- Department of Anatomy and Cell Biology, Oklahoma State University Center for Health Sciences, Tulsa, OK, United States
Annually, tetrapod vertebrate bone apposition is briefly interrupted, which typically coincides with the yearly environmental nadir. The temporary appositional hiatus is histologically recorded in bone cortex as a hypermineralized ring termed a line of arrested growth (LAG). One paleohistology study used this known LAG periodicity to quantify ontogenetic ages and growth rates in a dataset of fifty tibiae from the hadrosaurid dinosaur Maiasaura. In addition to LAGs, unusual cortical rings resulting from localized changes in vascular diameter were also noted in the tibiae, but not investigated further. The fifty Maiasaura tibiae from that study were histologically reexamined here to explore and document the composition, frequency, and cortical extent of localized vascular changes (LVCs) to assist with the recognition of similar structures in the cortices of other extinct taxa. This assessment reveals that the Maiasaura LVC is comprised of one or more laminae of primary osteons forming a ring of vascular canal diameters either uniformly larger or smaller than those of primary osteons adjacent to the vascular ring. With one exception, LVCs are only found prior to the first LAG in Maiasaura. However, LVC frequency is variable, ranging from specimens with no LVCs to as many as five prior to the first LAG. Because of their inconsistent frequency and restricted location within the cortex, LVCs likely do not have an annual periodicity in Maiasaura. Instead, LVCs may signal times of temporary but repeated stress. As both growth rates and mortality rates were highest during the first year in Maiasaura, illness or starvation seemed to have the greatest impact on growth during that time. A subadult tibia presenting with a pathologic directional cortical outgrowth is the only instance within the sample in which LVCs were found beyond the first LAG. Unfortunately, LVCs are under-explored in extant taxa, so the underlying cause(s) for Maiasaura LVCs cannot be established. As LVCs may provide yet another histological method for interpreting extinct vertebrate biology, foundational investigations of LVCs in extant vertebrates should be pursued.
Introduction
The hadrosaurid dinosaur Maiasaura peeblesorum was first described in 1979, from skeletal remains of nestling and adult individuals found within sediments of the Campanian Two Medicine Formation of Montana (Horner and Makela, 1979). Those fossils provided the first evidence that at least some dinosaur taxa nested in colonies and supplied parental care to their altricial young, which attracted considerable scientific interest (Horner and Makela, 1979; Barreto, 1997; Horner et al., 2001). The subsequent discovery and ongoing excavation of a 2 km2 monodominant (Schmitt et al., 2014) Maiasaura fossil horizon (Museum of the Rockies localities TM-003, TM-151, TM-158) geographically near the 1979 nesting locality resulted in the curation of over 1,000 skeletal elements from nearly every ontogenetic stage between nestling and adult (Horner et al., 2000; Woodward et al., 2015). By using this large ontogenetic sample, Maiasaura biology has been assessed in detail over the past 40 years (e.g., Horner and Makela, 1979; Barreto, 1997; Dilkes, 2000; Horner et al., 2000; Adams and Organ, 2005). Woodward et al. (2015) incorporated data from earlier Maiasaura studies into a population osteohistology synthesis utilizing 50 Maiasaura tibiae. The study produced statistically robust ontogenetic growth curves and permitted inferences on population structure, survivorship, and sexual maturity, making Maiasaura the most ontogenetically well-understood extinct vertebrate taxon to date (Woodward et al., 2015).
The bone microstructure analysis of Woodward et al. (2015) found that lines of arrested growth (LAGs) were absent from the majority of tibiae in the sample (n = 31). At high magnification, a LAG in Maiasaura tibiae appears as a continuous, thin black line adjacent to primary tissue laminae within the bone cortex (see Figure 4 from Woodward et al., 2015). The histology of extant vertebrates demonstrates that a LAG, much like a tree ring, is formed annually when growth temporarily ceases. In extant vertebrates, the growth hiatus typically coincides with the annual nadir, and the hiatus occurs regardless of metabolic rate, elevation, latitude, or captivity status (e.g., Peabody, 1961; Castanet et al., 1993, 2004; Woodward et al., 2011; Köhler et al., 2012).
The Maiasaura tibiae without LAGs in the Woodward et al. (2015) study were consistently the smallest within the sample (tibia lengths 32–48.8 cm), leading the authors to hypothesize that those individuals died before they were a year of age (or more accurately, before their first nadir). Larger tibiae in the sample (tibia lengths 56–99.7 cm) possessed between two and 9 LAGs, supporting the hypothesis that the small tibiae without LAGs were from young of the year. Although LAGs were not observed in the small tibiae between 32 and 48.8 cm in length, regions of cortex with cortical rings were visible at low magnifications, but the rings became difficult to distinguish from the surrounding bone laminae at higher magnifications (see Figure 5 from Woodward et al., 2015). Woodward et al. (2015) described the rings as “formed by a localized change in vascular canal size,” and therefore not true LAGs. As the focus of Woodward et al. (2015) was primarily on quantifying ontogenetic change in Maiasaura, these localized vascular rings were not discussed further.
Reexamination of the Maiasaura tibiae from Woodward et al. (2015) reveals that the localized vascular changes (abbreviated here as “LVCs”) are a common occurrence. The aim of this report is to describe LVCs in detail, including their composition, location about the cortex, and frequency within the ontogenetic sample of 50 tibiae, with a discussion of possible physiologic implications. The presence and extent of LVCs in extant vertebrates is largely unexplored, but the description and discussion of LVCs here will provide a basis for comparison should similar structures be observed in other tetrapods, extant or extinct.
Materials and Methods
All Maiasaura tibiae used in Woodward et al. (2015) are curated at the Museum of the Rockies (Bozeman, MT). The LVCs within the primary cortices of Maiasaura tibiae are not obvious at high magnifications and restricted fields of view associated with direct viewing of thin section slides. The LVCs are most easily observed in full transverse section digital composites at low magnification. Digital composites were previously produced for each thin section made for the study by Woodward et al. (2015), which are accessible through the Museum of the Rockies (MOR; Bozeman, MT, United States) or upon request from the senior author. The composite images were made using a Nikon Optiphot-Pol polarizing microscope with a Nikon DS Fi1 camera, Prior automated stage, and NIS Elements: BR software. For the present study, each of the fifty tibia digital composites was assessed for LVCs prior to the first LAG and within zones between each consecutive LAG. The presence and number of LVCs was recorded if one or more cortical rings were visible within the primary cortex when (1) the composite images were viewed in Photoshop CC at the “fit screen” (0% “zoom”) resolution, and (2) the structures did not fit the structural description of a LAG (Table 1). Once identified, Photoshop zoom was increased to 33% or higher in regions containing the LVCs, and the number and composition of LVCs was recorded (Table 1). Tibiae used in Woodward et al. (2015) and reexamined here are numbered T1 through T50.
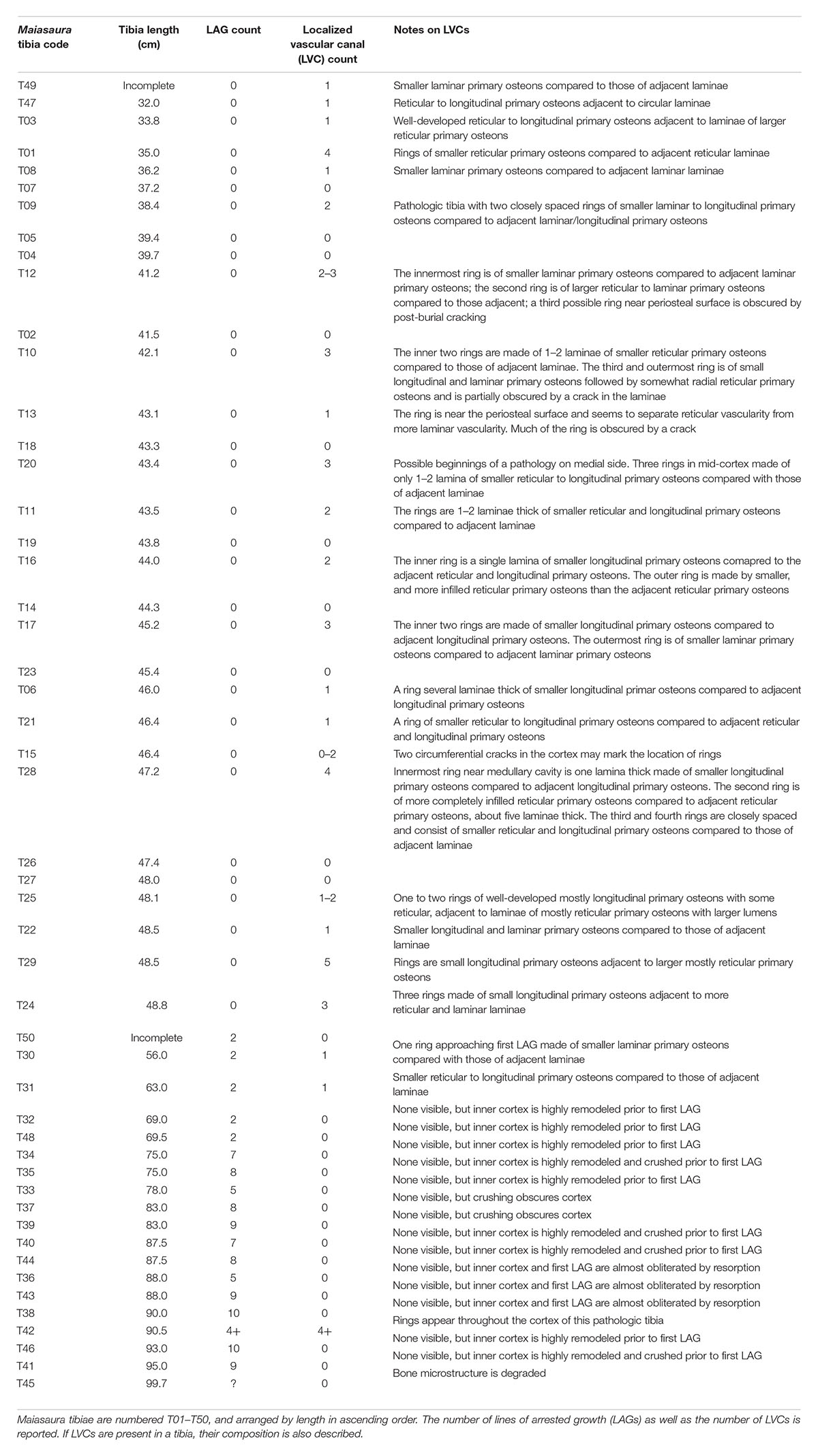
Table 1. Documentation of Maiasaura tibia parameters relevant to this localized vascular change (LVC) assessment.
Results
With just a single exception, LVCs were only observed within the cortices of small tibiae with no LAGs (presumed to be from individuals less than a year of age), or within the region of cortex prior to the first LAG in tibiae with multiple LAGs (Table 1). In the tibiae with no LAGs, the number of LVCs varied between none (n = 10), to between one and five (n = 20) (Table 1). The presence and number of LVCs prior to the first LAG in larger tibiae was often difficult to ascertain, as the cortex prior to the first LAG was frequently either partially or almost completely remodeled.
The primary tissue organization within regions of LVC remains woven; LVCs result not from a change in tissue organization, but from a change in vascular canal diameter within one or more subsequent laminae of primary osteons, relative to the vascular canal size of adjacent laminae. At low digital zoom, this localized change in vascular canal diameter appears as a discernible ring within the primary cortex. When the image is enlarged, ring composition is observed as either: (1) a region of primary osteons with larger vascular canals adjacent primary osteons with smaller vascular canals, but with the same vascular orientation (n = 1; Figures 1A,B); (2) a region of primary osteons with smaller vascular canals adjacent primary osteons with larger vascular canals, but with the same vascular orientation (n = 17; Figures 1C,D); or (3) a region of primary osteons with vascular canal orientation that differs from the adjacent primary osteons (n = 4; Figures 1E–G). In each case, the LVC ring can be as thin as a single lamina, or many laminae thick. Vascular canal orientation within the cortices and LVCs included reticular, laminar, longitudinal, and circular (least frequently).
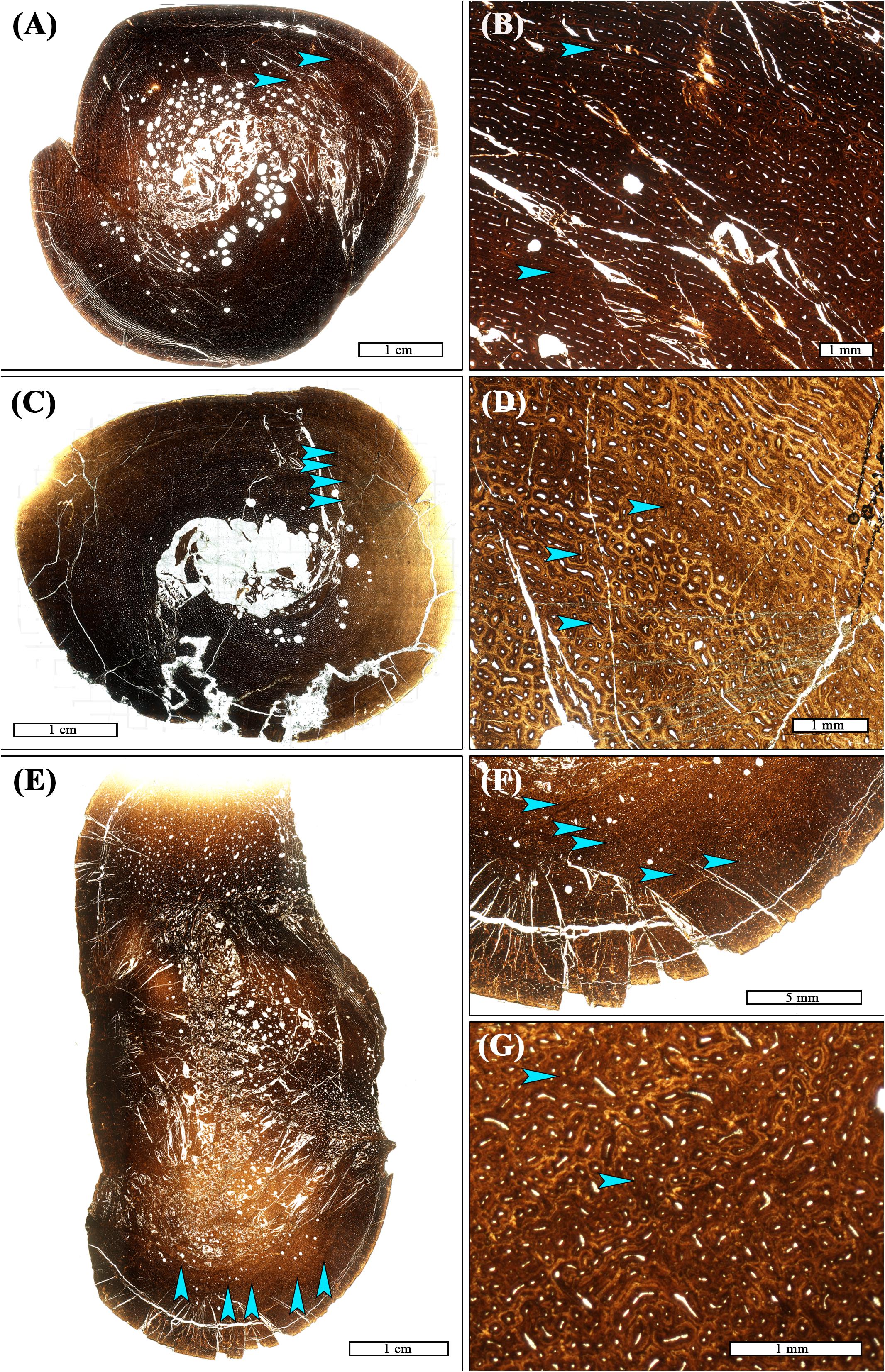
Figure 1. Transverse thin sections of Maiasaura tibiae from young of the year showing variation in frequency, spacing, and composition of localized vascular changes (LVCs). (A) There are 2–3 LVCs in the tibia T12. Two are located mid-cortex (arrows), and there may have been a third in the outer cortex but it is now obscured by post-burial fragmentation of the cortex. (B) Magnification of the cortex of T12 showing the two mid-cortex LVCs (arrows). The innermost LVC is made of smaller laminar primary osteons compared to adjacent laminar primary osteons. The second LVC is of larger reticular to laminar primary osteons compared to those adjacent (C) Four LVCs (arrows) are closely spaced within the mid-cortex of tibia T01. (D) Magnification of the T01 cortex shows that all four LVCs (arrows) are made of smaller reticular primary osteons compared to adjacent reticular laminae. (E) LVCs were most numerous in tibia T29, with a total of five (arrows). (F) Magnified view of T29, with arrows highlighting location of LVCs. (G) Magnification of the cortex of T29 showing the second and third LVC. They are comprised of small longitudinal primary osteons located adjacent to larger, mostly reticular, primary osteons. All images are shown in plane polarized light.
Some of the small tibiae (length <50 cm) with no LAGs had a concentric crack in the primary tissue laminae close to the periosteal surface (e.g., Figure 1A). There may have been an LVC on this crack, as larger tibiae with more than one LAG sometimes have an LVC just before the first LAG. LVCs were only found beyond the first LAG in a pathologic tibia [T42; see Cubo et al. (2015) for discussion of pathology] with at least four LAGs (Figure 2). In this particular tibia, at least four diffuse LVCs were found throughout the cortex.
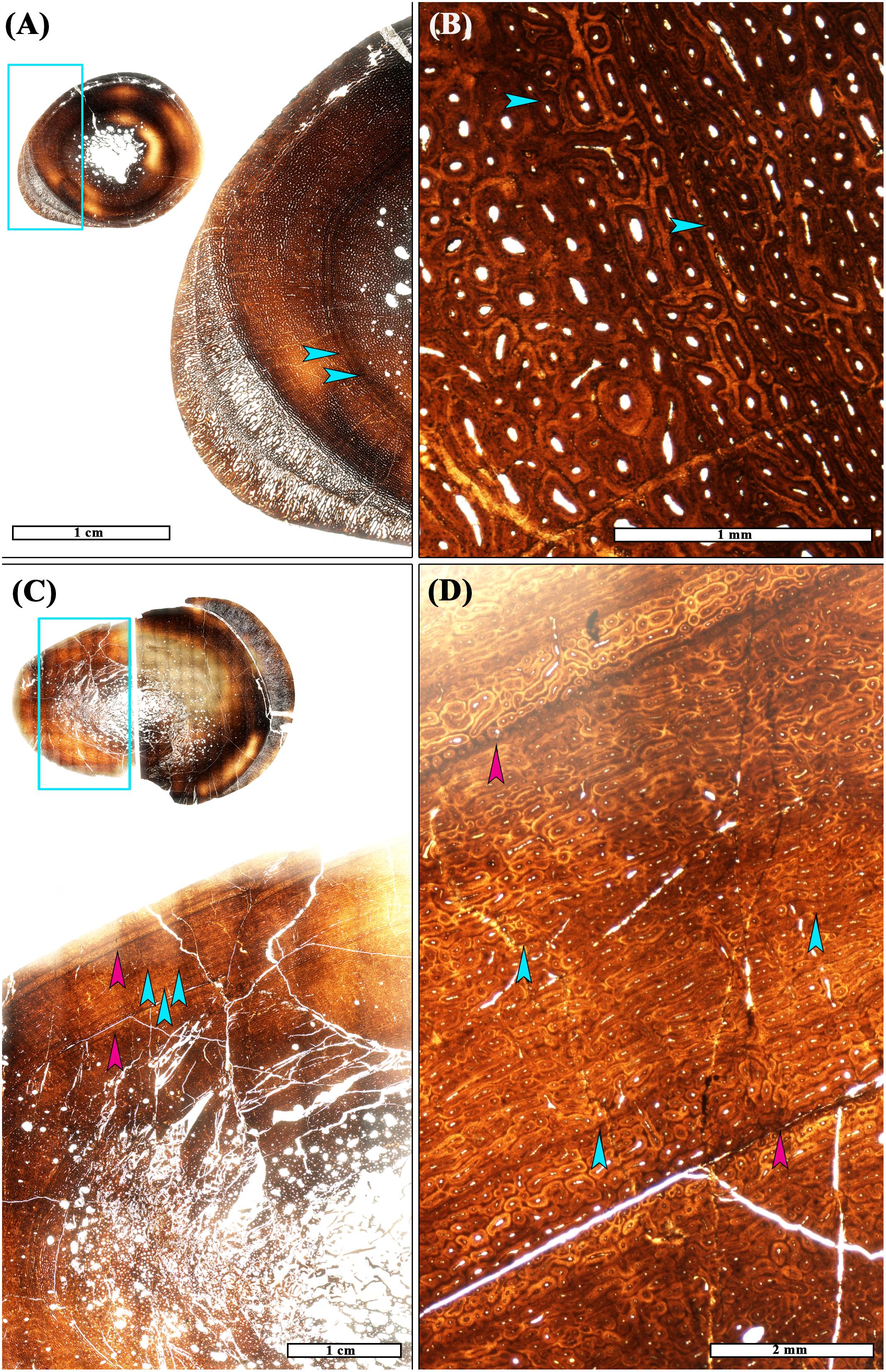
Figure 2. Two Maiasaura tibiae from the fifty tibia dataset show histological evidence of pathology in the form of directional radial outgrowths in transverse section, and both possess LVCs. (A) Small tibia T09 (inset) does not have lines of arrested growth (LAGs), but there are two pronounced LVCs visible within the mid-cortex (arrows). The blue box on the inset image indicates the enlarged region in the panel. (B) Magnification of T09 shows the LVCs to be made of smaller laminar to longitudinal primary osteons compared to adjacent laminar and longitudinal primary osteons. (C) Subadult-size tibia T42 (inset) possesses at least four LAGs (two are shown with pink arrows) and LVCs are observed throughout the cortex (the three most evident are shown with blue arrows). The blue box on the inset image indicates the enlarged region in the panel. (D) Magnification of cortical region showing three LVCs (blue arrows) in a zone of growth bounded by LAGs (pink arrows). These LVCs are made of smaller reticular to longitudinal primary osteons compared to those of adjacent laminae. All images are shown in plane polarized light.
Discussion
In their histologic assessment of Maiasaura tibiae, Woodward et al. (2015) remarked on unusual cortical rings resembling annually formed LAGs visible at low magnification (or low digital zoom). Upon further inspection using higher magnification objectives as well as higher digital image enlargement, Woodward et al. (2015) discovered the rings resulted from a localized (i.e., temporary) change in the vascular canal diameter of primary osteons. The morphology of LVCs was investigated in greater detail here. With a single exception (T42), LVCs were only observed in primary cortex formed prior to the first LAG. However, the number of LVCs was variable between individuals, ranging between zero and five. The composition of LVCs was also variable: they were either formed from a single lamina or were many laminae thick, consisting of either smaller vascular canals surrounded by larger vascular canals, or vice versa.
It is unclear whether slide thickness affects the number of observed LVCs. There may be a preferred slide thickness for identifying LVCs, and some may have been overlooked. However, when Maiasaura tibia slides are polished to an appropriate thickness for viewing other bone microstructures such as tissue organization or LAGs, LVCs were consistently observed prior to the first LAG and none thereafter. Therefore, while polishing a slide too thinly (or not thin enough) may affect the number of LVCs that can be recognized, slide thickness cannot explain the presence of LVCs prior to the first LAG but their absence thereafter in the same slide. Thus, the presence of LVCs only prior to the first LAG is a real phenomenon.
In addition to LVCs, Woodward et al. (2015) remarked on another unusual vascular pattern that occurred in tibiae but only after the second LAG. In the primary tissue zone following the second LAG, and within every zone thereafter, primary osteon vascular orientation is reticular, and transitions to sub-plexiform or circular for the remainder of the zone (see Figure 4 in Woodward et al., 2015). This vascular transition then repeats within consecutive zones. The vascular change from reticular to circular is so abrupt that it is observable at low magnifications (or low digital zoom) as a definite boundary between reticular and circular primary osteons. However, this kind of repeating vascular change differs from the LVCs observed prior to the first LAG in several respects. Within each zone after the second LAG, the vascular organization predictably changes from reticular to circular or sub-plexiform, and there is no reversion back to reticular prior to the LAG separating the consecutive cortical zones. In contrast, the cortical rings observed prior to the first LAG are localized, with the same vascular canal orientation and size to either side of the LVC. Because the frequency, extent, and cause of LVCs in extant vertebrates is currently uninvestigated, implications for their presence in Maiasaura tibiae is unclear. Several possible explanations for their occurrence are explored here.
An LVC may be comparable to an annulus, another ring-like structure associated with the growth hiatus. An annulus forms when apposition rate decreases appreciably, rather than ceasing altogether as is the case for LAGs. Annuli are recognized as diffuse bands of fairly avascular laminae, which are often parallel-fibered (Huttenlocker et al., 2013). These structures were not observed by Woodward et al. (2015) in the Maiasaura tibia sample. The rich vascular network of primary osteons within a woven-fibered matrix observed throughout the cortex in Maiasaura tibiae implies elevated growth rates similar to those observed in extant mammals and birds (Woodward et al., 2015). In addition, the vascular organization indicates the tibia growth rate of Maiasaura was highest in the juveniles, when LVCs were frequent (Woodward et al., 2015). Because the annual growth hiatus is likely a plesiomorphic characteristic of tetrapod growth (Köhler et al., 2012), LVCs may be comparable to annuli in that they result from annual decreases in the growth rate of an extremely fast growing vertebrate taxon.
A similar hypothesis was proposed to explain the absence of annual LAGs in the limb bones of sauropod dinosaurs: polish lines (Sander, 2000) are rings observed in the cortices of sauropod limb bones when using reflected light on polished transverse bone surfaces. Polish lines are hypothesized as annual occurrences appositional pauses or decreases in sauropods that may have been growing too quickly to form true LAGs (Sander, 2000). Vascular cycles are another histological feature reported in sauropod skeletal elements (de Ricqlès, 1983; Curry, 1999). Cycles [also referred to as modulations by Sander (2000)] are observed in transmitted, rather than reflected, light. They appear as rings within the cortex comprised of either smaller or larger vascular canals, relative to vascular canal diameters in laminae adjacent to the rings. Cycles were noted by Curry (1999) to consist of vascular arrangements differing from the vascular orientation in laminae adjacent to the cycle. Like polish lines, cycles are hypothesized to form annually in the rapidly growing sauropod appendicular skeleton and to indicate an annually occurring slight decrease in growth rate (Curry, 1999).
In appearance, LVCs in Maiasaura most closely resemble the vascular cycles figured by Curry (1999). However, the LVCs observed in Maiasaura are likely not equivalent to polish lines or cycles for two reasons: (1) LVCs are observable in the transmitted light of thin sections, whereas polish lines are identified using reflected light (Sander, 2000); (2) polish lines and cycles are present from inner to outer cortex and at various stages of ontogeny with predictable spacing (de Ricqlès, 1983; Curry, 1999; Sander, 2000). In contrast, LVCs appear with random frequency within and across individuals and are only present prior to the first LAG (with a single exception, discussed below).
If LVCs are yet another kind of cyclical growth mark formed with annual periodicity, the resulting individual variation in Maiasuara body size for a given age would be considerable. For instance, tibia T24, with a length of 48.8 cm, has three LVCs and no LAGs. If LVCs occur annually, this individual would be larger at 3 years than tibia T29, which has five LVCs and a tibia length of 48.5 cm. Another tibia (T22) is the same length as T29 and has only a single LVC. Extreme developmental plasticity is not unheard of for tetrapods. For instance, in archosaurs, substantial body size differences have been reported for same-age individuals in the Triassic dinosaur Plateosaurus (Sander and Klein, 2005), and farm-raised Alligator is documented to take 18 months to reach body lengths similar to 5 year-old wild counterparts (Coulson et al., 1973; de Ricqlès, 1983; Elsey et al., 1992). However, age in Plateosaurus was determined from counting LAGs, which do have a known periodicity of 1 year, and annual growth in crocodylians is also reliably documented histologically by the presence of LAGs (Peabody, 1961; Hutton, 1986). While it may turn out that the periodicity of LVCs is also annual, this hypothesis has yet to be validated with extant vertebrates. Because of this, and because of the inconsistency in the presence and frequency of LVCs in Maiasaura tibia cortices, LVCs should not be interpreted as annual in Maiasaura at present. With this approach, the tibiae T22, T24, and T29 from the aforementioned example would be from young of the year that were nearly the same body size, which presently the more realistic interpretation.
Instead of occurring annually, another possibility is that the LVCs correspond to episodic stresses such as an illness or starvation temporarily affecting growth rate. This may explain why LVCs are typically restricted to the first year of growth, as Woodward et al. (2015) demonstrated this was the period in which ontogenetic growth rate was highest (see Table 1 in Woodward et al., 2015), and also had the highest mortality rates. Young of the year may have experienced temporary physiologic stress if they encountered difficulty procuring nourishment or fell ill. On the other hand, in an LVC from individual T12 the vascular canal diameter was generally larger than those in adjacent laminae, and it is possible that this particular LVC corresponds to a brief apposition rate increase in response to favorable conditions.
Out of the 50 Maiasaura tibia sample, LVCs were only observed beyond the first LAG in T42, a pathologic tibia presenting a directional outgrowth of radial tissue at the periosteal surface (Figure 2). A similar directional radial outgrowth was also observed in a smaller tibia (T9) with no LAGs (i.e., young of the year) (Figure 2). Within this smaller tibia, its two LVCs are especially pronounced. While there may be a physiological connection between pronounced or cortically extensive LVCs and directional outgrowths, the reason for this apparent association (if any) is unclear at present. Ultimately, only investigations into the underlying cause(s) of LVCs in extant vertebrates will permit testing of the presented hypotheses.
Conclusion
The cortices of Maiasaura tibiae possess both LAGs and LVCs, with the latter occurring almost exclusively prior to the first LAG. While the utility of LAGs as skeletochronological indicators in fossil bone is firmly validated with studies on extant vertebrates, the same cannot be said of LVCs. Because LVCs may provide yet another tool for interpreting vertebrate biology from bone microstructure, foundational investigations with extant taxa are necessary. In the meantime, identification and description of LVCs in other extinct vertebrate taxa may refine the hypotheses proposed here. Until the cause(s) and possible periodicity of LVCs is established from extant vertebrates, the most conservative course of action at present is to utilize only LAGs and annuli (when present) in skeletochronological and annual growth rate investigations of Maiasaura.
Data Availability
All datasets generated for this study are included in the manuscript and/or the supplementary files.
Author Contributions
HW conceived the project, acquired data, interpreted data, and wrote the manuscript.
Conflict of Interest Statement
The author declares that the research was conducted in the absence of any commercial or financial relationships that could be construed as a potential conflict of interest.
Acknowledgments
The Museum of the Rockies (Bozeman, MT) provided access to Maiasaura tibia thin section slides and digital images. Thanks to Tom Ballard for helpful discussion. Comments from two reviewers helped to improve the manuscript.
References
Adams, J. S., and Organ, C. L. (2005). Histologic determination of ontogenetic patterns and processes in hadrosaurian ossified tendons. J. Vertebr. Paleontol. 25, 614–622. doi: 10.1671/0272-4634(2005)025[0614:HDOOPA]2.0.CO;2
Barreto, C. (1997). “Dinosaur growth plates and dinosaur bone growth,” in Dinofest International: Proceedings of a Symposium Held at Arizona State University, eds D. L. Wolberd, E. Stump, and G. D. Rosenberg (Philadelphia, PA: The Academy of Natural Sciences), 95–100.
Castanet, J., Croci, S., Aujard, F., Perret, M., Cubo, J., and Margerie, E. D. (2004). Lines of arrested growth in bone and age estimation in a small primate: Microcebus murinus. J. Zool. Lond. 263, 31–39. doi: 10.1017/S0952836904004844
Castanet, J., Francillon-Vieillot, H., Meunier, P. J., and Ricqlès, A. D. (1993). “Bone and individual aging,” in Bone, ed. B. K. Hall (London: CRC Press), 245–283.
Coulson, T. D., Coulson, R. A., and Hernandez, T. (1973). Some observations on the growth of captive alligators. Zoologica 58, 47–52.
Cubo, J., Woodward, H., Wolff, E., and Horner, J. (2015). First reported cases of biomechanically adaptive bone modeling in non-avian dinosaurs. PLoS One 10:e0131131. doi: 10.1371/journal.pone.0131131
Curry, K. A. (1999). Ontogenetic histology of Apatosaurus (Dinosauria: Sauropoda): new insights on growth rates and longevity. J. Vertebr. Paleontol. 19, 654–665. doi: 10.1080/02724634.1999.10011179
de Ricqlès, A. (1983). Cyclical growth in the long limb bones of a sauropod dinosaur. Acta Palaeontol. Pol. 28, 225–232.
Dilkes, D. W. (2000). Appendicular myology of the hadrosaurian dinosaur Maiasaura peeblesorum from the late cretaceous (Campanian) of Montana. Trans. R. Soc. Edinb. Earth Sci. 90, 87–125. doi: 10.1017/S0263593300007185
Elsey, R. M., Joanen, T., Mcnease, L., and Kinler, N. (1992). Growth rates and body condition factors of Alligator mississippiensis in coastal Louisiana wetlands: a comparison of wild and farm-released juveniles. Comp. Biochem. Physiol. 103, 667–672. doi: 10.1016/0300-9629(92)90164-L
Horner, J. R., de Ricqlès, A., and Padian, K. (2000). Long bone histology of the hadrosaurid dinosaur Maiasaura peeblesorum: growth dynamics and physiology based on an ontogenetic series of skeletal elements. J. Vertebr. Paleontol. 20, 115–129. doi: 10.1671/0272-4634(2000)020[0115:LBHOTH]2.0.CO;2
Horner, J. R., and Makela, R. (1979). Nest of juveniles provides evidence of family structure among dinosaurs. Nature 282, 296–298. doi: 10.1038/282296a0
Horner, J. R., Padian, K., and Ricqlès, A. D. (2001). Comparative osteohistology of some embryonic and perinatal archosaours: developmental and behavioral implications for dinosaurs. Paleobiology 27, 39–58. doi: 10.1666/0094-8373(2001)027<0039:COOSEA>2.0.CO;2
Huttenlocker, A., Woodward, H. N., and Hall, B. K. (2013). “Chapter 2: biology of bone,” in Bone Histology of Fossil Tetrapods: Advancing Methods, Analysis, and Interpretation, eds K. Padian and E.-T. Lamm (Berkeley: University of California Press), 13–34.
Hutton, J. M. (1986). Age determination of living Nile crocodiles from the cortical stratification of bone. Copeia 2, 332–341. doi: 10.2307/1444994
Köhler, M., Marin-Moratalla, N., Jordana, X., and Aanes, R. (2012). Seasonal bone growth and physiology in endotherms shed light on dinosaur physiology. Nature 487, 358–361. doi: 10.1038/nature11264
Peabody, F. E. (1961). Annual growth zones in living and fossil vertebrates. J. Morphol. 108, 11–62. doi: 10.1002/jmor.1051080103
Sander, P. M. (2000). Longbone histology of the Tendaguru sauropods: implications for growth and biology. Paleobiology 26, 466–488. doi: 10.1666/0094-8373 (2000)026<0466:LHOTTS>2.0.CO;2
Sander, P. M., and Klein, N. (2005). Developmental plasticity in the life history of a prosauropod dinosaur. Science 310, 1800–1802. doi: 10.1126/science.1120125
Schmitt, J. G., Jackson, F. D., and Hanna, R. R. (2014). “Debris flow origin of an unusual late Cretaceous hadrosaur bonebed in the Two medicine formation of western Montana,” in Hadrosaurs, eds D. Eberth and D. Evans (Bloomington: Indiana Press), 486–501.
Woodward, H. N., Freedman, E. A., Farlow, J. O., and Horner, J. R. (2015). Maiasaura, a model organism for extinct vertebrate population biology: a large sample statistical assessment of growth dynamics and survivorship. Paleobiology 41, 503–527. doi: 10.1017/pab.2015.19
Keywords: histology, dinosaur, growth rings, ontogeny, Maiasaura, skeletochronology
Citation: Woodward HN (2019) Maiasaura (Dinosauria: Hadrosauridae) Tibia Osteohistology Reveals Non-annual Cortical Vascular Rings in Young of the Year. Front. Earth Sci. 7:50. doi: 10.3389/feart.2019.00050
Received: 22 January 2019; Accepted: 04 March 2019;
Published: 20 March 2019.
Edited by:
Michel Laurin, UMR7207 Centre de Recherche sur la Paléobiodiversité et les Paléoenvironnements (CR2P), FranceReviewed by:
John Robert Horner, Chapman University, United StatesAurore Canoville, University of Cape Town, South Africa
Copyright © 2019 Woodward. This is an open-access article distributed under the terms of the Creative Commons Attribution License (CC BY). The use, distribution or reproduction in other forums is permitted, provided the original author(s) and the copyright owner(s) are credited and that the original publication in this journal is cited, in accordance with accepted academic practice. No use, distribution or reproduction is permitted which does not comply with these terms.
*Correspondence: Holly N. Woodward, aG9sbHkubi53b29kd2FyZEBnbWFpbC5jb20=; aG9sbHkuYmFsbGFyZEBva3N0YXRlLmVkdQ==