- 1Institute for Risk and Disaster Reduction, University College London, London, United Kingdom
- 2Department of Civil, Environmental, and Geomatic Engineering, University College London, London, United Kingdom
- 3Scuola Universitaria Superiore (IUSS) Pavia, Pavia, Italy
Every year, natural hazards affect millions of people around the world, causing significant economic and life losses. The rapid progress of technology and advances in understanding of the highly complex physical phenomena related to various natural hazards have promoted the development of new disaster-mitigation tools, such as earthquake early warning (EEW) systems. However, there is a general lack of integration between the multi- and cross-disciplinary elements of EEW, limiting its effectiveness and applications for end users. This paper reviews the current state-of-the-art in EEW, exploring both the technical components (i.e., seismological and engineering) as well as the socio-organizational components (i.e., social science, policy, and management) of EEW systems. This includes a discussion of specific evidence from case studies of Italy, United States’ West Coast, Japan, and Mexico, where EEW systems have reached varying levels of maturity. Our aim is to highlight necessary improvements for increasing the effectiveness of the technical aspects of EEW in terms of their implications on operational, political/legal, social, behavioral, and organizational drivers. Our analysis suggests open areas for research, associated with: 1) the information that needs to be included in EEW alerts to implement successful mitigation actions at both individual and organizational levels; 2) the need for response training to the community by official bodies, such as civil protection; 3) existing gaps in the attribution of accountability and development of liability policies involving EEW implementation; 4) the potential for EEW to increase seismic resilience of critical infrastructure and lifelines; 5) the need for strong organizational links with first responders and official EEW bodies; and 6) the lack of engineering-related (i.e., risk and resilience) metrics currently used to support decision making related to the triggering of alerts by various end users.
Introduction and Motivations
In recent decades, there has been an increased tendency in the literature to investigate both the technical and the social-science-related aspects of disaster prevention/mitigation, preparedness, response, and recovery. Events such as the 2004 Indian Ocean earthquake and tsunami, and the 2011 Tohoku (Japan) earthquake, tsunami, and nuclear meltdown, have raised awareness about the various domains/disciplines involved in risk forecasting/management strategies and the need to develop holistic approaches to early warning methods at a broader scale. According to the definition proposed by United Nation’s Office for Disaster Risk Reduction (UNDRR), early warning systems can be defined as “An integrated system of hazard monitoring, forecasting, disaster risk assessment, communication and preparedness activities, that enable individuals, communities, governments, businesses and others, to take timely action to reduce disaster risks in advance of hazardous events” (UNDRR, Terminology updated February 2017).1 The official definition also differentiates between early warning systems and multi-hazard early warning systems, suggesting the creation (where possible) of new platforms that could address several hazards and/or their impacts, increasing efficiency and coordination (UNISDR, 2017).
The topic of early warnings has acquired considerably more interest also in practice, which is reflected in various policies and international guidelines. In 2015, the member states of the United Nations endorsed the Sendai Framework for Disaster Risk Reduction (UNISDR, 2015), where it is specified that early warning must be a priority and early warning systems have to be substantially evolved by 2030 (UNISDR, 2015). Early warning systems can also play a crucial role in mitigating highly complex events, such as cascading and interacting hazards and risks (Pescaroli et al., 2018). In particular, Pescaroli and Alexander (2018) suggested that researchers should better integrate the technical components of early warning with practices of organizational resilience, for supporting the management of decisional uncertainties. Common training with these systems, including simulation exercises/drills, are also essential.
We specifically focus on earthquake early warning (EEW hereinafter) systems in this study. These systems combine real-time seismic instruments, fast telemetry capability, data processing software and methodologies/models/algorithms that can 1) provide real-time seismic-source information (e.g., rupture location and magnitude) and/or the ground-shaking intensity of ongoing earthquakes in the early stage of fault rupture; and 2) issue (based on some decisional rule) real-time warnings to the public or other end users in large urbanized areas before they experience the strong shaking that might cause damage/loss. The warning triggered by an EEW system can be considered a tool to improve emergency preparedness/rapid response and ultimately to minimize economic and human losses during earthquakes (e.g., Heaton, 1985; Wieland, 2001; Strauss and Allen, 2016). In the specific case of EEW, even recent review studies, such as the one by Allen and Melgar (2019), tend to focus on the technical aspects of EEW systems, without providing a full and systematic description of the multi- and cross-disciplinary challenges associated with EEW operation.
Implementation of EEW systems should always consider local specificities, for instance in terms of risk perceptions at both individual and community levels, governance and institutional arrangements (Twigg, 2003). New EEW methodologies (and their practical implementations) require a process of outreach and capacity building that should go beyond the seismology (and engineering) community (Allen et al., 2009b). EEW failures are not just technical in nature, such as missed or false alarms, but may be due to organizational weaknesses at the institutional level, i.e., lack of response training to end users/community by official bodies, such as civil protection. According to Herovic et al. (2019), there are still open questions on how societal drivers (such as the understanding of local culture and organizational needs), interact with both technological choices (such as decision support platforms and uncertainty modeling/communication) and information delivery (practices and policies needed for action). A better understanding on how decisional uncertainties in the warning process could influence preparedness or mitigation strategies, for example, in terms of differences in the available lead time and expected ground shaking in specific regions, is urgently needed (Wald, 2020).
This paper aims to develop a state-of-the-art review of both technical (seismology and engineering) and socio-organizational (social science, policy and management) components of EEW, in order to understand common gaps for research and practice. We aim to address some key questions that have had limited examination previously, such as: 1) What interactions, if any, exist between the technical and the socio-organizational components of EEW? 2) What improvements are required in the socio-organizational components to make EEW systems more effective? 3) What lessons can be learned from EEW systems implemented around the world?
Our review will focus in particular on case studies of Italy, United States’ West Coast, Japan, and Mexico. The choice of these case studies will be explained in the methodology section, while the common lessons learned will be integrated with other evidence from worldwide cases in the discussion section. The conclusions will highlight directions for future research.
It is worth noting that throughout this paper, “alert” and “warning” will be used as synonyms to indicate “an alarm signal or message coupled with a recommendation or order to take action such as mobilize or evacuate” (Alexander, 2002).
Beyond the Technical Components of Earthquake Early Warning Systems
The first challenge of approaching EEW systems from a multi- and cross-disciplinary perspective is deciding which components to include in the review, and how they interact with each other. The Third International Conference on Early Warning held in 2006 (UNISDR, 2006) defined the overall conceptual framework of early warning systems, highlighting the need to integrate four inter-related elements in their development. Effective “end-to-end” and “people-centered” early warning systems should include: 1) disaster risk knowledge, based on the systematic collection and understanding of data on the dynamic interrelations of exposure, hazards, and vulnerabilities; 2) detection, monitoring, analysis and forecasting of the hazards and possible consequences; 3) dissemination and communication, by an official source, of authoritative, timely, accurate and actionable warnings and associated information on likelihood and resulting impact. Multiple channels must be identified and used to reach the exposed population/users, defining simple messages and useful information; and 4) preparedness at all levels to respond to the warnings received. This should include emergency planning and community awareness education. These four interrelated elements need to be coordinated within and across sectors/disciplines for an early warning system to work effectively and to include a feedback mechanism for continuous improvement. Failure in one element or a lack of cross-component coordination could lead to failure of the whole system.
Moreover, the document by UNSIDR (2006) pointed out some cross-cutting issues, including the need for effective governance and institutional arrangements to support the development and implementation of early warning systems (including the legal and regulatory frameworks), and the involvement of the local community to activate bottom-up strategies for vulnerability reduction (UNISDR, 2006). According to Lindell et al. (2007), an essential element of warnings is that the communication has to produce a response action, so the information must be received and understood. Therefore, the technical elements cannot be considered alone but strategic decisions must be made on how the dissemination process is developed, defining appropriate communication mechanisms (e.g., television broadcasts and social media) according to the time of the day, and which elements of local culture, risk perceptions and people behaviors can influence this process. Similarly, Smith and Petley (2009) highlighted that warnings are more useful when information on upcoming hazards is combined with advice on short-term actions, such as the activation of evacuation procedures (if the warning time allows).
Various authors have suggested that the implementation of the technical components of early warning systems may be inadequate on its own (e.g., Quarantelli, 1984; Basher, 2006; Kelman and Glantz, 2014; Allen and Melgar, 2019). A classic work by Quarantelli (1984) defined very clearly that the reactions to warning for imminent threats are generally driven by factors such as institutional development and type of dissemination (formal vs. informal), perceived relevance, content (specific vs. general), proximity to the hazard, previous experience and validation (e.g., interactions with others). Effective early warning systems should not rely on a “top down” approach in which the emergency planning choices are imposed on the citizens; instead, these choices should be socially embedded. Patterns of inadequate engagement in the design and development of an early warning system could lead to a perceived lack of ownership, with consequent mistrust in the authorities/experts managing it and weaker political and economic support for further developments (Basher, 2006). Kelman and Glantz (2014) pointed out that the best approach to the design and implementation of an early warning system should be one where communities/end users are involved from the early phases of development rather than toward the end, in order to keep the technical tools oriented to their needs and specificities.
Describing specifically EEW systems, Allen and Melgar (2019) highlighted three main categories of users that need to be considered: a) individuals that undertake personal decisions and actions; b) automated response applications that need an institutional and company/organization background on how to implement the process (e.g., automatic stop to railroads); and c) individuals and institutions/organizations that use the information for situational awareness. In each context, users are likely to behave differently, and some categories may be more prominent than others. It has also been highlighted that early warning systems need to integrate mechanisms to incorporate feedback and experience from users to improve the effectiveness of how a warning is translated into action (e.g., Basher, 2006; Allen and Melgar, 2019). Moreover, when dealing with utility services and infrastructure, early warning systems should be considered/integrated into new practices of organizational resilience, defined as “the ability of an organization to anticipate, prepare for, and respond and adapt to incremental change and sudden disruptions in order to survive and prosper” (BS 65000:2014).2
Methodology
This paper develops a “traditional or narrative literature review,” according to the methodological criteria proposed by King et al. (1994) and Cronin et al. (2008).
First, we provide a brief overview of the technical components of EEW systems, on which most of the existing literature has concentrated, highlighting how they work, and the various challenges faced in EEW implementation. Secondly, we explore literature centered on the socio-organizational components of EEW, to understand how various related drivers can influence the effectiveness of the technical tools. We conduct the review through an in-depth analysis of four case studies covered by most of the literature, and then discuss it with the support of other worldwide evidence available. The case studies we selected represent different levels of advancement in the technical implementation of EEW systems, as explained by Allen and Melgar (2019):
–Italy, where EEW is technically advanced and subjected to real-time testing in the Campania region, but it is not open to the wider public;
–United States’ West Coast, where EEW is disseminated publicly to a fraction of the population (i.e., California). Selected end users receive alerts in areas where warnings are not public, and the development of a full public system is still in progress;
–Japan and Mexico, which have the most developed public alert distribution systems that disseminate alerts via multiple channels. EEW is available nationwide in Japan and is well integrated in safety procedures and practices, such as those for critical infrastructure management. In Mexico, EEW remains more fragmented and less integrated holistically into organizational resilience.
It should be noted that the selection of documents involved a replicable process to assure the consistency of our work and the possibility of future updates (Berg, 2001). An in-depth screening of scholarly literature was undertaken using both Google Scholar3 and Scopus,4 because the databases of the two research engines have different characteristics. Indeed, Google Scholar covers any document with a seemingly academic structure, including for example, conference proceedings, while Scopus comprises a database of documents–mainly journal papers—from approximately 5,000 publishers that have been selected by an independent committee.
We implemented a cross-reference approach between the identified documents to select additional publications of interest. A final check of gray documentation, such as policy reports, and open-source peer-reviewed papers/reports was performed on PreventionWeb,5 the knowledge platform for Disaster Risk Reduction of the United Nations.
We decided to focus our review primarily on literature published after 2004, i.e., in the period between 2005 and 2020, for the following reasons:
–Events, such as the 2004 Indian Ocean earthquake and tsunami, have significantly boosted the technical and non-technical development of early warning in general (and specifically EEW) since 2005;
–The technological evolution of society, associated with the widespread use of the internet, social media, and smartphones in the early 2000s, radically changed the components of disaster risk and the context of early warning systems, including EEW (Pescaroli and Alexander, 2018). This means that older papers will no longer contain valid concepts/lessons.
The key words used for the research were: “earthquake early warning systems,” “social sciences and early warnings,” “organizational resilience.” The first round of documents was then selected by searching for non-technical words/phrases in the abstract or title, including: social component/driver/sphere; organizational component/driver/sphere; operational component/driver/sphere; political component/driver/sphere; cultural component/driver/sphere; community; lessons learned; framework; review; state-of-the-art. The results in Scopus reflected the predominant focus of the literature on the technical components of EEW systems. Most of the work—approximately 1,700 papers—was concentrated in engineering, earth and planetary sciences, and computer sciences. Research in the domains of social sciences, medicine, business and management, decision sciences, arts and humanities, psychology, and economics comprised a total of 175 papers in English. However, many of these documents were not relevant for this study, because, for example, they focused on generic early warning systems or their technical components. Thus, the abstracts were filtered again to determine which papers: a) were focused on the socio-organizational aspects of EEW worldwide, with a particular focus on reactions to warnings or integration in policies; and b) supported the description of the case studies of interest.
Considered Domains
Each case study will be introduced by providing a general description of the local context and technical details of the EEW system implemented in the region. Then, relevant literature will be discussed with respect to different domains or spheres, i.e., operational, political and governance, social and behavioral, and organizational. Framing the discussion through the lens of the various spheres facilitates communication to a multidisciplinary audience. It is worth noting that some topics/findings can be seen as cross-cutting between different spheres, as discussed in the following sections; hence, the spheres should not be interpreted as rigid divisions.
Operational Sphere
This category includes tactical elements and tools that influence how EEW is developed and disseminated, such as social media/sirens, information on lead times (the time available before the arrival of the strong ground shaking at target sites) and expected impacts, including level of uncertainties in the predictions (when considered). In other words, the operational sphere includes what Alexander (2002) refers to as “the technological processes of conveying the message (i.e., communication systems).”
Political and Governance Sphere
This category includes contents associated with political and legislative issues, such as the legal framework in which EEW systems are implemented/operate, or accountability for dissemination and strategic decision making.
Social and Behavioral Sphere
This category includes people’s knowledge/understanding, perception, and opinion on EEW systems. The sphere is closely linked to the local context in which EEW systems operate, including the local culture, existing trust in institutions, local knowledge and experience with the considered hazard, informal training, household preparedness, community networks and vulnerability factors such as resource distribution.
Organizational Sphere
This sphere refers to organizations, such as civil protection, public utilities, and enterprises, that incorporate actions associated with EEW. We aim to understand the internal procedures of these organizations (if any) with respect to EEW, such as the activation of business continuity plans.
Earthquake Early Warning Systems: Physical Grounds, Technical Concepts, Methods, and Perspectives
From a technical perspective, the goal of an EEW system is to detect earthquakes in the early stages of fault rupture; to rapidly predict the source parameters (e.g., location and magnitude) and/or the intensity of the consequent ground motion; and to warn end users before they experience the strong shaking that might cause damage/loss (e.g., Zollo et al., 2014b). Figure 1 illustrates the technical principles of an EEW system, particularly in its regional configuration (see Regional Earthquake Early Warning Systems). The most damaging shaking is usually caused by seismic shear (or S-) and surface waves that travel at about half the speed of the fastest seismic waves (primary waves; or P-waves), and much slower than an electronic warning message (which essentially travels at 80% of the speed of light). EEW systems use P-waves (or early portion of S-waves) to detect strong shaking near the seismic source and transmit alerts ahead of the damaging S-waves at target sites. Hence, EEW systems can provide a few tens of seconds warning prior to the occurrence of damaging ground shaking at a target site, which allows for real-time seismic-risk-mitigating actions. Note that the actual/effective warning time depends mainly on the distance of the target site/structure/infrastructure with respect to the earthquake source. From an engineering perspective, if an earthquake is going to strike a target structure (or infrastructure/infrastructure component) and induce a response/damage level of enough severity, then planned emergency processes can be started immediately in order to limit potential losses. For instance, individuals can use the alert time to Drop-Cover-Hold On or move to safer locations within a building, reducing injuries and fatalities, or if the warning time allows, evacuate hazardous buildings. The alert time also (and especially) allows for many types of automated actions: stopping elevators at the nearest floor and opening the doors, opening firehouse doors, slowing high-speed trains to avoid accidents/derailments, turning traffic lights red—preventing cars from entering hazardous structures such as bridges and tunnels, shutting down gas pipelines to minimize fire hazards, shutting down manufacturing operations to decrease potential damage to equipment, saving vital computer information to avoid data losses, etc. This is not an exhaustive list but rather a snapshot of critical applications that could benefit from EEW.
According to the configuration of the networks/sensors, an EEW system can be conceptually classified as a regional or an on-site system (Figure 2). The following sections describe the specific features of each system type and provide some examples of locations where they have been installed.
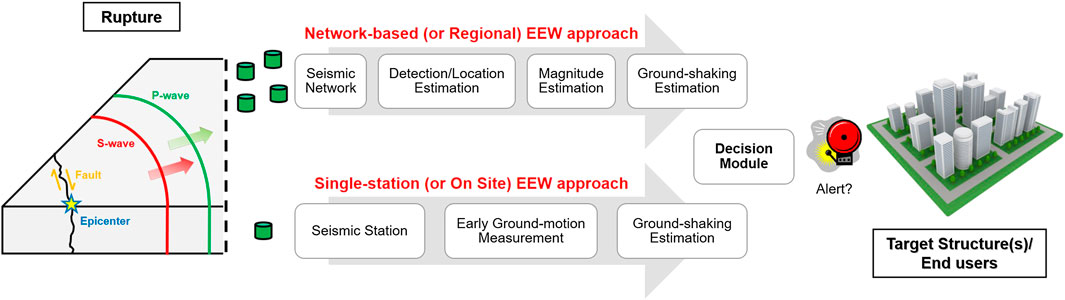
FIGURE 2. The two possible approaches to earthquake early warning (EEW). In the network-based (or regional) approach, the lead-time is equal to the S-arrival time at the target minus the first-P at the network minus any processing/computation time; in the single-station (or on-site) approach the lead-time is equal to the S-arrival time at the target minus the P-arrival at the target.
Regional Earthquake Early Warning Systems
A regional EEW system is based on a dense sensor network covering a geographical area of high seismicity. When an earthquake occurs, the relevant source parameters (e.g., event location and magnitude) are estimated from the early portion of recorded signals at sensors close to the rupture. Estimates of the source parameters are then used to predict, with a quantified confidence, a ground-motion intensity measure (IM) at a distant site where target structures/infrastructure of interest are located. Regional EEW systems typically require a number of stations that are triggered on arrival of the P-wave signal to provide stable early estimates of earthquake location (and source-to-site distance), earthquake magnitude, and ground-motion distribution in terms of the selected ground-motion IM.
Several approaches have been proposed for the real-time estimation of the earthquake location and magnitude, and are now implemented in various EEW algorithms around the world; a detailed review of these approaches is not within the scope of this paper but can be found in Zollo et al. (2014b) and Cremen and Galasso (2020), among many others. Approaches for regional EEW can be classified as either “point-source” (which simplistically treat the source as a concentrated volume) or “finite-fault” (which involve a more sophisticated and realistic characterization of source properties, considering the entire fault plane). Real-time point-source magnitude estimations are generally based on empirical relationships relating the earthquake size to parameters obtained in the early fraction (3–4 s) of P- and S-wave signals. These parameters are related to the low-frequency content of the ground motion (sensitive to the seismic moment) and can be associated with the maximum amplitude, dominant frequency, or the energy released by the event. Although many different studies have proven the reliability of these approaches for estimating the magnitudes of small-to-moderated events, it has been shown that they are not accurate for strong earthquakes (e.g., moment magnitude >6.5–7), due to saturation of the considered P-wave parameters. To solve this issue, different authors (e.g., Colombelli et al., 2012b) have proposed to estimate magnitude over longer time windows of the recorded P-wave and/or the S-wave signal (reducing the available lead time), to yield more accurate final values. Finite-fault magnitude calculations provide even more reliable estimations of the size of an incoming event, at the cost of requiring a larger amount of data and time. They typically involve empirical magnitude-rupture length relationships (Böse et al., 2012) or the use of slip estimates inferred from displacement measurements by geodetic data collection systems, such as Global Positioning or Global Navigational Satellite Systems (e.g., Crowell et al., 2012).
The estimation of earthquake location has also been addressed by various authors. A relevant (and state-of-the-art) example is the evolutionary approach by Satriano et al. (2008), which starts when the first station is triggered by an ongoing event and refines the location estimate as time passes. This method is based on the equal differential time formulation and provides a probabilistic density function for the earthquake location in a 3D space accounting for information from both triggered and not-yet-triggered stations at each time step. Tests performed on both synthetic and real data have shown that when a dense seismic network (i.e., with a mean station spacing of about 10 km) is deployed around the fault zone, high-accuracy in the location estimate is achieved within 1–3 s after the first arrival detection.
The source location and magnitude estimations are continuously updated (for example, through Bayesian approaches; Iervolino et al., 2009) by adding new station data as the P-wave front propagates through the regional EEW network. These source parameter estimates are then used to predict the severity of the ground shaking at sites far away from the source, by using region-specific attenuation relationships or ground-motion models (GMMs). The prediction of different IMs (e.g., Iervolino et al., 2006; Convertito et al., 2008), conditional on those parameters, may be performed by analogy to the well-known probabilistic seismic hazard analysis framework but in real-time, as discussed in detail in Iervolino et al. (2006).
On-Site Earthquake Early Warning Systems
Site-specific, or on-site, EEW systems consist of an array of sensors or a single sensor located in the vicinity of a single target site or structure/infrastructure of interest. Site-specific systems provide estimates of peak-ground-motion IMs [e.g., peak ground acceleration (PGA), or peak ground velocity (PGV)] based directly on the amplitude and/or predominant period of the initial recorded P-wave signal. This is achieved by implementing empirical regressions that correlate measurements obtained on the P-wave recordings and the amplitude of the final IM at the site. An extensive review of these approaches is not within the scope of this paper but can be found in Cremen and Galasso (2020), among many others.
For example, Wu and Kanamori (2005) derived a relationship between the maximum amplitude of high-pass filtered vertical-component records obtained in the first 3 s of the recorded P-wave train and site-specific PGV. Adopting the onsite approach enables faster warning issuance as independent magnitude estimates are typically not required, in contrast to the regional EEW approach described above. However, the accuracy of the estimates is generally lower (with respect to regional system) as real-time data from one station only (or a very few stations) is used.
On-site EEW systems are frequently threshold-based, i.e., the warning is given when the measured P-wave peak amplitude exceeds a pre-defined critical threshold that is based on the predicted S-wave peak ground-motion amplitude (Zollo et al., 2014b). However, small earthquakes might produce large amplitudes due to high-frequency spikes, and therefore false alarms can be triggered. To overcome this issue, a more advanced approach was proposed in which combinations of P-wave peaks and the P-wave predominant period are merged into a single proxy to provide more confident warnings (e.g., Wu and Kanamori, 2005). This idea has been furthermore updated by Colombelli et al. (2012a) and Zollo et al. (2010), who have developed a threshold-based EEW methodology combining real-time measurements of both parameters (in a 3-s window after the first P-arrival time) at sensors located at increasing distances away from the epicenter of the earthquake. For alert issuance, the measured values of the P-wave parameters are compared to threshold values, which are set for a specific minimum magnitude and instrumental intensity.
A variation of the on-site approach is the so-called front-detection system. This system resembles a fence against seismic waves, where sensors are strategically located between the earthquake’s likely source and the target to be protected, and alerts are issued when two or more nodes of the array record a ground acceleration amplitude larger than a defined critical value (Zollo et al., 2014b). Front-detection systems are especially useful when there is a significant distance between the seismic sources and the targets of interest, and ample warning time can be provided (Iervolino, 2011). This type of EEW approach is used on the west coast of Mexico to provides warnings for Mexico City (Espinosa-Aranda et al., 2011). Mexico City is located, on average, 300 km away from the west coast area of the country, where the most significant potential sources of seismicity are located (Lockman, 2005; Asgary et al., 2007; Wurman et al., 2007).
Engineering Approaches to Earthquake Early Warning
Research and development of EEW applications have not yet been emphasized in the engineering community, despite the very rapid seismology-centered improvements of EEW discussed in Regional Earthquake Early Warning Systems and On-Site Earthquake Early Warning System. In practice, two fundamental problems of EEW restrict its real-life implementation from an engineering viewpoint: 1) short warning time, particularly in epicentral areas; and 2) large uncertainty in the predicted ground motion (and consequent damage/loss). Regarding 1), to maximize warning time, the system must minimize delays in data acquisition/processing, communication, and delivery of alerts (e.g., increasing the number and density of seismic stations). Regarding 2), EEW information always involves some uncertainty due to the real-time estimation of source parameters and traditional uncertainties involved in probabilistic seismic hazard analysis (Iervolino et al., 2006). EEW applications may produce a substantial economic loss if a false alarm occurs (e.g., due to business interruption), ultimately affecting large communities (e.g., in the case of emergency stop of lifelines). On the other hand, there is a complex trade-off between the potential costs of false (and missed alarms) and the available warning time: as the seismic network collects more data on the earthquake, predictions will improve, but the time until shaking will decrease (Iervolino et al., 2009). The short warning time means that automated decision and mitigation actions are usually the preferred (or the only) option. From an engineering perspective, real-time probabilistic assessment of source parameters (e.g., magnitude and source-to-site distance) and/or earthquake-induced ground-motion IMs is a key link between real-time seismology and structural performance. However, it is well known that IMs may be poor indicators of structural response, consequent damage level and expected loss and therefore are not reliable metrics for deciding whether to issue an alarm. Direct real-time structural response or even loss (i.e., costs) quantification for a structure of interest is a sounder approach for warning management/alarm threshold setting.
Real-time engineering consequence quantification may be carried out within the framework of performance-based earthquake engineering (PBEE; e.g., Porter et al., 2007), as discussed in detail in Iervolino (2011), estimating the seismic performance of structure/infrastructure/engineering systems in terms of metrics of interest to stakeholders (i.e., “dollars, deaths, and downtime”), considering individual building/infrastructure components (structural, non-structural and contents), and accounting for all important sources of uncertainty. To achieve this, accurate yet (computationally) efficient regional structural response prediction methods must be coupled with real-time seismic hazard analysis to assess, in real-time, seismic damage and associated losses in order to trigger planned mitigation actions (e.g., alerting occupants, controlling elevators, etc.). Some recent studies (e.g., Maddaloni et al., 2013; Velazquez et al., 2017) have also discussed the control of structures as a possible advanced structural engineering application of EEW, especially in areas where the available warning time is very short. For instance, a building could change its dynamic properties (e.g., through seismic base isolation or structural damping) within a few seconds (or milliseconds) to better withstand the approaching ground shaking. The combined use of EEW and structural control, particularly semi-active devices, may reduce the structural vulnerability (and resulting losses) of specific systems. For instance, critical buildings that must be operational for emergency management purposes right after the event (e.g., hospitals, fire stations, or lifelines) could benefit from these combinations.
A PBEE approach can allow one to compare the expected losses corresponding to the three different possible outcomes of any EEW system: 1) performing an accurate mitigation action; 2) triggering false alarms; and 3) missing alarms. PBEE could additionally be combined with methods for multi-criteria decision analysis to create an advanced engineering-oriented decision-support system for EEW (e.g., Cremen and Galasso, 2020). This type of system would contain a harmonized mathematical framework for explicitly measuring all design variables that are integral to assessing the feasibility of implementing a given action (Iervolino, 2011), i.e., a) the required warning time; b) the false alarm acceptability; and c) the expected loss mitigation and related costs.
Case Studies Analysis: Italy, United States-West Coast, Japan, and Mexico
Four case studies have been considered for this state-of-the-art review: Italy, United States-West Coast, Japan, and Mexico. We explain the technicalities of each system and provide a description of the operational, political, socio-behavioral and organizational spheres, as described in Considered Domains.
Italy
The EEW system in Southern Italy has been implemented since 2005 due to the high seismicity in the Southern Apennine area directly affecting the Campania-Lucania region, where large urbanized cities, like Naples, are located. According to Zollo et al. (2014a), the system relies on the Irpinia Seismic Network (ISNet), which is formed of 31 seismic stations distributed in the Campania-Lucania region. The system is regional, and is based on a methodology that predicts the source parameters of the ongoing earthquake using various algorithms that are loaded into the main software platform called PRobabilistic and Evolutionary early warning SysTem (or PRESTo; Emolo et al., 2016).
PRESTo estimates the hypocenter location of an earthquake using the “RTLoc” approach proposed by Satriano et al. (2008), which is based on the equal differential time formulation introduced in Regional Earthquake Early Warning Systems. The magnitude of an earthquake is estimated by PRESTo according to the “RTMag” algorithm proposed by Lancieri and Zollo (2008). With location and magnitude defined, GMMs for the Campania-Lucania region are implemented to estimate the expected values of PGA, PGV, and instrumental intensity (or even macroseismic intensity) at the sites of interest (Satriano et al., 2011).
Several performance tests have been carried out on the system, using large and small historical earthquakes. If a dense seismic network is deployed around the fault region, PRESTo can provide reliable estimations of the location and magnitude of the event, as well as ground-motion intensities, within 5–6 s from the event origin time (Zollo et al., 2014a). In addition, Picozzi et al. (2015) considered the whole of Italy in a feasibility study to explore the potential of implementing a nationwide EEW system based on the PRESTo framework. The authors explained that reliable messages about large earthquakes could be provided to the community with lead times of the order of 25 s in several urbanized areas. This means the alarm could reach hundreds of municipalities in enough time, and residents could be supported by related education and training on basic protective actions (e.g., Drop-Cover-Hold On). However, despite the advanced, state-of-the-art algorithms used in PRESTo, public warnings are still not fully operational in the Campania Region.
Operational Sphere
When PRESTo issues an alarm, this is shared to a list of recipients (mainly researchers) that have been selected as beta testers by the system developers. These recipients have access to estimated earthquake parameters registered at every station in the network, estimates of the location and magnitude of the earthquake as well as arrival time, estimates of PGA, PGV, and other IMs at target sites, and theoretical P- and S-wave propagation paths. Users can adjust the threshold for triggering a warning alarm, which is disseminated via the Internet and SMS (Satriano et al., 2011).
Political and Governance Sphere
At the political and governance levels, there have been preliminary attempts to publicly operate an EEW system in Campania. However, the Italian Department of Civil Protection has prioritized different earthquake risk mitigation actions above EEW, such as reduction of the vulnerability of critical infrastructures and improved public seismic risk awareness (Clinton et al., 2016). More attention has been given to tsunami warning systems in which seismic detection, location, and magnitude estimations are integrated together (Bernardi et al., 2015).
Social and Behavioral Sphere
There is no specific study addressing the development of a holistic and bottom up EEW system in Italy, with explicit focus on the general public. This research gap is particularly pertinent in the context of this case study, given that previous work has highlighted a strong need for improving training and information practices of (general) earthquake preparedness in Italy (e.g., Pescaroli et al., 2012).
Organizational Sphere
Literature on the organizational sphere is very limited for Italy. Esposito and Emolo (2014) carried out a study to address the feasibility of adopting the Campania EEW system for the Circumvesuviana Napoli railway system. Although the research was quite preliminary in nature, the authors conclude that there is potential for EEW to be applied to the railway system in the region, in line with evidence of other case studies such as California.
Different authors have addressed the possibility of equipping existing seismic networks/stations with EEW capabilities for the entire Campania region. Emolo et al. (2016) presented a region-wide (Campania) case study that focused on public schools. The study finds that 3–5 s are enough for well-trained students to find shelter and protect themselves before the arrival of earthquakes. The authors ultimately conclude that implementing EEW systems for schools in the examined region would be effective, given that the largest concentration of residents in Campania is located in coastal areas away from where the most damaging earthquakes occur along the Apennine chain. The aforementioned work was motivated by a smaller case study presented by Picozzi et al. (2015), in which the EEW system of the Campania Region was tested in a specific school located in the Irpinia region. For this very particular case, it was shown that alerts can be provided to occupants of the school 13 s before the arrival of strong shaking in line with the historic M 6.9 1980 Irpinia event.
United States–West Coast
A prototype regional EEW system for California was proposed by Allen and Kanamori (2003) as a mitigation strategy to reduce potential impacts from earthquakes in the region of Southern California. The practical implementation of the system was initiated in 2007, funded mainly by the United States Geological Survey (USGS) and the following academic institutions: the California Institute of Technology (Caltech); the University of California, Berkeley (UC Berkeley); the University of Washington; the University of Oregon; the University of Southern California; and the Swiss Federal Institute of Technology (Böse et al., 2014; Kohler et al., 2018). The system, named CISN ShakeAlert, makes use of the existing California Integrated Seismic Network (CISN) that includes approximately 380 broadband and strong-motion stations across California. The stations detect ground motions coming from the high-potential seismic regions nearby, such as the San Andreas Fault, the Hayward Fault and the Cascadia subduction zone (Calkins and Lieberman, 2014; Johnson et al., 2016; Strauss and Allen, 2016; Given et al., 2018). The production prototype (v1.0) of ShakeAlert was established for California in early 2016 (Kohler et al., 2018). A newer version (v1.2) was established in 2017 for the West Coast states of Washington, Oregon, and California and provided earthquake notifications that were distributed to a group of local beta users.
The system was originally equipped with three different EEW algorithms (Böse et al., 2009; Chung et al., 2019) that worked simultaneously in order to estimate earthquake source parameters: 1) Onsite (Wu and Kanamori, 2005); 2) ElarmS (Allen et al., 2009a); and 3) Virtual Seismologist (Cua and Heaton, 2007).
In 2017, an evaluation of the ElarmS and Onsite algorithms resulted in a single unified point-source algorithm known as EPIC (Earthquake Point-source Integrated Code) that is primarily based on the most updated version of ElarmS, ElarmS-3 (E3) (Given et al., 2018). E3 was chosen as the basis of the system as it proved to be the fastest and most accurate algorithm of the three introduced above (Chung et al., 2019). In addition to EPIC, a second event source-detection algorithm called FinDer (Finite-fault Detector) has been included in the ShakeAlert platform. This finite-fault algorithm was proposed by Böse et al. (2012) to overcome the magnitude saturation challenges of point-source EEW approaches, as discussed in Regional Earthquake Early Warning Systems.
ShakeAlert combines information from the EPIC and FinDer algorithms through a decision module DM (Kohler et al., 2018). The DM receives event notifications from the EEW algorithms, which include the estimated earthquake location, magnitude and ground shaking, and creates alerts using a weighted average of the reporting algorithms (Chung et al., 2019).
The first “public” EEW in the West Coast of the United States was launched in Los Angeles in January 2019 (Hobbs and Rollins, 2019). Introduced as a smartphone application, and named ShakeAlertLA, this system provides warnings to 800,000 subscribers. Initially, the app alerted users if a magnitude 5.0+ was detected, but it was soon updated to provide warnings for Los Angeles county if earthquakes with magnitude 4.5+ were estimated. The California-wide EEW MyShake mobile application was launched in October 2019, as a collaboration between Caltech, UC Berkeley, USGS, and the Californian Office of Emergency Services (Allen et al., 2019). Both ShakeAlertLA and MyShake are intended to deliver warnings by receiving a real-time earthquake feed from the ShakeAlert platform.
Operational Sphere
MyShake is designed to release a warning if a magnitude 4.5 earthquake, or bigger is detected by the system (Allen et al., 2019). The contents of the warning message include the earthquake’s time of arrival at a given location, as well as its location and magnitude. When it comes to individuals, warning messages include advice on how to prepare for an earthquake, such as Drop-Cover-Hold On. Aside from delivering messages, the app also works as a seismic sensor, allowing crowdsourcing of information, by storing motion and location data (for the recording mobile phone) that are immediately sent to the developers for further research and improvement of the app’s algorithms. Additionally, MyShake can be downloaded anywhere in the world, to obtain information about earthquakes that have occurred anywhere and to share individuals’ post-earthquake feedback (e.g., experiences and observations); however, EEW alerts are exclusively for Californian events. As mentioned by the developers,6 the system is still a prototype, but it is a considerable advancement toward a public EEW system in California.
Political and Governance Sphere
MyShake represents a first attempt at a public EEW system for California. Obstacles to accomplishing more advanced and comprehensive EEW operability include the lack of essential related funding (e.g., Federal Government contributions). However, some researchers have shown that implementing a complete EEW system in California would be a cost-effective strategy for reducing human and economic losses due to earthquakes in the state. Strauss and Allen (2016) developed a cost-benefit analysis to understand the possible advantages of developing an EEW for the West Coast of the United States. The study pointed out that the annual cost of operating a public EEW system for the entire United States-West Coast would be repaid if it saved just three lives, warned two semiconductor plants, slowed one rapid transit train to avoid derailments, reduced 1% of non-fatal injuries, and led to a 0.25% decrease in gas-related fire damage triggered by an earthquake.
Legislative action requirements present some additional challenges to achieving a fully operational EEW service in the state of California. Johnson et al. (2016) pointed out that representatives from 24 organizations (representing 14 important sectors of the state’s infrastructure and economy) declared some concerns about the levels of liability associated with decisions based on warnings given by the system, particularly those related to actions undertaken as a consequence of false warnings. Similarly, participants of the study perceived a strong relation between EEW systems and life safety, raising financial, political and equity concerns that need to be addressed in the early stages of the system’s implementation. Access to the physical infrastructure of the system and the information it provides, as well as ensuring a stable governance structure and the reliability of continuous state-wide funding are key points that study participants included in the list of concerns (Johnson et al., 2016). It was argued that the inclusion of partners and stakeholders could improve the performance of broadcast notifications for EEW. Johnson et al. (2016) highlighted that representatives of the electric, telecommunications and information technology sectors should be included in the California EEW system Implementation Steering Committee, in addition to the government bodies and academic institutions already involved.
Social and Behavioral Sphere
The social perceptions of EEW in California and the West Coast of the United States strongly suggest that there is common public agreement on the need to implement the service. A survey conducted by the Probolsky Research Group in 2016 (Allen and Melgar, 2019) indicated that 88% of respondents (out of a total of 1,000) agreed that the state government should fund the warning system, and also expressed their willingness to pay small taxations to improve it.7 Similar findings have been identified by Dunn et al. (2016), who performed a 2,595-participant study in the states of Washington, Oregon and California, suggesting again that the perceived benefits of EEW systems were enough to justify some ad-hoc small tax contributions. The survey by Johnson et al. (2016) found that availability of EEW to the wider public and local communities was viewed by relevant stakeholders as a credible way to improve earthquake educational levels, as well as to raise related awareness and preparedness of both individuals and organizations. Strauss and Allen (2016) highlighted that a West Coast EEW system could reduce injuries in earthquakes by more than half. This is because actions such as Drop-Cover-Hold On could be done in advance of strong shaking, mitigating the impact of non-structural falling hazards that accounted for 50% of the injuries in cases such as the 1989 Loma Prieta earthquake (Allen and Melgar, 2019). In addition, Porter (2016) showed that if the whole San Francisco Bay Area community were properly trained to carry out Drop-Cover-Hold On and a public EEW system was operational in the area, any advance warning that allows the implementation for Drop-Cover-Hold On would be enough for 19,000 people to avoid injuries or death. The literature identifies a further potential benefit of a publicly available West Coast EEW, which is the enhancement of psychological resilience to earthquakes. For example, the information provided by a warning may reduce anxiety and other mental disorders triggered by sudden unexpected ground shaking, as discussed by Johnston et al. (2016).
Organizational Sphere
The United States-West Coast case study lacks associated research on the organizational sphere of EEW. However, the survey by Johnson et al. (2016) provides some insights that could be used as the basis for further analysis. A first point that emerges from the survey is that some private companies/organizations are already investing in their own EEW systems, and it is worth investigating whether these private efforts can be redirected to improve and sustain a public service. Indeed, the 24 organizations that participated in the survey indicated that a state-wide EEW system would be a beneficial risk mitigation measure, as a few seconds of advance warning could make a positive difference to the success of measures such as Drop-Cover-Hold On. The results of the survey highlighted consensus among stakeholders that EEW could be used for increasing the safety of employees by reducing the time needed to address “life-safety issues and complete life-safety assessments by directly informing people so they can take preventative actions” (Johnson et al., 2016). Interviewees felt that EEW could enhance business continuity management by facilitating faster organizational movement and efforts, allowing a more efficient restoration of operations. It could therefore reduce organizational downtime, economic losses, and disruptions, which would benefit end users and residents that avail of the services provided by those organizations. A final point that emerged from the survey of Johnson et al. (2016) is the need to tailor risk-mitigation actions to different target sectors, given variations in life and safety concerns. For example, larger companies might have different social dynamics (i.e., behaviors of groups resulting from the interactions of individual group members) when compared to smaller ones, requiring distinct EEW trainings or scenarios that integrate some considerations of external factors such as the period of the day or night in which an earthquake occurs and its implications for working timetables or shifts. Moreover, it was evident that implementation of the EEW system requires the definition of practices and training that must be properly explained and understood, involving improved communication between the companies and first responders.
One of the partners involved in ShakeAlert is the Bay Area Rapid Transit (BART) network (trains) in San Francisco, which has implemented an automated train-braking mechanism that is triggered by the EEW system. It takes 24 s to bring a train traveling at 112 km/h to a stop; during peak commuting times, about 64 trains are in operation across the BART network—each carrying approximately 1,000 passengers—and up to 45 trains travel at 112 km/h at any one time. Even one derailment at such a speed would be devastating. Moreover, minimizing earthquake damage to trains and tracks results in faster resumptions of services, which in turn supports the post-event restart of regional businesses. The BART system responded successfully to the EEW alert before the arrival of the M 6.0 South Napa Earthquake in 2014. Despite the fact that no trains were running at the time (3:20 am), the right procedures/protocol were in place (Strauss and Allen, 2016; Tajima and Hayashida, 2018).
Japan
The original on-site EEW system of Japan (developed in 1960) is considered the oldest implementation of EEW worldwide. This system was designed to offer protection against earthquakes for high-speed trains managed by the Japanese Railway System (Nakamura and Saita, 2007). Nakamura (1988) improved the initial platform by developing the Urgent Earthquake Detection and Alarm System (UrEDAS), a front-detection algorithm to detect the arrival of P-waves and estimate earthquake source parameters. Based on 3 s of the incoming P-wave train at a single station, UrEDAS calculates the magnitude of the event, the amplitude of the recorded motion and the epicenter of the earthquake (Nakamura and Saita, 2007). Compact UrEDAS is a complementary on-site EEW approach for the protection of Japanese Railways, estimating the “expected destructiveness” of the earthquake immediately from the earthquake motion just 1 s after the station has registered the P-wave arrival. In addition to train lines, Compact UrEDAS became operational in metro systems across Japan in 1998, following the Kobe earthquake in 1995 (Allen et al., 2009b).
A nationwide EEW system has been operating in Japan since 2006, which is managed by the Japanese Meteorological Agency (JMA) (Doi, 2011). The network is formed of 1,000 seismic stations deployed around the Japanese territory, spaced 20–25 km apart (Brown et al., 2011). The system follows a step-by-step algorithm that improves the estimations of earthquake magnitude and location as time passes (i.e., as more stations record the ground motion in time).
The hypocenter of the earthquake is determined using the approach proposed by Horiuchi et al. (2005), which geometrically constrains the location using geographical information on stations that have and have not yet recorded the arrival of P-waves (Nakamura et al., 2009), similar to the RtLoc algorithm mentioned in Italy. The magnitude of the event is calculated using separate scaling relationships between magnitude and the displacement amplitudes of the recorded P- and S-waves (Kamigaichi et al., 2009); the appropriate relationship to implement at a given time step (i.e., P- or S-wave one) is determined based on the expected arrival time of the S-wave.
The magnitude and location estimates of the nationwide system are then used to predict the value of PGV on bedrock at each location of interest, according to the GMM proposed by Si and Midorikawa (2000). Site amplification factors are used to convert the calculated amplitudes to peak velocities at the surface, which are finally translated to JMA seismic intensities via an empirical model (Kamigaichi, 2004). A warning is released whenever the value of predicted JMA intensity is larger than 3 (for advanced users, such as elevators operators and factory managers) or larger than 4 (for the general public) (Hoshiba et al., 2008; Hoshiba, 2014).
Operational Sphere
In Japan, warnings are disseminated to users that fall into two main categories (Hoshiba et al., 2008; Hoshiba, 2014): the first category includes online limited advanced users (organizations) that receive the warning through internet or dedicated telephone lines, which facilitates automatic actions related to their businesses (i.e., control of elevators) (Kamigaichi et al., 2009; Doi, 2011); this warning is intended for offices, schools, industry companies and critical facilities. The second group of users is the public that receive the warning and decide themselves on the optimal non-automated actions (depending on their circumstances) to be performed to mitigate their risk (Hoshiba et al., 2008). The second category of users receives the warning via media such as radio, e-mail, SMSs and the internet (Doi, 2011; Wald, 2020). The Fire and Disaster Management Agency also distributes warnings via speakers spread throughout different Japanese municipalities (Allen et al., 2009b), using a satellite communication system (J-Alert).
The contents of the warning messages differ for both categories: 1) advanced users receive the estimated magnitude and location of the epicenter, and lead time and predicted seismic intensities upon request (Doi, 2011); 2) the general public receives the earthquake’s origin time, the epicentral region name, and the names of subprefectural areas where the estimated JMA intensity is equal to or greater than four (Kamigaichi et al., 2009).
Previous work indicates that real-time warnings in Japan help to reduce seismic damage and losses for both factories/industrial facilities and critical infrastructure. For example, advanced warning facilitated by EEW enabled an electronics company located in the Miyagi Prefecture to cut the supply of chemicals in its factory 12 s before the arrival of ground shaking from the M 6.8 2007 Chuetsu-oki earthquake (Kamigaichi et al., 2009). During the same event, infants in a nursery located in the Fukushima prefecture moved into a safe zone 30 s before the earthquake hit the area. Offices, schools, shopping malls, and other factories also benefitted from the warning (Kamigaichi et al., 2009).
Political and Governance Sphere
The JMA has provided training to the population of Japan since 2007, when the system was launched publicly (Tajima and Hayashida, 2018). When an estimation is performed at a seismic station, the resulting values of earthquake source parameters and expected ground shaking are transmitted to the JMA on a real-time basis. JMA is then responsible for nationally disseminating the message, through disaster management organizations and broadcast companies (Doi, 2011). Also, many local governments (e.g., Tokyo) have installed EEW systems in public schools (Hoshiba, 2014). Kamigaichi et al. (2009) adds that implementing EEW in Japanese public facilities is a decision taken by the facility manager. JMA develops and disseminates public guidelines and manuals to understand the operability and capabilities of an EEW system put in place. These guidelines are also available to the public on the JMA’s website (in Japanese only).
Social and Behavioral Sphere
After the 2011 Tohoku Earthquake, JMA collected 2,820 survey responses from the general public of Iwate, Miyagi, and Fukushima, aiming to understand whether these people found EEW useful in general. The results of the survey indicated that 87% of the respondents were familiar with EEW systems and found them useful (Fujinawa and Noda, 2013). Positive public reaction and agreement toward EEW implementations in Japan can be also found in Hoshiba (2014), Nakayachi et al. (2019), and Santos-Reyes (2019). In addition, Fujinawa and Noda (2013) highlighted that the warning issued during the 2011 minor tremor provided people with a valuable chance to prepare for the strong incoming shaking. Users expressed a positive attitude toward false alarms after the Tohoku earthquake, arguing that they represented an opportunity to practice protective actions after the alarm is triggered (Allen et al., 2017). Ohara (2012) also carried out a survey in the Tokyo area to understand EEW awareness, before and after the 2011 Tohoku earthquake. The results present a remarkable increase in awareness toward EEW and the useful pre-event actions it accommodates. In addition, the results showed that after the 2011 event, people were more familiar with the technical limitations of the warning system and agreed that they prefer having a system that might provide false alarms than not having one at all (Ohara, 2012).
Warnings in Japan have clearly provided the population with enough time to implement risk-prevention strategies in past events, but Nakayachi et al. (2019) carried out a survey that revealed a possible practical issue: users tend to primarily react mentally and not physically, and therefore remain still once a warning is issued, without taking any kind of protection actions (Nakayachi et al., 2019). The reasons why most of the interviewees did not react after the alert mostly include: 1) “I expected small intensity, so I thought it was not necessary to do anything”; 2) “I thought the place I was in was safe”; and 3) “I did not know what to do”. Reason 1) seems to be related to the fact that the warning just indicated “strong shaking incoming” without any specific information about the available warning time nor the actual intensity expected at the area (Nakayachi et al., 2019) (for instance in the case of EEW delivered via speakers). It is of great concern for the JMA that individuals do not know what has to be done once the alarm is triggered (Doi, 2011). For these reasons, JMA has distributed brochures and videos among the Japanese population highlighting various scenarios that explain the best way to get prepared before the arrival of the incoming shaking (Doi, 2011). On the other hand, it has been noted that advanced users (i.e., organizations) have a clear understanding of the actions to be taken when an EEW is released (Doi, 2011).
Organizational Sphere
Public and organizational training on various EEW risk-mitigation actions has been put in place across different companies and institutions in Japan. Drills are performed nationally to exercise actions that can be taken once the EEW is triggered (Dai, 2011; Doi, 2011; Yamasaki, 2012; Fujinawa and Noda, 2013). Different levels of organizations and individuals participate in drills across the year, including: 1) organizations (private and public ones) across the country that carry out regular mandatory drills for their employees; 2) students, who are required to participate in a drill on the first day of each academic year; and 3) the general public, who participate in a nationwide drill on “National Disaster Prevention Day,” in collaboration with local governments.
Hospitals are a key piece of critical infrastructure that could benefit from EEW in Japan, according to previous research. Horiuchi (2009) analyzed potential EEW-related actions that could be performed to protect patients, staff, and delicate equipment, in a research project carried out at the National Hospital Organization Disaster Medical Center in Tokyo. By considering a scenario in which a patient is in the middle of a surgery when an earthquake occurs, the study provides a series of actions—both manual and automatic—that can be performed before the arrival of the tremor. Stopping elevators and opening all the doors of the premises are the main automatic actions identified for efficient evacuation of people once the EEW alarm is triggered. Interrupting medical procedures to prevent surgical errors on the patient due to shaking is the main manual EEW strategy discussed. A full list of the processes proposed can be found in Horiuchi (2009).
Finally, both UrEDAS and Compact UrEDAS provide warnings in about 90 locations along the lines of the Tokaido Shinkansen fast train, which travels with an average velocity of 360 km/h (Ashiya, 2004). If an alarm is triggered, trains are stopped if possible, or else run at low speeds to reduce potential damages.
Mexico
The Mexican EEW system was proposed by the Science and Technology National Council (CONACyT, Spanish acronym) and the Mexican National Research Council in 1986, as an urgent mitigation strategy against earthquake risk, given the M 8.1 1985 Michoacán earthquake that hit Mexico City with significant effects in terms of economic and human losses (Espinosa-Aranda et al., 2009; Espinosa-Aranda et al., 2011; Cuéllar et al., 2017). In August 1991, financed by Mexican City authorities, the Seismic Alert system (SAS) for Mexico City was deployed in the south-west of the country and had 12 seismic stations in the early stages of its implementation. In 1999, the state of Oaxaca, Mexico, experienced a catastrophic M 6.7 earthquake, which triggered the construction of the Seismic Alert System of Oaxaca (SASO), consisting of a network formed of 37 front-detection seismic stations.
Encouraged by the effective alerts of the two warning systems (SAS and SASO), the governments of Mexico City and Oaxaca decided to work together to create the Seismic Alert System of Mexico (SASMEX) in 2005. Designed as a front-detection system, the Mexican EEW system provides between 60 and 120 s of warning time to Mexico City (Cuéllar et al., 2018), which is significantly longer than the lead time provided in other countries (e.g., the Campania region, in Italy, where the maximum lead times are on the order of 15–20 s). A large warning time is possible with SASMEX, given that the distance between the network and Mexico City is 320 km (on average). The government of Mexico City financially funded the extension and update of SASMEX in 2010 to improve its performance, ultimately resulting in a total number of 97 stations within the network. SASMEX seismic sensors and alarms are currently located in seven states of Mexico (Jalisco, Michoacán, Colima, Guerrero, Oaxaca, Puebla, and Mexico City), (Cuéllar et al., 2017).
The detection and classification of large earthquakes performed by SASMEX is based on the 2 (ts − tp) algorithm (where ts refers to the arrival time of the S-wave, and tp to the arrival time of the P-wave) (Cuéllar et al., 2017). A second algorithm defined as tp + 3 was added to SASMEX in 2018, to calculate warning times for in-slab earthquakes that are located within the subducted Cocos Plate beneath central Mexico (Cuéllar et al., 2018). This algorithm does not consider S-wave arrivals, as historical events have shown that S-wave triggers result in the release of alerts that coincide with the arrival of ground shaking (no actual warning time delivered). SASMEX has proved to be an efficient and reliable system for the provision of long lead time warnings for Mexico City. In addition, it has been recognized as the first system in the world to provide public EEW (Lee and Espinosa-Aranda, 2003).
Operational Sphere
SASMEX warnings are issued through speakers located across Mexico City, and also disseminated through media messages via national television and radio broadcasts (Espinosa-Aranda et al., 2009; Suarez et al., 2009; Santos-Reyes, 2019). However, the speakers do not fully cover all 16 counties of the urban area in Mexico City, leaving some regions without any alert and with fragmented information about incoming earthquakes. Other services are available, such as private repeaters or speakers, but they require private investments in order to be accessed. The thresholds to issue an alert were updated for all cities that receive warnings in April 2019. Three different threshold cases have been established for triggering the alarm: 1) the estimated magnitude is larger than five and the estimated epicenter is within 250 km away of the target city; 2) the estimated magnitude is larger than 5.5 and the estimated epicenter is within 350 km of the target city; and 3) the estimated magnitude is larger than six and the estimated epicenter is outside a radius of 350 km.
A major concern highlighted by local media in Mexico,8 is the ineffective distribution of warning devices across the states that are equipped with EEW capabilities. In 2010–2011, Mexico City’s government and the federal government funded the extension of the country’s EEW system, paying for 88,000 extra alarming receivers. However, 60% of these devices are either stored, stolen/lost, installed excessively in some public buildings (e.g., 48 devices installed in buildings that only require 1) or simply missing in places where alarms are required, and some can even be purchased in e-shops. In addition, most of the installed speakers and receiver devices are located in the capitals of the seven states that receive alerts, such that few or no warnings are issued in other cities or towns that have been damaged in the past by ground shaking. For example, no speakers nor alarming devices were installed at the time of the September 7, 2017 earthquake in Juchitán, which was the Oaxacan town that registered the greatest number of casualties during the event.
A critical issue that commonly emerges in the literature is that the warning is limited to an alert about the incoming shaking, and does not provide any additional information about its characteristics, such as estimated arrival time and expected intensity/magnitude (Suarez et al., 2009). This incomplete information represents a major challenge, as indicated by a survey conducted after the September 2017 events in Mexico (Santos-Reyes, 2019), in which 2,400 residents of Mexico City expressed a preference for knowing the available lead time.
Political and Governance Sphere
The government has consistently lacked a clear strategy for disseminating SASMEX’s operational and warning procedures to end users. In addition, the system lacks efficient planning/coordination with first responders, and does not benefit from inter-agency coordination between local and federal governments (Suarez et al., 2009). Different issues have been highlighted in our analysis: first, the number of users is too low, considering the large population of Mexico City. Only a very small fraction of schools, first responders, critical service suppliers, and hospitals have access to the alerts (Suarez et al., 2009).9 Secondly, EEW systems are not exclusively managed by governmental bodies, presenting both advantages and shortfalls. Private organizations have developed mobile applications that provide warnings to the public located outside the range of SASMEX, improving the distribution of warnings but also creating possible sources of conflict. Reddy (2019) concludes that the most common of these applications, called “SkyAlert,” might jeopardize the functionality of the official Mexican EEW system. It has been noted that predictions from SkyAlert do not always match those of SASMEX, which can cause issues in terms of the reliability of both sources (Allen et al., 2017). Santos-Reyes (2019) suggested that decision makers, such as the civil protection department of Mexico City, should strengthen their policies and efforts to raise awareness of the capabilities (and limitations) of SASMEX. This need was clearly demonstrated during the M 7.1 earthquake on September 19, 2017. For this event, the EEW alert was issued in Mexico City at the same time as strong shaking occurred, which confused residents who commonly believed that EEW would always provide 60 s of warning time between the signal and the arrival of the strong shaking (Santos-Reyes, 2019). Users of SASMEX found it less useful after this event than they had before (Santos-Reyes, 2019).
Finally, the system does not provide coverage to all areas of the country that have experienced significant damage in previous earthquakes. Neither the state of Veracruz, where the second deadliest earthquake (1920) recorded in Mexico was located (Suárez and Novelo-Casanova, 2018), nor the state of Merelos, where the M 7.1 September 19, 2017 earthquake occurred, receive alerts from SASMEX. Other states of Mexico that have experienced the effects of earthquakes and do not receive EEW alerts include Estado de México and Tlaxcala.10 Finally, it has been shown that a denser seismic network in Mexico City could have resulted in a warning time of more than 20 s for the M 7.1 September 19, 2017 earthquake, instead of the 0–6 s lead time that actually occurred (Cuéllar et al., 2018; Tajima and Hayashida, 2018; Santos-Reyes, 2019).
Social and Behavioral Sphere
The lack of policies on delivering EEW-related training to the public is reflected in the socio-behavioral sphere. There is general agreement among the public that EEW is useful, but the majority of end users lack adequate training or tend to ignore the appropriate protection procedures to follow before, during and after an earthquake (Suarez et al., 2009; Santos-Reyes, 2019). Different analyses indicate that decision makers in Mexico need to improve communication and education practices among the residents of Mexico City, so that they react more efficiently to warnings and have a better understanding of the capabilities and limitations of SASMEX (Espinosa-Aranda et al., 2011; Santos-Reyes, 2019). Finally, it is important to note that residents from Mexico City view false and short-notice alarms as useful opportunities to conduct “additional exercises” (i.e., drills) on evacuation procedures (Allen et al., 2017; Allen et al., 2018; Reddy, 2019).
Organizational Sphere
All strategic buildings like hospitals, security buildings and offices, are legally required to contain devices for receiving EEW alerts in Mexico City (Suárez et al., 2018). Since 2015, more than 12,000 warning EEW speakers and devices have been installed across the city to broadcast alerts to different organizations and the general public (Santos-Reyes, 2019). However, the clear lack of training and information about response actions discussed previously is a major issue at the organization level, as it reduces the potential benefits of EEW for business continuity management (Suarez et al., 2009).9 A lack of communication between organizations and local and federal governments means that there is no formal strategy for identifying and prioritizing critical infrastructure, lifelines, and institutions to receive EEW alerts (Suarez et al., 2009).
On the other hand, drills are widely organized to practice the various risk-mitigation actions facilitated by the long warning times of SASMEX. For example, schools carry out these training procedures regularly (Suárez et al., 2018) and some critical facilities in Mexico City undertake EEW evacuation drills as part of their organizational procedures. A nationwide drill has been carried out once a year since 1986,11 when the National System of Civil Protection was launched in Mexico following the 1985 earthquake.12 Two more annual national drills (“Macrosimulacros”) were initiated in 2020.
Finally, EEW has been integrated into the organizational resilience of the Mexico City Metro (subway) (Cuéllar et al., 2014). Using radio receivers, the manager of the EEW system notifies train operators when it is necessary to stop trains and open the doors at the nearest station, so passengers can look for earthquake protection. However, the passengers do not receive the alarm while inside the carriage when the train is moving (Espinosa-Aranda et al., 2011).
Discussion
From the technical perspective, engineering-related research and development of EEW applications have not yet been emphasized, although very rapid improvements of EEW are being observed in the seismology community, as also noted in Earthquake Early Warning Systems: Physical Grounds, Technical Concepts, Methods, and Perspectives. An advanced, real-time PBEE framework is recommended for the design of structure-specific applications of EEW as an improved means of reducing casualties, economic and functionality losses due to earthquakes. Such a PBEE framework would require 1) accurately characterizing EEW-related uncertainty through an evolutionary and probabilistic approach for real-time seismic source and ground shaking modeling; 2) developing innovative computational building models for seismic alert and rapid damage assessment; and 3) pioneering technological and methodological solutions for interfacing real-time earthquake monitoring and engineering applications. This framework could additionally be unified with multi-criteria decision-making tools, to create an advanced engineering-oriented decision-support system for EEW that explicitly accounts for end-user preferences toward expected consequences associated with each potential action. The final goal of this type of system would be to enable end users to determine optimal real-time risk mitigating actions within the context of appropriately propagated and dynamically quantified uncertainties. This would ultimately increase the effectiveness of EEW within the continuum of technical risk-mitigation strategies that currently exist for earthquakes (Wald, 2020).
The four considered case studies highlight various common lessons learned from existing EEW systems. An interesting finding from countries with public EEW (i.e., Japan and Mexico) is that there is a clear tendency by residents to accept and tolerate false alarms (Allen et al., 2017; Allen et al., 2018; Reddy, 2019; Wald, 2020), which provide an opportunity to perform some additional evacuation exercises to those already implemented by institutions and governments. This evidence is in line with additional existing literature (Goltz, 2002), which indicates that false alarms can be perceived as unscheduled, inexpensive, evacuation exercises or drills. Finally, it has also been found in both Mexico and Japan that users prefer to receive false alarms from an implemented EEW system rather than not having any public EEW installed. However, no case study addresses possible negative mental health consequences that might be triggered in individuals given a false alarm. This is a significant gap in the state-of-the-art, as negative mental health effects could be particularly detrimental for people of vulnerable categories, such as those affected by Post-Traumatic Stress Disorder, which is the most common mental condition among earthquake survivors (Farooqui et al., 2017). As indicated by McBride et al. (2020), releasing too many false alerts can significantly reduce the public’s trust in a public EEW alert system, potentially endangering people if they consequently fail to take fast protective actions when necessary. To enhance trust in EEW, all of its limitations should be clearly communicated to end users and the public, including the potential for false alerts as well as very short warning times in specific areas (Wald, 2020).
A further element to consider in the discussion is the opinion of the public regarding EEW warnings across the case-studies. Public opinion of EEW warnings is a product of cultural or social features and is therefore region-specific. In Japan, most of the literature shows that residents of the country agree with the implementation of EEW and find it useful. However, Nakayachi et al. (2019) highlight that this is not always the case. In some regions of the country, the public does not believe that EEW alerts are effective, given their lack of detailed information about the strength of the incoming ground shaking and the expected arrival time. Related to the Mexican case-study, there is a common desire expressed by the public to receive more detailed information on the incoming event, so that the optimal risk-mitigation action can be undertaken (Santos-Reyes, 2019). This desire is shared worldwide, including by over 200 participants (organizations) of a survey that assessed the factors influencing organizational acceptance and use of EEW systems in California (Goltz, 2002). Tajima and Hayashida (2018) conclude that some types of information that can be used to determine the most appropriate actions to take, such as the arrival time, should be also be considered. In particular, the survey respondents in the study by Goltz (2002) indicated that mitigation actions such as “moving away from falling objects” or “shutting off gas lines” are the most appropriate measures in a short warning time (10 s), while “evacuating to an outside area” or “shutting down hazardous materials” are more effective options when a longer warning time is available (50 s). However, positive opinion on the use of detailed EEW alerts is not shared by other authors. For instance, Allen and Melgar (2019), Shrivastava (2003), and WMO (2017) argue that the processing of many details can delay responses, while simple warnings facilitate direct actions such as Drop-Cover-Hold On. Lindell et al. (2007) also indicates that warnings should be simple, clear and non-ambiguous, using appropriate language in a diverse environment. Finally, studies in the United States (Dunn et al., 2016) and Iran (Asgary et al., 2007) have identified public willingness to pay small taxations to contribute to the funding of EEW systems.
Official bodies, such as civil protection departments, are consistently regarded as crucial actors for the delivery of EEW training and education to the population (Gasparini et al., 2011), across all case studies examined. Concerns about the lack of effective political and organizational leadership related to EEW has been expressed in Mexico. For example, Santos-Reyes (2019) suggested that decision makers, such as the civil protection department of Mexico City, should increase their efforts to coordinate good practices of response to warnings, for instance through drills. This gap between EEW and monitoring services (civil protection and geological surveys) in Mexico has also been highlighted by Alexander (2015). Strong organizational links with representatives of official bodies and first responders are also required to make EEW a successful tool for enhancing business continuity in critical infrastructure such as energy, utilities, food supply, communications, and banking (Gasparini et al., 2011). As indicated by Tajima and Hayashida (2018), EEW systems are only effective if the resilience, strength, and operations of the system are ensured before ground shaking occurs.
Public transport systems, hospitals and schools are the most typical institutional beneficiaries of EEW (Gasparini et al., 2011). In all case studies examined, the transportation sector (i.e., the Circumvesuviana railway system in Naples, the BART system in San Francisco Bay Area, the Shinkansen train system in Japan and Mexico City’s Metro) has particularly clear potential to significantly benefit from EEW implementation. When a warning is released, these utilities can rapidly activate procedures to slow the traffic and to stop carriages to prevent likely damage and allow evacuation of occupants, if feasible. Similarly, it has been shown that schools can benefit from EEW because the consequences of false alarms will not be costly, but the alarms may have significant benefits for safety and support long term public education (Goltz, 2002). When it comes to hospitals, Horiuchi (2009) has listed a comprehensive series of protection/safety actions that can be carried out to safeguard the public, staff, equipment and materials, and the facilities themselves (e.g., operating rooms). These actions might reduce injuries and deaths of hospitals users and can allow a quick recovery and maintenance of facilities after an earthquake.
Figure 3 synthesizes the evidence derived from this discussion and summarizes the general findings for each sphere, considering all the case studies. The operational sphere, the political and governance sphere, the social and behavioral sphere, and the organizational sphere provide complementary considerations for the design and implementation of the technical components. This design and implementation process also requires a deep understanding of the local context as well as the prevailing application of legislative procedures, organizational standards and compliance assessments.
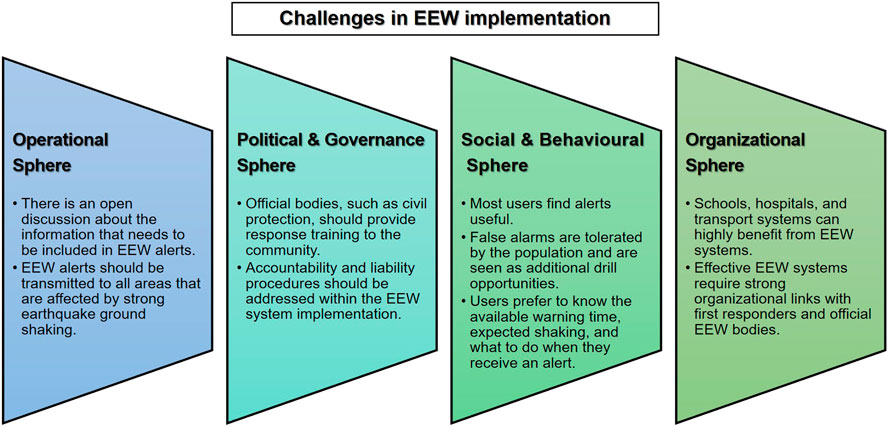
FIGURE 3. Challenges in implementing earthquake early warning (EEW) systems: common findings from the case studies, across all examined spheres.
The next section will introduce some open questions for future research and practice.
Conclusions
This paper developed a state-of-the-art review of the technical and socio-organizational components of EEW systems. This included a detailed discussion of specific evidence from case studies of Italy, California, Japan, and Mexico, where EEW systems have reached varying levels of maturity. The overall aim was to highlight improvements that are necessary to increase the effectiveness of the technical aspects of EEW in terms of their implications on the operational, political, social, behavioral, and organizational drivers.
From the technical perspective, EEW systems have benefitted from extensive seismology-related research and development and are now capable of rapidly yielding source characteristics/expected shaking estimations to a high degree of accuracy. However, engineering applications of EEW, which have the potential to significantly improve risk predictions (and therefore decision-making on the triggering of EEW alerts) for incoming events, rarely feature in the literature and are not used in real-life EEW implementations. Some studies have proposed to integrate the PBEE mathematical framework with EEW to quantitatively link seismological parameter estimations with structural performance consequences in real-time, while explicitly tracking all related uncertainties. Further work has suggested combining PBEE with multi-criteria decision-making (MCDM) techniques, to explicitly account for end-user preferences when determining real-time optimal risk-mitigation actions. A unified PBEE-MCDM approach would transform EEW into a powerful end-user-driven risk-management strategy for supporting seismic resilience.
In addition to significant technical improvements, there has been an increased awareness since the early 2000s around topics such as “people-centered” EEW systems. However, many multi- and cross-disciplinary aspects of EEW remain relatively unexplored, which could compromise EEW’s overall goal of increasing resilience and capacity.
First, our review has highlighted some open issues in the operational and socio-behavioral spheres. Most users find alerts useful and tolerate false alarms, viewing them as additional drill opportunities. However, there is an open discussion about the information that needs to be included in EEW alerts. The literature suggests that a ‘simple warning’ (e.g., no provision of the available warning time nor the characteristics of the incoming earthquake-induced ground shaking) is typically not preferred by users. Instead, they desire to know the available warning time, expected shaking, and what to do when they receive an alert. Similarly, organizations need information to facilitate the activation of prudent/cautious mitigation actions together with alert signals. It is worth noting that some literature argues that simple warning message may be enough and optimal as the processing of many details can delay responses, while simple warnings facilitate direct actions such as Drop-Cover-Hold On. This leads to open questions such as: What type of information is necessary to be communicated in EEW alert messages, to maximize their effectiveness? How should alert thresholds be determined, considering false alarm tolerance levels? How can a company perform shutdown operations if the time before the arrival of the shaking is unknown? What are the circumstances in which individuals evacuate, in a highly uncertain scenario?
Secondly, our review indicates that the effectiveness of EEW can be reduced by negative reactions within the political and governance sphere, and the organizational sphere. There is often little or no coordination between official bodies that provide warnings, and those organizations that may benefit from reliable EEW. For example, even where the technical components of EEW are perceived as useful, a lack of integration in operational functions and procedures can hamper their positive impact. The technical component may also be negatively influenced by the local context in terms of legislation, standardization, compliance, and management culture. Information and action on the political and governance sphere require special consideration when implementing EEW systems (Goltz, 2002; Gasparini et al., 2011). Complementary assessments should investigate questions such as Who is accountable for activating emergency procedures, following an alert, within an organization? Who is accountable for issuing alerts? What are the legal liabilities of disseminating false alerts?
All the examined case studies indicated that EEW is perceived as beneficial for critical infrastructure resilience. Our review found that hospitals, public transportation, and schools have particular potential to undertake risk mitigation actions when an EEW alert is received, assuming that the warning time is enough. However, Wald (2020) argues that there are only a limited number of EEW applications to critical infrastructure that have been well documented in the literature, many of which are hypothetical. In conclusion, further research is needed to understand if critical infrastructure should really be a priority target for EEW?
While this work is not exhaustive, it has leveraged a few pertinent case studies to provide important insight on the technical and socio-organizational components of EEW, and how their integration may be improved for more effective promotion of seismic resilience.
Author Contributions
GP and CG conceived and designed the study; OV, GC, and CG carried out the literature review/discussion on the technical components of EEW systems; GP defined the methodology, carried out the literature review/discussion on the socio-organizational component of EEWs, and supervised the study; and all the authors wrote the manuscript.
Conflict of Interest
The authors declare that the research was conducted in the absence of any commercial or financial relationships that could be construed as a potential conflict of interest.
Acknowledgments
GP and OV are supported by a British Academy/Leverhulme Small Research Grant by the United Kingdom’s Department for Business, Energy and Industrial Strategy (Grant Reference: SRG19/191797); and an Earthquake Engineering Field Investigation Team Award by the Institution of Structural Engineers in the UK. GC and CG are supported by the European Union’s Horizon 2020 research and innovation programme under grant agreement No 821046, project TURNkey (Towards more Earthquake-resilient Urban Societies through a Multi-sensor-based Information System enabling Earthquake Forecasting, Early Warning and Rapid Response actions).
Footnotes
1https://www.preventionweb.net
2https://www.bsigroup.com/en-GB/about-bsi/media-centre/press-releases/2014/november/Organizational-resilience-standard-published/
3https://scholar.google.com/intl/en/scholar/about.html
4https://www.elsevier.com/en-gb/solutions/scopus
5https://www.preventionweb.net
6https://eps.berkeley.edu/news/myshake-release-first-statewide-earthquake-early-warning-system
7https://www.moore.org/article-detail?newsUrlName=poll-nearly-nine-out-of-ten-california-voters-support-earthquake-early-warning-system
8https://www.animalpolitico.com/2017/09/gobierno-compra-alertas-sismicas-desaparecen-bodegas/
9https://www.seismosoc.org/news/mexicos-early-warning-system-perform-recent-earthquakes/
10https://www.milenio.com/estados/cuantos-estados-cuentan-con-alertas-sismicas
11https://verne.elpais.com/verne/2017/09/27/mexico/1506531283_511876.html
12https://mvsnoticias.com/noticias/estados/realizara-cnpc-macrosimulacro-de-sismo-en-septiembre/ (in Spanish)
References
Alexander, D. E. (2002). Principles of emergency planning and management. New York, NY: Oxford University Press, 340.
Alexander, D. E. (2015). Evaluation of civil protection programmes, with a case study from Mexico. Disaster Prev. Manag. 24, 263–283. doi:10.1108/DPM-12-2014-0268
Allen, R. M., Brown, H., Hellweg, M., Khainovski, O., Lombard, P., and Neuhauser, D. (2009a). Real-time earthquake detection and hazard assessment by ElarmS across California. Geophys. Res. Lett. 36, L00B08. doi:10.1029/2008GL036766
Allen, R. M., Cochran, E. S., Huggins, T., Miles, S., and Otegui, D. (2017). Quake warnings, seismic culture. Science 358, 1111. doi:10.1126/science.aar4640
Allen, R. M., Cochran, E. S., Huggins, T., Miles, S., and Otegui, D. (2018). Lessons from Mexico’s earthquake early warning system. Eos 99. doi:10.1029/2018eo105095
Allen, R. M., Gasparini, P., Kamigaichi, O., and Böse, M. (2009b). The status of earthquake early warning around the World: an introductory overview. Seismol. Res. Lett. 80, 682–693. doi:10.1785/gssrl.80.5.682
Allen, R. M., and Kanamori, H. (2003). The potential for earthquake early warning in southern California. Science 300, 786–789. doi:10.1126/science.1080912
Allen, R. M., Kong, Q., and Martin-Short, R. (2019). The MyShake platform: a global vision for earthquake early warning. Pure Appl. Geophys. 177, 1699–1712. doi:10.1007/s00024-019-02337-7
Allen, R. M., and Melgar, D. (2019). Earthquake early warning: advances, scientific challenges, and societal needs. Annu. Rev. Earth Planet Sci. 47, 361–388. doi:10.1146/annurev-earth-053018-060457
Asgary, A., Levy, J., and Mehregan, N. (2007). Estimating willingness to pay for a hypothetical earthquake early warning systems. Environ. Hazards. 7, 312–320. doi:10.1016/j.envhaz.2007.09.003
Ashiya, K. (2004). Earthquake alarm systems in Japan railways. J. Earthq. Eng. 4, 112–117. doi:10.5610/jaee.4.3_112
Basher, R. (2006). Global early warning systems for natural hazards: systematic and people-centred. Philos. Trans. A Math. Phys. Eng. Sci. 364, 2167–2182. doi:10.1098/rsta.2006.1819
Berg, B. L. (2001). Qualitative research methods for the social sciences. Boston, MA: Allyn and Bacon, 304. Available at: https://books.google.co.uk/books/about/Qualitative_Research_Methods_for_the_Soc.html?id=9SRHAAAAMAAJ (Accessed September 12, 2019).
Bernardi, F., Lomax, A., Michelini, A., Lauciani, V., Piatanesi, A., and Lorito, S. (2015). Appraising the early-est earthquake monitoring system for tsunami alerting at the Italian candidate tsunami service provider. Nat. Hazards Earth Syst. Sci. 15, 2019–2036. doi:10.5194/nhess-15-2019-2015
Böse, M., Allen, R., Brown, H., Gua, G., Fischer, M., Hauksson, E., et al. (2014). CISN ShakeAlert: an earthquake early warning demonstration system for California. Early Warn. Geol. Disasters, 49–69. doi:10.1007/978-3-642-12233-0_3
Böse, M., Hauksson, E., Solanki, K., Kanamori, H., Wu, Y.-M., and Heaton, T. H. (2009). A new trigger criterion for improved real-time performance of onsite earthquake early warning in Southern California. Bull. Seismol. Soc. Am. 99, 897–905. doi:10.1785/0120080034
Böse, M., Heaton, T. H., and Hauksson, E. (2012). Real-time Finite Fault Rupture Detector (FinDer) for large earthquakes. Geophys. J. Int. 191, 803–812. doi:10.1111/j.1365-246X.2012.05657.x
Brown, H. M., Allen, R. M., Hellweg, M., Khainovski, O., Neuhauser, D., and Souf, A. (2011). Development of the ElarmS methodology for earthquake early warning: realtime application in California and offline testing in Japan. Soil Dynam. Earthq. Eng. 31, 188–200. doi:10.1016/j.soildyn.2010.03.008
Calkins, A., and Lieberman, E. (2014). The benefits and costs of an earthquake early warning system in Washington State. Evans Sch. Rev. 5, 121–136.
Chung, A. I., Henson, I., and Allen, R. M. (2019). Optimizing earthquake early warning performance: ElarmS‐3. Seismol. Res. Lett. 90, 727–743. doi:10.1785/0220180192
Clinton, J., Zollo, A., Marmureanu, A., Zulfikar, C., and Parolai, S. (2016). State-of-the art and future of earthquake early warning in the European region. Bull. Earthq. Eng. 14, 2441–2458. doi:10.1007/s10518-016-9922-7
Colombelli, S., Amoroso, O., Zollo, A., and Kanamori, H. (2012a). Test of a threshold-based earthquake early-warning method using Japanese data. Bull. Seismol. Soc. Am. 102, 1266–1275. doi:10.1785/0120110149
Colombelli, S., Zollo, A., Festa, G., and Kanamori, H. (2012b). Early magnitude and potential damage zone estimates for the great Mw 9 Tohoku-Oki earthquake. Geophys. Res. Lett. 39, L22306. doi:10.1029/2012gl053923
Convertito, V., Iervolino, I., Zollo, A., and Manfredi, G. (2008). Prediction of response spectra via real-time earthquake measurements. Soil Dynam. Earthq. Eng. 28, 492–505. doi:10.1016/j.soildyn.2007.07.006
Cremen, G., and Galasso, C. (2020). Earthquake early warning: recent advances and perspectives. Earth-Sci. Rev. 205, 103184. doi:10.1016/j.earscirev.2020.103184
Cronin, P., Ryan, F., and Coughlan, M. (2008). Undertaking a literature review: a step-by-step approach. Br. J. Nurs. 17, 38–43. doi:10.12968/bjon.2008.17.1.28059
Crowell, B. W., Bock, Y., and Melgar, D. (2012). Real‐time inversion of GPS data for finite fault modeling and rapid hazard assessment. Geophys. Res. Lett. 39, L09305. doi:10.1029/2012gl051318
Cua, G., and Heaton, T. (2007). “The virtual seismologist (VS) method: a bayesian approach to earthquake early warning,” in Earthquake early warning systems. Editors P. Gasparini G. Manfredi and J. Zschau (Berlin, Germany: Springer), 97–132.
Cuéllar, A., Espinosa-Aranda, J. M., Suárez, R., Ibarrola, G., Uribe, A., Rodríguez, F. H., et al. (2014). “The Mexican seismic alert system (SASMEX): its alert signals,” in Broadcast results and performance during the M 7.4 punta maldonado earthquake of March 20th, 2012. Editors F. Wenzel and J. Zschau (Berlin, Germany: Springer), 71–87.
Cuéllar, A., Suárez, G., and Espinosa‐Aranda, J. M. (2017). Performance evaluation of the earthquake detection and classification algorithm 2(tS-tP) of the seismic alert system of Mexico (SASMEX). Bull. Seismol. Soc. Am. 107, 1451–1463. doi:10.1785/0120150330
Cuéllar, A., Suárez, G., and Espinosa‐Aranda, J. M. (2018). A fast earthquake early warning algorithm based on the first 3 s of the P‐wave coda. Bull. Seismol. Soc. Am. 108, 2068–2079. doi:10.1785/0120180079
Dai, A. (2011). “Early warning and emergency management in earthquakes,” in ICEMMS 2011–2011 2nd IEEE international conference on emergency management and management sciences, Beijing, China, August 8–10, 2008 (IEEE), 17–20. doi:10.1109/ICEMMS.2011.6015608
Doi, K. (2011). The operation and performance of earthquake early warnings by the Japan meteorological agency. Soil Dynam. Earthq. Eng. 31, 119–126. doi:10.1016/j.soildyn.2010.06.009
Dunn, P. T., Ahn, A. Y. E., Bostrom, A., and Vidale, J. E. (2016). Perceptions of earthquake early warnings on the U.S. West Coast. Int. J. Disaster Risk Reduct. 20, 112–122. doi:10.1016/j.ijdrr.2016.10.019
Emolo, A., Picozzi, M., Festa, G., Martino, C., Colombelli, S., Caruso, A., et al. (2016). Earthquake early warning feasibility in the Campania region (southern Italy) and demonstration system for public school buildings. Bull. Earthq. Eng. 14, 2513–2529. doi:10.1007/s10518-016-9865-z
Espinosa-Aranda, J. M., Cuellar, A., Garcia, A., Ibarrola, G., Islas, R., Maldonado, S., et al. (2009). Evolution of the Mexican seismic alert system (SASMEX). Seismol. Res. Lett. 80, 694. doi:10.1785/gssrl.80.5.694
Espinosa-Aranda, J. M., Cuéllar, ., Rodríguez, F. H., Frontana, B., Ibarrola, G., Islas, R., et al. (2011). The seismic alert system of Mexico (SASMEX): progress and its current applications. Soil Dynam. Earthq. Eng. 31, 154–162. doi:10.1016/j.soildyn.2010.09.011
Esposito, S., and Emolo, A. (2014). Final report for feasibility studies on EEW application to the Circumvesuviana Napoli railway. Strateg. Tools Real Time Earthq. Risk Reduct. REAKT. Available at: http://www.reaktproject.eu/deliverables/REAKT-D7.4.pdf (Accessed August 25, 2016).
Farooqui, M., Quadri, S. A., Suriya, S. S., Khan, M. A., Ovais, M., Sohail, Z., et al. (2017). Posttraumatic stress disorder: a serious post-earthquake complication. Trends Psychiatry Psychother. 39, 135–143. doi:10.1590/2237-6089-2016-0029
Fujinawa, Y., and Noda, Y. (2013). Japan’s earthquake early warning system on 11 March 2011: performance, shortcomings, and changes. Earthq. Spectra 29, 341–368. doi:10.1193/1.4000127
Gasparini, P., Manfredi, G., and Zschau, J. (2011). Earthquake early warning as a tool for improving society’s resilience and crisis response. Soil Dynam. Earthq. Eng. 31, 267–270. doi:10.1016/j.soildyn.2010.09.004
Given, D. D., Allen, R. M., Baltay, A. S., Bodin, P., Cochran, E. S., Creager, K., et al. (2018). Revised technical implementation plan for the ShakeAlert system-an earthquake early warning system for the West Coast of the United States. U.S. Geological Survey Open-File Report 2018-1155, 42 p. doi:10.3133/ofr20181155
Goltz, J. D. (2002). Introducing earthquake early warning in California: a summary of social science and public policy issues.
Heaton, T. H. (1985). A model for a seismic computerized alert network. Science 228, 987–990. doi:10.1126/science.228.4702.987
Herovic, E., Sellnow, T. L., and Sellnow, D. D. (2019). Challenges and opportunities for pre-crisis emergency risk communication: lessons learned from the earthquake community. J. Risk Res. 23, 349–364. doi:10.1080/13669877.2019.1569097
Hobbs, T., Rollins, C., and Rollins, C. (2019). Earthquake early warning system challenged by the largest SoCal shock in 20 years. Temblor. doi:10.32858/temblor.035
Horiuchi, S., Negishi, H., Abe, K., Kamimura, A., and Fujinawa, Y. (2005). An automatic processing system for broadcasting earthquake alarms. Bull. Seismol. Soc. Am. 95, 708–718. doi:10.1785/0120030133
Horiuchi, Y. (2009). Earthquake early warning hospital applications. Spec. Issue Early Warn. Nat. Disaster Mitigation 4, 237–241. doi:10.20965/jdr.2009.p0237
Hoshiba, M. (2014). Review of the nationwide earthquake early warning in Japan during its first five years. Earthq. Hazard Risk Disaster, 505–529. doi:10.1016/B978-0-12-394848-9.00019-5
Hoshiba, M., Kamigaichi, O., Saito, M., Tsukada, S., and Hamada, N. (2008). Earthquake early warning starts nationwide in Japan. Eos 89, 73–74. doi:10.1029/2008EO080001
Iervolino, I. (2011). Performance-based earthquake early warning. Soil Dynam. Earthq. Eng. 31, 209–222. doi:10.1016/j.soildyn.2010.07.010
Iervolino, I., Convertito, V., Giorgio, M., Manfredi, G., and Zollo, A. (2006). Real-time risk analysis for hybrid earthquake early warning systems. J. Earthq. Eng. 10, 867–885. doi:10.1080/13632460609350621
Iervolino, I., Giorgio, M., Galasso, C., and Manfredi, G. (2009). Uncertainty in early warning predictions of engineering ground motion parameters: what really matters? Geophys. Res. Lett. 36, L00B06. doi:10.1029/2008GL036644
Johnson, L. A., Rabinovici, S., Kang, G. S., Mahin, S. A., Curry, C., Arba, R., et al. (2016). PEER Report No. 2016/06. California earthquake early warning system benefit study. CSSC Publication 16-04.
Kamigaichi, O. (2004). JMA earthquake early warning. J. Earthq. Eng. 4, 134–137. doi:10.5610/jaee.4.3_134
Kamigaichi, O., Saito, M., Doi, K., Matsumori, T., Tsukada, S., Takeda, K., et al. (2009). Earthquake early warning in Japan: warning the general public and future prospects. Seismol. Res. Lett. 80, 717–726. doi:10.1785/gssrl.80.5.717
Kelman, I., and Glantz, M. H. (2014). “Early warning systems defined,” in Reducing disaster: early warning systems for climate change. Editors A. Singh and Z. Zommers (Dordrecht, Netherlands: Springer), 89–108.
King, G., Keohane, R. O., and Verba, S. (1994). Designing social inquiry: scientific inference in qualitative research. Contemp. Sociol. 24, 424. doi:10.2307/2076556
Kohler, M. D., Cochran, E. S., Given, D., Guiwits, S., Neuhauser, D., Henson, I., et al. (2018). Earthquake early warning ShakeAlert system: West Coast wide production prototype. Seismol. Res. Lett. 89, 99–107. doi:10.1785/0220170140
Lancieri, M., and Zollo, A. (2008). A Bayesian approach to the real-time estimation of magnitude from the early P and S wave displacement peaks. J. Geophys. Res. 113, B12302. doi:10.1029/2007JB005386
Lee, W. H. K., and Espinosa-Aranda, J. M. (2003). “Earthquake early warning systems: current status and perspectives,” in Early warning systems for natural disaster reduction. Editors J. Zschau and A. Küppers (Berlin, Germany: Springer), 409–423. doi:10.1007/978-3-642-55903-7_53
Lindell, M. K., Prater, C. S., and Peacock, W. G. (2007). Organizational communication and decision making for hurricane emergencies. Nat. Hazards Rev. 8, 50–60. doi:10.1061/(asce)1527-6988(2007)8:3(50)
Lockman, A. B. (2005). Single-station earthquake characterization for early warning. Bull. Seismol. Soc. Am. 95, 2029–2039. doi:10.1785/0120040241
Maddaloni, G., Caterino, N., Nestovito, G., and Occhiuzzi, A. (2013). Use of seismic early warning information to calibrate variable dampers for structural control of a highway bridge: evaluation of the system robustness. Bull. Earthq. Eng. 11, 2407–2428. doi:10.1007/s10518-013-9510-z
McBride, S. K., Bostrom, A., Sutton, J., de Groot, R. M., Baltay, A. S., Terbush, B., et al. (2020). Developing post-alert messaging for ShakeAlert, the earthquake early warning system for the west coast of the United States of America. Int. J. Disaster Risk Reduct. 50, 101713. doi:10.1016/j.ijdrr.2020.101713
Nakamura, H., Horiuchi, S., Wu, C., Yamamoto, S., and Rydelek, P. A. (2009). Evaluation of the real-time earthquake information system in Japan. Geophys. Res. Lett. 36, L00B01. doi:10.1029/2008GL036470
Nakamura, Y. (1988). “On the urgent earthquake detection and alarm system (UrEDAS),” in Proceedings of the 9th world conference on earthquake engineering, Tokyo, Japan, August 2–9, 1988, 673–678.
Nakamura, Y., and Saita, J. (2007). “UrEDAS, the earthquake warning system: today and tomorrow,” in Earthquake early warning systems. Editors P. Gasparini, G. Manfredi, and J. Zschau (Berlin, Germany: Springer), 249–281. doi:10.1007/978-3-540-72241-0_13
Nakayachi, K., Becker, J. S., Potter, S. H., and Dixon, M. (2019). Residents’ reactions to earthquake early warnings in Japan. Risk Anal. 39, 1723–1740. doi:10.1111/risa.13306
Ohara, M. (2012). “A study on people’s awareness of earthquake early warning before and after the 2011 off the Pacific coast of Tohoku earthquake, Japan,” in 15th World Conference on Earthquake Engineering, Lisbon, Portugal, September 24–28, 2012
Pescaroli, G., and Alexander, D. (2018). Understanding compound, interconnected, interacting, and cascading risks: a holistic framework. Risk Anal. 38, 2245–2257. doi:10.1111/risa.13128
Pescaroli, G., Nones, M., Galbusera, L., and Alexander, D. (2018). Understanding and mitigating cascading crises in the global interconnected system. Int. J. Disaster Risk Reduct. 30, 159–163. doi:10.1016/j.ijdrr.2018.07.004
Pescaroli, G., Pietrantoni, L., and Saccinto, E. (2012). Behavioural reactions to the Emilia-Romagna earthquake and implications for risk management. Auton. Locali Serv. Soc. 35, 505–514. doi:10.1447/73567
Picozzi, M., Emolo, A., Martino, C., Zollo, A., Miranda, N., Verderame, G., et al. (2015). Earthquake early warning system for schools: a feasibility study in southern Italy. Seismol. Res. Lett. 86, 398–412. doi:10.1785/0220140194
Porter, K. A. (2016). “How many injuries can be avoided through earthquake early warning and drop, cover, and hold on?” in Structural engineering and structural mechanics program, Boulder, CO, August 2016.
Porter, K. A., Kennedy, R., and Bachman, R. (2007). Creating fragility functions for performance-based earthquake engineering. Earthq. Spectra 23, 471–489. doi:10.1193/1.2720892
Quarantelli, E. L. (1984). Emergent citizen groups in disaster preparedness and recovery activities. Available at: http://udspace.udel.edu/handle/19716/1206 (Accessed September 12, 2019).
Reddy, E. (2019). Crying ‘crying wolf’: how misfires and Mexican engineering expertise are made meaningful. Ethnos 85, 335–350. doi:10.1080/00141844.2018.1561489
Santos-Reyes, J. (2019). How useful are earthquake early warnings? The case of the 2017 earthquakes in Mexico City. Int. J. Disaster Risk Reduct. 40, 101148. doi:10.1016/j.ijdrr.2019.101148
Satriano, C., Elia, L., Martino, C., Lancieri, M., Zollo, A., and Iannaccone, G. (2011). PRESTo, the earthquake early warning system for Southern Italy: concepts, capabilities and future perspectives. Soil Dynam. Earthq. Eng. 31, 137–153. doi:10.1016/j.soildyn.2010.06.008
Satriano, C., Lomax, A., and Zollo, A. (2008). Real-time evolutionary earthquake location for seismic early warning. Bull. Seismol. Soc. Am. 98, 1482–1494. doi:10.1785/0120060159
Shrivastava, P. (2003). Principles of emergency planning and management. Risk Manag. 5, 67. doi:10.1057/palgrave.rm.8240152
Si, H., and Midorikawa, S. (2000). “New attenuation relations for peak ground acceleration and velocity considering effects of fault type and site condition,” in 12th world conference on earthquake engineering, Auckland, New Zealand, January 30–February 4, 2000, 1–8.
Smith, K., and Petley, D. N. (2009). Environmental hazards. Assessing risk and reducing disaster. London, UK: Routledge.
Strauss, J. A., and Allen, R. M. (2016). Benefits and costs of earthquake early warning. Seismol. Res. Lett. 87, 765–772. doi:10.1785/0220150149
Suárez, G., Espinosa‐Aranda, J. M., Cuéllar, A., Ibarrola, G., García, A., Zavala, M., et al. (2018). A dedicated seismic early warning network: the Mexican seismic alert system (SASMEX). Seismol. Res. Lett. 89, 382–391. doi:10.1785/0220170184
Suarez, G., Novelo, D., and Mansilla, E. (2009). Performance evaluation of the seismic alert system (SAS) in Mexico City: a seismological and a social perspective. Seismol. Res. Lett. 80, 707–716. doi:10.1785/gssrl.80.5.707
Suárez, G., and Novelo‐Casanova, D. A. (2018). A pioneering aftershock study of the destructive 4 January 1920 Jalapa, Mexico, earthquake. Seismol. Res. Lett. 89, 1894–1899. doi:10.1785/0220180150
Tajima, F., and Hayashida, T. (2018). Earthquake early warning: what does “seconds before a strong hit” mean? Prog. Earth Planet. Sci. 5. doi:10.1186/s40645-018-0221-6
Twigg, J. (2003). “The human factor in early warnings: risk perception and appropriate communications,” in Early warning systems for natural disaster reduction. Editors J. Zschau et al. (Berlin: Springer Verlag), 19–26.
UNISDR (2006). “Developing early warning systems : a checklist,” in Third international conference on early warming, Bonn, Germany, March 27–29, 2006, 1–13.
UNISDR (2015). Sendai framework for disaster risk reduction 2015–2030. A/CONF.224/CRP.1. Geneva, Switzerland.
UNISDR (2017). Disaster resilience scorecards for cities–preliminary level assessment. Geneva, Switzerland. Available at: https://www.unisdr.org/files/58158_unisdr2017annualreport.pdf.
Wald, D. J. (2020). Practical limitations of earthquake early warning. Earthq. Spectra 36, 1412–1447. doi:10.1177/8755293020911388
Wieland, M. (2001). Earthquake alarm, rapid response, and early warning systems: low cost systems for seismic risk reduction. Zurich, Switzerland: Electrowatt Engineering Ltd.
WMO (2017). “Multi-hazard early warning systems: a checklist,” in Outcome of the first multi-hazard early warning conference, Cancún, Mexico, May 22–23, 2017.
Wu, Y.-M., and Kanamori, H. (2005). Rapid assessment of damage potential of earthquakes in Taiwan from the beginning of P waves. Bull. Seismol. Soc. Am. 95, 1181–1185. doi:10.1785/0120040193
Wurman, G., Allen, R. M., and Lombard, P. (2007). Toward earthquake early warning in northern California. J. Geophys. Res. 112, B08311. doi:10.1029/2006JB004830
Yamasaki, E. (2012). What we can learn from Japan's early earthquake warning system. Momentum 1, 2. Available at: https://repository.upenn.edu/momentum/vol1/iss1/2/ (Accessed October 19, 2019).
Zollo, A., Amoroso, O., Lancieri, M., Wu, Y.-M., and Kanamori, H. (2010). A threshold-based earthquake early warning using dense accelerometer networks. Geophys. J. Int. 183, 963–974. doi:10.1111/j.1365-246X.2010.04765.x
Zollo, A., Colombelli, S., Elia, L., Emolo, A., Festa, G., Iannaccone, G., et al. (2014a). “An integrated regional and on-site earthquake early warning system for southern Italy: concepts, methodologies and performances,” in Early warning for geological disasters. Editors F. Wenzel and J. Zschau (New York, NY: Springer), 117–137.
Keywords: earthquake early warnings, social resilience, organizational resilience, effectiveness of warning, seismic risk mitigation
Citation: Velazquez O, Pescaroli G, Cremen G and Galasso C (2020) A Review of the Technical and Socio-Organizational Components of Earthquake Early Warning Systems. Front. Earth Sci. 8:533498. doi:10.3389/feart.2020.533498
Received: 08 February 2020; Accepted: 14 September 2020;
Published: 21 October 2020.
Edited by:
Gordon Woo, Risk Management Solutions, United KingdomReviewed by:
Joern Lauterjung,Helmholtz Center Potsdam, GermanyKiran Kumar Singh Thingbaijam, Institute of Seismological Research, India
Copyright © 2020 Velazquez Ortiz, Pescaroli and Galasso. This is an open-access article distributed under the terms of the Creative Commons Attribution License (CC BY). The use, distribution or reproduction in other forums is permitted, provided the original author(s) and the copyright owner(s) are credited and that the original publication in this journal is cited, in accordance with accepted academic practice. No use, distribution or reproduction is permitted which does not comply with these terms.
*Correspondence: Carmine Galasso, Yy5nYWxhc3NvQHVjbC5hYy51aw==