- Department of Natural and Environmental Risks, Regional Agency for Environmental Protection of Piemonte, Turin, Italy
In northern Italy rainfall-triggered shallow landslides are recurrent hazardous phenomena that cause casualties and extensive damages. In the last decades several early warning systems (EWSs) have been developed based on rainfall intensity–event duration (I-D) thresholds derived by long rain gages time series. However, rain gages density and their representativeness limit reliability of such EWSs. In the past decades, several studies explored successfully the usefulness of reliable quantitative precipitation estimates (QPEs) by weather radar. The availability of high spatial and temporal resolution QPEs with short latency of the data makes those observations appealing as input data to automatic EWSs. Nevertheless, weather radar based QPEs can be affected by several sources of errors and uncertainties (miscalibration, partial beam blocking, overhanging precipitation, and so on). Analyzing the heavy precipitations that hit Piemonte, north-western Italy, on November 2016, causing floods and triggering widespread shallow landslides, this work presents a fruitful case study of operational weather radar application in shallow landslides early warning system.
Introduction
Shallow landslide early warning systems are normally implemented using rain gage network recording as input for rainfall values (Aleotti, 2004; Bosco et al., 2007; Guzzetti et al., 2007; Brunetti et al., 2010; Capparelli and Tiranti, 2010; Tiranti and Rabuffetti, 2010; Mercogliano et al., 2013; Rosi et al., 2015; Segoni et al., 2015, 2018; Devoli et al., 2018).
In the last decade, several researches focused on weather radar applications finalized to shallow landslide EWSs development (Chang et al., 2008; Chiang and Chang, 2009; Baum and Godt, 2010; Saito et al., 2010; Winter et al., 2010; Calvello et al., 2015; Jan and Chen, 2015; Nikolopoulos et al., 2015; Marra et al., 2016). In fact, the small size of the initiation catchments of debris flows, also caused by shallow landslides contribution, makes spatial resolution of observed precipitation the most relevant factor (Marra et al., 2017). Availability of high resolution observation of rainfall fields is thus mandatory in threshold-based EWSs. Especially in case of convective and localized precipitations, weather radar quantitative estimation of precipitations can overcome the poor representativeness of ground measurements, due to low density and geometry of the gage networks (Morrissey et al., 1995). Moreover, huge ground weather station networks may have longer latency of the data due to longer data transmission time. Weather radar typically scan the atmosphere close to the ground up to 150 km, with 1-km resolution or less, within few minutes. Both data resolution and short delay in data availability increase effectiveness of EWSs based weather radar.
Weather radar is thus successfully used in the real-time observations of rainfall triggering values for slope phenomena caused by short-duration and intense rainfalls, such as channelized debris flows (Borga et al., 1998; Tiranti et al., 2008, 2014; Marra et al., 2014; Berenguer et al., 2015); in fact, the distinguishing features of rainstorms are easier detected and characterized by weather radar than by rain gages. However, weather radar based QPEs in complex orography are affected by several errors and uncertainties residual clutter, partial beam blocking, rain-induced attenuation, variability of drop size distribution (DSD) and wet radome. Montopoli et al. (2017) provide a comprehensive review of limiting factors in QPEs in complex orography by ground weather radar. Due to nonuniform spatial distribution of errors, reliability of weather radar based EWSs in impervious areas or data quality of long-duration triggering rainfalls must be carefully evaluated. This paper discusses a successful operational implementation of weather radar utilization in shallow landslides EWSs in Piemonte. The areas examined in this study have good weather radar coverage and the performing weather radar data elaborating chain guarantees errors and uncertainties reduction in QPEs.
On November 2016, heavy rainfalls hit Piemonte in north-western Italy and triggered shallow landslides widely, mainly in the Alps. To estimate the rainfall parameters that triggered shallow landslides, rainfall-fields derived by weather radars observations merged with dense rain gage network data have been used. Based on higher number of shallow landslides occurred during the rainfall events, two study areas have been selected: the Pellice and Chisone Valleys and the Alto Tanaro catchments (Figure 1).
Materials and Methods
The Operational Weather Radar in Piemonte
Arpa Piemonte (the Regional Agency for Environmental Protection of Piemonte) operates two polarimetric Doppler C-band weather radar, located on the hill nearby Turin (Piemonte), installed on 1998, and in the Apennines near Savona (Liguria) installed on 2000. Table 1 summarizes the main characteristics of the weather radars.
Bric della Croce and Monte Settepani radars complete polarimetric volume scans, 170 km range, every 5 min, with, respectively, 11 and 8 elevations. Given the good visibility southward, Monte Settepani operates every 5 min an additional volume scan 250 km range by single polarization, composed of two elevations. A semisupervised robust hydrometeor classification is performed on polarimetric raw volumes (Bechini and Chandrasekar, 2015) to identify clutter, anaprop, attenuation, and meteorological echoes. Finally, completely free lowest beams are used to derive the product of surface rainfall intensity (SRI), according to beam visibility. During winter season, the radar reflectivity is later corrected for vertical profile reflectivity (VPR) according to Koistinen (1991). Single radar SRIs are merged in a 800 m resolution composite considering maximum reflectivity, where more than one weather radar observation is available. Additional weather radar data are merged to Piemonte composite, including the polarimetric weather radar in Monte Lema (Swiss) operated by MeteoSwiss, and weather radar in Carpiano (Italy) operated by the National Flight Assistance Company (ENAV).
Finally, composite radar reflectivity is converted in instantaneous rainfall intensity (mm/h) according to the well-known Z-R relationship:
where Z is the linear weather radar reflectivity expressed in mm/m3, R is rainfall intensity in mm/h and a = 300 and b = 1.5 are coefficients experimentally derived (Joss and Waldvogel, 1990). Further details on the operational weather radar operated by ARPA Piemonte can be found in Davini et al. (2011) and in Cremonini and Bechini (2010). Daily rainfall accumulations are corrected with tipping-bucket quality-controlled rain gage measurements by radar-gages assessment factors (Goudenhoofdt and Delobbe, 2009). On daily basis, the 24-h weather radar quantitative precipitation estimation (QPE) is compared with rain gages observations and for each ground weather station the following ratio is derived:
where Rx,y is the 24-h rainfall accumulation QPE co-located with the raingauge (Gx,y) placed at x,y coordinates. Only precipitation accumulations greater than 1.0 mm are considered and the outliers are also excluded and flagged as suspect ratios because they can be due to mechanical failure of raingauge or too large errors in weather radar QPE. Point assessment factors (AFs) are interpolated on the same weather radar composite grid using Ordinary Kriging method (Li and Heap, 2014) to derive the adjustment field. Finally, hourly weather radar QPEs are multiplied by assessment factor field to obtain the best unbiased hourly rainfall estimation.
Figure 2 shows the weather radar coverage in north-western Italy: stars indicate weather radar sites, circles the radar coverage and red polylines the two study basins of Pellice-Chisone and Alto Tanaro. Theoretical visibility maps, derived in standards propagation atmosphere, demonstrate that Piemonte, Lema and Carpiano weather radar have good visibility over target areas, being first completely free radar beams very close to the ground.
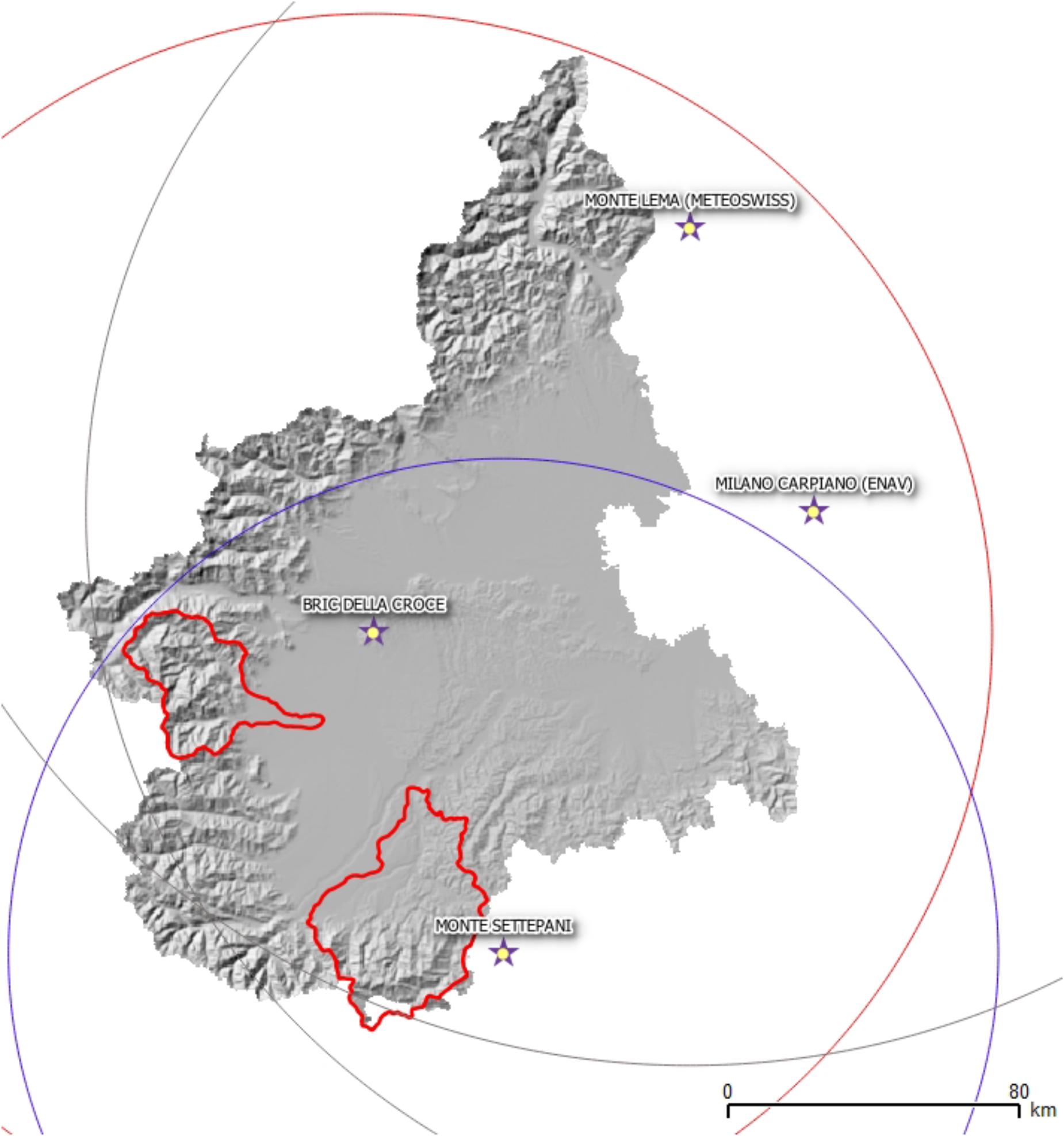
FIGURE 2. Weather radar coverage in north-western Italy. Star symbols show weather radar sites, circles volume scans coverage, polylines the target areas.
The Shallow Landslides EWS
On 2006, Tiranti and Rabuffetti (2010) developed a shallow landslide triggering model named “Shallow landslides Movements Announced through Rainfall Thresholds” (SMART) based on I-D empirical rainfall thresholds derived by statistical analysis on historical shallow landslides occurred in Piemonte from 1990 to 2006. SMART operates since 2008 both in forecast and monitoring modes and it is part of the regional warning system for natural hazards.
For shallow landslides, SMART recognizes two homogeneous areas (Figure 3), characterized by two different empirical thresholds (Equations 3 and 4) for two different environments (respectively, Alpine-Appennine and hylls), and defined on rainfall accumulation longer than 12 h. For shorter durations other slope phenomena can occur like diffused and accelerated soil erosion caused by run-off or channelized debris flows in the Alpine small catchments. Based on expected landslides density three levels of scenarios have been defined: (i) isolated landslides or soil erosion due to run-off; (ii) less than ten landslides per km2 expected; (iii) more than ten landslides per km2 expected.
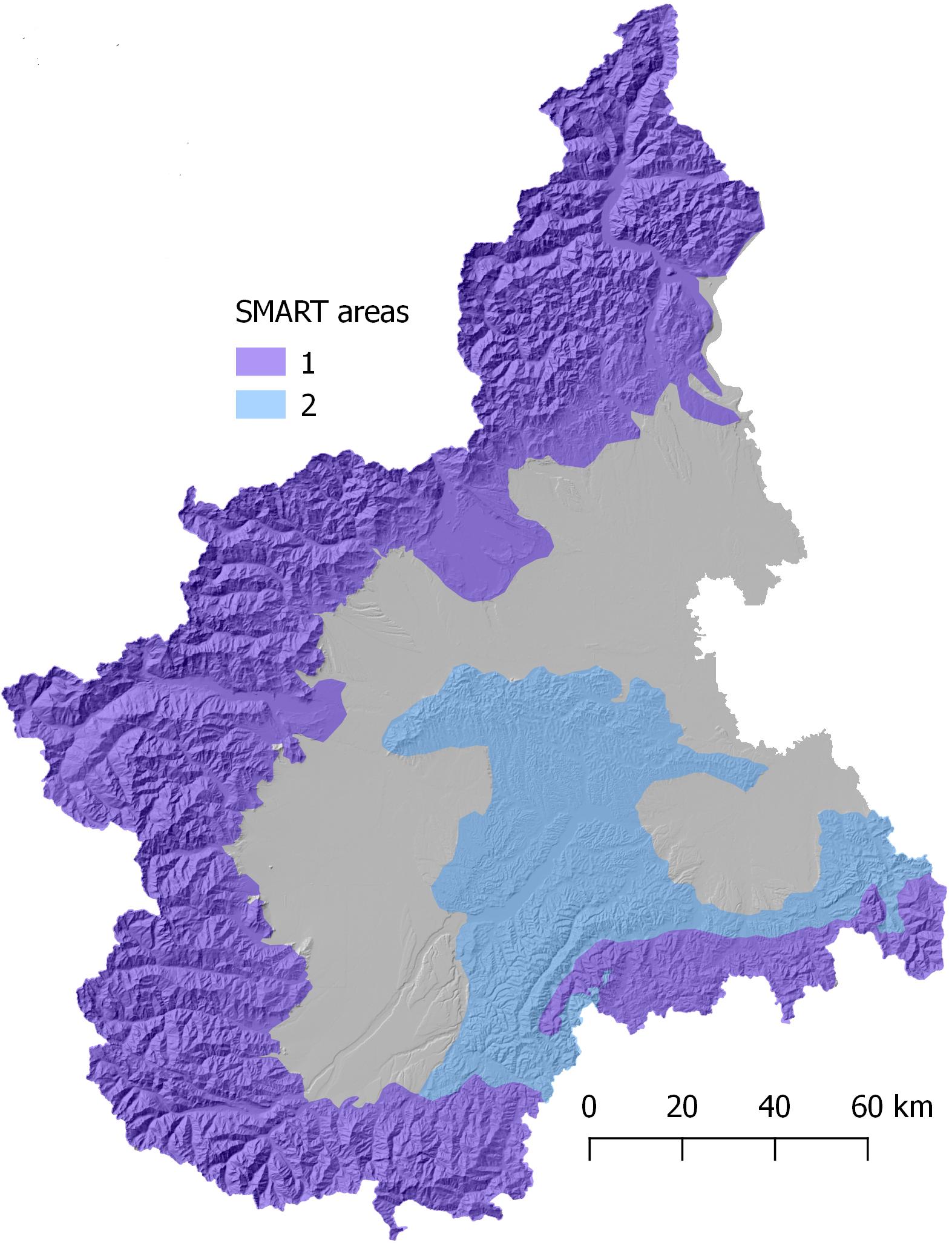
FIGURE 3. SMART homogeneous areas. Area 1: Alpine and Apennines environments characterized by metamorphic bedrock and high rainfall values to trigger shallow landslides; Area 2: hilly environment characterized by sedimentary bedrock and moderate rainfall values to trigger shallow landslides.
where l is the accumulated rainfall on D duration, the numerical coefficients are experimentally derived from statistical analysis of collected historical landslides and as exponent the Montana Coefficients (Boni and Parodi, 2001; Tiranti and Rabuffetti, 2010; Devoli et al., 2018) define rainfall effects according to the altitude.
The model rainfall inputs are the quantitative rainfall forecast and observed values by rain gage network, consisting in more than 400 weather stations distributed on about 10 km mesh grid for a total area of 25,402 km2. Thresholds are linked to each raingauge with an assigned buffer range of 5 km from rain gage centroid. For this reason, shallow landslides triggered at a distance > 5 km from rain gage are characterized by major uncertainty in forecasting. Recently, the weather radar observations have been introduced as new data input of SMART to fill this gap in ground network to identify triggering rainfall.
Results
Synoptic Forcing and Precipitations
From 21st to 25th November 2016, Piemonte was hit by heavy and persistent rainfalls, that have been initially localized in the southern areas, closed to the Ligurian Sea and then, they shifted to north-western Piemonte. On 21st November 2016 a deep trough approached western Europe, causing intense and humid south-westerly wind over the region. Low level wind convergence over Ligurian sea and diffuse atmospheric instability caused heavy localized precipitations over Apennines with more than 250 mm in 24 h. The following day, the low-pressure system isolated (cut-off), losing momentum, and becoming stationary over Iberian Peninsula. This atmospheric synoptic circulation caused intense stationary flow from Southeast over Piemonte, with heavy and diffuse precipitations. On 23rd and 24th November, low level flows turned from east, forcing heaviest rainfall in the western area of Piemonte. On 24th November rain gages recorded more than 350 mm in 24 h and, due to warm air advection, the snow level raised up to 2,000 m asl. Finally, on 25th November, the deep pressure system started to dissipate and precipitations over Piemonte progressively reduced. The largest rainfall amount recorded during 5 days was 632.6 mm; this accumulated precipitation corresponds to more than 50% mean annual rainfall in the region. The whole event was characterized by heavy convective rainfall, high spatial variability and peaks of more than 120 mm in 3 h. On 21st November, the snow level was located around 1500–1700 m asl and it increased on the following days, settling up to 1800–2000 m asl. On 24th November new cold air aloft caused a drop in the snow level up to 1400–1600 m asl, locally at lower altitude in the north-western areas of Piemonte. Rivers in the region had large increases in discharge recording maxima up to more than 50–100 years return period. About 150 shallow landslides were triggered, causing wide damages to residential buildings and infrastructures. These landslides were reported several days later after ground surveys by local authorities and Civil Protection. Their reports contain information about landslide type, location, reported damages but the triggering datetime of landslides is not available. Figure 4 shows rainfall accumulation from 21st to 25th November 2016 derived by weather radar observations and adjusted on daily basis with rain gages of meteorological ground network managed by Arpa Piemonte.
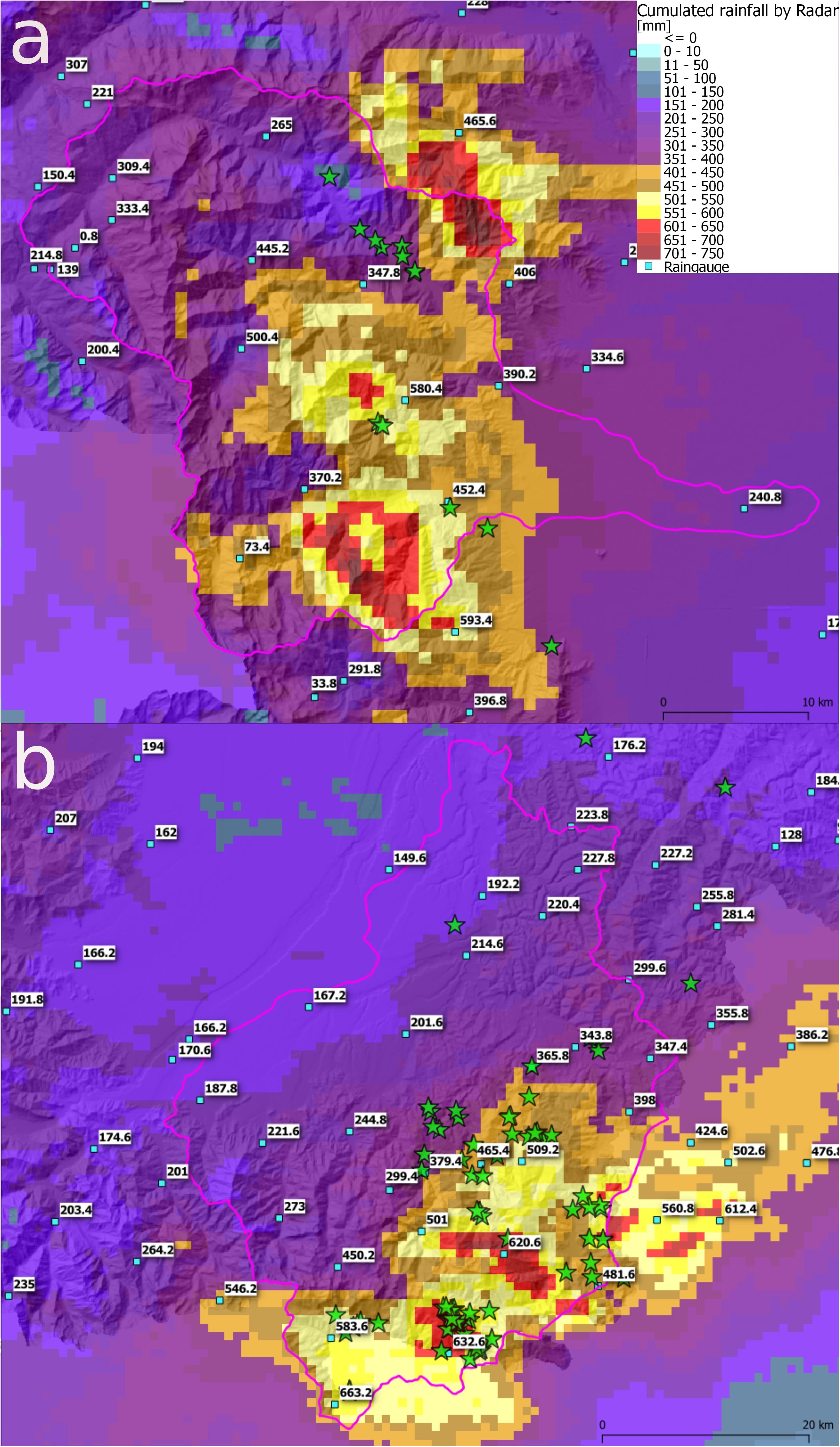
FIGURE 4. Total rainfall accumulation, estimated by weather radar (grid) and observed by rain gages (values) from 21st to 26th November 2016 in the study areas: (a) Pellice-Chisone; (b) Alto Tanaro.
Figure 4 demonstrates that the Piemonte raingauge network is not able to accurately describe the spatial variability of convective rainfall peaks, due to low spatial density. In Pellice-Chisone area the maximum rainfall accumulation recorded by rain gages over the whole period was 580.4 mm, meanwhile rainfall estimation by weather radar shows three peaks overpassing 700 mm.
An early warning system based trusting in rain gages observations would have probably failed where rainfall peaks are missed.
Rainfall Triggered Landslides
To evaluate the effectiveness of SMART thresholds in forecasting the triggering time of shallow landslides, the QPEs recorded during the event and derived by weather radar observations have been compared and filtered with the thresholds defined by Equation 3 in the Pellice-Chisone and Alto Tanaro mountain areas, where most landslides occurred (98 reported), and by Equation 4 for the hilly environment of Alto Tanaro (7 reported). It is possible to reconstruct the spatial-temporal evolution of phenomena, locating most prone areas to landslide triggering.
During night on 23rd November, in Alto Tanaro heavy rainfall started to overpass triggering thresholds. Figures 5a,b shows the accumulated rainfall estimated by weather radar from the beginning of event to 23rd November at 21:00 UTC, the green stars show the whole reported landslides after the rainfall event for which the triggering time is unknown. Figure 5b reports in red areas where thresholds were overpassed according to Equation 3. The yellow asterisk represents the raingauge that recorded an overpassing.
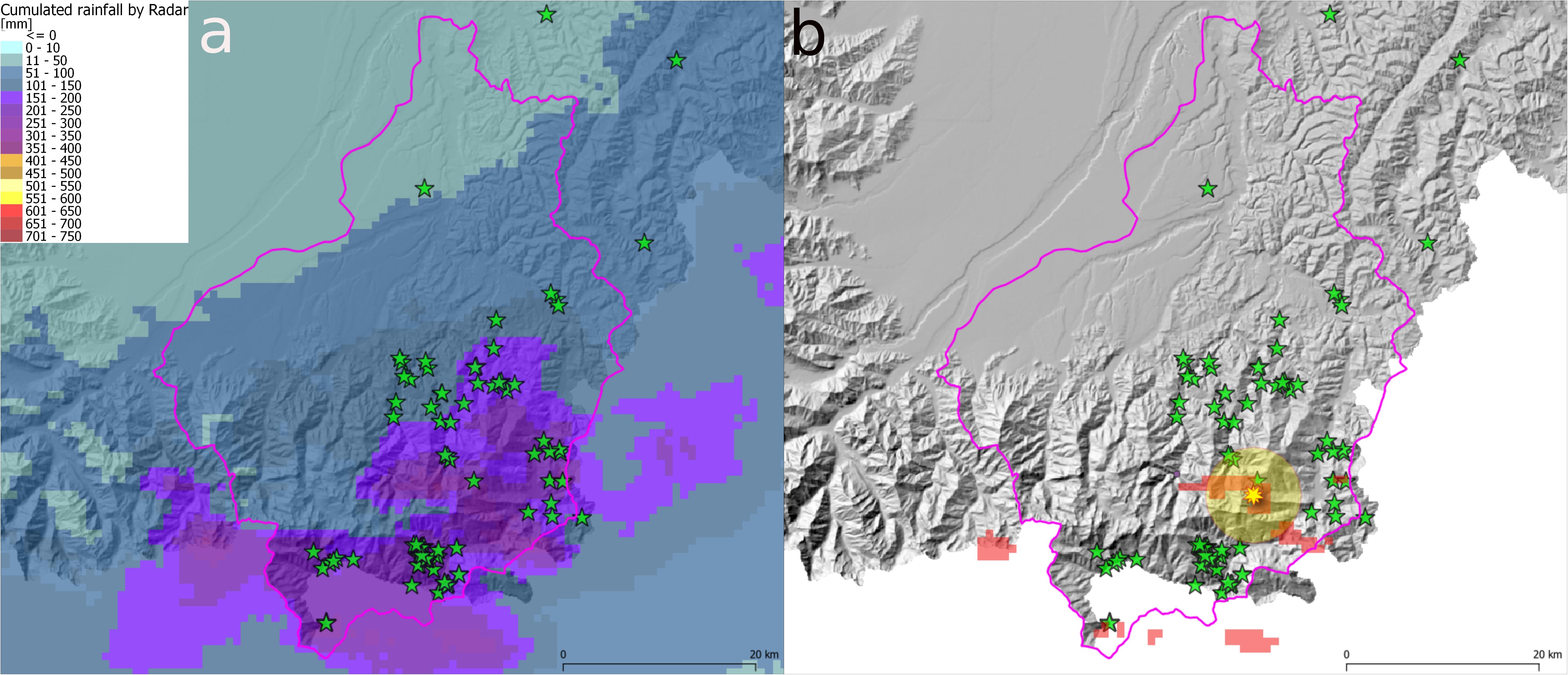
FIGURE 5. (a) Accumulated rainfall from 23rd November at 21:00 UTC in Alto Tanaro. (b) In red, areas interested by precipitations that overpassed thresholds of Equation 3 (critical rainfall accumulations) and rain gages overpassing threshold (yellow asterisks) and related buffers (yellow circles); green star labels indicate occurred landslides.
The critical rainfall interested wider areas in Alto Tanaro basin, causing an increase in landslides occurrence since 24th November 2016 at 03:00 UTC (Figures 6a,b). Only one raingauge issued a warning for threshold exceeding.
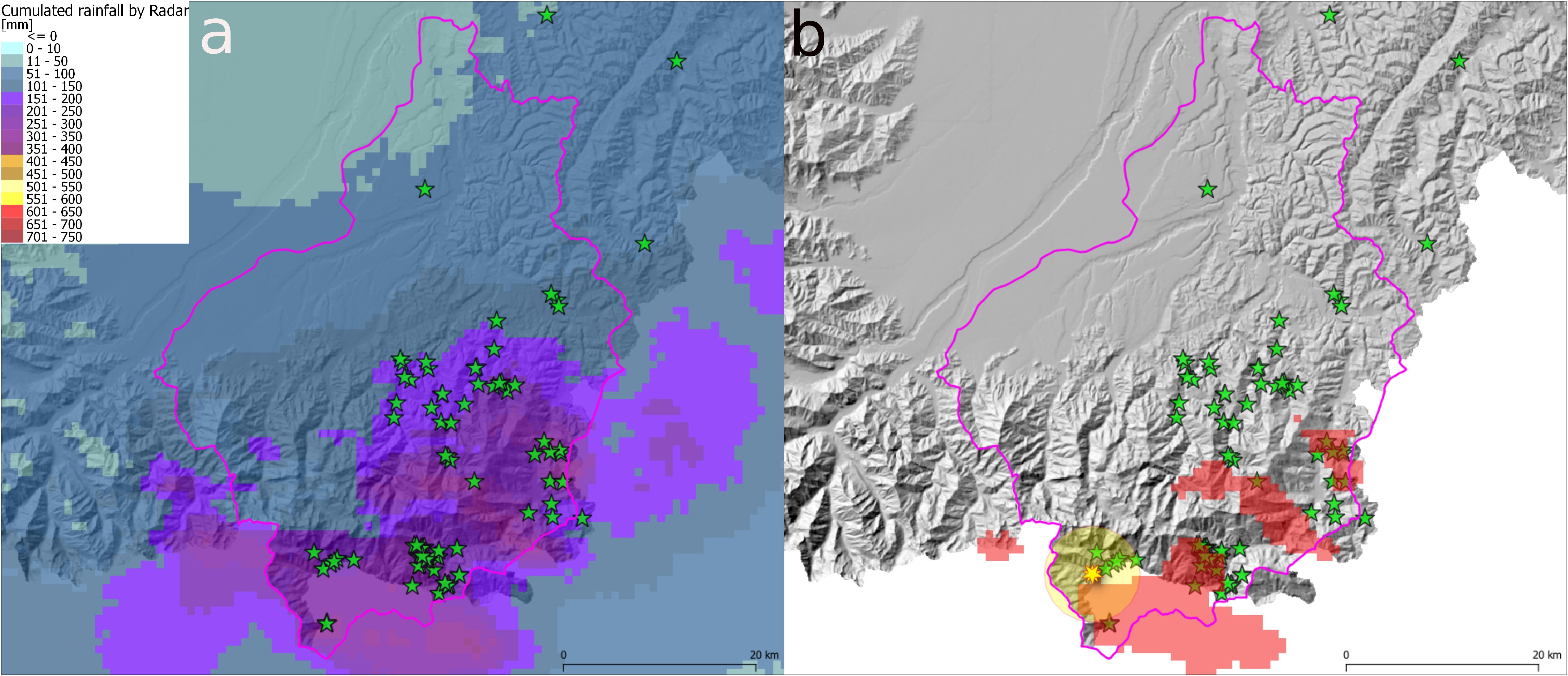
FIGURE 6. (a) Accumulated rainfall from 24th November at 03:00 UTC in Alto Tanaro. (b) In red, areas interested by precipitations that overpassed thresholds of Equation 2 (critical rainfall accumulations) and rain gages overpassing threshold (yellow asterisks) and related buffers (yellow circles); green star labels indicate occurred landslides.
In the Alto Tanaro, where the higher number of shallow landslides has been recorded, wider area interested by overpassing has been reached on 24th November at 15:00 UTC (Figures 7a,b). It is interesting noting that no raingauge recorded threshold exceedance at that time.
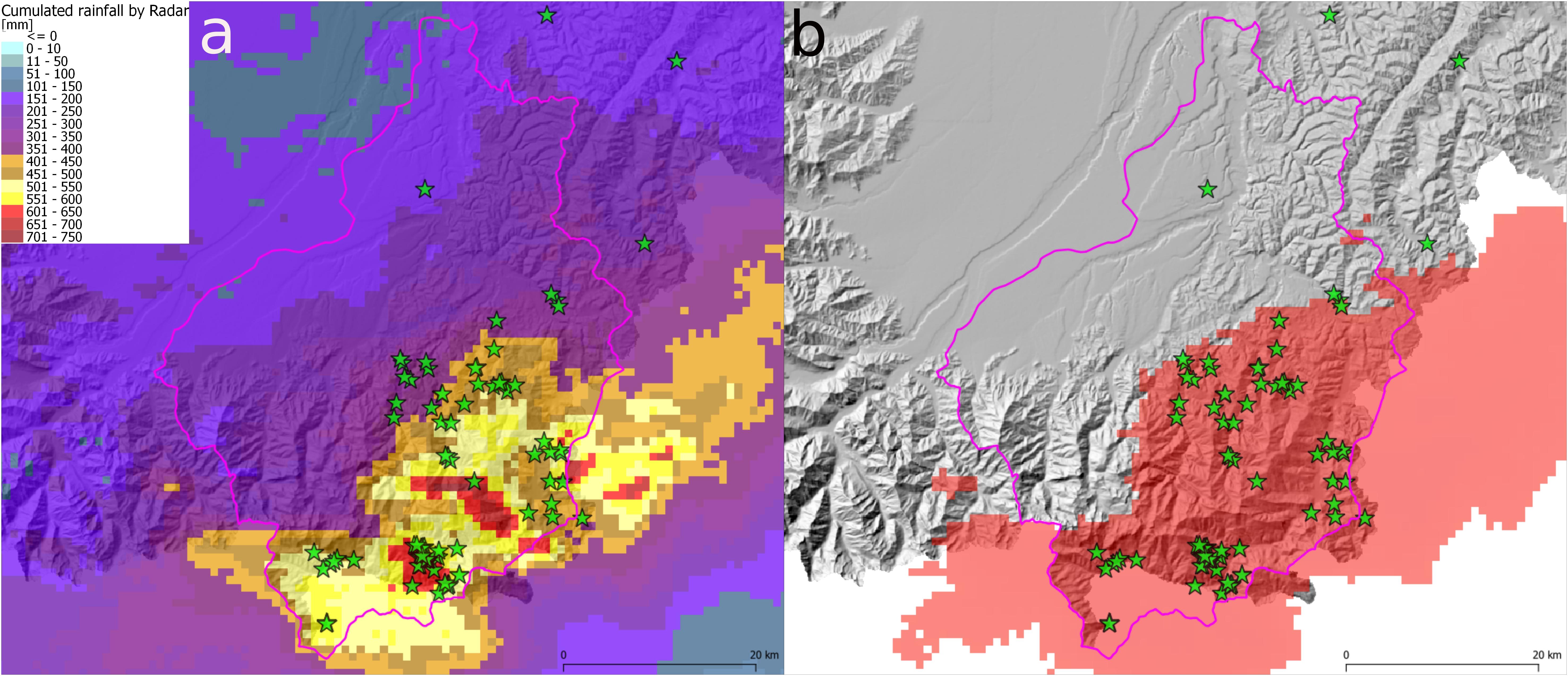
FIGURE 7. (a) Accumulated rainfall from 24th November at 15:00 UTC in Alto Tanaro. (b) In red, areas interested by precipitations that overpassed thresholds of Equation 4 (critical rainfall accumulations); green star labels indicate occurred landslides.
Some shallow landslides also occurred in hilly areas of Alto Tanaro. The same approach has been followed, applying the proper equation describing the behavior of intensity according to rainfall duration (Equation 4). Figure 8 shows that extent of rainfall critical values during the event.
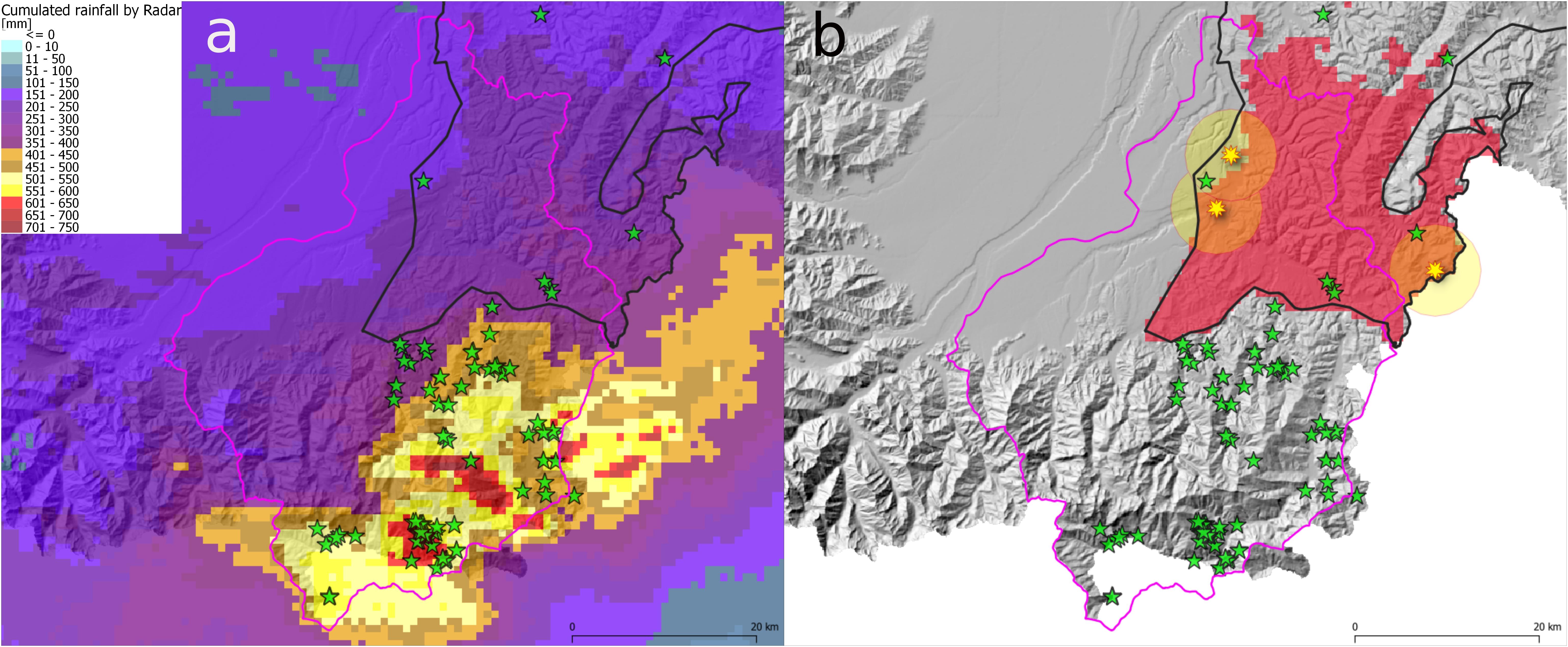
FIGURE 8. (a) Accumulated rainfall from 25th November at 06:00 UTC over hilly areas (delimited by black contour). (b) In red, areas interested by precipitations that overpassed thresholds of Equation 4 (critical rainfall accumulations) and rain gages overpassing threshold (yellow asterisks) and related buffers (yellow circles); green star labels indicate occurred landslides.
The total number of landslides in this hilly area has been seven but both rain gages and weather radar catched only five of them.
Over Pellice-Chisone the first critical rainfalls were reached on 24th November at 15:00 UTC (Figures 9a,b), also interesting the upper part of some catchments, where channelized debris flows were triggered due to the propagation of shallow landslides into channel network and observed in alluvial fans’ areas (stars in the red circle in Figure 9b). Three rain gages recorded threshold overpassing missing most of landslide sites.
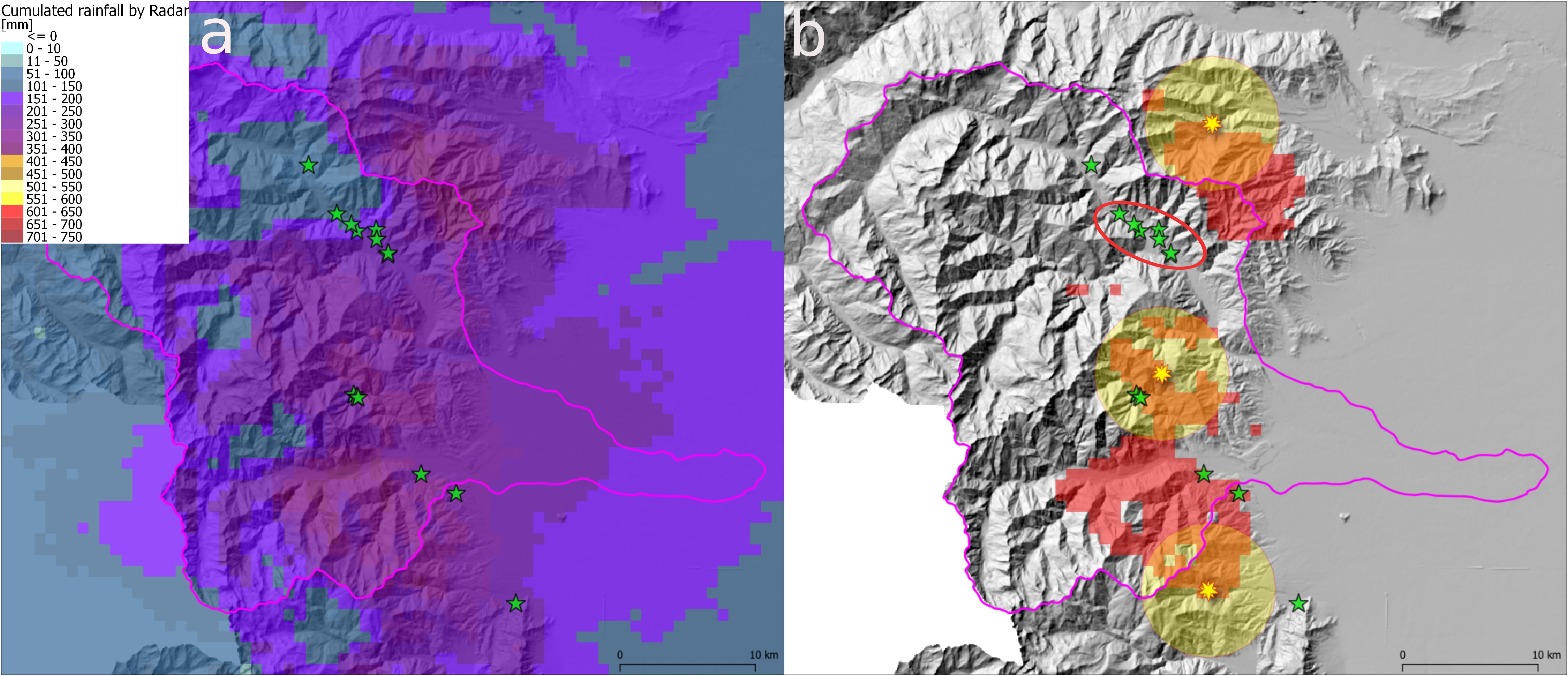
FIGURE 9. (a) Accumulated rainfall from 24th November at 15:00 UTC in Pellice-Chisone. (b) In red, areas interested by precipitations that overpassed thresholds of Equation 3 (critical rainfall accumulations) and rain gages overpassing threshold (yellow asterisks) and related buffers (yellow circles); green star labels indicate occurred landslides; red circle include reported debris flows that reached the alluvial fans’ areas generated from shallow landslides triggered at the basins’ head and propagated along channel networks.
The area of threshold exceeding reached maximum extent on 25th November 2016 at 04:00 UTC, and then rainfall intensities decreased until the end of event. Figure 10 shows reported landslides, the area of overpassing threshold according to weather radar QPEs, the rain gages and their datetime of overpassing threshold values.
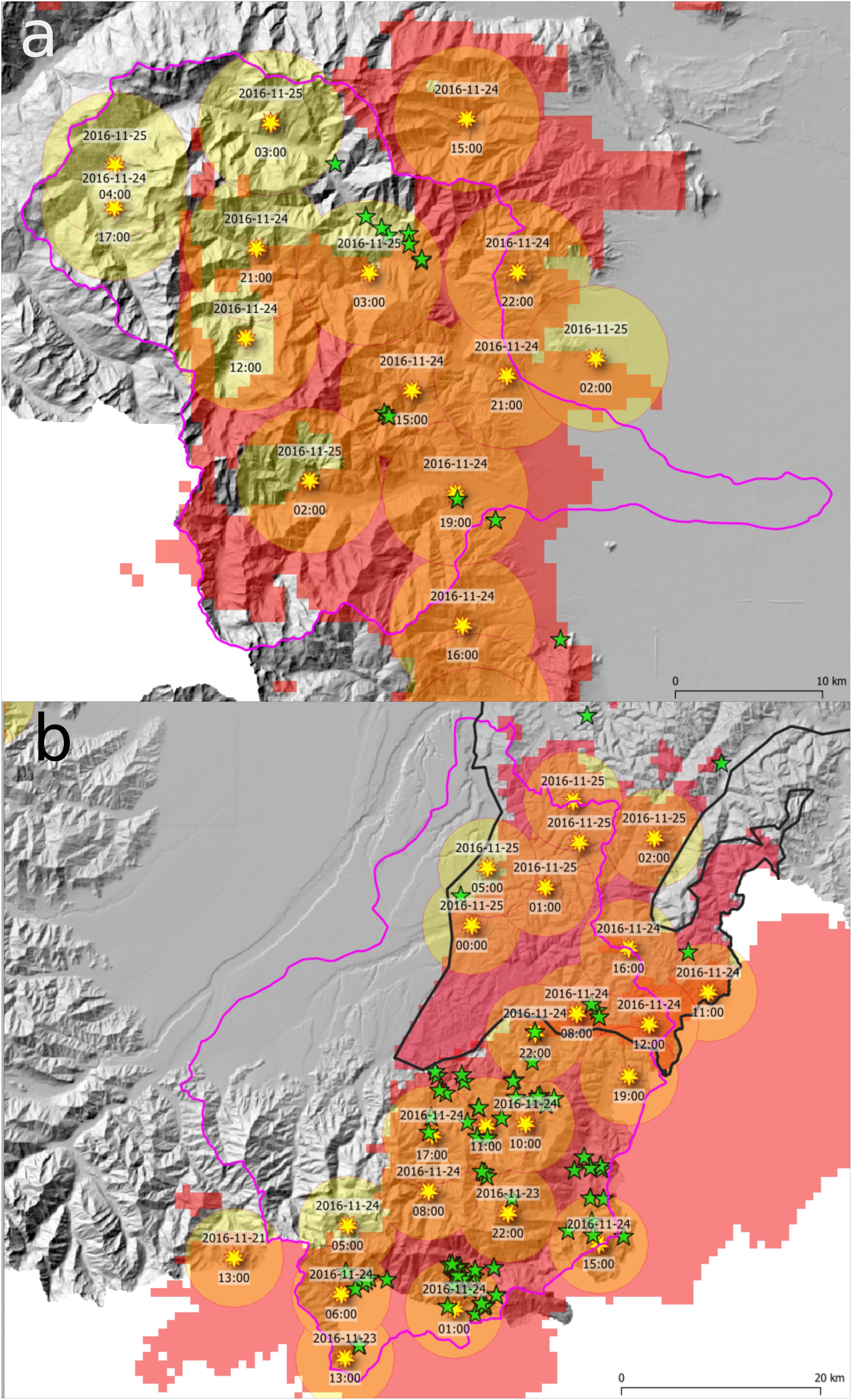
FIGURE 10. Rainfall accumulation over Pellice-Chisone (a) and Alto Tanaro (b) basins on 25th November 2016 at 04:00 UTC. In red, areas interested by precipitations that overpassed thresholds of Equation 4 and 4 (Alto Tanaro’s hilly environment delimited by black contour) from radar estimation, rain gages overpassing threshold (yellow asterisks) and related buffers (yellow circles) from the start of rainfall event to 25th November at 04:00 UTC; green star labels indicate all the reported landslides.
Discussion
Figures 5–9 show the evolution of severe rainfall event that hit Piemonte on November 2016. All shallow landslides in Alto Tanaro’s Alpine environment occurred between 400 and 1500 m asl. The four highest shallow landslides (1400–1500 m asl) have been checked with surrounding ground weather stations, assessing that no snow at ground was reported during the whole period. In Pellice-Chisone basin the seven landslides occurred between 500 and 1150 m asl, excluding a snow contribution in the phenomena triggering.
Given weather radar QPEs high spatial resolution (0.64 km2), the warning system is more effective in landslide triggering detection than the one exclusively based on rain gages. 14 landslides were reported in Pellice-Chisone basin of which 10 have been properly detected by weather radar, while only five ones were detected by rain gages (Figure 10a). In Alto Tanaro’s Alpine environment, 84 landslides correspond to proper detection by weather radar QPEs, while only 72 landslides were detected by rain gages, mainly due to low rain gages network density (Figure 10b). Nevertheless, five rain gages reported threshold exceeding corresponding to false alarms. In the hilly area, just five over seven landslides occurred were correctly detected by both radar QPEs and rain gages: this result suggests that a radar and rain gages integrated approach could have better performances in landslides triggering detection.
On 24th November 2014, temperature dropped and liquid precipitations turn to snowfall above 1200–1400 m asl in Pellice-Chisone basin. The following day, a sudden increase in temperature caused a new turn to liquid precipitation and melting of the snow accumulated over rain gages. Weather ground stations named Pragelato, Clot della Soma, Pra Catinat, Praly, and Massello (Figure 11) recorded rainfalls exceeding triggering threshold when heavy precipitation occurred but with a significant contribution due to snowmelting over the gage. These conditions determined false alarms in their surrounding area. It is worth noting that weather radar QPEs provided better estimations of the actual precipitation, correctly identifying that any threshold was not overpassed.
Conclusion
Heavy precipitation interested Piemonte (Italy) between 21st and 25th November 2016, causing floods and diffuse shallow landslides in Alto Tanaro and Pellice-Chisone basins. The illustrated case study shows that the operational use of QPEs, derived by merging weather radar observations and quality-controlled rain gages, is more reliable and effective in real-time determining a representative precipitation field responsible for triggering shallow landslides. The availability of QPEs with appropriate spatial and temporal resolution allowed characterizing the initiation of the torrential phenomena (debris flow) occurred in Pellice-Chisone basin by the observation of evolution of critical rainfalls occurred over the head of basins, where the mass transport was located. In Alto Tanaro basin weather radar based QPEs identified correctly the triggered shallow landslides distribution.
Furthermore, the widespread nature of triggers, which occurred during November 2016 heavy rainfalls, confirmed the effectiveness of the pre-announcement model of shallow landslides developed and operated by the Arpa Piemonte. The subsequent use of the distribution of critical rainfall values has finally allowed reconstructing with a good degree of reliability the timing of landslides triggering.
Given a long-time weather radar QPE dataset, a possible next development will be to derive weather radar based I-D thresholds and to compare them with gages-based ones. Although, weather radar based quantitative precipitation forecast (QPF) is not currently implemented into the Arpa Piemonte operational EWS, the real-time forecast of landslides is possible by making use of the time lag between slope failure and rainfall peak, as demonstrated during November 2016 heavy rainfall. A further development will consist in the application of weather radars QPFs to foresee the shallow landslides initiation one or 2 h ahead, improving the effectiveness of warnings.
Author Contributions
RC provide weather radar data and analysis and developed algorithm for QPEs. DT developed shallow landslide early warning system.
Conflict of Interest Statement
The authors declare that the research was conducted in the absence of any commercial or financial relationships that could be construed as a potential conflict of interest.
References
Aleotti, P. (2004). A warning system for rainfall-induced shallow failures. Eng. Geol. 73, 247–265. doi: 10.1016/j.enggeo.2004.01.007
Baum, R. L., and Godt, J. W. (2010). Early warning of rainfall-induced shallow landslides and debris flows in the USA. Landslides 7, 259–272. doi: 10.1007/s10346-009-0177-0
Bechini, R., and Chandrasekar, V. (2015). A semisupervised robust hydrometeor classification method for dual-polarization radar applications. J. Atmos. Ocean. Technol. 32, 22–47. doi: 10.1175/JTECH-D-14-00097.1
Berenguer, M., Sempere-Torres, D., and Hürlimann, M. (2015). Debris flow forecasting at regional scale by combining susceptibility mapping and radar rainfall. Nat. Hazards Earth Syst. Sci. 15, 587–602. doi: 10.5194/nhess-15-587-2015
Boni, G., and Parodi, A. (2001). Sintesi pluviometrica regionale: realizzazione di un atlante delle piogge intense sulle Alpi franco-italiane. Rapporto Finale, Progetto INTERREG II Italia-Francia. Azione 3, 61–80.
Borga, M., Dalla Fontana, G. D., Da Ros, D., and Marchi, L. (1998). Shallow landslide hazard assessment using a physically based model and digital elevation model. Environ. Geol. 35, 81–88.
Bosco, F., Campus, S., Mensio, L., Nicolò, G., and Tiranti, D. (2007). “Forecasting landslides -,” in Evaluation and Prevention of Natural Risks, Vol. 470, eds S. Campus, S. Barbero, S. Bovo, and F. Forlati (Amsterdam: Balkema), 353–390.
Brunetti, M. T., Peruccacci, S., Rossi, M., Luciani, S., Valigi, D., and Guzzetti, F. (2010). Rainfall thresholds for the possible occurrence of landslides in Italy. Nat. Hazards Earth Syst. Sci. 10, 447–458. doi: 10.5194/nhess-10-447-2010
Calvello, M., d’Orsi, R. N., Piciullo, L., Paes, N., Magalhaes, M. A., and Lacerda, W. A. (2015). The Rio de Janeiro early warning system for rainfall-induced landslides: analysis of performance for the years 2010–2013. Int. J. Disast. Risk Reduc. 12, 3–15. doi: 10.1016/j.ijdrr.2014.10.005
Capparelli, G., and Tiranti, D. (2010). Application of the MoniFLaIR early warning system for rainfall-induced landslides in the Piedmont region (Italy). Landslides 7, 401–410. doi: 10.1007/s10346-009-0189-9
Chang, K. T., Chiang, S. H., and Lei, F. (2008). Analysing the relationship between typhoon-triggered landslides and critical rainfall conditions. Earth Surf. Process. Landf. 33, 1261–1271. doi: 10.1002/esp.1611
Chiang, S. H., and Chang, K.-T. (2009). Application of radar data to modeling rainfall-induced landslides. Geomorphology 103, 299–309. doi: 10.1016/j.geomorph.2008.06.012
Cremonini, R., and Bechini, R. (2010). Heavy rainfall monitoring by polarimetric c-band weather radars. Water 2, 838–848. doi: 10.3390/w2040838
Davini, P., Bechini, R., Cremonini, R., and Cassardo, C. (2011). Radar-based analysis of convective storms over Northwestern Italy. Atmosphere 3, 33–58. doi: 10.3390/atmos3010033
Devoli, G., Tiranti, D., Cremonini, R., Sund, M., and Bøje, S. (2018). Comparison of landslide forecasting services in Piemonte (Italy) and in Norway, illustrated by events in late spring 2013. Nat. Hazards Earth Syst. Sci. 18, 1351–1372. doi: 10.5194/nhess-18-1351-2018
Goudenhoofdt, E., and Delobbe, L. (2009). Evaluation of radar-gauge merging methods for quantitative precipitation estimates. Hydrol. Earth Syst. Sci. 13, 195–203. doi: 10.5194/hess-13-195-2009
Guzzetti, F., Peruccacci, S., Rossi, M., and Stark, C. P. (2007). Rainfall thresholds for the initiation of landslides in central and southern Europe. Meteorol. Atmos. Phys. 98, 239–267. doi: 10.1007/s00703-007-0262-7
Jan, C. D., and Chen, C. L. (2015). “Debris flows caused by Typhoon Herb in Taiwan,” in Debris Flow Hazards and Related Phenomena, eds M. Jakob and O. Hungr (Berlin: Springer), 363–385.
Joss, J., and Waldvogel, A. (1990). “Precipitation measurement and hydrology,” in Radar in Meteorology: Battan Memorial and 40th Anniversary Radar Meteorology Conference, ed. D. Atlas (Boston, MA: American Meteorological Society), 577–606.
Koistinen, J. (1991). “Operational correction of radar rainfall errors due to the vertical reflectivity profile,” in Proceedings 25th International Conference on Radar Meteorology, (Providence, RI: AMS), 91–94. doi: 10.1175/2010JHM1201.1
Li, J., and Heap, A. D. (2014). Spatial interpolation methods applied in the environmental sciences: a review. Environ. Model. Softw. 53, 173–189. doi: 10.1016/j.envsoft.2013.12.008
Marra, F., Destro, E., Nikolopoulos, E. I., Zoccatelli, D., Dominique, J., Creutin, F. G., et al. (2017). Impact of rainfall spatial aggregation on the identification of debris flow occurrence thresholds. Hydrol. Earth Syst. Sci. 21, 4525–4532.
Marra, F., Nikolopoulos, E. I., Creutin, J. D., and Borga, M. (2014). Radar rainfall estimation for the identification of debris-flow occurrence thresholds. J. Hydrol. 519, 1607–1619. doi: 10.1016/j.jhydrol.2014.09.039
Marra, F., Nikolopoulos, E. I., Creutin, J. D., and Borga, M. (2016). Space–time organization of debris flows-triggering rainfall and its effect on the identification of the rainfall threshold relationship. J. Hydrol. 541, 246–255. doi: 10.1016/j.jhydrol.2015.10.010
Mercogliano, P., Segoni, S., Rossi, G., Sikorsky, B., Tofani, V., Schiano, P., et al. (2013). Brief communication “A prototype forecasting chain for rainfall induced shallow landslides”. Nat. Hazards Earth Syst. Sci. 13, 771–777. doi: 10.5194/nhess-13-771-2013
Montopoli, M., Roberto, N., Adirosi, E., Gorgucci, E., and Baldini, L. (2017). Investigation of weather radar quantitative precipitation estimation methodologies in complex orography. Atmosphere 8:34.
Morrissey, M. L., Maliekal, J. A., Greene, J. S., and Wang, J. (1995). The uncertainty of simple spatial averages using rain gauge networks. Water Resour. Res. 31, 2011–2017. doi: 10.1029/95WR01232
Nikolopoulos, E. I., Borga, M., Creutin, J. D., and Marra, F. (2015). Estimation of debris flow triggering rainfall: influence of rain gauge density and interpolation methods. Geomorphology 243, 40–50. doi: 10.1016/j.geomorph.2015.04.028
Rosi, A., Lagomarsino, D., Rossi, G., Segoni, S., Battistini, A., and Casagli, N. (2015). Updating EWS rainfall thresholds for the triggering of landslides. Nat. Hazards 78, 297–308. doi: 10.1007/s11069-015-1717-7
Saito, H., Nakayama, D., and Matsuyama, H. (2010). Relationship between the initiation of a shallow landslide and rainfall intensity—duration thresholds in Japan. Geomorphology 118, 167–175. doi: 10.1016/j.geomorph.2009.12.016
Segoni, S., Battistini, A., Rossi, G., Rosi, A., Lagomarsino, D., Catani, F., et al. (2015). Technical note: an operational landslide early warning system at regional scale based ospace–time–variable rainfall thresholds. Nat. Hazards Earth Syst. Sci. 15, 853–861. doi: 10.5194/nhess-15-853-2015
Segoni, S., Piciullo, L., and Gariano, S. L. (2018). A review of the recent literature on rainfall thresholds for landslide occurrence. Landslides 15, 1483–1501. doi: 10.1007/s10346-018-0966-4
Tiranti, D., Bonetto, S., and Mandrone, G. (2008). Quantitative basin characterization to refine debris-flow triggering criteria and processes: an example from the Italian Western Alps. Landslides 5, 45–57. doi: 10.1007/s10346-007-0101-4
Tiranti, D., Cremonini, R., Marco, F., Gaeta, A. R., and Barbero, S. (2014). The defense (DEbris Flows triggEred by storms - Nowcasting SystEm): an early warning system for torrential processes by radar storm tracking using a Geographic Information System (GIS). Comput. Geosci. 70, 96–109. doi: 10.1016/j.cageo.2014.05.004
Tiranti, D., and Rabuffetti, D. (2010). Estimation of rainfall thresholds triggering shallow landslides for an operational warning system implementation. Landslides 7, 471–481. doi: 10.1007/s10346-010-0198-8
Keywords: slope processes, early warning system, QPE, remote sensing, heavy rainfall
Citation: Cremonini R and Tiranti D (2018) The Weather Radar Observations Applied to Shallow Landslides Prediction: A Case Study From North-Western Italy. Front. Earth Sci. 6:134. doi: 10.3389/feart.2018.00134
Received: 16 March 2018; Accepted: 17 August 2018;
Published: 03 September 2018.
Edited by:
Steven L. Forman, Baylor University, United StatesReviewed by:
Stefano Luigi Gariano, Istituto di Ricerca per la Protezione Idrogeologica (IRPI), ItalyMilad Janalipour, K. N. Toosi University of Technology, Iran
Samuele Segoni, Università degli Studi di Firenze, Italy
Copyright © 2018 Cremonini and Tiranti. This is an open-access article distributed under the terms of the Creative Commons Attribution License (CC BY). The use, distribution or reproduction in other forums is permitted, provided the original author(s) and the copyright owner(s) are credited and that the original publication in this journal is cited, in accordance with accepted academic practice. No use, distribution or reproduction is permitted which does not comply with these terms.
*Correspondence: Roberto Cremonini, ci5jcmVtb25pbmlAYXJwYS5waWVtb250ZS5pdA==