- 1Laboratório de Paleontologia da Universidade Luterana do Brasil, Torres, Brazil
- 2Museu de Ciências Naturais da Fundação Zoobotânica do Rio Grande do Sul, Porto Alegre, Brazil
- 3Independent Researcher, Aleja Najświętszej Maryi Panny 20/20A, Częstochowa, Poland
- 4Facultad de Ciencias, Instituto de Ciencias Geológicas, Montevideo, Uruguay
Mesosauridae comprises the oldest known aquatic amniotes which lived in Gondwana during the Early Permian. Previous work in the Uruguayan mesosaur-bearing Mangrullo Formation suggested that mesosaurids lived in an inland water body, inferred as moderately hypersaline, with exceptional preservational conditions that justified describing these strata as a Fossil-Lagerstätte. Exquisitely preserved articulated mesosaur skeletons, including gastric content and associated coprolites, from the Brazilian Iratí Formation in the State of Goiás (central-western Brazil) indicate excellent conditions of preservation, extending the Konservat-Lagerstätte designation to both units in the Paraná Basin. The near-absence of more resistant fossil remains, like actinopterygian and temnospondyl bones, demonstrates the faunistic poverty of the mesosaur-bearing “salty sea.” Our studies of the alimentary habits of mesosaurids through the use of stereoscopic microscopy, light and electronic microscopy, and X-ray diffractometry suggest that the diet of mesosaurids was predominantly composed of pygocephalomorph crustaceans (possibly not exceeding 20 mm in length). However, the presence of bones and bone fragments of small mesosaurs in the gastric content, cololites, coprolites, and possible regurgitalites may also indicate cannibalistic and/or scavenging habits. Cannibalism is relatively common among vertebrates, particularly during conditions of environmental stress, like food shortage. Likewise, the apparent abundance of pygocephalomorph crustacean fossils in the Iratí and Mangrullo Formations, outside and within the studied gastric, cololite, and coprolite contents, might have to do with environmental stress possibly caused by volcanic activity, in particular ash spread into the basin during the Early Permian. In this context, casual necrophagy on the dead bodies of small mesosaurs and large pygocephalomorphs might have been an alternative alimentary behavior adopted for survival in mesosaurs.
Introduction
Fossilized feces, gastric contents, and regurgitations (“bromalites,” sensu Hunt et al., 1994; see also Hunt and Lucas, 2012) can tell us a lot about the dietary behavior of extinct organisms (Chin and Kirkland, 1998; Chin et al., 2008; Bajdek et al., 2014; Qvarnström et al., 2016). They mostly fossilize in situ (Hu et al., 2010; Bajdek, 2013), as isolated coprolites (Eriksson et al., 2011; Dentzien-Dias et al., 2012; Niedźwiedzki et al., 2016) or associated with the producer's skeleton (Hone et al., 2015; Wang et al., 2016). However, the latter is a far less frequent situation and the coprolite producers are usually very hard or impossible to identify. The mesosaur coprolites described herein represent one of the rare cases where the producer can be identified with confidence, because no other tetrapod or vertebrate is found in the mesosaur-bearing strata (Piñeiro, 2006; Piñeiro et al., 2012b). Also, the present study represents an exceptional case where coprolites, gastric contents, cololites, and regurgitalites of a single animal species, i.e., all the “basic” bromalite types, are described. Lower Permian vertebrate coprolites have been briefly described in several papers (e.g., Hunt and Lucas, 2005a,b; Hunt et al., 2005a,b; Shelton, 2013), but more detailed studies were until now restricted to Middle/Upper Permian materials (Smith and Botha-Brink, 2011; Dentzien-Dias et al., 2012, 2013; Owocki et al., 2012; Bajdek et al., 2016; Niedźwiedzki et al., 2016).
Mesosauridae comprises the oldest known aquatic reptiles which lived in Gondwana during the Early Permian. Most authors agree that Mesosauridae are basal sauropsids (e.g., Laurin and Reisz, 1995; Piñeiro et al., 2016) or basal parareptiles (Modesto, 1999; Tsuji and Müller, 2009), including three monotypic species: Mesosaurus tenuidens, Stereosternum tumidum, and Brazilosaurus sanpauloensis. All three species are found in Brazil, whereas only M. tenuidens has been reported from Uruguay (Piñeiro, 2004; Morosi, 2011; Piñeiro et al., 2012a). Nevertheless, recent studies would suggest the presence of not more than two species in the Paraná Basin (Piñeiro, 2004, 2006; Piñeiro et al., 2015a, 2016), and the taxonomic diversity of the mesosaurids from the African Karoo Basin remains uncertain (Piñeiro et al., 2015a).
Previously, the diet of mesosaurs was only inferred from indirect evidence. MacGregor (1908) suggested that mesosaurids preyed on fish because of their long snout and very peculiar tooth morphology. Romer (1966) and Carroll (1982) followed Frech's (1897–1902) suggestion that the long and horizontalized teeth of mesosaurs could indicate a sludge filter-type habit. Later, Oelofsen and Aráujo (1983), based on the distribution of the fauna in the “Iratí-Sea,” proposed that Stereosternum and Brazilosaurus, inhabitants of shallow waters, had a crustacean-based diet (e.g., Liocaris). Araújo (1976), Araújo-Barberena (1994), and Carroll (1988) followed the proposition that Stereosternum and Brazilosaurus were predators. Piñeiro (2002, 2004, 2006) questioned the hypothesis of MacGregor (1908) because of the absence of “fishes” in the mesosaur-bearing strata of the Lower Permian Mangrullo Formation of Uruguay and probably in the correlative Brazilian Iratí and South African Whitehill formations (Piñeiro, 2002, 2004). However, according to some Brazilian paleontologists, additional biostratigraphic studies will be needed to confirm this (Marina Bento-Soares, personal communication). Accordingly, Modesto (1996, 2006) considered that invertebrates of medium or large size would be preferred prey items of mesosaurs. In Uruguay, it is clear that isolated actinopterygian, acanthodian and coelacanthid scales, teeth and bones are present in levels that overlie and underlie those containing mesosaurs (see also Piñeiro et al., 2012b). Some bromalites, very probably produced by “fishes,” are found in the layers dominated by actinopterygian, coelacanthid, and acanthodian remains (Piñeiro, 2002, 2008; Piñeiro et al., 2012b). They are three-dimensional, sub-spherical structures containing tiny fragments of thick ganoid scales; despite their poor preservation, they are clearly spiral. Thus, because of their very different morphology with respect to the mesosaur coprolites, they are good stratigraphic markers in incompletely preserved sections of the Mangrullo Formation that do not contain mesosaur remains (but see critical notes on coprostratigraphy in Bajdek et al., 2014; Niedźwiedzki et al., 2016).
Recently, Pretto et al. (2014), based on histological studies of the tooth microstructure in Stereosternum tumidum, reported the presence of a tripartite alveolar periodontium that increased the resistance of the teeth against bite forces; they used this evidence to suggest that mesosaurids could have been predatory animals, feeding on crustaceans and juvenile mesosaurids.
The first direct evidence about mesosaurid dietary preferences was provided by Raimundo-Silva et al. (1997) and Raimundo-Silva (1999) through the study of an almost complete specimen assigned to Brazilosaurus sanpauloensis which preserves a cololite and some associated coprolites. Moreover, several isolated, well-preserved coprolites that could belong to the same species were also examined. Later, Piñeiro et al. (2012b) reported the discovery of similar coprolites from the Mangrullo Formation preserved in isolation or associated with adults, and also gastric contents in specimens assigned to Mesosaurus tenuidens. The material in both the gastric contents and the coprolites was identified as carapaces and abdomens of pygocephalomorph crustaceans, in some cases mixed with bones of small mesosaurids and putative plant remains. Recently, Ramos (2015) analyzed the coprolite and gastric contents from the Mangrullo Formation of Uruguay, concluding that mesosaurids could have been selective predators which fed on prey items smaller than 20 mm in length and that fragmentary, small mesosaur bones might have been accidentally ingested while catching pygocephalomorphs—taking into account the evidence presented therein (and in this contribution) that crustaceans could have been scavengers of mesosaur carcasses.
This paper aims to present an updated overview of the existing knowledge about the alimentary habits of Mesosauridae. Gastric contents, cololites, coprolites, and regurgitalites from the Lower Permian Iratí (Brazil) and Mangrullo (Uruguay) formations are described in detail. The trophic and taphonomic differences between these two paleoenvironments in the Paraná Basin and the mesosaurid species Brazilosaurus sanpauloensis and Mesosaurus tenuidens are discussed.
Materials and Methods
Material
The materials used in this study come from Lower Permian deposits from Brazil (Iratí Formation) and Uruguay (Mangrullo Formation; Figure 1). The specimens from the Mangrullo Formation were found at the Paso del Cuello locality, Tacuarembó County, northern Uruguay, and are housed at the Vertebrate Collection of the Facultad de Ciencias (FC-DPV), Universidad de la República Oriental del Uruguay (Figures 1A–C,E–G), while the samples from the Iratí Formation were collected from outcrops in the municipality of Perolândia (Goiás State, Brazil) and are deposited in the Museu de Ciências Naturais of the Fundação Zoobotânica do Rio Grande do Sul, Paleovertebrate Collection (MCN-PV; Figures 1D,H,I). The specimens (Figures 2–9) consist of: (1) partially articulated mesosaurid individuals preserving gastric and gut contents, plus associated coprolites (Figure 2), (2) partially articulated individuals preserving only gastric contents (Figure 6), (3) isolated coprolites (Figures 3,4,7,8), and (4) regurgitalites (Figure 9). Some other mesosaurid materials from São Paulo State, belonging to the fossil collection of the Instituto de Geociências, Universidade de São Paulo, Brazil (GP-2E) were also studied by GP. The complete list of the examined 136 specimens is provided as Supplementary Information. Skeletons from Brazil have been mostly preserved as original bones, in place or slightly displaced. Partial skeletons from the Mangrullo Formation are mostly preserved as molds.
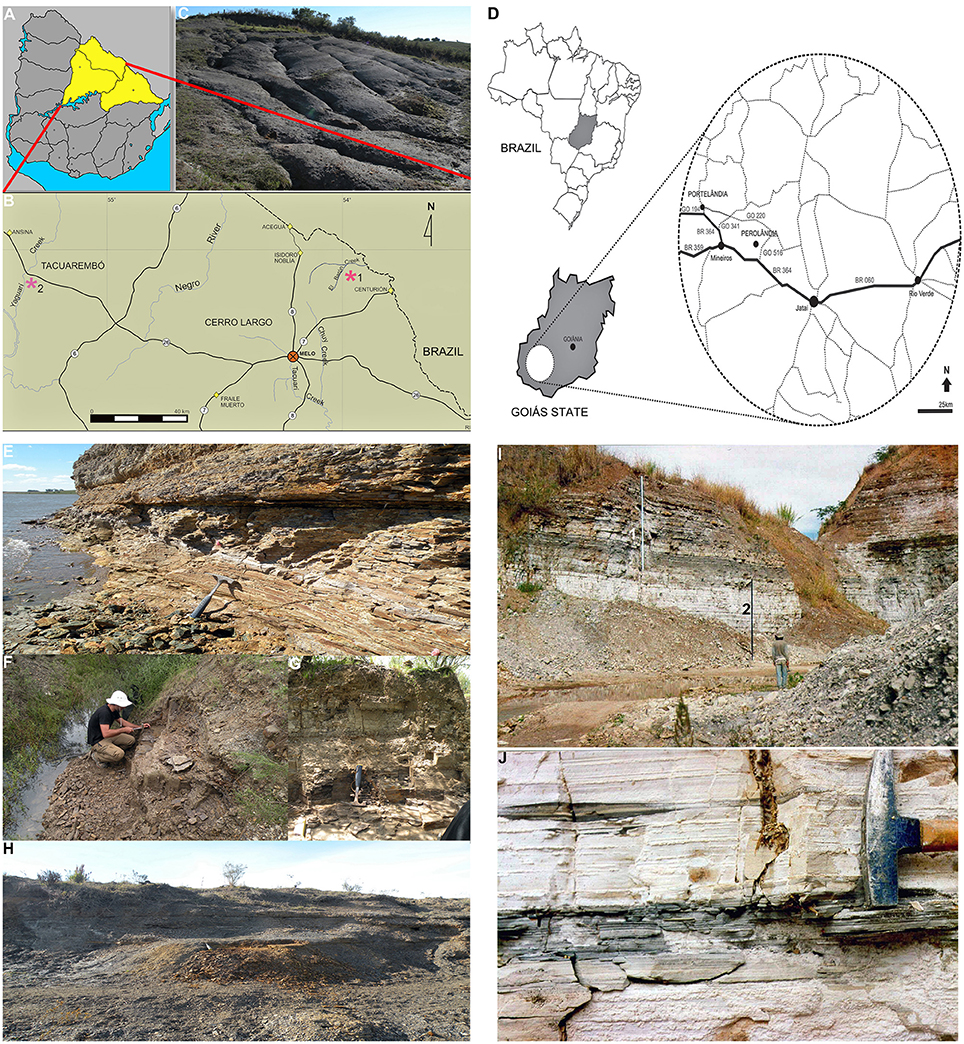
Figure 1. Geographic location of the Uruguayan Mangrullo and the Brazilian Iratí Lower Permian Konservat-Lagerstätten, and principal outcrops. (A) Map of Uruguay showing the area of outcrops of the Mangrullo Formation; (B) Main localities where the studied Uruguayan specimens were found; (C) Photograph of the Mangrullo Formation section at the El Barón locality; (D) Geographic location of outcrops of the Early Permian Iratí Formation that provided the studied specimens from Brazil; (E) Lithostratigraphic section at the El Barón locality (Cerro Largo County, Uruguay) showing the basalmost shales where mesosaurid, pygocephalomorph, and plant remains have appeared; (F) Limestones and siltstones at the Arroyo Yaguarí locality (Tacuarembó County, Uruguay) which yielded mesosaurid and pygocephalomorph specimens as the most frequent association; (G) Detail of the profile depicted in (F); (H) Bituminous shales at the El Barón locality (Cerro Largo County) intercalated by several bentonitic layers and levels of gypsum crystals; (I) General view of the litostratigraphic section at the SUCAL quarry (Perolândia, Goiás State, Brazil), which consists of limestones intercalated with shales at the middle and the top (1) and yellowish limestones at the base (2); (J) Detail of the basal limestone showing the gray, fossiliferous levels in Goiás. All the localities shown have yielded the mesosaur coprolites described herein but only Arroyo Yaguarí has provided specimens preserving gastric contents.
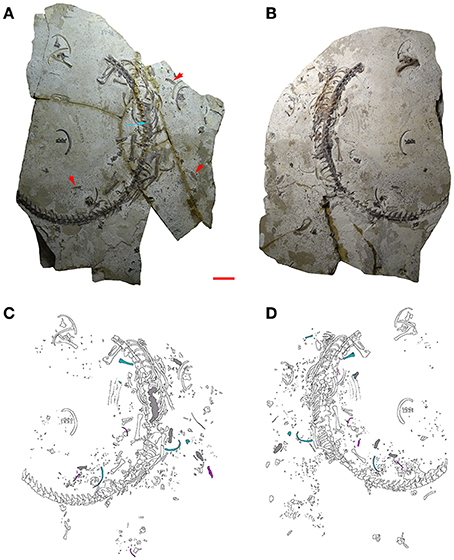
Figure 2. MCN-PV 2254. Part (A) and counterpart (B) of a mesosaur specimen assigned to the species Brazilosaurus sanpauloensis from Goiás State. Red arrows point to the small coprolites placed around the articulated skeleton. Blue arrow shows the location of the cololite. (C,D) Interpretive drawings of (A,B), respectively. Isolated bones from immature individuals of different ontogenetic stages associated with the adult are in blue and pink; cololite and coprolites are in gray. Scale bar: 10 mm.
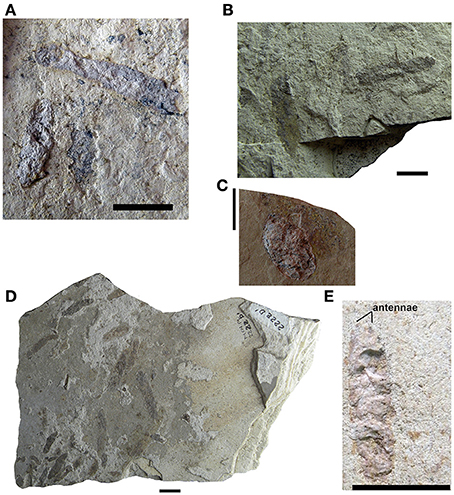
Figure 3. Mesosaur coprolites from the Iratí Formation (Lower Permian of Brazil). (A–C) (MCN-PV 2220, 2210, 2231), isolated coprolites showing the different morphologies found. (D) MCN-PV 2222, coprolite assemblage containing specimens of different sizes. (E) MCN-PV 2104. Coprolite from the Iratí Formation (Lower Permian, Brazil) showing a general morphology that mirrors that of a pygocephalomorph crustacean. This specimen seems to have preserved part of the bases of the antennae. Scale bars: 10 mm.
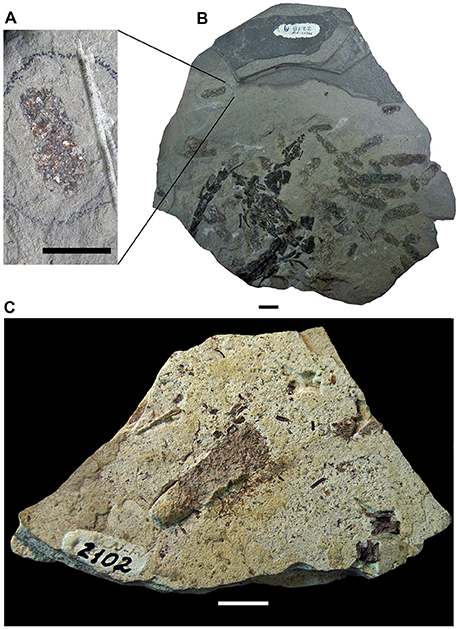
Figure 4. Mesosaur coprolites from the Iratí Formation (Lower Permian of Brazil). (A) Small coprolite showing bone inclusions which is part of an assemblage (MCN-PV 2218) of coprolites that otherwise lack such inclusions (B). (C) Specimen MCN-PV 2102 clearly showing that its original three-dimensional shape has been weathered and its contents dispersed. Scale bars: 10 mm.
All identifiable skeletal remains from Brazil here studied were attributed to Brazilosaurus sanpauloensis by Raimundo-Silva et al. (1997) and Raimundo-Silva (1999) based on the criteria established by Oelofsen and Aráujo (1983); Oelofsen and Araújo (1987): adults developing less pachyostotic ribs, and hemal arches which are U-shaped due to their marked pachyostosis. However, these diagnostic characters turned out not to be useful, considering that the ribs are in fact wider than the radius, ulna, tibia, or fibula in specimens assigned to Brazilosaurus, and that U-shaped hemal arches can be present along with V-shaped ones in the tail of a single individual (see Piñeiro et al., 2015a for a revision of the Brazilosaurus status and general mesosaurid diversity). Most of the materials were collected ex-situ in abandoned and active quarries, when rock debris and blocks formed as the result of mining activities. There are also specimens that were collected in situ. The materials collected from the debris of quarries often represent single blocks fragmented into several parts, which are here referred to as portions A, B, C of the same specimen. Moreover, most of the studied specimens have split into part and counterpart. They consist of two complementary plates that will be referred to as A/A′, B/B′ and so on. For instance, in MCN-PV 2254, a partially articulated adult individual, part, and counterpart (MCN-PV 2254 A and A′; Figure 2) are differentiated in that one (the part) shows bones and the other (the counterpart) just molds. Other specimens consist of molds in both slabs due to loss by weathering.
Methods
The isolated bones, and the dispersed material around the adult individuals preserving gut contents, were measured and compared to the bones of the specimens studied by Sedor (1994) and Piñeiro et al. (2016) in order to determine their relative age and the minimum number of juvenile individuals associated with the adults in each of the samples (from Brazil and from Uruguay).
Determination of the crustacean species in the gut contents and inside the coprolites was difficult because of their weathering and high degree of fragmentation, but they might be juvenile pygaspids (Dr. Irajá Damiani Pinto, personal communication).
The methods for a more profound study of the gut and coprolite contents were restricted to scanning electron microscopy and the X-ray diffraction. Further, both gut and coprolite contents were compared under normal light microscopy, stereoscopic microscopy, and scanning electron microscopy. Thin sections were made at the laboratory of the Instituto de Geociências, Universidade Federal do Rio Grande do Sul (UFRGS), and the Paleontological Laboratory at the Museu de Ciências e Tecnologia, Pontifícia Universidade do Rio Grande do Sul (PUCRS) and photographed under a Zeiss microscope, at magnifications between 80 and 500 times. The thin sections were made following standard histological techniques (e.g., Ferigolo, 1985).
For study under scanning electron microscopy, cololite/coprolite contents were removed from the slabs using electronic drills, dental probes, stylets, and tweezers. Some samples were etched in 5% hydrochloric acid for 10 min, whereas others were treated with phosphoric acid for a variable time. After this, the samples were placed in an oven at 60°C for 24 h, in order to remove any moisture. The samples were glued on stubs with silver paste, metalized with 24 carat gold, and examined and photographed under a Jeol Scanning Electron Microscope (SEM), JSM-5200, under 25 kV with variable magnification. Other samples, mostly those specimens (coprolites and gastric contents) from the Uruguayan Mangrullo Formation, were also analyzed under SEM, but they remained uncoated.
All measurements are given in millimeters. The measurements taken were anteroposterior length and dorsoventral diameter of the cololites, and minimum and maximum diameter of the coprolites. They were taken using a Mitutoyo caliper with a span of 15 cm opening and accuracy of 0.05 mm. Bones were measured following Sedor (1994) and fragmented bones were measured by combining the measurements taken from parts and counterparts (A, A′).
Results
MCN-PV 2254 (Figure 2)
MCN-PV 2254: Represented Mesosaur Individuals
Inside and around the adult individual MCN-PV 2254, indeed in almost every area of both slabs (A, A′), there is a large number of small crustacean fragments, bones, and bone fragments, all of them disarticulated and mostly badly preserved, maybe because they had already suffered different degrees of digestion. Thus, the very fragmentary elements could be part of the gastric or gut content of the adult individual but they also could have come from degradation of the coprolites preserved around the skeleton (Figure 2). About 25 bones/bone fragments and various teeth were identified as belonging to mesosaurs; others could not be identified because they are too small, and still poorly ontogenetically differentiated. Concerning crustacean remains, the degree of fragmentation does not allow identification, but most are probably pygocephalomorphs, the only crustacean group described thus far from the Iratí Formation.
Morphological and metric comparison among the bones associated with the adult individual allows us to infer the presence of at least two juvenile stages. These bones do not show the degree of erosion expected for bones originally retained in the stomach or the intestinal tract. Other tiny bones scattered around the adult skeleton, possibly from hatchling mesosaurs, seem not be related to the previously mentioned juvenile individuals due to fractures, missing parts, or excessive weathering because of their immaturity. These remains might represent elements formerly retained in the gastrointestinal tract of the adult mesosaur (see Figure 2).
MCN-PV 2254: Macroscopic Description of the Cololite
A macroscopic analysis of the cololite in MCN-PV 2254, which is associated with the vertebral column of the adult individual, shows that when the vertebral column is hyperextended, the cololite lies next to the vertebrae from the anterior portion of the 10th dorsal vertebra (D10) until the third caudal vertebra (3 Cd; see Figure 2). Three distinct types of bromalite preservation are seen in the specimen. First, part of the last meal is still contained in a mass in the intestinal tube (i.e., a cololite). Some remnants of the last meal are oval, reddish-brown, and of a slightly lighter shade than the bones of the adult mesosaur. The cololite lies between the medial surfaces of the right and left series of ribs and ventral to the vertebral column, indicating an intra-abdominal position. Second, numerous fragmented and weathered small bones and carapaces, representing part of the gastric contents, are dispersed around the skeleton of the adult. They lie close to the vertebral column, spanning the last dorsal and the sacral segments. Third, at least five coprolites are preserved close to the adult individual, here interpreted as the most probable producer (see Figures 2C,D).
MCN-PV 2254: Cololite Studied under Stereoscopic Microscopy
The cololite in specimen MCN-PV 2254 was studied under a stereoscopic microscope. A lot of crustacean fragments disrupted by the digestive processes can be seen inside the content, along with several small fragments of bones (Figure 5B). We interpret these fragmentary carapaces and bones as belonging to small pygocephalomorph crustaceans and very young mesosaurids respectively, but because they appear to be partially digested, no more precise taxonomic identification can be made. Nevertheless, one fragment of bone was removed for study under scanning electron microscopy.
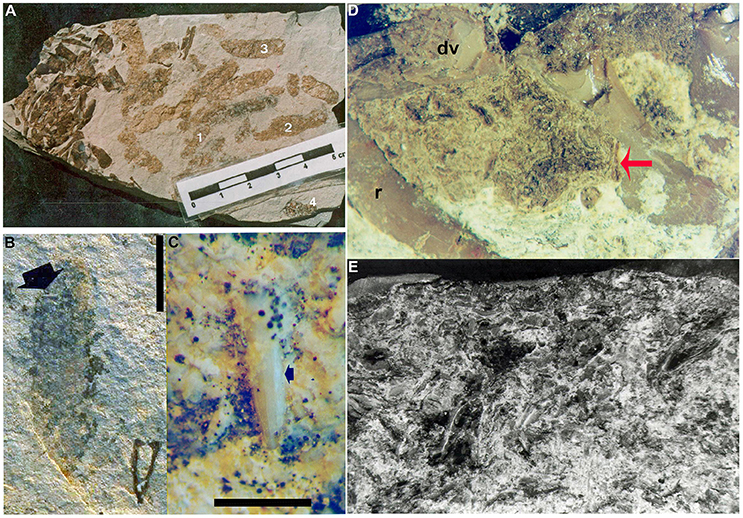
Figure 5. Mesosaur coprolites and cololites from the Lower Permian Iratí Formation: morphology and structure. (A) MCN-PV 2218 showing the different coprolite types (see text for a more detailed description). (B) MCN-PV 2220, type-4 coprolite bearing a very small tooth (arrow). (C) Detail showing the good preservation of the tooth included in FC-DPV 2220, even after the digestive process. Scale bars: 10 mm in (B) and 5 mm in (C). (D) Photograph of the cololite in MCN-PV 2254 to show the presence of bone inclusions (red arrow) with different degrees of preservation. (E) SEM image of the same cololite illustrated in (D), showing crustacean remains as part of its content. r, ribs; dv, dorsal vertebrae of the adult individual bearing the studied cololite.
MCN-PV 2254: Cololite Studied under Scanning Electron Microscopy (SEM)
Examination of the cololite under SEM has shown the crustacean fragments to be elongated bodies, twisted or very deformed, very homogeneous microscopically and without structure, except that multiple layers of exoskeleton are visible in some cases (Figure 5E). They also contain bones that appear to be not as weathered as the crustaceans. One bone fragment was separated from the cololite of MCN-PV 2254 for SEM study. It was identified as a bone fragment on the basis of its typical bone tissue microstructure, meaning concentric layers around vascular canals, and probable osteocyte vacuities.
X-ray diffraction
Of the analyzed cololite samples from MCN-PV 2254, only one showed peaks indicating a pure apatite composition; the others showed apatite and dolomite or apatite, dolomite and quartz. Apatite indicates the presence of bone debris in the cololite, since this mineral is not present in the dolomitic limestone that contains the specimen. It was also present in vertebral fragments of the skeleton of MCN-PV 2254, which were analyzed for comparison. The dolomite and quartz may be present as a result of fossilization processes and contamination of samples, since it is very difficult to remove cololite fragments without taking along small portions of the surrounding rock. However, the fossilized fecal matter often contains a few quartz grains and other mineral clasts (i.e., gastroliths) which may have been swallowed deliberately or incidentally with food and water, as exemplified by analyses of crocodile stomach contents (Pauwels et al., 2007; Wings, 2007).
The crystallographic parameters “a” and “c” (Tables 1, 2) suggest that the apatite present in the cololite of MCN-PV 2254 is virtually identical to that found in bone. The minimal and insignificant differences in chemical composition that exist are explained by recrystallization of apatite around the end of void-fillings (zeolitic channels) and alteration by factors such as pH, temperature, and exposure to chemicals, all resulting from digestive processes. The analysis did not find a difference between the carbonate of the dolomitic limestone and that found in the crustacean shells. This suggests that a diagenetic precipitation of calcium carbonate took place, and that the crustacean carapaces were not originally mineralized (Piñeiro et al., 2012c).
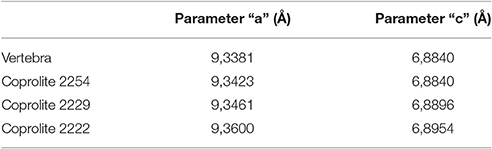
Table 2. Values of crystallographic parameters “a” and “c” for the apatite in the vertebra of MCN-PV 2254 and the coprolites found in the samples.
MCN-PV 2254: Coprolites
Several ellipsoid coprolites can be seen around the adult specimen. Their shape and appearance are similar to those of other mesosaur coprolites (Figure 2).
X-ray diffraction
Coprolites were analyzed using X-ray diffraction. The results obtained were very similar to those for the cololite. The sample of MCN-PV 2254 only presented apatite in contrast with other specimens (e.g., MCN-PV 2222 and 2229) which showed apatite, dolomite, and quartz.
The crystallographic parameters “a” and “c” (Table 2) indicate that the apatite type in the cololite is similar to that found in the sampled vertebrae of MCN-PV 2254 and that the small differences are due to the digestive process. As its contents show, the cololite is the product of partial digestion, whereas coprolites represent the end of the digestive process. For the carbonate we propose the same explanation given in the case of the cololite.
Study of Isolated Coprolites from the Iratí Formation (Figures 3–5)
Most of the specimens have an elongate ellipsoid, oval, or slightly curved shape (see Figures 3A,B,D,E, 5A). The specimen MCN-PV 2226 has a more rounded shape (Figure 3C). As a rule, coprolites have a reddish-brown color, and most of the exceptions have a lighter shade (Figure 4). Some, possibly due to diagenesis, have a slightly bluish (e.g., MCN-PV 2212 A/A′; MCN-PV 2220 A/A′) or brown-black hue (e.g., MCN-PV 2208, MCN-PV 2218 A/A′/B) and some show small bone inclusions (Figure 4A).
The coprolites are usually dispersed, or sometimes agglomerated, in slightly different planes. In the specimens in which there are a larger number of coprolites most are more or less parallel to each other, while some are oriented obliquely to the others (Figures 3D, 5A). Some specimens show coprolites overlapping each other (Figure 4B).
Some coprolites are complete (e.g., MCN-PV 2158 A/A′; MCN-PV 2254 A/A′), but some are broken and incomplete (MCN-PV 2158; MCN-PV 2210 A/A′; MCN-PV 2254). Most of them were split lengthwise by the fracture between the part and counterpart slabs (Figure 3). Some complete coprolites are well-preserved and visible on both slabs (e.g., MCN-PV 2102; MCN-PV 2231; MCN-PV 2254 A/A′). Others have only the thin outer layer preserved, and still others were completely lost so that just a slight halo remains. A few of them were in an initial stage of decomposition when fossilized, because their content is dispersed (Figures 3C, 4C).
Of the 220 coprolites studied, it was possible to establish both diameters of 107; only the largest diameter of a further six; and only the smallest diameter of the remaining 107. In the 113 coprolites for which the largest diameter could be measured, this parameter ranged from 9.8 to 32.5 mm (average largest diameter: 18.12 mm). In the smallest 214 coprolites for which the minimum diameter could be measured, this parameter ranged from 2.3 to 9.9 mm (average smallest diameter: 5.15 mm). Most coprolites have a largest diameter of 15.0–17.0 mm and a smallest diameter of 4.0–6.0 mm.
There was variation in content of the casting, not only between the different coprolites but between these preserved in the same slab. Four basic types were established: Type 1, only amorphous content; Type 2, with some crustacean fragments; Type 3, predominance of minuscule crustacean fragments; Type 4, crustacean and bone fragments and/or teeth (Figures 3–5).
MCN-PV 2220 is a coprolite containing an incomplete mesosaur tooth with a greatly altered enamel layer, which is opaque due to decalcification by the digestive process (Figures 5B,C).
Under microscopic stereoscopy, sometimes, the bone fragments appear very similar to fragmentary crustaceans, due to digestion. However, generally they are distinguished based on morphology, as the crustaceans are preserved as elongated, compact, fractured carapaces in continuity with each other. Other bone fragments are small polygonal pieces with rounded angles, due to digestion. Some of the best preserved of these were selected for further study under scanning electron microscopy, such as the specimen MCN-PV 2227 (Figure 5D).
Coprolites from the Iratí Formation under Scanning Electron Microscopy (SEM)
The mineralogical composition of coprolites from the Iratí Formation was analyzed under SEM equipped with EDS and the main component found was apatite (Figure 10). Some coprolites showed very small bone fragments with recognizable concentric bone layers. The fragments are much modified, bony microscopic structures, maybe suggesting they underwent a greater degree of digestion than the teeth (Figures 5B,C).
Study of the Bromalites from the Mangrullo Formation (Lower Permian) of Uruguay
Gastric Contents
Partially articulated skeletons of large adults from the Mangrullo Formation Konservat-Lagerstätte preserve gastric contents (Piñeiro et al., 2012b; Ramos, 2015; Figure 6). The main difference with respect to the cololite described from the Iratí Formation is that the elements show a lower digestive degradation of carapaces and bones by the acids, meaning that they were eaten a shorter time before the death of the animal. Although they are difficult to identify more precisely, we can identify isolated mesosaur bones (fragmentary humerus, vertebrae, ribs, mandibles, and skull; Figure 6A), different parts of pygocephalomorph crustacean bodies (e.g., thoracic and abdominal segments, and very probably also small carapaces; Figure 6C), and putative insect wings or plants (Figures 6B,D,E), as being part of the mesosaur gastric contents. These specimens demonstrate the presence of different-sized prey in the contents, as well as different degrees of degradation due to the digestion of the carapaces and bones. Thus, it may be possible to determine how much time passed between the last meal and death. Notably, crustacean remains are more degraded than the bones in all the examined specimens; this can be explained by the absence of mineralization of pygocephalomorph carapaces (see Piñeiro et al., 2012b,c; Ramos, 2015). Although chitin is well-resistant to digestion, many sauropsids secrete chitinases to hydrolyze it and also for defense against many pathogens (Marsh et al., 2001; Siroski et al., 2014). While we cannot know whether such enzymes were present in mesosaurs based on the fossilized specimens, we can say that the crustacean carapaces were almost completely digested in most of the coprolites found, with recognizable fragments present in just a few cases. The bones within the contents are mostly very small and moderately better-preserved vertebrae, ribs and other skeletal elements perhaps belonging to hatchling or juvenile mesosaurids. However, bones of large, adult mesosaurs can also be observed, even in the coprolites (see Figures 8B,D).
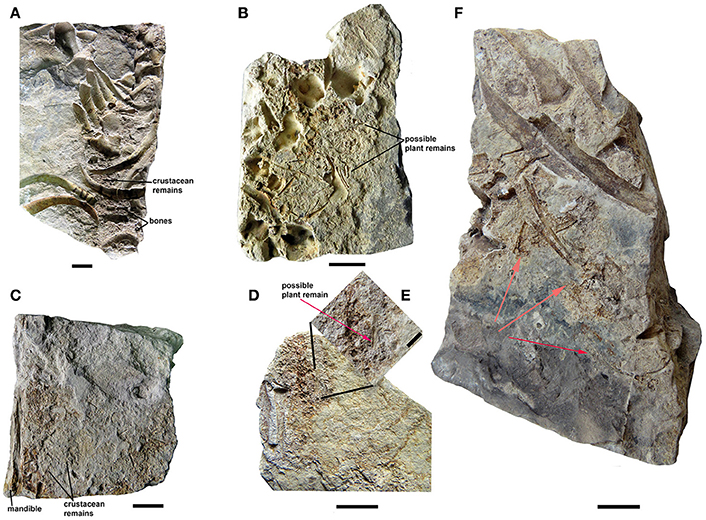
Figure 6. Mesosaurid gastric contents from the Mangrullo Formation (Lower Permian, Uruguay). (A) FC-DPV 2616 and (B) FC-DPV 2608, in partially articulated individuals; (C) FC-DPV 2636 and (D) FC-DPV 2651, identified based on the presence of well-preserved skeletal elements of an adult individual which were possibly separated from the rest of the skeleton by the excavation process using heavy machinery. (E) Close-up of the gastric content showing the presence of possible plant remains or an insect wing. Scale bars: 10 mm, with the exception of scale bar in (E) which is 5 mm. (F) FC-DPV 2650 showing a partial large individual preserving gastric content, associated with a small one (hatchling individual), whose body remains were possibly scavenged after death (red arrows), producing total disarticulation, and loss of most of bones. Scale bar: 10 mm.
Coprolites
Most of the preserved mesosaur coprolites in the Mangrullo Formation (Figures 7, 8) are more or less cylindrical with rounded ends, but some are anisopolar or irregular in shape (Figure 8D). Their morphology cannot be described in detail because of the nearly two-dimensional form of preservation caused by diagenetic compression (see also Hone et al., 2015; Wang et al., 2016). The putative coprolites differ from gastric contents in shape and in being masses constrained by better-defined regular or irregular margins. The state of preservation of remains in the amorphous groundmass differs too (see Figure 8). Coprolites from the Mangrullo Konservat-Lagerstätte can be classified as (1) long elliptical, groundmasses containing probably completely macerated crustacean remains and no bone inclusions; (2) short elliptical, groundmasses with putative bone inclusions; (3) more or less spherical forms with amorphous texture and some inclusions of uncertain origin; and (4) irregular bodies that can contain bones or teeth (Figures 8B,D). As expected for tetrapod coprolites, none show evidence of being spiral (Hunt et al., 1994; Niedźwiedzki et al., 2016). The major axis of the elliptical ones ranges from 20 to 40 mm and the minor one from 5 to 10 mm.
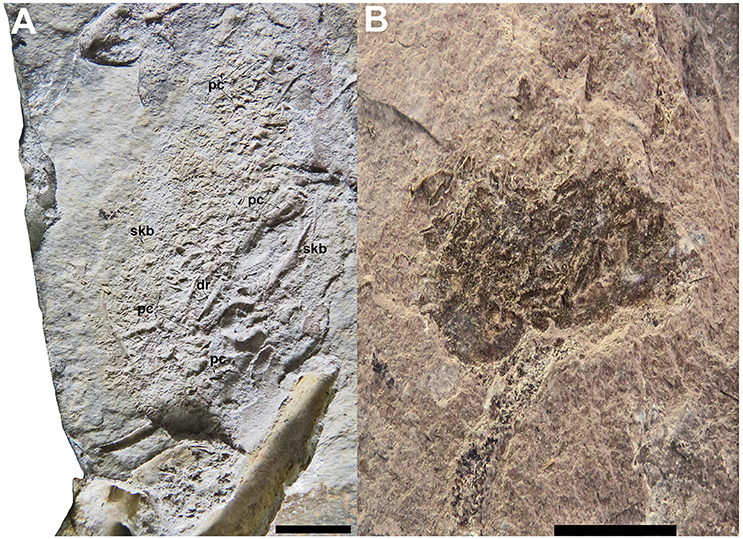
Figure 7. Mesosaur gastric contents and coprolites contrasted. (A) FC-DPV 2156, gastric content found in the Lower Permian Mangrullo Formation. (B) FC-DPV 2651, irregular coprolite from the Lower Permian Mangrullo Formation. dr, dorsal ribs; pc, pygocephalomorph crustaceans; skb, skull bones. Scale bars; 10 mm.
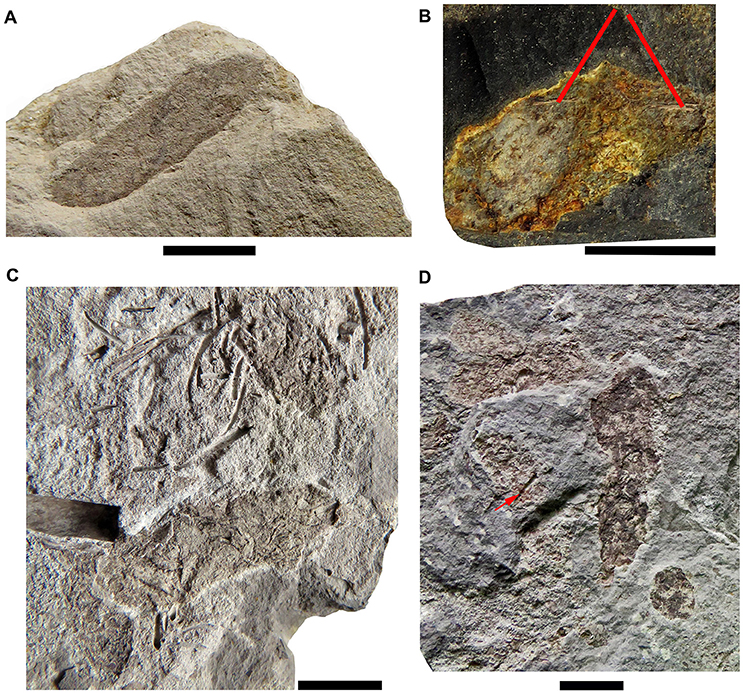
Figure 8. The different types of coprolites found in the Mangrullo Formation (Lower Permian, Uruguay). (A) FC-DPV 2619, elliptical coprolite without bone inclusions; (B) FC-DPV 2647, elliptical coprolite with bone inclusions; (C) FC-DPV 2210, ellipsoid amorphous coprolites without bone inclusions; (D) FC-DPV 2807 irregularly shaped coprolites bearing bone inclusions and teeth (red arrow). Scale bars: 10 mm.
The flattened condition of the coprolites is possibly due to compression of the shale and fine siltstone layers that contain them. Most were preserved as part and counterpart, making it possible to see their interiors and the texture of their contents. Otherwise, three-dimensional, not so well-preserved coprolites seem to have been weathered faster than the surrounding matrix and their exposed surfaces may have been destroyed fairly rapidly (see Figure 4C).
Coprolites can be associated with partially articulated skeletons or bones of adult individuals and with scattered, altered bones, and fragments of different-sized hatchling and juvenile mesosaurs (as described for MCN-PV 2154). Also, they can be associated with complete and fragmentary remains of pygocephalomorph crustaceans. As in the Iratí Formation, there are no bones that can be attributed to vertebrates other than mesosaurids in the levels that yielded the coprolites described herein. The gastric contents may be preserved mainly as phosphatized structures (Figure 10); their preservation was favored by the extreme conditions in the Mangrullo and Iratí formations, including low oxygen rates and increased salinity (see Figure 10C).
Regurgitalites
Small accumulations of moderately-preserved bones and well-preserved teeth are often found in the mesosaur-bearing levels of the Mangrullo Formation (Figure 9). They are interpreted as most likely mesosaur regurgitalites, consisting of indigestible parts that would cause intestinal occlusion if not expelled. They comprise relatively small bones revealing a lower degree of acid etching than bones found in cololites and coprolites, as well as large teeth which lack evident etching marks (Ramos, 2015). In fact, regurgitation may result in only slight chemical and structural alterations on bones, as exemplified by owl pellets (Dauphin et al., 2003). As in the mesosaur coprolites, there is some kind of groundmass surrounding the elements, but the groundmass gives the impression of having been less dense or more liquid in the regurgitalites. This is suggested by the absence of a well-defined outer border in the regurgitalites (Figure 9), in contrast to the coprolites.
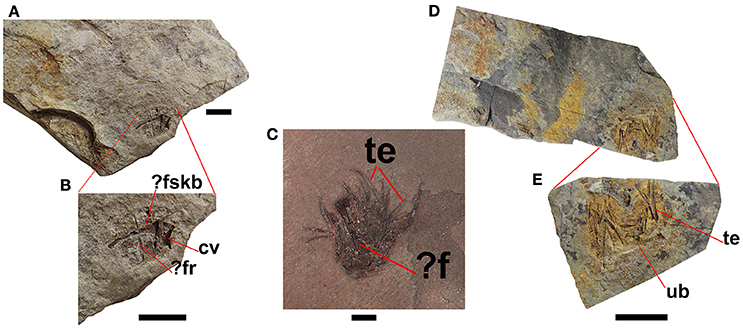
Figure 9. Regurgitalites from the Mangrullo Formation (Lower Permian, Uruguay). (A,B) FC-DPV 2648; (C) GP-2E 19A; (D,E) FC-DPV 2649. Scale bars: 10 mm. cv, cervical vertebra; f, femur; fr, fragmentary rib; fskb, fragmentary skull bone; te, teeth; ub, unidentified bone.
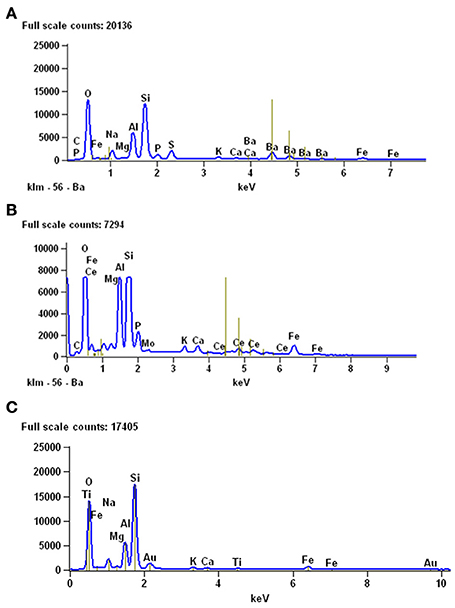
Figure 10. Results of EDS analyses performed to study the mineralogical composition of gastric contents (A) and coprolites (B) from the Mangrullo Formation (Lower Permian, Uruguay). (C) EDS analysis of the sediments around the studied bromalites, for comparative purposes.
Discussion
The mesosaur bromalites described in this study represent a rare case where the source animal can be identified with confidence, because no other tetrapod or vertebrate is found in the mesosaur-bearing strata (Piñeiro, 2006; Piñeiro et al., 2012a). Because all “fish” groups of the Permian period had a spiral valve and none of the coprolite specimens studied herein shows spiral morphology, they are interpreted as produced by tetrapods (see Gilmore, 1992; Hunt et al., 1994; Argyriou et al., 2016; Niedźwiedzki et al., 2016). The only tetrapods known from these particular strata are mesosaurids, represented by a large amount of bone remains. Moreover, the content of the coprolites does not differ substantially from the gut contents observed in mesosaurid skeletons. Therefore, the coprolites are attributed to mesosaurids, although some irregular coprolites could be also assigned to the large arthropods which are present in the Mangrullo Lagerstätte (Piñeiro et al., 2012b). Indeed, some of the pygocephalomorph crustaceans found in the Mangrullo Formation are unusually large (more than 80 mm) and they may also have been scavengers of dead mesosaurs. They are found in intimate association with the mesosaur skeletons, as can be seen even in the holotypic specimen of Mesosaurus tenuidens housed in the Muséum national d'Histoire naturelle in Paris (Figure 11). Therefore, pygocephalomorphs could potentially have produced some of the coprolites. However, the coprolites intimately associated with mesosaur specimens (e.g., MCN-PV 2254) and those found isolated are quite similar in morphology, texture, and color. They are also fairly comparable in size, ranging from 6 to 35 mm in length (see Table 3). This range allows us to infer that coprolites were produced by animals of mesosaur size, i.e., 40–100 cm in length.
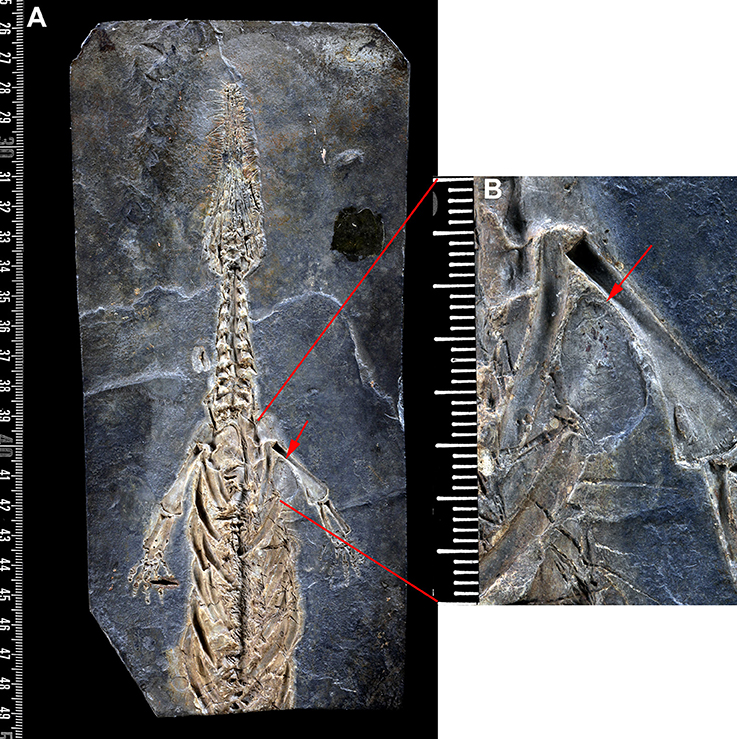
Figure 11. Mesosaurus tenuidens, Gervais, 1865: type specimen (AC 1865-77A). (A) The complete cranial and postcranial preserved skeleton (skull and anterior region of the trunk in ventral view). (B) Close-up of the shoulder region, showing a large pygalocephalomorph crustacean lodged under the humerus (red arrow).
In MCN-PV 2254 the adult skeleton, along with the cololite, coprolites, and bones of juveniles, possibly was preserved in the margins of the water body inhabited by the group. A part of the coprolite and cololite contents was lost and scattered around the adult skeleton. We can thus suggest that the last meal of the adult individual contained tiny bone fragments as well as small crustacean remains which can be distinguished by their morphology. Rapid burial would ensure the preservation of such delicate material. Thus, it seems even possible that the event that disarticulated the individuals also buried them. The presence of microbial mats would also ensure the preservation of bromalites and other fossils, since bacteria may play an important role in the fossilization of fecal matter (Hu et al., 2010; Bajdek et al., 2016).
The remains of a smaller, possibly young mesosaur and other bones that may belong to a hatchling are preserved adjacent to the adult individual and led us to analyze three hypotheses: (1) the smaller bones correspond to animals that died well-before, and were disarticulated by long exposure to air; (2) the small bones were part of the gastric content of the adult individual preserved in the same slab; (3) the bones belong to juvenile mesosaurs that died at the same time and perhaps for the same reason as the adult.
We consider hypothesis 2 least likely on the basis of the following evidence:
(1) The bones do not show any evidence of acid etching produced by gastric fluids.
(2) The skeletons of the juvenile individuals are too large to have passed through the oral cavity or the digestive tract of the articulated adult specimen.
(3) These bones belong to at least two juveniles of different ages associated with the adult. All bones are in the same layer and show similar preservation, and thus, it is unlikely that they were disarticulated and deposited close to an articulated, well-preserved individual by a separate event.
Even though the first hypothesis cannot be disproved, associations of bones from more than one individual or even of partially articulated skeletons are very common in the Mangrullo Formation shales and could suggest parental care and/or aggregation in mesosaurs (see Piñeiro et al., 2012d, 2015b).
It is very probable that the scenario represented by this specimen is more complex than initially thought. We can interpret the slab as bearing one adult and two young individuals (maybe belonging to the same species) that apparently died and were buried at the same time. It is evident that most of the elements are in situ, but there is evidence that the assemblage was not immediately buried after the death of the animals, as some alteration can be seen in the adult skeleton: the skull and front limbs are missing or incomplete, and the hind limbs and tail are very incomplete and have been moved from their original anatomical position. It is probable that the animals died very close to the shore, where the decay of the soft tissues was quicker than in the deep, anoxic bottom waters of the basin. The specimens could have been scavenged by pygocephalomorphs and other mesosaurs, as is shown also by some specimens found in the Mangrullo Formation (Figure 6E). The gut content spread away from the skeleton during the decay processes and, like the coprolites, was buried faster than the bones. The latter remained exposed for a prolonged time, accounting for the partial disarticulation of the adult skeleton. As explained above, there is enough evidence to support the presence of two juvenile individuals next to the adult. One juvenile might have been born a very short time before its death, and the other is somewhat older but still immature. Their bones were lighter and more easily swept away. However, another possible explanation for the presence of the isolated bones is that the original slab could have been broken by the bulldozers working in the quarry. This is a frequent occurrence in the Iratí and in the Mangrullo Formation as well. Indeed, the caterpillars are producing a taphonomic bias toward the young-adult associations but they do not explain why young adults represent the most frequently preserved ontogenetic stage in the two studied populations. Perhaps they were, along with the newborns, the most affected by the environmental stress produced by the evaporitic and progressive drought conditions in the basin engendered by continued volcanism that affected the region during the beginning of the Permian (López-Gamundi, 2006; Santos et al., 2006; Piñeiro et al., 2012d).
Interestingly, coprolite specimens from the Mangrullo and Iratí Formations are quite comparable in size in spite of the noticeably smaller body size of the Brazilian species Brazilosaurus sanpauloensis. This anomaly might suggest a rarity of coprolites of very mature mesosaurids in the Mangrullo Formation of Uruguay. Perhaps this was because just a small part of the population had the good luck to attain an old age. There is a positive but loose correlation between the feces size and the body size of the producer when comparing the individuals of the same or different species; big animals can produce large amounts of small feces (Chin, 1997; Chame, 2003; Flessa et al., 2012).
Ecological Inferences
Mesosaur bromalites tell us quite much about the ecological (trophic) interactions between the components of the peculiar “Mesosaur community” (Piñeiro et al., 2012b). As expected, mesosaurs fed on pygocephalomorph crustaceans, possibly as their preferred prey. They appear to have been size-selective, eating crustacean prey not exceeding 20 mm in length (Ramos, 2015). This can be asserted from almost all the coprolites and from all the studied gastric contents. We also show that small scattered mesosaur bones are present in the cololites, and exceptionally in coprolites (e.g., teeth), suggesting that these skeletal pieces must have been ingested. However, there is no evidence that large mesosaurs preyed on small ones, even though mesosaur bones are common in stomach contents and coprolites (see Table 4). Schwimmer et al. (2015) described partially articulated vertebrae of a baby turtle in a spiral bromalite (coprolite or cololite) of a shark. In contrast, bone elements present within cololites and coprolites in the material from the Mangrullo and Iratí Formations show strong acid etching and many fractures, and they are never articulated. In a hypothetical scenario of predation, we would expect to find more or less articulated skeletons in the gastric contents (where the digestive processes would still allow for a general identification of the bones). Mesosaur teeth seem not to be adapted to powerful biting (although see Pretto et al., 2014) and the jaw aperture in an average-sized mesosaur is much too small to allow even small newborn mesosaurs to be swallowed whole (see Figure 12). Thus, we support instead the hypothesis that mesosaurs were scavengers and fed on soft tissues of smaller decaying individuals. Some bones could have been accidentally ingested along with soft tissue. This could be why some of them are so eroded that they are difficult to identify. As the soft tissues are partially decomposing, the teeth need not be so strong and fixed to the jaws, explaining the high number of them preserved separately from the rest of the skeleton and the frequent occurrence of toothless skulls. Another hypothesis to explain the presence of mesosaur bones in the gastric contents and coprolites is that they were eaten accidentally, during the capture of pygocephalomorph crustaceans that were scavenging on the carrion accumulated on the bottom.
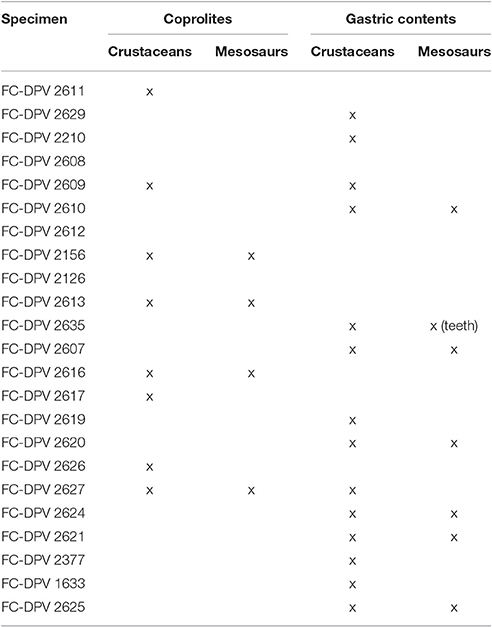
Table 4. Specimens from the Mangrullo Lagerstätte (Uruguay) with preserved cololites having identifiable content (modified from Ramos, 2015).
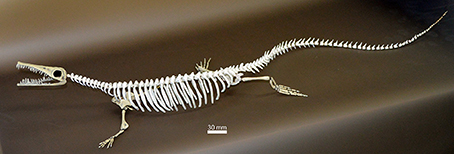
Figure 12. Skeletal reconstruction of a young adult Mesosaurus tenuidens Gervais, 1865 based on comparative measurements and anatomical observation of more than 50 individuals (see Villamil et al., 2015). This reconstruction allows us to obtain a more accurate idea of the size of the prey that a mesosaur of standard size could have eaten.
Teeth are among the skeletal elements that are most resistant to dissolution, although extant crocodiles normally decalcify ingested teeth (Fisher, 1981a,b). This may explain why the teeth found in the regurgitalites and gastric contents of mesosaurs tend to be better-preserved than bones. Interestingly, if tooth fragments found in the samples derive from prey, mesosaurs must have swallowed at least some cranial elements during acts of predation. Such a behavior is known in modern crocodiles, although very large prey needs to be dismembered first (Grigg and Gans, 1993; Webb and Manolis, 2009). But, in the case of mesosaurs, the evidence suggests that partial decomposition of the consumed carrion facilitated the presence of teeth in gastric contents and coprolites. Alternately, the mesosaurs might have sometimes swallowed their own teeth when they broke during feeding. Such tooth damage is known in some archosaurs, which are often characterized by fast tooth replacement (Grigg and Gans, 1993) as mesosaurs were Pretto et al. (2014). Stone et al. (2000) described from the Upper Jurassic of the Morrison Formation a large bone-rich phosphatic mass interpreted as a coprolite, which contained a broken distal end of an Allosaurus tooth. The authors suggested that the tooth broke and was accidentally ingested when the theropod used its jaws to break bones into smaller portions (Stone et al., 2000). Some mesosaur coprolites were found to contain teeth that could have either belonged to very young individuals or been recently erupted replacement teeth of adults, which are also small and short.
Based on the analyzed material, it is hard to determine whether the mesosaurids consumed carrion of the same or another species. Distinguishing between mesosaur species is a very complex issue, even in the case of well-preserved individuals (see Piñeiro et al., 2015a). Therefore, we prefer to identify mesosaurs as scavengers rather than cannibals. The largest crustacean remains (e.g., abdomens and some thoracic segments) often appear to be more weathered than expected, and thus it seems possible that they were also scavenged from the bottom. Moreover, some possible coprolites/regurgitalites resemble the crustaceans in shape and some aspects of internal structure (Figure 3D). It would be conceivable that crustaceans larger than 20 mm in length were swallowed whole, but were soon regurgitated in an incompletely digested state because of their large size. The fossil record contains some previously documented examples of that kind of inappropriate predator-prey interaction, in which a predator and an excessively large prey animal both died after the former swallowed the latter (see Bieńkowska-Wasiluk, 2010). Alternatively, the crustaceans may have been expelled as feces (see Figure 13).
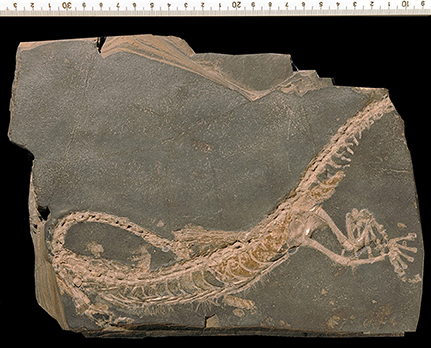
Figure 13. PIMUZ A III 192. Mesosaur specimen assigned to the species Brazilosaurus sanpauloensis showing a huge mass of fecal matter completely filling the distal part of intestine and some smaller feces already outside of the body (see text for a more detailed interpretation of the preservation).
The presence of apparent bone inclusions in the coprolites (Figure 4A) is intriguing. Reptiles are characterized by a long period of digestion and, for example, crocodiles digest the bones they ingest practically completely (Milàn, 2012; see Owocki et al., 2012). However, mesosaurs were fairly small; while large snakes may defecate even less than once a year, gracile arboreal snake species, which have low body mass, sometimes defecate within 24–48 h of ingesting a meal (Lillywhite et al., 2002). Relatively large bones are seen also in the putative regurgitalites, which also contain teeth of varied size and fragmentary bones that are difficult to identify, although they could be ribs or other long bones (Figure 9). Mesosaurs probably regurgitated bone fragments, perhaps consumed accidentally, which were too large to pass through the gastrointestinal tract. For example, raptor birds (e.g., Bond, 1936; Dauphin et al., 2003; Terry, 2004) and crocodiles (e.g., Grigg and Gans, 1993; Webb and Manolis, 2009; Semeniuk et al., 2011), as well as probably ichthyosaurs (Doyle and MacDonald, 1993; Nature News, 2002; Thies and Hauff, 2013), regurgitate most of the indigestible or hard-to-digest remains. Regurgitation might also have been caused by some kind of environmental stress, as suggested by the conditions under which the Mangrullo Formation is thought to have been deposited. Because digestion efficiency depends on body temperature in extant reptiles, undigested remnants of prey may be regurgitated during periods of unfavorable environmental temperature, as exemplified by crocodiles (Verdade et al., 1994) and snakes (Hailey and Davies, 1987), and regurgitation may also be caused by disease (Lock et al., 2003). Also, the presence of poorly digested remains in feces, caused by a short food-retention time in the gastrointestinal tract, may have to do with fluctuating food availability (see Chin et al., 2003).
Taphonomic Effects of the Environmental Conditions under Which the Iratí and Mangrullo Lagerstätten Were Deposited
Considering the sedimentary facies present in the Iratí and Mangrullo formations (Figure 1) and the data from the fossils described above, we can infer similar trophic conditions across the entire water body where mesosaurs lived (see Piñeiro et al., 2012b). Yet, it seems that specimens with gastric contents are comparatively more frequently found in the Uruguayan Mangrullo Formation than coprolites, the latter being more abundant and better preserved in the Brazilian deposits. In the Mangrullo Formation the evidence is suggestive of near-shore conditions, where not only its autochthonous communities are represented but also the parautochthonous one (plants and insects). Such combination of environmental and taphonomic conditions might in part have contributed to the decomposition of feces (see Chin and Gill, 1996; Wahl et al., 1998; Chin et al., 2009). Material preserved within the gastrointestinal tract may have a better preservation potential than fecal matter expelled in the form of excrement (see Section Discussion in the Supplementary Material in Niedźwiedzki et al., 2016), resulting in better preservation of such materials in the Mangrullo Formation. The presence of increased salinity and stagnant conditions at the bottom, associated with the presence of algal mats (Piñeiro et al., 2012b), delayed the decay processes and produced a very favorable environment for preservation of very delicate soft materials. In contrast, in the facies studied at the State of Goiás, Brazil, fossilization occurred in deeper water conditions. Feces may disintegrate in water and hence some believe that subaerial conditions are more suitable for their preservation (Poinar and Boucot, 2006), as seen in many coprolites from young archeological contexts preserved via desiccation (Wood and Wilmshurst, 2014). However, the process of mineralization and/or lithification may require some initial humidity of the fecal mass (see Bajdek et al., 2016). The most crucial factors allowing the preservation of feces are rapid burial followed by rapid lithification (Eriksson et al., 2011). For example, Bajdek (2013) described a putative coprolite preserved beneath sediments of a turbidity current. However, in the Mangrullo and Iratí Formations the sedimentation rate was very low. Preservation of the fossils described herein was also favored by the periodic volcanic events that affected the basin (López-Gamundi, 2006; Santos et al., 2006; Piñeiro et al., 2012b), which facilitated rapid burial of the specimens. However, it seems likely that the excrement masses were covered by microbial mats which are common in the sediments (Piñeiro et al., 2012b), essentially as suggested by Hu et al. (2010). The role of bacteria in the fossilization of feces has already been discussed by some authors (Chin and Kirkland, 1998; Hollocher et al., 2001; Hollocher and Hollocher, 2012; Bajdek et al., 2016; Qvarnström et al., 2016).
Interestingly, the fecal matter in the putative coprolite specimen described by Bajdek (2013), buried beneath sediments of a turbidity current, was replaced by fine clastic material, only preserving abundant shell fragments as content and the general excrement-shaped morphology. In contrast, the coprolite (and cololite) specimens from the Mangrullo and Iratí Formations, likely preserved as a result of microbial activity, are composed of fossilized original phosphatic fecal matter, like most carnivore coprolites known from the fossil record (Chin, 2002). As expected, EDS analysis of mesosaur stomach contents and coprolite matrix from the Mangrullo Formation reveals that the latter is characterized by a higher content of phosphate (Figure 10). This is explained by a higher degree of decomposition of the ingested bone material in the final stage of digestion. Finally, one of the interesting aspects of the material studied herein is the presence of some kind of groundmass surrounding the regurgitated elements, which nevertheless gives the impression of originally having been less dense or more liquid than in coprolites.
Conclusions
This study showed for the first time preserved residues from four distinct phases of the digestive process, which can all be assumed to belong to the same basal amniote: gastric contents, cololites, coprolites, and possibly regurgitalites. Coprolites are associated with mesosaur skeletons and bones and even those that were found isolated can be assigned to Mesosauridae, because of the absence of any other vertebrate in the studied outcrops. Therefore, this is one of the rare cases when coprolites can be directly ascribed to their producers.
Mesosaur diet included pygocephalomorph crustaceans as the main food item, corroborating some of the hypotheses previously suggested. However, contrary to other hypotheses based on indirect inferences, there is no evidence in support of the use of fish as a food item by any of the recognized mesosaurid species.
In addition, bone fragments occurring in cololites and gastric contents, and possibly also in coprolites and regurgitalites, are identified as belonging to juvenile Mesosauridae, which might suggest that mesosaurs developed a cannibalistic predatory behavior. However, the lack of any further evidence in support of this hypothesis, together with the morphology of the jaws and teeth of mesosaurids, leads us to suggest that mesosaurids were facultative scavengers, eating carrion of the same or different mesosaurid species. Both cannibalistic behavior and scavenging are quite typical under environmental stress, overcrowding and insufficient food resources. Also, the presence of undigested bone elements in the regurgitalites and feces of ectothermic reptiles sometimes results from environmental stress.
Perhaps the stress conditions were in part caused by the extended volcanism that began during the Early Permian. The volcanic events, as well as the presence of microbial mats on the bottoms of the water bodies, facilitated preservation of the fecal matter.
Author Contributions
RS: Studied the Brazilian materials, wrote part of the manuscript, made Tables 1, 2, performed some of the analyses. JF: Wrote part of the manuscript and provided relevant hypotheses to the better understanding of some aspects of the manuscript. PB: Wrote part of the manuscript and helped with the English language and bibliography, made important contributions to the article related to his specialty on the coprolite and cololite study. GP: Studied the Uruguayan materials, wrote the manuscript, and prepared all the figures, made Tables 3, 4 and performed part of the analyses detailed in the “Materials and Methods” Section.
Funding
This study was partially financed by ANII FCE_2011_6450 and by NGS grant 049714 to GP.
Conflict of Interest Statement
The authors declare that the research was conducted in the absence of any commercial or financial relationships that could be construed as a potential conflict of interest.
Acknowledgments
We are very grateful to the personnel and students of the Departamento de Paleontologia of the São Paulo University and the Paläontologisches Institut und Museum, University of Zürich for allowing the study of some specimens included in this contribution. We also thank Silvia Villar and Alejandro Márquez (Servicio de Microscopía Electrónica, Facultad de Ciencias, Montevideo, Uruguay) for assistance in the analyses performed under SEM. Joselyn Falconnet (Muséum National d'Histoire Naturelle, Paris) provided the photographs for Figure 11. We are grateful to the academic editor Corwin Sullivan and to David Marjanovíc and Eric M. Roberts for their constructive and very much thorough revision of this manuscript. We are indebted to William Idiart Rodriguez for assistance in the construction of Figure 1D.
Supplementary Material
The Supplementary Material for this article can be found online at: http://journal.frontiersin.org/article/10.3389/feart.2017.00023/full#supplementary-material
References
Araújo, D. C. (1976). Taxonomia e relações dos Proganosauria da Bacia do Paraná. Anais Acad. Brasil. Ciências 48, 91–116.
Araújo-Barberena, D. C. (1994). “Mesossauros e Pareiassauros: dúvidas e controvérsias,” in Workshop de Integração da Geologia e Paleontologia de Vertebrados no RS, Vol. 1 (Porto Alegre: Resumo das Comunicações), 21–22.
Argyriou, T., Clauss, M., Maxwell, E. E., Furrer, H., and Sánchez-Villagra, M. R. (2016). Exceptional preservation reveals gastrointestinal anatomy and evolution in early actinopterygian fishes. Sci. Rep. 6:18758. doi: 10.1038/srep18758
Bajdek, P. (2013). Coprolite of a durophagous carnivore from the Upper Cretaceous Godula Beds, Outer Western Carpathians, Poland. Geol. Q. 57, 361–364. doi: 10.7306/gq.1094
Bajdek, P., Owocki, K., and Niedźwiedzki, G. (2014). Putative dicynodont coprolites from the Upper Triassic of Poland. Palaeogeogr. Palaeoclimatol. Palaeoecol. 411, 1–17. doi: 10.1016/j.palaeo.2014.06.013
Bajdek, P., Qvarnström, M., Owocki, K., Sulej, T., Sennikov, A. G., Golubev, V. K., et al. (2016). Microbiota and food residues including possible evidence of pre-mammalian hair in Upper Permian coprolites from Russia. Lethaia 49, 455–477. doi: 10.1111/let.12156
Bieńkowska-Wasiluk, M. (2010). Taphonomy of oligocene teleost fishes from the outer carpathians of Poland. Acta Geol. Polonica 60, 479–533.
Bond, R. M. (1936). Eating habits of falcons with special reference to pellet analysis. The Condor 38, 72–76. doi: 10.2307/1363552
Carroll, R. L. (1982). The early evolution of reptiles. Ann. Rev. Ecol. Sys. 13, 87–109. doi: 10.1146/annurev.es.13.110182.000511
Carroll, R. L. (1988). Vertebrate Paleontology and Evolution. New York, NY: W.H. Freeman and Company, 698.
Chame, M. (2003). Terrestrial mammal feces: a morphometric summary and description. Mem. Inst. Oswaldo Cruz 98, 71–94. doi: 10.1590/s0074-02762003000900014
Chin, K. (1997). “Coprolites,” in Encyclopedia of Dinosaurs, eds P. J. Currie and K. Padian (New York, NY: Academic Press), 147–150.
Chin, K. (2002). “Analyses of coprolites produced by carnivorous vertebrates,” in The Fossil Record of Predation. Paleontological Society Special Papers 8, eds M. Kowalewski and P. H. Kelley, 43–49.
Chin, K., Bloch, J., Sweet, A., Tweet, J., Eberle, J., Cumbaa, S., et al. (2008). Life in a temperate polar sea: a unique taphonomic window on the structure of a Late Cretaceous Arctic marine ecosystem. Proc. R. Soc. B Biol. Sci. 275, 2675–2685. doi: 10.1098/rspb.2008.0801
Chin, K., Eberth, D. A., Schweitzer, M. H., Rando, T. A., Sloboda, W. J., and Horner, J. (2003). Remarkable preservation of undigested muscle tissue within a late cretaceous tyrannosaurid coprolite from Alberta, Canada. Palaios 18, 286–294. doi: 10.1669/0883-1351(2003)018<0286:RPOUMT>2.0.CO;2
Chin, K., and Gill, B. D. (1996). Dinosaurs, dung beetles, and conifers: participants in a Cretaceous food web. Palaios 11, 280–285. doi: 10.2307/3515235
Chin, K., Hartman, J. H., and Roth, B. (2009). Opportunistic exploitation of dinosaur dung: fossil snails in coprolites from the Upper Cretaceous two medicine formation of Montana. Lethaia 42, 185–198. doi: 10.1111/j.1502-3931.2008.00131.x
Chin, K., and Kirkland, J. I. (1998). Probable herbivore coprolites from the Upper Jurassic Mygatt-Moore Quarry, Western Colorado. Mod. Geol. 23, 249–275.
Dauphin, Y., Andrews, P., Denys, C., Fernández-Jalvo, Y., and Williams, T. (2003). Structural and chemical bone modifications in a modern owl pellet assemblage from Olduvai Gorge (Tanzania). J. Taphonomy 1, 209–232.
Dentzien-Dias, P. C., de Figueiredo, A. E. Q., Horn, B., Cisneros, J. C., and Schultz, C. L. (2012). Paleobiology of a unique vertebrate coprolites concentration from Rio do Rasto Formation (Middle/Upper Permian), Paraná Basin, Brazil. J. S. Am. Earth Sci. 40, 53–62. doi: 10.1016/j.jsames.2012.09.008
Dentzien-Dias, P. C., Poinar, G. Jr., de Figueiredo, A. E. Q., Pacheco, A. C. L., Horn, B. L. D., and Schultz, C. L. (2013). Tapeworm eggs in a 270 million-year-old shark coprolite. PLoS ONE 8:e55007. doi: 10.1371/journal.pone.0055007
Doyle, P., and MacDonald, D. I. M. (1993). Belemnite battlefields. Lethaia 26, 65–80. doi: 10.1111/j.1502-3931.1993.tb01513.x
Eriksson, M. E., Lindgren, J., Chin, K., and Månsby, U. (2011). Coprolite morphotypes from the Upper Cretaceous of Sweden: novel views on an ancient ecosystem and implications for coprolite taphonomy. Lethaia 44, 455–468. doi: 10.1111/j.1502-3931.2010.00257.x
Ferigolo, J. (1985). “O uso da Microscopia Eletrônica de Varredura na sistemática dos mamíferos,” in Congresso Brasileiro de Paleontologia 8 (Rio de Janeiro). Coletβnea de trabalhos paleontológicos (Brasilia: DNPM), 43–49 (Série Geologia, 27; Secção Paleontologia e Estratigrafia).
Fisher, D. C. (1981a). Crocodilian scatology, microvertebrate concentrations, and enamel-less teeth. Paleobiology 7, 262–275. doi: 10.1017/S0094837300004048
Fisher, D. C. (1981b). Taphonomic Interpretation of Enamel-Less Teeth in the Shotgun Local Fauna (Paleocene, Wyoming). Contributions from the Museum of Paleontology, The University of Michigan, Vol. 25, 259–275.
Flessa, K., Struthers, A., Fall, L. M., and Dexter, T. A. (2012). “Really crappy talk: fecal volume and body size in birds, mammals and dinosaurs,” in GSA Annual Meeting & Exposition 4–7 November 2012 (Paper No. 30774–206053) (Charlotte, NC).
Gilmore, B. (1992). Scroll coprolites from the Silurian of Ireland and the feeding of early vertebrates. Palaeontology 35, 319–333.
Grigg, G., and Gans, C. (1993). “Morphology and physiology of the Crocodylia,” in Fauna of Australia Vol 2A Amphibia and Reptilia (Canberra, ACT: Australian Government Publishing Service), 326–336.
Hailey, A., and Davies, P. M. C. (1987). Digestion, specific dynamic action, and ecological energetics of Natrix maura. Herpetol. J. 1, 159–166.
Hollocher, K., and Hollocher, T. C. (2012). Early processes in the fossilization of terrestrial feces to coprolites, and microstructure preservation. NMMNH Bull. 57, 79–91.
Hollocher, T. C., Chin, K., Hollocher, K. T., and Kruge, M. A. (2001). Bacterial residues in coprolite of herbivorous Dinosaurs: role of bacteria in mineralization of feces. Palaios 16, 547–565. doi: 10.1669/0883-1351(2001)016<0547:BRICOH>2.0.CO;2
Hone, D., Henderson, D. H., Therrien, F., and Habib, M. B. (2015). A specimen of Rhamphorhynchus with soft tissue preservation, stomach contents and a putative coprolite. PeerJ 3:e1191. doi: 10.7717/peerj.1191
Hu, S. X., Zhang, Q. Y., and Zhou, C. Y. (2010). Fossil coprolites from the Middle Triassic Luoping biota and ecological implication. J. Earth Sci. 21, 191–193. doi: 10.1007/s12583-010-0209-7
Hunt, A. P., Chin, K., and Lockley, M. G. (1994). “The paleobiology of vertebrate coprolites,” in The Paleobiology of Trace Fossils, ed S. K. Donovan (London: John Wiley), 221–240.
Hunt, A. P., and Lucas, S. G. (2005a). A new coprolite ichnotaxon fromthe Early Permian of Texas. NMMNH Bull. 30, 121–122.
Hunt, A. P., and Lucas, S. G. (2005b). The origin of large vertebrate coprolites from the Early Permian of Texas. NMMNH Bull. 30, 125–126.
Hunt, A. P., and Lucas, S. G. (2012). Classification of vertebrate coprolites and related trace fossils. NMMNH Bull. 57, 137–146.
Hunt, A. P., Lucas, S. G., and Spielmann, J. A. (2005a). Early Permian vertebrate coprolites from north-central New Mexico with description of a new ichnogenus. NMMNH Bull. 31, 39–42.
Hunt, A. P., Lucas, S. G., and Spielmann, J. A. (2005b). Biochronology of Early Permian vertebrate coprolites of the American Southwest. NMMNH Bull. 31, 43–45.
Laurin, M., and Reisz, R. R. (1995). A reevaluation of early amniote phylogeny. Zool. J. Linn. Soc. Lond. 113, 165–223. doi: 10.1111/j.1096-3642.1995.tb00932.x
Lillywhite, H. B., de Delva, P., and Noonan, B. P. (2002). “Patterns of gut passage time and the chronic retention of fecal mass in viperid snakes,” in Biology of the Vipers, eds G. W. Schuett, M. Höggren, and H. W. Greene (Traverse City, MI: Biological Sciences Press), 497–506.
Lock, B., Heard, D., Detrisac, C., and Elliott Jacobson, E. (2003). An epizootic of chronic regurgitation associated with chlamydophilosis in recently imported emerald tree boas (Corallus caninus). J. Zoo Wildl. Med. 34, 385–393. doi: 10.1638/02-065
López-Gamundi, O. (2006). Permian plate margin volcanism and tuffs in adjacent basins of west Gondwana: age constraints and common characteristics. J. S. Am. Earth Sci. 22, 227–238. doi: 10.1016/j.jsames.2006.09.012
MacGregor, J. H. (1908). “On Mesosaurus brasiliensis nov. sp. from the Permian of Brazil,” in Commissão dos Estudos das Minas de Carvão de Pedra do Brasil, Parte II, ed I. C. White (Rio de Janeiro: National Press), 301–336.
Marsh, R. S., Moe, C., Lomnethc, R. B., Fawcett, J. D., and Place, A. (2001). Characterization of gastrointestinal chitinase in the lizard Sceloporus undulatus garmani (Reptilia: Phrynosomatidae). Com. Biochem. Physiol. Part B Biochem. Mol. Biol. 128, 675–682. doi: 10.1016/S1096-4959(00)00364-X
Milàn, J. (2012). Crocodylian scatology – a look into morphology, internal architecture, inter- and intraspecific variation and prey remains in extant crocodylian feces. NMMNH Bull. 57, 65–71.
Modesto, S. P. (1996). The Anatomy, Relationships, and Palaeoecology of Mesosaurus tenuidens and Stereosternum tumidum (Amniota: Mesosauridae) from the Lower Permian of Gondwana. dissertation Ph.D. thesis, University of Toronto, Toronto, ON.
Modesto, S. P. (1999). Observations on the structure of the early permian reptile Stereosternum tumidum Cope. Palaeontol. Afr. 35, 7–19.
Modesto, S. P. (2006). The cranial skeleton of the Early Permian aquatic reptile Mesosaurus tenuidens: implications for relationships and palaeobiology. Zool. J. Linn. Soc. Lond. 146, 345–368. doi: 10.1111/j.1096-3642.2006.00205.x
Morosi, E. (2011). Estudio comparativo del cráneo en Mesosauridae de la Formación Mangrullo (Pérmico Temprano) de Uruguay. Montevideo: Facultad de Ciencias; Universidad de la República.
Niedźwiedzki, G., Bajdek, P., Qvarnström, M., Sulej, T., Sennikov, A. G., and Golubev, V. K. (2016). Reduction of vertebrate coprolite diversity associated with the end-Permian extinction event in Vyazniki region, European Russia. Palaeogeogr. Palaeoclimatol. Palaeoecol. 450, 77–90. doi: 10.1016/j.palaeo.2016.02.057
Oelofsen, B., and Araújo, D. C. (1987). Mesosaurus tenuidens and Stereosternum tumidum from the Permian Gondwana of both Southern Africa and South America. S. Afr. J. Sci. 83, 370–372.
Oelofsen, B., and Aráujo, D. C. (1983). Palaecological implications of the distribution of mesosaurid reptiles in the Permian Irati sea (Paraná Basin), South America. Rev. Brasil. Geociê. São Paulo 13, 1–6.
Owocki, K., Niedźwiedzki, G., Sennikov, A. G., Golubev, V. K., Janiszewska, K., and Sulej, T. (2012). Upper Permian vertebrate coprolites from Vyazniki and Gorokhovets, Vyatkian regional stage, Russian Platform. Palaios 27, 867–877. doi: 10.2110/palo.2012.p12-017r
Pauwels, O. S. G., Barr, B., Sanchez, M. L., and Burger, M. (2007). Diet records for the dwarf crocodile, Osteolaemus tetraspis tetraspis in Rabi Oil Fields and Loango National Park, southwestern Gabon. Hamadryad 31, 258–264.
Piñeiro, G. (2002). Paleofaunas del Pérmico-Eotriásico de Uruguay. dissertation/master's thesis, PEDECIBA, Universidad de la República, Montevideo.
Piñeiro, G. (2004). Paleofaunas del Pérmico y Permo-Triásico de Uruguay. Bioestratigrafía, Paleobiogeografía y Sistemática. dissertation/PhD thesis, Universidad de la República, Montevideo.
Piñeiro, G. (2006). “Nuevos aportes a la paleontología del Pérmico de Uruguay,” in Cuencas Sedimentarias de Uruguay: Geología, Paleontología y Recursos Naturales – Paleozoico, eds G. Veroslavsky, M. Ubilla, and S. Martínez (Montevideo: DI.R.A.C./FCien), 257–279.
Piñeiro, G. (2008). “Los mesosaurios y otros fósiles de fines del Paleozoico,” in Fósiles de Uruguay, ed D. Perea (Montevideo: DIRAC, Facultad de Ciencias), 179–205.
Piñeiro, G., Ferigolo, J., Meneghel, M., and Laurin, M. (2012d). The oldest known amniotic embryos suggest viviparity in mesosaurs. Hist. Biol. 24, 620–630. doi: 10.1080/08912963.2012.662230
Piñeiro, G., Ferigolo, J., Ramos, A., and Laurin, M. (2012a). Cranial morphology of the Early Permian mesosaurid Mesosaurus tenuidens and the evolution of the lower temporal fenestration reassessed. Comptes Rendus Palevol. 11, 379–391. doi: 10.1016/j.crpv.2012.02.001
Piñeiro, G., Ferigolo, J., Núñez, P., Meneghel, M., and Laurin, M. (2015a). “Mesosaurid taxonomy and systematics: the evolutive significance of the mesosaurid lower temporal fenestra: an overview of more than a century of research,” in Biology and Evolutionary History of Mesosaurs, the Oldest Known Gondwanan Aquatic Reptiles, Topic Research, Frontiers in Earth Science, eds G. Piñeiro and M. Laurin.
Piñeiro, G., Ferigolo, J., Núñez, P., Meneghel, M., Villar, S., and Laurin, M. (2015b). “Mesosaurid reproductive biology,” in Biology and Evolutionary History of Mesosaurs, the Oldest Known Gondwanan Aquatic Reptiles, Topic Research, Frontiers in Earth Science, eds G. Piñeiro, and M. Laurin.
Piñeiro, G., Meneghel, M., and Núñez-Demarco, P. (2016). The ontogenetic transformation of the mesosaurid tarsus: a contribution to the origin of the amniotic astragalus. PeerJ 4:e2036. doi: 10.7717/peerj.2036
Piñeiro, G., Morosi, E., Ramos, A., and Scarabino, F. (2012c). Pygocephalomorph crustaceans from the Early Permian of Uruguay: constraints on taxonomy. Rev. Brasil. Paleontol. 15, 33–48. doi: 10.4072/rbp.2012.1.03
Piñeiro, G., Ramos, A., Goso, C., Scarabino, F., and Laurin, M. (2012b). Unusual environmental conditions preserve a mesosaur-bearing Konservat-Lagerstätte from Uruguay. Acta Palaeontol. Pol. 57, 299–318. doi: 10.4202/app.2010.0113
Poinar, G., and Boucot, A. J. (2006). Evidence of intestinal parasites of dinosaurs. Parasitology 133, 245–249. doi: 10.1017/S0031182006000138
Pretto, F. A., Cabreira, S. F., and Schultz, C. L. (2014). Tooth microstructure of the Early Permian aquatic predator Stereosternum tumidum. Acta Palaeontol. Pol. 59, 125–133. doi: 10.4202/app.2011.0121
Qvarnström, M., Niedźwiedzki, G., and Žigaitė, Ž. (2016). Vertebrate coprolites (fossil faeces): an underexplored Konservat-Lagerstätte. Earth Sci. Rev. 162, 44–57. doi: 10.1016/j.earscirev.2016.08.014
Raimundo-Silva, R. (1999). Hábito Alimentar de Brazilosaurus Sanpauloensis (Reptilia, Mesosauridae) Formação Iratí, Estado de Goiás, com Base em Conteúdo Digestivo e Coprólitos. dissertation/master's thesis, Instituto de Geociências, Universidade Federal do Rio Grande do Sul, Porto Alegre.
Raimundo-Silva, R., Ferigolo, J., and Sedor, F. A. (1997). “Primeiras evidências de conteúdo digestivo em Brazilosaurus sanpauloensis (Reptilia, Mesosauridae) da Formação Iratí, Bacia do Paraná,” in XV Congresso Brasileiro de Paleontologia (São Pedro: Resumos), 85.
Ramos, A. (2015). La Dieta de Los Reptiles Mesosauridae (Reptilia: Proganosauria) del Pérmico Temprano de Uruguay. dissertation/graduation's thesis, Universidad de la República, Montevideo.
Santos, R. V., Souza, P. A., Alvarenga, C. J. S., Dantas, E. L., Pimentel, E. L., Oliveira, C. G., et al. (2006). Shrimp U−Pb zircon dating and palynology of bentonitic layers from the permian irati formation Parana basin, Brazil. Gondwana Res. 9, 456–463. doi: 10.1016/j.gr.2005.12.001
Schwimmer, D. R., Weems, R. E., and Sanders, A. E. (2015). A late cretaceous shark coprolite with baby freshwater turtle vertebrae inclusions. Palaios 30, 707–713. doi: 10.2110/palo.2015.019
Sedor, F. A. (1994). Estudo Pós-Craniano de Brazilosaurus Sanpauloensis Shikama & Ozaki, 1966 (Anapsida, Proganosauria, Mesosauridae) da Formação Irati, Permiano da Bacia do Paraná, Brasil. dissertation/master's thesis, Programa de Pós-graduação em Geociências, Universidade Federal do Rio Grande do Sul, Porto Alegre.
Semeniuk, V., Manolis, C., Webb, G. J. W., and Mawson, P. R. (2011). The saltwater crocodile, Crocodylus porosus Schneider, 1801, in the Kimberley coastal region. J. R. Soc. West. Aust. 94, 407–416.
Shelton, C. D. (2013). A new method to determine volume of bromalites: morphometrics of Lower Permian (Archer City Formation) heteropolar bromalites. Swiss J. Palaeontol. 132, 221–238. doi: 10.1007/s13358-013-0057-z
Siroski, P. A., Poletta, G. L., Parachu Marco, M. V., Ortega, H. H., and Merchant, M. E. (2014). Presence of chitinase enzymes in crocodilians. Acta Herpetol. 9, 139–146. doi: 10.13128/Acta_Herpetol-13237
Smith, R. M. H., and Botha-Brink, J. (2011). Morphology and composition of bone-bearing coprolites from the Late Permian Beaufort Group, Karoo Basin, South Africa. Palaeogeogr. Palaeoclimatol. Palaeoecol. 312, 40–53. doi: 10.1016/j.palaeo.2011.09.006
Stone, D. D., Crisp, E. L., and Bishop, J. T. (2000). A large meat-eating dinosaur coprolite from the jurassic morrison formation of Utah. Geol. Soc. Am. Abstr. Programs 32, 220.
Terry, R. C. (2004). Owl pellet taphonomy: a preliminary study of the post-regurgitation taphonomic history of pellets in a temperate forest. Palaios 19, 497–506. doi: 10.1669/0883-1351(2004)019<0497:OPTAPS>2.0.CO;2
Thies, D., and Hauff, R. B. (2013). A Speiballen from the lower jurassic posidonia shale of South Germany. N. Jb. Geol. Paläont. Abh. 267, 117–124. doi: 10.1127/0077-7749/2012/0301
Tsuji, L. A., and Müller, J. (2009). Assembling the history of the Parareptilia: phylogeny, diversification, and a new definition of the clade. Fossil Rec. 12, 71–81. doi: 10.1002/mmng.200800011
Verdade, L. M., Packer, I. U., Michelloti, F., and Rangel, M. C. (1994). “Thermoregulatory behavior of broad snouted caiman (Caiman latirostris) under different thermal regimes,” in Workshop Sobre Conservación y Manejo del Yacare Overo Caiman latirostris, Vol. 4 (Santa Fé), 84–94.
Villamil, J., Meneghel, M., Blanco, R. E., Jones, W., Núñez Demarco, P., Rinderknecht, A., et al. (2015). Optimal swimming speed estimates in the Early Permian mesosaurid Mesosaurus tenuidens (Gervais, 1865) from Uruguay. Hist. Biol. 28, 963–971. doi: 10.1080/08912963.2015.1075018
Wahl, A. M., Martin, A. J., and Hasiotis, S. (1998). “Vertebrate coprolites and coprophagy traces, Chinle Formation (Late Triassic), Petrified Forest National Park, Arizona, in National Park Service; Paleontological Research,” in National Park Service Geological Resources Division Technical Report NPS/NRGRD/GRDTR-98/01, eds V. L. Santucci and L. McClelland (Lakewood, CO: National Park Service, Geological Resources Division), 144–148.
Wang, M., Zhou, Z., and Sullivan, C. (2016). A fish-eating enantiornithine bird from the early cretaceous of china provides evidence of modern avian digestive features. Curr. Biol. 26, 1–7. doi: 10.1016/j.cub.2016.02.055
Webb, G., and Manolis, C. (2009). Green Guide to Crocodiles of Australia. Sydney, NSW: New Holland Publishers.
Wings, O. (2007). A review of gastrolith function with implications for fossil vertebrates and a revised classification. Acta Palaeontol. Pol. 52, 1–16.
Keywords: Mesosauridae, Early Permian, Iratí Formation, Mangrullo Formation, Fossil-Lagerstätte, diet, bromalites
Citation: Silva RR, Ferigolo J, Bajdek P and Piñeiro G (2017) The Feeding Habits of Mesosauridae. Front. Earth Sci. 5:23. doi: 10.3389/feart.2017.00023
Received: 08 October 2016; Accepted: 21 February 2017;
Published: 13 March 2017.
Edited by:
Corwin Sullivan, Institute of Vertebrate Paleontology and Paleoanthropology (CAS), ChinaReviewed by:
David Marjanović, Museum für Naturkunde, GermanyEric M. Roberts, James Cook University Townsville, Australia
Copyright © 2017 Silva, Ferigolo, Bajdek and Piñeiro. This is an open-access article distributed under the terms of the Creative Commons Attribution License (CC BY). The use, distribution or reproduction in other forums is permitted, provided the original author(s) or licensor are credited and that the original publication in this journal is cited, in accordance with accepted academic practice. No use, distribution or reproduction is permitted which does not comply with these terms.
*Correspondence: Graciela Piñeiro, Zm9zc2lsQGZjaWVuLmVkdS51eQ==