- Department of Glaciology and Climate, Geological Survey of Denmark and Greenland, Copenhagen, Denmark
Thirty-four experts took part in a survey of the most important and challenging topics in the field of meltwater retention in snow and firn, to reveal those topics that present the largest potential for scientific advancement. The most important and challenging topic to the expert panel is spatial heterogeneity of percolation, both in measurement and model studies. Studying percolation blocking by ice layering, particularly in modeling, also provides large potential for science advancement, as well as hydraulic conductivity and capillary forces in snow/firn. Model studies can benefit from improved initialization, and improved calculation of accumulation and liquid water at the surface. Firn coring should be performed more often, though we argue that also data that are relatively simple to collect, but of great importance to retention such as surface accumulation, density and temperature, are too sparse due to the high logistical expenses involved in field campaigns. Generally speaking, retention changes are expected to be of importance to the climatic (surface) mass balance and thus ice loss in coming decades, more so for Greenland than Antarctica or ice masses elsewhere.
Introduction
The mass balances of ice sheets, ice caps, and glaciers worldwide include one large mass input, namely snow accumulation. Yet depending on the location, several large mass loss components exist such as ice-dynamic mass transfer to the oceans, basal melting, surface meltwater runoff, and sublimation. Globally, the loss components are found to out-compete the gains in the current climate, which is largely attributed to increased melting at the air-ice and ocean-ice interfaces, also impacting ice dynamic discharge (IPCC, 2013).
Surface runoff occurs when melting, rainfall, and condensation exceed evaporation and retention in snow, firn, porous ice, and supraglacial lakes (Cuffey and Paterson, 2010). Our ability to determine surface runoff thus depends on our ability to determine several entirely independent processes, each with their own complexities. Out of these processes, retention of water in snow, firn or ice may not be the largest contributor averaged over an entire glacier, ice cap or ice sheet, yet regionally (in the percolation area) it can be large enough to accommodate all liquid water that percolates into the surface layer (e.g., Benson, 1962). At lower elevations, the snow and firn (or even porous ice) periodically gets overwhelmed by liquid water in the runoff area. Challenges in determining runoff are largest due to the uncertainties in quantifying retention in snow and firn, as this is the only component that takes place at depth (in the order of meters), thus out of sight and reach of basic, direct observational methods (Harper et al., 2012). Indirect methods have existed for decades, such as determining changes in density/stratigraphy from repeat snow pits (e.g., Techel and Pielmeier, 2011) or firn cores (e.g., Vallelonga et al., 2014), continuous subsurface temperature measurements for capturing the release of latent heat from refreezing (Humphrey et al., 2012; Charalampidis et al., 2016), or radar systems capable of identifying strong reflectors in porous snow or firn as ice layers (e.g., Koenig et al., 2016). Such methods are excellent for determining the location and quantity of the retained mass, but do not allow us to track and comprehend all physical processes involved in percolation and refreezing. This then limits our ability to model these processes with accuracy.
Whereas part of the difficulty in studying retention stems from not being able to track water movement through snow/firn with detail without altering the medium itself by digging pits or drilling cores, another aspect is the memory of the system. Namely, when meltwater refreezes it will impact future percolation due to changes in snow/firn density and temperature (Bezeau et al., 2013). This continues until the layer and the changes it caused in the snow/firn, migrated downward far enough to escape the direct influence of surface processes, i.e., the maximum percolation depth (Harper et al., 2012). Depending on the net accumulation rate (Mosley-Thompson et al., 2001), this can take many years. So if a model is forced by inaccurate surface energy quantities, or initialized with inaccurate snow/firn densities and temperatures, or struggles representing physical processes such as gravitational densification (Arthern et al., 2010; Morris and Wingham, 2014), thermal conduction (Sturm et al., 1997), and movement of liquid water (Colbeck, 1974; Hirashima et al., 2010), then not only the first retention event may be calculated inaccurately, but in a cascade effect all retention events may be affected. The impact on the climatic mass balance can be substantial for instance when consecutive high melt seasons occur, with ice layer formation shifting conditions in favor of surface runoff as opposed to percolation (Machguth et al., 2016).
In this paper, we aim to identify the most crucial topics in meltwater retention in order to reveal the largest potential for scientific advancement in this field. We apply expert elicitation, which is common practice in investigations with high uncertainty due to the lack of data, for instance in case of rare events or future predictions. In glaciology the expert survey methodology was applied e.g., in estimating future sea level contributions from the ice sheets (Bamber and Aspinall, 2013). Our approach differs in that we will not attempt quantification of meltwater retention in snow and firn through expert elicitation.
Methods
The questionnaire used to conduct the expert elicitation on meltwater retention in snow and firn, consisted of 18 entries listing snow/firn processes and properties (Table 1). On a scale of 1–10, 10 being highest, the expert was asked to rate each topic in terms of: (1) importance to meltwater retention, (2) difficulty level in measuring with accuracy, and (3) difficulty level in modeling with accuracy (providing ratings Rret1−3 for each topic/question). Also, the expert was asked to rate “the importance of the change in meltwater retention in snow and firn in a warming climate on decadal time scales for mass loss from” (1) Antarctica, (2) Greenland, and (3) other glaciated regions (ratings Rreg1−3). In case of substantial doubt the expert was asked not to provide an answer. In order to be able to assess the expert levels of the sources, the questionnaire also asked for the self-assessed (1) expert level on the topic of meltwater retention in snow and firn, (2) experience level performing firn measurements, (3) experience level modeling meltwater retention in snow and firn (ratings Rexp1−3), and (4) the years (Y) since (or until) obtaining a Ph.D. degree.
We invited those present at the “Workshop on observing and modeling meltwater retention processes in snow and firn on ice sheets and glaciers” hosted by the Geological Survey of Denmark and Greenland (GEUS) on 1–3 June 2016, and other known topical experts to take part in the survey. With the aim of publishing the results indicated on the questionnaire, all subjects gave their written consent by returning the document. The expert panel of 34 excludes the first author of this study. On average, the retention expert level is found to be 6.4 (Rexp1, av). Experience levels are lower for modeling of retention (Rexp2, av = 5.6), and particularly for measuring (Rexp3, av = 4.9), the latter of which is likely related to the high cost associated with field expeditions.
We apply expert weighting to each answer by multiplication with an individual expert factor, calculated as:
Note, that the self-assessed expert level and scientific seniority weigh equally in calculating one's expert level. Ratings Rret1 and Rreg1−3 are weighted using expert level rating Rexp1, ratings Rret2 using Rexp2, and ratings Rret3 using Rexp3. Expert factors vary between 0.29 and 2.18, a factor of 7.4 difference.
Likewise, we apply importance weighting by multiplication with the importance factor of each snow/firn topic:
where Rret1, EW is the expert-weighted importance averaged over all experts. Importance factors range between 0.67 and 1.26.
Using these methods, we obtain averages and expert-weighted (EW) averages for every surveyed snow/firn topic, in terms of their importance to meltwater retention, and their difficulty to quantify by means of measuring and modeling (Table 1). We also present expert- and importance-weighted (EIW) averages of the difficulties in measuring and modeling. Note, that after the weighting values can exceed the 1–10 range.
Results
The 34 experts attributed average values between 4.4 and 8.5 with an overall average of 6.7 to the importance of snow/firn topics (Table 1). Expert weighting has little impact on the average (6.8), nor on the ranking of the importance of processes/properties. Surface accumulation (8.6) and the availability of liquid water at the surface (8.4) are considered most important, followed by the spatial heterogeneity of meltwater percolation (7.9), percolation blocking by ice layering (7.7), and surface albedo through the melt-albedo feedback (7.3). Least important of the selected topics is considered to be near-surface ventilation by wind (4.6).
EW-values of the difficulty level in measuring the snow/firn processes/properties vary considerably (Table 1). Whereas measuring surface temperature (or in fact any surface variable) is considered relatively straightforward (3.3), measuring the spatial heterogeneity of meltwater percolation is judged to be the largest challenge (8.6). Other large challenges are found in measuring the effect of capillary forces on liquid water (8.2), hydraulic conductivity (8.1), ventilation by wind (7.8), and processes occurring in the bottom half of the firn (7.6).
Also in modeling, surface temperature is considered least difficult to determine with accuracy (4.5), and spatial heterogeneity most difficult (9.1, Table 1). The top five modeling challenges align well with the high ranking observational challenges, except that the modeling of percolation blocking by ice layering ranks second (8.5). On average, measuring and modeling retention-related topics (6.4) are judged to be equally complicated (6.3 vs. 6.4).
In Figure 1A, we identify four quadrants in a plot of importance vs. difficulty of the survey topics. Most topics are located in the quadrants of high importance and low difficulty, or low importance and high difficulty. Three topics are judged to be of above-average importance and difficulty: spatial heterogeneity in, and blocking of, meltwater percolation, and the hydraulic conductivity of the snow/firn.
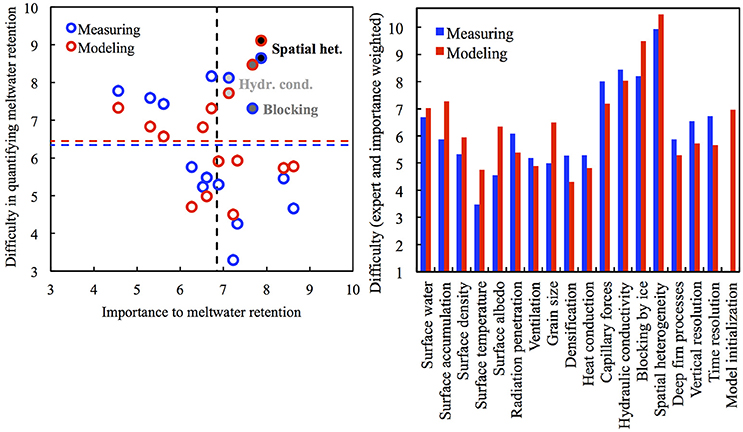
Figure 1. (A) The expert-weighted difficulty in quantifying aspect in observational (blue) and modeling (red) studies of meltwater retention in snow and firn, plotted against their expert-weighted importance. Dashed lines represent average values. (B) The expert- and importance-weighted difficulty in measuring (blue) and modeling (red) studies. Ratings were provided on a scale of 1–10.
EIW-values confirm that these three are top ranking both in measuring and modeling (Table 1, Figure 1B). Also the topic of capillary forces acting on liquid water ranks high (8.0 and 7.2). The top five in measuring is completed by temporal resolution, i.e., the difficulty in resolving relevant firn process cycles from repeat cores. In modeling, surface accumulation has a top-ranking EIW-value (7.3).
Finally, we turn to the question on how important meltwater retention in snow/firn is to the change in the mass balance of ice masses around the globe in coming decades (Rreg1−3). This expert judgment assessment indicates that, on these timescales, retention may be an important factor for ice loss from Antarctica (5.2), Greenland (8.4), and glaciated regions elsewhere (6.5).
Discussion
On some topics nearly all experts agree as shown by a small standard deviation (Table 1). For instance, all but one expert assess the modeling of meltwater percolation blocking by ice layers to be difficult (7–10), resulting in a small standard deviation of 1.3. In the other extreme, in judging the difficulty level of measuring surface accumulation density, a considerable disagreement is found with entries over the entire 1–10 range, resulting in a larger standard deviation of 2.7. To remedy the situation in which the less experienced potentially increase disagreement, we added weight to the opinions of those with more experience in the field of retention by calculating expert factors for all survey partakers, based on the expert's seniority level and self-assessed expert levels (see Methods Section). The calculation is arguably arbitrary, and therefore kept simple. However, we find that expert weighting does not significantly change the average topic score; expert weighting contributes between −0.16 and +0.71 with an average of +0.22 to the Rret questions. Therefore, the results are expert-level insensitive, because the survey part takers with a below-average expert level generally agree with their more experienced peers. The adjustment through importance weighting is larger with adjustment between −2.59 (snow/firn ventilation being difficult to measure, but not important for retention studies) and +1.49 (surface accumulation being somewhat difficult to model, but very important for retention studies), yet the average importance-adjustment is negligible at −0.04.
If a topic related to meltwater retention in snow/firn is considered to be both important and difficult to assess, we regard this as a topic in which the largest scientific advances can be made. These topics have an above-average EIW score (Table 1). Yet, we also need to consider the topics that are important, but less difficult to quantify. In retention modeling, these topics leave little room for advancement. On the other hand, in measuring, an additional challenge exists in reaching the often remote and inaccessible accumulation areas of glaciers and ice caps/sheets. So while the measurement may be relatively simple, observational data remain sparse due to high logistical expense. A data shortage is also reflected in the experts' opinion that relevant cycles in retention are not well-resolved by repeat firn coring (Table 1). Therefore, we argue that also the relatively simple, yet important, measurements should be targeted for scientific advancement in retention. Strikingly, these are all the measurements of surface variables listed in Table 1 (surface water, accumulation, density, temperature, and albedo) for which automated solutions exist or can be developed, such as equipping weather stations (e.g., Mölg et al., 2008; Van As et al., 2011) with radiometers, sonic rangers, and snow-water-equivalent sensors.
With sparsity of observational data, it becomes of primary importance that existing data are available to everyone. Excellent examples of publicly available databases containing firn data exist (e.g., the SUMup database; Koenig et al., 2016), and efforts should be made by the global glaciological community to expand those databases. Likewise, only few models are capable of detailed calculations of meltwater retention in snow and firn. The more the model codes become available to the entire research community, the more researchers can build on previous efforts, and the largest the scientific advancement will be.
Conclusion
In this study, we used the results of 34 experts that took part in an expert elicitation of meltwater retention in snow and firn, to identify those snow/firn processes and properties that are both important and challenging to quantify, thus providing the largest potential for scientific advancement. We applied expert- and importance-weighting, only the latter of which proved influential.
We find the following topics to present the largest potential for scientific advancement, both in measuring and modeling:
- spatial heterogeneity of meltwater percolation in snow/firn,
- blocking of percolating meltwater by ice layers, especially in model studies,
- hydraulic conductivity,
- and capillary forces acting on liquid water.
Additionally, in modeling the following topics are worthy of further emphasis:
- quantifying surface water,
- quantifying accumulation,
- and model initialization.
Based on the survey results, we also argue for:
- obtaining more firn cores and to sample them in more detail,
- and measuring surface variables (liquid water, accumulation, density, temperature, and albedo) at more sites, since data sparsity is not caused by the difficulty of the measurement itself, but by the difficulty in getting to the accumulation areas of glaciers and ice caps/sheets.
In a final question on the future change of retention due to climate change, the expert panel answered that in the coming decades, meltwater retention is expected to be of importance to mass loss in glaciated regions worldwide, especially in Greenland.
Author Contributions
DV, RF, and JB conceived the study. DV compiled the questionnaire, analyzed the data, and wrote the text with contributions by JB and RF.
Conflict of Interest Statement
The authors declare that the research was conducted in the absence of any commercial or financial relationships that could be construed as a potential conflict of interest.
Acknowledgments
We are grateful to the anonymous experts taking part in this study. Support for this study was provided by Denmark's Nature and Universe grant DFF 4002-00234 through the Retain project (retain.geus.dk) and the Danish Energy Agency (www.ENS.dk) through the Programme for Monitoring of the Greenland Ice Sheet (www.PROMICE.dk). This study with human subjects was carried out in accordance with the ethical guidelines by the Geological Survey of Denmark and Greenland (GEUS).
References
Arthern, R. J., Vaughan, D. G., Rankin, A. M., Mulvaney, R., and Thomas, E. R. (2010). In situ measurements of Antarctic snow compaction compared with predictions of models. J. Geophys. Res. 115:F03011. doi: 10.1029/2009JF001306
Bamber, J. L., and Aspinall, W. P. (2013). An expert judgement assessment of future sea level rise from the ice sheets. Nat. Clim. Change 3, 424–427. doi: 10.1038/nclimate1778
Benson, C. S. (1962). Stratigraphic Studies in the Snow and Firn of the Greenland Ice Sheet. Research Report 70, SIPRE.
Bezeau, P., Sharp, M., Burgess, D., and Gascon, G. (2013). Firn profile changes in response to extreme 21st-century melting at Devon Ice Cap, Nunavut, Canada. J. Glaciol. 59, 981–991. doi: 10.3189/2013JoG12J208
Charalampidis, C., Van As, D., Colgan, W. T., Fausto, R. S., MacFerrin, M., and Machguth, H. (2016). Thermal tracing of retained meltwater in the lower accumulation area of the southwestern Greenland ice sheet. Ann. Glaciol. 57. doi: 10.1017/aog.2016.2. Available online at: https://www.igsoc.org/annals/57/72/accepted.html
Colbeck, S. C. (1974). The capillary effects on water percolation in homogeneous snow. J. Glaciol. 13, 85–97.
Cuffey, K. M., and Paterson, W. S. B. (2010). The Physics of Glaciers. Burlington, MA: Academic Press, 704.
Harper, J., Humphrey, N., Pfeffer, W. T., Brown, J., and Fettweis, X. (2012). Greenland ice-sheet contribution to sea-level rise buffered by meltwater storage in firn. Nature 491, 240–243. doi: 10.1038/nature11566
Hirashima, H., Yamaguchi, S., Sato, A., and Lehning, M. (2010). Numerical modeling of liquid water movement through layered snow based on new measurements of the water retention curve. Cold Regions Sci. Technol. 64, 94–103. doi: 10.1016/j.coldregions.2010.09.003
Humphrey, N. F., Harper, J. T., and Pfeffer, W. T. (2012). Thermal tracking of meltwater retention in Greenland's accumulation area. J. Geophys. Res. 117:F01010. doi: 10.1029/2011JF002083
IPCC (2013). “Climate change 2013: the physical science basis,” in Contribution of Working Group I to the Fifth Assessment Report of the Intergovernmental Panel on Climate Change, eds T. F. Stocker, D. Qin, G.-K. Plattner, M. Tignor, S. K. Allen, J. Boschung, A. Nauels, Y. Xia, V. Bex, and P. M. Midgley (Cambridge; New York, NY: Cambridge University Press), 1535.
Koenig, L. S., Ivanoff, A., Alexander, P. M., MacGregor, J. A., Fettweis, X., Panzer, B., et al. (2016). Annual Greenland accumulation rates (2009–2012) from airborne snow radar. Cryosphere 10, 1739–1752. doi: 10.5194/tc-10-1739-2016
Machguth, H., MacFerrin, M., Van As, D., Box, J. E., Charalampidis, C., Colgan, W., et al. (2016). Greenland meltwater storage in firn limited by near-surface ice formation. Nat. Clim. Change 6, 390–393. doi: 10.1038/nclimate2899
Mölg, T., Cullen, N. J., Hardy, D. R., Kaser, G., and Klok, L. (2008). Mass balance of a slope glacier on Kilimanjaro and its sensitivity to climate. Int. J. Climatol. 28, 881–892. doi: 10.1002/joc.1589
Morris, E. M., and Wingham, D. J. (2014). Densification of polar snow: measurements, modeling, and implications for altimetry. J. Geophys. Res. 119, 349–365. doi: 10.1002/2013JF002898
Mosley-Thompson, E., McConnell, J. R., Bales, R. C., Lin, P. N., Steffen, K., Thompson, L. G., et al. (2001). Local to regional-scale variability of annual net accumulation on the Greenland ice sheet from PARCA cores. J. Geophys. Res. 106, 33839–33851. doi: 10.1029/2001JD900067
Sturm, M., Holmgren, J., Konig, M., and Morris, K. (1997). The thermal conductivity of seasonal snow. J. Glaciol. 43, 26–41.
Techel, F., and Pielmeier, C. (2011). Point observations of liquid water content in wet snow–investigating methodical, spatial and temporal aspects. Cryosphere 5, 405–418. doi: 10.5194/tc-5-405-2011
Vallelonga, P., Christianson, K., Alley, R. B., Anandakrishnan, S., Christian, J. E. M., Dahl-Jensen, D., et al. (2014). Initial results from geophysical surveys and shallow coring of the Northeast Greenland Ice Stream (NEGIS). Cryosphere 8, 1275–1287. doi: 10.5194/tc-8-1275-2014
Keywords: snow, firn, melt, percolation, retention, refreezing, spatial heterogeneity, ice layer blocking
Citation: van As D, Box JE and Fausto RS (2016) Challenges of Quantifying Meltwater Retention in Snow and Firn: An Expert Elicitation. Front. Earth Sci. 4:101. doi: 10.3389/feart.2016.00101
Received: 20 September 2016; Accepted: 09 November 2016;
Published: 29 November 2016.
Edited by:
Martin Hoelzle, University of Fribourg, SwitzerlandReviewed by:
Shad O'Neel, USGS Alaska Science Center, USAChristoph Schneider, Humboldt University of Berlin, Germany
Copyright © 2016 van As, Box and Fausto. This is an open-access article distributed under the terms of the Creative Commons Attribution License (CC BY). The use, distribution or reproduction in other forums is permitted, provided the original author(s) or licensor are credited and that the original publication in this journal is cited, in accordance with accepted academic practice. No use, distribution or reproduction is permitted which does not comply with these terms.
*Correspondence: Dirk van As, ZHZhQGdldXMuZGs=