur samples of hyperacidic crater lakes, even if sulfur-oxidizing bacteria have been observed seasonally at Yugama crater-lake (Takano et al., 1997).
Sulfur layers or pore-filling liquid S have been reported, or their presence inferred, at several andesitic volcanoes hosting active hydrothermal systems (e.g., Oppenheimer and Stevenson, 1989; Takano et al., 1994; Scolamacchia and Dingwell, 2014; Delmelle and Bernard, 2015). The ubiquitous presence of sulfur in volcanic areas suggests that also its accumulation at depth is common in many vent-hydrothermal systems. The ubiquitous pore-filling elemental sulfur in ejected ballistics from phreatic eruptions from hydrothermal systems (e.g., Nairn et al., 1979; Kilgour et al., 2010, at Ruapehu; Breard et al., 2014, at Tongariro) supports this hypothesis, at volcanoes with crater-lakes. Nevertheless, the absence of elemental S from ejecta should not be considered an indication of its absence from hydrothermal systems. In fact, the presence of crater lakes can be only a feature limited in time. Several volcanoes have shown transitions between periods of dome growth and periods with crater lakes (e.g., Pinatubo, Philippines Stimac et al., 2004; Soufrière, St.Vincent, Lesser Antilles; Fournier et al., 2011), and sulfur melting and remobilization has been observed at volcanoes with no records of historical eruptions, and no crater lakes (e.g., Lastarria, Chile, Naranjo, 1985). Accumulated sulfur can be subjected to “combustion” (Oppenheimer, 1996) or “volatilization” (Christenson, 2000), during different eruptive phases, contributing to an increase in the SO2 released.
Considering sulfur's unusual viscosity variations under the complex temperature and chemical regimes at volcanoes and hydrothermal systems, it could be a common trigger for unheralded eruptions. Modern techniques to evaluate the variations of impure sulfur viscosity, and a robust temporal scaling of experimental results to volcanic environments, are urgently needed to better understand the situations that lead to gas-overpressures in volcanic systems.
Author Contributions
TS reviewed the literature on sulfur properties, suggested that the hypothesis applies to volcanic areas and interpreted the data at Ruapehu and Poás. SC applied the hypothesis to Ontake, Tongariro. Both authors drafted the manuscript.
Conflict of Interest Statement
The authors declare that the research was conducted in the absence of any commercial or financial relationships that could be construed as a potential conflict of interest.
Acknowledgments
SC contribution is supported by the Living with Volcanic Risk Programme, funded by the New Zealand Natural Hazards Research Platform.
Claus Siebe is thanked for fruitful discussions during early stages of the draft of this manuscript.
Very detailed reviews were provided by Eisuke Fujita, Pierre Delmelle, and an anonymous reviewer, who contributed to clarify the ideas stated in this manuscript and strengthen the hypotheses made.
References
Bacon, R. F., and Fanelli, R. (1942). Purification of sulfur. Industr. Engin. Chem. 34, 1043–1048. doi: 10.1021/ie50393a006
Bacon, R. F., and Fanelli, R. (1943). The viscosity of sulfur. J. Am. Chem. Soc. 65, 639–648. doi: 10.1021/ja01244a043
Bernard, A., Escobar, C. D., Mazot, A., and Gutierrez, R. E. (2004). “The acid volcanic lake of Santa Ana volcano, El Salvador,” in Natural Hazards in El Salvador eds W. I. Rose, J. J. Bommer, D. L. López, M. J. Carr, and J. J. Major (Boulder, CO: Geological Society of America Special Papers), 121–133.
Breard, E. C. P., Lube, G., Cronin, S. J., Fitzgerald, R., Kennedy, B., Scheu, B., et al. (2014). Using the spatial distribution and lithology of ballistic blocks to interpret eruption sequence and dynamics: August 6 2012 Upper Te Maari eruption, New Zealand. J. Volcanol. Geotherm. Res. 286, 373–386 doi: 10.1016/j.jvolgeores.2014.03.006
Cernosek, Z., Holubova, E., Cernoskova, E., and Ruzicka, A. (2009). Sulfur- a new information on this seemingly well-known element. J. Non-oxide Glasses 1, 38–42. Available online at: http://www.chalcogen.ro/index.php/journals/journal-of-non-oxide-glasses/14-jnog/342-volume-1-number-1-january-march-2009
Christenson, B. W. (2000). Geochemistry of fluids associated with the 1995-1996 eruption of Mt. Ruapehu, New Zealand: signatures and processes in the magmatic-hydrothermal system. J. Volcanol. Geotherm. Res. 97, 1–30. doi: 10.1016/S0377-0273(99)00167-5
Christenson, B. W., Reyes, A. G., Young, R., Moebis, A., Sherburn, S., Cole-Baker, J., et al. (2010). Cyclic processes and factors leading to phreatic eruption events: insights from the 25 September 2007 eruption through Ruapehu Crater Lake, New Zealand. J. Volcanol. Geotherm. Res. 191, 15–32. doi: 10.1016/j.jvolgeores.2010.01.008
Christenson, B. W., and Wood, C. P. (1993). Evolution of a vent-hosted hydrothermal system beneath Ruapehu Crater Lake, New Zealand. Bull. Volcanol. 55, 547–565. doi: 10.1007/BF00301808
Delmelle, P., and Bernard, A. (2015). “The remarkable chemistry of sulfur in hyper-acid crater lakes: a scientific tribute to Bokuichiro Takano and Minoru Kusakabe,” in Volcanic Lakes, Advances in Volcanology, eds D. Rouwet, B. Christenson, F. Tassi, and J. Vandemeulebrouck (Berlin; Heidelberg: Springer-Verlag), 239–259.
Fournier, N., Moreau, M., and Robertson, R. (2011). Disappearance of a crater lake: implications for potential explosivity at Soufriére volcano, St Vincent, Lesser Antilles. Bull. Volcanol. 73, 543–555. doi: 10.1007/s00445-010-0422-3
Hurst, A. W., Bibby, H. M., Scott, B. J., and McGuinness, M. J. (1991). The heat source of Ruapehu Crater Lake: deductions from the energy and mass balances. J. Volcanol. Geotherm. Res. 46, 1–20. doi: 10.1016/0377-0273(91)90072-8
Hurst, A. W., and McGinty, P. J. (1999). Earthquake swarms to the west of Mt Ruapehu preceeding its 1995 eruption. J. Volcanol. Geotherm. Res. 90, 19–28. doi: 10.1016/S0377-0273(99)00019-0
Kilgour, G., Manville, V., Della Pasqua, F., Graettinger, A., Hogdson, K. A., and Jolly, G. E. (2010). The 25 September 2007 eruption of Mount Ruapehu, New Zealand: direected ballistics, surtseyan jets and ice-slurry lahars. J. Volcanol. Geotherm. Res. 191, 1–14. doi: 10.1016/j.jvolgeores.2009.10.015
Nairn, I. A., Wood, C. P., and Hewson, C. A. Y. (1979). Phreatic eruptions of Ruapehu: April 1975, N. Z. J. Volcanol. Geophys. 22, 155–173. doi: 10.1080/00288306.1979.10424215
Naranjo, J. A. (1985). Sulphur flows at Lastarria volcano in the North Chilean Andes. Nature 313, 778–780.
Oppenheimer, C. (1996). On the role of hydrothermal systems in the transfer of volcanic sulfur to the atmosphere. Geophys. Res. Lett. 23, 2057–2060. doi: 10.1029/96GL02061
Oppenheimer, C. (2000). Ultraviolet sensing of volcanic sulfur emissions. Elements 6, 87–92. doi: 10.2113/gselements.6.2.87
Oppenheimer, C., and Stevenson, D. (1989). Liquid sulphur lakes at Poás volcano. Nature 342, 790–793. doi: 10.1038/342790a0
Procter, J. N., Cronin, S. J., Zernack, A. V., Lube, G., Stewart, R. B., Nemeth, K., et al. (2014). Debris flow evolution and activation of an explosive hydrothermal system; Te Maari, Tongariro, New Zealand. J. Volcanol. Geotherm. Res. 286, 303–316. doi: 10.1016/j.jvolgeores.2014.07.006
Rowe, L. G. Jr., Ohsawa, S., Takano, B., Brantley, S. L., Fernandez, J. L., and Barquero, J. (1992). Using Crater Lake chemistry to predict volcanic activity at Poás volcano, Costa Rica. Bull. Volcanol. 54, 494–503. doi: 10.1007/BF00301395
Scolamacchia, T., and Dingwell, D. B. (2014). Sulfur as a binding agent of aggregates in explosive eruptions. Bull. Volcanol. 76:871. doi: 10.1007/s00445-014-0871-1
Stimac, J. A., Goff, F., Counce, D., Laroque, A. C. L., Hilton, D. R., and Morgenstern, U. (2004). The crater lake and hydrothermal system of Mount Pinatubo, Philippines: evolution in the decade after the eruption. Bull. Volcanol. 66, 149–167. doi: 10.1007/s00445-003-0300-3
Takano, B. (1987). Correlation of volcanic activity with sulfur oxyanion speciation in a crater lake. Science 235, 1633–1635. doi: 10.1126/science.235.4796.1633
Takano, B., Koshida, M., Fujiwara, Y., Sugimori, K., and Takayanagi, S. (1997). Influence of sulfur-oxidizing bacteria on the budget of sulfate in Yugama crater lake, Kusatsu-Shirane volcano, Japan. Biogeochemistry 38, 227–253. doi: 10.1023/A:1005805100834
Takano, B., Saitoh, H., and Takano, E. (1994). Geochemical implications of subaqueous molten sulfur at Yugama crater lake, Kusatsu-Shirane volcano, Japan. Geochem. J. 28, 199–216. doi: 10.2343/geochemj.28.199
Taran, Y., and Giggenbach, W. A. (2003). “Geochemistry of light hydrocarbons in subduction-related volcanic and hydrothermal fluids,” in Volcanic, Geothermal, and Ore-Forming Fluids: Rulers and Witnesses of Processes Within the Earth, Vol. 10, eds S. F. Simmons and I. Graham (Society of Economic Geologists- Special Publication), 61–74. Available online at: https://www.segweb.org/store/detail.aspx?id=SP10
Keywords: sulfur viscosity, impure sulfur, explosive eruptions, geothermal systems, volcanic lakes
Citation: Scolamacchia T and Cronin SJ (2016) Idiosyncrasies of Volcanic Sulfur Viscosity and the Triggering of Unheralded Volcanic Eruptions. Front. Earth Sci. 4:24. doi: 10.3389/feart.2016.00024
Received: 29 July 2015; Accepted: 23 February 2016;
Published: 15 March 2016.
Edited by:
Luis E. Lara, Servicio Nacional de Geología y Minería, ChileReviewed by:
Eisuke Fujita, National Research Institute for Earth Science and Disaster Prevention, JapanPierre Delmelle, Université Catholique de Louvain, Belgium
Copyright © 2016 Scolamacchia and Cronin. This is an open-access article distributed under the terms of the Creative Commons Attribution License (CC BY). The use, distribution or reproduction in other forums is permitted, provided the original author(s) or licensor are credited and that the original publication in this journal is cited, in accordance with accepted academic practice. No use, distribution or reproduction is permitted which does not comply with these terms.
*Correspondence: Teresa Scolamacchia, dHNjb2xhbWFjY2hpYUBnbWFpbC5jb20=