- 1Institute of Tibetan Plateau Research, Chinese Academy of Sciences, Beijing, China
- 2CAS Center for Excellence in Tibetan Plateau Earth Sciences, Chinese Academy of Sciences, Beijing, China
- 3University of Chinese Academy of Sciences, Beijing, China
- 4Institute of Atmospheric Physics, Chinese Academy of Sciences, Beijing, China
Lakes have important influence on local temperature and precipitation and are also regulators of regional climate. Using satellite and climate reanalysis datasets and a regional weather research forecasting (WRF) model, we carried out two WRF sensitivity experiments: one including the lake (WRF_lake) and the other with lake replaced by land surface (WRF_nolake). The comparison between the two sensitivity WRF experiments revealed that, in summer, the lake was strong heat sink in the daytime and heat source at night. In autumn, the lake was weak heat sink in the daytime and strong heat source at night. Correspondingly, the precipitation was reduced by about 45–70% over the lake because of the cooling effect in summer of 2008, and the precipitation over the lake and to the east of the lake was enhanced by 60% because of the warming effect of 2008.
Introduction
The landscape of the Tibetan Plateau (TP) is characterized by extremely complex topography. The local climate varies greatly. Lake represents a significant feature of the surface because its thermal inertia has a large potential impact on the climate (Bonan, 1995; Laird et al., 2009; Xu et al., 2009; Zhou et al., 2015; Kirillin et al., 2017; Wang et al., 2018; Zhan et al., 2019). The lake system of the TP is also closely linked to local climate (Haginoya et al., 2009; Tsujimoto and Koike, 2013; Huang et al., 2017; Wang et al., 2017; Song et al., 2019). The contribution of lake effects to local or regional climate is becoming more and more important. Due to the lake-air temperature difference in different seasons, there are different seasonal distribution characteristics of lake effect precipitation. To understand the seasonal characteristics of lake effect precipitation in the lake area is conducive to further understanding the regional water cycle characteristics.
Seasonal contributions of lake effects have attracted broad research attention given their dramatic impacts on local precipitation. In summer, the precipitation in Lake Ladoga and Great Lakes reduces 20–70% of convective precipitation because of lake effect (Scott and Huff, 1996; Samuelsson et al., 2010). In winter, the lake effect increases the precipitation. For example, Lake Ladoga in Russia can increase convective precipitation by up to 20–40% (Samuelsson et al., 2010). The Great Lakes in the United States, which are unfrozen and induces large lake effect precipitation (more than 100%) in the winter (Umek and Gohm, 2016; Welsh et al., 2016). The effect is different from Ngoring Lake and Gyaring Lake on the TP in summer. Both of these two lakes cause an increase of precipitation in early summer (Wen et al., 2015). The increase precipitation was attributed to the hollowness of these two lakes, which allows them to easily warm in the early summer.
To our knowledge, up to now few quantitative studies have been done regarding the seasonal contributions of lake effects to local precipitation in such cold and high-mountain environment.
Nam Co Lake is one of the largest lakes on the TP, with an area of more than 2000 km2. The maximum and average depths of Nam Co Lake are 98 and 45 m, respectively. The shape of Nam Co Lake is in the East-West direction, and the length of the fetch distance is about 73 km (Figure 1). The south area of the lake is the Nianqing Tanggula Mountains. Previous studies have demonstrated the role of Nam Co Lake in local climate (Lazhu et al., 2016; Huang et al., 2017; Dai et al., 2018a,b). Based on station meteorological data and reanalysis data, we analyzed the qualitative characteristics of lake effect precipitation in the Nam Co basin and identified the lake cooling effect during July–August and lake heating effect during November–December (Dai et al., 2018b). With weather research forecasting (WRF) model simulation and station meteorological data, we pointed out that the lake effect could contribute more than half of the precipitation to the downwind heavy precipitation events around Nam Co Lake during October to November (Dai et al., 2018a). However, the impact of the lake cooling effect of Nam Co on the regional climate during summer hasn’t been quantitatively evaluated. The diurnal character of lake effect of Nam Co on regional climate hasn’t been well-explored. In this paper, we focus on the lake effect of Nam Co on regional climate during daytime and nighttime, and its seasonal variations of the diurnal character of lake effect of Nam Co in summer and autumn.
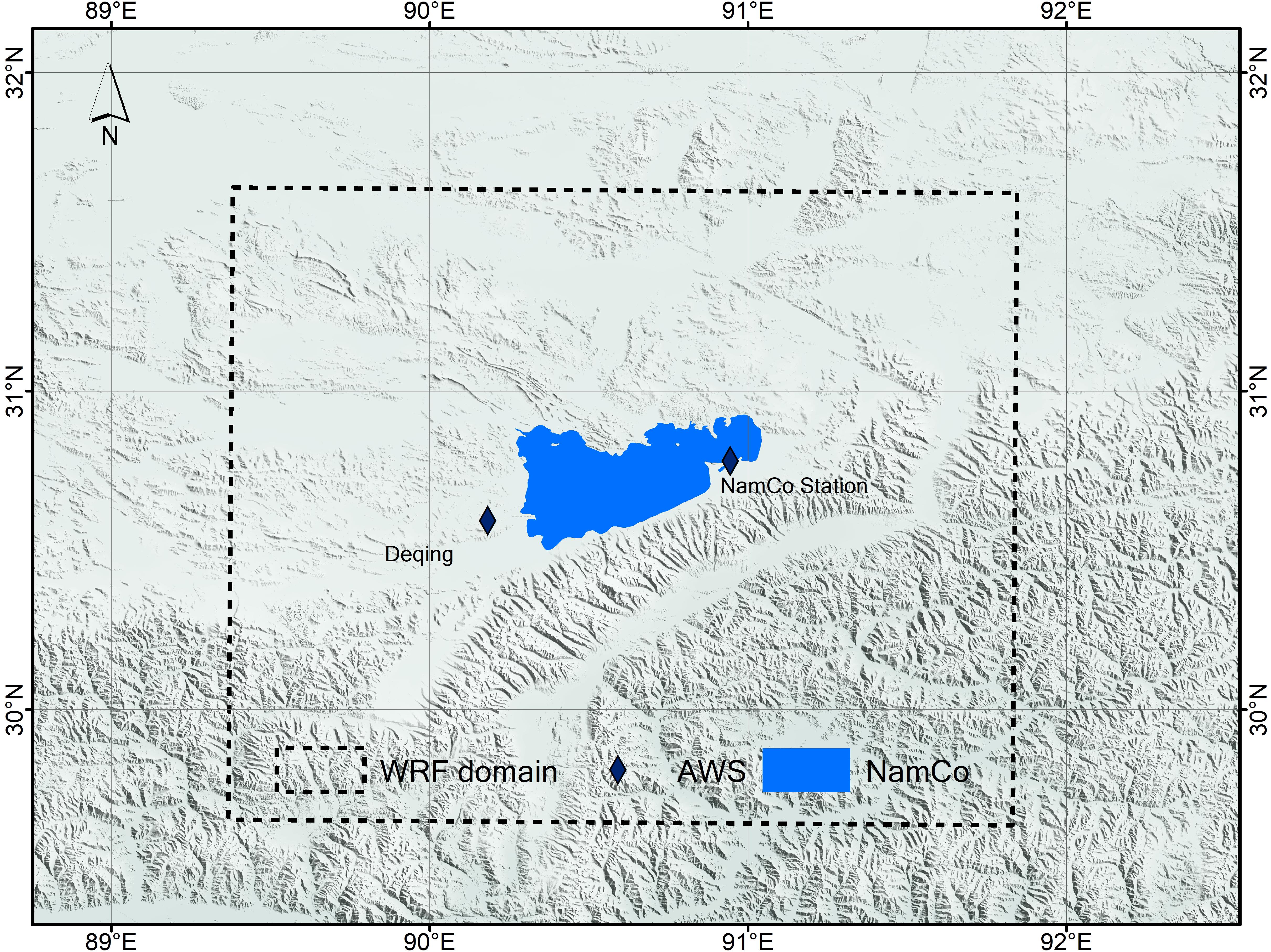
Figure 1. Location and topography of Nam Co Lake and the two nearby meteorological stations (Nam Co and Deqing stations). The black dash box denotes the position of the simulation domain. The AWS is the abbreviation of Automatic Weather Station.
Materials and Methods
Multiple Data Products
We used MODIS Land Surface Temperature Data Product (MOD11A2) collected during the daytime (10:30 Local Time; 04:30 UTC) and at night (22:30 Local Time; 16:30 UTC), and we calculated diurnal surface temperatures separately by using Modis_day and Modis_night. We then used these surface temperatures to calculate the monthly averages of surface temperature. More detailed information of MOD11A2 data can be found in the MODIS Products Users’ Guide1.
We used 10 m wind speed and 2 m air temperature data in 2008 from ERA 5 monthly reanalysis datasets, with a horizontal resolution of 0.25° × 0.25° (Copernicus Climate Change Service (C3S), 2017). We also used 2 m air temperature, and precipitation data (0.1° × 0.1° resolution) in 2008 from the China Meteorological Forcing Dataset (CMFD; Yang et al., 2010; He et al., 2020) that combines in-situ observations of TP, provided by the National Tibetan Plateau Data Center. Finally, we used monthly Tropical Rainfall Measuring Mission (TRMM; Kummerow et al., 2000) data in 2008.
WRF Model
WRF model is a useful tool to study regional lake-effect precipitation (Zhao et al., 2012; Nicholls and Toumi, 2014; Sun et al., 2015; Wen et al., 2015; Dai et al., 2018a; Shi and Xue, 2019). Using the WRF-Lake model to simulate the regional climate of Nam Co in October of 2006, we showed that Nam Co causes lake-effect precipitation in October (Dai et al., 2018a). Similar to the study of Dai et al. (2018a), in this study we used the WRF model to simulate the impact of Nam Co Lake on local climate, with same physics options choices and similar boundary configuration. Please see more details in Dai et al. (2018a). The simulation start time was 0000 UTC 1 May 2008, and the end time was 0000 UTC 1 December 2008, and the model produced output every 3 h. The averages of the simulated data at two times during the day (03:00 and 06:00 UTC) and at two times during the night (15:00 and 18:00 UTC) are reported here to compare with the corresponding MODIS daytime (04:30 UTC) and nighttime (16:30 UTC) data. We carried out two WRF experiments: one including lake (WRF_lake) and the other with lake replaced by land surface (WRF_nolake). Comparison of WRF_lake and WRF_nolake showed the influence of the lake on the regional climate.
The aim of this study was to investigate the seasonal variety of Nam Co lake effects in summer and autumn using observations, reanalysis data, and numerical modeling. The key factor to understand the lake effect is lake-air temperature difference (Lavoie, 1972). In this study, we verified and analyzed the factors such as lake temperature, air temperature and precipitation. An observation station located in the Nam Co area since 2005, in the watershed of the southeastern part of the lake, 2 km away from the lake shore, where lake effect precipitation occurs in October and November (Dai et al., 2018a). The precipitation measurements of the west part of the lake (Deqing station) have been carried out in 2008 (Zhou et al., 2013). We therefore chose to analyze the characteristics of the lake effect in 2008. Nam Co Lake is ice-covered for about 5 months and ice free for about 7 months per year (Kropacek et al., 2013). From December to April, as the complex influence of lake ice and the total precipitation over the lake and surrounding is few to negligible. In our analysis we therefore focused on the period from May to November. According to the seasonal variations in the simulated latent and sensible heat fluxes of Nam Co (Lazhu et al., 2016), the result indicate that the evaporation is small in summer and reaches a peak in October. We chose July in summer and October in autumn for detailed analysis.
Evaluation of WRF Simulations: Temperature and Precipitation
Comparison of the Skin Surface Temperatures Between WRF and MODIS
The diurnal changes of MODIS lake surface temperature were relatively small compared to the corresponding temperature changes of the surrounding land surface. We first obtained the temperature of surface skin (TSK) over the lake by WRF simulation and by MODIS data. The spatial pattern of the WRF simulated TSK was in agreement with the MODIS data, though the simulated TSK over land is slightly higher with WRF than MODIS data in July and lower in October (Figure 2). In particular, the TSK difference between the lake and land and its diurnal variety are well-captured by WRF. Based on MODIS data, the TSK over Nam Co Lake was cooler (warmer) than the TSK in surrounding land during daytime (nighttime) (Figure 2). In July (Figures 2A–D), the TSK difference between the lake surface temperature and land surface temperature was −16°C in the daytime and 5°C at nighttime according to MODIS data. The simulated TSK difference between the lake and land with WRF was −12°C in the daytime and 3°C at nighttime. In October (Figures 2E–H), the TSK difference between the lake and land was −6°C in the daytime and 15°C at nighttime according to MODIS data. The simulated TSK difference between the lake and land with WRF was −3°C in the daytime and 12°C at nighttime. The simulated TSK difference between the lake surface temperature and land surface temperature was consistent with that based on MODIS data, which the deviation was about 4°C in the daytime and 3°C at nighttime. Therefore, WRF simulation was able to mimic the characteristics of diurnal variety the TSK difference between the lake and land in July and October.
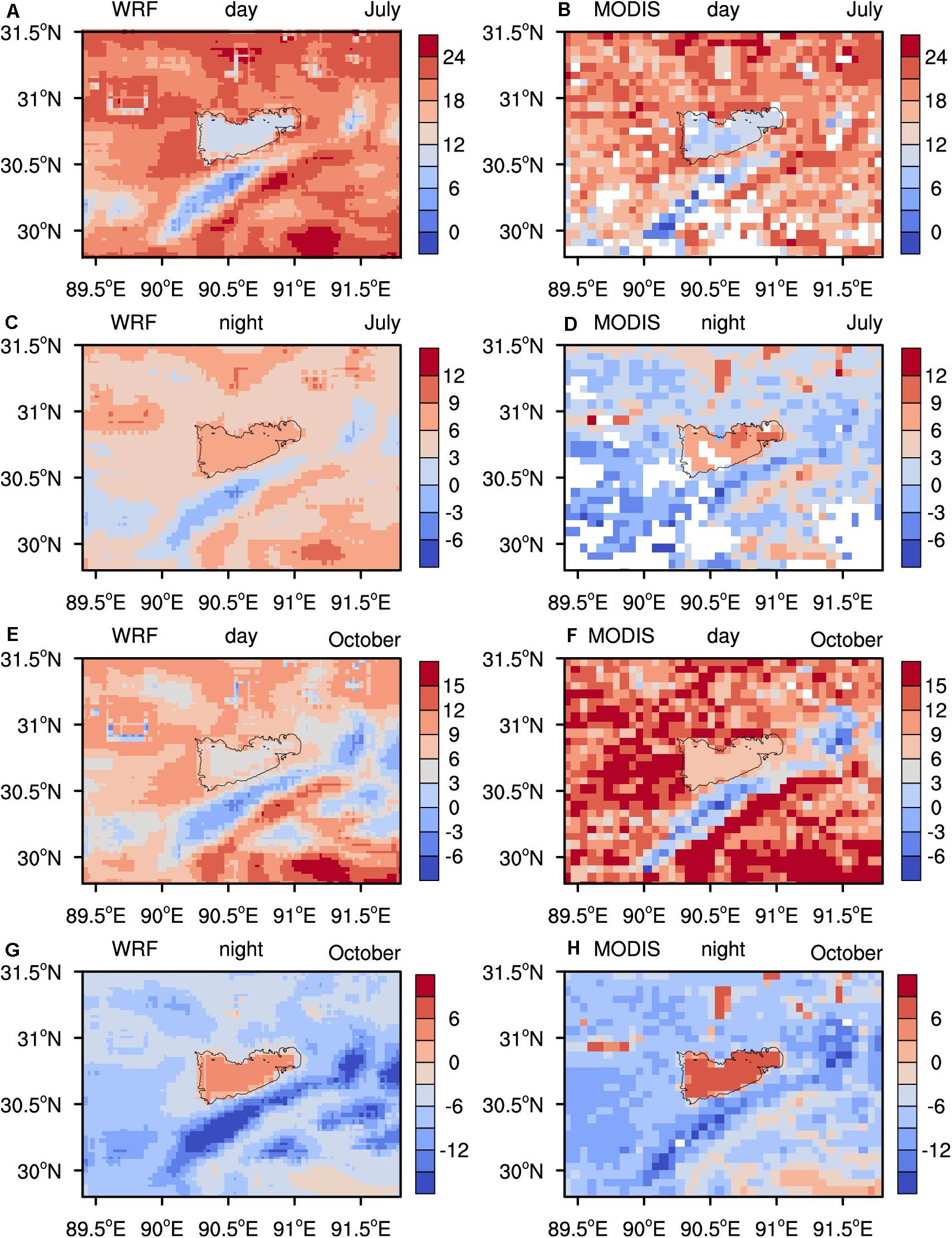
Figure 2. Diurnal spatial pattern of temperature of skin surface (TSK, units: °C) of WRF_lake simulation in July (A,C) and October (E,G), and the TSK from MODIS data in July (B,D) and October (F,H). The gridded squares with missed MODIS value are filled by white color.
Comparison of 2 m Temperatures and 500 hPa Temperatures Between WRF, ERA5, and CMFD
We also used the ERA 5 and CMFD data in July (Figure 3) and October (Figure 4) of 2008 to compare with the WRF simulation in terms of two varieties: 2 m air temperature and 10 m wind speed. The resolutions of WRF, ERA 5, and CMFD were 0.06° × 0.06°, 0.25° × 0.25°, and 0.1° × 0.1°, respectively. Nearly at each 3-h time slices, the WRF reasonably reproduced the spatial pattern of CMFD 2 m temperature. It is also noticed that the WRF-simulated temperature over the lake was closer to the ERA5 data. The WRF-simulated wind pattern was also consistent with the ERA5 wind. The WRF generally well-simulated both the wind in July and in October based on reanalysis data and gave more details.
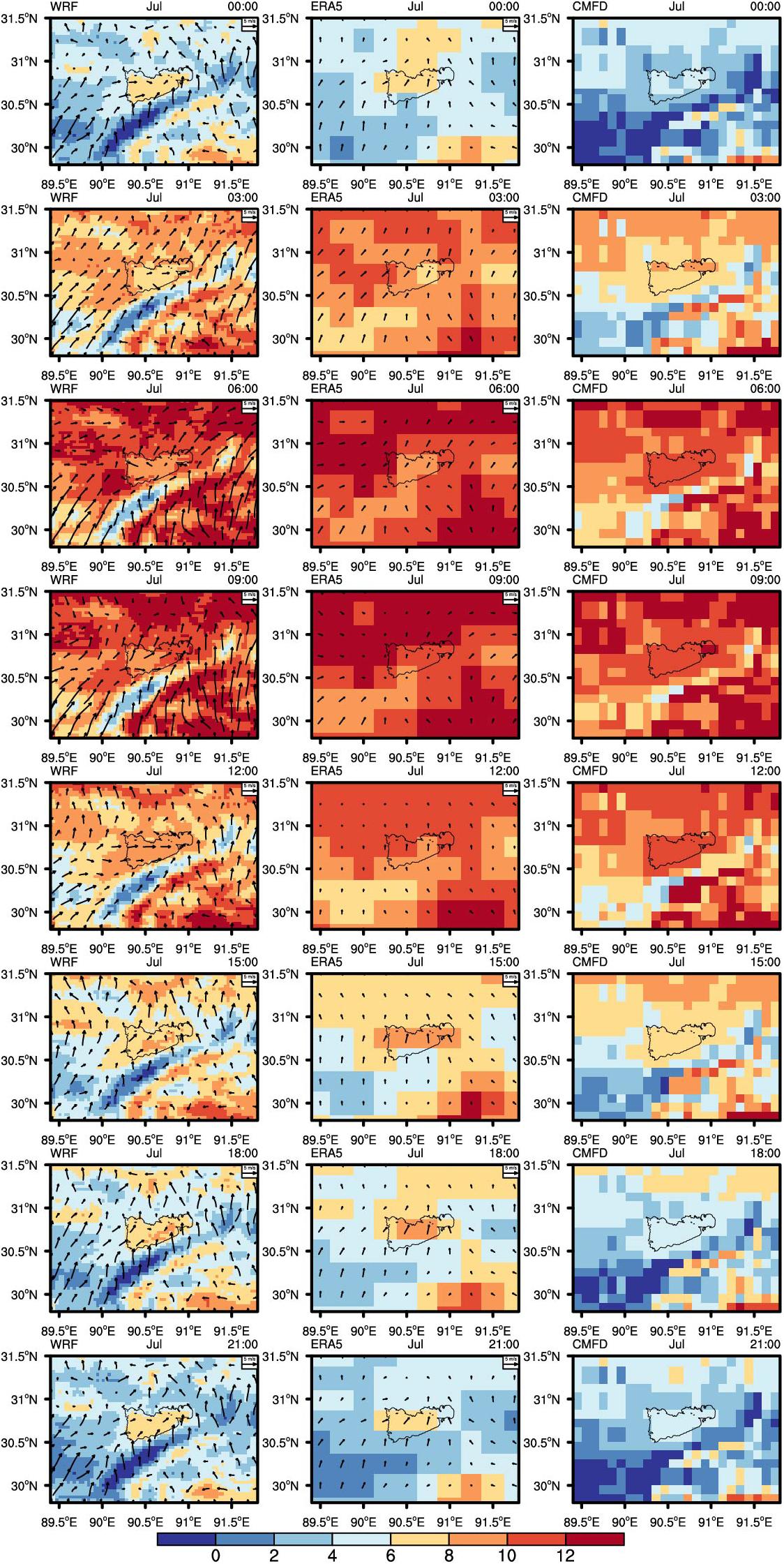
Figure 3. Comparison of the monthly mean 3-h 2 m temperature (shading, units: °C) and 10 m wind (vector, units: mm/s) between the WRF_lake simulation, the ERA5 and the CMFD data in July 2008.
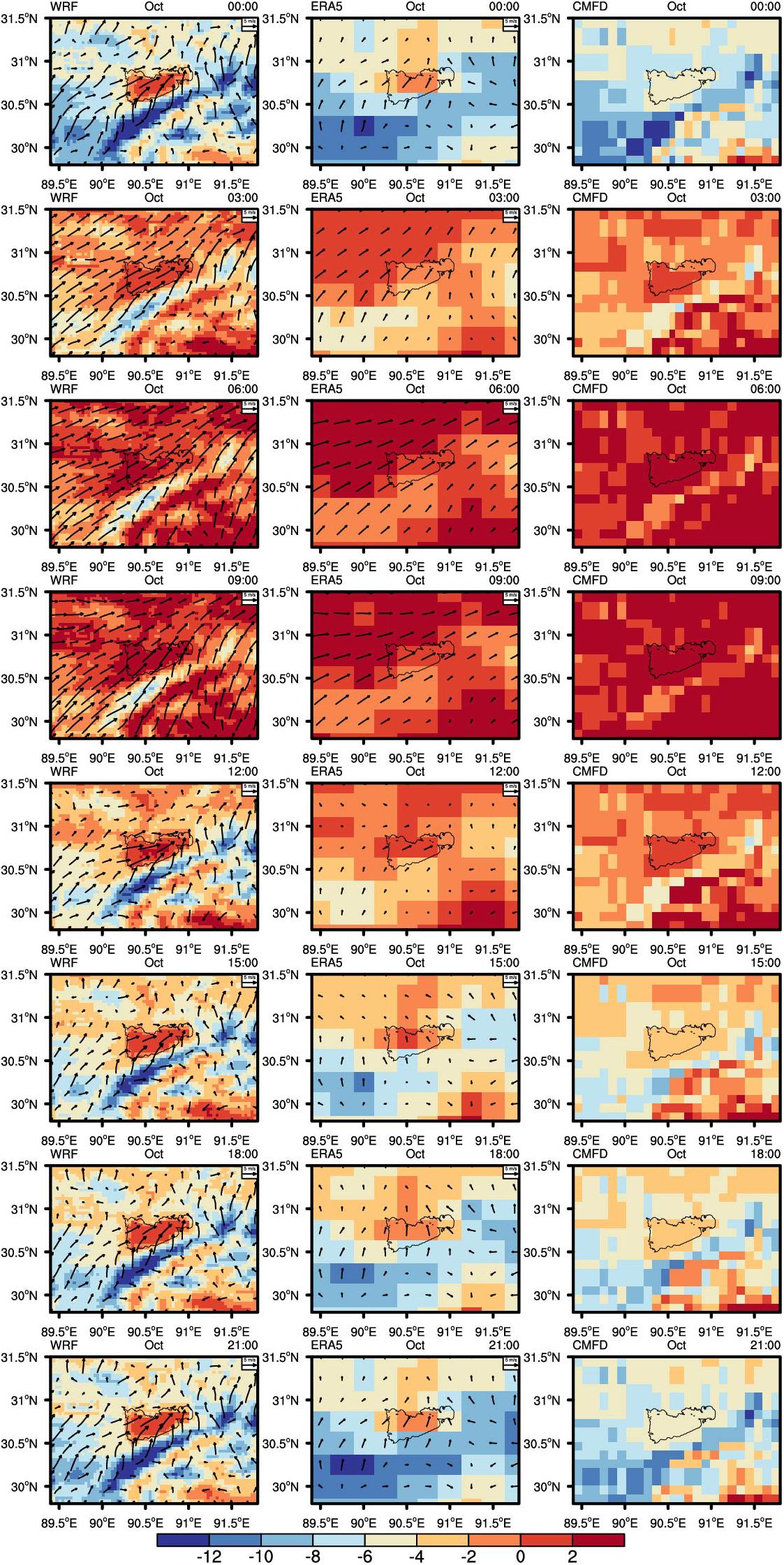
Figure 4. Same as Figure 3, but for October 2008.
Precipitation Comparison: Spatial and Temporal Distribution
The Nam Co Lake is a relatively gentle terrain in the basin. The simulated precipitation over the lake was larger than the TRMM and CMFD data (Figure 5). The deviation between the WRF simulation and the satellite data also appears in previous study on the TP (Gao et al., 2015). Compared with two stations on the east and west side of the lake, in July, the WRF-simulated precipitation was higher than the precipitation at the Nam Co station on the east coast and lower than the precipitation at the Deqing station on the west coast. In contrary, the WRF-simulated precipitation in October was slightly lower than the precipitation at the Nam Co station and higher than the precipitation at the Deqing station. However, WRF reasonably reproduced the seasonal rainfall amount in July and October. Regarding the spatial patterns, nearly at each 3 h time interval, the WRF produced a comparable spatial pattern of precipitation to that of the CMFD (Figure 6). We also found discrepancies between the WRF simulation and the CMFD data from 0:00 to 6:00 UTC, which may be attributed to their different 2 m temperature over the lake. In general, the WRF can reasonably depict the lake effect on the regional precipitation.
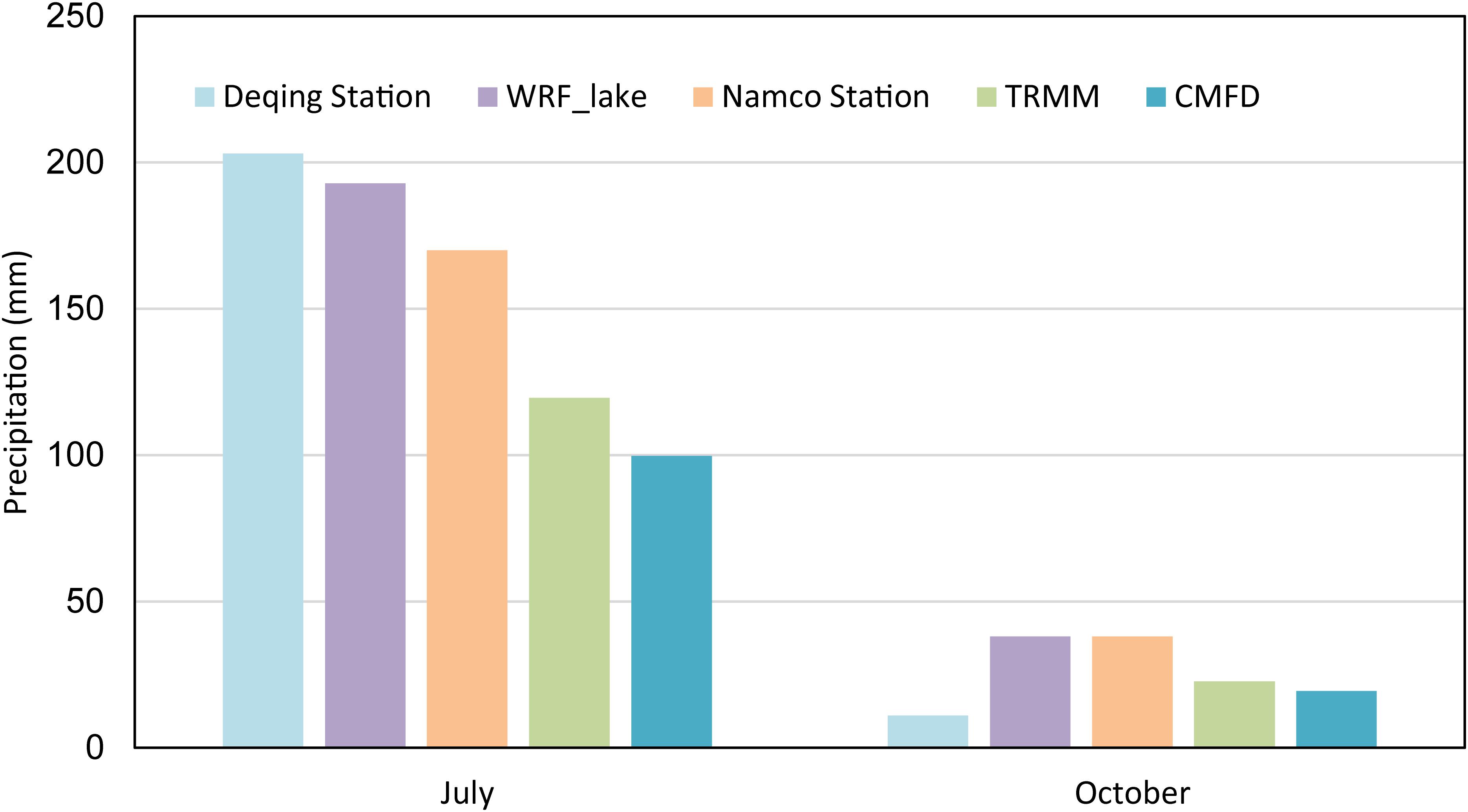
Figure 5. The monthly precipitation at the upwind Deqing station (blue), and the downwind Nam Co station (orange), as well as the monthly precipitation averaged over the Nam Co Lake by the WRF simulation (purple) and from the TRMM product (green) and CMFD (dark blue), in July and October, respectively.
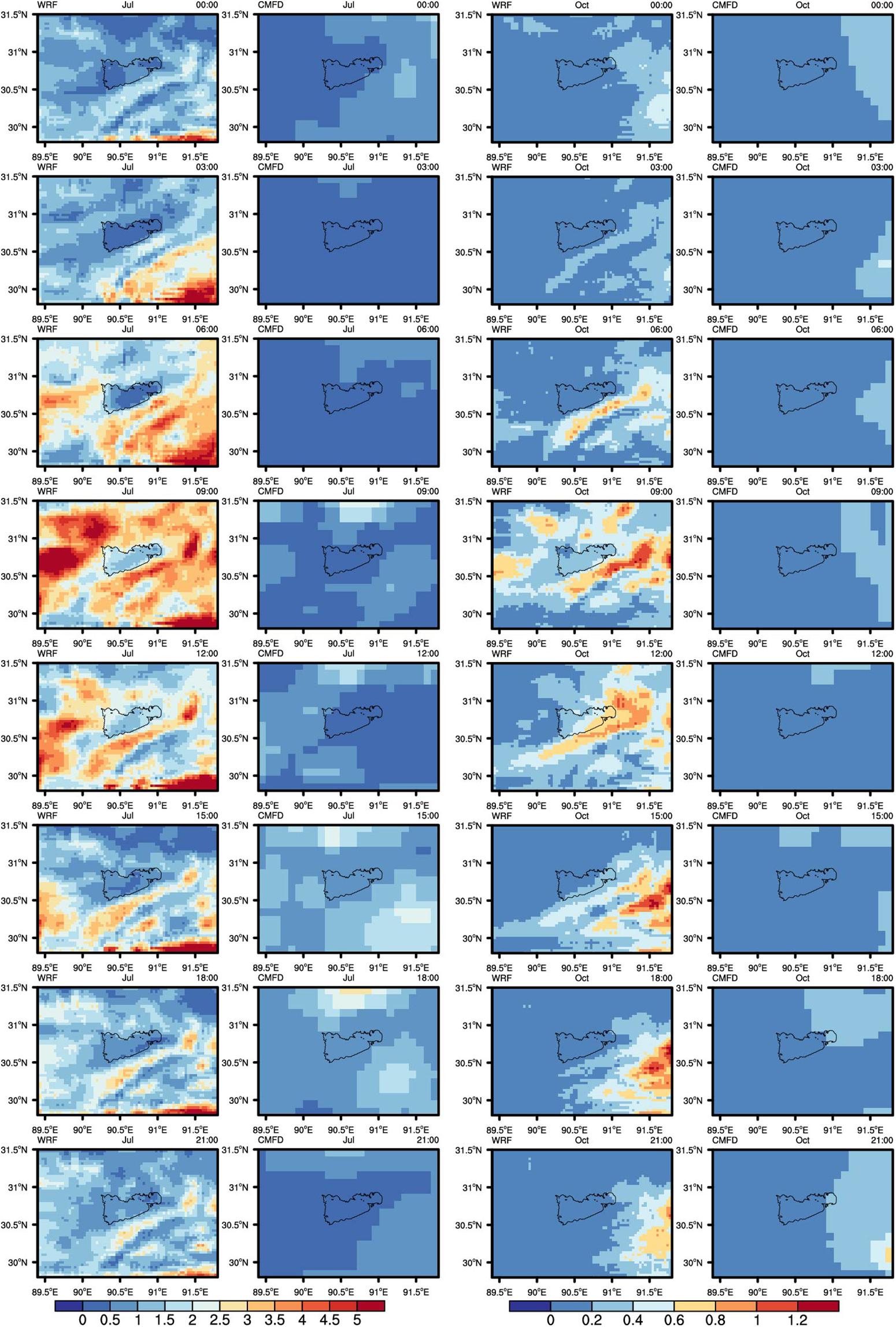
Figure 6. Spatial distribution of monthly mean 3-h precipitation (shading, units: mm) from the WRF_lake simulation and the CMFD data in July (left two panels) and October (right two panels) of 2008.
Results and Discussion
Heat Sinks and Sources
Because the temperature difference between the lake and land is very different during the day and night, we analyzed the diurnal variety of the temperature difference between the lake and land during different seasons based on the WRF-simulated TSK. After the sun rises in the daytime (03:00–09:00 UTC) during July, the TSK of the land rises faster than the TSK of the lake, which is about 9°C cooler than the land TSK at 09:00 UTC (Figure 7). Six hours after sunset (15:00–18:00 UTC), the TSK difference between the lake and land reaches a minimum. The land cools faster at night (21:00–00:00 UTC), and the lake TSK is about 6°C warmer than the land TSK. The maximum temperature difference between the lake and land can be as low as −17°C during the day, whereas the temperature difference is about 10°C at night. The lake is a strong heat sink during the day, and a source of heat at night in the summer.
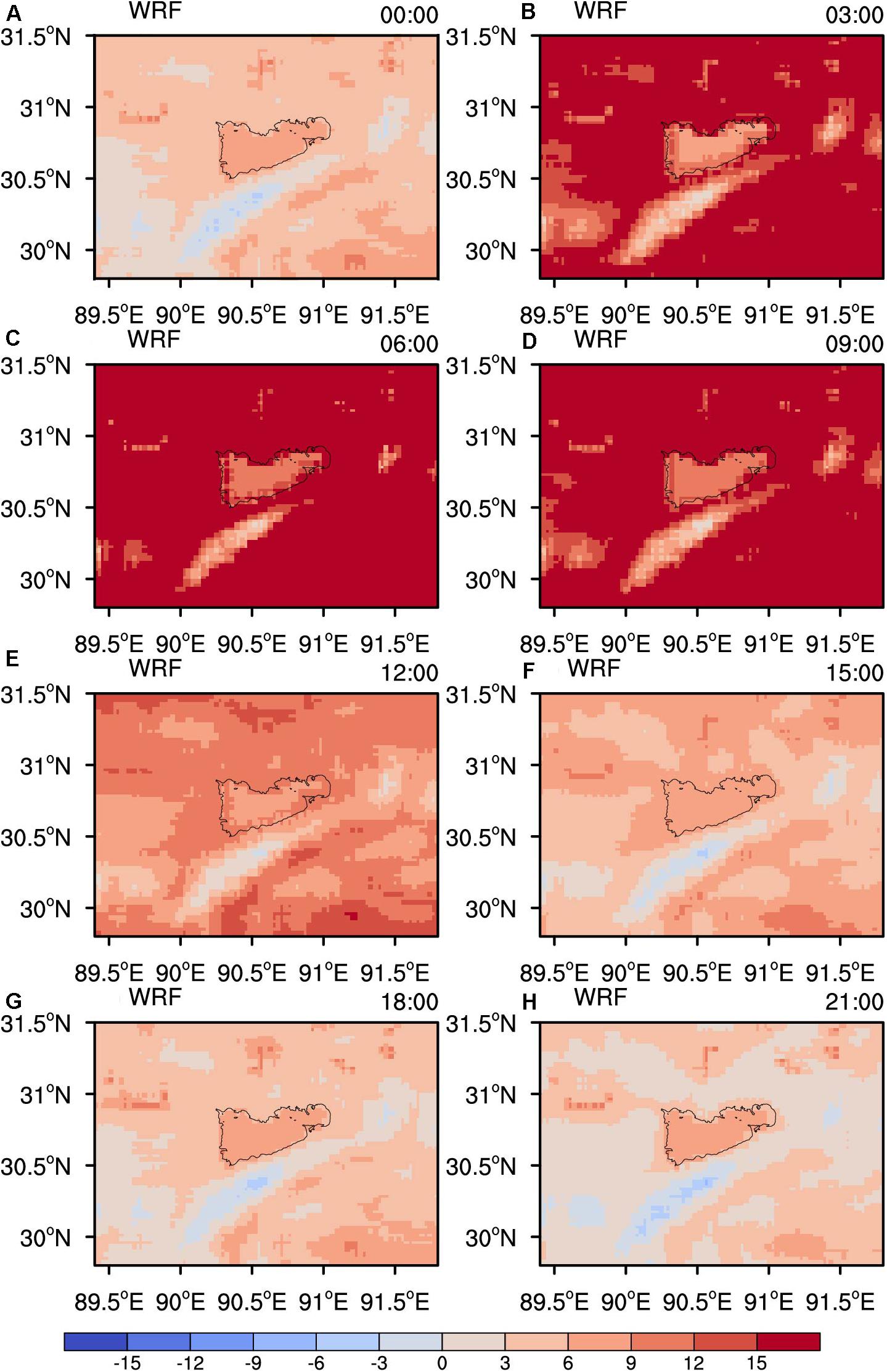
Figure 7. Spatial pattern of monthly mean 3-h TSK (A–H, shading, units: °C) of WRF_lake simulation in July 2008.
In October, during the 6 h after the sun rises (03:00–09:00 UTC), the TSK of the land rises faster than the TSK of the lake (Figure 8). During evening and early morning (12:00–00:00 UTC), the TSK of the land decreases much faster than the TSK of the lake. The maximum TSK difference between the lake and land is greater than 18°C at night, whereas the difference between the land and lake temperature is less than 5°C during the daytime. The lake acted mainly as a strong heat source in the autumn.
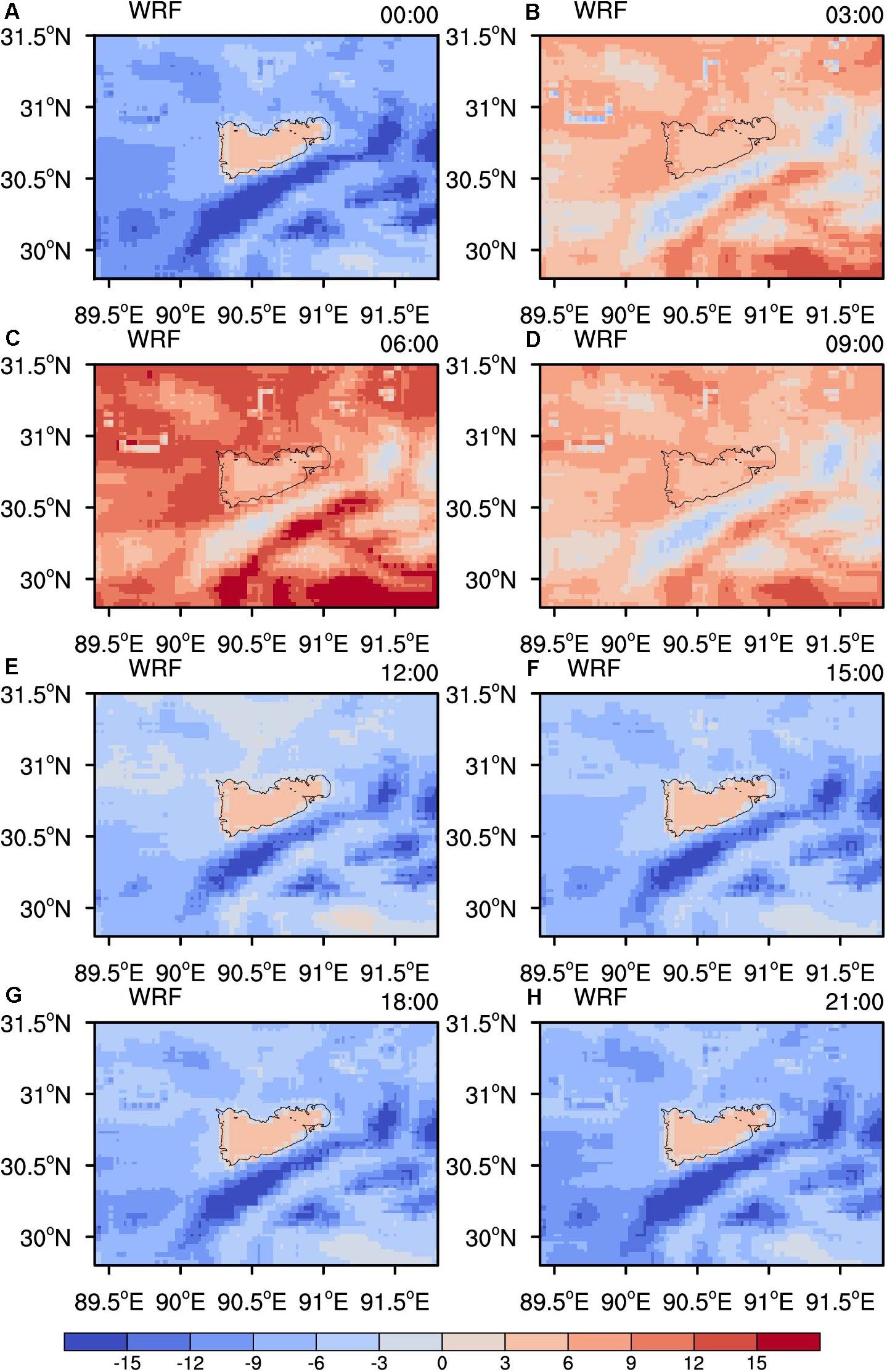
Figure 8. Same as Figure 7, but for October 2008.
Cooling and Warming Effects on 2 m Temperatures
The comparison of WRF_lake and WRF_nolake showed that the lake had a cooling effect in July, whereas the lake had a warming effect in October, in terms of 2 m air temperature. Due to the air temperature varies more diurnally than the lake temperature, we analyzed the temperatures during the daytime and night with WRF_lake and WRF_nolake models to determine the influence of the lake on the daily maximum and daily minimum air temperatures.
The lake effect was essentially limited to the immediate vicinity of the lake (Figure 9). In July, the lake was characterized by a negative temperature anomaly of up to 3°C during the day and by the positive anomalies of up to 3°C during the night. The presence of the lakes reduces the 2 m temperature within a distance of 10 km around the lake by an average of 0.4°C during the day (03:00–12:00 UTC), and increased it by about 0.3°C at night (18:00–03:00 UTC). The diurnal temperature difference around the lake was reduced by 0.7°C because of the lake effect. In July, there was still a warming effect of Nam Co Lake that could be explained by its relatively warm surface water at night.
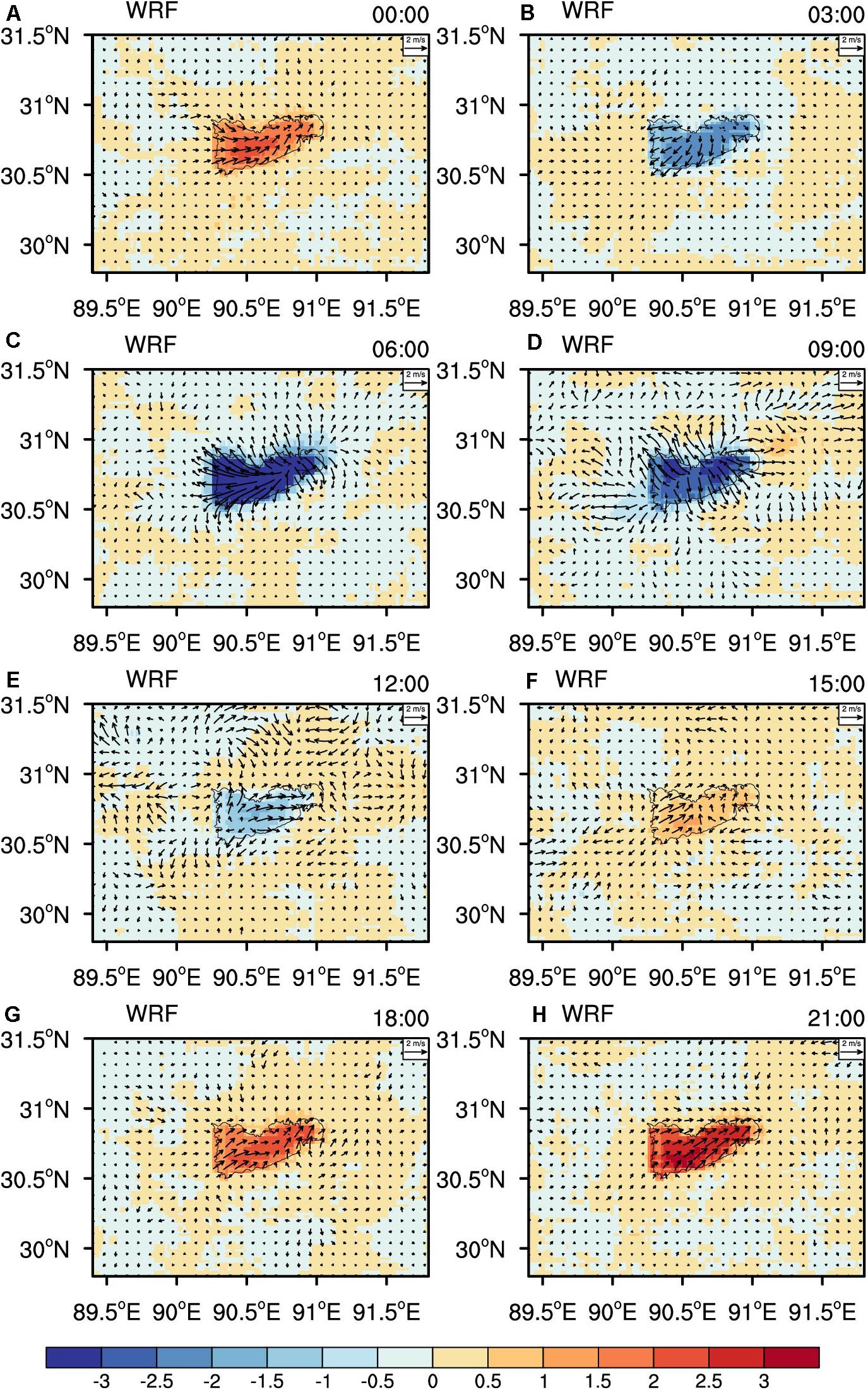
Figure 9. Spatial pattern of simulated of monthly mean 3-h 2 m air temperature (A–H, shading, units: °C) and 10 m wind (A–H, vector, units: m/s) anomaly (difference between WRF_lake and WRF_nolake simulations) in July 2008.
In October, Nam Co Lake is characterized by a negative temperature anomaly of up to 1°C during the day and by the positive temperature anomalies of up to 4°C at night. According to the spatial pattern of simulated diurnal 2 m air temperature anomaly (difference between WRF_lake and WRF_nolake simulations) in October (Figure 10), The presence of the lakes reduced the temperature around the lake by 0.6°C in the daytime (06:00–12:00 UTC), and its influence extended from the shore for as much as 50 km to the east. In the middle of the night (21:00–06:00 UTC), the temperature within 10 km of the lake increased by an average of 0.4°C. The diurnal temperature difference around the lake was reduced by 1°C.
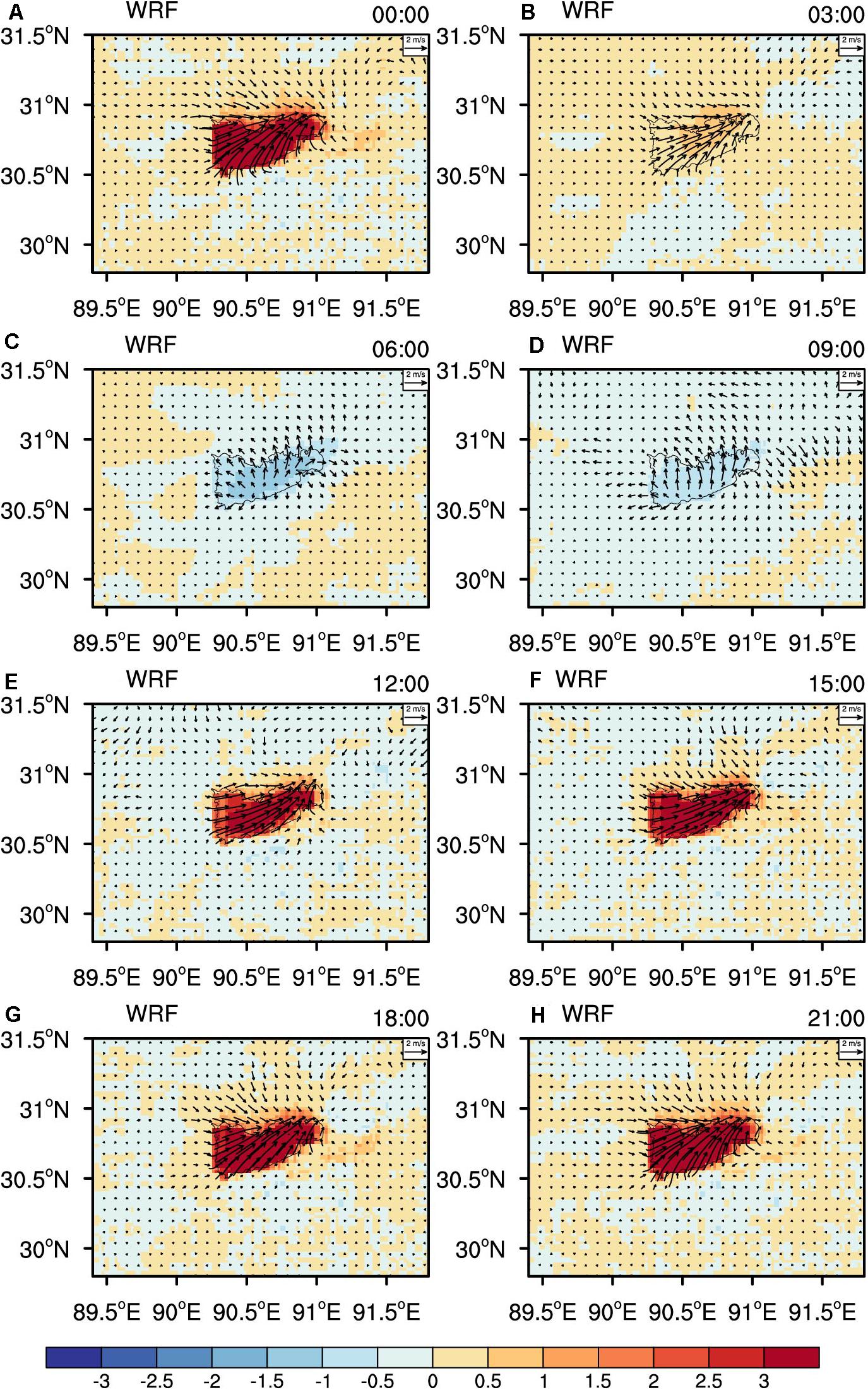
Figure 10. Same as Figure 9, but for October 2008.
The cooling effect during the day led to a decrease of the daily maximum temperature in areas adjacent to the lake, and the warming effect at night led to an increase of the daily minimum temperature in the region. The lake therefore reduced the amplitude of the daily temperature difference cycle. Previous studies of Ngoring Lake and Gyaring Lake on the TP have also revealed that most lakes reduce the maximum temperature all year round and increase the minimum temperature, except in March (Wen et al., 2015). This temperature effect has an impact on the flora of the region.
Examination of the spatial pattern of the 10 m wind field (Figures 9, 10) revealed that during the day (06:00–12:00 UTC) the presence of the lake caused a strong lake breeze around the lake, which was strongest in the afternoon (15:00–18:00 Local Time) in July. The Lake breeze emanated from the lake to the surrounding area and transported water vapor to the land and increased the humidity. The lake breeze not only changed the temperature but also caused the formation of low clouds and fogs. A land breeze, which blew from the land over the lake, was apparent during 1200–0000 UTC (18:00–06:00 Local Time) in October.
We compared the temperature difference between the surface and 500 hPa simulated by WRF_lake and WRF_nolake models to calculate the influence of the lake on the temperature difference between lake and 500 hPa. During the period from May to August, the lake-500 hpa temperature difference was less than 6°C based on the WRF_lake simulation, whereas lake-500 hPa temperature difference exceeded 12°C according to the WRF_nolake simulation. In July, the presence of the lakes reduced the lake-500 hPa temperature difference over Nam Co Lake by about 5°C (Figure 11). In September, the presence of the lake caused a slight increase in the lake-500 hPa temperature difference. In October and November, the lake-500 hPa temperature difference exceeded 12°C according to the WRF_lake simulation, whereas the lake-500 hPa temperature difference was less than 12°C based on the WRF_nolake simulation. In October, the presence of the lake increased the lake-500 hPa temperature difference by about 7°C (Figure 11). Theoretically, changes of the lake effect are reflected by changes of lake-500 hPa temperature difference.
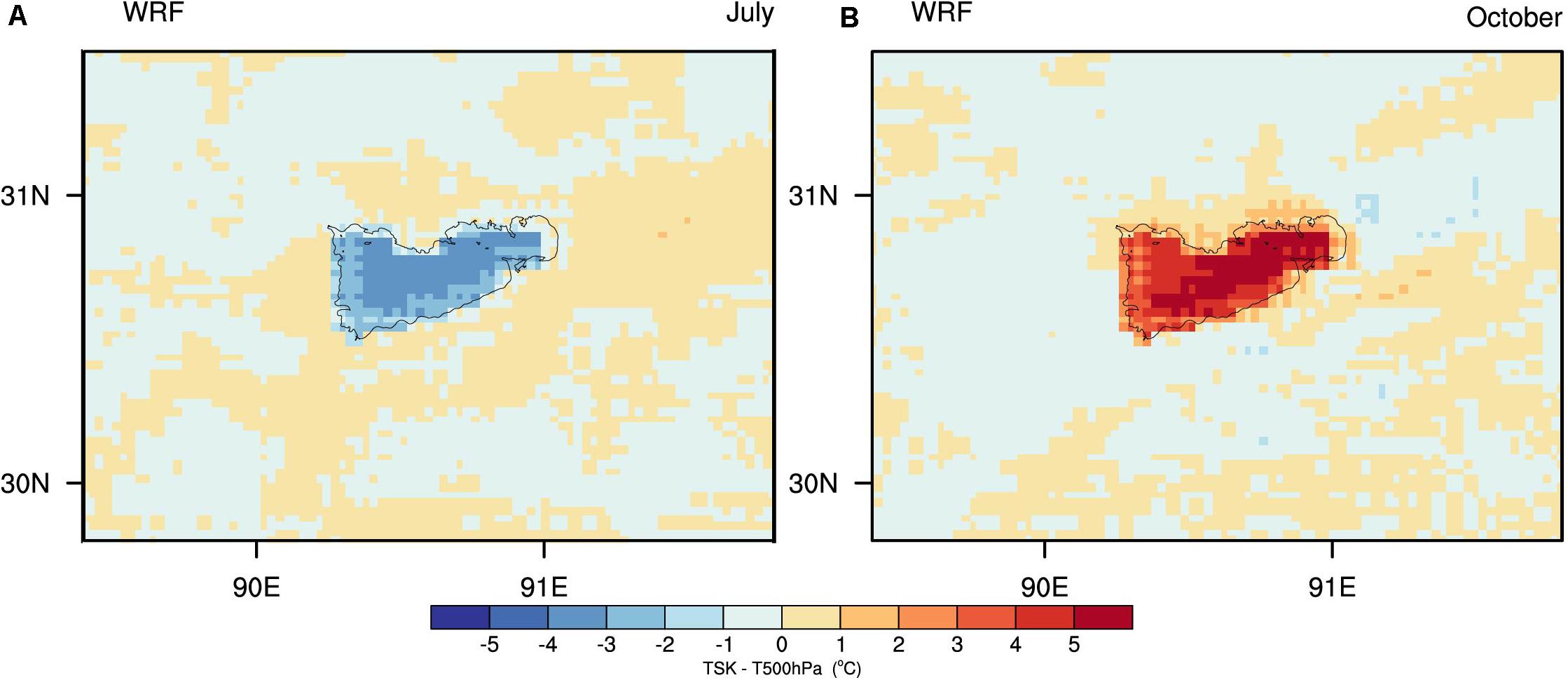
Figure 11. Spatial pattern of the difference between the simulated results by the WRF_lake and the WRF_nolake, which is the gap between the lake surface and 500 hPa temperatures (units: °C) in July (A) and October (B) of 2008.
Lake Effect: Enhancement and Suppression on Precipitation
The WRF_lake and WRF_nolake simulations indicated that there was no significant change in the distribution of large scale precipitation. Because of the cooling effects of the lake, there was characterized by much less precipitation over the lake during May to August. The presence of the lake reduced precipitation over the lake and thereby increased the spatial differences of regional precipitation. The precipitation over the lake simulated by the WRF_lake model was less than the simulated precipitation in the absence of the lake (WRF_nolake). The difference was greatest in July (Figure 12A). There was no significant change in May and September. During May to August, the presence of the lake reduced precipitation over the lake by about 45–70%.
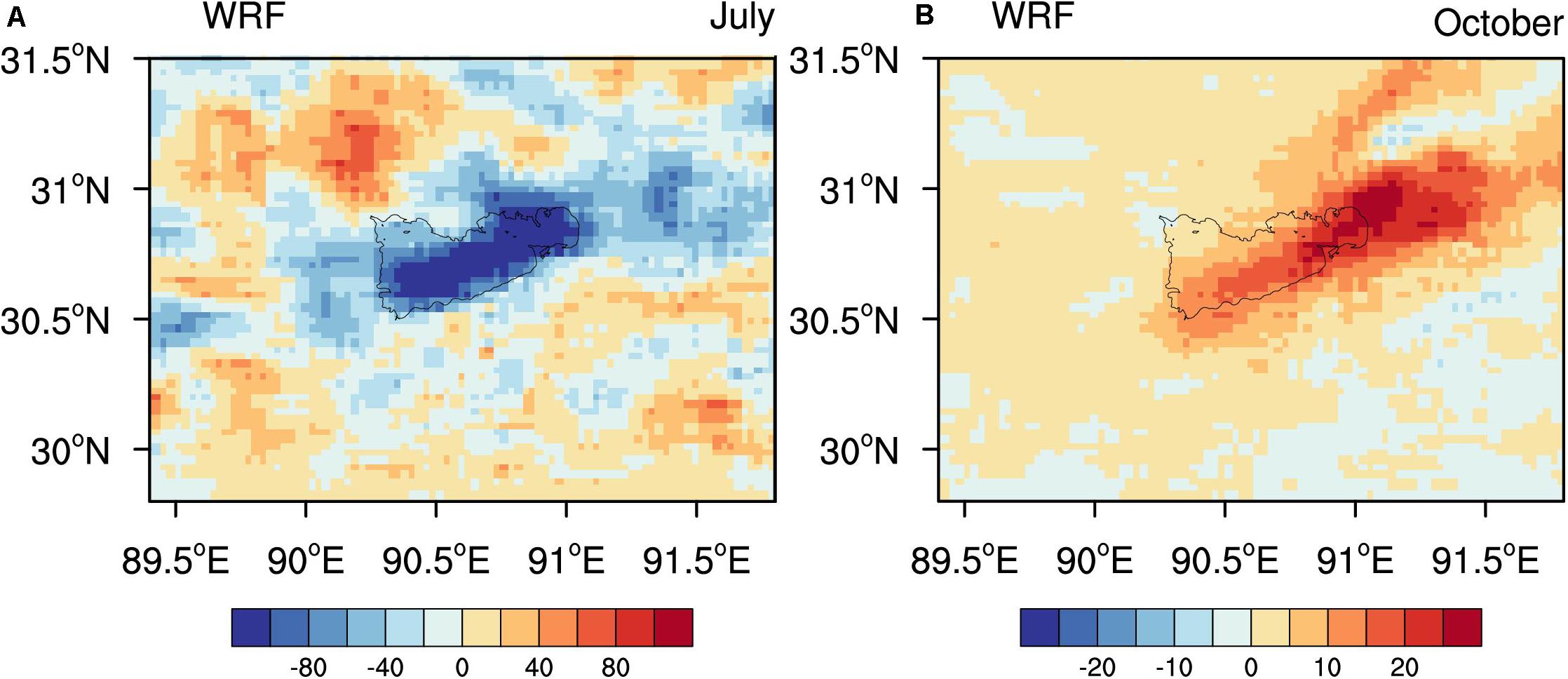
Figure 12. Precipitation difference (mm) between the WRF_lake and the WRF_nolake simulations in July (A) and October (B) of 2008.
The WRF_lake and WRF_nolake simulation showed that there was no significant change in the distribution of large scale precipitation from October to November too. At a smaller scale, parts of the basin east of 90.25°E were dominated by lake-effect precipitation. The precipitation over the lake simulated by WRF_lake model exceeded the precipitation simulated in the absence of the lake (WRF_nolake) by more than 60% (Figure 12B). Furthermore, precipitation was greater in the eastern than in the western part of the basin because of the lake.
The precipitation over Nam Co Lake is suppressed in summer and increased in autumn as a result of the lake effect. Although the Nam Co lake effect did not change the large-scale distribution of precipitation, it significantly affected the temporal pattern of precipitation locally. Specifically, the lake effect reduced precipitation in the wet season and increased precipitation in the dry season.
Conclusion
Although the monthly mean lake–land temperature difference was small in summer, the surface WRF-simulated temperature showed that in July the lake was a heat sink during the day and a source of heat during the night. The monthly mean lake–land temperature difference was significant in October and November, when the lake temperature was higher than the land temperature. In October, the lake was mainly a source of heat, especially between midnight and early morning. The distribution of precipitation in the Nam Co basin was characterized by two patterns. the precipitation was less over the lake than over the surrounding land in summer; and the precipitation was greater in the eastern than in the western part of the Nam Co basin in autumn.
A comparison of two WRF sensitivity experiments, one including the lake (WRF_lake) and the other with lake replaced by land surface (WRF_nolake), showed that the lake cooled the air during the day and warmed the air at night by up to several degrees, depending on the large-scale meteorological conditions. The cooling effect of the lake was manifested by lower air temperatures in the summer, and the warming effect of the lake was manifested by higher air temperatures in autumn. Both the cooling and warming effects were limited to the immediate vicinity of the lake and were normally not apparent more than a few kilometers from the shore. The presence of the lake reduced the lake–500-hPa temperature difference over Nam Co Lake by up to 6°C from May to August in 2008. In October and November of 2008, the presence of the lake increased the lake–500-hPa temperature difference by up to 7°C. The lake effect did not change the large-scale distribution of precipitation, but it affected local precipitation significantly. In 2008, from June through August, the lake reduced precipitation over the lake by about 45–70%; while from October through November in 2008, the WRF-simulated precipitation was 60% greater than the precipitation in the absence of the lake.
Data Availability Statement
Publicly available datasets were analyzed in this study. This data can be found here: https://e4ftl01.cr.usgs.gov/MODIS_Dailies_E/MOLT/MOD11A1.005/; https://disc2.gesdisc.eosdis.nasa.gov/opendap/TRMM_L3/; http://data.tpdc.ac.cn; https://reanalyses.org/atmosphere/era5-references.
Author Contributions
YD designed the study and led the drafting of the manuscript. LW and TY contributed to the interpretation of results and the overall framing of the study. XL and XZ performed data analysis and prepared figures. All authors contributed to the article and approved the submitted version.
Funding
This study was funded by the National Natural Science Foundation of China (Grants 41801049 and 91747201).
Conflict of Interest
The authors declare that the research was conducted in the absence of any commercial or financial relationships that could be construed as a potential conflict of interest.
Acknowledgments
We would like to acknowledge the U.S. Geological Survey (USGS) and U.S. National Aeronautics and Space Administration (NASA) for providing MODIS data (https://e4ftl01.cr.usgs.gov/MODIS_Dailies_E/MOLT/MOD11A 1.005/) and TRMM data (https://disc2.gesdisc.eosdis.nasa.gov/o pendap/TRMM_L3/), the National Tibetan Plateau Data Center providing CMFD data (http://data.tpdc.ac.cn), and the European Center for Medium-Range Weather Forecasts for providing the ERA 5 data (https://reanalyses.org/atmosphere/era5-references).
Footnotes
References
Bonan, G. B. (1995). Sensitivity of a gcm simulation to inclusion of inland water surfaces. J. Clim. 8, 2691–2704. doi: 10.1175/1520-0442(1995)008<2691:soagst>2.0.co;2
Copernicus Climate Change Service (C3S) (2017). ERA5: Fifth generation of ECMWF atmospheric reanalyses of the global climate. Copernicus Climate Change Service Climate Data Store (CDS). Available online at: https://cds.climate.copernicus.eu/cdsapp#!/home
Dai, Y., Wang, L., Yao, T., Li, X., Zhu, L., and Zhang, X. (2018a). Observed and simulated lake effect precipitation over the tibetan plateau: an initial study at nam co lake. J. Geophys. Res. Atmos. 123, 6746–6759. doi: 10.1029/2018jd028330
Dai, Y., Yao, T., Li, X., and Ping, F. (2018b). The impact of lake effects on the temporal and spatial distribution of precipitation in the nam co basin, tibetan plateau. Quat. Int. 475, 63–69. doi: 10.1016/j.quaint.2016.01.075
Gao, Y., Xu, J., and Chen, D. (2015). Evaluation of wrf mesoscale climate simulations over the tibetan plateau during 1979-2011. J. Clim. 28, 2823–2841. doi: 10.1175/jcli-d-14-00300.1
Haginoya, S., Fujii, H., Kuwagata, T., Xu, J., Ishigooka, Y., Kang, S., et al. (2009). Air-lake interaction features found in heat and water exchanges over nam co on the tibetan plateau. Sola 5, 172–175. doi: 10.2151/sola.2009-044
He, J., Yang, K., Tang, W., Lu, H., Qin, J., Chen, Y. Y., et al. (2020). The first high-resolution meteorological forcing dataset for land process studies over China. Sci. Data 7:25. doi: 10.1038/s41597-020-0369-y
Huang, L., Wang, J., Zhu, L., Ju, J., and Daut, G. (2017). The warming of large lakes on the tibetan plateau: evidence from a lake model simulation of nam co, china, during 1979-2012. J. Geophys. Res. Atmos. 122, 13095–13107. doi: 10.1002/2017jd027379
Kirillin, G., Wen, L., and Shatwell, T. (2017). Seasonal thermal regime and climatic trends in lakes of the tibetan highlands. Hydrol. Earth Syst. Sci. 21, 1895–1909. doi: 10.5194/hess-21-1895-2017
Kropacek, J., Maussion, F., Chen, F., Hoerz, S., and Hochschild, V. (2013). Analysis of ice phenology of lakes on the tibetan plateau from modis data. Cryosphere 7, 287–301. doi: 10.5194/tc-7-287-2013
Kummerow, C., Simpson, J., Thiele, O., Barnes, W., Chang, A. T. C., Stocker, E., et al. (2000). The status of the tropical rainfall measuring mission (trmm) after two years in orbit. J. Appl. Meteorol. 39, 1965–1982.
Laird, N., Sobash, R., and Hodas, N. (2009). The frequency and characteristics of lake-effect precipitation events associated with the New York state finger lakes. J. Appl. Meteorol. Climatol. 48, 873–886. doi: 10.1175/2008jamc2054.1
Lavoie, R. L. (1972). A mesoseale numerical model of lake-fffect storms. J. Atmos. Sci. 29, 1025–1040. doi: 10.1175/1520-0469(1972)029<1025:amnmol>2.0.co;2
Lazhu, Y. K., Wang, J., Lei, Y., Chen, Y., Zhu, L., Ding, B., et al. (2016). Quantifying evaporation and its decadal change for Lake Nam Co, central Tibetan Plateau. J. Geophys. Res. Atmos. 121, 7578–7591. doi: 10.1002/2015JD024523
Nicholls, J. F., and Toumi, R. (2014). On the lake effects of the caspian sea. Q. J. R. Meteorol. Soc. 140, 1399–1408. doi: 10.1002/qj.2222
Samuelsson, P., Kourzeneva, E., and Mironov, D. (2010). The impact of lakes on the european climate as simulated by a regional climate model. Boreal Environ. Res. 15, 113–129.
Scott, R. W., and Huff, F. A. (1996). Impacts of the Great Lakes on regional climate conditions. J. Gt. Lakes Res. 22, 845–863. doi: 10.1016/S0380-1330(96)71006-7
Shi, Q., and Xue, P. F. (2019). Impact of lake surface temperature variations on lake effect snow over the Great Lakes region. J. Geophys. Res. Atmos. 124, 12553–12567. doi: 10.1029/2019JD031261
Song, C., Sheng, Y., Zhan, S., Wang, J., Ke, L., and Liu, K. (2019). Impact of amplified evaporation due to lake expansion on the water budget across the inner Tibetan Plateau. Int. J. Climatol. 40, 2091–2105. doi: 10.1002/joc.6320
Sun, X., Xie, L., Semazzi, F., and Liu, B. (2015). Effect of lake surface temperature on the spatial distribution and intensity of the precipitation over the lake victoria basin. Mon. Weather Rev. 143, 1179–1192. doi: 10.1175/mwr-d-14-00049.1
Tsujimoto, K., and Koike, T. (2013). Land-lake breezes at low latitudes: the case of tonle sap lake in cambodia. J. Geophys. Res. Atmos. 118, 6970–6980. doi: 10.1002/jgrd.50547
Umek, L., and Gohm, A. (2016). Lake and orographic effects on a snowstorm at lake constance. Mon. Weather Rev. 144, 4687–4707. doi: 10.1175/mwr-d-16-0032.1
Wang, B., Ma, Y., Ma, W., and Su, Z. (2017). Physical controls on half-hourly, daily, and monthly turbulent flux and energy budget over a high-altitude small lake on the tibetan plateau. J. Geophys. Res. Atmos. 122, 2289–2303. doi: 10.1002/2016jd026109
Wang, W., Lee, X., Xiao, W., Liu, S., Schultz, N., Wang, Y., et al. (2018). Global lake evaporation accelerated by changes in surface energy allocation in a warmer climate. Nat. Geosci. 11, 410–414. doi: 10.1038/s41561-018-0114-8
Welsh, D., Geerts, B., Jing, X., Bergmaier, P. T., Minder, J. R., Steenburgh, W. J., et al. (2016). Understanding heavy lake-effect snowfall: The vertical structure of radar reflectivity in a deep snowband over and downwind of lake ontario. Mon. Weather Rev. 144, 4221–4244. doi: 10.1175/mwr-d-16-0057.1
Wen, L., Lv, S., Li, Z., Zhao, L., and Nagabhatla, N. (2015). Impacts of the two biggest lakes on local temperature and precipitation in the yellow river source region of the tibetan plateau. Adv. Meteorol. 2015:248031. doi: 10.1155/2015/248031
Xu, J., Yu, S., Liu, J., Haginoya, S., and Yasunari, T. (2009). The implication of heat and water balance changes in a lake basin on the tibetan plateau. Hydrol. Res. Lett. 3, 1–5. doi: 10.3178/hrl.3.1
Yang, K., He, J., Tang, W. J., Qin, J., and Cheng, C. C. K. (2010). On downward shortwave and longwave radiations over high altitude regions: observation and modeling in the Tibetan Plateau. Agric. Forest Meteorol. 150, 38–46. doi: 10.1016/j.agrformet.2009.08.004
Zhan, S., Song, C., Wang, J., Sheng, Y., and Quan, J. (2019). A global assessment of terrestrial evapotranspiration increase due to surface water area change. Earth’s Future 7, 266–282. doi: 10.1029/2018EF001066
Zhao, L., Jin, J., Wang, S.-Y., and Ek, M. B. (2012). Integration of remote-sensing data with wrf to improve lake-effect precipitation simulations over the great lakes region. J. Geophys. Res. Atmos. 117:D09102. doi: 10.1029/2011jd016979
Zhou, J., Wang, L., Zhang, Y., Guo, Y., Li, X., and Liu, W. (2015). Exploring the water storage changes in the largest lake (selin co) over the tibetan plateau during 2003-2012 from a basin-wide hydrological modeling. Water Resour. Res. 51, 8060–8086. doi: 10.1002/2014wr015846
Keywords: cooling effect, warming effect, Nam Co Lake, WRF model, Tibetan Plateau
Citation: Dai Y, Yao T, Wang L, Li X and Zhang X (2020) Contrasting Roles of a Large Alpine Lake on Tibetan Plateau in Shaping Regional Precipitation During Summer and Autumn. Front. Earth Sci. 8:358. doi: 10.3389/feart.2020.00358
Received: 05 January 2020; Accepted: 04 August 2020;
Published: 03 September 2020.
Edited by:
Nick Van De Giesen, Delft University of Technology, NetherlandsReviewed by:
Hongkai Gao, East China Normal University, ChinaLijuan Wen, Northwest Institute of Eco-Environment and Resources (CAS), China
Copyright © 2020 Dai, Yao, Wang, Li and Zhang. This is an open-access article distributed under the terms of the Creative Commons Attribution License (CC BY). The use, distribution or reproduction in other forums is permitted, provided the original author(s) and the copyright owner(s) are credited and that the original publication in this journal is cited, in accordance with accepted academic practice. No use, distribution or reproduction is permitted which does not comply with these terms.
*Correspondence: Yufeng Dai, eXVmZW5nZGFpQGl0cGNhcy5hYy5jbg==; Lei Wang, d2FuZ2xlaUBpdHBjYXMuYWMuY24=